- 1Oncoematologia e Officina Farmaceutica, Bambino Gesù Children's Hospital, IRCCS, Rome, Italy
- 2General Directorate for Research and Health Innovation, Italian Ministry of Health, Rome, Italy
- 3Department of Pediatrics, Catholic University of the Sacred Heart, Rome, Italy
- 4Department of Infectious, Tropical Diseases and Microbiology, IRCCS Sacro Cuore Don Calabria Hospital, Negrar di Valpolicella, Verona, Italy
- 5Unicamillus, International Medical University of Rome, Rome, Italy
The immune response to invading pathogens is characterized by the rapid establishment of a complex network of cellular interactions and soluble signals. The correct balancing of activating and regulating pathways and tissue-homing signals determines its effectiveness and persistence over time. Emerging viral pathogens have always represented a great challenge to the immune system and an often uncontrolled/imbalanced immune response has been described (e.g. cytokine storm, immune paralysis), contributing to the severity of the disease. Several immune biomarkers and cell subsets have been identified as major players in the cascade of events leading to severe diseases, highlighting the rationale for host-directed intervention strategy. There are millions of immunocompromised pediatric and adult patients worldwide (e.g. transplant recipients, hematologic patients, subjects with primary immune-deficiencies), experiencing an impaired immune reactivity, due to diseases and/or to the medical treatments. The reduced immune reactivity could have two paradoxical non-exclusive effects: a weak protective immunity on one hand, and a reduced contribution to immune-mediated pathogenetic processes on the other hand. In these sensitive contexts, the impact of emerging infections represents a still open issue to be explored with several challenges for immunologists, virologists, physicians and epidemiologists. In this review, we will address emerging infections in immunocompromised hosts, to summarize the available data concerning the immune response profile, its influence on the clinical presentation, the possible contribution of persistent viral shedding in generating new viral variants with improved immune escape features, and the key role of vaccination.
1 Introduction
In the last years, we continuously and suddenly faced with viruses that cause serious health concern. Immunocompromised (IC) subjects represent a heterogeneous group of pediatric and adult patients at increased risk of morbidity and mortality for infectious diseases compared to the general population, due to altered susceptibility to infectious agents and to impaired capacity to fight them, with possible critical clinical course and eventual long-term sequelae.
The reduced immune reactivity of IC patients may be due to the underlying disease itself (e.g., hematologic malignancy, solid tumors, congenital immunodeficiency, acquired immunodeficiency including AIDS with low CD4 counts, autoimmune disease, etc.) and/or to ongoing immunosuppressive treatments (e.g., hematopoietic stem cell transplantation (HSCT), solid organ transplantation (SOT), B-cell depleting drugs, steroids, etc.), which can strongly affect the immune system reactivity. Alongside these risk factors, IC patients have frequent contacts with the healthcare system that, in turn, may increase the risk of acquiring infectious diseases. In recent years, the pediatric and adult IC populations reached about 2-3% of the general population and the growing use of biological agents in several clinical settings will certainly contribute to increase the proportion of people showing some immunosuppressed traits.
An emerging infectious disease is one that either has appeared and affects a given population for the first time, or has existed previously, but is rapidly spreading, in terms of either number of people getting infected, or new geographical areas invaded. The majority of these infections are zoonotic, like SARS-CoV, West Nile Virus, Yellow Fever, and H1N1, and several factors (climatic, demographic, social) favour their spread (1). Other infections may re-emerge as a consequence of the failure in the healthcare system, as it occurred recently for measles (‘officially’ eradicated from the United States in 2000) and poliovirus, that reappeared in western countries afters years of silence (2, 3) possibly by new or previously underestimated way of transmission (4). In addition, already known viruses are recognized as potentially leading to important sequelae in vulnerable patients (e.g., Human Herpesviruses 6 and 7, or Adenoviruses) or cause of new diseases as a consequence of more potent immunosuppressive regimens recently available (e.g., polyomavirus BK). The list of rare and emerging viral pathogens in the immunocompromised population is quite long and unfortunately it is continuously growing, as demonstrated by recent relevant public health treats [Ebola 2013-2016, Zika 2015, Coronaviruses (MERS 2012, SARS-CoV-2 2019-ongoing), Influenza H1N1 (2009), H7N9 and Monkeypox]. Here, we aimed to summarize the evidences concerning: i) the complex scenario of the immune response to emerging viral infection in IC patients; ii) the impact of immune frailties on the clinical presentation of emerging viral infections and on viral shedding and iii) the vaccine efficacy in IC patients.
2 The immune response in IC patients: A complex scenario
Most viral infections cause self-limiting diseases of short duration. A well-coordinated humoral and cell-mediated immune response can effectively control and clear the infectious agent without causing damage to the host. However, the same virus in an IC host can result in more severe and longer disease, worsened by increased rates of bacterial and fungal superinfections (5, 6). The immune deficiency in IC patients can have different consequences, depending on the duration, the degree of immunosuppression and on the type of immune targets (humoral, cellular response or both).
One common feature in the pathogenesis of several emerging diseases (e.g. Flaviviruses, Filoviruses, SARS-CoV-2, etc.) is the role of early uncontrolled cytokine storm as the main driver of tissue damage, leading to immune-mediated multi-organ injury and disease progression (7–10). In this scenario, we could speculate that immunosuppression could paradoxically have a beneficial effect, dampening the excessive inflammatory response and reducing the immune-mediated damage (11). The huge number of SARS-CoV-2 infections in different clinical settings allowed scientists to study the impact of different immune frailties on the delicate balance between pathogenetic and protective actions during the course of a viral infection. The effect of immunosuppression induced by disease and/or treatment on COVID-19 clinical outcome can highlight a paradox: immunosuppressive conditions could be harmful in the initial phase of COVID-19, when the host immune response is necessary to inhibit viral replication. On the other hand, they might have a beneficial effect in the later, more severe, phase of COVID-19, preventing hyperinflammation and massive cytokine release that are, in fact, the main trigger of tissue injury and disease worsening (12). The observation that IC patients receiving biological drugs have a non-increased risk of disease severity (11, 13) suggests that the reduction of the immune reactivity might, indeed, contribute to dampen the cytokine storm-induced pathogenetic mechanisms. According to this hypothesis, the effectiveness of immunosuppressive treatment (e.g. dexamethasone, tocilizumab, baricitinib) has been tested in several randomised controlled trials, showing survival benefits in non-IC patients with SARS-CoV-2 infection (14–17). The detrimental role of inflammatory immune reactivity has been also confirmed in young children, whose tolerogenic immune profile has been associated with a generally asymptomatic/mild clinical course (18). Of note, the loss of this regulatory system can be responsible for the severe hyperinflammatory disorders occurring rarely in children with recent SARS-CoV-2 infection, such as MIS-C (Multisystem Inflammatory Syndrome Related in Children) (18, 19).
