- 1Department of Geriatric, Hospital of Chengdu University of Traditional Chinese Medicine, Chengdu, China
- 2The General Hospital of Western Theater Command, Chengdu, China
- 3TCM Regulating Metabolic Diseases Key Laboratory of Sichuan Province, Hospital of Chengdu University of Traditional Chinese Medicine, Chengdu, China
- 4Clinical Medicine Teaching Department, Hospital of Chengdu University of Traditional Chinese Medicine, Chengdu, China
- 5The First Clinical Medical College, Guangxi University of Chinese Medicine, Guangxi, China
- 6Xi’an Hospital of Traditional Chinese Medicine, Xi’an, China
- 7Hengyang Medical School, University of South China, Hengyang, China
Background: Common vaccinations may have impacts on dementia risk, but current evidence is inconsistent. We therefore investigated the association between vaccinations and dementia risk by systematic review and meta-analysis approach.
Methods: We conducted an extensive search of PubMed, Embase, Cochrane Library, and Web of Science to identify studies that compared the risk of dementia in vaccinated versus unvaccinated populations. The adjusted hazard ratio (HR) and corresponding 95% confidence intervals (CIs) were pooled as measures.
Results: Of the 9124 records initially retrieved, 17 studies with 1857134 participants were included in our analysis. The overall pooled results showed that vaccinations were associated with a 35% lower dementia risk (HR=0.65, 95% CI: 0.60-0.71, P overall effect < 0.001; I2 =91.8%, P heterogeneity<0.001). All types of vaccination were associated with a trend toward reduced dementia risk, with rabies (HR=0.43), tetanus & diphtheria & pertussis (Tdap) (HR=0.69), herpes zoster (HR=0.69), influenza (HR=0.74), hepatitis A (HR=0.78), typhoid (HR=0.80), and hepatitis B (HR=0.82) vaccinations being significant. Individuals with more full vaccination types and more annual influenza vaccinations were less likely to develop dementia. Gender and age had no effect on this association.
Conclusion: Routine adult vaccinations are associated with a significant reduction in dementia risk and may be an effective strategy for dementia prevention. Further research is needed to elucidate the causal effects of this association and the underlying mechanisms.
Introduction
Dementia is a syndrome that manifests as progressive cognitive decline and is the seventh leading cause of death among all diseases (1). Age is the greatest known risk factor, and the number of people living with dementia is increasing as the global population ages. More than 55 million people worldwide currently suffer from dementia, and its prevalence almost doubles every two decades, with the total number of people with dementia expected to reach 139 million in 2050 (1). Dementia not only adversely affects the physical and psychological well-being, ability to perform daily living activities, and quality of life of the individual, but also has long-lasting negative effects on their caregivers and families. The enormous burden makes dementia one of the greatest challenges to global health and social care in the 21st century (1, 2).
Unfortunately, to date, with the exception of aducanumab, which was approved by the FDA in 2021 for the treatment of Alzheimer’s disease even if its efficacy is highly debated (3), no therapies have emerged that can reverse or cure dementia, so investigating and identifying measures that may reduce the risk of its onset is critical to managing the disease and has a significant impact on individuals and society. The onset of dementia is often caused by the interaction of lifestyle, environmental factors, and genes; 12 modifiable risk/protective factors have been confirmed, including hypertension, diabetes, hearing impairment, excessive alcohol consumption, smoking, obesity, depression, head injury, air pollution, infrequent social contact, lack of physical activity, and low level of education. Modifying these 12 factors may prevent or delay dementia by 40% (4). Moreover, many other modifiable protective/risk factors for dementia have been identified, such as sleep disturbances, sleep duration, Mediterranean diet, marital status, vitamin D deficiency, and cardiovascular disease (5–10). A growing body of research suggests that the immune system and infections play an important role in the development of dementia; bacterial, fungal, and viral infections may cause neurotoxic inflammation and oxidative stress in the brain, which can lead to neurodegeneration (11–13). In addition, many sources of inflammation, such as periodontal disease, may be involved in the development of dementia (14–16). Thus, vaccination, the most effective and affordable method to prevent and control infectious diseases, may have a positive effect on dementia risk.
For years, public health authorities have wanted to dispel the myth that vaccinated people are more likely to develop Alzheimer’s disease. This is because vaccination stimulates the immune system and thus causes inflammation, which is part of the pathology of Alzheimer’s disease. But a more important reason is that the occasional neuropsychiatric disorders occurring after vaccination are over-reported in the media as a cause-and-effect relationship to appeal to public sentiment (17). A previous meta-analysis has found a significantly lower risk of dementia after influenza vaccination, but this study did not further explore the factors that might influence this association (18). In addition, several studies have examined the association between various other common vaccines, such as shingles, diphtheria, and tetanus vaccines, and the risk of dementia (19–24). Nevertheless, the current evidence on the association between vaccination and dementia risk is inconsistent and there is a lack of studies that have comprehensively explored this topic, so we conducted this meta-analysis and systematic review to investigate the effect of different vaccinations on dementia risk, taking into account the influence of age, sex, and dose.
Materials and Methods
The present systematic review and meta-analysis was conducted according to Preferred Reporting Items for Systematic Reviews and Meta-Analyses (PRISMA 2020) guidelines (25), and the protocol for this study is not registered.
Search Strategy
Two authors extensively searched the PubMed, Embase, Cochrane Library, and Web of Science for relevant studies published from database inception to November 30, 2021. Subject terms (vaccine(s), vaccination, dementia, Alzheimer disease) and corresponding synonyms were combined to form the search strategy. No filters or language restrictions were used. The specific search strategy for each database is provided in the Appendix. We also manually searched reference lists of included studies and relevant reviews to avoid missing additional eligible studies.
