- Department of Comparative Biomedical Sciences, School of Veterinary Medicine, Louisiana State University, Baton Rouge, LA, United States
Cre-LoxP system has been widely used to induce recombination of floxed genes of interest. Currently available macrophage promoter-specific Cre recombinase mice strains have various limitations that warrants the testing of additional Cre strains. V-maf musculoaponeurotic fibrosarcoma oncogene family, protein b -Cre (Mafb-Cre) mice label macrophages in most organs such as spleen, small intestine, lung, bone marrow, and peritoneal cavity. However, whether Mafb-Cre recombinase targets the gene recombination in alveolar macrophage remains untested. Here, we utilized MafbCre/WTR26mTmG/WT strain that expresses mTOM protein in all the cells of mouse body except for those that express Mafb-Cre-regulated mEGFP. We performed fluorescent microscopy and flow cytometry to analyze mTOM and mEGFP expression in alveolar macrophages from MafbCre/WTR26mTmG/WT mice. Our analyses revealed that the Mafb-Cre is active in only ~40% of the alveolar macrophages in an age-independent manner. While Mafb- (mTOM+/mEGFP-) and Mafb+ (mEGFP+) alveolar macrophages exhibit comparable expression of CD11b and CD11c surface markers, the surface expression of MHCII is elevated in the Mafb+ (mEGFP+) macrophages. The bone marrow-derived macrophages from MafbCre/WTR26mTmG/WT mice are highly amenable to Cre-LoxP recombination in vitro. The bone marrow depletion and reconstitution experiment revealed that ~98% of alveolar macrophages from MafbCre/WTR26mTmG/WT → WT chimera are amenable to the Mafb-Cre-mediated recombination. Finally, the Th2 stimulation and ozone exposure to the MafbCre/WTR26mTmG/WT mice promote the Mafb-Cre-mediated recombination in alveolar macrophages. In conclusion, while the Mafb-/Mafb+ dichotomy thwarts the use of Mafb-Cre for the induction of floxed alleles in the entire alveolar macrophage population, this strain provides a unique tool to induce gene deletion in alveolar macrophages that encounter Th2 microenvironment in the lung airspaces.
Introduction
Cre-LoxP system has been widely used to induce recombination of floxed genes of interest (1, 2). In this system, the Cre recombinase enzyme recognizes two target 34bp LoxP (locus of x-over, P1) unidirectional sequences in genomic DNA and catalyzes a recombination reaction where floxed allelic region, i.e., a sequence that is flanked by two LoxP sequences, is excised (3). The gene recombination can be restricted to a particular cell type by employing a cell type-specific promoter to induce Cre recombinase transgene expression in a cell type-specific manner. Numerous mouse strains with Cre recombinase expression in single cell type including hepatocytes (4), alveolar type II cells (5, 6), club cells (7), myocardium (8) are available. Due to the relatively higher degree of plasticity in immune cells (9), including macrophages (10), widely acceptable immune cell-specific Cre recombinase mouse strains are very limited.
Several Cre recombinase mouse strains utilizing myeloid-associated promoters such as Lysozyme M (11–14), Colony Stimulating Factor 1 Receptor (Csf1r) (15), CD11b (16–18), CD11c (19–22), C-X3-C Motif Chemokine receptor 1 (Cx3cr1) (23), and F4/80 (24) have been used to explore macrophage-specific roles. These strains, however, are known to target non-macrophage cell types as well (14, 23–32). Therefore, mouse strains that restrict Cre recombinase specifically to the macrophages are still awaited. V-maf musculoaponeurotic fibrosarcoma oncogene family, protein b (Mafb) promoter has been reported to express in macrophages (33). In that report, Mafb-Cre mouse strain was employed to demonstrate that there was a clear separation of macrophage and dendritic cell (DC) populations based on the expression of MAFB and ZBTB46, respectively (33). Moreover, Mafb-Cre mice appeared to be a reliable alternative to trace and distinguish macrophages from other cell types, especially DCs, in most organs such as spleen, small intestine, lung, bone marrow, and peritoneal cavity (33). However, the capability of Mafb-cre recombinase in inducing floxed gene recombination in alveolar macrophage remains untested.
In the current study, we sought to determine the effectiveness of Mafb-regulated Cre recombinase in the induction of recombination within a floxed mTOM/mEGFP (mTmG) reporter allele. Therefore, we utilized R26mTmG/mTmG reporter strain that expresses mTOM protein in all the cells of mouse body except for those that express Cre recombinase enzyme (34). The Cre recombinase-expressing cells in R26mTmG/mTmG reporter mice translate mEGFP protein instead of mTOM, a readout for a successful recombination event. We hypothesized that all the alveolar macrophages from the MafbCre/WTR26mTmG/WT mice express mEGFP protein. To address this hypothesis, we harvested alveolar macrophages from MafbCre/WTR26mTmG/WT mice and analyzed their mTOM and mEGFP expression status using fluorescent microscopy and flow cytometry. Our data suggest that Mafb gene determines the alveolar macrophage dichotomy, which is independent of the recruitment of bone marrow-derived macrophages.
Materials and methods
Generation of MafbCre/WTR26mTmG/WT mice and animal husbandry
Mafb-regulated Cre recombinase expressing line (B6N(129S4)-Mafbtm1.1(cre)Kmm/J), Rosa promoter (R26) regulated mTOM/mEGFP (mTmG) dual fluorescent reporter line (B6.129(Cg)-Gt26Sortm4(ACTB-tdTomato,-EGFP)Luo/J) were procured from Jackson Laboratory (Bar Harbor, ME). MafbCre/WT mice had mixed C57BL/6J and C57BL/6N background while mTOM/mEGFP reporter mice were from C57BL/6J background. These two strains were crossed to generate MafbCre/WTR26mTmG/WT mice. All mice used in this study were maintained in hot-washed, individual ventilated cages, strictly followed 12-hour dark/light cycle and were fed regular diet with water ad libitum. All animal procedures were performed under animal protocol approved by the Institutional Animal Care and Use Committee (IACUC) of the Louisiana State University.
Bronchoalveolar lavage fluid collection
MafbCre/WTR26mTmG/WT neonates (PND 3) and adults (PND 42) were anesthetized via intraperitoneal injection (11) of 2,2,2-tribromoethanol (Millipore Sigma, Burlington, MA). After midline laparotomy, lung and trachea were exposed via thoracotomy. Whole lung was lavaged with phosphate buffered saline (PBS) and processed, as previously described (35). BAL cells were processed for flow cytometry and fluorescent microscopic analyses.
Flow cytometry
BAL cells were Fc-blocked with CD16/32 (Thermo Fisher Scientific, Waltham, MA) and stained with leukocyte panel, including AF700 CD45 (BioLegend, San Diego, CA), BV510 CD11c (Thermo Fisher Scientific, Waltham, MA), BV785 CD11b (BioLegend, San Diego, CA), BV605 Ly6G (BioLegend, San Diego, CA), BV421 CD64 (BioLegend, San Diego, CA), APC CD24 (BioLegend, San Diego, CA), and PeCP-Cy5.5 MHCII (BioLegend, San Diego, CA) to characterize the alveolar macrophage population, which was further analyzed for mTOM+ and mEGFP+ subpopulations using Cytoflex (Beckman Coulter, Inc., CA). Flow cytometry data was analyzed by CytExpert Software (Beckman Coulter, Inc., CA).
Oropharyngeal M1/M2 challenge
MafbCre/WTR26mTmG/WT mice were anesthetized with isoflurane and oropharyngeal challenged with a cocktail of Th1 stimulants LPS [10 μl (10 μg) LPS + 40 μl saline] and IFN-γ, or Th2 stimulant IL-33 (1.25 μg IL-33 in 50 μl saline) for alternative (M2) activation. For M1 activation, mice were challenged with 10 μg of LPS on day 1 and the IFN-γ was instilled on day 7, followed by BALF collection of day 8. For M2 activation, mice were oropharyngeal challenged with 1.25 μg of IL-33 on days 1, 3, 5, 7 and BALF was collected on day 8.
Ozone exposure
Ozone exposure procedure has been previously established (36). Ozone was generated from the Ozone Generator (TSE, Chesterfield, MO) and was supplied to 1.3m3 glass chambers. Briefly, mice were transferred into cages with perforated lids and were placed inside the dark chambers without feed and water before the start of DLAM night cycle. Ozone concentration was maintained at ~ 800 ppb throughout the 4-hour duration of the exposure. Ozone concentration along with chamber temperature, pressure and humidity were monitored and recorded at hourly interval during exposures. The timing of exposures was maintained strictly throughout the 14-day exposure.
