- 1School of Biological Sciences, Southampton General Hospital, University of Southampton, Southampton, United Kingdom
- 2Dundee Imaging Facility, School of Life Sciences, University of Dundee, Dundee, United Kingdom
- 3Centre for Therapeutics Discovery, LifeArc, Accelerator Building, Stevenage, United Kingdom
- 4Eli Lilly Research Centre, Surrey, United Kingdom
- 5Eisai Limited, European Knowledge Centre, Hertfordshire, United Kingdom
- 6Abbvie, Foundational Neuroscience Centre, Cambridge, MA, United States
- 7Alzheimer's Research UK, Cambridge, United Kingdom
The proliferation and activation of microglia, the resident macrophages in the brain, is a hallmark of many neurodegenerative diseases such as Alzheimer's disease (AD) and prion disease. Colony stimulating factor 1 receptor (CSF1R) is critically involved in regulating microglial proliferation, and CSF1R blocking strategies have been recently used to modulate microglia in neurodegenerative diseases. However, CSF1R is broadly expressed by many cell types and the impact of its inhibition on the innate immune system is still unclear. CSF1R can be activated by two independent ligands, CSF-1 and interleukin 34 (IL-34). Recently, it has been reported that microglia development and maintenance depend on IL-34 signaling. In this study, we evaluate the inhibition of IL-34 as a novel strategy to reduce microglial proliferation in the ME7 model of prion disease. Selective inhibition of IL-34 showed no effects on peripheral macrophage populations in healthy mice, avoiding the side effects observed after CSF1R inhibition on the systemic compartment. However, we observed a reduction in microglial proliferation after IL-34 inhibition in prion-diseased mice, indicating that microglia could be more specifically targeted by reducing IL-34. Overall, our results highlight the challenges of targeting the CSF1R/IL34 axis in the systemic and central compartments, important for framing any therapeutic effort to tackle microglia/macrophage numbers during brain disease.
Introduction
Neuroinflammation is a critical component of neurodegenerative diseases, including Alzheimer's disease (AD), Parkinson's disease (PD), or prion diseases. The neuroinflammatory process is characterized by a robust activation of the innate immune system, with an increase in the number of microglial cells associated with an activated and phagocytic phenotype (1–3). Experimental models of prion disease present several shared features of neurodegenerative diseases including protein misfolding, progressive synaptic degeneration followed by loss of neurons, microglial activation, and production of inflammatory cytokines and chemokines (4).
The contribution of local proliferation of microglia, regulated by the activation of the colony stimulating factor 1 receptor (CSF1R), has been shown as a disease-modifying mechanism during the progression of the ME7 prion model of progressive chronic neurodegeneration (5). Similarly, a prolonged inhibition of the tyrosine kinase activity of CSF1R blocks microglial proliferation and prevents synaptic degeneration, ameliorating disease progression, in the APP/PS1 model (6), the 3xTg model (7), and the 5xFAD model (8, 9) of AD-like pathology. More recently, our group has validated this disease-modifying mechanism in the P301S mouse of tauopathy, demonstrating that inhibition of CSF1R is effective in repolarising microglia to a homeostatic phenotype, preventing neuronal degeneration (10). In recent years, the therapeutic potential of blocking antibodies and small molecule CSF1R kinase inhibitors has been demonstrated in inflammatory diseases, neurological disorders, bone diseases, and cancer, with some candidates currently in clinical phase testing (11–13). However, the broader impact of this approach on the innate immune system remains unclear. CSF1R is expressed by cells of the myeloid lineage (14) and therefore it is anticipated that the inhibition of CSF1R would not only affect microglia, but also have potential on-target effects in circulating and tissue-resident myeloid populations, with a possible downstream immunosuppressive effect. CSF1R can be activated by two independent ligands with high affinity, the colony stimulating factor 1 (CSF-1) (15) and the recently identified interleukin 34 (IL-34) (16). A potential avenue to block this pathway more selectively is the specific modulation of the binding of its ligands, to increase tissue specificity and reduce side effects. Both ligands have been shown to promote microglial proliferation (5) but also show a differential temporal and spatial pattern of expression. Whereas CSF-1 is broadly expressed (spleen, lymph nodes, cortex, bone marrow, amongst others), the expression of IL-34 is restricted to a few tissues, predominantly produced in the skin and the brain, with minimal overlap with the CSF-1 expression pattern (17, 18). Interestingly, mice lacking IL-34 (Il34LacZ) displayed a marked reduction of Langerhans cells in the skin and microglial cells in the brain, whereas other tissue macrophages were unaffected, showing that IL-34 specifically controls the development and maintenance of these populations (19, 20).
Since IL-34 has been shown to be a tissue-restricted ligand of CSF1R, and it is crucial for the differentiation and maintenance of microglial cells in the brain, we aimed to investigate whether IL-34 inhibition could be used as a selective approach to reduce microglial proliferation during chronic neurodegeneration with minimal effects on other tissue-resident myeloid populations. Here, we first describe the effects of the selective inhibition of IL-34, compared to CSF1R inhibition, on different tissue-resident populations in healthy mice, supporting tissue-selectivity of IL-34. We also demonstrate that IL-34 inhibition decreases the proliferation of microglial cells in the ME7 prion model, showing that IL-34 is involved in the regulation of microglial proliferation and supporting that the inhibition of this cytokine could be used as a more selective approach to modulate microglial proliferation in neurodegenerative diseases.
Methods
In vitro Assessment of CSF1R Phosphorylation
The N13 murine microglia cell line (21) was cultured in Dulbecco's modified Eagle's medium (DMEM, Thermo Fisher Scientific), supplemented with 10% fetal bovine serum and 50 U/mL penicillin/ streptomycin (Thermo Fisher Scientific). Cells were maintained in T75 flasks at 37°C in a 5% CO2 humidified atmosphere. Cells were plated at a density of 2 × 105 cells/cm2 in 6-well-plates and cultured overnight to allow adherence. Cells were plated at a density of 2 × 105 cells/cm2 in 6-well-plates and cultured overnight to allow adherence. Cells were kept in serum-free medium for 4 h prior to stimulation and then incubated for the indicated time points (5 or 10 min) with recombinant CSF-1 (50 or 100 ng/mL), IL-34 (50 or 100 ng/mL) (R&D Systems) or LPS (1 μg/mL) as a negative control for CSF1R pathway activation (22, 23), after which cells were immediately lysed in RIPA buffer (Thermo Fisher Scientific), supplemented with protease and phosphatase inhibitor cocktails (Roche, Thermo Fisher Scientific). Protein lysates were concentrated using Microcon-10kDa Centrifugal Filter Units (Merck Millipore), according to manufacturer's instructions and protein concentration was determined using the Pierce BCA Protein Assay Kit (Thermo Fisher Scientific). Protein lysates were subjected to SDS-PAGE and Western blot.
In vitro Assessment of IL-34 Neutralizing Antibodies Using CellTiter Glo
Mouse myelogenous leukemia (M-NFS-60) cells were CSF-1 (R&D systems, 216-MC/CF) starved for 24 h. In white clear bottom 96-well-plates 10 μL IL-34 antibody (mouse monoclonal IgG2A (v1.1 manufactured by Genscript, (24, 25)), rat monoclonal IgG2A (MAB5195, R&D Systems) and sheep polyclonal IgG (AF5195, R&D Systems) and 10 μL IL-34 stimulus (R&D systems, 5195-ML-CF) were incubated at 37°C for 30 min before 80 μL M-NFS-60 cells (103 cells/well) were added. After two days incubation at 37°C cell viability was assessed using CellTiterGlo (Promega, G7570) following manufacturer's instructions. Hundred microliter reconstituted CellTiterGlo was added per well, plates were shaken for 2 min and incubated at room temperature for 10 min before luminescence was read.
Experimental Model of Prion Disease and Pharmacological Treatments
C57BL/6J mice (Harlan laboratories) and c-fms-eGFP (macgreen) mice (26), characterized by eGFP expression under the c-fms (CSF1R) promoter, were bred and maintained in local facilities. Mice were housed in groups of 4–10, under a 12 h light/12 h dark cycle at 21°C, with food and water ad libitum. To induce prion disease 6 weeks old C57BL/6J or macgreen mice were anesthetized with a ketamine/xylazine mixture (85 and 13 mg/kg), and 1 μL of ME7-derived (ME7 group) brain homogenate (10% w/v) was injected stereotaxically and bilaterally at the dorsal hippocampus, coordinates from bregma: anteroposterior, −2.0 mm; lateral, ±1.7 mm; depth, −1.6 mm. Naïve animals were used as controls. All procedures were performed in accordance with U.K. Home Office licensing. Group sizes were defined after performing power calculations, in order to achieve a significant difference of p < 0.05, in light of a retrospective analysis of our previous published results, to reach a power between 0.80 and 0.90, depending on the specific experimental conditions. The effect size was calculated taking into consideration the strength of association between the variables, the sensitivity, and the variation of any dependent variable. The calculations are the customary ones based on normal distributions.
