- 1Department of Anatomy, Histology and Embryology, Faculty of Medicine, University of Debrecen, Debrecen, Hungary
- 2Center of Excellence in Genomic Medicine Research (CEGMR), Department of Medical Laboratory Technology, Faculty of Applied Medical Sciences, King Abdulaziz University, Jeddah, Saudi Arabia
- 3Center for Transdisciplinary Research, Department of Pharmacology, Saveetha Dental College and Hospitals, Saveetha Institute of Medical and Technical Sciences, Chennai, India
- 4FibroHealth Interdisciplinary Research Programme, Fibrobesity Cluster, Research Unit of Health Sciences and Technology, Faculty of Medicine, University of Oulu, Oulu, Finland
- 5Department of Regenerative Medicine, State Research Institute Centre for Innovative Medicine, Vilnius, Lithuania
- 6Department of Joint Surgery, First Affiliated Hospital of Sun Yat-sen University, Guangzhou, Guangdong, China
- 7World Health Organization Collaborating Center for Public Health Aspects of Musculoskeletal Health and Aging, Université de Liège, Liège, Belgium
Introduction: Clusterin is a moonlighting protein that has many functions. It is a multifunctional holdase chaperone glycoprotein that is present intracellularly and extracellularly in almost all bodily fluids. Clusterin is involved in lipid transport, cell differentiation, regulation of apoptosis, and clearance of cellular debris, and plays a protective role in ensuring cellular survival. However, the possible involvement of clusterin in arthritic disease remains unclear. Given the significant potential of clusterin as a biomarker of osteoarthritis (OA), a more detailed analysis of its complex network in an inflammatory environment, specifically in the context of OA, is required. Based on the molecular network of clusterin, this study aimed to identify interacting partners that could be developed into biomarker panels for OA.
Methods: The STRING database and Cytoscape were used to map and visualize the clusterin connectome. The Qiagen Ingenuity Pathway Analysis (IPA) software was used to analyze and study clusterin associated signaling networks in OA. We also analyzed transcription factors known to modulate clusterin expression, which may be altered in OA.
Results: The top hits in the clusterin network were intracellular chaperones, aggregate-forming proteins, apoptosis regulators and complement proteins. Using a text-mining approach in Cytoscape, we identified additional interacting partners, including serum proteins, apolipoproteins, and heat shock proteins.
Discussion: Based on known interactions with proteins, we predicted potential novel components of the clusterin connectome in OA, including selenoprotein R, semaphorins, and meprins, which may be important for designing new prognostic or diagnostic biomarker panels.
1 Introduction
Epidemiological studies have estimated that over 500 million people worldwide suffer from osteoarthritis (OA) (1). However, the true global burden of the disease is likely to be much higher (2). OA is a common inflammatory joint disorder that causes degeneration of articular cartilage and affects joint movement, resulting in significant disability (3). Despite its considerable personal, economic, and societal tolls, OA has generally been neglected. The development of therapies for OA has not made significant progress, unlike for many other chronic non-communicable diseases. Currently, there are no effective pharmacological treatments or disease-modifying OA drugs (DMOADs) available (2). The current therapeutic approaches include exercise, weight loss, and education. OA patients are generally administered non-steroidal anti-inflammatory drugs (NSAIDs) to reduce inflammation and alleviate joint pain. The lack of effective therapies likely stems from the heterogeneous nature of the disease and incomplete understanding of its pathophysiology (4). This can be facilitated by identifying clinical, biological, or medical markers specific to disease phenotypes. Soluble biomarkers are of interest and many candidate biomarkers have been identified. Systemic biomarkers have the potential to report the overall burden of disease and therefore provide holistic endpoints for generalized disease analyses (5). One such systemic biomarker that has recently attracted special attention in the context of OA is clusterin (6).
Clusterin (also known as apolipoprotein J and several other aliases) is a multifunctional holdase chaperone glycoprotein present in almost every bodily fluid, interstitial fluid, and intracellularly (7, 8). It is the first extracellular chaperone to facilitate the clearance of misfolded extracellular proteins, similar to heat shock proteins (HSP) inside the cell (9). Molecular chaperones are characterized by their selective binding to non-native protein conformations to form stable complexes, thus inhibiting irreversible aggregation (10). The importance of extracellular chaperones is underpinned by the fact that more than 40 human degenerative diseases are associated with the deposition of fibrillar proteinaceous aggregates called amyloids, including Alzheimer’s disease (AD) and Parkinson’s disease (PD) (10). In addition to the brain and central nervous system, amyloids also affect many tissues and organs, including the musculoskeletal system (11). Amyloid deposits derived from transthyretin (TTR) and apolipoprotein A-1 (APOA1) are frequently found in knee joints of patients with OA (12). The molecular structure of clusterin comprises molten globular-like features with putative amphipathic α-helices, which allow it to interact with the hydrophobic regions of proteins exposed to stress (13, 14). Upon binding, clusterin either stabilizes these proteins or facilitates their degradation (15). Clusterin has traditionally been associated with neuroprotection, mainly because of its role in clearing misfolded proteins, such as β-amyloid in AD (16). In addition, clusterin levels are associated with myocardial infarction (17). Clusterin is also associated with pain and inflammation. For example, lower clusterin serum concentrations were linked to higher pain scores in patients with erosive hand OA (18). Clusterin is used as a translational preclinical biomarker of various conditions, such as renal injury (19, 20), AD (21), cognitive disorders (22), and inflammatory conditions, such as vasculitis (23). Although clusterin levels in body fluids clearly reflect pathophysiological processes in many settings, and its use as a biomarker or biomarker candidate seems promising, it is unsuitable as a single unique diagnostic tool.
Clusterin is a moonlighting protein with many functions, including lipid transport, cell differentiation, regulation of apoptosis, and clearance of cellular debris, and seems to play a protective role in ensuring cellular survival (7). However, the possible involvement of clusterin in arthritic and rheumatic diseases has been relatively understudied, and only two published studies have examined its potential as a biomarker for cartilage lesions (24, 25). Therefore, further research is needed to study the roles of the secreted and intracellular forms of clusterin in osteoarticular tissues and to confirm whether clusterin could be used as a biomarker candidate in OA. Clusterin has been reported to be secreted by articular cartilage and chondrocytes (26, 27). Exposure to the pro-inflammatory cytokine interleukin-1β (IL-1β) resulted in reduced levels of clusterin precursor, but increased levels of mature clusterin (~35 kDa) released into the secretome of equine articular cartilage explants (26). Using in vitro models of low-grade inflammation in OA (which relies on a combination of tumor necrosis factor-α (TNF-α) and IL-1β), clusterin secretion into the secretome was attenuated (27). Clusterin is a robust marker of local synovial inflammation, as its level is significantly elevated in synovial fluid samples from patients with OA (28).
Despite accumulating (and often seemingly controversial) data, clusterin may have cytoprotective and anti-apoptotic effects, or other moonlighting functions that have not been studied in OA (6). Given the significant potential of synovial and systemic clusterin as biomarkers of OA, a more detailed analysis of its complex network in an inflammatory environment, specifically in the context of OA, is required. In order to address this, in the present study, we first used the STRING database and Cytoscape (29) to map and visualise the clusterin connectome. QIAGEN Ingenuity Pathway Analysis (IPA; Qiagen, Germantown, MD, USA) software, an advanced bioinformatics tool with a massive built-in scientific literature-based knowledge database, was employed to analyze and study clusterin-associated signalling networks in OA. The purpose of this study was to identify, based on the connectome and interactome available in public databases and the IPA knowledgebase, putative novel entities that could be developed into biomarkers (or rather panels of biomarkers) in OA. To this end, the interactions between clusterin and its partners in the broader connectome and interactome networks were investigated, highlighting their putative or established roles in arthritic diseases.
2 Methods
2.1 Elaborating the clusterin connectome using STRING and Cytoscape
We first employed the STRING database (version 11.5; string-db.org) to search for known protein interactions and Gene Ontology (GO) annotations of clusterin. STRING (Search Tool for the Retrieval of Interacting Genes/Proteins) is a biological database and web resource of known and predicted protein–protein interactions, and it is not exclusive to joint tissues or OA. We then used the PubMed query service in Cytoscape to import the top 50 protein interaction data for clusterin (confidence cut-off:0.4; network type: full-string network; query: clusterin; or clusterin AND osteoarthritis) based on publications indexed in PubMed. Owing to spatial limitations, the interactants identified using the PubMed query are included and discussed in the context of inflammatory joint disorders in the Supplementary Material.
2.2 Ingenuity pathway analysis
Clusterin interactome-associated genes were decoded using the Ingenuity Pathway Analysis (IPA) knowledge database (Qiagen, USA). The core analysis module was selected to identify significant upstream and downstream effects of the clusterin interactome on canonical pathways, diseases, biofunctions, causal networks, unique non-directional networks, tox functions, and pathological functions (30, 31). Fisher’s exact test with a p-value cut-off ≤ 0.05 and Benjamini-Hochberg (B-H) correction were used to calculate statistical significance. Activation or inhibition of canonical signaling pathways, diseases and disorders, molecular and cellular functions, and physiological system development and function were computed based on the Z-score algorithm of IPA and compared with an idealized activation or inhibition pattern for a signaling pathway, disease/disorder, or biological function. The IPA molecular activity predictor tool (MAP) (31) was used to assess the effects of clusterin activation or inhibition on the signaling pathways associated with OA.
2.3 Transcription factor analysis
Genome-wide RNA sequencing datasets of normal and OA-affected joint articular cartilage were downloaded from the Gene Expression Omnibus (GSE1140071) (32). Normalized read counts of individual samples (18 normal and 20 OA) were averaged before the analysis. The GeneHancer (GH) regulatory elements were then evaluated. Clusterin promoter/enhancer GH08J027610 had the highest gene association score of 255.90. The GeneHancer dataset contains 248 potential transcription factors that can bind to this sequence of genes of interest. The expression levels of these factors were compared between the control and OA groups.
3 Results and discussion
3.1 Clusterin has multiple interacting partners and is involved in diverse biological processes
Clusterin has 455 known interacting partners according to the STRING database; however, we only processed the top 25 interactants in this study2. The clusterin connectome, based on the top 25 interactants, contained 53 edges (connections), and the average node (protein) degree was 4.08, with a PPI enrichment p-value < 1.0e-16 (Tables 1, 2; Figure 1). The top hits in the clusterin network included intracellular chaperones (HSPA5 and HSP90B1) and aggregate-forming proteins (APP, SNCA, and PRNP), which is not surprising given their historic association with neurodegenerative disorders. Below we are focusing on the direct connections of clusterin.
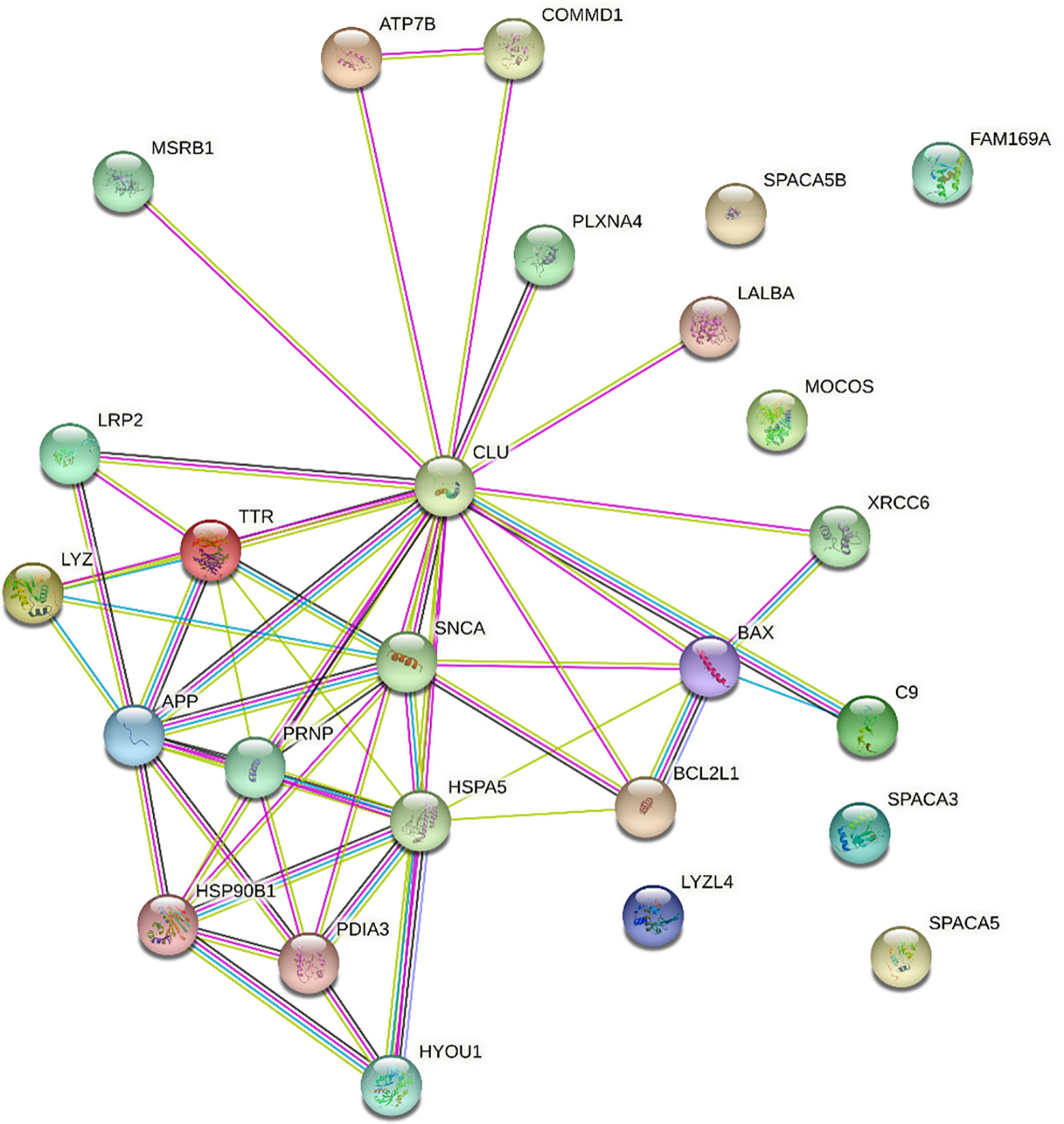
Figure 1 STRING interaction network for clusterin. Only direct connections are discussed. APP,amyloid-beta A4 protein; ATP7B, ATPase copper transporting beta; BAX, BCL2 associated X apoptosis regulator; BCL2L1, BCL2 like 1; C9, complement protein C9; CLU, clusterin; COMMD1, copper metabolism Murr1 domain; FAM169A, family with sequence similarity 169 member A; HSP90B1, heat shock protein 90 beta family member 1; HSPA5, heat shock protein family A (Hsp70) member 5; HYOU1, hypoxia up-regulated 1; LALBA, α-lactalbumin; LRP2, low-density lipoprotein receptor related protein 2; LYZ, lysozyme; LYZL4, lysozyme like 4; MOCOS, molybdenum cofactor sulfurase; MSRB1, methionine sulfoxide reductase B1; PDIA3, protein disulfide isomerase family A member 3; PLXNA4, plexin A4; PRNP, prion protein; SNCA, synuclein alpha; SPACA3, sperm acrosome associated 3; SPACA5, sperm acrosome associated 5; SPACA5B, sperm acrosome associated 5B; TTR, transthyretin; XRCC6, X-Ray Repair Cross-Complementing Protein 6. Edge colours are as follows: known interactions: light blue, from curated databases; magenta, experimentally determined; predicted interactions: green, gene neighbourhood; red, gene fusions; dark blue, gene co-occurrence; others: lime, text-mining; black, co-expression; purple, protein homology.
