- 1Thoracic Service, Department of Surgery, Memorial Sloan Kettering Cancer Center, New York, NY, United States
- 2Department of Pathology, New York University (NYU) Grossman School of Medicine, New York, NY, United States
- 3Thoracic Oncology Service, Department of Medicine, Memorial Sloan Kettering Cancer Center, New York, NY, United States
- 4Center for Cell Engineering, Memorial Sloan Kettering Cancer Center, New York, NY, United States
Background: The attenuated, genetically engineered vaccinia virus has been shown to be a promising oncolytic virus for the treatment of patients with solid tumors, through both direct cytotoxic and immune-activating effects. Whereas systemically administered oncolytic viruses can be neutralized by pre-existing antibodies, locoregionally administered viruses can infect tumor cells and generate immune responses. We conducted a phase I clinical trial to investigate the safety, feasibility and immune activating effects of intrapleural administration of oncolytic vaccinia virus (NCT01766739).
Methods: Eighteen patients with malignant pleural effusion due to either malignant pleural mesothelioma or metastatic disease (non-small cell lung cancer or breast cancer) underwent intrapleural administration of the oncolytic vaccinia virus using a dose-escalating method, following drainage of malignant pleural effusion. The primary objective of this trial was to determine a recommended dose of attenuated vaccinia virus. The secondary objectives were to assess feasibility, safety and tolerability; evaluate viral presence in the tumor and serum as well as viral shedding in pleural fluid, sputum, and urine; and evaluate anti-vaccinia virus immune response. Correlative analyses were performed on body fluids, peripheral blood, and tumor specimens obtained from pre- and post-treatment timepoints.
Results: Treatment with attenuated vaccinia virus at the dose of 1.00E+07 plaque-forming units (PFU) to 6.00E+09 PFU was feasible and safe, with no treatment-associated mortalities or dose-limiting toxicities. Vaccinia virus was detectable in tumor cells 2-5 days post-treatment, and treatment was associated with a decrease in tumor cell density and an increase in immune cell density as assessed by a pathologist blinded to the clinical observations. An increase in both effector (CD8+, NK, cytotoxic cells) and suppressor (Tregs) immune cell populations was observed following treatment. Dendritic cell and neutrophil populations were also increased, and immune effector and immune checkpoint proteins (granzyme B, perforin, PD-1, PD-L1, and PD-L2) and cytokines (IFN-γ, TNF-α, TGFβ1 and RANTES) were upregulated.
Conclusion: The intrapleural administration of oncolytic vaccinia viral therapy is safe and feasible and generates regional immune response without overt systemic symptoms.
Clinical trial registration: https://clinicaltrials.gov/ct2/show/NCT01766739, identifier NCT01766739.
Introduction
With an estimated annual incidence of at least 150,000 patients in the United States, malignant pleural effusions (MPEs) occur in 15% of all patients with cancer during the course of their disease (1). In addition to causing symptoms that limit quality of life, such as shortness of breath that requires interventions (2), the presence of MPE represents advanced cancer and contributes to poor prognosis (3). Palliative interventions have been the mainstay for symptomatic relief and prevention of MPE recurrence that can interrupt cancer therapy in patients with MPE (2, 4). There has been limited success following systemic immune checkpoint inhibitor agent therapy, chemotherapy or a combination of chemo immunotherapies in patients with MPE (5, 6). Intrapleural biological therapies, such as oncolytic viral therapy and chimeric antigen receptor (CAR) T-cell therapy, have been investigated to promote effector immune responses in patients with MPEs (7–10). However, local immune suppressive mechanisms in MPEs that inhibit the efficacy of effector immune responses have been well described (11, 12). Specifically, macrophages and TGFβ in MPEs have been shown to play a pivotal role in hampering the antitumor immune responses (11, 13). Correlative analysis of pre- and post-treatment MPEs and pleural tumor biopsies to characterize the effector and suppressor immune responses following intrapleural therapies can shed light on changes in the immune microenvironment and aid in developing regimens to further enhance functional efficacy.
Malignant pleural mesothelioma (MPM), metastatic non-small cell lung cancer, and breast cancers are common causes of MPEs. Malignant pleural mesothelioma is a rare cancer with diffuse involvement of the pleural cavity. Immune checkpoint inhibitors have shown promising results in patients with MPM; however, the increase in survival is limited to mostly biphasic and sarcomatoid forms of MPM (14, 15). Epithelioid MPM, the most common form of MPM, is known to have the lowest tumor mutational burden and PD-L1 expression among solid tumors (16), with equivalent survival compared to chemotherapy (15). Regional oncolytic viral therapies that can generate effector immune responses in patients with MPM may provide an opportunity for subsequent immune checkpoint inhibitor agent therapy (17).
Oncolytic viruses, which selectively infect and exert cytopathic effects on tumor cells, are a potential therapeutic option for MPM. As a member of the poxvirus family of the genus orthopoxvirus, the vaccinia virus is one such oncolytic virus that possesses multiple favorable features for use as a therapeutic agent. It exhibits rapid cell-to-cell spread, is cytolytic across a broad range of tumor cell types, has a large insertion capacity for exogenous genes, and is genetically stable with low potential for mutagenesis (18). It is amenable to large-scale manufacture, storage and production, and is safe to administer intravenously (19).
In in vitro studies, the vaccinia virus has shown to be efficient in killing multiple cancer cell lines, including breast, lung, thyroid, prostate, pancreas, squamous cell carcinoma, and MPM (20). In in vivo studies, the vaccinia virus has caused tumor elimination in mouse models of breast cancer and MPM, as well as tumor growth inhibition in mouse models of lung adenocarcinoma, anaplastic thyroid cancer (21), prostate cancer (22), ovarian cancer, pancreatic cancer, and melanoma (23). Isolated case reports have documented complete remission in a patient with multiple myeloma (24) and a patient with chronic lymphocytic leukemia (25, 26) following vaccinia virus administration. Vaccinia virus has also been used in phase I clinical trials to treat patients with bladder cancer (27), metastatic melanoma (28), and advanced hepatocellular carcinoma (29, 30).
We conducted a single-center phase I clinical trial (NCT01766739) to study the intrapleural administration of attenuated vaccinia virus (GL-ONC1, Genelux Corporation) in patients with MPEs due to MPM or metastatic disease (non-small cell lung cancer or breast cancer). In 2019, the United States Adopted Names Council (USAN) granted Genelux adoption of the name Olvimulogene nanivacirepvec (referred to as Olvi-vec) in place of the name GL-ONC1; henceforth referred to as Olvi-vec throughout the manuscript.
Materials and methods
Trial design and patients
An open-label, dose-escalating, non-randomized, single-center phase I study was conducted to study the intrapleural administration of attenuated vaccinia virus (Olvi-vec) as a bolus. Olvi-vec was administered either as a single dose or as three consecutive daily doses to patients with a histologically or cytologically documented diagnosis of MPE, as detailed in the study protocol (Supplementary Material).
Study oversight
The study protocol and amendments were approved by the Memorial Sloan Kettering Cancer Center Institutional Review Board (IRB# 12-169, NCT01766739). All patients provided written informed consent to participate in the study, and all response and toxicity outcomes were documented. Patients were enrolled in groups of three and individually assessed for safety and dose-limiting toxicity. Inclusion and exclusion criteria are listed in the protocol (Supplementary Material). Patients were treated following the diagnosis of histologically or cytologically documented MPEs (due to primary non-small-cell lung carcinoma, MPM, and other histologies) and had free pleural space (partial or total) that permitted intrapleural drug instillation.
Olvi-vec manufacturing
A genetically engineered vaccinia virus, designated as GLV-1h68, was used in preclinical investigation. GLV-1h68 was derived from the LIVP strain by inserting RUC-GFP (a fusion gene of Renilla luciferase and green fluorescent protein), LacZ (beta-galactosidase), and gusA (beta-glucuronidase) expression cassettes into F14.5L (located between F14L and F15L), thymidine kinase (TK), and hemagglutinin loci, respectively. Disruption of these non-essential genes and expression of the foreign gene expression cassettes not only attenuated the virus but also enhanced its tumor-specific targeting. The GMP-derived material of this same virus is called Olvi-vec. Olvi-vec has been used primarily for all safety pharmacology and toxicological experiments, as well as for in vitro potency comparisons (in cell cultures) and in vivo potency comparisons (in tumorous animals). Details of the virus manufacturing process and analyses are described in the study protocol (Supplementary Material).
Intrapleural treatment
Eligible patients were admitted into the hospital for treatment on protocol. The pleural effusion was drained via insertion of a chest tube or pleural catheter (PleurX™ Catheter, Becton, Dickinson and Company, Franklin Lakes, NJ). A chest CT scan was performed to document drainage of the effusion and to assess the extent of pleural disease. Within 72 hours of the CT scan, the virus was instilled as a bolus into the pleural space via the chest tube or pleural catheter. Up to 150 ml of additional saline was used to flush the chest tube or pleural catheter to ensure that all the treatment drug was instilled into the pleural space. The chest tube or pleural catheter was left clamped for 4 hours (+/- 1 hour), after which it was reopened and placed to drainage in order to drain the pleural space. As dictated by the patient’s clinical status, the chest tube was either left inserted or removed until the surgical procedure (video-assisted thoracoscopic surgery, VATS) was performed 2-7 days after treatment to collect MPE and obtain pleural biopsy.
Study objectives and assessment
The primary objective of this study was to determine a recommended dose of Olvi-vec. The secondary objectives included the assessment of feasibility, safety and tolerability, evaluation of viral presence in the tumor, pleural fluid, serum, sputum, and urine, and evaluation of anti-vaccinia virus immune response. All patients were included in the reporting of adverse events (AEs). The safety of Olvi-vec was assessed by the evaluation of the type, frequency, and severity of AEs, changes in clinical laboratory tests (hematological and chemistry), immunogenicity, and physical examination. All AEs and laboratory toxicities were graded using the Common Terminology Criteria for Adverse Events (National Cancer Institute, version 4.0). Laboratory testing was performed at baseline (i.e., within 14 days before treatment), daily during the first 3 days after treatment, and at termination of study (day 60 ± 5).
Hematoxylin and eosin staining
Hematoxylin and eosin staining was performed on FFPE blocks of tumor biopsies collected before (pre-treatment) and 2-5 days after (post-treatment) Olvi-vec therapy. Semi-quantitative scoring of tumor cell density and immune cell density (0: very low density; 1: low density; 2: moderate density; 3: high density) was performed by a primary and secondary pathologist who were blinded to sample identity.
