- 1Bone Marrow Transplantation Center, The First Affiliated Hospital, School of Medicine, Zhejiang University, Hangzhou, China
- 2Institute of Hematology, Zhejiang University, Hangzhou, China
- 3Zhejiang Engineering Laboratory for Stem Cell and Immunotherapy, Hangzhou, China
- 4School of Medicine, Zhejiang University, Hangzhou, China
Histone deacetylase inhibitors are currently the most studied drugs because of their beneficial effects on inflammatory response. Emerging data from numerous basic studies and clinical trials have shown that histone deacetylase inhibitors can suppress immune-mediated diseases, such as graft-vs.-host disease (GVHD), while retaining beneficial graft-vs.-leukemia (GVL) effects. These drugs prevent and/or treat GVHD by modifying gene expression and inhibiting the production of proinflammatory cytokines, regulating the function of alloreactive T cells, and upregulating the function and number of regulatory T cells. Some of these drugs may become new immunotherapies for GVHD and other immune diseases.
Introduction
Allogeneic hematopoietic stem cell transplantation (allo-HSCT) is one of the most effective therapies for hematological malignancies. Although the overall effect of allo-HSCT has improved with the improvement in conditioning regimens, effective control of infection, HLA matching technology, and donor selection, the incidence of graft-vs.-host disease (GVHD) is still 30–60%, with a mortality rate of 30–50% (1). GVHD is the main cause of death after transplantation (2), which limits the success of allo-HSCT. Acute GVHD (aGVHD) has been reported to mainly involve the skin, liver, and gastrointestinal tract of patients within 100 days after transplantation. Chronic GVHD is usually diagnosed after day 100 and mainly manifests as autoimmune symptoms, including dry syndrome, scleroderma and obliterative bronchitis. The pathogenesis of GVHD has been confirmed to involve an alloreactive immune response mediated by the activation of donor T lymphocytes (3).
The combination of a calcineurin inhibitor (CNI; i.e., tacrolimus or cyclosporine) plus methotrexate (MTX) and/or mycophenolate mofetil (MMF) is a standard GVHD prophylaxis regimen used with posttransplantation cyclophosphamide (PTCy) in most haploidentical donor transplant (HIDT) protocols (4–6). Alternative immunosuppressive drug combinations may further help reduce the risk of treatment failure. The incorporation of proteasome inhibitors into GVHD-prevention regimens represents one such strategy, which has generated significant interest (7, 8). However, the incidence of aGVHD is still high and CNIs can also inhibit graft-vs.-leukemia (GVL) effect, thereby increasing relapse rate. In addition, currently, methylprednisolone is the first-line treatment for GVHD. However, the probability of complete remission of patients treated with methylprednisolone is <50% (9), and the long-term use of steroids may lead to steroid dependency and steroid-related adverse events, such as infection. Therefore, there is an urgent need to develop a new, safe, and effective strategy for prophylaxis and treatment of GVHD in the field of allo-HSCT.
Histone deacetylase (HDAC) inhibitors (HDACis) are currently used as anticancer drugs. Their effects in immune-mediated diseases have been studied. For instance, butyrate (pan-HDACi) has been reported to inhibit inflammatory response in a murine model of GVHD (10). This paper reviews advances in research on the application of HDACis for GVHD, and discusses their profound implications in immune cells involved in GVHD.
Overview of Histone Deacetylation Inhibitors
Histones, as structural proteins, are an important component of chromatin. According to the “histone code hypothesis,” specific residues of histone tails exposed to the chromatin surface can be covalently modified, such as through lysine acetylation, to form “histone codes” and then trigger downstream events (11). Histone acetylation level is a result of the interaction between histone acetyltransferases (HATs) and HDACs. These two groups can acetylate or deacetylate histones (mainly H3 and H4) or some specific lysine residues of certain proteins, thereby altering the chromatin structure and ultimately affecting gene expression.
HDACs can be divided into four categories, of which classical classes I, II, and IV have sequence similarity, and their enzyme activities are dependent on Zn+. Class I HDACs (HDAC1, 2, 3, and 8) are mainly located in the nucleus, class II HDACs (HDAC4, 5, 6, 7, 9, and 10) often shuttle between the nucleus and cytoplasm, and class IV HDAC (only HDAC11) is mainly located in the nucleus. Class III HDACs are Sir2-related enzymes (SIRT), which are deacetylases that depend on nicotinamide adenine dinucleotide, and this class has seven members, SIRT1–7, which are located in various organelles based on their functions (12, 13).
HDACis can inhibit the activity of specific histone deacetylases and upregulate the acetylation level of histones in specific cells as well as other specific non-histone molecules, thus regulating cell growth, differentiation, and immune response. HDACis have different effects on different cells. Their mechanisms include influencing DNA damage, DNA repair, and glycometabolism; altering gene expression; influencing cell growth; and inducing apoptosis, mitosis abnormalities, active oxygen redox, antiangiogenesis, antitumour metastasis, and autophagy of tumors (Figure 1). HDACis can be divided into six categories based on their chemical structure, including hydroxamic acids, short-chain fatty acids, cyclopeptides, electrophilic ketones, benzoamides, and other compounds. At present, four kinds of HDACis have been approved as anti-tumor drugs by the Food and Drug Administration (FDA). Among them, suberoylanilide hydroxamic acid (SAHA) and romidepsin can be used to treat T-cell lymphoma, panobinostat can be used to treat multiple melanomas, and belinostat to treat peripheral T-cell lymphoma (14). Notably, several HDACis have been used in clinical trials for prophylaxis or treatment of GVHD and show dramatic effects, such as a reduction in proinflammatory cytokine secretion and improvement of clinical symptoms (15) (Table 1).