Inclusion and Exclusion Criteria
Studies were considered for inclusion if all the following conditions were met: (a) the study subjects were humans. (b) For cohort studies, there was a vaccinated cohort and a non-vaccinated cohort; for case-control studies, the case group was patients with dementia and the control group was not. Dementia was clearly defined, and the use of diagnosis codes from the patient medical records and standard clinical criteria were acceptable. (c) Studies compared the risk of dementia in vaccinated population versus unvaccinated populations. (d) The study design was a longitudinal study, including cohort or case-control studies.
Studies that met any of the following criteria would be excluded: (a) Literature that did not generate raw data such as reviews, meta-analyses, comments. (b) Non-human studies such as animal models and cells. (c) The intervention is a therapeutic vaccine specifically for dementia or Alzheimer’s disease. (d) Case reports, case series, or research which did not establish a temporal relationship between vaccinations and dementia onset, such as cross-sectional study.
Study Selection and Data Extract
Two authors independently browsed the titles and abstracts of the retrieved records. Full-text reading was performed for potentially eligible papers. Excluded papers would be checked again by a third author.
For eligible studies, two authors independently extracted the following information: first author, year of publication, sample size, gender, age, region, population source, study period, type of vaccine, measures of identifying dementia and vaccination, study design, effect size, adjusted confounding factors, and duration of follow-up. Any disagreements that arose during the above process were resolved by consensus among all authors.
Study Quality Assessment
The quality of included studies was assessed using the Newcastle-Ottawa Scale (NOS) (26). For cohort studies, scores were given in terms of selection, comparability, and outcome, while case-control studies were scored in terms of selection, comparability, and exposure, both with a maximum score of nine. The study with scores greater than six was considered high quality studies, otherwise it was considered to be at high risk of bias.
Statistical Analysis
We calculated the pooled hazard ratio (HR) and the corresponding 95% CI for the dementia in the vaccinated population compared to the non-vaccinated population (27). When both adjusted and crude effect sizes were present, the adjusted effect size was used. Heterogeneity among included studies was assessed by Cochran’s Q test and Higgins’ I2 statistics (28). Heterogeneity was considered significant when I2 > 50% or P heterogeneity < 0.05, and results of the random-effects model were reported; otherwise, heterogeneity was considered acceptable, and results from the fixed-effects model were reported. Stratified analyses were performed according to vaccine type, dose, gender, age, and type of dementia. Sensitivity analyses were performed by excluding one study at a time to observe changes in the pooled results. In addition, we compared the results of the fixed-effects and random-effects models to check the stability of the pooled results. Egger’s and Begg’s tests were used to quantitatively assess publication bias, and we also observed the symmetry of funnel plots by visual inspection (29, 30). No publication bias was considered to exist when both Egger’s and Begg’s P-values were greater than 0.05 and the funnel plot was generally symmetrical; otherwise, we assessed the impact of potentially unpublished studies on the results by the trim-and-fill method (31).
All data synthesis and analysis in this study was done using STATA MP/16.0 (Stata Corp LLC, TX, USA). All P values were two-tailed and less than 0.05 was considered significant.
Results
The pre-developed search strategies yielded a total of 9124 potentially relevant papers. After removing 4015 duplicates, the titles and abstracts of the remaining records were screened, and 4739 clearly non-relevant papers were excluded. Full-text reading was performed on 370 papers, and 17 studies were finally included in this systematic review. The study selection process and the reasons for exclusion after full-text reading are presented in Figure 1.
Description of the Included Studies
A total of 17 studies involving 1857,134 participants assessed the association between common vaccinations and subsequent risk of dementia (19–24, 32–43). All included studies were population-based studies with sample sizes ranging from 1290 to 551344. Eight cohorts were from the United States, four from China, and two each from the United Kingdom, Canada, and Israel. Vaccine types evaluated in more than one cohort for association with dementia risk included influenza, herpes zoster, tetanus & diphtheria & pertussis (Tdap), Bacillus Calmette-Guerin (BCG), pneumonia, and poliomyelitis vaccines. Hepatitis A, hepatitis B, typhoid, rabies, yellow fever, cholera, and meningitis vaccines were also evaluated. 13 studies were retrospective cohort studies, one was a prospective cohort study, and three were nested case-control studies. The median/mean follow-up ranged from 2.9 to 10.6 years, with a maximum follow-up of 20 years. The incidence of dementia in almost all studies was identified according to diagnostic codes, while vaccination was identified according to readable codes and drug names. The detailed characteristics of the included studies are presented in Table 1.
The overall level of quality of the included studies was excellent, with NOS scores ranging from 7-9 (Table 2). Most studies considered the effects of sociodemographics, comorbidities, medications, detection bias, and competing events on dementia risk (Table 1).
Overall Association Between Vaccination and Dementia Risk
The heterogeneity test showed substantial heterogeneity among individual studies (I2 =91.8%, P heterogeneity<0.001), so the random-effects model was used. The pooled 17 studies was 0.65 (95% CI: 0.60-0.71, P overall effect < 0.001), suggesting that vaccinations overall reduced the risk of future dementia by 35% (Figure 2).