3.1.1 Heat shock proteins and intracellular chaperones
Among known interacting partners, heat shock protein family A (Hsp70) member 5 (HSPA5, also known as 78 kDa glucose-regulated protein, GRP78; BiP) has the highest number of edges (10). HSPA5 is a chaperone in the endoplasmic reticulum (ER) lumen, which is known to regulate clusterin stability under ER stress (33) and is involved in the molecular mechanisms of ER stress induced during chondrogenesis (34). HSP90B1 (Grp94) also has a very high number of edges (7). HSP90B1 is involved in ER stress triggered by excessive mechanical load and hypoxia in chondrocytes (35).
3.1.2 Amyloidogenic proteins and protein misfolding
Amyloid-β A4 protein (APP) is one of the top entities in the clusterin connectome (36). The clusterin–amyloid β-peptide complex interacts with low-density lipoprotein (LDL) receptor-related protein 2 (LRP2; megalin, known to act as a clusterin receptor), which offers a mechanism to clear the pathological accumulation of aggregates (37). Autoantibodies against LRP2 have been detected in 87% of patients with rheumatoid arthritis (RA) and 15% of patients with OA, indicating that these anti-LRP2 autoantibodies may play pathological roles by inhibiting the protein reabsorbing function of LRP2 in the proximal tubule (38). α-synuclein (SNCA) also forms a major component of amyloid plaques in AD and PD, and this process is blocked by clusterin (39). Clusterin interacts with extracellular α-synuclein fibrils and limits their uptake by astrocytes (40). Transthyretin (TTR) is an amyloidogenic protein. Clusterin is known to interact with aggregated forms of TTR, and serum clusterin levels in patients with transthyretin amyloid cardiomyopathy are significantly lower than those in healthy controls (41). TTR deposition in articular cartilage has been reported to increase disease severity in a murine model of OA (42). Moreover, both clusterin and TTR levels were higher in synovial fluid samples of patients with knee OA than in those with hand OA, indicating that they are involved in similar molecular pathways during OA pathogenesis (43). Clusterin interacts with amyloidogenic variants of lysozyme (LYZ) (44). Lysozyme has long been known to be present in the cartilage ECM (45), and cartilage degradation leads to increased serum and synovial fluid lysozyme levels in patients with OA (46). Clusterin is also involved in the folding/unfolding pathway of the extracellular protein α-lactalbumin (LALBA) (47).
Protein disulfide isomerase family A member 3 (PDIA3, ERp57), an oxidoreductase involved in native disulfide bond formation, is required for efficient clusterin oxidative folding (48). In the case of protein misfolding in the ER of chondrocytes, ECM proteins aggregate, resulting in ER stress, and the unfolded protein response (UPR) is initiated. Persistent ER stress is a pathogenic mechanism underlying OA (49). Hypoxia upregulated 1 (HYOU1), a marker of protein misfolding under cellular stress, is involved in the chondrocyte response to IL-1α (50).
3.1.3 Anti-apoptotic proteins
Clusterin has a well-known anti-apoptotic role, partly because it reduces the activity of the pro-apoptotic protein BCL2 associated X apoptosis regulator (BAX) (51, 52). However, the regulatory mechanisms of BAX underlying chondrocyte apoptosis in OA remain largely unknown (53), and clusterin involvement in this pathway has not been implicated in OA chondrocytes. In contrast, using a text-mining-based approach, anti-apoptotic BCL2L1 (BCL-XL) was recently identified as a gene that could be exploited as a potential drug target in OA (54) and is known to regulate apoptosis through the BCL-XL protein in kidney cells (55).
3.1.4 Copper homeostasis
ATPase copper-transporting β (ATP7B) is an important regulator of intracellular Cu homeostasis (15). COMMD1 (Copper metabolism Murr1 domain 1) is expressed in most tissues and plays a role in controlling protein degradation and stability (56). Clusterin and COMMD1 interact with ATP7B independently. As a consequence of these interactions, degradation of misfolded Cu-ATPase molecules is facilitated, which is an important factor in the quality control of ATP7B required for the maintenance of normal copper homeostasis (15). Literature on the function of copper transporters in chondrocytes is sparse (57), although a genetic predisposition to physiologically higher circulating copper and zinc status may increase the risk of OA (58). Significantly higher Cu concentrations have been detected in the synovial fluid of patients with OA than in healthy subjects (59). Therefore, Cu levels and transporter status in combination with clusterin should be further investigated in the context of OA. In contrast, COMMD1 is an important mediator of NF-κB signalling, a key player in inflammatory pathways, and clusterin has been linked to COMMD1 protein levels (60). Clusterin also has a complex regulatory interaction with NF-κB signalling (61).
3.1.5 Complement system
The complement system is involved in host defense mechanisms that aim to eliminate potentially harmful structures from the body. Clusterin potently inhibits terminal complement assembly by blocking complement protein (C9), thereby reducing the rate of complement-mediated cytolysis and providing higher levels of protection (62). Complement protein C9 has been described in the hypertrophic zone of the epiphyseal growth plate (63). C9 appears to be predominantly present in SC5b-9 complexes in synovial membrane samples from patients with OA (64). In cases of acute arthritis, such as OA flare-up, marked C9 deposits were detected in the synovium; however, C9 deposits were not found in chronic conditions associated with degenerative diseases, such as OA (65).
3.1.6 DNA repair
Clusterin is also implicated in DNA repair. Given the often fatal consequences of DNA breaks, several pathways exist for the recognition and repair of these lesions. One such pathway involves the DNA-dependent protein kinase (DNA-PK) complex, which consists of a catalytic subunit and heterodimeric Ku autoantigen comprising Ku70 (XRCC6) and Ku80 proteins (66). Clusterin was identified as an interacting partner of Ku70, likely initiating complex signalling mechanisms leading to cell death (66). In colon cancer, interleukin 6 (IL-6) affects pro-survival pathways by modulating the expression and molecular interactions between the pro-apoptotic factor BAX, DNA repair proteins Ku70/86, and clusterin (67). However, no experimental data are available on the role of clusterin in mediating repair pathways involving Ku70 in OA.
3.1.7 Selenium homeostasis
Selenoprotein R (SelR, also known as methionine sulfoxide reductase B1, MSRB1) plays an important role in maintaining intracellular redox balance by reducing the R-form of methionine sulfoxide. Given that selenium is an essential trace element, selenoproteins that mediate its metabolism are involved in key cellular functions such as redox homeostasis (68). SelR interacts with clusterin (69). Co-overexpression of SelR and clusterin in an AD model significantly decreased intracellular ROS levels. Furthermore, the interaction between clusterin and β-amyloid peptide was confirmed, suggesting a putative effect of SelR and β-amyloid peptide via clusterin (69). Appropriate selenium levels are required to maintain cartilage development and homeostasis (68), and experimental evidence suggests that selenoproteins are expressed in chondrocyte cell lines in vitro (70). Selenium deficiency is linked to the development of Kashin–Beck disease (KBD), which is an endemic osteoarthropathy (prevalent in low-selenium areas of China, North Korea, and Siberia in Russia) caused by disturbances in the closure of the epiphyseal plate and manifests as skeletal deformities and movement disorders (71). Certain polymorphisms in selenoprotein genes are associated with a higher risk of KBD (72). Furthermore, a cross-sectional analysis of dietary selenium intake revealed that high dietary selenium consumption may be associated with an increased risk of OA (73). However, SelR has not yet been directly discussed in the context of OA development.
3.1.8 Semaphorins
Plexins are receptors of the semaphorin family of signalling proteins (74). Plexin A4 (PLXNA4) acts as a clusterin receptor in the central nervous system and is an emerging therapeutic target for AD (68). Although plexins in chondrocytes have only been partially mapped, semaphorin-3A (Sema3A) has been implicated in OA chondrocyte physiology because excessive Sema3A signalling stimulated by the pro-inflammatory cytokines interleukin-1β (IL-1β) and tumor necrosis factor-α (TNF-α) promotes apoptosis (75). Sema4D has recently been shown to be involved in chondrocyte apoptosis triggered by lipopolysaccharide (LPS) (76). Therefore, elucidating aberrant semaphorin signalling in the context of plexins and clusterin may lead to the identification of new targets in OA.
3.2 A text-mining approach further expanded the clusterin connectome
To further identify interacting partners with clusterin, we used the PubMed query text-mining service in Cytoscape to expand the clusterin connectome (Figure 2). In the expanded connectome, additional interacting partners or proteins that were discussed together with clusterin in the research articles indexed in PubMed were retrieved (Table 3). Among these, APP, TTR, LRP2, C9, and XRCC6 have been discussed above. Owing to spatial limitations, most of the interacting partners identified using this text-mining approach are discussed in detail in the Supplementary Material.
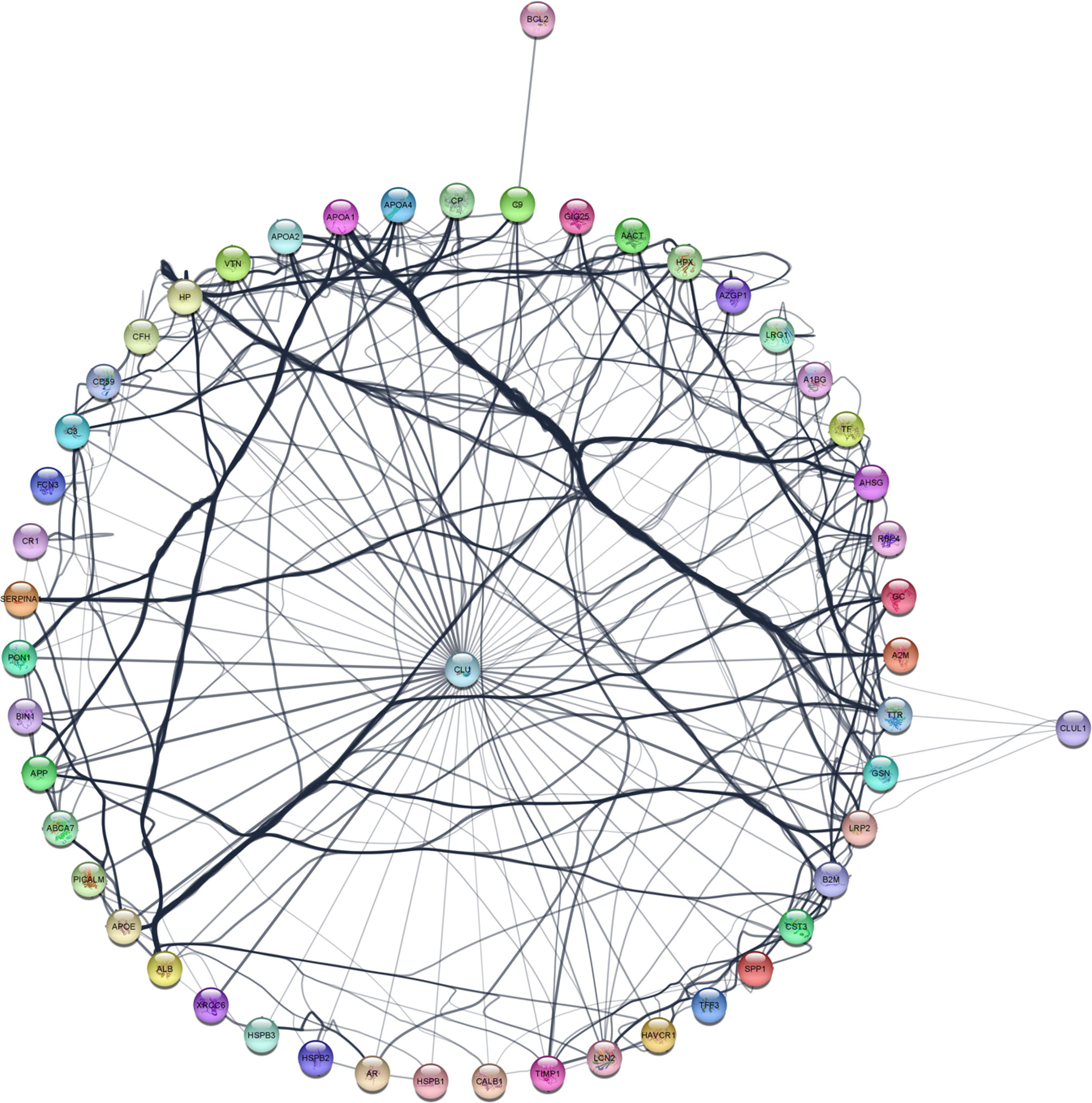
Figure 2 The expanded clusterin connectome retrieved using the PubMed query service in Cytoscape. See further details in text.
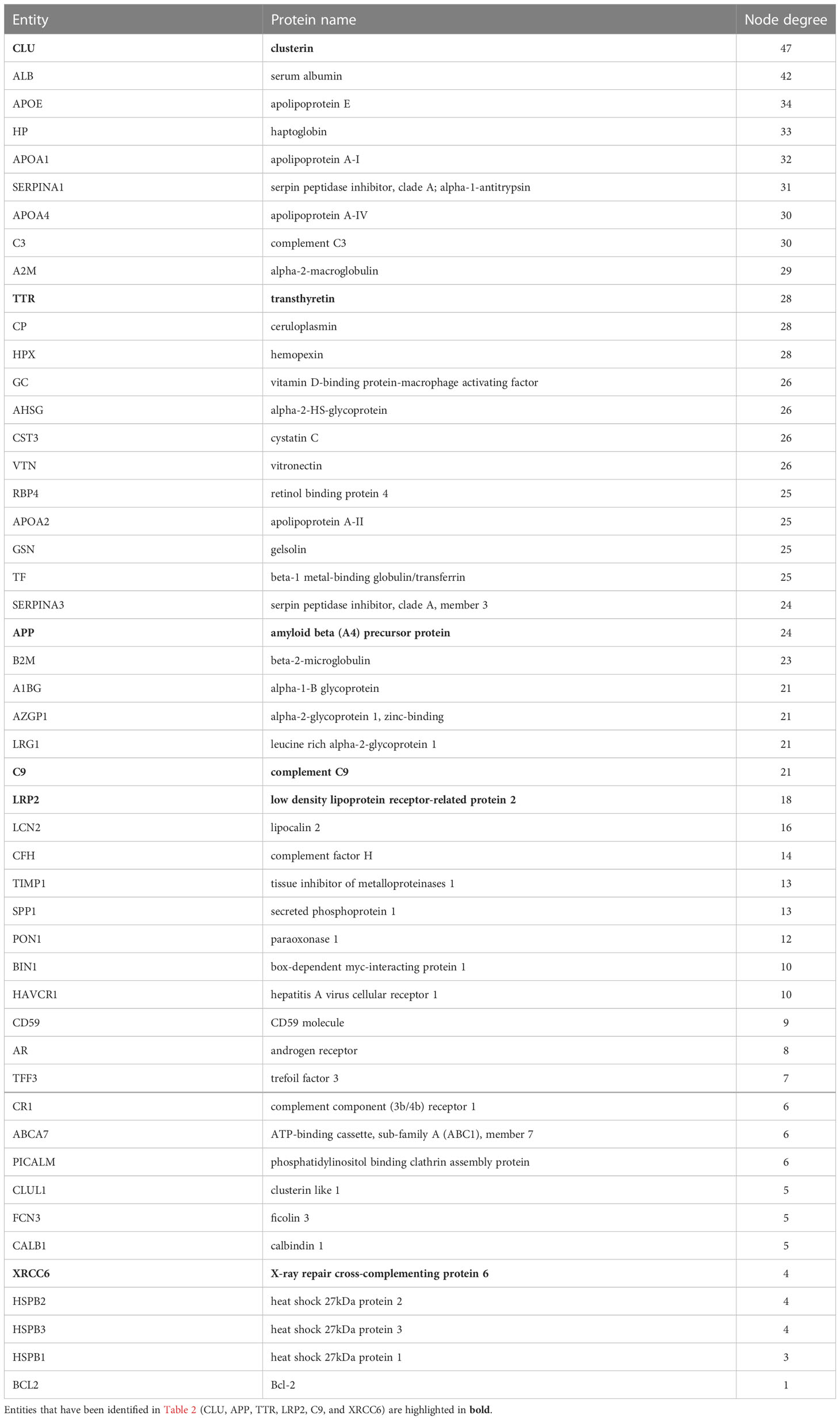
Table 3 Entities in the extended connectome network of clusterin ranked by node degree as identified by text-mining using Cytoscape.