Bone marrow transplantation
8-10-week-old WT mice on C57BL/6J background were lethally irradiated with 6 Megavolt X-rays from a Linear Accelerator (Varian Clinac 21EX) with two (dorsal and ventral) 525-rad (525 cGy) doses (37). Femur and tibia bones of donor MafbCre/WTR26mTmG/WT mice were collected to prepare single suspension of bone marrow cells for transplantation. A total of 8 × 106 cells were injected into the tail vein of lethally irradiated recipient mice. After receiving bone marrow cells via tail vein injection, the recipient mice were given 0.2% neomycin sulfate dissolved in acidified water for the first 2 weeks post-transplantation to reduce the bacterial growth in water bottles due to regurgitated food. Necropsies were performed 5 weeks post-bone marrow injections.
Statistics
Student’s t test was used for two-group comparisons. One-way ANOVA was used for three-group comparisons. The p values <0.05 were considered statistically significant. All data represented at least three different experiments. GraphPad Prism 8.0 (La Jolla, CA) was used for statistical analyses. The values were represented as mean ± SEM.
Results
Steady-state alveolar macrophages exist as Mafb+ and Mafb- mixed populations
We crossed MafbCre/WT and R26mTmG/mTmG reporter mice to generate MafbCre/WTR26mTmG/WT strain (Figure 1A). First, we analyzed alveolar macrophages in 3-day-old MafbCre/WTR26mTmG/WT neonates. To our surprise, only ~40% (34 ± 2.4%) of the harvested alveolar macrophages expressed mEGFP green fluorescent protein, indicating Mafb promoter activity and associated Cre-LoxP recombination. The remaining ~60% (64 ± 2.1%) of alveolar macrophages exclusively exhibited mTOM expression suggesting the absence of Mafb activity and therefore the lack of Cre recombinase expression and recombination in the floxed reporter allele (Figures 1B–D). Because macrophages from different origins colonize the lung in three successive waves throughout the embryonic developmental stages and spatially distribute during the first week of postnatal life (38), we hypothesized that, as compared to the neonatal alveolar macrophage population, the alveolar macrophage population collected from adult MafbCre/WTR26mTmG/WT mice possesses different composition of mTOM+ and mEGFP+ macrophages. Therefore, we analyzed alveolar macrophages from 6-week-old MafbCre/WTR26mTmG/WT mice. Again, to our surprise, the adult mice also had ~40% (36.6 ± 2%) and ~60% (62.9 ± 2.1%) alveolar macrophages that exhibited mEGFP and exclusive mTOM expression, respectively (Figures 1E–G). These data suggest that the effectiveness of Mafb-regulated Cre recombination is comparable in the neonatal and matured stage of the lung, and that Mafb promoter is not expressed in all the steady-state alveolar macrophages.
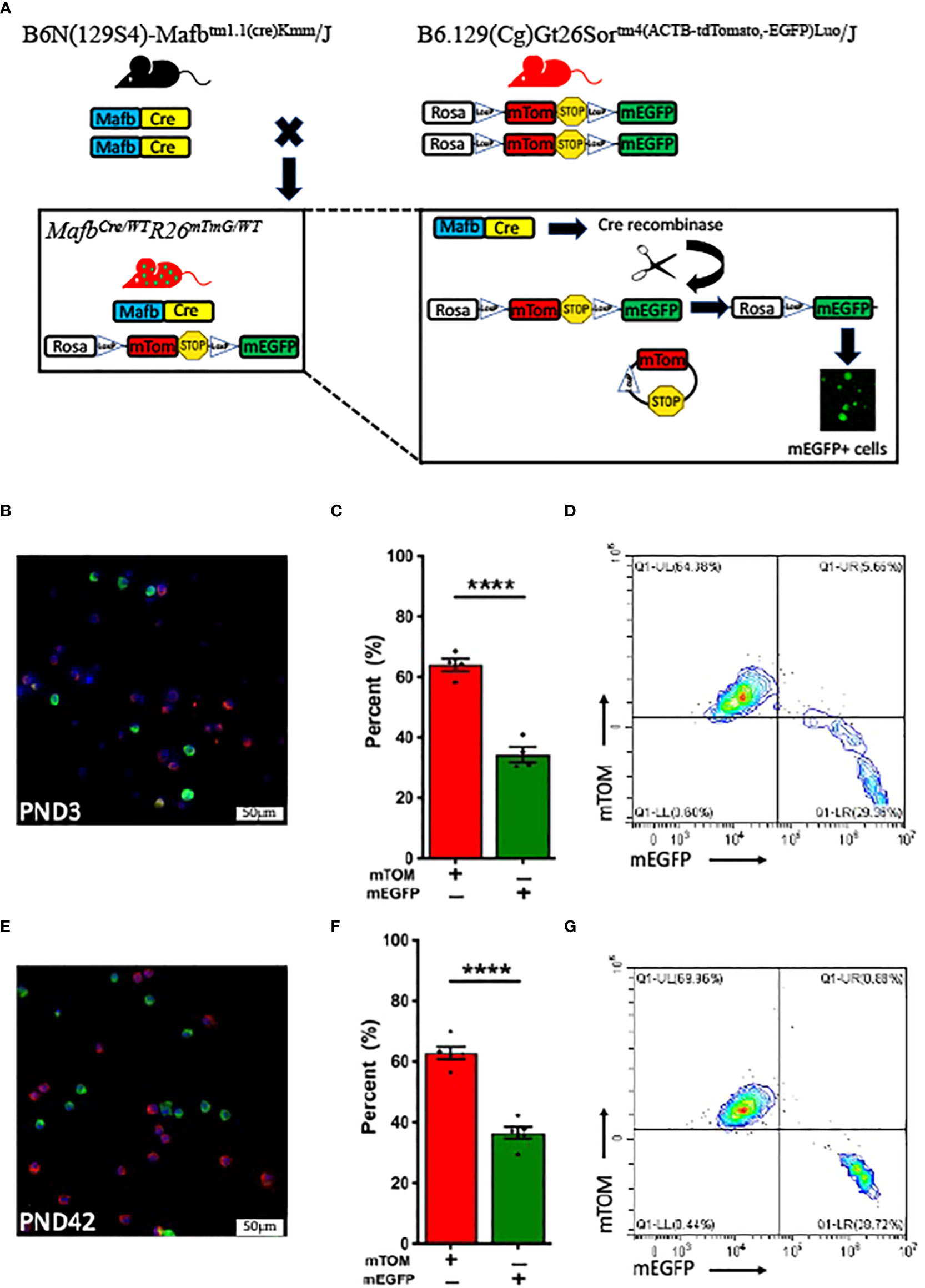
Figure 1 MafbCre/WTR26mTmG/WT mice at various stages of postnatal development exhibit comparable Cre-LoxP efficiency. (A) Generation of transgenic MafbCre/WTR26mTmG/WT mice. Mafb-regulated Cre recombinase expressing strain (B6N(129S4)-Mafbtm1.1(cre)Kmm/J) was crossed with Rosa promoter (R26)-regulated dual fluorescent mTOM/mEGFP reporter strain (B6.129(Cg)-Gt26Sortm4(ACTB-tdTomato,-EGFP)Luo/J) to generate MafbCre/WTR26mTmG/WT mice. In cells with active Mafb promoter in MafbCre/WTR26mTmG/WT mice, Cre recombinase excises the mTOM and PolyA Stop sequences, which are flanked by the LoxP sites, and allows the translation of mEGFP protein. Representative fluorescent photomicrographs of (B) PND 3 (n=4) and (E) PND 42 (6-week-old) (n=5) BALF cells from MafbCre/WTR26mTmG/WT mice depicting the fluorescent cell composition and respective percentage of exclusively mTOM+ and mEGFP+ cells in (C) PND 3 and (F) PND 42 MafbCre/WTR26mTmG/WT mice. Error bars represent SEM ****p<0.0001 using Student’s t test. Representative flow cytometry graphs depicting exclusively mTOM+ (UL) and mEGFP+ (UR+LR) cells, respectively in BALF from (D) PND 3 and (G) PND 42 MafbCre/WTR26mTmG/WT mice.
