- 1Respiratory Immunology Division, Lovelace Respiratory Research Institute, Albuquerque, NM, United States
- 2Department of Immunology and Nanomedicine, Herbert Wertheim College of Medicine, Florida International University, Miami, FL, United States
- 3Department of Microbiology and Immunology, Galveston, TX, United States
- 4Department of Pediatrics, University of New Mexico Health Sciences Center, Albuquerque, NM, United States
Rationale: Gestational cigarette smoke (CS) impairs lung angiogenesis and alveolarization, promoting transgenerational development of asthma and bronchopulmonary dysplasia (BPD). Hydrogen sulfide (H2S), a proangiogenic, pro-alveolarization, and anti-asthmatic gasotransmitter is synthesized by cystathionine-γ-lyase (CSE), cystathionine-β-synthase (CBS), and 3-mercaptopyruvate sulfur transferase (3MST).
Objective: Determine if gestational CS exposure affected the expression of H2S synthesizing enzymes in the mouse lung and human placenta.
Methods: Mice were exposed throughout gestational period to secondhand CS (SS) at approximating the dose of CS received by a pregnant woman sitting in a smoking bar for 3 h/days during pregnancy. Lungs from 7-days old control and SS-exposed pups and human placenta from mothers who were either non-smokers or smokers during pregnancy were analyzed for expression of the enzymes.
Measurements: Mouse lungs and human placentas were examined for the expression of CSE, CBS, and 3MST by immunohistochemical staining, qRT-PCR and/or Western blot (WB) analyses.
Results: Compared to controls, mouse lung exposed gestationally to SS had significantly lower levels of CSE, CBS, and 3MST. Moreover, the SS-induced suppression of CSE and CBS in F1 lungs was transmitted to the F2 generation without significant change in the magnitude of the suppression. These changes were associated with impaired epithelial-mesenchymal transition (EMT)—a process required for normal lung angiogenesis and alveolarization. Additionally, the placentas from mothers who smoked during pregnancy, expressed significantly lower levels of CSE, CBS, and 3MST, and the effects were partially moderated by quitting smoking during the first trimester.
Conclusions: Lung H2S synthesizing enzymes are downregulated by gestational CS and the effects are transmitted to F2 progeny. Smoking during pregnancy decreases H2S synthesizing enzymes is human placentas, which may correlate with the increased risk of asthma/BPD in children.
Introduction
Maternal smoking during pregnancy remains relatively common (1, 2) and about 1/4th of mothers, who smoke during pregnancy, misreport as quitters (3). Epidemiological data and animal studies suggest that exposure to CS, including secondhand CS (SS) during pregnancy increases the risk of allergic asthma (AA) and BPD in the progeny (4–7); the latter encompasses alveolar simplification (8). Gestational exposure of mice to CS/SS impairs angiogenesis, exacerbates AA, and induces BPD-like alveolar simplification through downregulation of HIF-1α; this phenotype is transmitted to the F2 progeny (9–11). The mechanisms by which gestation CS promotes AA and BPD are unclear. H2S is the newest member of gasotransmitter that affects many physiological systems (12). H2S is an anti-inflammatory that promotes angiogenesis/vascularization and wound healing (7, 13). In the lung, H2S attenuates lipopolysaccharide-induced acute lung injury (14), confers protection against ventilation-induced pulmonary inflammation and injury (15), promotes alveolarization and airway development (16), and protects against asthma and allergic inflammation (17, 18).
In mammals, H2S is mainly produced from L-cysteine by three enzymes: cystathionine γ-lyase (CSE), cystathionine β-synthase (CBS), and 3-mercaptopyruvate sulfur transferase (3MST) (19, 20). The distribution of these enzymes in various tissue is somewhat uncertain. It is generally believed that CSE and CBS are the two most prominent H2S synthesizing enzymes, where CBS is primarily localized to the brain and CSE in non-neuronal tissues (21, 22). However, this is not an inflexible rule. For example, adult rat lung expresses CSE and 3MST, but insignificant levels of CBS (23), but CBS has been reported in airway vasculature and lung epithelial cells, and CSE is present in the lung parenchyma (16). All three H2S synthesizing enzymes (CSE, CBS, and 3MST) are present in the lungs of cows and sea lions (24) and lung biopsies from non-small cell lung cancer patients (25) and the lung epithelial cell line A549 (26) also express all the three enzymes. In a recent report, 3MST was shown to be upregulated in the lung adenocarcinoma (27). Similarly, while the portal vein and thoracic aorta contain CSE, ileum expresses both CSE and CBS (28). Thus, the expression of H2S enzymes depends on the tissue type and the state of cell differentiation.
Epithelial mesenchymal transition (EMT) is an important process for cell differentiation during development, organogenesis, and carcinogenesis (29, 30). While dysregulated EMT in the adult lung promotes multiple respiratory diseases, it is indispensable for the development of lung epithelium (31), where the TGF-β/Smad pathway plays a key role (31, 32). Although, H2S has been shown to inhibit EMT in lung cancers through Wnt/Catenin signaling and the activation of HIF-1α (25, 33), HIF-1α is dramatically downregulated by gestational CS in the 7-days old mouse lung (11, 34) and, in some lung injuries, H2S promotes EMT and lung repair (35, 36). Moreover, HIF-1α mediates cellular differentiation through TGF-β (37, 38)—a key participant in EMT (39, 40). Thus, EMT is important in lung development and organogenesis, and requires H2S-induced HIF-1α/TGF-β.
In this communication we demonstrate that gestational SS suppresses TGF-β, EMT, and anti-asthmatic factors, and the 7-days old mouse lung and human placentas contains all the three H2S synthesizing enzymes. Gestational exposure to CS suppresses the expression of these enzymes in the mouse lung and human placentas from mothers' who smoke during pregnancy. The latter prompts the possibility that the placental levels of H2S synthesizing enzymes may correlate with the risk of AA and BPD in children.
Materials and Methods
Animals
Pathogen-free BALB/c mice were purchased from the FCR Facility (Frederick, MD). The animals were housed at the Animal Facility of Lovelace Respiratory Research Institute, Albuquerque, NM in accordance with the Guidelines from the Association for the Assessment and Accreditation for Laboratory Animal Care International. Animals were kept in exposure chambers maintained at 26 ± 2°C with 12-h light/dark cycle. Food and water were provided ad libitum.
Study Approval
All animal protocols were approved by the Institutional Animal Care and Use Committee in accordance with the Guide for Laboratory Animal Practice under the Association for the Assessment and Accreditation for Laboratory Animal Care International.
Gestational Exposure to Sidestream Cigarette Smoke (SS)
Adult (3–4 months old) male and female mice (BALB/c) were separately acclimatized to SS or filtered air (FA) for 2 weeks before being paired for mating under the same exposure conditions. Briefly, mice were exposed to whole-body SS or FA for 6 h/days, 7 days/weeks (total particulate matter 1.52 ± 0.41 mg/m3) using Type 1,300 smoking machine (AMESA Electronics, Geneva, Switzerland) that generated two 70 cm3 puffs/min from 2R1 cigarettes as described previously (9, 10). The dose of SS was approximately equivalent to the amount of SS a pregnant woman would receive by sitting in a smoking bar for 3 h/days throughout the gestational period (10). After pregnancy was established, male mice were removed and the pregnant mice continued to receive SS or FA until the pups were born. Immediately after the birth of pups the exposures were stopped. On the postnatal day 7, some animals were sacrificed by an intraperitoneal injection of 0.2 ml Euthasol. Some adult F1 mice from FA and SS groups were mated to obtain the F2 progeny as described previously (10). Representative results are presented using animal from two different sets of SS-exposure. At least 15 animals per group were used; each analysis used 5 mice/group and the analysis was repeated twice. Specific details are given under figure legends.
Human Placenta Samples
Placentas were collected at the University of New Mexico Hospital (UNMH), Albuquerque, NM according to protocol #17-064 approved by the University of New Mexico Medical Center Institutional Review Board and Human Research Protection Office in accordance with the NIH guidelines. All donors had agreed to donate the tissues. We were able to collect 10 placentas in a span of 7 months (by Dr. A. Sheybani and Dr. V. Exil, both from UNMH) representing three controls (mothers who did not smoke during the pregnancy), 4 CS-exposed (mothers who smoked throughout the pregnancy), two first-trimester quitters (mothers who stopped smoking during the first trimester of pregnancy), one false-control (mother who claimed to have quitted smoking during pregnancy, but the placenta had high level of cotinine). A 3 cm3 section of each placenta was dissected and frozen immediately for RNA and protein assays. Rest of the placentas were kept at −80°Cuntil use. Tissue slides (5 μm) were prepared by the institutional Pathology Core.
