- Department of Medicine, Division of Cardiology, Columbia University Irving Medical Center, Columbia University, New York, NY, United States
Heart transplant candidates sensitized to HLA antigens wait longer for transplant, are at increased risk of dying while waiting, and may not be listed at all. The increasing prevalence of HLA sensitization and limitations of current desensitization strategies underscore the urgent need for a more effective approach. In addition to pregnancy, prior transplant, and transfusions, patients with end-stage heart failure are burdened with unique factors placing them at risk for HLA sensitization. These include homograft material used for congenital heart disease repair and left ventricular assist devices (LVADs). Moreover, these risks are often stacked, forming a seemingly insurmountable barrier in some cases. While desensitization protocols are typically implemented uniformly, irrespective of the mode of sensitization, the heterogeneity in success and post-transplant outcomes argues for a more tailored approach. Achieving this will require progress in our understanding of the immunobiology underlying the innate and adaptive immune response to these varied allosensitizing exposures. Further attention to B cell activation, memory, and plasma cell differentiation is required to establish methods that durably abrogate the anti-HLA antibody response before and after transplant. The contribution of non-HLA antibodies to the net state of sensitization and the potential implications for graft longevity also remain to be comprehensively defined. The aim of this review is to first bring forth select issues unique to the sensitized heart transplant candidate. The current literature on desensitization in heart transplantation will then be summarized providing context within the immune response. Building on this, newer approaches with therapeutic potential will be discussed emphasizing the importance of not only addressing the short-term pathogenic consequences of circulating HLA antibodies, but also the need to modulate alloimmune memory.
Introduction: Sensitization to Human Leukocyte Antigens (HLA) in Heart Transplantation
HLA sensitization is a major barrier to heart transplantation, the incidence of which is increasing (1, 2). Sensitized patients wait longer for transplant and are at higher risk of dying on the waiting list or being delisted (3). Post-transplant, there is an increased incidence of adverse outcomes including both cellular and antibody mediated rejection as well as cardiac allograft vasculopathy (4–6). Heart transplant candidates bear the burden of all the same risk factors for sensitization as other transplant candidates, namely pregnancy, prior transplant, and transfusions. However, additional sensitizing events also contribute. These include homografts (7) used for congenital heart disease repair, and mechanical circulatory support (5), most commonly left ventricular assist devices (LVADs). Given that immune ‘insults’ can accumulate over time, this risk can be quickly magnified.
As is the case across solid organ transplantation, desensitization strategies are limited by variable efficacy and frequently accompanied by brisk rebound. In the case of end-stage heart disease, this is further complicated by tenuous hemodynamics, time sensitivity, and in some cases chronic device infections. In the United States, sensitized transplant candidates do not receive priority status on the waitlist (8). While policy change is one approach, prioritization can be a double-edged sword, resulting in hesitancy to develop robust, mechanistically driven desensitization strategies with the potential to improve post-transplant outcomes (9). Instead, prioritization may favor peri-transplant antibody management approaches that temporize the situation but do not modulate the underlying immune response. Multidrug regimens may more comprehensively address the underlying immune response, particularly for patients in whom years of repeated allosensitizing events result in high titer HLA antibodies with cytotoxic capabilities (10–12). However, the factors driving differences in B cell memory and plasma cell characteristics are incompletely understood, the response to treatment is heterogeneous, and therapeutic options remain limited. Moreover, while emphasis has been placed on therapeutic approaches that directly target humoral alloimmunity, it is important to consider that allosensitization includes T-cell memory and that there is growing appreciation of the complex interaction between innate and adaptive immunity. The objective of this review is to 1) summarize the current state of desensitization in heart transplantation supported by experience in kidney, 2) provide context within the immune response, and 3) building on these findings, introduce rational strategies with the potential to improve long-term outcomes. A brief overview of HLA sensitization, the methods used to detect allosensitization, and considerations unique to heart transplantation will first be provided to contextualize the review.
Sensitization and Unique Considerations for the Heart Transplant Candidate
Overview of the Immune Response to Foreign HLA
Exposure to foreign HLA can initiate a complex set of immune reactions which may result in a short-term ‘effector response’, long-lived plasma cells (LLPCs) capable of sustained antibody secretion over decades, and/or the establishment of B and T cell memory [reviewed in (13)]. As a result, HLA allosensitization may either be overt (detectable HLA antibodies) or cryptic (the presence of cellular memory in the absence of detectable HLA antibodies). Although only the former is commonly considered in the context of desensitization, both are associated with increased risk of rejection and/or worse outcomes after transplant (14, 15). Importantly, because HLA antibodies are the product of a T cell-dependent response (16), and because alloreactive effector T cells themselves are potently pathogenic, both T and B cell memory, as well as their ability to sustain reciprocal interactions, should be considered (17). Notably, monocytes, dendritic cells (DCs) and natural killer (NK) cells, which are at the boundary between innate and adaptive immunity, feature prominently in the response to the allograft, yet their importance in the context of desensitization is only beginning to be considered. Figure 1 provides a schematic overview along with the most common current approaches to desensitization based on a recent international survey (4). The primary and recall humoral response are detailed in several recent reviews and summarized below (13, 18, 19). Alloreactive T cells and innate immunity will also be briefly considered.
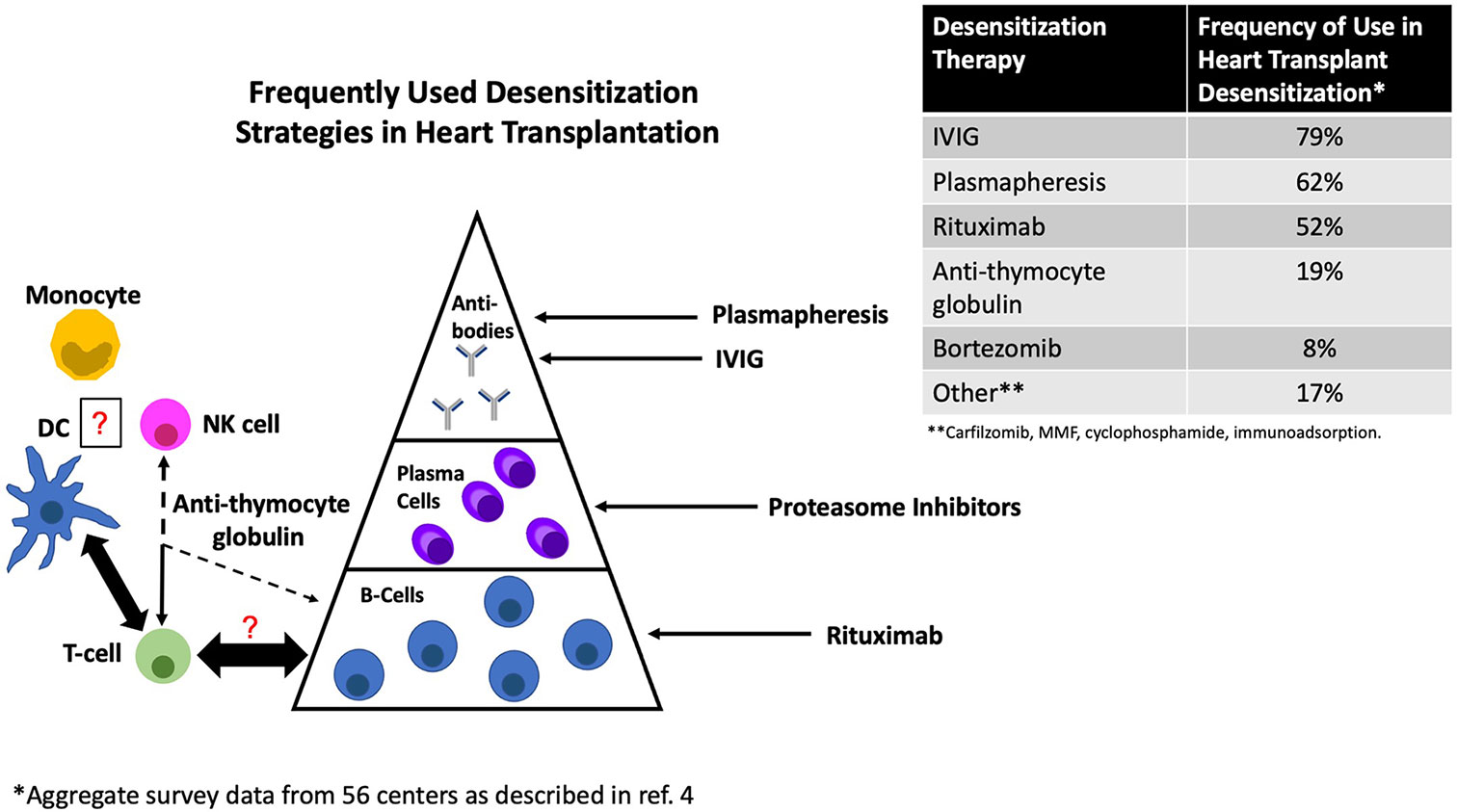
Figure 1 Common approaches to desensitization in heart transplantation and their primary site of action. DC, dendritic cell; NK, natural killer cell.
The Adaptive Response to HLA Antigens: Humoral Alloimmunity
In brief, during the primary immune response, non-self HLA encountered during pregnancy (paternal), exposure to foreign tissue (transplant, homografts), or blood products (transfusions) is transported to secondary lymphoid organs (e.g., lymph nodes or spleen) which are broadly divided into B cell rich follicles and T cells zones (18). T and B cells first encounter antigen independently in their respective zones. T cells acquire a T follicular helper (Tfh) like phenotype (upregulating BCL-6, CXCR5, PD1, and ICOS) while B cells downregulate CXCR5 and upregulate CCR7 and EBI2 allowing them to migrate to the T cell-B cell border (20). Here, cognate interactions with T cells occur in the presence of costimulatory signals (CD28, CD40L, and ICOS). At this stage, B cells can either directly differentiate in an extrafollicular manner or enter the germinal center. Extrafollicular B cells can differentiate into antibody secreting cells (ASCs) or acquire a memory phenotype (13). Alternatively, B cells can enter the germinal center (GC) which marks another critical collaboration between T and B cells ultimately resulting in selection and clonal proliferation of B cells with a high affinity B cell receptor (BCR). Some of these may migrate to the bone marrow and become plasma cells (BMPCs), while others differentiate into quiescent memory B cells (Bmem). The understanding of these different fates and factors driving them is rapidly evolving and beyond the scope of this review. However, three concepts emerge with particular relevance from the desensitization perspective. Firstly, it is important to consider that both extrafollicular and GC B cells can acquire a memory phenotype with heterogeneity in Ig subclass, antigen affinity (resulting from somatic hypermutation), and longevity (19, 21, 22). Secondly, because BMPCs secrete antibodies with specificities that parallel those found in the blood (23), they represent an important therapeutic target. Establishing the phenotype and characteristics of anti-HLA-secreting BMPCs and the factors driving their differentiation will be critical to designing targeted therapies that ideally eliminate HLA-secreting PCs while leaving protective immunity intact. Thirdly, the opportunity for continual T cell-B cell interactions both within and outside of the GC suggests that strategies targeting these interactions may be beneficial both to dampen the active alloresponse and prevent rebound (24, 25).
The Adaptive Response to HLA Antigens: T-Cell Alloimmunity
Sensitized transplant candidates are at increased risk of cellular rejection highlighting the effect of enhanced T-cell reactivity towards the donor graft independent of the T-dependent antibody response (26, 27). In addition to overt exposure to antigenic HLA, the recipient’s history of encounter with environmental antigens can shape the donor reactive response through cross-reactivity or heterologous immunity (28, 29). In kidney transplant recipients, the extent of HLA molecular mismatch also influences alloimmune risk (30). For the sensitized heart transplant candidate, these factors can fine tune the donor reactive response and influence post-transplant outcomes. For the patient without an apparent sensitization history, this implies that absence of overt HLA exposure is not synonymous with low risk. At the epidemiological level, this may contribute to the heterogeneity in reported outcomes amongst HLA sensitized heart transplant recipients.
The Innate-Adaptive Interface in HLA Sensitization
While treatment of HLA sensitization is focused on the aforementioned adaptive immune response, innate immune cells, including monocytes, dendritic cells (DCs), and natural killer (NK) cells play an important role in the response to the allograft. DCs serve as antigen presenting cells (APCs) to activate T cells and are therefore a critical first step in the adaptive response. However, because this can occur through the direct, indirect, and semi-direct pathways [reviewed in (31–33)] activation of both CD4 and CD8 T cell can theoretically occur at any timepoint post-transplant. Monocytes are the precursors to some subsets of DCs and also macrophages the latter featuring prominently in the allograft during AMR, secreting proinflammatory cytokines, recruiting additional immune cells, present antigen locally, and provide costimulatory signals (34). NK cells are also present in the allograft during antibody mediated rejection (AMR) suggesting a logical link between DSA and graft damage via antibody-dependent cellular cytotoxicity (ADCC) (35). However, NK cells can function independent of DSA secreting cytotoxic, proinflammatory molecules in the setting of ‘non-self’ recognition (36, 37). While the above characterization suggests a pathogenic role for the innate response, tolerogenic potential has also been described (31, 34). Moreover, at least some innate immune cells can develop memory adding a layer of complexity to their role in the allograft over time. This suggests that manipulation, rather than inhibition of the innate immune response may be of benefit. Whether strategies that synergistically modulate innate immunity while abrogating the alloreactive adaptive response can lead to sustained improvement in long-term outcomes for highly sensitized transplant recipients, remains to be established.
Assessment of Allosensitization
In addition to HLA antibody detection, which is most commonly done using the Luminex Single Antigen Bead (SAB) assay, numerous approaches have been developed to detect alloreactive memory T and B cells. Table 1 provides an overview of some of these assays. It should be noted that, at the present time most of these remain exploratory in nature. For further descriptions, the reader is referred to Sensitization in Transplantation: Assessment of Risk (2017 & 2019) (38, 39) as well as several recent reviews (40, 47–49).
Unique Mechanisms of HLA Sensitization in Heart Transplantation and Their Implications for Desensitization
Congenital Heart Disease
Advances in surgical techniques to repair congenital heart defects have revolutionized the prognosis for children with complex congenital heart disease (CHD) with many surviving to adulthood (50–52). Some will develop end stage disease making CHD the second most common indication for heart transplantation between the ages of 18-39 (53). The risk of HLA sensitization is increased, in many cases driven by homograft material used during previous surgical repair leading to chronic exposure to foreign HLA (54). In the absence of immunosuppression brisk allosensitization ensues, which is often broadly reactive, high titer, and resistant to desensitization (7, 55–58). This is further compounded by multiple sternotomies, blood transfusions, and in some cases left ventricular assist device (LVAD) implantation (59). Given that many such patients may be turned down for transplant, the true scope of the problem is likely underestimated. Whether children, with perhaps greater immune plasticity and a shorter duration of exposure, respond differently is difficult to parse out (60–62).
Pregnancy & Peripartum Cardiomyopathy
Peripartum cardiomyopathy (PPCM) is an important cause of morbidity and mortality globally with a geographically polarized incidence ranging from 1:100 in the developing world to 1:2000-4000 in the US where cases may be on the rise (62–67). While many women will recover, a subset progress to end-stage disease necessitating advanced therapies. In the transplant setting, PPCM is associated with higher PRA, increased risk of rejection and worse survival (68). The divergence between the potential for a tolerogenic T cell state and the development of antibodies against paternal antigens raises important questions in the context of transplantation (69). Whether the ‘T-cell centric’ focus of our current immunosuppressive regimens, and relative lack of adequate B cell control is particularly detrimental in this setting is unknown. In a mouse model of pregnancy induced allosensitization followed by heart transplant with a semi-allogenic graft, T cell tolerance to the graft was overcome in the presence of pregnancy-sensitized B cells, whether or not DSA was present. B cell depletion (α-CD20) restored allograft acceptance (70). The clinical implications remain to be defined, but may be of particular relevance when transplant occurs in close proximity to the sensitizing event.
LVAD
Nearly half of patients who undergo heart transplantation are supported on an LVAD prior to transplant (71). While LVAD implant is associated with increased risk of developing HLA-reactive antibodies, their significance in terms of post-transplant outcomes is less clear (72). In an early UNOS registry analysis, LVAD recipients waited longer for transplant but there was no difference in 1-year survival or rejection episodes between sensitized and non-sensitized patients, even when highly sensitized (PRA>90%) patients were considered (72). Similarly, Chiu et al. used propensity matching to compare sensitized transplant candidates with and without mechanical circulatory support (MCS) (5). In contrast to non-MCS sensitized candidates who had increased 1-year mortality, patients transplanted from MCS had similar outcomes to non-sensitized transplant recipients.