Next, to test the hypothesis that the mTOM+ alveolar macrophages reflect immature macrophages and have the potential to express Mafb-regulated Cre and mEGFP expression at maturity, we harvested alveolar macrophages and plated them in a cell culture dish. During the observation period, we did not observe mEGFP positivity in the mTOM+ cells (Supplemental Figure 1). Consistent with this finding, the flow cytometry analyses did not reveal double positive cells (Figure 1G), i.e., transitional population that reflect the read out from pre-recombination (mTOM expression) as well as post-recombination (mEGFP expression) events. These data suggest a coexistence of exclusively mEGFP- and mTOM-expressing macrophage populations in the lung airspace at homeostasis.
Further, we analyzed mTOM+ and mEGFP+ macrophages for the expression of selected myeloid cell surface markers such as CD11b, CD11c, and MHCII (Figure 2). Interestingly, the expression of these surface markers, except for MHCII, were comparable between the analyzed mTOM+ and mEGFP+ macrophages (Figure 2). mEGFP+ macrophages exhibited higher MHCII expression as compared to mTOM+ counterparts (Figure 2J). These data indicate a coexistence of alveolar macrophage subpopulations that display distinct MHCII expression, indicating their different antigen presentation potential.
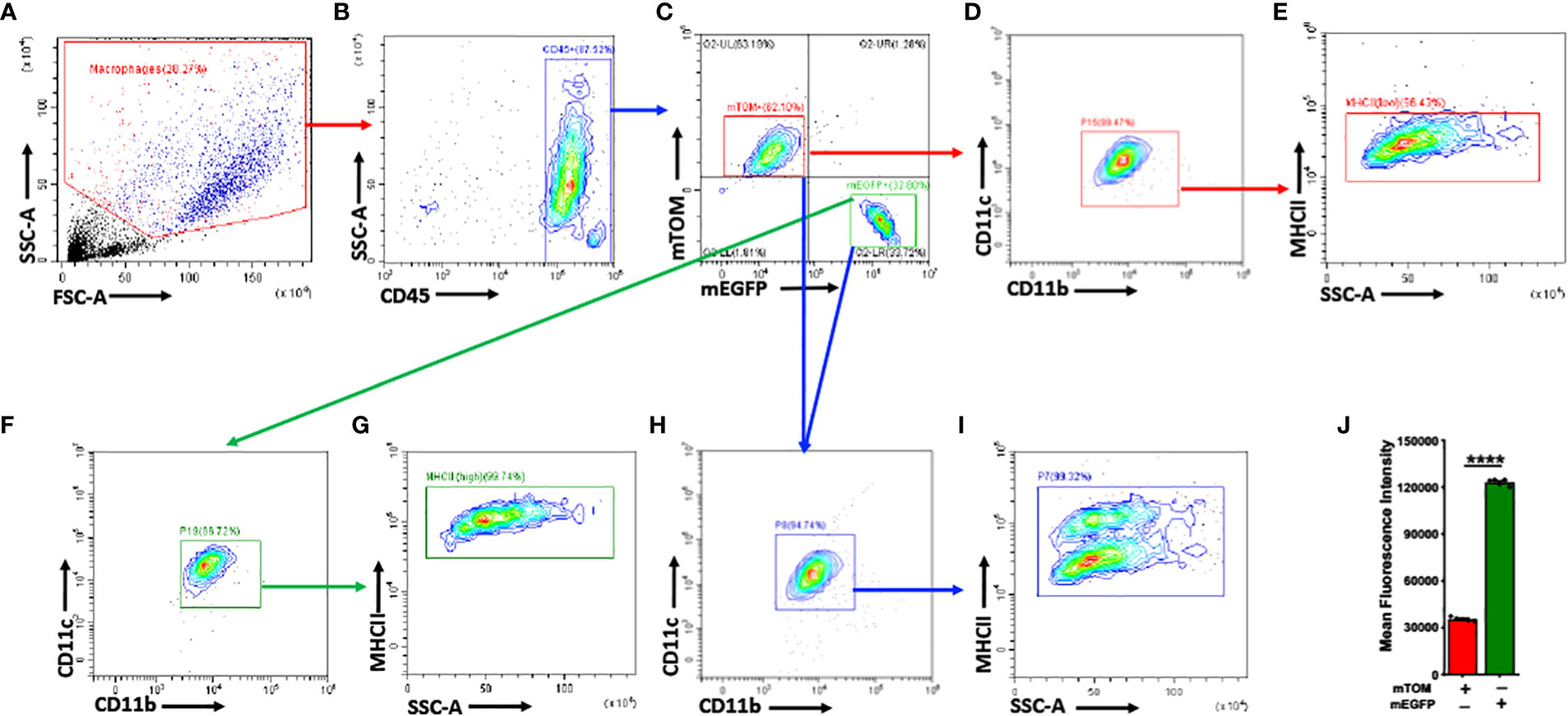
Figure 2 mTOM+ and mEGFP+ subpopulations share comparable myeloid surface markers, except for MHCII, in naïve MafbCre/WTR26mTmG/WT. (A) Applied Side Scatter (SSC) and Forward Scatter (FSC) to eliminate dead cells and debris. (B) Leukocyte CD45+ cells were identified. (C) mTOM+ and mEGFP+ subpopulations were isolated. Myeloid markers such as CD11c, CD11b, MHCII, were assessed in (D, E) mTOM+ and (F, G) mEGFP+ subpopulations. (H) Overlapping mTOM+ and mEGFP+ cells revealed comparable expression of CD11c and CD11b between these subpopulations. (I) mEGFP+ cells exhibited higher MHCII expression as compared to mTOM+ cells. (J) Histogram depicting mean fluorescence intensity (MFI) of MHCII expression in mTOM+ and mEGFP+ populations. Error bars represent SEM ****p<0.0001 using Student’s t test. Data shown are from PND 42 naïve MafbCre/WTR26mTmG/WT mice (n=5).
Bone marrow-derived macrophages are amenable to Mafb-regulated Cre recombinase expression and recombination in floxed alleles
Previous report suggests that macrophages under homeostasis are seeded from three separate lineages: yolk sac, fetal liver and bone marrow (38). Therefore, to test whether the concurrent existence of these two alveolar macrophage subpopulations in the lung is dictated by the lung tissue microenvironment or by their differential origins, we analyzed bone marrow-derived macrophages (BMDMs), both in vitro as well as in vivo, for Mafb-regulated induction of mEGFP expression. We generated bone marrow-derived macrophages (BMDMs) using bone marrow progenitors that were harvested from MafbCre/WTR26mTmG/WT. Our analyses for the composition of mTOM+ and mEGFP+ BMDMs revealed that ~95% (94 ± 0.4%) of BMDMs were amenable to Cre-LoxP recombination, as indicated by the upregulated mEGFP expression (Figures 3A–C). These data suggest that the bone marrow-derived macrophages possess robust Mafb expression and Mafb-mediated Cre-LoxP recombination.
