- 1Department of Neurosurgery, Graduate School of Medical Sciences, Kyushu University, Fukuoka, Japan
- 2Department of Neuropsychiatry, Graduate School of Medical Sciences, Kyushu University, Fukuoka, Japan
- 3Department of Neuropathology, Graduate School of Medical Sciences, Kyushu University, Fukuoka, Japan
- 4Department of Psychosomatic Medicine, Kyushu University Hospital, Fukuoka, Japan
Targeting the unique glioma immune microenvironment is a promising approach in developing breakthrough immunotherapy treatments. However, recent advances in immunotherapy, including the development of immune checkpoint inhibitors, have not improved the outcomes of patients with glioma. A way of monitoring biological activity of immune cells in neural tissues affected by glioma should be developed to address this lack of sensitivity to immunotherapy. Thus, in this study, we sought to examine the feasibility of non-invasive monitoring of glioma-associated microglia/macrophages (GAM) by utilizing our previously developed induced microglia-like (iMG) cells. Primary microglia (pMG) were isolated from surgically obtained brain tissues of 22 patients with neurological diseases. iMG cells were produced from monocytes extracted from the patients’ peripheral blood. Quantitative reverse transcription-polymerase chain reaction (qRT-PCR) revealed a significant correlation of the expression levels of representative markers for M1 and M2 microglia phenotypes between pMG and the corresponding iMG cells in each patient (Spearman’s correlation coefficient = 0.5225, P <0.0001). Synchronous upregulation of CD206 expression levels was observed in most patients with glioma (6/9, 66.7%) and almost all patients with glioblastoma (4/5, 80%). Therefore, iMG cells can be used as a minimally invasive tool for monitoring the disease-related immunological state of GAM in various brain diseases, including glioma. CD206 upregulation detected in iMG cells can be used as a surrogate biomarker of glioma.
Introduction
Gliomas are tumors of the central nervous system (CNS) derived from neural tissues. Among them, glioblastoma (GBM) has a highly aggressive phenotype and accounts for most gliomas. Despite advances in surgical resection, chemotherapy, and radiation therapy, GBM prognosis remains poor, and <5% of patients survive beyond five years post-diagnosis (1).
Among the recent attempts to develop multimodal treatment strategies to modify the extremely poor survival of GBM patients, immune checkpoint inhibitors were expected to bring about a paradigm shift, similar to that achieved in the treatment of other malignancies, such as melanoma (2, 3). However, clinical trials have failed to observe significant therapeutic benefits of immune checkpoint inhibitors in patients with GBM (4, 5). Such unfavorable results may be partly due to the peculiar state of the immune system in glioma tissues and the CNS in general.
Microglia are the brain’s immune system cells responsible for maintaining brain homeostasis (6). Microglia exhibit a spectrum of phenotypes. The classically activated microglia/macrophages stimulate anti-tumor immune responses through the secretion of pro-inflammatory cytokines, such as tumor necrosis factor-alpha (TNF-α), interleukin (IL)-1β, and inducible nitric oxide synthase, defined as M1 markers. However, the alternatively activated microglia/macrophages promote tumor survival by producing anti-inflammatory cytokines such as IL-4, transforming growth factor-beta (TGF-β), and IL-10, defined as M2 markers (7–10). Microglia and peripheral macrophages recruited by glioma cells, defined as glioma-associated microglia/macrophages (GAM), were shown to contribute to tumor growth and invasion (11).
GBM is a complex solid tumor containing neoplastic and non-neoplastic cells, and the majority of the non-neoplastic cells are GAM, which account for 30%-50% of the cells in GBM (12, 13). GAM have been reported to play various roles in the malignancy features of GBM, including proliferation, growth, invasion, and immunosuppression (14–17). GAM are recruited to GBM microenvironment, where they release a wide array of chemokines and cytokines in response to the factors secreted by the tumor cells (14). Recently, small extracellular vesicles secreted by GAM have been reported to promote the progression of glioma (18). Within the tumor microenvironment, GAM are forced to transform to M2 phenotypes by GBM cells that secrete factors such as IL-10, IL-4, IL-6, macrophage colony-stimulating factor, macrophage inhibitory factor, TGFβ, and prostaglandin E2, which subsequently supports tumor growth and invasion (19). Several studies of tumor tissue samples have documented a correlation between GAM characteristics and pathological grade/prognosis of gliomas (20, 21).
We have previously developed a technique to generate induced microglia-like (iMG) cells from peripheral blood (22). We reported that iMG cells expressed the essential characteristics of human microglia, such as surface markers and drug responses, phagocytosis, and cytokine production (22). We applied this technique for translational research focusing on neurological, psychiatric, and pain-related disorders (23, 24). Other research groups and ours have revealed that iMG cells express microglia-specific surface markers (CX3CR1, P2RY12, TMEM119, and others) and possess phagocytic activity (22, 25, 26). Recently, iMG cells have been reported to be distinct from monocytes and macrophages but clustered closer with human brain microglia [Ohgidani 2020 under review] (25). These results suggest that iMG cells are a promising research tool for less-invasive monitoring of the immunological state of microglia in the CNS.
CD206 is a 175 kDa transmembrane protein encoded by the mannose receptor C-type 1 gene (MRC1). It is mostly expressed in macrophages, dendritic cells, and endothelial cells, where it functions as a receptor for mannosylated ligands, such as microbial antigens (27). In neural tissues, expression of CD206 is observed in microglia (28, 29) and astrocytes (30, 31). CD206 is widely recognized as a representative M2 microglial marker (29, 32). CD206 is involved in important cellular functions, especially in pinocytosis and phagocytosis (28, 31, 33). Therefore, CD206 is suggested to play a critical role in the first step of the recognition and capture of pathogens in neural tissues (31). A recent study has suggested a positive correlation between the World Health Organization pathological grades and the numbers of CD206-positive GAM in human glioma tumor tissues (21). Interestingly, we reported that CD206 expression in iMG cells was downregulated in patients with bipolar disorder during the manic state (34). Based on that study, we proposed that iMG cells could be candidate surrogate cells to monitor the immune environment that comprises other CD206-expressing cells in CNS diseases.
We hypothesized further that iMG cells from patients with glioma might reflect the immune properties of GAM and thereby serve as a novel biomarker of glioma. To clarify this hypothesis, we compared the immunological states of blood-derived iMG cells with those of brain-derived microglia. Further, we investigated the specificity of biological properties of these cells, which were isolated from glioma patients.
Materials and Methods
Patients
Microglia were isolated from the residual resected brain tissue after sampling for the pathological diagnosis from the surgical removal of subcortical or deeply located mass lesions (N = 15) or following epileptic surgery (N = 7) in a total of 22 patients (Table 1). During surgeries for the removal of nine gliomas (grade II, N = 2; grade III, N = 2; grade IV, N = 5) and six radiographic glioma-like mass lesions (meningioma, N = 1; metastatic tumor, N = 2; brain abscess, N = 1; encephalitis, N = 1; radiation necrosis, N = 1), the samples for microglia isolation were obtained from the surgical corridor during the approach to the lesion. In five of the seven patients with epilepsy, sampling for microglia isolation was performed at the resected epileptogenic lesion detected using chronic subdural electrodes placed during a prior surgery 1 or 2 weeks before. Resected brain tissues were immediately placed on ice and transferred to the laboratory for microglia isolation within 2 h of resection.