Hydroxamic Acids
Hydroxamic acids chelate with metal atoms and bind reversibly with the zinc ions required for HDAC catalytic activity. This type of HDACi, including trichostatin A (TSA), vorinostat (SAHA), panobinostat (PANO), and belinostat, inhibits HDAC activities through competitive binding with the active site. TSA is a natural pan-HDACi that can inhibit class I and II HDACs. It has been used in diverse studies, including in vitro and in vivo experiments on various cancer strains and immune diseases. TSA reduces the expression of interleukin (IL)-12, interferon γ (IFN-γ), and IL-6 at both the mRNA and protein levels by promoting the acetylation of histones H3 and H4, and ultimately reduces renal disease in lupus mice (20). TSA has also been reported to regulate the expression of various costimulatory/adhesion molecules (such as CD28 and CD154) to alter T-cell function (21). However, the toxicity of TSA limits its clinical application (22). SAHA is a synthetic analog of TSA, but it has significantly lower toxicity. Therefore, SAHA is more widely used than TSA in experimental investigations and clinical applications. SAHA has been shown to prevent GVHD after bone marrow transplantation in mice in an indoleamine-2,3-dioxygenase-dependent manner (23). Another study has also shown that the prevention of GVHD by SAHA is related to the regulation of the inflammatory cytokine environment and the inhibition of signal transducer and activator of transcription 1 (STAT1) (24). The feasibility of SAHA (100 mg, twice a day) combined with tacrolimus and MTX for GVHD prophylaxis after allo-HSCT was evaluated in a prospective phase I/II clinical trial (NCT00810602). Fifty patients diagnosed with high-risk hematological malignant diseases were enrolled in this trial. All the patients had an available 8/8 or 7/8 HLA-matched related donor and underwent reduced-intensity conditioning. The cumulative incidence of grade II–IV acute GVHD by day 100 was 22% (95% confidence interval [CI] 13–36%). The most common non-hematological adverse events included electrolyte disturbances (n = 15), hyperglycemia (n = 11), infections (n = 6), mucositis (n = 4), and increased activity of liver enzymes (n = 3) (16).
In addition, in a single-center prospective phase II clinical trial, a novel regimen, consisting of SAHA and standard prophylatic drugs, was evaluated after unrelated-donors HSCT. The results showed that the addition of SAHA reduced the incidence of grade II–IV aGVHD on day 100 from 48 to 28% or lower, assuming a type I error of 5%, and enhanced the acetylation of histone H3 in peripheral blood mononuclear cells while reducing IL-6 secretion (median, 4.2 vs. 7.6 pg/mL; P = 0.028) (NCT01790568) (17).
PANO has been approved by the FDA as a third-line treatment for multiple myeloma (25). Bug et al.1 reported a phase I/II clinical trial of oral maintenance therapy using PANO for patients with myelodysplastic syndrome or acute myeloid leukemia who underwent allo-HSCT. Furthermore, in a phase I/II clinical study (NCT01111526), PANO was used in combination with glucocorticoids for the treatment of GVHD2. All participants in this study took PANO at a maximum tolerated dose (5 mg) three times a week for a month. At 36 days after study initiation, complete responses were observed in 12 patients (75%), partial responses were observed in 3 (19%), and progressive disease in 1 (6%). These trials demonstrated that PANO is safe to use after allo-HSCT; moreover, it can control GVHD and additionally function in targeting minimal residual lesions.
Short-Chain Fatty Acids (SCFAs)
SCFAs cannot bind to Zn2+ in the active center of HDAC; therefore, their inhibitory effect on HDAC is weaker than that of hydroxamic acids. SCFAs are the products of bacterial degradation of unabsorbed starch and non-starch polysaccharides (e.g., fibers). They are important anions in the colon's cavity and affect the morphology and function of colon epithelial cells (26). Some studies have shown that SCFAs can be absorbed by the intestinal epithelial cells (IECs), from where they enter the circulatory system through the liver, and ultimately affect cardiovascular function and inflammatory response (27, 28). Acetate, propionate, and butyrate are the main components of SCFAs. Among these, butyrate is the most important HDACi; it inhibits class I and II HDACs and has been shown to inhibit inflammatory responses in various inflammatory models. Cleophas et al. (29) found that butyrate can inhibit the expression of proinflammatory cytokines (IL-1β, IL-6, IL-8, and IFN-γ) in gouty arthritis and has strong anti-inflammatory effects. Furthermore, it has been found that fecal butyrate levels are decreased in patients after allo-HSCT. In a clinical study, stool samples from patients were obtained at baseline (before conditioning regimen), and on day 0 (day of allo-HSCT), day 7 post transplant, and day 14 post transplant. The results showed that the level of butyrate was significantly lower on day 14 than at the baseline, which was collected before allo-HSCT (P = 0.0039) (18). In 2016, to verify the effectiveness of butyrate in GVHD prophylaxis, a prospective phase II clinical trial (NCT02763033) was initiated to determine whether resistant starch can reduce the incidence of aGVHD3. In their ongoing study, the investigators speculated that the short-term administration of resistant starch increases intestinal butyric acid levels, thereby reducing the incidence of GVHD. In addition, in a previous study by Mathewson et al., (10) butyrate was effective in the treatment of GVHD in a mice model. The reduced butyrate in IECs after allo-HSCT resulted in decreased histone acetylation, whereas butyrate restoration improved the intestinal epithelium junction, re-established the intestinal flora structure, decreased IECs apoptosis, influenced IECs to present major histocompatibility complex (MHC) class II antigens.