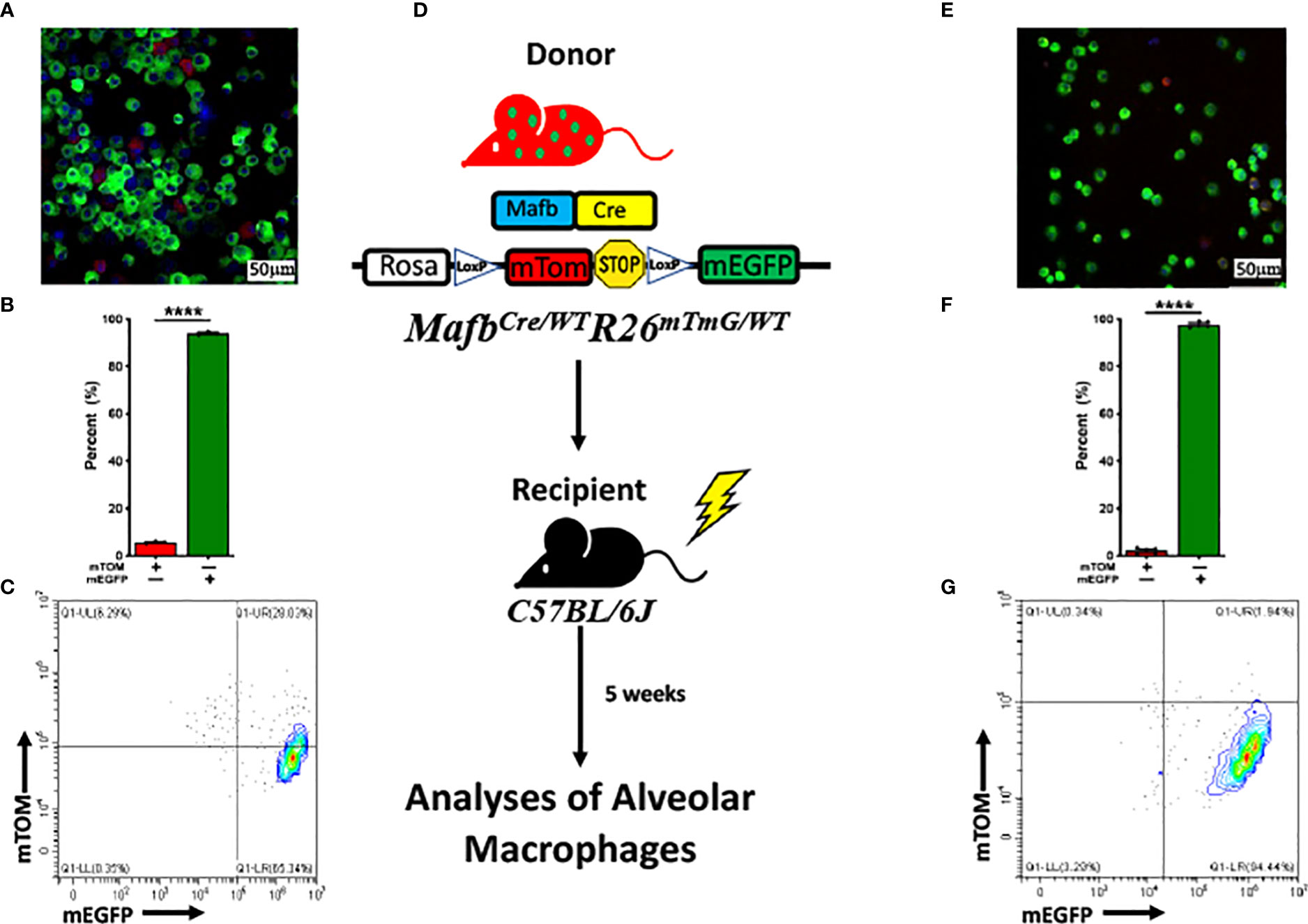
Figure 3 MafbCre/WTR26mTmG/WT bone marrow-derived macrophages (BMDMs) are highly amenable to Cre-LoxP recombination in vitro and in vivo. (A) Representative fluorescent photomicrograph depicting the fluorescent cell composition, (B) respective percentage of exclusively mTOM+ and mEGFP+ cells. The experiment was repeated three times. Error bars represent SEM ****p<0.0001 using Student’s t test. (C) Representative flow cytometry graph depicting exclusively mTOM+ (UL) and mEGFP+ (UR+LR) in bone marrow-derived macrophages (BMDMs) cultured from MafbCre/WTR26mTmG/WT bone marrow progenitors. (D) Generation of chimeric mice via transplantation of bone marrow cells from sex- and age-matched MafbCre/WTR26mTmG/WT donors to lethally irradiated C57BL/6J recipients. Lung macrophages of chimeric mice were repopulated before subjected to flow cytometry and fluorescent microscopy analyses. (E) Representative fluorescent photomicrograph depicting the fluorescent cell composition and (F) respective percentage of exclusively mTOM+ and mEGFP+ cells in BALF of chimeric mice. Error bars represent SEM ****p<0.0001 using Student’s t test. (G) Representative flow cytometry graph depicting exclusively mTOM+ (UL) and mEGFP+ (UR+LR) cells, respectively in alveolar macrophages of chimeric mice (n=5).
Since in vitro-differentiated BMDMs do not recapitulate the lung tissue microenvironment, further in vivo experiments were planned to test whether the bone marrow cells can populate the lung airspaces predominantly with mEGFP+ cells. We hypothesized that bone marrow cells possess robust Mafb expression that cause reconstitution of alveolar macrophage population with mEGFP+ cells. Accordingly, 8-week-old C57BL/6 mice were lethally irradiated to deplete cells of hematopoietic lineage, followed by bone marrow transplantation from MafbCre/WTR26mTmG/WT age- and sex-matched donor mice. After reconstitution phase, BALF harvested from the chimeric mice were examined (Figure 3D). Consistent with the in vitro BMDM experiment, ~98% (97.8 ± 0.6%) of the alveolar macrophages were amenable to Cre-LoxP recombination, as indicated by the robust mEGFP expression (Figures 3E–G). This data suggest that the majority of alveolar macrophages originated from the bone marrow express Mafb gene as compared to fetal liver-derived macrophages.
Type-2 environment enhances the activity of Mafb promoter and promote the Mafb-Cre-driven Cre-LoxP recombination
Mafb gene is known to be upregulated in Th2 inflammation-associated alternatively-activated (M2) macrophages (39). We further speculated that M2 polarization also promotes Mafb promoter upregulation in vivo, we challenged adult MafbCre/WTR26mTmG/WT mice with LPS [10 μl (10 μg) LPS +40 μl saline]/IFN-γ (40) or IL-33 (1.25 μg IL-33 in 50 μl saline) to induce M1 and M2 activation, respectively. The BALF immune cells collected from these mice were subjected to flow cytometry and fluorescent microscopy analyses to determine the composition of mTOM+ and mEGFP+ macrophages. The Cre-LoxP recombination efficiency, as indicated by the composition of mTOM+ and mEGFP+ macrophages, in LPS/IFN-γ-treated mice (Figures 4A–C) was comparable to the naïve MafbCre/WTR26mTmG/WT (59.88 ± 1.69% mTOM+ vs 39.63 ± 1.48% mEGFP+). As expected, ~90% (89.4 ± 2.1%) of cells were mEGFP+ in the BALF of IL-33-treated mice (Figures 4D–F). These data suggest that M2 polarization promotes Cre-LoxP recombination in macrophages regardless of their origins.