Both the quality and duration of de novo detectable HLA antibody responses following LVAD implant may contribute to the discrepancy in outcomes. Using the Luminex Single Antigen Bead Assay (SAB), Shankar et al. described an increase in cPRA from 20% to 53% (p=0.024) after LVAD implant without evidence of cytotoxicity (defined as a CDC PRA>10%) (73). Similarly, a recent comparison of the HeartMate II and HeartMate 3 (HM3) device found that de novo sensitization, defined by SAB, persists with the newer generation HM3 although the development of high MFI (>10,000) class I antibodies was less frequent. Similar to the findings by Shankar et al, cytotoxicity defined by a de novo positive CDC PRA >10%, was low (74). In contrast to pregnancy and prior transplant, where high MFI HLA antibodies can persist for decades, the response post-LVAD may decline more quickly. In a cohort of 268 patients, 30 (23%) developed newly detectable HLA sensitization (defined as cPRA>10%) after LVAD implant, which declined over time in 67% of these transplant candidates (cPRA<10%) without desensitization (75). Nonetheless, compared to non-sensitized or previously sensitized transplant recipients, the risk of ACR and AMR was higher suggesting that memory persist.
Potential device-intrinsic effects on cellular and humoral immunity have been investigated (76). Whether the type of device and generation affects this is still unclear. Early studies in patients bridged to transplant with first-generation LVADs demonstrated diminished T cell responses in mixed lymphocyte reactions while non-TCR mediated activation was less affected (77). Apoptosis was enhanced, especially in the CD4 compartment although the specificity was not established. In vivo, the response to intradermal skin challenge with mumps and candida albicans was impaired raising the possibility of T cell dysfunction. Non-HLA humoral sensitization with reactivity to autoantigens has also been described (78, 79). In a cohort of patients bridged to transplant with a second-generation LVAD, this was attributed to natural (polyreactive) antibodies. A potential association with primary graft dysfunction but not rejection was found (80). Collectively, the increased incidence of allosensitization and predisposition to early cellular rejection raises the question of whether selective inhibition of B cell – T cell interactions and/or enhancing regulatory T cell function would be of particular benefit in this population.
Antibodies to Non-HLA Antigens and Their Relevance to the Sensitized Heart Transplant Candidate
Antibodies to non-HLA antigens including the angiotensin II type 1 receptor (AT1R), major histocompatibility complex class I chain-related molecule A (MICA), cardiac myosin, and vimentin have been associated with increased risk of adverse outcomes in heart transplant recipients (81–86). Many of these studies were retrospective, single-center analyses and dissecting the role of pre-transplant non-HLA antibodies versus their development post-transplant has also been difficult. In the multicenter CTOT-5 long-term follow up cohort, the persistence at 12-months of anti-cardiac myosin antibodies (but not vimentin) present before transplant was associated with the composite endpoint of death, retransplantation, coronary stent, myocardial infarction, and cardiac allograft vasculopathy, albeit that this association was weak (87). Some but not all studies have described an association between pre-transplant anti-AT1R antibodies and increased risk of ACR, AMR, and CAV (83, 88, 89). Notably, a link between LVAD implantation and the development of anti-AT1R has also been described making this antibody of particular relevance to the heart transplant population (82). Functionally, anti-AT1R can exert agonistic effects on the AT1 receptor, induced ERK kinase signaling in endothelial cells, and promoted vascular changes in a rat kidney transplant model (90) suggesting that a complement/Fc receptor independent mechanism may contribute to its pathogenic effects.
The possibility that non-HLA antibodies synergize with DSA to exert allograft damage is salient to the highly HLA sensitized transplant candidate (83, 91). Non-HLA antibodies may either be directed towards donor polymorphisms or be reactive to autoantigens. Zhang et al, found that transplant recipients who developed anti-MICA antibodies directed at donor polymorphisms (but not against non-donor polymorphisms) were at risk of AMR (84). In a separate study, pre-transplant anti-MICA was associated with inferior kidney allograft survival (92). Thus, in already high-risk HLA sensitized heart transplant candidates, assessment of the non-HLA antibody repertoire and careful donor selection might be warranted. Conversely, in the setting of autoreactive non-HLA antibodies, donor selection would be expected to have less relevance from this perspective.
An important question is whether contemporary desensitization strategies used for HLA antibodies are effective for non-HLA sensitization. This may depend in part on their mechanism of action and the relative importance of antibody concentration/titer to downstream effect. In kidney transplant recipients with malignant AT1R antibodies, plasmapheresis and IVIG, with the addition of an ARB improved allograft survival (90). In the small subset of patients studied, anti-AT1R became undetectable. Furthermore, in highly HLA sensitized heart transplant candidates, bortezomib was associated with a reduction in anti-AT1R (93). The success of using an ARB in conjunction with plasmapheresis/IVIG ± rituximab in anti-AT1R+ heart transplant recipients with allograft dysfunction is not clear. In a recent report, although symptoms were ameliorated, only a fraction of patients experiencing improvement in graft function (94). Further work is needed to establish how anti-AT1R and other non-HLA antibodies should be managed, particularly in highly HLA sensitized heart transplant candidates.
Current Approaches to Desensitization in Heart Transplantation
Antibody Reduction Strategies
Clinical
The use of IVIG to permit transplantation amongst sensitized transplant candidates was described in the early 1990s as an extension of its observed effects in autoimmune mediated disease (95–99). Today it continues to be included in up to 79% of heart transplant desensitization protocols making it the most commonly used treatment (4). While there have been no randomized controlled trials of IVIG for desensitization in heart transplantation, its efficacy in kidney transplant was formally addressed in the randomized, placebo-controlled IG02 trial (100). Monthly IVIG infusions (2g/kg) led to a modest but significant reduction in PRA and a trend towards an increase in transplantation, albeit after 6-months when the PRA had returned to near baseline.
In a study of 13 sensitized children awaiting heart transplantation, 77% of sensitized patients defined by C1q-single antigen bead (SAB) PRA >18% were successfully desensitized with IVIG and transplanted with 100% 1-year survival (101). Similarly, in 13 children with homograft repair, those receiving higher cumulative doses of IVIG were more likely to respond to treatment although longer follow-up time was a confounding factor (60). IVIG has also been used to desensitize LVAD recipients with newly elevated CDC PRAs after implant. Dowling et al. reported on 4 LVAD recipients that developed an elevated CDC PRA after LVAD implant. All four resolved their CDC PRA to baseline within 6 months of starting IVIG (102). Similarly, John et al. described a cohort of 26 heart transplant candidates supported on an LVAD who were treated with monthly IVIG and cyclophosphamide. Compared to untreated sensitized candidates (n=45) desensitization reduced the time to transplant and decreased the risk of post-transplant rejection down to that of non-sensitized recipients (103, 104). However, these observations should be considered in the context of the aforementioned points regarding LVAD-related HLA sensitization. Indeed, the magnitude of IVIG’s effect has been challenged with the use of the more sensitive SAB assay. Nair et al, described a modest reduction in mean MFI with interindividual variability in response (105). The cPRA was not significantly reduced. A similarly heterogeneous response was observed when both the microcytotoxicity assay (CDC PRA) and Luminex SAB were performed in a small cohort of kidney transplant candidates, albeit that the dose of IVIG was lower (106).
More commonly in heart transplantation, IVIG has been used in conjunction with plasmapheresis (PP). Pisani et al. used PP and IVIG immediately prior to transplant and suggested that outcomes were similar to those of a contemporary unsensitized cohort (107). Similarly, Leech et al. found that plasmapheresis, with low dose IVIG reduced the PRA in many but not all heart transplant candidates (108). Underlying etiology and magnitude of the HLA antibody response may contribute to this heterogeneity as all non-responders in this study were women. Contemporary studies of perioperative plasmapheresis with IVIG also suggest that post-transplant rejection is common, likely because this approach does not address the underlying immune response (9, 109, 110).
Plasmapheresis has also been used as an adjunct to plasma cell therapies (discussed below) with the concept that antibody removal may decrease negative feedback inhibition on plasma cells enhancing protein production thereby sensitizing them to proteasome inhibition (PI). This effect remains to be formally evaluated but recent studies challenge this view. In an iterative trial of PI with and without intermittent plasmapheresis, both strategies led to a similar reduction in bone marrow plasma cells and circulating immunodominant HLA antibodies (10). Our preliminary work has also recently questioned the need for plasmapheresis during desensitization (12). Given i) the increased risk for infection in an already tenuous patient population, ii) challenges managing periprocedural anticoagulation, and hence iii) need for inpatient treatment, contemporary strategies may forgo plasmapheresis. Nonetheless, in very highly sensitized patients, when crossing DSA, its use in the peri-transplant setting may still be warranted.
Mechanistic Considerations
Multiple immunomodulatory effects have been proposed including the neutralization and enhanced elimination of pathogenic antibodies, inhibition of downstream complement activation, and direct inhibitory effects on the cellular immune response (reviewed in (97, 98, 111, 112)). From a desensitization perspective, early work proposed that IVIG effectively neutralized the pathogenic effects of anti-HLA antibodies through antiidiotypic antibodies and potentially complement inhibition (95, 113–116). The presence of anti-idiotypic antibodies to autoantibodies in IVIG preparations has been described (117). In a similar manner, Tyan et al, found that IVIG effectively inhibited cytotoxicity in vitro, which was attributed to the Fab2’ portion of anti-idiotypic antibodies (114, 115). It was further proposed that IVIG may stimulate endogenous production of anti-idiotypic antibodies with potential protective effects (118). While this may explain the observed reduction in CDC PRA, it has also been attributed to complement inhibition. Either way, this could explain the reduction in CDC PRA seen in at least some patients but would have lesser effect on antibody titer.
IVIG may also enhance the elimination of pathogenic antibodies by saturating endogenous Fc neonatal receptors (FcRns) (119–121). Under physiological conditions, endogenous FcRns rescue endocytosed immunoglobulin, recycling it to the cell surface thereby preventing degradation and extending its half-life in the serum. When FcRns become saturated due to supraphysiological levels of IgG, immunoglobulin is targeted for lysosomal degradation thereby reducing its half-life in the serum. Since FcRn can mediate IgG recycling at a rate 42% higher than production (122), this pathway is critical for maintaining protective immunity but may also drive the persistence of pathogenic antibodies. FcRn-/- mice eliminate IgG markedly faster than wild type, an effect which has been capitalized on to study the use of exogenous IVIG in the treatment of autoimmune disease. In an experimental model of bullous pemphigus, FcRn-deficient mice were resistant to disease and had lower levels of pathogenic IgG, an effect that could be recapitulated in disease-prone wild-type mice by the administration of high-dose IVIG (123). In time course studies, Bleeker et al, used a monoclonal antibody (mAb) to trace the effect of single-dose IVIG on antibody elimination (124). In mice this resulted in a 40% reduction with maximal effect at 3 days. Extrapolating this model to humans, a 25% reduction in autoantibody was predicted with maximal effects at 3-4 weeks although differences between species make interpretation difficult (121). Importantly, it should be noted that enhanced Ig elimination has ramifications for treatment regimens that incorporate mAb therapies with high-dose IVIG. Total dose of mAbs may be reduced or augmented depending on their Fc properties. Thus, until strategic, rigorously tested multidrug regimens are designed to specifically capitalize on these properties, administration of IVIG should be temporally separated from that of mAbs by at least 2 weeks (125).
While IVIG and therapeutic modulation of its pathway may reduce pathological antibodies in the circulation, the underlying immune response is largely unaffected. In patients treated with IVIG and plasmapheresis followed by splenectomy at the time of transplant, histological analysis suggested that immune cell composition was undisturbed (110). Moreover, examination of paired bone marrow samples obtained before and after treatment with plasmapheresis, IVIG, and anti-thymocyte globulin found no significant effect of treatment on the number of alloantibody secreting cells (109).
New Antibody Targeted Therapies for Highly Sensitized Transplant Candidates
Taking advantage of a potent bacterial immune evasion strategy, the IgG-degrading enzyme derived from Streptococcus pyogenes (IdeS) has been used for perioperative desensitization in kidney transplant recipients (126). IdeS hydrolizes IgG into the Fab2’ fragment and Fc portion thus effectively preventing antibody dependent cellular cytotoxicity (ADCC) and complement activation. Twenty-five kidney transplant candidates received IdeS 4-6 hours before transplant. All received lymphodepletion (Atgam® or alemtuzumab). Some patients also received IVIG and rituximab. As expected, all circulating IgG was cleaved within 6 hours and remained inactivated for 1-2 weeks after which IgG levels increased. DSA rebound was especially notable in the absence of adjunctive B cell therapies. However, patients who received rituximab/IVIG as adjunctive therapy nonetheless had inflammation on 6-month protocol biopsies. Furthermore, infectious complications were common, and would be anticipated to be even higher/more serious amongst heart transplant recipients many of whom are supported on a LVAD as bridge to transplant. A more ‘tempered’ approach could be to enhance elimination of pathogenic antibodies by blocking FcRN, a strategy being actively studied in the setting of autoimmunity (127–130). IgG concentrations (but not IgA or IgM) are reduced by ~50% but interestingly, tetanus and influenza A reactive antibodies were preserved (127). The implications for HLA antibodies are currently being investigated. If proven, FcRN inhibition with adjunctive therapies to modulate the underlying immune response (discussed below) could reduce HLA antibodies, preserve protective immunity, and be used to suppress DSA until cellular donor reactivity is controlled.
An alternative approach is to block the downstream effects of pathogenic HLA antibodies. The complement inhibitor eculizumab has been studied in kidney transplant recipients and more recently in heart transplantation with favorable short-term outcomes (131, 132). The strength of this approach is that it permits transplantation across DSA and a positive flow-crossmatch without hyperacute rejection. However, long-term outcomes remain suboptimal. Amongst 30 sensitized kidney transplant recipients with a positive FCXM treated with eculizumab at the time of transplant, 56.7% had evidence of chronic AMR on 5-year protocol biopsy (133). Death-censored allograft survival was similar to FCXM+ controls both of which were reduced compared to those with a negative FCXM. Because this approach does not target the underlying immune response, >50% of patients in this cohort remained FCXM+ at 1-year post-transplant, albeit that the channel shift was reduced. Whether eculizumab can be used in conjunction with approaches that better target the underlying alloimmune response requires further study.
Plasma Cell Therapies
Clinical
Proteasome inhibitors (PIs) induce apoptosis in response to the accumulation of misfolded proteins. The exceptionally high rate of immunoglobulin synthesis by antibody secreting cells (ASCs) underlies their susceptibility to PI-based therapies and their efficacy in treating plasma cell dyscrasias (134, 135). Bortezomib is a first-generation PI that reversibly binds the 20S proteasome. Patel et al. were amongst the first to describe its use in 7 highly sensitized heart transplant candidates all except one of whom had undergone attempted desensitization with other therapies (7 to 177 days prior) (136). Four doses of Bortezomib with corticosteroids were administered, each immediately following 2 sessions of plasmapheresis which was used in an attempt to stimulate protein synthesis by PCs. Treatment led to a marked reduction in cPRA from 62% to 35% (p=0.01) although this was determined 1-2 weeks following treatment making it difficult to differentiate between the effect of plasmapheresis, bortezomib, and prior cycles of treatment. Five patients were transplanted, 4 without evidence of rejection. One died early post-transplant in the setting of graft dysfunction and sepsis. Notably however, only one patient was transplanted against a moderate level DSA. These preliminary results were to be further tested in a multicenter, randomized-controlled trial (CTOT-13). However, this was terminated due to inadequate enrollment highlighting the challenges of these nonetheless important clinical trials. Larger studies in kidney transplant candidates suggest a modest response to bortezomib. Woodle and colleagues used an iterative study design to investigate the use of a bortezomib based protocol (with rituximab and plasmapheresis) (137). In this cohort, 43.2% of patients were successfully desensitized and transplanted, all with a negative flow crossmatch and undetectable DSA. An encouraging reduction in immunodominant antibody was observed, especially when more frequent/higher density dosing was used (8 doses). Yet the response for very high MFI antibodies was less robust. Using a cPRA MFI cut off of 8000, only 50% of patients were defined as responders and this decreased to 38.3% when the CDC PRA was used. Thus, while promising, when extrapolating to the very highly sensitized cohort, the approach remains somewhat limited. In a separate cohort of kidney transplant candidates, 32 doses of bortezomib monotherapy did not significantly reduce cPRA despite a modest reduction in unacceptable antigens (138). Whether the addition of plasmapheresis and rituximab would have improved outcomes, as was used in the study by Woodle et al, is unknown. Either way, the regimen was poorly tolerated with only 50% of candidates receiving the full treatment course without dose reduction.