Cyclic Peptides
Cyclic peptides are the most complex class of HDACis. They inhibit the enzyme activity of class I and II HDACs by interacting with Zn2+ at HDAC's active sites. Cyclic peptide HDACis can be classified as sulfur-containing and sulfur-free inhibitors. FR235222 is a sulfur-free cyclotetrapeptide inhibitor. It was first isolated from the fermentation broth of Cladosporium (Acremonium sp. No. 27082) by Mori et al. (30). They found that FR235222 has a strong immunosuppressive ability, which effectively inhibited the proliferation of T cells and delayed hypersensitivity in mice and adjuvant-induced arthritis in rats. AS1387392 is an analog of FR235222, but it has better pharmacokinetic characteristics and is an orally bioavailable HDACi. Therefore, AS1387392 can be used as a new and effective immunosuppressant (31). Romidepsin is a sulfur-containing peptide HDACi, with a unique ring structure. It has been approved by the FDA for the treatment of cutaneous T-cell lymphoma (32). In addition, recent studies have found that romidepsin can inhibit the activation of STAT1 and STAT3 by inducing suppressor of cytokine signaling 1 expression, and it can suppress the expression of proinflammatory cytokines (e.g., IL-1β) induced by sodium urate crystals (33). In an ongoing phase I clinical trial (NCT02512497), romidepsin was administered in combination with fludarabine and busulfan before and after allo-HSCT to verify whether it helps in controlling leukemia or lymphoma and evaluate the safety of this combination4.
Benzamides
Entinostat (MS-275) is a typical synthetic benzamide HDACi that selectively inhibits class I HDAC enzyme activity. Saito et al. (34) first discovered that MS-275 has a pronouned antitumour activity in mice. In addition to this activity, MS-275 has been used as an effective anti-inflammatory agent in recent studies and has been verified to be effective in some inflammatory models, such as experimental autoimmune encephalomyelitis and rheumatoid arthritis (35, 36). Lin et al. (35) found that MS-275 can effectively improve collagen-induced arthritis in animal models of rheumatoid arthritis; considerably reduce claw swelling, bone erosion, and absorption; and reduce serum IL-6 and IL-1β levels. Moreover, another study found that MS-275 can effectively inhibits inflammatory response in experimental autoimmune neuritis (EAN) in rats by inhibiting inflammatory T cells, macrophages, and proinflammatory cytokines, and inducing anti-inflammatory immune cells and molecules. This indicates that MS-275 may be an effective candidate drug for treating autoimmune neuropathy (36).
Chidamide is an orally absorbed benzamide pan-HDACi that was independently developed in China. It can stimulate the expression of Foxp3, a key transcription factor of regulatory T (Treg) cells, in patients with idiopathic thrombocytopenic purpura (ITP) and in ITP model mice. Moreover, it upregulates the expression of intracellular cytotoxic T-lymphocyte-associated protein 4 in Treg cells, induces Treg cell expansion, and restores immune tolerance (37). However, it is still worth exploring further whether benzamides HDACi can effectively prevent GVHD.
Sirtuin (SIRT) Inhibitors
Sirtuin inhibitors include nicotinamide, which inhibits all class III HDACs, and specific SIRT1/2 inhibitors, such as sirtinol, cambinol, and EX-527. Nicotinamide can inhibit proliferation and induce the apoptosis of chronic lymphoblastic leukemia cells by activating the p53/miR-34a/SIRT1 network (38). In a phase I/II clinical trial, it was confirmed that nicotinamide can be used to expand umbilical cord blood cells in vitro, and the median recovery times of neutrophils and platelets were shortened by 9.5 days (95% CI, 7–12 days) and 12 days (95% CI, 3–16.5 days), respectively, after umbilical cord blood transplantation, which significantly improved the safety of cord blood transplantation and reduced the incidence of GVHD (19). Anusara et al. (39) found that SIRT-1 knockout in mice enhanced p53 acetylation in T cells and promoted Treg stability. Furthermore, selective inhibition of SIRT1 by EX-527 significantly alleviated GVHD, improved survival of the mice, and preserved the GVL effect mediated by donor T cells.
Effects of HDACis on GVHD
The pathological mechanism of GVHD has now mostly been clarified and can be divided into three stages. In the first stage, tissue damage is caused by conditioning chemotherapy or infection, which activates “dangerous signal pathways” and leads to the secretion of proinflammatory cytokines (such as IL-1 and TNF-α). The second stage is the activation and amplification of effector T cells, in which antigen-presenting cells (APCs) and proinflammatory cytokines jointly activate donor T cells and cause their proliferation and the secretion of proinflammatory cytokines. The third stage is the immune effect stage, in which the activated donor T cells and abundant proinflammatory cytokines lead to tissue damage of the host, and this organ injury can further activate T cells. The following section summarizes the possible mechanism of action of HDACis in the treatment of GVHD in terms of occurrence and development stages of GVHD (Figure 2).
