- 1Department of Immunology, College of Basic Medical Science, Dalian Medical University, Dalian, Liaoning, China
- 2Department of Rheumatology and Immunology, Dalian Municipal Central Hospital, Dalian, Liaoning, China
- 3Department of Rheumatology and Immunology, The Affiliated Drum Tower Hospital of Nanjing University Medical School, Nanjing, Jiangsu, China
T follicular helper (Tfh) cells are heterogeneous and mainly characterized by expressing surface markers CXCR5, ICOS, and PD-1; cytokine IL-21; and transcription factor Bcl6. They are crucial for B-cell differentiation into long-lived plasma cells and high-affinity antibody production. T follicular regulatory (Tfr) cells were described to express markers of conventional T regulatory (Treg) cells and Tfh cells and were able to suppress Tfh-cell and B-cell responses. Evidence has revealed that the dysregulation of Tfh and Tfr cells is positively associated with the pathogenic processes of autoimmune diseases. Herein, we briefly introduce the phenotype, differentiation, and function of Tfh and Tfr cells, and review their potential roles in autoimmune diseases. In addition, we discuss perspectives to develop novel therapies targeting Tfh/Tfr balance.
Introduction
Autoimmune diseases refer to a category of diseases with high prevalence (7%–9%) in the general population, causing considerable mortality. Autoimmune diseases can be initiated by immune responses mistakenly targeting an individual’s cellular components, resulting in tissue damage and organ dysfunction. According to the tissues involved, they can be categorized as organ-specific diseases, including type 1 diabetes (T1D) and multiple sclerosis (MS), and multiple organs involving systemic diseases, including rheumatoid arthritis (RA), systemic lupus erythematosus (SLE), Sjögren’s syndrome (SS), and granulomatosis with polyangiitis (GPA) (1, 2). Many autoimmune diseases are characterized by autoantibody production. Autoantibodies promote disease pathogenesis by forming immune complexes, which mediate tissue inflammation and damage by activating complement and effector cells (3). Helper T cells play crucial roles in the pathogenesis of autoimmune diseases by secreting immune mediators and helping B cell-mediated long-lived humoral immunity development (4).
T follicular helper (Tfh) cells are a CD4+ T-cell subset that promotes germinal center (GC) formation, antibody affinity maturation, and memory B-cell generation (5). Recent studies have found a specialized subset of T regulatory (Treg) cells named T follicular regulatory (Tfr) cells, which can negatively regulate GC responses (6). Here, we review the established phenotype and function of Tfh and Tfr cells and their roles in the pathogenesis of autoimmune diseases, and highlight the potential therapies targeting these cells.
Phenotypes of Tfh and Tfr cells
Tfh cells are a heterogeneous subset of CD4+ T cells (Figure 1). Initial studies of experimental animal models revealed that Tfh cells mainly reside in GCs of secondary lymphoid organs. These canonical GC Tfh cells are characterized by expressing transcription factor B-cell lymphoma 6 (Bcl6), CXC-chemokine receptor 5 (CXCR5), inducible T-cell co-stimulator (ICOS), and programmed cell death protein-1 (PD-1) (7, 8). The clinical studies mainly focus on Tfh cells from the peripheral blood of patients. Circulating Tfh (cTfh) cells share phenotypic surface markers CXCR5, ICOS, and PD-1 with GC Tfh cells. Based on the expression of CXCR3 and CCR6, cTfh cells are further divided into four major subsets: CXCR3+CCR6− cTfh1, CXCR3−CCR6− cTfh2, CXCR3−CCR6+ cTfh17, and CXCR3+CCR6+ cTfh17.1 cells. These cTfh cell subsets share common precursors with their equivalent helper T cell (Th) 1, Th2, Th17 or Th1, and Th17 cells, respectively (9–11).
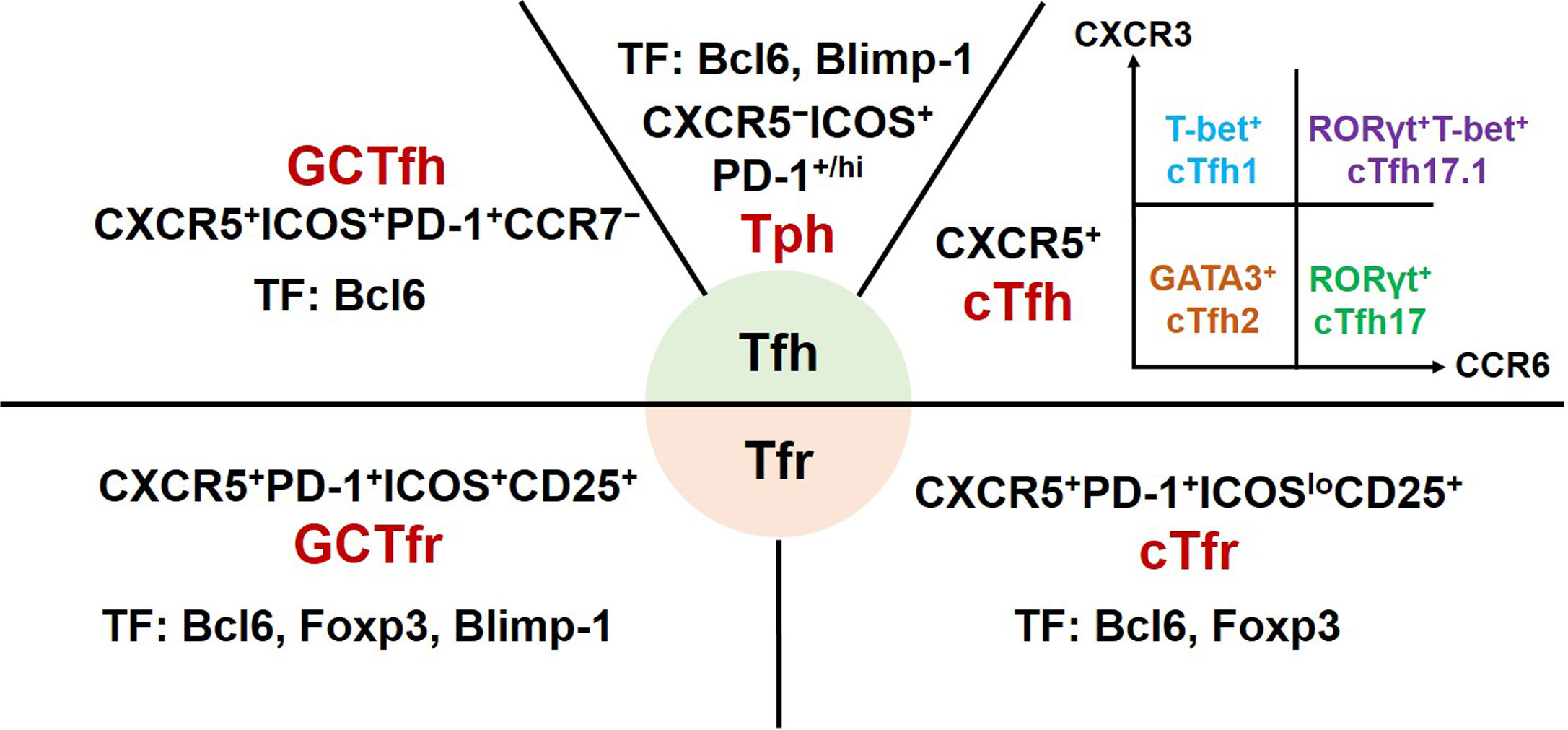
Figure 1 Phenotype and transcription factors of Tfh effector cell subsets and Tfr cells. GC, germinal centers; Tfh, T follicular helper; cTfh, circulating Tfh; Tph, T peripheral helper; Tfr, T follicular regulatory; cTfr, circulating Tfr; CXCR, chemokine receptor; ICOS, inducible T-cell co-stimulator; PD-1, programmed cell death protein-1; Bcl6, B cell lymphoma 6; Foxp3, forkhead box P3; GATA3, GATA-binding protein 3; ROR, retinoid-related orphan receptor; Blimp-1, B lymphocyte-induced maturation protein 1; TF, transcription factors.
Tfr cells, a subset of regulatory T cells, were described as sharing phenotypic markers with conventional Treg cells (CD4+CD25+Foxp3+) and Tfh cells (12, 13). Subsequent studies reported that circulating Tfr (cTfr) cells should be defined as CD4+CXCR5+Foxp3+ T cells and tissue-resident Tfr cells fully expressing CXCR5, ICOS, and PD-1 (14, 15). In addition, Tfr cells as a heterogeneous subset may express different phenotypic characteristics at different development stages in distinct inflammatory milieus (10).
Differentiation of Tfh and Tfr cells
Transcription factors
Bcl6 is the essential transcription factor for Tfh cell differentiation by controlling the expression of CXCR5, interleukin-6 receptor (IL-6R), IL-21, and IL-21R in naïve CD4+ T cells. Bcl-6 can also inhibit the effects of the transcription factors T-bet for Th1 cells, GATA3 for Th2 cells, and RORγt for Th17 cells by downregulating the expression of B lymphocyte-induced maturation protein 1 (Blimp-1) (4, 11, 16, 17). In particular, CXCR5+ cTfh cells, with effector memory phenotype, do not express Bcl6 owing to the lack of persistent antigen stimulation (18). Upon antigen re-encounter, these pre-Tfh cells can rapidly differentiate into mature GC Tfh cells (19, 20).
Unlike Tfh cells, Tfr cells can differentiate from thymic-derived natural Treg cells and peripheral Treg cells (12, 13, 21). These precursor cells differentiate into Tfr cells requiring transcription factor Bcl6. Similar to Tfh cell differentiation, ICOS signaling promotes the development of Tfr cells by upregulating Bcl6 expression (22). Compared with GC Tfr cells, cTfr cells expressed a similar level of CXCR5, but a lower level of ICOS (15). Blimp-1 is necessary for the Treg-like suppressive function and homing into GCs of Tfr cells (13, 23).
