- 1Rehabilitation Department, Guangdong Work Injury Rehabilitation Hospital, Guangzhou, China
- 2Department of Spleen and Stomach, GuangZhou Tianhe District Hospital of Chinese Medicine, Guangzhou, China
- 3The Second Affiliated Hospital, Guangzhou University of Chinese Medicine, Guangzhou, China
- 4The First Affiliated Hospital, Guangzhou University of Chinese Medicine, Guangzhou, China
- 5Science and Innovation Center, Guangzhou University of Chinese Medicine, Guangzhou, China
Gastric precancerous lesions (GPL) are a major health concern worldwide due to their potential to progress to gastric cancer (GC). Understanding the mechanism underlying the transformation from GPL to GC can provide a fresh insight for the early detection of GC. Although chronic inflammation is prevalent in the GPL, how the inflammatory microenvironment monitored the progression of GPL-to-GC are still elusive. Inflammation has been recognized as a key player in the progression of GPL. This review aims to provide an overview of the inflammatory microenvironment in GPL and its implications for disease progression and potential therapeutic applications. We discuss the involvement of inflammation in the progression of GPL, highlighting Helicobacter pylori (H. pylori) as a mediator for inflammatory microenvironment and a key driver to GC progression. We explore the role of immune cells in mediating the progression of GPL, and focus on the regulation of inflammatory molecules in this disease. Furthermore, we discuss the potential of targeting inflammatory pathways for GPL. There are currently no specific drugs for GPL treatment, but traditional Chinese Medicine (TCM) and natural antioxidants, known as antioxidant and anti-inflammatory properties, exhibit promising effects in suppressing or reversing the progression of GPL. Finally, the challenges and future perspectives in the field are proposed. Overall, this review highlights the central role of the inflammatory microenvironment in the progression of GPL, paving the way for innovative therapeutic approaches in the future.
Key points
• Chronic inflammation stimulates cells to secrete inflammatory factors and changes in immune cell function, which further promotes inflammatory changes in the gastric mucosa and even leads to cancer.
• Altered inflammatory immune microenvironment due to H. pylori infection enhances cytogenic Correa cascade progression based on epidemiological investigation and basic research.
• Inflammatory molecules regulation in gastric precancerous lesions progression.
• Traditional Chinese Medicine (TCM) and natural antioxidants, known as antioxidant and anti-inflammatory properties, exhibit promising effects in suppressing or reversing the progression of GPL.
1 Introduction
Gastric cancer(GC) ranks as the fifth most common tumor globally and stands as the third leading cause of cancer-related mortality across the world (1, 2) Its incidence is most pronounced in East Asia (3). Notably, GC is twice as likely to afflict men compared to women (1). The prognosis for advanced GC, with a 5-year survival rate of under 20%, is grim, while early gastric cancer (EGC) enjoys a favorable outlook, boasting a 5-year survival rate ranging from 90% to 95% (4).
Several prominent risk factors for GC encompass H. pylori infection, age, and dietary patterns. The progression of gastric lesions, from superficial gastritis (SG) to chronic atrophic gastritis (CAG), spasmolytic polypeptide-expressing metaplasia (SPEM), intestinal metaplasia (IM), and low-grade intraepithelial gastritis neoplasia (LGIN), can eventually lead to high-grade intraepithelial neoplasia (HGIN) and aggressive GC (5). These precancerous states (CAG, SPEM, and IM) and precancerous lesions (LGIN and HGIN) are associated with an elevated risk of GC. Chronic infection of the gastric mucosa lays the foundation for the progression of CAG and IM to gastric mucosal cancer. Approximately 5% of dysplasia (Dys) patients develop GC within two decades (6). Evidence suggests that IM arises from SPEM in humans, indicating that SPEM is the key initial pretumor metaplasia in gastric adenocarcinoma. Biopsies obtained before cancer diagnosis have shown that SPEM was detected in more than 4 out of 5 tumor patients, compared to only 1 out of 3 gastritis patients (7).
The clinical presentation of GPL is marked by nonspecific manifestations, including upper abdominal discomfort, acid reflux, and nausea, among others. It should be noted that there is no clear correlation between the severity of the pathology and the symptoms. The development of precancerous lesions is closely related to inflammatory processes and immune responses. Assessing the immune molecule expression in GPL is pivotal in evaluating the inflammatory status. Nevertheless, inflammatory response and immune response in GPL still poorly elucidated. On the other hand, treatment options for GPL encompass surgical intervention, H. pylori eradication, cyclooxygenase-2(COX-2) inhibitor and other symptomatic treatment, without specific therapy. However, traditional Chinese medicine and its active ingredients are effective in the treatment of GPL and more and more related studies, but there is a lack of systematic summary. This review aims to provide a concise overview of our current comprehension of diverse inflammatory immune response, and the treatment of traditional Chinese medicine and its active ingredients, unveiling the most recent research findings regarding their potential mechanisms of GPL.
2 Inflammation participates in the gastric precancerous lesion progression
Substantial epidemiological evidence has demonstrated that chronic inflammation of the gastric epithelium is important in GC development. This connection between inflammation and GC in humans has been meticulously documented through lifelong studies by Correa, which documents a clear association between inflammation and GC. Inflammatory microenvironments are common pathological characteristics and drive the development of multiple GPL (8). The cells and mediators responsible for inflammation constitute a substantial portion of the epithelial inflammatory microenvironment. In GPL-to-GC, inflammatory conditions often precede the onset of malignancy. Moreover, oncogenic changes create a tumor-promoting inflammatory milieu (9).
In 1994, H. pylori was unequivocally designated as a Class I carcinogen with a proven link to GC (10). The infection statistics reveal that 1%-3% of individuals harboring H. pylori will ultimately develop GC (11). This persistent infection takes root by inciting chronic and active inflammation within the gastric mucosa, setting the stage for a cascade of pathological events. The stepwise progression from H. pylori-induced GPL-to-GC has been meticulously defined in various animal models, including mice and Mongolian gerbils (12). The stomach’s enduring inflammation often triggers metaplastic alterations in the mucosa, characterized by the atrophy of mature oxyntic cells and the emergence of novel metaplastic lineages. Consequently, the degenerating mucosa can adopt a more proliferative phenotype, substantially elevating the risk of GPL transitioning to GC (13).
H. pylori, which harbors the cag pathogenicity island, triggers immune cell infiltration. Paradoxically, this robust immune and inflammatory response fails to eradicate the infection, leaving the host gastric mucosa ensnared in the enduring throes of inflammation. H. pylori infection stimulates inflammation and altered immune cell function promoting malignant transformation of GPL, according to growing evidence. Inflammatory response on gastric from H. pylori infection shows “sui generis” characteristics that are scarcely observed in other organs (14). Furthermore, research has unveiled that an immune response induced by Helicobacter pylori can promote genetic changes. The alterations encompass changes in transcription factors (CDX2, RUNX3, TLR1), interleukins (IL1β, IL8), and the generation of oxidative stress-induced DNA damage. These genetic modifications activate genes that drive tumor development while concurrently suppressing tumor suppressor genes (15), essentially placing GPL patients in a chronic inflammatory state (16). It’s important to note that while cell proliferation alone does not inevitably lead to cancer, within the context of an inflammatory microenvironment teeming with inflammatory cells and growth factors, heightened cell proliferation undeniably amplifies the risk of tumorigenesis (17). Oxidative damage and DNA damage accumulate gradually along GPL-GC (18). With inflammation, parietal cells undergo apoptosis, paving the way for the emergence of CAG and metaplastic cells (19). The gastric environment accumulates a mass of immune cells, which in turn, produce a multitude of inflammatory cytokines. Immune molecules triggered by antigen stimulation, including antibodies, complement, and lymphokines, assume pivotal roles. Dysregulation of immune cell activity or imbalances in immune-related factors can yield profound consequences in this intricate interplay of factors.
3 Immune cells mediated gastric precancerous lesions progression
Malignant tumor cells thrive within a multifaceted cellular microenvironment, comprising a dynamic interplay of endothelial cells, fibroblasts, and an array of immune cells. This diverse cast of immune cell types encompasses innate immune cells, adaptive immune cells, and immunosuppressive cells. The insidious influence of H. pylori infection amplifies the production of cytokines, thereby instigating a cascade of events that recruit and activate immune cells within this intricate milieu (20). Neutrophils and sometimes eosinophils represent the initial responders in the acute inflammatory reaction. Following neutrophils infiltrate, immune cells are summoned to the site of injury or infection. Inflammation is critical to orchestrating the migration and function of macrophages and T cells. As the inflammatory response concludes, both macrophages and T cells must adopt a pro-resolving phenotype to gradually terminate the inflammatory process. But under specific conditions, immune responses and inflammation persist, leading to the progression of chronic inflammatory diseases (Figure 1).
3.1 Neutrophil immune regulation in gastric precancerous lesions progression
Neutrophil infiltration is a typical occurrence in cases of acute gastritis induced by H. pylori (21). In the realm of tissue pathology, the extent of neutral granulocyte infiltration serves as a key indicator of gastritis inflammation severity (22). Typically, neutral granulocytes predominantly infiltrate the proliferation zone in normal conditions. However, in specific situations, they can extend into the surface region, potentially leading to the development of depressed abscesses (23). Throughout this process, neutral granulocytes may undergo apoptosis and be phagocytosed by foveolar cells (24).
The neutrophil recruitment is often attributed to the signaling of endogenous and bacterial chemoattractants (25). Furthermore, immune and epithelial cells participate in the immune response which triggered by H. pylori infection. Some types of inflammatory factors (such as IL-8, IL-1β and tumor necrosis factor-α(TNF-α)) are the major participants of the reaction. These factors can stimulate IL-8, causing neutrophil infiltration and the exacerbation of inflammation (26). Research has established that neutrophils activated by the water-soluble surface proteins of H. pylori (27). Furthermore, nicotinamide adenine dinucleotide phosphate (NADPH) oxidase subunits in H. pylori’s fatty polysaccharides within neutrophils can lead to an overwhelming generation of reactive oxygen species (ROS), thereby promoting inflammatory damage (28). In this process, the protein domain 3 of ARRDC3 facilitates the accumulation and migration of neutrophils in the gastric mucosa (29). Scientific investigations have found that neutrophil extracellular traps (NETs) can enhance more aggressive mesenchymal phenotypes, thereby contributing to GC progression both in vitro and in vivo. Targeting NETs holds promise as a potential therapeutic approach (30). Moreover, the neutrophil-to-lymphocyte ratio has emerged as a potential prognostic indicator for cancer, owing to its ease of acquisition in clinical settings (31). Preoperative NLR serves as an independent prognostic factor for GC patients, providing stratified prognostic value, especially in cases classified as AJCC stage III (32).