Multiplex immunofluorescence staining
Multiplex immunofluorescence (mIF) staining was performed on tumor biopsies collected before (pre-treatment) and 2-5 days after (post-treatment) Olvi-vec therapy. Formalin-fixed and paraffin-embedded (FFPE) blocks were cut into sections of 5 µm thickness. Sections from each biopsy were stained with antibodies (Supplementary Table 1) using the Opal™ 7-Color Kit for Multiplex Immunohistochemistry (Akoya Biosciences, Marlborough, MA). After mIF staining, slides were scanned using the Vectra® 3.0 Automated Quantitative Pathology Imaging System (PerkinElmer Inc., Hopkinton, MA). Quantitative assessment of cell markers was performed using inForm® software (version 2.2.1, PerkinElmer Inc., Hopkinton, MA). Cell segmentation and phenotyping algorithms were reviewed and confirmed by study pathologists.
Viral plaque assay and vaccinia virus neutralization assay
Viral plaque assays were performed on body fluid samples (blood, sputum, urine, pleural fluid) collected from patients immediately before and 24, 48, 72, and 96 hours after Olvi-vec treatment to assess for the presence of viral particles. Post-treatment tumor biopsies collected 2-5 days after treatment also underwent assessment for viral particles using viral plaque assays. In brief, patient samples were plated on confluent layers of CV-1 cells. Evaluation of virus infection was done by visual assessment of viral plaque in wells with both CV-1 cells and patient samples. Additionally, post-treatment serum samples obtained from patients 60 days after Olvi-vec treatment were assessed for the presence of Olvi-vec neutralizing antibodies via standard vaccinia virus neutralization assay and compared to corresponding pre-treatment serum samples.
Effusion and pleural biopsy analysis
Pleural fluid and serum samples were obtained from patients both pre-treatment (baseline) and post-treatment (at 24, 48, 72, and 96 hours, and on days 2, 3, and 60). All specimens available were assessed for a panel of effector and suppressive cytokines using a 41-plex MILLIPLEX® MAP Human Cytokine/Chemokine kit (MilliporeSigma, Burlington, MA). The kit was run on a Luminex® 100/200™ System (Luminex Corporation, Austin, TX).
Values represent the mean of the duplicate wells ± standard deviation. These data were analyzed using IS 2.3 software (Luminex Software, Inc., Riverside, CA), Microsoft Excel and GraphPad Prism. Additionally, RNA isolation was performed on FFPE sections of tumor biopsies taken pre- and post-treatment using the RNeasy® FFPE Kit in accordance with the manufacturer’s protocol (Qiagen, Germantown, MD). RNA concentration and purity were measured using the NanoDrop™ 2000/2000c Spectrophotometer (Thermo Scientific, Waltham, MA). RNA profiling was performed using the nCounter® PanCancer Immune Profiling Panel by NanoString Technologies, Inc. (Seattle, WA).
Statistical analyses
The sample size was based on a standard dose-escalation design. All statistical tests were two-sided, and statistical significance was defined as p<0.05. Data with normal distribution was assessed using paired t-test. Data without normal distribution was assessed using Wilcoxon matched-pairs signed-rank test. Analyses were conducted using R 4.1.2 (R Foundation for Statistical Computing, Vienna, Austria).
Results
Patient characteristics
From February 2013 to April 2015, 18 patients were enrolled who were treated in a dose-escalating fashion (Table 1). Fifteen patients had MPM (13 epithelioid, 1 biphasic, and 1 sarcomatoid), 2 had non-small cell lung cancer (1 adenocarcinoma and 1 squamous cell carcinoma), and 1 had triple negative breast cancer. 5 out of 18 patients were female, and the mean age of all patients was 66 years. All 3 patients with NSCLC or breast cancer and 1 patient with MPM had received previous lines of therapy.
Feasibility and safety
Attenuated vaccinia virus (Olvi-vec) was administered intrapleurally as a bolus through a pleural catheter after complete evacuation of pleural effusion in all patients (Figure 1). The intrapleural administration of vaccinia virus was feasible, and there were no failures in administration of the agent. Table 2 lists the adverse events that occurred at any grade (1–4) in ≥15% of the total cohort (n=18), up to day 60 post-treatment.
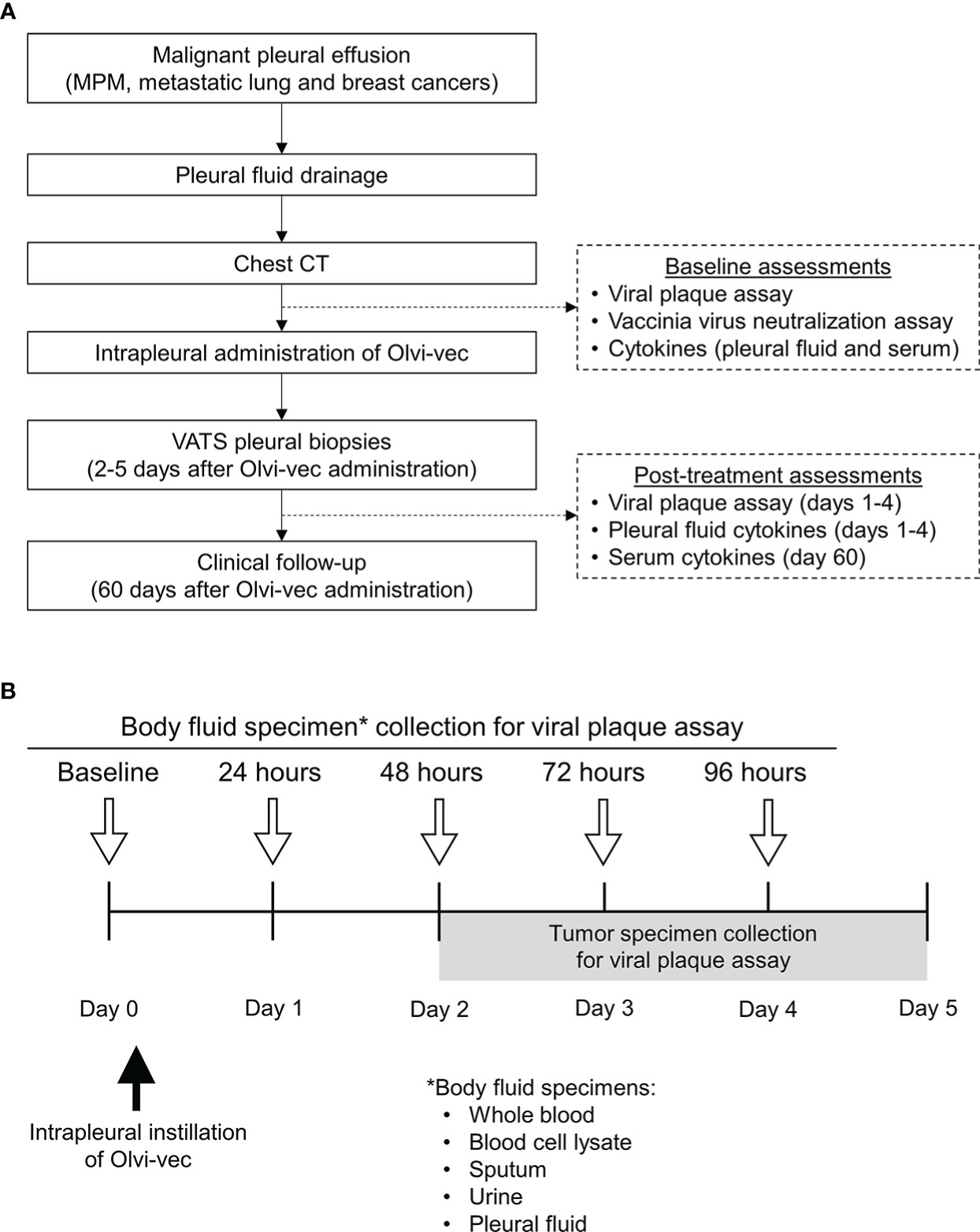
Figure 1 Protocol schema (A) and specimen collection schema for viral plaque assay (B). *List of specimens collected.
There was 1 reversible grade-4 laboratory abnormality (hypocalcemia). The most frequent grade 3 adverse events were lymphopenia (3 patients, 17%), fatigue (2 patients, 11%), and hypophosphatemia (2 patients, 11%). The most frequent grade 2 adverse events were anemia (5 patients, 28%), hyperglycemia (5 patients, 28%), and fever (4 patients, 22%). There were no dose-limiting toxicities, and maximally tolerated dose was not reached. As a result, the primary objective of establishing a recommended dose was not reached.
Vaccinia detection and qualitative assessment of treatment effect in tumor
Resected post-treatment samples from 14 patients were stained by immunohistochemistry with an antibody against Olvi-vec (A27L). Positive cytoplasmic expression was observed in 7 of 14 specimens (representative images shown in Figures 2A, B). When the A27L antibody was tested in a multiplex immunofluorescence panel with anti-mesothelin antibody on pre- and post-treatment specimens from Patient #16, cytoplasmic expression of Olvi-vec was observed in the post-treatment specimen but not the pre-treatment specimen (representative images shown in Figure 2C).