More recently carfilzomib, a second-generation proteasome inhibitor that binds irreversibly to the 20S proteasome has been used for desensitization. Its superior efficacy to bortezomib for the treatment of multiple myeloma and reduced incidence of peripheral neuropathy make it an attractive alternative (139, 140). In a recent report, 9 treatment naïve heart transplant candidates underwent desensitization with plasmapheresis and carfilzomib 20mg/m2 followed by 2g/kg of IVIG (141). IgG-cPRA decreased from 76% to 40% (p=0.01) immediately after the last dose of carfilzomib (day 16). Six patients were transplanted, 5 across previously moderate MFI DSA which responded to desensitization (mean MFI pre/post desensitization 5360 and 2012 respectively). All patients received thymoglobulin induction. There was only 1 documented rejection during a median follow up of 35.1 months. This occurred in the patient with a C1q-PRA of 54% suggesting yet again a limitation for more highly sensitized candidates. Carfilzomib was studied in a cohort of kidney transplant candidates divided into two groups (10). A major strength of the study design was that it allowed for the effect of carfilzomib alone to be determined and directly compared to a protocol using pre-carfilzomib plasmapheresis. Both groups received 2 cycles with each cycle consisting of 6 doses of carfilzomib up to 36mg/m2. Group A received 3 plasmapheresis sessions after the last dose of carfilzomib while Group B had an additional plasmapheresis session added each week prior to carfilzomib. Median maximal immunodominant antibody reduction was 72.8% (Group 1, 69.8%; Group B, 80.1%) with no significant difference between treatment groups (p=0.698). Notably, this included an assessment at day 45 (before both groups received post-carfilzomib plasmapheresis) therefore demonstrating the direct effect of PI on reducing circulating HLA antibodies in the absence of plasmapheresis (Group A). However, antibody rebound was observed within 30 days of completing treatment underscoring the need for adjunctive therapies to sustain the response.
Both bortezomib and carfilzomib carry distinct, but not insignificant side-effect profiles, the former being associated with peripheral neuropathy while the latter, based on the myeloma literature, carries a theoretical risk of cardiotoxicity (140, 142). The magnitude of risk in heart transplant candidates is unknown and must be balanced with the potential benefits given the ultimate goal. Other complications include reversible acute kidney injury, thrombocytopenia, gastrointestinal side effects, and infection (143).
Collectively, these studies highlight the potential of plasma cell directed therapies as part of desensitization regimens, but this remains limited by i) inadequate response, particularly in very highly sensitized candidates, ii) antibody rebound/PI resistance, and iii) their side-effect profile.
Mechanistic Considerations
Bone marrow CD138+ plasma cells (PCs) secrete antibodies with specificities that mirror those in the peripheral blood underscoring their contribution to HLA sensitization (23). Bortezomib and carfilzomib reduce CD138+ bone marrow plasma cells (BMPCs) by ≥ 50%, including the HLA reactive repertoire in sensitized patients (23, 144, 145). PIs disrupt the balance between protein synthesis and destruction leading to accumulation of misfolded proteins, activation of the unfolded protein response (UPR), and apoptosis. In a mouse model of lupus, bortezomib reduces both short and long-lived BMPCs which correlated with the activation of the unfolded protein response (UPR) (146). Similar activation of the UPR has been observed in BMPCs from patients undergoing desensitization with carfilzomib (23). Nonetheless, the response is incomplete and accompanied by brisk rebound following treatment completion (10). Several factors may contribute. Firstly, some PCs may be particularly resistant to PIs owing to their tightly regulated and highly protective bone marrow niche. Indeed, protective immunity appears to be only partially affected with persistence of adequate, albeit somewhat reduced titers of tetanus and measles antibodies (145, 147, 148). Whether CD19-CD38hiCD138+ long-lived plasma cells (LLPCs), which harbor specificities for protective viral immunity also contribute to HLA specificities requires further study (149). Secondly, some PCs may develop PI resistance, potentially through β5 subunit mutations and/or upregulation of components of the immunoproteasome (23, 150). Thirdly, whether PI-induced apoptosis enhances the secretory rate of remaining PCs to maintain equilibrium remain to be established. Importantly, homeostatic proliferation in germinal centers (GCs) is thought to drive repopulation and has been elegantly demonstrated in a non-human primate model of AMR (151). This highlights the critical contribution of memory B cells, which can enter the GC leading to robust recall responses. Taken together, these observations argue for coordinated combinatorial therapies targeting not only the PC compartment but also GCs and memory B cells.
New Plasma Cell Therapies With Potential for Desensitization
Daratumumab is a mAb targeting CD38 which is highly expressed on the surface of plasma cells. Its success in treating myeloma (152) and more recently AL amyloidosis (153), has raised interest in its use for desensitization/AMR (154, 155). Notably, CD38 is also expressed on NK cells, which may provide additional benefit, particularly in the setting of AMR and chronic rejection (35, 36). Doberer et al. described a case of combined smoldering myeloma and DSA+ chronic active AMR treated with daratumumab (156). This report is notable for the extensive analysis of the peripheral blood, bone marrow, and allograft tissue. Consistent with the established mechanism of daratumumab, blood and bone marrow PCs were effectively depleted. This included DSA secreting BMPCs and was paralleled by the elimination of circulating DSA. NK cells were also reduced in the peripheral blood and allograft. Importantly, this was accompanied by a reduction in the molecular AMR score and microcirculatory inflammation. Similar resolution of AMR was described in another case (157). However, in contrast, class II DSA did not appear to be reduced by daratumumab. The authors suggested NK cell depletion as the mechanism underlying this apparently divergent response. While cautious enthusiasm is merited, a concern is that CD38 is also expressed on multiple suppressor cell lineages including IL-10 secreting Bregs, a subset of CD4+CD25+CD127lo Tregs with particularly potent suppressive capabilities, and myeloid-derived suppressor cells (158). In myeloma patients, this was accompanied by augmented CD8+ T effector memory responses with enhanced IFNγ secretion in response to viral antigens (158). Given the potential role of heterologous immunity and/or bystander T-cells in rejection, this latter finding is also noteworthy (28, 159). Interestingly, both of the aforementioned cases showed signs of TCMR on follow up biopsies consistent with the findings in a non-human primate model (155–157). Finally, as with PIs, daratumumab is unlikely to abolish upstream GC reactions and thus, antibody rebound may occur. Nonetheless, daratumumab, potentially in combination with additional immunomodulatory therapies may provide benefit in the pre-transplant setting. Proof-of-concept has been described with partial response in a heart transplant candidate allowing for transplantation across two previously unacceptable antigens (155). A pilot study in highly sensitized heart transplant candidates is awaited (NCT04088903).
The inability to adequately deplete the bone marrow compartment of HLA secreting PCs suggests that their tightly regulated microenvironments (i.e. ‘bone marrow niche’) may be a barrier to direct PC targeted therapies. Plerixafor inhibits CXCR4-CXCL12 interactions between PCs and bone marrow stromal cells, with the potential to release them from their niche thus raising the possibility that it could enhance PI mediated effects (160). This question is being addressed in kidney transplant desensitization with preliminary results showing promise (161, 162). Other possible approaches of interest include cytokine modulation and metabolic regulation. These have been reviewed elsewhere (160).
B-Cell Therapies
Clinical
The brisk rebound after plasma cell directed therapies emphasizes the need to target B cell memory. To date, the most commonly used B cell therapy in heart transplantation is rituximab, a chimeric murine/human monoclonal IgG1 antibody directed against CD20, expressed on mature B cells. Near complete depletion of peripheral blood B cells is achieved through a combination of antibody-dependent cellular cytotoxicity (ADCC), complement-dependent cytotoxicity, and apoptosis (163).
While there are no randomized controlled trials in heart transplant desensitization, evidence from the kidney literature suggests that rituximab 1) has a modest, albeit heterogenous effect on the CDC PRA and T-cell flow crossmatch, 2) may be more effective than IVIG alone at preventing severe AMR/graft loss, and 3) has some effect on rebound but this is often incomplete (164). In an early clinical trial, Vo et al. investigated the benefit of adding rituximab (1g given on days 7 and 22) to an IVIG based desensitization protocol. This approach reduced the mean CDC PRA by 33%. However, like IG02, the PRA remained above 40% and confidence intervals were wide, consistent with a heterogeneous response (mean CDC T-cell PRA 77 ± 18% before desensitization, 44 ± 30% after desensitization) (165). Furthermore, 50% of the patients transplanted had a rejection episode, 31% of which had evidence of C4d staining suggesting that constraint of the amnestic response is incomplete. Nonetheless, the addition of rituximab may have some benefit over IVIG at tempering rebound. A trial comparing IVIG alone to IVIG + rituximab was stopped early due to AMR and 2 graft losses, both of which occurred in the placebo arm (166).
In heart transplantation, Patel et al. described the successful desensitization of 4 sensitized heart transplant candidates with rituximab and IVIG (167). The same group later reported long-term outcomes in 21 heart transplant recipients treated with IVIG and plasmapheresis, 5 of whom also received rituximab (168). Desensitization led to a reduction in antibody, albeit with a heterogeneous response, and patients were transplanted with a negative CDC crossmatch. Five-year survival and freedom from CAV were comparable to non-sensitized recipients. However, this study only considered patients who were successfully transplanted and therefore does evaluate treatment effect per se. Further small single-center experience in pediatric heart transplantation has also been cautiously favourable (169, 170). Schumacher et al, described a cohort of 14 heart transplant candidates of whom 8 were classified as responders and 5 were transplanted (170). Treatment with IVIG and rituximab increased the donor pool from 10% to 85% (range 2-100%) amongst responders. Rituximab was continued post-transplant with good short-term outcomes. However, despite cautious optimism based on these select, non-randomized reports, clinical experience parallels the heterogeneous and often inadequate response observed in the kidney RCTs.
Several other clinical observations are noteworthy. Firstly, as seen in CTOT-11, a trial of rituximab in non-sensitized heart transplant recipients, rituximab does not appear to prevent de novo DSA, a finding which has also been described in kidney transplantation (171, 172). Secondly, non-selective B cell depletion also affects B cells with regulatory/tolerogenic properties. This has been raised as a potential explanation for the increase in percent atheromatous volume (PAV) in CTOT-11, as well as the higher rate of cellular rejection in at least one rituximab study in kidney transplantation (171, 172). More recently, the ratio of IL-10/TNFα expressed by transitional B cells (T1B, CD24+++CD38+++) was found to predict T-cell mediated rejection in kidney transplant recipients (173). Perhaps most importantly, as further discussed below, B cell depletion in lymph nodes, spleen, and bone marrow is incomplete. Therefore, there is persistent potential for ongoing GC and extrafollicular antibody responses, as well as antigen presentation to T cells.
Mechanistic Considerations
While the CD20 antigen is widely expressed on most B cell subsets, it is absent on cells at both extremes of the B cell lineage, namely B cell precursors and antibody secreting plasmablasts/plasma cells. With respect to the former, this implies that B cell repopulation will follow depletion, as is typically seen within 12 months post-infusion (174). In terms of the latter, because bone marrow plasma cells can survive decades in protective bone marrow microenvironments, removing precursor B cells will not address HLA secreting PCs persisting in these reservoirs.
However, beyond this, rituximab incompletely eliminates B cells, and in particular, CD27+ memory B cells (Bmem), from the bone marrow, spleen, and lymph nodes (110, 175–178). Ramos and colleagues determined the effect of desensitization on splenic histology and cellular composition in five splenectomized patients who had undergone recent desensitization with rituximab/IVIG/plasmapheresis (110). Despite depletion of CD20+ and CD79+ B cells, CD27+ Bmem persisted. Similarly, CD27+IgD- switched Bmem persisted in lymph nodes from patients with rheumatoid arthritis (176). In contrast, naïve and unswitched Bmem were reduced. Clinically this is consistent with the robust recall response to influenza vaccination following rituximab monotherapy despite the near absence of circulating influenza specific Bmem in peripheral blood (179). Similarly, amongst sensitized kidney transplant recipients treated with rituximab, 34 of 39 HLA antibodies that increased after transplant were associated with epitopes shared with previous allografts or pregnancy (164).
An important implication is the persistence of lymphoid germinal center (GC)-like structures in patients treated with rituximab (180). Histological assessment is further supported by the failure to suppress LN IL-21 mRNA transcription, a surrogate for Tfh activity (74). Consistent with this, in a humanized CD20+ transgenic mouse model, GC B cells were most resistant to rituximab (181). Together, the persistence of Bmem, GCs, and LLPCs underly the limited ability of rituximab to adequately suppress established HLA antibody responses.
New B cell Targeting Strategies
Inebilizumab is a CD19-directed antibody recently approved for use in patients with neuromyelitis optica spectrum disorder (NMOSD) (182). Because CD19 is expressed on a subset of plasma cells, inebilizumab is an attractive target for desensitization which is being investigated in sensitized kidney transplant candidates (NCT04174677). An alternative approach is to modulate the factors required for B cell survival. To this end, BAFF (anti-B cell activating factor of the tumor necrosis alpha family) modulation has been attempted for desensitization without significant benefit. More recently, the BAFF inhibitor belimumab had modest benefit in preventing proteasome inhibitor induced rebound (183). Targeting survival factors and cytokines may be of benefit when used as part of a multidrug regimen but this requires further study.
Costimulation Blockade Disrupts the Germinal Center and Suppresses the DSA Response
Thus far, the therapeutic strategies discussed focus on eliminating antibodies or the cells responsible for their production. The T-dependent nature of the anti-HLA antibody response suggests T cell- B cell interactions as a rationale therapeutic target. The critical players include CD28:CD80/86, CD40:CD40L, and ICOS : ICOSL and the ability to block these interactions is collectively referred to as costimulation blockade (184). The major clinical target to date has been the CD28:CD80/86 pathway. This has been achieved using the fusion protein CTLA4-Ig (abatacept) and its high affinity variant belatacept (185). CTLA4 binds to CD80/86 thereby preventing CD28 mediated signaling critical for T cell activation (signal 2). This underlies its rationale as maintenance immunosuppression in kidney transplantation with the theoretical advantage of inducing anergy in an alloantigen specific manner when T-cell receptor signaling is left undisturbed (signal 1 in the absence of signal 2). Importantly, in pre-clinical non-human primate studies, belatacept potently suppressed the primary antibody response following sheep red blood cell immunization (185) suggesting a potential advantage over contemporary T-cell focused immunosuppression. Clinically this translated into a strikingly low incidence of de novo DSA in phase 3 clinical trials (186).
The ability to control established immune responses with costimulation blockade has been addressed in mouse and non-human primate models which we have recently translated to the clinical setting amongst some of the most highly sensitized heart transplant candidates (cPRA>99%). Early work in mouse models from Anita Chong’s lab demonstrated that CTLA4-Ig could inhibit the memory B cell response, collapse ongoing GC B cell reactions, and halt the rise in alloantibody following heart transplantation (24, 187). While post-GC plasma cells were less well controlled, the addition of bortezomib to a continuous CTLA4-Ig regimen, but not bortezomib alone, led to sustained alloantibody suppression which mirrored the clinical response in a small cohort of kidney transplant recipients (188). Parallel studies, in non-human primates performed by Kwun/Knechtle and colleagues demonstrated that belatacept could abrogate the potent GC homeostatic proliferation associated with PI administration (25). This ‘dual targeting’ approach, tested in a rigorous model of allosensitization, decreased bone marrow plasma cells, lymph node isotype switched B cells and T follicular helper cells resulting in a reduction in DSA before transplant and improved post-transplant survival (148, 189). Moreover, a direct effect on BMPCs has been proposed given the expression of CD28 on a subset of long-lived plasma cells (190). Building on this work, we recently reported on the combination of belatacept with sequential cycles of PI therapy in four extremely highly sensitized heart transplant candidates (12). Our studies revealed a marked reduction in both class I and II HLA antibodies, including those with high MFI that were C1q positive and successful transplantation with a negative CDC crossmatch in three cases. Preliminary findings suggest that the reduction in many HLA antibodies may be sustained with ongoing monthly belatacept infusions. This is bolstered by observations in kidney transplant recipients where, compared to calcineurin inhibitors, belatacept more effectively constrained pre-existing DSA and led to a modest reduction in non-DSA (191, 192). Taken together, this highlights the relevance of rationally designed strategies that integrate clinical observations, with hypothesis driven findings, that have been specifically tested in model systems.