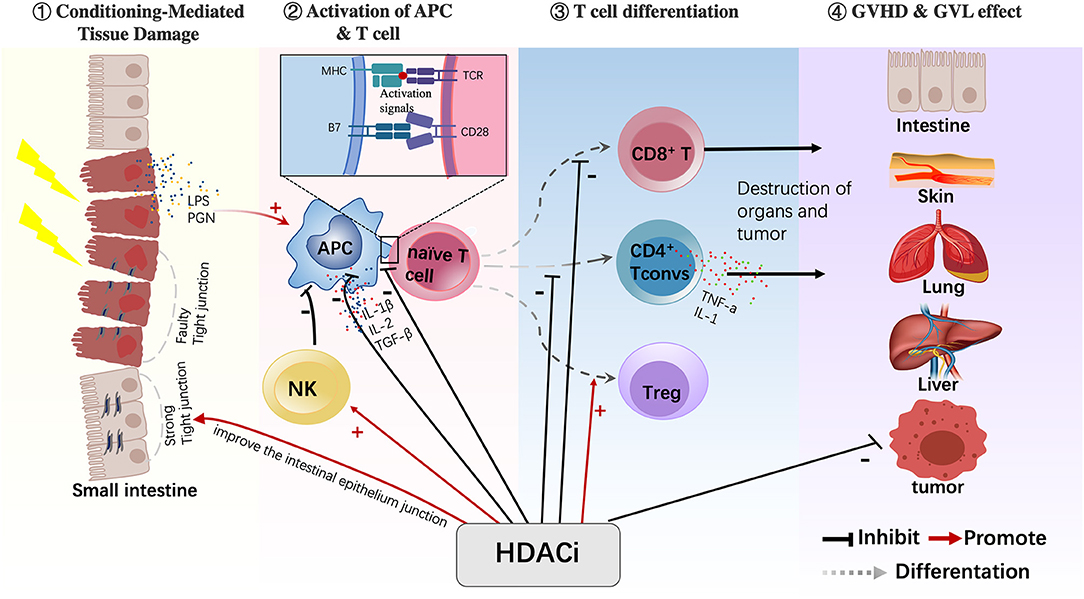
Figure 2. HDACis have various immunomodulatory effects on different cells. HDACis play an important role in regulating the maturation of APCs, reducing their antigen-presenting capacity and inhibiting the production of proinflammatory cytokines. HDACis also promote the conversion of naive T cells into Tregs and increase their function. In addition, HDACis activate NK cells and inhibit CD4+ Tconv cells and CD8+T cells. Moreover, HDACis can improve the intestinal epithelium junction during the GVHD process (HDACi, histone deacetylase inhibitor; APC, antigen-presenting cell; Treg, regulatory T cell; NK, natural killer cell; CD4+ Tconv, CD4+ conventional T cells).
HDACis Inhibit the Production of Inflammatory Mediators
Reducing the secretion of proinflammatory cytokines, thereby downregulating immune response, is an effective strategy for the prophylaxis and treatment of GVHD. ITF2357 has been reported to decrease the expression of various mRNAs induced by IL-1β, including those of cytokines (IL-6 and IL-8), chemokines (CXCL2, CXCL5, CXCL6, and CXCL10), matrix-degrading enzymes (MMP1 and ADAMTS1), and other inflammatory mediators, and promote the degradation of IL-6, IL-8, PTGS2, and CXCL2 mRNAs (40). SAHA, which has been approved by FDA for the treatment of T-cell lymphoma, has antitumor effects at micromolar concentrations, whereas a nanomolar SAHA concentration can reduce the secretion of inflammatory cytokines, such as IFN-γ, TNF-α, IL-1, and IL-12. SAHA can downregulate the mRNA levels of TNF-α and IFN-γ by enhancing the acetylation level of histone H3 and inhibit the secretion of inflammatory cytokines (41, 42). Moreover, butyrate can enhance the acetylation of TNF-α and IL-6 promoters and block the binding of RNA polymerase II with TNF-α and IL-6 gene promoters. In other words, transcription initiation is inhibited, and the expression of TNF-α and IL-6 is reduced (43).
HDACis Regulate the Function of APCs
Dendritic cells (DCs) are the most effective type of APCs; they play an important role in the pathological process of GVHD. On the one hand, DCs can activate donor T cells by presenting host antigens; on the other hand, they can secrete numerous pro-inflammatory cytokines to further aggravate tissue damage. Pretreatment with TSA reduces the antigen-presenting activity of lipopolysaccharide (LPS)-induced DCs in a dose-dependent manner. TSA plays a role in regulating the maturation of DC cells; thus, pretreatment of DCs with TSA before LPS stimulation reduces the expression of maturation markers to the same level as that of immature DCs. In addition, TSA reduces the production of IL-2 in mature DCs stimulated by LPS (44). Furthermore, TSA can reduce the levels of proinflammatory cytokines (IL-1β, IL-12, and TGF-β) secreted by DCs (45).
HDACis and Regulatory T Cells
Sakaguchi et al. (46) first discovered that a small group of CD4+ T cells, named Tregs, expresses high levels of CD25 and that the removal of Tregs leads to autoimmune diseases. Tregs play a key role in maintaining peripheral immune tolerance by preventing autoimmunity and chronic inflammation (46). Subsequently, Hori S et al. demonstrated that Foxp3 is specifically expressed in Tregs and is a key regulator of cell development and function (47). In recent years, preclinical studies have shown that adoptive retransfusion of Tregs can inhibit GVHD and prevent or delay allograft rejection (48).
The acetylation of lysine in Foxp3 is necessary to maintain Treg function. Foxp3 acetylation promotes its binding with the IL-2 promoter and subsequently inhibits endogenous IL-2 production. HDAC can inhibit FOXP3 gene transcription to some extent, whereas HDACis can enhance the homeostasis mediated by Treg proliferation. Therefore, HDACis are considered to be effective for increasing the number and inhibitory function of Tregs (49). Choi et al. (50) analyzed the immune response of patients receiving vorinostat for GVHD prevention after HSCT. Their results showed increases in the number of Tregs, methylation level of the Treg-specific demethylated region, and CD45RA and CD31 expression on the surface of Tregs, as well as enhanced inhibitory function.