Determination of Cotinine Levels in Placentas
The smoking status of the mothers was confirmed by determining the cotinine levels in the placental tissues using the cotinine ELISA kit (Calbiotech Inc., CA) with a sensitivity of 5 ng/ml. Immunoblots were developed using placental homogenates.
Assays for H2S Synthesizing Enzymes
The expression of CSE, CBS, and 3MST was determined by WB analysis, IF-IHC, and/or qPCR. Assay details are given under relevant figure legends.
Immunostaining and Immunofluorescent Imaging
For immunohistochemical (IHC) staining, deparaffinized and hydrated lung and placental tissue sections were washed in 0.05% v Brij-35 in PBS (pH 7.4) and immunostained for antigen expression as described previously (41). Briefly, the antigens were unmasked by steaming the sections in 10 mM Citrate buffer (pH 6.0) followed by incubation in a blocking solution containing 3% BSA, 1% Gelatin and 1% normal donkey serum with 0.1% Triton X-100 and 0.1% Saponin. Serial sections were stained with antibodies to Vimentin, E-cadherin, and ZO-1 (Invitrogen Inc., Carlsbad, CA), or isotype control antibodies. The immunolabelled tissues were detected using respective secondary antibodies conjugated with fluorescent dyes (Jackson ImmunoResearch Lab Inc., West Grove, PA). Where indicated, the sections were stained with 4′,6-diamidino-2-phenylindole (DAPI) containing Fluormount-G (SouthernBiotech, Birmingham, AL) to visualize nuclei. Immunofluorescent images were captured with BZX700 Microscopy system (Keyence, Tokyo, Japan). Specific details are given under appropriate figure legends.
Western Blot Analysis
Western blot (WB) analysis of mouse lung and human placenta homogenates was carried out as described previously (10). Briefly, lung or placental tissues were homogenized in RIPA buffer and the protein content of the extracts was determined by the BCA Protein Assay Kit (Pierce, Rockford, IL). The homogenates were run on SDS-PAGE on 10% precast polyacrylamide gels (BioRad Lab, Hercules CA). The gels were transferred electrophoretically to nitrocellulose membranes (BioRad Lab). The blots were incubated with the respective antibodies. The mouse anti-β actin antibody (Santa Cruz Biotech) was used as the house-keeping protein. After incubating with an appropriate secondary antibody, the blots were developed with Amersham ECL Western Blotting Detection Reagent (GE Healthcare Bio-Science Corp. Piscataway, NJ) and the images were captured by Fujiform LAS-4000 luminescent image analyzer (FUJIFILM Corporation, Tokyo). Densitometry was used to quantitate the expression of specific proteins and expressed as the protein/β-actin band ratio.
Quantitative Real-Time PCR (qPCR)
Total RNA was extracted by using a ToTALLY RNA kit (catalog number AM1910; Ambion, Austin, TX, USA). RNA samples were quantified by using a NanoDrop spectrophotometer and quality was analyzed on an RNA Nano-drop by using the Agilent 2100 bioanalyzer (Agilent Technologies). Synthesis of cDNA used 1 μg of total RNA in a 20 μl reaction mixture and TaqMan Reverse Transcription Reagents kit from ABI (catalog number N8080234; Applied Biosystems). qPCR amplification (performed in triplicate) used 1 μl of cDNA in a total volume of 25 μl of Faststart Universal SYBR green master mix (Roche Applied Science #04913850001). The mRNA sequences for CSE, and CBS for mouse and human reported under GenBank accession numbers NM145953 (CSE mouse), NM144855.3 (CBS mouse), NM_001902 (CSE human), and NM000071 (CBS (human) and were used to design primers for qRT-PCR assay (42–44). Expression of 3MST mRNA was performed using total RNA from lung and placental tissues by qPCR analysis and One-Step Real-Time PCR MasterMix containing TaqMan probes and a specific-labeled primer/probe set (Applied Biosystems). 18S RNA was used as housekeeping gene for normalization. PCR assays were run in the ABI Prism 7500 Sequence Detection System. Triplicate cycle threshold (CT) values were analyzed using the comparative CT (ΔΔCT) method as per manufacturer's instructions (Applied Biosystems). The amount of target (2−ΔΔCT) was obtained by normalization to the endogenous reference (18S) sample. RNA isolation, primer design, and qRT-PCR assays were performed using the Molecular Genomic Core, UTMB, Galveston, TX.
Statistical Analysis
Grouped results were expressed as mean ± SD and p ≤ 0.05 were considered significant. The data were normalized via natural log transformations and when the data was normally distributed, statistical significance among the groups was determined by one-way ANOVA with Bonferroni correction with multiple pairwise comparisons. When the data was not normally distributed, we used Kruskal-Wallis assessment on ranks followed by Dunn's multiple comparison tests. Student's t-test was employed for comparison between two groups at 95% confidence interval using Prizm software (GraphPad Software Inc., San Diego, CA). p ≤ 0.05 was considered statistically significant.
Results and Discussion
Gestational SS Inhibits EMT in the F1-Progeny Lung
Gestational exposure to SS impairs alveolarization and promotes BPD in the progeny, and these effects are transmitted to the F2 progeny and associated with suppressed levels of HIF-1α (11, 34). EMT is a biological process that allows epithelial cells to assume mesenchymal phenotype, which is critical for normal alveolarization (45) and regulated by HIF-1α, TGF-β, and VEGF (38, 39, 46, 47). VEGF promotes angiogenesis that stimulates EMT and alveolarization (48, 49) and intratracheal transplantation of mesenchymal stem cells attenuate lung injury in newborn mice (50). During embryogenesis and organ development, epithelial markers such as E-cadherin and ZO-1 are decreased and mesenchymal markers such as vimentin are increased (48, 51). The transcription factor HIF-1α promotes synthesis of TGF-β–the most potent inducer of EMT (52, 53) and HIF-1α is potently reduced in gestationally SS-exposed lungs (11). To determine whether gestational SS affected EMT, we determined the lung levels of epithelial (E-cadherin and ZO-1) and mesenchymal (vimentin) cell markers by IHC and Western blot analysis in 7-days old lungs from control and gestationally SS-exposed mice. Compared to control lungs, levels of E-cadherin (Figure 1A) and ZO-1 (Figure 1B) were significantly higher than those of vimentin (Figures 1A,C) in gestationally SS-exposed lungs. Moreover, the concentrations of TGF-β by Western blot analysis (Figure 1D) and of the anti-asthmatic factor SOX2 by IHC (Figure 1E), were significantly lower in gestationally SS-exposed lungs. SOX2 is a pluripotent transcription factor in bronchoalveolar progenitors, which promotes the Club cells to express Clara-cell secretory protein (CCSP) and surfactant protein-C (SP-C) (54). CCSP and SP-C are suppressed by gestational SS (10) and reduced SOX2 and CCSP levels are associated with higher risk of asthma (55); humans and mice deficient in CCSP, exhibit airway hyperresponsiveness (56). Together, these results suggest that gestational exposure to CS inhibits EMT and is associated with decreased numbers of SOX2-positive Clara cell progenitors.