On the other hand, in more complex clinical settings (such as cancer patients and transplant recipients), the higher severity of SARS-CoV-2 infection could be explained better by the overall fragility, multiorgan involvement and chemotherapy-induced cytopenia inflammatory/suppressive profile rather than by impaired antiviral status (11, 20–23).
The tumor microenvironment (TME) is characterized by several cell types (e.g., fibroblasts, endothelial cells, macrophages, neutrophils, etc.) exerting potent immunomodulatory effects and contribute to cancer progression (24–26). The inflammatory/suppressive signals in TME promote the recruitment and expansion of several type of immunosuppressive cells such as myeloid derived suppressor cells (MDSCs), tumor-associated macrophages (TAMs) or Treg responsible for the strong inhibition of the host immune response (27–29). A similar suppressor cell expansion as well the increase in the neutrophil/lymphocyte ratio that characterize cancer patients (30) was also observed during viral emerging infection such as COVID-19 (31, 32). Their effects in dampening both antiviral and antitumoral immunity can therefore be exacerbated in cancer patients experiencing emerging infections.
A crucial role of T cells in protecting against the severe diseases has been defined in several emerging infections such as Zika (33), Dengue (34), SARS-CoV-2 (35) and influenza (36). The antiviral T cell effectiveness, together with their cross-recognition capability, give to these cells the role of crucial actor in IC patients with a deficit in humoral response, such as patients treated with B-cell depleting agents. Accordingly, CD8 T cells were associated with improved survival after SARS-CoV-2 infection in hematologic cancer patients showing an impaired humoral response, including those treated with anti-CD20 therapy (37). On the other hand, a loss of T cells can result in worsened morbidity and mortality after ZIKA infection (38). These results highlight the potential protective role of T cells in IC patients, and strengthen the beneficial approach of vaccination where available (39, 40) as well as of other cell-based approaches (41).
3 Immune failure, clinical outcome and persistent infection
The poor immune responsiveness in IC patients may reduce the inflammatory storm after infection, but, on the other hand, may impair the host’s ability to clear the virus, resulting in a persistent infection characterized by a prolonged viral replication at low level, in the absence of immune pathogenic processes. Common examples are represented by RNA viruses such as influenza A (42), rhinoviruses (43), and also polioviruses for whom chronic replication up to 28 years has been reported (44). In addition to these prominent examples, increasing evidences suggest that also emerging/re-emerging viruses can represent a relevant issue due to prolonged viral shedding in IC hosts.
A critical point in persistent infections is their association with enhanced viral genetic diversity with potentially higher rates of viral evolution compared to acute self-limiting infections, leading to the suggestions that IC hosts may represent an important reservoir for the emergence of novel viral variants possessing an immune-escape (45–47), or drug-resistant strain (48) phenotype. Among the emerging/re-emerging viruses, the most common persistent infections in IC patients have been observed with Dengue viruses, West Nile virus (WNV), SARS-CoV-2, hepatitis E virus, Molluscum contagiosum (49, 50), but also with new variants of adenoviruses (51).
In IC patients with a severe form of Dengue (e.g. shock syndrome or hemorrhagic fever), a prolonged period (median 30 days, range 18-80 days) of illness has been described (52, 53), and the full resolution of the infection coincided with the CD8 T-cell recovery, suggesting a role for cellular immunity in limiting the extent and duration of the infection (54). Main hematological dysfunctions of Dengue fever are leukopenia, thrombocytopenia and bone marrow impairment that can further worsen the chemotherapy-induced myelosuppression, resulting in bleeding and deep immune impairment that, in turn, is associated with an increased risk of secondary bacterial infections (55) and a high mortality rate (52, 56).
While WNV infection occurs asymptomatically in the vast majority of persons, the risk for neuroinvasive disease is significantly increased in IC patients (57). Moreover, IC patients may experience a severe neuropathological damage and multiorgan localization associated with prolonged infection (58). Of note, WNV can be detected in the blood for up to 28 days in IC patients, as compared to a maximum duration of 10 days in immunocompetent subjects (59).
Prolonged SARS-CoV-2 infections have been very well documented in IC patients. Among factors associated to this phenomenon, the B-cell depletion induced by a range of clinical or therapeutical conditions (e.g. hematological malignancies, bi-specific T-cell engagers (BiTEs), Chimeric Antigen Receptor (CAR)-modified T-cell therapy, HSCT or anti-CD20 monoclonal antibodies administration) represents a good example (60, 61). A few reported cases provide compelling evidence of prolonged infection even in SOT, AIDS, and/or other conditions (62). Nonetheless, several key questions remain about immunocompromised individuals with protracted COVID-19 not only to improve the well-being of the patient, but also to better understand intra-host viral evolution leading to the emergence of novel variants and to immune escape mechanisms (63).