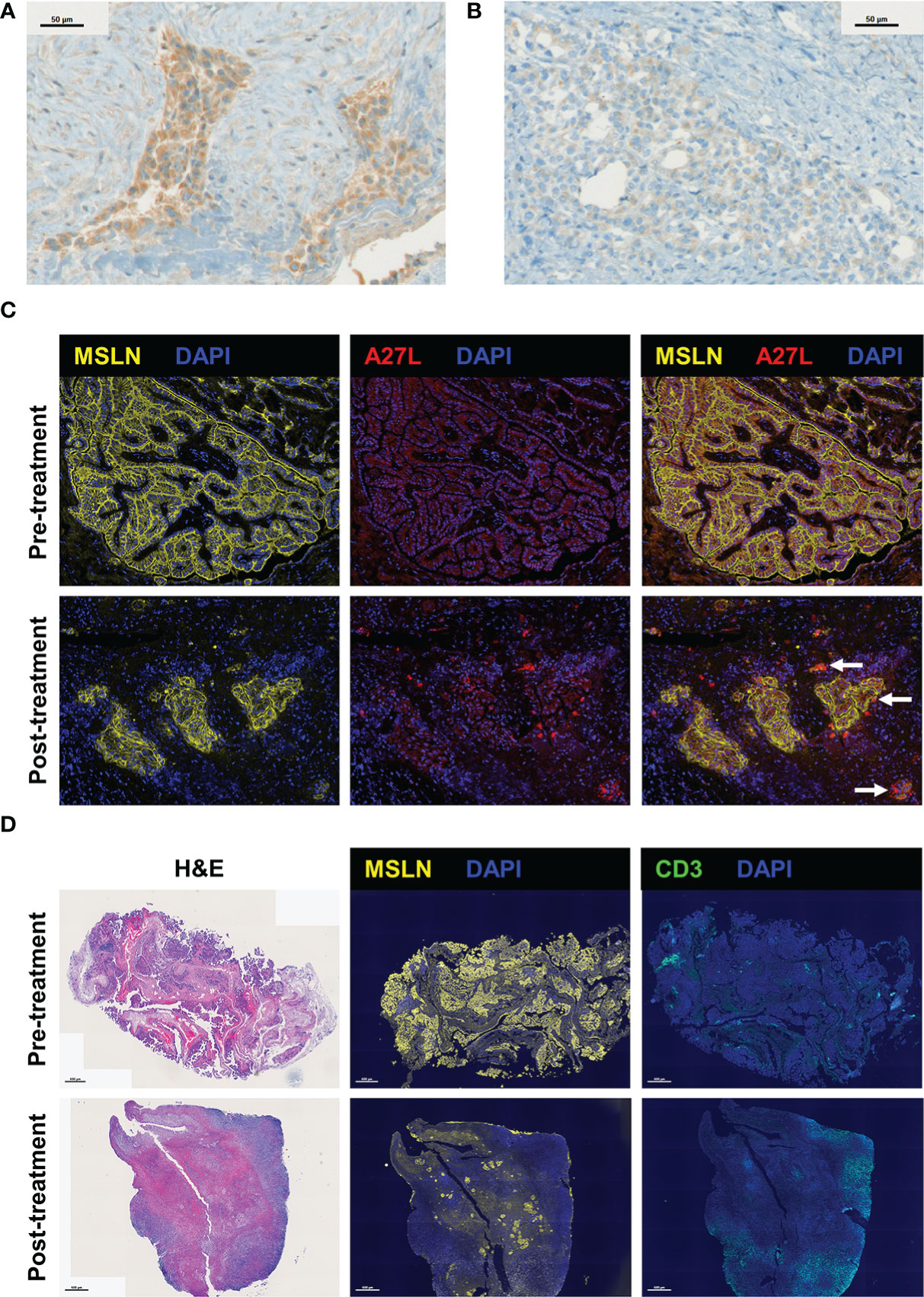
Figure 2 Visualization of Olvi-vec within tumor cells and the immune cell infiltrate in malignant pleural mesothelioma (MPM) tumors following Olvi-vec treatment. (A) Representative image of an immunohistochemistry (IHC)-stained section of a post-treatment tumor specimen from Patient #1 showing cytoplasmic positivity for Olvi-vec. (B) Representative image of an IHC-stained section from Patient #1 nine months later, showing weak/absent Olvi-vec staining. (C) Representative multiplex immunofluorescence (mIF) images of pre- and post-treatment tumor specimens from Patient #16, stained with anti-mesothelin and A27L (anti-Olvi-vec) antibodies. Arrows indicate tumor cells with cytoplasmic positivity for Olvi-vec, which are observed in the post-treatment specimen but not the pre-treatment specimen. (D) Representative images of pre- and post-treatment tumor specimens from Patient #16 stained with hematoxylin and eosin (H&E) (left panel), anti-mesothelin (MSLN) antibody (middle panel), and anti-CD3 antibody (right panel) in three consecutive cut sections. In the post-treatment specimen compared to pre-treatment, the density of tumor cells positive for MSLN is observed to be lower, and the density of immune cells positive for CD3 is observed to be higher.
Resected samples from 13 patients were stained by multiplex immunofluorescence with a panel of antibodies against mesothelin (MSLN), CD3, CD4, CD8, and FoxP3. 4 patients had matched pre- and post-treatment specimens, and 9 patients had post-treatment specimens only. The density of MSLN+ tumor cells was qualitatively observed to decrease and the density of CD3+ immune cells was qualitatively observed to increase from pre-treatment to post-treatment specimens (representative images shown in Figure 2D).
Quantitative assessment of treatment effect in tumor
Resected samples from 16 patients were stained with hematoxylin and eosin and independently scored semi-quantitatively for tumor-cell density and immune-cell density by two pathologists (Table 3). Four patients had matched pre- and post-treatment specimens, and 12 patients had post-treatment specimens only. When matched tumor specimens were compared (n=4), tumor cell density score decreased from pre-treatment to post-treatment in all patients (Figure 3). Immune cell density score increased from pre-treatment to post-treatment in 3 of 4 patients. Among all post-treatment tumor specimens (n=16), the average score per high power field (1 mm2) was lower for tumor cell density compared to immune cell density.
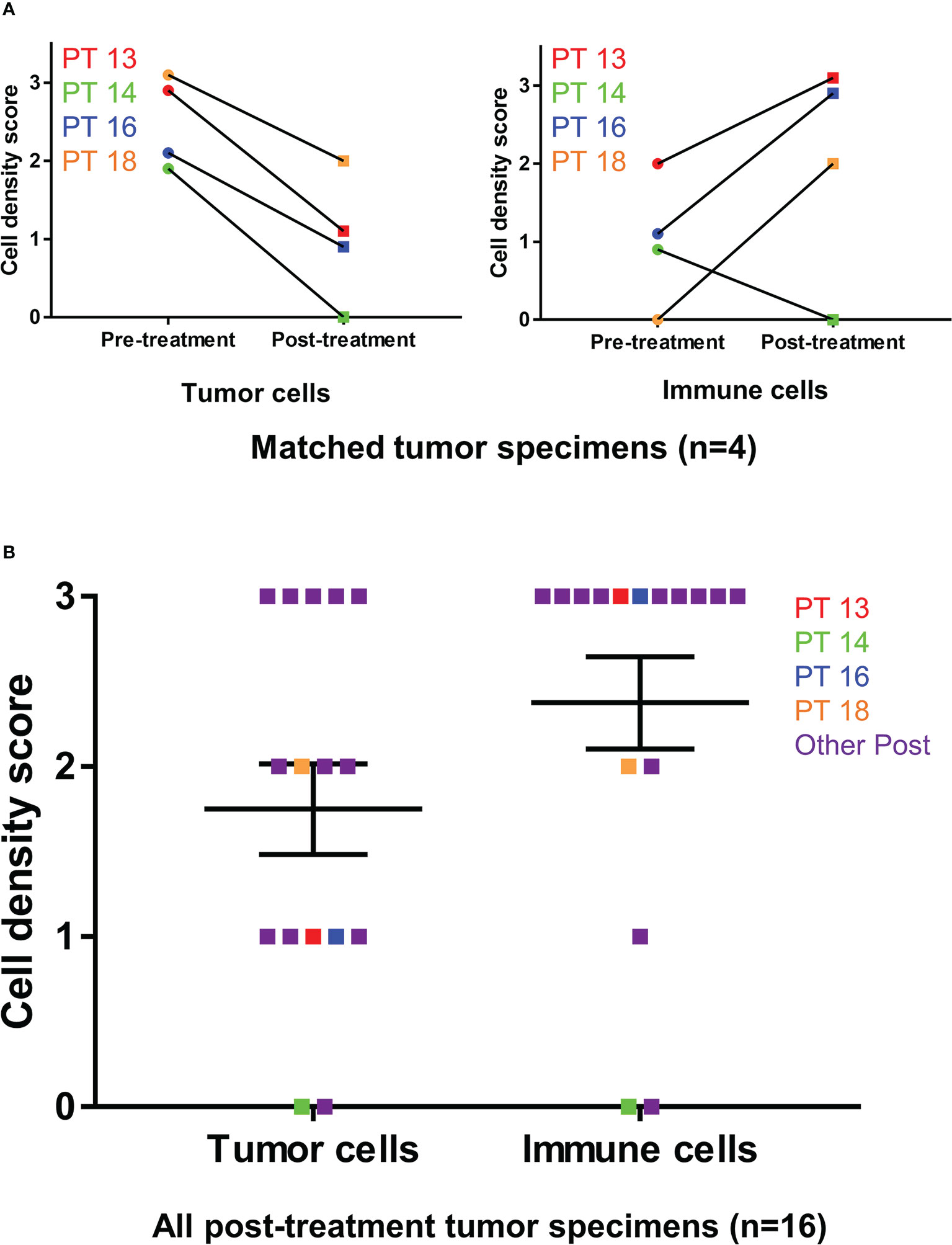
Figure 3 Tumor cell and immune cell density scoring before and after Olvi-vec therapy. (A) Matched MPM tumor sections obtained before and after Olvi-vec therapy underwent staining with hematoxylin and eosin and were scored for tumor cell density and immune cell density. (B) Tumor cell density scores and immune cell density were scored for all post-treatment tumor sections. Bars indicate mean and standard error of the mean (SEM).
When matched tumor specimens were stained using multiplex immunofluorescence and then compared (n=4), mean CD8+ cells per mm2 increased in 3 of 4 patients (Figure 4). Mean MSLN+ tumor cells per mm2 decreased from pre-treatment to post-treatment in all patients. Comparing available pre-treatment specimens to all post-treatment specimens (n=13), mean CD8+ cells increased and mean MSLN+ tumor cells decreased, although neither achieved statistical significance (Figure 5).