Subgroup Analyses
We performed stratified analyses according to vaccine type, gender, age, dose, and type of dementia. Subgroup analyses showed that influenza (HR=0.74, 95% CI: 0.63-0.87, P<0.001), herpes zoster (HR=0.69, 95% CI: 0.67-0.72, P<0.001), and Tdap (HR=0.69, 95% CI: 0.58-0.82, P<0.001) vaccinations and subsequent lower dementia risk were significantly associated. The greatest reduction in dementia risk was seen after BCG vaccination, but statistical significance was borderline (HR=0.42, 95% CI: 0.17-1.07, P=0.069). Pneumonia (HR=0.68, 95% CI: 0.41-1.13) and poliomyelitis (HR=0.78, 95% CI: 0.44-1.40) vaccinations also tended to be associated with a lower risk of dementia, but without statistical significance (P=0.137 and 0.406, respectively). Furthermore, Wilkinson et al. explored the association between seven other vaccinations and dementia risk using the Secure Anonymised Information Linkage databank containing the population of Wales, UK. All vaccinations were associated with a reduced risk of dementia, with reduced risk ranging from 18% to 57% among those vaccinated against hepatitis A, hepatitis B, typhoid, and rabies, whereas statistical significance was absent for yellow fever, cholera, and meningitis vaccines. Only studies on influenza vaccine explored the effects of vaccination dose on dementia risk. Individuals who received 1 or 2-3 influenza vaccines during the follow-up period had no reduced risk of dementia compared to unvaccinated individuals, whereas people who received at least 4 annual influenza vaccinations had a 49% reduced risk of dementia (HR=0.51, 95% CI: 0.32-0.80, P=0.003) (Table 3). Moreover, Wiemken et al. found that people who received both Tdap and herpes zoster vaccines had a lower risk of dementia compared to those who received Tdap only or herpes zoster only vaccines (23); Wilkinson et al. found a greater reduced risk of dementia in individuals who received typhoid + hepatitis A vaccinations and hepatitis A + B vaccinations than in those who received only one of these vaccines (20). These findings are suggestive of a dose-response effect. Pooled results did not differ significantly by age, sex, or type of dementia (Table 3).
Evaluation for Publication Bias
The P-values of Begg’s and Egger’s tests were 0.6343 and 0.2127, respectively, indicating a low possibility of publication bias. However, the funnel plot exhibited a slight asymmetry, so publication bias was still suspected (Figure 3A). The trim-and-fill analysis estimated that four hypothetical studies were missing (Figure 3B). When four studies were imputed and added to the meta-analysis, the overall HR was 0.677 (95% CI: 0.598-0.755, P<0.001), indicating that the effect of potentially unpublished studies on the current pooled results was subtle.
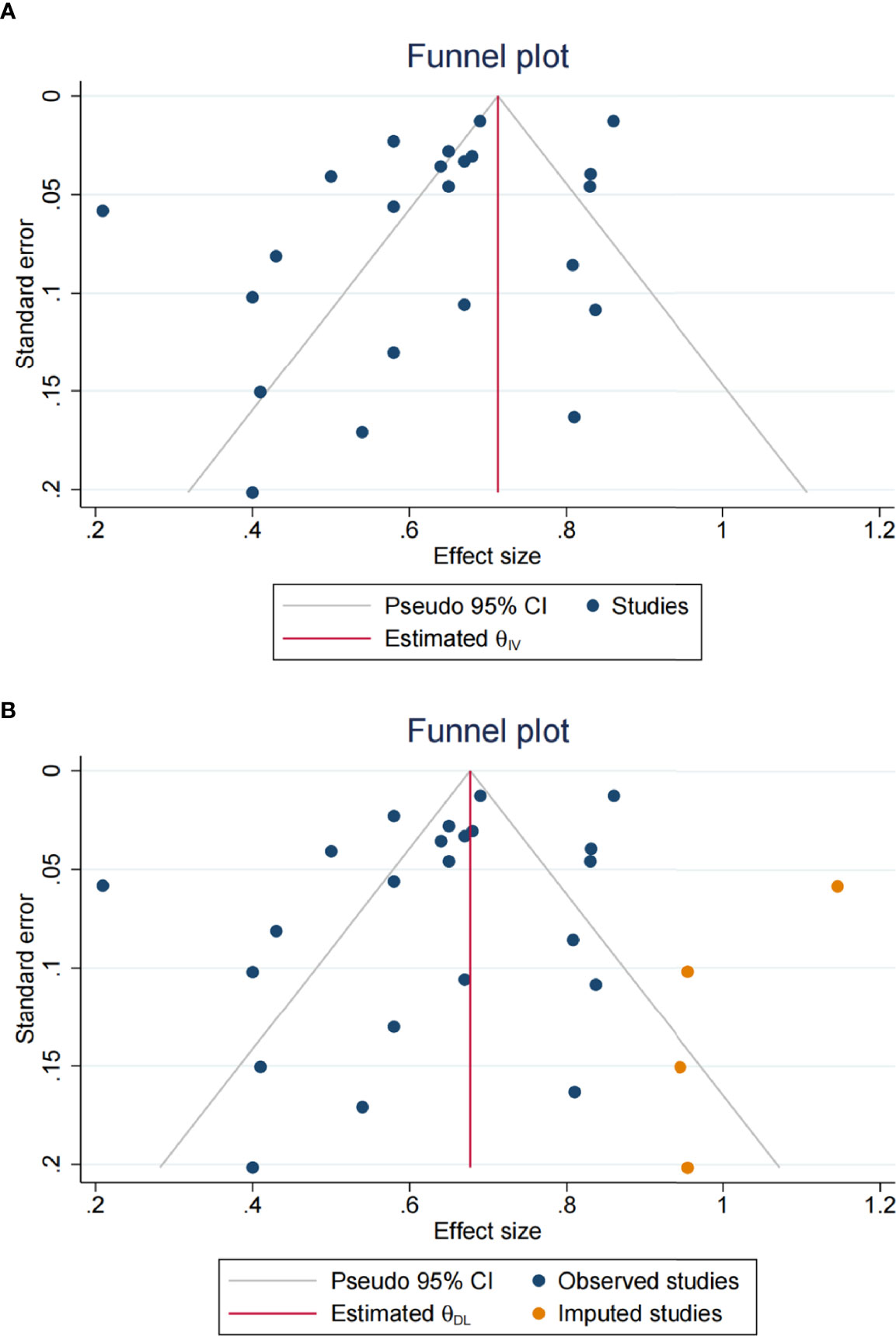
Figure 3 Publication bias assessment. (A) The funnel plot; (B) four hypothetical studies were observed through the trim-and-fill method.