The diagnosis of persistent hepatitis E virus infection has been rarely reported in IC patients, and the paucity of symptoms associated with persistent HEV infection strongly contributes to the delayed or missed diagnosis (64). Molluscum contagiosum virus is a double-stranded DNA virus component of the Poxviridae family, commonly seen in the pediatric population. It infects human keratinocytes resulting in small, umbilicated, flesh-coloured papules. Normally considered a self-limited disease, in IC patients, the presentation of MC is prolonged and can have different clinical presentation. Extensive and atypical presentation of normally self-limited infectious dermatosis has been reported in SOT recipients, patients with hematologic malignancies such as lymphoma and leukemia and in other iatrogenic immunosuppressive states (65). Moreover, it is well known that adenoviral infections can cause viral persistence and disseminated disease in IC patients. Recent observations rely to the potential role of new variants emerged both in United States and Asia. The primary source of disseminated infections in IC patients seems to be related to the reactivation of persistent ADV infection (66).
4 Vaccine efficacy: A critical point in immunocompromised patients
The impaired immune response in IC patients can make these patients more susceptible to infections, to develop severe diseases and, on the other hand, to be less responsive to vaccination. Influenza represents a good example: IC people can experience complicated disease characterized by progression to lower respiratory infection and bacterial superinfection, and, on the other hand, they show a poor response to vaccination (67). Indeed, the overall immunogenicity of influenza vaccine is lower in HSCT, where risk factors associated with a poor response include use of calcineurin inhibitors, chronic GvHD, shorter time post-transplant, as well as low IgM levels (68, 69). Accordingly, a lower immunogenicity has also been reported in SOT recipients as compared to the general population (70), and is dependent both on type of transplant and immunosuppressive regimen, being mycophenolate mofetil the drug with the greatest negative impact (71).
The number of IC patients at risk of encountering an emerging virus is growing both in endemic countries and in the context of tourist travel. Indeed, the advancement in the clinical and therapeutical management of IC hosts has promoted a significant improvement of their quality of life, which, in turn, has allowed them to travel abroad. The negative side of this improvement is the consequent risk to be exposed to tropical diseases, including rabies. Animal-associated injuries are common in travellers (72) and, in rabies enzootic countries, should lead to a prompt post-exposure prophylaxis (PEP). PEP regimen consists of the administration of a series of four rabies vaccine shots and rabies immunoglobulins (73) and results highly effective in preventing the disease in injured persons. IC patients usually receive a fifth vaccine dose, and should be tested for seroconversion 7 to 14 days following completion of the PEP regimen. Despite an additional vaccine dose, PEP seems to be less immunogenic in IC patients, as a detectable humoral response could be detected in a minority of tested patients (74). Nevertheless, further screening of immune response in IC patients is mandatory to define factors associated with inadequate response (75).
The incredibly successful development of highly effective SARS-CoV-2 vaccine platforms has changed the history of the COVID-19 pandemic, avoiding millions of deaths worldwide (76). In parallel with the success of the clinical standpoint, mass vaccination with mRNA vaccines has offered us a great opportunity to fully understand the immunological defects associated with different fragilities. The injection of the same antigen with the same schedule in millions of people with different ages, races, diseases, therapies, comorbidities, and the parallel analysis of both humoral and cellular immune response has opened a new scenario to fully define the mechanisms of immune-suppression and to design new host-directed immunological approaches.
A recent meta-analysis summarized that the seroconversion rate after two COVID-19 vaccine doses in IC patients was lower than that observed in healthy control: 92% (88–94%) for patients with solid cancer, 78% (69–95) for patients with immune-mediated inflammatory disorders, 64% (50–76) for patients with haematological neoplasia, and 27% (16–42) for transplant recipients (77). Accordingly, IC patients were at higher risk for symptomatic and severe COVID-19 (78) and account for more than 40% of hospitalised breakthrough cases, despite representing a much smaller proportion of the general population (79). In a prospective study including several of IC groups (hematological, solid tumors, neurological and rheumatologic patients) the independent predictors of seroconversion were age, diseases and treatment (39). In particular, the B cell-targeting therapy (e.g. anti-CD20 monoclonal antibody, anti-CD19 CAR T cells) was associated with the worst humoral response to vaccination, making these patients particularly vulnerable to SARS-CoV-2 infection. In contrast, spike-specific T-cell response is less susceptible to both diseases and treatment, highlighting its main role as a backbone of the immune response also in highly IC patients where they can exert a protective activities against the severe COVID-19 (35, 39, 80). The booster doses (third and fourth doses) improved the humoral response in all disease groups, although to a lesser extent in patients with hematological malignancies, whereas the T-cell response increased similarly in all IC groups (39, 81).
The analysis of the vaccine response in patients with transfusion-dependent thalassemia shed light on another aspect of immunity impairment. These patients were able to mount a good immune response to mRNA vaccination, with a strength similar to that observed in healthy donors, but their response decreased overtime faster than in healthy donors, highlighting a fragility in the persistence rather than in the power of the immune reactivity, which can be interpreted in light of an early immune senescence (82).
A huge effort has been making to define factors associated with a poor immunogenicity in different fragile patients with the awareness that these results could have a much greater impact than that of the COVID-19 vaccination context. The definition of the molecular mechanisms of immune fragility linked to individual pathologies, therapies, comorbidities, age, etc., can indeed provide new useful tools for improving the management of these patients and for designing the best vaccination strategy. Several critical issues need to be pointed out when addressing the vaccination in the fragile clinical settings, such as the best time for the vaccine administration (e.g. time from transplantation, time from anti-CD20 therapy start, etc.), the possibility to temporarily discontinue the immunosuppressive treatment and the definition of the optimal vaccine schedule.
Finally, in the development of new vaccination strategies against emerging and re-emerging infectious diseases, we need to remind that IC patients cannot receive live vaccines because their immune suppression condition may lead to the uncontrolled replication of the vaccine virus, with consequent trigger of virus-induced pathogenic events.
5 Conclusion
Fragile patients are characterized by different immune impairment signals affecting the overall immune protection that can be further worsened by emerging viral infections. Accordingly, the management of these infections in IC patients represents a relevant clinical challenge.
