- 1Post-Graduation Program in Science of Human and Rehabilitation, Federal University of São Paulo (UNIFESP), Santos, Brazil
- 2ENT Research Lab, Department of Otorhinolaryngology –Head and Neck Surgery, Federal University of Sao Paulo (UNIFESP), São Paulo, Brazil
- 3Post-Graduation Program in Health Sciences, Santo Amaro University (UNISA), São Paulo, Brazil
- 4Programa de Pós-Graduação em Patologia Ambiental e Experimental, Universidade Paulista - Unip, São Paulo, Brazil
- 5Laboratory of Clinical and Molecular Virology, Department of Microbiology, Institute of Biomedical Science of the University of São Paulo, São Paulo, Brazil
- 6Scientific Platform Pasteur, University of São Paulo, São Paulo, Brazil
- 7Albert Einstein Institute for Teaching and Research (IIEP), Hospital Israelita Albert Einstein, São Paulo, Brazil
- 8Post Graduate Program in Dentistry, Universidade Cruzeiro Do Sul, São Paulo, Brazil
- 9Department of Periodontology, School of Dentistry, University of Texas Health Science Center at San Antonio, San Antonio, TX, United States
- 10Department of Gastroenterology (LIM07), Faculdade de Medicina, Universidade de São Paulo, São Paulo, Brazil
- 11Division of Clinical Laboratories (LIM 03), Hospital das Clínicas, Faculdade de Medicina, Universidade de São Paulo, São Paulo, Brazil
- 12Post-Graduation Program in Human Movement and Rehabilitation, Unievangélica, Anápolis, Brazil
- 13Post-Graduation Program in Bioengineering, Universidade Brasil, São Paulo, Brazil
Background: Relevant aspects regarding the SARS-CoV-2 pathogenesis and the systemic immune response to this infection have been reported. However, the mucosal immune response of the upper airways two months after SARS-CoV-2 infection in patients with mild/moderate symptoms is still not completely described. Therefore, we investigated the immune/inflammatory responses of the mucosa of the upper airways of mild/moderate symptom COVID-19 patients two months after the SARS-CoV-2 infection in comparison to a control group composed of non-COVID-19 healthy individuals.
Methods: A cohort of 80 volunteers (age 37.2 ± 8.2), including non-COVID-19 healthy individuals (n=24) and COVID-19 patients (n=56) who presented mild/moderate symptoms during a COVID-19 outbreak in Brazil in November and December of 2020. Saliva samples were obtained two months after the COVID-19 diagnosis to assess the levels of SIgA by ELISA and the cytokines by multiplex analysis.
Results: Salivary levels of SIgA were detected in 39 volunteers into the COVID-19 group and, unexpectedly, in 14 volunteers in the control group. Based on this observation, we distributed the volunteers of the control group into without SIgA or with SIgA sub-groups, and COVID-19 group into without SIgA or with SIgA sub-groups. Individuals with SIgA showed higher levels of IL-10, IL-17A, IFN-γ, IL-12p70, IL-13, and IFN-α than those without SIgA. In intergroup analysis, the COVID-19 groups showed higher salivary levels of IL-10, IL-13, IL-17A, and IFN-α than the control group. No statistical differences were verified in the salivary levels of IL-6 and IFN-β. Lower IL-12p70/IL-10 and IFN-γ/IL-10 ratios were found in the control group without SIgA than the control group with SIgA and the COVID-19 group with SIgA.
Conclusion: We were able to present, for the first time, that associations between distinct immunological profiles can help the mucosal immunity to maintain the salivary levels of SIgA in COVID-19 patients two months after the SARS-CoV-2 infection.
Introduction
The World Health Organization (WHO) declared, in March 2020, that humanity was facing a pandemic situation originated by the SARS-CoV-2 virus, which causes the named coronavirus disease 19 or COVID-19 (1). Since then, many studies have been conducted in order to understand not only the pathogenic aspects involved in this viral disease but also how the immune and inflammatory response is induced by this infection (2, 3).
In this respect, as the SARS-CoV-2 is a respiratory virus, and its presence in the airway elicits a local immune/inflammatory response (4) that, in a “controlled situation”, can help the host to clear the virus through the production and release of immunoglobulins, mainly secretory immunoglobulin A (SIgA) and cytokines (5–7). It is broadly accepted that the dysregulated inflammatory response promoted by the SARS-CoV-2 infection drives a cytokine storm, which is closely associated with severe symptoms and viral lethality (8). Therefore, the “dose” of immune/inflammatory responses elicited by SARS-CoV-2 infection seems to be crucial for driving a “good” or “bad” outcome in this disease. In fact, our group previously reported that individuals with severe COVID-19 presented higher levels of SIgA, interferons (IFN) type I (IFN-α and IFN-β) and type II (IFN-γ), and interleukin (IL)-37 in nasopharyngeal and oropharyngeal swabs samples as compared to the groups with mild COVID-19 and individuals with other respiratory infections (9).
In terms of immune/inflammatory responses in the airway mucosa, it has been shown that the presence of a respiratory virus, such as SARS-CoV-2, can trigger the expression of different types of cytokines that can assist not only in the clearance of the pathogenic agent, but can also generate a protective immunity against viral infection (10, 11). Moreover, it is worth mentioning that the analysis of cytokines released on airways mucosa in response to COVID-19 can be useful to define a signature of this infection, which could help to guide medical assistance, medicine development, and patients’ follow-up (12). In this sense, we have demonstrated that COVID-19 patients presenting mild symptoms showed significant correlations between different types of cytokines. One of the most interesting positive correlations found was between the levels of SIgA and IL-17A (9).
According to the literature, the production and release of cytokines by the upper airway’s mucosa, in response to respiratory infection, can elicit different immune response profiles such as Th1, Th2, and Th17, which can help in the production of mucosal specific-SIgA against the infective agent (10). Among some SIgA features, it is paramount to point out that it was reported that reduction in SIgA levels is closely associated with illness severity (13). Secretory immunoglobulin A is considered as the “first line of defense” against many different pathogens due to its capacity to directly bind and inhibit many pathogenic agents in the mucosa (14–16). Corroborating this important action, a lower level of SIgA in the airways mucosa is related to a higher risk to present upper respiratory infections (URTI) (17), particularly by a virus (18).
In terms of mucosal immunity in the upper airways, it was reported that both the oral cavity and nasal passages contain higher frequencies of sIgA+ B-cells, which suggests a similarity between these mucosal effector tissues. Based on this fact, the use of saliva is useful to evaluate not only diseases and conditions in the oral cavity, but also as well as the systemic health of individuals (19–21). These affirmations are especially valid for the immune/inflammatory response in the mucosa of the upper airways considering that saliva is an easily accessible external fluid that can be used to measure the antigen-specific SIgA antibodies following immunological challenges, such as infection and immunization, in both human and experimental animal models (14). Beyond the presence of antibodies, saliva is a biofluid composed of other biomolecules, including different types of cytokines (22, 23). Based on this information, investigation about the immune and inflammatory responses in the mucosa of the upper airway against the COVID-19 not only can improve our knowledge concerning this disease, but can allow us to better understand why some individuals are asymptomatic or why the symptomatic develop mild, moderate, or severe COVID-19. Furthermore, it is worthy to note that, until now, the overwhelming majority of studies that aimed to evaluate the antibodies and cytokines profile in COVID-19 patients were focused on systemic aspects, mainly in patients with severe disease, whom, in general, presented the cytokine storm. Therefore, in the present study, we evaluated the immune/inflammatory responses in the mucosa of the upper airway, particularly assessing SIgA and cytokines, two months after SARS-CoV-2 infection in a group of patients who presented mild/moderate symptoms in comparison to a control group composed of healthy individuals.
Materials and Methods
Subjects of the Study
In the present study, 80 individuals (mean age 37.2 ± 8.2), 28 men and 52 women, were enrolled. The volunteers were distributed into two groups: the control group (n=24) was composed of non-COVID-19 healthy individuals and the COVID-19 group (n=56) was composed of individuals infected with SARS-CoV-2, who presented mild/moderate symptoms between the months of November and December of 2020, during a COVID-19 outbreak in Brazil. We clarify that on this occasion none of the volunteers were submitted to the vaccination for COVID-19. All the participants were informed about the study and signed the informed consent form previously approved by the Ethics Committee of the Albert Einstein Hospital (number 4.159.565). It is noteworthy to highlight that both the study and all experiments were performed in accordance with the Declaration of Helsinki. Data concerning age, gender, and clinical parameters, including the COVID-19 symptoms, are shown in Table 1.
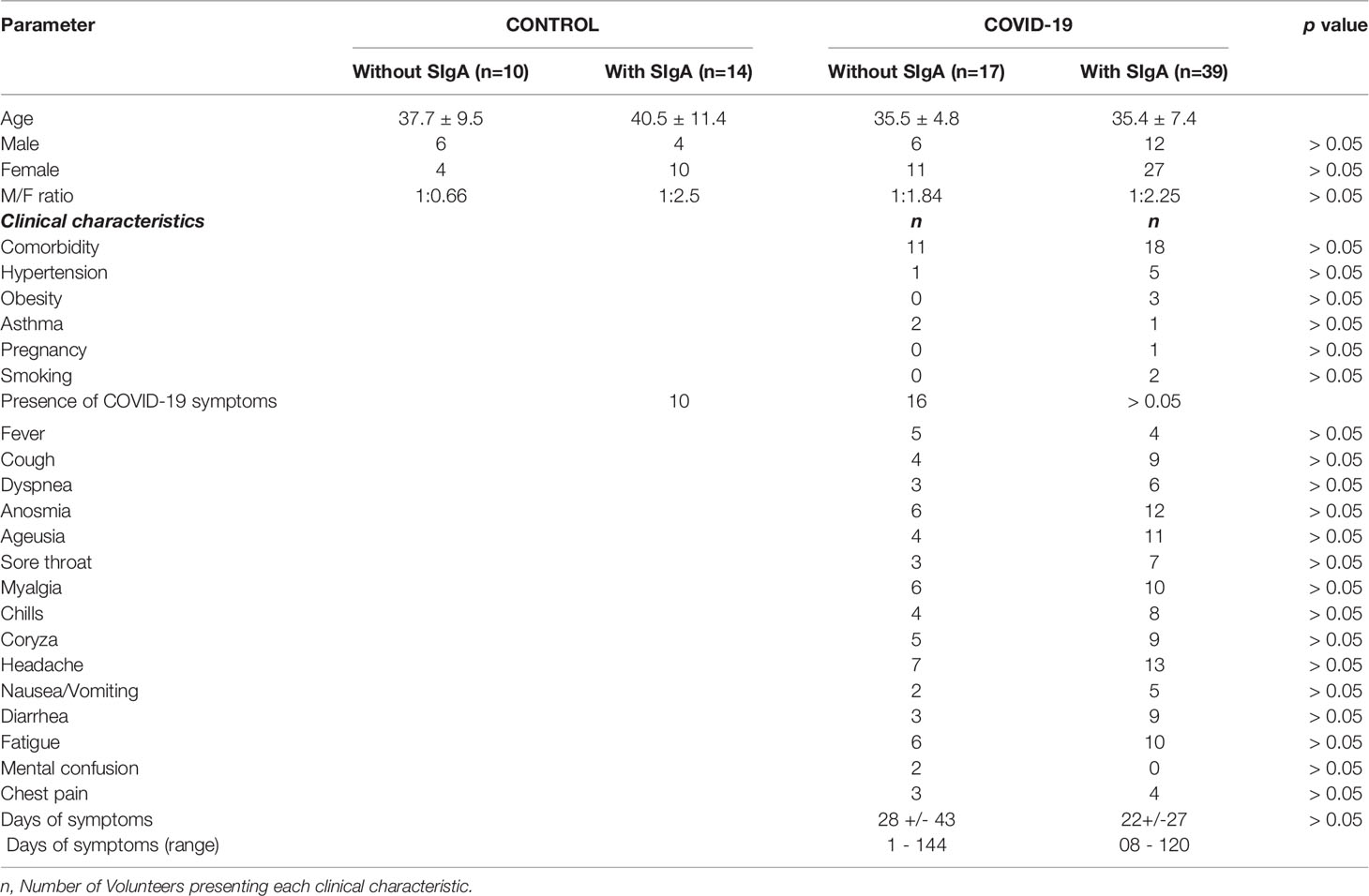
Table 1 Demographic, clinical characteristics, and symptoms presented by the patients who composed the COVID-19 sub-groups without or with SIgA.