Cytokines
Besides antigen stimulation to activate T-cell receptor (TCR) signaling and costimulatory signals via CD28 and ICOS, the expression and activity of Bcl6 are regulated by several specific cytokine-initiated cell-intrinsic signaling cascades (Figure 2). The IL-2–STAT5 pathway inhibits GC Tfh cell formation by inducing Blimp-1 to suppress Bcl6 expression (24). The IFN-α/β-STAT1 pathway contributes to CXCR5 and PD-1 expression in naive mice CD4+ T cells by inducing Bcl6 (25). Co-stimulation of IFN-α and IL-2 can convert CXCR5+PD-1+ cTfh cells to CXCR5−PD-1+ T peripheral helper (Tph) cells through promoting the binding of STAT5 to the Bcl6 locus at the expense of STAT1 (26). IL-12 is the most efficient cytokine inducing human naive CD4+ T cells to express IL-21. The IL-21–STAT3 pathway can promote GC Tfh cell differentiation by inducing Bcl6 expression and antagonizing IL-2 signals (27, 28). IL-23, as the substitute for IL-12, can also induce IL-21 expression and human cTfh cell differentiation in vitro (29). The IL-6 is a potent inducer for IL-21 expression and GC Tfh cell differentiation of naive murine CD4+ T cells by inducing STAT3 phosphorylation (27, 30). Of special interest is that IL-29 may suppress cTfh differentiation through decreasing STAT3 activation-induced Bcl6 expression (31). In addition, TGF-β and TGF-β superfamily member Activin A seems to be important for human, but not murine, Tfh cell differentiation (29, 32).
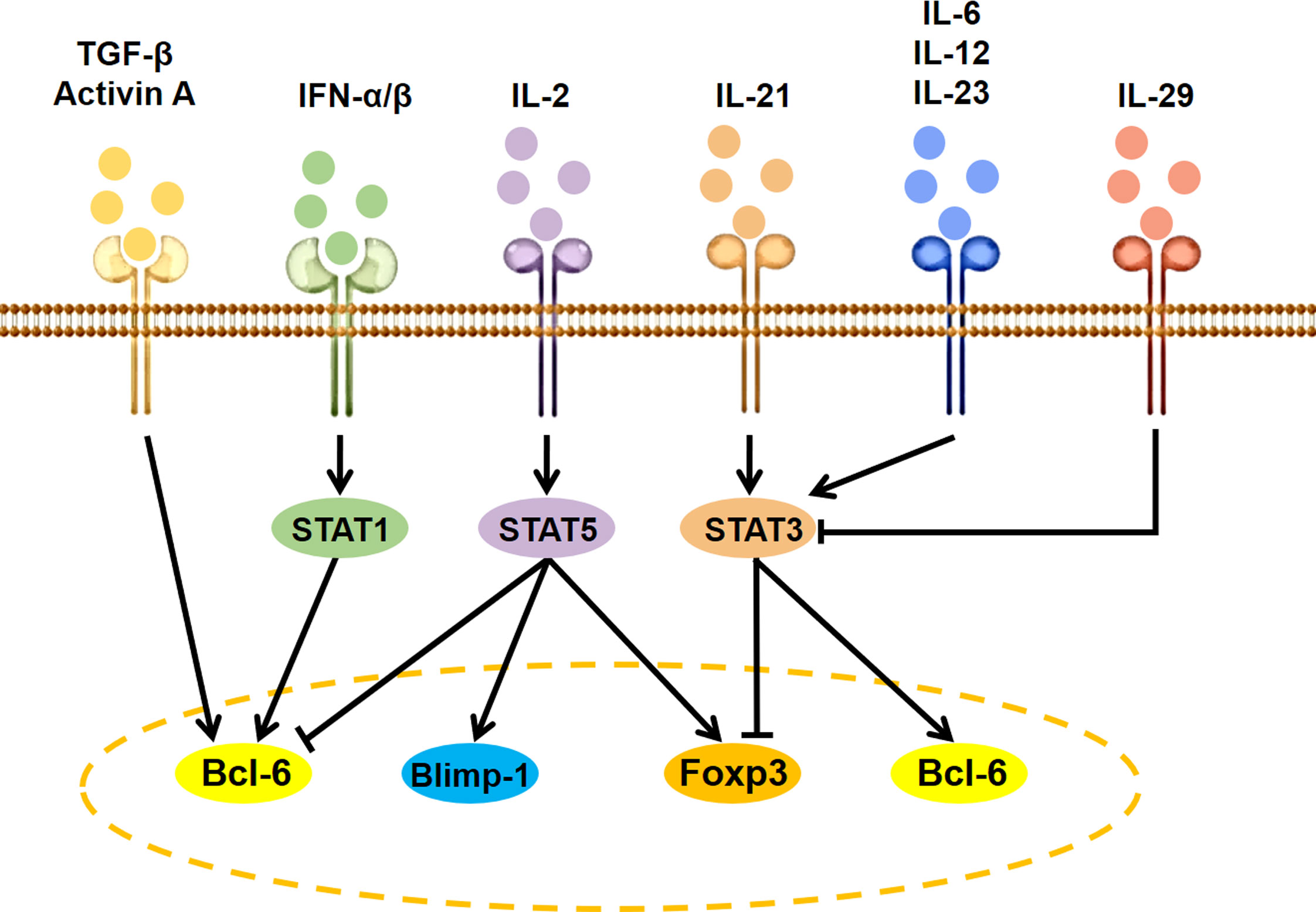
Figure 2 Signaling modulation of Tfh and Tfr cell differentiation. IL, interleukin; TGF, transforming growth factor; IFN, interferon; STAT, signal transducers and activators of transcription; Bcl6, B cell lymphoma 6; Foxp3, forkhead box P3; Blimp-1, B lymphocyte induced maturation protein 1.
IL-2, as a positive factor for Treg cell differentiation and a negative factor for Tfh cell differentiation (33), can facilitate cTfr cell development by upregulating Foxp3 and Bcl6 expression (34, 35). IL-21 and IL-6 as the positive factor for Tfh cell differentiation can inhibit Tfr cell development by suppressing Foxp3, TGF-β, or CD25 expression via activating the STAT3 signaling pathway (36–38), while STAT3 deficiency decreased both Tfr and Tfh cell differentiation (39).
Functions of Tfh and Tfr cells
Tfh cells are a subset of effector T cells that can assist B-cell maturation, high-affinity antibody production, and memory B-cell development in GCs (Table 1). CXCR5, a receptor for chemokine ligand CXCL13, can guide Tfh cells into GCs and interact with B cells. It has been shown that a high level of CXCR5 combined with a low level of CCR7 is required for T cells to migrate to the T–B border (10). Within GCs, Tfh cells interact with B cells relying on ICOS-ICOSL, CD40L-CD40, and TCR-peptide-MHC II, which induces IL-4, IL-21, and Bcl-6 expression in Tfh cells and promotes B-cell activation (4, 40, 41). Furthermore, IL-21 binding to IL-21R on Tfh and B cells can facilitate their proliferation and differentiation to maintain GC responses (42, 43), while PD-1 signals can restrain GC Tfh cell proliferation by inhibiting ICOS signaling, avoiding excessive B-cell proliferation and antibody production (53).
Upon re-encountering antigen, extrafollicular cTfh cells rapidly differentiate into mature GC Tfh cells and are guided by CXCR5-CXCL13 to GCs in secondary lymphoid organs (19, 20). cTfh1 cells express high levels of IFN-γ; cTfh2 cells express high levels of IL-4, IL-5, and IL-13; and cTfh17 cells express high levels of IL-17 and IL-22. All these cytokines help class-switching of GC B cells (10, 44). Studies reported that distinct cTfh cell subsets, except cTfh1 cells, are prone to induce naive B cells to differentiate into plasma cells secreting different classes of immunoglobulins (8, 45).
CXCR5 can also guide Tfr cells to migrate to GCs (6). GC Tfr cells show durable and persistent suppression of high-affinity autoantibody production by inhibiting glucose metabolism in B cells (49). GC Tfr cells may limit GC reactions by physically interrupting Tfh–B cell recognition via cytotoxic T-lymphocyte antigen 4 (CTLA-4) competitive binding with the co-stimulatory molecules on GC B cells (49, 50). Moreover, similar to Treg cells, Tfr cells control Tfh- and B cell-mediated immune responses by secreting IL-10, TGF-β, and granzyme B (51). However, compared to GC Tfr cells, the cTfr cells showed less suppressive function on B-cell responses (52).
Involvement of Tfh and Tfr cells in autoimmune diseases
The interactions between B and Tfh cells are crucial for autoantibody production, which is the hallmark of autoimmune diseases. Considerable evidence has revealed that the imbalance of Tfh and Tfr cells is involved in the development of autoimmune pathology (Table 2).
Rheumatoid arthritis
RA is a common systemic autoimmune disease mainly characterized by chronic inflammation affecting the joints and other organs (99). Studies indicated that CXCR5+ Tfh cells were present in the B-cell area of lymphoid tissue from early RA patients, and both CXCR5+ and CXCR5+PD-1+ cTfh cell proportions were higher in untreated early RA patients than in healthy controls (HC) (54–56). The increased circulating plasmablasts in RA patients promoted CXCR5+ICOS+ cTfh cell differentiation via IL-6 production (100). Furthermore, in patients with low or high active RA, the frequencies of cTfh1 cells were comparable with those in HC. However, the frequencies of cTfh2 and cTfh17 cells were higher than those in HC. Patients with high active RA had more cTfh2 and cTfh17 cells than patients with low active RA (57). OX40 expressed cTfh, especially cTfh17 cells, were increased, and negatively correlated with autoantibody sialylation in RA patients (101).
A novel CXCR5−PD-1hi Tfh cell population in the synovial tissues and peripheral blood of seropositive RA patients was defined as Tph cells. Tph cells were increased only in patients with high active RA and might contribute to chronic autoimmune phenomena at the inflammatory foci (48, 58). Adiponectin (AD) promoted fibroblast-like synoviocytes producing IL-6 to enhance CXCR5+PD-1+ cTfh cell responses in RA patients. Intra-articular injection of AD aggravated synovial inflammation with increased Tfh cells in the joint tissue of collagen-induced arthritis (CIA) mice (100).
Moreover, compared with HC, RA patients showed decreased CXCR5+CD25+ CD127lo or CXCR5+Foxp3+ cTfr cells and Tfr/Tfh ratio. The ratio of Tfr/Tfh was negatively correlated with C-reactive protein (CRP), erythrocyte sedimentation rate (ESR), serum anti-cyclic citrullinated peptide antibodies (ACPA), and disease activity score-28 (DAS28) index of RA patients (59–61). Studies on targeting Tfh and Tfr cells revealed that CTLA-4-Ig, iguratimod, abatacept, low-dose IL-2, and alcohol consumption could ameliorate RA by inhibiting Tfh cell responses and restoring the Tfr/Tfh balance (102–107).