Effect of HDACis on Natural Killer (NK) Cell Function
Donor NK cells can reduce the occurrence of GVHD by eliminating host APCs and secreting IL-10 in the early stage of transplantation, and they can directly eliminate recipient tumor cells. Delayed expansion of NK cells, especially immature NK cells, is associated with an increased aGVHD incidence and severity. Compared with patients without GVHD, patients with GVHD showed a significant decrease in the number of NK cells in peripheral blood (51). Entinostat enhanced NK cell function through epigenetic upregulation of the IFIT1-STING-STAT4 pathway. In that study, the researchers found that entinostat significantly increased the expression of NKG2D, an essential NK cell-activating receptor. Furthermore, the killing function of NK cells was also enhanced. In terms of its mechanism, entinostat increases the accessibility of the chromatin in the promoter region of interferon-induced protein with tetratripeptide repeats 1 (IFIT1), thus upregulating the mRNA and protein expression levels of IFIT1 and enhancing the IFIT1–STING–STAT4 pathways mediated by IFIT1 (52). However, further studies investigating whether HDACi also promotes the killing function of NK cells by regulating acetylation, thereby eliminating recipient APCs and inhibiting GVHD, are warranted.
Effect of HDACis on Helper T Cells
As mentioned above, donor-derived T cells are the key cell subsets in the development of GVHD, whereas GVL also requires allogeneic T cells. Th1, Th17, and Th2 subpopulations contribute to GVHD, but they mediate GVHD to different degrees of severity (Th1 and Th17 mediating more severe GVHD) and different distributions of GVHD in target tissues (conversion to Th1 or Th17 cells is related to intestinal GVHD, whereas conversion to Th2 cells is related to lung GVHD) (53). Long et al. (54) demonstrated that valproic acid (VPA) can reduce the incidence and lethality of GVHD after allo-HSCT in mice, which is related to the downregulation of Akt phosphorylation and thus, inhibition of Th1 and Th17. In addition, TSA can inhibit inflammation by increasing the number of Th2 cells and enhancing their ability to secrete IL-4 (55).
Discussion
This paper reviews the classification of HDACi and their direct or indirect effects on immune cells involved in GVHD. HDACi are an important class of anti-tumor drugs that have been used to treat a variety of tumors. Moreover, an increasing number of basic research and clinical trials have shown that HDACi have a strong anti-inflammatory effect and can negatively regulate GVHD while retaining beneficial GVL effects. Therefore, HDACi may become new immunotherapeutic options for prophylaxis and treatment of GVHD or other immune diseases.
Author Contributions
Under the supervision of HH and YZ, the manuscript was written by XX and XL. All authors contributed to the article and approved the submitted version.
Funding
This work was supported by the National Natural Science Foundation of China (81670148) and Key Project of Science and Technology Department of Zhejiang Province (2019C03016).
Conflict of Interest
The authors declare that the research was conducted in the absence of any commercial or financial relationships that could be construed as a potential conflict of interest.
Abbreviations
HDAC, histone deacetylase; GVHD, graft-vs.-host disease; Allo-HSCT, allogeneic hematopoietic stem cell transplantation; HDACi, HDAC inhibitors; HATs, histone acetyltransferases; SIRT, Sir2-related enzymes; NAD, niacinamide adenine dinucleotide; SAHA, suberoylanilide hydroxamic acid; PANO, panobinostat; TSA, trichostatin A; IFN-γ, interferon γ; SCFAs, short-chain fatty acids; IECs, intestinal epithelial cells; MHC, major histocompatibility complex; VPA, valproic acid; Treg, regulatory T; ITP, idiopathic thrombocytopenic purpura; APCs, antigen-presenting cells; NK, natural killer; CD4+ Tconvs, CD4+ conventional T cells; IFIT1, tetratripeptide repeats 1.
Footnotes
1. ^ClinicalTrials, n.d., ClinicalTrials.gov. NCT01451268.
2. ^ClinicalTrials, n.d., ClinicalTrials.gov. NCT01111526.
3. ^ClinicalTrials, n.d., ClinicalTrials.gov. NCT02763033.
4. ^ClinicalTrials, n.d., ClinicalTrials.gov. NCT02512497.