Using Il-6 Inhibition to Modulate the Immune Response in Highly Sensitized Transplant Candidates
A conceivable alternative to direct immune cell targeting is to modulate the cytokine milieu. The theoretical advantage is that multiple levels of the immune response can be simultaneously targeted. Given its pleiotropic nature, IL-6 is an attractive cytokine that is important for both the innate and adaptive immune response. IL-6 supports Th1 and Th2 proliferation, promotes proinflammatory Th17 lineage commitment at the expense of Tregs, contributes to Tfh development, sustains B cell survival, and favors plasmablast differentiation (193). Inhibition of IL-6 can be achieved either by blocking the IL-6 receptor (tocilizumab) or direct neutralization (clazakizumab). Both have been used in kidney transplantation for desensitization and AMR. Tocilizumab is also being studied in non-highly sensitized heart transplant recipients early post-transplant to establish whether it can reduce the incidence of rejection, de novo DSA, re-transplantation and death at 1-year post transplant (NCT03644667).
Tocilizumab has been used for desensitization in two distinct cohorts of kidney transplant recipients. Vo et al. reported their experience with tocilizumab + IVIG in a phase I/II desensitization study (194). All patients had failed treatment with IVIG and rituximab. Five of 10 patients were transplanted with a reduction in their immunodominant DSA. However, the pre-treatment MFIs were <10,000 suggesting a potential limitation for patients with high titer antibodies. In a separate study of 13 highly sensitized (mean cPRA>97%) kidney transplant candidates, a more muted response was observed (195). Twelve patients had at least 1 prior transplant and in 92% the prior allograft remained in situ (off immunosuppression). Efficacy assessment was evaluated based on the immunodominant MFI, which in most cases was >10,000. In this cohort, tocilizumab monotherapy had only a marginal effect on HLA antibodies as assessed by MFI with dilutions used when prozone was detected. While an increase in naïve B cells and decrease in plasmablasts was observed, tocilizumab did not significantly change the percentage of pre/post-germinal center B cells, Tfh, and Tfh subsets between baseline and 6 months and there was no significant augmentation of CD3+CD4+CD127-FoxP3+ Tregs. Nonetheless, Treg augmentation with tocilizumab has been reported by others (196, 197). Subsequently, Vo et al, described the use of clazakizumab in combination with plasma exchange and IVIG for desensitization (198). A reduction in class I and II HLA antibody MFI was observed although there was substantial variability in response between patients. Alemtuzumab was used for induction along with standard immunosuppression and clazakizumab was continued post-transplant. Notably, only 1 patient had detectable DSA at 12 months raising the possibility that adjunctive post-transplant IL-6 blockade may be of benefit.
A potential role for IL-6 inhibition has also been described in the setting of AMR. Choi et al, reported on a cohort of 36 kidney transplant recipients with cAMR treated with tocilizumab after failing standard therapies (199). A significant reduction in DSA, C4d deposition, glomerulitis and peritubular capillaritis scores was observed. Graft survival was 80% at 6-years which is higher than that reported in the literature without treatment although a control arm was not included. A subsequent study randomized 20 patients with DSA positive late AMR to clazakizumab vs. placebo followed by an open label extension where all participants received study drug (200). Clazakizumab reduced DSA with improvements in intragraft AMR associated findings in some patients during the extended follow up period. Early attenuation of eGFR decline was observed in the clazakizumab arm compared with placebo which was no longer apparent when the placebo arm was transitioned to clazakizumab. Serious infection (n=5) and diverticular disease with complications (n=2) were noted suggesting the need for careful pre-treatment evaluation and close monitoring.
Collectively, these studies suggest that IL-6 inhibition may be of benefit to the allosensitized transplant patient both before and after transplant. The full potential of IL-6 inhibition together with adjunctive strategies for the highly sensitized candidate remains to be determined. Conceptually, synergism between IL-6 inhibition and costimulation blockade could augment GC inhibition while counterbalancing the potential reduction in Tregs. Other combinations are also possible and future studies, perhaps driven by a translational approach, should be considered.
Considering Cell-Based Therapies for the Allosensitized Heart Transplant Candidate
A potential alternative or complementary strategy to the ones described thus far is to modulate the immune response using cell-based therapies such as mesenchymal stromal cells (MSCs) or regulatory immune cells (e.g. Tregs, dendritic cells, macrophages). This approach remains largely speculative for desensitization and a full discussion of the topic is beyond the scope of this review. However, several pre-clinical and conceptual considerations are noteworthy. MSCs have been shown to inhibit alloantibody production in vitro (201), reduce DSA in a rat model of transfusion associated allosensitization (202), and increase rejection-free survival in a high-risk corneal transplant model (203). Clinical translation was reported in three HLA sensitized patients (204) although cautious interpretation is warranted given the need to address potential risks of MSC-based therapy. Firstly, the use of allogeneic MSCs has been associated with the development of low-level DSA to the MSC and/or shared kidney-HLA (205). This was prevented when repeat mismatches (MSC and allograft) were avoided (206). Secondly, MSCs have been associated with transient kidney graft dysfunction when given in the immediate post-transplant period (207). This was attributed to early post-transplant inflammation resulting in MSC recruitment to the allograft where they potentiated the proinflammatory response. MSC administration prior to transplant may prevent this. Nonetheless, the possible implications for the heart transplant recipient are noteworthy given that hemodynamic compromise can be fatal. Thirdly, the risk of opportunistic infection and malignancy requires ongoing evaluation (208). Nonetheless, in addition to the aforementioned humoral alloimmune effects, MSCs may modulate multiple layers of the immune response enhancing T-cell suppression, augmenting transitional/regulatory B cells, modulating dendritic cells and macrophage activity (reviewed in (208, 209)). The potential to use 3rd party MSCs in the pre-transplant setting would significantly enhance feasibility in heart transplantation where the timing of transplant is not known a priori.
An alternative strategy is to use Tregs to suppress the anti-donor response, ideally in an antigen specific manner. This could theoretically target alloreactive effector T cells while leaving infectious immunity intact. Safety has been preliminarily established in phase I/II trials including those in the multicenter ONE Study (210, 211). However, these trials were done in low-to-intermediate risk subjects with the goal of demonstrating safety and/or permitting immunosuppression minimization. Less is known about the effect on humoral alloimmunity and more saliently, the relevance to pre-transplant allosensitization. In a mouse model of AMR, induced Tregs reduced DSA and allograft infiltration/damage in some, but not all animals (212). More recently, Sicard et al, investigated the effect of donor specific CAR-Tregs (dsCAR-Tregs) on the humoral response in a mouse allogeneic skin transplant model (213). Compared to controls, dsCAR-Tregs reduced the number of DSA secreting B cells, decreased the amount of de novo DSA, and prolonged survival. In contrast, when mice were sensitized prior to transplant, dsCAR-Tregs were neither able to constrain the humoral alloimmune response nor prolong survival suggesting that this approach may not be as effective for sensitized transplant candidates. Whether combining Tregs with adjunctive strategies such as B cell depletion would be of benefit, requires further study. However, practical issues (such as the unpredictability of timing in heart transplantation) and safety implications (including the stability of Treg phenotype) are important to consider in this setting.
Discussion: Challenges and Future Directions
In this review the literature relevant to heart transplant desensitization has been summarized, supported by finding at the cellular level in human studies, and objective rationale derived from model systems. Interpretation is limited by 1) a paucity of standardized clinical trials in heart transplantation, 2) ethical limitations in assigning a control arm, 3) immense variability in the etiology and ‘degree’ of sensitization, 4) lack of standardization across HLA labs, and 5) deficits in our understanding of the factors driving memory B cell and plasma cell differentiation, persistence, and resistance to desensitization. Given that humoral sensitization rates have doubled over the past 2 decades (4) and that highly sensitized candidates are more likely to die waiting for transplant or become too sick (3), developing safe and effective desensitization strategies is an urgent priority.
One aspect that has as yet not been addressed in this review is the sensitization ‘cut-off’ meriting desensitization. This remains a challenging issue. Using cPRA based on MFI can be problematic. Not only is MFI an inadequate surrogate for antibody titer, but the choice of MFI cut-off will determine whether the candidate is desensitized thus introducing heterogeneity. Attesting to the variability in practice, in a recent international survey, 21% of centers used cPRA>80% and 21% used cPRA>50% as their cut off. The remainder described using a range of cut-offs from >10% to >90% (4). The next important consideration is whether the desensitization strategy will alter long-term outcomes. Approaches that have the potential to modulate the underlying donor reactive immune phenotype and/or repertoire, as discussed in this review, may be of benefit even when sensitization is more modest. This has yet to be formally evaluated. However, at present, the most commonly used approaches for desensitization are plasmapheresis, IVIG, and rituximab (4). Neither alone nor in combination are these likely to suffice, particularly in the very highly sensitized candidate and the potential for durable effects is modest.
Developing a prioritization policy, as has been implemented in Canada (9), may be of value but is limited by the lack of standardization across HLA labs in the US, differences in what constitutes an unacceptable antigen, and difficulties defining an optimal cut-off. Furthermore, this approach may foster hesitancy to pursue robust immunomodulatory desensitization strategies with the potential to improve long-term outcomes. Whether some patients, perhaps those who are more advanced in age, may be better served by destination LVAD is another consideration. However, this is not an acceptable option in many cases where heart transplantation remains the gold-standard. The focus should therefore be on designing multidrug regimens, driven by drug repurposing, and developed in conjunction with findings from pre-clinical models. While the present review has put forth several strategies with potential to target each layer contributing to the immune response (Figure 2), other approaches are forthcoming. Improvements in our understanding of HLA reactive memory B cells and plasma cell characteristics is critical to defining who will derive the greatest benefit from targeted therapies. Post-transplant immunosuppression with better control of B cell memory, ideally in an antigen specific manner, will also be required. Long-term benefit will likely be derived from strategies that modulate multiple layers of the immune response. Despite inroads being made, much remains to be done to ensure equal access to heart transplantation for the highly sensitized patient with end-stage heart disease. While desensitization continues to be a ‘niche’ field, the growing incidence in an already high-risk population along with the poor prognosis for those denied transplant underscores the urgent need for attention.
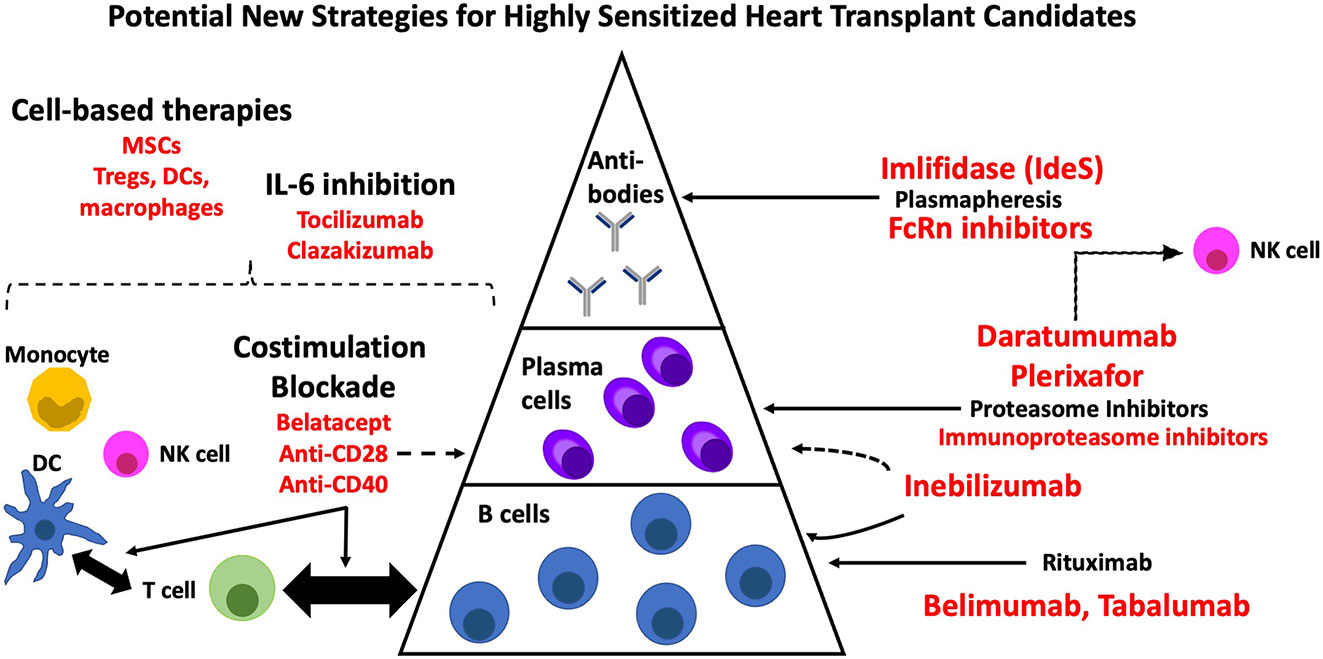
Figure 2 New therapies with potential for desensitization in heart transplantation. DC, dendritic cell; MSC, mesenchymal stromal cell; NK, natural killer cell.
Author Contributions
The author confirms being the sole contributor of this work and has approved it for publication.
Funding
Mendez National Institute of Transplantation Foundation (PG012633).
Conflict of Interest
The author declares that the research was conducted in the absence of any commercial or financial relationships that could be construed as a potential conflict of interest.
Publisher’s Note
All claims expressed in this article are solely those of the authors and do not necessarily represent those of their affiliated organizations, or those of the publisher, the editors and the reviewers. Any product that may be evaluated in this article, or claim that may be made by its manufacturer, is not guaranteed or endorsed by the publisher.
Acknowledgments
Thanks are due to Dr. Anita S. Chong for many insightful discussions on desensitization and to Dr. Adriana Zeevi for continued expert advice on HLA interpretation.
Abbreviations
ACR, Acute Cellular Rejection; ADCC, Antibody-Dependent Cellular Cytotoxicity; AMR, Antibody Mediated Rejection; AT1R - angiotensin II type 1 receptor; Bmem, Memory B cell; BMPC, Bone Marrow Plasma Cell; CDC, Complement Dependent Cytotoxicity; CHD, Congenital Heart Disease; cPRA, calculated Panel Reactive Antibody; CTLA4, Cytotoxic T-Lymphocyte-Associated Protein 4; DSA, Donor Specific Antibody; FcRN, Neonatal Fc Receptor; FCXM, Flow cytometric crossmatch; GC, Germinal Center; HLA, Human Leukocyte Antigen; IdeS - IgG-degrading enzyme derived from Streptococcus pyogenes; IVIG, Intravenous Immunoglobulin; LLPC, Long Lived Plasma Cell; LN, Lymph Node; LVAD, Left Ventricular Assist Device; MCS, Mechanical Circulatory Support; MFI, Mean Fluorescence Intensity; MICA, Major Histocompatibility Complex Class I Chain-Related Molecule A; PI, Proteasome Inhibitor; PPCM, Peripartum Cardiomyopathy; PRA, Panel Reactive Antibody; SAB, Single Antigen Bead; TCR, T-cell Receptor; UPR, Unfolded Protein Response.