Systemic lupus erythematosus
SLE is a prototypic autoimmune disease with aberrant activation of T and B cells. Multiple serum autoantibodies against nuclear antigens lead to systemic tissue damage (108). According to studies, the increased cTfh cells and serum IL-21 were associated with the pathogenesis of SLE patients (109). Furthermore, in SLE patients, the percentages of cTfh1 and cTfh2 cells were comparable with those in HC, and the percentages of cTfh17 cells were higher than those in HC (110, 111). A population of Tfh cells with active NLRP3 inflammasome was increased and essential for optimal humoral responses and GC formation in SLE patients and mice (62). Circulating CXCR5−PD-1+/hi Tph cells were increased significantly, which stimulated B-cell responses via secreting IL-21 in SLE patients (26, 63, 64). CXCX5−PD-1hi CXCR3+ Tfh-like cells expanded in blood and the tubulointerstitial areas of SLE patients, providing B-cell help, independently of IL-21, by producing IL-10 and succinate (65). IL-10-producing CCR6+IL7R+ Tfh-like cells lacking Bcl6 expression were elevated in peripheral blood and lymph nodes of SLE patients, and these cells were associated with the presence of pathogenic anti-dsDNA antibodies in SLE patients and promoted B-cell IgG production ex vivo (66).
Chronic type I IFN production plays a pathogenic role in SLE patients (112). Studies found that type I IFN signals inhibited Tfh cell expansion, but induced Tph cell generation and IL-21 and IFNγ production in Tfh cells by activating STAT4 in lupus mice (113, 114). Circulating immunogenic self-DNA in SLE patients could induce IL-17+ Tfh cell expansion via RORγt supporting IgG anti-dsDNA responses (115). OX40L (a TNF superfamily ligand) on myeloid antigen-presenting cells induced human naive and memory CD4+ T cells to express Tfh-associated molecules including CXCR5, CD40L, and IL-21 (116).
Xu et al. found that CXCR5+CD25+CD127lo cTfr cells and the ratio of Tfr/Tfh were decreased significantly in SLE patients. Both cTfr cell frequencies and Tfr/Tfh ratio were negatively correlated with serum IL-21, anti-dsDNA antibody levels, and disease activity of SLE patients (69). However, another study reported that CXCR5+Foxp3+ cTfr cells and the ratio of Tfr/Tfh were increased in SLE patients. Although the suppressive capacity of cTfr cells was not altered, the cTfr cell frequencies were positively correlated with auto-antibodies and disease activity scores of SLE patients (67). Kurata et al. found that the frequencies of CXCR5+Foxp3+ cTfr cells were similar in HC and SLE patients, while the expression of PD-1 on cTfr cells was increased and positively correlated with anti-DNA antibody levels and disease activity scores of SLE patients. These cTfr cells had impaired suppressive function with decreased expression of Foxp3, CTLA4, and IL-2 receptors (68).
Clinical studies showed that methylprednisolone pulse treatment decreased the percentages and absolute number of cTfh cells in SLE patients (117). Dexamethasone treatment reduced the frequencies of cTfh2 cells but increased the percentages of cTfh17 cells in SLE patients (111). Ex vivo, IL-2 stimulation downregulated the expression of PD-1 along with the increased expression of Foxp3 and CTLA-4 on cTfr cells (68), and converted memory Tfh cells to cTfr cells by promoting STAT3 and STAT5 phosphorylation in SLE patients (34). In vivo, sustained low-dose IL-2 therapy reduced cTfh cells significantly but had little effect on cTfr cells, which resulted in recovered Tfr/Tfh ratio in lupus mice and patients (118, 119). IL-2 therapy might inhibit GC Tfh early development from primed CD4+ T cells by inhibiting Bcl6 expression (120).
Mesenchymal stem cells (MSCs) ameliorated lupus symptoms in B6.lpr mice by producing iNOS to decrease CXCR5+PD-1hi Tfh cell expansion (121). Baicalin and TLR7 agonist imiquimod treatment could relieve lupus mice by inhibiting Tfh cell differentiation and IL-21 production, and promoting Tfr cell differentiation (122, 123). Cotreatment of soluble OX40L and Jagged-1 (a Notch family ligand) alleviated lupus nephritis via increasing Tfr/Tfh ratio, leading to decreased GC B cells and anti-dsDNA antibody levels in NZBWF1/j mice (124). Research revealed that ATP-gated ionotropic P2X7 receptor stimulation limited the expansion of pathogenic Tfh cells by promoting caspase-mediated pyroptosis in a lupus mouse model. Restoring P2X7 activity may limit the progressive amplification of pathogenic autoantibodies in SLE patients (125).
Sjögren’s syndrome
SS is a heterogeneous systemic autoimmune disease mainly characterized by exocrine gland dysfunction. Anti-Ro/SSA and anti-La/SSB antibodies are important diagnostic indicators of SS (126). CXCR5+ICOS+PD-1+ cTfh cells were significantly increased in SS patients, especially in anti-Ro/SSA antibody-positive patients (70, 71). Compared with HC, SS patients with a high degree of focal lymphocytic sialadenitis had more CCR7loPD-1hi cTfh cells, which were positively correlated with disease activity scores and plasma cell percentages of SS patients (72). IL-21+ or ICOS+ cTfh cells were positively correlated with transitional B cells, plasmablasts, and plasma cells in SS patients (70, 79). Although cTfh1, cTfh2, and cTfh17 cells were comparable in the peripheral blood of HC and SS patients, they were increased in minor salivary glands (SGs) of SS patients (71, 73). CXCR5+PD1+ICOS+Foxp3− Tfh cells and CXCR5−PD1hiICOS+Foxp3− Tph cells were enriched in both SS peripheral blood and salivary gland with GCs (75, 76). Foxp3+CXCR5+ cTfr cells were increased in SS patients, especially in autoantibody-positive SS patients (71, 77), while another study reported that the percentages of CXCR5+Foxp3+CD25+ cTfr cells and the ratio of Tfr/Tfh were decreased in SS patients (78).
The elevated enhancer of zeste homolog 2 (EZH2, an epigenetic regulator) in CD4+ T cells facilitated CXCR5+PD-1+ cTfh cell differentiation by enhancing STAT3 phosphorylation in SS patients (127). Elevated CCL25 expression in labial salivary glands could facilitate the attraction of circulating CCR9+ Th cells, which expressed high levels of PD-1 and ICOS in pSS patients. The CCR9+ Th cells promoted IgG production and displayed higher levels of IFN-γ, IL-17, IL-4, and IL-21 than CXCR5+ Th cells with antigen or IL-7 stimulation (74). Blocking ICOS reduced the levels of IL-21, IL-6, IL-8, and tumor necrosis factor-α (TNF-α) in SG–organ cultures, which indicated that T-cell costimulatory pathways were crucial for proinflammatory cytokine production of Tfh cells (76).
MSCs inhibited naive CD4+ T cells of SS patients to differentiate into cTfh cells via secreting indoleamine 2,3-dioxygenase (IDO) with high enzymic activity, which could be partly reversed by the IDO inhibitor 1-MT (128). Rituximab (RTX, B cell-depleting anti-CD20 monoclonal antibodies) therapy reduced cTfh cells in SS patients (129). Catalpol, sirolimus, and abatacept (CTLA-4-Ig fusion protein) therapy could reduce cTfh cells and upregulate cTfr cells and restore Tfh/Tfr ratio, which led to attenuate Tfh cell-dependent B-cell hyperactivity of SS (78, 130, 131).
Granulomatosis with polyangiitis
GPA is a rare and severe systemic autoimmune disease with the classic hallmark of antineutrophilic cytoplasmic autoantibody (ANCA) specific for PR3 affecting systemic small vessels (132). Studies reported that TCR-activated naive CD4+ T cells from GPA patients expressed high levels of Bcl6, which was associated with decreased IL-2R/STAT5 signaling (133). CXCR5+PD-1+ cTfh cells (80) and CD4+IL-21+ IL-17A+ T cells were increased significantly in GPA patients, and ANCA-positive GPA patients had more CD4+IL-21+ and CD4+BCL6+ T cells in peripheral blood than HC and ANCA-negative GPA patients (81). RTX treatment decreased disease activity scores and cTfh cell percentages of active GPA patients (80).
Multiple sclerosis
MS is a T cell-dominant chronic neuro-inflammatory disorder characterized by demyelination and axonal damage. Autoreactive CD4+ T cells from peripheral lymphoid organs or CD4+ T cells activated by central nervous system (CNS) local antigen play a crucial role in the pathogenesis of MS and its animal model, experimental autoimmune encephalomyelitis (EAE) (134). Genome-wide association studies (GWAS) showed that polymorphisms in the Tfh signature genes IL-21 (135), CXCR5 (136), and PD-1 (137) are either diagnostic or prognostic risk factors for MS.
Intrathecal inflammatory environment promoted the recruitment of cTfh cells (89), especially cTfh1 and CXCR3+CCR6+ cTfh17.1 cells into CNS (46), and CD4+IL-21+ T cells were found in the lesions of MS patients (82). The frequencies of CCR7+ICOS+ circulating memory Tfh cells, cTfh17 cells, and cTfh17.1 cells were increased and cTfh1 cells (83) and cTfh2 cells (84) were decreased significantly in MS patients (85–87). CXCR5+PD-1+ Tfh cells were also increased in the cerebrospinal fluid (CSF) of MS patients and EAE mice (88). Adoptive transfer of myelin antigen-activated splenic CXCR5+ Tfh cells exacerbated MS-like autoimmunity of EAE mice (138).
The frequencies of CXCR5+CD25+PD-1+Foxp3+/CD127− or CXCR5+CD25hi/+ CD127dim/− Tfr cells were decreased significantly and exhibited reduced suppressive capacity in blood and CSF of MS patients (85–87, 89). Blimp-1 deficiency impaired the suppressive activity and promoted the expression of pro-inflammatory cytokine IL17A in Tfr cells and their homing into the GC, which led to severe CNS autoimmunity in EAE mice (23, 139). The Tfr/Tfh ratio was decreased and negatively correlated with IgG production in serum and CSF of MS patients (87, 90).