Determination of Virus Infection by RT-PCR
The diagnosis for SARS-Cov-2 infection was carried out by real-time (RT) PCR using nasal/oropharyngeal samples obtained in the individuals of the COVID-19 group and healthy individuals. The RT-PCR kits XGEN MASTER COVID-19 (Mobius, Pinhais, Paraná, Brazil); COBAS® SARS-CoV-2 Test (Roche Molecular Systems, Branchburg, NJ, USA); Xpert®Xpress SARS-CoV-2 (Cepheid, Sunnyvale, CA, USA); and Abbott RealTime SARS-C0V-2 (Abbott Molecular Inc.,DesPlaines, IL,USA) were used following manufacturer’s instructions (24–32).
Saliva Sampling
Unstimulated saliva was self-collected by the COVID-19 patients or healthy non-COVID-19 volunteers in a sterile 50-mL tube as previously described (33) in the “Hospital Israelita Albert Einstein” located in São Paulo, Brazil. Regarding the COVID-19 group, the samples were obtained 55 to 60 days after the COVID-19 diagnosis. In relation to the healthy non-COVID-19 volunteer group, none of them presented SARS-CoV-2 infection on the salivary sample collection day. All saliva samples were centrifuged at 3000 rpm for 5 min and the supernatants were stored in -80°C to perform cytokines and secretory immunoglobulin A assays. The volunteers enrolled in this study presented good oral health conditions at the moment of salivary samples collection. In addition, it is noteworthy to point out that all the volunteers were submitted for clinical examination in order to attest to their good health status before the saliva sampling.
Determination of Secretory Immunoglobulin A (SIgA)
Secretory IgA immunoglobulin (SIgA) was detected by ELISA in-house test, which was previously standardized, in order to define not only the optimum concentration of SARS-CoV-2 antigens but also the better saliva samples dilution. Briefly, 96-well plates (Corning, New York, USA) were coated with a mixture of antigens (0.12 ug/mL in sodium carbonate–sodium bicarbonate buffer) from nCoV-PS-Ag7 (Fapon Biotech Inc., Dongguan, China) containing the nucleoprotein (N), membrane (M) and spike (S) and was incubated overnight. Unspecific binding of antibodies was avoided by blocking with the buffer PBS-BSA-T containing 1% of bovine serum albumin (Invitrogen by Thermo Fisher Scientific, Vienna, Austria) in PBS (1X, pH: 7.3) + 0.05% of Tween 20 (Synth, Diadema, Brazil) at 37°C for 2h. After washing three times with a PBS-T solution (PBS 1X, pH:7.3 + 0.05% of Tween), 100μL of saliva [diluted at 1:2,000 in PBS-BSA (PBS 1X, pH: 7.3 + 0.1% of BSA)] was added and incubated for 2h at 37°C. After washing three times with a PBS-T solution, it was added the secondary antibody conjugated with horseradish peroxidase diluted at 1:2,000 (in PBS-BSA) of goat anti-human IgA (Sigma-Aldrich Co., Deisenhofen, Germany). After incubation for 1h at 37°C and three PBS-T washes, 100 μL of TMB solution (3.3′.5.5′- tetramethylbenzidine. Thermo Scientific, Massachusetts, USA) was added and incubated for 10 min at room temperature, avoiding direct exposure to light. The reaction was stopped by adding a solution of sulfuric acid (0.2 N) to each well, and the optical density at 450nm was measured.
Determination of Cytokines
Cytokine concentrations were determined in the saliva samples by a multiplex assay (LEGENDplex™ bead-based multiplex assays, Biolegend, San Diego, CA, USA). The biomarkers assessed were: IL-6, IL-10, IL-12p70, IL-13, IL-17A, IFN-α, IFN-β, and IFN-γ following the manufacturer’s instructions. In this regard, all salivary samples were initially diluted 2-fold and after 25microL of the sample diluted was used to perform this assay. The concentration of cytokines was calculated using appropriate standard curves (following instructions from manufacturers). The linearity of the multiplex assay was within the 2.4–10,000pg/mL range, which includes the range of sample determinations. The correlation coefficients of all standard curves were ranged from 0.95 to 0.99, while intra-assay variance coefficients were 3–5%, and interassay variance coefficients were 8–10%. Analysis was performed with the BD Accuri™ C6 Plus Flow Cytometer (BD Biosciences San Jose. CA. USA) and the data obtained were analyzed with LEGENDPlex™ V8.0 software (Biolegend).
Statistical Analysis
All data obtained from the SIgA and cytokines analysis were initially compared with the Gauss curve and the normality for each parameter assessed was determined by the Shapiro-Wilk test, followed by the homogeneity of variance analysis by the Levene test. Salivary concentrations of SIgA and the cytokines in the volunteer groups were analyzed using the Mann-Whitney test and were presented as the median with the respective quartiles. In addition, the correlation test was performed by Spearman’s test. Differences between age, number of men and women, and the salivary concentrations ratio between IL-10 and the other cytokines were analyzed using the Student T-test and were presented as mean and standard deviation, whereas the differences between the clinical characteristics and symptoms were evaluated using the Chi-square test. Significance was established with α risk at 5.0% level (p ≤ 0.05) and all the analysis was performed data using GraphPad Prism (version 8.1.2) software.
Results
As shown in Figure 1, some volunteers in the control group did not present SIgA for SARS-Cov-2 antigens (n=10) as expected, whereas other volunteers presented this antibody in saliva (n=14). It is worthy to note that, as mentioned in the “Material and Methods” section, these healthy volunteers were allocated to the control group based on the observation that all of them were RT-PCR negative for COVID-19. In addition, the volunteers infected by SARS-Cov-2 were also separated into two groups in accordance with the presence of SIgA: COVID-19 with SIgA (n=39) and COVID-19 without SIgA (n=17). Significant differences were found in the intragroup analysis between the volunteers in the subgroups control (p = 0.002) or COVID-19 (p < 0.0001). Moreover, in the intergroup analysis, the salivary SIgA levels observed in the COVID-19 group were higher than the levels found in the control group (p = 0.04).
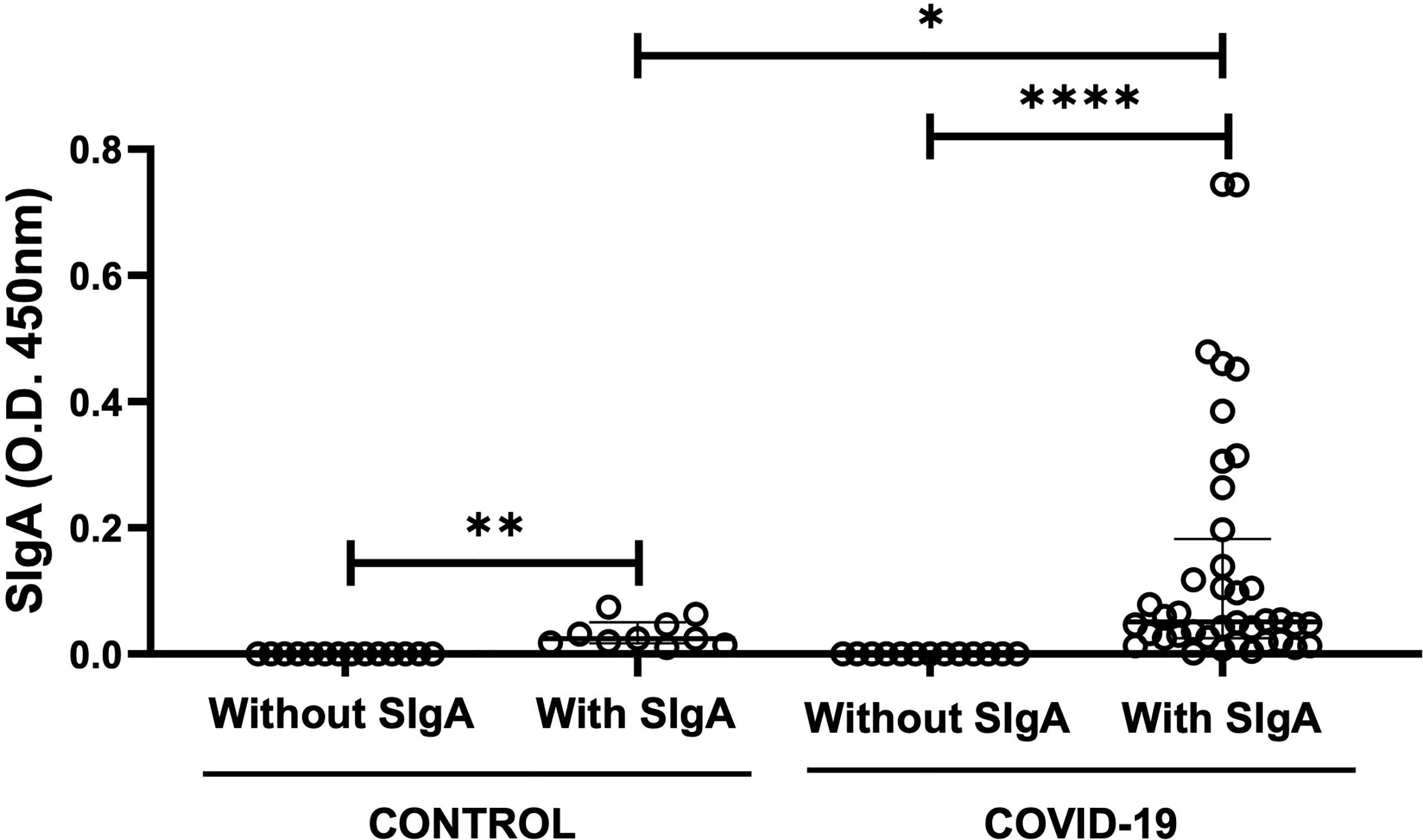
Figure 1 Comparison of the levels of salivary specific-SIgA for SARS-CoV-2 between control and COVID-19 groups with or without the presence of SIgA. The level of significance was established at 5% (*p < 0.05; **p < 0.05; ****p < 0.0001).