References
1. Colvin MM, Cook JL, Chang PP, Hsu DT, Kiernan MS, Kobashigawa JA, et al. Sensitization in Heart Transplantation: Emerging Knowledge: A Scientific Statement From the American Heart Association. Circulation (2019) 139(12):e553–78. doi: 10.1161/CIR.0000000000000598
2. Nikolova AP, Kobashigawa JA. Donor-Specific Antibodies in Heart Transplantation: Can We Afford the Price or Is It Too Steep to Pay? Curr Opin Organ Transplant (2020) 25(6):555–62. doi: 10.1097/MOT.0000000000000818
3. Kransdorf EP, Kittleson MM, Patel JK, Pando MJ, Steidley DE, Kobashigawa JA. Calculated Panel-Reactive Antibody Predicts Outcomes on the Heart Transplant Waiting List. J Heart Lung Transplant (2017) 36(7):787–96. doi: 10.1016/j.healun.2017.02.015
4. Kobashigawa J, Colvin M, Potena L, Dragun D, Crespo-Leiro MG, Delgado JF, et al. The Management of Antibodies in Heart Transplantation: An ISHLT Consensus Document. J Heart Lung Transplant (2018) 37(5):537–47. doi: 10.1016/j.healun.2018.01.1291
5. Chiu P, Schaffer JM, Oyer PE, Pham M, Banerjee D, Joseph Woo Y, et al. Influence of Durable Mechanical Circulatory Support and Allosensitization on Mortality After Heart Transplantation. J Heart Lung Transplant (2016) 35(6):731–42. doi: 10.1016/j.healun.2015.12.023
6. Nwakanma LU, Williams JA, Weiss ES, Russell SD, Baumgartner WA, Conte JV. Influence of Pretransplant Panel-Reactive Antibody on Outcomes in 8,160 Heart Transplant Recipients in Recent Era. Ann Thorac Surge (2007) 84(5):1556–62; discussion 1562-1553. doi: 10.1016/j.athoracsur.2007.05.095
7. Shaddy RE, Hunter DD, Osborn KA, Lambert LM, Minich LL, Hawkins JA, et al. Prospective Analysis of HLA Immunogenicity of Cryopreserved Valved Allografts Used in Pediatric Heart Surgery. Circulation (1996) 94(5):1063–7. doi: 10.1161/01.CIR.94.5.1063
8. Organ Procurement and Transplantation Network (OPTN). Policy 6. Allocation of Heart and Heart Lungs. pp. 90–106. Available at: https://optn.transplant.hrsa.gov/media/1200/optn_policies.pdf. Accessed August 6th, 2021.
9. Clarke B, Ducharme A, Giannetti N, Kim D, McDonald M, Pflugfelder P, et al. Multicenter Evaluation of a National Organ Sharing Policy for Highly Sensitized Patients Listed for Heart Transplantation in Canada. J Heart Lung Transplant (2017) 36(5):491–8. doi: 10.1016/j.healun.2017.01.003
10. Tremblay S, Driscoll JJ, Rike-Shields A, Hildeman DA, Alloway RR, Girnita AL, et al. A Prospective, Iterative, Adaptive Trial of Carfilzomib-Based Desensitization. Am J Transplant (2020) 20(2):411–21. doi: 10.1111/ajt.15613
11. Ho TP, Jignesh K, Nishihara K, Kittleson MM, Chang DH, Kransdorf EP, et al. Treatment of Highly Sensitized Patients Before Heart Transplant Associated With Long-Term Post-Transplant Benefit. Circulation (2020) 142:A13046. doi: 10.1161/circ.142.suppl_3.13046
12. Alishetti S, Farr M, Jennings D, Serban G, Uriel N, Sayer G, et al. Desensitizing Highly Sensitized Heart Transplant Candidates With the Combination of Belatacept and Proteasome Inhibition. Am J Transplant (2020) 20(12):3620–30. doi: 10.1111/ajt.16113
13. Chong AS. Mechanisms of Organ Transplant Injury Mediated by B Cells and Antibodies: Implications for Antibody-Mediated Rejection. Am J Transplant (2020) 20(Suppl 4):23–32. doi: 10.1111/ajt.15844
14. Lucia M, Luque S, Crespo E, Melilli E, Cruzado JM, Martorell J, et al. Preformed Circulating HLA-Specific Memory B Cells Predict High Risk of Humoral Rejection in Kidney Transplantation. Kidney Int (2015) 88(4):874–87. doi: 10.1038/ki.2015.205
15. Luque S, Lucia M, Melilli E, Lefaucheur C, Crespo M, Loupy A, et al. Value of Monitoring Circulating Donor-Reactive Memory B Cells to Characterize Antibody-Mediated Rejection After Kidney Transplantation. Am J Transplant (2019) 19(2):368–80. doi: 10.1111/ajt.15055
16. Chong AS. B Cells as Antigen-Presenting Cells in Transplantation Rejection and Tolerance. Cell Immunol (2020) 349:104061. doi: 10.1016/j.cellimm.2020.104061
17. Karahan GE, Claas FHJ, Heidt S. Pre-Existing Alloreactive T and B Cells and Their Possible Relevance for Pre-Transplant Risk Estimation in Kidney Transplant Recipients. Front Med (Lausanne) (2020) 7:340. doi: 10.3389/fmed.2020.00340
18. Akkaya M, Kwak K, Pierce SK. B Cell Memory: Building Two Walls of Protection Against Pathogens. Nat Rev Immunol (2020) 20(4):229–38. doi: 10.1038/s41577-019-0244-2
19. Cyster JG, Allen CDC. B Cell Responses: Cell Interaction Dynamics and Decisions. Cell (2019) 177(3):524–40. doi: 10.1016/j.cell.2019.03.016
20. Cyster JG, Dang EV, Reboldi A, Yi T. 25-Hydroxycholesterols in Innate and Adaptive Immunity. Nat Rev Immunol (2014) 14(11):731–43. doi: 10.1038/nri3755
21. Shinnakasu R, Inoue T, Kometani K, Moriyama S, Adachi Y, Nakayama M, et al. Regulated Selection of Germinal-Center Cells Into the Memory B Cell Compartment. Nat Immunol (2016) 17(7):861–9. doi: 10.1038/ni.3460
22. Sundling C, Lau AWY, Bourne K, Young C, Laurianto C, Hermes JR, et al. Positive Selection of IgG(+) Over IgM(+) B Cells in the Germinal Center Reaction. Immunity (2021) 54(5):988–1001.e5. doi: 10.1016/j.immuni.2021.03.013
23. Woodle ES, Tremblay S, Brailey P, Girnita A, Alloway RR, Aronow B, et al. Proteasomal Adaptations Underlying Carfilzomib-Resistance in Human Bone Marrow Plasma Cells. Am J Transplant (2020) 20(2):399–410. doi: 10.1111/ajt.15634
24. Chen J, Yin H, Xu J, Wang Q, Edelblum KL, Sciammas R, et al. Reversing Endogenous Alloreactive B Cell GC Responses With Anti-CD154 or CTLA-4ig. Am J Transplant (2013) 13(9):2280–92. doi: 10.1111/ajt.12350
25. Kim EJ, Kwun J, Gibby AC, Gibby JJ, Gibby AB 3rd, Iwakoshi NN, et al. Costimulation Blockade Alters Germinal Center Responses and Prevents Antibody-Mediated Rejection. Am J Transplant (2014) 14(1):59–69. doi: 10.1111/ajt.12526
26. Potena L, Bontadini A, Iannelli S, Fruet F, Leone O, Barberini F, et al. Occurrence of Fatal and Nonfatal Adverse Outcomes After Heart Transplantation in Patients With Pretransplant Noncytotoxic HLA Antibodies. J Transplant (2013) 2013:519680. doi: 10.1155/2013/519680
27. Itescu S, Tung TC, Burke EM, Weinberg A, Moazami N, Artrip JH, et al. Preformed IgG Antibodies Against Major Histocompatibility Complex Class II Antigens Are Major Risk Factors for High-Grade Cellular Rejection in Recipients of Heart Transplantation. Circulation (1998) 98(8):786–93. doi: 10.1161/01.CIR.98.8.786
28. Adams AB, Williams MA, Jones TR, Shirasugi N, Durham MM, Kaech SM, et al. Heterologous Immunity Provides a Potent Barrier to Transplantation Tolerance. J Clin Invest (2003) 111(12):1887–95. doi: 10.1172/JCI200317477
29. Selin LK, Brehm MA, Naumov YN, Cornberg M, Kim SK, Clute SC, et al. Memory of Mice and Men: CD8+ T-Cell Cross-Reactivity and Heterologous Immunity. Immunol Rev (2006) 211:164–81. doi: 10.1111/j.0105-2896.2006.00394.x
30. Wiebe C, Kosmoliaptsis V, Pochinco D, Gibson IW, Ho J, Birk PE, et al. HLA-DR/DQ Molecular Mismatch: A Prognostic Biomarker for Primary Alloimmunity. Am J Transplant (2019) 19(6):1708–19. doi: 10.1111/ajt.15177
31. Lechler R, Ng WF, Steinman RM. Dendritic Cells in Transplantation–Friend or Foe? Immunity (2001) 14(4):357–68. doi: 10.1016/S1074-7613(01)00116-9
32. Alegre ML, Lakkis FG, Morelli AE. Antigen Presentation in Transplantation. Trends Immunol (2016) 37(12):831–43. doi: 10.1016/j.it.2016.09.003
33. Siu JHY, Surendrakumar V, Richards JA, Pettigrew GJ. T Cell Allorecognition Pathways in Solid Organ Transplantation. Front Immunol (2018) 9:2548. doi: 10.3389/fimmu.2018.02548
34. Ordikhani F, Pothula V, Sanchez-Tarjuelo R, Jordan S, Ochando J. Macrophages in Organ Transplantation. Front Immunol (2020) 11:582939. doi: 10.3389/fimmu.2020.582939
35. Loupy A, Duong Van Huyen JP, Hidalgo L, Racapé M, Aubert O, Venner JM, et al. Gene Expression Profiling for the Identification and Classification of Antibody-Mediated Heart Rejection. Circulation (2017) 135(10):917–35. doi: 10.1161/CIRCULATIONAHA.116.022907
36. Koenig A, Chen CC, Marcais A, Barba T, Mathias V, Sicard A, et al. Missing Self Triggers NK Cell-Mediated Chronic Vascular Rejection of Solid Organ Transplants. Nat Commun (2019) 10(1):5350. doi: 10.1038/s41467-019-13113-5
37. Adenugba A. NK Cells in Transplantation. Transplantation (2017) 101(10):2262–4. doi: 10.1097/TP.0000000000001914
38. Tambur AR, Campbell P, Chong AS, Feng S, Ford ML, Gebel H, et al. Sensitization in Transplantation: Assessment of Risk (STAR) 2019 Working Group Meeting Report. Am J Transplant (2020) 20(10):2652–68. doi: 10.1111/ajt.15937
39. Tambur AR, Campbell P, Claas FH, Feng S, Gebel HM, Jackson AM, et al. Sensitization in Transplantation: Assessment of Risk (STAR) 2017 Working Group Meeting Report. Am J Transplant (2018) 18(7):1604–14. doi: 10.1111/ajt.14752
40. Bestard O, Cravedi P. Monitoring Alloimmune Response in Kidney Transplantation. J Nephrol (2017) 30(2):187–200. doi: 10.1007/s40620-016-0320-7
41. Liwski RS, Gebel HM. Of Cells and Microparticles: Assets and Liabilities of HLA Antibody Detection. Transplantation (2018) 102(1S Suppl 1):S1–6. doi: 10.1097/TP.0000000000001818
42. Chen G, Sequeira F, Tyan DB. Novel C1q Assay Reveals a Clinically Relevant Subset of Human Leukocyte Antigen Antibodies Independent of Immunoglobulin G Strength on Single Antigen Beads. Hum Immunol (2011) 72(10):849–58. doi: 10.1016/j.humimm.2011.07.001
43. Chen G, Tyan DB. C1q Assay for the Detection of Complement Fixing Antibody to HLA Antigens. Methods Mol Biol (Clifton NJ) (2013) 1034:305–11. doi: 10.1007/978-1-62703-493-7_16
44. Mangiola M, Marrari M, Qingyong X, Sanchez PG, Zeevi A. Approaching the Sensitized Lung Patient: Risk Assessment for Donor Acceptance. J Thoracic Dis (2021). doi: 10.21037/jtd-2021-21
45. Morris H, DeWolf S, Robins H, Sprangers B, LoCascio SA, Shonts BA, et al. Tracking Donor-Reactive T Cells: Evidence for Clonal Deletion in Tolerant Kidney Transplant Patients. Sci Transl Med (2015) 7(272):272ra210. doi: 10.1126/scitranslmed.3010760
46. DeWolf S, Grinshpun B, Savage T, Lau SP, Obradovic A, Shonts B, et al. Quantifying Size and Diversity of the Human T Cell Alloresponse. JCI Insight (2018) 3(15). doi: 10.1172/jci.insight.121256
47. DeWolf S, Sykes M. Alloimmune T Cells in Transplantation. J Clin Invest (2017) 127(7):2473–81. doi: 10.1172/JCI90595
48. Chong AS, Rothstein DM, Safa K, Riella LV. Outstanding Questions in Transplantation: B Cells, Alloantibodies, and Humoral Rejection. Am J Transplant (2019) 19(8):2155–63. doi: 10.1111/ajt.15323
49. Habal MV, Farr M, Restaino S, Chong A. Desensitization in the Era of Precision Medicine: Moving From the Bench to Bedside. Transplantation (2019) 103(8):1574–81. doi: 10.1097/TP.0000000000002737
50. Khairy P, Ionescu-Ittu R, Mackie AS, Abrahamowicz M, Pilote L, Marelli AJ. Changing Mortality in Congenital Heart Disease. J Am Coll Cardiol (2010) 56(14):1149–57. doi: 10.1016/j.jacc.2010.03.085
51. Marelli AJ, Mackie AS, Ionescu-Ittu R, Rahme E, Pilote L. Congenital Heart Disease in the General Population: Changing Prevalence and Age Distribution. Circulation (2007) 115(2):163–72. doi: 10.1161/CIRCULATIONAHA.106.627224
52. Crumb SR, Cook SC, Cheatham JP, Galantowicz M, Feltes TF, Phillips A, et al. Quality Outcomes of ACHD Patients Undergoing Cardiovascular Procedures and Hospital Admissions in a Free-Standing Children’s Hospital. Int J Cardiol (2011) 146(3):326–9. doi: 10.1016/j.ijcard.2009.07.007
53. Khush KK, Cherikh WS, Chambers DC, Goldfarb S, Hayes D Jr, Kucheryavaya AY, et al. The International Thoracic Organ Transplant Registry of the International Society for Heart and Lung Transplantation: Thirty-Fifth Adult Heart Transplantation Report-2018; Focus Theme: Multiorgan Transplantation. J Heart Lung Transplant (2018) 37(10):1155–68. doi: 10.1016/j.healun.2018.07.022
54. Ross HJ, Law Y, Book WM, Broberg CS, Burchill L, Cecchin F, et al. Transplantation and Mechanical Circulatory Support in Congenital Heart Disease: A Scientific Statement From the American Heart Association. Circulation (2016) 133(8):802–20. doi: 10.1161/CIR.0000000000000353
55. Breinholt JP 3rd, Hawkins JA, Lambert LM, Fuller TC, Profaizer T, Shaddy RE. A Prospective Analysis of the Immunogenicity of Cryopreserved Nonvalved Allografts Used in Pediatric Heart Surgery. Circulation (2000) 102(19 Suppl 3):III179–182. doi: 10.1161/01.CIR.102.suppl_3.III-179
56. Hooper DK, Hawkins JA, Fuller TC, Profaizer T, Shaddy RE. Panel-Reactive Antibodies Late After Allograft Implantation in Children. Ann Thoracic Surge (2005) 79(2):641–4. discussion 645. doi: 10.1016/j.athoracsur.2004.07.052
57. Hoekstra F, Witvliet M, Knoop C, Akkersdijk G, Jutte N, Bogers A, et al. Donor-Specific Anti-Human Leukocyte Antigen Class I Antibodies After Implantation of Cardiac Valve Allografts. J Heart Lung Transplant (1997) 16(5):570–2.