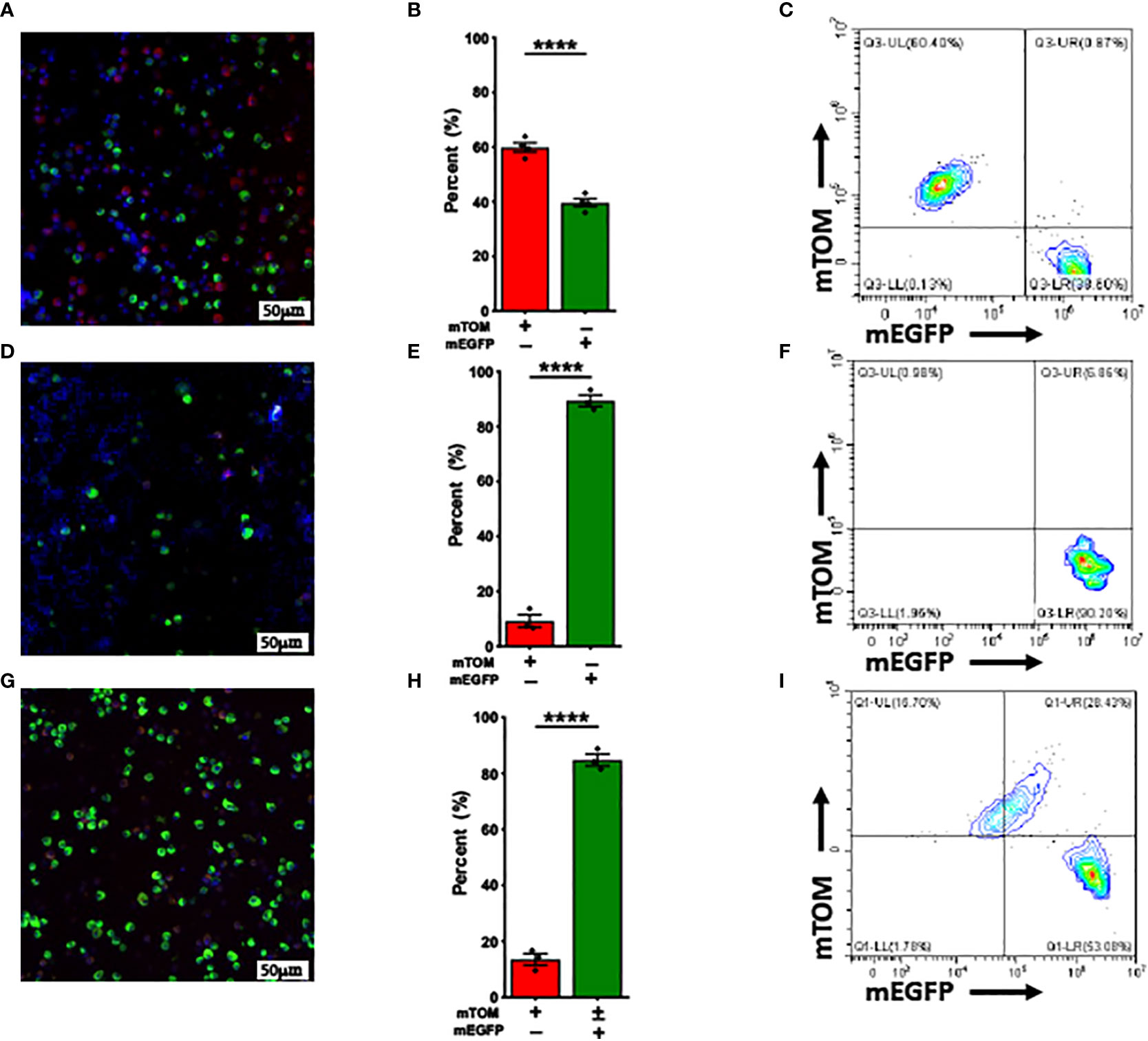
Figure 4 Alternative activation promotes while Classical activation doesn’t affect Mafb-Cre-mediated recombination in vivo. Representative fluorescent photomicrographs of (A) LPS/INF-γ, (D) IL-33 and (G) ozone-exposed MafbCre/WTR26mTmG/WT mice depicting the fluorescent cell composition. Respective percentage of exclusively mTOM+ and mEGFP+ cells in (B) LPS/INF-γ, (E) IL-33 and (H) ozone-exposed MafbCre/WTR26mTmG/WT mice. Error bars represent SEM ****p<0.0001 using Student’s t test. Representative flow cytometry graphs depicting exclusively mTOM+ (UL) and mEGFP+ (UR+LR), respectively of (C) LPS/INF-γ, (F) IL-33 and (I) ozone-exposed MafbCre/WTR26mTmG/WT mice. LPS/IFN-γ-treated mice (n=4), IL-33-treated (n=3) mice, ozone-treated mice (n=3).
Ozone is known to promote M2 activation in alveolar macrophages (36, 41). Therefore, we hypothesized that ozone exposure will activate Mafb promoter in mTOM+ alveolar macrophages of MafbCre/WTR26mTmG/WT mice that will facilitate Cre-LoxP-mediated recombination. Therefore, MafbCre/WTR26mTmG/WT were repetitively exposed to 800ppb of ozone at 4 hours/day for 14 days and the alveolar macrophages were harvested within 16-24h after the last exposure. BALF cells from these mice were subjected to flow cytometry and fluorescent microscopy analyses. Approximately 85% (84.8 ± 2.1%) of alveolar macrophages collected from ozone-exposed mice lungs expressed mEGFP protein, suggesting that ozone exposure activates Mafb promoter in mTOM+ cells that subsequently induces Cre-LoxP-mediated recombination in the reporter allele and thus translates mEGFP protein (Figures 4G–I; Supplemental Figure 2). Approximately 29% of the alveolar macrophages were double positive suggesting continuous transition of mTOM+ into mEGFP+ macrophages (Figure 4G–I; Supplemental Figure 2).
Discussion
Alveolar macrophages exhibit remarkable plasticity in their response to the extracellular milieu that enables them to perform a variety of functions including maintenance of homoeostasis, immune surveillance, microbial clearance, removal of inhaled biotic/abiotic materials and cellular debris, and resolution of inflammation (42). To elucidate the role of various genes in alveolar macrophage functions, various promoters such as Lysozyme, Cd11c, Cd11b, Csf1r, Cx3cr1, and F4/80, are commonly employed to induce Cre-LoxP-mediated gene deletion. However, these promoters not only target macrophage but also induce recombination in non-macrophage cell populations. For example, Lysozyme-Cre (LysM-Cre) targets macrophages, granulocytes, dendritic cells (14), Myeloid-derived suppressor cells (MDSCs) (26), and AT2 cells (23). Similarly, Csf1r-Cre targets all leukocyte population (23), Cd11c-Cre also targets dendritic cells (29), Cd11b-Cre also targets granulocytes, Cx3cr1-Cre targets interstitial macrophages (IMs) but not resident alveolar macrophages (23), and F4/80-Cre targets only some macrophage subpopulations (24). Therefore, alveolar macrophage-specific promoter amenable to efficient Cre-LoxP-mediated recombination is still awaited.
Mafb is a reliable promoter for macrophage lineage tracking in many major organs, such as spleen, small intestine, lung, bone marrow and peritoneal cavity (33). However, whether Mafb is specific to alveolar macrophages is not yet known. In this study, we examined MafbCre/WTR26mTmG/WT mice where the expression of mTOM/mEGFP (mTmG), a dual reporter floxed allele, was used as a readout for Mafb-regulated Cre-LoxP recombination. We hypothesized that Mafb promoter activity induces the Cre-LoxP-mediated recombination and the expression of mEGFP protein in alveolar macrophages. To test this hypothesis, first, Mafb-regulated recombination efficiency was examined in alveolar macrophages from neonatal versus adult mice. Second, we assessed Mafb-regulated recombination efficiency in BMDMs, in vitro as well as in vivo. Third, the effects of Th1 versus Th2 stimuli on Mafb-regulated Cre-LoxP-mediated efficiency were compared. Our findings provide interesting insight into the previously unknown association between Mafb expression and possibly differential macrophage functionality.
The analyses for Mafb-regulated Cre-LoxP-mediated recombination efficiency in steady-state alveolar macrophages from neonatal (PND 3) versus adult (PND 42) mice revealed that ~60% of the harvested alveolar macrophages are not targeted by Mafb-regulated Cre recombinase. Further, the lack of double positive (mTOM+ mEGFP+) alveolar macrophages suggest little to negligible ongoing transition of mTOM+ cells into mEGFP+ cells. These data suggest a tightly-regulated distribution of Mafb- and Mafb+ macrophages in steady-state lung airspaces. Tan et al. reported that the fetal liver-derived macrophages enter the alveoli within 1 week after birth and become resident alveolar macrophages (38). Other studies have demonstrated that the bone marrow-derived monocytes contribute to the alveolar macrophage populations (43–45). Our comparison of neonatal and adult BAL macrophages revealed that the dichotomy in the Mafb promoter activity is not affected by the early neonatal versus adult age.
Because the Mafb expression was restricted to ~40% of the alveolar macrophages, we speculated that the Mafb expression pattern might parallel to the expression of other alveolar macrophage-relevant surface markers. The flow cytometry data revealed that both Mafb- and Mafb+ populations exhibit comparable surface expression patterns for CD11b and CD11c. However, mEGFP+ cells exhibited higher MHCII expression, indicating a more robust antigen presentation potential as compared to mTOM+/mEGFP-cells. Mafb expression in macrophages is known to promote their differentiation (46) and maintenance of their M2 phenotype (47, 48). Moreover, Mafb gene expression is often accompanied with the upregulation of MHCII expression (49–51). In human, MAFB gene was reported to be upregulated in fibrotic lung macrophage clusters of patients with Th2-associated diseases such as Idiopathic Pulmonary Fibrosis (IPF) (49), smoking-related lung cancer (52) and SARS-CoV-2 (53). The MafbCre/WTR26mTmG/WT mice repetitively exposed to ozone, a known induced of Th2 inflammation with M2 macrophage predominance (39), exhibited replacement of Mafb- alveolar macrophage populations with Mafb+ macrophages. Interestingly, a double positive mTOM+/mEGFP+ macrophage population with intermediate MHCII expression was identified in ozone-exposed MafbCre/WTR26mTmG/WT mice, suggesting that higher MHCII expression is linked to the robust expression of Mafb in alveolar macrophages. M2 macrophages possess enhanced antigen presentation ability, thus also express MHCII (54). Therefore, the observed high expression of MHCII in Mafb+ macrophages was expected. These data suggest that Th2 tissue environment upregulated the Mafb expression and M2 macrophage activation in mice.