3.2 T Lymphocyte immune regulation in gastric precancerous lesions progression
The immune response of the human body to H. pylori is a multifaceted and constantly evolving process. During childhood, notable features include a significant increase in FoxP3+ Treg cells within the gastric mucosa, along with substantial elevations in the levels of Treg, Transforming growth factor beta 1 (TGF-β1), and IL-10. This pattern stands in stark contrast to the immune response observed in infected adults (33). In the case of adults, diverse scenarios unfold. The gastric mucosa exhibits a TH1 reaction and TH17 reaction, characterized by a reduction in TGF-β1 concentration and an upsurge in IFN-γ, IL-12P70, IL-17A, IL-23, and other cytokines. Of particular interest is the synergy between TGF-β1 and IL-6, which collaboratively promote the expression of IL-23, thereby enhancing the TH17 response. This unique immune reaction is intricately linked to the damage observed in gastric mucosal cells. Consequently, adults are more predisposed to the progression of GPL (34–36).
In patients with GC, specific subgroups of immune cells within tumor tissues are associated with prognosis. Tumors characterized by high expression of CD8 (+) cytotoxic T lymphocytes often correlate with a favorable prognosis. Conversely, the risen of Foxp3 (+)/CD8 (+) and Foxp3 (+)/CD4 (+) ratios may serve as indicators for a poorer prognosis (37).
3.3 Macrophages immune regulation in gastric precancerous lesions progression
Monocytes and macrophages initiate from bone marrow progenitor cells before entering the bloodstream. In response to inflammation, circulating monocytes respond to local growth factors by migrating into tissues, where they can differentiate into macrophages. Guided by chemotactic factors, tissue-resident macrophages then migrate to the site of tissue damage (38). In 1908, Elie Metchnikoff and Paul Ehrlich made pioneering observations concerning macrophages and their phagocytic activity. Macrophages play a pivotal role as the immune system’s first line of defense, contributing to the defense against infection by generating pro-inflammatory factors, including IL-1β (39). Activated macrophages serve as the primary source of growth factors and cytokines, exerting profound effects on local mucosal tissues and thus shaping the chronic inflammatory microenvironment (40). In cases of mild gastritis, macrophages infiltrate the stomach after parietal cell loss, promoting metaplasia progression (41). However, macrophages also exhibit specialization in response to local environmental cues, resulting in distinct gene expression profiles and functions across various organ systems (42). M2 macrophages, driven by Th2 cytokines, are characterized as anti-inflammatory tumor-associated inflammatory cells, which can detrimentally influence gastric tumors in GPL mice (43). Inflammatory process in gastric is associated with an increase of secretory activity of macrophages. This heightened M1 macrophage activation can exacerbate gastric inflammation and result in a reduced bacterial load (44). Studies have demonstrated that in mouse model tissues, M2 macrophages can promote cellular SPEM by inflammatory stimulation (41). Moreover, macrophages possess the capability to release cytokines and chemokines into the bloodstream, including IL-1β, TNF-α, IL-6, IFN-γ, and PGE2 (45), contributing to systemic chronic inflammation. It is widely believed that inflammation underlies gastric dysfunction. In GC, macrophage polarization transitions from the anti-inflammatory M1 state to the pro-inflammatory M2 state. Pathogens such as H. pylori can impede the M1 macrophage response, induce macrophage polarization into the M2 state, and increase ROS-induced macrophage apoptosis, thereby advancing the progression of GPL (44).
However, in a persistent inflammatory state, immune functions can become detrimental, leading to the production of mutagenic agents like peroxynitrite, which can react with DNA, promoting uncontrolled division of epithelial and stromal cells. Macrophage infiltration can release TNF-α, exacerbating DNA damage, potentially linked to IL-33 (43). Notably, H. pylori induces apoptosis in macrophages and is a strategy to escape the immune response. Phagocytosis of H. pylori triggers apoptosis in macrophages, releasing bacteria to infect the next cell (46, 47).
3.4 Fibroblasts immune regulation in gastric precancerous lesions progression
Stromal fibroblasts are pivotal contributors to the intricate web of chronic cancer-related inflammation and the initiation and progression of malignant diseases (48). Fibroblasts can produce IL-6, thereby inducing TNF, IL-17, IL-1β, LPS and IFNs (49). In addition, H. pylori increases caspases and sST2, causing deleterious effects on gastric barrier cells. Gastric epithelial cells and fibroblasts can upregulate type I collagen and repair early cell damage caused by H. pylori (50). Various immune cells circulate in the blood in response to specific environmental signals. When the gastric mucosa is damaged, these immune cells will be recruited to the damaged tissue, promote the formation of new blood vessels, and create an immunosuppressive environment. Studies have found that cancer-associated fibroblasts (CAFs) participate in ECM remodeling and promote angiogenesis, leading to the progression of GPL (51). CAFs are also capable of secreting miR-522 to suppress ferroptosis and bolster angiogenesis (52). Furthermore, CAFs-derived IL-8 amplifies the inflammatory response by activating signaling pathways such as nuclear factor kappa-B (NF-κB) (53). Additionally, H. pylori infection has been shown to induce the transformation of fibroblasts into myofibroblasts, elevating the early oncogenic marker HIF-1α (54). H. pylori-activated gastric fibroblasts are central to promoting the transition of normal gastric epithelial cells into a precancerous state, driving EMT through the regulation of TGFβ R1/R2-dependent signaling. In summary, H. pylori infection intensifies CAFs differentiation, subsequently promoting EMT through pathways involving NF-κB, STAT3, and TGF-β. Given the pivotal role of CAFs in the microenvironment of gastric, targeting CAFs emerges as a potential strategy for enhancing patient prognosis (53, 55).
The mechanism of GPL is correlated with neutrophils, T cells, macrophages and fibroblasts. As a key inflammatory factor, IL-8 interacts with different immune cells, triggering the release of more inflammatory factors and aggravating the cascade reaction of GPL.
4 Inflammatory molecules and microorganisms regulation in gastric precancerous lesions progression
In the progression of gastric precancerous lesions, the regulation of inflammatory molecules and microorganisms plays a crucial role. Several types of inflammatory molecules have been implicated in this process, including the gut microbiome, bile acid, and cytokines (Table 1). Understanding the intricate interplay between these components is crucial for comprehending the mechanisms underlying GPL and may pave the way for novel therapeutic interventions.
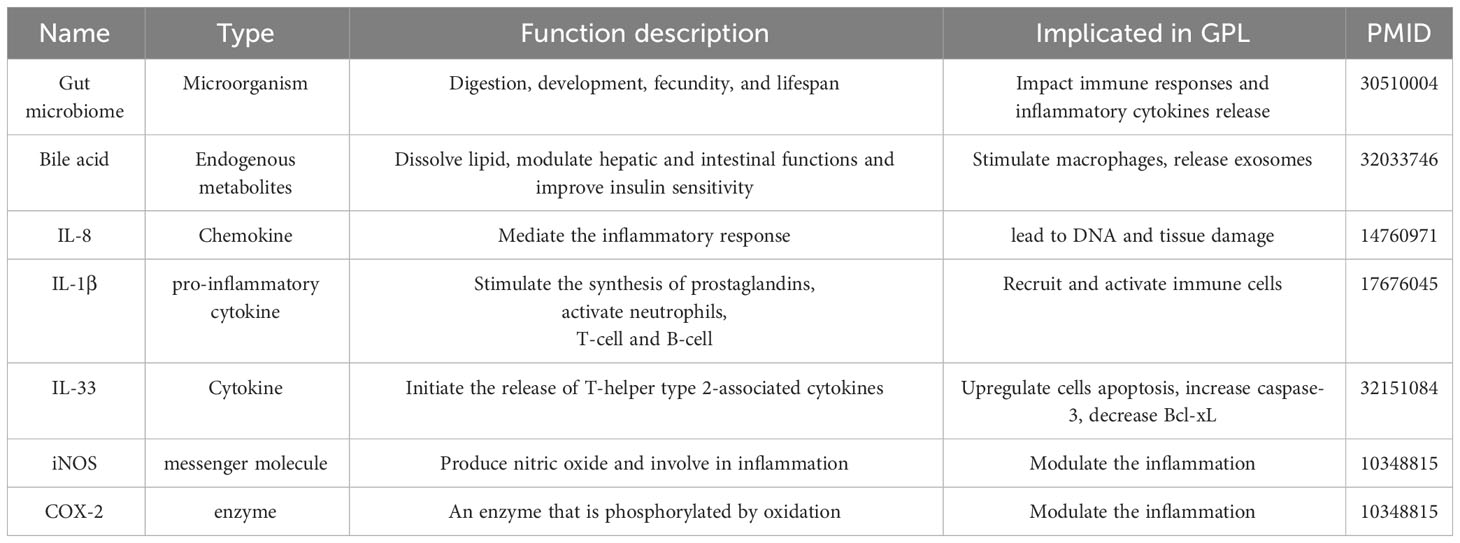
Table 1 Summary of inflammatory molecules and microorganisms implicated in gastric precancerous lesions.