58. Meyer SR, Campbell PM, Rutledge JM, Halpin AM, Hawkins LE, Lakey JR, et al. Use of an Allograft Patch in Repair of Hypoplastic Left Heart Syndrome may Complicate Future Transplantation. Eur J Cardiothor Surg (2005) 27(4):554–60. doi: 10.1016/j.ejcts.2004.12.033
59. Colvin M, Smith JM, Hadley N, Skeans MA, Uccellini K, Lehman R, et al. OPTN/SRTR 2017 Annual Data Report: Heart. Am J Transplant (2019) 19(Suppl 2):323–403. doi: 10.1111/ajt.15278
60. Edwards JJ, Seliktar N, White R, Heron SD, Lin K, Rossano J, et al. Impact and Predictors of Positive Response to Desensitization in Pediatric Heart Transplant Candidates. J Heart Lung Transplant (2019) 38(11):1206–13. doi: 10.1016/j.healun.2019.08.018
61. Castleberry C, Ryan TD, Chin C. Transplantation in the Highly Sensitized Pediatric Patient. Circulation (2014) 129(22):2313–9. doi: 10.1161/CIRCULATIONAHA.113.001378
62. Stergiopoulos K, Lima FV. Peripartum Cardiomyopathy-Diagnosis, Management, and Long Term Implications. Trends Cardiovasc Med (2019) 29(3):164–73. doi: 10.1016/j.tcm.2018.07.012
63. Sliwa K, Hilfiker-Kleiner D, Petrie MC, Mebazaa A, Pieske B, Buchmann E, et al. Current State of Knowledge on Aetiology, Diagnosis, Management, and Therapy of Peripartum Cardiomyopathy: A Position Statement From the Heart Failure Association of the European Society of Cardiology Working Group on Peripartum Cardiomyopathy. Eur J Heart Fail (2010) 12(8):767–78. doi: 10.1093/eurjhf/hfq120
64. Brar SS, Khan SS, Sandhu GK, Jorgensen MB, Parikh N, Hsu JW, et al. Incidence, Mortality, and Racial Differences in Peripartum Cardiomyopathy. Am J Cardiol (2007) 100(2):302–4. doi: 10.1016/j.amjcard.2007.02.092
65. Fett JD, Christie LG, Carraway RD, Murphy JG. Five-Year Prospective Study of the Incidence and Prognosis of Peripartum Cardiomyopathy at a Single Institution. Mayo Clin Proc (2005) 80(12):1602–6. doi: 10.4065/80.12.1602
66. Desai D, Moodley J, Naidoo D. Peripartum Cardiomyopathy: Experiences at King Edward VIII Hospital, Durban, South Africa and a Review of the Literature. Trop Doct (1995) 25(3):118–23. doi: 10.1177/004947559502500310
67. Isogai T, Kamiya CA. Worldwide Incidence of Peripartum Cardiomyopathy and Overall Maternal Mortality. Int Heart J (2019) 60(3):503–11. doi: 10.1536/ihj.18-729
68. Rasmusson K, Brunisholz K, Budge D, Horne BD, Alharethi R, Folsom J, et al. Peripartum Cardiomyopathy: Post-Transplant Outcomes From the United Network for Organ Sharing Database. J Heart Lung Transplant (2012) 31(2):180–6. doi: 10.1016/j.healun.2011.11.018
69. Kinder JM, Stelzer IA, Arck PC, Way SS. Immunological Implications of Pregnancy-Induced Microchimerism. Nat Rev Immunol (2017) 17(8):483–94. doi: 10.1038/nri.2017.38
70. Suah AN, Tran DV, Khiew SH, Andrade MS, Pollard JM, Jain D, et al. Pregnancy-Induced Humoral Sensitization Overrides T Cell Tolerance to Fetus-Matched Allografts in Mice. J Clin Invest (2021) 131(1):e140715. doi: 10.1172/JCI140715
71. Khush KK, Cherikh WS, Chambers DC, Harhay MO, Hayes D Jr, Hsich E, et al. The International Thoracic Organ Transplant Registry of the International Society for Heart and Lung Transplantation: Thirty-Sixth Adult Heart Transplantation Report - 2019; Focus Theme: Donor and Recipient Size Match. J Heart Lung Transplant (2019) 38(10):1056–66. doi: 10.1016/j.healun.2019.08.004
72. Arnaoutakis GJ, George TJ, Kilic A, Weiss ES, Russell SD, Conte JV, et al. Effect of Sensitization in US Heart Transplant Recipients Bridged With a Ventricular Assist Device: Update in a Modern Cohort. J Thorac Cardiovasc Surg (2011) 142(5):1236–45, 1245 e1231. doi: 10.1016/j.jtcvs.2011.07.019
73. Shankar N, Daly R, Geske J, Kushwaha SK, Timmons M, Joyce L, et al. LVAD Implant as a Bridge to Heart Transplantation Is Associated With Allosensitization as Measured by Single Antigen Bead Assay. Transplantation (2013) 96(3):324–30. doi: 10.1097/TP.0b013e3182985371
74. Jain R, Habal MV, Clerkin KJ, Latif F, Restaino SW, Zorn E, et al. De Novo Human Leukocyte Antigen Allosensitization in Heartmate 3 Versus Heartmate II Left Ventricular Assist Device Recipients. ASAIO J (2021). doi: 10.1097/MAT.0000000000001451
75. Ko BS, Drakos S, Kfoury AG, Hurst D, Stoddard GJ, Willis CA, et al. Immunologic Effects of Continuous-Flow Left Ventricular Assist Devices Before and After Heart Transplant. J Heart Lung Transplant (2016) 35(8):1024–30. doi: 10.1016/j.healun.2016.05.001
76. Itescu S, Ankersmit JH, Kocher AA, Schuster MD. Immunobiology of Left Ventricular Assist Devices. Prog Cardiovasc Dis (2000) 43(1):67–80. doi: 10.1053/pcad.2000.7191
77. Ankersmit HJ, Tugulea S, Spanier T, Weinberg AD, Artrip JH, Burke EM, et al. Activation-Induced T-Cell Death and Immune Dysfunction After Implantation of Left-Ventricular Assist Device. Lancet (1999) 354(9178):550–5. doi: 10.1016/S0140-6736(98)10359-8
78. Itescu S, John R. Interactions Between the Recipient Immune System and the Left Ventricular Assist Device Surface: Immunological and Clinical Implications. Ann Thoracic Surge (2003) 75(6 Suppl):S58–65. doi: 10.1016/S0003-4975(03)00480-6
79. Grosman-Rimon L, Ajrawat P, Lioe J, Tumiati LC, Rao V, Billia F, et al. Increases in Serum Autoantibodies After Left Ventricular Assist Device Implantation. J Card Fail (2019) 25(4):301–6. doi: 10.1016/j.cardfail.2019.01.002
80. See SB, Clerkin KJ, Kennel PJ, Zhang F, Weber MP, Rogers KJ, et al. Ventricular Assist Device Elicits Serum Natural IgG That Correlates With the Development of Primary Graft Dysfunction Following Heart Transplantation. J Heart Lung Transplant (2017) 36(8):862–70. doi: 10.1016/j.healun.2017.03.018
81. Barten MJ, Dragun D, Dieterlen M-T, Klein S, von Salisch S, Garbade J. Occurrence of Non-HLA Antibodies Against MICA, AT1R and ETAR After Heart Transplantation Is Associated With Cardiac Allograft Vasculopathy: 1745. Transplantation (2012) 94(10S):152. doi: 10.1097/00007890-201211271-00282
82. Chau VQ, Flattery M, Nicholson KS, Mcdougan F, Gupta G, Uber P, et al. Elevated AT1R Antibody and Morbidity in Patients Bridged to Heart Transplant Using Continuous Flow Left Ventricular Assist Devices. J Card Fail (2020) 26(11):959–67. doi: 10.1016/j.cardfail.2020.06.010
83. Reinsmoen NL, Lai CH, Mirocha J, Cao K, Ong G, Naim M, et al. Increased Negative Impact of Donor HLA-Specific Together With Non-HLA-Specific Antibodies on Graft Outcome. Transplantation (2014) 97(5):595–601. doi: 10.1097/01.TP.0000436927.08026.a8
84. Zhang Q, Cecka JM, Gjertson DW, Ge P, Rose ML, Patel JK, et al. HLA and MICA: Targets of Antibody-Mediated Rejection in Heart Transplantation. Transplantation (2011) 91(10):1153–8. doi: 10.1097/TP.0b013e3182157d60
85. Nath DS, Ilias Basha H, Tiriveedhi V, Alur C, Phelan D, Ewald GA, et al. Characterization of Immune Responses to Cardiac Self-Antigens Myosin and Vimentin in Human Cardiac Allograft Recipients With Antibody-Mediated Rejection and Cardiac Allograft Vasculopathy. J Heart Lung Transplant (2010) 29(11):1277–85. doi: 10.1016/j.healun.2010.05.025
86. Zhang X, Reinsmoen NL. Impact of Non-Human Leukocyte Antigen-Specific Antibodies in Kidney and Heart Transplantation. Front Immunol (2017) 8:434. doi: 10.3389/fimmu.2017.00434
87. Starling RC, Stehlik J, Baran DA, Armstrong B, Stone JR, Ikle D, et al. Multicenter Analysis of Immune Biomarkers and Heart Transplant Outcomes: Results of the Clinical Trials in Organ Transplantation-05 Study. Am J Transplant (2016) 16(1):121–36. doi: 10.1111/ajt.13422
88. Hiemann NE, Meyer R, Wellnhofer E, Schoenemann C, Heidecke H, Lachmann N, et al. Non-HLA Antibodies Targeting Vascular Receptors Enhance Alloimmune Response and Microvasculopathy After Heart Transplantation. Transplantation (2012) 94(9):919–24. doi: 10.1097/TP.0b013e3182692ad2
89. Urban M, Slavcev A, Gazdic T, Ivak P, Besik J, Netuka I. The Impact of Angiotensin II Type 1 Receptor Antibodies on Post-Heart Transplantation Outcome in Heart Mate II Bridged Recipients. Interact Cardiovasc Thorac Surg (2016) 22(3):292–7. doi: 10.1093/icvts/ivv344
90. Dragun D, Muller DN, Brasen JH, Fritsche L, Nieminen-Kelhä M, Dechend R, et al. Angiotensin II Type 1-Receptor Activating Antibodies in Renal-Allograft Rejection. N Engl J Med (2005) 352(6):558–69. doi: 10.1056/NEJMoa035717
91. Zhang Q, Reed EF. The Importance of Non-HLA Antibodies in Transplantation. Nat Rev Nephrol (2016) 12(8):484–95. doi: 10.1038/nrneph.2016.88
92. Zou Y, Stastny P, Susal C, Dohler B, Opelz G. Antibodies Against MICA Antigens and Kidney-Transplant Rejection. N Engl J Med (2007) 357(13):1293–300. doi: 10.1056/NEJMoa067160
93. Zhang X, Patel J, Kobashigawa J. Effect of Bortezomib Desensitization Therapy on Levels of AT1R Antibody. J Heart Lung Transplant (2019) 38(4):S278. doi: 10.1016/j.healun.2019.01.694
94. Moreno J, Verma A, Kopecky B, Dehner C, Kostelecky N, Schilling J, et al. Angiotensin II Type 1 Receptor Antibody Mediated Rejection Following Orthotopci Heart Transplant: A Singel Center Experience. J Heart Lung Transplant (2020) 39(4):S30. doi: 10.1016/j.healun.2020.01.1176
95. Ronda N, Kaveri SV, Kazatchkine MD. Treatment of Autoimmune Diseases With Normal Immunoglobulin Through Manipulation of the Idiotypic Network. Clin Exp Immunol (1993) 93(Suppl 1):14–5. doi: 10.1111/j.1365-2249.1993.tb06218.x
96. Bain PG, Motomura M, Newsom-Davis J, Misbah SA, Chapel HM, Lee ML, et al. Effects of Intravenous Immunoglobulin on Muscle Weakness and Calcium-Channel Autoantibodies in the Lambert-Eaton Myasthenic Syndrome. Neurology (1996) 47(3):678–83. doi: 10.1212/WNL.47.3.678
97. Gelfand EW. Intravenous Immune Globulin in Autoimmune and Inflammatory Diseases. N Engl J Med (2012) 367(21):2015–25. doi: 10.1056/NEJMra1009433
98. Jordan SC, Toyoda M, Kahwaji J, Vo AA. Clinical Aspects of Intravenous Immunoglobulin Use in Solid Organ Transplant Recipients. Am J Transplant (2011) 11(2):196–202. doi: 10.1111/j.1600-6143.2010.03400.x
99. Jordan SC, Tyan D, Czer L, Toyoda M. Immunomodulatory Actions of Intravenous Immunoglobulin (IVIG): Potential Applications in Solid Organ Transplant Recipients. Pediatr Transplant (1998) 2(2):92–105.
100. Jordan SC, Tyan D, Stablein D, McIntosh M, Rose S, Vo A, et al. Evaluation of Intravenous Immunoglobulin as an Agent to Lower Allosensitization and Improve Transplantation in Highly Sensitized Adult Patients With End-Stage Renal Disease: Report of the NIH IG02 Trial. J Am Soc Nephrol (2004) 15(12):3256–62. doi: 10.1097/01.ASN.0000145878.92906.9F
101. Lal AT,D, Chin C. IVIG TO Desensitize Sensitized Pediatric Heart Transplant Candidates as Measured by a Novel C1q SAB Assay. Am J Transplant (2012) 12(S1):392.
102. Dowling RD, Jones JW, Carroll MS, Gray LA Jr. Use of Intravenous Immunoglobulin in Sensitized LVAD Recipients. Transplant Proc (1998) 30(4):1110–1. doi: 10.1016/S0041-1345(98)00172-9
103. John R, Lietz K, Burke E, Ankersmit J, Mancini D, Suciu-Foca N, et al. Intravenous Immunoglobulin Reduces Anti-HLA Alloreactivity and Shortens Waiting Time to Cardiac Transplantation in Highly Sensitized Left Ventricular Assist Device Recipients. Circulation (1999) 100(19 Suppl):II229–235.doi: 10.1161/01.CIR.100.suppl_2.II-229
104. John R, Lietz K, Schuster M, Naka Y, Rao V, Mancini DM, et al. Immunologic Sensitization in Recipients of Left Ventricular Assist Devices. J Thorac Cardiovasc Surg (2003) 125(3):578–91. doi: 10.1067/mtc.2003.30
105. Nair V, Sawinski D, Akalin E, Friedlander R, Ebcioglu Z, Sehgal V, et al. Effect of High-Dose Intravenous Immunoglobulin on Anti-HLA Antibodies in Sensitized Kidney Transplant Candidates. Clin Transplant (2012) 26(3):E261–268. doi: 10.1111/j.1399-0012.2012.01657.x
106. Ferrari-Lacraz S, Aubert V, Buhler L, Pascual M, Andresen I, Binet I, et al. Anti-HLA Antibody Repertoire After IVIg Infusion in Highly Sensitized Patients Waiting for Kidney Transplantation. Swiss Med Wkly (2006) 136(43-44):696–702.
107. Pisani BA, Mullen GM, Malinowska K, Lawless CE, Mendez J, Silver MA, et al. Plasmapheresis With Intravenous Immunoglobulin G Is Effective in Patients With Elevated Panel Reactive Antibody Prior to Cardiac Transplantation. J Heart Lung Transplant (1999) 18(7):701–6. doi: 10.1016/S1053-2498(99)00022-4
108. Leech SH, Lopez-Cepero M, LeFor WM, DiChiara L, Weston M, Furukawa S, et al. Management of the Sensitized Cardiac Recipient: The Use of Plasmapheresis and Intravenous Immunoglobulin. Clin Transplant (2006) 20(4):476–84. doi: 10.1111/j.1399-0012.2006.00509.x
109. Perry DK, Pollinger HS, Burns JM, Rea D, Ramos E, Platt JL, et al. Two Novel Assays of Alloantibody-Secreting Cells Demonstrating Resistance to Desensitization With IVIG and rATG. Am J Transplant (2008) 8(1):133–43. doi: 10.1111/j.1600-6143.2007.02039.x
110. Ramos EJ, Pollinger HS, Stegall MD, Gloor JM, Dogan A, Grande JP. The Effect of Desensitization Protocols on Human Splenic B-Cell Populations In Vivo. Am J Transplant (2007) 7(2):402–7. doi: 10.1111/j.1600-6143.2006.01632.x
111. Shehata N, Palda VA, Meyer RM, Blydt-Hansen TD, Campbell P, Cardella C, et al. The Use of Immunoglobulin Therapy for Patients Undergoing Solid Organ Transplantation: An Evidence-Based Practice Guideline. Transfus Med Rev (2010) 24(Suppl 1):S7–27. doi: 10.1016/j.tmrv.2009.09.010
112. Boros P, Gondolesi G, Bromberg JS. High Dose Intravenous Immunoglobulin Treatment: Mechanisms of Action. Liver Transpl (2005) 11(12):1469–80. doi: 10.1002/lt.20594
113. Rossi F, Kazatchkine MD. Antiidiotypes Against Autoantibodies in Pooled Normal Human Polyspecific Ig. J Immunol (1989) 143(12):4104–9.