3.3 Gene ontology annotations for the clusterin connectome reveal key biological pathways and molecular functions
GO annotations for human clusterin were retrieved from QuickGo Web Services3. The top biological processes included “positive/negative regulation of protein-containing complex assembly,” “positive regulation of gene expression,” “positive regulation of receptor-mediated endocytosis,” “protein targeting to lysosome involved in chaperone-mediated autophagy,” “negative regulation of cell death,” “positive/negative regulation of amyloid fibril formation,” “negative regulation of response to endoplasmic reticulum stress,” “positive regulation of proteasomal ubiquitin-dependent protein catabolic process,” “protein stabilization,” and chaperone-mediated protein folding” (Table 4A). Clusterin is involved in the following key molecular pathways: “protein binding,” “signaling receptor binding,” “amyloid-beta binding,” “protein carrier chaperone,” “protein-containing complex binding,” “tau protein binding,” “low-density lipoprotein particle receptor binding,” “chaperone binding,” and “misfolded protein binding” (Table 4B).
3.4 The clusterin network in the IPA knowledgebase
3.4.1 Overall clusterin network in the IPA knowledgebase
We then analyzed the overall clusterin interactome in the IPA knowledge base and identified additional pathways and interacting partners. In the overall molecular network of clusterin, the top canonical pathways were “colorectal cancer metastasis signaling,” “regulation of the epithelial mesenchymal transition by growth factors pathway,” “pancreatic adenocarcinoma signaling,” “IL-12 signaling and production in macrophages,” and “glucocorticoid receptor signaling” (Table 5A). These results are in line with the well-established role of clusterin in tumor biology.
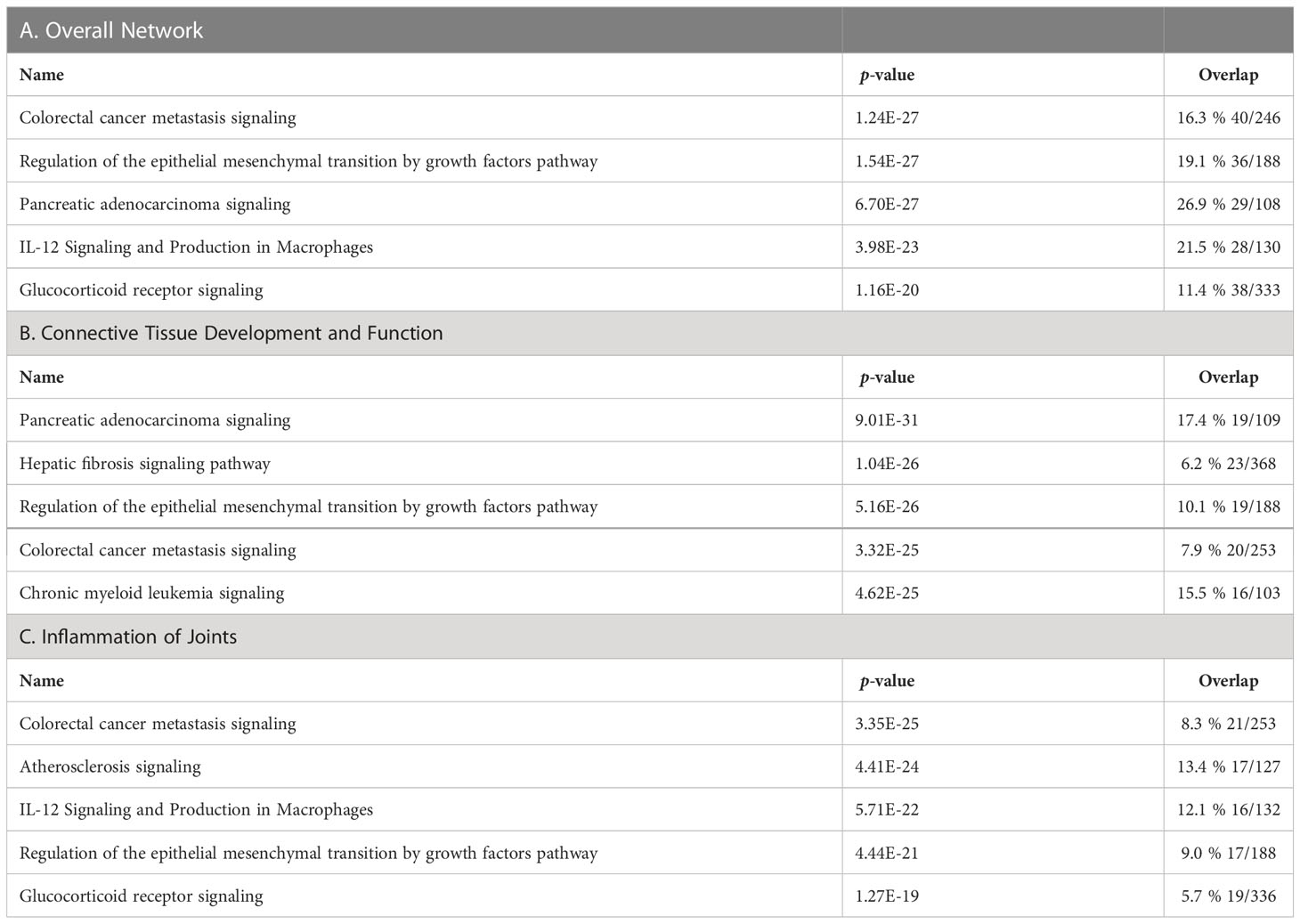
Table 5 Top canonical pathways of clusterin in the overall network (A), in connective tissue development and function (B), and in inflammation of joints (C).
The top upstream regulators were IL-6, CLU, TGFB1, TP53, and EZH2 (Table 6A). Interleukin-6 (IL-6) has been reported to influence pro-survival pathways in colon cancer progression via Bax, Ku70/86, and clusterin (67). IL-6 is especially relevant in the context of OA; an increase in IL-6 serum levels has been associated with decreased physical function and increased risk of knee OA progression (77). Transforming growth factor β1 (TGFB1) regulates clusterin expression (78–80). TGFB1 is an essential factor in chondrogenesis and cartilage maintenance, and a recent study confirmed that a SNP associated with OA susceptibility affects TGFB1 expression by influencing its enhancer (81). The tumor suppressor protein p53 (TP53) represses clusterin expression, which may be important for p53-mediated cell death (82). p53 has a well-established role in OA (82). EZH2, a histone methyltransferase involved in polycomb repressor complex 2 (PRC2), represses clusterin expression; therefore, aberrant upregulation of EZH2 may contribute to the progression of various tumors (83). EZH2 is upregulated in OA (84); however, the link between EZH2, clusterin, and OA progression has not yet been established.
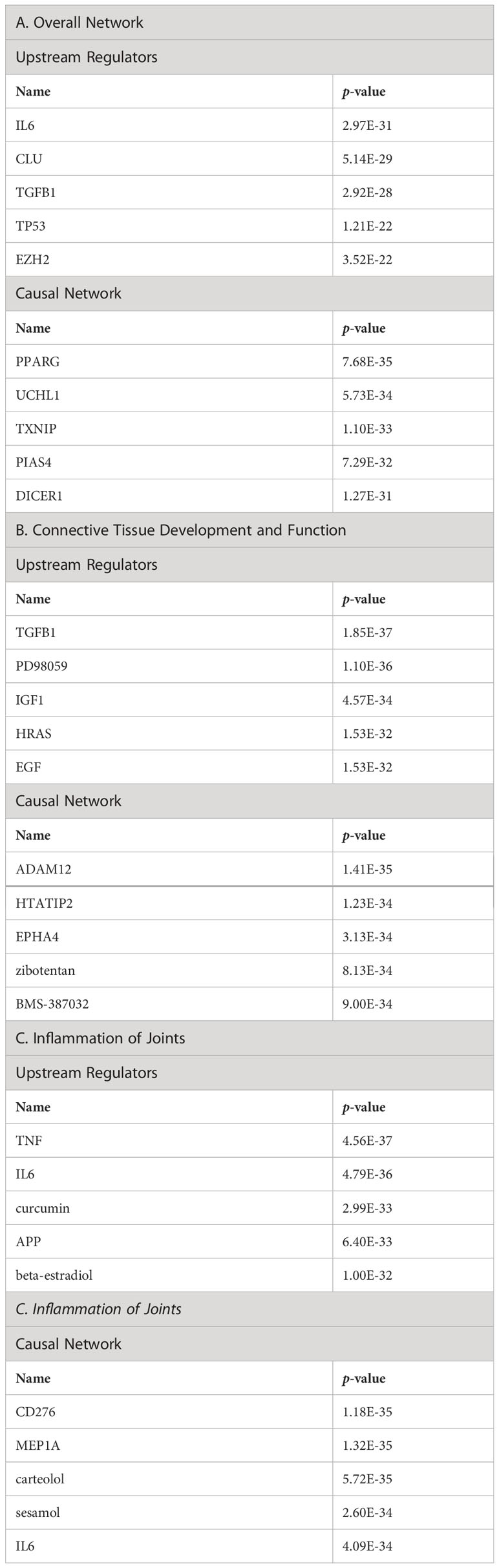
Table 6 Top upstream regulators and casual network of clusterin in the overall network (A), in connective tissue development and function (B), and in inflammation of joints (C).
The entities in the causal network of clusterin were PPARG, UCH1, TXNIP, PIAS4, and DICER1 (Table 6A). Clusterin overexpression upregulates the adipogenic marker peroxisome proliferator-activated receptor γ (PPARG) during adipocyte differentiation (85). PPARG signalling is involved in skeletal muscle regeneration via growth/differentiation factor 3 (GDF3) (86). PPARG expression is upregulated in synovitis, indicating its role in mediating tissue recovery (87). Although ubiquitin carboxyl-terminal hydrolase (UCL1), thioredoxin-interacting protein (TXNIP), and DICER1 are among the top members of the causal network, we did not find a direct association between these factors and clusterin. Nevertheless, TXNIP, an inhibitor of antioxidant activity, is downregulated by sirtuin 6 (SIRT6) in chondrocytes (88). Furthermore, TXNIP forms a complex with DDIT/REDD1, an endogenous inhibitor of mTOR that regulates cellular stress responses; the TXNIP/REDD1 complex is required for the activation of autophagy in chondrocytes, but its expression is reduced in OA (89). A protein inhibitor of activated STAT (PIAS4/PIASY) interferes with the binding of NF-κB, an important regulator of inflammation, to its target genes (60). As previously discussed, clusterin may mediate COMMD1, which induces NF-κB destabilization and proteasomal degradation (60). DICER-dependent pathways play critical roles in chondrocyte proliferation and differentiation during skeletal development (90).
The top five networks with the involvement of clusterin were as follows: “cell death and survival, cellular assembly and organization, cancer,” “cancer, organismal injury and abnormalities, cellular development,” “cellular development, connective tissue development and function, tissue development,” “cell death and survival, lipid metabolism, molecular transport,” and “cellular assembly and organization, DNA replication, recombination, and repair, cellular compromise” (Table 7A).
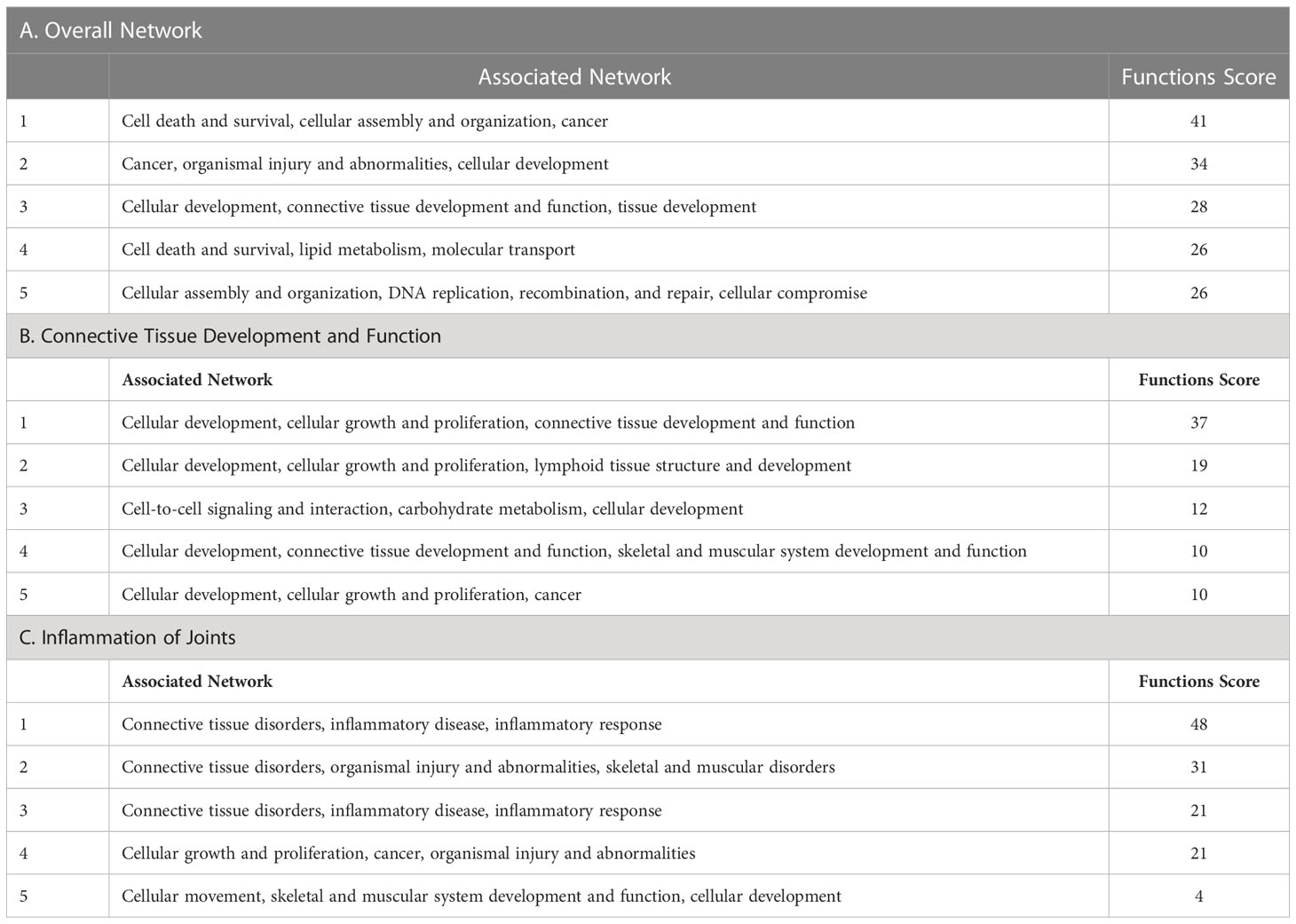
Table 7 Top networks associated with clusterin in the overall network (A), in connective tissue development and function (B), and in inflammation of joints (C).