In healthy subjects, emerging infection may induce an excessive innate inflammatory response (cytokine storm), representing the main diver of tissue injury and disease progression. At the same time, the immunocompetent immune system recognizes viral antigens, differentiates, and mediates a protective immune response, resulting in virus clearance and prompt clinical recovery (Figure 1A). On the contrary, in the IC individual, we can observe a paradox: the low immune reactivity significantly reduces the inflammatory response partially avoiding tissue damage; on the other hand, the immune impairment can be unable to effectively clear the virus, resulting in persistent non-severe infections (Figure 1B). In this perspective, immunosuppression might be non-detrimental or even advantageous, as demonstrated in several clinical trial addressing SARS-CoV-2 infection.
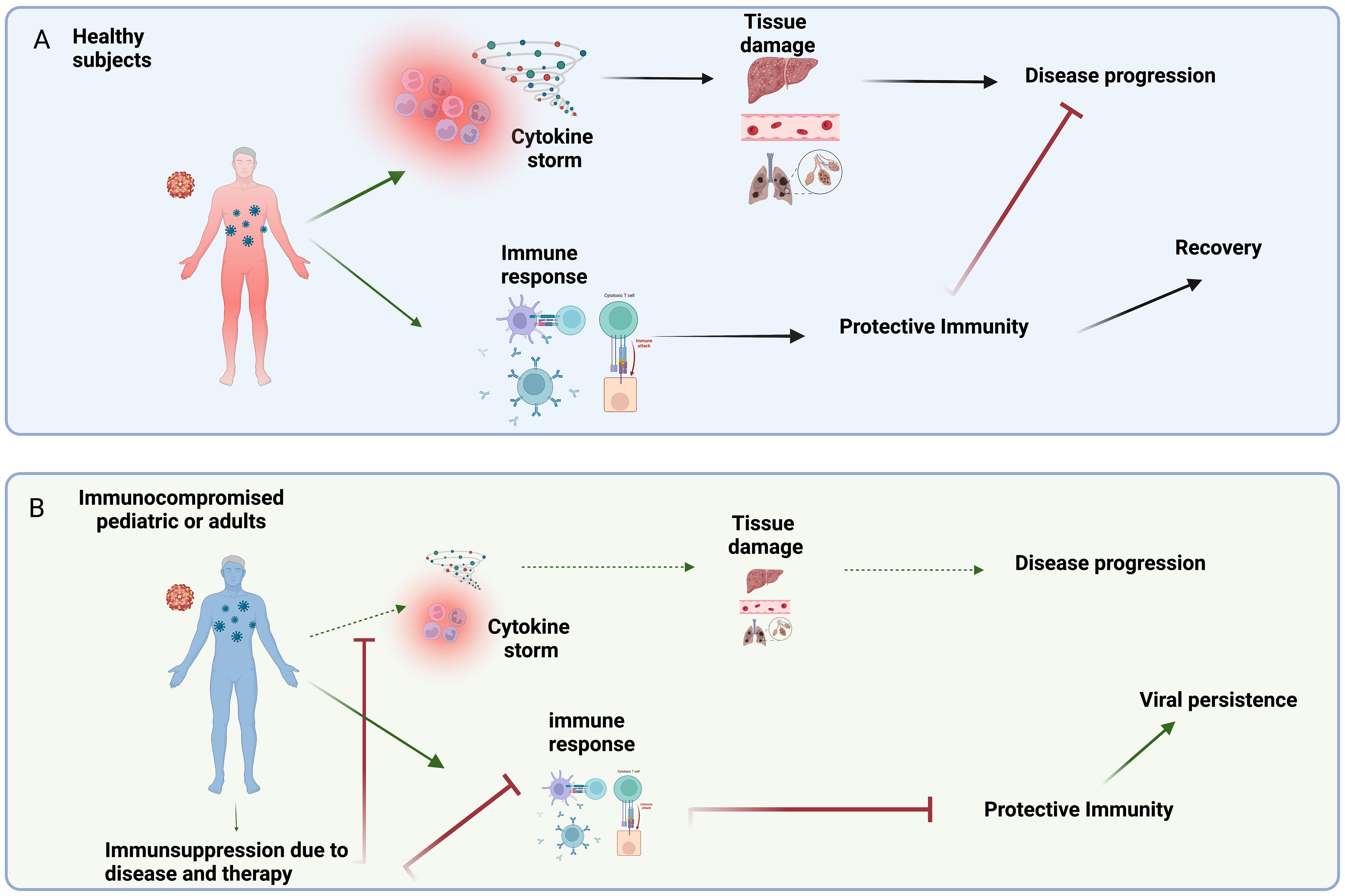
Figure 1 (A) In healthy subjects, emerging viral infections induce an uncontrolled/imbalanced release of pro-inflammatory mediators (e.g. cytokine storm) that can contribute to tissue damage and disease progression. On the other hand, the competent immune system can mediate a protective immune response leading to clinical recovery. (B) The low immune reactivity characterizing the IC patients, significantly reduces the inflammatory response partially avoiding tissue damage and disease progression. On the other hand, the immunosuppression due to disease and/or therapy can reduce the ability to induce a protective immunity, thus resulting in persistent non-severe infections. Created in BioRender.com.bio.
Author contributions
CA, FL, AC, MC, GI conceived the review; CA, BB, CC and VB drafted the paper; CA and VB prepared the figure; FL, AC and GI reviewed the paper; all authors agreed on the final version.
Funding
This research was supported by the Italian Ministry of Health “Fondi Ricerca Corrente” to IRCCS Sacro Cuore Don Calabria Hospital – L3P1, and by EU funding within the MUR PNRR Extended Partnership initiative on Emerging Infectious Diseases (Project no. PE00000007, INF-ACT. This research was supported by the Italian Ministry of Health “Fondi Ricerca Corrente” to Bambino Gesù Children's Hospital, IRCCS.
Conflict of interest
The authors declare that the research was conducted in the absence of any commercial or financial relationships that could be construed as a potential conflict of interest.
Publisher’s note
All claims expressed in this article are solely those of the authors and do not necessarily represent those of their affiliated organizations, or those of the publisher, the editors and the reviewers. Any product that may be evaluated in this article, or claim that may be made by its manufacturer, is not guaranteed or endorsed by the publisher.