For chronic treatment of healthy mice, rat monoclonal CSF1R-blocking antibodies (BE0213, Bio X Cell) and rat monoclonal IL-34 antibodies (MAB5195, R&D Systems) were diluted in PBS, pH 7.4 and administered by intraperitoneal injections 3 times a week for 3 weeks at a dose of 250 μg per injection. For chronic treatment in ME7 prion mice, mouse monoclonal IL-34 IgG2A (24) was administered biweekly for 4 weeks at a dose of 60 mg/kg, starting 12 weeks after prion inoculation. For acute treatment in ME7 prion mice, 1 μg of mouse or human-specific IL-34 neutralizing antibody (sheep polyclonal IgG, AF5195, or AF5265, R&D Systems) was stereotaxically and bilaterally injected into the dorsal hippocampus, coordinates from bregma: anteroposterior, ?2.0 mm; lateral, ±1.7 mm; depth, ?1.6 mm, 12 weeks after induction of prion disease. Mice received daily intraperitoneal BrdU injections (7.5 mg/mL, 0.1 mL/10 g weight in sterile saline; Sigma-Aldrich) and were sacrificed 1 week after intracerebral antibody administration. Weight of the mice was monitored in all experiments, and no differences were observed between treated and untreated groups. All the experimental groups were randomized to avoid gender and cage effects, and all the experiments were performed double-blinded.
Histology
Mice were terminally anesthetized with an overdose of sodium pentobarbital and transcardially perfused with heparinised 0.9% saline. Brain and peripheral organs (liver, kidney, and spleen) were fixed in 4% paraformaldehyde overnight and immersed in 30% sucrose in PBS for at least 24 h. Tissue was cut in serial sections (35 μm thick, coronal sections of the brain) with a cryostat (Leica) and stored free-floating in cryoprotectant solution [30% sucrose, 30% ethylene glycol, 1% Polyvinyl-pyrrolidone (PVP-40) in 0.1 M PB, pH 7.4] at −20°C. For histological analysis of peripheral organs, 4–6 sections from each organ were randomly selected. For analysis of cortex, hippocampal CA1 and dentate gyrus, every 6th systematically sampled section, spanning the entire area of the hippocampus and totalling 6–9 sections were used for quantification. Tissue sections taken from macgreen mice were directly mounted on slides with Mowiol/DABCO (Sigma-Aldrich) mixture. Immunohistochemistry of brain sections from C57BL/6J mice was performed as previously described (5). Briefly, sections were subjected to DNA denaturation with 2N HCl (30 min, 37°C), followed by incubation with 5% normal serum and 5% BSA in PBS to block non-specific binding. After rinses with PBS-0.1% Tween 20 (PBST), sections were incubated overnight at 4°C with rabbit anti-Iba1 (Wako, 019-19741) and anti-BrdU (Biorad, MCA2060). After washes with PBST, sections were incubated with host-specific Alexa 488- and 568-conjugated secondary antibodies (Invitrogen). Brain sections and sections of peripheral organs from macgreen mice mounted with Mowiol/DABCO were imaged with a Leica DM5000B microscope coupled to a Leica DFC300FX camera. Iba1-positive, BrdU/Iba1 double positive and eGFP-positive cells in brain and organs of macgreen mice were counted using the cell counter tool of the ImageJ software and cell number was normalized to the quantified area.
Analysis of Skin-Resident Langerhans Cells
Ears were fixed in 4% paraformaldehyde overnight and then stored in PBS. The ears were split into dorsal and ventral halves and each pair was mounted on slide under coverslip mounted in Vectashield anti-fade mounting medium. For each half ear, 5 fields were chosen at random and 0.9-μm thick sections were collected on a LSM700 confocal microscope (Zeiss) using settings for eGFP fluorescence with a 40 × objective. For each field a z-stack was taken to cover the full thickness of the Langerhans cells layer-typically 5–100 images depending on the flatness of the ear. Cell volume and number were measured using Volocity software (Quorum Technologies). Cells were identified as those with a GFP intensity >2SD from the mean image intensity. Non-cellular objects <200 or >5,000 μm3 were excluded. Objects with a long axis >100 μm were also excluded-this eliminated most auto-fluorescent hairs in the z stacks. Each image was checked manually to remove cell doublets and unexcluded hair profiles.
Flow Cytometric Analysis of Brain and Blood Samples
Mice were terminally anesthetized with an overdose of sodium pentobarbital and transcardially perfused with heparinised 0.9% saline. Brain hemispheres were harvested in PBS with 2% FCS and 2 mM EDTA (FACS buffer) and mechanically triturated and enzymatically dissociated using the Neural Tissue Dissociation Kit (P) (Miltenyi), according to manufacturer's instructions. Samples were passed through a cell strainer of 70 μm mesh (BD2 Falcon) with FACS buffer, and centrifuged at 500 × g for 10 min at 4°C. After the second wash, cells were re-suspended in 37% Percoll (GE Healthcare) and centrifuged at 500 × g for 30 min at 18°C. The supernatant and myelin layers were discarded, and the cell pellet enriched with mononuclear cells was resuspended in FACS buffer. Blood samples were harvested by cardiac puncture and collected in EDTA-coated tubes. Brain cells and blood samples were split in several tubes and immunostained. Primary antibody labeling was performed for 1 h at 4°C, using the following antibodies: rat-anti-mouse CD11b (BD Biosciences, clone M1/70), rat-anti-mouse CD45 (Biolegend, clone 30-F11) and rat-anti-mouse Ly6C (BD Biosciences, clone AL-21) and Fixable Viability Dye eFluor™ 450 (eBioscience). Moreover, unstained cells and isotype-matched control samples were used to control for autofluorescence and non-specific binding of antibodies. After staining, erythrocytes in blood samples were lysed in RBC lysis buffer (eBioscience). Cells were washed and resuspended in FACS buffer. Samples were run on a BD FACS Aria Flow cytometer, recording 100,000 events per sample. Data was analyzed using FlowJo software.
SDS-PAGE and Western Blot
Frozen brain samples and peripheral organs were homogenized in T-PER™ Tissue Protein Extraction Reagent (Thermo Fisher), N13 cells were lysed in RIPA buffer (Thermo Scientific), supplemented with protease inhibitors (EASYpack, Roche) and phosphatase inhibitors (PhosSTOP, Roche). Homogenates were centrifuged at 13,000 rpm and the supernatant was collected. Protein was quantified using BCA assay (Thermo Fisher) following the manufacturer's instructions.
40 μg protein of N13 cell lysates was loaded to 7.5% Mini-PROTEAN® TGX Stain-Free™ Protein Gels (BioRad) and transferred to nitrocellulose membrane using the Trans-Blot® Turbo™ RTA Mini Transfer Kit (BioRad). After blocking with 5% BSA in TBS/0.1% Tween20, membranes were incubated with a combination of rabbit polyclonal antibodies against phospho-M-CSF receptor (Tyr546, Tyr708, Tyr723, and Tyr923, Cell signaling), phospho-AKT (Ser473, Cell signaling), or phospho-p44/42 MAPK (Erk1/2) (Thr202/Tyr204, Cell signaling) over night at 4°C. Membranes were washed in TBS and further incubated with an HRP-labeled anti-rabbit IgG (BioRad) for 2 h at room temperature. Membranes were incubated with the SuperSignal™ West Pico Chemiluminescent Substrate (Thermo Fisher Scientific) and signal was detected on the ChemiDoc Imaging System (BioRad). Membranes were stripped using the Restore™ Western Blot Stripping Buffer (Thermo Fisher Scientific) and reprobed with mouse monoclonal CSF-1R antibody (D-8, Santa Cruz Biotechnology), anti-AKT (Cell signaling), or anti-ERK1/ERK2 antibody (9B3, abcam), followed by HRP-labeled anti-mouse or anti-rabbit IgG antibody (BioRad). Intensity of protein bands was quantified using Adobe Photoshop.
Elisa
Nunc MaxiSorp™ flat-bottom 96-well-plates (Thermo Scientific) were coated with F(ab')2 fragment anti-rat or anti-mouse IgG (H+L) (Jackson ImmunoResearch) overnight. Plates were washed with PBS + 0.05% Tween20 and incubated with blocking buffer (PBS containing 0.05% Tween20 and 1% BSA) to block non-specific binding sites. Plasma samples or tissue lysates diluted in blocking buffer were incubated for 2 h or overnight. A standard was generated using respective anti-IL-34 or anti-CSF1R antibodies that were used for in vivo treatment. After washing, plates were incubated with peroxidase-conjugated anti-rat or anti-mouse Fcγ subclass 2a-specific IgG (Jackson ImmunoResearch) for 2 h, then washed and incubated with 1-Step™ Ultra TMB-ELISA Substrate Solution (Thermo Scientific). The reaction was stopped with 2N H2SO4 and the signal was measured on a plate reader at 450 nm.
CSF-1 and IL-34 in plasma or brain were measured by commercially available immunoassays (R&D systems), according to manufacturer's instructions.
Statistics
Data are shown as mean ± SEM and were analyzed using the GraphPad Prism 6 software package (GraphPad Software), using two-way ANOVA with Tukey's post-hoc test for multiple comparisons, Student's t-test or one-way ANOVA followed by Tukey's post-hoc test for multiple comparisons, as indicated. Differences were considered significant at p < 0.05.
Results
IL-34 Induces Activation of the CSF1R Signaling Pathway and IL-34-Mediated Cell Growth Can Be Inhibited Using Neutralizing Antibodies
To investigate whether IL-34 activates the CSF1R pathway in microglia, we stimulated a murine microglia cell line (N13) with either IL-34 or with CSF-1 and analyzed tyrosine phosphorylation of the receptor and downstream signaling molecules ERK1/ERK2 and AKT. Stimulation with either IL-34 or CSF-1 leads to increased phosphorylation of CSF1R and downstream mediators, indicating that IL-34 binds to and activates the CSF1R pathway, triggering downstream signaling pathways related to survival and proliferation (Figure 1A). IL-34-mediated growth of myelogenous leukemia cell line M-NFS-60 can be inhibited by three different IL-34 neutralizing antibodies, which were further used in this study and showed similar potencies [mouse monoclonal v1.1 (25): IC50 0.43 nM, rat monoclonal MAB5195: IC50 0.53 nM, sheep polyclonal AF5195: IC50 2.05 Nm] (Figure 1B), indicating that CSF1R-dependent signaling can be modulated by IL-34 inhibition.