3.4.2 Connective tissue development and function
Given that one of the top networks above was “cellular development, connective tissue development and function, and tissue development,” we repeated the IPA knowledgebase analysis focusing on connective tissue development and function. In this analysis, the top five canonical pathways were as follows: “pancreatic adenocarcinoma signaling,” “hepatic fibrosis signaling pathway,” “regulation of the epithelial mesenchymal transition by growth factors pathway,” “colorectal cancer metastasis signaling,” and “chronic myeloid leukemia signaling” (Table 5B).
The top upstream regulators were TGFB1, PD98059, IGF-1, HRAS, and EGF (Table 6B). TGFB1, an upstream regulator of clusterin, has been discussed previously. PD98059, an inhibitor of mitogen-activated protein kinase kinase (MEK1/MAPKK), abrogated clusterin-stimulated proliferation, indicating that clusterin may activate the extracellular signal-regulated kinase 1/2 (ERK1/2) pathway (91). As discussed previously, clusterin stimulates MMP-9 expression via ERK1/2 and NF-κB pathways (92). In an in vitro model of OA, ADAMTS and MMP upregulation correlated with the activation of ERK1/2 signalling, and PD98059 reversed the overexpression of matrix metalloproteinases (93). Insulin-like growth factor-1 (IGF-1) is also known to induce clusterin expression (94) and is involved in protecting cells from premature senescence (95). IGF-1 plays key roles in cartilage by promoting chondrocyte proliferation, enhancing ECM production, and inhibiting chondrocyte apoptosis, and is therefore highly relevant in OA therapy (96). Induction of the HRAS proto-oncogene represses clusterin expression in a MEK/ERK and methylation-dependent manner, indicating that DNA hypermethylation of the clusterin promoter is controlled by oncogenic signalling pathways (97). HRAS is involved in modulating chondrocyte apoptosis, senescence, and ECM degradation via MAPK signalling in OA (98). Epidermal growth factor (EGF) regulates clusterin expression via the Ras/ERK/AP-1 signalling pathway (99). While EGF signalling plays an important role in endochondral bone formation and joint homeostasis, conflicting results on its role in OA have been reported, which is likely attributable to the activation of specific downstream molecules as well as crosstalk with other signalling pathways (100).
The entities in the causal network were ADAM12, HTATIP2, EPHA4, Zibotentan, and BMS-387032 (Table 6B). We discussed the inclusion of ADAMs in an extended clusterin connectome (see Supplementary Material). HTATIP2 is an oxidoreductase required for tumor suppression in gliomas (101). Ephrin type-A receptor 4 (EPHA4) plays an emerging role in OA. Activation of EPHA4 signalling attenuates pro-inflammatory cytokine and MMP production in synoviocytes and augments the expression of chondrogenic genes in chondrocytes (102). Zibotentan is an endothelin A receptor antagonist (103). In vascular smooth muscle cells, endothelin was found to be significantly differentially expressed in response to clusterin (104). Endothelin-1 signalling plays an emerging role in OA pathogenesis by stimulating the expression of MMP-1 and MMP-13 (105). BMS-387032 is a potent inhibitor of cyclin-dependent kinases (CDK) 2, 7, and 9 (106). CDK inhibitors reduce the injury response after joint trauma, indicating that this pathway can be exploited for the prevention and/or treatment of early OA (107).
The top five networks in the clusterin interactome in the context of connective tissue development and function were as follows: “cellular development, cellular growth and proliferation, connective tissue development and function,” “cellular development, cellular growth and proliferation, lymphoid tissue structure and development,” “cell-to-cell signaling and interaction, carbohydrate metabolism, cellular development,” “cellular development, connective tissue development and function, skeletal and muscular system,” and “cellular development, cellular growth and proliferation, cancer” (Table 7B).
3.4.3 Molecules regulated by clusterin in the IPA knowledgebase
We extracted these molecules from the IPA knowledgebase, and their expression levels were modulated by clusterin, as shown in the published literature (Figure 3). In OA, clusterin-dependent regulation of several molecules is particularly relevant.
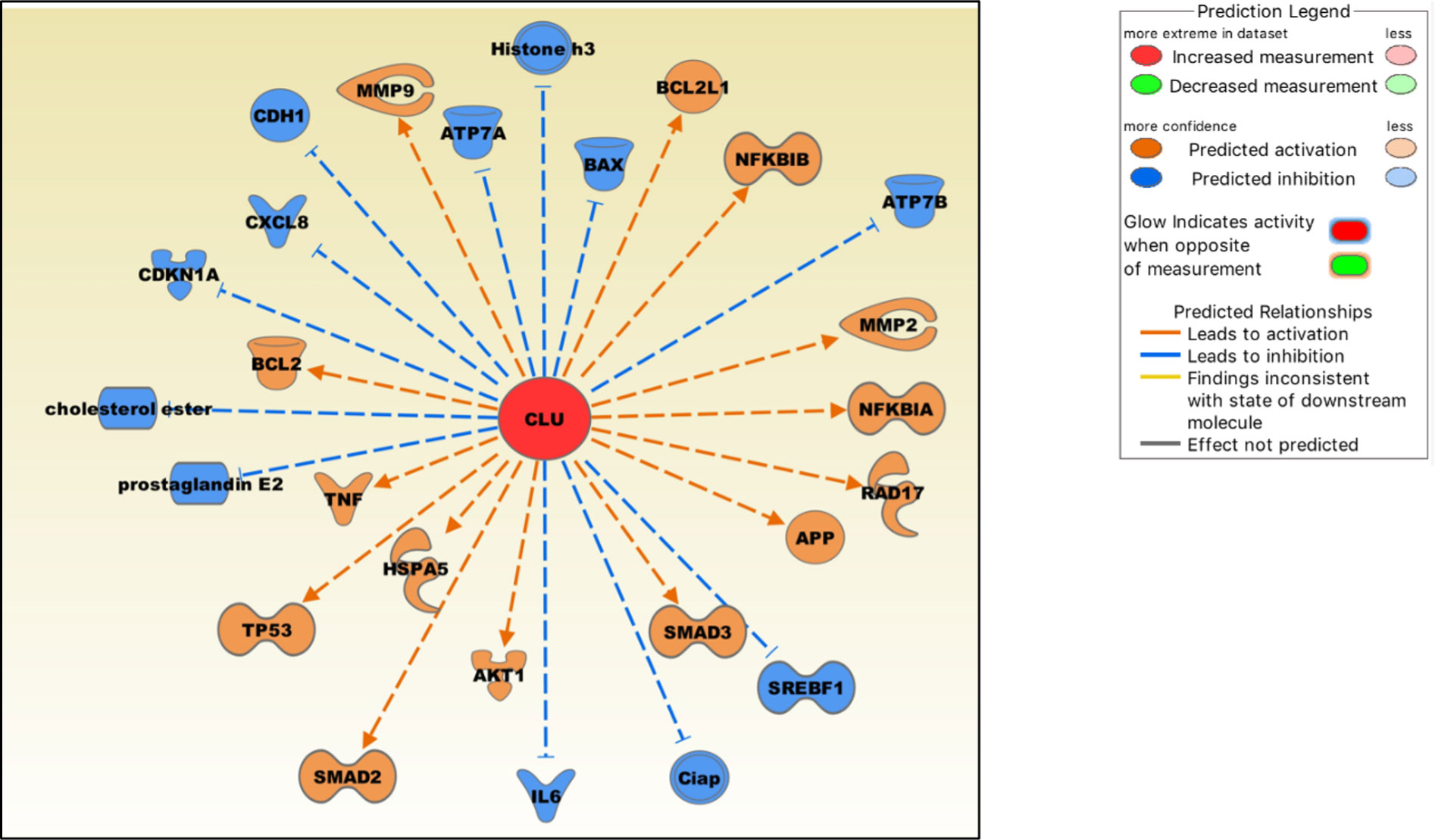
Figure 3 Molecules either up-regulated (orange) or down-regulated (blue) by clusterin based on the IPA knowledgebase. Image generated by the IPA software.
Clusterin increases the production of TNF-α and MMP-9 in macrophages (108), and both proteins are critical mediators of the OA pathophysiology (109). In cultured human fibroblast-like synoviocytes, clusterin knockdown by siRNA increased the production of pro-inflammatory cytokines IL-6 and IL-8 (CXCL8), indicating that clusterin plays a negative regulatory role in NF-κB-regulated cytokine production (24). Clusterin inhibits NF-κB signalling by stabilizing IκBs in neuroblastoma cells (110). Clusterin decreases the production of PGE2 (prostaglandin E2) (111), a principal mediator of inflammation, in RA and OA (112). Clusterin gene silencing in human OA chondrocytes shifted the cell phenotype towards hypertrophy and increased apoptosis, downregulated NF-κB-regulated genes, and increased MMP13 and TNF-α levels, suggesting a protective role of clusterin in these cells (113). Clusterin is involved in upregulating MMP-2 and downregulating E-cadherin expression in tumor cells (114). As discussed previously, clusterin and COMMD1 interact to downregulate ATP7A and ATP7B copper-transporting ATPases, thereby mediating Cu homeostasis (15). Overexpression of clusterin blocks TNF-α-mediated induction of p21 (CDKN1A) and abrogates proteolytic activation of the apoptosis regulator BAX, rendering clusterin-overexpressing breast cancer cells significantly more resistant to cytokines (115). Clusterin regulates the expression of proteins in mitochondrial apoptosis pathways, such as Bcl‐2, BAX, Bcl‐xL and caspase‐9, and phosphorylation of Akt (116). Clusterin blocks hepatic lipid accumulation by inhibiting SREBP-1c expression, suggesting that it may be a suitable target for preventing hepatic fat accumulation in insulin-resistant patients (117).
Clusterin is also an emerging modulator of TGF-β signalling that regulates SMAD2/3 proteins (118). These proteins are essential for the formation and maintenance of healthy cartilage and SMAD3 mutations are associated with OA (119). Clusterin overexpression increased SMAD2/3 protein levels via enhancing TGF-β-induced transcriptional activity (118). Clusterin is also involved in stabilising SMAD2/3. In tumor cells, clusterin plays a protective role against ER stress-induced apoptosis by interacting with glucose-regulated protein 78 (GRP78; also known as HSPA5), a central regulator of the unfolded protein response (120). GRP78 is upregulated in advanced OA, suggesting that chondrocytes experience ER stress during its pathogenesis (121). Clusterin may also be involved in regulating cellular cholesterol homeostasis under both normal and pathological conditions (122). Cholesterol homeostasis plays a key role in skeletal development, the dysregulation of which contributes to the development of cartilage diseases, including OA (123).
3.5 Clusterin network in OA (molecular activity prediction) in the IPA knowledgebase
We also analyzed the clusterin connectome in the context of joint inflammation (Figure 4). In this context, the top canonical pathways, similar to the previous two analyses, included “colorectal cancer metastasis signaling,” “atherosclerosis signaling,” “IL-12 signaling and production in macrophages,” “regulation of the epithelial mesenchymal transition by growth factors pathway,” and “glucocorticoid receptor signaling” (Table 5C).
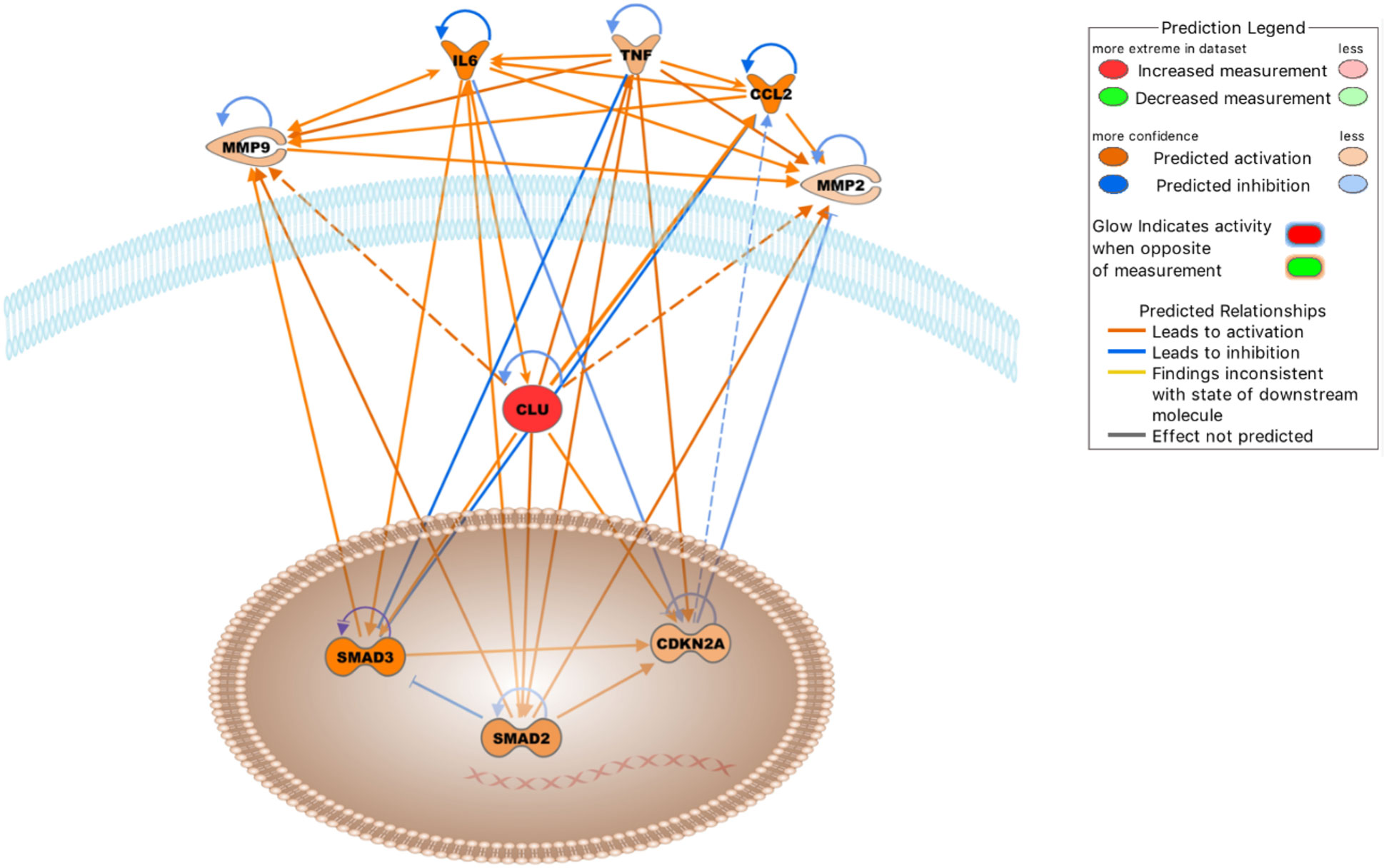
Figure 4 Molecules either activated (orange lines) or inhibited (blue lines) in the clusterin network in the context of OA based on the IPA knowledgebase (Dashed lines: probable activation). Image generated by the IPA software.