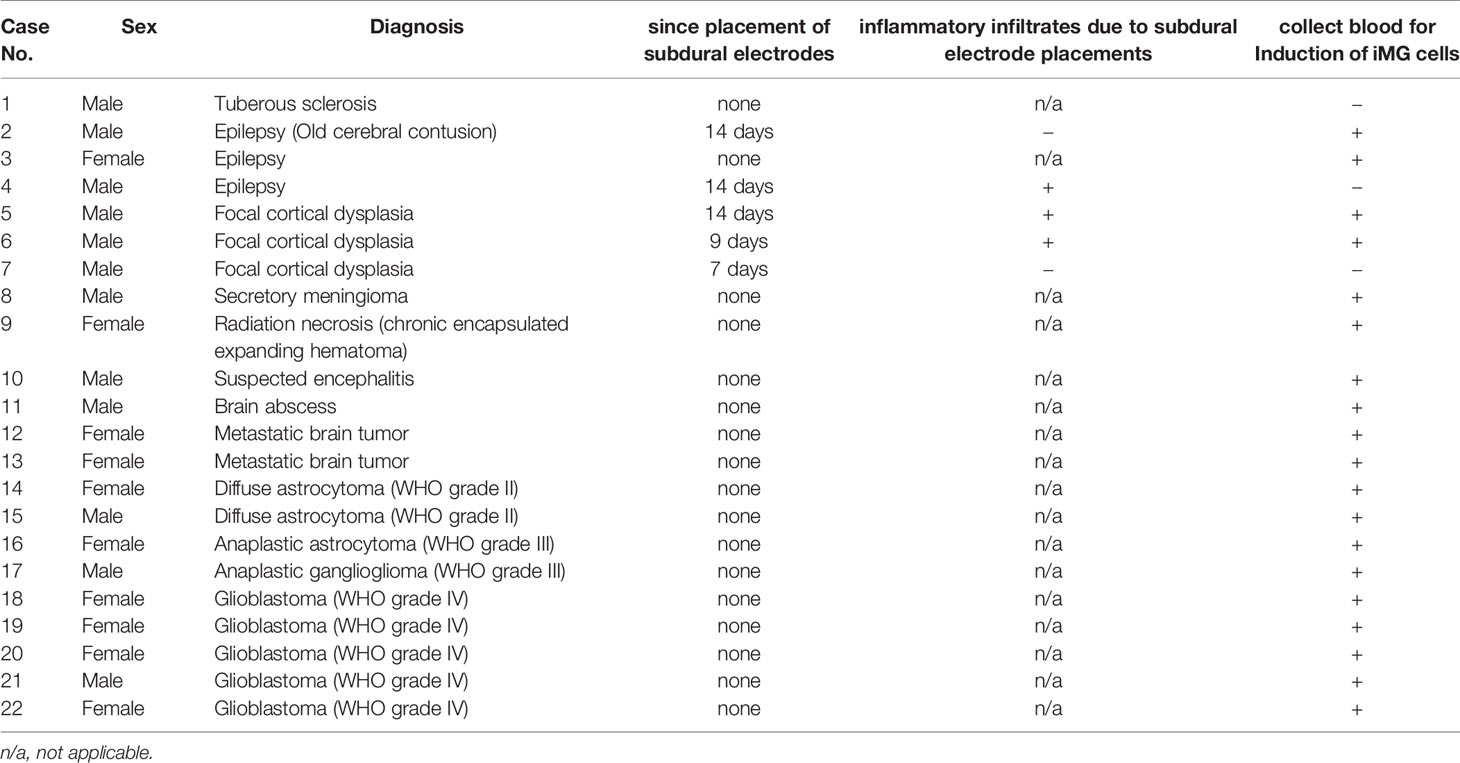
Table 1 Summary of the pathological characteristics of samples and period from subdural electrode placements.
Primary Microglia Isolation
Human tissue samples were dissociated using a Neural Tissue Dissociation Kit (Miltenyi Biotec, Bergisch-Gladbach, Germany), according to the manufacturer’s instructions (Figure 1). The cell suspension was incubated with CD11b microbeads (Miltenyi Biotec) in the MACS buffer (Miltenyi Biotec) for 15 min at 4°C. Afterward, the cells were washed, resuspended, and transferred to an LS column (Miltenyi Biotec) within a magnetic field. The positively selected (CD11b+) microglia were collected and resuspended in the Microglia Medium (ScienCell, Carlsbad, CA, USA). Primary microglia (pMG) were plated on culture dishes at a density of 3 × 105 cells/mL and cultured overnight in standard culture conditions (37 °C, 5% CO2). After overnight incubation, culture supernatant and non-adherent cells were removed. Microglia were cultured in RPMI-1640 Glutamax (Invitrogen, Carlsbad, CA, USA) supplemented with 1% antibiotic/antimycotic, recombinant human granulocyte macrophage colony-stimulating factor (GM-CSF) (10 ng/mL; R&D Systems, Minneapolis, MN, USA) and recombinant human IL-34 (100 ng/mL; R&D Systems) for 5 days.
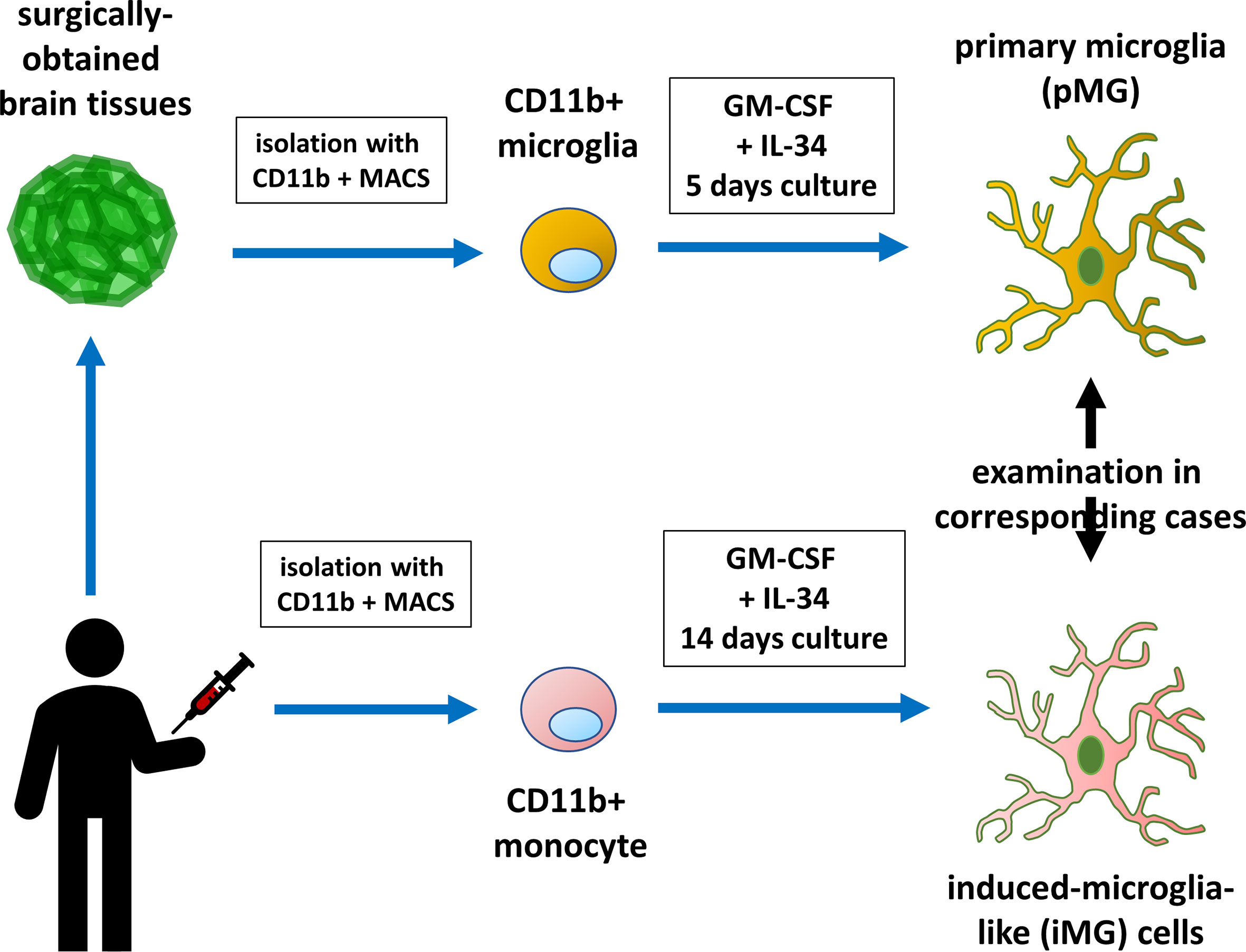
Figure 1 Diagram illustrating primary microglia and induced microglia-like cell collection and experiment. Monocytes isolated from the patients were induced to develop into microglia-like (iMG) cells. The biological properties of iMG cells were compared with those of brain-derived primary microglia.