Number of reports suggest that tissue macrophages originate from the bone marrow-derived circulating monocytes (55–57) and that the tissue microenvironment, not the lineage, determine the macrophage morphology and function (58). Accordingly, we hypothesized that the higher degree of Mafb expression in steady-state alveolar macrophages will be observed in mice that are populated with bone marrow-derived macrophages. Our analyses revealed that the majority of BMDMs in vitro are amenable to the Mafb-regulated recombination. Consistent with the in vitro findings, the in vivo model of alveolar macrophages repopulation with BMDMs also revealed Mafb-regulated recombination in ~96% alveolar macrophages. These data suggest that the lineage, not the tissue microenvironment, determines the differential expression of Mafb in alveolar macrophages.
Mafb expression is upregulated in M2 macrophages in vitro (39) and is known to promote anti-inflammatory properties in M2 macrophages (59). We reasoned that if Th2-predominated milieu upregulates the Mafb expression in alveolar macrophages and that, in turn, promotes the Cre-LoxP recombination, the Mafb-Cre strain might be useful for Cre-LoxP-mediated recombination in Th2-associated studies such as allergic asthma or parasitic diseases. Our data demonstrated that the Th2 cytokines, indeed, promote the Mafb-Cre expression, as indicated by increased number of mEGFP+ macrophages. On the other hand, the Th1 stimulation did not increase the proportion of mEGFP+ macrophages. These outcomes were comparable between the in vitro and in vivo stimulation experiments.
Ozone is one of the six criteria environmental pollutants according to the National Ambient Air Quality Standard (NAAQS) (60). The repetitive exposure to ozone results in Th2-mediated responses that promote the M2 alveolar macrophages (35, 36, 61–63). Importantly, ozone exposure results in the robust upregulation of Mafb transcripts in alveolar macrophages (36). Based on these reports, we hypothesized that the ozone exposure will promote the activation of Mafb promoter that, in turn, will induce the Cre-LoxP-mediated recombination in mTmG allele of MafbCre/WTR26mTmG/WT mice. As expected, the proportion of mEGFP+ cells increased remarkably and the presence of mTOM+/mEGFP+ cells suggest the induction of Mafb-regulated mEGFP expression in the originally mTOM+ cells. Our findings indicate that the presence of Th2-predominated responses in the lung airspaces may assist in the high efficiency of Mafb-regulated Cre-LoxP-mediated recombination.
The current study has some limitations as well. First, we were not able to examine alveolar macrophages from aged mice. However, we anticipate that the BAL macrophages from aged mice will have greater proportion of mEGFP+ cells. This speculation is consistent with a previous report that suggest age-associated progressive replacement of the embryonically-derived alveolar macrophages with BMDMs (64, 65). Second, we were not able to demonstrate that the mEGFP+ alveolar macrophages are indeed embryonically derived macrophages and were not populated by BMDMs. Although these limitations will be addressed in future studies, the current findings provide a robust foundation for these forthcoming investigations.
In conclusion, this study presents interesting findings: 1) Mafb gene expression in alveolar macrophages is lineage-dependent; 2) Bone marrow-derived macrophages exhibit robust Mafb expression, in vitro as well as in vivo; 3) Th2, but not Th1, environment promotes the activation of Mafb promoter, in vitro as well as in vivo. Finally, this study provides evidence of the coexistence of two macrophage subpopulations, i.e., Mafb+ and Mafb-, in the lung airspaces. While this dichotomy thwarts the use of Mafb-Cre in the induction of floxed alleles in alveolar macrophages, this strain provides a unique tool to induce gene deletion in alternatively-activated alveolar macrophages in various Th2 disease models.
Data availability statement
The original contributions presented in the study are included in the article/Supplementary Material. Further inquiries can be directed to the corresponding author.
Ethics statement
The animal study was reviewed and approved by Louisiana State University Institutional Animal Care and Use Committee (IACUC).
Author contributions
TV and YS conceived and designed the study. TV and YS performed all the experiments. TV and YS wrote and reviewed the manuscript for intellectual contents. All authors contributed to the article and approved the submitted version.
Funding
The work was supported by NIH R01 (NIEHS Grant # R01ES030125).
Acknowledgments
We thank Dhruthi Singamsetty and Richa Lamichhane with their technical assistance with the bone marrow transplantation experiments.
Conflict of interest
The authors declare that the research was conducted in the absence of any commercial or financial relationships that could be construed as a potential conflict of interest.
Publisher’s note
All claims expressed in this article are solely those of the authors and do not necessarily represent those of their affiliated organizations, or those of the publisher, the editors and the reviewers. Any product that may be evaluated in this article, or claim that may be made by its manufacturer, is not guaranteed or endorsed by the publisher.
Supplementary material
The Supplementary Material for this article can be found online at: https://www.frontiersin.org/articles/10.3389/fimmu.2022.1050494/full#supplementary-material
Supplementary Figure 1 | Mafb- alveolar macrophages are not precursors of Mafb+ alveolar macrophages during steady-state. Representative fluorescent photomicrographs of the cultured BAL macrophage cluster from adult naïve MafbCre/WTR26mTmG/WT mice (n=3), recorded at day 1, day 2, day 3, day 4, and day 5.
Supplementary Figure 2 | Mafb upregulation induces expression of MHCII in alveolar macrophages following ozone exposure of MafbCre/WTR26mTmG/WT mice. (A) Applied Side Scatter (SSC) and Forward Scatter (FSC) to eliminate dead cells and debris. (B) Leukocyte CD45+ cells were identified. (C) GR1+ cells are identified. (D) CD64+ CD24(-) macrophage population is identified (E) mTOM+ and mEGFP+ subpopulations were isolated. Myeloid markers such as CD11c, CD11b, MHCII, were assessed in (F, G) mTOM+, (H, I) mTOM+ mEGFP+ (J, K) mEGFP+ subpopulations. (L) Histogram depicting mean fluorescence intensity (MFI) of MHCII expression in mTOM+, mTOM+/mEGFP+ and mEGFP+ populations. Error bars represent SEM ****p<0.0001 using One-way ANOVA. Data shown are from ozone-exposed MafbCre/WTR26mTmG/WT mice (n=3).