Sensitivity Analysis
We assessed the effect of individual studies on the overall result through excluding one study at a time and then combining the remaining studies. The pooled HR did not change significantly when any single study was excluded, indicating that the current overall result is robust (Figure 4). Furthermore, we examined the robustness of each meta-analysis by comparing the results of random- and fixed-effects models. The conclusions remained unchanged in all groups except for the 2-3 dose and BCG groups where their results shifted from non-significant to significant (Table 4).
Discussion
This systematic review and meta-analysis comprehensively investigated the current evidence regarding the effect of routine adult vaccinations on the risk of dementia, and the pooled results from 17 studies with more than 1.8 million participants showed a 35% reduction in the dementia risk after vaccinations. This reduction was observed to be significant in influenza, herpes zoster, Tdap, hepatitis A, hepatitis B, typhoid, and rabies vaccinations, with similar trends but not significant in other vaccines. The reduced risk did not vary with age, gender, or type of dementia. Compared to the previous meta-analysis on influenza vaccination conducted by Veronese et al, we added four new studies related to influenza vaccination and overall pooled results were similar. Interestingly, we found a dose-response effect of vaccination on the incidence of dementia. More full vaccination types and a greater number of annual influenza vaccinations were associated with a lower risk of dementia.
The exact biological mechanism underlying the association between vaccination and reduced risk of dementia is unclear. One possible explanation is that vaccination can reduce the probability of developing infectious diseases. Studies have shown that infectious events may impair cognition and increase the risk of dementia (44, 45). A large multi-cohort study suggests that infections causing an increased risk of dementia are not limited to central nervous system infections, which suggested that the systemic effects of general inflammation may affect the brain (11). Recent research suggests that even infectious diseases like COVID-19, which is dominated by respiratory symptoms, may still be associated with structural changes in the brain and cognitive decline (46–49). However, the association between vaccinations and dementia risk may not be fully explained by preventing the relevant pathogens, as the efficacy of vaccines against different pathogens is not equal but significant reductions in dementia risk can be observed after almost all types of vaccination. In addition, two studies found no attenuation of the association between vaccinations and reduced risk of dementia after adjusting for infection with vaccine-associated pathogens (23, 24). Hence, another possible theory is the non-specific effects (NSEs), also called off-target effects (50). Vaccinations may enable the body’s immune system to be trained and maintain appropriate immune responses thereby increasing the ability to resist bacteria and viruses from entering the brain and reducing the risk of dementia caused by chronic inflammation and abnormal neuroinflammation. Studies have found that multiple basic vaccinations reduced all-cause mortality in children, which supports the NSEs for vaccines (51). The dose-dependent relationship further supports the NSEs hypothesis. Furthermore, specific vaccines may also affect dementia onset through other unique mechanisms. For example, the influenza vaccine reduces cerebrovascular events, a known risk factor for dementia (52, 53); the herpes zoster vaccine prevents reactivation of herpes virus in the brain; and intravesical BCG instillation significantly increases serum IL-2 levels, which leads to an expansion of the number of neuroprotective Treg cells (54, 55). In fact, previous studies have also shown that influenza and BCG vaccines in animal models can enhance and maintain microglia activation, restore brain immune homeostasis and reduce Aβ burden, ultimately improving cognitive impairment (56, 57). It is therefore possible that these vaccines have preventive benefits by intervening in the pathological process of dementia.
It may also be argued that the apparent protective effect of vaccination simply reflects the different health characteristics and lifestyles between vaccinated population and unvaccinated population, such as better knowledge of disease prevention, higher education and income, and greater social support (58). Of note, most of the included studies adjusted for demographics, comorbidities, and medications use variables. Moreover, several studies considered detection bias and socioeconomic factors such as income, marital status, insurance status, urbanization, and education. Wiemken et al. also explored the effect of health adherence bias, and their findings remained unchanged, with little change in the effect size (23). However, unmeasured or residual confounding may still bias the analyses in the original studies. Dementia is a chronically progressive disease, and mild cognitive decline may exist years before dementia is formally diagnosed. It is also possible that people with reduced cognitive reserve are more likely to neglect vaccination. Wilkinson et al. conducted a stratified analysis based on follow-up time, and interestingly, all vaccine types had lower HRs for dementia ten years after vaccination than five years after vaccination, suggesting that the reverse causality is less likely (20). However, given the lack of prospective randomized controlled studies, the current evidence is not sufficient to conclude any causal effect.
Even if the evidence from observational studies cannot definitively show that vaccinations will prevent dementia, the benefits of timely and full vaccination for adults are still substantial. As the body’s immunity declines with age, especially in people with underlying diseases such as hypertension, coronary heart disease, and diabetes, following the recommended immunization schedule not only prevents vaccine-related infectious diseases, but also prevents the exacerbation of existing chronic diseases due to infections (59). Moreover, a recent nationwide prospective study from Denmark found that patients with dementia had a significantly higher mortality rate following infections compared to people without dementia, more than six times that of people with neither dementia nor infection; this markedly increased risk can persist for up to 10 years (60). Therefore, the full complement of recommended vaccines is critical for both the general elderly and those already suffering from dementia. Vaccinations now recommended by the U.S. Centers for Disease Control and Prevention for people aged≥65 include influenza, Tdap, varicella, zoster, pneumococcal, meningococcal, hepatitis A, hepatitis B, haemophilus influenzae type b, and COVID-19 vaccines (61). Of note, although there are currently no studies on the effect of COVID-19 vaccines on dementia risk, given the multiple neurological complications of SARS-CoV-2, COVID-19 vaccination may be beneficial in preventing cognitive decline, but future studies are needed to confirm this (62). Reports from different regions indicate that coverage of influenza vaccine, the recommended annual vaccine, is low among older adults (63, 64). This suggests the need for specific interventions to increase vaccination coverage in older adults. In addition to making vaccination as a key component of healthy aging, vaccination could also be considered as an important component of dementia prevention strategies, like losing weight, stopping smoking, limiting alcohol, and regular exercise.