4.1 Gut microbiome implicated in gastric precancerous lesions progression
The equilibrium of the intestinal microbiota is intricately intertwined with the host’s well-being. The presence of H. pylori can disrupt the balance of the intestinal flora, thereby fostering the advancement of GPL. This phenomenon is intricately linked to the persistent activation of the host’s immune system by the intestinal microbiota, which, in turn, results in localized chronic inflammation. On one hand, the intestinal flora is central to regulating anti-tumor immune responses, while, on the other hand, it can facilitate the generation of carcinogenic metabolites. An abnormal immune response can precipitate an imbalance in the intestinal flora, ultimately leading to an abnormal release of inflammatory factors (56). Therefore, elimination of H. pylori can correct intestinal flora disorder and have a healthy impact on the gastrointestinal tract (57). GPL progression is related to the abundance of H. pylori and other gastrointestinal flora. This suggests that intestinal dysbiosis has the potential to serve as a biomarker to differentiate between gastritis and GC (58, 59). This has been confirmed both in rat models and in human tissues, where the changes observed are very similar (60). One thing needs attention, although the infection abundance of H. pylori gradually increases in different stages of GPL, GC has less H. pylori, and the bacterial flora is dominated by oral and intestinal pathogenic microbial strains (61). Furthermore, IM patients are colonized with abundant oral bacterial genera, including Peptostreptococcus oralis, Neisseria elongatus, Johnsonella martensi, and Neisseria flavus (62). In addition, Acinetobacter may promote the development of intraepithelial neoplasia. Certain bacterial genera show a higher degree of centrality in the progression of GPL, such as gastric mucosal genera (including Gemini, Streptococcus, etc) (59). The gut microbiota and its metabolites may be central to the progression of GPL. Studies have shown that H. pylori can regulate gut microbiota. This regulate may include species changes in the microbiota, metabolites of the microbiota. And intestinal microbiota in turn can regulate the inflammatory immunity of gastric mucosa, resulting in the progression of GPL. This interaction between Helicobacter pylori and the gut microbiota can be referred to as the Helicobacter pylori-Gut microbiota Metabolism (HGM) axis (60, 63, 64).
4.2 Bile acid in gastric precancerous lesions progression
Bile acid reflux is a critical factor in the occurrence of gastrointestinal metaplasia (GIM), and this pathogenesis does not require the involvement of H. pylori (65, 66). The underlying mechanism involves deoxycholic acid stimulating macrophages to release exosomes encapsulating inflammatory factors (67). These exosomes, in turn, promote the overexpression of hsa-miR-30a-5p in gastric mucosal epithelial cells. Overexpression of this miRNA targets Forkhead Box D1 (FOXD1) and leads to the increased expression of CDX2, thereby promoting the development of intestinal metaplasia and GIM (68). The possible mechanisms of bile acid-induced gastritis have been documented, but the final substrates are all related to changes in miRNA and CDX2 substrates, which activate the expression of KLF4, cadherin 17, and HNF4α, leading to the progression of IM to GC (69–71).
4.3 Cytokines and inflammatory factors
The inflammatory microenvironment is central to the progression of GPL to GC. Cytokines and inflammatory factors are central to immune response and are associated with a multitude of pathological changes associated with GPL (Figure 2).
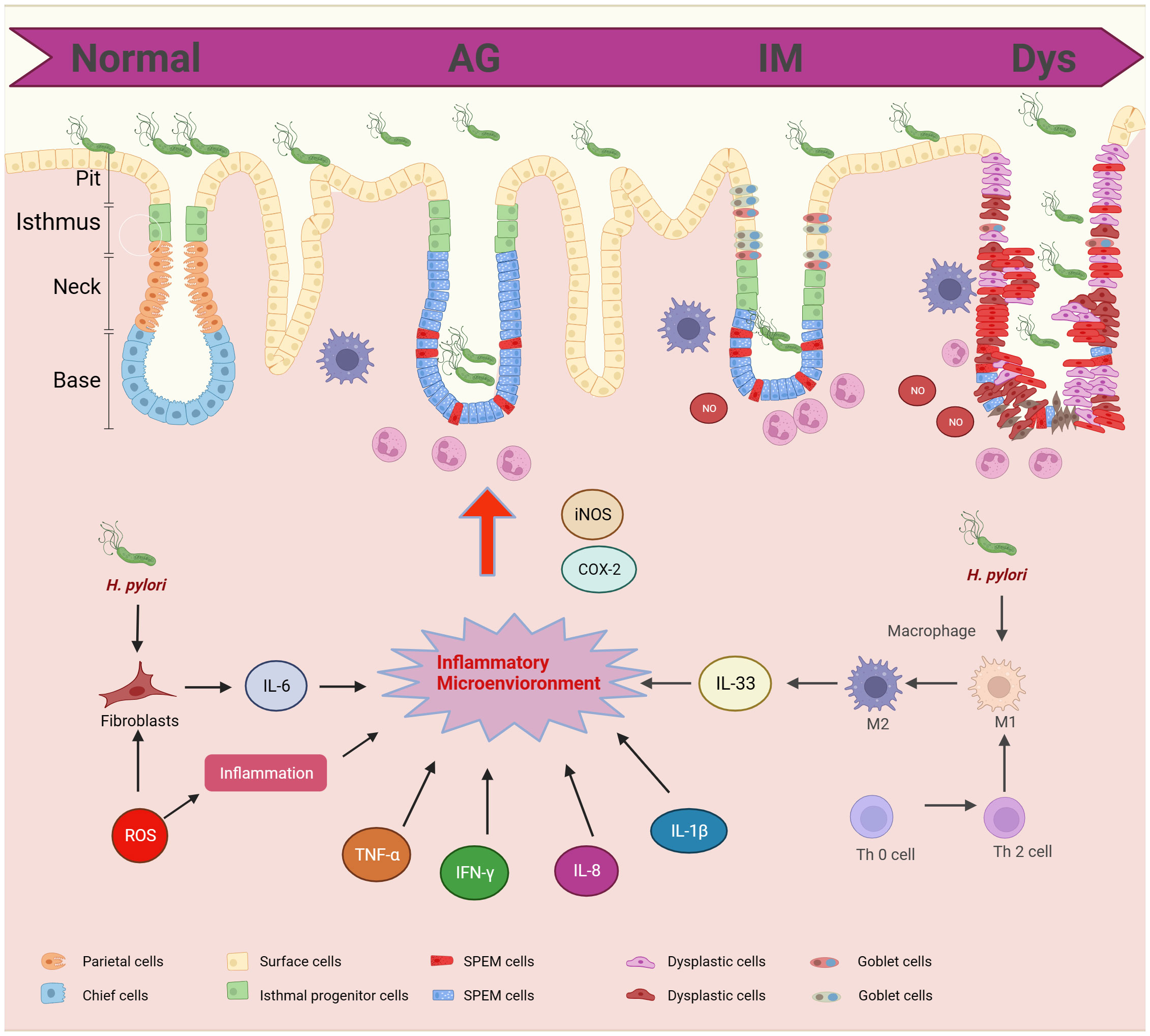
Figure 2 Cytokines and inflammatory factors in gastric precancerous lesions progression. GPL includes different pathological stages: AG, IM, and Dys. The pathological morphology is related to the inflammatory microenvironment. H.Pylori and T cells can promote the polarization of macrophages and induce the release of IL-33. Fibroblasts promote the release of IL-6 and ROS promotes the release of hormones. The inflammatory microenvironment promotes the infiltration of neutrophils into epithelial cells.
4.3.1 Interleukin-8 in gastric precancerous lesions progression
Interleukin-8 (IL-8), an important member of the CXC chemokine family, is a potent chemoattractant for neutrophils and lymphocytes and is critical to promoting gastric mucosal inflammation. IL-8 expression is significantly elevated in H. pylori-associated gastritis. IL-8 causes sustained overproduction of nitric oxide, which may induce DNA and tissue damage, thereby increasing the risk of neoplastic transformation (72). In histomorphology, IL-8 complements established predictors such as gastrin and pepsinogen A/C ratio (73). Highly expressed IL-8 can continuously infiltrate tissues and increase VEGF levels, leading to GPL (74).
4.3.2 IL-1β in gastric precancerous lesions progression
Gastric IL-1β is closely linked to high grade mucosal inflammation (75), and is critical to the progression of CAG to GC (76, 77). The IL-1β exhibits polymorphic characteristics that are significantly linked to gastric acid secretion and GPL (78). IL-1β may collaborate with other inflammatory cytokines, such as promoting the upregulation of IL-17A, recruiting and activating immune cells within the gastric mucosa, collectively inciting inflammation (79, 80). Furthermore, IL-1β has been demonstrated to establish a positive feedback loop, inducing the expression of IL-8. It is noteworthy that while gastritis occurrence is associated with IL-1β and IL-18, exhibiting a declining trend from chronic gastritis to GC (81).
4.3.3 Interleukin -33 in gastric precancerous lesions progression
Interleukin-33 (IL-33) is a recently characterized alarmin with high expression levels in the gastric mucosa, capable of potently activating Th2 immunity. after exposure to H. pylori, silencing IL-33 in GES-1 cells has been shown to lead to decrease cell metabolic activity, migration, adhesion and proliferation. Additionally, it resulted in an upregulation of cell apoptosis, marked by an increase in caspase-3 activity and a decrease in Bcl-xL expression. The findings suggest a proregenerative role of IL-33 (82). H. pylori infection can activate IL-33 pro-regenerative activity in apoptotic gastric tissue cells (83).
4.3.4 Inducible nitric oxide synthase and cyclooxygenase-2 in gastric precancerous lesions progression
In patients with gastritis, especially those infected with H. pylori, nitric oxide produced by inducible nitric oxide synthase(iNOS) and COX-2 is induced to regulate epithelial cell growth and inflammatory changes (84). Studies have underscored the significance of iNOS as an inflammation-inducing enzyme and a key contributing factor to gastritis (85). Furthermore, iNOS can bind to H. pylori and induce apoptosis in gastric mucosal. Study shown that iNOS-KO mice exhibited persistent inflammation but not apoptosis after H. pylori infection (86).
There are many other cellular inflammatory molecules involved in the progression of GPL, and their mechanisms of action still need to be further explored. The involvement of inflammatory molecules may be closely related to the mechanism of action of immune cells.
5 Inflammatory pathways and TCM intervention in the prevention and treatment of GPL
In the process of inflammatory stimulation of gastric mucosa leading to GPL, it is closely related to some inflammatory pathways, including classic pathways: MAPK, Wnt/β-catenin, JAK/STAT3 and PI3K/AKT/mTOR signaling pathway (87), and non-classic inflammatory pathways: the Hippo and Hedgehog signaling pathway (Figure 3). Traditional Chinese medicine (TCM) and TCM-derived natural products, known as antioxidant and anti-inflammatory properties, can interfere with the outcome of GPL by affecting the above pathways (Table 2).