Since the groups were distributed due to the presence or absence of SIgA in saliva, Table 1 presents the number of individuals allocated in the control group without SIgA or with SIgA, as well as in the COVID-19 group without SIgA or with SIgA. In addition, it presents the age, the number of men and women, and its ratio in each volunteer group along with the comorbidities and symptoms found in COVID-19 groups during the SARS-CoV-2 infection. In a general way, no differences were observed in these parameters.
Figure 2 shows the analysis of salivary cytokines, both pro (IL-6, IL-12p70, IL-13, IL-17A, IFN-α, IFN-β, and IFN-γ) and anti-inflammatory (IL-10), in the volunteers enrolled in the present study. Concerning the results obtained in the intragroup evaluation, it was found that the control group with SIgA presented higher levels of IL-10 (Figure 2B), IL-17A (Figure 2E), and IFN-γ (Figure 2H), as well as lower levels of IL-13 (Figure 2D) than the values observed in the control group without SIgA. In a similar way, the COVID-19 group with SIgA presented higher levels of IL-10 (Figure 2B) and IFN-γ (Figure 2H) than the values found in the COVID-19 group without SIgA. However, increased salivary levels of IL-12p70 (Figure 2C), IL-13 (Figure 2D), and IFN-α (Figure 2F), and no differences in IL-17 levels (Figure 2E) were also observed in the COVID-19 group with SIgA as compared to the results obtained in the COVID-19 group without SIgA. In addition to these findings, it was observed in the intergroup analysis that the COVID-19 group without SIgA showed higher levels of IL-10 (Figure 2B), IL-13 (Figure 2D), and IL-17A (Figure 2E) than the control group without SIgA. In relation to the groups with SIgA, increased levels of IL-10 (Figure 2B), IL-13 (Figure 2D), IL-17A (Figure 2E), and IFN-α (Figure 2F) were found in the COVID-19 group as compared to the values observed in the control group. No statistical differences were verified in the salivary levels of IL-6 (Figure 2A) and IFN-β (Figure 2G).
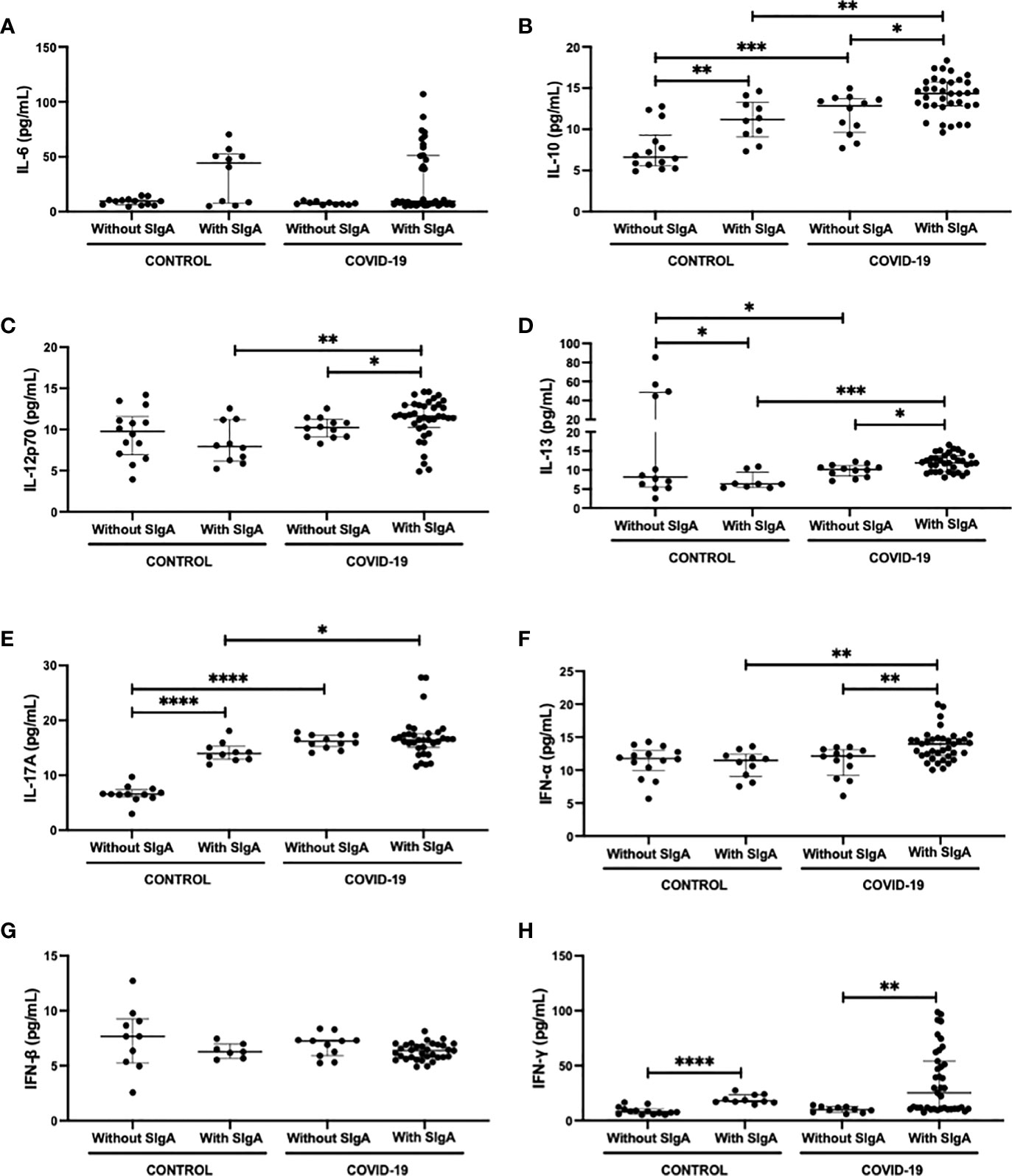
Figure 2 Comparison of the salivary cytokines levels of IL-6 (A), IL-10 (B), IL-12p70 (C), IL-13 (D), IL-17 (E), IFN-α (F), IFN-β (G), and IFN-γ (H) between control without or with SIgA and COVID-19 without or with SIgA group. Values are presented in the median and interquartile range. Statistical analysis: Mann-Whitney test. The level of significance was established at 5% (*p < 0.05; **p < 0.01; ***p < 0.001; ****p < 0.0001).
Table 2 presents the results obtained in the Spearman coefficient correlation analysis. Interestingly, a positive correlation between IFN-α and IL-12p70 was verified in all volunteer groups. In a different way, whereas IFN-α showed a positive correlation with IL-6 in the control group without SIgA, the IFN-α showed a positive correlation with IL-10 in the control group with SIgA, with IFN-γ in the COVID-19 group with SIgA, and with IL-13 in both COVID-19 groups. Concerning IL-6, it was found a positive correlation with IL-12p70 in the control group without SIgA, whereas the control group with SIgA IL-6 showed negative correlations with IL-13 and IFN-γ. Particularly in the COVID-19 groups, IL-6 presented a positive correlation with IFN-γ and also IL-12p70 presented a positive correlation with IL-17A. In addition, the groups that presented SIgA showed a positive correlation between IL-12p70 and IL-10. Another cytokine that showed significant correlations in the groups presenting SIgA was IL-13, but with different cytokines, since in the control group with SIgA, a negative correlation with IFN-β was observed, and in the COVID-19 group with SIgA, two other positive correlations were evidenced, with IFN-γ and IL-17A. Lastly, the COVID-19 group without SIgA exclusively showed a positive correlation between IL-12p70 and IL-13, whereas the COVID-19 group with SIgA presented positive correlations between the levels of SIgA and IFN-γ, as well as IL-17A.
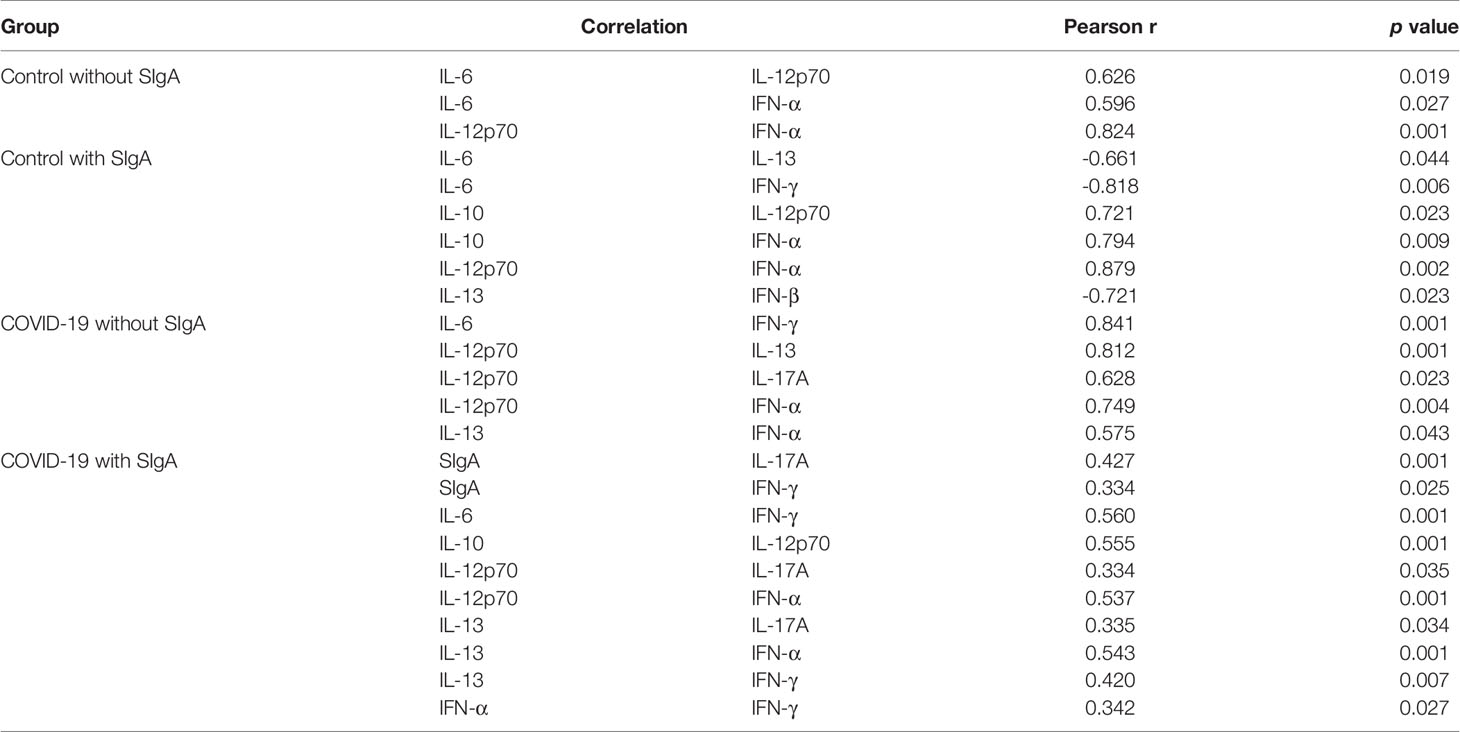
Table 2 Significant correlations between analysis of SIgA and cytokines of COVID-19 and control with and without SIgA groups.