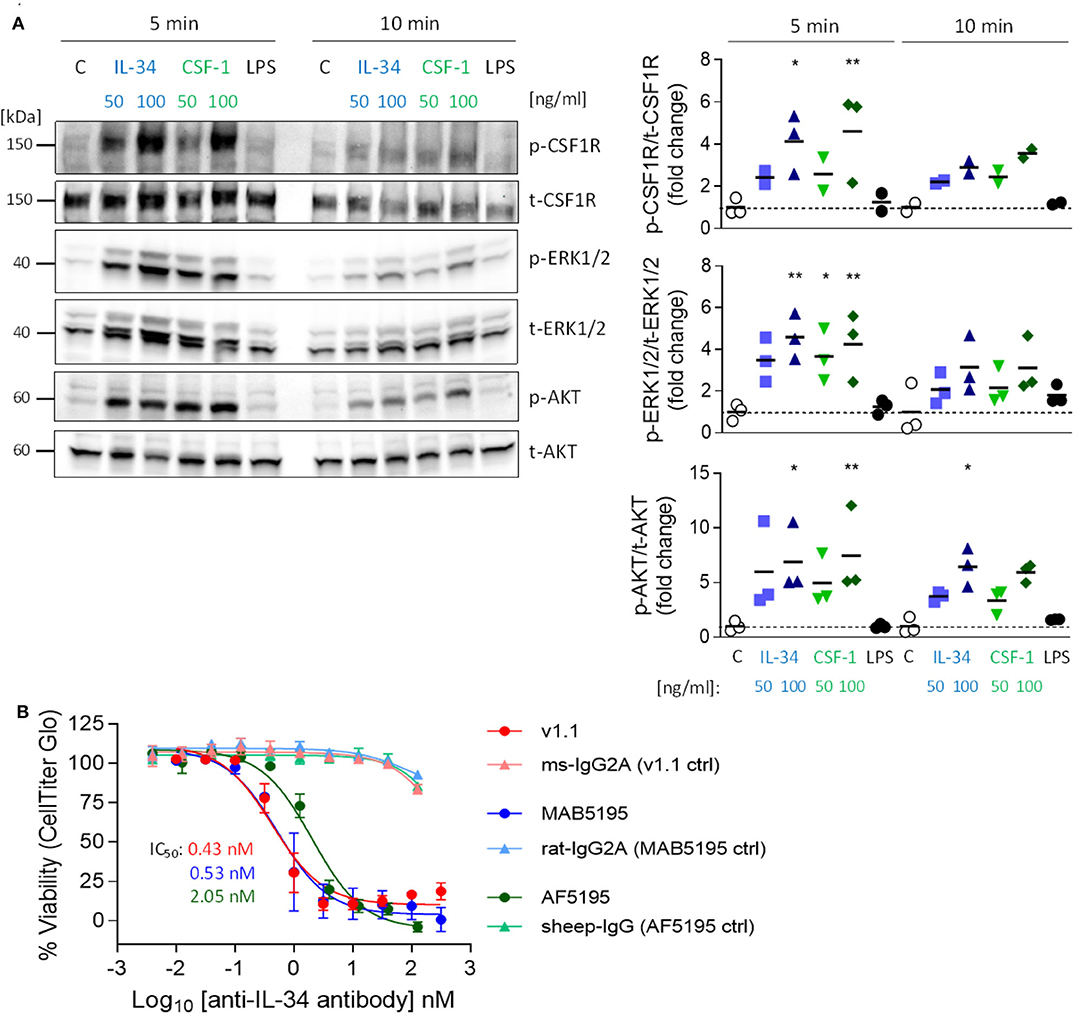
Figure 1. Activation of the CSF1R signaling pathway by IL-34 and CSF-1 and inhibition of IL-34-mediated cell growth using IL-34 neutralizing antibodies. (A) N13 microglia cell line was stimulated with IL-34 (50 or 100 ng/mL), CSF-1 (50 or 100 ng/mL) or LPS (1 μg/mL) for 5 or 10 min. Cell lysates were subjected to Western blotting which showed increased phosphorylation of CSF1R and downstream ERK1/2 and AKT after IL-34 and CSF-1 stimulation. (B) IL-34 neutralizing antibodies used in this study (mouse monoclonal IgG2A [v1.1, (24)), rat monoclonal IgG2A (MAB5195, R&D Systems) and sheep polyclonal IgG (AF5195, R&D Systems)] prevented IL-34-dependent cell growth of M-NFS-60 in a similar nanomolar range. n = 3, data shown represent mean ± SEM, two-way ANOVA followed by Tukey's multiple comparison test. *p < 0.05, **p < 0.01, ***p < 0.001, comparisons are stimulations vs. unstimulated control (C) for each time point.
CSF1R- but Not IL-34 Antibody-Mediated Inhibition Leads to a Reduction of CSF1R+/Ly6Clo Blood Monocytes
To determine the effect of CSF1R vs. IL-34 blockade on blood immune cells, macgreen mice were treated by intraperitoneal injections of CSF1R- or IL-34- neutralizing antibodies (monoclonal rat IgG2a, 250 μg per injection, 3 injections per week), vehicle (PBS), or rat IgG2a isotype for 3 weeks (Figure 2A). The use of macgreen mice allows to monitor the abundance of CSF1R+ cells based on the csf1r-eGFP transgene reporter expression. Measuring antibody titers in the plasma following treatment using a rat IgG2a-specific ELISA showed comparable levels of antibody in anti-IL-34 and anti-CSF1R treated mice, while levels of isotype control were found to be significantly higher (Figure 2B). Administration of CSF1R antibodies, but not IL-34 antibodies, increased CSF-1 levels in the plasma (Figure 2C), which has been described as an indication of target engagement (27). Levels of IL-34 were found to be low in the plasma at baseline (~30 times lower than CSF-1 levels) and were increased after CSF1R antibody treatment similar to CSF-1 levels, while IL-34 was undetectable after IL-34 antibody treatment (Figure 2D). Flow cytometric analysis of blood immune cells demonstrated a significant decrease in CSF1R+ monocytes after CSF1R antibody treatment, which, although a slight trend toward a reduction in cell number can be observed, was not significant after IL-34 antibody administration (Figure 2E, for gating strategy see Supplementary Figure 1). Further analysis of CSF1R+ subpopulations showed that the reduction in blood monocytes after CSF1R antibody treatment is largely due to an effect on cells with high CSF1R expression (eGFPhi) as opposed to cells with intermediate CSF1R expression (eGFPint, Figure 2E). The anti-CSF1R-sensitive eGFPhi cells have been previously identified as CD14+ CD16++ human non-classical monocytes, while the eGFPint population constitutes the CD14++ CD16+/− intermediate and classical monocytes (28), equivalent to the murine non-classical Ly6Clo monocytes and the classical Ly6Chi monocytes, respectively. In accordance with an effect on eGFPhi cells, the non-classical Ly6Clo monocytes were predominantly reduced after anti-CSF1R treatment, while classical Ly6Chi were not affected (Figure 2F). Again, both Ly6Chi and Ly6Clo populations were not significantly reduced after IL-34 antibody administration. In contrast to blood immune cells, CSF1R+ cells in the bone marrow were not affected by either CSF1R or IL-34 antibody treatment, indicating that CSF1R is not required for the differentiation of myeloid progenitors in the marrow (Supplementary Figures 2A,B). Taken together, these results indicate that the number of CSF1R+ monocytes in the blood depends on CSF1R, and is less dependent on IL-34.