Preparation of iMG Cells From Human Peripheral Blood
Peripheral blood was collected into a heparinized tube from patients with brain tumors, epilepsy, or other brain diseases (Figure 1). In three patients with epilepsy, consent to collect blood was not obtained. Peripheral blood mononuclear cells (PBMCs) were isolated using Histopaque-1077 (Sigma Chemical Co., St. Louis, MO, USA) density gradient centrifugation. PBMCs were resuspended in RPMI-1640 (Nacalai Tesque, Kyoto, Japan) supplemented with 10% heat-inactivated fetal bovine serum (Japan Bio Serum, Hiroshima, Japan) and 1% antibiotic/antimycotic (Invitrogen). PBMCs were plated on culture dishes at a density of 4 × 105 cells/mL and cultured overnight in standard culture conditions (37 °C, 5% CO2). After overnight incubation, culture supernatant and non-adherent cells were removed. Adherent cells (monocytes) were cultured in RPMI-1640 Glutamax supplemented with 1% antibiotic/antimycotic, recombinant human GM-CSF (10 ng/mL), and recombinant human IL-34 (100 ng/mL) for 14 days to obtain iMG cells (22, 23).
Quantitative Reverse Transcription-Polymerase Chain Reaction
To assess gene expression patterns in microglia and iMG cells, qRT-PCR was performed using a LightCycler 480 system (Roche Diagnostics, Mannheim, Germany). Microglia and iMG cells were washed. Total RNA was extracted using a High Pure RNA Isolation kit (Roche Diagnostics) according to the manufacturer’s protocol and used for cDNA synthesis using a Transcriptor First Strand cDNA Synthesis kit (Roche Diagnostics). qRT-PCR for representative markers of M1 and M2 microglia phenotypes was performed using their respective primers (Table 2). Normalization was performed using the reference gene glyceraldehyde-3-phosphate dehydrogenase (GAPDH) from the Universal ProbeLibrary (Roche Diagnostics) and the ΔΔCt method.
Immunohistochemistry
The resected brain tissues around the tumors were fixed in 10% neutral buffered formalin, embedded in paraffin, and processed for immunohistochemistry. The expression levels of the following microglia/macrophage markers were investigated in 4 μm serial paraffin sections: Iba-1 (pan-microglia/macrophage marker), CD68 (lysosomal protein; highly expressed by macrophages and activated microglia), and CD206 (mannose receptor, M2 marker). Goat antibody for Iba-1 (Cat. #ab5076, Abcam, Cambridge, MA, USA; 1:500 dilution, RRID: AB_2224402), rabbit antibody for CD206 (Cat. #ab64693, Abcam, Cambridge, MA, USA; 1:1000 dilution, RRID: AB_1523910), and mouse antibody for CD68 (Cat. #M0814, Dako, Carpinteria, CA, USA; 1:200 dilution, RRID: AB_2314148) were used as the primary antibodies. The sections were incubated with primary antibodies at 4°C overnight. Immunoreaction products were detected using the polymer immunocomplex method by an Envision system (Dako). The sections were counterstained with hematoxylin. Immunoreactivity was detected using 3,3′-diaminobenzidine (Dojindo, Kumamoto, Japan). The negative control experiments for CD 206, Iba-1, and CD68 were performed without primary antibodies (Supplementary Figure S1).
Statistical Analyses
Results are expressed as the mean ± standard deviation (SD). The Spearman’s correlation coefficient was used for analyzing the correlation between parameters in pMG and iMG cells. Statistical significance was determined at α = 0.05 level. Differences were considered statistically significant when P-values were <0.05. Each experiment was conducted with four independent cell cultures; however, in some cases, the number of pMG or iMG cells was small and the number of samples was <4 [N = 3.68 (SD ±0.7790)].
Results
Gene Expression in Human GAM and iMG Cells
Total RNA was isolated from these paired samples to investigate the expression profiles of pMG cells isolated from brain tissue and the corresponding blood-derived iMG cells. PCR was performed to determine the expression levels of inflammation-related genes known as representative markers for M1 microglia and macrophage phenotype (CD45, CD80, HLA-DR, TNF-α, IL-1β, and IL-23) and M2 phenotype (CD206, CD209, CD23, BDNF, IL-10, and CCL18) (Supplementary Table S1). Spearman’s correlation analysis revealed that expression levels of inflammation-related genes in the paired pMG and iMG cells derived from the same patient significantly correlated (Spearman’s correlation coefficient = 0.5225, P < 0.0001), indicating that iMG cells exhibited disease-related phenotypes and were regulated synchronously with the corresponding pMG (Figure 2).
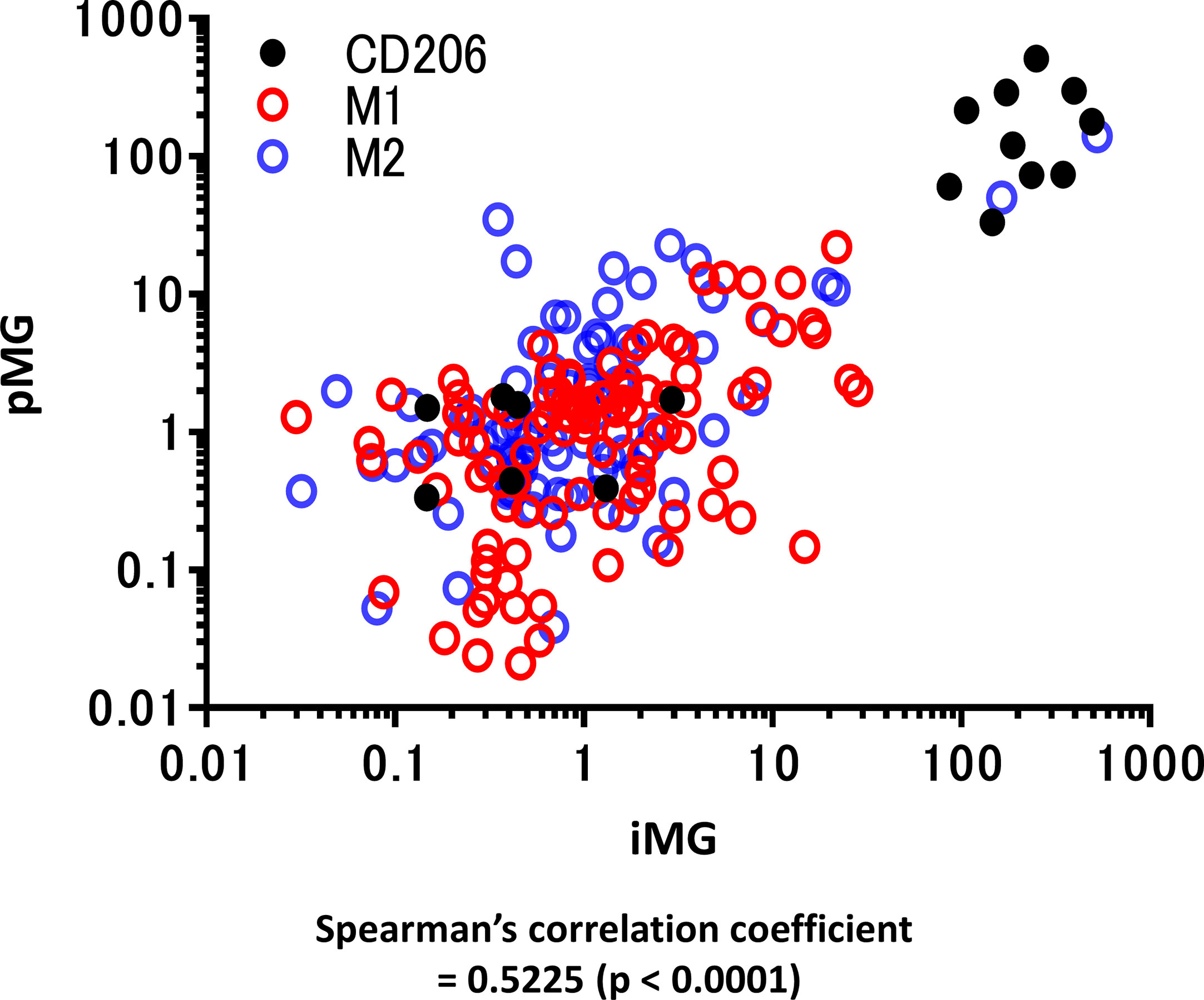
Figure 2 qRT-PCR for representative M1 and M2 microglial markers in primary microglia and induced microglia-like cells. mRNA levels of M1 and M2 microglia markers (12 types in total) were measured using qRT-PCR in 19 cases in which total mRNA was extracted from both induced microglia-like (iMG) cells and primary microglia (pMG). Each value was compared with the control (epilepsy cases without inflammatory infiltrates due to subdural electrode placements, N = 2) to investigate the correlation between iMG and pMG parameters. The expression levels of inflammation-related genes significantly correlated in pMG and iMG cells from the same patients (Spearman’s correlation coefficient = 0.5225, P < 0.0001). Red circles indicate M1 markers, M2 markers are indicated by blue circles, and CD206 is indicated by black dots. qRT-PCR was performed in four independent cell cultures; however, in some cases, the number of pMG or iMG cells was small, and the number of samples was <4 [N = 3.68 (SD ±0.7790)].