The top upstream regulators were TNF, IL6, curcumin, APP, and β-estradiol (Table 6C). TNF, IL-6, and APP have previously been discussed in the context of the clusterin connectome. Curcumin, an anti-inflammatory compound derived from Curcuma spp., has been used in clinical trials to determine its effectiveness in OA patients. Patients experienced improvement in pain, physical function, and quality of life after taking curcumin; these effects are attributable to the fact that curcumin blocks activation of the NF-κB system in chondrocytes (124). As discussed previously, various components of NF-κB signalling are directly associated with clusterin. β-estradiol regulates clusterin expression (125). The prevalence of OA was higher in women than in men in all age groups. In a study performed on total knee arthroplasty samples, sex differences were found in the synovial fluid levels of vitamin D metabolites, cytokines, and metalloproteinases, as well as in the cellular expression of 17β-estradiol receptors (126).
Entities in the causal network of clusterin were CD276, MEP1A, carteolol, sesamol, and IL6 (Table 6C). CD276 (also known as B7-H3), a member of the immunoglobulin superfamily, has been identified in chondrocytes by our group and others (127, 128). The expression of CD276 is correlated with poor prognosis in several pathologies, including RA (129), and is implicated as a promising therapeutic target for autoimmune diseases. Meprin α (MEP1A) is the largest secreted extracellular protease that hydrolyzes, activates, or inactivates several cytokines and growth factors. For example, it cleaves various MMPs, ADAMs, BMPs, DKK-1, collagen, syndecans, and fibronectin, many of which are relevant in the context of OA. It also cleaved clusterin (130). However, meprins have not been implicated in OA. Carteolol is a nonselective β-adrenoceptor antagonist. The β-adrenergic receptor signalling pathway plays a detrimental role in temporomandibular joint OA (131) and regulates cartilage catabolism induced by IL-1β (132). Sesamol, a natural organic compound present in sesame seeds and sesame oil, exerts its protective effect by blocking MMP expression via NF-κB or ERK/p38 MAPK signalling (133), offering a potential chondroprotective strategy in OA.
In the context of joint inflammation, the top five networks were as follows: “connective tissue disorders, inflammatory disease, inflammatory response,” “connective tissue disorders, organismal injury and abnormalities, skeletal and muscular disorders,” “connective tissue disorders, inflammatory disease, inflammatory response,” “cellular growth and proliferation, cancer, organismal injury and abnormalities,” and “cellular movement, skeletal and muscular system development and function, cellular development” (Table 7C). These networks further support the relevance of clusterin in inflammatory joint disease.
IPA identified five key regulatory networks that are especially relevant in the context of OA. These interactions have been previously described in detail, and are briefly discussed below.
1. IL-6 increases clusterin expression. Clusterin has been shown to be regulated by the pro-inflammatory cytokine IL-6 in various models (67, 134, 135). Importantly, an association between clusterin and IL-6 has been documented in cultured human fibroblast-like synoviocytes (FLSs) (24). Knockdown of clusterin using siRNA induced a significant and reproducible increase in the baseline production of IL-6 in FLSs, highlighting its negative regulatory role in NF-κB-dependent cytokine production.
2. Clusterin induces the expression of TNF-α and other cytokines. NF-κB, which is activated by extracellular stimuli including inflammatory cytokines such as TNF-α, is a key regulator of gene expression programs that culminate in stress-like responses. IKKs are upstream mediators of NF-κB activation (136). Clusterin has been identified as one of several genes that is dependent on IKK activation upon stimulation by TNF-α, suggesting that this pathway could protect against immune complex-mediated inflammatory reactions (137). TNF-α significantly alters the biogenesis of clusterin, leading to the appearance and nuclear accumulation of a 50–53 kDa uncleaved, non-glycosylated, disulfide-linked isoform (138). TNF-α also increases the level of the cytoplasmic 36–38.5 kDa clusterin isoform (139). These anomalous intracellular forms are likely attributable to aberrant glycosylation of clusterin released from the secretory system into the cytosol under ER stress (8). Conversely, exogenous clusterin increased TNF-α release from activated microglial cells (140). Clusterin binds to TNF-α in the BioPlex human interactome network (141). Furthermore, clusterin upregulates the expression of chemotactic cytokines such as monocyte chemotactic protein-1 (MCP-1) and macrophage inflammatory protein-1β (MIP-1β), regulated upon activation, normal T cell expressed and secreted (RANTES), and TNF-α in macrophages (108). However, clusterin is a negative regulator of TNF-α in OA chondrocytes, as increased TNF-α levels have been detected in clusterin-silenced human OA chondrocytes (113). Based on the above, the effects of clusterin on cytokine (e.g., TNF-α) production depend on the cell type, disease state, and the interplay between other intracellular pathways, depending on the available upstream or downstream factors.
3. Clusterin modulates the enzymatic activity and expression of MMPs. In both RA and OA, inflammatory cytokines, such as IL-1β and TNF-α stimulate the production of ECM-degrading MMPs (142). A direct interaction between clusterin and MMP-9 has been demonstrated in human epithelial cells, where clusterin binding prevents stress-induced MMP-9 aggregation and inhibits MMP-9 enzymatic activity. Clusterin also inhibits the enzymatic activities of MMP-2, MMP-3, and MMP-7. Treatment with pro-inflammatory cytokines (such as IL-1β and TNF-α) reduced clusterin expression (143). In contrast, clusterin knockdown resulted in a significant downregulation of MMP-2 in human hepatocellular carcinoma cells (114). Clusterin facilitates the nuclear translocation of NF-κB along with IκB-α degradation and phosphorylation in macrophages, leading to MMP-9 upregulation. Notably, only the intact secretory form of clusterin promotes MMP-9 activation; glycosylation-deficient and non-glycosylated recombinant clusterin is unable to stimulate MMP-9 (92, 108). Clusterin increases MMP-9 activity by increasing the phosphorylation status of p38 MAPK in platelet-stimulated colon carcinoma cells, thereby increasing invasion (144). The carcinogenic factor dinitrosopiperazine increased the binding of CLU to MMP-9 and upregulated MMP-9 expression via clusterin (145). In contrast, increased MMP13 levels were observed in human OA chondrocytes following clusterin silencing (113). These data also highlight that MMP regulation by clusterin is cell-type- and context-dependent, relying on concurrent active signal transduction pathways.
4. Clusterin is required for CDKN2A up-regulation. Forkhead box transcription factor L2 (FOXL2) stimulates clusterin expression in pituitary tumors. Clusterin induces the expression of cyclin-dependent kinase inhibitor p16 (CDKN2A), thereby inhibiting pituitary cell proliferation (146). FOXL2 is a major transcription factor in various developmental pathways, including bone and cartilage development, and its actions overlap with those of SOX9 (147). FOXL2 regulates ECM components (Col1a2, Col3a1, Col4a1, fibronectin, and laminin) in the ovaries (148). Senescence-promoting p16INK4a (CDKN2A), which is expressed in synovial tissue, is an OA marker and its somatic deletion partially protects against cartilage degeneration (149).
5. Clusterin increases the expression of SMAD2 and SMAD3. As discussed earlier, clusterin regulates SMAD2/3 proteins, which are key modulators of cartilage formation, by interacting with TGF-β type II receptor. Clusterin can also stabilize SMAD2/3 proteins, potentially via proteasomal degradation (118).
3.6 Clusterin transcription factor analysis in OA
Ten transcription factors that can bind to promoter/enhancer GH08J027610 were selected based on our criteria, which were two major conditions: first, the expression level to be in the top 10 percentile among the average OA read counts; and second, the fold change value to be above 1.0, on average, of OA samples normalized to the average of normal ones. These transcription factors include CTBP1, KLF6, MBD2, REST, SMARCE1, SOX5, TEAD1, XRCC5, ZEB1, and ZNF280D. According to the PANTHER™ Gene Ontology classification (150), the term ‘metabolic process’ (GO:0008152) was the most abundant, with eight of the 10 genes annotated by this term. Other notable categories included ‘biological regulation’ (GO:0065007), ‘cellular process’ (GO:0009987), and ‘developmental process’ (GO:0032502), all of which included at least three of the 10 genes. Finally, according to the Signor 2.0 curated interactions database (151), GDNF is a transcriptional up-regulator of clusterin (based on data obtained in Rattus norvegicus (152)). According to the datasets we analyzed, GDNF did not show robust expression in any of the groups investigated (normal versus OA cartilage); however, it is still notable that its expression level increased from the 23rd percentile in normal cartilage to the 35th percentile in OA cartilage in terms of ranking within the total transcriptome.
4 Summary
Clusterin, a multifunctional holdase chaperone, is an enigmatic protein with a wide range of functions that exerts its moonlighting role by acting in concert with an array of interacting proteins reviewed in this article. Clusterin is a moonlighting protein because, in addition to its conventional role as an extracellular chaperone in proteostasis, it is involved in a variety of other functions, including cell survival, complement inhibition, and cell differentiation (8). Clusterin is enigmatic, because we are far from understanding the actions of its cytoplasmic form. Here, we used an in silico approach to examine the interaction partners and connections of clusterin in OA. Clusterin interacts with a large number of proteins, as is evident from this study, as well as the additional information contained in the Supplementary Material that further analyses an extended list of clusterin interactants. Clusterin interactome is likely to expand further with the identification of new partners.
Based on known interactions with proteins, we predicted potentially novel components of the clusterin connectome in OA that may be important for designing new prognostic or diagnostic biomarker panels. The cytoprotective role of clusterin during cellular stress could be attributed to several mechanisms, such as anti-apoptotic signaling via Bax and/or Ku70, protection against oxidative stress, inhibition of the membrane attack complex (MAC) of locally activated complement proteins, and binding to stressed/misfolded proteins in a chaperone-like manner, preventing their aggregation. As discussed previously, clusterin has anti-apoptotic activity in various models by preventing Bax from entering the mitochondria or by blocking Bax phosphorylation via activation of the PI3K-AKT pathway (7). At the same time, however, clusterin also has pro-apoptotic functions by binding to Ku70, promoting active cell death through a caspase 3-dependent pathway (7). One of the most important roles of clusterin is the regulation of NF-κB activity. NF-κB-induced gene expression has been widely documented to contribute to the pathogenesis of inflammatory diseases including OA (24). Given the emerging role of clusterin in the regulation of apoptosis and NF-κB signalling, it is a potentially interesting and important target for RA and OA therapy. Understanding and defining the exact role(s) of this multifunctional protein in the pathogenesis of these two arthritic diseases are crucial.
Some of the interactions discussed in this paper have been described in various in vitro models or in vivo experiments, and have not (yet) been identified in the context of OA. However, given that, in addition to the complex role of clusterin, these interacting partners are also key players in OA pathogenesis and/or prognosis, it is likely that these interactions also exist in OA-affected joints. The interacting partners that warrant further experimental confirmation of OA are as follows. Clusterin is a known interacting partner of Ku70, which is a component of the DNA-dependent protein kinase complex that triggers cell death. However, the role of clusterin in mediating repair pathways involving Ku70 in OA has not yet been investigated. Selenoprotein R (SelR) maintains intracellular redox balance in cells, and clusterin interacts with SelR. Co-overexpression of SelR and clusterin significantly decreased intracellular ROS levels. Although selenoproteins are expressed in chondrocyte cell lines, SelR itself has not been explored in the context of OA. Other interesting candidates that have emerged as potentially relevant markers are semaphorins, a versatile group of proteins involved in various processes including axonal growth and bone development. Sema3A signalling stimulated by IL-1β and TNF-α promotes apoptosis, and Sema4D has recently been shown to be involved in chondrocyte apoptosis. Elucidating aberrant semaphorin signalling in the context of clusterin may lead to the identification of new targets in OA. Furthermore, meprins, which are extracellular proteases involved in connective tissue homeostasis, cleave procollagen I, amyloid precursor protein (APP), and IL-6R (153). Despite their roles as extracellular proteases and their specific targets, meprins have not been implicated in OA, highlighting the need for further research.
5 Conclusions and perspectives
Given that it is unlikely that any single biomarker can be sufficiently sensitive and specific to fulfil all needs, such as early disease detection, prediction of disease progression, and monitoring response to therapy as an effective intervention marker, it is likely that a combination of biochemical and imaging markers will ultimately be used serially and in combination to optimize OA drug development and patient therapy in OA. This is probably the case with clusterin; as a single biomarker, it will likely be insufficient to aid in the diagnosis and prognosis of patients with OA.
It is now evident that clusterin levels in bodily fluids are altered in various pathological conditions. Furthermore, it is involved in a plethora of intracellular signalling pathways, the outcomes of which are context-dependent. A growing body of evidence suggests that clusterin is a promising biomarker for OA (6). The diverse roles of this protein should be carefully considered in future translational and clinical orthopaedic studies, and special attention should be paid to its involvement in other comorbidities. It is important that future biomarker studies, especially when clusterin levels are measured in bodily fluids such as serum or urine, should not correlate clusterin levels exclusively to the process of OA pathogenesis. Clusterin in the synovial fluid is likely to be more suitable for further development as a biomarker candidate.
One such comorbidity is obesity. Clusterin plasma concentration is closely associated with metabolic disorders, such as obesity, and a high-fat and high-sucrose diet (Western diet) leading to diet-induced obesity is accompanied by increased clusterin levels in mice (154). Aberrant metabolism has been linked to different phenotypes of OA, and obesity is one of the most important risk factors of the disease (155). Clusterin is increasingly used as a biomarker for obesity-related AD (156), and different levels of clusterin in the CSF are associated with various stages of AD pathology (157). Although adipocyte-derived adipokines, including clusterin, may play a direct role in OA pathology, future studies are needed to determine whether clusterin is a viable biomarker for at least certain OA phenotypes or molecular endotypes and if it offers a key link between obesity, metabolic disease, and OA.
Future research is necessary on clusterin as a soluble biomarker candidate to establish whether it can provide new insights into OA pathogenesis progression and determine whether it can be used to aid in defining molecular endotypes, along with other biomarker candidates, perhaps the network of proteins identified and discussed in this article, including selenoprotein R, semaphorins, and meprin. Thus, clusterin will be a great asset for future research on OA pathogenesis, progression and potentially also for assessing responses to therapeutic interventions.
Data availability statement
Publicly available datasets were analyzed in this study. This data can be found here: Gene Expression Omnibus, GSE114007.
Author contributions
Conceptualization: AM, CM. conducting the research: network/pathway analysis using STRING/Cytoscape, PK. IPA analysis, PP. transcription factor analysis, RT literature search, CM. significant contribution to discussions, all authors. All authors contributed to the article and approved the submitted version.
Funding
CM and PK were supported by the Young Researcher Excellence Program (grant number: FK-134304) of the National Research, Development, and Innovation Office, Hungary. Project no. TKP2020-NKA-04 was implemented with support provided by the National Research, Development, and Innovation Fund of Hungary, financed under the 2020-4.1.1-TKP2020 funding scheme. AM acknowledges financial support from the Academy of Finland through the Profi6 336449 grant awarded to the University of Oulu, the European Commission Horizon Health programme and the PROTO Consortium, (Grant agreement ID: 101095635, https://cordis.europa.eu/project/id/101095635) and the European Structural and Social Funds through the Research Council of Lithuania (Lietuvos Mokslo Taryba), according to the Programme Attracting Foreign Researchers for Research Implementation (Grant No. 01.2.2-LMT-K-718-02-0022). CM, AM and RT also acknowledge financial support from the European Cooperation in Science and Technology COST Association Action CA21110 - Building an open European Network on OsteoArthritis research (NetwOArk); https://www.cost.eu/actions/CA21110/). PNP acknowledges the financial support from the Deputyship for Research and Innovation, Ministry of Education in Saudi Arabia, through project number (1045).