References
1. Long DP, Tan B, Zhao AC, Xu LX, Xiang ZH. [Progress in cre/lox site-specific recombination system in higher eukaryotes]. Yi Chuan (2012) 34:177–89. doi: 10.3724/SP.J.1005.2012.00177
2. Yarmolinsky M, Hoess R. The legacy of nat sternberg: The genesis of cre-lox technology. Annu Rev Virol (2015) 2:25–40. doi: 10.1146/annurev-virology-100114-054930
3. Hamilton DL, Abremski K. Site-specific recombination by the bacteriophage P1 lox-cre system. cre-mediated synapsis of two lox sites. J Mol Biol (1984) 178:481–6. doi: 10.1016/0022-2836(84)90154-2
4. Lee YH, Sauer B, Johnson PF, Gonzalez FJ. Disruption of the c/ebp alpha gene in adult mouse liver. Mol Cell Biol (1997) 17:6014–22. doi: 10.1128/MCB.17.10.6014
5. Gui YS, Wang L, Tian X, Feng R, Ma A, Cai B, et al. SPC-Cre-ERT2 transgenic mouse for temporal gene deletion in alveolar epithelial cells. PloS One (2012) 7:e46076. doi: 10.1371/journal.pone.0046076
6. Chung MI, Hogan BLM. Ager-CreER(T2): A new genetic tool for studying lung alveolar development, homeostasis, and repair. Am J Respir Cell Mol Biol (2018) 59:706–12. doi: 10.1165/rcmb.2018-0125OC
7. Bertin G, Poujeol C, Rubera I, Poujeol P, Tauc M. In vivo Cre/loxP mediated recombination in mouse Clara cells. Transgenic Res (2005) 14:645–54. doi: 10.1007/s11248-005-7214-0
8. Shai SY, Harpf AE, Babbitt CJ, Jordan MC, Fishbein MC, Chen J, et al. Cardiac myocyte-specific excision of the beta1 integrin gene results in myocardial fibrosis and cardiac failure. Circ Res (2002) 90:458–64. doi: 10.1161/hh0402.105790
9. Holzel M, Bovier A, Tuting T. Plasticity of tumour and immune cells: a source of heterogeneity and a cause for therapy resistance? Nat Rev Cancer (2013) 13:365–76. doi: 10.1038/nrc3498
10. Biswas SK, Chittezhath M, Shalova IN, Lim JY. Macrophage polarization and plasticity in health and disease. Immunol Res (2012) 53:11–24. doi: 10.1007/s12026-012-8291-9
11. Roda JM, Sumner LA, Evans R, Phillips GS, Marsh CB, Eubank TD. Hypoxia-inducible factor-2alpha regulates GM-CSF-derived soluble vascular endothelial growth factor receptor 1 production from macrophages and inhibits tumor growth and angiogenesis. J Immunol (2011) 187:1970–6. doi: 10.4049/jimmunol.1100841
12. Xu J, Tao B, Guo X, Zhou S, Li Y, Zhang Y, et al. Macrophage-restricted Shp2 tyrosine phosphatase acts as a rheostat for MMP12 through TGF-beta activation in the prevention of age-related emphysema in mice. J Immunol (2017) 199:2323–32. doi: 10.4049/jimmunol.1601696
13. Becerra-Diaz M, Strickland AB, Keselman A, Heller NM. Androgen and androgen receptor as enhancers of M2 macrophage polarization in allergic lung inflammation. J Immunol (2018) 201:2923–33. doi: 10.4049/jimmunol.1800352
14. Clausen BE, Burkhardt C, Reith W, Renkawitz R, Forster I. Conditional gene targeting in macrophages and granulocytes using LysMcre mice. Transgenic Res (1999) 8:265–77. doi: 10.1023/A:1008942828960
15. Bhattacharyya A, Boostanpour K, Bouzidi M, Magee L, Chen TY, Wolters R, et al. IL10 trains macrophage profibrotic function after lung injury. Am J Physiol Lung Cell Mol Physiol (2022) 322:L495–502. doi: 10.1152/ajplung.00458.2021
16. Ferron M, Vacher J. Targeted expression of cre recombinase in macrophages and osteoclasts in transgenic mice. Genesis (2005) 41:138–45. doi: 10.1002/gene.20108
17. Awad AS, You H, Gao T, Cooper TK, Nedospasov SA, Vacher J, et al. Macrophage-derived tumor necrosis factor-alpha mediates diabetic renal injury. Kidney Int (2015) 88:722–33. doi: 10.1038/ki.2015.162
18. Morris SM Jr., You H, Gao T, Vacher J, Cooper TK, Awad AS. Distinct roles of arginases 1 and 2 in diabetic nephropathy. Am J Physiol Renal Physiol (2017) 313:F899–905. doi: 10.1152/ajprenal.00158.2017
19. Sennello JA, Misharin AV, Flozak AS, Berdnikovs S, Cheresh P, Varga J, et al. Lrp5/beta-catenin signaling controls lung macrophage differentiation and inhibits resolution of fibrosis. Am J Respir Cell Mol Biol (2017) 56:191–201. doi: 10.1165/rcmb.2016-0147OC
20. Farrell HE, Lawler C, Oliveira MT, Davis-Poynter N, Stevenson PG. Alveolar macrophages are a prominent but nonessential target for murine cytomegalovirus infecting the lungs. J Virol (2015) 90:2756–66. doi: 10.1128/JVI.02856-15
21. Todd EM, Zhou JY, Szasz TP, Deady LE, D'Angelo JA, Cheung MD, et al. Alveolar macrophage development in mice requires l-plastin for cellular localization in alveoli. Blood (2016) 128:2785–96. doi: 10.1182/blood-2016-03-705962
22. Hu Y, Wen J, Zhang B, Xiao H. Precision control of mTORC1 is crucial for the maintenance and IL-13 responsiveness of alveolar macrophages. Int Immunopharmacol (2021) 95:107552. doi: 10.1016/j.intimp.2021.107552
23. McCubbrey AL, Allison KC, Lee-Sherick AB, Jakubzick CV, Janssen WJ. Promoter specificity and efficacy in conditional and inducible transgenic targeting of lung macrophages. Front Immunol (2017) 8:1618. doi: 10.3389/fimmu.2017.01618
24. Abram CL, Roberge GL, Hu Y, Lowell CA. Comparative analysis of the efficiency and specificity of myeloid-cre deleting strains using ROSA-EYFP reporter mice. J Immunol Methods (2014) 408:89–100. doi: 10.1016/j.jim.2014.05.009
25. Reindl W, Weiss S, Lehr HA, Forster I. Essential crosstalk between myeloid and lymphoid cells for development of chronic colitis in myeloid-specific signal transducer and activator of transcription 3-deficient mice. Immunology (2007) 120:19–27. doi: 10.1111/j.1365-2567.2006.02473.x
26. Cekic C, Day YJ, Sag D, Linden J. Myeloid expression of adenosine A2A receptor suppresses T and NK cell responses in the solid tumor microenvironment. Cancer Res (2014) 74:7250–9. doi: 10.1158/0008-5472.CAN-13-3583
27. Karsten CM, Laumonnier Y, Eurich B, Ender F, Broker K, Roy S, et al. Monitoring and cell-specific deletion of C5aR1 using a novel floxed GFP-C5aR1 reporter knock-in mouse. J Immunol (2015) 194:1841–55. doi: 10.4049/jimmunol.1401401
28. Wiese AV, Ender F, Quell KM, Antoniou K, Vollbrandt T, Konig P, et al. The C5a/C5aR1 axis controls the development of experimental allergic asthma independent of LysM-expressing pulmonary immune cells. PloS One (2017) 12:e0184956. doi: 10.1371/journal.pone.0184956
29. Dominguez S, Montgomery AB, Haines GK 3rd, Bloomfield CL, Cuda CM. The caspase-8/RIPK3 signaling axis in antigen presenting cells controls the inflammatory arthritic response. Arthritis Res Ther (2017) 19:224. doi: 10.1186/s13075-017-1436-4
30. Osero BO, Cele Z, Aruleba RT, Maine RA, Ozturk M, Lutz MB, et al. Interleukin-4 responsive dendritic cells are dispensable to host resistance against leishmania mexicana infection. Front Immunol (2021) 12:759021. doi: 10.3389/fimmu.2021.759021
31. Mai LT, Smans M, Silva-Barrios S, Fabie A, Stager S. IRF-5 expression in myeloid cells is required for splenomegaly in l. donovani infected mice. Front Immunol (2019) 10:3071. doi: 10.3389/fimmu.2019.03071
32. Soung do Y, Kalinowski J, Baniwal SK, Jacome-Galarza CE, Frenkel B, Lorenzo J, et al. Runx1-mediated regulation of osteoclast differentiation and function. Mol Endocrinol (2014) 28:546–53. doi: 10.1210/me.2013-1305
33. Wu X, Briseno CG, Durai V, Albring JC, Haldar M, Bagadia P, et al. Mafb lineage tracing to distinguish macrophages from other immune lineages reveals dual identity of langerhans cells. J Exp Med (2016) 213:2553–65. doi: 10.1084/jem.20160600
34. Muzumdar MD, Tasic B, Miyamichi K, Li L, Luo L. A global double-fluorescent cre reporter mouse. Genesis (2007) 45:593–605. doi: 10.1002/dvg.20335
35. Choudhary I, Vo T, Paudel K, Yadav R, Mao Y, Patial S, et al. Postnatal ozone exposure disrupts alveolar development, exaggerates mucoinflammatory responses, and suppresses bacterial clearance in developing Scnn1b-tg(+) mice lungs. J Immunol (2021) 207:1165–79. doi: 10.4049/jimmunol.2001286