Next, to identify glioma-specific transcripts in GAM, the qPCR results were compared between the glioma group (GLI group; N = 9) and radiographic glioma-like mass lesion group (RGM group, N = 6; meningioma, N = 1; metastatic tumor, N = 2; brain abscess, N = 1; encephalitis, N = 1; and radiation necrosis, N = 1). Among the analyzed markers, the expression of CD206, known as an M2 marker, was synchronously upregulated in pMG and iMG cells in six of the nine patients (67%) in the GLI group (Figure 3A). Upregulation of CD206 expression in pMG and iMG cells was particularly pronounced in individuals with GBM, as this phenomenon was observed in four of the five patients studied (80%). The characteristics of the patients from the GLI group are summarized in Table 3, revealing no clear predictive biomarker or background characteristics correlating with CD206 upregulation. In the RGM group, synchronous upregulation of CD206 expression was detected only in two (meningioma and brain abscess) of the six (33%) patients (Figure 3B). The brain tissue around the tumor of the patient with meningioma showed reactive astrocytes and edematous change probably because of the physical compression (Supplementary Figure S2). The surrounding tissue of the patient with brain abscess showed edematous change and infiltration of inflammatory cells. There was no increment in CD206 expression level in two patients with metastatic tumor and two patients who underwent removal of tumor-suspected lesions (radiation necrosis) and encephalitis suspected lesion. Altogether, CD206 upregulation in microglia shown in the surrounding tissue might be a hallmark of glioma, especially in GBM, and can be detected by isolating iMG cells from the peripheral blood of patients.
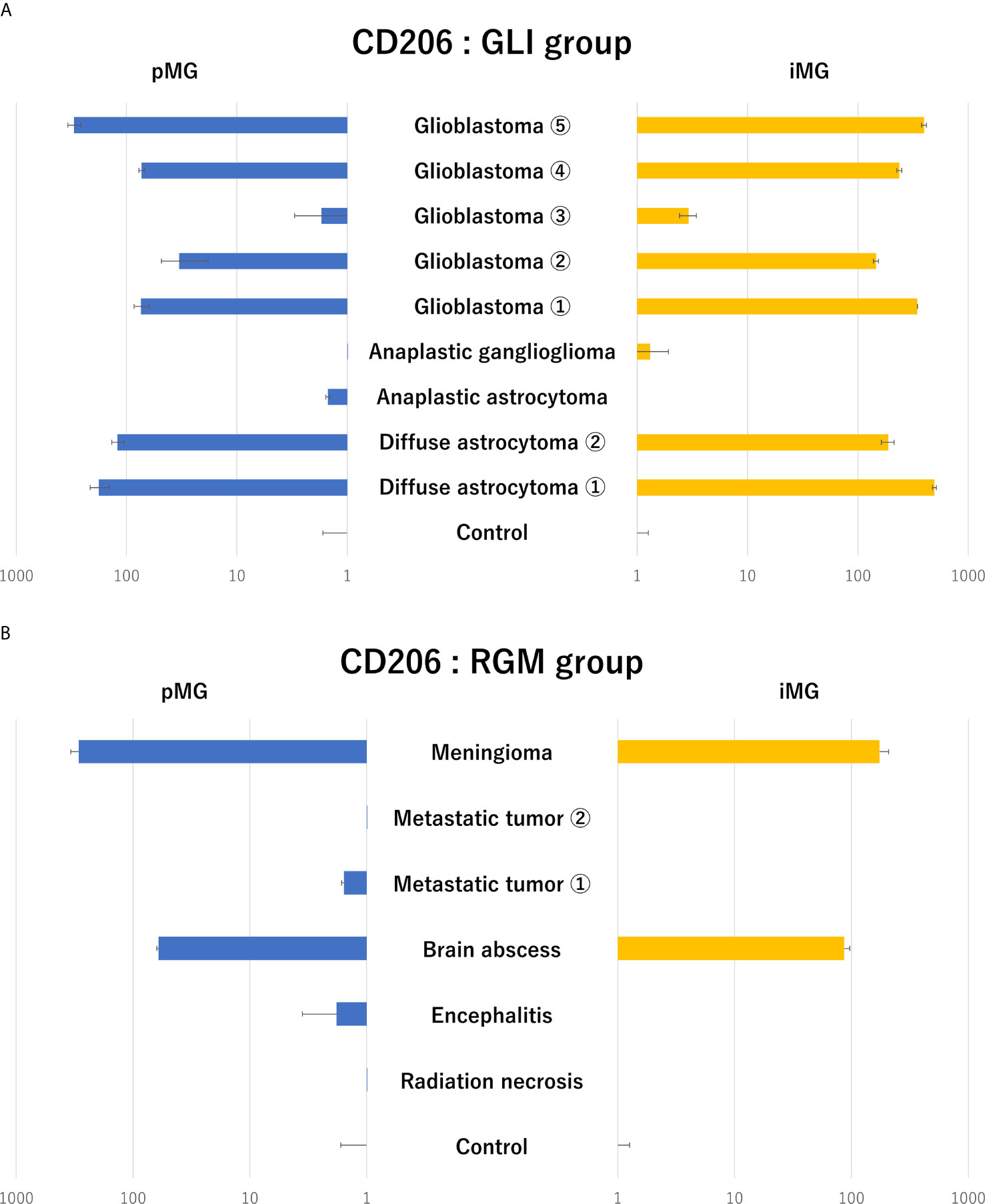
Figure 3 Expression levels of CD206 in primary microglia and induced microglia-like cells in patients with glioma and radiographic glioma-like mass lesions. (A) CD206 expression levels in primary microglia (pMG) and induced microglia-like (iMG) cells in patients with glioma (GLI group) compared to those in control samples. Synchronous upregulation in CD206 expression was detected in pMG and iMG cells in six of the nine (66%) patients, and especially in four of the five (80%) patients with GBM. (B) CD206 expression levels in pMG and iMG cells in patients with a radiographic glioma-like mass lesion (RGM group) compared to those in control samples. Synchronous upregulation of CD206 expression was detected in two of the six (33%) patients. We compared CD206 expression levels in the GLI and RGM groups with those from epilepsy patients as control due to the lack of samples from healthy individuals.