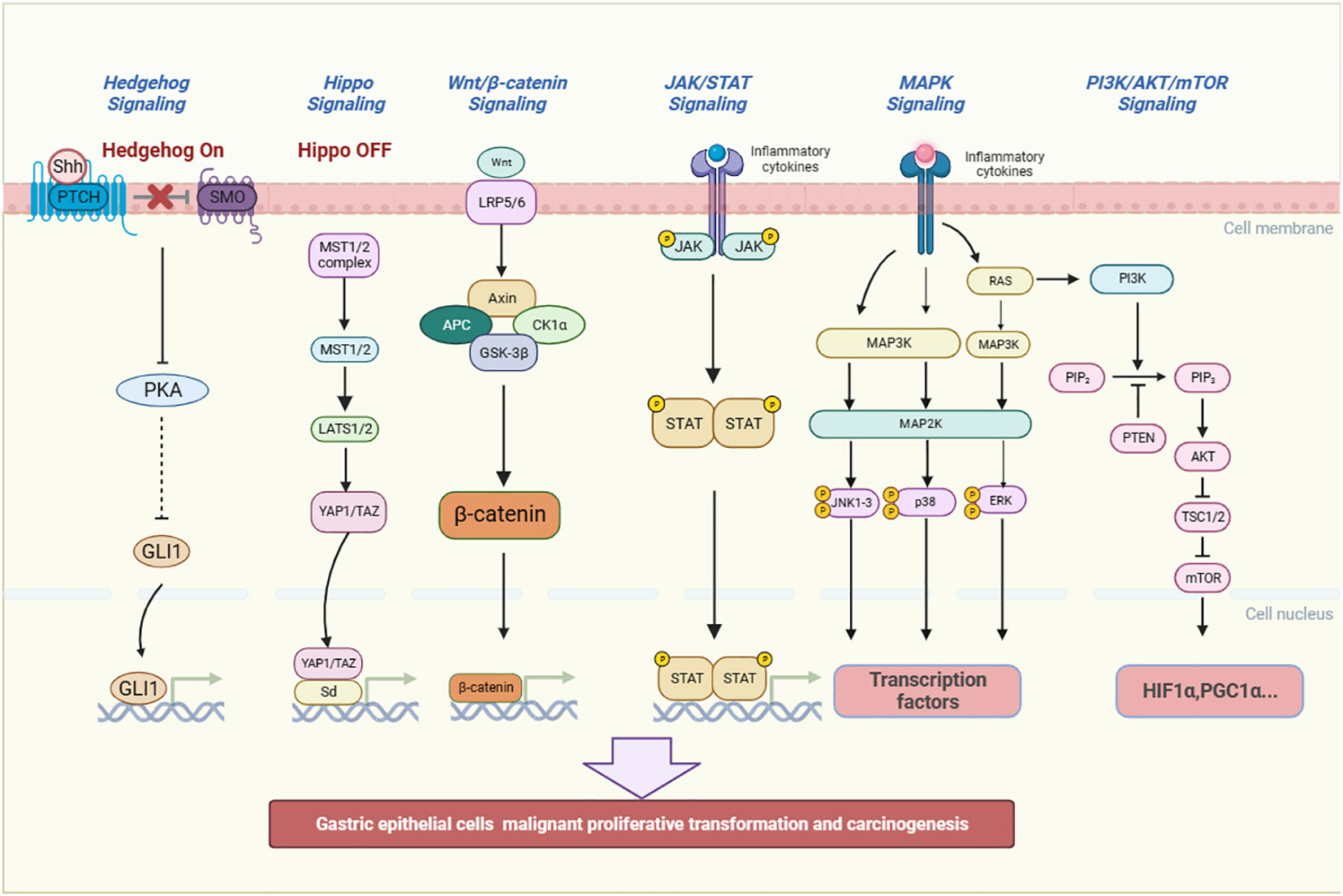
Figure 3 The inflammatory pathways related to the occurrence and development of GPL. They include MAPK, JAK/STAT3, PI3K/AKT/mTOR, the Hippo, Hedgehog and Wnt/β-catenin signaling pathway. The signaling pathways can promote gastric epithelial cells to undergo malignant proliferative transformation and carcinogenesis. (Shh, Sonic hedgehog, PTCH, Patched, SMO, Smoothened, PKA, Protein Kinase A, GLI1, glioma-associated oncogene homolog 1, MST, Mammalian Sterile 20-like kinase 1/2, LATS 1/2:large tumor suppressor kinase 1/2, YAP/TAZ, Yes-associated protein/transcriptional co-activator with PDZ-binding motif, Sd, Scalloped, Wnt, Wingless and INT-1, Axin1, Axis inhibition protein 1, APC, Adenomatous polyposis coli, CK1α:casein kinase 1α, GSK-3β:Glycogen synthase kinase 3β, JAK, Janus Kinase, STAT, Signal transducer and activator of transcription, RAS, Reliability, Availability and Serviceability, MAPK, Mitogen-activated protein kinase, JNK, c-Jun N-terminal kinase, p38:Peroxidase 38, ERK, Extracellular regulated protein kinases, PI3K:Phosphatidylinositol-3-kinase, PIP2:Phosphatidylinositol(4,5)bisphosphate, PTEN, Phosphatase and tensin homolog, AKT, Protein kinase B, TSC1/2:Tuberous sclerosis 1/2, mTOR, mammalian target of rapamycin, HIF1α:Hypoxia-inducible factor-1α, PGC1α, Peroxisome proliferator-activated receptor-γ coactivator 1α).
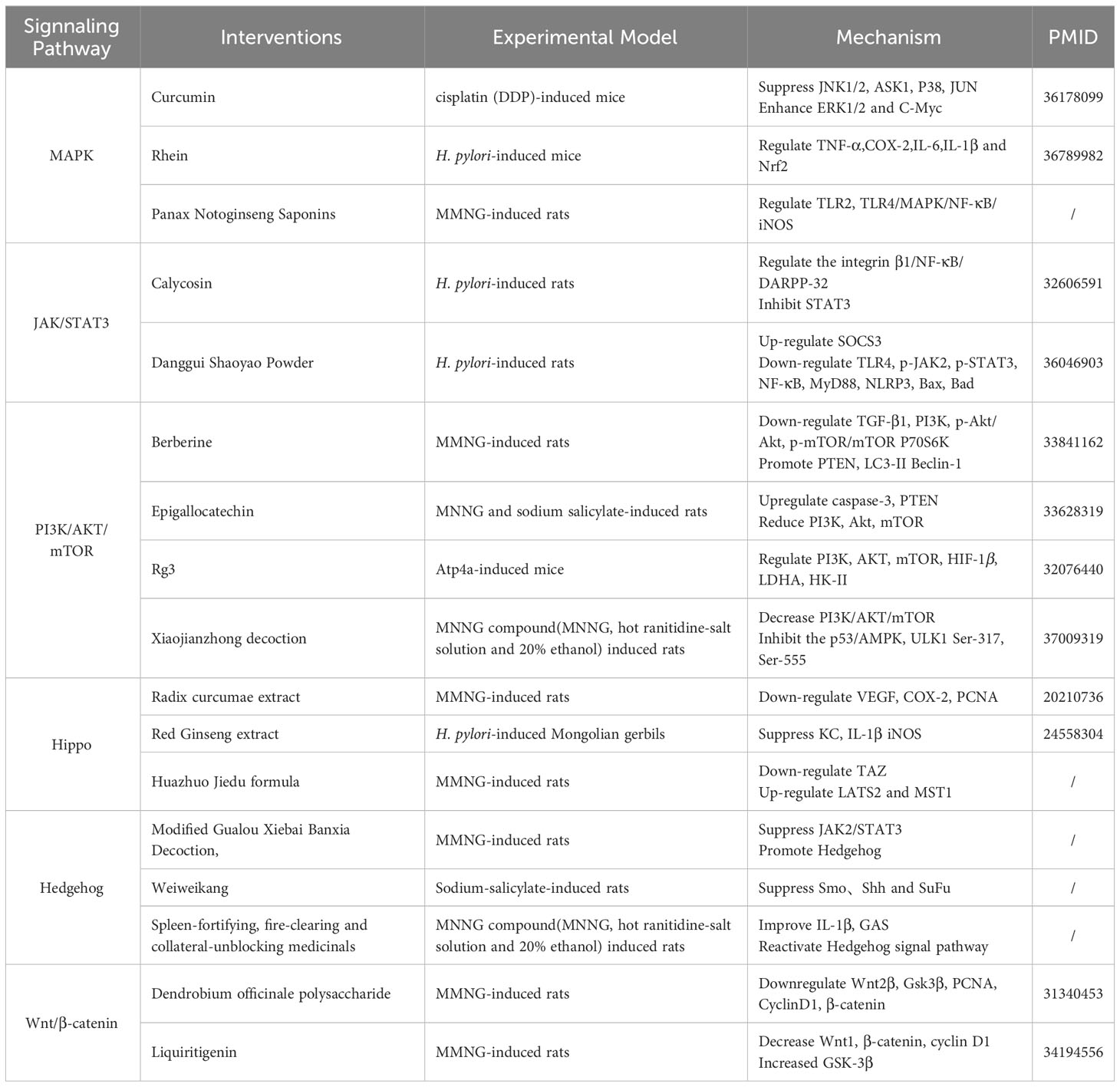
Table 2 Summary of mechanism of traditional Chinese medicine targeting inflammatory pathways in the Prevention and Treatment of GPL.
5.1 MAPK signaling pathway
The activation of MAPK signaling pathways involves three main components: MAP3K, MAP2K, and MAPK. MAPK contains P38, JNK, ERK. Once MAPKs are activated, they go on to stimulate various substrate proteins, thereby regulating a wide range of cellular activities (88). The p38 signal regulates the activation of ROS and EMT. ROS, in turn, can activate EGFR, thereby initiating the Ras/MAPK pathway and participating in activating NF-κB and COX-2. Ultimately, this intricate signaling cascade promotes the progression of GPL cell canceration (89). Curcumin, a phenolic compound renowned for its robust antioxidant properties, effectively mitigates cisplatin-induced inflammation and apoptosis in the gastric mucosa by modulating the NF-κB and MAPKs signaling pathways (90). Rhein exerts anti-inflammatory and antioxidant effects in CAG. It can improve the mouse model of CAG infected with H.pylori by inhibiting inflammation and oxidative stress. The repair of gastric mucosal damage is achieved through the activation of Nrf2 and MAPK signaling (91). Panax Notoginseng Saponins have the ability to stimulate the JNK signaling pathway, trigger apoptosis, suppress inflammatory responses, slow down the malignant progression of gastric mucosa, and provide protective benefits for the gastric mucosa (92).