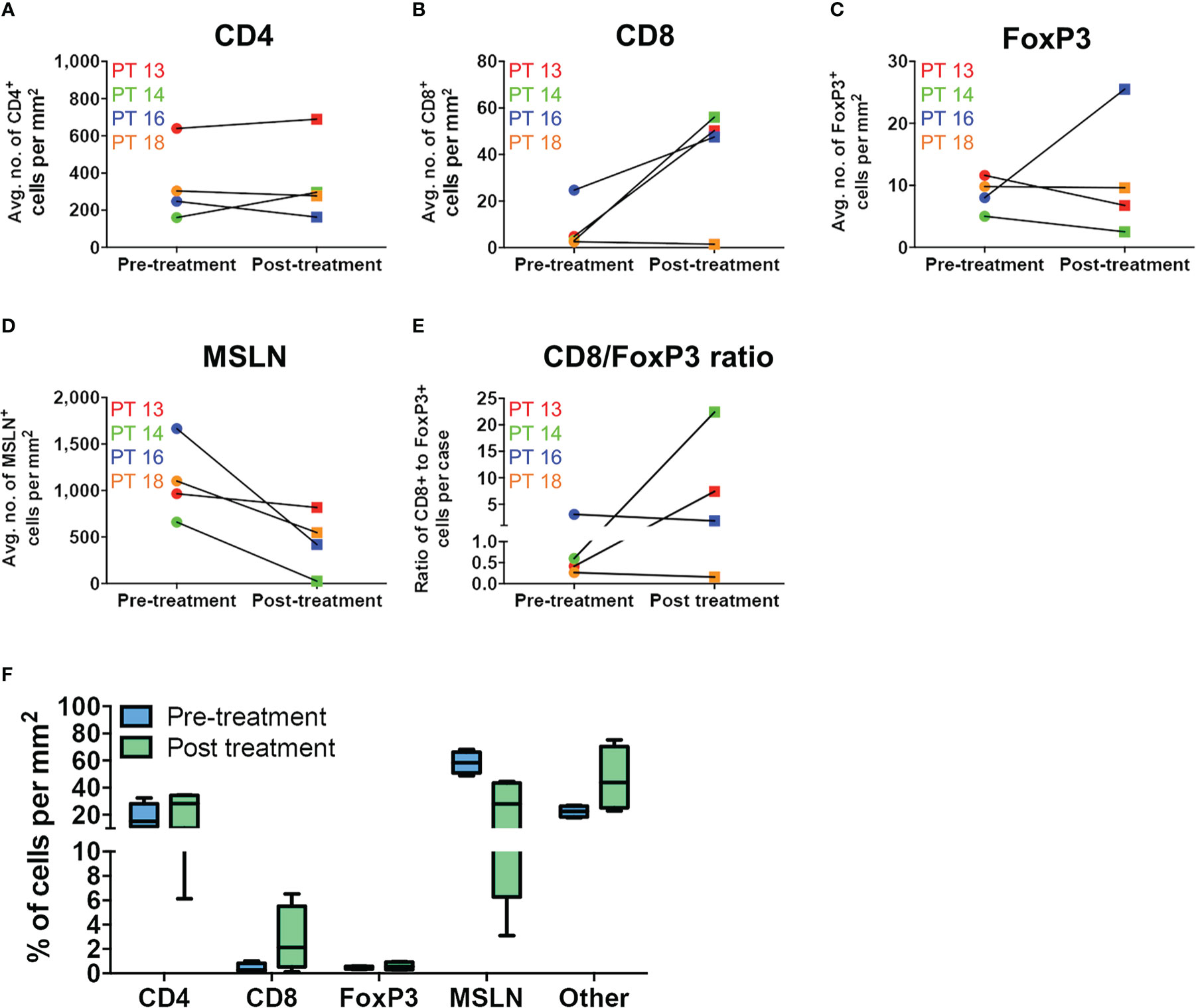
Figure 4 Immune cell infiltration in matched MPM tumor specimens before and after Olvi-vec therapy. (A–D) Matched MPM tumor sections obtained before and after Olvi-vec therapy underwent mIF staining and quantification of (A) CD4+ T cells, (B) CD8+ T cells, (C) FoxP3+ T cells, and (D) mesothelin (MSLN)+ tumor cells. (E) The ratio of CD8+ to FoxP3+ T cells in pre- and post-treatment tumor sections was calculated to identify patients with immunogenic (CD8+/FoxP3+ >1) vs. immune suppressive (CD8+/FoxP3+ <1) tumor microenvironments. (F) Immune cell populations present in matched pre- and post-treatment tumor sections were expressed as a percentage of total cells per mm2. “Other” cells are DAPI+ but negative for CD4, CD8, FoxP3, and MSLN—they may be fibroblasts, mesothelial cells, or other classes of immune cells. Bars indicate mean, interquartile range, and SEM.
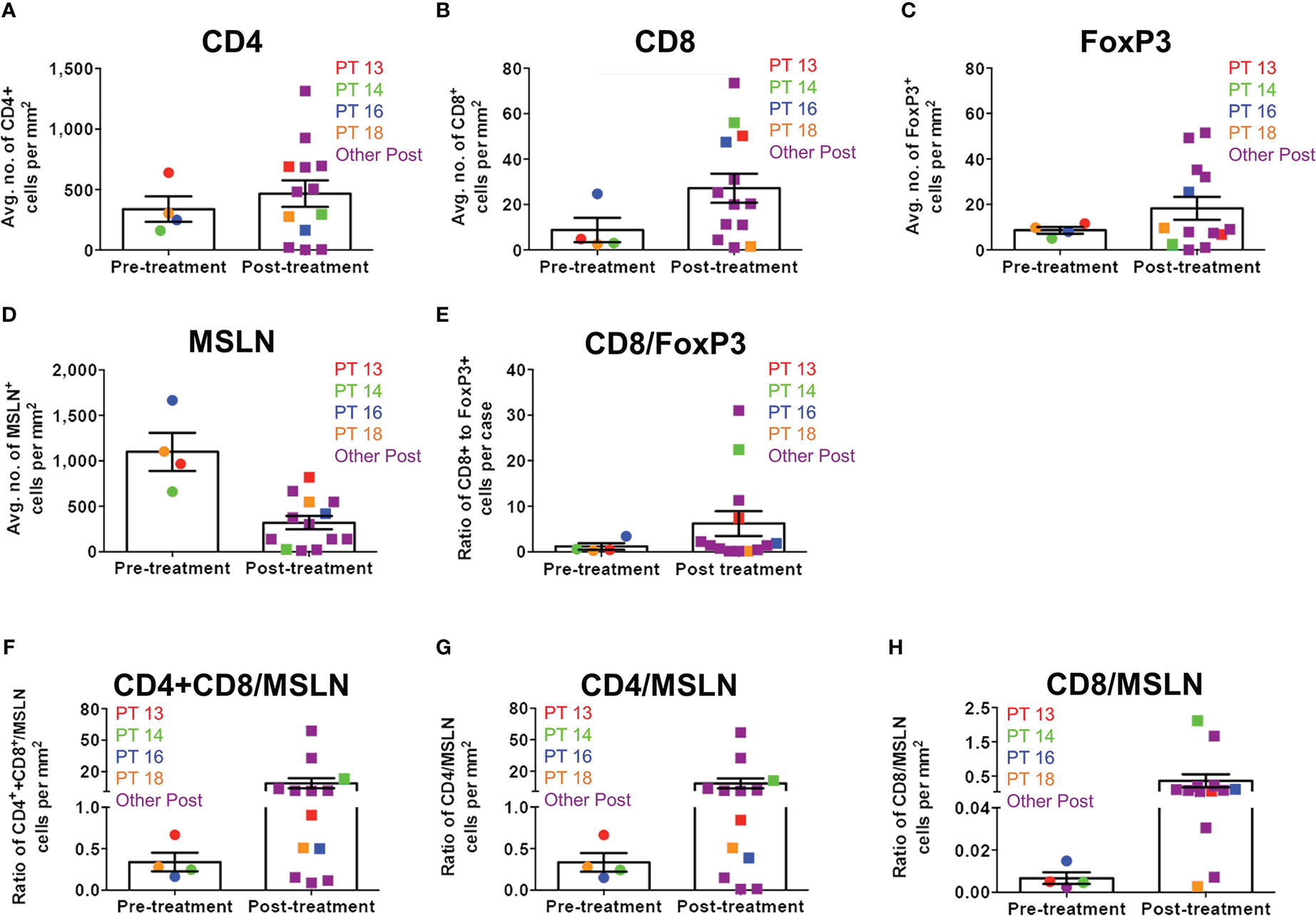
Figure 5 Immune cell infiltration ratios in all MPM tumor specimens before and after Olvi-vec therapy. (A–D) MPM tumor sections obtained before and after Olvi-vec therapy underwent multiplex immunofluorescence (mIF) staining and quantification of (A) CD4+ T cells, (B) CD8+ T cells, (C) FoxP3+ T cells, and (D) mesothelin (MSLN)+ tumor cells. (E) The ratio of CD8+ to FoxP3+ T cells (high ratio indicating relative immunogenicity, low ratio indicating relative immune suppression) in pre- and post-treatment tumor sections was calculated. (F–H) Ratios of (F) total T cells (CD4+ and CD8+) to MSLN+ tumor cells, (G) CD4+ T cells to MSLN+ tumor cells, and (H) CD8+ T cells to MSLN+ tumor cells were calculated. Bars indicate mean and SEM.
Gene expression analysis of pre- and post-treatment tumor specimens (n=16), using nCounter immune cell type scoring module, revealed increased scores (i.e., change in score by 1 unit, indicating twice the abundance of that cell type) for CD45+, Th1+, Tregs, CD8+, exhausted CD8+, NK+, cytotoxic cells, dendritic cells, macrophage, and neutrophil immune cell populations in post-treatment tumor specimens compared to pre-treatment specimens (Figure 6). Similarly, scoring of individual protein mRNA levels pre- and post-treatment tumor specimens (n=13) revealed increased scores in immune effector proteins (IFN-γ, granzyme B, perforin), immune suppressive proteins (TGFβ, FoxP3), and immune checkpoint regulatory proteins (PD-1, PD-L1, PD-L2) following treatment (Figure 7). The data discussed in this publication have been deposited in NCBI’s Gene Expression Omnibus (31) and are accessible through GEO Series accession number GSE223395 (https://www.ncbi.nlm.nih.gov/geo/query/acc.cgi?acc=GSE223395).