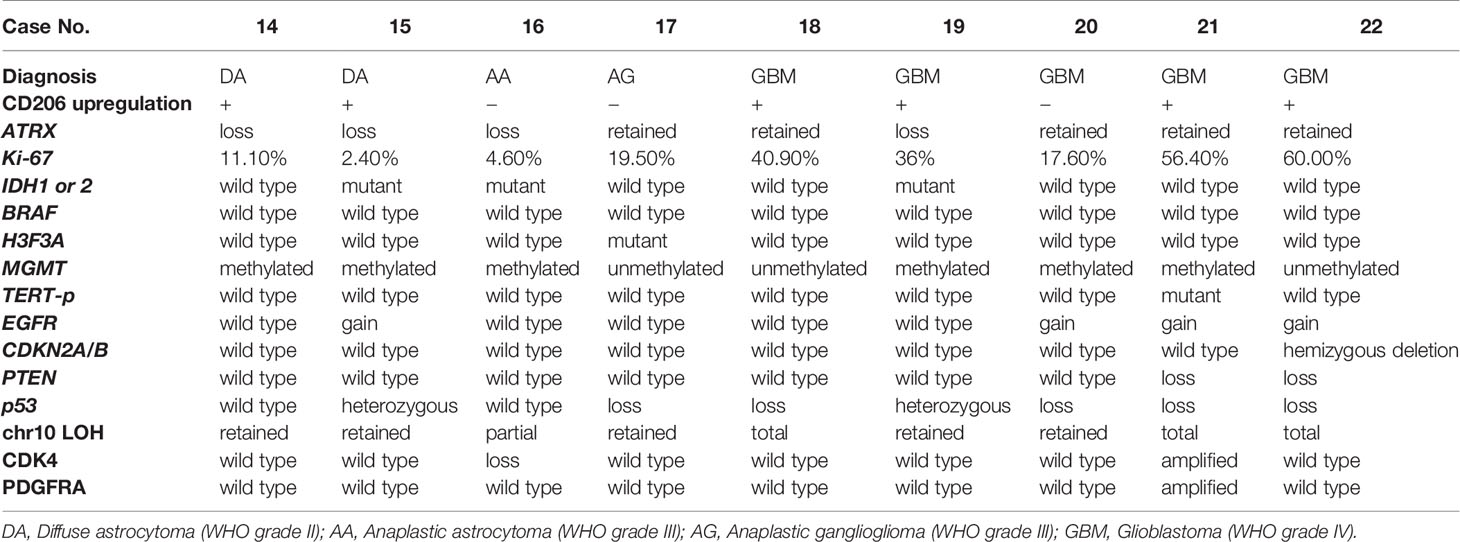
Table 3 Summary of the pathological characteristics and genetic mutations in patients from the glioma group.
Expression CD206, Iba-1, and CD68 in Human Brain Tumor Samples
To assess the invasion of microglia/macrophages in the brain tissue around the tumor, tissue samples of grade II–IV glioma and metastatic tumor were analyzed using immunohistochemistry (Figures 4A–D). Interestingly, the Iba1-positive GAM fraction was the most predominant in all tumors, followed by a slightly lower proportion of cells positive for CD68, a marker for the activated microglia. CD206 tended to be highly expressed in brain tissue surrounding GBM, and conversely, it was weakly expressed in all metastatic brain tumors and grade III gliomas. In grade II gliomas, CD206-positive GAM were present in high numbers. These results were consistent with the PCR results in all tumor cases.
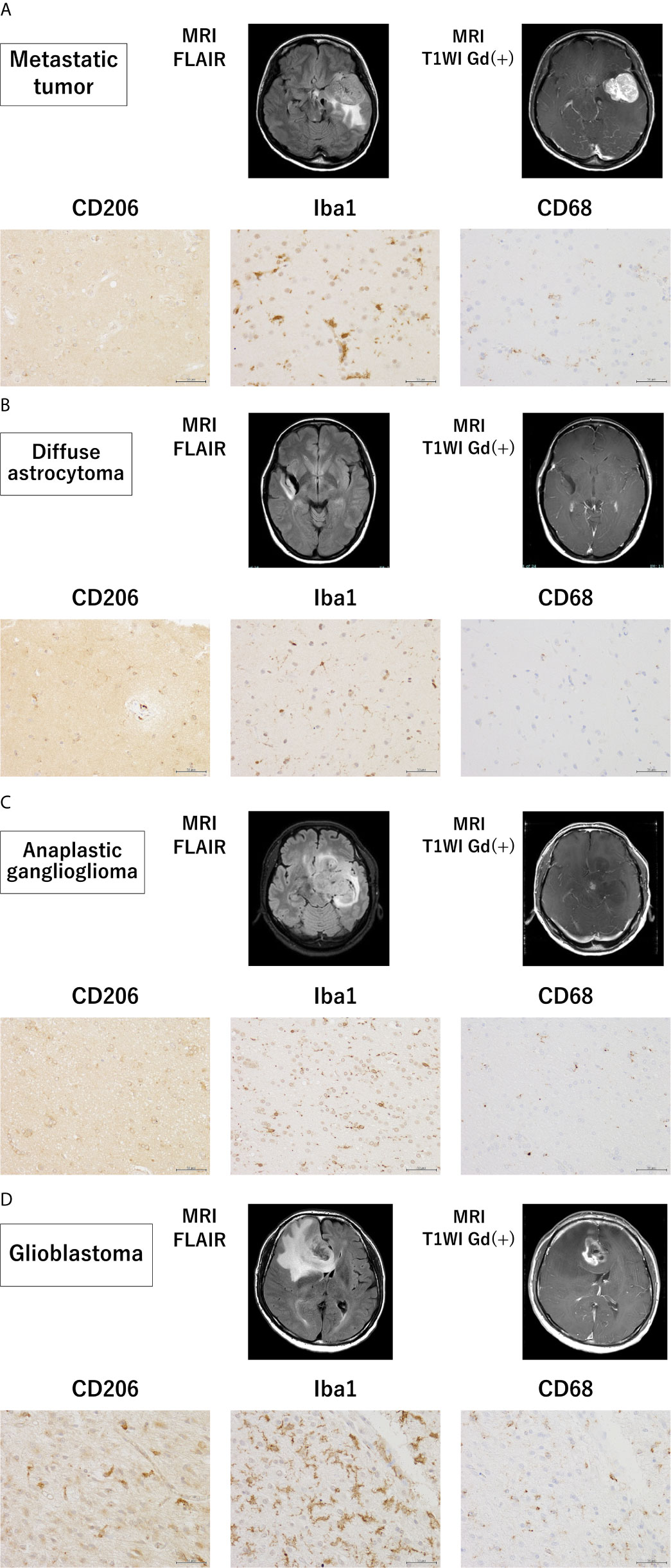
Figure 4 Magnetic resonance imaging findings and immunohistochemistry staining in samples from patients with grade II–IV glioma and metastatic tumor. Magnetic resonance imaging findings and representative immunohistochemical staining for CD206, Iba-1, and CD68 of the brain tissues surrounding a metastatic tumor (A) and glioma (WHO grade II–IV) (B–D) (scale bars = 50 µm).
Discussion
Our study demonstrates that some immune properties of GAM can be monitored using iMG cells isolated from peripheral blood. In recent years, the development of drugs targeting tumor immunity has progressed rapidly. In particular, immune checkpoint inhibitors have already been confirmed to be effective in clinical trials for tumors such as melanoma (2); however, these drugs have not been proven effective for GBM. Mounting evidence has demonstrated that immune response is involved in the development and progression of GBM (14); therefore, a thorough investigation of the underlying molecular and immunologic mechanisms of GBM tumorigenesis is important for developing novel interventions. Our study demonstrated that the specific immune status of glioma might be monitored using peripheral iMG cells, which can be utilized as an effective research tool for the elucidation of immunologic mechanisms of tumorigenesis. Furthermore, GAM that play a role in supporting tumor invasion (35) may become a new target for immunotherapy of GBM in the future.