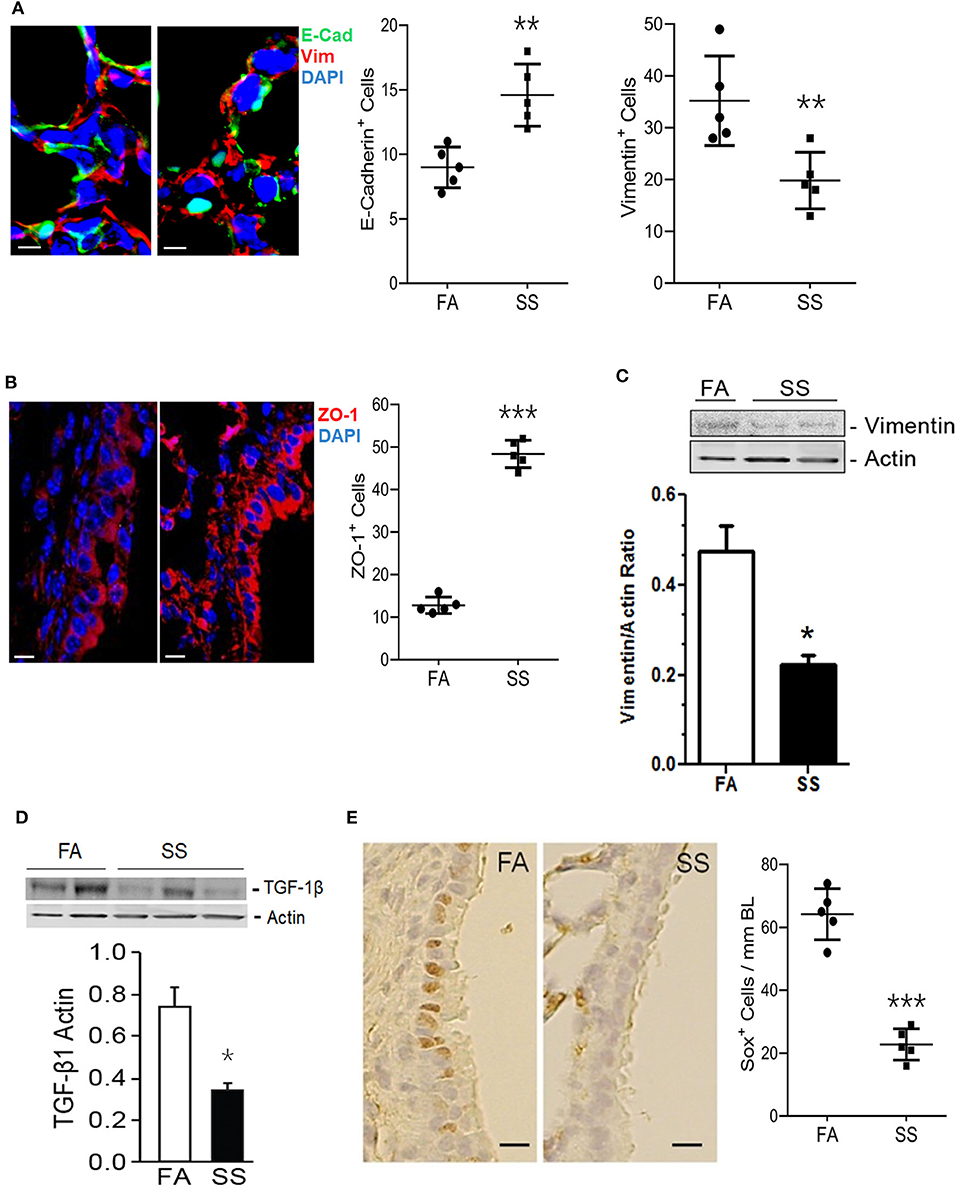
Figure 1. Gestational exposure to SS inhibits EMT in the mouse F1 lung. (A) Representative micrographs of lung sections from mice exposed gestationally to filtered-air (FA) or side-stream cigarette smoke (SS) and co-stained with vimentin (red) and cadherin (green); DAPI-stained nuclei (blue). E-Cadherin+ and Vimentin+ cells per unit area (18,000 μm2) were counted blind using NDP View on a Nanozoomer (Hamamatsu Photonics Inc.). (B) Representative micrographs of lung sections from gestationally FA or SS-exposed mice and stained with ZO-1 (red). ZO-1+ cells (12,400 μm2; NDP scanner). (C) Representative Western blot of lung tissue homogenates (70 μg) from FA or SS-exposed mice and probed with anti-vimentin antibody. Lower panel is the densitometry of the blot and expressed as Vimentin/Actin ratio. (D) Western blot analysis of lung tissue homogenates (70 μg) from FA or SS-exposed lungs probed with anti-TGF-β1 antibody (Cat# ab92486, Abcam). Lower panel is densitometry of the blot presented as TGF-β1/Actin ratio. (E) Representative image of the lung sections (5 μm) stained with anti-Sox2 antibody and detected by immunohistochemical staining. Right panel shows Sox+ cells (17,000 μm2; NDP scanner) counted blind. Data shown as mean±SD (n = 5/gp; *p < 0.05; **p < 0.01; ***p < 0.001).
Gestational SS Suppresses CSE and CBS in the F1 and F2 Progeny Lungs
H2S is required for normal angiogenesis and alveolarization (16, 25) and produced in the periphery mainly by CSE and CBS (12). H2S attenuates lung injury (15, 57) and CSE deficiency exacerbates airway hyperreactivity (44) and impairs alveolarization. Impaired angiogenesis and alveolarization in CSE- and CBS-deficient mice are partially restored by H2S donor compounds (16). Moreover, H2S levels are lower in the exhaled air from asthma and COPD patients and correlates with lower FEV1 (58). Expression level of H2S enzymes is reported to be tissue specific. Thus, the brain and the vascular endothelium have a strong expression of CBS and CSE, respectively; however, both tissues also express 3MST (22). The situation in the lung is somewhat confusing. Lungs were reported to primarily express CSE (21); however, human lung cell lines such as A549 (26) and the lungs from cow and sea lions express all the three H2S synthesizing enzymes (24).
To ascertain whether gestational SS affected H2S production in the lung, we determined the mRNA levels of CSE (Figure 2A) and CBS (Figure 2B) and 3MST (Figure 2C) by qPCR in 7-day-old lungs from control and SS-exposed mice. Gestational CS inhibited mRNA levels of CSE, CBS, and 3MST, which would decrease the level of H2S in the lung and increase the risk of inflammatory lung diseases in these animals.
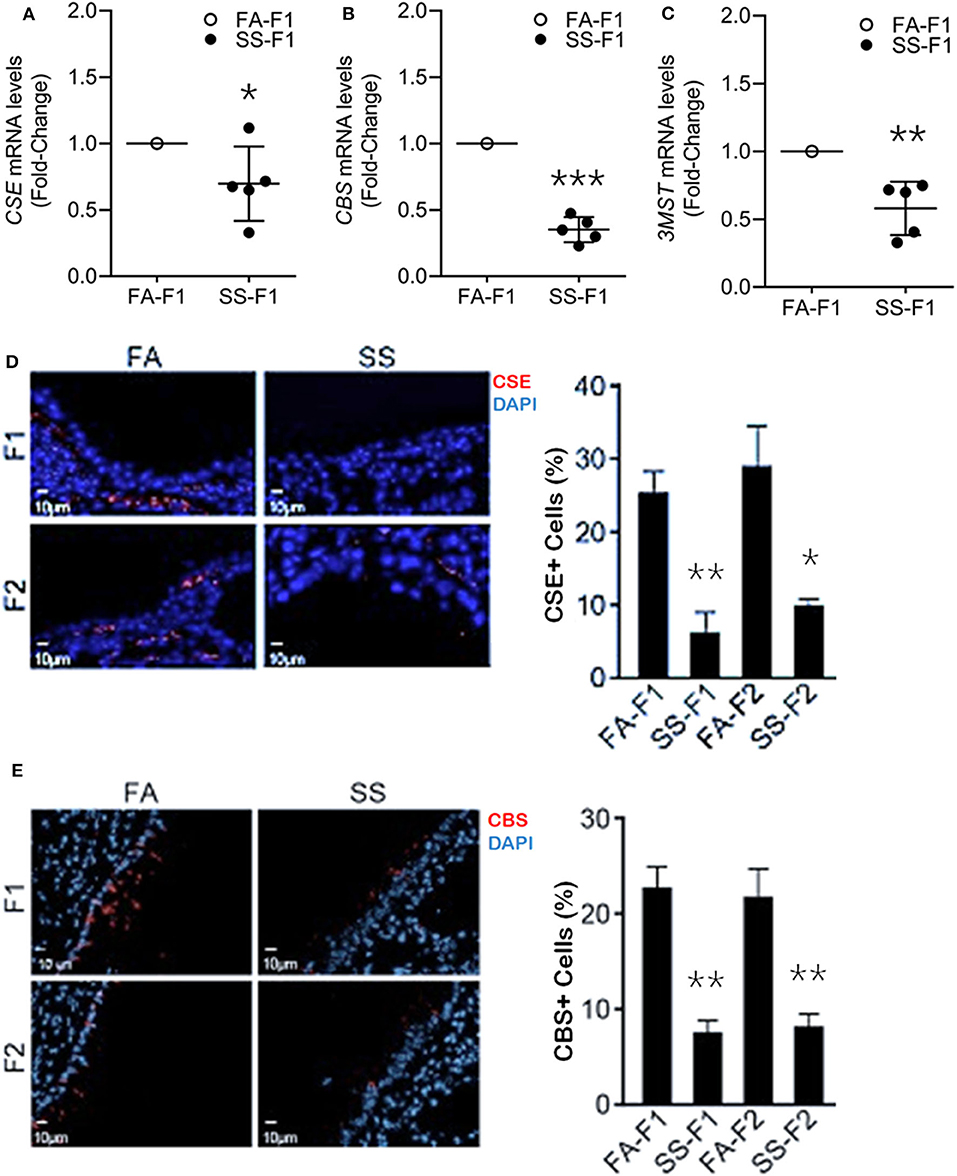
Figure 2. Gestational SS suppresses H2S biogenesis enzymes, CSE, CBS and 3MST in 7d old F1 mouse lung. Relative mRNA levels of CSE (A), CBS (B), and 3MST (C) in F1 lungs exposed gestationally to FA or SS. (D) Representative micrographs of lung sections from F1 and F2 progenies exposed gestationally to SS or FA. Sections were stained for CSE (red) and DAPI (blue). Right pane is quantitation of CSE+ cells (%) in each group. (E) Micrographs of lung sections from F1 and F2 stained for CBS (red) and DAPI (blue). Right panel shows quantitation of CBS+ cells (%) in each group. Data shown as mean ± SD (n = 5/gp; *p < 0.05; **p < 0.01; ***p < 0.001).