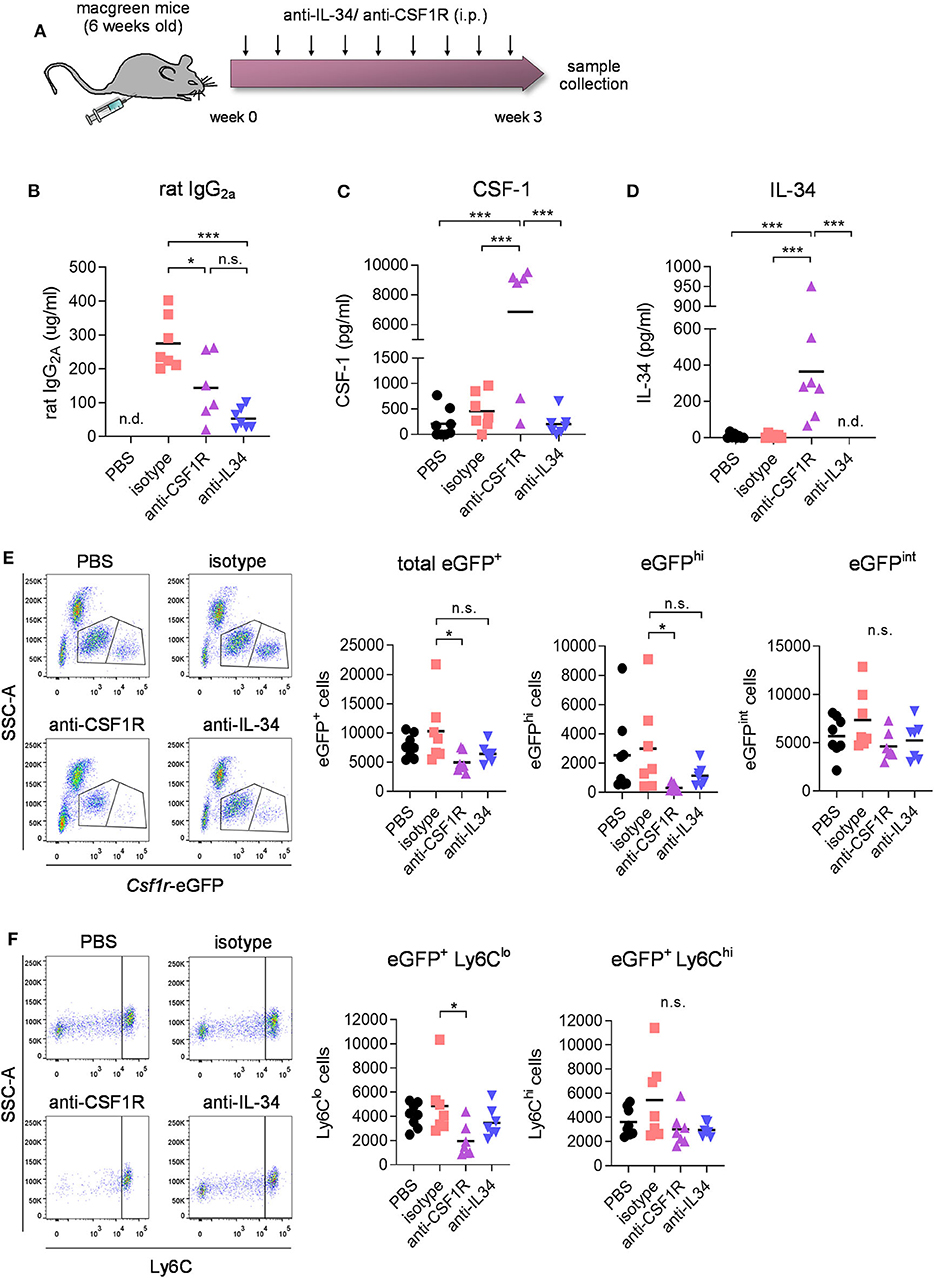
Figure 2. Effect of CSF1R- and IL-34 antibody treatment on blood immune cell compartment. (A) Macgreen mice were treated with anti-CSF1R or anti-IL-34 (both rat monoclonal IgG2A) by intraperitoneal injections of 250 μg antibody 3x a week for 3 weeks. (B–D) Levels of rat IgG2a, CSF-1 and IL-34 were measured in blood plasma after the treatment by ELISA, which showed increased IL-34 and CSF-1 levels after CSF1R- but not IL-34 blockade. (E) Flow cytometric analysis of CSF1R+ cells in the blood of anti-CSF1R- and anti-IL-34- treated mice demonstrated a significant reduction after CSF1R- but not IL-34 antibody treatment. Graphs indicate respective cell numbers per 5 × 104 CD45+ cells. (F) Flow cytometry of eGFP+ Ly6Chi and eGFP+ Ly6Clo subpopulations of CSF1R-expressing cells in the blood shows a reduction in Ly6Clo cells after anti-CSF1R treatment, while Ly6Chi cells were not affected. Graphs indicate respective cell numbers per 5 × 104 CD45+ cells. PBS n = 8, isotype n = 8, anti-CSF1R n = 8, anti-IL-34 n = 7, data shown represent mean ± SEM, two-way ANOVA followed by Tukey's multiple comparison test. *p < 0.05, **p < 0.01, ***p < 0.001.
Systemic IL-34 Blockade Reduces Epidermal Langerhans Cells, but Not Macrophage Populations in Liver and Kidney or Microglia in the Brain
We next aimed to determine the effect of IL-34 and CSF1R antibody treatment on different populations of tissue-resident macrophages. Measurement of rat IgG2a levels in liver, kidney, spleen, and brain by ELISA showed equal distribution of IL-34- and CSF1R- neutralizing antibodies in each organ, with a distribution between different organs from highest to lowest as follows: spleen > kidney > liver > brain (Supplementary Figure 3). Administration of IL-34 neutralizing antibodies for 3 weeks did not change the number of CSF1R+ macrophages in the liver and in the kidney (Figure 3A). In contrast treatment with a CSF1R blocking antibody lead to a pronounced reduction of macrophages in both organs, demonstrating a 41% reduction in liver-resident macrophages and a 85% reduction of macrophages in the kidney (Figure 3A). Skin-resident CSF1R+ Langerhans cells, were significantly decreased after treatment with either IL-34- or CSF1R blocking antibodies (Figure 3A). This indicates that skin-resident Langerhans cells depend on IL-34- mediated signaling through CSF1R, while macrophages in liver and kidney are maintained by IL-34- independent CSF1R signaling.
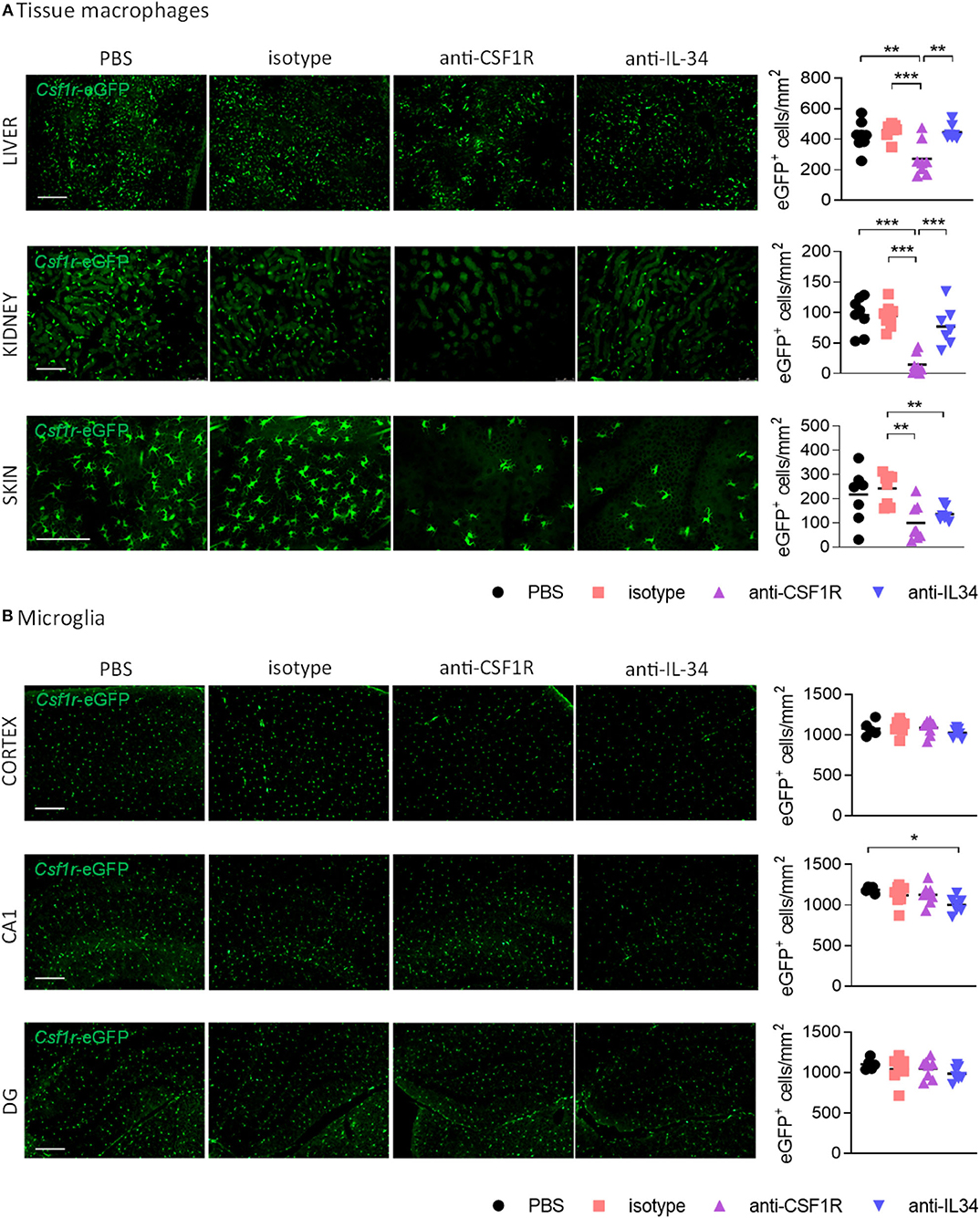
Figure 3. Effect of CSF1R- and IL-34 antibody treatment on tissue macrophages. (A) Histological analysis of CSF1R-positive cells in liver and kidney shows a reduction after anti-CSF1R treatment, but not after anti-IL-34 treatment. Skin Langerhans cells were significantly reduced after both CSF1R – and IL-34 blockade. (B) Microglia in cortex, hippocampal CA1 and dentate gyrus were not majorly affected by CSF1R or IL-34 antibody administration. Scale bar 100 μm. PBS n = 8, isotype n = 8, anti-CSF1R n = 8, anti-IL-34 n = 7, data shown represent mean ± SEM, two-way ANOVA followed by Tukey's multiple comparison test. *p < 0.05, **p < 0.01, ***p < 0.001.
To find out whether IL-34 antibody treatment affects microglia number in the brain we quantified the number of CSF1R+ cells in the cerebral cortex, hippocampal CA1, and dentate gyrus. Peripheral administration of CSF1R or IL-34 blocking antibodies for 3 weeks did not overtly affect the number of microglia in the brain, with only a small reduction in the CA1 region of the hippocampus observed after anti-IL-34 administration (Figure 3B). Since previous reports showed that CSF1R inhibition using small molecule inhibitors leads to a reduction in microglia (5), it is likely that we did not reach optimal antibody penetration into the brain with the administered dose of antibody to achieve sufficient target engagement.