Figure 3 shows the analysis of the ratio between salivary levels of IL-10 and the other pro-inflammatory cytokines assessed in this study. It was found a significant decrease in the IL-12p70/IL-10 ratio (Figure 3B) between the control group without SIgA and the control group with SIgA, as well as in the IFN-γ/IL-10 ratio (Figure 3G) between the control group without SIgA, the control group with SIgA, and the COVID-19 group with SIgA. No other differences were observed.
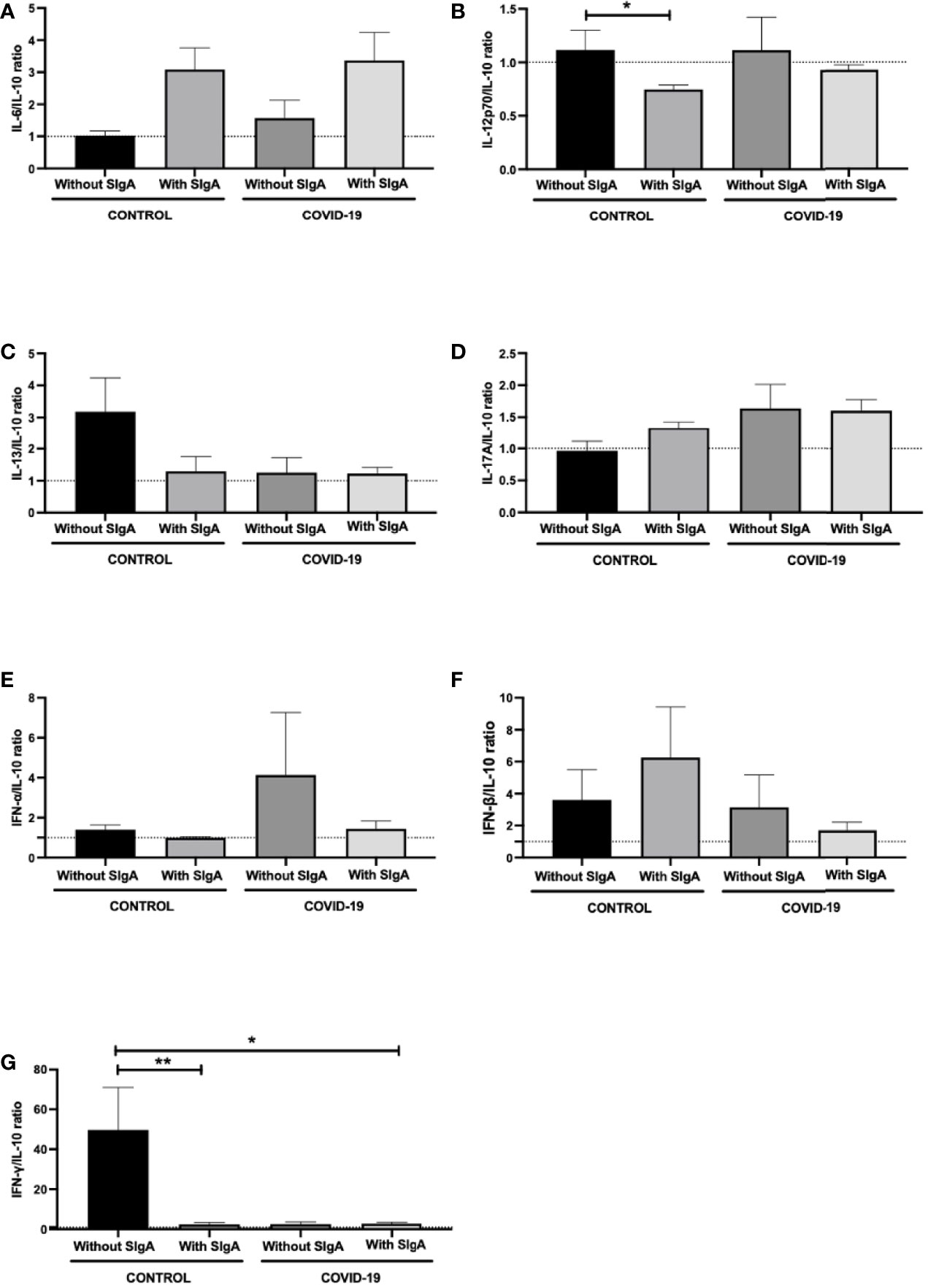
Figure 3 Comparison of the salivary cytokines ratios of IL-6/IL-10 (A), IL-12p70/IL-10 (B), IL-13/IL-10 (C), IL-17/IL-10 (D), IFN-α/IL-10 (E), IFN-β/IL-10 (F), and IFN-γ/IL-10 (G) between control without or with SIgA and COVID-19 without or with SIgA group. Values are presented in the median and interquartile range. Statistical analysis: Kruskal Wallis test. The level of significance was established at 5% (*p < 0.05; **p < 0.01).
Figure 4 summarizes all data found in the volunteer groups of this study.
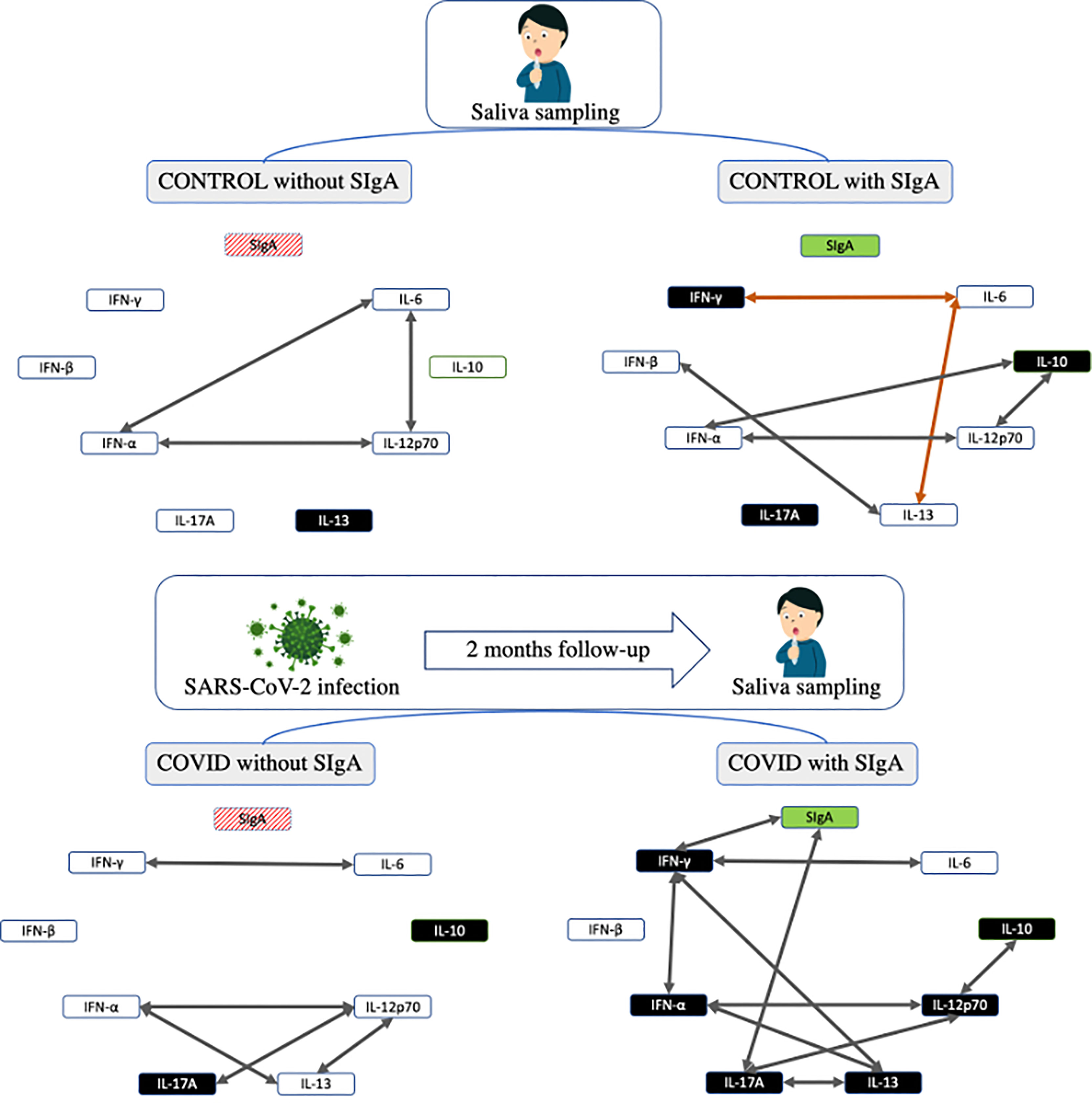
Figure 4 Summarized representation of the main results found in the study. The boxes representing the cytokines are colored in black color indicating an increase in their concentration when compared to the other groups. The arrows represent the correlations found in the study, in which positive correlations appear in gray and negative in orange.
Discussion
The present study shows, for the first time, that two months after SARS-CoV-2 infection, the COVID-19 group with SIgA showed increased salivary SIgA levels than the control group with SIgA, as well as pro-inflammatory cytokines, such as IL-12p70, IL-13, IL-17A, IFN-α, and IFN-γ, and the anti-inflammatory cytokine IL-10, as compared to the other groups. In an interesting way, no differences in the salivary levels of IL-6 and IFN-β were verified between the groups. Furthermore, the correlation analysis demonstrated remarkable associations in all volunteer groups, highlighting the results obtained in the COVID-19 group with SIgA.
