- 1Department of Nephrology, Shanghai East Hospital, Tongji University School of Medicine, Shanghai, China
- 2Department of Medicine, Rhode Island Hospital and Alpert Medical School, Brown University, Providence, RI, United States
Background: Peritoneal dialysis (PD) is an effective replacement therapy for end-stage renal disease patients. However, long-term exposure to peritoneal dialysate will lead to the development of peritoneal fibrosis. Epigenetics has been shown to play an important role in peritoneal fibrosis, but the role of histone deacetylases 8 (HDAC8) in peritoneal fibrosis have not been elucidated. In this research, we focused on the role and mechanisms of HDAC8 in peritoneal fibrosis and discussed the mechanisms involved.
Methods: We examined the expression of HDAC8 in the peritoneum and dialysis effluent of continuous PD patients. Then we assessed the role and mechanism of HDAC8 in peritoneal fibrosis progression in mouse model of peritoneal fibrosis induced by high glucose peritoneal dialysis fluid by using PCI-34051. In vitro, TGF-β1 or IL-4 were used to stimulate human peritoneal mesothelial cells (HPMCs) or RAW264.7 cells to establish two cell injury models to further explore the role and mechanism of HDAC8 in epithelial-mesenchymal transition (EMT) and macrophage polarization.
Results: We found that HDAC8 expressed highly in the peritoneum from patients with PD-related peritonitis. We further revealed that the level of HDAC8 in the dialysate increased over time, and HDAC8 was positively correlated with TGF-β1 and vascular endothelial growth factor (VEGF), and negatively correlated with cancer antigen 125. In mouse model of peritoneal fibrosis induced by high glucose dialysate, administration of PCI-34051 (a selective HDAC8 inhibitor) significantly prevented the progression of peritoneal fibrosis. Treatment with PCI-34051 blocked the phosphorylation of epidermal growth factor receptor (EGFR) and the activation of its downstream signaling pathways ERK1/2 and STAT3/HIF-1α. Inhibition of HDAC8 also reduced apoptosis. In vitro, HDAC8 silencing with PCI-34051 or siRNA inhibited TGF-β1-induced EMT and apoptosis in HPMCs. In addition, continuous high glucose dialysate or IL-4 stimulation induced M2 macrophage polarization. Blockade of HDAC8 reduced M2 macrophage polarization by inhibiting the activation of STAT6 and PI3K/Akt signaling pathways.
Conclusions: We demonstrated that HDAC8 promoted the EMT of HPMCs via EGFR/ERK1/2/STAT3/HIF-1α, induced M2 macrophage polarization via STAT6 and PI3K/Akt signaling pathways, and ultimately accelerated the process of peritoneal fibrosis.
Introduction
Peritoneal dialysis (PD) is an effective renal replacement therapy (1), however, the proportion of ESRD patients treated with PD is lower than hemodialysis in developed countries (2). Clinical studies have demonstrated that peritoneal ultrafiltration declines gradually 2 - 4 years after the initiation of PD (3–5). The induction of peritoneal fibrosis is a complex pathological event, and is characterized by epithelial-to-mesenchymal transition (EMT), activation of fibroblasts, deposition of extracellular matrix (ECM) components and angiogenesis (6). The release of several growth factors/cytokines, especially transforming growth factor-1 (TGF-β1) and vascular endothelial growth factor (VEGF), and activation of various signaling pathways contribute to the progression of peritoneal fibrosis. Our previous studies have demonstrated that epidermal growth factor receptor (EGFR) promotes the development and progression of peritoneal fibrosis via the activation of multiple pro-fibrosis signaling pathways, inflammatory responses and angiogenesis (7). The phosphorylation of EGFR subsequently leads to the activation of several intracellular signaling pathways, including extracellular signal-regulated kinases 1/2 (ERK 1/2) and signal transducer and activator of transcription 3 (STAT3) during peritoneal fibrosis (8). Thus, targeting EGFR may be an effective approach to preserve peritoneal membrane ultrafiltration capacity.
Macrophages are key components of the peritoneal immune system (9). Macrophages are usually divided into two functional subtypes (M1 and M2), which play different roles in various physiological and pathological environments (10). Analysis of experimental evidences from PD patient samples demonstrates that the majority of peritoneal macrophages tend to be M2 macrophages in phenotype and function (11). Predominance of M2 macrophage response leads to induction of EMT, and upregulate the production of ECM protein, angiogenesis, and fibrosis (12). Our previous studies have demonstrated that M2 macrophage polarization in the peritoneum is regulated by the phosphorylation of signal transducer and activator of transcription 6 (STAT6) and phosphatidylinositol-3-kinase (PI3K)/Akt pathways (13).
According to previous studies by our group, epigenetic regulation plays an important role in the process of peritoneal fibrosis (6, 7, 13, 14). Histone deacetylase 8 (HDAC8) is a class I HDAC that catalyzes the deacetylation of histones and non-histones (15). It has been demonstrated that HDAC8 plays a multifunctional role in cancer progression, such as stimulating tumor growth and metastasis by enhancing cell proliferation and activating EMT via acting on histones and non-histone substrates (15). However, the specific mechanism and target proteins of HDAC8 in regulating peritoneal fibrosis have not been revealed.
In this research, we examined the expression of HDAC8 in the peritoneum and dialysis effluent of continuous PD patients. Then we assessed the role and mechanism of HDAC8 in peritoneal fibrosis progression in mouse model of peritoneal fibrosis induced by high glucose peritoneal dialysis fluid (PDF) by using PCI-34051. In vitro, TGF-β1 and IL-4 were used to stimulate human peritoneal mesothelial cells (HPMCs) and RAW264.7 cells to establish two cell injury models to further explore the role and mechanism of HDAC8 in EMT and macrophage polarization.
Materials and methods
Additional details for all methods are provided in the Supplementary Material and Methods.
Clinical sample collection and ethics statement
We collected peritoneal tissue during catheterization initiation operations (n = 6) and refractory peritonitis-induced catheter migration (n = 6) at Shanghai East Hospital affiliated with Tongji University and completed co-immunofluorescent staining of HDAC8 and α-SMA. Human PD effluents from patients with different durations were collected at Shanghai East Hospital, Shanghai Baoshan Hospital and Shanghai Songjiang District Central Hospital from September 2017 to March 2022. These patients were divided into 5 groups according to duration: group 1 (duration ≤ 1 month, n=16), group 2 (1<duration ≤ 12 months, n=22), group 3 (12<duration ≤ 24 months, n=20), group 4 (24<duration ≤ 36 months, n=16), and group 5 (duration>36 months, n=14). This study was approved by the Medical Ethics Committee of Shanghai East Hospital and was conducted in accordance with the Declaration of Helsinki. Written informed consent was obtained from each patient. And we have obtained the registration number from the Chinese Clinical Trial Register (ChiCTR): ChiCTR2100052103.
Animals and treatment
Male C57BL mice (purchased from Shanghai Super-B&K Laboratory Animal Corp. Ltd) weighed 20-25g were used in this study. The peritoneal fibrosis model was created by daily i.p. injection of 100 ml/kg peritoneal dialysis fluid with 4.25% glucose for 28 days (6). To examine the efficacy of PCI-34051 in peritoneal fibrosis, two different concentrations of PCI-34051 (10 or 20 mg/kg) in DMSO was intraperitoneally every day and the mice were sacrificed on day 28 to collect peritoneum. The animal protocol was reviewed and approved by the Institutional Animal Care and Use Committee at Tongji University (Shanghai, China). The details of animals and treatment are provided in the Supplementary Material and Methods.
Cell culture and treatments
Cells were collected and cultured as described previously (13, 16). HPMCs (American Type Culture Collection, ATCC; Rockville, MD, United States) were cultured in Dulbecco’s modified Eagle’s medium (DMEM) with F12 containing 10% fetal bovine serum (FBS), 1% penicillin, and streptomycin. Raw264.7 cells (American Type Culture Collection, ATCC; Rockville, MD, United States) were cultured in RPMI-1640 medium containing 10% FBS, 1% penicillin and streptomycin. All cells were cultured in an atmosphere of 5% CO2, and 95% air at 37°C and were experimented after three generations. To examine the role and mechanisms of HDAC8 in TGF-β1-induced EMT of HPMCs, we starved HPMCs for 24 hours with DMEM/F12 containing 0.5% FBS and then exposed to TGF-β1 (2ng/ml) in the presence or absence of PCI-34051 (5mM). for 36 hours. To examine the role and mechanisms of HDAC8 in IL-4-induced macrophage polarization of Raw264.7 cells, we starved Raw264.7 cells for 24 hours with RPMI-1640 containing 0.5% FBS and then exposed to IL-4 (10 ng/ml) for 24 hours in the presence or absence of PCI-34051 (5μM). Then, cells were harvested for further immunoblot analysis or immunofluorescent staining. All the in vitro experiments were repeated at least three times.
Results
HDAC8 is highly expressed in peritoneum from long-term PD patients, positively correlated with TGF-β1 and VEGF and negatively correlated with CA125 in human PD effluent
We collected peritoneal tissue during catheterization initiation operations (n = 6) and refractory peritonitis-induced catheter migration (n = 6) and completed co-immunofluorescent staining of HDAC8 and α-SMA. As shown in Figure 1A, HDAC8 was highly expressed in the thickened peritoneum of long-term PD patients with PD-related peritonitis, and HDAC8 was co-expressed with α-SMA positive cells.