References
1. Holtan SG, Pasquini M, Weisdorf DJ. Acute graft-versus-host disease: a bench-to-bedside update. Blood. (2014) 124:363–73. doi: 10.1182/blood-2014-01-514786
2. Martin PJ, Weisdorf D, Przepiorka D, Hirschfeld S, Farrell A, Rizzo JD, et al. National Institutes of Health consensus development project on criteria for clinical trials in chronic graft-versus-host disease: VI. Design of clinical trials working group report. Biol. Blood Marrow Transplant. (2006) 12:491–505. doi: 10.1016/j.bbmt.2006.03.004
3. Zhang LL, Chu JH, Yu JH, Wei W. Cellular and molecular mechanisms in graft-versus-host disease. J. Leukoc. Biol. (2016) 99:279–87. doi: 10.1189/jlb.4RU0615-254RR
4. Kornblit B, Storer BE, Andersen NS, Maris MB, Chauncey TR, Petersdorf EW, et al. Sirolimus with CSP and MMF as GVHD prophylaxis for allogeneic transplantation with HLA antigen-mismatched donors. Blood. (2020) 136:1499–506. doi: 10.1182/blood.2020005338
5. Choi SW, Reddy P. Current and emerging strategies for the prevention of graft-versus-host disease. Nat. Rev. Clin. Oncol. (2014) 11:536–47. doi: 10.1038/nrclinonc.2014.102
6. Chhabra S, Liu Y, Hemmer MT, Costa L, Pidala JA, Courie DR, et al. Comparative analysis of calcineurin inhibitor-based methotrexate and mycophenolate mofetil-containing regimens for prevention of graft-versus-host disease after reduced-intensity conditioning allogeneic transplantation. Biol. Blood Marrow Transplant. (2019) 25:73–85. doi: 10.1016/j.bbmt.2018.08.018
7. Blanco B, Sanchez-Abarca LI, Caballero-Velazquez T, Santamaria C, Inoges S, Perez-Simon JA. Depletion of alloreactive T-cells in vitro using the proteasome inhibitor bortezomib preserves the immune response against pathogens. Leuk. Res. (2011) 35:1412–5. doi: 10.1016/j.leukres.2011.05.018
8. Moran E, Carbone F, Augusti V, Patrone F, Ballestrero A, Nencioni A. Proteasome inhibitors as immunosuppressants: biological rationale and clinical experience. Semin. Hematol. (2012) 49:270–6. doi: 10.1053/j.seminhematol.2012.04.004
9. Martin PJ, Rizzo JD, Wingard JR, Ballen K, Curtin PT, Cutler C, et al. First- and second-line systemic treatment of acute graft-versus-host disease: recommendations of the American Society of Blood and Marrow Transplantation. Biol. Blood Marrow Transplant. (2012) 18:1150–63. doi: 10.1016/j.bbmt.2012.04.005
10. Mathewson ND, Jenq R, Mathew AV, Koenigsknecht M, Hanash A, Toubai T, et al. Gut microbiome-derived metabolites modulate intestinal epithelial cell damage and mitigate graft-versus-host disease. Nat. Immunol. (2016) 17:505–13. doi: 10.1038/ni.3400
11. Strahl BD, Allis CD. The language of covalent histone modifications. Nature. (2000) 403:41–5. doi: 10.1038/47412
12. Seto E, Yoshida M. Erasers of histone acetylation: the histone deacetylase enzymes. Csh Perspect. Biol. (2014) 6:4. doi: 10.1101/cshperspect.a018713
13. Yoo CB, Jones PA. Epigenetic therapy of cancer: past, present and future. Nat. Rev. Drug Discov. (2006) 5:37–50. doi: 10.1038/nrd1930
14. Khan O, La Thangue NB. HDAC inhibitors in cancer biology: emerging mechanisms and clinical applications. Immunol. Cell Biol. (2012) 90:85–94. doi: 10.1038/icb.2011.100
15. Choi SW, DiPersio JF, Braun TM, Hou GQ, Stockerl-Goldstein K, Tawara I, et al. Targeting histone deacetylases as a new strategy for graft versus host disease prevention. Blood. (2012) 120:740. doi: 10.1182/blood.V120.21.740.740
16. Choi SW, Braun T, Chang L, Ferrara JLM, Pawarode A, Magenau JM, et al. Vorinostat plus tacrolimus and mycophenolate to prevent graft-versus-host disease after related-donor reduced-intensity conditioning allogeneic haemopoietic stem-cell transplantation: a phase 1/2 trial. Lancet Oncol. (2014) 15:87–95. doi: 10.1016/S1470-2045(13)70512-6
17. Choi SW, Braun T, Henig I, Gatza E, Magenau J, Parkin B, et al. Vorinostat plus acrolimus/methotrexate to prevent GVHD after myeloablative conditioning, unrelated donor HCT. Blood. (2017) 130:1760–7. doi: 10.1182/blood-2017-06-790469
18. Romick-Rosendale LE, Haslam DB, Lane A, Denson L, Lake K, Wilkey A, et al. Antibiotic exposure and reduced short chain fatty acid production after hematopoietic stem cell transplant. Biol. Blood Marrow Transplant. (2018) 24: 2418–24. doi: 10.1016/j.bbmt.2018.07.030
19. Horwitz ME, Wease S, Blackwell B, Valcarcel D, Sanz G, et al. Phase I/II study of stem-cell transplantation using a single cord blood unit expanded ex vivo with nicotinamide. J. Clin. Oncol. (2019) 37:367–74. doi: 10.1200/JCO.18.00053
20. Mishra N, Reilly CM, Brown DR, Ruiz P, Gilkeson GS. Histone deacetylase inhibitors modulate renal disease in the MRL-lpr/lpr mouse. J. Clin. Invest. (2003) 111:539–52. doi: 10.1172/JCI16153
21. Moreira JMA, Scheipers P, Sorensen P. The histone deacetylase inhibitor Trichostatin A modulates CD4+ T cell responses. BMC Cancer. (2003) 3:30. doi: 10.1186/1471-2407-3-30
22. Jasek E, Lis GJ, Jasinska M, Jurkowska H, Litwin JA. Effect of histone deacetylase inhibitors trichostatin A and valproic acid on etoposide-induced apoptosis in leukemia cells. Anticancer Res. (2012) 32:2791–9. doi: 10.1093/annonc/mds166
23. Reddy P, Sun YP, Toubai T, Duran-Struuck R, Clouthier SG, Weisiger E, et al. Histone deacetylase inhibition modulates indoleamine 2,3-dioxygenase-dependent DC functions and regulates experimental graft-versus-host disease in mice. J. Clin. Invest. (2008) 118:2562–73. doi: 10.1172/JCI34712
24. Leng C, Gries M, Ziegler J, Lokshin A, Mascagni P, Lentzsch S, et al. Reduction of graft-versus-host disease by histone deacetylase inhibitor suberonylanilide hydroxamic acid is associated with modulation of inflammatory cytokine milieu and involves inhibition of STAT1. Exp. Hematol. (2006) 34:776–87. doi: 10.1016/j.exphem.2006.02.014