Abbreviations
IC, Immunocompromised; HSCT, Hematopoietic Stem Cell Transplant; SOT, Solid Organ Transplant; SARS, Severe Acute Respiratory Syndrome; MERS, Middle-East Respiratory Syndrome; WNV, West Nile Virus; CAR, Chimeric Antigen Receptor; GvHD, Graft-versus-Host Disease; PEP, Post-Exposure Prophylaxis.
References
1. Plowright RK, Parrish CR, McCallum H, Hudson PJ, Ko AI, Graham AL, et al. Pathways to zoonotic spillover. Nat Rev Microbiol (2017) 15:502–10. doi: 10.1038/nrmicro.2017.45
2. (2022). Available at: https://polioeradication.org/news-post/report-of-polio-detection-in-united-states/.
3. (2022). Available at: https://polioeradication.org/news-post/vaccine-derived-poliovirus-type-2-vdpv2-detected-in-environmental-samples-in-london-uk/.
4. John TJ, Dharmapalan D. Lessons from vaccine-related poliovirus in Israel, UK and USA. Vaccines (Basel) (2022) 10:1969. doi: 10.3390/vaccines10111969
5. Manuel O, Estabrook M, American Society of Transplantation Infectious Diseases Community of Practice. RNA Respiratory viral infections in solid organ transplant recipients: Guidelines from the American society of transplantation infectious diseases community of practice. Clin Transplant (2019) 33:e13511. doi: 10.1111/ctr.13511
6. Waghmare A, Englund JA, Boeckh M. How I treat respiratory viral infections in the setting of intensive chemotherapy or hematopoietic cell transplantation. Blood (2016) 127:2682–92. doi: 10.1182/blood-2016-01-634873
7. Falasca L, Agrati C, Petrosillo N, Di Caro A, Capobianchi MR, Ippolito G, et al. Molecular mechanisms of Ebola virus pathogenesis: Focus on cell death. Cell Death Differ (2015) 22:1250–9. doi: 10.1038/cdd.2015.67
8. Del Valle DM, Kim-Schulze S, Huang HH, Beckmann ND, Nirenberg S, Wang B, et al. An inflammatory cytokine signature predicts COVID-19 severity and survival. Nat Med (2020) 26:1636–43. doi: 10.1038/s41591-020-1051-9
9. Srikiatkhachorn A, Mathew A, Rothman AL. Immune-mediated cytokine storm and its role in severe dengue. Semin Immunopathol (2017) 39:563–74. doi: 10.1007/s00281-017-0625-1
10. Agrati C, Carsetti R, Bordoni V, Sacchi A, Quintarelli C, Locatelli F, et al. The immune response as a double-edged sword: The lesson learnt during the COVID-19 pandemic. Immunology (2022) 167:287–302. doi: 10.1111/imm.13564
11. Fung M, Babik JM. COVID-19 in immunocompromised hosts: What we know so far. Clin Infect Dis (2021) 72:340–50. doi: 10.1093/cid/ciaa863
12. Schoot TS, Kerckhoffs APM, Hilbrands LB, van Marum RJ. Immunosuppressive drugs and COVID-19: A review. Front Pharmacol (2020) 11:1333. doi: 10.3389/fphar.2020.01333
13. Salvarani C, Bajocchi G, Mancuso P, Galli E, Muratore F, Boiardi L, et al. Susceptibility and severity of COVID-19 in patients treated with bDMARDS and tsDMARDs: A population-based study. Ann Rheum Dis (2020) 79:986–8. doi: 10.1136/annrheumdis-2020-217903
14. Group RC, Horby P, Lim WS, Emberson JR, Mafham M, Bell JL, et al. Dexamethasone in hospitalized patients with covid-19. N Engl J Med (2021) 384:693–704. doi: 10.1056/NEJMoa2021436
15. Group RC. Tocilizumab in patients admitted to hospital with COVID-19 (RECOVERY): a randomised, controlled, open-label, platform trial. Lancet (2021) 397:1637–45. doi: 10.1016/S0140-6736(21)00676-0
16. Kalil AC, Patterson TF, Mehta AK, Tomashek KM, Wolfe CR, Ghazaryan V, et al. Baricitinib plus remdesivir for hospitalized adults with covid-19. N Engl J Med (2021) 384:795–807. doi: 10.1056/NEJMoa2031994
17. Mehta P, McAuley DF, Brown M, Sanchez E, Tattersall RS, Manson JJ, et al. COVID-19: consider cytokine storm syndromes and immunosuppression. Lancet (2020) 395:1033–4. doi: 10.1016/S0140-6736(20)30628-0
18. Nicastro E, Verdoni L, Bettini LR, Zuin G, Balduzzi A, Montini G, et al. COVID-19 in immunosuppressed children. Front Pediatr (2021) 9:629240. doi: 10.3389/fped.2021.629240
19. Cheung EW, Zachariah P, Gorelik M, Boneparth A, Kernie SG, Orange JS, et al. Multisystem inflammatory syndrome related to COVID-19 in previously healthy children and adolescents in new York city. JAMA (2020) 324:294–6. doi: 10.1001/jama.2020.10374
20. Kuderer NM, Choueiri TK, Shah DP, Shyr Y, Rubinstein SM, Rivera DR, et al. Covid and c. cancer, clinical impact of COVID-19 on patients with cancer (CCC19): a cohort study. Lancet (2020) 395:1907–18. doi: 10.1016/S0140-6736(20)31187-9
21. Lee LY, Cazier JB, Angelis V, Arnold R, Bisht V, Campton NA, et al. COVID-19 mortality in patients with cancer on chemotherapy or other anticancer treatments: A prospective cohort study. Lancet (2020) 395:1919–26. doi: 10.1016/S0140-6736(20)31173-9