Chronic Systemic Administration of IL-34 Blocking Antibody Lacks Sufficient Central Target Engagement, Not Modifying the Microglial Population in ME7 Prion Mice
We next aimed to investigate whether chronic systemic IL-34 antibody treatment would affect microglia numbers in the ME7 prion mice, a model of neurodegeneration which is characterized by a pronounced expansion of the microglia population (5). Based on our previous observation showing a lack of effect in the brain with 250 μg antibody per injection (~10 mg/kg, Figure 3B), we increased the administered dose to 60 mg/kg per injection (Figure 4A). After 4 weeks of biweekly intraperitoneal injections of the antibody in prion diseased mice, microglia populations were analyzed by flow cytometry and histology. While ME7 prion mice showed increased numbers of microglia compared to naïve animals, as assessed by flow cytometry (CD45int CD11b+ cells) and histology (Csf1r-eGFP+ cells), there was no difference in microglia numbers in brains of anti-IL-34 treated animals compared to control-treatment (Figures 4B,C). The proportion of CD45hi CD11b+ cells was not affected by disease or treatment, indicating no effect on infiltration of peripheral myeloid cells into the brain (Figure 4B). Levels of isotype and anti-IL-34 antibody were detectable in plasma and brain using a mouse-IgG2a specific ELISA, which revealed higher levels of isotype compared to IL-34 antibody in both compartments, with a brain/plasma ratio of 0.165 for the isotype and 0.141 for the IL-34 antibody (Figure 4D). CSF-1 levels in the brain were significantly increased in prion mice compared to naïve mice, but unaffected by the anti-IL34 treatment (Figure 4E). IL-34 levels were around 300 times higher in the naïve brain than CSF-1 levels, but not changed in the context of prion disease or after IL-34 antibody treatment (Figure 4E). In order to determine whether the absence of an effect of IL-34 antibody treatment on microglia numbers could be due to insufficient target engagement, we developed an ELISA to capture mouse IgG2a from brain lysates, followed by detection of IL-34 molecules bound to captured IgG2a. Using this assay we detected bound IL-34 exclusively in brain lysates of mice treated with IL-34 antibody, but not with isotype or PBS (Figure 4F). The percentage of IL-34 bound to IL-34 antibody showed a dose dependent increase after 2 injections in healthy mice, demonstrating increased target engagement with increasing doses of IL-34 antibody (Figure 4F). However, ME7 prion mice treated for 4 weeks with biweekly injections of 60 mg/kg showed that only ~13% of total IL-34 was bound to the antibody, suggesting a low degree of target engagement (Figure 4F).
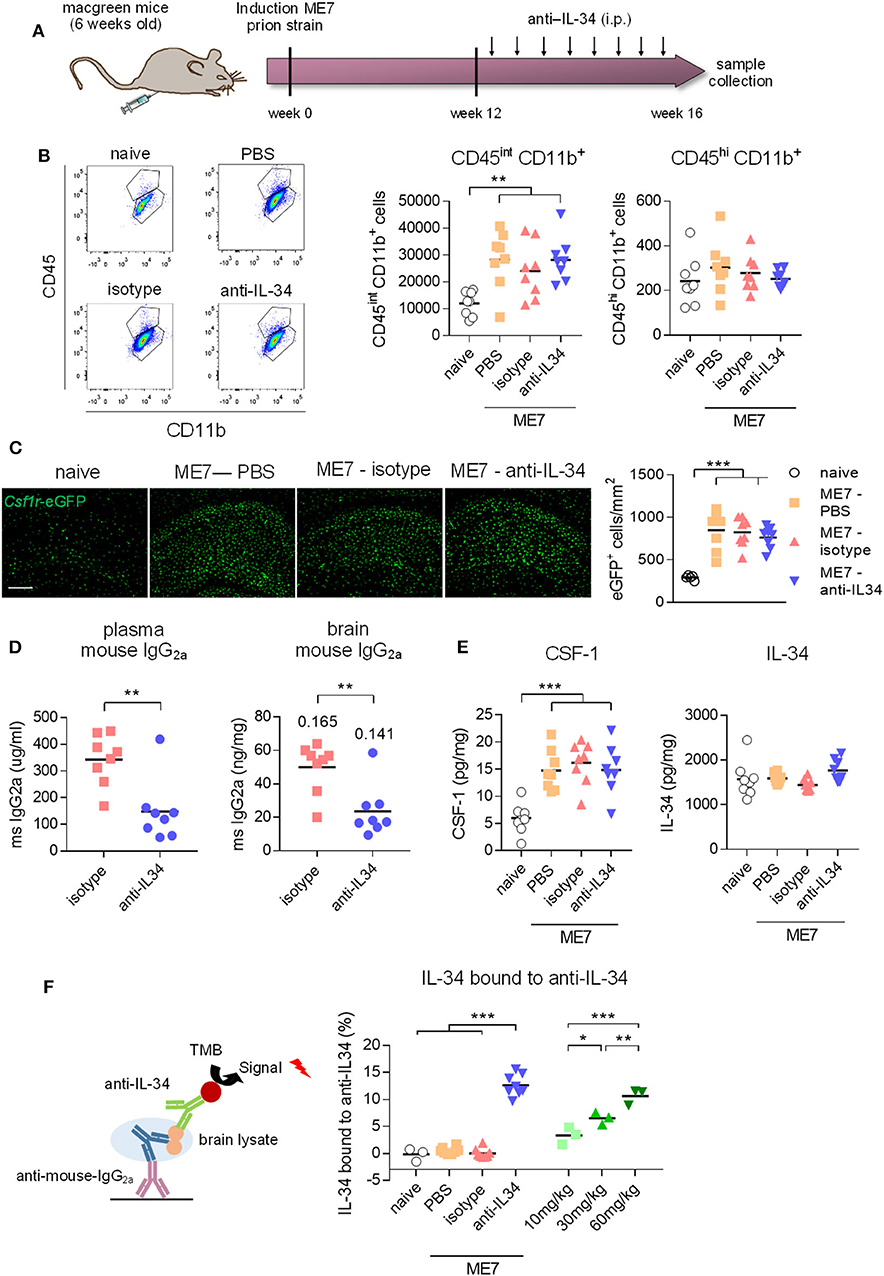
Figure 4. Peripheral IL-34 antibody injections in ME7 prion mice did not affect microglia numbers. (A) Macgreen mice infected with prion disease were treated with anti-IL-34 (mouse monoclonal IgG2A) by intraperitoneal injections at a dose of 60 mg/kg twice a week for 4 weeks. (B) Flow cytometric analysis of CSF1R-positive cells in the brain of anti-IL-34- treated mice showed no effect on number of CD45int CD11b+ or CD45hi CD11b+ cells. Graphs indicate respective cell numbers per 105 living cells. (C) Histological analysis of CSF1R-positive cells in the cortical brain shows unchanged microglial numbers after anti-IL-34 treatment. (D) Levels of mouse IgG2a were measured in blood plasma and brain lysates by ELISA, which showed higher levels of isotype than anti-IL-34. Values for tissue/plasma ratio are indicated above bars. (E) CSF-1 and IL-34 were measured in brain lysates, showing no alterations of IL-34 and CSF-1 levels after anti-IL-34 administration. (F) IL-34 bound to anti-IL34 in brain lysates were detected by ELISA, by coating plates with anti-mouse IgG2a to capture IL-34 antibodies present in brain lysates and detecting IL-34 bound to IL-34 antibodies with an IL-34 specific detection antibody (R&D systems). Levels of IL-34 bound to IL-34 antibodies were <15% from total IL-34 levels. Scale bar 100 μm. n = 8 per group, data shown represent mean ± SEM, two-way ANOVA followed by Tukey's multiple comparison test. *p < 0.05, **p < 0.01, ***p < 0.001.
Intracerebral Administration of IL-34 Blocking Antibodies Reduces Microglia Proliferation in ME7 Prion Mice
Since we did not achieve a significant degree of target engagement in the brain with peripheral antibody administration, we aimed to determine whether administering the antibody directly into the brain of prion mice could affect microglia numbers. IL-34 antibodies were stereotactically injected into the hippocampus 12 weeks after induction of prion disease, a timepoint of pronounced microglia proliferation (5), and brains were collected 1 week after injection (Figure 5A). Analysis of cells double-positive for the proliferation marker BrdU and Iba1, a marker of microglia/macrophages, showed increased microglial proliferation and increased total number of Iba1-positive cells in the hippocampus of prion mice compared to naïve mice, while injection of a mouse-specific IL-34 neutralizing antibody significantly reduced microglia proliferation by about 50% (Figure 5B). Administration of a human-specific IL-34 antibody did not have an effect on microglia proliferation, possibly due to reduced homology with mouse IL-34 [71% (16)]. Microglial proliferation was higher in ME7 compared to ME7 mice treated with isotype, probably due to the injection itself causing microgliosis associated to the local injury, which naïve and untreated ME7 mice did not receive. The reduction of microglial proliferation however did not result in a reduction of total microglia numbers after IL-34 antibody administration at the analyzed timepoint, probably due to the acute and transient nature of the intervention, which merely affected the small proliferating sub-population of the total microglial population (Figure 5B). The finding that microglia proliferation was locally reduced after direct intracerebral injection provides proof-of-concept that IL-34 is involved in regulating microglia proliferation in the context of chronic neurodegeneration and that IL-34 blockade could be used as a strategy to reduce microglia proliferation.