Clinical studies showed that laquinimod treatment inhibited the expansion of PD-1+CXCR5+BCL6+ Tfh and IL-21-producing activated CD4+CD44+ T cells in the lymph nodes of EAE mice (140). Methylprednisolone pulse, abatacept, and RTX decreased cTfh cells and serum IL-21 in MS patients (141–143). Dimethyl fumarate treatment decreased the frequencies of cTfh1, cTfh17, and cTfh17.1 cells and increased cTfh2 cells in MS patients (84, 144). Fingolimod (sphingosine 1-phosphate receptor agonist) reduced frequencies of cTfh17, cTfh17.1, and CXCR5+CD25hi cTfr cells, but increased cTfh1 cells in MS patients (145).
Type 1 diabetes
T1D is a T cell-mediated organ-specific autoimmune disease. The pancreatic infiltrated islet-autoreactive T cells elicit hyperglycemia by destroying insulin-producing β cells (146). Unlike systemic autoimmune disease, Tfh cells are programmed differently in T1D. Although T–B cell interactions are essential to driving high-affinity islet autoantibody production predicting T1D development, the β-cell destruction can arise independently of autoantibody (91, 96, 147). Both Tfh and Tph cells were increased and associated with T1D progression in human and mouse models by producing IL-21 and recruiting and activating B cells in the pancreas (91–95). Furthermore, pathogenic Tfh1 cells were observed in the pancreas and promoted T1D development in nonobese diabetic (NOD) mice (96). Tfr cells were decreased and had attenuated suppressive ability in the peripheral blood, spleen, and pancreatic lymph nodes of T1D patients. The adoptive transfer of Tfr cells prevented T1D development in NOD mice (97, 98). RTX administration decreased the percentages of cTfh and CXCR5+PD-1+ cTfr cells but increased CXCR5+ICOS+ cTfr cells in T1D patients (148). Thus, Tfh cell analysis may be a biomarker and stratification tool to predict diabetes progression and clinical response for therapies in T1D patients (149, 150).
Conclusions
Despite the fact that Tfh cells have been detected in many studies, their phenotypic surface markers vary in different studies. The expression of surface molecules and cytokines in Tfh cells changes over time to help B-cell responses more efficiently (10). In summary, Tfh cells are increased in multiple autoimmune diseases and promote the development of systemic autoimmune diseases by assisting B cell-mediated long-lasting humoral immunity (8). Islet autoantibodies are not thought to be the pathogenic effector molecules for T1D processes, but B cells, as antigen-presenting cells, can present islet autoantigen to active Tfh cells, which are necessary to cause β-cell destruction (96). Thus, the number of Tfh cells can be a novel predicting biomarker for clinical diagnosis and treatment of autoimmune diseases.
Tfr cells show different responses to distinct antigens or diverse disease contexts. The changes in Tfr cell percentages are not consistent in different studies on autoimmunity (10). Based on the discoveries to date, Tfr cells may also be induced and expanded by self-antigens, but their suppressive capacity is impaired in autoimmune diseases. The broken balance between Tfr and Tfh cells is responsible for the aggravated autoimmune responses. Targeting Tfr/Tfh balance may be a promising therapy for autoimmune diseases.
Theoretically, as a key transcription factor governing Tfh/Tfr differentiation, BCL6 degraders may be a potential therapeutic option by targeting Tfh cells in the treatment of autoimmune diseases. BCL6 is also an oncogenic driver for B-cell lymphoma and follicular lymphoma (151, 152). BCL6 targeting degraders have been well studied for lymphoma therapy (153, 154). Mechanistically, BCL6 contributes to lymphomagenesis by promoting the survival and proliferation of GC B cells and preventing premature terminal differentiation into memory or plasma cells, which can be beneficial for autoimmune diseases (151, 155). Hence, BCL6 degrader treatment may be a double-edged sword in autoimmune diseases and needs further basic and clinical research.
According to present clinical studies, methylprednisolone pulse, fingolimod, RTX, and other medication and biologics treatment decreased the expansion of pathogenic cTfh and/or increased cTfr cells in patients with autoimmune diseases (84, 117, 129, 141–145). However, some clinical studies showed unexpected results that the number of cTfh cells was not altered in RA patients treated with anti-TNFα agents (156) and the percentages of cTfh17 cells were increased in SLE patients treated with dexamethasone (111). Thus, further studies are required to better understand the delicate role of Tfh cell subsets in stratifying patients, which may help design personalized treatment schemes for individuals with autoimmune diseases.
Author contributions
GY and JQ conceived and designed the project. JQ, CL, and ZB drafted and wrote the manuscript. JQ, GY, and XL supervised the project and revised the manuscript. All authors contributed to the article and approved the submitted version.
Funding
This work was supported by the National Natural Science Foundation of China (NSFC) (grant no. 81970062 to GY, grant no. 82201990 to JQ), the Science and Technology Talent Innovation Support Project of Dalian (grant no. 2022RQ070 to JQ), and the Dalian Key Laboratory of Human Micro-organism Homeostasis and Immunological Mechanism Research of Diseases.
Conflict of interest
The authors declare that the research was conducted in the absence of any commercial or financial relationships that could be construed as a potential conflict of interest.
Publisher’s note
All claims expressed in this article are solely those of the authors and do not necessarily represent those of their affiliated organizations, or those of the publisher, the editors and the reviewers. Any product that may be evaluated in this article, or claim that may be made by its manufacturer, is not guaranteed or endorsed by the publisher.
References
1. Richard-Eaglin A, Smallheer BA. Immunosuppressive/Autoimmune disorders. Nurs Clin North Am (2018) 53(3):319–34. doi: 10.1016/j.cnur.2018.04.002
2. Theofilopoulos AN, Kono DH, Baccala R. The multiple pathways to autoimmunity. Nat Immunol (2017) 18(7):716–24. doi: 10.1038/ni.3731
3. Xiao ZX, Miller JS, Zheng SG. An updated advance of autoantibodies in autoimmune diseases. Autoimmun Rev (2021) 20(2):102743. doi: 10.1016/j.autrev.2020.102743
4. Crotty S. T Follicular helper cell biology: a decade of discovery and diseases. Immunity (2019) 50(5):1132–48. doi: 10.1016/j.immuni.2019.04.011
5. Tangye SG, Ma CS, Brink R, Deenick EK. The good, the bad and the ugly - TFH cells in human health and disease. Nat Rev Immunol (2013) 13(6):412–26. doi: 10.1038/nri3447
6. Gong Y, Tong J, Wang S. Are follicular regulatory T cells involved in autoimmune diseases? Front Immunol (2017) 8:1790. doi: 10.3389/fimmu.2017.01790
7. Scherm MG, Ott VB, Daniel C. Follicular helper T cells in autoimmunity. Curr Diabetes Rep (2016) 16(8):75. doi: 10.1007/s11892-016-0770-2
8. Wei X, Niu X. T Follicular helper cells in autoimmune diseases. J Autoimmun (2022) 134:102976. doi: 10.1016/j.jaut.2022.102976
9. Schmitt N, Bentebibel SE, Ueno H. Phenotype and functions of memory tfh cells in human blood. Trends Immunol (2014) 35(9):436–42. doi: 10.1016/j.it.2014.06.002
10. Deng J, Wei Y, Fonseca VR, Graca L, Yu D. T Follicular helper cells and T follicular regulatory cells in rheumatic diseases. Nat Rev Rheumatol (2019) 15(8):475–90. doi: 10.1038/s41584-019-0254-2
11. Gensous N, Charrier M, Duluc D, Contin-Bordes C, Truchetet ME, Lazaro E, et al. T Follicular helper cells in autoimmune disorders. Front Immunol (2018) 9:1637. doi: 10.3389/fimmu.2018.01637
12. Chung Y, Tanaka S, Chu F, Nurieva RI, Martinez GJ, Rawal S, et al. Follicular regulatory T cells expressing Foxp3 and bcl-6 suppress germinal center reactions. Nat Med (2011) 17(8):983–8. doi: 10.1038/nm.2426
13. Linterman MA, Pierson W, Lee SK, Kallies A, Kawamoto S, Rayner TF, et al. Foxp3+ follicular regulatory T cells control the germinal center response. Nat Med (2011) 17(8):975–82. doi: 10.1038/nm.2425
14. Maceiras AR, Fonseca VR, Agua-Doce A, Graca L. T Follicular regulatory cells in mice and men. Immunology (2017) 152(1):25–35. doi: 10.1111/imm.12774
15. Sage PT, Alvarez D, Godec J, von Andrian UH, Sharpe AH. Circulating T follicular regulatory and helper cells have memory-like properties. J Clin Invest (2014) 124(12):5191–204. doi: 10.1172/JCI76861
16. Ueno H. T Follicular helper cells in human autoimmunity. Curr Opin Immunol (2016) 43:24–31. doi: 10.1016/j.coi.2016.08.003
17. Nurieva RI, Chung Y, Martinez GJ, Yang XO, Tanaka S, Matskevitch TD, et al. Bcl6 mediates the development of T follicular helper cells. Science (2009) 325(5943):1001–5. doi: 10.1126/science.1176676
18. Baumjohann D, Preite S, Reboldi A, Ronchi F, Ansel KM, Lanzavecchia A, et al. Persistent antigen and germinal center b cells sustain T follicular helper cell responses and phenotype. Immunity (2013) 38(3):596–605. doi: 10.1016/j.immuni.2012.11.020
19. He J, Tsai LM, Leong YA, Hu X, Ma CS, Chevalier N, et al. Circulating precursor CCR7(lo)PD-1(hi) CXCR5(+) CD4(+) T cells indicate tfh cell activity and promote antibody responses upon antigen reexposure. Immunity (2013) 39(4):770–81. doi: 10.1016/j.immuni.2013.09.007
20. Sallusto F, Geginat J, Lanzavecchia A. Central memory and effector memory T cell subsets: function, generation, and maintenance. Annu Rev Immunol (2004) 22:745–63. doi: 10.1146/annurev.immunol.22.012703.104702