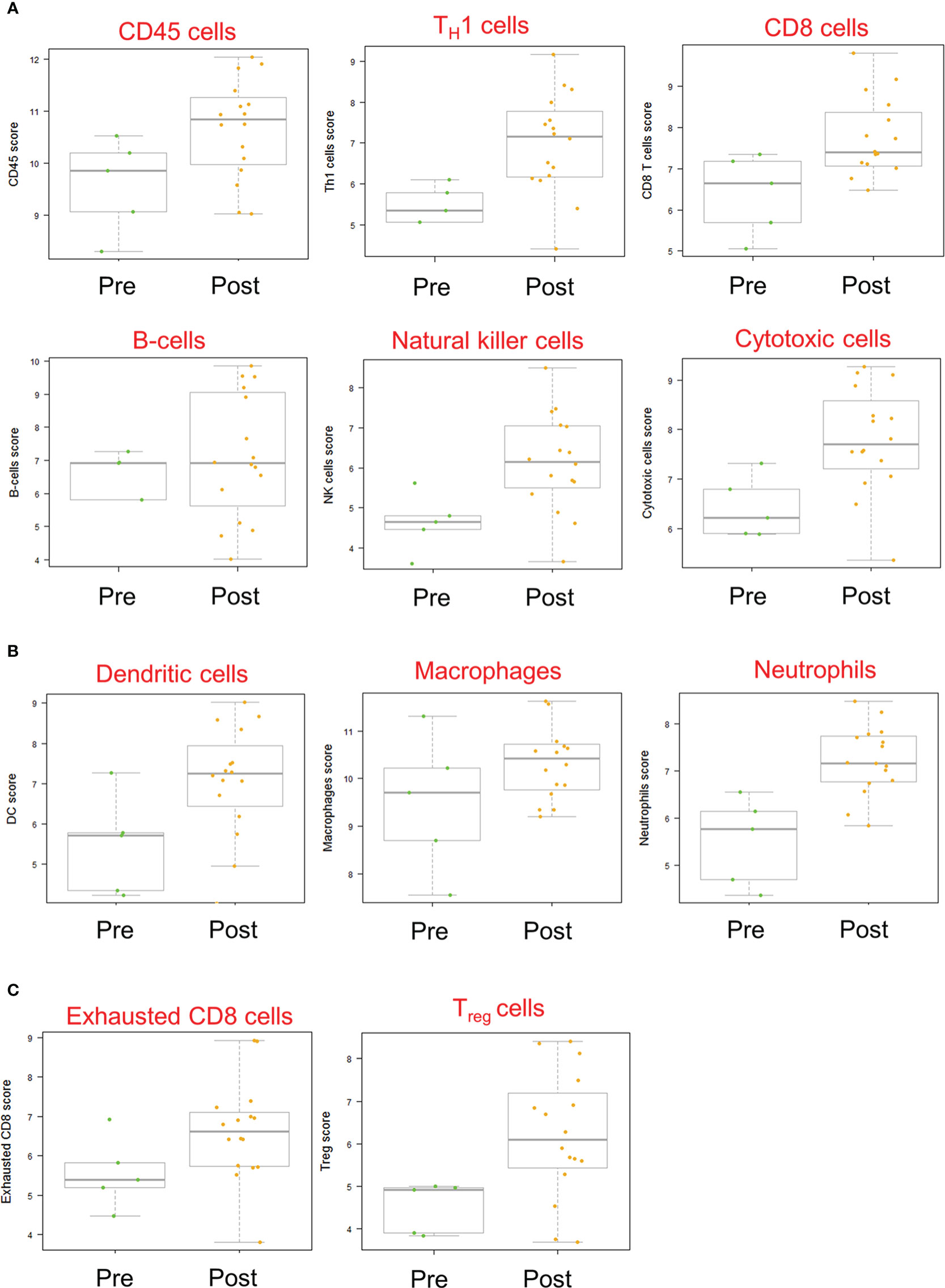
Figure 6 Immune cell scores based on mRNA transcript abundance before and after Olvi-vec therapy. (A–C) Total RNA was isolated from MPM tumor sections before and after Olvi-vec therapy. The number of mRNA transcripts specific to (A) lymphoid, (B) myeloid, and (C) exhausted and regulatory cell types were quantified. A score increase of one indicates a two-fold increase in cell population abundance in a sample. Bars indicate mean, interquartile range, and range.
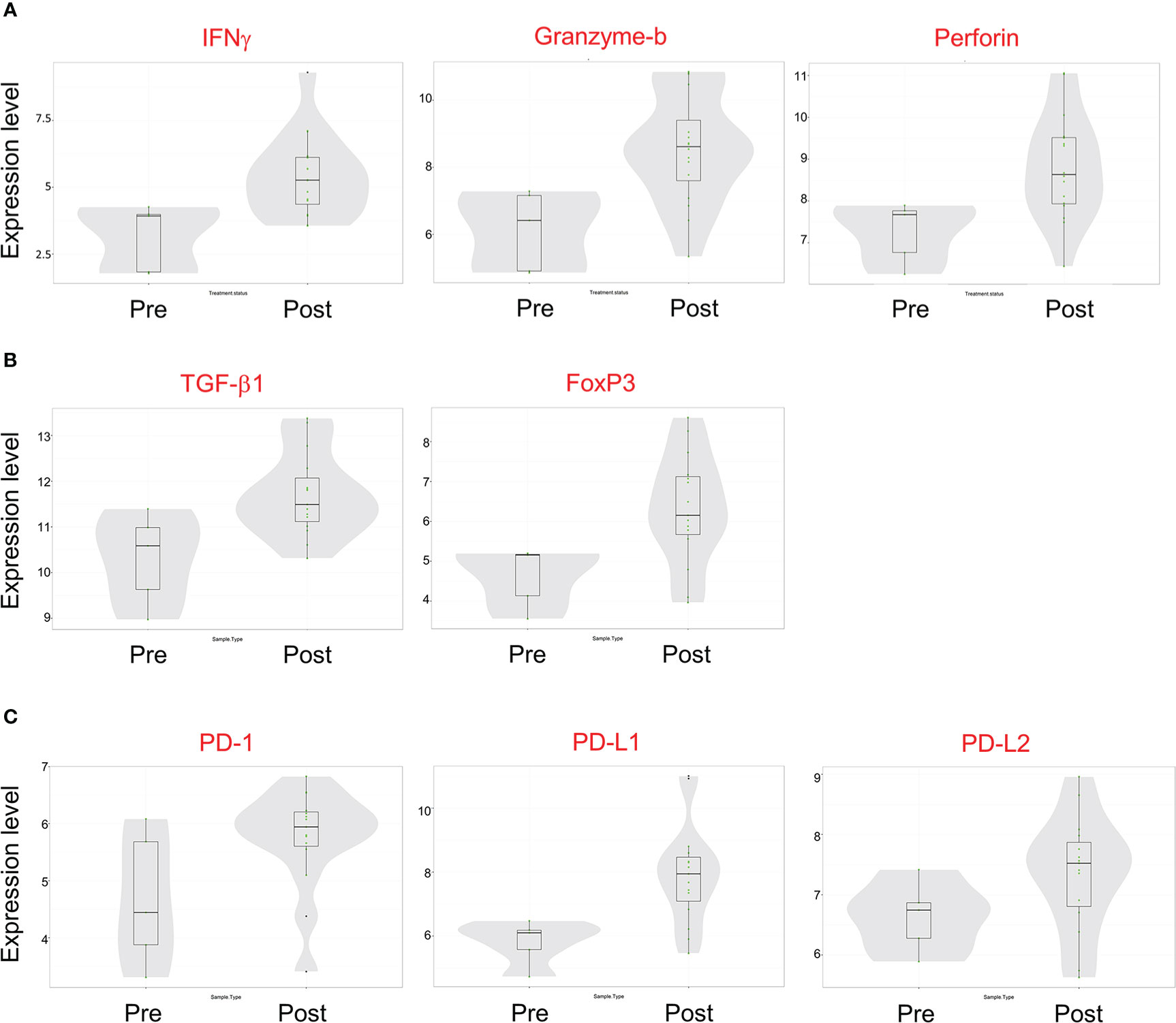
Figure 7 mRNA transcript expression of immune-modulatory proteins before and after Olvi-vec therapy. (A–C) Total RNA was isolated from MPM tumor sections before and after Olvi-vec therapy. The number of mRNA transcripts for (A) immune effector proteins, (B) immune suppressive proteins, and (C) immune checkpoint regulatory proteins were quantified. Bars indicate mean, interquartile range, and range.
Vaccinia detection in body fluids and anti-vaccinia immune response
Vaccinia virus was detectable by viral plaque assay in the pleural fluid of 7 patients (Table 4A). Vaccinia was initially detected in pleural fluid at the dose 1.00E8 PFU (Cohort 2) and exhibited dose-dependent increase in PFU/mL in Cohorts 3, 4, 5, and 6. Viral plaque assay was also positive for vaccinia in the post-treatment tumor lysate of 4 patients. There was minimal viral shedding into other compartments, as shown by low positivity in the urine of 2 patients, blood lysate of 1 patient, and sputum of 1 patient (Table 4B). Of note, among patients who received multiple doses of Olvi-vec (Cohorts 5 and 6), significant increase in the number of plaque-forming units was observed in the pleural fluid of 2 patients.
Among 7 patients whose baseline serum was available to perform vaccinia virus neutralization assay, 4 patients had low levels of anti-vaccinia neutralizing antibodies pre-treatment, and 3 had no neutralizing antibodies (Table 5). Five of the patients had high levels of neutralizing antibodies at day 60 post-treatment.
Pleural fluid and serum cytokine analysis
Luminex analysis of pleural fluid specimens from baseline to up to 96 hours following treatment indicated significant increase in the levels of the following cytokines by 48 hours: IFN-γ, TNF-α, VEGF, IL-1ra, IL-1β, and IP-10 (Figure 8). In contrast, analysis of serum specimens showed an increase only in IL-8 levels from baseline to day 3 following treatment (p=0.0065; Figure 8). By day 60, only RANTES (CCL5) was found to be significantly elevated in serum compared to baseline (p=0.0276; Figure 9).