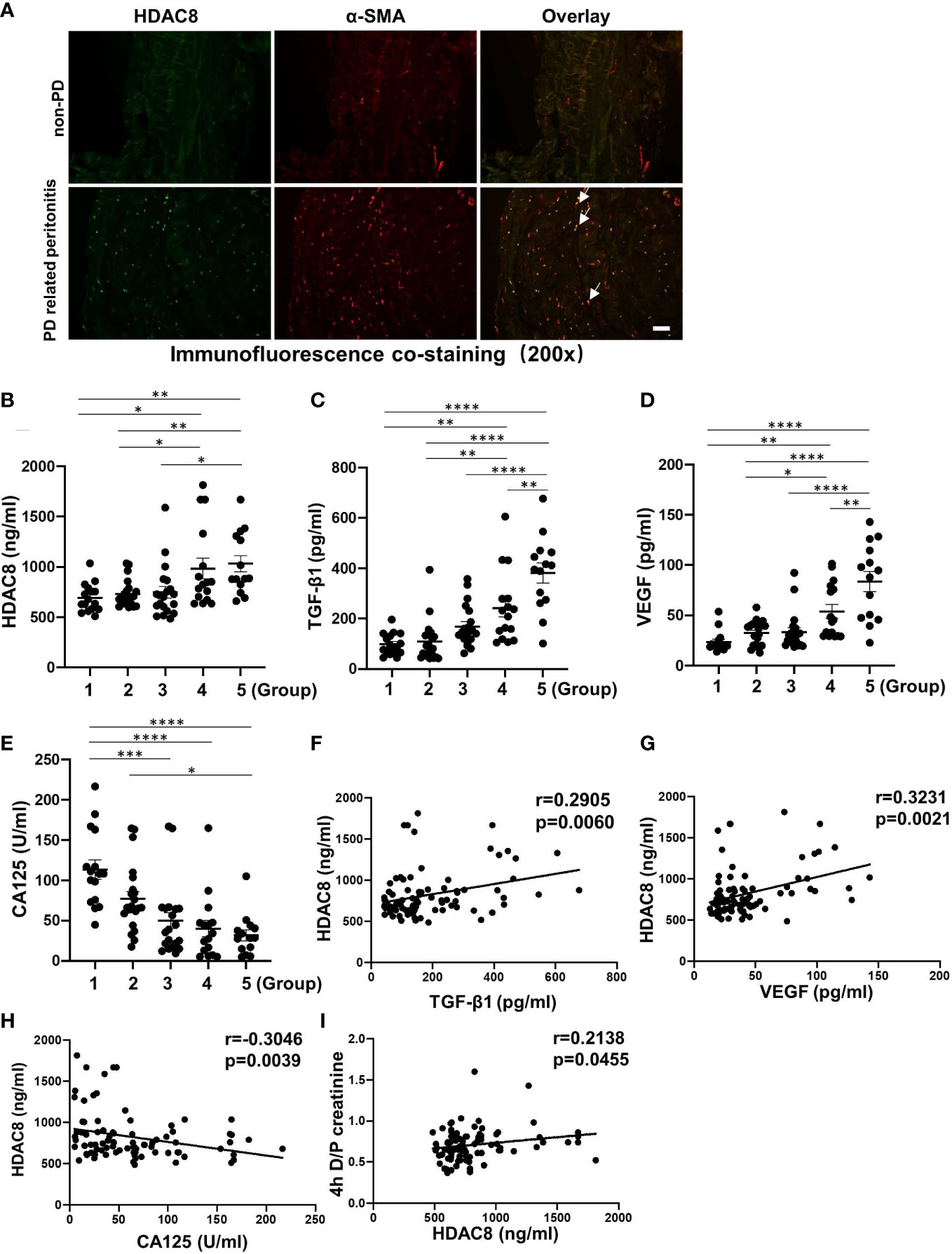
Figure 1 HDAC8 is highly expressed in peritoneum from long-term PD patients, positively correlated with TGF-β1 and VEGF and negatively correlated with CA125 in human PD effluent (A) Immunofluorescent co-staining of α-SMA and HDAC8 in the peritoneum from patients with non-PD and PD-related peritonitis. And HDAC8 was co-expressed with α-SMA-positive cells (white arrows). (B–E) Levels of HDAC8 and cytokines in dialysis effluent according to ELISA kits. The dialysis effluents from 88 PD patients were divided into 5 groups according to duration: group 1 (duration ≤ 1 month, n=16), group 2 (1<duration ≤ 12 months, n=22), group 3 (12<duration ≤ 24 months, n=20), group 4 (24<duration ≤ 36 months, n=16), and group 5 (duration>36 months, n=14). The expression levels of HDAC8 (B), TGF-β1 (C), VEGF (D), and CA125 (E) were indicated in each group. (F–I) HDAC8 was positively correlated with enhanced expression of TGF-β1, VEGF and 4h D/P creatinine, and negatively with CA125 in dialysis effluent of PD patients. Correlation analysis were conducted between HDAC8 and TGF-β1 (F), HDAC8 and VEGF (G), HDAC8 and CA125 (H), HDAC8 and 4h D/P creatinine (I). Data were expressed as means ± SEM. *P<0.05; **P<0.01; ***P<0.001; ****P<0.0001. N.S., statistically not significant, with the comparisons labeled. All scale bars = 50 μm.
ELISA kit assays showed that the expressions of HDAC8, TGF-β1 and VEGF in dialysis effluent were significantly increased, while the level of CA125 decreased with the prolongation of dialysis time (Figures 1B–E). CA125 is reported to be a marker of peritoneal mesothelial cell, with the severity of peritoneal fibrosis, the expression of CA125 is decreased due to the loss of peritoneal mesothelial cell (17). Further correlation analysis showed that HDAC8 was positively correlated with TGF-β1 (r = 0.2905, p = 0.0060) and VEGF (r= 0.3231, p = 0.0021), and negatively correlated with CA125 (r= −0.3046, p = 0.0039) (Figures 1F–H). In addition, it has been reported that patients characterized as high transporters had an increased sub-mesothelial fibrous layer (18). According to the results of PET tests in PD patients (Figure 1I), HDAC8 was positively correlated with the peritoneal transport rate of patients (r= 0.2138, p = 0.0455). Table S1 showed the clinical characteristics of the enrolled PD patients. There was no significant difference between different groups of PD patients except creatinine and serum sodium. These data suggest that HDAC8 might be a clinically noninvasive biomarker in dialysis efflux for predicting peritoneal injury and transport status in PD patients.
Inhibition of HDAC8 suppresses development of peritoneal fibrosis and improves functional impairments of peritoneal membrane in the high glucose PDF-injured peritoneum
In the preliminary experiment, we used two different concentrations of PCI-34051 (10mg/kg or 20mg/kg) to intervene high glucose PDF induced peritoneal fibrosis in mice. Our results showed that two different concentrations of PCI-34051 could prevent the development of peritoneal fibrosis and improve functional impairments of peritoneal membrane in the high glucose PDF-injured peritoneum, and high concentration of PCI (20mg/kg) had a better effect on inhibiting peritoneal fibrosis and conserving peritoneum function (Figure S1). Therefore, we chose a concentration of 20mg/kg PCI-34051 to treat mice for further study. As shown by Masson trichrome staining in Figure 2A, we successfully established a mouse model of peritoneal fibrosis with continuous intraperitoneal injection of 4.25% glucose PDF, which was characterized by an increase in the thickness of the sub-mesothelial area. Treatment of PCI-34051 (20mg/kg/d) after injection of 4.25% glucose PDF significantly reduced these pathological changes (Figures 2A–C), suggesting that HDAC8 is a key mediator of peritoneal fibrosis. EMT is an important mechanism of peritoneal fibrosis (19, 20). We examined the expression of α-SMA, collagen I and E-cadherin by western blot. As shown in Figures 2D–G, expressions of α-SMA and collagen I were significantly increased, and expression of E-cadherin was decreased after exposure to 4.25% glucose PDF. Treatment of PCI-34051 blocked the EMT process.
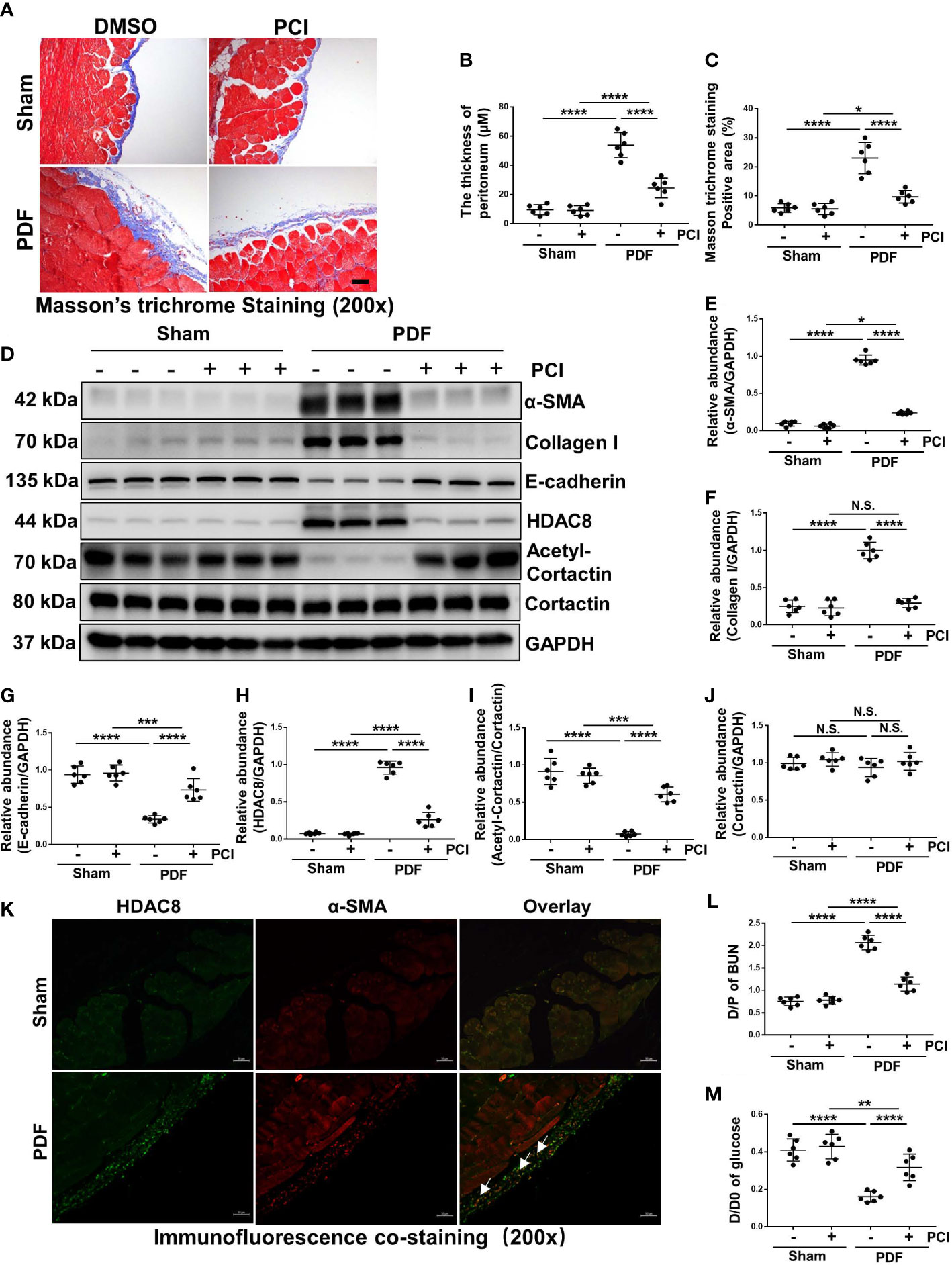
Figure 2 Inhibition of HDAC8 suppresses development of peritoneal fibrosis and improves functional impairments of peritoneal membrane in the high glucose PDF-injured peritoneum (A) Masson trichrome staining of the peritoneum in different groups of mice. (B) The thickness of peritoneum according to Masson trichrome staining. (C) The positive area of Masson trichrome staining-positive sub-mesothelial area (blue). (D) Western blot analysis showed the protein levels of α-SMA, collagen I, E-cadherin, HDAC8, cortactin, acetyl-cortactin and GAPDH in peritoneum from different groups of mice. Expression levels of (E) α-SMA, (F) collagen I, (G) E-cadherin, (H) HDAC8, (I) acetyl-cortactin, (J) cortactin in different groups were quantified by densitometry and normalized with GAPDH and cortactin respectively. (K) Immunofluorescent co-staining of α-SMA and HDAC8 (white arrows) in the peritoneum from mice with or without high glucose PDF injection. (L) The dialysate-to-plasma (D/P) ratio of blood urea nitrogen (BUN). (M) Ratio of dialysate glucose at 2 h after PDF injection to dialysate glucose at 0 hour (D/D0). Data were expressed as means ± SEM. *P<0.05; **P<0.01; ***P<0.001; ****P<0.0001. N.S., statistically not significant, with the comparisons labeled. All scale bars = 50 μm.