21. Aloulou M, Carr EJ, Gador M, Bignon A, Liblau RS, Fazilleau N, et al. Follicular regulatory T cells can be specific for the immunizing antigen and derive from naive T cells. Nat Commun (2016) 7:10579. doi: 10.1038/ncomms10579
22. Sage PT, Francisco LM, Carman CV, Sharpe AH. The receptor PD-1 controls follicular regulatory T cells in the lymph nodes and blood. Nat Immunol (2013) 14(2):152–61. doi: 10.1038/ni.2496
23. Shen E, Rabe H, Luo L, Wang L, Wang Q, Yin J, et al. Control of germinal center localization and lineage stability of follicular regulatory T cells by the Blimp1 transcription factor. Cell Rep (2019) 29(7):1848–61.e6. doi: 10.1016/j.celrep.2019.10.012
24. Johnston RJ, Choi YS, Diamond JA, Yang JA, Crotty S. STAT5 is a potent negative regulator of TFH cell differentiation. J Exp Med (2012) 209(2):243–50. doi: 10.1084/jem.20111174
25. Nakayamada S, Poholek AC, Lu KT, Takahashi H, Kato M, Iwata S, et al. Type I IFN induces binding of STAT1 to Bcl6: divergent roles of STAT family transcription factors in the T follicular helper cell genetic program. J Immunol (2014) 192(5):2156–66. doi: 10.4049/jimmunol.1300675
26. Jiang Q, Wang J, Jiang H, Li W, Sun Y, Shan Y, et al. Competitive binding of transcription factors underlies flexibility of T peripheral helper cells and T follicular helper cells in SLE. Rheumatol (Oxford) (2022) 61(11):4547–57. doi: 10.1093/rheumatology/keac112
27. Nurieva RI, Chung Y, Hwang D, Yang XO, Kang HS, Ma L, et al. Generation of T follicular helper cells is mediated by interleukin-21 but independent of T helper 1, 2, or 17 cell lineages. Immunity (2008) 29(1):138–49. doi: 10.1016/j.immuni.2008.05.009
28. Read KA, Powell MD, Baker CE, Sreekumar BK, Ringel-Scaia VM, Bachus H, et al. Integrated STAT3 and ikaros zinc finger transcription factor activities regulate bcl-6 expression in CD4(+) Th cells. J Immunol (2017) 199(7):2377–87. doi: 10.4049/jimmunol.1700106
29. Schmitt N, Liu Y, Bentebibel SE, Ueno H. Molecular mechanisms regulating T helper 1 versus T follicular helper cell differentiation in humans. Cell Rep (2016) 16(4):1082–95. doi: 10.1016/j.celrep.2016.06.063
30. Crotty S. T Follicular helper cell differentiation, function, and roles in disease. Immunity (2014) 41(4):529–42. doi: 10.1016/j.immuni.2014.10.004
31. Xu T, Yan T, Li P. Interleukin-29 regulates T follicular helper cells by repressing BCL6 in rheumatoid arthritis patients. Clin Rheumatol (2020) 39(12):3797–804. doi: 10.1007/s10067-020-05151-y
32. Locci M, Wu JE, Arumemi F, Mikulski Z, Dahlberg C, Miller AT, et al. Activin a programs the differentiation of human TFH cells. Nat Immunol (2016) 17(8):976–84. doi: 10.1038/ni.3494
33. Papillion A, Powell MD, Chisolm DA, Bachus H, Fuller MJ, Weinmann AS, et al. Inhibition of IL-2 responsiveness by IL-6 is required for the generation of GC-T(FH) cells. Sci Immunol (2019) 4(39):eaaw7636. doi: 10.1126/sciimmunol.aaw7636
34. Hao H, Nakayamada S, Yamagata K, Ohkubo N, Iwata S, Inoue Y, et al. Conversion of T follicular helper cells to T follicular regulatory cells by interleukin-2 through transcriptional regulation in systemic lupus erythematosus. Arthritis Rheumatol (2021) 73(1):132–42. doi: 10.1002/art.41457
35. Botta D, Fuller MJ, Marquez-Lago TT, Bachus H, Bradley JE, Weinmann AS, et al. Dynamic regulation of T follicular regulatory cell responses by interleukin 2 during influenza infection. Nat Immunol (2017) 18(11):1249–60. doi: 10.1038/ni.3837
36. Jandl C, Liu SM, Canete PF, Warren J, Hughes WE, Vogelzang A, et al. IL-21 restricts T follicular regulatory T cell proliferation through bcl-6 mediated inhibition of responsiveness to IL-2. Nat Commun (2017) 8:14647. doi: 10.1038/ncomms14647
37. Leon B, Bradley JE, Lund FE, Randall TD, Ballesteros-Tato A. FoxP3+ regulatory T cells promote influenza-specific tfh responses by controlling IL-2 availability. Nat Commun (2014) 5:3495. doi: 10.1038/ncomms4495
38. Ding Y, Li J, Yang P, Luo B, Wu Q, Zajac AJ, et al. Interleukin-21 promotes germinal center reaction by skewing the follicular regulatory T cell to follicular helper T cell balance in autoimmune BXD2 mice. Arthritis Rheumatol (2014) 66(9):2601–12. doi: 10.1002/art.38735
39. Wu H, Xie MM, Liu H, Dent AL. Stat3 is important for follicular regulatory T cell differentiation. PloS One (2016) 11(5):e0155040. doi: 10.1371/journal.pone.0155040
40. Wan S, Ni L, Zhao X, Liu X, Xu W, Jin W, et al. Costimulation molecules differentially regulate the ERK-Zfp831 axis to shape T follicular helper cell differentiation. Immunity (2021) 54(12):2740–55 e6. doi: 10.1016/j.immuni.2021.09.018
41. Gonzalez DG, Cote CM, Patel JR, Smith CB, Zhang Y, Nickerson KM, et al. Nonredundant roles of IL-21 and IL-4 in the phased initiation of germinal center b cells and subsequent self-renewal transitions. J Immunol (2018) 201(12):3569–79. doi: 10.4049/jimmunol.1500497
42. Vogelzang A, McGuire HM, Yu D, Sprent J, Mackay CR, King C. A fundamental role for interleukin-21 in the generation of T follicular helper cells. Immunity (2008) 29(1):127–37. doi: 10.1016/j.immuni.2008.06.001
43. Kuchen S, Robbins R, Sims GP, Sheng C, Phillips TM, Lipsky PE, et al. Essential role of IL-21 in b cell activation, expansion, and plasma cell generation during CD4+ T cell-b cell collaboration. J Immunol (2007) 179(9):5886–96. doi: 10.4049/jimmunol.179.9.5886
44. Morita R, Schmitt N, Bentebibel SE, Ranganathan R, Bourdery L, Zurawski G, et al. Human blood CXCR5(+)CD4(+) T cells are counterparts of T follicular cells and contain specific subsets that differentially support antibody secretion. Immunity (2011) 34(1):108–21. doi: 10.1016/j.immuni.2010.12.012
45. Kim SJ, Lee K, Diamond B. Follicular helper T cells in systemic lupus erythematosus. Front Immunol (2018) 9:1793. doi: 10.3389/fimmu.2018.01793
46. Morille J, Mandon M, Rodriguez S, Roulois D, Leonard S, Garcia A, et al. Multiple sclerosis CSF is enriched with follicular T cells displaying a Th1/Eomes signature. Neurology(R) neuroimmunology Neuroinflamm (2022) 9(6):e200033. doi: 10.1212/NXI.0000000000200033
47. Annunziato F, Cosmi L, Santarlasci V, Maggi L, Liotta F, Mazzinghi B, et al. Phenotypic and functional features of human Th17 cells. J Exp Med (2007) 204(8):1849–61. doi: 10.1084/jem.20070663
48. Rao DA, Gurish MF, Marshall JL, Slowikowski K, Fonseka CY, Liu Y, et al. Pathologically expanded peripheral T helper cell subset drives b cells in rheumatoid arthritis. Nature (2017) 542(7639):110–4. doi: 10.1038/nature20810
49. Sage PT, Ron-Harel N, Juneja VR, Sen DR, Maleri S, Sungnak W, et al. Suppression by T(FR) cells leads to durable and selective inhibition of b cell effector function. Nat Immunol (2016) 17(12):1436–46. doi: 10.1038/ni.3578
50. Zhu Y, Zou L, Liu YC. T Follicular helper cells, T follicular regulatory cells and autoimmunity. Int Immunol (2016) 28(4):173–9. doi: 10.1093/intimm/dxv079
51. Fonseca VR, Ribeiro F, Graca L. T Follicular regulatory (Tfr) cells: dissecting the complexity of tfr-cell compartments. Immunol Rev (2019) 288(1):112–27. doi: 10.1111/imr.12739
52. Fonseca VR, Agua-Doce A, Maceiras AR, Pierson W, Ribeiro F, Romao VC, et al. Human blood t(fr) cells are indicators of ongoing humoral activity not fully licensed with suppressive function. Sci Immunol (2017) 2(14):eaan1487. doi: 10.1126/sciimmunol.aan1487
53. Kawamoto S, Tran TH, Maruya M, Suzuki K, Doi Y, Tsutsui Y, et al. The inhibitory receptor PD-1 regulates IgA selection and bacterial composition in the gut. Science (2012) 336(6080):485–9. doi: 10.1126/science.1217718
54. Bemani P, Eklund KK, Ali-Hassanzadeh M, Kabelitz D, Schmidt RE, Meri S, et al. Proportion of T follicular helper cells in peripheral blood of rheumatoid arthritis patients: a systematic review and meta-analysis. Expert Rev Clin Immunol (2021) 17(6):667–80. doi: 10.1080/1744666X.2021.1915770
55. Cao G, Chi S, Wang X, Sun J, Zhang Y. CD4+CXCR5+PD-1+ T follicular helper cells play a pivotal role in the development of rheumatoid arthritis. Med Sci Monit (2019) 25:3032–40. doi: 10.12659/MSM.914868
56. Anang DC, Ramwadhdoebe TH, Hahnlein JS, van Kuijk B, Smits N, van Lienden KP, et al. Increased frequency of CD4(+) follicular helper T and CD8(+) follicular T cells in human lymph node biopsies during the earliest stages of rheumatoid arthritis. Cells (2022) 11(7):1104. doi: 10.3390/cells11071104
57. Sun WK, Bai Y, Yi MM, Wu LJ, Chen JL, Wu DM, et al. Expression of T follicular helper lymphocytes with different subsets and analysis of serum IL-6, IL-17, TGF-beta and MMP-3 contents in patients with rheumatoid arthritis. Eur Rev Med Pharmacol Sci (2019) 23(1):61–9. doi: 10.26355/eurrev_201901_16748
58. Fortea-Gordo P, Nuno L, Villalba A, Peiteado D, Monjo I, Sanchez-Mateos P, et al. Two populations of circulating PD-1hiCD4 T cells with distinct b cell helping capacity are elevated in early rheumatoid arthritis. Rheumatol (Oxford) (2019) 58(9):1662–73. doi: 10.1093/rheumatology/kez169
59. Ribeiro F, Romao VC, Rosa S, Jesus K, Agua-Doce A, Barreira SC, et al. Different antibody-associated autoimmune diseases have distinct patterns of T follicular cell dysregulation. Sci Rep (2022) 12(1):17638. doi: 10.1038/s41598-022-21576-8
60. Wang X, Yang C, Xu F, Qi L, Wang J, Yang P. Imbalance of circulating Tfr/Tfh ratio in patients with rheumatoid arthritis. Clin Exp Med (2019) 19(1):55–64. doi: 10.1007/s10238-018-0530-5