Corroborating to the present study, our group (9) has previously demonstrated a significant positive correlation between IL-17A and SIgA in COVID-19 patients presenting mild/moderate symptoms. According to the literature, IL-17A is one of the major cytokines involved in the Th17 immune response, including the upper airway mucosal immunity, by improving its protection in association with a specific-SIgA response (10). Hence, it was reported that IL-17 can enhance the expression of the polymeric immunoglobulin receptor (pIgR), which is responsible to mediate the transport of polymeric immunoglobulins such as SIgA, across the mucosal epithelial cells, delivering this antibody to the mucosal surface (14). In fact, it is utmost of importance to highlight that IL-17 is associated with an upper airway mucosal protective immunity since its neutralization impaired the immune response against not only nasal vaccination but also influenza infection (10, 34, 35). In a recent review, Hoffmann et al. (2021) (36) showcased the corollary action of IL-17 in the airway’s viral infection and secondary bacterial infections (37). In this context, in association with IL-22, IL-17 can also regulate the function of the epithelial barrier and mediate host response to infections (38–40) by inducing the production of antimicrobial proteins and mucus, recruitment of monocytes and neutrophils, favoring tight junction formation, and the mucosal repair (41–44). Moreover, it has been reported that the improvement of secondary bacterial infections clearance during influenza infection was related to IL-17 production (45). In line with these findings, Smith and collaborators (14) declared that “the strategic positioning of TH17 cells at barriers surfaces reflects their importance in the neutralization of pathogens”. So, as COVID-19 is also a respiratory disease, the production of IL-17A in the upper airway of individuals with SARS-Cov-2 infection can be crucial to elicit an immune response that limits disease severity (36), particularly by inducing specific-SIgA for COVID-19 as observed in the present study.
Based on the previous report of Mahallawia and collaborators (2018) (44), in which it was shown that patients with acute MERS-CoV infection presented both Th1 and Th17 responses, our findings show a significant positive correlation between IL-12p70, a classical Th1 cytokine, and IL-17 in the COVID-19 groups. Beyond that, higher levels of IL-12p70 and IL-17 were also observed in the COVID-19 group with SIgA compared to the COVID-19 group without SIgA. Therefore, it shows that the SARS-CoV-2 infection can elicit a similar immune response denoted by MERS-CoV. In terms of mucosal immunity, IL-12p70 is a cytokine that not only can induce interferon production (46), but can also act directly in B cells (47) stimulating their growth and immunoglobulin secretion (48, 49). Moreover, it was demonstrated that IL-12 can be important to enhance the pIgR expression on upper airways (50) and also that the nasal immunization with this cytokine was able to increase antigen-specific antibody response (14). Taking these data together, we can hypothesize that the concomitant increase of IL-12p70 with IL-17A observed in the COVID-19 group with SIgA could be an important feature in order to promote an efficient stimulation of the immune response in the airway’s mucosa, leading to SIgA production. However, this association between the salivary levels of IL-12p70 and IL-17A, seemingly, is not the unique factor able to induce the SIgA production in COVID-19 patients, since the COVID-19 group without SIgA did also present the same correlation.
In this sense, another interesting finding in the samples collected after two months of SARS-CoV-2 infection in the COVID-19 group with SIgA was not only the increased IL-13 levels but also the positive correlation between IL-13 and IL-17. Although elevations in the plasma levels of both IL-13 and IL-17, among other cytokines, were observed in symptomatic patients infected by SARS-CoV-2 as compared to controls (51), higher IL-13 levels in saliva of COVID-19 patients presenting SIgA than COVID-19 patients without SIgA or control with SIgA has not been reported before. It is broadly known that IL-13 is a cytokine involved in the Th2 immune response and its elevation is closely associated with several respiratory diseases, such as asthma, COPD, polyposis, and allergy, notably involved in hypersecretion of mucus in such diseases. However, it is also accepted that some Th2-derived cytokines, including IL-13, are able not only to induce IgA+ B cells proliferation, but also the differentiation of these cells into IgA-secreting plasma cells (52), which can promote the increase of SIgA production in the mucosa.
In terms of viral infection, it was demonstrated that the presence of innate lymphocyte cells (ILC), a type of immune cell that shares many characteristics of the CD4+ T helper cell but without adaptive immunity receptors/lymphoid lineage expression, can induce the production of IL-13 (53, 54) when it presents the Th2 profile (called ILC2) in the respiratory tract. Concerning ILC2 cells, the number of these cells increased in the mucosa. particularly in the intestines, lungs, and tonsils (55), playing a prominent role in the maintenance of the epithelial cell barrier since its depletion led to profound damages in the epithelial barrier following H1N1 infection (56). Also, it was demonstrated that in an H1N1 infection there was an accumulation of ILC2 cells in the lung, regardless of viral load (57). Regarding ILC cells profiles, as previously mentioned, ILC2 cells were associated with a Th2 immune profile characterized by the production of IL-13, whereas type 1 ILC (ILC1) cells produce interferon-gamma (IFN-γ), type 3 ILC (ILC3) cells IL-17A, and IL-22 (58). However, recently it was reported that ILC2 cells also can transdifferentiate to an ILC3-like cell and produce IL-17, which shows that the ILC2 cells in the airways mucosa present remarkable plasticity and can contribute with ILC3 cells in IL-17 production following infection. Therefore, the positive correlation between IL-13 and IL-17 could be putatively attributed to ILC2 cells responses, which lead to the improvement of the mucosa protective immune response, particularly by eliciting the SIgA production.
Corroborating this suggestion that the association between IL-13 and IL-17 can improve the conditions to promote SIgA production in COVID-19 patients, including two months after SARS-CoV-2 infection, we found that the COVID-19 group without SIgA did not show this same association. Instead, the COVID-19 group without SIgA showed an exclusive positive correlation between the salivary levels of IL-13 and IL-12p70.
According to the classical immunological knowledge, both in physiologic or even some infection and inflammation situations, when the immune response is driven to the Th1 profile (IL-12), the Th2 profile (IL-13) is diminished, or even some cytokines belonging to the same Th response can be preferentially produced than others (59, 60). For instance. it was demonstrated that during RSV responses, the IL-12 production was negatively related to IL-13 production, that IL-12/IL-13 axis was central to elicit an effective or innefective immune response to RSV infection and central to dictate the infection severity (61). Furthermore, it has been pointed out that the control of IL-13 release is crucial to avoid or minimize the dangerous effect of this cytokine in some diseases and infection processes in the airways (50, 61–63) and the positive correlation between the levels of IL-13 and IL-12p70 could represent a remarkable way to putatively control the harmful IL-13 effects in COVID-19 patients. Therefore, this association also could be responsible for the lack of association between IL-13 and IL-17, two months after SARS-CoV-2 infection, which led some COVID-19 patients to not present salivary SIgA levels.
In an interesting finding two months after SARS-CoV-2 infection, COVID-19 group patients with SIgA, the salivary level of IL-13 did not show a positive correlation with IL-17 and IFN-γ. This last association can favor the activation of an effective immune response to SARS-CoV-2 infection since it was reported that the concomitant production of IFN-γ and IL-13 in the airways increased the numbers of CD11c-positive cells expressing MHC class II, as well as the co-stimulator molecule CD86 (64), which can improve not only antigen-presentation but also the activation of CD4+ T cell. Furthermore, Jartti and collaborators (2014) (65) demonstrated that the presence of viral infection on tonsils or nasopharyngeal mucosa induced an “unexpected” strong correlation between IL-13 and the cluster of antiviral cytokines, which included all types of interferons.
According to these authors, the concomitant secretion of IL-13 and antiviral interferons can decrease inflammation and injury due to the capacity of IL-13 in inhibiting the pro-inflammatory factors synthesis by monocytes and macrophages during viral infection (66). Based on the information presented above, we can putatively suggest that, the positive correlation between IL-13 and IFN-α observed in both COVID-19 groups two months after the SARS-CoV-2 infection was important to control the inflammation and consequently minimize an eventual tissue injury in the airways, and, also, the positive correlation between IL-13 and IFN-γ can help the activation of the immune response leading to the SIgA production.
Beyond these observations, the COVID-19 group with SIgA showed increased IFN-α and IFN-γ levels as compared to the COVID-19 group without SIgA, as well as a positive correlation between these interferons. Higher IFN-α and IFN-γ levels were verified in patients with MERS-CoV (67) and SARS-CoV (9, 68), and it was postulated that these elevations could be useful both to improve the antigen presentation and also to develop a robust antiviral response against these infections (44, 69).
Although the elevation of IFN-γ in COVID-19 patients with mild/moderate symptoms have already been demonstrated by our group (9), the present study found significant positive correlations between the levels of IFN-γ with SIgA, IFN-α, IL-6, and IL-13 which were found in the same group in salivary samples obtained two months after SARS-CoV-2 infection, which we found particularly concerning.
It is noteworthy to point out that regardless of the type of interferons [type-I (IFN-α and IFN-β); type II (IFN-γ); or type-III (IFN-λ)], in a viral infection, these molecules are the main antiviral cytokines that promote the infection control and the ability to elicit an adaptive immune response to improve the viral clearance (70). Regarding the coronavirus infection, mainly in MERS-CoV and SARS-CoV infections, it was documented that the elevation in interferons both type-I and type-II was able to elicit an efficient host defense through its essential property of inhibiting coronavirus replication (9, 70). In terms of SARS-CoV-2 infection, the presence of the protective action of IFNs in the upper airway is very important to promote viral clearance with or without mild/moderate symptoms (71). Therefore, the increase in salivary levels of IFN-α and IFN-γ, especially in the COVID-19 group with SIgA, may elicit an effective immune response in the mucosa of the upper airway leading to SIgA production.
In this respect, according to the literature, IFN-γ is another cytokine that, similar to IL-17, is capable to upregulate the pIgR expression in the mucosal epithelial cells, which can drive to the better secretion of SIgA in the mucosa surfaces. Furthermore, it was reported that, in response to pro-inflammatory stimulus, IFN-γ can also up-regulate the expression of MHC class II molecules on mucosal epithelial cells. It is also paramount to highlight that the elevation of the IFN-γ levels concomitant with IL-6 by cells in the submandibular glands was able to create conditions for IgA synthesis in this site (14).
Thus, its direct effect on MHC class II and pIgR expression on the mucosal epithelial cells and the positive correlation with IL-6, demonstrates that IFN-γ is a very important cytokine to induce a favorable molecular environment in the mucosa of the upper airway to promote the secretion of SIgA (14). However, our finding that the COVID-19 group without SIgA also presented a positive correlation between IFN-γ and IL-6, two months after the SARS-CoV-2 infection, can reinforce the importance of the other features formerly described in the response necessary to guarantee SIgA production.