Conflict of interest
The authors declare that the research was conducted in the absence of any commercial or financial relationships that could be construed as a potential conflict of interest.
Publisher’s note
All claims expressed in this article are solely those of the authors and do not necessarily represent those of their affiliated organizations, or those of the publisher, the editors and the reviewers. Any product that may be evaluated in this article, or claim that may be made by its manufacturer, is not guaranteed or endorsed by the publisher.
Supplementary material
The Supplementary Material for this article can be found online at: https://www.frontiersin.org/articles/10.3389/fimmu.2023.1103097/full#supplementary-material
Footnotes
- ^ https://www.ncbi.nlm.nih.gov/geo/query/acc.cgi?acc=GSE114007 (Last accessed: 19-Feb-2023)
- ^ https://version-11-5.string-db.org/cgi/network?networkId=bBHxWsZnQ9sG (Last accessed: 19-Feb-2023)
- ^ https://www.ebi.ac.uk/QuickGO/annotations?geneProductId=P10909 (last accessed: 19-Feb-2023)
References
1. Hunter DJ, March L, Chew M. Osteoarthritis in 2020 and beyond: A lancet commission. Lancet (2020) 396:1711–2. doi: 10.1016/S0140-6736(20)32230-3
2. Mobasheri A, Mahmoudian A, Kalvaityte U, Uzieliene I, Larder CE, Iskandar MM, et al. A white paper on collagen hydrolyzates and ultrahydrolyzates: Potential supplements to support joint health in osteoarthritis? Curr Rheumatol Rep (2021) 23:78. doi: 10.1007/s11926-021-01042-6
3. Hunter DJ, Bierma-Zeinstra S. Osteoarthritis. Lancet (2019) 393:1745–59. doi: 10.1016/S0140-6736(19)30417-9
4. Henrotin Y. Osteoarthritis in year 2021: biochemical markers. Osteoarthritis Cartilage (2021) 30(2):237–48. doi: 10.1016/j.joca.2021.11.001
5. Kraus VB, Karsdal MA. Osteoarthritis: Current molecular biomarkers and the way forward. Calcif Tissue Int (2021) 109:329–38. doi: 10.1007/s00223-020-00701-7
6. Kalvaityte U, Matta C, Bernotiene E, Pushparaj PN, Kiapour AM, Mobasheri A. Exploring the translational potential of clusterin as a biomarker of early osteoarthritis. J Orthop Translat (2022) 32:77–84. doi: 10.1016/j.jot.2021.10.001
7. Rodriguez-Rivera C, Garcia MM, Molina-Alvarez M, Gonzalez-Martin C, Goicoechea C. Clusterin: Always protecting. synthesis, function and potential issues. BioMed Pharmacother (2021) 134:111174. doi: 10.1016/j.biopha.2020.111174
8. Satapathy S, Wilson MR. The dual roles of clusterin in extracellular and intracellular proteostasis. Trends Biochem Sci (2021) 46:652–60. doi: 10.1016/j.tibs.2021.01.005
9. Wyatt AR, Yerbury JJ, Berghofer P, Greguric I, Katsifis A, Dobson CM, et al. Clusterin facilitates in vivo clearance of extracellular misfolded proteins. Cell Mol Life Sci (2011) 68:3919–31. doi: 10.1007/s00018-011-0684-8
10. Wilson MR, Yerbury JJ, Poon S. Potential roles of abundant extracellular chaperones in the control of amyloid formation and toxicity. Mol Biosyst (2008) 4:42–52. doi: 10.1039/B712728F
11. Wininger AE, Phelps BM, Le JT, Harris JD, Trachtenberg BH, Liberman SR. Musculoskeletal pathology as an early warning sign of systemic amyloidosis: A systematic review of amyloid deposition and orthopedic surgery. BMC Musculoskelet Disord (2021) 22:51. doi: 10.1186/s12891-020-03912-z
12. Yanagisawa A, Ueda M, Sueyoshi T, Nakamura E, Tasaki M, Suenaga G, et al. Knee osteoarthritis associated with different kinds of amyloid deposits and the impact of aging on type of amyloid. Amyloid (2016) 23:26–32. doi: 10.3109/13506129.2015.1115758
13. Bailey RW, Dunker AK, Brown CJ, Garner EC, Griswold MD. Clusterin, a binding protein with a molten globule-like region. Biochemistry (2001) 40:11828–40. doi: 10.1021/bi010135x
14. Humphreys DT, Carver JA, Easterbrook-Smith SB, Wilson MR. Clusterin has chaperone-like activity similar to that of small heat shock proteins. J Biol Chem (1999) 274:6875–81. doi: 10.1074/jbc.274.11.6875
15. Materia S, Cater MA, Klomp LW, Mercer JF, La Fontaine S. Clusterin and COMMD1 independently regulate degradation of the mammalian copper ATPases ATP7A and ATP7B. J Biol Chem (2012) 287:2485–99. doi: 10.1074/jbc.M111.302216
16. Foster EM, Dangla-Valls A, Lovestone S, Ribe EM, Buckley NJ. Clusterin in alzheimer’s disease: Mechanisms, genetics, and lessons from other pathologies. Front Neurosci (2019) 13:164. doi: 10.3389/fnins.2019.00164
17. Turkieh A, Fertin M, Bouvet M, Mulder P, Drobecq H, Lemesle G, et al. Expression and implication of clusterin in left ventricular remodeling after myocardial infarction. Circ Heart Fail (2018) 11:e004838. doi: 10.1161/CIRCHEARTFAILURE.117.004838
18. Kropackova T, Sleglova O, Ruzickova O, Vencovsky J, Pavelka K, Senolt L. Lower serum clusterin levels in patients with erosive hand osteoarthritis are associated with more pain. BMC Musculoskelet Disord (2018) 19:264. doi: 10.1186/s12891-018-2179-3
19. Cofiell R, Kukreja A, Bedard K, Yan Y, Mickle AP, Ogawa M, et al. Eculizumab reduces complement activation, inflammation, endothelial damage, thrombosis, and renal injury markers in aHUS. Blood (2015) 125:3253–62. doi: 10.1182/blood-2014-09-600411
20. Wijewickrama ES, Mohamed F, Gawarammana IB, Endre ZH, Buckley NA, Isbister GK. Serum and urinary biomarkers for early detection of acute kidney injury following hypnale spp. envenoming. PloS Negl Trop Dis (2021) 15:e0010011. doi: 10.1371/journal.pntd.0010011
21. Tumati S, Herrmann N, Marotta G, Li A, Lanctot K. Blood-based biomarkers of agitation in alzheimer’s disease: Advances and future prospects. Neurochem Int (2022) 152:105250. doi: 10.1016/j.neuint.2021.105250
22. Ha J, Moon MK, Kim H, Park M, Cho SY, Lee J, et al. Plasma clusterin as a potential link between diabetes and Alzheimer disease. J Clin Endocrinol Metab (2020) 105(9):dgaa378. doi: 10.1210/clinem/dgaa378
23. Yoon T, Ahn SS, Pyo JY, Song JJ, Park YB, Lee SW. Serum clusterin level could reflect the current activity of antineutrophil cytoplasmic antibody-associated vasculitis. Yonsei Med J (2021) 62:1016–22. doi: 10.3349/ymj.2021.62.11.1016
24. Devauchelle V, Essabbani A, De Pinieux G, Germain S, Tourneur L, Mistou S, et al. Characterization and functional consequences of underexpression of clusterin in rheumatoid arthritis. J Immunol (2006) 177:6471–9. doi: 10.4049/jimmunol.177.9.6471
25. Kiapour AM, Sieker JT, Proffen BL, Lam TT, Fleming BC, Murray MM. Synovial fluid proteome changes in ACL injury-induced posttraumatic osteoarthritis: Proteomics analysis of porcine knee synovial fluid. PloS One (2019) 14:e0212662. doi: 10.1371/journal.pone.0212662
26. Clutterbuck AL, Smith JR, Allaway D, Harris P, Liddell S, Mobasheri A. High throughput proteomic analysis of the secretome in an explant model of articular cartilage inflammation. J Proteomics (2011) 74:704–15. doi: 10.1016/j.jprot.2011.02.017
27. Matta C, Fellows CR, Quasnichka H, Williams A, Jeremiasse B, Allaway D, et al. Clusterin secretion is attenuated by the proinflammatory cytokines interleukin-1beta and tumor necrosis factor-alpha in models of cartilage degradation. J Orthop Res (2021) 39:1017–29. doi: 10.1002/jor.24814
28. Fandridis E, Apergis G, Korres DS, Nikolopoulos K, Zoubos AB, Papassideri I, et al. Increased expression levels of apolipoprotein j/clusterin during primary osteoarthritis. In Vivo (2011) 25:745–9.
29. Shannon P, Markiel A, Ozier O, Baliga NS, Wang JT, Ramage D, et al. Cytoscape: a software environment for integrated models of biomolecular interaction networks. Genome Res (2003) 13:2498–504. doi: 10.1101/gr.1239303
30. Jafri MA, Kalamegam G, Abbas M, Al-Kaff M, Ahmed F, Bakhashab S, et al. Deciphering the association of cytokines, chemokines, and growth factors in chondrogenic differentiation of human bone marrow mesenchymal stem cells using an ex vivo osteochondral culture system. Front Cell Dev Biol (2019) 7:380. doi: 10.3389/fcell.2019.00380
31. Kalamegam G, Alfakeeh SM, Bahmaid AO, AlHuwait EA, Gari MA, Abbas MM, et al. In vitro evaluation of the anti-inflammatory effects of thymoquinone in osteoarthritis and in silico analysis of inter-related pathways in age-related degenerative diseases. Front Cell Dev Biol (2020) 8:646. doi: 10.3389/fcell.2020.00646
32. Fisch KM, Gamini R, Alvarez-Garcia O, Akagi R, Saito M, Muramatsu Y, et al. Identification of transcription factors responsible for dysregulated networks in human osteoarthritis cartilage by global gene expression analysis. Osteoarthritis Cartilage (2018) 26:1531–8. doi: 10.1016/j.joca.2018.07.012
33. Li N, Zoubeidi A, Beraldi E, Gleave ME. GRP78 regulates clusterin stability, retrotranslocation and mitochondrial localization under ER stress in prostate cancer. Oncogene (2013) 32:1933–42. doi: 10.1038/onc.2012.212
34. Han X, Zhou J, Zhang P, Song F, Jiang R, Li M, et al. IRE1alpha dissociates with BiP and inhibits ER stress-mediated apoptosis in cartilage development. Cell Signal (2013) 25:2136–46. doi: 10.1016/j.cellsig.2013.06.011
35. Huang Z, Zhou M, Wang Q, Zhu M, Chen S, Li H. Mechanical and hypoxia stress can cause chondrocytes apoptosis through over-activation of endoplasmic reticulum stress. Arch Oral Biol (2017) 84:125–32. doi: 10.1016/j.archoralbio.2017.09.021
36. Wojtas AM, Sens JP, Kang SS, Baker KE, Berry TJ, Kurti A, et al. Astrocyte-derived clusterin suppresses amyloid formation in vivo. Mol Neurodegener (2020) 15:71. doi: 10.1186/s13024-020-00416-1
37. Hammad SM, Ranganathan S, Loukinova E, Twal WO, Argraves WS. Interaction of apolipoprotein J-amyloid beta-peptide complex with low density lipoprotein receptor-related protein-2/megalin. A mechanism to prevent pathological accumulation of amyloid beta-peptide. J Biol Chem (1997) 272:18644–9. doi: 10.1074/jbc.272.30.18644
38. Ooka S, Matsui T, Nishioka K, Kato T. Autoantibodies to low-density-lipoprotein-receptor-related protein 2 (LRP2) in systemic autoimmune diseases. Arthritis Res Ther (2003) 5:R174–180. doi: 10.1186/ar754
39. Yuste-Checa P, Trinkaus VA, Riera-Tur I, Imamoglu R, Schaller TF, Wang H, et al. The extracellular chaperone clusterin enhances tau aggregate seeding in a cellular model. Nat Commun (2021) 12:4863. doi: 10.1038/s41467-021-25060-1
40. Filippini A, Mutti V, Faustini G, Longhena F, Ramazzina I, Rizzi F, et al. Extracellular clusterin limits the uptake of alpha-synuclein fibrils by murine and human astrocytes. Glia (2021) 69:681–96. doi: 10.1002/glia.23920
41. Greene MJ, Sam F, Soo Hoo PT, Patel RS, Seldin DC, Connors LH. Evidence for a functional role of the molecular chaperone clusterin in amyloidotic cardiomyopathy. Am J Pathol (2011) 178:61–8. doi: 10.1016/j.ajpath.2010.11.015
42. Matsuzaki T, Akasaki Y, Olmer M, Alvarez-Garcia O, Reixach N, Buxbaum JN, et al. Transthyretin deposition promotes progression of osteoarthritis. Aging Cell (2017) 16:1313–22. doi: 10.1111/acel.12665
43. Barreto G, Soliymani R, Baumann M, Waris E, Eklund KK, Zenobi-Wong M, et al. Functional analysis of synovial fluid from osteoarthritic knee and carpometacarpal joints unravels different molecular profiles. Rheumatol (Oxford) (2019) 58:897–907. doi: 10.1093/rheumatology/key232
44. Kumita JR, Poon S, Caddy GL, Hagan CL, Dumoulin M, Yerbury JJ, et al. The extracellular chaperone clusterin potently inhibits human lysozyme amyloid formation by interacting with prefibrillar species. J Mol Biol (2007) 369:157–67. doi: 10.1016/j.jmb.2007.02.095
45. Greenwald RA, Josephson AS, Diamond HS, Tsang A. Human cartilage lysozyme. J Clin Invest (1972) 51:2264–70. doi: 10.1172/JCI107035
46. Bennett RM, Skosey JL. Lactoferrin and lysozyme levels in synovial fluid: differential indices of articular inflammation and degradation. Arthritis Rheum (1977) 20:84–90. doi: 10.1002/art.1780200115
47. Poon S, Treweek TM, Wilson MR, Easterbrook-Smith SB, Carver JA. Clusterin is an extracellular chaperone that specifically interacts with slowly aggregating proteins on their off-folding pathway. FEBS Lett (2002) 513:259–66. doi: 10.1016/S0014-5793(02)02326-8
48. Jessop CE, Chakravarthi S, Garbi N, Hammerling GJ, Lovell S, Bulleid NJ. ERp57 is essential for efficient folding of glycoproteins sharing common structural domains. EMBO J (2007) 26:28–40. doi: 10.1038/sj.emboj.7601505
49. Rellmann Y, Gronau I, Hansen U, Dreier R. 4-phenylbutyric acid reduces endoplasmic reticulum stress in chondrocytes that is caused by loss of the protein disulfide isomerase ERp57. Oxid Med Cell Longev (2019) 2019:6404035. doi: 10.1155/2019/6404035
50. Wilson R, Golub SB, Rowley L, Angelucci C, Karpievitch YV, Bateman JF, et al. Novel elements of the chondrocyte stress response identified using an in vitro model of mouse cartilage degradation. J Proteome Res (2016) 15:1033–50. doi: 10.1021/acs.jproteome.5b01115
51. Koltai T. Clusterin: A key player in cancer chemoresistance and its inhibition. Onco Targets Ther (2014) 7:447–56. doi: 10.2147/OTT.S58622
52. Pereira RM, Mekary RA, da Cruz Rodrigues KC, Anaruma CP, Ropelle ER, da Silva ASR, et al. Protective molecular mechanisms of clusterin against apoptosis in cardiomyocytes. Heart Fail Rev (2018) 23(1):123–9. doi: 10.1007/s10741-017-9654-z
53. Miao G, Zang X, Hou H, Sun H, Wang L, Zhang T, et al. Bax targeted by miR-29a regulates chondrocyte apoptosis in osteoarthritis. BioMed Res Int (2019) 2019:1434538. doi: 10.1155/2019/1434538
54. Yu RG, Zhang JY, Liu ZT, Zhuo YG, Wang HY, Ye J, et al. Text mining-based drug discovery in osteoarthritis. J Healthc Eng (2021) 2021:6674744. doi: 10.1155/2021/6674744
55. Takase O, Minto AW, Puri TS, Cunningham PN, Jacob A, Hayashi M, et al. Inhibition of NF-kappaB-dependent bcl-xL expression by clusterin promotes albumin-induced tubular cell apoptosis. Kidney Int (2008) 73:567–77. doi: 10.1038/sj.ki.5002563
56. Burkhead JL, Morgan CT, Shinde U, Haddock G, Lutsenko S. COMMD1 forms oligomeric complexes targeted to the endocytic membranes via specific interactions with phosphatidylinositol 4,5-bisphosphate. J Biol Chem (2009) 284:696–707. doi: 10.1074/jbc.M804766200
57. Fife RS, Moody S, Houser D, Proctor C. Studies of copper transport in cultured bovine chondrocytes. Biochim Biophys Acta (1994) 1201:19–22. doi: 10.1016/0304-4165(94)90145-7
58. Zhou J, Liu C, Sun Y, Francis M, Ryu MS, Grider A, et al. Genetically predicted circulating levels of copper and zinc are associated with osteoarthritis but not with rheumatoid arthritis. Osteoarthritis Cartilage (2021) 29:1029–35. doi: 10.1016/j.joca.2021.02.564
59. Yazar M, Sarban S, Kocyigit A, Isikan UE. Synovial fluid and plasma selenium, copper, zinc, and iron concentrations in patients with rheumatoid arthritis and osteoarthritis. Biol Trace Elem Res (2005) 106:123–32. doi: 10.1385/BTER:106:2:123
60. Bartuzi P. Tuning NF-κβ activity: A touch of COMMD proteins. Biochim Biophys Acta (2013) 1832:2315–21. doi: 10.1016/j.bbadis.2013.09.014
61. Wang Y, Wang X, Zhao H, Liang B, Du Q. Clusterin confers resistance to TNF-alpha-induced apoptosis in breast cancer cells through NF-kappaB activation and bcl-2 overexpression. J Chemother (2012) 24:348–57. doi: 10.1179/1973947812Y.0000000049
62. McDonald JF, Nelsestuen GL. Potent inhibition of terminal complement assembly by clusterin: Characterization of its impact on C9 polymerization. Biochemistry (1997) 36:7464–73. doi: 10.1021/bi962895r
63. Andrades JA, Nimni ME, Becerra J, Eisenstein R, Davis M, Sorgente N. Complement proteins are present in developing endochondral bone and may mediate cartilage cell death and vascularization. Exp Cell Res (1996) 227:208–13. doi: 10.1006/excr.1996.0269
64. Kemp PA, Spragg JH, Brown JC, Morgan BP, Gunn CA, Taylor PW. Immunohistochemical determination of complement activation in joint tissues of patients with rheumatoid arthritis and osteoarthritis using neoantigen-specific monoclonal antibodies. J Clin Lab Immunol (1992) 37(4):147–62.