61. Niu Q, Huang ZC, Wu XJ, Jin YX, An YF, Li YM, et al. Enhanced IL-6/phosphorylated STAT3 signaling is related to the imbalance of circulating T follicular helper/T follicular regulatory cells in patients with rheumatoid arthritis. Arthritis Res Ther (2018) 20(1):200. doi: 10.1186/s13075-018-1690-0
62. Zhao Z, Xu B, Wang S, Zhou M, Huang Y, Guo C, et al. Tfh cells with NLRP3 inflammasome activation are essential for high-affinity antibody generation, germinal centre formation and autoimmunity. Ann rheumatic Dis (2022) 81(7):1006–12. doi: 10.1136/annrheumdis-2021-221985
63. Bocharnikov AV, Keegan J, Wacleche VS, Cao Y, Fonseka CY, Wang G, et al. PD-1hiCXCR5- T peripheral helper cells promote b cell responses in lupus via MAF and IL-21. JCI Insight (2019) 4(20):e130062. doi: 10.1172/jci.insight.130062
64. Makiyama A, Chiba A, Noto D, Murayama G, Yamaji K, Tamura N, et al. Expanded circulating peripheral helper T cells in systemic lupus erythematosus: association with disease activity and b cell differentiation. Rheumatol (Oxford) (2019) 58(10):1861–9. doi: 10.1093/rheumatology/kez077
65. Caielli S, Veiga DT, Balasubramanian P, Athale S, Domic B, Murat E, et al. A CD4(+) T cell population expanded in lupus blood provides b cell help through interleukin-10 and succinate. Nat Med (2019) 25(1):75–81. doi: 10.1038/s41591-018-0254-9
66. Facciotti F, Larghi P, Bosotti R, Vasco C, Gagliani N, Cordiglieri C, et al. Evidence for a pathogenic role of extrafollicular, IL-10-producing CCR6(+)B helper T cells in systemic lupus erythematosus. Proc Natl Acad Sci U.S.A. (2020) 117(13):7305–16. doi: 10.1073/pnas.1917834117
67. Liu C, Wang D, Song Y, Lu S, Zhao J, Wang H. Increased circulating CD4(+)CXCR5(+)FoxP3(+) follicular regulatory T cells correlated with severity of systemic lupus erythematosus patients. Int Immunopharmacol (2018) 56:261–8. doi: 10.1016/j.intimp.2018.01.038
68. Kurata I, Mikami N, Ohyama A, Osada A, Kondo Y, Tsuboi H, et al. Impaired function of PD-1(+) follicular regulatory T cells in systemic lupus erythematosus. Clin Exp Immunol (2021) 206(1):28–35. doi: 10.1111/cei.13643
69. Xu B, Wang S, Zhou M, Huang Y, Fu R, Guo C, et al. The ratio of circulating follicular T helper cell to follicular T regulatory cell is correlated with disease activity in systemic lupus erythematosus. Clin Immunol (2017) 183:46–53. doi: 10.1016/j.clim.2017.07.004
70. Szabo K, Papp G, Szanto A, Tarr T, Zeher M. A comprehensive investigation on the distribution of circulating follicular T helper cells and b cell subsets in primary sjogren's syndrome and systemic lupus erythematosus. Clin Exp Immunol (2016) 183(1):76–89. doi: 10.1111/cei.12703
71. Szabo K, Jambor I, Szanto A, Horvath IF, Tarr T, Nakken B, et al. The imbalance of circulating follicular T helper cell subsets in primary sjogren's syndrome associates with serological alterations and abnormal b-cell distribution. Front Immunol (2021) 12:639975. doi: 10.3389/fimmu.2021.639975
72. Kim JW, Lee J, Hong SM, Lee J, Cho ML, Park SH. Circulating CCR7(lo)PD-1(hi) follicular helper T cells indicate disease activity and glandular inflammation in patients with primary sjogren's syndrome. Immune Netw (2019) 19(4):e26. doi: 10.4110/in.2019.19.e26
73. Hernandez-Molina G, Soto-Abraham V, Zamora-Legoff V, Furuzawa-Carballeda J. Differential Th follicular cell subsets in minor salivary glands of patients with primary sjogren's syndrome and systemic lupus erythematosus associated with sjogren's syndrome. Clin Exp Rheumatol (2021) 39 Suppl 133(6):49–56. doi: 10.55563/clinexprheumatol/yuj21y
74. Blokland SLM, Hillen MR, Kruize AA, Meller S, Homey B, Smithson GM, et al. Increased CCL25 and T helper cells expressing CCR9 in the salivary glands of patients with primary sjogren's syndrome: potential new axis in lymphoid neogenesis. Arthritis Rheumatol (2017) 69(10):2038–51. doi: 10.1002/art.40182
75. Blokland SLM, van Vliet-Moret FM, Hillen MR, Pandit A, Goldschmeding R, Kruize AA, et al. Epigenetically quantified immune cells in salivary glands of sjogren's syndrome patients: a novel tool that detects robust correlations of T follicular helper cells with immunopathology. Rheumatol (Oxford) (2020) 59(2):335–43. doi: 10.1093/rheumatology/kez268
76. Pontarini E, Murray-Brown WJ, Croia C, Lucchesi D, Conway J, Rivellese F, et al. Unique expansion of IL-21+ tfh and tph cells under control of ICOS identifies sjogren's syndrome with ectopic germinal centres and MALT lymphoma. Ann rheumatic Dis (2020) 79(12):1588–99. doi: 10.1136/annrheumdis-2020-217646
77. Fonseca VR, Graca L. Contribution of FoxP3(+) tfr cells to overall human blood CXCR5(+) T cells. Clin Exp Immunol (2019) 195(3):302–4. doi: 10.1111/cei.13245
78. Wang Y, Guo H, Liang Z, Feng M, Wu Y, Qin Y, et al. Sirolimus therapy restores the PD-1+ICOS+Tfh:CD45RA-Foxp3(high) activated tfr cell balance in primary sjogren's syndrome. Mol Immunol (2022) 147:90–100. doi: 10.1016/j.molimm.2022.04.006
79. Brokstad KA, Fredriksen M, Zhou F, Bergum B, Brun JG, Cox RJ, et al. T Follicular-like helper cells in the peripheral blood of patients with primary sjogren's syndrome. Scand J Immunol (2018):e12679. doi: 10.1111/sji.12679
80. Zhao Y, Lutalo PM, Thomas JE, Sangle S, Choong LM, Tyler JR, et al. Circulating T follicular helper cell and regulatory T cell frequencies are influenced by b cell depletion in patients with granulomatosis with polyangiitis. Rheumatol (Oxford) (2014) 53(4):621–30. doi: 10.1093/rheumatology/ket406
81. Abdulahad WH, Lepse N, Stegeman CA, Huitema MG, Doornbos-van der Meer B, Tadema H, et al. Increased frequency of circulating IL-21 producing Th-cells in patients with granulomatosis with polyangiitis (GPA). Arthritis Res Ther (2013) 15(3):R70. doi: 10.1186/ar4247
82. Tzartos JS, Craner MJ, Friese MA, Jakobsen KB, Newcombe J, Esiri MM, et al. IL-21 and IL-21 receptor expression in lymphocytes and neurons in multiple sclerosis brain. Am J Pathol (2011) 178(2):794–802. doi: 10.1016/j.ajpath.2010.10.043
83. Romme Christensen J, Bornsen L, Ratzer R, Piehl F, Khademi M, Olsson T, et al. Systemic inflammation in progressive multiple sclerosis involves follicular T-helper, Th17- and activated b-cells and correlates with progression. PloS One (2013) 8(3):e57820. doi: 10.1371/journal.pone.0057820
84. Cunill V, Massot M, Clemente A, Calles C, Andreu V, Nunez V, et al. Relapsing-remitting multiple sclerosis is characterized by a T follicular cell pro-inflammatory shift, reverted by dimethyl fumarate treatment. Front Immunol (2018) 9:1097. doi: 10.3389/fimmu.2018.01097
85. Haque R, Kim Y, Park K, Jang H, Kim SY, Lee H, et al. Altered distributions in circulating follicular helper and follicular regulatory T cells accountable for imbalanced cytokine production in multiple sclerosis. Clin Exp Immunol (2021) 205(1):75–88. doi: 10.1111/cei.13596
86. Fan X, Jin T, Zhao S, Liu C, Han J, Jiang X, et al. Circulating CCR7+ICOS+ memory T follicular helper cells in patients with multiple sclerosis. PloS One (2015) 10(7):e0134523. doi: 10.1371/journal.pone.0134523
87. Dhaeze T, Peelen E, Hombrouck A, Peeters L, Van Wijmeersch B, Lemkens N, et al. Circulating follicular regulatory T cells are defective in multiple sclerosis. J Immunol (2015) 195(3):832–40. doi: 10.4049/jimmunol.1500759
88. Schafflick D, Xu CA, Hartlehnert M, Cole M, Schulte-Mecklenbeck A, Lautwein T, et al. Integrated single cell analysis of blood and cerebrospinal fluid leukocytes in multiple sclerosis. Nat Commun (2020) 11(1):247. doi: 10.1038/s41467-019-14118-w
89. Holm Hansen R, Talbot J, Hojsgaard Chow H, Bredahl Hansen M, Buhelt S, Herich S, et al. Increased intrathecal activity of follicular helper T cells in patients with relapsing-remitting multiple sclerosis. Neurology(R) neuroimmunology Neuroinflamm (2022) 9(5):e200009. doi: 10.1212/NXI.0000000000200009
90. Puthenparampil M, Zito A, Pantano G, Federle L, Stropparo E, Miante S, et al. Peripheral imbalanced TFH/TFR ratio correlates with intrathecal IgG synthesis in multiple sclerosis at clinical onset. Multiple sclerosis (2019) 25(7):918–26. doi: 10.1177/1352458518779951
91. Vecchione A, Madley R, Danzl N, Borsotti C, Marharlooei MK, Li HW, et al. T1D patient-derived hematopoietic stem cells are programmed to generate tph, tfh, and autoimmunity-associated b cell subsets in human immune system mice. Clin Immunol (2022) 240:109048. doi: 10.1016/j.clim.2022.109048
92. Vandamme C, Kinnunen T. B cell helper T cells and type 1 diabetes. Scand J Immunol (2020) 92(4):e12943. doi: 10.1111/sji.12943
93. Ferreira RC, Simons HZ, Thompson WS, Cutler AJ, Dopico XC, Smyth DJ, et al. IL-21 production by CD4+ effector T cells and frequency of circulating follicular helper T cells are increased in type 1 diabetes patients. Diabetologia (2015) 58(4):781–90. doi: 10.1007/s00125-015-3509-8
94. Ekman I, Ihantola EL, Viisanen T, Rao DA, Nanto-Salonen K, Knip M, et al. Circulating CXCR5(-)PD-1(hi) peripheral T helper cells are associated with progression to type 1 diabetes. Diabetologia (2019) 62(9):1681–8. doi: 10.1007/s00125-019-4936-8
95. Shao F, Zheng P, Yu D, Zhou Z, Jia L. Follicular helper T cells in type 1 diabetes. FASEB J (2020) 34(1):30–40. doi: 10.1096/fj.201901637R
96. Bonami RH, Nyhoff LE, McNitt DH, Hulbert C, Felton JL, Kendall PL, et al. T-B lymphocyte interactions promote type 1 diabetes independently of SLAM-associated protein. J Immunol (2020) 205(12):3263–76. doi: 10.4049/jimmunol.1900464