Our study revealed the specific upregulation of CD206 in iMG cells isolated from the peripheral blood of patients with glioma. These findings indicate that our technique can be used to develop a diagnostic marker for glioma. Although neuroradiological examinations, such as computed tomography and magnetic resonance imaging, are mainly performed as standard clinical examinations for the preoperative diagnosis of GBM, radiographic characteristics of GBM are similar to those of other neuronal diseases, including metastatic brain tumor, brain abscess, and primary CNS lymphoma. Therefore, the development of a tumor-specific marker for GBM would greatly facilitate the presurgical confirmation of diagnosis. There have been several recent attempts of molecular diagnosis of gliomas using cerebrospinal fluid (36, 37); however, those techniques have not achieved the detection of glioma-specific mutations in peripheral blood cells. Recently, several reports on serum microRNAs can distinguish patients with gliomas from healthy controls with high sensitivity and specificity (38, 39). Zhou et al. (40) reviewed 28 reports on the diagnosis of glioma using microRNAs and reported overall sensitivity of 85% and specificity of 90%. Although these studies suggested that microRNA levels are useful for distinguishing glioma and non-glioma cases, a complete consensus has not been reached to date. Our report provides a novel alternative approach for developing a non-invasive diagnostic tool for brain tumors based on a peripheral blood test.
We have previously reported that CD206 expression in iMG cells was downregulated in manic patients with bipolar disorder (34). We hypothesized that psychiatric states change microglial polarization and affect the immune environment, associated with changes in CD206 expression levels. The present study extends the significance of CD206 expression in iMG cells as a marker to glioma, another CNS disease.
Our study revealed the upregulation of CD206 expression using qRT-PCR of microglia extracted from brain tissues around GBM in four of the five studied cases. Few studies have reported that GAM are associated with the pathological grade and prognosis of gliomas. A positive correlation between the World Health Organization pathological grades and the expression of CD206-positive GAM has been reported in human glioma tumor tissues (21). GAM may be induced to M2 polarization by glioma-secreted factors, thereby supporting tumor invasion and growth (35). In low-grade gliomas, Lee et al. reported correlations between malignant transformation, CX3CR1 V249I gene polymorphisms, and tumor immune microenvironment. Tumors from patients that were heterozygous or homozygous for CX3CR1 V249I polymorphisms showed less infiltration of M2 macrophages and had a better prognosis than those in patients without these mutations (41).
We found that iMG cells derived from the peripheral blood showed similar CD206 profiles to pMG in the brain. Recently, some reports have shown that extracellular vesicles derived from GBM can change the phenotype of GAM to an M2-like anti-inflammatory phenotype (42, 43). Gabrusiewicz et al. showed that exosomes secreted from GBM stem cells mainly targeted peripheral blood monocytes to induce immune suppressive M2 phenotype by secreting cytokines such as monocyte chemotactic protein-3 and chemokine (C-X-C motif) ligand 1 (44). Thus, not only the interaction between GBM and GAM, but also between GBM and peripheral blood monocytes through humoral factors and/or extracellular vesicles may reflect the microenvironment of gliomas and can be detected by analyzing iMG cells derived from peripheral monocytes.
Limitations
Studying human GAM has two major challenges. Control tissues are derived from post-mortem tissues or diseased non-tumor patients and, most notably, from epileptic patients because of the lack of naive control samples. However, the brain tissues obtained from patients with epilepsy are not completely normal. The second challenge is the current lack of reliable surface markers for distinguishing brain-resident microglia from infiltrating myeloid cells in the human brain. Experiments with functional assays and flow cytometry would be required to evaluate how closely iMG cells reflect the immunological activity of GAM. However, these experiments could not be performed in this study because the number of cells collected was limited. In several epilepsy patients, non-specific upregulation of microglial markers, including CD206, were observed in tissue samples obtained by the second look surgeries performed approximately 1 or 2 weeks after craniotomies for subdural electrode placements. Histopathological examination of such tissue samples revealed infiltration of chronic inflammatory cells in the subarachnoid space. Physical brain damage caused the infiltration of inflammatory cells, which was thought to have affected the upregulation of CD206 expression (45–47).
In this study, synchronous upregulation of CD206 expression levels was observed in most patients with glioma; however, patients with meningioma and brain abscess showed similar levels. Identifying differences in the properties of microglia and iMG cells between glioma, meningioma, and brain abscess is recommended for future studies. As recent attempts to identify markers distinguishing microglia and monocytes/macrophages have not reached any consensus (48, 49), we analyzed human data using a sorting method based on CD11b+ MACS: this approach assesses all myeloid cells, including monocytes, macrophages, dendritic cells, and neutrophils, besides microglia. Our findings were based on a limited number of patients with brain tumors, including GBM, and a limited number of epilepsy patients as control. Therefore, future studies with a larger number of patients will be necessary to confirm our results.
Conclusion
In summary, our study revealed that peripheral iMG cells obtained by our previously developed technique can be used to gauge the properties of pMG from the tumor lesion microenvironment in the CNS. Therefore, iMG cells are novel, less-invasive tools for monitoring the disease-related immunological state of microglia. They can be used to investigate the roles of microglia in various brain diseases, including glioma. The upregulation of CD206 expression detected using iMG cells has the potential to be used as a glioma biomarker. Our study represents the first step towards understanding the contribution of GAM to the proliferation and invasion of GBM cells. Further studies are needed for investigating the role of microglia in GBM as a better understanding of GAM roles in GBM may provide a new therapeutic target for GBM treatment.
Data Availability Statement
The original contributions presented in the study are included in the article/Supplementary Material, further inquiries can be directed to the corresponding author/s.
Ethics Statement
The studies involving human participants were reviewed and approved by The ethics committee of the Graduate School of Medical Sciences, Kyushu University (application number: 26-406 and 29-624). Written informed consent to participate in this study was provided by the participants’ legal guardian/next of kin. Informed consent was obtained from the patients whose brain tissues had to be resected during the operative procedure for the treatment of brain diseases under a protocol approved by the Ethics Committee of the Graduate School of Medical Sciences at the Kyushu University (application number: 26-406 and 29-624). Freshly resected patient samples and blood samples were provided by the Department of Neurosurgery of the Kyushu University Hospital. Consent to collect blood was not obtained in three patients with epilepsy due to their young age and low body weight. Handling and analysis of these tissues were performed with the approval of the Ethical Committee and according to the principles of the Declaration of Helsinki.
Author Contributions
ST, MO, NH, and TK designed the study. ST and MO conducted the experiments, analyzed the results, and wrote the manuscript. SI, NSa, NSh, YFuj, KT, and YFun performed the experiments. SS and HH analyzed the data. NH, NM, RH, YS, TI, MH, KI, MM, and TK critically revised the manuscript. All authors contributed to the article and approved the submitted version.
Funding
This work was partially supported by Grants-in-Aid for Scientific Research from (1) The Japan Agency for Medical Research and Development (Syogaisya-Taisaku-Sogo-Kenkyu-Kaihatsu-Jigyo to TK (JP18dk0307075), Yugo-No to TK (JP19dm0107095), and MH (JP19ek0610015)), (2) KAKENHI - the Japan Society for the Promotion of Science (JP26713039, JP15K15431, JP16H03741, JP16H06403, JP18H04042 & JP19K21591 to TK, JP19K17065 to MO, JP20K09392 to NH, JP21H03044 to MM), and (3) SENSHIN Medical Research Foundation (to TK). The funders had no role in study design, data collection, and analysis, decision to publish, or manuscript preparation.
Conflict of Interest
The authors declare that the research was conducted in the absence of any commercial or financial relationships that could be construed as a potential conflict of interest.
Acknowledgments
We thank Ms. Aki Sako and Ms. Aya Yamada for their technical assistance. We would like to thank Editage (www.editage.com) for English language editing.
Supplementary Material
The Supplementary Material for this article can be found online at: https://www.frontiersin.org/articles/10.3389/fimmu.2021.670131/full#supplementary-material
Supplementary Figure 1 | Negative control staining for CD 206, Iba-1, and CD68 in patients with grade II–IV glioma and metastatic tumor. The negative controls for CD 206, Iba-1, and CD68 of the brain tissues surrounding metastatic tumor (A) and glioma (WHO grade II–IV) (B–D) (scale bars = 50 µm).
Supplementary Figure 2 | Hematoxylin and eosin staining of the brain tissue around the tumor of a patient with secretory meningioma. The brain tissue around the tumor of a patient with secretory meningioma showed reactive astrocytes and edematous change (scale bars = 50 µm).