To demonstrate the effectiveness of PCI-34051 in vivo, we examined the impact of PCI-34051 on the expression of HDAC8 and its substrate protein cortactin. The expression of HDAC8 increased significantly after the injection of 4.25% glucose PDF for 28 days, while the expression of acetyl-cortactin decreased (Figures 2D, H–J). Treatment of PCI-34051 decreased the expression of HDAC8 to the base level in mice receiving 4.25% glucose PDF, and increased acetyl-cortactin. Immunofluorescent staining showed that HDAC8 was mainly expressed in cells present in the sub-mesothelial zone and co-localized with α-SMA (Figure 2K). These results suggested that PCI-34051 may prevent the progression of peritoneal fibrosis by suppressing EMT. In addition, PCI-34051 improved high glucose PDF-associated peritoneal functional impairments according to the PET test results (Figures 2L, M).
Inhibition of HDAC8 blocks the activation of EGFR/ERK1/2/STAT3/HIF-1α signaling pathway in the peritoneum exposed to high glucose dialysate
Studies from our group have demonstrated that the activation of EGFR/ERK/1/2/STAT3 signaling pathway is related to the progression of peritoneal fibrosis (21, 22). Therefore, we investigated the effect of HDAC8 on the activation of EGFR and its downstream signaling molecules ERK1/2 and STAT3. Immunofluorescent staining showed that HDAC8 was observed in EGFR positive cells (Figure 3A). As shown in Figures 3B–H, the phosphorylation of EGFR, ERK1/2 and STAT3 were significantly increased after exposure to 4.25% glucose PDF, while PCI-34051 administration inhibited their phosphorylation. Recent studies have demonstrated that the expression of HIF-1α in mesenchymal cells is mainly dependent on the activation of STAT3, and the inhibition of STAT3 will further suppressed the activation of HIF-1α, thus affecting the EMT of mesothelial cells (23). As shown in Figures 3B, I, the expression of HIF-1α was significantly increased after exposure to 4.25% glucose PDF, while PCI-34051 administration inhibited its expression. These data suggested that inhibition of HDAC8 suppressed the activation of the EGFR/ERK1/2/STAT3/HIF-1α signaling pathway.
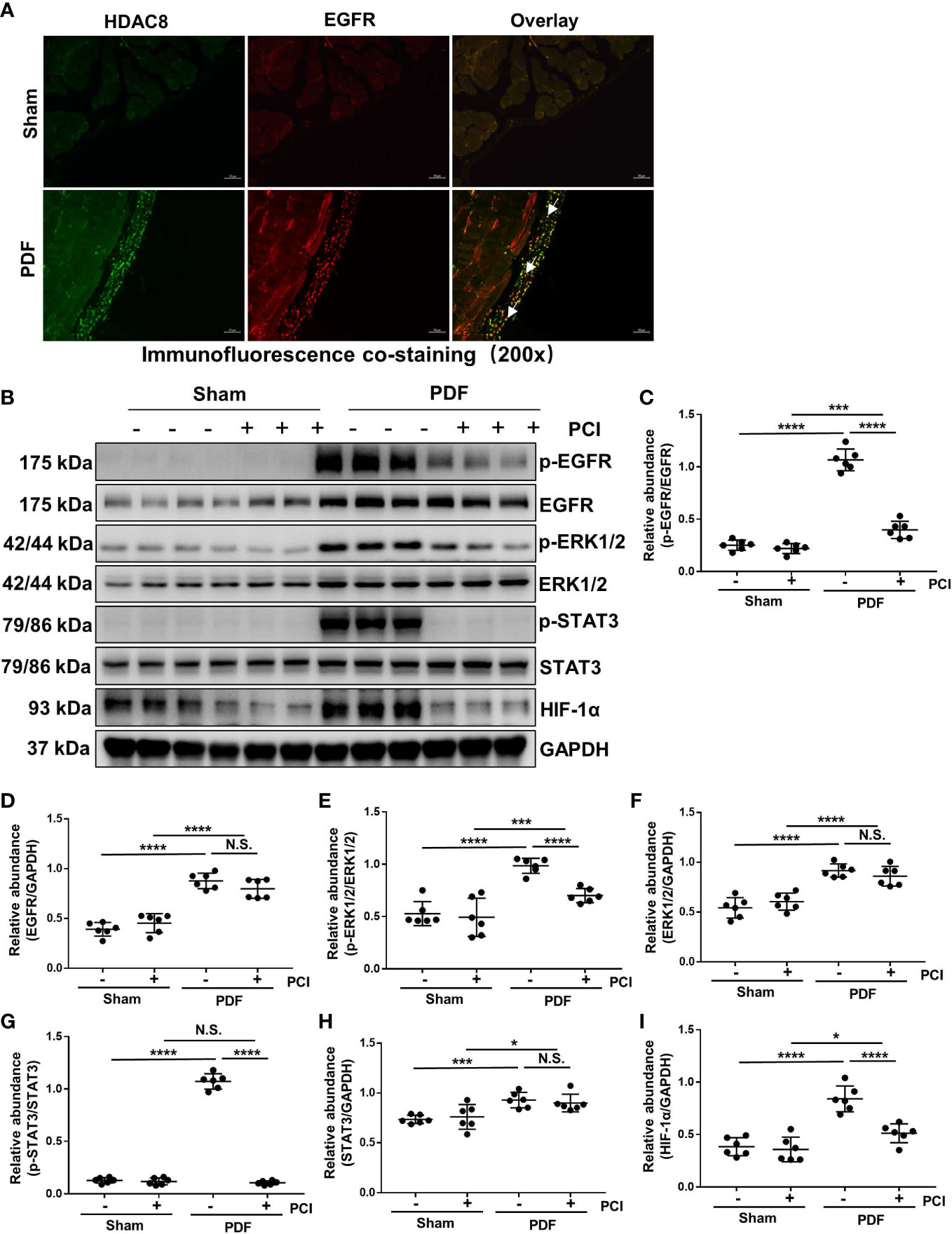
Figure 3 Inhibition of HDAC8 blocks the activation of EGFR/ERK1/2/STAT3/HIF-1α signaling pathway in the peritoneum exposed to high glucose dialysate (A) Immunofluorescent co-staining of EGFR and HDAC8 (white arrows) in the peritoneum from mice with or without high glucose PDF injection. (B) Western blot analysis showed the protein levels of p-EGFR, EGFR, p-ERK1/2, ERK1/2, p-STAT3, STAT3, HIF-1α and GAPDH. Expression levels of (C) p-EGFR, (D) EGFR, (E) p-ERK1/2, (F) ERK1/2, (G) p-STAT3, (H) STAT3, (I) HIF-1α were quantified by densitometry and normalized with GAPDH, EGFR, ERK1/2 and STAT3 respectively. Data were expressed as means ± SEM. *P<0.05; **P<0.01; ***P<0.001; ****P<0.0001. N.S., statistically not significant, with the comparisons labeled. All scale bars = 50 μm.
Blockade of HDAC8 with PCI-34051 or siRNA abrogates EMT by inhibition of EGFR/ERK1/2/STAT3/HIF-1α signaling pathway
TGF-β1 is an important cytokine that can stimulate EMT and induce peritoneal fibrosis (7). HPMCs exposure to TGF-β1 increased the expression of α-SMA and collagen I, and decreased the expression of epithelial cell marker E-cadherin, indicating that TGF-β1 promoted the EMT of HPMCs (Figures 4A–D, 5A–D). Treatment of PCI-34051 or siRNA, decreased the expression of HDAC8 and increased the expression of acetyl-cortactin (Figures 4A, E–G, 5A, E–G). In addition, TGF-β1 induced the phosphorylation of EGFR and activation of its downstream signaling pathways ERK1/2 and STAT3/HIF-1α (Figures 4H–O, 5H–O). Blockade of HDAC8 suppressed all of these responses. These data supported our in vivo observation that HDAC8 is a key protein in regulating peritoneal fibrosis via EGFR/ERK1/2/STAT3/HIF-1α signaling pathway.