97. Vecchione A, Jofra T, Gerosa J, Shankwitz K, Di Fonte R, Galvani G, et al. Reduced follicular regulatory T cells in spleen and pancreatic lymph nodes of patients with type 1 diabetes. Diabetes (2021) 70(12):2892–902. doi: 10.2337/db21-0091
98. Xu X, Shen M, Zhao R, Cai Y, Jiang H, Shen Z, et al. Follicular regulatory T cells are associated with beta-cell autoimmunity and the development of type 1 diabetes. J Clin Endocrinol Metab (2019) 104(9):4199–213. doi: 10.1210/jc.2019-00093
99. Benucci M, Bernardini P, Coccia C, De Luca R, Levani J, Economou A, et al. JAK inhibitors and autoimmune rheumatic diseases. Autoimmun Rev (2023) 22(4):103276. doi: 10.1016/j.autrev.2023.103276
100. Chavele KM, Merry E, Ehrenstein MR. Cutting edge: circulating plasmablasts induce the differentiation of human T follicular helper cells via IL-6 production. J Immunol (2015) 194(6):2482–5. doi: 10.4049/jimmunol.1401190
101. Kurata I, Matsumoto I, Ohyama A, Osada A, Ebe H, Kawaguchi H, et al. Potential involvement of OX40 in the regulation of autoantibody sialylation in arthritis. Ann rheumatic Dis (2019) 78(11):1488–96. doi: 10.1136/annrheumdis-2019-215195
102. Bai Z, Lu Z, Liu R, Tang Y, Ye X, Jin M, et al. Iguratimod restrains circulating follicular helper T cell function by inhibiting glucose metabolism via Hif1alpha-HK2 axis in rheumatoid arthritis. Front Immunol (2022) 13:757616. doi: 10.3389/fimmu.2022.757616
103. Fukuyo S, Nakayamada S, Iwata S, Kubo S, Saito K, Tanaka Y. Abatacept therapy reduces CD28+CXCR5+ follicular helper-like T cells in patients with rheumatoid arthritis. Clin Exp Rheumatol (2017) 35(4):562–70.
104. Wu R, Li N, Zhao X, Ding T, Xue H, Gao C, et al. Low-dose interleukin-2: biology and therapeutic prospects in rheumatoid arthritis. Autoimmun Rev (2020) 19(10):102645. doi: 10.1016/j.autrev.2020.102645
105. Azizov V, Dietel K, Steffen F, Durholz K, Meidenbauer J, Lucas S, et al. Ethanol consumption inhibits T(FH) cell responses and the development of autoimmune arthritis. Nat Commun (2020) 11(1):1998. doi: 10.1038/s41467-020-15855-z
106. Ding T, Niu H, Zhao X, Gao C, Li X, Wang C. T-Follicular regulatory cells: potential therapeutic targets in rheumatoid arthritis. Front Immunol (2019) 10:2709. doi: 10.3389/fimmu.2019.02709
107. Aldridge J, Andersson K, Gjertsson I, Hultgard Ekwall AK, Hallstrom M, van Vollenhoven R, et al. Blood PD-1+TFh and CTLA-4+CD4+ T cells predict remission after CTLA-4Ig treatment in early rheumatoid arthritis. Rheumatol (Oxford) (2022) 61(3):1233–42. doi: 10.1093/rheumatology/keab454
108. Gomez-Banuelos E, Fava A, Andrade F. An update on autoantibodies in systemic lupus erythematosus. Curr Opin Rheumatol (2023) 35(2):61–7. doi: 10.1097/BOR.0000000000000922
109. Wang L, Zhao P, Ma L, Shan Y, Jiang Z, Wang J, et al. Increased interleukin 21 and follicular helper T-like cells and reduced interleukin 10+ b cells in patients with new-onset systemic lupus erythematosus. J Rheumatol (2014) 41(9):1781–92. doi: 10.3899/jrheum.131025
110. Jin X, Chen J, Wu J, Lu Y, Li B, Fu W, et al. Aberrant expansion of follicular helper T cell subsets in patients with systemic lupus erythematosus. Front Immunol (2022) 13:928359. doi: 10.3389/fimmu.2022.928359
111. Mao M, Xu S, Lin L, Dong D, Xue M, He S, et al. Impact of corticosteroids on the proportions of circulating tfh cell subsets in patients with systemic lupus erythematous. Front Med (2022) 9:949334. doi: 10.3389/fmed.2022.949334
112. Psarras A, Wittmann M, Vital EM. Emerging concepts of type I interferons in SLE pathogenesis and therapy. Nat Rev Rheumatol (2022) 18(10):575–90. doi: 10.1038/s41584-022-00826-z
113. Tanemura S, Tsujimoto H, Seki N, Kojima S, Miyoshi F, Sugahara K, et al. Role of interferons (IFNs) in the differentiation of T peripheral helper (Tph) cells. Int Immunol (2022) 34(10):519–32. doi: 10.1093/intimm/dxac026
114. Dong X, Antao OQ, Song W, Sanchez GM, Zembrzuski K, Koumpouras F, et al. Type I interferon-activated STAT4 regulation of follicular helper T cell-dependent cytokine and immunoglobulin production in lupus. Arthritis Rheumatol (2021) 73(3):478–89. doi: 10.1002/art.41532
115. Wen Z, Xu L, Xu W, Xiong S. Retinoic acid receptor-related orphan nuclear receptor gammat licenses the differentiation and function of a unique subset of follicular helper T cells in response to immunogenic self-DNA in systemic lupus erythematosus. Arthritis Rheumatol (2021) 73(8):1489–500. doi: 10.1002/art.41687
116. Jacquemin C, Schmitt N, Contin-Bordes C, Liu Y, Narayanan P, Seneschal J, et al. OX40 ligand contributes to human lupus pathogenesis by promoting T follicular helper response. Immunity (2015) 42(6):1159–70. doi: 10.1016/j.immuni.2015.05.012
117. Feng X, Wang D, Chen J, Lu L, Hua B, Li X, et al. Inhibition of aberrant circulating tfh cell proportions by corticosteroids in patients with systemic lupus erythematosus. PloS One (2012) 7(12):e51982. doi: 10.1371/journal.pone.0051982
118. Liang K, He J, Wei Y, Zeng Q, Gong D, Qin J, et al. Sustained low-dose interleukin-2 therapy alleviates pathogenic humoral immunity via elevating the Tfr/Tfh ratio in lupus. Clin Transl Immunol (2021) 10(6):e1293. doi: 10.1002/cti2.1293
119. Miao M, Xiao X, Tian J, Zhufeng Y, Feng R, Zhang R, et al. Therapeutic potential of targeting Tfr/Tfh cell balance by low-dose-IL-2 in active SLE: a post hoc analysis from a double-blind RCT study. Arthritis Res Ther (2021) 23(1):167. doi: 10.1186/s13075-021-02535-6
120. Ballesteros-Tato A, Leon B, Graf BA, Moquin A, Adams PS, Lund FE, et al. Interleukin-2 inhibits germinal center formation by limiting T follicular helper cell differentiation. Immunity (2012) 36(5):847–56. doi: 10.1016/j.immuni.2012.02.012
121. Zhang Z, Feng R, Niu L, Huang S, Deng W, Shi B, et al. Human umbilical cord mesenchymal stem cells inhibit T follicular helper cell expansion through the activation of iNOS in lupus-prone B6.MRL-fas(lpr) mice. Cell Transplant (2017) 26(6):1031–42. doi: 10.3727/096368917X694660
122. Duan X, Shen C, Zhang X, Wu L, Chen J, Ma B, et al. Toll-like receptor 7 agonist imiquimod prevents the progression of SLE in MRL/lpr mice via inhibiting the differentiation of T follicular helper cells. Int Immunopharmacol (2020) 80:106239. doi: 10.1016/j.intimp.2020.106239
123. Yang J, Yang X, Yang J, Li M. Baicalin ameliorates lupus autoimmunity by inhibiting differentiation of tfh cells and inducing expansion of tfr cells. Cell Death Dis (2019) 10(2):140. doi: 10.1038/s41419-019-1315-9
124. Kumar P, Balakrishnan S, Surendra Lele S, Setty S, Dhingra S, Epstein AL, et al. Restoration of follicular T Regulatory/Helper cell balance by OX40L-JAG1 cotreatment suppresses lupus nephritis in NZBWF1/j mice. J Immunol (2022) 208(11):2467–81. doi: 10.4049/jimmunol.2200057
125. Faliti CE, Gualtierotti R, Rottoli E, Gerosa M, Perruzza L, Romagnani A, et al. P2X7 receptor restrains pathogenic tfh cell generation in systemic lupus erythematosus. J Exp Med (2019) 216(2):317–36. doi: 10.1084/jem.20171976
126. Ritter J, Chen Y, Stefanski AL, Dorner T. Current and future treatment in primary sjogren's syndrome - a still challenging development. Joint Bone Spine (2022) 89(6):105406. doi: 10.1016/j.jbspin.2022.105406
127. He C, Yang Y, Chen Z, Liu S, Lyu T, Zeng L, et al. EZH2 promotes T follicular helper cell differentiation through enhancing STAT3 phosphorylation in patients with primary sjogren's syndrome. Front Immunol (2022) 13:922871. doi: 10.3389/fimmu.2022.922871
128. Liu R, Su D, Zhou M, Feng X, Li X, Sun L. Umbilical cord mesenchymal stem cells inhibit the differentiation of circulating T follicular helper cells in patients with primary sjogren's syndrome through the secretion of indoleamine 2,3-dioxygenase. Rheumatol (Oxford) (2015) 54(2):332–42. doi: 10.1093/rheumatology/keu316
129. Verstappen GM, Kroese FG, Meiners PM, Corneth OB, Huitema MG, Haacke EA, et al. B cell depletion therapy normalizes circulating follicular Th cells in primary sjogren syndrome. J Rheumatol (2017) 44(1):49–58. doi: 10.3899/jrheum.160313
130. Lin R, Hao D, Dong Y, Wang Y. Catalpol ameliorates sjogren's syndrome by modulating interplay of T and b cells. BioMed Pharmacother (2020) 123:109806. doi: 10.1016/j.biopha.2019.109806
131. Verstappen GM, Meiners PM, Corneth OBJ, Visser A, Arends S, Abdulahad WH, et al. Attenuation of follicular helper T cell-dependent b cell hyperactivity by abatacept treatment in primary sjogren's syndrome. Arthritis Rheumatol (2017) 69(9):1850–61. doi: 10.1002/art.40165
132. Nakazawa D, Masuda S, Tomaru U, Ishizu A. Pathogenesis and therapeutic interventions for ANCA-associated vasculitis. Nat Rev Rheumatol (2019) 15(2):91–101. doi: 10.1038/s41584-018-0145-y
133. Kim S, Boehme L, Nel L, Casian A, Sangle S, Nova-Lamperti E, et al. Defective STAT5 activation and aberrant expression of BCL6 in naive CD4 T cells enhances follicular Th cell-like differentiation in patients with granulomatosis with polyangiitis. J Immunol (2022) 208(4):807–18. doi: 10.4049/jimmunol.2001331
134. Angelini G, Bani A, Constantin G, Rossi B. The interplay between T helper cells and brain barriers in the pathogenesis of multiple sclerosis. Front Cell Neurosci (2023) 17:1101379. doi: 10.3389/fncel.2023.1101379
135. Gharibi T, Kazemi T, Aliparasti MR, Farhoudi M, Almasi S, Dehghanzadeh R, et al. Investigation of IL-21 gene polymorphisms (rs2221903, rs2055979) in cases with multiple sclerosis of Azerbaijan, Northwest Iran. Am J Clin Exp Immunol (2015) 4(1):7–14.