22. Aries JA, Davies JK, Auer RL, Hallam SL, Montoto S, Smith M, et al. Clinical outcome of coronavirus disease 2019 in haemato-oncology patients. Br J Haematol (2020) 190:e64–7. doi: 10.1111/bjh.16852
23. Robilotti EV, Babady NE, Mead PA, Rolling T, Perez-Johnston R, Bernardes M, et al. Determinants of COVID-19 disease severity in patients with cancer. Nat Med (2020) 26:1218–23. doi: 10.1038/s41591-020-0979-0
24. Cassetta L, Pollard JW. A timeline of tumour-associated macrophage biology. Nat Rev Cancer (2023). doi: 10.1038/s41568-022-00547-1
25. Galdiero MR, Varricchi G, Loffredo S, Mantovani A, Marone G. Roles of neutrophils in cancer growth and progression. J Leukoc Biol (2018) 103:457–64. doi: 10.1002/JLB.3MR0717-292R
26. Zhao H, Wu L, Yan G, Chen Y, Zhou M, Wu Y, et al. Inflammation and tumor progression: Signaling pathways and targeted intervention. Signal Transduct Target Ther (2021) 6:263. doi: 10.1038/s41392-021-00658-5
27. Ya G, Ren W, Qin R, He J, Zhao S. Role of myeloid-derived suppressor cells in the formation of pre-metastatic niche. Front Oncol (2022) 12:975261. doi: 10.3389/fonc.2022.975261
28. Solinas G, Germano G, Mantovani A, Allavena P. Tumor-associated macrophages (TAM) as major players of the cancer-related inflammation. J Leukoc Biol (2009) 86:1065–73. doi: 10.1189/jlb.0609385
29. Munn DH, Bronte V. Immune suppressive mechanisms in the tumor microenvironment. Curr Opin Immunol (2016) 39:1–6. doi: 10.1016/j.coi.2015.10.009
30. Scilla KA, Bentzen SM, Lam VK, Mohindra P, Nichols EM, Vyfhuis MA, et al. Neutrophil-lymphocyte ratio is a prognostic marker in patients with locally advanced (Stage IIIA and IIIB) non-small cell lung cancer treated with combined modality therapy. Oncologist (2017) 22:737–42. doi: 10.1634/theoncologist.2016-0443
31. Grassi G, Notari S, Gili S, Bordoni V, Casetti R, Cimini E, et al. Myeloid-derived suppressor cells in COVID-19: The paradox of good. Front Immunol (2022) 13:842949. doi: 10.3389/fimmu.2022.842949
32. Liu Y, Du X, Chen J, Jin Y, Peng L, Wang HHX, et al. Neutrophil-to-lymphocyte ratio as an independent risk factor for mortality in hospitalized patients with COVID-19. J Infect (2020) 81:e6–e12. doi: 10.1016/j.jinf.2020.04.002
33. Lai L, Rouphael N, Xu Y, Natrajan MS, Beck A, Hart M, et al. Innate, T-, and b-cell responses in acute human zika patients. Clin Infect Dis (2018) 66:1–10. doi: 10.1093/cid/cix732
34. Tian Y, Grifoni A, Sette A, Weiskopf D. Human T cell response to dengue virus infection. Front Immunol (2019) 10:2125. doi: 10.3389/fimmu.2019.02125
35. Le Bert N, Clapham HE, Tan AT, Chia WN, Tham CYL, Lim JM, et al. Highly functional virus-specific cellular immune response in asymptomatic SARS-CoV-2 infection. J Exp Med (2021) 218:e20202617. doi: 10.1084/jem.20202617
36. Tsang TK, Lam KT, Liu Y, Fang VJ, Mu X, Leung NHL, et al. Investigation of CD4 and CD8 T cell-mediated protection against influenza a virus in a cohort study. BMC Med (2022) 20:230. doi: 10.1186/s12916-022-02429-7
37. Bange EM, Han NA, Wileyto P, Kim JY, Gouma S, Robinson J, et al. CD8(+) T cells contribute to survival in patients with COVID-19 and hematologic cancer. Nat Med (2021) 27:1280–9. doi: 10.1038/s41591-021-01386-7
38. Pardy RD, Richer MJ. Protective to a T: The role of T cells during zika virus infection. Cells (2019) 8:820. doi: 10.3390/cells8080820
39. Corradini P, Agrati C, Apolone G, Mantovani A, Giannarelli D, Marasco V, et al. Humoral and T-cell immune response after three doses of mRNA SARS-CoV-2 vaccines in fragile patients: the Italian VAX4FRAIL study. Clin Infect Dis (2022) 76(3):e426–e438. doi: 10.1101/2022.01.12.22269133
40. Caldera F, Mercer M, Samson SI, Pitt JM, Hayney MS. Influenza vaccination in immunocompromised populations: Strategies to improve immunogenicity. Vaccine (2021) 39 Suppl 1:A15–23. doi: 10.1016/j.vaccine.2020.11.037
41. Hanajiri R, Sani GM, Hanley PJ, Silveira CG, Kallas EG, Keller MD, et al. Generation of zika virus-specific T cells from seropositive and virus-naive donors for potential use as an autologous or "off-the-shelf" immunotherapeutic. Cytotherapy (2019) 21:840–55. doi: 10.1016/j.jcyt.2019.06.008
42. Pinsky BA, Mix S, Rowe J, Ikemoto S, Baron EJ. Long-term shedding of influenza a virus in stool of immunocompromised child. Emerg Infect Dis (2010) 16:1165–7. doi: 10.3201/eid1607.091248
43. Kaiser L, Aubert JD, Pache JC, Deffernez C, Rochat T, Garbino J, et al. Chronic rhinoviral infection in lung transplant recipients. Am J Respir Crit Care Med (2006) 174:1392–9. doi: 10.1164/rccm.200604-489OC
44. Dunn G, Klapsa D, Wilton T, Stone L, Minor PD, Martin J. Twenty-eight years of poliovirus replication in an immunodeficient individual: Impact on the global polio eradication initiative. PloS Pathog (2015) 11:e1005114. doi: 10.1371/journal.ppat.1005114