Abbreviations
CNS, Central nervous system; GAM, Glioma-associated microglia/macrophages; GBM, Glioblastoma; GLI group, Glioma group; GM-CSF, Granulocyte macrophage colony-stimulating factor; IL, Interleukin; iMG cells, Induced microglia-like cells; PBMCs, Peripheral blood mononuclear cells; pMG, Primary microglia; qRT-PCR, Quantitative reverse transcription-polymerase chain reaction; RGM group, radiographic glioma-like mass lesion group; TGF-β, Transforming growth factor-beta; TNF-α, Tumor necrosis factor-alpha.
References
1. Dolecek TA, Propp JM, Stroup NE, Kruchko C. CBTRUS Statistical Report: Primary Brain and Central Nervous System Tumors Diagnosed in the United States in 2005-2009. Neuro Oncol (2012) 14 Suppl 5:v1–49. doi: 10.1093/neuonc/nos218
2. Robert C, Ribas A, Schachter J, Arance A, Grob J-J, Mortier L, et al. Pembrolizumab Versus Ipilimumab in Advanced Melanoma (KEYNOTE-006): Post-Hoc 5-Year Results From an Open-Label, Multicentre, Randomised, Controlled, Phase 3 Study. Lancet Oncol (2019) 20(9):1239–51. doi: 10.1016/s1470-2045(19)30388-2
3. Wolchok JD, Chiarion-Sileni V, Gonzalez R, Rutkowski P, Grob JJ, Cowey CL, et al. Overall Survival With Combined Nivolumab and Ipilimumab in Advanced Melanoma. N Engl J Med (2017) 377(14):1345–56. doi: 10.1056/NEJMoa1709684
4. Omuro A, Vlahovic G, Lim M, Sahebjam S, Baehring J, Cloughesy T, et al. Nivolumab With or Without Ipilimumab in Patients With Recurrent Glioblastoma: Results From Exploratory Phase I Cohorts of CheckMate 143. Neuro Oncol (2018) 20(5):674–86. doi: 10.1093/neuonc/nox208
5. Filley AC, Henriquez M, Dey M. Recurrent Glioma Clinical Trial, CheckMate-143: The Game is Not Over Yet. Oncotarget (2017) 8(53):91779–94. doi: 10.18632/oncotarget.21586
6. Wolf SA, Boddeke HW, Kettenmann H. Microglia in Physiology and Disease. Annu Rev Physiol (2017) 79:619–43. doi: 10.1146/annurev-physiol-022516-034406
7. Mosser DM, Edwards JP. Exploring the Full Spectrum of Macrophage Activation. Nat Rev Immunol (2008) 8(12):958–69. doi: 10.1038/nri2448
8. Kettenmann H, Hanisch UK, Noda M, Verkhratsky A. Physiology of Microglia. Physiol Rev (2011) 91(2):461–553. doi: 10.1152/physrev.00011.2010
9. Mantovani A, Sica A, Sozzani S, Allavena P, Vecchi A, Locati M. The Chemokine System in Diverse Forms of Macrophage Activation and Polarization. Trends Immunol (2004) 25(12):677–86. doi: 10.1016/j.it.2004.09.015
10. Ye XZ, Xu SL, Xin YH, Yu SC, Ping YF, Chen L, et al. Tumor-Associated Microglia/Macrophages Enhance the Invasion of Glioma Stem-Like Cells Via TGF-beta1 Signaling Pathway. J Immunol (2012) 189(1):444–53. doi: 10.4049/jimmunol.1103248
11. da Fonseca AC, Badie B. Microglia and Macrophages in Malignant Gliomas: Recent Discoveries and Implications for Promising Therapies. Clin Dev Immunol (2013) 2013:264124. doi: 10.1155/2013/264124
12. Rossi ML, Hughes JT, Esiri MM, Coakham HB, Brownell DB. Immunohistological Study of Mononuclear Cell Infiltrate in Malignant Gliomas. Acta Neuropathol (1987) 74(3):269–77. doi: 10.1007/BF00688191
13. Wood GW, Morantz RA. Immunohistologic Evaluation of the Lymphoreticular Infiltrate of Human Central Nervous System Tumors. J Natl Cancer Inst (1979) 62(3):485–91. doi: 10.1093/jnci/62.3.485
14. Hambardzumyan D, Gutmann DH, Kettenmann H. The Role of Microglia and Macrophages in Glioma Maintenance and Progression. Nat Neurosci (2016) 19(1):20–7. doi: 10.1038/nn.4185
15. Bettinger I, Thanos S, Paulus W. Microglia Promote Glioma Migration. Acta Neuropathol (2002) 103(4):351–5. doi: 10.1007/s00401-001-0472-x
16. Watters JJ, Schartner JM, Badie B. Microglia Function in Brain Tumors. J Neurosci Res (2005) 81(3):447–55. doi: 10.1002/jnr.20485
17. Qian J, Luo F, Yang J, Liu J, Liu R, Wang L, et al. Tlr2 Promotes Glioma Immune Evasion by Downregulating Mhc Class Ii Molecules in Microglia. Cancer Immunol Res (2018) 6(10):1220–33. doi: 10.1158/2326-6066.Cir-18-0020
18. Zhang Z, Xu J, Chen Z, Wang H, Xue H, Yang C, et al. Transfer of MicroRNA Via Macrophage-Derived Extracellular Vesicles Promotes Proneural-to-Mesenchymal Transition in Glioma Stem Cells. Cancer Immunol Res (2020) 8(7):966–81. doi: 10.1158/2326-6066.Cir-19-0759
19. Wei J, Gabrusiewicz K, Heimberger A. The Controversial Role of Microglia in Malignant Gliomas. Clin Dev Immunol (2013) 2013:285246. doi: 10.1155/2013/285246
20. Gjorgjevski M, Hannen R, Carl B, Li Y, Landmann E, Buchholz M, et al. Molecular Profiling of the Tumor Microenvironment in Glioblastoma Patients: Correlation of Microglia/Macrophage Polarization State With Metalloprotease Expression Profiles and Survival. Biosci Rep (2019) 39(6):BSR20182361. doi: 10.1042/BSR20182361
21. Ding P, Wang W, Wang J, Yang Z, Xue L. Expression of Tumor-Associated Macrophage in Progression of Human Glioma. Cell Biochem Biophys (2014) 70(3):1625–31. doi: 10.1007/s12013-014-0105-3
22. Ohgidani M, Kato TA, Setoyama D, Sagata N, Hashimoto R, Shigenobu K, et al. Direct Induction of Ramified Microglia-Like Cells From Human Monocytes: Dynamic Microglial Dysfunction in Nasu-Hakola Disease. Sci Rep (2014) 4:4957. doi: 10.1038/srep04957
23. Ohgidani M, Kato TA, Kanba S. Introducing Directly Induced Microglia-Like (iMG) Cells From Fresh Human Monocytes: A Novel Translational Research Tool for Psychiatric Disorders. Front Cell Neurosci (2015) 9:184. doi: 10.3389/fncel.2015.00184
24. Sato-Kasai M, Kato TA, Ohgidani M, Mizoguchi Y, Sagata N, Inamine S, et al. Aripiprazole Inhibits polyI:C-Induced Microglial Activation Possibly Via TRPM7. Schizophr Res (2016) 178(1-3):35–43. doi: 10.1016/j.schres.2016.08.022
25. Atoshi Banerjee YL, Do K, Mize T, Wu X, Chen X, Chen J. Validation of Induced Microglia-Like Cells (Img Cells) for Future Studies of Brain Diseases. Front Cell Neurosci (2021) 15:629279. doi: 10.3389/fncel.2021.629279
26. Sellgren CM, Sheridan SD, Gracias J, Xuan D, Fu T, Perlis RH. Patient-Specific Models of Microglia-Mediated Engulfment of Synapses and Neural Progenitors. Mol Psychiatry (2017) 22(2):170–7. doi: 10.1038/mp.2016.220
27. Stahl PD, Ezekowitz RAB. The Mannose Receptor is a Pattern Recognition Receptor Involved in Host Defense. Curr Opin Immunol (1998) 10(1):50–5. doi: 10.1016/S0952-7915(98)80031-9