25. US Food and Drug Administration. FDA approves Farydak for treatment of multiple myeloma [media release] (2015).
26. Mortensen PB, Clausen MR. Short-chain fatty acids in the human colon: relation to gastrointestinal health and disease. Scand. J. Gastroenterol. (1996) 31:132–48. doi: 10.3109/00365529609094568
27. Comalada M, Bailon E, de Haro O, Lara-Villoslada F, Xaus J, Zarzuelo A, et al. The effects of short-chain fatty acids on colon epithelial proliferation and survival depend on the cellular phenotype. J. Cancer Res. Clin. (2006) 132:487–97. doi: 10.1007/s00432-006-0092-x
28. Pluznick JL. A novel SCFA receptor, the microbiota, and blood pressure regulation. Gut Microbes. (2014) 5:202–7. doi: 10.4161/gmic.27492
29. Cleophas MCP, Crisan TO, Lemmers H, Toenhake-Dijkstra H, Fossati G, Jansen TL, et al. Suppression of monosodium urate crystal-induced cytokine production by butyrate is mediated by the inhibition of class I histone deacetylases. Ann. Rheumatol. Dis. (2016) 75:593–600. doi: 10.1136/annrheumdis-2014-206258
30. Mori H, Abe F, Furukawa S, Furukawa S, Sakai F, Hino M, et al. Fr235222, a fungal metabolite, is a novel immunosuppressant that inhibits mammalian histone deacetylase (hdac) ii. biological activities in animal models. Cheminform. (2003) 56:181. doi: 10.1002/chin.200329154
31. Sasamura S, Sakamoto K, Takagaki S, Yamada T, Takase S, Mori H, et al. AS1387392, a novel immunosuppressive cyclic tetrapeptide compound with inhibitory activity against mammalian histone deacetylase. J. Antibiot. (2010) 63:633–6. doi: 10.1038/ja.2010.51
32. VanderMolen KM, McCulloch W, Pearce CJ, Oberlies NH. Romidepsin (Istodax, NSC 630176, FR901228, FK228, depsipeptide): a natural product recently approved for cutaneous T-cell lymphoma. J. Antibiot. (2011) 64:525–31. doi: 10.1038/ja.2011.35
33. Cleophas MCP, Crisan TO, Kluck V, Hoogerbrugge N, Netea-Maier RT, Dinarello CA, et al. Romidepsin suppresses monosodium urate crystal-induced cytokine production through upregulation of suppressor of cytokine signaling 1 expression. Arthritis Res. Ther. (2019) 21:50. doi: 10.1186/s13075-019-1834-x
34. Saito A, Yamashita T, Mariko Y, Nosaka Y, Tsuchiya K, Ando T, et al. A synthetic inhibitor of histone deacetylase, MS-27-275, with marked in vivo antitumor activity against human tumors. Proc. Natl. Acad. Sci. U.S.A. (1999) 96: 4592–7. doi: 10.1073/pnas.96.8.4592
35. Lin HS, Hu CY, Chan HY, Liew YY, Huang HP, Lepescheux L, et al. Anti-rheumatic activities of histone deacetylase (HDAC) inhibitors in vivo in collagen-induced arthritis in rodents. Br. J. Pharmacol. (2007) 150:862–72. doi: 10.1038/sj.bjp.0707165
36. Zhang Z, Zhang Z, Schluesener HJ. MS-275, a histone deacetylase inhibitor, reduces the inflammatory reaction in rat experimental autoimmune neuritis. Neuroscience. (2010) 169:370–7. doi: 10.1016/j.neuroscience.2010.04.074
37. Zhao HY, Ma YH, Li DQ, Sun T, Li LZ, Li P, et al. Low-dose chidamide restores immune tolerance in ITP in mice and humans. Blood. (2019) 133:730–42. doi: 10.1182/blood-2018-05-847624
38. Audrito V, Vaisitti T, Rossi D, Gottardi D, D'Arena G, Laurenti L, et al. Nicotinamide blocks proliferation and induces apoptosis of chronic lymphocytic leukemia cells through activation of the p53/miR-34a/SIRT1 tumor suppressor network. Cancer Res. (2011) 71:4473–83. doi: 10.1158/0008-5472.CAN-10-4452
39. Daenthanasanmak A, Iamsawat S, Chakraborty P, Nguyen HD, Bastian D, Liu C, et al. Targeting Sirt-1 controls GVHD by inhibiting T-cell allo-response and promoting Treg stability in mice. Blood. (2019) 133:266–79. doi: 10.1182/blood-2018-07-863233
40. Angiolilli C, Kabala PA, Grabiec AM, Rossato M, Lai WS, Fossati G, et al. Control of cytokine mRNA degradation by the histone deacetylase inhibitor ITF2357 in rheumatoid arthritis fibroblast-like synoviocytes: beyond transcriptional regulation. Arthritis Res. Ther. (2018) 20:148. doi: 10.1186/s13075-018-1638-4
41. Leoni F, Zaliani A, Bertolini G, Porro G, Pagani P, Pozzi P, et al. The antitumor histone deacetylase inhibitor suberoylanilide hydroxamic acid exhibits antiinflammatory properties via suppression of cytokines. Proc. Natl. Acad. Sci. U.S.A. (2002) 99:2995–3000. doi: 10.1073/pnas.052702999