Several limitations of this study need to be considered. The number of studies exploring the association between certain vaccine types and dementia risk is small, so it is not clear whether this association varies by region and ethnicity. The populations in some studies were limited to those with specific conditions, such as chronic kidney failure, chronic obstructive pulmonary disease, and bladder cancer. Furthermore, the predominant type of vaccine used against the same pathogens differed across time and regions, for example, there were inactivated, recombinant, and live attenuated influenza vaccines. These may have contributed to the heterogeneity of this meta-analysis. Finally, current findings may be affected by publication bias, but the conclusions remain unchanged after adding hypothetical unpublished studies.
Conclusion
Our study shows that routine vaccinations are significantly associated with dementia risk reduction and more vaccinations have a stronger protective effect on dementia development. Vaccination may be an inexpensive and accessible intervention to prevent cognitive decline and deserve to be incorporated into the management of dementia prevention strategies. Prospective studies and basic experiments are needed to clarify the causal relationship and underlying mechanisms of this association.
Data Availability Statement
The original contributions presented in the study are included in the article/Supplementary Material. Further inquiries can be directed to the corresponding author.
Author Contributions
Conception and design: ZS and ML. Database search, study selection, and data extraction: XW and SH, Statistical analysis: TX and DC. Drafting of manuscript: XW, HY, ML, YZ, and JL. Editing and critical revision of the manuscript: ZS and ML. All authors contributed to the article and approved the submitted version.
Conflict of Interest
The authors declare that the research was conducted in the absence of any commercial or financial relationships that could be construed as a potential conflict of interest.
Publisher’s Note
All claims expressed in this article are solely those of the authors and do not necessarily represent those of their affiliated organizations, or those of the publisher, the editors and the reviewers. Any product that may be evaluated in this article, or claim that may be made by its manufacturer, is not guaranteed or endorsed by the publisher.
Supplementary Material
The Supplementary Material for this article can be found online at: https://www.frontiersin.org/articles/10.3389/fimmu.2022.872542/full#supplementary-material
References
1. World Health Organization. Global Status Report on the Public Health Response to Dementia. In: Geneva World Heal Organ, vol. 251. (2021). Available at: https://www.who.int/publications/i/item/9789240033245.
2. World Alzheimer Report. World Alzheimer Report 2018 | Alzheimer’s Disease International. In: World Alzheimer Rep (2018). p. 1–46. Available at: https://www.alzint.org/what-we-do/research/world-alzheimer-report/.
4. Livingston G, Sommerlad A, Orgeta V, Costafreda SG, Huntley J, Ames D, et al. Dementia Prevention, Intervention, and Care: 2020 Report of the Lancet Commission. Lancet (2020) 390:2673–734. doi: 10.1016/S0140-6736(17)31363-6
5. Shi L, Chen SJ, Ma MY, Bao YP, Han Y, Wang YM, et al. Sleep Disturbances Increase the Risk of Dementia: A Systematic Review and Meta-Analysis. Sleep Med Rev (2018) 40:4–16. doi: 10.1016/j.smrv.2017.06.010
6. Sabia S, Fayosse A, Dumurgier J, van Hees VT, Paquet C, Sommerlad A, et al. Association of Sleep Duration in Middle and Old Age With Incidence of Dementia. Nat Commun (2021) 12:2289. doi: 10.1038/s41467-021-22354-2
7. Sommerlad A, Ruegger J, Singh-Manoux A, Lewis G, Livingston G. Marriage and Risk of Dementia: Systematic Review and Meta-Analysis of Observational Studies. J Neurol Neurosurg Psychiatry (2018) 89:231–8. doi: 10.1136/jnnp-2017-316274
8. Charisis S, Ntanasi E, Yannakoulia M, Anastasiou CA, Kosmidis MH, Dardiotis E, et al. Mediterranean Diet and Risk for Dementia and Cognitive Decline in a Mediterranean Population. J Am Geriatr Soc (2021) 69:1548–59. doi: 10.1111/jgs.17072