5.2 JAK/STATs signaling pathway
In the JAK/STATs pathway, H.pylori elevates levels of inflammatory factors such as IL-6, stimulate the excessive activation of JAK/STATs signaling, leading to the progression of GPL. Once activated, JAKs proceed to phosphorylate the primary substrate Signal Transducers and Activator of Transcription (STATs). Subsequently, STATs molecules form dimers, which are subsequently transported to the nucleus. Within the nucleus, these dimers bind to specific regulatory sequences, thereby regulating the transcription of target genes. One such example of target genes is the Suppressor of Cytokine Signaling (SOCS) family, which can be activated or suppressed by this intricate signaling pathway, leading to the progression of GPL (93, 94). Normally, interferon gamma activates JAK/STATs to participate in the immune response. In the early stages of H. pylori infection, cholesterol in the gastric epithelial cells can be consumed, preventing interferon gamma signaling from activating the JAK/STAT signaling pathway and thus evading the immune response (95). However, in the progression of GPL to gastric cancer, STATs increased with the cascade of GPL to gastric cancer, which possible mechanism is the activation of PD-L1, which led to the progression of gastric cancer (93). Calycosin is a flavonoid derived from the root of Astragalus membranaceus, known for its antioxidant and anti-inflammatory properties. It prevents gastric mucosal damage in MNNG-induced GPL rats by inhibiting STAT3 expression in GPL (96). Danggui Shaoyao Powder has been found to up-regulate SOCS3 protein levels, down-regulate TLR4, p-JAK2, p-STAT3 and NF-κB protein levels, and reduce gastric mucosal atrophy in rats (97).
5.3 PI3K/AKT/mTOR signaling pathway
The PI3K/AKT/mTOR signaling pathway is closely linked to apoptosis and autophagy (98). H.pylori and inflammatory factors trigger RAS, subsequently stimulating downstream PI3K, in turn, facilitates the activation of AKT through phosphorylation and is drawn to the cell membrane by AKT. This activates mTOR complex 1 (mTORC1). The effectors of mTORC1, including proteins like HIF1a and PGC-1a, play a pivotal role in regulating various cellular functions associated with oncogenic phenotypes (99). Several natural antioxidants have been reported to be effective for GPL via regulation of this signaling pathway. For example, berberine has been shown to down-regulate TGF-β1, PI3K/AKT/mTOR signaling, and P70S6K, while promoting PTEN, LC3-II, and Beclin-1, ultimately leading to an improvement in CAG (100). Epigallocatechin Gallate (EGCG), a natural antioxidant abundant in tea, has been found to improve GPL (101). Moreover, Ginsenoside Rg3, a natural compound found in Ginseng, has gained attention for anti-inflammatory, antioxidant, and anticancer properties. Ginsenoside Rg3 has been demonstrated to regulate PI3K/AKT/mTOR and HIF-1α. Ginsenoside Rg3 can be used to induce apoptosis and treat GPL (102). Xiaojianzhong decoction has been shown to reduce gastric mucosal hypoxia, regulate the PI3K/AKT/mTOR pathway to improve GPL (103).
5.4 The Hippo pathway
In the signal cascade of the Hippo pathway, there exist two states known as “Hippo on” and “Hippo off.” In the “Hippo on” state, the transcription factors, YAP/TAZ can associate with the 14-3-3 protein complex and become sequestered in the cytoplasm, ultimately undergoing degradation via ubiquitination. However, in the “Hippo off” state, YAP/TAZ cannot bind to the 14-3-3 complex, enabling their entry into the nucleus where they participate in complex formation, regulate the downstream target (104). The Hippo is link to inflammatory endothelial injury. Study has found Red Ginseng extract has also been found to inhibit IL-1β and iNOS associated with H.pylori infection, suppress the phosphorylation of IκBα, and reduce the increase of gastric mucosal LPO level and MPO activity, thereby delaying the evolution of GPL (105). Additionally, radix curcumae extract has been shown inhibit VEGF, COX-2, offering a potential treatment for GPL (106). Huazhuo Jiedu formula has been shown to down-regulate the expression of Hippo/TAZ signaling pathway and its related protein transcriptional coactivators PDZ-binding motif (TAZ), tumor suppressor kinase (LATS2), and mammalian sterile line 20-like kinase (MST1) levels in gastric mucosa tissue, thereby improving chronic atrophic gastritis (107).
5.5 Hedgehog signaling pathway
Hedgehog signaling starts with by various Hedgehog ligands, such as Shh, Ihh, and Dhh, which bind to membrane-bound receptor known as Patched. Interestingly, Patched receptors have shown to promote the progression of GC (108). Inflammation can accelerate the expression of the Hedgehog signaling pathway and induce IFNα, which regulates the level of SLFN4 and leads to atrophic gastritis in infected gastric mucosa (109). Modified Gualou Xiebai Banxia Decoction, a traditional Chinese medicine prescription, promotes the Hedgehog pathway to improve the inflammatory activity in rats with CAG (110). The Chinese patent medicine Weiweikang can regulate the levels of Smo, Shh, and SuFu proteins in the gastric mucosa of rats, which improves the inflammatory changes in the gastric mucosa and treats GPL (111). Spleen-fortifying, fire-clearing and collateral-unblocking medicinals affects the up-regulation of Shh, Ptch1, Smo, and Gli1 protein expression, the down-regulation of Gli2, Gli3, and Sufu protein expression, reduces serum IL-1β levels, and improves the pathological changes in CAG rats (112).
5.6 Wnt/β-catenin pathway
In the Wnt/β-catenin pathway, H.pylori infection activates the levels of Wnt pathway-related proteins as gastric disease progresses (113). Under the action of Wnt ligands, CK1α, Axin, GSKβ and LRP5/6 are recruited to form a complex. This results in an increase in large amounts of free beta-catenin, which increases the progression of gastric disease (114). Traditional Chinese medicine can intervene GPL by regulating the Wnt/β-catenin pathway. Dendrobium officinale polysaccharide can reduce Wnt2β and β-catenin to inhibit the progression of GPL (115). Liquiritigenin and hesperidin in Jianpiyiqi formula can improve mucosal atrophy and inflammation and help treat GC by down-regulating Wnt1, β-catenin and up-regulating GSK-3β (116).
6 Challenges and future perspectives
The inflammatory microenvironment in GPL has shed light on the crucial part of inflammation in the progression of GC. However, several challenges need to be addressed to achieve a comprehensive understanding of this complex process and develop effective prevention and treatment strategies. Here we discuss some of the key challenges and propose future perspectives in this field.
One major challenge in studying the inflammatory microenvironment in GPL is the heterogeneity of these lesions. Though GPL provides a critical stage for clinical intervention of GC, GPL can vary in terms of histopathological features, molecular alterations, and inflammatory cell infiltration patterns. It is central to uncover the underlying mechanisms driving this heterogeneity and identifying specific biomarkers for GPL. Nowadays, application of single-cell sequencing and imaging methods to explore the cellular heterogeneity within GPL, will shed light on unraveling distinct subpopulations of inflammatory cells, and understanding their functional diversity and interaction patterns (117).
Another big challenge lies in deciphering the intricate interplay between immune cells and inflammation in GPL progression. Immune cells and cytokines play an important role in the transformation of GPL. Their interactions and communication, along with their specific contributions to GPL development, remain unclear. It is also necessary to delineate the dynamic interactions between immune cells and the inflammatory microenvironment, which may provide novel targets for immunotherapeutic approaches (118).
The inflammatory microenvironments are common pathological features that drive the development of multiple chronic diseases such as cancer. In GPL, abnormal activation of inflammatory microenvironment has been shown to be link to the progression of the disease. However, it is important to recognize that the inflammatory microenvironment consists of a complex network of cells, molecules, and signaling pathways. Targeting inflammatory microenvironment by chemical interventions may disrupt the delicate balance necessary for normal physiological functions, leading to potential side effects and toxicity. Thus, the proper target should be rigorously validation in vivo before implementing any intervention strategies (119).
Traditional Chinese medicine and its active components have demonstrated distinct advantages in influencing the release of inflammatory factors and treating GPL (120). However, the underlying mechanisms how they affect the inflammatory microenvironment to prevent GPL, along with their true effect in GPL clinical trials, remain unclear. In the future, investigating the molecular mechanisms how TCM interventions modulate inflammatory microenvironment and exploring their synergistic effects via drug combinations, will pave the way for the integration of TCM into clinical practice.
Collectively, the challenges presented necessitate further research efforts. By addressing these challenges and exploring the proposed future perspectives, we can advance our understanding of the inflammatory microenvironment in GPL and develop effective strategies for the prevention and treatment of GPL.
Author contributions
SZ: Writing – original draft. YS: Writing – original draft. HL: Writing – original draft. DZ: Writing – original draft. JF: Writing – review & editing. HP: Writing – review & editing. WL: Funding acquisition, Writing – review & editing.
Funding
The author(s) declare financial support was received for the research, authorship, and/or publication of this article. National Natural Science Foundation of China (No.82374343, No.82004300), Basic and Applied Basic Research Foundation of Guangdong Province (No.2023A1515011107), Guangzhou Health Science and Technology Project (No. 20222A011025), Medical Science and Technology Research Fund of Guangdong Province (B2023168), Basic Research Program of Guangzhou Science and Technology Bureau (No.2023A04J1169), The General Program of Open Project of Joint Construction of State Key Laboratory for Dampness Syndrome of Traditional Chinese Medicine by Guangdong Province and Ministry of Science and Technology(No.SZ2022KF17), Guangdong University Research Platform and Projects-Key Field Projects (No. 2023ZDZX2013), Open Bidding for Selecting the Best Teams in Guangzhou University of Chinese Medicine (A1-2601-23-414-110Z77), National Natural Science Foundation of China (No.81973816), Innovation Team and Talents Cultivation Program of National Administration of Traditional Chinese Medicine (No: ZYYCXTD-C-202208).
Conflict of interest
The authors declare that the research was conducted in the absence of any commercial or financial relationships that could be construed as a potential conflict of interest.
The author(s) declared that they were an editorial board member of Frontiers, at the time of submission. This had no impact on the peer review process and the final decision.
Publisher’s note
All claims expressed in this article are solely those of the authors and do not necessarily represent those of their affiliated organizations, or those of the publisher, the editors and the reviewers. Any product that may be evaluated in this article, or claim that may be made by its manufacturer, is not guaranteed or endorsed by the publisher.