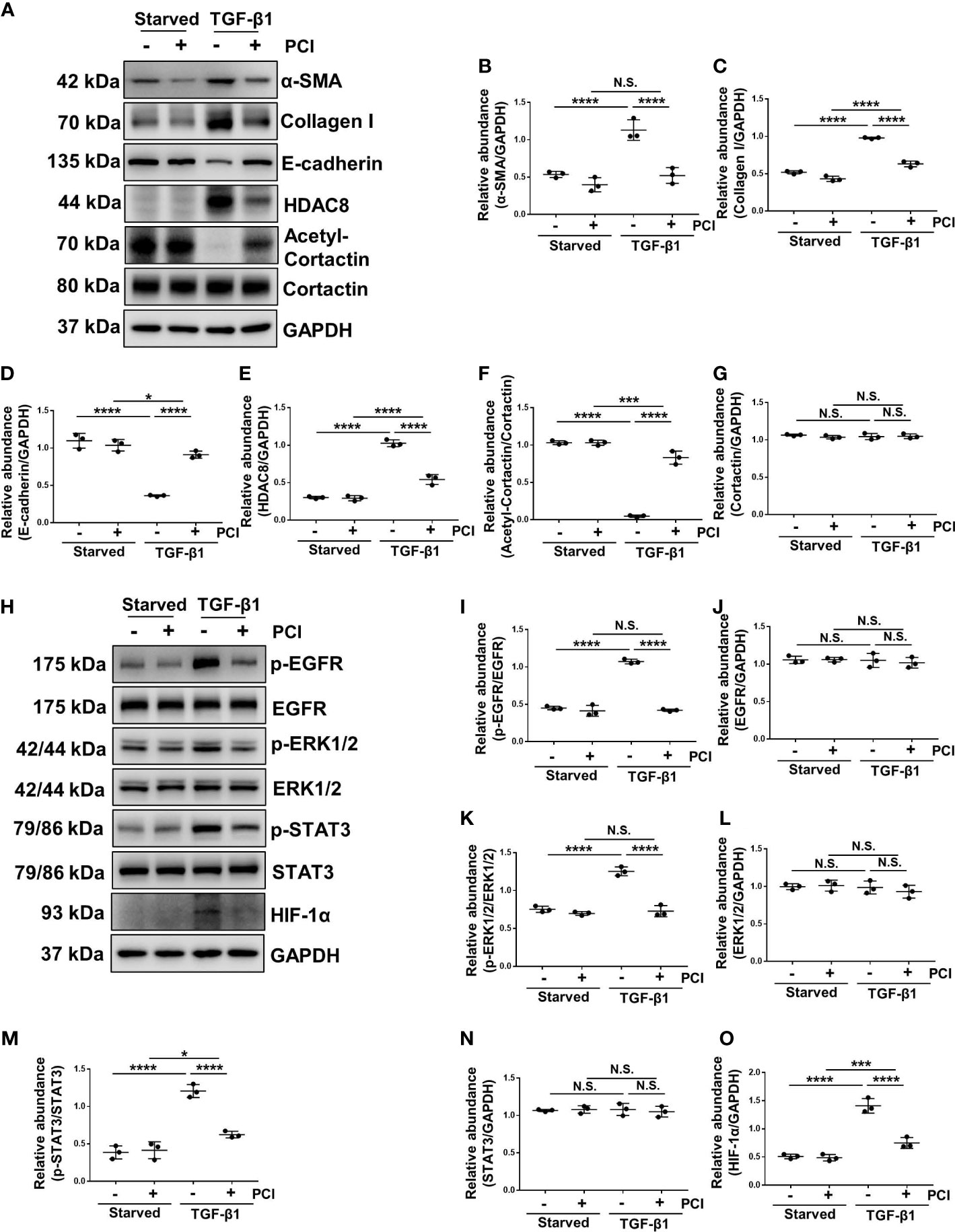
Figure 4 Blockade of HDAC8 with PCI-34051 abrogates EMT by inhibition of EGFR/ERK1/2/STAT3/HIF-1α signaling pathway (A) Serum-starved HPMCs were pretreated with PCI-34051 (5μM) and then exposed to TGF-β1 (2 ng/ml) for 36 h. Cell lysates were subjected to immunoblot analysis with specific antibodies against α-SMA, collagen I, E-cadherin, HDAC8, cortactin, acetyl-cortactin and GAPDH. Expression levels of (B) α-SMA, (C) collagen I, (D) E-cadherin, (E) HDAC8, (F) acetyl-cortactin, (G) cortactin in different groups were quantified by densitometry and normalized with GAPDH and cortactin respectively. (H) Cell lysates were subjected to immunoblot analysis with specific antibodies against p-EGFR, EGFR, p-ERK1/2, ERK1/2, p-STAT3, STAT3, HIF-1α and GAPDH. Expression levels of (I) p-EGFR, (J) EGFR, (K) p-ERK1/2, (L) ERK1/2, (M) p-STAT3, (N) STAT3, (O) HIF-1α were quantified by densitometry and normalized with GAPDH, EGFR, ERK1/2 and STAT3 respectively. Data were expressed as means ± SEM. *P<0.05; **P<0.01; ***P<0.001; ****P<0.0001. N.S., statistically not significant, with the comparisons labeled.
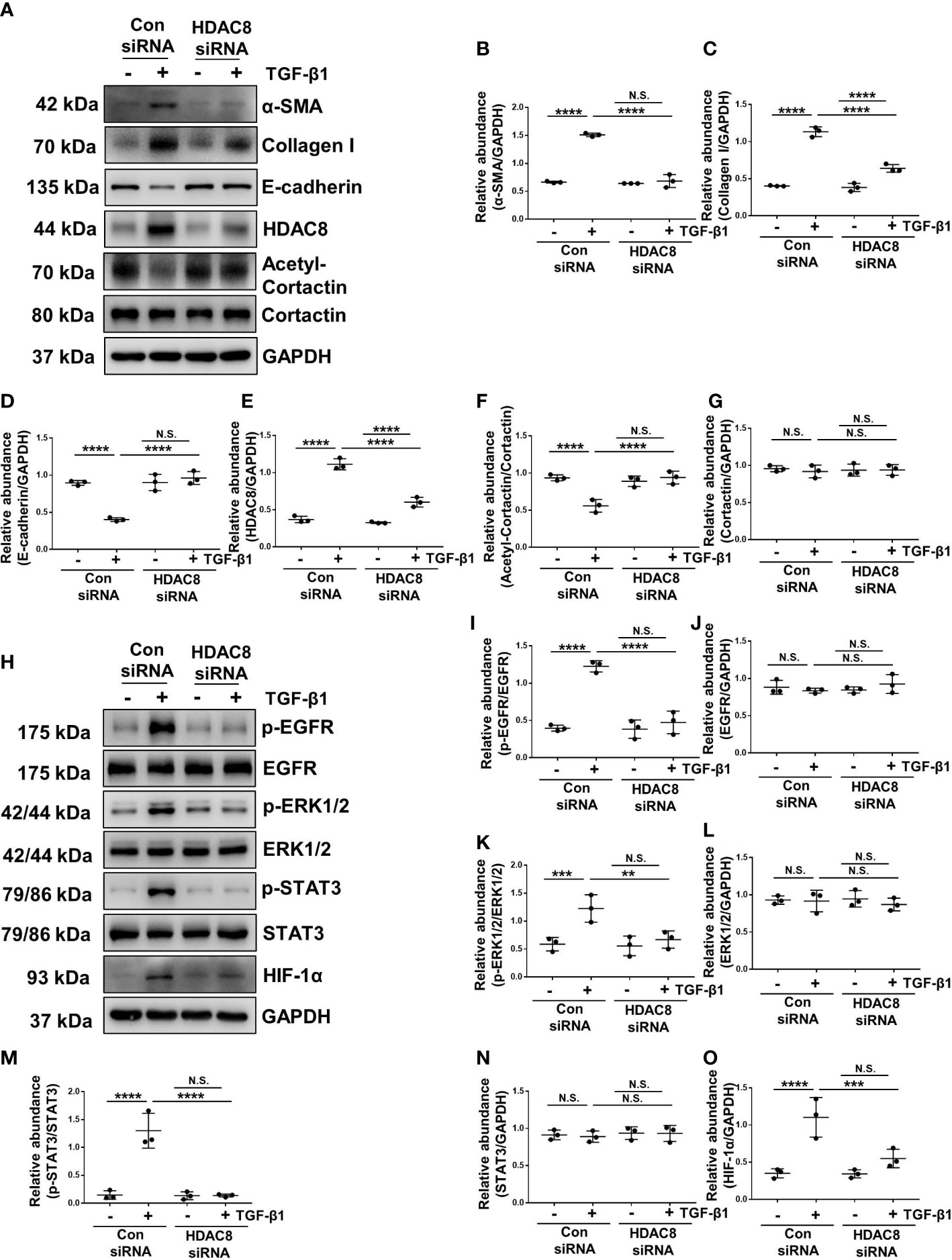
Figure 5 Blockade of HDAC8 with siRNA abrogates EMT by inhibition of EGFR/ERK1/2/STAT3/HIF-1α signaling pathway (A) Serum-starved HPMCs were pretreated with siRNA and then exposed to TGF-β1 (2 ng/ml) for 36 h. Cell lysates were subjected to immunoblot analysis with specific antibodies against α-SMA, collagen I, E-cadherin, HDAC8, cortactin, acetyl-cortactin and GAPDH. Expression levels of (B) α-SMA, (C) collagen I, (D) E-cadherin, (E) HDAC8, (F) acetyl-cortactin, (G) cortactin in different groups were quantified by densitometry and normalized with GAPDH and cortactin respectively. (H) Cell lysates were subjected to immunoblot analysis with specific antibodies against p-EGFR, EGFR, p-ERK1/2, ERK1/2, p-STAT3, STAT3, HIF-1α and GAPDH. Expression levels of (I) p-EGFR, (J) EGFR, (K) p-ERK1/2, (L) ERK1/2, (M) p-STAT3, (N) STAT3, (O) HIF-1α were quantified by densitometry and normalized with GAPDH, EGFR, ERK1/2 and STAT3 respectively. Data were expressed as means ± SEM. *P<0.05; **P<0.01; ***P<0.001; ****P<0.0001. N.S., statistically not significant, with the comparisons labeled.
Inhibition of HDAC8 prevents M2 macrophage polarization via suppressing STAT6 and PI3K/Akt signaling pathways in the high glucose PDF-injured peritoneum
Macrophages play a pivotal role in peritoneal fibrosis process (13). Therefore, we detected the infiltration and polarization of macrophages in a mouse peritoneal fibrosis model established by 4.25% glucose PDF. Western blot showed increased expressions of arginase-1 (Arg-1) and CD163 expression [cell markers of M2 phenotype (13)] after exposure to 4.25% glucose PDF, while PCI-34051 administration effectively decreased their expressions (Figures 6A, C, D). These results suggested that M2 macrophage polarization was involved in the process of peritoneal fibrosis, and the inhibition of HDAC8 by PCI-34051 could prevent M2 macrophage polarization.