45. Debbink K, Lindesmith LC, Ferris MT, Swanstrom J, Beltramello M, Corti D, et al. Within-host evolution results in antigenically distinct GII.4 noroviruses. J Virol (2014) 88:7244–55. doi: 10.1128/JVI.00203-14
46. Doerflinger SY, Weichert S, Koromyslova A, Chan M, Schwerk C, Adam R, et al. Human norovirus evolution in a chronically infected host. mSphere (2017) 2:e00352-16. doi: 10.1128/mSphere.00352-16
47. Quaranta EG, Fusaro A, Giussani E, D'Amico V, Varotto M, Pagliari M, et al. SARS-CoV-2 intra-host evolution during prolonged infection in an immunocompromised patient. Int J Infect Dis (2022) 122:444–8. doi: 10.1016/j.ijid.2022.06.023
48. Rogers MB, Song T, Sebra R, Greenbaum BD, Hamelin ME, Fitch A, et al. Intrahost dynamics of antiviral resistance in influenza a virus reflect complex patterns of segment linkage, reassortment, and natural selection. mBio (2015) 6. doi: 10.1128/mBio.02464-14
49. Fisher C, McLawhorn JM, Adotama P, Stasko T, Collins L, Levin J. Pulsed dye laser repurposed: Treatment of refractory molluscum contagiosum in renal transplant patient. Transpl Infect Dis (2019) 21:e13036. doi: 10.1111/tid.13036
50. Siddiqui N, Mansfield BS, Olmesdahl NP, Swart P, Nel J. A severe case of molluscum contagiosum immune reconstitution inflammatory syndrome in a patient with human immunodeficiency virus. Eur J Case Rep Intern Med (2022) 9:003115. doi: 10.12890/2022_003115
51. Lynch JP 3rd, Kajon AE. Adenovirus: Epidemiology, global spread of novel types, and approach to treatment. Semin Respir Crit Care Med (2021) 42:800–21. doi: 10.1055/s-0041-1733802
52. de Souza Pereira BB, Darrigo Junior LG, de Mello Costa TC, Felix AC, Simoes BP, Stracieri AB, et al. Prolonged viremia in dengue virus infection in hematopoietic stem cell transplant recipients and patients with hematological malignancies. Transpl Infect Dis (2017) 19. doi: 10.1111/tid.12721
53. Sharma SK, Seth T, Mishra P, Gupta N, Agrawal N, Broor S, et al. Clinical profile of dengue infection in patients with hematological diseases. Mediterr J Hematol Infect Dis (2011) 3:e2011039. doi: 10.4084/mjhid.2011.039
54. Ng KH, Zhang SL, Tan HC, Kwek SS, Sessions OM, Chan CY, et al. Persistent dengue infection in an immunosuppressed patient reveals the roles of humoral and cellular immune responses in virus clearance. Cell Host Microbe (2019) 26:601–605 e3. doi: 10.1016/j.chom.2019.10.005
55. Visuthranukul J, Bunworasate U, Lawasut P, Suankratay C. Dengue hemorrhagic fever in a peripheral blood stem cell transplant recipient: The first case report. Infect Dis Rep (2009) 1:e3. doi: 10.4081/idr.2009.1338
56. Jain H, Sengar M, Menon H, Dangi U, Biswas S, Chandrakanth MV. Dengue fever as a cause of febrile neutropenia in adult acute lymphoblastic leukemia: A single center experience. Hematol Oncol Stem Cell Ther (2014) 7:125–6. doi: 10.1016/j.hemonc.2014.04.002
57. Kleinschmidt-DeMasters BK, Beckham JD. West Nile Virus encephalitis 16 years later. Brain Pathol (2015) 25:625–33. doi: 10.1111/bpa.12280
58. Armah HB, Wang G, Omalu BI, Tesh RB, Gyure KA, Chute DJ, et al. Systemic distribution of West Nile virus infection: postmortem immunohistochemical study of six cases. Brain Pathol (2007) 17:354–62. doi: 10.1111/j.1750-3639.2007.00080.x
59. Batsis JA, Phy MP. West Nile Virus meningitis in a chronic immunosuppressed patient with rheumatoid arthritis. Clin Rheumatol (2005) 24:548–50. doi: 10.1007/s10067-005-1085-x
60. Choi B, Choudhary MC, Regan J, Sparks JA, Padera RF, Qiu X, et al. Persistence and evolution of SARS-CoV-2 in an immunocompromised host. N Engl J Med (2020) 383:2291–3. doi: 10.1056/NEJMc2031364
61. Dioverti V, Salto-Alejandre S, Haidar G. Immunocompromised patients with protracted COVID-19: a review of "Long persisters". Curr Transplant Rep (2022) 9:209–18. doi: 10.1007/s40472-022-00385-y
62. Abbasi J. Researchers tie severe immunosuppression to chronic COVID-19 and virus variants. JAMA (2021) 325:2033–5. doi: 10.1001/jama.2021.7212
63. Kemp SA, Collier DA, Datir RP, Ferreira I, Gayed S, Jahun A, et al. SARS-CoV-2 evolution during treatment of chronic infection. Nature (2021) 592:277–82. doi: 10.1038/s41586-021-03291-y
64. Ankcorn M, Said B, Morgan D, Elsharkawy AM, Maggs J, Ryder S, et al. Persistent hepatitis e virus infection across England and Wales 2009-2017: Demography, virology and outcomes. J Viral Hepat (2021) 28:420–30. doi: 10.1111/jvh.13424
65. Kaufman WS, Ahn CS, Huang WW. Molluscum contagiosum in immunocompromised patients: AIDS presenting as molluscum contagiosum in a patient with psoriasis on biologic therapy. Cutis (2018) 101:136–40.