References
1. Smyth EC, Nilsson M, Grabsch HI, van Grieken NC, Lordick F. Gastric cancer. Lancet (2020) 396:635–48. doi: 10.1016/S0140-6736(20)31288-5
2. Sung H, Ferlay J, Siegel RL, Laversanne M, Soerjomataram I, Jemal A, et al. Global cancer statistics 2020: GLOBOCAN estimates of incidence and mortality worldwide for 36 cancers in 185 countries. CA Cancer J Clin (2021) 71:209–49. doi: 10.3322/caac.21660
3. Bray F, Ferlay J, Soerjomataram I, Siegel RL, Torre LA, Jemal A. Global cancer statistics 2018: GLOBOCAN estimates of incidence and mortality worldwide for 36 cancers in 185 countries. CA Cancer J Clin (2018) 68:394–424. doi: 10.3322/caac.21492
4. Yuasa N, Nimura Y. Survival after surgical treatment of early gastric cancer, surgical techniques, and long-term survival. Langenbecks Arch Surg (2005) 390:286–93. doi: 10.1007/s00423-004-0482-y
5. You WC, Li JY, Blot WJ, Chang YS, Jin ML, Gail MH, et al. Evolution of precancerous lesions in a rural Chinese population at high risk of gastric cancer. Int J Cancer (1999) 83:615–9. doi: 10.1002/(SICI)1097-0215(19991126)83:5<615::AID-IJC8>3.0.CO;2-L
6. de Vries AC, van Grieken NC, Looman CW, Casparie MK, de Vries E, Meijer GA, et al. Gastric cancer risk in patients with premalignant gastric lesions: a nationwide cohort study in the Netherlands. Gastroenterology (2008) 134:945–52. doi: 10.1053/j.gastro.2008.01.071
7. Halldórsdóttir AM, Sigurdardóttrir M, Jónasson JG, Oddsdóttir M, Magnússon J, Lee JR, et al. Spasmolytic polypeptide-expressing metaplasia (SPEM) associated with gastric cancer in Iceland. Dig Dis Sci (2003) 48:431–41. doi: 10.1023/A:1022564027468
8. Balkwill F, Mantovani A. Inflammation and cancer: back to Virchow. Lancet (2001) 357(9255):539–45. doi: 10.1016/S0140-6736(00)04046-0
9. Diakos CI, Charles KA, McMillan DC, Clarke SJ. Cancer-related inflammation and treatment effectiveness. Lancet Oncol (2014) 15:e493–503. doi: 10.1016/S1470-2045(14)70263-3
10. Marshall BJ, Warren JR. Unidentified curved bacilli in the stomach of patients with gastritis and peptic ulceration. Lancet (1984) 1(8390):1311–5. doi: 10.1016/S0140-6736(84)91816-6
11. Perillo M, Becker-Weidman D, Bezuidenhout AF, Siewert B, Eisenberg RL. Infections of the gastrointestinal tract. Semin Roentgenol (2017) 52(2):63–72. doi: 10.1053/j.ro.2017.04.009
12. Honda S, Fujioka T, Tokieda M, Satoh R, Nishizono A, Nasu M. Development of Helicobacter pylori-induced gastric carcinoma in Mongolian gerbils. Cancer Res (1998) 58(19):4255–9.
13. Goldenring JR. Pyloric metaplasia, pseudopyloric metaplasia, ulcer-associated cell lineage and spasmolytic polypeptide-expressing metaplasia: reparative lineages in the gastrointestinal mucosa. J Pathol (2018) 245(2):132–7. doi: 10.1002/path.5066
14. Ieni A, Barresi V, Rigoli L, Fedele F, Tuccari G, Caruso RA. Morphological and cellular features of innate immune reaction in helicobacter pylori gastritis: A brief review. Int J Mol Sci (2016) 17(1):109. doi: 10.3390/ijms17010109
15. Itzkowitz SH, Yio X. Inflammation and cancer IV. Colorectal cancer in inflammatory bowel disease: the role of inflammation. Am J Physiol Gastrointest Liver Physiol (2004) 287(1):G7–17. doi: 10.1152/ajpgi.00079.2004
16. Nasr R, Shamseddine A, Mukherji D, Nassar F, Temraz S. The crosstalk between microbiome and immune response in gastric cancer. Int J Mol Sci (2020) 21(18):6586. doi: 10.3390/ijms21186586
17. Coussens LM, Werb Z. Inflammation and cancer. Nature (2002) 420(6917):860–7. doi: 10.1038/nature01322
18. Frick A, Khare V, Paul G, Lang M, Ferk F, Knasmüller S, et al. Overt increase of oxidative stress and DNA damage in murine and human colitis and colitis-associated neoplasia. Mol Cancer Res (2018) 16(4):634–42. doi: 10.1158/1541-7786.MCR-17-0451
19. Goldenring JR, Mills JC. Cellular plasticity, reprogramming, and regeneration: metaplasia in the stomach and beyond. Gastroenterology (2022) 162(2):415–30. doi: 10.1053/j.gastro.2021.10.036
20. Jeong HY, Ham IH, Lee SH, Ryu D, Son SY, Han SU, et al. Spatially distinct reprogramming of the tumor microenvironment based on tumor invasion in diffuse-type gastric cancers. Clin Cancer Res (2021) 27(23):6529–42. doi: 10.1158/1078-0432.CCR-21-0792
21. Allen LA. Modulating phagocyte activation: the pros and cons of Helicobacter pylori virulence factors. J Exp Med (2000) 191(9):1451–4. doi: 10.1084/jem.191.9.1451
22. Dixon MF, Genta RM, Yardley JH, Correa P. Classification and grading of gastritis. The updated Sydney System. International Workshop on the Histopathology of Gastritis, Houston 1994. Am J Surg Pathol (1996) 20(10):1161–81. doi: 10.1097/00000478-199610000-00001
23. Lee I. Critical pathogenic steps to high risk Helicobacter pylori gastritis and gastric carcinogenesis. World J Gastroenterol (2014) 20(21):6412–9. doi: 10.3748/wjg.v20.i21.6412
24. Caruso RA, Fedele F, Di Bella C, Mazzon E, Rigoli L. Foveolar cells phagocytose apoptotic neutrophils in chronic active Helicobacter pylori gastritis. Virchows Arch (2012) 461(5):489–94. doi: 10.1007/s00428-012-1308-x
25. Heit B, Tavener S, Raharjo E, Kubes P. An intracellular signaling hierarchy determines direction of migration in opposing chemotactic gradients. J Cell Biol (2002) 159(1):91–102. doi: 10.1083/jcb.200202114
26. Ren WK, Xu YF, Wei WH, Huang P, Lian DW, Fu LJ, et al. Effect of patchouli alcohol on Helicobacter pylori-induced neutrophil recruitment and activation. Int Immunopharmacol (2019) 68:7–16. doi: 10.1016/j.intimp.2018.12.044
27. Unemo M, Aspholm-Hurtig M, Ilver D, Bergström J, Borén T, Danielsson D, et al. The sialic acid binding SabA adhesin of Helicobacter pylori is essential for nonopsonic activation of human neutrophils. J Biol Chem (2005) 280(15):15390–7. doi: 10.1074/jbc.M412725200
28. Teshima S, Tsunawaki S, Rokutan K. Helicobacter pylori lipopolysaccharide enhances the expression of NADPH oxidase components in cultured Guinea pig gastric mucosal cells. FEBS Lett (1999) 452(3):243–6. doi: 10.1016/S0014-5793(99)00636-5
29. Liu YG, Teng YS, Shan ZG, Cheng P, Hao CJ, Lv YP, et al. Arrestin domain containing 3 promotes Helicobacter pylori-associated gastritis by regulating protease-activated receptor 1. JCI Insight (2020) 5(15):e135849. doi: 10.1172/jci.insight.135849
30. Zhu T, Zou X, Yang C, Li L, Wang B, Li R, et al. Neutrophil extracellular traps promote gastric cancer metastasis by inducing epithelial−mesenchymal transition. Int J Mol Med (2021) 48(1):127. doi: 10.3892/ijmm.2021.4960
31. Zahorec R. Ratio of neutrophil to lymphocyte counts–rapid and simple parameter of systemic inflammation and stress in critically ill. Bratisl Lek Listy (2001) 102(1):5–14.
32. Han QY, Zhang X, Zhang JG, Zhou WJ, Chen QY, Chen YY, et al. Pre-operative neutrophil-to-lymphocyte ratio is an independent prognostic factor in patients with gastric cancer. Int Immunopharmacol (2022) 113(Pt A):109371. doi: 10.1016/j.intimp.2022.109371
33. Araújo G, Marques HS, Santos M, da Silva F, da Brito BB, Correa Santos GL, et al. Helicobacter pylori infection: How does age influence the inflammatory pattern. World J Gastroenterol (2022) 28(4):402–11. doi: 10.3748/wjg.v28.i4.402
34. Freire de Melo F, Rocha AM, Rocha GA, Pedroso SH, de Assis Batista S, Fonseca de Castro LP, et al. A regulatory instead of an IL-17 T response predominates in Helicobacter pylori-associated gastritis in children. Microbes Infect (2012) 14(4):341–7. doi: 10.1016/j.micinf.2011.11.008
35. Freire de Melo F, Rocha GA, Rocha AM, Teixeira KN, Pedroso SH, Pereira Junior JB, et al. Th1 immune response to H. pylori infection varies according to the age of the patients and influences the gastric inflammatory patterns. . Int J Med Microbiol (2014) 304(3-4):300–6. doi: 10.1016/j.ijmm.2013.11.001
36. Razavi A, Bagheri N, Azadegan-Dehkordi F, Shirzad M, Rahimian G, Rafieian-Kopaei M, et al. Comparative immune response in children and adults with H. pylori infection. J Immunol Res (2015) 2015:315957. doi: 10.1155/2015/315957
37. Chang WJ, Du Y, Zhao X, Ma LY, Cao GW. Inflammation-related factors predicting prognosis of gastric cancer. World J Gastroenterol (2014) 20(16):4586–96. doi: 10.3748/wjg.v20.i16.4586
38. Epelman S, Lavine KJ, Randolph GJ. Origin and functions of tissue macrophages. Immunity (2014) 41:21–35. doi: 10.1016/j.immuni.2014.06.013
39. Qiu D, Chu X, Hua L, Yang Y, Li K, Han Y, et al. Gpr174-deficient regulatory T cells decrease cytokine storm in septic mice. Cell Death Dis (2019) 10(3):233. doi: 10.1038/s41419-019-1462-z
40. Maeda H, Akaike T. Nitric oxide and oxygen radicals in infection, inflammation, and cancer. Biochem (Mosc) (1998) 63(7):854–65. doi: 10.1007/978-1-4615-5081-5_18
41. Petersen CP, Weis VG, Nam KT, Sousa JF, Fingleton B, Goldenring JR. Macrophages promote progression of spasmolytic polypeptide-expressing metaplasia after acute loss of parietal cells. Gastroenterology (2014) 146(7):1727–38.e8. doi: 10.1053/j.gastro.2014.02.007
42. Gautier EL, Shay T, Miller J, Greter M, Jakubzick C, Ivanov S, et al. Gene-expression profiles and transcriptional regulatory pathways that underlie the identity and diversity of mouse tissue macrophages. Nat Immunol (2012) 13(11):1118–28. doi: 10.1038/ni.2419
43. Petersen CP, Meyer AR, De Salvo C, Choi E, Schlegel C, Petersen A, et al. A signalling cascade of IL-33 to IL-13 regulates metaplasia in the mouse stomach. Gut (2018) 67(5):805–17. doi: 10.1136/gutjnl-2016-312779
44. Hardbower DM, Asim M, Murray-Stewart T, Casero RA Jr, Verriere T, Lewis ND, et al. Arginase 2 deletion leads to enhanced M1 macrophage activation and upregulated polyamine metabolism in response to Helicobacter pylori infection. Amino Acids (2016) 48(10):2375–88. doi: 10.1007/s00726-016-2231-2
45. Hart PH, Cooper RL, Finlay-Jones JJ. IL-4 suppresses IL-1 beta, TNF-alpha and PGE2 production by human peritoneal macrophages. Immunology (1991) 72(3):344–9.