Gestational CS/SS/nicotine increases the risk of asthma and/or BPD transgenerationally in humans and animals (10, 59–61). To determine whether the transgenerational pro-asthmatic/pro-BDP effects of gestational SS were related to changes in H2S, 7-days old lungs from control and gestationally SS-exposed F1 and F2 mice were analyzed for CSE (Figure 2D) and CBS (Figure 2E) levels by IHC staining. Results showed that control lungs contained about equal numbers (~25%of total cells) of CSE- and CBS-positive cells, and gestational exposure to SS significantly reduced the number CSE/CBS-positive cells in both F1 and F2 animals. Thus, as reported for HIF-1α, angiogenesis, and alveolar volumes (11), gestational SS suppresses the levels of CSE and CBS in F1 progeny and the effects are transmitted to F2. Given the relationship between HIF-1α, TGF-β, EMT, angiogenesis, alveolarization, BPD, AA, and H2S, it is likely that the CS-induced proinflammatory lung responses in F1 and F2 progenies are related to changes in H2S levels regulated by H2S synthesizing enzymes. These data suggest that mouse lungs contain all three H2S synthesizing enzymes and gestational exposure to CS suppresses their expression. Reduced levels of these enzymes has the potential to promote lung diseases such as asthma and BPD.
Placentas From Women Who Smoked During Pregnancy Express Low Levels of H2S Synthesizing Enzymes
Children from women who smoke during pregnancy have increased risk of AA and BPD (4–7) and herein our results suggest that the increased susceptibility may correlate with decreased levels of H2S synthesizing enzymes. Thus, the lung levels of H2S enzymes at birth may predict the risk of AA and BPD in children; however, it is unrealistic to obtain lung samples from newborn babies. Because, H2S synthesizing enzymes are present in most tissues (12, 62), we ascertained whether the enzymes were present in human placentas and, if so, whether smoking during pregnancy affected their expression. We were able to obtain 10 human placentas representing 3 controls (mothers who did not smoke during the pregnancy), four CS-exposed (mothers who smoked throughout the pregnancy), two first-trimester quitters (mothers who stopped smoking during the first trimester of pregnancy), one false-control (mother who claimed to have quitted smoking during pregnancy, but the placental showed high cotinine). Cotinine was determined on all placentas by ELISA to ensure that the tissues were from smoking/non-smoking mothers. CSE expression was determined by WB analysis, qPCR, and IF-IHC; CBS by WB and IF-IHC, and 3MST by qPCR analysis.
Immunoblot analysis of the placental homogenates from mothers who smoked throughout the pregnancy (GesCS) showed very low expression of CSE as compared to control non-smokers (CONT) or the mothers who quit during the 1st trimester (GesCS1/3) (Figures 3A,B). Similarly, as determined by qPCR analysis, CSE-specific mRNA content of GesCS placentas was significantly lower than CONT and GesCS1/3 (Figure 3C). Although the protein content of CSE in GesCS1/3 was higher than GesCS, it was still significantly lower than CONT (Figure 3B), suggesting that quitting smoking during the first trimester may be beneficial; however, the effects are not totally reversible and may persists after the birth. CSE expression was further confirmed by immunostaining of placental sections showing a 4-fold lower expression of CSE in GesCS than CONT; CSE expression in GesCS1/3 placentas was intermediate between CONT and GesCS (Figures 3D,E).
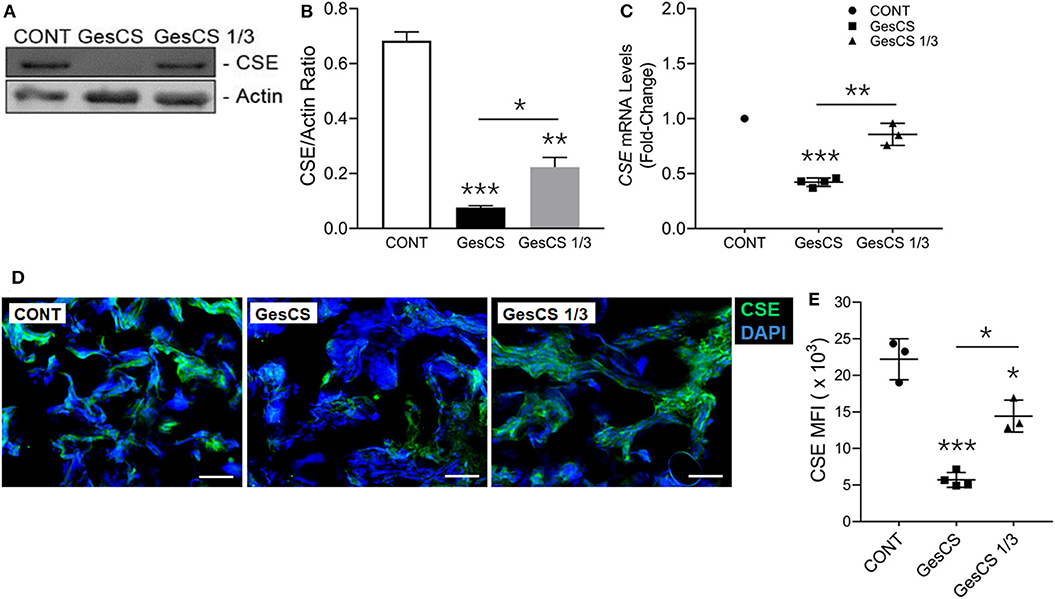
Figure 3. Gestational exposure to CS suppresses H2S biosynthetic enzyme CSE in human placenta. Placentas were analyzed for CSE expression using WB, qPCR and IF-IHC staining. (A) Western blot analysis of the placental tissue homogenate (150 μg protein) with anti-CSE antibody (Abcam, MA, USA). (B) Densitometry of CBS normalized to β-actin levels. (C) CSE mRNA detection by qPCR and expressed relative to CONT group. (D) Representative micrographs showing placental CSE (green) along with DAPI-stained nuclei (blue), scale−10 μ. (E) Quantification of CSE expression by MFIs (mean fluorescence intensity). CONT, control non-smoker; GesCS, cigarette smoking during whole pregnancy; GesCS1/3, CS exposure during first trimester; Data shown as mean ± SD (n = 3–4/gp; *p < 0.05; **p < 0.01; ***p < 0.001).
WB analysis also indicated that the expression of CBS was lower in GesCS than CONT or GesCS1/3 (Figures 4A,B). Furthermore, IHC analysis of CBS in placentas showed the expression was 3-fold lower in GesCS than CONT; however, the difference between CONT and GesCS 1/3 groups was not statistically significant (Figures 4C,D). We also examined the status of 3MST mRNA expression in human placentas by qPCR. Like CSE and CBS, exposure to cigarette smoke significantly inhibited the expression of 3MST (Figure 4E) indicating that, like CSE and CBS, gestational CS also downregulates 3MST expression in human placentas.