Interestingly, in the control group with SIgA, negative correlations were observed between the levels of IFN-γ and IL-6, between IFN-β and IL-13, and between IL-13 and IL-6. These findings are in agreement with the classical notion that, in a physiologic situation, without infection and inflammation, when the immune response is driven to the Th1 profile (IFN-γ), the Th2 profile (IL-6 and IL-13) is diminished. However, Th1 and Th2 cytokines may co-exist depending of the stimuli. Therefore, we can suggest that in this asymptomatic group, the presence of SARS-CoV-2 infection led to the activation of the immune response in the mucosa of the upper airway and after the viral clearance, confirmed by the PCR test negative, the physiological conditions have been stabilized.
Another very interesting result is related to the positive association between the salivary levels of IFN-α and IL-12p70, regardless of the SARS-Cov-2 infection or SIgA production. In agreement with the literature, both IFN-α and IL-12 are cytokines present in the The immune response and can induce the IFN-γ production in the oral cavity (72, 73). It was reported that low doses of IFN-α are able to prime the mucosal immune responses and that its functions are upstream of IL-12 action in the Th1 response against different antigens (self and non-self) and pathogens, mainly viruses in the oral cavity (74, 75). Regarding IFN-α action, its presence can induce the differentiation of monocytes to dendritic cells, when released during antigen presentation to CD4 T lymphocytes. This not only elicits signals in favor of Th1 immune response but also promotes upregulation of high-affinity IL-12 receptors (IL-12b1/b2), which leads the Th1 cells to be more responsive to IL-12 actions, as well as be useful to amplify the CD8 T lymphocyte resistance to viral infections (74, 76, 77). Furthermore, IFN-α also demonstrated a potential capacity to improve the neutrophils’ antifungal activity in the oral cavity (78, 79). Taking together all these data, our observation of the positive correlation between IFN-α and IL-12p70 could be understood as a hallmark of oral cavity immunity since all the volunteer groups presented this same association.
Specifically in the control group without SIgA, the positive correlation between IL-6 and IFN-α or IL-12p70 showed an interesting regulation of the immune response in the oral cavity since as formerly described, IL-6 is associated with Th2 immune response whereas IFN-α and IL-12 are associated with Th1 immune response. These positive correlations can corroborate our previous report that this association (Th1/Th2) is favorable to create a mucosal environment more protective by guaranteeing conditions to produce SIgA (14), particularly in response to the presence of a pathogenic agent. Furthermore, IL-6 is a cytokine that presents both pro and anti-inflammatory effects, depending on the context. It is accepted that this cytokine can be produced by a variety of cells, such as neutrophils, macrophages, fibroblasts, keratinocytes, endothelial cells, and also that it is involved in several biological processes, such as antibody production, T and B cells activation and differentiation, hematopoiesis, vascular permeability, and angiogenesis (80–82). In the oral cavity, IL-6 is a crucial cytokine involved in the host response against bacterial infection (83) and together with IFN-α, is important in host defense to influenza infection (84). So, the association between these cytokines allows us to speculate that this immune profile is necessary to create a protective environment improving the host defenses against potential pathogens.
Alternatively to what is described above, the groups that presented SIgA in saliva showed a positive correlation between IL-10 and IFN-α and/or IL-12p70, which demonstrates that the control of inflammation was elicited. It is broadly known that IL-10 is one of the most important anti-inflammatory cytokines and its production is crucial to regulate the inflammatory process (85). In the present study, we show that salivary IL-10 levels were increased in the COVID-19 groups (two months after the SARS-CoV-2 infection) and also in the control group that presented SIgA, demonstrating that IL-10 production was promoted and necessary to control the inflammation induced by SARS-CoV-2 infection. To support this idea, we observed no difference in the analysis of the ratio between salivary levels of IL-10 and the other pro-inflammatory cytokines, with the exception of a significant decrease of IL-12p70/IL-10 and IFN-γ/IL-10 ratios, which were closely associated with the increase of IL-10. According to the literature, the analysis of the ratio between pro and anti-inflammatory cytokines is considered a keystone that can clarify whether the inflammatory process is controlled (86), and mitigating dangerous situations that occur when the inflammation is not regulated, such as the cytokine storm in SARS-CoV-2 infection (87). Therefore, we can putatively suggest that IL-10 was able to control the inflammation in the COVID-19 groups, even two months after the SARS-CoV-2 infection, and in the control group with SIgA, which allows us to hypothesize that this regulation contributed to these volunteers being asymptomatic or presenting moderate/mild symptoms.
Taking our findings together, we were able to reinforce the importance of IL-17 in the immune response of airways’ mucosa and show, for the first time, relevant characteristics and associations between cytokines in COVID-19 groups, two months after the SARS-CoV-2 infection, in producers or non-producers of salivary specific-SIgA for SARS-CoV-2 virus.
Data Availability Statement
The raw data supporting the conclusions of this article will be made available by the authors, without undue reservation.
Ethics Statement
The studies involving human participants were reviewed and approved by Ethics Committee of the Albert Einstein Hospital. The patients/participants provided their written informed consent to participate in this study.
Author Contributions
JS, RV, and AB conceived the study. JS, JP, RV, ED, DO, and AB participated in the planning and development of this study. JS, JA, CF, FM, AA-S, SK, and EW participated in the laboratory analysis. SR, DH, EW, and JP laboratory diagnosis analysis, hospital clinical evaluation, sample collection, and patients’ clinical evaluation. JS, RV, and AB wrote the initial and final draft of the manuscript. All authors contributed to the article, revised the final draft, and approved the submitted version of the manuscript.
Funding
Funding was granted by Fundação de Amparo à Pesquisa do Estado de São Paulo (FAPESP), Grants No. 2016/20045-7 and 2020/06409-1 (ED), No. 2012/15165-2 (RV) and 2019/14115-0 (AB).
Conflict of Interest
The authors declare that the research was conducted in the absence of any commercial or financial relationships that could be construed as a potential conflict of interest.
Publisher’s Note
All claims expressed in this article are solely those of the authors and do not necessarily represent those of their affiliated organizations, or those of the publisher, the editors and the reviewers. Any product that may be evaluated in this article, or claim that may be made by its manufacturer, is not guaranteed or endorsed by the publisher.
Acknowledgments
The authors thank all the volunteers of this study.
Supplementary Material
The Supplementary Material for this article can be found online at: https://www.frontiersin.org/articles/10.3389/fimmu.2022.890887/full#supplementary-material
References
1. World Health Organization. Who Director-General’s Opening Remarks at the Media Briefing on COVID-19-11 March 2020. Geneva, Switzerland: World Health Organization (2020).
2. Lowery SA, Sariol A, Perlman S. Innate Immune and Inflammatory Responses to SARS-CoV-2: Implications for COVID-19. Cell Host Microbe (2021) 29(7):1052–62. doi: 10.1016/j.chom.2021.05.004
3. Gustine JN, Jones D. Immunopathology of Hyperinflammation in COVID-19. Am J Pathol (2021) 191(1):4–17. doi: 10.1016/j.ajpath.2020.08.009
4. Anka AU, Tahir MI, Abubakar SD, Alsabbagh M, Zian Z, Hamedifar H, et al. Coronavirus Disease 2019 (COVID-19): An Overview of the Immunopathology, Serological Diagnosis and Management. Scand J Immunol (2021) 93(4):e12998. doi: 10.1111/sji.12998
5. Béné MC, de CarvalhoBittencourt M, Eveillard M, Le Bris Y. Good IgA Bad IgG in SARS-CoV-2 Infection? Clin Infect Dis (2020) 71(15):897–98. doi: 10.1093/cid/ciaa426
6. Moore JB, June CH. Cytokine Release Syndrome in Severe COVID-19. Science (2020) 368(6490):473–4. doi: 10.1126/science.abb8925
7. Liu J, Li S, Liu J, Liang B, Wang X, Wang H, et al. Longitudinal Characteristics of Lymphocyte Responses and Cytokine Profiles in the Peripheral Blood of SARS-CoV-2 Infected Patients. EBioMedicine (2020) 55:102763. doi: 10.1016/j.ebiom.2020.102763
8. Jin Y, Yang H, Ji W, Wu W, Chen S, Zhang W, et al. Virology, Epidemiology, Pathogenesis, and Control of COVID-19. Viruses (2020) 12(4):372. doi: 10.3390/v12040372
9. Dos Santos JMB, Soares CP, Monteiro FR, Mello R, do Amaral JB, Aguiar AS, et al. In Nasal Mucosal Secretions, Distinct IFN and IgA Responses Are Found in Severe and Mild Sars-CoV-2 Infection. Front Immunol (2021) 12:595343. doi: 10.3389/fimmu.2021.595343
10. Noda K, Kodama S, Umemoto S, Nomi N, Hirano T, Suzuki M, et al. Th17 Cells Contribute to Nontypeable Haemophilus Influenzae-Specific Protective Immunity Induced by Nasal Vaccination With P6 Outer Membrane Protein and α-Galactosylceramide. Microbiol Immunol (2011) 55(8):574–81. doi: 10.1111/j.1348-0421.2011.00352.x
11. Yao Z, Zheng Z, Wu K, Junhua Z. Immune Environment Modulation in Pneumonia Patients Caused by Coronavirus: SARS-CoV, Mers-CoV and SARS-Cov-2. Aging (Albany NY) (2020) 12(9):7639–51. doi: 10.18632/aging.103101
12. Dezfuli NK, Adcock IM, Montazami N, Mortaz E, Velayati A. Update on Immunology of COVID-19 Disease and Potential Strategy for Controlling. Tanaffos (2020) 19(4):274–90.
13. Zhao S, Yuan L, Li Y, Liu L, Luo Z, Lv Q, et al. Secretory IgA in Mucosa of Pharynx and Larynx Plays an Important Role Against Influenza A Virus Infection in Kidney Yang Deficiency Syndrome Model. Evid Based Complement Alternat Med (2020) 2020:9316763. doi: 10.1155/2020/9316763
14. Smith P, MacDonald T, Blumberg R. Principles of Mucosal Immunology. Garland Sci 2nd Edition, New York: Garland Sci (2012). ISBN 9780429258459
15. Woof JM, Kerr MA. The Function of Immunoglobulin A in Immunity. J Pathol (2006) 208(2):270–82. doi: 10.1002/path.1877
16. Kumar N, Arthur CP, Ciferri C, Matsumoto ML. Structure of the Secretory Immunoglobulin A Core. Science (2020) 367(6481):1008–14. doi: 10.1126/science.aaz5807
17. Vaisberg M, Paixão V, Almeida EB, Santos JMB, Foster R, Rossi M, et al. Daily Intake of Fermented Milk Containing. Nutrients (2019) 11(7):1678. doi: 10.3390/nu11071678
18. Liang B, Hyland L, Hou S. Nasal-Associated Lymphoid Tissue is a Site of Long-Term Virus-Specific Antibody Production Following Respiratory Virus Infection of Mice. J Virol (2001) 75(11):5416–20. doi: 10.1128/JVI.75.11.5416-5420.2001
19. Buczko P, Zalewska A, Szarmach I. Saliva and Oxidative Stress in Oral Cavity and in Some Systemic Disorders. J Physiol Pharmacol (2015) 66(1):3–9.