46. Menaker RJ, Ceponis PJ, Jones NL. Helicobacter pylori induces apoptosis of macrophages in association with alterations in the mitochondrial pathway. Infect Immun (2004) 72(5):2889–98. doi: 10.1128/IAI.72.5.2889-2898.2004
47. Schwartz JT, Allen LA. Role of urease in megasome formation and Helicobacter pylori survival in macrophages. J Leukoc Biol (2006) 79(6):1214–25. doi: 10.1189/jlb.0106030
48. Shen XJ, Zhang H, Tang GS, Wang XD, Zheng R, Wang Y, et al. Caveolin-1 is a modulator of fibroblast activation and a potential biomarker for gastric cancer. Int J Biol Sci (2015) 11(4):370–9. doi: 10.7150/ijbs.10666
49. Nguyen HN, Noss EH, Mizoguchi F, Huppertz C, Wei KS, Watts G, et al. Autocrine loop involving IL-6 family member LIF, LIF receptor, and STAT4 drives sustained fibroblast production of inflammatory mediators. Immunity (2017) 46(2):220–32. doi: 10.1016/j.immuni.2017.01.004
50. Gonciarz W, Krupa A, Moran AP, Tomaszewska A, Chmiela M. Interference of LPS H. pylori with IL-33-Driven Regeneration of Caviae porcellus Primary Gastric Epithelial Cells and Fibroblasts. Cells (2021) 10(6):1385. doi: 10.3390/cells10061385
51. Sahai E, Astsaturov I, Cukierman E, DeNardo DG, Egeblad M, Evans RM, et al. A framework for advancing our understanding of cancer-associated fibroblasts. Nat Rev Cancer (2020) 20(3):174–86. doi: 10.1038/s41568-019-0238-1
52. Zhang H, Deng T, Liu R, Ning T, Yang H, Liu D, et al. CAF secreted miR-522 suppresses ferroptosis and promotes acquired chemo-resistance in gastric cancer. Mol Cancer (2020) 19(1):43. doi: 10.1186/s12943-020-01168-8
53. Zhai J, Shen J, Xie G, Wu J, He M, Gao L, et al. Cancer-associated fibroblasts-derived IL-8 mediates resistance to cisplatin in human gastric cancer. Cancer Lett (2019) 454:37–43. doi: 10.1016/j.canlet.2019.04.002
54. Krzysiek-Maczka G, Targosz A, Ptak-Belowska A, Korbut E, Szczyrk U, Strzalka M, et al. Molecular alterations in fibroblasts exposed to Helicobacter pylori: a missing link in bacterial inflammation progressing into gastric carcinogenesis. J Physiol Pharmacol (2013) 64(1):77–87.
55. Fujii S, Fujihara A, Natori K, Abe A, Kuboki Y, Higuchi Y, et al. TEM1 expression in cancer-associated fibroblasts is correlated with a poor prognosis in patients with gastric cancer. Cancer Med (2015) 4(11):1667–78. doi: 10.1002/cam4.515
56. Guo Y, Zhang Y, Gerhard M, Gao JJ, Mejias-Luque R, Zhang L, et al. Effect of Helicobacter pylori on gastrointestinal microbiota: a population-based study in Linqu, a high-risk area of gastric cancer. Gut (2020) 69:1598–607. doi: 10.1136/gutjnl-2019-319696
57. Mowat C, Williams C, Gillen D, Hossack M, Gilmour D, Carswell A, et al. Omeprazole, Helicobacter pylori status, and alterations in the intragastric milieu facilitating bacterial N-nitrosation. Gastroenterology (2000) 119:339–47. doi: 10.1053/gast.2000.9367
58. Ferreira RM, Pereira-Marques J, Pinto-Ribeiro I, Costa JL, Carneiro F, MaChado JC, et al. Gastric microbial community profiling reveals a dysbiotic cancer-associated microbiota. Gut (2018) 67:226–36. doi: 10.1136/gutjnl-2017-314205
59. Liu D, Chen S, Gou Y, Yu W, Zhou H, Zhang R, et al. Gastrointestinal microbiota changes in patients with gastric precancerous lesions. Front Cell Infect Microbiol (2021) 11:749207. doi: 10.3389/fcimb.2021.749207
60. Chu F, Li Y, Meng X, Li Y, Li T, Zhai M, et al. Gut microbial dysbiosis and changes in fecal metabolic phenotype in precancerous lesions of gastric cancer induced with N-methyl-N'-nitro-N-nitrosoguanidine, sodium salicylate, ranitidine, and irregular diet. Front Physiol (2021) 12:733979. doi: 10.3389/fphys.2021.733979
61. Liatsos C, Papaefthymiou A, Kyriakos N, Galanopoulos M, Doulberis M, Giakoumis M, et al. Helicobacter pylori, gastric microbiota and gastric cancer relationship: Unrolling the tangle. World J Gastrointest Oncol (2022) 14(5):959–72. doi: 10.4251/wjgo.v14.i5.959
62. Wu F, Yang L, Hao Y, Zhou B, Hu J, Yang Y, et al. Oral and gastric microbiome in relation to gastric intestinal metaplasia. Int J Cancer (2022) 150(6):928–40. doi: 10.1002/ijc.33848
63. Peng L, Guo Y, Gerhard M, Gao JJ, Liu ZC, Mejías-Luque R, et al. Metabolite alterations and interactions with microbiota in helicobacter pylori-associated gastric lesions. Microbiol Spectr (2023) 11(4):e0534722. doi: 10.1128/spectrum.05347-22
64. Zang H, Wang J, Wang H, Guo J, Li Y, Zhao Y, et al. Metabolic alterations in patients with Helicobacter pylori-related gastritis: The H. pylori-gut microbiota-metabolism axis in progression of the chronic inflammation in the gastric mucosa. Helicobacter (2023) 28(4):e12984. doi: 10.1111/hel.12984
65. Straub D, Oude Elferink R, Jansen P, Bergman J, Parikh K, Krishnadath KK. Glyco-conjugated bile acids drive the initial metaplastic gland formation from multi-layered glands through crypt-fission in a murine model. PloS One (2019) 14(7):e0220050. doi: 10.1371/journal.pone.0220050
66. Matsuhisa T, Arakawa T, Watanabe T, Tokutomi T, Sakurai K, Okamura S, et al. Relation between bile acid reflux into the stomach and the risk of atrophic gastritis and intestinal metaplasia: a multicenter study of 2283 cases. Dig Endosc (2013) 25(5):519–25. doi: 10.1111/den.12030
67. Xu X, Cheng J, Luo S, Gong X, Huang D, Xu J, et al. Deoxycholic acid-stimulated macrophage-derived exosomes promote spasmolytic polypeptide-expressing metaplasia in the stomach. Biochem Biophys Res Commun (2020) 524(3):649–55. doi: 10.1016/j.bbrc.2020.01.159
68. Xu X, Cheng J, Luo S, Huang D, Xu J, Qian Y, et al. Deoxycholic acid-stimulated macrophage-derived exosomes promote intestinal metaplasia and suppress proliferation in human gastric epithelial cells. Eur J Pharmacol (2020) 887:173566. doi: 10.1016/j.ejphar.2020.173566
69. Li T, Guo H, Li H, Jiang Y, Zhuang K, Lei C, et al. MicroRNA-92a-1-5p increases CDX2 by targeting FOXD1 in bile acids-induced gastric intestinal metaplasia. Gut (2019) 68(10):1751–63. doi: 10.1136/gutjnl-2017-315318
70. Wang N, Wu S, Zhao J, Chen M, Zeng J, Lu G, et al. Bile acids increase intestinal marker expression via the FXR/SNAI2/miR-1 axis in the stomach. Cell Oncol (Dordr) (2021) 44(5):1119–31. doi: 10.1007/s13402-021-00622-z
71. Yuan T, Ni Z, Han C, Min Y, Sun N, Liu C, et al. SOX2 interferes with the function of CDX2 in bile acid-induced gastric intestinal metaplasia. Cancer Cell Int (2019) 19:24. doi: 10.1186/s12935-019-0739-8
72. Rieder G, Hofmann JA, Hatz RA, Stolte M, Enders GA. Up-regulation of inducible nitric oxide synthase in Helicobacter pylori-associated gastritis may represent an increased risk factor to develop gastric carcinoma of the intestinal type. Int J Med Microbiol (2003) 293(6):403–12. doi: 10.1078/1438-4221-00280
73. Sanduleanu S, Bruïne AD, Biemond I, Stridsberg M, Jonkers D, Lundqvist G, et al. Ratio between serum IL-8 and pepsinogen A/C: a marker for atrophic body gastritis. Eur J Clin Invest (2003) 33(2):147–54. doi: 10.1046/j.1365-2362.2003.01101.x
74. Siregar GA, Halim S, Sitepu VR. Serum TNF-a, IL-8, VEGF levels in Helicobacter pylori infection and their association with degree of gastritis. Acta Med Indones (2015) 47(2):120–6.