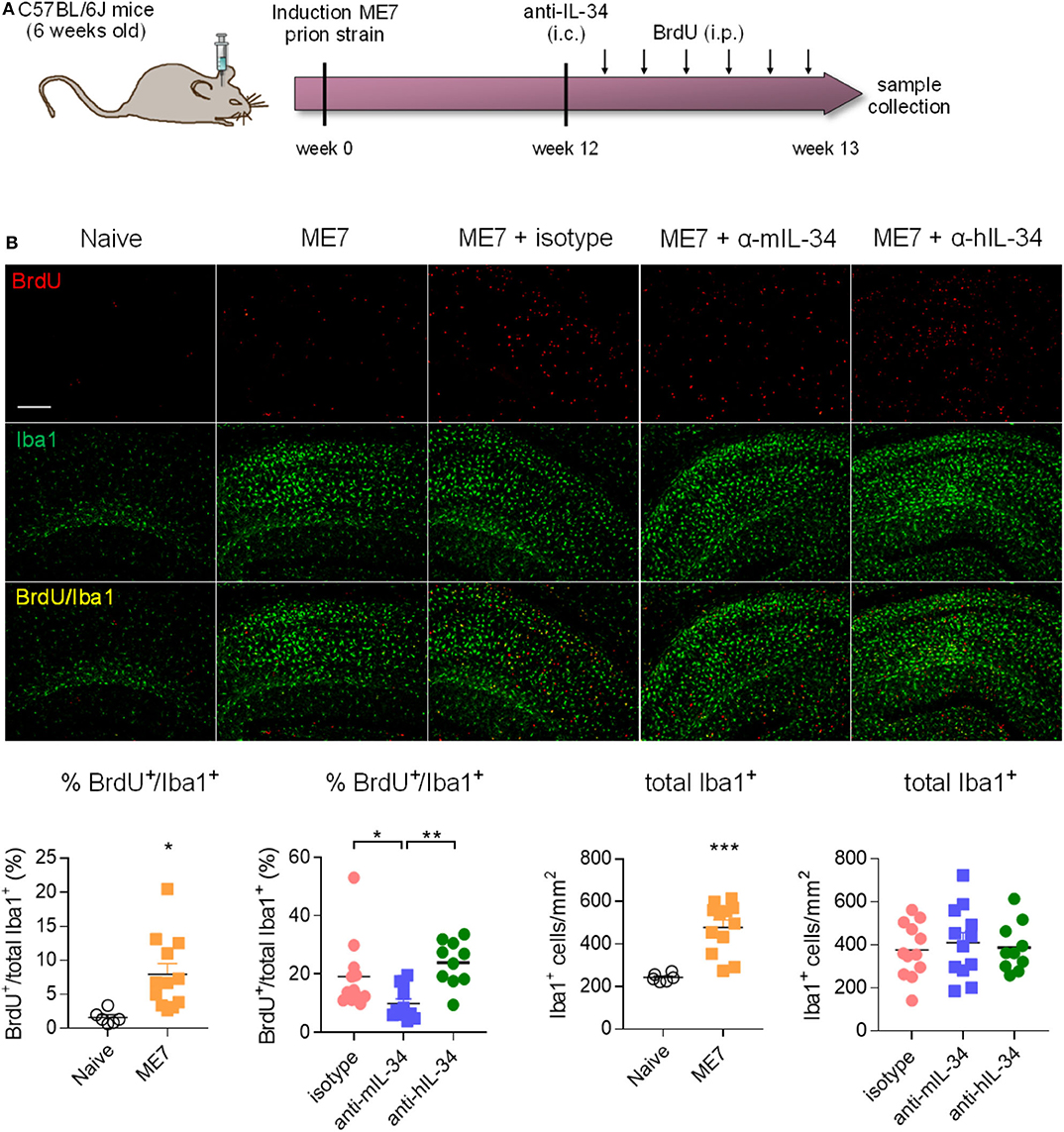
Figure 5. Intracerebral IL-34 antibody administration resulted in reduced microglia proliferation in ME7 prion mice. (A) Mice infected with prion disease received a single intracerebral injection of mouse- or human-specific anti-IL-34 (sheep polyclonal IgG) and brains were analyzed 1 week later. (B) Histological analysis of BrdU/Iba1-positive microglial cells in the cortex showed a reduction after treatment with a mouse-, but not with a human-specific antibody. Scale bar 100 μm. Naïve n = 6, ME7 n = 12, ME7 + isotype n = 10, ME7 + anti-mIL-34 n = 8, ME7 + anti-hIL-34 n = 7, data shown represent mean ± SEM, two-way ANOVA followed by Tukey's multiple comparison test. *p < 0.05, **p < 0.01, ***p < 0.001.
Discussion
In this study we explored the effect of inhibition of IL-34 on blood monocytes, systemic tissue macrophages, and microglia in health and neurodegenerative disease, modeled by a murine model of prion disease. IL-34 is a tissue-specific ligand of the CSF-1 receptor predominantly expressed in the brain and in the skin and has been shown to be crucial for the development and survival of microglia and epidermal Langerhans cells (19, 20). We showed here that inhibition of IL-34 does not affect monocytes and macrophage populations in many peripheral tissues, with an exception of skin-resident Langerhans cells. Even though we did not achieve sufficient target engagement in the brain after peripheral administration of IL-34 blocking antibodies, we observed a local reduction of microglia proliferation when injecting IL-34 antibodies directly into the brain of mice infected with prion disease, indicating that IL-34 is involved in driving proliferation of microglia in the context of neurodegenerative disease.
IL-34 and CSF-1 were shown to activate the CSF1R signaling cascade in a similar manner (29) and have an overall similar effect on human monocyte differentiation in vitro as shown by transcriptional profiling and pathway analysis (30). We have also found that the activation pattern of CSF1R and downstream pathway components AKT and ERK1/2 induced by IL-34 resembles the one induced by CSF-1 in microglia. Although IL-34 and CSF-1 seem to similarly affect CSF1R activation and macrophage differentiation, they have distinct tissue expression pattern with limited spatial overlap (29). A more recent report confirms the distinct spatial distribution of IL-34 and CSF-1 in the brain parenchyma, however describes differential transcriptional signatures in microglia exposed to CSF-1 and IL-34, suggesting divergent effects of the two CSF1R ligands on microglia phenotype that might account for the observed differences in regional microglial transcriptional profiles (31). In accordance with previous reports which showed that IL-34 is more widely expressed in the brain than CSF-1 (17, 19, 20), we observed that overall levels of IL-34 protein are ~300 times higher than CSF-1, highlighting its predominant role in the brain. However, we also observed that IL-34 levels did not further increase in the brain of prion-diseased mice.
It was demonstrated previously that CSF1R inhibition using tyrosine kinase inhibitors can be used as a strategy to decrease microglia proliferation in neurodegenerative disease models, which led to beneficial effects such as reduced neuronal loss and behavioral deficits in mouse models of prion disease (5), tau pathology (10), and Aβ pathology (7, 9). Long-term CSF1R inhibition could potentially increase risk of infections and lead to disturbance of tissue homeostasis due to the reduction of CSF1R-dependent macrophage populations in multiple organs. In a Listeria monocytogenes infection model, CSF-1/IL-34 blockade or treatment with anti-CSF-1 alone increased susceptibility to bacterial infection, although to a lower degree than anti-TNF therapy (32). Interestingly, IL-34 blockade did not alter infection susceptibility (32), indicating an immunosuppressive effect as observed with CSF-1 blockade can be prevented with anti-IL-34 treatment. CSF1R antagonism also lead to higher susceptibility of mice to lethal West Nile virus infection and lack of virologic control in both brain and periphery, highlighting the importance of myeloid cells in restriction of viral replication and the restimulation of antiviral T cells recruited to the CNS (33). On the other hand, a CSF1R tyrosine kinase inhibitor showed a good safety and tolerability profile in patients with rheumatoid arthritis over a course of 3 months, causing only minor side effects related to compromised Kupffer cell function (27), however the long-term consequences of CSF1R pharmacological inhibition are not well-understood. Other possible side-effects of long-term CSF1R blockade might include disturbances of bone formation and resorption as well as the function of pancreatic β cells (see Martin-Estebane and Gomez-Nicola (34) for further discussion).