36. Choudhary I, Vo T, Paudel K, Patial S, Saini Y. Compartment-specific transcriptomics of ozone-exposed murine lungs reveals sex- and cell type-associated perturbations relevant to mucoinflammatory lung diseases. Am J Physiol Lung Cell Mol Physiol (2021) 320:L99–L125. doi: 10.1152/ajplung.00381.2020
37. Choudhary I, Vo T, Bathula CS, Lamichhane R, Lewis BW, Looper J, et al. Tristetraprolin overexpression in non-hematopoietic cells protects against acute lung injury in mice. Front Immunol (2020) 11:2164. doi: 10.3389/fimmu.2020.02164
38. Tan SY, Krasnow MA. Developmental origin of lung macrophage diversity. Development (2016) 143:1318–27. doi: 10.1242/dev.129122
39. Daassi D, Hamada M, Jeon H, Imamura Y, Nhu Tran MT, Takahashi S. Differential expression patterns of MafB and c-maf in macrophages in vivo and in vitro. Biochem Biophys Res Commun (2016) 473:118–24. doi: 10.1016/j.bbrc.2016.03.063
40. Ishizuka EK, Ferreira MJ, Grund LZ, Coutinho EM, Komegae EN, Cassado AA, et al. Role of interplay between IL-4 and IFN-gamma in the in regulating M1 macrophage polarization induced by nattectin. Int Immunopharmacol (2012) 14:513–22. doi: 10.1016/j.intimp.2012.08.009
41. Choudhary I, Vo T, Paudel K, Wen X, Gupta R, Kesimer M, et al. Vesicular and extravesicular protein analyses from the airspaces of ozone-exposed mice revealed signatures associated with mucoinflammatory lung disease. Sci Rep (2021) 11:23203. doi: 10.1038/s41598-021-02256-5
42. Wynn TA, Chawla A, Pollard JW. Macrophage biology in development, homeostasis and disease. Nature (2013) 496:445–55. doi: 10.1038/nature12034
43. Takahashi K, Yamamura F, Naito M. Differentiation, maturation, and proliferation of macrophages in the mouse yolk sac: a light-microscopic, enzyme-cytochemical, immunohistochemical, and ultrastructural study. J Leukoc Biol (1989) 45:87–96. doi: 10.1002/jlb.45.2.87
44. Hoeffel G, Chen J, Lavin Y, Low D, Almeida FF, See P, et al. C-myb(+) erythro-myeloid progenitor-derived fetal monocytes give rise to adult tissue-resident macrophages. Immunity (2015) 42:665–78. doi: 10.1016/j.immuni.2015.03.011
45. Higashi K, Naito M, Takeya M, Ando M, Araki S, Takahashi K. Ontogenetic development, differentiation, and phenotypic expression of macrophages in fetal rat lungs. J Leukoc Biol (1992) 51:444–54. doi: 10.1002/jlb.51.5.444
46. Kelly LM, Englmeier U, Lafon I, Sieweke MH, Graf T. MafB is an inducer of monocytic differentiation. EMBO J (2000) 19:1987–97. doi: 10.1093/emboj/19.9.1987
47. Cuevas VD, Anta L, Samaniego R, Orta-Zavalza E, Vladimir de la Rosa J, Baujat G, et al. MAFB determines human macrophage anti-inflammatory polarization: Relevance for the pathogenic mechanisms operating in multicentric carpotarsal osteolysis. J Immunol (2017) 198:2070–81. doi: 10.4049/jimmunol.1601667
48. Kang K, Park SH, Chen J, Qiao Y, Giannopoulou E, Berg K, et al. Interferon-gamma represses M2 gene expression in human macrophages by disassembling enhancers bound by the transcription factor MAF. Immunity (2017) 47:235–250 e4. doi: 10.1016/j.immuni.2017.07.017
49. Aran D, Looney AP, Liu L, Wu E, Fong V, Hsu A, et al. Reference-based analysis of lung single-cell sequencing reveals a transitional profibrotic macrophage. Nat Immunol (2019) 20:163–72. doi: 10.1038/s41590-018-0276-y
50. Dick SA, Macklin JA, Nejat S, Momen A, Clemente-Casares X, Althagafi MG, et al. Self-renewing resident cardiac macrophages limit adverse remodeling following myocardial infarction. Nat Immunol (2019) 20:29–39. doi: 10.1038/s41590-018-0272-2
51. Kikuchi K, Iida M, Ikeda N, Moriyama S, Hamada M, Takahashi S, et al. Macrophages switch their phenotype by regulating maf expression during different phases of inflammation. J Immunol (2018) 201:635–51. doi: 10.4049/jimmunol.1800040
52. Samir O, Kobayashi N, Nishino T, Siyam M, Yadav MK, Inoue Y, et al. Transcription factor MAFB as a prognostic biomarker for the lung adenocarcinoma. Int J Mol Sci (2022) 23(17):9945. doi: 10.3390/ijms23179945
53. Vega MA, Simon-Fuentes M, Gonzalez de la Aleja A, Nieto C, Colmenares M, Herrero C, et al. MAFB and MAF transcription factors as macrophage checkpoints for COVID-19 severity. Front Immunol (2020) 11:603507. doi: 10.3389/fimmu.2020.603507
54. Murray PJ, Allen JE, Biswas SK, Fisher EA, Gilroy DW, Goerdt S, et al. Macrophage activation and polarization: nomenclature and experimental guidelines. Immunity (2014) 41:14–20. doi: 10.1016/j.immuni.2014.06.008
55. van Furth R, Cohn ZA. The origin and kinetics of mononuclear phagocytes. J Exp Med (1968) 128:415–35. doi: 10.1084/jem.128.3.415
56. Geissmann F, Manz MG, Jung S, Sieweke MH, Merad M, Ley K. Development of monocytes, macrophages, and dendritic cells. Science (2010) 327:656–61. doi: 10.1126/science.1178331
57. Aguzzi A, Barres BA, Bennett ML. Microglia: scapegoat, saboteur, or something else? Science (2013) 339:156–61. doi: 10.1126/science.1227901
58. Naito M, Umeda S, Yamamoto T, Moriyama H, Umezu H, Hasegawa G, et al. Development, differentiation, and phenotypic heterogeneity of murine tissue macrophages. J Leukoc Biol (1996) 59:133–8. doi: 10.1002/jlb.59.2.133
59. Kim H. The transcription factor MafB promotes anti-inflammatory M2 polarization and cholesterol efflux in macrophages. Sci Rep (2017) 7:7591. doi: 10.1038/s41598-017-07381-8
60. Patial S, Saini Y. Lung macrophages: current understanding of their roles in ozone-induced lung diseases. Crit Rev Toxicol (2020) 50:310–23. doi: 10.1080/10408444.2020.1762537
61. Harkema JR, Wagner JG. Innate lymphoid cell-dependent airway epithelial and inflammatory responses to inhaled ozone: A new paradigm in pathogenesis. Toxicol Pathol (2019) 47:993–1003. doi: 10.1177/0192623319873872
62. Kumagai K, Lewandowski RP, Jackson-Humbles DN, Buglak N, Li N, White K, et al. Innate lymphoid cells mediate pulmonary eosinophilic inflammation, airway mucous cell metaplasia, and type 2 immunity in mice exposed to ozone. Toxicol Pathol (2017) 45:692–704. doi: 10.1177/0192623317728135
63. Kumagai K, Lewandowski R, Jackson-Humbles DN, Li N, Van Dyken SJ, Wagner JG, et al. Ozone-induced nasal type 2 immunity in mice is dependent on innate lymphoid cells. Am J Respir Cell Mol Biol (2016) 54:782–91. doi: 10.1165/rcmb.2015-0118OC
64. Bain CC, MacDonald AS. The impact of the lung environment on macrophage development, activation and function: diversity in the face of adversity. Mucosal Immunol (2022) 15:223–34. doi: 10.1038/s41385-021-00480-w
Keywords: MAFB, Cre-LoxP, macrophage-specific Cre, alveolar macrophages, lung
Citation: Vo T and Saini Y (2022) Case report: Mafb promoter activity may define the alveolar macrophage dichotomy. Front. Immunol. 13:1050494. doi: 10.3389/fimmu.2022.1050494
Received: 21 September 2022; Accepted: 25 November 2022;
Published: 12 December 2022.
Edited by:
Teun J. De Vries, VU Amsterdam, NetherlandsReviewed by:
Satoru Takahashi, University of Tsukuba, JapanEkhtear Hossain, Southern University and A&M College, United States
Jagjit S. Yadav, College of Medicine, University of Cincinnati, United States
Copyright © 2022 Vo and Saini. This is an open-access article distributed under the terms of the Creative Commons Attribution License (CC BY). The use, distribution or reproduction in other forums is permitted, provided the original author(s) and the copyright owner(s) are credited and that the original publication in this journal is cited, in accordance with accepted academic practice. No use, distribution or reproduction is permitted which does not comply with these terms.
*Correspondence: Yogesh Saini, ysaini@lsu.edu