28. Marzolo MP, von Bernhardi R, Inestrosa NC. Mannose Receptor is Present in a Functional State in Rat Microglial Cells. J Neurosci Res (1999) 58(3):387–95. doi: 10.1002/(Sici)1097-4547(19991101)58:3<387::Aid-Jnr4>3.0.Co;2-L
29. Durafourt BA, Moore CS, Zammit DA, Johnson TA, Zaguia F, Guiot MC, et al. Comparison of Polarization Properties of Human Adult Microglia and Blood-Derived Macrophages. Glia (2012) 60(5):717–27. doi: 10.1002/glia.22298
30. Burudi EME, Riese S, Stahl PD, Rgnier-Vigouroux A. Identification and Functional Characterization of the Mannose Receptor in Astrocytes. Glia (1999) 25(1):44–55. doi: 10.1002/(sici)1098-1136(19990101)25:1<44::Aid-glia5>3.0.Co;2-c
31. Régnier-Vigouroux A. The Mannose Receptor in the Brain. Int Rev Cytol (2003) 226:321–42. doi: 10.1016/s0074-7696(03)01006-4
32. Kobayashi K, Imagama S, Ohgomori T, Hirano K, Uchimura K, Sakamoto K, et al. Minocycline Selectively Inhibits M1 Polarization of Microglia. Cell Death Dis (2013) 4:e525. doi: 10.1038/cddis.2013.54
33. Zimmer H, Riese S, Regnier-Vigouroux A. Functional Characterization of Mannose Receptor Expressed by Immunocompetent Mouse Microglia. Glia (2003) 42(1):89–100. doi: 10.1002/glia.10196
34. Ohgidani M, Kato TA, Haraguchi Y, Matsushima T, Mizoguchi Y, Murakawa-Hirachi T, et al. Microglial CD206 Gene Has Potential as a State Marker of Bipolar Disorder. Front Immunol (2016) 7:676. doi: 10.3389/fimmu.2016.00676
35. Pyonteck SM, Akkari L, Schuhmacher AJ, Bowman RL, Sevenich L, Quail DF, et al. Csf-1R Inhibition Alters Macrophage Polarization and Blocks Glioma Progression. Nat Med (2013) 19(10):1264–72. doi: 10.1038/nm.3337
36. Huang SW, Ali ND, Zhong L, Shi J. MicroRNAs as Biomarkers for Human Glioblastoma: Progress and Potential. Acta Pharmacol Sin (2018) 39(9):1405–13. doi: 10.1038/aps.2017.173
37. Fujioka Y, Hata N, Akagi Y, Kuga D, Hatae R, Sangatsuda Y, et al. Molecular Diagnosis of Diffuse Glioma Using a Chip-Based Digital PCR System to Analyze IDH, TERT, and H3 Mutations in the Cerebrospinal Fluid. J Neurooncol (2021) 152(1):47–54. doi: 10.1007/s11060-020-03682-7
38. Ohno M, Matsuzaki J, Kawauchi J, Aoki Y, Miura J, Takizawa S, et al. Assessment of the Diagnostic Utility of Serum Microrna Classification in Patients With Diffuse Glioma. JAMA Netw Open (2019) 2(12):e1916953. doi: 10.1001/jamanetworkopen.2019.16953
39. Zhi F, Shao N, Wang R, Deng D, Xue L, Wang Q, et al. Identification of 9 Serum microRNAs as Potential Noninvasive Biomarkers of Human Astrocytoma. Neuro Oncol (2015) 17(3):383–91. doi: 10.1093/neuonc/nou169
40. Zhou Q, Liu J, Quan J, Liu W, Tan H, Li W. MicroRNAs as Potential Biomarkers for the Diagnosis of Glioma: A Systematic Review and Meta-Analysis. Cancer Sci (2018) 109(9):2651–9. doi: 10.1111/cas.13714
41. Lee S, Latha K, Manyam G, Yang Y, Rao A, Rao G. Role of CX3CR1 Signaling in Malignant Transformation of Gliomas. Neuro Oncol (2020) 22(10):1463–73. doi: 10.1093/neuonc/noaa075
42. van der Vos KE, Abels ER, Zhang X, Lai C, Carrizosa E, Oakley D, et al. Directly Visualized Glioblastoma-Derived Extracellular Vesicles Transfer RNA to Microglia/Macrophages in the Brain. Neuro Oncol (2016) 18(1):58–69. doi: 10.1093/neuonc/nov244
43. Abels ER, Maas SLN, Nieland L, Wei Z, Cheah PS, Tai E, et al. Glioblastoma-Associated Microglia Reprogramming Is Mediated by Functional Transfer of Extracellular Mir-21. Cell Rep (2019) 28(12):3105–19.e7. doi: 10.1016/j.celrep.2019.08.036
44. Gabrusiewicz K, Li X, Wei J, Hashimoto Y, Marisetty AL, Ott M, et al. Glioblastoma Stem Cell-Derived Exosomes Induce M2 Macrophages and PD-L1 Expression on Human Monocytes. Oncoimmunology (2018) 7(4):e1412909. doi: 10.1080/2162402X.2017.1412909
45. Jin X, Ishii H, Bai Z, Itokazu T, Yamashita T. Temporal Changes in Cell Marker Expression and Cellular Infiltration in a Controlled Cortical Impact Model in Adult Male C57BL/6 Mice. PloS One (2012) 7(7):e41892. doi: 10.1371/journal.pone.0041892
46. Loane DJ, Kumar A. Microglia in the TBI Brain: The Good, the Bad, and the Dysregulated. Exp Neurol (2016) 275 Pt 3:316–27. doi: 10.1016/j.expneurol.2015.08.018
47. Wang G, Zhang J, Hu X, Zhang L, Mao L, Jiang X, et al. Microglia/Macrophage Polarization Dynamics in White Matter After Traumatic Brain Injury. J Cereb Blood Flow Metab (2013) 33(12):1864–74. doi: 10.1038/jcbfm.2013.146
48. Butovsky O, Jedrychowski MP, Moore CS, Cialic R, Lanser AJ, Gabriely G, et al. Identification of a Unique TGF-beta-dependent Molecular and Functional Signature in Microglia. Nat Neurosci (2014) 17(1):131–43. doi: 10.1038/nn.3599
Keywords: microglia, glioma, CD206, surrogate biomarker, induced microglia-like cells
Citation: Tanaka S, Ohgidani M, Hata N, Inamine S, Sagata N, Shirouzu N, Mukae N, Suzuki SO, Hamasaki H, Hatae R, Sangatsuda Y, Fujioka Y, Takigawa K, Funakoshi Y, Iwaki T, Hosoi M, Iihara K, Mizoguchi M and Kato TA (2021) CD206 Expression in Induced Microglia-Like Cells From Peripheral Blood as a Surrogate Biomarker for the Specific Immune Microenvironment of Neurosurgical Diseases Including Glioma. Front. Immunol. 12:670131. doi: 10.3389/fimmu.2021.670131
Received: 20 February 2021; Accepted: 04 June 2021;
Published: 29 June 2021.
Edited by:
Valérie Dutoit, Université de Genève, SwitzerlandReviewed by:
Michael Rückert, University Hospital Erlangen, GermanyMichel Guy André Mittelbronn, National Health Laboratory, Luxembourg
Copyright © 2021 Tanaka, Ohgidani, Hata, Inamine, Sagata, Shirouzu, Mukae, Suzuki, Hamasaki, Hatae, Sangatsuda, Fujioka, Takigawa, Funakoshi, Iwaki, Hosoi, Iihara, Mizoguchi and Kato. This is an open-access article distributed under the terms of the Creative Commons Attribution License (CC BY). The use, distribution or reproduction in other forums is permitted, provided the original author(s) and the copyright owner(s) are credited and that the original publication in this journal is cited, in accordance with accepted academic practice. No use, distribution or reproduction is permitted which does not comply with these terms.
*Correspondence: Takahiro A. Kato, dGFrYWhpcm9AbnBzeWNoLm1lZC5reXVzaHUtdS5hYy5qcA==
†These authors have contributed equally to this work