A potential way to circumvent affecting multiple macrophage populations is to target IL-34, which is predominantly expressed in the brain. We aimed to evaluate whether inhibition of this more tissue-restricted ligand of CSF1R would cause a reduction in microglia proliferation without having a major impact on peripheral myeloid cell populations. In support of this strategy, the administration of recombinant IL-34 into the brain caused locally increased microglia proliferation similar to CSF-1 (5), showing that IL-34 can induce proliferation of microglia in the brain. In IL-34lacZ/lacZ mice which lack IL-34, microglial numbers are strongly reduced in many brain regions such as cortex, hippocampus and striatum (19, 20), indicating that IL-34 is at least partially responsible for maintenance of the population. Targeting IL-34 using an antibody-based approach, we showed that specific inhibition of IL-34 was sufficient to reduce microglia proliferation present in prion pathology, at least when IL-34 was inhibited over a short period of time by administration of IL-34 antibodies directly into the brain. This finding suggests that IL-34 is partially involved in regulating microglia proliferation in neurodegenerative disease. However, it proved to be challenging in our study to target brain-intrinsic IL-34 using systemically administered neutralizing antibodies probably due to their poor brain penetrance which prevented sufficient antibody titers to efficiently neutralize biological function of IL-34 in the brain. Although two recent reports showed a reduction in microglial numbers after peripheral administration of IL-34-specific monoclonal antibodies at high doses (32, 35), we did not observe a modulation of microglial numbers after chronic systemic antibody treatment in healthy mice or mice infected with prion disease. We found IL-34 to be ~300 times higher in the brain than CSF-1, while in the blood it was nearly absent. This high abundance of IL-34 in the brain emphasizes the difficulty in efficiently targeting this cytokine with neutralizing antibodies. Similarly, peripheral administration of CSF1R blocking antibodies did not lead to a reduction in microglia in cortex, dentate gyrus and CA1. The fact that blocking CSF1R using small molecule inhibitors resulted in pronounced depletion of microglia in several mouse models (5, 7, 9, 10), further indicates that the strategy applied in this study using blocking antibodies is not favorable. Lin et al. and Easley-Neal et al. provide first proof that manipulation of IL-34 can be used to modify the microglia population in the gray matter of most brain regions (35) and that this approach might be relevant in the context of inflammatory diseases and cancer (32). We extend these findings by showing that in a model of neurodegenerative disease, which is characterized by a pronounced expansion of the microglia population predominantly in the hippocampus, inhibition of IL-34 leads to reduced microglial proliferation. In order to provide further proof-of-concept that IL-34 inhibition can be used to tackle neurodegenerative disease, it is inevitable to apply other strategies which offer an improved brain penetrance profile, ideally by using small molecule inhibitors, which to date does not exist. By contrast, it was recently shown that prion disease induced in IL-34lacZ/lacZ mice did not change the number and activation of microglia and rather accelerated prion disease progression (36). It is possible that in the genetically modified mice with a constitutive loss of function of IL-34, CSF-1 compensates for the absence of IL-34 and drives proliferation through CSF1R to expand the microglial population in prion disease. It is also possible that during long term anti-IL34 treatment, CSF-1 will take over the function of IL-34 in maintaining and expanding the microglial population during neurodegeneration, given that it is also increased during disease. This possibility needs to be investigated in the future using more suitable pharmacological strategies than peripheral antibody administration.
Chronic inhibition of IL-34 did not majorly affect the abundance of blood monocytes or tissue-resident macrophage populations in liver and kidney, which were sensitive to CSF1R blockade, suggesting IL-34- independent mechanisms of survival, most likely through CSF-1. In line with this, a natural mutation in the Csf-1 gene (op/op) caused a reduction in macrophages in most tissues of the body (12), and long-term treatment with a CSF1R-blocking antibody lead to a complete depletion of Kupffer cells in the liver and prevented the development of non-classical Ly6Clo monocytes in the blood (37), which we likewise observed. It was previously shown, that genetic deficiency of IL-34 resulted in decreased numbers of microglia in most brain regions, while there was no effect on myeloid cells in blood and bone marrow, Kupffer cells in the liver, lung alveolar macrophages, and dendritic cells in the lung and spleen (20). Similarly, a specific impact of IL-34 blockade on Langerhans cell homeostasis, but not on liver, intestine and kidney macrophages after IL-34 antibody administration has been recently shown (32). We have also observed an effect on Langerhans cells, which were reduced after both CSF1R- and IL-34 antibody treatment, confirming a role of IL-34 in regulating their survival as well as the efficacy of anti-IL-34 antibodies. Overall, sensitivity of macrophage populations to IL-34 inhibition seems to be defined by the spatial expression pattern of IL-34, which rarely overlaps with CSF-1 expression. Thus, myeloid cells located in regions dominated by IL-34 expression can be targeted by inhibition of IL-34, which is restricted to fewer organs, potentially reducing unwanted side effects caused by therapeutic intervention targeting the CSF1R pathway. We propose that IL-34 inhibition could be a viable strategy to decrease proliferation of microglia in the context of neurodegenerative disease, with restricted impact on peripheral myeloid cells.
Data Availability Statement
Requests to access the datasets should be directed to Diego Gomez-Nicola, d.gomez-nicola@soton.ac.uk.
Ethics Statement
The animal study was reviewed and approved by University of Southampton Ethics Review Group.
Author Contributions
DG-N, VHP, DT, JB, HN, PA, EK, and CR conceived the study. DG-N supervised the project. JO prepared the figures and wrote the manuscript. ES, MM-E, EP, LB, IG-R, FB, AP, DF, and SF performed in vivo experiments and analyzed the data. All authors contributed to drafting the manuscript. All authors contributed to the article and approved the submitted version.
Funding
This research was funded by the Alzheimer's Research UK Dementia Consortium and Medical Research Council (MR/P024572/1).
Conflict of Interest
HN was employed by the company Eli Lilly and Company, PA was employed by the company Eisai Limited, EK was employed by the company Abbvie, and CR was employed by Alzheimer's Research UK.
The remaining authors declare that the research was conducted in the absence of any commercial or financial relationships that could be construed as a potential conflict of interest.
Acknowledgments
We thank Georgina Dawes for technical assistance. We thank the Southampton Flow Cytometry Facility for technical advice, and the Biomedical Research Facility for assistance with animal breeding and maintenance. This manuscript has been released as a pre-print at bioRxiv 2020.03.09.976118; (38).
Supplementary Material
The Supplementary Material for this article can be found online at: https://www.frontiersin.org/articles/10.3389/fimmu.2020.579000/full#supplementary-material
Supplementary Figure 1. Gating strategy used for flow cytometric analysis of brain, blood, and bone marrow after CSF1R- and IL-34 antibody treatment.
Supplementary Figure 2. Effect of CSF1R- and IL-34 antibody treatment on bone marrow. Macgreen mice were treated with anti-CSF1R or anti-IL-34 (both rat monoclonal IgG2A) by intraperitoneal injections of 250 μg antibody 3x a week for 3 weeks. (A) Flow cytometric analysis of CSF1R+ cells in the bone marrow of anti-CSF1R- and anti-IL-34- treated mice did not result in significant alterations. Graphs indicate respective cell numbers per 5 × 104 CD45+ cells. (B) Flow cytometry of eGFP+ Ly6Chi and eGFP+ Ly6Clo subpopulations of CSF1R-expressing cells in the bone marrow did not reveal any significant changes due to the treatment. Graphs indicate respective cell numbers per 5 × 104 CD45+ cells. PBS n = 8, isotype n = 8, anti-CSF1R n = 8, anti-IL-34 n = 7, data shown represent mean ± SEM, two-way ANOVA followed by Tukey's multiple comparison test. *p < 0.05, **p < 0.01, ***p < 0.001.
Supplementary Figure 3. Distribution of CSF1R- and IL-34 antibodies in peripheral organs and brain. Macgreen mice were treated with anti-CSF1R or anti-IL-34 (both rat monoclonal IgG2A) by intraperitoneal injections of 250 μg antibody 3x a week for 3 weeks. Levels of rat IgG2a were measured in tissue lysates of brain, liver, kidney and spleen after the treatment by ELISA, showing no significant differences between anti-CSF1R and anti-IL-34 in individual organs. Brain: PBS n = 8, isotype n = 8, anti-CSF1R n = 8, anti-IL-34 n = 7, liver/kidney/spleen: PBS n = 4, isotype n = 4, anti-CSF1R n = 3, anti-IL-34 n = 4, data shown represent mean ± SEM, two-way ANOVA followed by Tukey's multiple comparison test. *p < 0.05, **p < 0.01, ***p < 0.001.
References
1. Heneka MT, Golenbock DT, Latz E. Innate immunity in Alzheimer's disease. Nat Immunol. (2015) 16:229–36. doi: 10.1038/ni.3102
2. Hugh Perry V, O'Connor V. The role of microglia in synaptic stripping and synaptic degeneration: a revised perspective. ASN Neuro. (2010) 2:281–91. doi: 10.1042/AN20100024
3. Perry VH, Cunningham C, Boche D. Atypical inflammation in the central nervous system in prion disease. Curr Opin Neurol. (2002) 15:349–54. doi: 10.1097/00019052-200206000-00020
4. Obst J, Simon E, Mancuso R, Gomez-Nicola D. The role of microglia in prion diseases: a paradigm of functional diversity. Front Aging Neurosci. (2017) 9:207. doi: 10.3389/fnagi.2017.00207
5. Gomez-Nicola D, Fransen NL, Suzzi S, Perry VH. Regulation of microglial proliferation during chronic neurodegeneration. J Neurosci. (2013) 33:2481–93. doi: 10.1523/JNEUROSCI.4440-12.2013
6. Olmos-Alonso A. Pharmacological targeting of CSF1R inhibits microglial proliferation and prevents the progression of Alzheimer'slike pathology. Brain. (2015) 139:1–17. doi: 10.1093/brain/awv379
7. Dagher NN, Najafi AR, Neely Kayala KM, Elmore MRP, White TE, Medeiros R, et al. Colony-stimulating factor 1 receptor inhibition prevents microglial plaque association and improves cognition in 3xTg-AD mice. J Neuroinflamm. (2015) 12:1–14. doi: 10.1186/s12974-015-0366-9
8. Sosna J, Philipp S, Albay RI, Reyes-Ruiz JM, Baglietto-Vargas D, LaFerla FM, et al. Early long-term administration of the CSF1R inhibitor PLX3397 ablates microglia and reduces accumulation of intraneuronal amyloid, neuritic plaque deposition and pre-fibrillar oligomers in 5XFAD mouse model of Alzheimer's disease. Mol Neurodegener. (2018) 13:1–11. doi: 10.1186/s13024-018-0244-x
9. Spangenberg EE, Lee RJ, Najafi AR, Rice RA, Elmore MRP, Blurton-Jones M, et al. Eliminating microglia in Alzheimer's mice prevents neuronal loss without modulating amyloid-β pathology. Brain. (2016) 139:1265–81. doi: 10.1093/brain/aww016
10. Mancuso R, Fryatt G, Cleal M, Obst J, Pipi E, Monzón-Sandoval J, et al. CSF1R inhibitor JNJ-40346527 attenuates microglial proliferation and neurodegeneration in P301S mice. Brain. (2019) 142:3243–64. doi: 10.1093/brain/awz241
11. El-Gamal MI, Al-Ameen SK, Al-Koumi DM, Hamad MG, Jalal NA, Oh CH. Recent advances of colony-stimulating factor-1 receptor (CSF-1R) kinase and its inhibitors. J Med Chem. (2018) 61:5450–66. doi: 10.1021/acs.jmedchem.7b00873
12. Hume DA, MacDonald KP. Therapeutic applications of macrophage colony-stimulating factor-1 (CSF-1) and antagonists of CSF-1 receptor (CSF-1R) signaling. Blood. (2012) 119:1810–20. doi: 10.1182/blood-2011-09-379214
13. Masteller EL, Wong BR. Targeting IL-34 in chronic inflammation. Drug Discov Today. (2014) 19:1212–6. doi: 10.1016/j.drudis.2014.05.016
14. Hume DA, Caruso M, Ferrari-Cestari M, Summers KM, Pridans C, Irvine KM. Phenotypic impacts of CSF1R deficiencies in humans and model organisms. J Leukoc Biol. (2020) 107:205–19. doi: 10.1002/JLB.MR0519-143R