65. Konttinen YT, Ceponis A, Meri S, Vuorikoski A, Kortekangas P, Sorsa T, et al. Complement in acute and chronic arthritides: Assessment of C3c, C9, and protectin (CD59) in synovial membrane. Ann Rheum Dis (1996) 55:888–94. doi: 10.1136/ard.55.12.888
66. Yang CR, Yeh S, Leskov K, Odegaard E, Hsu HL, Chang C, et al. Isolation of Ku70-binding proteins (KUBs). Nucleic Acids Res (1999) 27:2165–74. doi: 10.1093/nar/27.10.2165
67. Pucci S, Mazzarelli P, Sesti F, Boothman DA, Spagnoli LG. Interleukin-6 affects cell death escaping mechanisms acting on bax-Ku70-Clusterin interactions in human colon cancer progression. Cell Cycle (2009) 8:473–81. doi: 10.4161/cc.8.3.7652
68. Kang SS, Kurti A, Wojtas A, Baker KE, Liu CC, Kanekiyo T, et al. Identification of plexin A4 as a novel clusterin receptor links two alzheimer’s disease risk genes. Hum Mol Genet (2016) 25:3467–75. doi: 10.1093/hmg/ddw188
69. Chen P, Wang C, Ma X, Zhang Y, Liu Q, Qiu S, et al. Direct interaction of selenoprotein r with clusterin and its possible role in alzheimer’s disease. PloS One (2013) 8(6):e66384. doi: 10.1371/journal.pone.0066384
70. Yan J, Zheng Y, Min Z, Ning Q, Lu S. Selenium effect on selenoprotein transcriptome in chondrocytes. Biometals (2013) 26:285–96. doi: 10.1007/s10534-013-9610-x
71. Li S, Cao J, Caterson B, Hughes CE. Proteoglycan metabolism, cell death and kashin-beck disease. Glycoconj J (2012) 29:241–8. doi: 10.1007/s10719-012-9421-2
72. Xiong YM, Mo XY, Zou XZ, Song RX, Sun WY, Lu W, et al. Association study between polymorphisms in selenoprotein genes and susceptibility to kashin-beck disease. Osteoarthritis Cartilage (2010) 18:817–24. doi: 10.1016/j.joca.2010.02.004
73. Deng X, Tan Y. A national cross-sectional analysis of selenium intake and risk of osteoarthritis: NHANES 2003-2016. Front Public Health (2022) 10:1047605. doi: 10.3389/fpubh.2022.1047605
74. Alto LT, Terman JR. Semaphorins and their signaling mechanisms. Methods Mol Biol (2017) 1493:1–25. doi: 10.1007/978-1-4939-6448-2_1
75. Sun J, Wei X, Wang Z, Liu Y, Lu J, Lu Y, et al. Inflammatory milieu cultivated Sema3A signaling promotes chondrocyte apoptosis in knee osteoarthritis. J Cell Biochem (2018) 119:2891–9. doi: 10.1002/jcb.26470
76. Lei J, Fu Y, Zhuang Y, Zhang K. Sema4D aggravated LPS-induced injury via activation of the MAPK signaling pathway in ATDC5 chondrocytes. BioMed Res Int (2020) 2020:8691534. doi: 10.1155/2020/8691534
77. Greene MA, Loeser RF. Aging-related inflammation in osteoarthritis. Osteoarthritis Cartilage (2015) 23:1966–71. doi: 10.1016/j.joca.2015.01.008
78. Laping NJ, Morgan TE, Nichols NR, Rozovsky I, Young-Chan CS, Zarow C, et al. Transforming growth factor-beta 1 induces neuronal and astrocyte genes: Tubulin alpha 1, glial fibrillary acidic protein and clusterin. Neuroscience (1994) 58:563–72. doi: 10.1016/0306-4522(94)90081-7
79. Morgan TE, Laping NJ, Rozovsky I, Oda T, Hogan TH, Finch CE, et al. Clusterin expression by astrocytes is influenced by transforming growth factor beta 1 and heterotypic cell interactions. J Neuroimmunol (1995) 58:101–10. doi: 10.1016/0165-5728(94)00194-S
80. Wegrowski Y, Perreau C, Martiny L, Haye B, Maquart FX, Bellon G. Transforming growth factor beta-1 up-regulates clusterin synthesis in thyroid epithelial cells. Exp Cell Res (1999) 247:475–83. doi: 10.1006/excr.1998.4378
81. Rice SJ, Roberts JB, Tselepi M, Brumwell A, Falk J, Steven C, et al. Genetic and epigenetic fine-tuning of TGFB1 expression within the human osteoarthritic joint. Arthritis Rheumatol (2021) 73:1866–77. doi: 10.1002/art.41736
82. Criswell T, Klokov D, Beman M, Lavik JP, Boothman DA. Repression of IR-inducible clusterin expression by the p53 tumor suppressor protein. Cancer Biol Ther (2003) 2:372–80. doi: 10.4161/cbt.2.4.430
83. Wang C, Liu Z, Woo CW, Li Z, Wang L, Wei JS, et al. EZH2 mediates epigenetic silencing of neuroblastoma suppressor genes CASZ1, CLU, RUNX3, and NGFR. Cancer Res (2012) 72:315–24. doi: 10.1158/0008-5472.CAN-11-0961
84. Allas L, Brochard S, Rochoux Q, Ribet J, Dujarrier C, Veyssiere A, et al. EZH2 inhibition reduces cartilage loss and functional impairment related to osteoarthritis. Sci Rep (2020) 10:19577. doi: 10.1038/s41598-020-76724-9
85. Oh GS, Yoon J, Kim G, Kim GH, Kim DS, Choi B, et al. Regulation of adipocyte differentiation by clusterin-mediated kruppel-like factor 5 stabilization. FASEB J (2020) 34:16276–90. doi: 10.1096/fj.202000551RR
86. Varga T, Mounier R, Patsalos A, Gogolak P, Peloquin M, Horvath A, et al. Macrophage PPARgamma, a lipid activated transcription factor controls the growth factor GDF3 and skeletal muscle regeneration. Immunity (2016) 45:1038–51. doi: 10.1016/j.immuni.2016.10.016
87. Menarim BC, El-Sheikh Ali H, Loux SC, Scoggin KE, Kalbfleisch TS, MacLeod JN, et al. Transcriptional and histochemical signatures of bone marrow mononuclear cell-mediated resolution of synovitis. Front Immunol (2021) 12:734322. doi: 10.3389/fimmu.2021.734322
88. Collins JA, Kapustina M, Bolduc JA, Pike JFW, Diekman BO, Mix K, et al. Sirtuin 6 (SIRT6) regulates redox homeostasis and signaling events in human articular chondrocytes. Free Radic Biol Med (2021) 166:90–103. doi: 10.1016/j.freeradbiomed.2021.01.054
89. Alvarez-Garcia O, Olmer M, Akagi R, Akasaki Y, Fisch KM, Shen T, et al. Suppression of REDD1 in osteoarthritis cartilage, a novel mechanism for dysregulated mTOR signaling and defective autophagy. Osteoarthritis Cartilage (2016) 24:1639–47. doi: 10.1016/j.joca.2016.04.015
90. Kobayashi T, Lu J, Cobb BS, Rodda SJ, McMahon AP, Schipani E, et al. Dicer-dependent pathways regulate chondrocyte proliferation and differentiation. Proc Natl Acad Sci U.S.A. (2008) 105(6):1949–54. doi: 10.1073/pnas.0707900105
91. Shin YJ, Kang SW, Jeong SY, Shim YJ, Kim YH, Kim BM, et al. Clusterin enhances proliferation of primary astrocytes through extracellular signal-regulated kinase activation. Neuroreport (2006) 17:1871–5. doi: 10.1097/WNR.0b013e328010ac99
92. Shim YJ, Kang BH, Jeon HS, Park IS, Lee KU, Lee IK, et al. Clusterin induces matrix metalloproteinase-9 expression via ERK1/2 and PI3K/Akt/NF-kappaB pathways in monocytes/macrophages. J Leukoc Biol (2011) 90:761–9. doi: 10.1189/jlb.0311110
93. Prasadam I, Crawford R, Xiao Y. Aggravation of ADAMTS and matrix metalloproteinase production and role of ERK1/2 pathway in the interaction of osteoarthritic subchondral bone osteoblasts and articular cartilage chondrocytes – possible pathogenic role in osteoarthritis. J Rheumatol (2012) 39:621–34. doi: 10.3899/jrheum.110777
94. Takeuchi A, Shiota M, Beraldi E, Thaper D, Takahara K, Ibuki N, et al. Insulin-like growth factor-I induces CLU expression through Twist1 to promote prostate cancer growth. Mol Cell Endocrinol (2014) 384:117–25. doi: 10.1016/j.mce.2014.01.012
95. Luo X, Suzuki M, Ghandhi SA, Amundson SA, Boothman DA. ATM Regulates insulin-like growth factor 1-secretory clusterin (IGF-1-sCLU) expression that protects cells against senescence. PloS One (2014) 9:e99983. doi: 10.1371/journal.pone.0099983
96. Wen C, Xu L, Xu X, Wang D, Liang Y, Duan L. Insulin-like growth factor-1 in articular cartilage repair for osteoarthritis treatment. Arthritis Res Ther (2021) 23:277. doi: 10.1186/s13075-021-02662-0
97. Lund P, Weisshaupt K, Mikeska T, Jammas D, Chen X, Kuban RJ, et al. Oncogenic HRAS suppresses clusterin expression through promoter hypermethylation. Oncogene (2006) 25:4890–903. doi: 10.1038/sj.onc.1209502
98. Lu G, Li L, Wang B, Kuang L. LINC00623/miR-101/HRAS axis modulates IL-1beta-mediated ECM degradation, apoptosis and senescence of osteoarthritis chondrocytes. Aging (Albany NY) (2020) 12:3218–37. doi: 10.18632/aging.102801
99. Gutacker C, Klock G, Diel P, Koch-Brandt C. Nerve growth factor and epidermal growth factor stimulate clusterin gene expression in PC12 cells. Biochem J (1999) 339(Pt 3):759–66. doi: 10.1042/bj3390759
100. Qin L, Beier F. EGFR signaling: Friend or foe for cartilage? JBMR Plus (2019) 3:e10177. doi: 10.1002/jbm4.10177
101. Dong X, Deng Q, Nie X, Zhang M, Jia W, Chen C, et al. Downregulation of HTATIP2 expression is associated with promoter methylation and poor prognosis in glioma. Exp Mol Pathol (2015) 98:192–9. doi: 10.1016/j.yexmp.2015.01.013
102. Stiffel VM, Thomas A, Rundle CH, Sheng MH, Lau KW. The EphA4 signaling is anti-catabolic in synoviocytes but pro-anabolic in articular chondrocytes. Calcif Tissue Int (2020) 107:576–92. doi: 10.1007/s00223-020-00747-7
103. Shore N, Mason M, de Reijke TM. New developments in castrate-resistant prostate cancer. BJU Int (2012) 109 Suppl 6:22–32. doi: 10.1111/j.1464-410X.2012.11217.x
104. Sivamurthy N, Stone DH, Logerfo FW, Quist WC. Apolipoprotein J inhibits the migration, adhesion, and proliferation of vascular smooth muscle cells. J Vasc Surg (2001) 34:716–23. doi: 10.1067/mva.2001.116301
105. Sin A, Tang W, Wen CY, Chung SK, Chiu KY. The emerging role of endothelin-1 in the pathogenesis of subchondral bone disturbance and osteoarthritis. Osteoarthritis Cartilage (2015) 23:516–24. doi: 10.1016/j.joca.2014.11.002
106. Heath EI, Bible K, Martell RE, Adelman DC, Lorusso PM. A phase 1 study of SNS-032 (formerly BMS-387032), a potent inhibitor of cyclin-dependent kinases 2, 7 and 9 administered as a single oral dose and weekly infusion in patients with metastatic refractory solid tumors. Invest New Drugs (2008) 26:59–65. doi: 10.1007/s10637-007-9090-3
107. Fukui T, Yik JHN, Doyran B, Davis J, Haudenschild AK, Adamopoulos IE, et al. Bromodomain-containing-protein-4 and cyclin-dependent-kinase-9 inhibitors interact synergistically in vitro and combined treatment reduces post-traumatic osteoarthritis severity in mice. Osteoarthritis Cartilage (2021) 29:68–77. doi: 10.1016/j.joca.2020.07.012
108. Shim YJ, Kang BH, Choi BK, Park IS, Min BH. Clusterin induces the secretion of TNF-alpha and the chemotactic migration of macrophages. Biochem Biophys Res Commun (2012) 422:200–5. doi: 10.1016/j.bbrc.2012.04.162
109. Kapoor M, Martel-Pelletier J, Lajeunesse D, Pelletier JP, Fahmi H. Role of proinflammatory cytokines in the pathophysiology of osteoarthritis. Nat Rev Rheumatol (2011) 7:33–42. doi: 10.1038/nrrheum.2010.196
110. Santilli G, Aronow BJ, Sala A. Essential requirement of apolipoprotein J (clusterin) signaling for IkappaB expression and regulation of NF-kappaB activity. J Biol Chem (2003) 278:38214–9. doi: 10.1074/jbc.C300252200
111. Zhang F, Sha J, Wood TG, Galindo CL, Garner HR, Burkart MF, et al. Alteration in the activation state of new inflammation-associated targets by phospholipase A2-activating protein (PLAA). Cell Signal (2008) 20:844–61. doi: 10.1016/j.cellsig.2008.01.004
112. Park JY, Pillinger MH, Abramson SB. Prostaglandin E2 synthesis and secretion: The role of PGE2 synthases. Clin Immunol (2006) 119:229–40. doi: 10.1016/j.clim.2006.01.016
113. Tarquini C, Pucci S, Scioli MG, Doldo E, Agostinelli S, D’Amico F, et al. Clusterin exerts a cytoprotective and antioxidant effect in human osteoarthritic cartilage. Aging (Albany NY) (2020) 12:10129–46. doi: 10.18632/aging.103310