20. Wang J, Schipper HM, Velly AM, Mohit S, Gornitsky M. Salivary Biomarkers of Oxidative Stress: A Critical Review. Free Radic Biol Med (2015) 85:95–104. doi: 10.1016/j.freeradbiomed.2015.04.005
21. Almeida EB, Santos JMB, Paixão V, Amaral JB, Foster R, Sperandio A, et al. L-Glutamine Supplementation Improves the Benefits of Combined-Exercise Training on Oral Redox Balance and Inflammatory Status in Elderly Individuals. Oxid Med Cell Longev (2020) 2020:2852181. doi: 10.1155/2020/2852181
22. Zhang Y, Sun J, Lin CC, Abemayor E, Wang MB, Wong DT. The Emerging Landscape of Salivary Diagnostics. Periodontol (2000) 70(1):38–52.
23. Fernandes L, Pacheco VB, Borges L, Athwal HK, de PaulaEduardo F, Bezinelli L, et al. Saliva in the Diagnosis of COVID-19: A Review and New Research Directions. J Dental Res (2020) 99(13):1435–43. doi: 10.1177/0022034520960070
24. Corman VM, Landt O, Kaiser M, Molenkamp R, Meijer A, Chu DK, et al. Detection of 2019 Novel Coronavirus (2019-nCoV) by Real-Time RT-PCR. Eurosurveillance (2020) 25(3):2000045. doi: 10.2807/1560-7917.ES.2020.25.3.2000045
25. Dare RK, Fry AM, Chittaganpitch M, Sawanpanyalert P, Olsen SJ, Erdman DD. Human Coronavirus Infections in Rural Thailand: A Comprehensive Study Using Real-Time Reverse-Transcription Polymerase Chain Reaction Assays. J Infect Dis (2007) 196(9):1321–8. doi: 10.1086/521308
26. Emery SL, Erdman DD, Bowen MD, Newton BR, Winchell JM, Meyer RF, et al. Real-Time Reverse Transcription-Polymerase Chain Reaction Assay for SARS-associated Coronavirus. Emerg Infect Dis (2004) 10(2):311–6. doi: 10.3201/eid1002.030759
27. Fry AM, Chittaganpitch M, Baggett HC, Peret TC, Dare RK, Sawatwong P, et al. The Burden of Hospitalized Lower Respiratory Tract Infection Due to Respiratory Syncytial Virus in Rural Thailand. PloS One (2010) 5(11):e15098. doi: 10.1371/journal.pone.0015098
28. Heim A, Ebnet C, Harste G, Pring-Akerblom P. Rapid and Quantitative Detection of Human Adenovirus DNA by Real-Time PCR. J Med Virol (2003) 70(2):228–39. doi: 10.1002/jmv.10382
29. Kodani M, Yang G, Conklin LM, Travis TC, Whitney CG, Anderson LJ, et al. Application of TaqMan Low-Density Arrays for Simultaneous Detection of Multiple Respiratory Pathogens. J Clin Microbiol (2011) 49(6):2175–82. doi: 10.1128/JCM.02270-10
30. Lu X, Erdman DD. Molecular Typing of Human Adenoviruses by PCR and Sequencing of a Partial Region of the Hexon Gene. Arch Virol (2006) 151(8):1587–602. doi: 10.1007/s00705-005-0722-7
31. Lu X, Holloway B, Dare RK, Kuypers J, Yagi S, Williams JV, et al. Real-Time Reverse transcription-PCR Assay for Comprehensive Detection of Human Rhinoviruses. J Clin Microbiol (2008) 46(2):533–9. doi: 10.1128/JCM.01739-07
32. Morgan OW, Chittaganpitch M, Clague B, Chantra S, Sanasuttipun W, Prapasiri P, et al. Hospitalization Due to Human Parainfluenza Virus-Associated Lower Respiratory Tract Illness in Rural Thailand. Influenza Other Respir Viruses (2013) 7(3):280–5. doi: 10.1111/j.1750-2659.2012.00393.x
33. de Paula Eduardo F, Bezinelli LM, de Araujo CAR, Moraes JVV, Birbrair A, Pinho JRR, et al. Self-Collected Unstimulated Saliva, Oral Swab, and Nasopharyngeal Swab Specimens in the Detection of SARS-Cov-2. Clin Oral Investig (2022) 26(2):1561–7. doi: 10.1007/s00784-021-04129-7
34. Hamada H, Garcia-Hernandez Mde L, Reome JB, Misra SK, Strutt TM, McKinstry KK, et al. Tc17, a Unique Subset of CD8 T Cells That can Protect Against Lethal Influenza Challenge. J Immunol (2009) 182(6):3469–81. doi: 10.4049/jimmunol.0801814
35. Trondsen M, Aqrawi LA, Zhou F, Pedersen G, Trieu MC, Zhou P, Cox RJ. Induction of Local Secretory IgA and Multifunctional Cd4+ T-helper Cells Following Intranasal Immunization With a H5N1 Whole Inactivated Influenza Virus Vaccine in BALB/c Mice. Scand J Immunol (2015) 81(5):305–17. doi: 10.1111/sji.12288
36. Hoffmann JP, Kolls JK, McCombs JE. Regulation and Function of ILC3s in Pulmonary Infections. Front Immunol (2021) 12:672523. doi: 10.3389/fimmu.2021.672523
37. Prasso JE, Deng JC. Postviral Complications: Bacterial Pneumonia. Clin Chest Med (2017) 38(1):127–38. doi: 10.1016/j.ccm.2016.11.006
38. Cella M, Fuchs A, Vermi W, Facchetti F, Otero K, Lennerz JK, et al. A Human Natural Killer Cell Subset Provides an Innate Source of IL-22 for Mucosal Immunity. Nature (2009) 457(7230):722–5. doi: 10.1038/nature07537
39. Sawa S, Cherrier M, Lochner M, Satoh-Takayama N, Fehling HJ, Langa F, et al. Lineage Relationship Analysis of RORgammat+ Innate Lymphoid Cells. Science (2010) 330(6004):665–9. doi: 10.1126/science.1194597
40. Sawa S, Lochner M, Satoh-Takayama N, Dulauroy S, Bérard M, Kleinschek M, et al. Rorγt+ Innate Lymphoid Cells Regulate Intestinal Homeostasis by Integrating Negative Signals From the Symbiotic Microbiota. Nat Immunol (2011) 12(4):320–6. doi: 10.1038/ni.2002
41. Khalid I, Alraddadi BM, Dairi Y, Khalid TJ, Kadri M, Alshukairi AN, Qushmaq IA, et al. Acute Management and Long-Term Survival Among Subjects With Severe Middle East Respiratory Syndrome Coronavirus Pneumonia and ARDS. Respir Care (2016) 61(3):340–8. doi: 10.4187/respcare.04325
42. Assiri A, McGeer A, Perl TM, Price CS, Al Rabeeah AA, Cummings DA, et al. Hospital Outbreak of Middle East Respiratory Syndrome Coronavirus. N Engl J Med (2013) 369(5):407–16. doi: 10.1056/NEJMoa1306742
43. Ko JH, Park GE, Lee JY, Lee JY, Cho SY, Ha YE, et al. Predictive Factors for Pneumonia Development and Progression to Respiratory Failure in MERS-CoV Infected Patients. J Infect (2016) 73(5):468–75. doi: 10.1016/j.jinf.2016.08.005
44. Mahallawi WH, Khabour OF, Zhang Q, Makhdoum HM, Suliman BA. Mers-CoV Infection in Humans is Associated With a Pro-Inflammatory Th1 and Th17 Cytokine Profile. Cytokine (2018) 104:8–13. doi: 10.1016/j.cyto.2018.01.025
45. Kudva A, Scheller EV, Robinson KM, Crowe CR, Choi SM, Slight SR, et al. Influenza A Inhibits Th17-mediated Host Defense Against Bacterial Pneumonia in Mice. J Immunol (2011) 186(3):1666–74. doi: 10.4049/jimmunol.1002194
46. Boyaka PN, Lillard JW, McGhee J. Interleukin 12 and Innate Molecules for Enhanced Mucosal Immunity. Immunol Res (1999) 20(3):207–17. doi: 10.1007/BF02790404
47. Vogel LA, Showe LC, Lester TL, McNutt RM, Van Cleave VH, Metzger DW. Direct Binding of IL-12 to Human and Murine B Lymphocytes. Int Immunol (1996) 8(12):1955–62. doi: 10.1093/intimm/8.12.1955
48. Metzger DW, McNutt RM, Collins JT, Buchanan JM, Van Cleave VH, Dunnick WA. Interleukin-12 Acts as an Adjuvant for Humoral Immunity Through Interferon-γ-Dependent and-Independent Mechanisms. Eur J Immunol (1997) 27(8):1958–65. doi: 10.1002/eji.1830270820
49. Li L, Young D, Wolf SF, Choi YS. Interleukin-12 Stimulates B Cell Growth by Inducing IFN-γ. Cell Immunol (1996) 168(2):133–40. doi: 10.1006/cimm.1996.0059
50. Hupin C, Rombaux P, Bowen H, Gould H, Lecocq M, Pilette C. Downregulation of Polymeric Immunoglobulin Receptor and Secretory IgA Antibodies in Eosinophilic Upper Airway Diseases. Allergy (2013) 68(12):1589–97. doi: 10.1111/all.12274
51. Huang C, Wang Y, Li X, Ren L, Zhao J, Hu Y. Clinical Features of Patients Infected With 2019 Novel Coronavirus in Wuhan, China. Lancet (2020) 395(10223):497–506. doi: 10.1016/S0140-6736(20)30183-5
52. Wu M, Xiao H, Liu G, Chen S, Tan B, Ren W, et al. Glutamine Promotes Intestinal SIgA Secretion Through Intestinal Microbiota and IL-13. Mol Nutr Food Res (2016) 60(7):1637–48. doi: 10.1002/mnfr.201600026
53. Allen JE, Maizels RM. Diversity and Dialogue in Immunity to Helminths. Nat Rev Immunol (2011) 11(6):375–88. doi: 10.1038/nri2992
54. Anthony RM, Rutitzky LI, Urban JF Jr, Stadecker MJ, Gause WC. Protective Immune Mechanisms in Helminth Infection. Nat Rev Immunol (2007) 7(12):975–87. doi: 10.1038/nri2199
55. Spits H, Cupedo T. Innate Lymphoid Cells: Emerging Insights in Development, Lineage Relationships, and Function. Annu Rev Immunol (2012) 30:647–75. doi: 10.1146/annurev-immunol-020711-075053
56. Fukushi M, Ito T, Oka T, Kitazawa T, Miyoshi-Akiyama T, Kirikae T, et al. Serial Histopathological Examination of the Lungs of Mice Infected With Influenza A Virus PR8 Strain. PloS One (2011) 6(6):e21207. doi: 10.1371/journal.pone.0021207
57. Marashian SM, Mortaz E, Jamaati HR, Alavi-Moghaddam M, Kiani A, Abedini A, et al. Role of Innate Lymphoid Cells in Lung Disease. Iran J Allergy Asthma Immunol (2015) 14(4):346–60.