15. Richard E, Heard PM. Factors regulating macrophage production and growth. J Biol Chem. (1977) 252:4305–12.
16. Lin H, Lee E, Hestir K, Leo C, Huang M, Bosch E, et al. Discovery of a cytokine and its receptor by functional screening of the extracellular proteome. Science. (2008) 320:807–11. doi: 10.1126/science.1154370
17. Nandi S, Gokhan S, Dai XM, Wei S, Enikolopov G, Lin H, et al. The CSF-1 receptor ligands IL-34 and CSF-1 exhibit distinct developmental brain expression patterns and regulate neural progenitor cell maintenance and maturation. Dev Biol. (2012) 367:100–13. doi: 10.1016/j.ydbio.2012.03.026
18. Wang Y, Colonna M. Interleukin-34, a cytokine crucial for the differentiation and maintenance of tissue resident macrophages and Langerhans cells. Eur J Immunol. (2014) 44:1575–81. doi: 10.1002/eji.201344365
19. Greter M, Lelios I, Pelczar P, Hoeffel G, Price J, Leboeuf M, et al. Stroma-derived interleukin-34 controls the development and maintenance of langerhans cells and the maintenance of microglia. Immunity. (2012) 37:1050–60. doi: 10.1016/j.immuni.2012.11.001
20. Wang Y, Szretter KJ, Vermi W, Gilfillan S, Rossini C, Cella M, et al. IL-34 is a tissue-restricted ligand of CSF1R required for the development of Langerhans cells and microglia. Nat Immunol. (2012) 13:753–60. doi: 10.1038/ni.2360
21. Righi M, Sassano M, Valsasnini P, Shammah S, Ricciardi-Castagnoli P. Activation of the M-CSF gene in mouse macrophages immortalized by retroviruses carrying a v-myc oncogene. Oncogene. (1991) 6:103–11.
22. Sester DP, Trieu A, Brion K, Schroder K, Ravasi T, Robinson JA, et al. LPS regulates a set of genes in primary murine macrophages by antagonising CSF-1 action. Immunobiology. (2005) 210:97–107. doi: 10.1016/j.imbio.2005.05.004
23. Sweet MJ, Campbell CC, Sester DP, Xu D, McDonald RC, Stacey KJ, et al. Colony-stimulating factor-1 suppresses responses to CpG DNA and expression of toll-like receptor 9 but enhances responses to lipopolysaccharide in murine macrophages. J. Immunol. 168:392–9. doi: 10.4049/jimmunol.168.1.392
24. Ma D, Doi Y, Jin S, Li E, Sonobe Y, Takeuchi H, et al. TGF-β induced by interleukin-34-stimulated microglia regulates microglial proliferation and attenuates oligomeric amyloid β neurotoxicity. Neurosci Lett. (2012) 529:86–91. doi: 10.1016/j.neulet.2012.08.071
25. Ma X, Lin WY, Chen Y, Stawicki S, Mukhyala K, Wu Y, et al. Structural basis for the dual recognition of helical cytokines IL-34 and CSF-1 by CSF-1R. Structure. (2012) 20:676–87. doi: 10.1016/j.str.2012.02.010
26. Sasmono RT, Oceandy D, Pollard JW, Tong W, Pavli P, Wainwright BJ, et al. A macrophage colony-stimulating factor receptor – green fluorescent protein transgene is expressed throughout the mononuclear phagocyte system of the mouse. Blood. (2003) 101:1155–63. doi: 10.1182/blood-2002-02-0569
27. Genovese MC, Hsia E, Belkowski SM, Chien C, Masterson T, Thurmond RL, et al. Results from a phase IIA parallel group study of JNJ-40346527, an oral disease-modifying antirheumatic drug therapy results from a phase IIA parallel group study of JNJ-40346527, an oral CSF-1R inhibitor, in patients with active rheumatoid arthritis de. J Rheumatol. (2015) 42:1752–60. doi: 10.3899/jrheum.141580
28. Pridans C, Davis GM, Sauter KA, Lisowski ZM, Corripio-Miyar Y, Raper A, et al. A Csf1r -EGFP transgene provides a novel marker for monocyte subsets in sheep. J Immunol. (2016) 197:2297–305. doi: 10.4049/jimmunol.1502336
29. Wei S, Nandi S, Chitu V, Yeung Y-G, Yu W, Huang M, et al. Functional overlap but differential expression of CSF-1 and IL-34 in their CSF-1 receptor-mediated regulation of myeloid cells. J Leukoc Biol. (2010) 88:495–505. doi: 10.1189/jlb.1209822
30. Barve RA, Zack MD, Weiss D, Song RH, Beidler D, Head RD. Transcriptional profiling and pathway analysis of CSF-1 and IL-34 effects on human monocyte differentiation. Cytokine. (2013) 63:10–7. doi: 10.1016/j.cyto.2013.04.019
31. Kana V, Desland FA, Casanova-Acebes M, Ayata P, Badimon A, Nabel E, et al. CSF-1 controls cerebellar microglia and is required for motor function and social interaction. J Exp Med. (2019) 216:2265–81. doi: 10.1084/jem.20182037
32. Lin W, Xu D, Austin CD, Caplazi P, Senger K, Sun Y, et al. Function of CSF1 and IL34 in macrophage homeostasis, inflammation, and cancer. Front Immunol. (2019) 10:1. doi: 10.3389/fimmu.2019.00001
33. Funk KE, Klein RS. CSF1R antagonism limits local restimulation of antiviral CD8+ T cells during viral encephalitis. J Neuroinflam. (2019) 16:1–19. doi: 10.1186/s12974-019-1397-4
34. Martin-Estebane M, Gomez-Nicola D. Targeting microglial population dynamics in alzheimer's disease: are we ready for a potential impact on immune function? Front Cell Neurosci. (2020) 14:149. doi: 10.3389/fncel.2020.00149
35. Easley-Neal C, Foreman O, Sharma N, Zarrin AA, Weimer RM. CSF1R Ligands IL-34 and CSF1 are differentially required for microglia development and maintenance in white and gray matter brain regions. Front Immunol. (2019) 10:2199. doi: 10.3389/fimmu.2019.02199
36. Zhu C, Herrmann US, Falsig J, Abakumova I, Nuvolone M, Schwarz P, et al. A neuroprotective role for microglia in prion diseases. J Exp Med. (2016) 213:1047–59. doi: 10.1084/jem.20151000
37. MacDonald KP, Palmer JS, Cronau S, Seppanen E, Olver S, Raffelt NC. An antibody against the colony-stimulating factor 1 receptor depletes the resident subset of monocytes and tissue- and tumor-associated macrophages but does not inhibit inflammation. Blood. (2010) 116:3955–63. doi: 10.1182/blood-2010-02-266296
Keywords: CSF1R (colony-stimulating factor 1 receptor), prion disease, tissue-resident macrophage, chronic neurodegeneration, proliferation
Citation: Obst J, Simon E, Martin-Estebane M, Pipi E, Barkwill LM, Gonzalez-Rivera I, Buchanan F, Prescott AR, Faust D, Fox S, Brownlees J, Taylor D, Perry VH, Nuthall H, Atkinson PJ, Karran E, Routledge C and Gomez-Nicola D (2020) Inhibition of IL-34 Unveils Tissue-Selectivity and Is Sufficient to Reduce Microglial Proliferation in a Model of Chronic Neurodegeneration. Front. Immunol. 11:579000. doi: 10.3389/fimmu.2020.579000
Received: 01 July 2020; Accepted: 27 August 2020;
Published: 08 October 2020.
Reviewed by:
Charlotte Madore, INRAE Nouvelle-Aquitaine Bordeaux, FranceMelanie Greter, University of Zurich, Switzerland
Copyright © 2020 Obst, Simon, Martin-Estebane, Pipi, Barkwill, Gonzalez-Rivera, Buchanan, Prescott, Faust, Fox, Brownlees, Taylor, Perry, Nuthall, Atkinson, Karran, Routledge and Gomez-Nicola. This is an open-access article distributed under the terms of the Creative Commons Attribution License (CC BY). The use, distribution or reproduction in other forums is permitted, provided the original author(s) and the copyright owner(s) are credited and that the original publication in this journal is cited, in accordance with accepted academic practice. No use, distribution or reproduction is permitted which does not comply with these terms.
*Correspondence: Diego Gomez-Nicola, d.gomez-nicola@soton.ac.uk