114. Glotz D, Haymann JP, Sansonetti N, Francois A, Menoyo-Calonge V, Bariety J, et al. Suppression of HLA-Specific Alloantibodies by High-Dose Intravenous Immunoglobulins (IVIg). A Potential Tool for Transplantation of Immunized Patients. Transplantation (1993) 56(2):335–7. doi: 10.1097/00007890-199308000-00015
115. Tyan DB, Li VA, Czer L, Trento A, Jordan SC. Intravenous Immunoglobulin Suppression of HLA Alloantibody in Highly Sensitized Transplant Candidates and Transplantation With a Histoincompatible Organ. Transplantation (1994) 57(4):553–62. doi: 10.1097/00007890-199402270-00014
116. Magee JC, Collins BH, Harland RC, Lindman BJ, Bollinger RR, Frank MM, et al. Immunoglobulin Prevents Complement-Mediated Hyperacute Rejection in Swine-to-Primate Xenotransplantation. J Clin Invest (1995) 96(5):2404–12. doi: 10.1172/JCI118297
117. Kaveri SV, Dietrich G, Hurez V, Kazatchkine MD. Intravenous Immunoglobulins (IVIg) in the Treatment of Autoimmune Diseases. Clin Exp Immunol (1991) 86(2):192–8. doi: 10.1111/j.1365-2249.1991.tb05794.x
118. Reed E, Ho E, Cohen DJ, Ramey W, Marboe C, D'Agati V, et al. Anti-Idiotypic Antibodies Specific for HLA in Heart and Kidney Allograft Recipients. Immunol Res (1993) 12(1):1–11. doi: 10.1007/BF02918364
119. Gable KL, Guptill JT. Antagonism of the Neonatal Fc Receptor as an Emerging Treatment for Myasthenia Gravis. Front Immunol (2019) 10:3052. doi: 10.3389/fimmu.2019.03052
120. Brambell FW, Hemmings WA, Morris IG. A Theoretical Model of Gamma-Globulin Catabolism. Nature (1964) 203:1352–4. doi: 10.1038/2031352a0
121. Roopenian DC, Akilesh S. FcRn: The Neonatal Fc Receptor Comes of Age. Nat Rev Immunol (2007) 7(9):715–25. doi: 10.1038/nri2155
122. Kim J, Hayton WL, Robinson JM, Anderson CL. Kinetics of FcRn-Mediated Recycling of IgG and Albumin in Human: Pathophysiology and Therapeutic Implications Using a Simplified Mechanism-Based Model. Clin Immunol (2007) 122(2):146–55. doi: 10.1016/j.clim.2006.09.001
123. Li N, Zhao M, Hilario-Vargas J, Prisayanh P, Warren S, Diaz LA, et al. Complete FcRn Dependence for Intravenous Ig Therapy in Autoimmune Skin Blistering Diseases. J Clin Invest (2005) 115(12):3440–50. doi: 10.1172/JCI24394
124. Bleeker WK, Teeling JL, Hack CE. Accelerated Autoantibody Clearance by Intravenous Immunoglobulin Therapy: Studies in Experimental Models to Determine the Magnitude and Time Course of the Effect. Blood (2001) 98(10):3136–42.doi: 10.1182/blood.V98.10.3136
125. Jordan SC, Kucher K, Bagger M, Hockey HU, Wagner K, Ammerman N, et al. Intravenous Immunoglobulin Significantly Reduces Exposure of Concomitantly Administered Anti-C5 Monoclonal Antibody Tesidolumab. Am J Transplant (2020) 20(9):2581–8. doi: 10.1111/ajt.15922
126. Jordan SC, Lorant T, Choi J. IgG Endopeptidase in Highly Sensitized Patients Undergoing Transplantation. N Engl J Med (2017) 377(17):1693–4. doi: 10.1056/NEJMc1711335
127. Kiessling P, Lledo-Garcia R, Watanabe S, Langdon G, Tran D, Bari M, et al. The FcRn Inhibitor Rozanolixizumab Reduces Human Serum IgG Concentration: A Randomized Phase 1 Study. Sci Transl Med (2017) 9(414). doi: 10.1126/scitranslmed.aan1208
128. Robak T, Kazmierczak M, Jarque I, Musteata V, Treliński J, Cooper N, et al. Phase 2 Multiple-Dose Study of an FcRn Inhibitor, Rozanolixizumab, in Patients With Primary Immune Thrombocytopenia. Blood Adv (2020) 4(17):4136–46. doi: 10.1182/bloodadvances.2020002003
129. Howard JF Jr, Bril V, Burns TM, Mantegazza R, Bilinska M, Szczudlik A, et al. Randomized Phase 2 Study of FcRn Antagonist Efgartigimod in Generalized Myasthenia Gravis. Neurology (2019) 92(23):e2661–73. doi: 10.1212/WNL.0000000000007600
130. Bril V, Benatar M, Andersen H, Vissing J, Brock M, Greve B, et al. Efficacy and Safety of Rozanolixizumab in Moderate to Severe Generalized Myasthenia Gravis: A Phase 2 Randomized Control Trial. Neurology (2021) 96(6):e853–65. doi: 10.1212/WNL.0000000000011108
131. Marks WH, Mamode N, Montgomery RA, Stegall MD, Ratner LE, Cornell LD, et al. Safety and Efficacy of Eculizumab in the Prevention of Antibody-Mediated Rejection in Living-Donor Kidney Transplant Recipients Requiring Desensitization Therapy: A Randomized Trial. Am J Transplant (2019) 19(10):2876–88. doi: 10.1111/ajt.15364
132. Patel JK, Coutance G, Loupy A, Dilibero D, Hamilton M, Kittleson M, et al. Complement Inhibition for Prevention of Antibody-Mediated Rejection in Immunologically High-Risk Heart Allograft Recipients. Am J Transplant (2021) 21(7):2479–88. doi: 10.1111/ajt.16420
133. Schinstock CA, Bentall AJ, Smith BH, Cornell LD, Everly M, Gandhi MJ, et al. Long-Term Outcomes of Eculizumab-Treated Positive Crossmatch Recipients: Allograft Survival, Histologic Findings, and Natural History of the Donor-Specific Antibodies. Am J Transplant (2019) 19(6):1671–83. doi: 10.1111/ajt.15175
134. Salmon SE, Smith BA. Immunoglobulin Synthesis and Total Body Tumor Cell Number in IgG Multiple Myeloma. J Clin Invest (1970) 49(6):1114–21. doi: 10.1172/JCI106327
135. Eyer K, Doineau RCL, Castrillon CE, Briseño-Roa L, Menrath V, Mottet G, et al. Single-Cell Deep Phenotyping of IgG-Secreting Cells for High-Resolution Immune Monitoring. Nat Biotechnol (2017) 35(10):977–82. doi: 10.1038/nbt.3964
136. Patel J, Everly M, Chang D, Kittleson M, Reed E, Kobashigawa J. Reduction of Alloantibodies via Proteasome Inhibition in Cardiac Transplantation. J Heart Lung Transplant (2011) 30(12):1320–6. doi: 10.1016/j.healun.2011.08.009
137. Woodle ES, Shields AR, Ejaz NS, Sadaka B, Girnita A, Walsh RC, et al. Prospective Iterative Trial of Proteasome Inhibitor-Based Desensitization. Am J Transplant (2015) 15(1):101–18. doi: 10.1111/ajt.13050
138. Moreno Gonzales MA, Gandhi MJ, Schinstock CA, Moore NA, Smith BH, Braaten NY, et al. 32 Doses of Bortezomib for Desensitization Is Not Well Tolerated and Is Associated With Only Modest Reductions in Anti-HLA Antibody. Transplantation (2017) 101(6):1222–7. doi: 10.1097/TP.0000000000001330
139. Dimopoulos MA, Moreau P, Palumbo A, Joshua D, Pour L, Hájek R, et al. Carfilzomib and Dexamethasone Versus Bortezomib and Dexamethasone for Patients With Relapsed or Refractory Multiple Myeloma (ENDEAVOR): A Randomised, Phase 3, Open-Label, Multicentre Study. Lancet Oncol (2016) 17(1):27–38. doi: 10.1016/S1470-2045(15)00464-7
140. Martin TG. Peripheral Neuropathy Experience in Patients With Relapsed and/or Refractory Multiple Myeloma Treated With Carfilzomib. Oncol (Williston Park) (2013) 27(Suppl 3):4–10.
141. Sriwattanakomen R, Xu Q, Demehin M, Shullo MA, Mangiola M, Hickey GW, et al. Impact of Carfilzomib-Based Desensitization on Heart Transplantation of Sensitized Candidates. J Heart Lung Transplant (2021) 40(7):595–603. doi: 10.1016/j.healun.2021.03.001
142. Bishnoi R, Xie Z, Shah C, Bian J, Murthy HS, Wingard JR, et al. Real-World Experience of Carfilzomib-Associated Cardiovascular Adverse Events: SEER-Medicare Data Set Analysis. Cancer Med (2021) 10(1):70–8. doi: 10.1002/cam4.3568
143. KYPROLIS Highlights of Prescribing Information. Amgen. 1. Kyprolis [package insert]. U.S. Food and Drug Administration. website. Available at: https://www.accessdata.fda.gov/drugsatfda_docs/label/2012/202714lbl.pdf. Revised 07/12. Accessed August 6, 2021.
144. Perry DK, Burns JM, Pollinger HS, Amiot BP, Gloor JM, Gores GJ, et al. Proteasome Inhibition Causes Apoptosis of Normal Human Plasma Cells Preventing Alloantibody Production. Am J Transplant (2009) 9(1):201–9. doi: 10.1111/j.1600-6143.2008.02461.x
145. Alexander T, Sarfert R, Klotsche J, Kühl AA, Rubbert-Roth A, Lorenz HM, et al. The Proteasome Inhibitior Bortezomib Depletes Plasma Cells and Ameliorates Clinical Manifestations of Refractory Systemic Lupus Erythematosus. Ann Rheum Dis (2015) 74(7):1474–8. doi: 10.1136/annrheumdis-2014-206016
146. Neubert K, Meister S, Moser K, Weisel F, Maseda D, Amann K, et al. The Proteasome Inhibitor Bortezomib Depletes Plasma Cells and Protects Mice With Lupus-Like Disease From Nephritis. Nat Med (2008) 14(7):748–55. doi: 10.1038/nm1763
147. Burghuber CK, Manook M, Ezekian B, Gibby AC, Leopardi FV, Song M, et al. Dual Targeting: Combining Costimulation Blockade and Bortezomib to Permit Kidney Transplantation in Sensitized Recipients. Am J Transplant (2019) 19(3):724–36. doi: 10.1111/ajt.15067
148. Ezekian B, Schroder PM, Mulvihill MS, Barbas A, Collins B, Freischlag K, et al. Pretransplant Desensitization With Costimulation Blockade and Proteasome Inhibitor Reduces DSA and Delays Antibody-Mediated Rejection in Highly Sensitized Nonhuman Primate Kidney Transplant Recipients. J Am Soc Nephrol (2019) 30(12):2399–411. doi: 10.1681/ASN.2019030304
149. Halliley JL, Tipton CM, Liesveld J, Rosenberg AF, Darce J, Gregoretti IV, et al. Long-Lived Plasma Cells Are Contained Within the CD19(-)CD38(hi)CD138(+) Subset in Human Bone Marrow. Immunity (2015) 43(1):132–45. doi: 10.1016/j.immuni.2015.06.016
150. Franke NE, Niewerth D, Assaraf YG, van Meerloo J, Vojtekova K, van Zantwijk CH, et al. Impaired Bortezomib Binding to Mutant Beta5 Subunit of the Proteasome Is the Underlying Basis for Bortezomib Resistance in Leukemia Cells. Leukemia (2012) 26(4):757–68. doi: 10.1038/leu.2011.256
151. Kwun J, Burghuber C, Manook M, Iwakoshi N, Gibby A, Hong JJ, et al. Humoral Compensation After Bortezomib Treatment of Allosensitized Recipients. J Am Soc Nephrol (2017) 28(7):1991–6. doi: 10.1681/ASN.2016070727
152. Lokhorst HM, Plesner T, Laubach JP, Nahi H, Gimsing P, Hansson M, et al. Targeting CD38 With Daratumumab Monotherapy in Multiple Myeloma. N Engl J Med (2015) 373(13):1207–19. doi: 10.1056/NEJMoa1506348
153. Sanchorawala V, Sarosiek S, Schulman A, Mistark M, Migre ME, Cruz R, et al. Safety, Tolerability, and Response Rates of Daratumumab in Relapsed AL Amyloidosis: Results of a Phase 2 Study. Blood (2020) 135(18):1541–7. doi: 10.1182/blood.2019004436
154. Jordan SC, Ammerman N, Choi J, Huang E, Peng A, Sethi S, et al. The Role of Novel Therapeutic Approaches for Prevention of Allosensitization and Antibody-Mediated Rejection. Am J Transplant (2020) 20(Suppl 4):42–56. doi: 10.1111/ajt.15913
155. Kwun J, Matignon M, Manook M, Guendouz S, Audard V, Kheav D, et al. Daratumumab in Sensitized Kidney Transplantation: Potentials and Limitations of Experimental and Clinical Use. J Am Soc Nephrol (2019) 30(7):1206–19. doi: 10.1681/ASN.2018121254
156. Doberer K, Klager J, Gualdoni GA, Mayer KA, Eskandary F, Farkash EA, et al. CD38 Antibody Daratumumab for the Treatment of Chronic Active Antibody-Mediated Kidney Allograft Rejection. Transplantation (2021) 105(2):451–7. doi: 10.1097/TP.0000000000003247
157. Jordan S, Vescio R, Ammerman N, Toyoda M, Ge S, Chu M, et al. Daratumumab for Desensitization and Antibody Mediated Rejection Treatment in Highly-HLA Sensitized Patients. Am J Transplant (2020) 20(suppl 3).
158. Krejcik J, Casneuf T, Nijhof IS, Verbist B, Bald J, Plesner T, et al. Daratumumab Depletes CD38(+) Immune Regulatory Cells, Promotes T-Cell Expansion, and Skews T-Cell Repertoire in Multiple Myeloma. Blood (2016) 128(3):384–94. doi: 10.1182/blood-2015-12-687749
159. Habal MV, Miller AMI, Rao S, Lin S, Obradovic A, Khosravi-Maharlooei M, et al. T Cell Repertoire Analysis Suggests a Prominent Bystander Response in Human Cardiac Allograft Vasculopathy. Am J Transplant (2020) 21(4):1465–76. doi: 10.1111/ajt.16333
160. Woodle ES, Tremblay S, Rossi A, Rojas CC, Alloway R, Roskin K, et al. Plasma Cell Targeting to Prevent Antibody-Mediated Rejection. Am J Transplant (2020) 20(Suppl 4):33–41. doi: 10.1111/ajt.15889
161. Tremblay S, Castro-Rojas C, Driscoll J, Knechtle S, Shields A, Alloway R, et al. Pilot Study of Plasma Cell Niche-Targeted Therapy for Enhancement of Proteasome Inhibitor Effectiveness. Am J Transplant (2017) 17(suppl 3).
162. Woodle E, Tremblay S, Castro-Rojas C, Knechtle S, Shields A, Alloway R, et al. In Vivo Administration of Plerixafor and Bortezomib Results in Bone Marrow Plasma Cell Mobilization and Death While Inducing Biphasic Plasma Cell Apoptosis in Peripheral Blood. Am J Transplant (2017) 17 (suppl 3).