66. Radke JR, Cook JL. Human adenovirus infections: update and consideration of mechanisms of viral persistence. Curr Opin Infect Dis (2018) 31:251–6. doi: 10.1097/QCO.0000000000000451
67. Vilchez RA, McCurry K, Dauber J, Lacono A, Griffith B, Fung J, et al. Influenza virus infection in adult solid organ transplant recipients. Am J Transplant (2002) 2:287–91. doi: 10.1034/j.1600-6143.2002.20315.x
68. Fukatsu Y, Nagata Y, Adachi M, Yagyu T, Ono T. Serum IgM levels independently predict immune response to influenza vaccine in long-term survivors vaccinated at >1 year after undergoing allogeneic hematopoietic stem cell transplantation. Int J Hematol (2017) 105:638–45. doi: 10.1007/s12185-016-2163-3
69. Mohty B, Bel M, Vukicevic M, Nagy M, Levrat E, Meier S, et al. Marrow transplant and H.N.s.g. Geneva university hospitals, graft-versus-host disease is the major determinant of humoral responses to the AS03-adjuvanted influenza A/09/H1N1 vaccine in allogeneic hematopoietic stem cell transplant recipients. Haematologica (2011) 96:896–904. doi: 10.3324/haematol.2011.040386
70. Mazzone PJ, Mossad SB, Mawhorter SD, Mehta AC, Schilz RJ, Maurer JR. The humoral immune response to influenza vaccination in lung transplant patients. Eur Respir J (2001) 18:971–6. doi: 10.1183/09031936.01.00215201
71. Karbasi-Afshar R, Izadi M, Fazel M, Khedmat H. Response of transplant recipients to influenza vaccination based on type of immunosuppression: A meta-analysis. Saudi J Kidney Dis Transpl (2015) 26:877–83. doi: 10.4103/1319-2442.164556
72. Piyaphanee W, Kittitrakul C, Lawpoolsri S, Gautret P, Kashino W, Tangkanakul W, et al. Risk of potentially rabid animal exposure among foreign travelers in southeast Asia. PloS Negl Trop Dis (2012) 6:e1852. doi: 10.1371/journal.pntd.0001852
73. World Health Organization. WHO expert consultation on rabies. second report. World Health Organ Tech Rep Ser (2013), 1–139.
74. Kopel E, Oren G, Sidi Y, David D. Inadequate antibody response to rabies vaccine in immunocompromised patient. Emerg Infect Dis (2012) 18:1493–5. doi: 10.3201/eid1809.111833
75. Parize P, Poujol P, Morineau Le Houssine P, Goesch J, Lucet C, Basuyau L, et al. Immune response to rabies post-exposure prophylaxis in patients with non-HIV secondary immunodeficiencies. Vaccine (2020) 38:5091–4. doi: 10.1016/j.vaccine.2020.06.037
76. Haas EJ, Angulo FJ, McLaughlin JM, Anis E, Singer SR, Khan F, et al. Impact and effectiveness of mRNA BNT162b2 vaccine against SARS-CoV-2 infections and COVID-19 cases, hospitalisations, and deaths following a nationwide vaccination campaign in Israel: an observational study using national surveillance data. Lancet (2021) 397:1819–29. doi: 10.1016/S0140-6736(21)00947-8
77. Lee A, Wong SY, Chai LYA, Lee SC, Lee MX, Muthiah MD, et al. Efficacy of covid-19 vaccines in immunocompromised patients: Systematic review and meta-analysis. BMJ (2022) 376:e068632. doi: 10.1136/bmj-2021-068632
78. Whitaker HJ, Tsang RSM, Byford R, Andrews NJ, Sherlock J, Sebastian Pillai P, et al. Pfizer-BioNTech and Oxford AstraZeneca COVID-19 vaccine effectiveness and immune response amongst individuals in clinical risk groups. J Infect (2022) 84:675–83. doi: 10.1016/j.jinf.2021.12.044
79. Plumb ID, Feldstein LR, Barkley E, Posner AB, Bregman HS, Hagen MB, et al. Effectiveness of COVID-19 mRNA vaccination in preventing COVID-19-Associated hospitalization among adults with previous SARS-CoV-2 infection - united states, June 2021-February 2022. MMWR Morb Mortal Wkly Rep (2022) 71:549–55. doi: 10.15585/mmwr.mm7115e2
80. Aiello A, Grossi A, Meschi S, Meledandri M, Vanini V, Petrone L, et al. Coordinated innate and T-cell immune responses in mild COVID-19 patients from household contacts of COVID-19 cases during the first pandemic wave. Front Immunol (2022) 13:920227. doi: 10.3389/fimmu.2022.920227
81. Maria Rescigno CA, Salvarani C, Mantovani DGMC, Massafra R, Zinzani PL, Morrone A, et al. Neutralizing antibodies to omicron after the fourth SARS-CoV-2 mRNA vaccine dose in immunocompromised patients highlight the need of additional boosters. Front Immunol (2023) 14:1104124. doi: 10.1101/2022.11.19.22282537
Keywords: immune deficiency, immunopathogenesis, children, adult, persistent infection
Citation: Agrati C, Bartolini B, Bordoni V, Locatelli F, Capobianchi MR, Di Caro A, Castilletti C and Ippolito G (2023) Emerging viral infections in immunocompromised patients: A great challenge to better define the role of immune response. Front. Immunol. 14:1147871. doi: 10.3389/fimmu.2023.1147871
Received: 19 January 2023; Accepted: 22 February 2023;
Published: 09 March 2023.
Edited by:
Iole Macchia, National Institute of Health (ISS), ItalyReviewed by:
Valentyn Oksenych, University of Oslo, NorwayMohammad Reza Aslani, Ardabil University of Medical Sciences, Iran
Copyright © 2023 Agrati, Bartolini, Bordoni, Locatelli, Capobianchi, Di Caro, Castilletti and Ippolito. This is an open-access article distributed under the terms of the Creative Commons Attribution License (CC BY). The use, distribution or reproduction in other forums is permitted, provided the original author(s) and the copyright owner(s) are credited and that the original publication in this journal is cited, in accordance with accepted academic practice. No use, distribution or reproduction is permitted which does not comply with these terms.
*Correspondence: Chiara Agrati, Y2hpYXJhLmFncmF0aUBvcGJnLm5ldA==
†Present address: Barbara Bartolini, National Institute for Infectious Diseases Istituto Nazionale Malattie Infettive (INMI) L Spallanzani – IRCCS, Rome, Italy
‡These authors share first authorship
§These authors share last authorship