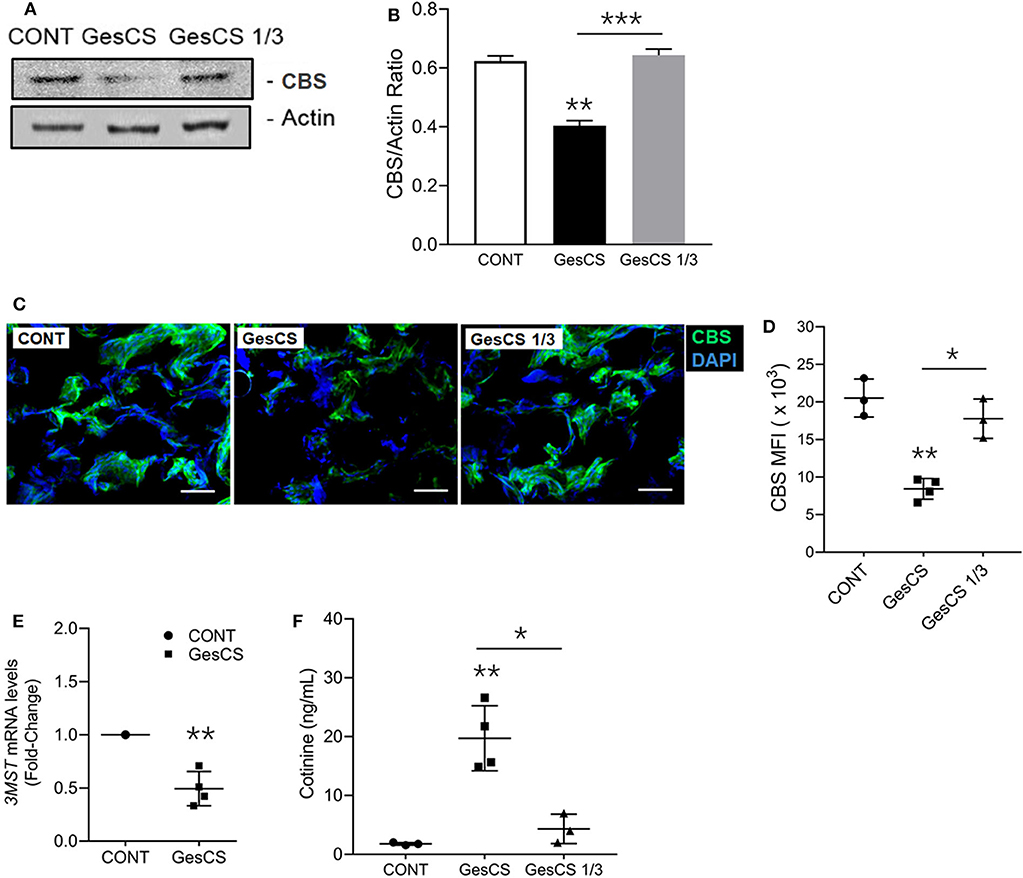
Figure 4. Gestational exposure to CS suppresses CBS and 3MST in human placenta. (A) Western blot analysis of placental tissue homogenates (150 μg protein) probed with anti-CBS antibody (Abcam, MA). (B) Densitometry of CBS normalized to β-actin. (C) Representative micrographs showing placental CBS (green) and DAPI-stained nuclei (blue), scale−10 μ (D) Quantification of CBS expression by MFI (mean fluorescence intensity) of CBS-immunoreactive fluorescence. (E) Quantitative RT-PCR of 3MST mRNA expression (n = 5). (F) The smoking status of mother's was ascertained by the cotinine levels in the placental homogenate using cotinine ELISA kit (Calbiotech Inc., CA) with sensitivity of 5 ng/ml. CONT, non-smoker control; GesCS, cigarette smoker during pregnancy; GesCS1/3, CS exposure during first trimester; Data shown as mean ± SD (n = 3–4/gp; *p < 0.05; **p < 0.01; ***p < 0.001).
The smoking status of the mothers who donated the placentas was verified by measuring the cotinine levels (Figure 4F) and, in general, corroborated their assertion. However, we observed one outlier, where the WB and qPCR analyses of the placenta indicated very low levels of CSE (data not shown), yet the donor claimed to have quitted smoking during the pregnancy. The placenta contained high cotinine levels and was not included in the analyses. Sadly, it is not uncommon for the mothers to falsely assert quitting smoking during the pregnancy (3, 63).
The current study does not clearly define the stage(s) of pregnancy, where the fetus is completely resistant to the effects of CS on placental H2S enzymes. While the epidemiological evidence strongly suggests that CS exposure during pregnancy promotes wheeze and asthma in children (64), but the identity of the susceptible stage(s) of the pregnancy is not unequivocal and may vary from first trimester (6) to third trimester (5). Our data with placental levels of H2S enzymes suggest that the effects of smoking during first trimester are moderate, but not negligible; however, we have not correlated these levels to the actual incidence of asthma in the progeny. Interestingly, perinatal exposure to nicotine induces asthma in rats (61), suggesting that late stages of embryonic development might be more sensitive to gestational CS. Nonetheless, it is highly likely that there is a correlation between placental levels of H2S enzymes and the risk of asthma/BPD in children and H2S or H2S-donor compounds may have therapeutic value to reduce this risk. The manner by which H2S inhibits allergic asthma is related to its ability to suppress Th2 immune responses (65) and CSE deficient mice have elevated GATA3 nuclear content, higher levels of Th2 cytokines, and exaggerated asthma response; H2S donors attenuate asthma (17, 66). Thus, gestational exposure to CS downregulates H2S synthesizing enzymes that in turn may increase the susceptibility of children to respiratory diseases associated with gestational exposure to CS. Taken together, the data presented herein provide a basic outline of the potential interaction between gestational CS exposure, de novo synthesis of H2S, and development of lung developmental diseases as described schematically in Figure 5.
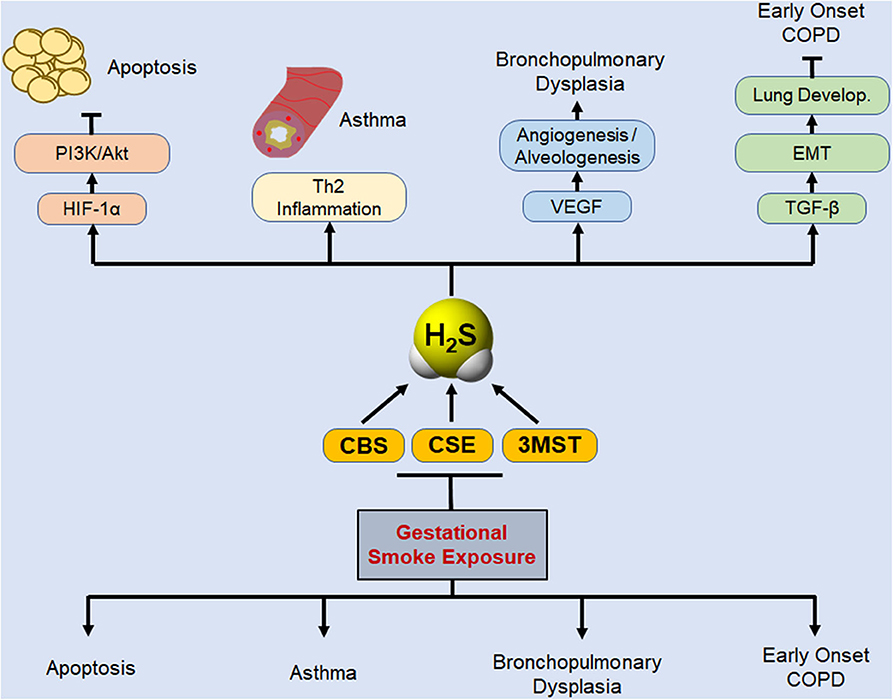
Figure 5. Schematic representation of potential protective role of biosynthesized H2S in basic lung pathophysiologies that are disrupted by gestational exposure to CS. Gestational exposure to CS is primarily linked to four major pulmonary predicaments: cell apoptosis, asthma, BPD, and susceptibility to early development of COPD. Lung cell apoptosis is associated with allergic inflammation (18) and decreased levels of HIF-1α and PI3K/Akt (34). PI3K/Akt inhibits apoptosis and promotes cell proliferation (67), and activation of Akt protects the neonatal lung against injuries (68). H2S donors inhibit apoptosis, attenuate lung damage, and promote normal lung development (21, 69). Asthma development as a consequence of gestational CS exposure or the deficiency of H2S enzymes is associated with increased Th2 inflammation (9, 17, 70), and exogenous H2S was shown to reverse the exacerbated asthma response in CSE-deficient mice (17). Gestation SS suppresses VEGF and angiogenesis, leading to impaired alveolarization and BPD (11, 34), and H2S stimulates VEGF expression and angiogenesis, (71), and alveolarization (72, 73). Maternal smoking affects lung development and has been linked to early onset of COPD in the progeny (74, 75). TGF-β is critical for EMT and normal lung development (31, 53) and herein we have shown that gestational CS downregulates TGF-β and inhibits EMT. Thus, gestational exposure to CS downregulates H2S synthesizing enzymes that in turn may increase the susceptibility of children to respiratory diseases associated with gestational exposure to CS.