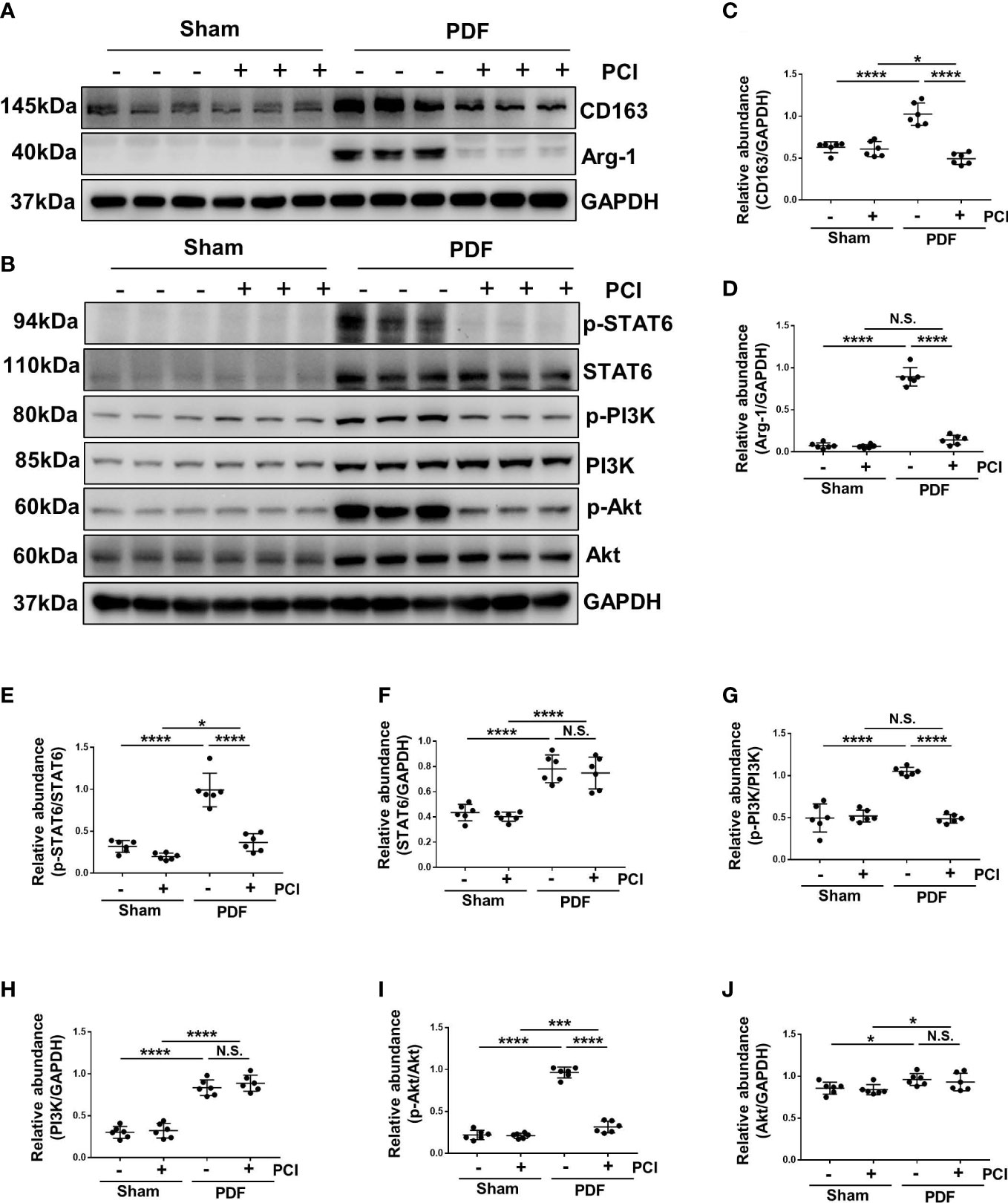
Figure 6 Inhibition of HDAC8 prevents M2 macrophage polarization via suppressing STAT6 and PI3K/Akt signaling pathways in the high glucose PDF-injured peritoneum (A) Western blot analysis showed the protein levels of CD163, Arg-1 and GAPDH in peritoneum of mice. (B) Western blot analysis showed the protein levels of p-STAT6, STAT6, p-PI3K, PI3K p-Akt, Akt and GAPDH in peritoneum of mice. Expression levels of (C) CD163, (D) Arg-1, (E) p-STAT6, (F) STAT6, (G) p-PI3K, (H) PI3K, (I) p-Akt, (J) Akt were quantified by densitometry and normalized with GAPDH, STAT6, PI3K and Akt respectively. Data were expressed as means ± SEM. *P<0.05; **P<0.01; ***P<0.001; ****P<0.0001. N.S., statistically not significant, with the comparisons labeled.
The phosphorylation of STAT6 and activation of PI3K/AKT signaling pathway are involved in the regulation of M2 macrophage polarization (13). In our research, the phosphorylation of STAT6, PI3K and Akt were significantly increased after exposure to 4.25% glucose PDF, while PCI-34051 administration inhibited their phosphorylation (Figures 6B, E, G, I). In addition, injection of 4.25% glucose PDF increased the expression of total STAT6, PI3K and Akt, and PCI-34051 administration did not decrease their expressions (Figures 6B, E–J). These data suggested that inhibition of HDAC8 might inhibit the M2 macrophage polarization via STAT6 and PI3K/Akt pathways.
Blockade of HDAC8 with PCI-34051 or siRNA prevents M2 macrophage polarization via suppressing STAT6 and PI3K/Akt signaling pathways
IL-4 stimulation can induce M2 macrophage polarization (13). As shown in Figures 7A and 8A, immunofluorescent staining of CD163 indicated that IL-4 induced M2 polarization in RAW264.7 cells, while blockade of HDAC8 prevented M2 macrophage polarization. Western blot further verified this result (Figures 7B, C, E–I, 8B, C, E–I). In addition, IL-4 induced the phosphorylation of STAT6 and activation of PI3K/Akt pathway (Figures 7D, J–L, 8D, J–L, S2, S3). Blockade of HDAC8 with PCI-34051 or siRNA suppressed all of these responses. Immunofluorescent staining of p-STAT6 further verified that blockade of HDAC8 could suppress the phosphorylation of STAT6 (Figures 7M, 8M). These data supported our in vivo observation that HDAC8 could regulate M2 macrophage polarization via STAT6 and PI3K/Akt signaling pathways.
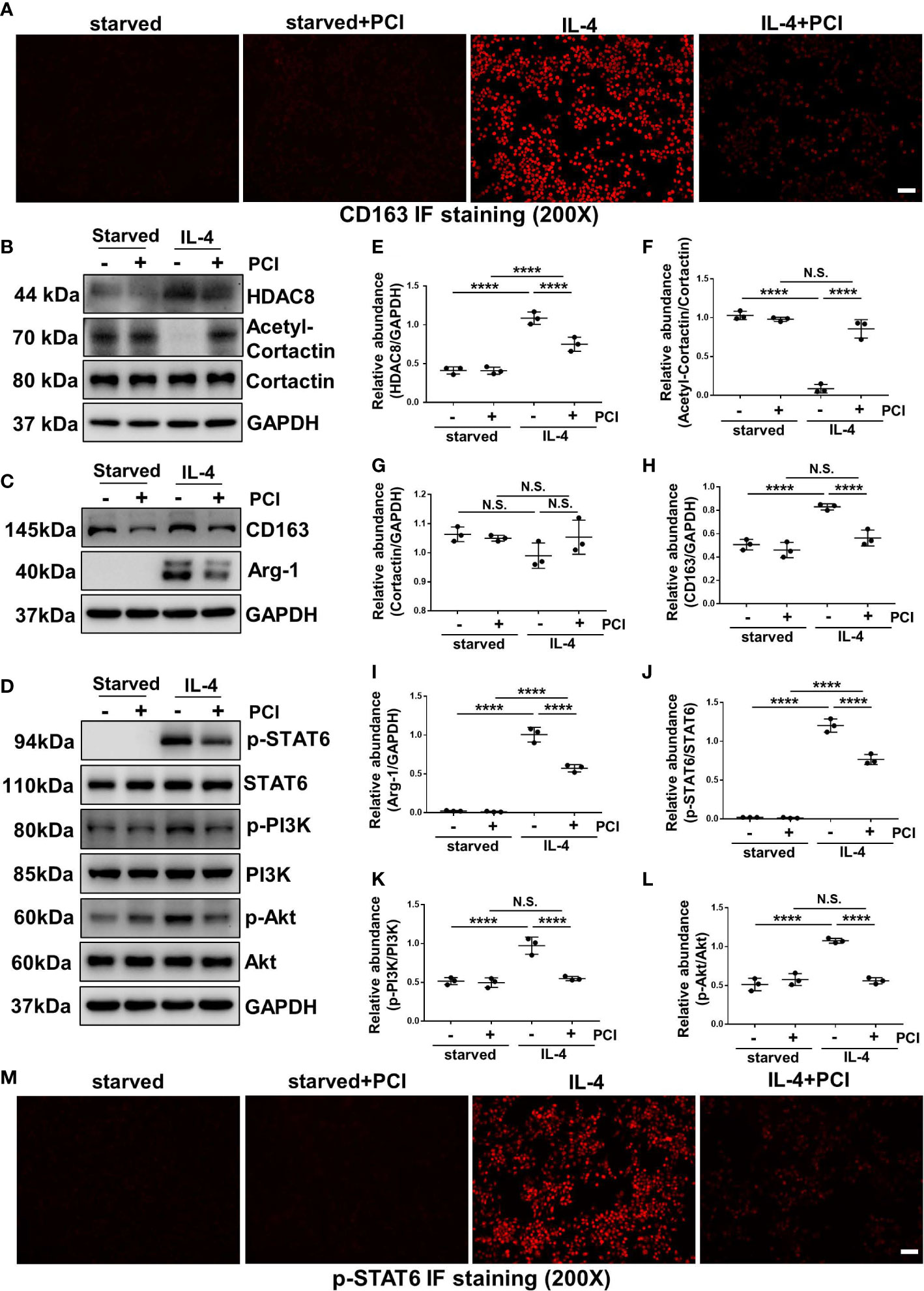
Figure 7 Blockade of HDAC8 with PCI-34051 prevents M2 macrophage polarization via suppressing STAT6 and PI3K/Akt signaling pathways (A) Immunofluorescent staining of CD163 in Raw264.7 cells with different treatments (B–D) Serum-starved RAW264.7 were pretreated with PCI-34051 (5μM) and then exposed to IL-4 (10 ng/ml) for 24 h. Cell lysates were subjected to immunoblot analysis with specific antibodies against HDAC8, acetyl-cortactin, cortactin, CD163, Arg-1, p-STAT6, STAT6, p-PI3K, PI3K p-Akt, Akt and GAPDH. Expression levels of (E) HDAC8, (F) acetyl-cortactin, (G) cortactin, (H) CD163, (I) Arg-1, (J) p-STAT6, (K) p-PI3K, (L) p-Akt were quantified by densitometry and normalized with GAPDH, cortactin, STAT6, PI3K and Akt respectively. (M) Immunofluorescent staining of p-STAT6 in Raw264.7 cells with different treatments. Data were expressed as means ± SEM. *P<0.05; **P<0.01; ***P<0.001; ****P<0.0001. N.S., statistically not significant, with the comparisons labeled. All scale bars = 50 μm.