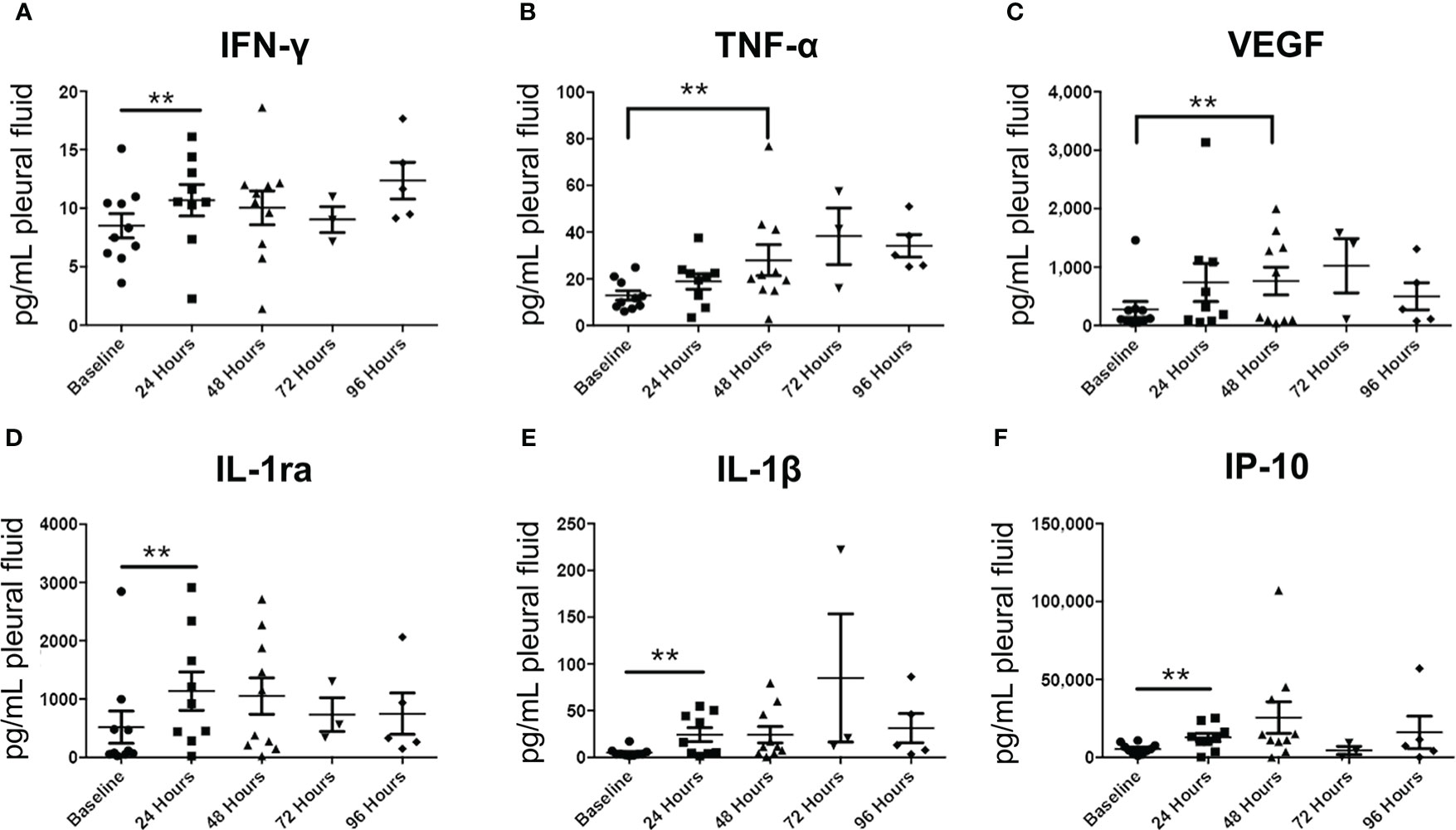
Figure 8 Pleural fluid cytokine profiles before and after Olvi-vec therapy. (A–F) Pleural fluid sampled before and after Olvi-vec therapy (at 24 hours, 48 hours, 72 hours, and 96 hours post-treatment) was evaluated for concentrations of cytokines. Bars indicate mean and SEM. **p<0.05, paired t-test.
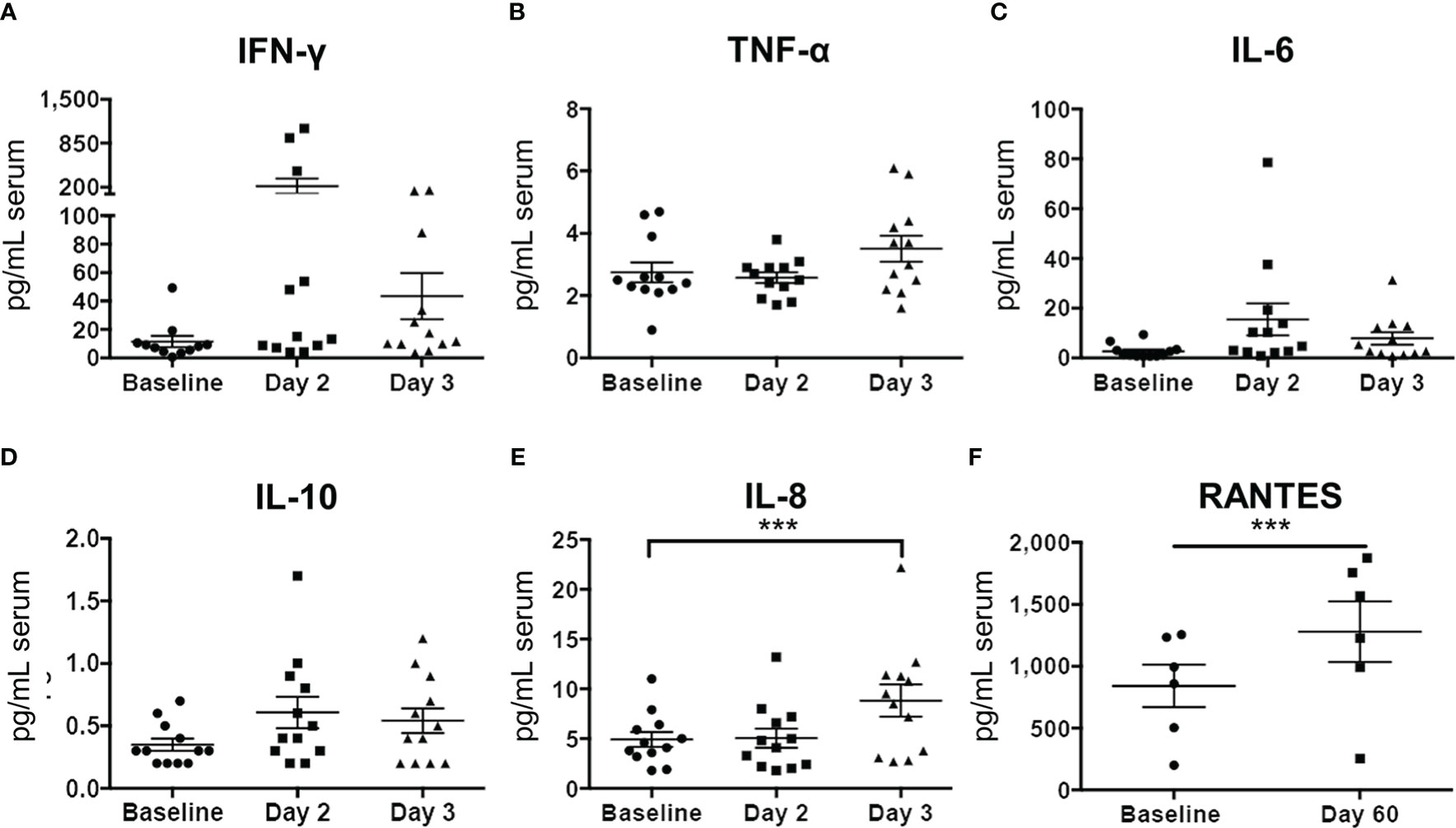
Figure 9 Serum cytokine profiles before and after Olvi-vec therapy. (A–F) Serum sampled before and after Olvi-vec therapy (at Day 2, Day 3, and Day 60) was evaluated for concentrations of cytokines. Bars indicate mean and SEM. ***p<0.01, paired t-test.
Long-term outcomes
All patients received subsequent other therapies as determined by treating physician following participation in the trial. Among all patients, median overall survival (OS) was 19.5 months. The median OS among patients who had MPM was 22 months (Figure 10). One patient with epithelioid MPM is alive; 87 months after treatment, the patient received other treatments that are standard of care for patients with MPM.
Discussion
Our phase I study of intrapleural oncolytic viral therapy is based on strong rationale developed in preclinical models of malignant pleural mesothelioma (32–34). The strength of our phase I study is the correlative analysis performed on pre- and post-treatment pleural effusions and pleural biopsies along with systemic immune response assessment by cytokine analysis following intrapleural oncolytic viral therapy. Intrapleural administration of Olvi-vec treatment is feasible, safe, and associated with induction of effector immune responses. All patients received treatment with the established dose via intrapleural delivery. Olvi-vec was detected using direct and indirect methods in resected tumor specimens and pleural fluid collected post-treatment. The treatment was safe, with one grade 4 laboratory abnormality and no treatment-associated mortalities noted. There were no dose-limiting toxicities or dose de-escalations, and the maximally tolerated dose was not reached. Therefore, a recommended dose was not established. Presence of the vaccinia virus within tumor cells was detectable 2-5 days after treatment and associated with local reduction in tumor cell density and an increase in immune cell density. Specifically, CD8+ T-cell density increased, indicating the generation of treatment-induced immunogenicity. Gene expression analysis showed increases in multiple immune cell populations (including CD8+, CD45+, Th1+, Tregs, NK cells, macrophages, neutrophils, dendritic cells, and cytotoxic cells), as well as increased concentration of effector proteins, immune checkpoint proteins, and cytokines in post-treatment tumor and pleural fluid samples. Viral shedding outside the pleural compartment was observed in only 4 patients. The number of plaque-forming units in pleural fluid was significantly increased in 2 patients who received multiple doses of Olvi-vec (cohorts 5 and 6). Most importantly, there was minimal systemic immune activation following intrapleural treatment, with only 2 cytokines noted to be elevated in serum. Among trial patients who had MPM, median OS was 22 months following treatment.
Our observations in treating patients with MPE-associated immunosuppressive microenvironment are similar to published studies of vaccinia viral therapy without immunosuppression at the administered site. Administered as systemic therapy in clinical trials, vaccinia viral therapy was associated with a trend toward improved progression-free survival and increased CD4+/Treg ratio in patients with metastatic breast cancer (35). In patients with advanced colorectal liver metastases and metastatic melanoma, vaccinia viral therapy was associated with significant increases in IFN-stimulated and pro-inflammatory cytokines, as well as NK-cell activation and CD8+ proliferation (36). Vaccinia viral therapy had no observed benefit in patients with advanced soft tissue sarcoma (37).
Intrapleural oncolytic viral therapy has been investigated by use of multiple oncolytic viruses. In patients with MPM, intrapleural delivery of adenoviral gene-mediated cytotoxic therapy has been investigated. Adenoviral vectors have been used for gene transfer of cytokines [such as IFN-α (8) and IFN-β (38)] and enzymes (such as TK) that potentiate cytotoxic activity of subsequently administered ganciclovir (39) or valacyclovir (7). These trials reported isolated instances of grade 4 pericardial tamponade (38), grade 4 hypotension (7), and severe flu-like symptoms requiring dose de-escalation (8). We did not observe any dose-limiting or treatment-related grade ≥4 toxicities in our trial. Adenovirus-mediated IFN-β and IFN-α gene transfer were reported to be associated with increases in activated NK-cell populations (8, 40). Our correlative data noted a 2-fold increase in NK cells based on gene expression analysis. Adenovirus-mediated TK and IFN gene transfers were associated with survival greater than 2 years in multiple patients (7, 8, 40, 41), as was observed following vaccinia treatment in our study. However, the survival observed cannot be attributed to the treatment agent due to the phase I nature of the study and limited clinical anti-tumor efficacy observed immediately after the treatment.