Research Impact
Exposure to cigarette smoke (CS) during pregnancy impairs epithelial-mesenchymal transition (EMT) and angiogenesis in the lung, increasing the risk of allergic asthma and bronchopulmonary dysplasia (BPD), transgenerationally. Hydrogen sulfide (H2S), a recently recognized gasotransmitter, promotes angiogenesis and inhibits asthma and alveolar simplification. H2S is synthesized by cystathionine-β-synthase (CBS), cystathionine-γ-lyase (CSE), and 3-mercaptopyruvate sulfur transferase (3MST). Results presented herein show that exposure of mice to CS during pregnancy suppressed the lung expression of CSE, CBS, 3-MST, and the CS-induced suppression of CSE and CBS was transmitted to F2. Similarly, smoking during pregnancy downregulated the expression of CSE, CBS, and 3MST in human placentas; the downregulated expression of the enzymes might be a biomarker for asthma susceptibility in children.
Data Availability Statement
All datasets generated for this study are included in the article.
Ethics Statement
The studies involving human participants were reviewed and approved by University of New Mexico Medical Center's Institutional Review Board and Human Research Protection Office in accordance with the NIH guidelines. The patients/participants provided their written informed consent to participate in this study. The animal study was reviewed and approved by Lovelace Respiratory Research Institute IACUC.
Author Contributions
SS performed the experiments, analyzed the data, and wrote the manuscript, DD, MM, AS, and TI analyzed the data, VE, HA, and VR performed the sample analysis and analyzed the data. RG analyzed the data and wrote the manuscript. HC performed the sample analysis, analyzed the data, and wrote the manuscript. MS designed the studies, analyzed the data, and wrote the manuscript. All authors reviewed the manuscript.
Funding
SS and MS acknowledge the support by NIH R01-HL125000 and HC was in part supported by NIH R21AI144724 and FIU Start-up Funds.
Conflict of Interest
The authors declare that the research was conducted in the absence of any commercial or financial relationships that could be construed as a potential conflict of interest.
Acknowledgments
We thank the staff and veterinarians of the Inhalation Core and Histology Core facility of Lovelace Respiratory Research Institute.
Abbreviations
AA, allergic asthma; BPD, bronchopulmonary dysplasia; CBS, cystathionine-β-synthase; CCSP, Clara-cell secretory protein; COPD, chronic obstructive pulmonary disease; CS, cigarette smoke; CSE, cystathionine-γ-lyase; CS, cigarette smoke; EMT, epithelial mesenchymal transition; FA, filtered air; GesCS, gestational cigarette smoke; GesCS1/3, mothers who quit smoking during the 1st trimester; HIF-1α, hypoxia Inducible factor-1α; H2S, Hydrogen sulfide; 3MST, 3-mercaptopyruvate sulfur transferase; MFI, mean fluorescence intensity; NaHS, sodium hydrosulfide; SS, secondhand CS; SP-C, surfactant protein-C; TGF-β, transforming growth factor beta; ZO-1, zonula occludens-1.
References
1. Hylkema MN, Blacquiere MJ. Intrauterine effects of maternal smoking on sensitization, asthma, and chronic obstructive pulmonary disease. Proc Am Thorac Soc. (2009) 6:660–2. doi: 10.1513/pats.200907-065DP
2. Mazurek JM, England LJ. Cigarette smoking among working women of reproductive age-United States, 2009-2013. Nicotine Tob Res. (2016) 18:894–9. doi: 10.1093/ntr/ntv292
3. England LJ, Grauman A, Qian C, Wilkins DG, Schisterman EF, Yu KF, et al. Misclassification of maternal smoking status and its effects on an epidemiologic study of pregnancy outcomes. Nicotine Tob Res. (2007) 9:1005–13. doi: 10.1080/14622200701491255
4. Hu FB, Persky V, Flay BR, Zelli A, Cooksey J, Richardson J. Prevalence of asthma and wheezing in public schoolchildren: association with maternal smoking during pregnancy. Ann Allergy Asthma Immunol. (1997) 79:80–4. doi: 10.1016/S1081-1206(10)63090-6
5. Xepapadaki P, Manios Y, Liarigkovinos T, Grammatikaki E, Douladiris N, Kortsalioudaki C, et al. Association of passive exposure of pregnant women to environmental tobacco smoke with asthma symptoms in children. Pediatr Allergy Immunol. (2009) 20:423–9. doi: 10.1111/j.1399-3038.2008.00820.x
6. Neuman A, Hohmann C, Orsini N, Pershagen G, Eller E, Kjaer HF, et al. Maternal smoking in pregnancy and asthma in preschool children: a pooled analysis of eight birth cohorts. Am J Respir Crit Care Med. (2012) 186:1037–43. doi: 10.1164/rccm.201203-0501OC
7. Wang G, Li W, Chen Q, Jiang Y, Lu X, Zhao X. Hydrogen sulfide accelerates wound healing in diabetic rats. Int J Clin Exp Pathol. (2015) 8:5097–104.
8. Martinez S, Garcia-Meric P, Millet V, Aymeric-Ponsonnet M, Alagha K, Dubus JC. Tobacco smoke in infants with bronchopulmonary dysplasia. Eur J Pediatr. (2015) 174:943–8. doi: 10.1007/s00431-015-2491-y
9. Singh SP, Gundavarapu S, Pena-Philippides JC, Rir-Sima-ah J, Mishra NC, Wilder JA, et al. Prenatal secondhand cigarette smoke promotes Th2 polarization and impairs goblet cell differentiation and airway mucus formation. J Immunol. (2011) 187:4542–52. doi: 10.4049/jimmunol.1101567
10. Singh SP, Gundavarapu S, Smith KR, Chand HS, Saeed AI, Mishra NC, et al. Gestational exposure of mice to secondhand cigarette smoke causes bronchopulmonary dysplasia blocked by the nicotinic receptor antagonist mecamylamine. Environ Health Perspect. (2013) 121:957–64. doi: 10.1289/ehp.1306611
11. Singh SP, Chand HS, Langley RJ, Mishra N, Barrett T, Rudolph K, et al. Gestational exposure to Sidestream (Secondhand) cigarette smoke promotes transgenerational epigenetic transmission of exacerbated allergic Asthma and Bronchopulmonary Dysplasia. J Immunol. (2017) 198:3815–22. doi: 10.4049/jimmunol.1700014
12. Polhemus DJ, Lefer DJ. Emergence of hydrogen sulfide as an endogenous gaseous signaling molecule in cardiovascular disease. Circ Res. (2014) 114:730–7. doi: 10.1161/CIRCRESAHA.114.300505
13. Katsouda A, Bibli SI, Pyriochou A, Szabo C, Papapetropoulos A. Regulation and role of endogenously produced hydrogen sulfide in angiogenesis. Pharmacol Res. (2016) 113:175–85. doi: 10.1016/j.phrs.2016.08.026
14. Ali FF, Abdel-Hamid HA, Toni ND. H2S attenuates acute lung inflammation induced by administration of lipopolysaccharide in adult male rats. Gen Physiol Biophys. (2018) 37:421–31. doi: 10.4149/gpb_2018002
15. Spassov SG, Faller S, Hummel M, Helo K, Ihle A, Ryter SW, et al. Hydrogen sulfide confers lung protection during mechanical ventilation via cyclooxygenase 2, 15-deoxy Delta12,14-Prostaglandin J2, and peroxisome proliferator-activated receptor gamma. Crit Care Med. (2017) 45:e849–57. doi: 10.1097/CCM.0000000000002440
16. Madurga A, Golec A, Pozarska A, Ishii I, Mizikova I, Nardiello C, et al. The H2S-generating enzymes cystathionine beta-synthase and cystathionine gamma-lyase play a role in vascular development during normal lung alveolarization. Am J Physiol Lung Cell Mol Physiol. (2015) 309:L710–24. doi: 10.1152/ajplung.00134.2015