114. Chen D, Wang Y, Zhang K, Jiao X, Yan B, Liang J. Antisense oligonucleotide against clusterin regulates human hepatocellular carcinoma invasion through transcriptional regulation of matrix metalloproteinase-2 and e-cadherin. Int J Mol Sci (2012) 13:10594–607. doi: 10.3390/ijms130810594
115. Flanagan L, Whyte L, Chatterjee N, Tenniswood M. Effects of clusterin over-expression on metastatic progression and therapy in breast cancer. BMC Cancer (2010) 10:107. doi: 10.1186/1471-2407-10-107
116. Zhang H, Kim JK, Edwards CA, Xu Z, Taichman R, Wang CY. Clusterin inhibits apoptosis by interacting with activated bax. Nat Cell Biol (2005) 7:909–15. doi: 10.1038/ncb1291
117. Seo HY, Kim MK, Jung YA, Jang BK, Yoo EK, Park KG, et al. Clusterin decreases hepatic SREBP-1c expression and lipid accumulation. Endocrinology (2013) 154:1722–30. doi: 10.1210/en.2012-2009
118. Lee KB, Jeon JH, Choi I, Kwon OY, Yu K, You KH. Clusterin, a novel modulator of TGF-beta signaling, is involved in Smad2/3 stability. Biochem Biophys Res Commun (2008) 366:905–9. doi: 10.1016/j.bbrc.2007.12.033
119. Thielen NGM, van der Kraan PM, van Caam APM. TGFβ/BMP signaling pathway in cartilage homeostasis. Cells (2019) 8(9):969. doi: 10.3390/cells8090969
120. Wang C, Jiang K, Gao D, Kang X, Sun C, Zhang Q, et al. Clusterin protects hepatocellular carcinoma cells from endoplasmic reticulum stress induced apoptosis through GRP78. PloS One (2013) 8:e55981. doi: 10.1371/journal.pone.0055981
121. Nugent AE, Speicher DM, Gradisar I, McBurney DL, Baraga A, Doane KJ, et al. Advanced osteoarthritis in humans is associated with altered collagen VI expression and upregulation of ER-stress markers Grp78 and bag-1. J Histochem Cytochem (2009) 57:923–31. doi: 10.1369/jhc.2009.953893
122. Gelissen IC, Hochgrebe T, Wilson MR, Easterbrook-Smith SB, Jessup W, Dean RT, et al. (clusterin) induces cholesterol export from macrophage-foam cells: a potential anti-atherogenic function? Biochem J (1998) 331(Pt 1):231–7. doi: 10.1042/bj3310231
123. Papathanasiou I, Anastasopoulou L, Tsezou A. Cholesterol metabolism related genes in osteoarthritis. Bone (2021) 152:116076. doi: 10.1016/j.bone.2021.116076
124. Chin KY. The spice for joint inflammation: Anti-inflammatory role of curcumin in treating osteoarthritis. Drug Des Devel Ther (2016) 10:3029–42. doi: 10.2147/DDDT.S117432
125. Wunsche W, Tenniswood MP, Schneider MR, Vollmer G. Estrogenic regulation of clusterin mRNA in normal and malignant endometrial tissue. Int J Cancer (1998) 76:684–8. doi: 10.1002/(SICI)1097-0215(19980529)76:5<684::AID-IJC12>3.0.CO;2-4
126. Pan Q, O’Connor MI, Coutts RD, Hyzy SL, Olivares-Navarrete R, Schwartz Z, et al. Characterization of osteoarthritic human knees indicates potential sex differences. Biol Sex Differ (2016) 7:27. doi: 10.1186/s13293-016-0080-z
127. La Rocca G, Lo Iacono M, Corsello T, Corrao S, Farina F, Anzalone R. Human wharton’s jelly mesenchymal stem cells maintain the expression of key immunomodulatory molecules when subjected to osteogenic, adipogenic and chondrogenic differentiation in vitro: new perspectives for cellular therapy. Curr Stem Cell Res Ther (2013) 8:100–13. doi: 10.2174/1574888X11308010012
128. Matta C, Zhang X, Liddell S, Smith JR, Mobasheri A. Label-free proteomic analysis of the hydrophobic membrane protein complement in articular chondrocytes: a technique for identification of membrane biomarkers. Biomarkers (2015) 20:572–89. doi: 10.3109/1354750X.2015.1130191
129. Chen Y, Guan SY, Deng J, Yang H, Xu W, Xu S, et al. B7-H3: A promising therapeutic target for autoimmune diseases. Cell Immunol (2020) 352:104077. doi: 10.1016/j.cellimm.2020.104077
130. Jefferson T, Auf dem Keller U, Bellac C, Metz VV, Broder C, Hedrich J, et al. The substrate degradome of meprin metalloproteases reveals an unexpected proteolytic link between meprin beta and ADAM10. Cell Mol Life Sci (2013) 70:309–33. doi: 10.1007/s00018-012-1106-2
131. Jiao K, Niu LN, Li QH, Ren GT, Zhao CM, Liu YD, et al. beta2-adrenergic signal transduction plays a detrimental role in subchondral bone loss of temporomandibular joint in osteoarthritis. Sci Rep (2015) 5:12593. doi: 10.1038/srep12593
132. Hwang HS, Lee MH, Go DJ, Kim HA. Norepinephrine modulates IL-1beta-induced catabolic response of human chondrocytes. BMC Musculoskelet Disord (2021) 22:724. doi: 10.1186/s12891-021-04598-7
133. Lu YC, Jayakumar T, Duann YF, Chou YC, Hsieh CY, Yu SY, et al. Chondroprotective role of sesamol by inhibiting MMPs expression via retaining NF-kappaB signaling in activated SW1353 cells. J Agric Food Chem (2011) 59:4969–78. doi: 10.1021/jf1046738
134. Van Lenten BJ, Wagner AC, Navab M, Fogelman AM. Oxidized phospholipids induce changes in hepatic paraoxonase and ApoJ but not monocyte chemoattractant protein-1 via interleukin-6. J Biol Chem (2001) 276:1923–9. doi: 10.1074/jbc.M004074200
135. Zhao L, Melenhorst JJ, Hennighausen L. Loss of interleukin 6 results in delayed mammary gland involution: A possible role for mitogen-activated protein kinase and not signal transducer and activator of transcription 3. Mol Endocrinol (2002) 16:2902–12. doi: 10.1210/me.2001-0330
136. Mercurio F, Manning AM. NF-kappaB as a primary regulator of the stress response. Oncogene (1999) 18:6163–71. doi: 10.1038/sj.onc.1203174
137. Li X, Massa PE, Hanidu A, Peet GW, Aro P, Savitt A, et al. IKKalpha, IKKbeta, and NEMO/IKKgamma are each required for the NF-kappa b-mediated inflammatory response program. J Biol Chem (2002) 277:45129–40. doi: 10.1074/jbc.M205165200
138. O’Sullivan J, Whyte L, Drake J, Tenniswood M. Alterations in the post-translational modification and intracellular trafficking of clusterin in MCF-7 cells during apoptosis. Cell Death Differ (2003) 10:914–27. doi: 10.1038/sj.cdd.4401254
139. Humphreys D, Hochgrebe TT, Easterbrook-Smith SB, Tenniswood MP, Wilson MR. Effects of clusterin overexpression on TNFalpha- and TGFbeta-mediated death of L929 cells. Biochemistry (1997) 36:15233–43. doi: 10.1021/bi9703507
140. Xie Z, Harris-White ME, Wals PA, Frautschy SA, Finch CE, Morgan TE. (clusterin) activates rodent microglia in vivo and in vitro. J Neurochem (2005) 93:1038–46. doi: 10.1111/j.1471-4159.2005.03065.x
141. Huttlin EL, Ting L, Bruckner RJ, Gebreab F, Gygi MP, Szpyt J, et al. The BioPlex network: A systematic exploration of the human interactome. Cell (2015) 162:425–40. doi: 10.1016/j.cell.2015.06.043
142. Burrage PS, Mix KS, Brinckerhoff CE. Matrix metalloproteinases: role in arthritis. Front Biosci (2006) 11:529–43. doi: 10.2741/1817
143. Jeong S, Ledee DR, Gordon GM, Itakura T, Patel N, Martin A, et al. Interaction of clusterin and matrix metalloproteinase-9 and its implication for epithelial homeostasis and inflammation. Am J Pathol (2012) 180:2028–39. doi: 10.1016/j.ajpath.2012.01.025
144. Radziwon-Balicka A, Santos-Martinez MJ, Corbalan JJ, O’Sullivan S, Treumann A, Gilmer JF, et al. Mechanisms of platelet-stimulated colon cancer invasion: role of clusterin and thrombospondin 1 in regulation of the P38MAPK-MMP-9 pathway. Carcinogenesis (2014) 35:324–32. doi: 10.1093/carcin/bgt332
145. Li Y, Lu J, Zhou S, Wang W, Tan G, Zhang Z, et al. Clusterin induced by N,N’-dinitrosopiperazine is involved in nasopharyngeal carcinoma metastasis. Oncotarget (2016) 7:5548–63. doi: 10.18632/oncotarget.6750
146. Chesnokova V, Zonis S, Wawrowsky K, Tani Y, Ben-Shlomo A, Ljubimov V, et al. Clusterin and FOXL2 act concordantly to regulate pituitary gonadotroph adenoma growth. Mol Endocrinol (2012) 26:2092–103. doi: 10.1210/me.2012-1158
147. Marongiu M, Marcia L, Pelosi E, Lovicu M, Deiana M, Zhang Y, et al. FOXL2 modulates cartilage, skeletal development and IGF1-dependent growth in mice. BMC Dev Biol (2015) 15:27. doi: 10.1186/s12861-015-0072-y
148. Marongiu M, Deiana M, Marcia L, Sbardellati A, Asunis I, Meloni A, et al. Novel action of FOXL2 as mediator of Col1a2 gene autoregulation. Dev Biol (2016) 416:200–11. doi: 10.1016/j.ydbio.2016.05.022
149. Malaise O, Tachikart Y, Constantinides M, Mumme M, Ferreira-Lopez R, Noack S, et al. Mesenchymal stem cell senescence alleviates their intrinsic and seno-suppressive paracrine properties contributing to osteoarthritis development. Aging (Albany NY) (2019) 11:9128–46. doi: 10.18632/aging.102379
150. Mi H, Ebert D, Muruganujan A, Mills C, Albou LP, Mushayamaha T, et al. PANTHER version 16: A revised family classification, tree-based classification tool, enhancer regions and extensive API. Nucleic Acids Res (2021) 49:D394–403. doi: 10.1093/nar/gkaa1106
151. Licata L, Lo Surdo P, Iannuccelli M, Palma A, Micarelli E, Perfetto L, et al. SIGNOR 2.0, the SIGnaling network open resource 2.0: 2019 update. Nucleic Acids Res (2020) 48(D1):D504–10. doi: 10.1093/nar/gkz949
152. Pahnke J, Mix E, Knoblich R, Muller J, Zschiesche M, Schubert B, et al. Overexpression of glial cell line-derived neurotrophic factor induces genes regulating migration and differentiation of neuronal progenitor cells. Exp Cell Res (2004) 297:484–94. doi: 10.1016/j.yexcr.2004.03.037
153. Arnold P, Otte A, Becker-Pauly C. Meprin metalloproteases: Molecular regulation and function in inflammation and fibrosis. Biochim Biophys Acta Mol Cell Res (2017) 1864:2096–104. doi: 10.1016/j.bbamcr.2017.05.011
154. Park JS, Lee WK, Kim HS, Seo JA, Kim DH, Han HC, et al. Clusterin overexpression protects against western diet-induced obesity and NAFLD. Sci Rep (2020) 10:17484. doi: 10.1038/s41598-020-73927-y
155. Mobasheri A, Rayman MP, Gualillo O, Sellam J, van der Kraan P, Fearon U. The role of metabolism in the pathogenesis of osteoarthritis. Nat Rev Rheumatol (2017) 13:302–11. doi: 10.1038/nrrheum.2017.50
156. Bradley D. Clusterin as a potential biomarker of obesity-related alzheimer’s disease risk. biomark Insights (2020) 15:1177271920964108. doi: 10.1177/1177271920964108
Keywords: clusterin, osteoarthritis, ingenuity pathway analysis, STRING, Cytoscape, connectome
Citation: Kovács P, Pushparaj PN, Takács R, Mobasheri A and Matta C (2023) The clusterin connectome: Emerging players in chondrocyte biology and putative exploratory biomarkers of osteoarthritis. Front. Immunol. 14:1103097. doi: 10.3389/fimmu.2023.1103097
Received: 19 November 2022; Accepted: 23 February 2023;
Published: 15 March 2023.
Edited by:
Pietro Ghezzi, University of Urbino Carlo Bo, ItalyReviewed by:
Magali Cucchiarini, Saarland University Medical Center, GermanyMary B. Goldring, Hospital for Special Surgery, United States
Copyright © 2023 Kovács, Pushparaj, Takács, Mobasheri and Matta. This is an open-access article distributed under the terms of the Creative Commons Attribution License (CC BY). The use, distribution or reproduction in other forums is permitted, provided the original author(s) and the copyright owner(s) are credited and that the original publication in this journal is cited, in accordance with accepted academic practice. No use, distribution or reproduction is permitted which does not comply with these terms.
*Correspondence: Csaba Matta, bWF0dGEuY3NhYmFAbWVkLnVuaWRlYi5odQ==; Ali Mobasheri, YWxpLm1vYmFzaGVyaUBvdWx1LmZp
†These authors share senior authorship