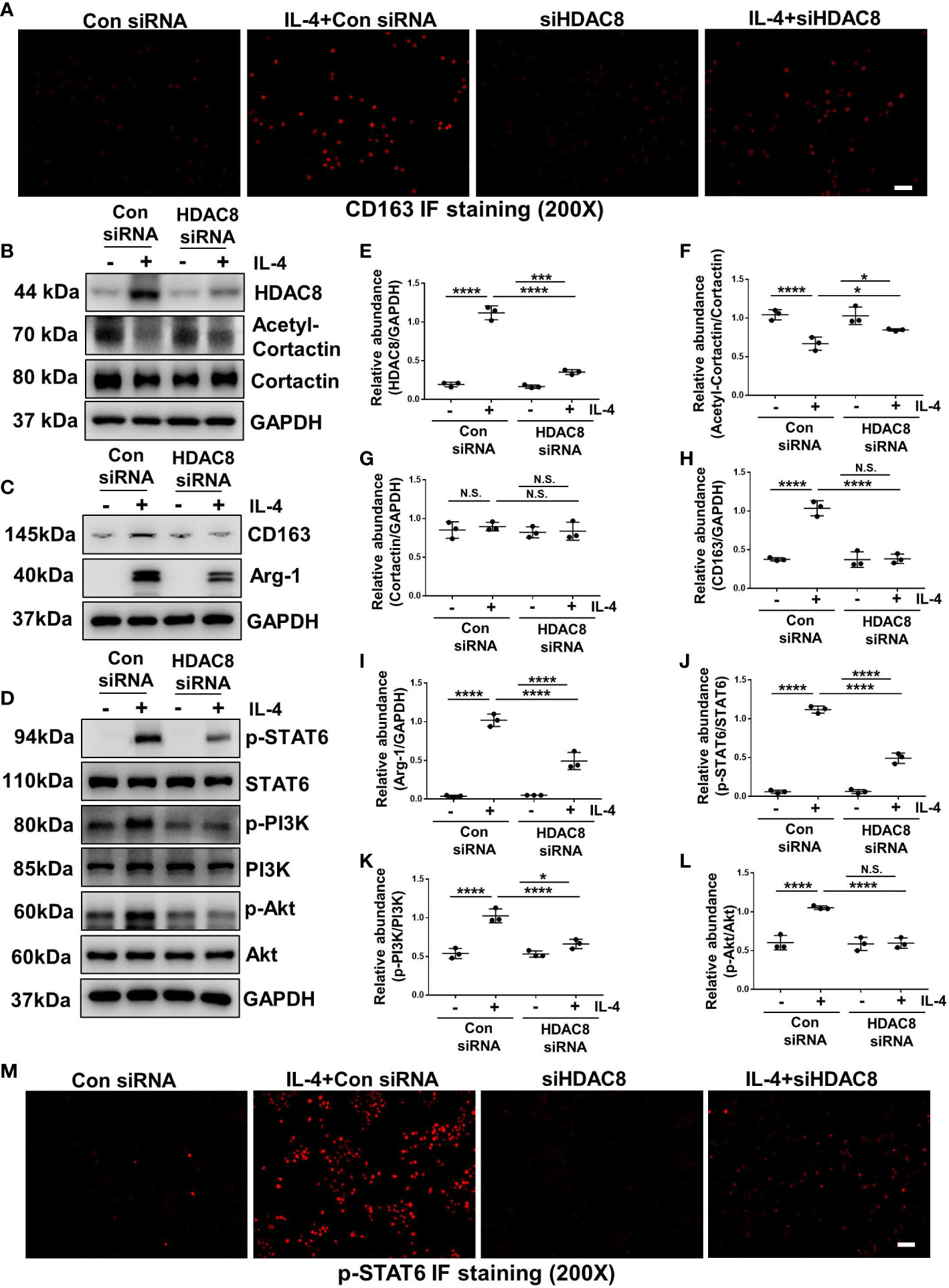
Figure 8 Blockade of HDAC8 with siRNA prevents M2 macrophage polarization via suppressing STAT6 and PI3K/Akt signaling pathways (A) Immunofluorescent staining of CD163 in Raw264.7 cells with different treatments (B–D) Serum-starved RAW264.7 were pretreated with siRNA and then exposed to IL-4 (10 ng/ml) for 24 h. Cell lysates were subjected to immunoblot analysis with specific antibodies against HDAC8, acetyl-cortactin, cortactin, CD163, Arg-1, p-STAT6, STAT6, p-PI3K, PI3K p-Akt, Akt and GAPDH. Expression levels of (E) HDAC8, (F) acetyl-cortactin, (G) cortactin, (H) CD163, (I) Arg-1, (J) p-STAT6, (K) p-PI3K, (L) p-Akt were quantified by densitometry and normalized with GAPDH, cortactin, STAT6, PI3K and Akt respectively. (M) Immunofluorescent staining of p-STAT6 in Raw264.7 cells with different treatments. Data were expressed as means ± SEM. *P<0.05; **P<0.01; ***P<0.001; ****P<0.0001. N.S., statistically not significant, with the comparisons labeled. All scale bars = 50 μm.
Inhibition of HDAC8 reduces cell apoptosis in vivo and vitro
BAX and cleaved-caspase-3 are both pivotal proteins involved in cell apoptosis (24, 25). In vivo and in vitro experiments (Figure S4), we demonstrated that exposure to 4.25% glucose PDF or TGF-β1 stimulation would promote the cell apoptosis, while blockade of HDAC8 could reduce cell apoptosis.
Discussion
Peritoneal fibrosis is one of the most significant complications for PD patients, however, up to now there is no effective solution (3, 26). In this research, we demonstrated that inhibition of HDAC8 with PCI-34051 or siRNA suppressed EMT, apoptosis and M2 macrophage polarization, ultimately attenuated peritoneal fibrosis and peritoneal dysfunction. Thus, inhibition of HDAC8 may be a potential therapeutic strategy for prevention and treatment of peritoneal fibrosis in long term PD patients.
HDAC8 is a sex-linked gene located at chromosomal position Xq13.1 (27) and expressed both in nucleus and cytoplasm (28–30). HDAC8 has a variety of histone and non-histone (SMC3, α-tubulin, cortactin, HSP20, p53, PKM2, AKT, ERRα and c-Jun) substrates (31–34). As one of the target substrates of HDAC8, cortactin is a ubiquitous multidomain protein involved in the regulation of actin cytoskeleton, integrin signaling and ECM degradation (35). Acetyl-cortactin is localized in the nucleus and can be stimulated by growth factors to transport into the cytoplasm (36, 37). In our study, we found that HDAC8 expression was increased in peritoneum stimulated by high glucose PDF or HPMCs exposed to TGF-β1, and overexpression of HDAC8 inhibited the acetylation of cortactin. Since cortactin is only expressed in the cytoplasm of cells (37), we hypothesized that HDAC8 regulated peritoneal fibrosis by participating in cytoplasmic protein regulation.
EGFR is a tyrosine kinase receptor that binds to ligands and phosphorylates, subsequently leading to the activation of several signaling pathways, including ERK1/2 and STAT3 (8). Our previous studies have demonstrated that activated EGFR promotes peritoneal fibrosis by regulating EMT, inflammation, and angiogenesis (8). In addition, previous studies have demonstrated that EGFR activation is regulated by several histone deacetylases, including HDAC1 (38), HDAC4 (39) and HDAC6 (40, 41). In this research, we found that overexpression of HDAC8 could promote the phosphorylation of EGFR and the activation of its downstream signal molecules. Although the mechanism by which HDAC8 regulates EGFR is still unknown, we speculate that HDAC8 may act by affecting the endocytic trafficking and degradation of EGFR. Activation of EGFR signaling is terminated by endocytosis, and vesicles containing the receptor-ligand complex target lysosomes for degradation along microtubule tracks (42). Acetylation of microtubule component α-tubulin affects the stability of microtubule and further regulates intracellular cargo (such as EGFR-containing vesicles) transport (42). The deacetylation of α-tubulin is mainly mediated by HDAC6, but HDAC8 has also recently been characterized to be involved in the deacetylation of α-tubulin (43). HDAC8 is significantly overexpressed in HeLa cells and may take over the function of HDAC6 as a major deacetylase of α-tubulin (43). Therefore, we speculate that HDAC8 might inhibit EGFR endocytosis by deacetylating α-tubulin in the cytoplasm, and result in the continuous activation of EGFR.
M2 macrophages are believed to be involved in peritoneal fibrosis, and consumption of M2 macrophages in the peritoneum can alleviate peritoneal fibrosis (44). Our study found that blockade of HDAC8 could prevent M2 macrophage polarization via STAT6 and PI3K/Akt signaling pathways. STAT6 is a major factor in the M2 polarization process of macrophages, and its activation drives M2 polarization (45). Recent studies have shown that acetylation of STAT6 can inhibit its transcriptional activity and thus inhibit M2 polarization (46). Although the specific mechanism by which HDAC8 regulates STAT6 has not been reported, several studies have demonstrated the direct regulatory effect of HDACs on STAT transcription factors. A recent study has demonstrated that IL-4-STAT6 signaling is dependent on HDAC3, which performs post-translational modifications and allosteric regulation of STAT6 by occupying STAT6-repressed enhancers (47). The expression and phosphorylation of STAT3 are also regulated by several HDACs, including HDAC1 and HDAC3 (48, 49). Whether HDAC8 affects the activation of STAT6 through acetylation remains to be further investigated. The PI3K/Akt pathway can influence the survival, migration and polarization of macrophages (50). It has been demonstrated that HDAC8 induced tri-methylation of histone H3 lysine 27 through down-regulating the H3K27me3 eraser Jumonji Domain Containing 3 could suppress PTEN expression, thus activating the PI3K/Akt signaling pathway, and further determining susceptibility to cell cycle arrest induced by anthrax lethal toxin (51). In this research, we also found that knockdown of HDAC8 could suppress the activation of PI3K/Akt signaling pathway. The regulatory effect of HDA8 on Akt, on one hand, is mediated by its upstream signaling molecule PI3K; On the other hand, Soon-Duck Ha et al. have demonstrated that HDAC8 activates Akt through upregulating PLCB1 and suppressing DESC1 expression (52). In conclusion, targeting HDAC8 could further regulate M2 macrophage polarization.