17. Zhang G, Wang P, Yang G, Cao Q, Wang R. The inhibitory role of hydrogen sulfide in airway hyperresponsiveness and inflammation in a mouse model of asthma. Am J Pathol. (2013) 182:1188–95. doi: 10.1016/j.ajpath.2012.12.008
18. Mendes JA, Ribeiro MC, Reis Filho G, Rocha T, Muscara MN, Costa SKP, et al. Hydrogen sulfide inhibits apoptosis and protects the bronchial epithelium in an allergic inflammation mice model. Int Immunopharmacol. (2019) 73:435–41. doi: 10.1016/j.intimp.2019.05.041
19. Szabo C. Hydrogen sulphide and its therapeutic potential. Nat Rev Drug Discov. (2007) 6:917–35. doi: 10.1038/nrd2425
20. Kimura H. Hydrogen sulfide: its production, release and functions. Amino Acids. (2011) 41:113–21. doi: 10.1007/s00726-010-0510-x
21. Bazhanov N, Ansar M, Ivanciuc T, Garofalo RP, Casola A. Hydrogen sulfide: a novel player in airway development, pathophysiology of respiratory diseases, and antiviral defenses. Am J Respir Cell Mol Biol. (2017) 57:403–10. doi: 10.1165/rcmb.2017-0114TR
22. Kimura H. Production and physiological effects of hydrogen sulfide. Antioxid Redox Signal. (2014) 20:783–93. doi: 10.1089/ars.2013.5309
23. Madden JA, Ahlf SB, Dantuma MW, Olson KR, Roerig DL. Precursors and inhibitors of hydrogen sulfide synthesis affect acute hypoxic pulmonary vasoconstriction in the intact lung. J Appl Physiol. (1985) (2012) 112:411–8. doi: 10.1152/japplphysiol.01049.2011
24. Olson KR, Whitfield NL, Bearden SE, St Leger J, Nilson E, Gao Y, et al. Hypoxic pulmonary vasodilation: a paradigm shift with a hydrogen sulfide mechanism. Am J Physiol Regul Integr Comp Physiol. (2010) 298:R51–60. doi: 10.1152/ajpregu.00576.2009
25. Wang M, Yan J, Cao X, Hua P, Li Z. Hydrogen sulfide modulates epithelial-mesenchymal transition and angiogenesis in non-small cell lung cancer via HIF-1α activation. Biochem Pharmacol. (2020) 172:113775. doi: 10.1016/j.bcp.2019.113775
26. Wang M, Cao X, Luan C, Li Z. Hydrogen sulfide attenuates hydrogen peroxide-induced injury in human lung Epithelial A549 cells. Int J Mol Sci. (2019) 20:3975. doi: 10.3390/ijms20163975
27. Augsburger F, Szabo C. Potential role of the 3-mercaptopyruvate sulfurtransferase (3-MST)-hydrogen sulfide (H2S) pathway in cancer cells. Pharmacol Res. (2020) 154:104083. doi: 10.1016/j.phrs.2018.11.034
28. Hosoki R, Matsuki N, Kimura H. The possible role of hydrogen sulfide as an endogenous smooth muscle relaxant in synergy with nitric oxide. Biochem Biophys Res Commun. (1997) 237:527–31. doi: 10.1006/bbrc.1997.6878
29. Chen T, You Y, Jiang H, Wang ZZ. Epithelial-mesenchymal transition (EMT): a biological process in the development, stem cell differentiation, and tumorigenesis. J Cell Physiol. (2017) 232:3261–72. doi: 10.1002/jcp.25797
30. Pei D, Shu X, Gassama-Diagne A, Thiery JP. Mesenchymal-epithelial transition in development and reprogramming. Nat Cell Biol. (2019) 21:44–53. doi: 10.1038/s41556-018-0195-z
31. Jolly MK, Ward C, Eapen MS, Myers S, Hallgren O, Levine H, et al. Epithelial-mesenchymal transition, a spectrum of states: role in lung development, homeostasis, and disease. Dev Dyn. (2018) 247:346–58. doi: 10.1002/dvdy.24541
32. Guan S, Xu W, Han F, Gu W, Song L, Ye W, et al. Ginsenoside Rg1 attenuates cigarette smoke-induced pulmonary epithelial-mesenchymal transition via inhibition of the TGF- β1/Smad pathway. Biomed Res Int. (2017) 2017:7171404. doi: 10.1155/2017/7171404
33. Guo L, Peng W, Tao J, Lan Z, Hei H, Tian L, et al. Hydrogen sulfide inhibits transforming growth factor-β1-induced EMT via Wnt/catenin pathway. PLoS One. (2016) 11:e0147018. doi: 10.1371/journal.pone.0147018
34. Singh SP, Chand HS, Gundavarapu S, Saeed AI, Langley RJ, Tesfaigzi Y, et al. HIF-1α plays a critical role in the gestational sidestream smoke-induced Bronchopulmonary Dysplasia in mice. PLoS One. (2015) 10:e0137757. doi: 10.1371/journal.pone.0137757
35. Pan Y, Zhou C, Yuan D, Zhang J, Shao C. Radiation exposure promotes Hepatocarcinoma cell invasion through epithelial mesenchymal transition mediated by H2S/CSE pathway. Radiat Res. (2016) 185:96–105. doi: 10.1667/RR14177.1
36. Zhang H, Song Y, Zhou C, Bai Y, Yuan D, Pan Y, et al. Blocking endogenous H2S signaling attenuated radiation-induced long-term metastasis of residual HepG2 cells through inhibition of EMT. Radiat Res. (2018) 190:374–84. doi: 10.1667/RR15074.1
37. Caniggia I, Mostachfi H, Winter J, Gassmann M, Lye SJ, Kuliszewski M, et al. Hypoxia-inducible factor-1 mediates the biological effects of oxygen on human trophoblast differentiation through TGFβ(3). J Clin Invest. (2000) 105:577–87. doi: 10.1172/JCI8316
38. Yoshimoto S, Tanaka F, Morita H, Hiraki A, Hashimoto S. Hypoxia-induced HIF-1α and ZEB1 are critical for the malignant transformation of ameloblastoma via TGF-β-dependent EMT. Cancer Med. (2019) 8:7822–32. doi: 10.1002/cam4.2667
39. Xu J, Lamouille S, Derynck R. TGF-β-induced epithelial to mesenchymal transition. Cell Res. (2009) 19:156–72. doi: 10.1038/cr.2009.5
40. Lamouille S, Xu J, Derynck R. Molecular mechanisms of epithelial-mesenchymal transition. Nat Rev Mol Cell Biol. (2014) 15:178–96. doi: 10.1038/nrm3758
41. Chand HS, Harris JF, Tesfaigzi Y. IL-13 in LPS-induced inflammation causes Bcl-2 expression to sustain hyperplastic mucous cells. Sci Rep. (2018) 8:436. doi: 10.1038/s41598-017-18884-9
42. Li H, Ma Y, Escaffre O, Ivanciuc T, Komaravelli N, Kelley JP, et al. Role of hydrogen sulfide in paramyxovirus infections. J Virol. (2015) 89:5557–68. doi: 10.1128/JVI.00264-15
43. Ivanciuc T, Sbrana E, Casola A, Garofalo RP. Protective role of nuclear factor erythroid 2-related factor 2 against respiratory syncytial virus and human Metapneumovirus infections. Front Immunol. (2018) 9:854. doi: 10.3389/fimmu.2018.00854
44. Ivanciuc T, Sbrana E, Casola A, Garofalo RP. Cystathionine gamma-lyase deficiency enhances airway reactivity and viral-induced disease in mice exposed to side-stream tobacco smoke. Pediatr Res. (2019) 86:39–46. doi: 10.1038/s41390-019-0396-6
45. Morrisey EE, Hogan BL. Preparing for the first breath: genetic and cellular mechanisms in lung development. Dev Cell. (2010) 18:8–23. doi: 10.1016/j.devcel.2009.12.010
46. Hines EA, Sun X. Tissue crosstalk in lung development. J Cell Biochem. (2014) 115:1469–77. doi: 10.1002/jcb.24811
47. Duan L, Ye L, Zhuang L, Zou X, Liu S, Zhang Y, et al. VEGFC/VEGFR3 axis mediates TGFbeta1-induced epithelial-to-mesenchymal transition in non-small cell lung cancer cells. PLoS One. (2018) 13:e0200452. doi: 10.1371/journal.pone.0200452
48. Fantozzi A, Gruber DC, Pisarsky L, Heck C, Kunita A, Yilmaz M, et al. VEGF-mediated angiogenesis links EMT-induced cancer stemness to tumor initiation. Cancer Res. (2014) 74:1566–75. doi: 10.1158/0008-5472.CAN-13-1641