42. Reddy P, Maeda Y, Hotary K, Liu C, Reznikov LL, Dinarello CA, et al. Histone deacetylase inhibitor suberoylanilide hydroxamic acid reduces acute graft-versus-host disease and preserves graft-versus-leukemia effect. Proc. Natl. Acad. Sci. U.S.A. (2004) 101:3921–6. doi: 10.1073/pnas.0400380101
43. Zhang HY, Du M, Yang QY, Zhu MJ. Butyrate suppresses murine mast cell proliferation and cytokine production through inhibiting histone deacetylase. J. Nutr. Biochem. (2016) 27:299–306. doi: 10.1016/j.jnutbio.2015.09.020
44. Kim ES, Lee JK. Histone deacetylase inhibitors decrease the antigen presenting activity of murine bone marrow derived dendritic cells. Cell Immunol. (2010) 262:52–7. doi: 10.1016/j.cellimm.2009.12.007
45. Jiang HY, Zhang SW, Song TT, Guan X, Zhang RJ, Chen X. Trichostatin a protects dendritic cells against oxygen-glucose deprivation via the SRSF3/PKM2/glycolytic pathway. Front. Pharmacol. (2018) 9:612. doi: 10.3389/fphar.2018.00612
46. Sakaguchi S, Sakaguchi N, Asano M, Itoh M, Toda M. Immunologic self-tolerance maintained by activated T cells expressing IL-2 receptor alpha-chains (CD25) breakdown of a single mechanism of self-tolerance causes various autoimmune diseases. J. Immunol. (1995) 155:1151–64.
47. Hori S, Nomura T, Sakaguchi S. Control of regulatory T cell development by the transcription factor Foxp3. Science. (2003) 299:1057–61. doi: 10.1126/science.1079490
48. Trenado A, Sudres M, Tang Q, Maury S, Charlotte F, Gregoire S, et al. Ex vivo-expanded CD4+CD25+ immunoregulatory T cells prevent graft-versus-host-disease by inhibiting activation/differentiation of pathogenic T cells. J. Immunol. (2006) 176:1266–73. doi: 10.4049/jimmunol.176.2.1266
49. Tao R, de Zoeten EF, Ozkaynak E, Chen CX, Wang LQ, Porrett PM, et al. Deacetylase inhibition promotes the generation and function of regulatory T cells. Nat. Med. (2007) 13:1299–307. doi: 10.1038/nm1652
50. Choi SW, Gatza E, Hou GQ, Sun YP, Whitfield J, Song YH, et al. Histone deacetylase inhibition regulates inflammation and enhances Tregs after allogeneic hematopoietic cell transplantation in humans. Blood. (2015) 125:815–9. doi: 10.1182/blood-2014-10-605238
51. Chan YLT, Zuo JM, Inman C, Croft W, Begum J, Croudace J, et al. NK cells produce high levels of IL-10 early after allogeneic stem cell transplantation and suppress development of acute GVHD. Eur. J. Immunol. (2018) 48:316–29. doi: 10.1002/eji.201747134
52. Idso JM, Lao S, Schloemer NJ, Knipstein J, Burns R, Thakar MS, et al. Entinostat augments NK cell functions via epigenetic upregulation of IFIT1-STING-STAT4 pathway. Oncotarget. (2020) 11:1799–815. doi: 10.18632/oncotarget.27546
53. Yi T, Chen Y, Wang L, Du G, Huang D, Zhao D, et al. Reciprocal differentiation and tissue-specific pathogenesis of Th1, Th2, and Th17 cells in graft-versus-host disease. Blood. (2009) 114:3101–12. doi: 10.1182/blood-2009-05-219402
54. Long J, Chang L, Shen Y, Gao WH, Wu YN, Dou HB, et al. Valproic acid ameliorates graft-versus-host disease by downregulating Th1 and Th17 cells. J. Immunol. (2015) 195:1849–57. doi: 10.4049/jimmunol.1500578
Keywords: allo-reactive T cells, allogeneic hematopoietic stem cell transplantation, epigenetic regulation, graft-vs.-host disease, histone deacetylase inhibitors
Citation: Xu X, Li X, Zhao Y and Huang H (2021) Immunomodulatory Effects of Histone Deacetylation Inhibitors in Graft-vs.-Host Disease After Allogeneic Stem Cell Transplantation. Front. Immunol. 12:641910. doi: 10.3389/fimmu.2021.641910
Received: 16 December 2020; Accepted: 08 February 2021;
Published: 24 February 2021.
Edited by:
Michaela Lucas, University of Western Australia, AustraliaReviewed by:
Benny Chen, Duke University, United StatesHonglin Wang, Shanghai Jiao Tong University, China
Copyright © 2021 Xu, Li, Zhao and Huang. This is an open-access article distributed under the terms of the Creative Commons Attribution License (CC BY). The use, distribution or reproduction in other forums is permitted, provided the original author(s) and the copyright owner(s) are credited and that the original publication in this journal is cited, in accordance with accepted academic practice. No use, distribution or reproduction is permitted which does not comply with these terms.
*Correspondence: Yanmin Zhao, emp6aGFveWFubWluQDE2My5jb20=; He Huang, aHVhbmdoZUB6anUuZWR1LmNu