Following adenovirus-mediated TK/ganciclovir therapy, gene transfer was observed to be limited to superficial layers of the tumor (39). Yet, radiologic response and persistent clinical response were observed in 3 patients (41), prompting the authors to hypothesize that the therapy had immune activating effects in addition to direct cytotoxicity. Indeed, administration of adenovirus TK has been shown to increase PD-L1 expression on tumor cells (7). In the current trial we observed increased expression of PD-1, PD-L1, and PD-L2 mRNA transcripts following vaccinia virus treatment. We also observed presence of vaccinia virus in the tumor as identified by multiplex immunofluorescence along with associated immune activation signature by nanostring and cytokine analyses. Oncolytic virus-induced tumor cytotoxicity may potentially shift the balance of the immune microenvironment towards activation through pathogen-associated and damage-associated molecular pattern signaling. These observations provide rationale to the addition of checkpoint blockade to vaccinia virus treatment (42) to enhance immune activation and antitumor efficacy. In preclinical studies, vaccinia virus combined with anti-PD1 therapy caused tumor reduction in glioblastoma (42), and vaccinia virus combined with MEK inhibitory therapy resulted in enhanced cytotoxicity in doxorubicin-resistant ovarian cancer (43).
In addition to effector immune responses (an increase in CD45+, Th1+, CD8+, NK+, cytotoxic T cells, and dendritic cells), we also observed an increase in exhausted CD8+ T cells and macrophages indicating the suppressor immune response. However, it is not certain whether these alterations are limited to tumors with pre-existing immune suppressor responses that are augmented following vaccinia viral therapy. In addition, our clinical trial was limited by the inclusion of a small number of patients from a single institution, who had heterogeneous types and stages of disease. Not all specimens investigated were available from all patients, a limitation inherent in a phase I clinical trial. Nevertheless, our correlative analyses demonstrating immune activation support the potential utility of vaccinia virus as an intrapleural oncolytic treatment for patients with MPM.
Data availability statement
The data discussed in this publication have been deposited in NCBI’s Gene Expression Omnibus (31) and are accessible through GEO Series accession number GSE223395 (https://www.ncbi.nlm.nih.gov/geo/query/acc.cgi?acc=GSE223395).
Ethics statement
The studies involving human participants were reviewed and approved by the Memorial Sloan Kettering Cancer Center Institutional Review Board (IRB# 12-169). The patients/participants provided their written informed consent to participate in this study.
Author contributions
NC: Data curation, Formal analysis, Investigation, Methodology, Software, Validation, Visualization, Roles/Writing – original draft, Writing – review & editing; JC: Data curation, Formal analysis, Investigation, Methodology, Software, Validation, Visualization, Roles/Writing – original draft, Writing – review & editing; EM: Data curation, Formal analysis, Investigation, Methodology, Writing – review & editing; RB: Data curation, Investigation, Writing – review & editing; JS: Data curation, Investigation, Writing – review & editing; SB: Data curation, Investigation, Writing – review & editing; AM: Data curation, Investigation, Writing – review & editing; MZ: Data curation, Investigation, Writing – review & editing; PA: Conceptualization, Data curation, Formal analysis, Funding acquisition, Investigation, Methodology, Project Administration, Resources, Software, Supervision, Validation, Visualization, Roles/Writing – original draft, Writing – review & editing; VR: Conceptualization, Data curation, Funding acquisition, Investigation, Resources, Supervision, Writing – review & editing. All authors contributed to the article and approved the submitted version.
Funding
Funding support for this investigator-initiated phase I clinical trial is provided by Genelux Corporation. No employee of Genelux Corporation had any influence on the study analysis, interpretation of data, or manuscript writing. JC is supported, in part, by a grant from the National Institutes of Health (T32CA009501-33). PA’s laboratory work is supported by grants from the National Institutes of Health (P30 CA008748, R01 CA236615-01, and R01 CA235667), the U.S. Department of Defense (BC132124, LC160212, CA170630, CA180889 and CA200437), the Batishwa Fellowship, the Comedy vs Cancer Award, the DallePezze Foundation, the Derfner Foundation, the Esophageal Cancer Education Fund, the Geoffrey Beene foundation, the Memorial Sloan Kettering Technology Development Fund, the Miner Fund for Mesothelioma Research, the Mr. William H. Goodwin and Alice Goodwin, the Commonwealth Foundation for Cancer Research, and the Experimental Therapeutics Center of Memorial Sloan Kettering Cancer Center. PA’s laboratory received research support from the ATARA Biotherapeutics. The research support for all authors was not involved in the study design; in the collection, analysis, and interpretation of data; in the writing of the report; and in the decision to submit the article for publication.
Acknowledgments
We acknowledge excellent editorial assistance from Summer Koop of the MSK Thoracic Surgery Service.
Conflict of interest
In the last 3 years, MZ has received consulting fees from Curis, Ikena, Takeda, GlaxoSmithKline, Aldeyra Therapeutics, and Novocure and honoraria for CME content from PER, Medscape, Research to Practice, Medical Learning Institute and OncLive. Memorial Sloan Kettering receives research funding from the Department of Defense, the National Institutes of Health, Precog, GlaxoSmithKline, Epizyme, Polaris, Sellas Life Sciences, Bristol Myers Squibb, Millenium/Takeda, Curis, and Atara for research conducted by MZ. MZ serves as Chair of the Board of Directors of the Mesothelioma Applied Research Foundation, uncompensated. PA declares research funding from ATARA Biotherapeutics; Scientific Advisory Board Member and Consultant for Abound Bio, ATARA Biotherapeutics, Bayer, Bio4T2, Carisma Therapeutics, Imugene, ImmPactBio, Johnson&Johnson, Orion Pharma, Outpace Bio, Pleuri-tech, Putnam associates; Patents, royalties and intellectual property on mesothelin-targeted CAR and other T-cell therapies licensed to ATARA Biotherapeutics, issued patent method for detection of cancer cells using virus, and pending patent applications on PD-1 dominant negative receptor, wireless pulse-oximetry device, and on an ex vivo malignant pleural effusion culture system. Memorial Sloan Kettering Cancer Center MSK has licensed intellectual property related to mesothelin-targeted CARs and T-cell therapies to ATARA Biotherapeutics, and has associated financial interests.
The remaining authors declare that the research was conducted in the absence of any commercial or financial relationships that could be construed as a potential conflict of interest.
Publisher’s note
All claims expressed in this article are solely those of the authors and do not necessarily represent those of their affiliated organizations, or those of the publisher, the editors and the reviewers. Any product that may be evaluated in this article, or claim that may be made by its manufacturer, is not guaranteed or endorsed by the publisher.
Supplementary material
The Supplementary Material for this article can be found online at: https://www.frontiersin.org/articles/10.3389/fimmu.2023.1112960/full#supplementary-material
References
1. American Thoracic Society. Management of malignant pleural effusions. Am J Respir Crit Care Med (2000) 162(5):1987–2001. doi: 10.1164/ajrccm.162.5.ats8-00
2. Dipper A, Jones HE, Bhatnagar R, Preston NJ, Maskell N, Clive A. Interventions for the management of malignant pleural effusions: An updated network meta-analysis. Eur Respir Rev (2021) 30(160):210025. doi: 10.1183/16000617.0025-2021
3. Yang Y, Du J, Wang YS, Kang HY, Zhai K, Shi HZ. Prognostic impact of pleural effusion in patients with malignancy: A systematic review and meta-analysis. Clin Transl Sci (2022) 15(6):1340–54. doi: 10.1111/cts.13260
4. Suzuki K, Servais EL, Rizk NP, Solomon SB, Sima CS, Park BJ, et al. Palliation and pleurodesis in malignant pleural effusion: The role for tunneled pleural catheters. J Thorac Oncol (2011) 6(4):762–7. doi: 10.1097/JTO.0b013e31820d614f
5. Ghanim B, Rosenmayr A, Stockhammer P, Vogl M, Celik A, Bas A, et al. Tumour cell pd-L1 expression is prognostic in patients with malignant pleural effusion: The impact of c-reactive protein and immune-checkpoint inhibition. Sci Rep (2020) 10(1):5784. doi: 10.1038/s41598-020-62813-2
6. Kawachi H, Tamiya M, Taniguchi Y, Yokoyama T, Yokoe S, Oya Y, et al. Efficacy of immune checkpoint inhibitor with or without chemotherapy for nonsquamous nsclc with malignant pleural effusion: A retrospective multicenter cohort study. JTO Clin Res Rep (2022) 3(7):100355. doi: 10.1016/j.jtocrr.2022.100355
7. Aggarwal C, Haas AR, Metzger S, Aguilar LK, Aguilar-Cordova E, Manzanera AG, et al. Phase I study of intrapleural gene-mediated cytotoxic immunotherapy in patients with malignant pleural effusion. Mol Ther (2018) 26(5):1198–205. doi: 10.1016/j.ymthe.2018.02.015
8. Sterman DH, Haas A, Moon E, Recio A, Schwed D, Vachani A, et al. A trial of intrapleural adenoviral-mediated interferon-Alpha2b gene transfer for malignant pleural mesothelioma. Am J Respir Crit Care Med (2011) 184(12):1395–9. doi: 10.1164/rccm.201103-0554CR
9. Adusumilli PS, Zauderer MG, Riviere I, Solomon SB, Rusch VW, O’Cearbhaill RE, et al. A phase I trial of regional mesothelin-targeted car T-cell therapy in patients with malignant pleural disease, in combination with the anti-Pd-1 agent pembrolizumab. Cancer Discovery (2021) 11(11):2748–63. doi: 10.1158/2159-8290.CD-21-0407