49. Jakkula M, Le Cras TD, Gebb S, Hirth KP, Tuder RM, Voelkel NF, et al. Inhibition of angiogenesis decreases alveolarization in the developing rat lung. Am J Physiol Lung Cell Mol Physiol. (2000) 279:L600–7. doi: 10.1152/ajplung.2000.279.3.L600
50. Kim YE, Park WS, Ahn SY, Sung DK, Chang YS. Intratracheal transplantation of mesenchymal stem cells attenuates hyperoxia-induced lung injury by down-regulating, but not direct inhibiting formyl peptide receptor 1 in the newborn mice. PLoS One. (2018) 13:e0206311. doi: 10.1371/journal.pone.0206311
51. Kalluri R, Weinberg RA. The basics of epithelial-mesenchymal transition. J Clin Invest. (2009) 119:1420–8. doi: 10.1172/JCI39104
52. Nahomi RB, Nagaraj RH. The role of HIF-1α in the TGF-β2-mediated epithelial-to-mesenchymal transition of human lens epithelial cells. J Cell Biochem. (2018) 119:6814–27. doi: 10.1002/jcb.26877
53. Saito A, Horie M, Nagase T. TGF-β signaling in lung health and disease. Int J Mol Sci. (2018) 19:2460. doi: 10.3390/ijms19082460
54. Archer F, Abi-Rizk A, Desloire S, Dolmazon C, Gineys B, Guiguen F, et al. Lung progenitors from lambs can differentiate into specialized alveolar or bronchiolar epithelial cells. BMC Vet Res. (2013) 9:224. doi: 10.1186/1746-6148-9-224
55. Laing IA, Hermans C, Bernard A, Burton PR, Goldblatt J, Le Souef PN. Association between plasma CC16 levels, the A38G polymorphism, and asthma. Am J Respir Crit Care Med. (2000) 161:124–7. doi: 10.1164/ajrccm.161.1.9904073
56. Zhai J, Insel M, Addison KJ, Stern DA, Pederson W, Dy A, et al. Club cell secretory protein deficiency leads to altered lung function. Am J Respir Crit Care Med. (2019) 199:302–12. doi: 10.1164/rccm.201807-1345OC
57. Esechie A, Kiss L, Olah G, Horvath EM, Hawkins H, Szabo C, et al. Protective effect of hydrogen sulfide in a murine model of acute lung injury induced by combined burn and smoke inhalation. Clin Sci (Lond). (2008) 115:91–7. doi: 10.1042/CS20080021
58. Zhang J, Wang X, Chen Y, Yao W. Exhaled hydrogen sulfide predicts airway inflammation phenotype in COPD. Respir Care. (2015) 60:251–8. doi: 10.4187/respcare.03519
59. Li YF, Langholz B, Salam MT, Gilliland FD. Maternal and grandmaternal smoking patterns are associated with early childhood asthma. Chest. (2005) 127:1232–41. doi: 10.1016/S0012-3692(15)34472-X
60. Accordini S, Calciano L, Johannessen A, Portas L, Benediktsdottir B, Bertelsen RJ, et al. A three-generation study on the association of tobacco smoking with asthma. Int J Epidemiol. (2018) 47:1106–17. doi: 10.1093/ije/dyy031
61. Rehan VK, Liu J, Sakurai R, Torday JS. Perinatal nicotine-induced transgenerational asthma. Am J Physiol Lung Cell Mol Physiol. (2013) 305:L501–7. doi: 10.1152/ajplung.00078.2013
62. Levitt MD, Abdel-Rehim MS, Furne J. Free and acid-labile hydrogen sulfide concentrations in mouse tissues: anomalously high free hydrogen sulfide in aortic tissue. Antioxid Redox Signal. (2011) 15:373–8. doi: 10.1089/ars.2010.3525
63. Burstyn I, Kapur N, Shalapay C, Bamforth F, Wild TC, Liu J, et al. Evaluation of the accuracy of self-reported smoking in pregnancy when the biomarker level in an active smoker is uncertain. Nicotine Tob Res. (2009) 11:670–8. doi: 10.1093/ntr/ntp048
64. Silvestri M, Franchi S, Pistorio A, Petecchia L, Rusconi F. Smoke exposure, wheezing, and asthma development: a systematic review and meta-analysis in unselected birth cohorts. Pediatr Pulmonol. (2015) 50:353–62. doi: 10.1002/ppul.23037
65. Xu S, Liu Z, Liu P. Targeting hydrogen sulfide as a promising therapeutic strategy for atherosclerosis. Int J Cardiol. (2014) 172:313–7. doi: 10.1016/j.ijcard.2014.01.068
66. Wang P, Wu L, Ju Y, Fu M, Shuang T, Qian Z, et al. Age-dependent allergic asthma development and cystathionine gamma-lyase deficiency. Antioxid Redox Signal. (2017) 27:931–44. doi: 10.1089/ars.2016.6875
67. Krasilnikov MA. Phosphatidylinositol-3 kinase dependent pathways: the role in control of cell growth, survival, and malignant transformation. Biochemistry (Mosc). (2000) 65:68–78.
68. Alphonse RS, Vadivel A, Coltan L, Eaton F, Barr AJ, Dyck JR, et al. Activation of Akt protects alveoli from neonatal oxygen-induced lung injury. Am J Respir Cell Mol Biol. (2011) 44:146–54. doi: 10.1165/rcmb.2009-0182OC
69. Liu WL, Liu ZW, Li TS, Wang C, Zhao B. Hydrogen sulfide donor regulates alveolar epithelial cell apoptosis in rats with acute lung injury. Chin Med J (Engl). (2013) 126:494–9. doi: 10.3760/cma.j.issn.0366-6999.20120809
70. Ivanciuc T, Sbrana E, Ansar M, Bazhanov N, Szabo C, Casola A, et al. Hydrogen sulfide is an antiviral and antiinflammatory endogenous gasotransmitter in the airways. Role in respiratory syncytial virus infection. Am J Respir Cell Mol Biol. (2016) 55:684–96. doi: 10.1165/rcmb.2015-0385OC
71. Longchamp A, Mirabella T, Arduini A, MacArthur MR, Das A, Trevino-Villarreal JH, et al. Amino acid restriction triggers angiogenesis via GCN2/ATF4 regulation of VEGF and H2S production. Cell. (2018) 173:117–29.e14. doi: 10.1016/j.cell.2018.03.001
72. Madurga A, Mizikova I, Ruiz-Camp J, Vadasz I, Herold S, Mayer K, et al. Systemic hydrogen sulfide administration partially restores normal alveolarization in an experimental animal model of bronchopulmonary dysplasia. Am J Physiol Lung Cell Mol Physiol. (2014) 306:L684–97. doi: 10.1152/ajplung.00361.2013
73. Vadivel A, Alphonse RS, Ionescu L, Machado DS, O'Reilly M, Eaton F, et al. Exogenous hydrogen sulfide (H2S) protects alveolar growth in experimental O2-induced neonatal lung injury. PLoS One. (2014) 9:e90965. doi: 10.1371/journal.pone.0090965
74. Bush A. Lung development and aging. Ann Am Thorac Soc. (2016) 13(Suppl. 5):S438–46. doi: 10.1513/AnnalsATS.201602-112AW
Keywords: gestational cigarette smoke, H2S biogenesis, human placenta, lungs, transgenerational effect
Citation: Singh SP, Devadoss D, Manevski M, Sheybani A, Ivanciuc T, Exil V, Agarwal H, Raizada V, Garofalo RP, Chand HS and Sopori ML (2020) Gestational Exposure to Cigarette Smoke Suppresses the Gasotransmitter H2S Biogenesis and the Effects Are Transmitted Transgenerationally. Front. Immunol. 11:1628. doi: 10.3389/fimmu.2020.01628
Received: 28 February 2020; Accepted: 17 June 2020;
Published: 28 July 2020.
Edited by:
Christian Herr, Saarland University Hospital, GermanyReviewed by:
Tanima Bose, Ludwig Maximilian University of Munich, GermanyIrene Marafini, Policlinico Tor Vergata, Italy
Copyright © 2020 Singh, Devadoss, Manevski, Sheybani, Ivanciuc, Exil, Agarwal, Raizada, Garofalo, Chand and Sopori. This is an open-access article distributed under the terms of the Creative Commons Attribution License (CC BY). The use, distribution or reproduction in other forums is permitted, provided the original author(s) and the copyright owner(s) are credited and that the original publication in this journal is cited, in accordance with accepted academic practice. No use, distribution or reproduction is permitted which does not comply with these terms.
*Correspondence: Mohan L. Sopori, bXNvcG9yaUBscnJpLm9yZw==