9. Chai B, Gao F, Wu R, Dong T, Gu C, Lin Q, et al. Vitamin D Deficiency as a Risk Factor for Dementia and Alzheimer’s Disease: An Updated Meta-Analysis. BMC Neurol (2019) 19:284. doi: 10.1186/s12883-019-1500-6
10. Grande G, Ljungman PLS, Eneroth K, Bellander T, Rizzuto D. Association Between Cardiovascular Disease and Long-Term Exposure to Air Pollution With the Risk of Dementia. JAMA Neurol (2020) 77:801–9. doi: 10.1001/jamaneurol.2019.4914
11. Sipilä PN, Heikkilä N, Lindbohm JV, Hakulinen C, Vahtera J, Elovainio M, et al. Hospital-Treated Infectious Diseases and the Risk of Dementia: A Large, Multicohort, Observational Study With a Replication Cohort. Lancet Infect Dis (2021) 21:1557–67. doi: 10.1016/S1473-3099(21)00144-4
12. Sochocka M, Zwolińska K, Leszek J. The Infectious Etiology of Alzheimer’s Disease. Curr Neuropharmacol (2017) 15:996–1009. doi: 10.2174/1570159x15666170313122937
13. Perry VH, Cunningham C, Holmes C. Systemic Infections and Inflammation Affect Chronic Neurodegeneration. Nat Rev Immunol (2007) 7:161–7. doi: 10.1038/nri2015
14. Sochocka M, Donskow-Łysoniewska K, Diniz BS, Kurpas D, Brzozowska E, Leszek J. The Gut Microbiome Alterations and Inflammation-Driven Pathogenesis of Alzheimer’s Disease—a Critical Review. Mol Neurobiol (2019) 56:1841–51. doi: 10.1007/s12035-018-1188-4
15. Wang XX, Zhang B, Xia R, Jia QY. Inflammation, Apoptosis and Autophagy as Critical Players in Vascular Dementia. Eur Rev Med Pharmacol Sci (2020) 24:9601–14. doi: 10.26355/eurrev_202009_23048
16. Nadim R, Tang J, Dilmohamed A, Yuan S, Wu C, Bakre AT, et al. Influence of Periodontal Disease on Risk of Dementia: A Systematic Literature Review and a Meta-Analysis. Eur J Epidemiol (2020) 35:821–33. doi: 10.1007/s10654-020-00648-x
17. Gasparini R, Panatto D, Lai PL, Amicizia D. The Urban Myth of the Association Between Neurological Disorders and Vaccinations. J Prev Med Hyg (2015) 56:E1–8. doi: 10.15167/2421-4248/jpmh2015.56.1.467
18. Veronese N, Demurtas J, Smith L, Michel JP, Barbagallo M, Bolzetta F, et al. Influenza Vaccination Reduces Dementia Risk: A Systematic Review and Meta-Analysis. Ageing Res Rev (2022) 73:101534. doi: 10.1016/j.arr.2021.101534
19. Tyas SL, Manfreda J, Strain LA, Montgomery PR. Risk Factors for Alzheimer’s Disease: A Population-Based, Longitudinal Study in Manitoba, Canada. Int J Epidemiol (2001) 30:590–7. doi: 10.1093/ije/30.3.590
20. Wilkinson T, Schnier C, Bush K, Rannikmäe K, Lyons RA, McTaggart S, et al. Drug Prescriptions and Dementia Incidence: A Medication-Wide Association Study of 17000 Dementia Cases Among Half a Million Participants. J Epidemiol Community Health (2021) 76:223–9. doi: 10.1136/jech-2021-217090
21. Verreault R, Laurin D, Lindsay J, De Serres G. Past Exposure to Vaccines and Subsequent Risk of Alzheimer’s Disease. Cmaj (2001) 165:1495–8.
22. Scherrer JF, Salas J, Wiemken TL, Jacobs C, Morley JE, Hoft DF. Lower Risk for Dementia Following Adult Tetanus, Diphtheria, and Pertussis (Tdap) Vaccination. J Gerontol - Ser A Biol Sci Med Sci (2021) 76:1436–43. doi: 10.1093/gerona/glab115
23. Wiemken TL, Salas J, Morley JE, Hoft DF, Jacobs C, Scherrer JF. Comparison of Rates of Dementia Among Older Adult Recipients of Two, One, or No Vaccinations. J Am Geriatr Soc (2021) 70:1157–68. doi: 10.1111/jgs.17606
24. Scherrer JF, Salas J, Wiemken TL, Hoft DF, Jacobs C, Morley JE. Impact of Herpes Zoster Vaccination on Incident Dementia: A Retrospective Study in Two Patient Cohorts. PloS One (2021) 16:e0257405. doi: 10.1371/journal.pone.0257405
25. Page MJ, McKenzie JE, Bossuyt PM, Boutron I, Hoffmann TC, Mulrow CD, et al. The PRISMA 2020 Statement: An Updated Guideline for Reporting Systematic Reviews. BMJ (2021) 372:n71. doi: 10.1136/bmj.n71
26. Wells G, Shea B, O’Connell D, Peterson J. The Newcastle-Ottawa Scale (NOS) for Assessing the Quality of Nonrandomised Studies in Meta-Analyses. Ottawa: Ottawa Hosp Res Inst (2000). Available at: http://www.ohri.ca/programs/clinical_epidemiology/oxford.asp.