At present, the development of HDAC8 inhibitors focuses on improving their activity and high selectivity. Meanwhile, the multi-target pharmacological approach on HDAC8 has gained attention for its benefits from achieving the simultaneous modulation of multiple targets, especially in complex diseases such as cancer and fibrosis (53, 54). PCI-34051 is currently the most widely used HDAC8 inhibitor in the validation of various target diseases due to its considerable subtype selectivity. PCI-34051 in combination with conventional antitumor agents has shown to have a synergistic effect in the reversal of disease (55, 56). Whether the combination of PCI-34051 and other drugs could play a reverse role in fibrosis diseases, which remains to be further studied.
In conclusion, we demonstrated that blockade of HDAC8 could prevent and reverse peritoneal fibrosis. Mechanistically, HDAC8 promoted EMT by inducing the phosphorylation of EGFR and in turn led to the activation of its downstream fibrotic signaling pathways, including STAT3/HIF-1α and ERK1/2. HDAC8 is also a key protein in regulating M2 macrophage polarization via STAT6 and PI3K/Akt signaling pathways (Figure S5). As such, targeting HDAC8 might be a new strategy to lessen the severity of peritoneal fibrosis.
Data availability statement
The original contributions presented in the study are included in the article/Supplementary Materials. Further inquiries can be directed to the corresponding author.
Ethics statement
This study was approved by the Medical Ethics Committee of Shanghai East Hospital and was conducted in accordance with the Declaration of Helsinki. Written informed consent was obtained from each patient. And we have obtained the registration number from the Chinese Clinical Trial Register (ChiCTR): ChiCTR2100052103. The animal protocol was reviewed and approved by the Institutional Animal Care and Use Committee at Tongji University (Shanghai, China).
Author contributions
NL participated in research design. XZ, HC, YS, JL, XM, LD, YH, MT, QZ and DY conducted experiments. XZ, HC, YS and NL contributed new reagents or analytic tools. XZ performed data analysis. XZ, SZ and NL wrote or contributed to the writing of the manuscript. All authors read and approved the final version of the article.
Funding
This study was supported by the Project of the Outstanding Leaders Training Program of Pudong Health Bureau of Shanghai (PWR12021-02 to NL), the National Nature Science Foundation of China grants (82070791 and 81670690 to NL), the Key Discipline Construction Project of Shanghai Pudong New Area Health Commission (PWZxk2022-05 to NL), the Pudong Health Bureau of Shanghai (PW2021D-04 and PWYgf2021-03 to NL), the Shanghai Health Bureau and Shanghai administration of traditional Chinese Medicine of China (ZHYY-ZXYJHZX-202114 to NL), the clinical investigation grant of Shanghai East Hospital (DFLC2022016 to NL), the China Postdoctoral Science Foundation (2021M692436 to YS), the Youth Cultivation Talent Fund of Shanghai East Hospital (DFPY2022011 to YS), the Shanghai Scientific Committee of China (20ZR1445800 to NL).
Conflict of interest
The authors declare that the research was conducted in the absence of any commercial or financial relationships that could be construed as a potential conflict of interest.
Publisher’s note
All claims expressed in this article are solely those of the authors and do not necessarily represent those of their affiliated organizations, or those of the publisher, the editors and the reviewers. Any product that may be evaluated in this article, or claim that may be made by its manufacturer, is not guaranteed or endorsed by the publisher.
Supplementary material
The Supplementary Material for this article can be found online at: https://www.frontiersin.org/articles/10.3389/fimmu.2023.1137332/full#supplementary-material
References
1. Javaid MM, Khan BA, Subramanian S. Peritoneal dialysis as initial dialysis modality: a viable option for late-presenting end-stage renal disease. J Nephrol (2019) 32:51–6. doi: 10.1007/s40620-018-0485-3
2. Kramer A, Pippias M, Noordzij M, Stel VS, Andrusev AM, Aparicio-Madre MI, et al. The European renal association - European dialysis and transplant association (ERA-EDTA) registry annual report 2016: a summary. Clin Kidney J (2019) 12:702–20. doi: 10.1093/ckj/sfz011
3. Masola V, Bonomini M, Borrelli S, Di Liberato L, Vecchi L, Onisto M, et al. Fibrosis of peritoneal membrane as target of new therapies in peritoneal dialysis. Int J Mol Sci (2022) 23:4831. doi: 10.3390/ijms23094831
4. Dzekova-Vidimliski P, Nikolov IG, Gjorgjievski N, Selim G, Trajceska L, Stojanoska A, et al. Peritoneal dialysis-related peritonitis: Rate, clinical outcomes and patient survival. Pril (Makedon Akad Nauk Umet Odd Med Nauki) (2021) 42:47–55. doi: 10.2478/prilozi-2021-0034
5. Davies SJ, Mushahar L, Yu Z, Lambie M. Determinants of peritoneal membrane function over time. Semin Nephrol (2011) 31:172–82. doi: 10.1016/j.semnephrol.2011.01.006
6. Shi Y, Tao M, Wang Y, Zang X, Ma X, Qiu A, et al. Genetic or pharmacologic blockade of enhancer of zeste homolog 2 inhibits the progression of peritoneal fibrosis. J Pathol (2020) 250:79–94. doi: 10.1002/path.5352
7. Wang L, Liu N, Xiong C, Xu L, Shi Y, Qiu A, et al. Inhibition of EGF receptor blocks the development and progression of peritoneal fibrosis. J Am Soc Nephrol (2016) 27:2631–44. doi: 10.1681/ASN.2015030299
8. Liu N, Guo JK, Pang M, Tolbert E, Ponnusamy M, Gong R, et al. Genetic or pharmacologic blockade of EGFR inhibits renal fibrosis. J Am Soc Nephrol (2012) 23:854–67. doi: 10.1681/ASN.2011050493
9. Terri M, Trionfetti F, Montaldo C, Cordani M, Tripodi M, Lopez-Cabrera M, et al. Mechanisms of peritoneal fibrosis: Focus on immune cells-peritoneal stroma interactions. Front Immunol (2021) 12:607204. doi: 10.3389/fimmu.2021.607204
10. Sica A, Mantovani A. Macrophage plasticity and polarization: In vivo veritas. J Clin Invest (2012) 122:787–95. doi: 10.1172/JCI59643
11. Lin J, Kong Q, Hao W, Hu W. High glucose contributes to the polarization of peritoneal macrophages to the M2 phenotype in vivo and in vitro. Mol Med Rep (2020) 22:127–34. doi: 10.3892/mmr.2020.11130
12. Bellón T, Martínez V, Lucendo B, Del PG, Castro MJ, Aroeira LS, et al. Alternative activation of macrophages in human peritoneum: Implications for peritoneal fibrosis. Nephrol Dial Transplant (2011) 26:2995–3005. doi: 10.1093/ndt/gfq771
13. Shi Y, Li J, Chen H, Hu Y, Tang L, Zhou X, et al. Pharmacologic inhibition of histone deacetylase 6 prevents the progression of chlorhexidine gluconate-induced peritoneal fibrosis by blockade of M2 macrophage polarization. Front Immunol (2022) 13:899140. doi: 10.3389/fimmu.2022.899140
14. Shi Y, Tao M, Ni J, Tang L, Liu F, Chen H, et al. Requirement of histone deacetylase 6 for interleukin-6 induced epithelial-mesenchymal transition, proliferation, and migration of peritoneal mesothelial cells. Front Pharmacol (2021) 12:722638. doi: 10.3389/fphar.2021.722638
15. Kim JY, Cho H, Yoo J, Kim GW, Jeon YH, Lee SW, et al. Pathological role of HDAC8: Cancer and beyond. CELLS-BASEL (2022) 11:3161. doi: 10.3390/cells11193161
16. Shi Y, Ni J, Tao M, Ma X, Wang Y, Zang X, et al. Elevated expression of HDAC6 in clinical peritoneal dialysis patients and its pathogenic role on peritoneal angiogenesis. Ren Fail (2020) 42:890–901. doi: 10.1080/0886022X.2020.1811119
17. Ditsawanon P, Supasyndh O, Aramwit P. Dialysate cancer antigen 125 in long-term peritoneal dialysis patients. Clin Exp Nephrol (2014) 18:10–5. doi: 10.1007/s10157-013-0823-7
18. Plum J, Hermann S, Fusshöller A, Schoenicke G, Donner A, Röhrborn A, et al. Peritoneal sclerosis in peritoneal dialysis patients related to dialysis settings and peritoneal transport properties. Kidney Int Suppl (2001) 78:S42–7. doi: 10.1046/j.1523-1755.2001.07801.x
19. Liu Y, Ma Z, Huang Z, Zou D, Li J, Feng P. MiR-122-5p promotes peritoneal fibrosis in a rat model of peritoneal dialysis by targeting Smad5 to activate wnt/β-catenin pathway. Ren Fail (2022) 44:191–203. doi: 10.1080/0886022X.2022.2030360
20. Tian L, Yu Q, Liu D, Chen Z, Zhang Y, Lu J, et al. Epithelial-mesenchymal transition of peritoneal mesothelial cells is enhanced by M2c macrophage polarization. Immunol Invest (2022) 51:301–15. doi: 10.1080/08820139.2020.1828911
21. Xu L, Liu N, Gu H, Wang H, Shi Y, Ma X, et al. Histone deacetylase 6 inhibition counteracts the epithelial-mesenchymal transition of peritoneal mesothelial cells and prevents peritoneal fibrosis. Oncotarget (2017) 8:88730–50. doi: 10.18632/oncotarget.20982