136. International Multiple Sclerosis Genetics, C, Lill CM, Schjeide BM, Graetz C, Ban M, Alcina A, et al. MANBA, CXCR5, SOX8, RPS6KB1 and ZBTB46 are genetic risk loci for multiple sclerosis. Brain J Neurol (2013) 136(Pt 6):1778–82. doi: 10.1093/brain/awt101
137. Pawlak-Adamska E, Nowak O, Karabon L, Pokryszko-Dragan A, Partyka A, Tomkiewicz A, et al. PD-1 gene polymorphic variation is linked with first symptom of disease and severity of relapsing-remitting form of MS. J neuroimmunology (2017) 305:115–27. doi: 10.1016/j.jneuroim.2017.02.006
138. Guo J, Zhao C, Wu F, Tao L, Zhang C, Zhao D, et al. T Follicular helper-like cells are involved in the pathogenesis of experimental autoimmune encephalomyelitis. Front Immunol (2018) 9:944. doi: 10.3389/fimmu.2018.00944
139. Luo L, Hu X, Dixon ML, Pope BJ, Leavenworth JD, Raman C, et al. Dysregulated follicular regulatory T cells and antibody responses exacerbate experimental autoimmune encephalomyelitis. J Neuroinflamm (2021) 18(1):27. doi: 10.1186/s12974-021-02076-4
140. Varrin-Doyer M, Pekarek KL, Spencer CM, Bernard CC, Sobel RA, Cree BA, et al. Treatment of spontaneous EAE by laquinimod reduces tfh, b cell aggregates, and disease progression. Neurology(R) neuroimmunology Neuroinflamm (2016) 3(5):e272. doi: 10.1212/NXI.0000000000000272
141. Ratzer R, Romme Christensen J, Romme Nielsen B, Sorensen PS, Bornsen L, Sellebjerg F. Immunological effects of methylprednisolone pulse treatment in progressive multiple sclerosis. J neuroimmunology (2014) 276(1-2):195–201. doi: 10.1016/j.jneuroim.2014.08.623
142. Yahyazadeh S, Esmaeil N, Shaygannejad V, Mirmosayyeb O. Comparison of follicular T helper cells, monocytes, and T cells priming between newly diagnosed and rituximab-treated MS patients and healthy controls. Res Pharm Sci (2022) 17(3):315–23. doi: 10.4103/1735-5362.343085
143. Yuzefpolskiy Y, Morawski P, Fahning M, Speake C, Lord S, Chaudhary A, et al. Cutting edge: effect of disease-modifying therapies on SARS-CoV-2 vaccine-induced immune responses in multiple sclerosis patients. J Immunol (2022) 208(7):1519–24. doi: 10.4049/jimmunol.2101142
144. Holm Hansen R, Hojsgaard Chow H, Christensen JR, Sellebjerg F, von Essen MR. Dimethyl fumarate therapy reduces memory T cells and the CNS migration potential in patients with multiple sclerosis. Multiple sclerosis related Disord (2020) 37:101451. doi: 10.1016/j.msard.2019.101451
145. Huber JE, Chang Y, Meinl I, Kumpfel T, Meinl E, Baumjohann D. Fingolimod profoundly reduces frequencies and alters subset composition of circulating T follicular helper cells in multiple sclerosis patients. J Immunol (2020) 204(5):1101–10. doi: 10.4049/jimmunol.1900955
146. Jing Z, Li Y, Ma Y, Zhang X, Liang X, Zhang X. Leverage biomaterials to modulate immunity for type 1 diabetes. Front Immunol (2022) 13:997287. doi: 10.3389/fimmu.2022.997287
147. Zou X, Wang S, Zhang Y, Wang X, Yang W. The role of follicular T helper cells in the onset and treatment of type 1 diabetes. Int Immunopharmacol (2020) 84:106499. doi: 10.1016/j.intimp.2020.106499
148. Xu X, Shi Y, Cai Y, Zhang Q, Yang F, Chen H, et al. Inhibition of increased circulating tfh cell by anti-CD20 monoclonal antibody in patients with type 1 diabetes. PloS One (2013) 8(11):e79858. doi: 10.1371/journal.pone.0079858
149. Edner NM, Heuts F, Thomas N, Wang CJ, Petersone L, Kenefeck R, et al. Follicular helper T cell profiles predict response to costimulation blockade in type 1 diabetes. Nat Immunol (2020) 21(10):1244–55. doi: 10.1038/s41590-020-0744-z
150. Kenefeck R, Wang CJ, Kapadi T, Wardzinski L, Attridge K, Clough LE, et al. Follicular helper T cell signature in type 1 diabetes. J Clin Invest (2015) 125(1):292–303. doi: 10.1172/JCI76238
151. Basso K, Dalla-Favera R. Roles of BCL6 in normal and transformed germinal center b cells. Immunol Rev (2012) 247(1):172–83. doi: 10.1111/j.1600-065X.2012.01112.x
152. Bosga-Bouwer AG, Haralambieva E, Booman M, Boonstra R, van den Berg A, Schuuring E, et al. BCL6 alternative translocation breakpoint cluster region associated with follicular lymphoma grade 3B. Genes Chromosomes Cancer (2005) 44(3):301–4. doi: 10.1002/gcc.20246
153. Kerres N, Steurer S, Schlager S, Bader G, Berger H, Caligiuri M, et al. Chemically induced degradation of the oncogenic transcription factor BCL6. Cell Rep (2017) 20(12):2860–75. doi: 10.1016/j.celrep.2017.08.081
154. Gu H, He J, Li Y, Mi D, Guan T, Guo W, et al. B-cell lymphoma 6 inhibitors: current advances and prospects of drug development for diffuse Large b-cell lymphomas. J Med Chem (2022) 65(23):15559–83. doi: 10.1021/acs.jmedchem.2c01433
155. Cattoretti G, Pasqualucci L, Ballon G, Tam W, Nandula SV, Shen Q, et al. Deregulated BCL6 expression recapitulates the pathogenesis of human diffuse large b cell lymphomas in mice. Cancer Cell (2005) 7(5):445–55. doi: 10.1016/j.ccr.2005.03.037
Keywords: T follicular helper cells (TFH cells), T follicular regulatory cells (TFR), autoimmune diseases, rheumatoid arthritis, systemic lupus erythematosus, Sjögren’s syndrome, type 1 diabetes, multiple sclerosis
Citation: Qi J, Liu C, Bai Z, Li X and Yao G (2023) T follicular helper cells and T follicular regulatory cells in autoimmune diseases. Front. Immunol. 14:1178792. doi: 10.3389/fimmu.2023.1178792
Received: 03 March 2023; Accepted: 13 April 2023;
Published: 28 April 2023.
Edited by:
Valentyn Oksenych, University of Oslo, NorwayReviewed by:
Dirk Baumjohann, University Hospital Bonn, GermanyArtem Kalinichenko, St. Anna Children’s Cancer Research Institute (CCRI), Austria
Susan John, King’s College London, United Kingdom
Esther C Zumaquero Martinez, University of Alabama at Birmingham, United States
Yunbo Wei, Qilu University of Technology, China
Copyright © 2023 Qi, Liu, Bai, Li and Yao. This is an open-access article distributed under the terms of the Creative Commons Attribution License (CC BY). The use, distribution or reproduction in other forums is permitted, provided the original author(s) and the copyright owner(s) are credited and that the original publication in this journal is cited, in accordance with accepted academic practice. No use, distribution or reproduction is permitted which does not comply with these terms.
*Correspondence: Genhong Yao, eWFvZ2VuaG9uZ0BuanUuZWR1LmNu; Jingjing Qi, cWpqMTkxMDAxQGRtdS5lZHUuY24=
†These authors have contributed equally to this work