10. Ghosn M, Cheema W, Zhu A, Livschitz J, Maybody M, Boas FE, et al. Image-guided interventional radiological delivery of chimeric antigen receptor (Car) T cells for pleural malignancies in a phase I/Ii clinical trial. Lung Cancer (2022) 165:1–9. doi: 10.1016/j.lungcan.2022.01.003
11. Lievense LA, Cornelissen R, Bezemer K, Kaijen-Lambers ME, Hegmans JP, Aerts JG. Pleural effusion of patients with malignant mesothelioma induces macrophage-mediated T cell suppression. J Thorac Oncol (2016) 11(10):1755–64. doi: 10.1016/j.jtho.2016.06.021
12. Lievense LA, Bezemer K, Cornelissen R, Kaijen-Lambers ME, Hegmans JP, Aerts JG. Precision immunotherapy; dynamics in the cellular profile of pleural effusions in malignant mesothelioma patients. Lung Cancer (2017) 107:36–40. doi: 10.1016/j.lungcan.2016.04.015
13. Kosti CN, Vaitsi PC, Pappas AG, Iliopoulou MP, Psarra KK, Magkouta SF, et al. Csf1/Csf1r signaling mediates malignant pleural effusion formation. JCI Insight (2022) 7(6):e155300. doi: 10.1172/jci.insight.155300
14. Baas P, Scherpereel A, Nowak AK, Fujimoto N, Peters S, Tsao AS, et al. First-line nivolumab plus ipilimumab in unresectable malignant pleural mesothelioma (Checkmate 743): A multicentre, randomised, open-label, phase 3 trial. Lancet (2021) 397(10272):375–86. doi: 10.1016/S0140-6736(20)32714-8
15. Offin M, Rusch VW, Rimner A, Adusumilli PS, Zauderer MG. Evolving landscape of initial treatments for patients with malignant pleural mesotheliomas: Clinical trials to clinical practice. Oncologist (2022) 27(8):610–4. doi: 10.1093/oncolo/oyac113
16. Yarchoan M, Albacker LA, Hopkins AC, Montesion M, Murugesan K, Vithayathil TT, et al. Pd-L1 expression and tumor mutational burden are independent biomarkers in most cancers. JCI Insight (2019) 4(6):e126908. doi: 10.1172/jci.insight.126908
17. Mankor JM, Disselhorst MJ, Poncin M, Baas P, Aerts J, Vroman H. Efficacy of nivolumab and ipilimumab in patients with malignant pleural mesothelioma is related to a subtype of effector memory cytotoxic T cells: Translational evidence from two clinical trials. EBioMedicine (2020) 62:103040. doi: 10.1016/j.ebiom.2020.103040
18. Liu X, Zhao J, Li X, Lao F, Fang M. Design strategies and precautions for using vaccinia virus in tumor virotherapy. Vaccines (Basel) (2022) 10(9):1552. doi: 10.3390/vaccines10091552
19. Mastrangelo MJ, Lattime EC. Virotherapy clinical trials for regional disease: In situ immune modulation using recombinant poxvirus vectors. Cancer Gene Ther (2002) 9(12):1013–21. doi: 10.1038/sj.cgt.7700538
20. Kelly KJ, Woo Y, Brader P, Yu Z, Riedl C, Lin SF, et al. Novel oncolytic agent glv-1h68 is effective against malignant pleural mesothelioma. Hum Gene Ther (2008) 19(8):774–82. doi: 10.1089/hum.2008.036
21. Lin SF, Price DL, Chen CH, Brader P, Li S, Gonzalez L, et al. Oncolytic vaccinia virotherapy of anaplastic thyroid cancer in vivo. J Clin Endocrinol Metab (2008) 93(11):4403–7. doi: 10.1210/jc.2008-0316
22. Donat U, Weibel S, Hess M, Stritzker J, Hartl B, Sturm JB, et al. Preferential colonization of metastases by oncolytic vaccinia virus strain glv-1h68 in a human pc-3 prostate cancer model in nude mice. PloS One (2012) 7(9):e45942. doi: 10.1371/journal.pone.0045942
23. Mastrangelo MJ, Maguire HC, Lattime EC. Intralesional Vaccinia/Gm-csf recombinant virus in the treatment of metastatic melanoma. Adv Exp Med Biol (2000) 465:391–400. doi: 10.1007/0-306-46817-4_34
24. Kawa A, Arakawa S. The effect of attenuated vaccinia virus as strain on multiple myeloma; a case report. Jpn J Exp Med (1987) 57(1):79–81.
25. Hansen RM, Libnoch JA. Remission of chronic lymphocytic leukemia after smallpox vaccination. Arch Intern Med (1978) 138(7):1137–8. doi: 10.1001/archinte.1978.03630320073024
26. Yettra M. Remission of chronic lymphocytic leukemia after smallpox vaccination. Arch Intern Med (1979) 139(5):603.
27. Gomella LG, Mastrangelo MJ, McCue PA, Maguire HJ, Mulholland SG, Lattime EC. Phase I study of intravesical vaccinia virus as a vector for gene therapy of bladder cancer. J Urol (2001) 166(4):1291–5. doi: 10.1016/S0022-5347(05)65755-2
28. Mastrangelo MJ, Maguire HC Jr., Eisenlohr LC, Laughlin CE, Monken CE, McCue PA, et al. Intratumoral recombinant gm-Csf-Encoding virus as gene therapy in patients with cutaneous melanoma. Cancer Gene Ther (1999) 6(5):409–22. doi: 10.1038/sj.cgt.7700066
29. Liu TC, Hwang T, Park BH, Bell J, Kirn DH. The targeted oncolytic poxvirus jx-594 demonstrates antitumoral, antivascular, and anti-hbv activities in patients with hepatocellular carcinoma. Mol Ther (2008) 16(9):1637–42. doi: 10.1038/mt.2008.143
30. Park BH, Hwang T, Liu TC, Sze DY, Kim JS, Kwon HC, et al. Use of a targeted oncolytic poxvirus, jx-594, in patients with refractory primary or metastatic liver cancer: A phase I trial. Lancet Oncol (2008) 9(6):533–42. doi: 10.1016/S1470-2045(08)70107-4
31. Edgar R, Domrachev M, Lash AE. Gene expression omnibus: NCBI gene expression and hybridization array data repository. Nucleic Acids Res (2002) 30(1):207–10. doi: 10.1093/nar/30.1.207
32. Adusumilli PS, Stiles BM, Chan M, Mullerad M, Eisenberg DP, Ben-Porat L, et al. Imaging and therapy of malignant pleural mesothelioma using replication-competent herpes simplex viruses. J Gene Med (2006) 8(5):603–15. doi: 10.1002/jgm.877
33. Adusumilli PS, Stiles BM, Chan M, Eisenberg DP, Yu Z, Stanziale SF, et al. Real-time diagnostic imaging of tumors and metastases by use of a replication-competent herpes vector to facilitate minimally invasive oncological surgery. FASEB J (2006) 20(6):726–8. doi: 10.1096/fj.05-5316fje
34. Adusumilli PS, Chan M, Hezel M, Yu Z, Stiles BM, Chou T, et al. Radiation-induced cellular DNA damage repair response enhances viral gene therapy efficacy in the treatment of malignant pleural mesothelioma. Ann Surg Oncol (2007) 14(1):258–69. doi: 10.1245/s10434-006-9127-4
35. Heery CR, Ibrahim NK, Arlen PM, Mohebtash M, Murray JL, Koenig K, et al. Docetaxel alone or in combination with a therapeutic cancer vaccine (Panvac) in patients with metastatic breast cancer: A randomized clinical trial. JAMA Oncol (2015) 1(8):1087–95. doi: 10.1001/jamaoncol.2015.2736
36. Samson A, West EJ, Carmichael J, Scott KJ, Turnbull S, Kuszlewicz B, et al. Neoadjuvant intravenous oncolytic vaccinia virus therapy promotes anticancer immunity in patients. Cancer Immunol Res (2022) 10(6):745–56. doi: 10.1158/2326-6066.CIR-21-0171
37. Toulmonde M, Cousin S, Kind M, Guegan JP, Bessede A, Le Loarer F, et al. Randomized phase 2 trial of intravenous oncolytic virus jx-594 combined with low-dose cyclophosphamide in patients with advanced soft-tissue sarcoma. J Hematol Oncol (2022) 15(1):149. doi: 10.1186/s13045-022-01370-9
38. Sterman DH, Recio A, Haas AR, Vachani A, Katz SI, Gillespie CT, et al. A phase I trial of repeated intrapleural adenoviral-mediated interferon-beta gene transfer for mesothelioma and metastatic pleural effusions. Mol Ther (2010) 18(4):852–60. doi: 10.1038/mt.2009.309
39. Sterman DH, Molnar-Kimber K, Iyengar T, Chang M, Lanuti M, Amin KM, et al. A pilot study of systemic corticosteroid administration in conjunctionwith intrapleural adenoviral vector administration in patients with malignant pleural mesothelioma. Cancer Gene Ther (2000) 7(12):1511–8. doi: 10.1038/sj.cgt.7700269
40. Sterman DH, Recio A, Carroll RG, Gillespie CT, Haas A, Vachani A, et al. A phase I clinical trial of single-dose intrapleural ifn-beta gene transfer for malignant pleural mesothelioma and metastatic pleural effusions: High rate of antitumor immune responses. Clin Cancer Res (2007) 13(15 Pt 1):4456–66. doi: 10.1158/1078-0432.CCR-07-0403
41. Sterman DH, Recio A, Vachani A, Sun J, Cheung L, DeLong P, et al. Long-term follow-up of patients with malignant pleural mesothelioma receiving high-dose adenovirus herpes simplex thymidine Kinase/Ganciclovir suicide gene therapy. Clin Cancer Res (2005) 11(20):7444–53. doi: 10.1158/1078-0432.CCR-05-0405
42. Sun Y, Zhang Z, Zhang C, Zhang N, Wang P, Chu Y, et al. An effective therapeutic regime for treatment of glioma using oncolytic vaccinia virus expressing il-21 in combination with immune checkpoint inhibition. Mol Ther Oncolytics (2022) 26:105–19. doi: 10.1016/j.omto.2022.05.008
Keywords: pleural cancers, oncolytic viral therapy, tumor microenvironment, regional therapy, malignant pleural effusion (MPE)
Citation: Chintala NK, Choe JK, McGee E, Bellis R, Saini JK, Banerjee S, Moreira AL, Zauderer MG, Adusumilli PS and Rusch VW (2023) Correlative analysis from a phase I clinical trial of intrapleural administration of oncolytic vaccinia virus (Olvi-vec) in patients with malignant pleural mesothelioma. Front. Immunol. 14:1112960. doi: 10.3389/fimmu.2023.1112960
Received: 30 November 2022; Accepted: 06 February 2023;
Published: 16 February 2023.
Edited by:
Christophe Blanquart, Centre National de la Recherche Scientifique (CNRS), FranceReviewed by:
Fonteneau Jean-francois, UMR1307 Centre de Recherche en Cancérologie et Immunologie Intégré Nantes Angers (CRCI2NA) (INSERM), FranceLuciano Castiello, National Institute of Health (ISS), Italy
Copyright © 2023 Chintala, Choe, McGee, Bellis, Saini, Banerjee, Moreira, Zauderer, Adusumilli and Rusch. This is an open-access article distributed under the terms of the Creative Commons Attribution License (CC BY). The use, distribution or reproduction in other forums is permitted, provided the original author(s) and the copyright owner(s) are credited and that the original publication in this journal is cited, in accordance with accepted academic practice. No use, distribution or reproduction is permitted which does not comply with these terms.
*Correspondence: Prasad S. Adusumilli, adusumip@mskcc.org
†These authors have contributed equally to this work and share first authorship