163. Pescovitz MD. Rituximab, an Anti-Cd20 Monoclonal Antibody: History and Mechanism of Action. Am J Transplant (2006) 6(5 Pt 1):859–66. doi: 10.1111/j.1600-6143.2006.01288.x
164. Jackson AM, Kraus ES, Orandi BJ, Segev DL, Montgomery RA, Zachary AA. A Closer Look at Rituximab Induction on HLA Antibody Rebound Following HLA-Incompatible Kidney Transplantation. Kidney Int (2015) 87(2):409–16. doi: 10.1038/ki.2014.261
165. Vo AA, Lukovsky M, Toyoda M, Wang J, Reinsmoen NL, Lai CH, et al. Rituximab and Intravenous Immune Globulin for Desensitization During Renal Transplantation. N Engl J Med (2008) 359(3):242–51. doi: 10.1056/NEJMoa0707894
166. Vo AA, Choi J, Cisneros K, Reinsmoen N, Haas M, Ge S, et al. Benefits of Rituximab Combined With Intravenous Immunoglobulin for Desensitization in Kidney Transplant Recipients. Transplantation (2014) 98(3):312–9. doi: 10.1097/TP.0000000000000064
167. Patel J, Kittleson M, Rafiei M, Stern L, Chang D, Czer L, et al. Desensitization Therapy With Immunoglobulin (IVIG) and Rituximb for Patients Awaiting Heart Transplantation [Abstract]. J Heart Lung Transplant (2012) 31(4):S161. doi: 10.1016/j.healun.2012.01.468
168. Kobashigawa JA, Patel JK, Kittleson MM, Kawano MA, Kiyosaki KK, Davis SN, et al. The Long-Term Outcome of Treated Sensitized Patients Who Undergo Heart Transplantation. Clin Transplant (2011) 25(1):E61–67. doi: 10.1111/j.1399-0012.2010.01334.x
169. Asante-Korang A, Amankwah EK, Lopez-Cepero M, Ringewald J, Carapellucci J, Krasnopero D, et al. Outcomes in Highly Sensitized Pediatric Heart Transplant Patients Using Current Management Strategies. J Heart Lung Transplant (2015) 34(2):175–81.doi: 10.1016/j.healun.2014.09.027
170. Schumacher KR, Ramon DS, Kamoun M, Caruthers R, Gajarski RJ. HLA Desensitization in Pediatric Heart Transplant Candidates: Efficacy of Rituximab and IVIg. J Heart Lung Transplant (2012) 31(9):1041–2. doi: 10.1016/j.healun.2012.05.009
171. Starling RC, Armstrong B, Bridges ND, Eisen H, Givertz MM, Kfoury AG, et al. Accelerated Allograft Vasculopathy With Rituximab After Cardiac Transplantation. J Am Coll Cardiol (2019) 74(1):36–51. doi: 10.1016/j.jacc.2019.04.056
172. Clatworthy MR, Watson CJ, Plotnek G, Bardsley V, Chaudhry AN, Bradley JA, et al. B-Cell-Depleting Induction Therapy and Acute Cellular Rejection. N Engl J Med (2009) 360(25):2683–5. doi: 10.1056/NEJMc0808481
173. Cherukuri A, Salama AD, Mehta R, Mohib K, Zheng L, Magee C, et al. Transitional B Cell Cytokines Predict Renal Allograft Outcomes. Sci Transl Med (2021) 13(582). doi: 10.1126/scitranslmed.abe4929
174. Breedveld F, Agarwal S, Yin M, Ren S, Li NF, Shaw TM, et al. Rituximab Pharmacokinetics in Patients With Rheumatoid Arthritis: B-Cell Levels do Not Correlate With Clinical Response. J Clin Pharmacol (2007) 47(9):1119–28. doi: 10.1177/0091270007305297
175. Nakou M, Katsikas G, Sidiropoulos P, Bertsias G, Papadimitraki E, Raptopoulou A, et al. Rituximab Therapy Reduces Activated B Cells in Both the Peripheral Blood and Bone Marrow of Patients With Rheumatoid Arthritis: Depletion of Memory B Cells Correlates With Clinical Response. Arthritis Res Ther (2009) 11(4):R131. doi: 10.1186/ar2798
176. Ramwadhdoebe TH, van Baarsen LGM, Boumans MJH, Bruijnen STG, Safy M, Berger FH, et al. Effect of Rituximab Treatment on T and B Cell Subsets in Lymph Node Biopsies of Patients With Rheumatoid Arthritis. Rheumatol (Oxford) (2019) 58(6):1075–85. doi: 10.1093/rheumatology/key428
177. Teng YK, Levarht EW, Hashemi M, Bajema IM, Toes RE, Huizinga TW, et al. Immunohistochemical Analysis as a Means to Predict Responsiveness to Rituximab Treatment. Arthritis Rheumatol (2007) 56(12):3909–18. doi: 10.1002/art.22967
178. Kamburova EG, Koenen HJ, Boon L, Hilbrands LB, Joosten I. In Vitro Effects of Rituximab on the Proliferation, Activation and Differentiation of Human B Cells. Am J Transplant (2012) 12(2):341–50. doi: 10.1111/j.1600-6143.2011.03833.x
179. Cho A, Bradley B, Kauffman R, Priyamvada L, Kovalenkov Y, Feldman R, et al. Robust Memory Responses Against Influenza Vaccination in Pemphigus Patients Previously Treated With Rituximab. JCI Insight (2017) 2(12). doi: 10.1172/jci.insight.93222
180. Rosengren S, Wei N, Kalunian KC, Zvaifler NJ, Kavanaugh A, Boyle DL. Elevated Autoantibody Content in Rheumatoid Arthritis Synovia With Lymphoid Aggregates and the Effect of Rituximab. Arthritis Res Ther (2008) 10(5):R105. doi: 10.1186/ar2497
181. Gong Q, Ou Q, Ye S, Lee WP, Cornelius J, Diehl L, et al. Importance of Cellular Microenvironment and Circulatory Dynamics in B Cell Immunotherapy. J Immunol (2005) 174(2):817–26. doi: 10.4049/jimmunol.174.2.817
182. Highlights of Prescribing Information: UPLIZNA. Available at: https://www.accessdata.fda.gov/drugsatfda_docs/label/2020/761142s000lbl.pdf (Accessed April 25th, 2021).
183. Tremblay S, Rike-Shields A, Govil A, Cardi M, Brailey P, Alloway R, et al. BAFF Inhibition to Prevent Antibody Rebound Following Proteasome Inhibitor-Based Desensitization: A Randomized, Controlled Trial. Am J Transplant (2020) 20(suppl 3).
184. Schroder PM, Fitch ZW, Schmitz R, Choi AY, Kwun J, Knechtle SJ. The Past, Present, and Future of Costimulation Blockade in Organ Transplantation. Curr Opin Organ Transplant (2019) 24(4):391–401. doi: 10.1097/MOT.0000000000000656
185. Larsen CP, Pearson TC, Adams AB, Tso P, Shirasugi N, Strobert E, et al. Rational Development of LEA29Y (Belatacept), a High-Affinity Variant of CTLA4-Ig With Potent Immunosuppressive Properties. Am J Transplant (2005) 5(3):443–53. doi: 10.1111/j.1600-6143.2005.00749.x
186. Bray RA, Gebel HM, Townsend R, Roberts ME, Polinsky M, Yang L, et al. De Novo Donor-Specific Antibodies in Belatacept-Treated vs Cyclosporine-Treated Kidney-Transplant Recipients: Post Hoc Analyses of the Randomized Phase III BENEFIT and BENEFIT-EXT Studies. Am J Transplant (2018) 18(7):1783–9. doi: 10.1111/ajt.14721
187. Chen J, Wang Q, Yin D, Vu V, Sciammas R, Chong AS. Cutting Edge: CTLA-4ig Inhibits Memory B Cell Responses and Promotes Allograft Survival in Sensitized Recipients. J Immunol (2015) 195(9):4069–73. doi: 10.4049/jimmunol.1500940
188. Jain D, Rajab A, Young JS, Yin D, Nadasdy T, Chong AS, et al. Reversing Donor-Specific Antibody Responses and Antibody-Mediated Rejection With Bortezomib and Belatacept in Mice and Kidney Transplant Recipients. Am J Transplant (2020) 20(10):2675–85. doi: 10.1111/ajt.15881
189. Kwun J, Burghuber C, Manook M, Ezekian B, Park J, Yoon J, et al. Successful Desensitization With Proteasome Inhibition and Costimulation Blockade in Sensitized Nonhuman Primates. Blood Adv (2017) 1(24):2115–9. doi: 10.1182/bloodadvances.2017010991
190. Rozanski CH, Arens R, Carlson LM, Nair J, Boise LH, Chanan-Khan AA, et al. Sustained Antibody Responses Depend on CD28 Function in Bone Marrow-Resident Plasma Cells. J Exp Med (2011) 208(7):1435–46. doi: 10.1084/jem.20110040
191. Bray RA, Gebel HM, Townsend R, Roberts ME, Polinsky M, Yang L, et al. Posttransplant Reduction in Preexisting Donor-Specific Antibody Levels After Belatacept- Versus Cyclosporine-Based Immunosuppression: Post Hoc Analyses of BENEFIT and BENEFIT-EXT. Am J Transplant (2018) 18(7):1774–82. doi: 10.1111/ajt.14738
192. Parsons RF, Zahid A, Bumb S, Decker H, Sullivan HC, Eun-Hyung Lee F, et al. The Impact of Belatacept on Third-Party HLA Alloantibodies in Highly Sensitized Kidney Transplant Recipients. Am J Transplant (2020) 20(2):573–81. doi: 10.1111/ajt.15585
193. Rose-John S, Winthrop K, Calabrese L. The Role of IL-6 in Host Defence Against Infections: Immunobiology and Clinical Implications. Nat Rev Rheumatol (2017) 13(7):399–409.doi: 10.1038/nrrheum.2017.83
194. Vo AA, Choi J, Kim I, et al. A Phase I/II Trial of the Interleukin-6 Receptor-Specific Humanized Monoclonal (Tocilizumab) + Intravenous Immunoglobulin in Difficult to Desensitize Patients. Transplantation (2015) 99(11):2356–63. doi: 10.1097/TP.0000000000000741
195. Jouve T, Laheurte C, Noble J, Weinhard J, Daligault M, Renaudin A, et al. Immune Responses Following Tocilizumab Therapy to Desensitize HLA-Sensitized Kidney-Transplant Candidates. Am J Transplant (2021). doi: 10.1111/ajt.16709
196. Chandran S, Leung J, Hu C, Laszik ZG, Tang Q, Vincenti FG. Interleukin-6 Blockade With Tocilizumab Increases Tregs and Reduces T Effector Cytokines in Renal Graft Inflammation: A Randomized Controlled Trial. Am J Transplant (2021) 21(7):2543–54. doi: 10.1111/ajt.16459
197. Stanley J, Shili G, Nori A, Mieko T, Edmund H, Alice P, et al. Clazakizumab (Anti-IL-6) Induces Foxp3+ Tregs in Highly HLA Sensitized Patients Desensitized for HLAi Transplantation (Nct03380962). Transplantation (2020) 104(S3):S105. doi: 10.1097/01.tp.0000698800.17823.68
198. Vo AAAN, Huang E, Toyoda M, Ge S, Peng A, Najjar R, et al. Desensitization Using Clazakizumab® (Anti-Il-6) in Highly-Hla Sensitized Patients Awaiting Kidney Transplant. Am J Transplant (2021) 104(S3):S104–5. doi: 10.1097/01.tp.0000698796.63079.e4
199. Choi J, Aubert O, Vo A, Loupy A, Haas M, Puliyanda D, et al. Assessment of Tocilizumab (Anti-Interleukin-6 Receptor Monoclonal) as a Potential Treatment for Chronic Antibody-Mediated Rejection and Transplant Glomerulopathy in HLA-Sensitized Renal Allograft Recipients. Am J Transplant (2017) 17(9):2381–9. doi: 10.1111/ajt.14228
200. Doberer K, Duerr M, Halloran PF, Eskandary F, Budde K, Regele H, et al. A Randomized Clinical Trial of Anti-IL-6 Antibody Clazakizumab in Late Antibody-Mediated Kidney Transplant Rejection. J Am Soc Nephrol (2021) 32(3):708–22. doi: 10.1681/ASN.2020071106
201. Comoli P, Ginevri F, Maccario R, Avanzini MA, Marconi M, Groff A, et al. Human Mesenchymal Stem Cells Inhibit Antibody Production Induced In Vitro by Allostimulation. Nephrol Dial Transplant (2008) 23(4):1196–202. doi: 10.1093/ndt/gfm740
202. Zhang Z, Wilson NA, Chinnadurai R, Panzer SE, Redfield RR 3rd, Reese SR, et al. Autologous Mesenchymal Stromal Cells Prevent Transfusion-Elicited Sensitization and Upregulate Transitional and Regulatory B Cells. Transplant Direct (2018) 4(9):e387. doi: 10.1097/TXD.0000000000000827
203. Lohan P, Murphy N, Treacy O, Lynch K, Morcos M, Chen B, et al. Third-Party Allogeneic Mesenchymal Stromal Cells Prevent Rejection in a Pre-Sensitized High-Risk Model of Corneal Transplantation. Front Immunol (2018) 9:2666. doi: 10.3389/fimmu.2018.02666
204. Saadi G, Fadel F, El Ansary M, El-Hamid SA. Mesenchymal Stem Cell Transfusion for Desensitization of Positive Lymphocyte Cross-Match Before Kidney Transplantation: Outcome of 3 Cases. Cell Prolif (2013) 46(2):121–6. doi: 10.1111/cpr.12012
205. Erpicum P, Weekers L, Detry O, Bonvoisin C, Delbouille MH, Grégoire C, et al. Infusion of Third-Party Mesenchymal Stromal Cells After Kidney Transplantation: A Phase I-II, Open-Label, Clinical Study. Kidney Int (2019) 95(3):693–707. doi: 10.1016/j.kint.2018.08.046
206. Dreyer GJ, Groeneweg KE, Heidt S, Roelen DL, van Pel M, Roelofs H, et al. Human Leukocyte Antigen Selected Allogeneic Mesenchymal Stromal Cell Therapy in Renal Transplantation: The Neptune Study, a Phase I Single-Center Study. Am J Transplant (2020) 20(10):2905–15. doi: 10.1111/ajt.15910
207. Perico N, Casiraghi F, Remuzzi G. Clinical Translation of Mesenchymal Stromal Cell Therapies in Nephrology. J Am Soc Nephrol (2018) 29(2):362–75. doi: 10.1681/ASN.2017070781
208. Podesta MA, Remuzzi G, Casiraghi F. Mesenchymal Stromal Cells for Transplant Tolerance. Front Immunol (2019) 10:1287. doi: 10.3389/fimmu.2019.01287
209. Hickson LJ, Herrmann SM, McNicholas BA, Griffin MD. McNicholas and Matthew D. Griffin. Progress Toward the Clinical Application of Mesenchymal Stromal Cells and Other Disease-Modulating Regenerative Therapies: Examples From the Field of Nephrology. Kidney360 (2021) 2(3):542–57. doi: 10.34067/KID.0005692020
210. Sawitzki B, Harden PN, Reinke P, Moreau A, Hutchinson JA, Game DS, et al. Regulatory Cell Therapy in Kidney Transplantation (The ONE Study): A Harmonised Design and Analysis of Seven Non-Randomised, Single-Arm, Phase 1/2A Trials. Lancet (2020) 395(10237):1627–39. doi: 10.1016/S0140-6736(20)30167-7
211. Roemhild A, Otto NM, Moll G, Abou-El-Enein M, Kaiser D, Bold G, et al. Regulatory T Cells for Minimising Immune Suppression in Kidney Transplantation: Phase I/IIa Clinical Trial. BMJ (2020) 371:m3734. doi: 10.1136/bmj.m3734
212. Liao T, Xue Y, Zhao D, Li S, Liu M, Chen J, et al. In Vivo Attenuation of Antibody-Mediated Acute Renal Allograft Rejection by Ex Vivo TGF-Beta-Induced CD4(+)Foxp3(+) Regulatory T Cells. Front Immunol (2017) 8:1334. doi: 10.3389/fimmu.2017.01334
Keywords: HLA, sensitization, desensitization, non-HLA antibodies, heart transplantation, cPRA, humoral immune response
Citation: Habal MV (2021) Current Desensitization Strategies in Heart Transplantation. Front. Immunol. 12:702186. doi: 10.3389/fimmu.2021.702186
Received: 29 April 2021; Accepted: 26 July 2021;
Published: 24 August 2021.
Edited by:
Annette M. Jackson, Duke University, United StatesReviewed by:
Georg Böhmig, Medical University of Vienna, AustriaRusan Ali Catar, Charité – Universitätsmedizin Berlin, Germany
Copyright © 2021 Habal. This is an open-access article distributed under the terms of the Creative Commons Attribution License (CC BY). The use, distribution or reproduction in other forums is permitted, provided the original author(s) and the copyright owner(s) are credited and that the original publication in this journal is cited, in accordance with accepted academic practice. No use, distribution or reproduction is permitted which does not comply with these terms.
*Correspondence: Marlena V. Habal, bWgzNjk2QGN1bWMuY29sdW1iaWEuZWR1