27. DerSimonian R, Laird N. Meta-Analysis in Clinical Trials. Control Clin Trials (1986) 7:177–88. doi: 10.1016/0197-2456(86)90046-2
28. Higgins JPT, Thomas J, Chandler J, Cumpston M, Li T, Page MJ, et al. Cochrane Handbook for Systematic Reviews of Interventions. Cochrane Handb Syst Rev Interv (2019) 1–694. doi: 10.1002/9781119536604
29. Sterne JAC, Becker BJ, Egger M. The Funnel Plot. Publ Bias Meta-Analysis Prev Assess Adjust (2005) 75–98. doi: 10.1002/0470870168.ch5
30. Egger M, Smith GD, Schneider M, Minder C. Bias in Meta-Analysis Detected by a Simple, Graphical Test. Br Med J (1997) 315:629–34. doi: 10.1136/bmj.315.7109.629
31. Duval S, Tweedie R. Trim and Fill: A Simple Funnel-Plot-Based Method of Testing and Adjusting for Publication Bias in Meta-Analysis. Biometrics (2000) 56:455–63. doi: 10.1111/j.0006-341X.2000.00455.x
32. Wiemken TL, Salas J, Hoft DF, Jacobs C, Morley JE, Scherrer JF. Dementia Risk Following Influenza Vaccination in a Large Veteran Cohort. Vaccine (2021) 39:5524–31. doi: 10.1016/j.vaccine.2021.08.046
33. Luo CS, Chi CC, Fang YA, Liu JC, Lee KY. Influenza Vaccination Reduces Dementia in Patients With Chronic Obstructive Pulmonary Disease: A Nationwide Cohort Study. J Investig Med (2020) 68:838–45. doi: 10.1136/jim-2019-001155
34. Liu JC, Hsu YP, Kao PF, Hao WR, Liu SH, Lin CF, et al. Influenza Vaccination Reduces Dementia Risk in Chronic Kidney Disease Patients: A Population-Based Cohort Study. Med (United States) (2016) 95:e2868. doi: 10.1097/MD.0000000000002868
35. Lee CY, Chang CC, Lin CS, Yeh CC, Hu CJ, Wu CZ, et al. Risk of Dementia in Patients With Periodontitis and Related Protective Factors: A Nationwide Retrospective Cohort Study. J Clin Periodontol (2020) 47:1428–36. doi: 10.1111/jcpe.13372
36. Ju-Chi Liu J-C, Sung L-C, Hao W-R. Influenza Vaccination Reduces Relative Risk of Dementia in Patients With Heart Failure. Eur J Heart Fail (2016) 18:344. doi: 10.1002/ejhf.539
37. Amran A, Lin Y, Kim Y, Bernstam E, Jiang X, Schulz PE. Influenza Vaccination is Associated With a Reduced Incidence of Alzheimer’s Disease. Alzheimer’s Dement (2020) 16:e041693 . doi: 10.1002/alz.041693
38. Lophatananon A, Mekli K, Cant R, Burns A, Dobson C, Itzhaki R, et al. Shingles, Zostavax Vaccination and Risk of Developing Dementia: A Nested Case-Control Study - Results From the UK Biobank Cohort. BMJ Open (2021) 11:e045871. doi: 10.1136/bmjopen-2020-045871
39. Klinger D, Hill BL, Barda N, Halperin E, Gofrit ON, Greenblatt CL, et al. Bladder Cancer Immunotherapy by BCG Is Associated With a Significantly Reduced Risk of Alzheimer’s Disease and Parkinson’s Disease. Vaccines (2021) 9:491. doi: 10.3390/vaccines9050491
40. Kim JI, Zhu D, Barry E, Kovac E, Aboumohamed A, Agalliu I, et al. Intravesical Bacillus Calmette-Guérin Treatment Is Inversely Associated With the Risk of Developing Alzheimer Disease or Other Dementia Among Patients With Non–muscle-Invasive Bladder Cancer. Clin Genitourin Cancer (2021) 19:e409–16. doi: 10.1016/j.clgc.2021.05.001
41. Gofrit ON, Klein BY, Cohen IR, Ben-Hur T, Greenblatt CL, Bercovier H. Bacillus Calmette-Guérin (BCG) Therapy Lowers the Incidence of Alzheimer’s Disease in Bladder Cancer Patients. PloS One (2019) 14:e0224433. doi: 10.1371/journal.pone.0224433
42. Ukraintseva S, Akushevich I, Yashkin A, MattDuan, Arbeev K, Wu D, et al. P4-436: Vaccination Against Pneumonia in the Elderly May Reduce Alzheimer’s Risk. Alzheimer’s Dement (2019) 15:P1469–70:e0224433. doi: 10.1016/j.jalz.2019.06.4108
43. Ukraintseva S, Yashkin A, Duan M, Akushevich I, Arbeev K, Wu D, et al. Repurposing of Existing Vaccines for Personalized Prevention of Alzheimer’s Disease: Vaccination Against Pneumonia may Reduce AD Risk Depending on Genotype. Alzheimer’s Dement (2020) 16:e046751. doi: 10.1002/alz.046751
44. Douros A, Santella C, Dell’aniello S, Azoulay L, Renoux C, Suissa S, et al. Infectious Disease Burden and the Risk of Alzheimer’s Disease: A Population-Based Study. J Alzheimer’s Dis (2021) 81:329–38. doi: 10.3233/JAD-201534
45. Muzambi R, Bhaskaran K, Brayne C, Davidson JA, Smeeth L, Warren-Gash C. Common Bacterial Infections and Risk of Dementia or Cognitive Decline: A Systematic Review. J Alzheimer’s Dis (2020) 76:1609–26. doi: 10.3233/JAD-200303
46. Douaud G, Lee S, Alfaro-Almagro F, Arthofer C, Wang C, McCarthy P, et al. SARS-CoV-2 is Associated With Changes in Brain Structure in UK Biobank. Nature (2022). doi: 10.1038/s41586-022-04569-5
47. Hosp JA, Dressing A, Blazhenets G, Bormann T, Rau A, Schwabenland M, et al. Cognitive Impairment and Altered Cerebral Glucose Metabolism in the Subacute Stage of COVID-19. Brain (2021) 144:1263–76. doi: 10.1093/brain/awab009
48. Toniolo S, Scarioni M, Di Lorenzo F, Hort J, Georges J, Tomic S, et al. Management Group of the EAN Dementia and Cognitive Disorders Scientific Panel. Dementia and COVID-19, a Bidirectional Liaison: Risk Factors, Biomarkers, and Optimal Health Care. J Alzheimers Dis (2021) 82:883–98. doi: 10.3233/JAD-210335