58. Guo L, Junttila IS, Paul WE. Cytokine-Induced Cytokine Production by Conventional and Innate Lymphoid Cells. Trends Immunol (2012) 33(12):598–606. doi: 10.1016/j.it.2012.07.006
59. Abbas AK, Murphy KM, Sher A. Functional Diversity of Helper T Lymphocytes. Nature (1996) 383(6603):787–93. doi: 10.1038/383787a0
60. Moser M, Murphy KM. Dendritic Cell Regulation of TH1-TH2 Development. Nat Immunol (2000) 1(3):199–205. doi: 10.1038/79734
61. Tekkanat KK, Maassab HF, Cho DS, Lai JJ, John A, Berlin A, et al. Il-13-induced Airway Hyperreactivity During Respiratory Syncytial Virus Infection is STAT6 Dependent. J Immunol (2001) 166(5):3542–8. doi: 10.4049/jimmunol.166.5.3542
62. Wills-Karp M. Il-12/IL-13 Axis in Allergic Asthma. J Allergy Clin Immunol (2001) 107(1):9–18. doi: 10.1067/mai.2001.112265
63. Tekkanat KK, Maassab H, Berlin AA, Lincoln PM, Evanoff HL, Kaplan MH. Role of interleukin-12 and Stat-4 in the Regulation of Airway Inflammation and Hyperreactivity in Respiratory Syncytial Virus Infection. Am J Pathol (2001) 159(2):631–8. doi: 10.1016/S0002-9440(10)61734-8
64. Ford JG, Rennick D, Donaldson DD, Venkayya R, McArthur C, Hansell E, et al. Il-13 and IFN-gamma: Interactions in Lung Inflammation. J Immunol (2001) 167(3):1769–77. doi: 10.4049/jimmunol.167.3.1769
65. Jartti T, Palomares O, Waris M, Tastan O, Nieminen R, Puhakka T, et al. Distinct Regulation of Tonsillar Immune Response in Virus Infection. Allergy (2014) 69(5):658–67. doi: 10.1111/all.12396
66. Gern JE, Brooks GD, Meyer P, Chang A, Shen K, Evans MD, et al. Bidirectional Interactions Between Viral Respiratory Illnesses and Cytokine Responses in the First Year of Life. J Allergy Clin Immunol (2006) 117(1):72–8. doi: 10.1016/j.jaci.2005.10.002
67. Faure E, Poissy J, Goffard A, Fournier C, Kipnis E, Titecat M, et al. Distinct Immune Response in Two MERS-CoV-infected Patients: can We Go From Bench to Bedside? PLos One (2014) 9(2):e88716. doi: 10.1371/journal.pone.0088716
68. Contoli M, Papi A, Tomassetti L, Rizzo P, Vieceli DallaSega F, Fortini F, et al. Blood Interferon-α Levels and Severity, Outcomes, and Inflammatory Profiles in Hospitalized Covid-19 Patients. Front Immunol (2021) 12:648004. doi: 10.3389/fimmu.2021.648004
69. DeDiego ML, Nieto-Torres JL, Jimenez-Guardeño JM, Regla-Nava JA, Castaño-Rodriguez C, Fernandez-Delgado R, et al. Coronavirus Virulence Genes With Main Focus on SARS-CoV Envelope Gene. Virus Res (2014) 194:124–37. doi: 10.1016/j.virusres.2014.07.024
70. Totura AL, Baric RS. SARS Coronavirus Pathogenesis: Host Innate Immune Responses and Viral Antagonism of Interferon. Curr Opin Virol (2012) 2(3):264–75. doi: 10.1016/j.coviro.2012.04.004
71. Jafarzadeh A, Nemati M, Saha B, Bansode YD, Jafarzadeh S. Protective Potentials of Type Iii Interferons in COVID-19 Patients: Lessons From Differential Properties of Type I- and III Interferons. Viral Immunol (2021) 34(5):307–20. doi: 10.1089/vim.2020.0076
72. Malmgaard L. Induction and Regulation of IFNs During Viral Infections. J Interferon Cytokine Res (2004) 24(8):439–54. doi: 10.1089/1079990041689665
73. Trinchieri G, Gerosa F. Immunoregulation by Interleukin-12. J Leukoc Biol (1996) 59(4):505–11. doi: 10.1002/jlb.59.4.505
74. Tompkins WA. Immunomodulation and Therapeutic Effects of the Oral Use of Interferon-Alpha: Mechanism of Action. J Interferon Cytokine Res (1999) 19(8):817–28. doi: 10.1089/107999099313325
75. Malmgaard L, Paludan SR. Interferon (IFN)-Alpha/Beta, Interleukin (IL)-12 and IL-18 Coordinately Induce Production of IFN-gamma During Infection With Herpes Simplex Virus Type 2. J Gen Virol (2003) 84(Pt 9):2497–500. doi: 10.1099/vir.0.19251-0
76. Spadaro F, Lapenta C, Donati S, Abalsamo L, Barnaba V, Belardelli F, et al. Ifn-α Enhances Cross-Presentation in Human Dendritic Cells by Modulating Antigen Survival, Endocytic Routing, and Processing. Blood (2012) 119(6):1407–17. doi: 10.1182/blood-2011-06-363564
77. Santini SM, Lapenta C, Logozzi M, Parlato S, Spada M, Di Pucchio T, Belardelli F, et al. Type I Interferon as a Powerful Adjuvant for Monocyte-Derived Dendritic Cell Development and Activity In Vitro and in Hu-PBL-SCID Mice. J Exp Med (2000) 191(10):1777–88. doi: 10.1084/jem.191.10.1777
78. Katz MH, Greenspan D, Westenhouse J, Hessol NA, Buchbinder SP, Lifson AR, et al. Progression to AIDS in HIV-infected Homosexual and Bisexual Men With Hairy Leukoplakia and Oral Candidiasis. AIDS (1992) 6(1):95–100. doi: 10.1097/00002030-199201000-00013
79. Coates RA, Farewell VT, Raboud J, Read SE, Klein M, MacFadden DK, et al. Using Serial Observations to Identify Predictors of Progression to AIDS in the Toronto Sexual Contact Study. J Clin Epidemiol (1992) 45(3):245–53. doi: 10.1016/0895-4356(92)90084-Z
80. Matsuki Y, Yamamoto T, Hara K. Detection of Inflammatory Cytokine Messenger RNA (mRNA)-expressing Cells in Human Inflamed Gingiva by Combined in Situ Hybridization and Immunohistochemistry. Immunology (1992) 76(1):42–7.
81. Hirano T, Matsuda T, Turner M, Miyasaka N, Buchan G, Tang B, et al. Excessive Production of Interleukin 6/B Cell Stimulatory Factor-2 in Rheumatoid Arthritis. Eur J Immunol (1988) 18(11):1797–801. doi: 10.1002/eji.1830181122
82. Ridker PM, Cushman M, Stampfer MJ, Tracy RP, Hennekens CH. Inflammation, Aspirin, and the Risk of Cardiovascular Disease in Apparently Healthy Men. N Engl J Med (1997) 336(14):973–9. doi: 10.1056/NEJM199704033361401
83. Naruishi K, Nagata T. Biological Effects of Interleukin-6 on Gingival Fibroblasts: Cytokine Regulation in Periodontitis. J Cell Physiol (2018) 233(9):6393–400. doi: 10.1002/jcp.26521
84. Hayden FG, Fritz R, Lobo MC, Alvord W, Strober W, Straus SE, et al. Local and Systemic Cytokine Responses During Experimental Human Influenza A Virus Infection. Relation to Symptom Formation and Host Defense. J Clin Invest (1998) 101(3):643–9. doi: 10.1172/JCI1355
85. Foster R, Vaisberg M, Bachi ALL, Dos Santos JMB, de PaulaVieira R, Luna-Junior LA, et al. Premenstrual Syndrome, Inflammatory Status, and Mood States in Soccer Players. Neuroimmunomodulation (2019) 26(1):1–6. doi: 10.1159/000494559
86. Samuel PJ. Cytokine Analysis: A Fresh Perspective. J Interferon Cytokine Res (2020) 40(11):511–4. doi: 10.1089/jir.2020.0089
Keywords: mucosal immunity, saliva, cytokines, interferon, interleukin, SARS-CoV-2
Citation: Santos JdMBd, Amaral JBd, França CN, Monteiro FR, Alvares-Saraiva AM, Kalil S, Durigon EL, Oliveira DBL, Rodrigues SS, Heller D, Welter EAR, Pinho JRR, Vieira RP and Bachi ALL (2022) Distinct Immunological Profiles Help in the Maintenance of Salivary Secretory IgA Production in Mild Symptoms COVID-19 Patients. Front. Immunol. 13:890887. doi: 10.3389/fimmu.2022.890887
Received: 07 March 2022; Accepted: 19 April 2022;
Published: 24 May 2022.
Edited by:
Sarah Vreugde, University of Adelaide, AustraliaReviewed by:
Willem Van De Veen, University of Zurich, SwitzerlandVignesh Ramachandran, University of Kuala Lumpur, Malaysia
Copyright © 2022 Santos, Amaral, França, Monteiro, Alvares-Saraiva, Kalil, Durigon, Oliveira, Rodrigues, Heller, Welter, Pinho, Vieira and Bachi. This is an open-access article distributed under the terms of the Creative Commons Attribution License (CC BY). The use, distribution or reproduction in other forums is permitted, provided the original author(s) and the copyright owner(s) are credited and that the original publication in this journal is cited, in accordance with accepted academic practice. No use, distribution or reproduction is permitted which does not comply with these terms.
*Correspondence: Juliana de Melo Batista dos Santos, anVsaWFuYS5tZWxvQHVuaWZlc3AuYnI=
†These authors have contributed equally to this work and share senior authorship