22. Shi Y, Li J, Chen H, Hu Y, Tang L, Wang Y, et al. Inhibition of EZH2 suppresses peritoneal angiogenesis by targeting a VEGFR2/ERK1/2/HIF-1α-dependent signaling pathway. J Pathol (2022) 258:164–78. doi: 10.1002/path.5987
23. Yang X, Bao M, Fang Y, Yu X, Ji J, Ding X. STAT3/HIF-1α signaling activation mediates peritoneal fibrosis induced by high glucose. J Transl Med (2021) 19:283. doi: 10.1186/s12967-021-02946-8
24. Jenner A, Peña-Blanco A, Salvador-Gallego R, Ugarte-Uribe B, Zollo C, Ganief T, et al. DRP1 interacts directly with BAX to induce its activation and apoptosis. EMBO J (2022) 41:e108587. doi: 10.15252/embj.2021108587
25. Savitskaya MA, Onishchenko GE. Mechanisms of apoptosis. Biochem (Mosc) (2015) 80:1393–405. doi: 10.1134/S0006297915110012
26. Nakayama M, Zhu WJ, Watanabe K, Gibo A, Sherif AM, Kabayama S, et al. Dissolved molecular hydrogen (H(2)) in peritoneal dialysis (PD) solutions preserves mesothelial cells and peritoneal membrane integrity. BMC Nephrol (2017) 18:327. doi: 10.1186/s12882-017-0741-0
27. Van den Wyngaert I, de Vries W, Kremer A, Neefs J, Verhasselt P, Luyten WH, et al. Cloning and characterization of human histone deacetylase 8. FEBS Lett (2000) 478:77–83. doi: 10.1016/S0014-5793(00)01813-5
28. Ahn MY. HDAC inhibitor apicidin suppresses murine oral squamous cell carcinoma cell growth in vitro and in vivo via inhibiting HDAC8 expression. Oncol Lett (2018) 16:6552–60. doi: 10.3892/ol.2018.9468
29. Huang C, Shu Y, Zhu Y, Liu H, Wang X, Wen H, et al. Discovery of non-substrate, environmentally sensitive turn-on fluorescent probes for imaging HDAC8 in tumor cells and tissue slices. Bioorg Med Chem (2022) 68:116821. doi: 10.1016/j.bmc.2022.116821
30. Li Y, Liang R, Sun M, Li Z, Sheng H, Wang J, et al. AMPK-dependent phosphorylation of HDAC8 triggers PGM1 expression to promote lung cancer cell survival under glucose starvation. Cancer Lett (2020) 478:82–92. doi: 10.1016/j.canlet.2020.03.007
31. Lopez JE, Haynes SE, Majmudar JD, Martin BR, Fierke CA. HDAC8 substrates identified by genetically encoded active site photocrosslinking. J Am Chem Soc (2017) 139:16222–7. doi: 10.1021/jacs.7b07603
32. Alam N, Zimmerman L, Wolfson NA, Joseph CG, Fierke CA, Schueler-Furman O. Structure-based identification of HDAC8 non-histone substrates. STRUCTURE (2016) 24:458–68. doi: 10.1016/j.str.2016.02.002
33. Kee HJ, Kim I, Jeong MH. Zinc-dependent histone deacetylases: Potential therapeutic targets for arterial hypertension. Biochem Pharmacol (2022) 202:115111. doi: 10.1016/j.bcp.2022.115111
34. Adhikari N, Amin SA, Jha T. Selective and nonselective HDAC8 inhibitors: A therapeutic patent review. Pharm Pat Anal (2018) 7:259–76. doi: 10.4155/ppa-2018-0019
35. Weaver AM. Cortactin in tumor invasiveness. Cancer Lett (2008) 265:157–66. doi: 10.1016/j.canlet.2008.02.066
36. Kirkbride KC, Sung BH, Sinha S, Weaver AM. Cortactin: A multifunctional regulator of cellular invasiveness. Cell Adh Migr (2011) 5:187–98. doi: 10.4161/cam.5.2.14773
37. Zhang X, Yuan Z, Zhang Y, Yong S, Salas-Burgos A, Koomen J, et al. HDAC6 modulates cell motility by altering the acetylation level of cortactin. Mol Cell (2007) 27:197–213. doi: 10.1016/j.molcel.2007.05.033
38. Kakiuchi A, Kakuki T, Ohwada K, Kurose M, Kondoh A, Obata K, et al. HDAC inhibitors suppress the proliferation, migration and invasiveness of human head and neck squamous cell carcinoma cells via p63−mediated tight junction molecules and p21−mediated growth arrest. Oncol Rep (2021) 45:46. doi: 10.3892/or.2021.7997
39. Ahmad R, Kumar B, Pan K, Dhawan P, Singh AB. HDAC-4 regulates claudin-2 expression in EGFR-ERK1/2 dependent manner to regulate colonic epithelial cell differentiation. Oncotarget (2017) 8:87718–36. doi: 10.18632/oncotarget.21190
40. Chen X, Yu C, Hou X, Li J, Li T, Qiu A, et al. Histone deacetylase 6 inhibition mitigates renal fibrosis by suppressing TGF-β and EGFR signaling pathways in obstructive nephropathy. Am J Physiol Renal Physiol (2020) 319:F1003–14. doi: 10.1152/ajprenal.00261.2020
41. Deribe YL, Wild P, Chandrashaker A, Curak J, Schmidt M, Kalaidzidis Y, et al. Regulation of epidermal growth factor receptor trafficking by lysine deacetylase HDAC6. Sci Signal (2009) 2:a84. doi: 10.1126/scisignal.2000576
42. Lee SJ, Li Z, Litan A, Yoo S, Langhans SA. EGF-induced sodium influx regulates EGFR trafficking through HDAC6 and tubulin acetylation. BMC Cell Biol (2015) 16:24. doi: 10.1186/s12860-015-0070-8
43. Vanaja GR, Ramulu HG, Kalle AM. Overexpressed HDAC8 in cervical cancer cells shows functional redundancy of tubulin deacetylation with HDAC6. Cell Commun Signal (2018) 16:20. doi: 10.1186/s12964-018-0231-4
44. Wang J, Jiang ZP, Su N, Fan JJ, Ruan YP, Peng WX, et al. The role of peritoneal alternatively activated macrophages in the process of peritoneal fibrosis related to peritoneal dialysis. Int J Mol Sci (2013) 14:10369–82. doi: 10.3390/ijms140510369
45. Gong M, Zhuo X, Ma A. STAT6 upregulation promotes M2 macrophage polarization to suppress atherosclerosis. Med Sci Monit Basic Res (2017) 23:240–9. doi: 10.12659/MSMBR.904014
46. Yu T, Gan S, Zhu Q, Dai D, Li N, Wang H, et al. Modulation of M2 macrophage polarization by the crosstalk between Stat6 and Trim24. Nat Commun (2019) 10:4353. doi: 10.1038/s41467-019-12384-2
47. Czimmerer Z, Daniel B, Horvath A, Rückerl D, Nagy G, Kiss M, et al. The transcription factor STAT6 mediates direct repression of inflammatory enhancers and limits activation of alternatively polarized macrophages. IMMUNITY (2018) 48:75–90. doi: 10.1016/j.immuni.2017.12.010
48. Du W, Wang N, Li F, Jia K, An J, Liu Y, et al. STAT3 phosphorylation mediates high glucose-impaired cell autophagy in an HDAC1-dependent and -independent manner in schwann cells of diabetic peripheral neuropathy. FASEB J (2019) 33:8008–21. doi: 10.1096/fj.201900127R
49. Icardi L, Mori R, Gesellchen V, Eyckerman S, De Cauwer L, Verhelst J, et al. The Sin3a repressor complex is a master regulator of STAT transcriptional activity. Proc Natl Acad Sci U.S.A. (2012) 109:12058–63. doi: 10.1073/pnas.1206458109
50. Vergadi E, Ieronymaki E, Lyroni K, Vaporidi K, Tsatsanis C. Akt signaling pathway in macrophage activation and M1/M2 polarization. J Immunol (2017) 198:1006–14. doi: 10.4049/jimmunol.1601515
51. Ha SD, Cho W, Kim SO. HDAC8 prevents anthrax lethal toxin-induced cell cycle arrest through silencing PTEN in human monocytic THP-1 cells. Toxins (Basel) (2017) 9:162. doi: 10.3390/toxins9050162
52. Ha SD, Lewin N, Li S, Kim SO. HDAC8 activates AKT through upregulating PLCB1 and suppressing DESC1 expression in MEK1/2 inhibition-resistant cells. CELLS-BASEL (2021) 10:1101. doi: 10.3390/cells10051101
53. Smalley JP, Cowley SM, Hodgkinson JT. Bifunctional HDAC therapeutics: One drug to rule them all? MOLECULES (2020) 25:4394. doi: 10.3390/molecules25194394
54. Fontana A, Cursaro I, Carullo G, Gemma S, Butini S, Campiani G, et al. A therapeutic perspective of HDAC8 in different diseases: An overview of selective inhibitors. Int J Mol Sci (2022) 23:10014. doi: 10.3390/ijms231710014
55. Pantelaiou-Prokaki G, Mieczkowska I, Schmidt GE, Fritzsche S, Prokakis E, Gallwas J, et al. HDAC8 suppresses the epithelial phenotype and promotes EMT in chemotherapy-treated basal-like breast cancer. Clin Epigenet (2022) 14:7. doi: 10.1186/s13148-022-01228-4
Keywords: histone deacetylase 8, epithelial-mesenchymal transition, epidermal growth factor receptor, macrophage polarization, peritoneal fibrosis
Citation: Zhou X, Chen H, Shi Y, Li J, Ma X, Du L, Hu Y, Tao M, Zhong Q, Yan D, Zhuang S and Liu N (2023) Histone deacetylase 8 inhibition prevents the progression of peritoneal fibrosis by counteracting the epithelial-mesenchymal transition and blockade of M2 macrophage polarization. Front. Immunol. 14:1137332. doi: 10.3389/fimmu.2023.1137332
Received: 04 January 2023; Accepted: 10 February 2023;
Published: 23 February 2023.
Edited by:
Liang Ma, Sichuan University, ChinaReviewed by:
Fujun Lin, Shanghai Jiao Tong University, ChinaQi Bian, Changhai Hospital, China
Bing Li, Second Affiliated Hospital of Hainan Medical University, China
Copyright © 2023 Zhou, Chen, Shi, Li, Ma, Du, Hu, Tao, Zhong, Yan, Zhuang and Liu. This is an open-access article distributed under the terms of the Creative Commons Attribution License (CC BY). The use, distribution or reproduction in other forums is permitted, provided the original author(s) and the copyright owner(s) are credited and that the original publication in this journal is cited, in accordance with accepted academic practice. No use, distribution or reproduction is permitted which does not comply with these terms.
*Correspondence: Na Liu, bmFsaXVicm93bkAxNjMuY29t