49. Hampshire A, Trender W, Chamberlain SR, Jolly AE, Grant JE, Patrick F, et al. Cognitive Deficits in People Who Have Recovered From COVID-19. E Clin. Med, (2021) 39:101044. doi: 10.1016/j.eclinm.2021.101044
50. Benn CS, Netea MG, Selin LK, Aaby P. A Small Jab - A Big Effect: Nonspecific Immunomodulation By Vaccines. Trends Immunol (2013) 34:431–9. doi: 10.1016/j.it.2013.04.004
51. McGovern ME, Canning D. Vaccination and All-Cause Child Mortality From 1985 to 2011: Global Evidence From the Demographic and Health Surveys. Am J Epidemiol (2015) 182:791–8. doi: 10.1093/aje/kwv125
52. Tsivgoulis G, Katsanos AH, Zand R, Ishfaq MF, Malik MT, Karapanayiotides T, et al. The Association of Adult Vaccination With the Risk of Cerebrovascular Ischemia: A Systematic Review and Meta-Analysis. J Neurol Sci (2018) 386:12–8. doi: 10.1016/j.jns.2018.01.007
53. Raz L, Knoefel J, Bhaskar K. The Neuropathology and Cerebrovascular Mechanisms of Dementia. J Cereb Blood Flow Metab (2016) 36:172–86. doi: 10.1038/jcbfm.2015.164
54. Baek H, Ye M, Kang GH, Lee C, Lee G, Choi DB, et al. Neuroprotective Effects of CD4+CD25+Foxp3+ Regulatory T Cells in a 3xtg-AD Alzheimer’s Disease Model. Oncotarget (2016) 7:69347–57. doi: 10.18632/oncotarget.12469
55. Taniguchi K, Koga S, Nishikido M, Yamashita S, Sakuragi T, Kanetake H, et al. Systemic Immune Response After Intravesical Instillation of Bacille Calmette-Guerin (BCG) for Superficial Bladder Cancer. Clin Exp Immunol (1999) 115:131–5. doi: 10.1046/j.1365-2249.1999.00756.x
56. Yang Y, He Z, Xing Z, Zuo Z, Yuan L, Wu Y, et al. Influenza Vaccination in Early Alzheimer’s Disease Rescues Amyloidosis and Ameliorates Cognitive Deficits in APP/PS1 Mice by Inhibiting Regulatory T Cells. J Neuroinflamm (2020) 17:65. doi: 10.1186/s12974-020-01741-4
57. Cossu D, Ruberto S, Yokoyama K, Hattori N, Sechi LA. Efficacy of BCG Vaccine in Animal Models of Neurological Disorders. Vaccine (2022) 40:432–6. doi: 10.1016/j.vaccine.2021.12.005
58. Jain A, van Hoek AJ, Boccia D, Thomas SL. Lower Vaccine Uptake Amongst Older Individuals Living Alone: A Systematic Review and Meta-Analysis of Social Determinants of Vaccine Uptake. Vaccine (2017) 35:2315–28. doi: 10.1016/j.vaccine.2017.03.013
59. Pandolfi F, Franza L, Todi L, Carusi V, Centrone M, Buonomo A, et al. The Importance of Complying With Vaccination Protocols in Developed Countries: “Anti-Vax” Hysteria and the Spread of Severe Preventable Diseases. Curr Med Chem (2018) 25:6070–81. doi: 10.2174/0929867325666180518072730
60. Janbek J, Taudorf L, Musaeus CS, Frimodt-Møller N, Laursen TM, Waldemar G. Increased Excess Short- and Long-Term Mortality Following Infections in Dementia: A Prospective Nationwide and Registry-Based Cohort Study. Alzheimer’s Dement (2020) 28:411–20. doi: 10.1002/alz.038941
61. Centers for Disease Control and Prevention. Immunization Schedules. Recommended Adult Immunization Schedule for Ages 19 Years or Older, United States (2021). Available at: https://www.cdc.gov/vaccines/schedules/hcp/imz/adult.html (Accessed January 15, 2022).
62. Harapan BN, Yoo HJ. Neurological Symptoms, Manifestations, and Complications Associated With Severe Acute Respiratory Syndrome Coronavirus 2 (SARS-CoV-2) and Coronavirus Disease 19 (COVID-19). J Neurol (2021) 268:3059–71. doi: 10.1007/s00415-021-10406-y
63. Andrew MK, Bowles SK, Pawelec G, Haynes L, Kuchel GA, McNeil SA, et al. Influenza Vaccination in Older Adults: Recent Innovations and Practical Applications. Drugs Aging (2019) 36:29–37. doi: 10.1007/s40266-018-0597-4
Keywords: vaccination, dementia, epidemiology, meta-analysis, systematic review, protective factor
Citation: Wu X, Yang H, He S, Xia T, Chen D, Zhou Y, Liu J, Liu M and Sun Z (2022) Adult Vaccination as a Protective Factor for Dementia: A Meta-Analysis and Systematic Review of Population-Based Observational Studies. Front. Immunol. 13:872542. doi: 10.3389/fimmu.2022.872542
Received: 09 February 2022; Accepted: 04 April 2022;
Published: 03 May 2022.
Edited by:
Francesco Di Lorenzo, Santa Lucia Foundation (IRCCS), ItalyReviewed by:
Sofia Toniolo, University of Oxford, United KingdomCaterina Motta, Santa Lucia Foundation (IRCCS), Italy
Copyright © 2022 Wu, Yang, He, Xia, Chen, Zhou, Liu, Liu and Sun. This is an open-access article distributed under the terms of the Creative Commons Attribution License (CC BY). The use, distribution or reproduction in other forums is permitted, provided the original author(s) and the copyright owner(s) are credited and that the original publication in this journal is cited, in accordance with accepted academic practice. No use, distribution or reproduction is permitted which does not comply with these terms.
*Correspondence: Zhen Sun, c2x6bXNAZm94bWFpbC5jb20=