75. Yamaoka Y, Kita M, Kodama T, Sawai N, Kashima K, Imanishi J. Induction of various cytokines and development of severe mucosal inflammation by cagA gene positive Helicobacter pylori strains. Gut (1997) 41(4):442–51. doi: 10.1136/gut.41.4.442
76. Correa P. Human gastric carcinogenesis: a multistep and multifactorial process–First American Cancer Society Award Lecture on Cancer Epidemiology and Prevention. Cancer Res (1992) 52(24):6735–40.
77. Outlioua A, Badre W, Desterke C, Echarki Z, El Hammani N, Rabhi M, et al. Gastric IL-1β, IL-8, and IL-17A expression in Moroccan patients infected with Helicobacter pylori may be a predictive signature of severe pathological stages. Cytokine (2020) 126:154893. doi: 10.1016/j.cyto.2019.154893
78. Rech TF, Mazzoleni LE, Mazzoleni F, Francesconi C, Sander GB, Michita RT, et al. Analysis of the influence of interleukin-1β Gene polymorphism on gastric inflammatory response and precancerous lesions development in patients with functional dyspepsia. Immunol Invest (2020) 49(5):585–96. doi: 10.1080/08820139.2019.1710532
79. Acosta-Rodriguez EV, Napolitani G, Lanzavecchia A, Sallusto F. Interleukins 1beta and 6 but not transforming growth factor-beta are essential for the differentiation of interleukin 17-producing human T helper cells. Nat Immunol (2007) 8:942–9. doi: 10.1038/ni1496
80. Acosta-Rodriguez EV, Rivino L, Geginat J, Jarrossay D, Gattorno M, Lanzavecchia A, et al. Surface phenotype and antigenic specificity of human interleukin 17-producing T helper memory cells. Nat Immunol (2007) 8:639–46. doi: 10.1038/ni1467
81. Bartchewsky W Jr, Martini MR, Masiero M, Squassoni AC, Alvarez MC, Ladeira MS, et al. Effect of Helicobacter pylori infection on IL-8, IL-1beta and COX-2 expression in patients with chronic gastritis and gastric cancer. Scand J Gastroenterol (2009) 44:153–61. doi: 10.1080/00365520802530853
82. Gonciarz W, Krupa A, Chmiela M. Proregenerative activity of IL-33 in gastric tissue cells undergoing helicobacter pylori-induced apoptosis. Int J Mol Sci (2020) 21(5):1801. doi: 10.3390/ijms21051801
83. Buzzelli JN, Chalinor HV, Pavlic DI, Sutton P, Menheniott TR, Giraud AS, et al. IL33 is a stomach alarmin that initiates a skewed th2 response to injury and infection. Cell Mol Gastroenterol Hepatol (2015) 1(2):203–21.e3. doi: 10.1016/j.jcmgh.2014.12.003
84. Fu S, Ramanujam KS, Wong A, Fantry GT, Drachenberg CB, James SP, et al. Increased expression and cellular localization of inducible nitric oxide synthase and cyclooxygenase 2 in Helicobacter pylori gastritis. Gastroenterology (1999) 116(6):1319–29. doi: 10.1016/S0016-5085(99)70496-8
85. Kaise M, Miwa J, Iihara K, Suzuki N, Oda Y, Ohta Y. Helicobacter pylori stimulates inducible nitric oxide synthase in diverse topographical patterns in various gastroduodenal disorders. Dig Dis Sci (2003) 48(4):636–43. doi: 10.1023/A:1022855818944
86. Miyazawa M, Suzuki H, Masaoka T, Kai A, Suematsu M, Nagata H, et al. Suppressed apoptosis in the inflamed gastric mucosa of Helicobacter pylori-colonized iNOS-knockout mice. Free Radic Biol Med (2003) 34(12):1621–30. doi: 10.1016/S0891-5849(03)00218-1
87. Yeung YT, Aziz F, Guerrero-Castilla A, Arguelles S. Signaling pathways in inflammation and anti-inflammatory therapies. Curr Pharm Des (2018) 24(14):1449–84. doi: 10.2174/1381612824666180327165604
88. Yang SH, Sharrocks AD, Whitmarsh AJ. MAP kinase signalling cascades and transcriptional regulation. Gene (2013) 513(1):1–13. doi: 10.1016/j.gene.2012.10.033
89. Spirina LV, Avgustinovich AV, Afanas'ev SG, Cheremisina OV, Volkov MY, Choynzonov EL, et al. Molecular mechanism of resistance to chemotherapy in gastric cancers, the role of autophagy. Curr Drug Targets (2020) 21(7):713–21. doi: 10.2174/1389450120666191127113854
90. Gao J, Liu Y, Chen J, Tong C, Wang Q, Piao Y. Curcumin treatment attenuates cisplatin-induced gastric mucosal inflammation and apoptosis through the NF- κ B and MAPKs signaling pathway. Hum Exp Toxicol (2022) 41:9603271221128738. doi: 10.1177/09603271221128738
91. Liu S, Shu L, Yang J, Liu Y, Zhang S, Wang N, et al. Rhein exhibits anti-inflammatory effects in chronic atrophic gastritis via Nrf2 and MAPK signaling. Turk J Gastroenterol (2023) 34(5):525–32. doi: 10.5152/tjg.2023.22251
92. Cai Tian-tian LL, Pan Hua-feng ZX, Zi-ming Z. Protective effect of panax notoginseng saponins on gastric mucosa of rats with gastric. China J Traditional Chin Med Pharm (2019) 34(12):5877–80.
93. Li X, Pan K, Vieth M, Gerhard M, Li W, Mejías-Luque R. JAK-STAT1 signaling pathway is an early response to helicobacter pylori infection and contributes to immune escape and gastric carcinogenesis. Int J Mol Sci (2022) 23(8):4147. doi: 10.3390/ijms23084147
94. Seif F, Khoshmirsafa M, Aazami H, Mohsenzadegan M, Sedighi G, Bahar M. The role of JAK-STAT signaling pathway and its regulators in the fate of T helper cells. Cell Commun Signal (2017) 15(1):23. doi: 10.1186/s12964-017-0177-y
95. Morey P, Pfannkuch L, Pang E, Boccellato F, Sigal M, Imai-Matsushima A, et al. Helicobacter pylori depletes cholesterol in gastric glands to prevent interferon gamma signaling and escape the inflammatory response. Gastroenterology (2018) 154(5):1391–404.e9. doi: 10.1053/j.gastro.2017.12.008
96. Li D, Zhao L, Li Y, Kang X, Zhang S. Gastro-protective effects of calycosin against precancerous lesions of gastric carcinoma in rats. Drug Des Devel Ther (2020) 14:2207–19. doi: 10.2147/DDDT.S247958
97. Zheng XJ, Chen PP, Liu Y, Sun JH, Zhang NL, Wang B, et al. Effect of modified Danggui Shaoyao Powder on SOCS3/TLR4 signaling pathway in rats with chronic atrophic gastritis. Zhongguo Zhong Yao Za Zhi (2022) 47(15):4128–35. doi: 10.19540/j.cnki.cjcmm.20220325.701
98. Wu S, Chen M, Huang J, Zhang F, Lv Z, Jia Y, et al. ORAI2 promotes gastric cancer tumorigenicity and metastasis through PI3K/Akt signaling and MAPK-dependent focal adhesion disassembly. Cancer Res (2021) 81(4):986–1000. doi: 10.1158/0008-5472.CAN-20-0049
99. Li N, Tang B, Jia YP, Zhu P, Zhuang Y, Fang Y, et al. Helicobacter pylori CagA Protein Negatively Regulates Autophagy and Promotes Inflammatory Response via c-Met-PI3K/Akt-mTOR Signaling Pathway. Front Cell Infect Microbiol (2017) 7:417. doi: 10.3389/fcimb.2017.00417
100. Tong Y, Liu L, Wang R, Yang T, Wen J, Wei S, et al. Berberine attenuates chronic atrophic gastritis induced by MNNG and its potential mechanism. Front Pharmacol (2021) 12:644638. doi: 10.3389/fphar.2021.644638
101. Zhu F, Xu Y, Pan J, Li M, Chen F, Xie G. Epigallocatechin Gallate Protects against MNNG-Induced Precancerous Lesions of Gastric Carcinoma in Rats via PI3K/Akt/mTOR Pathway. Evid Based Complement Alternat Med (2021) 2021:8846813. doi: 10.1155/2021/8846813
102. Liu W, Pan HF, Yang LJ, Zhao ZM, Yuan DS, Liu YL, et al. Panax ginseng C.A. Meyer (Rg3) Ameliorates Gastric Precancerous Lesions in Atp4a(-/-) Mice via Inhibition of Glycolysis through PI3K/AKT/miRNA-21 Pathway. Evid Based Complement Alternat Med (2020) 2020:2672648. doi: 10.1155/2020/2672648
103. Zhang JX, Bao SC, Chen J, Chen T, Wei HL, Zhou XY, et al. Xiaojianzhong decoction prevents gastric precancerous lesions in rats by inhibiting autophagy and glycolysis in gastric mucosal cells. World J Gastrointest Oncol (2023) 15(3):464–89. doi: 10.4251/wjgo.v15.i3.464
104. Cao Z, An L, Han Y, Jiao S, Zhou Z. The Hippo signaling pathway in gastric cancer. Acta Biochim Biophys Sin (Shanghai) (2023) 55(6):893–903. doi: 10.3724/abbs.2023038
105. Bae M, Jang S, Lim JW, Kang J, Bak EJ, Cha JH, et al. Protective effect of Korean Red Ginseng extract against Helicobacter pylori-induced gastric inflammation in Mongolian gerbils. J Ginseng Res (2014) 38(1):8–15. doi: 10.1016/j.jgr.2013.11.005
106. Lu B, Yu L, Xu L, Chen H, Zhang L, Zeng Y. The effects of radix curcumae extract on expressions of VEGF, COX-2 and PCNA in gastric mucosa of rats fed with MNNG. Curr Pharm Biotechnol (2010) 11(3):313–7. doi: 10.2174/138920110791111915
107. Tang Xin XX, Wang Wenlin RC, Cheng P. Toxicity efficacy of Radix Aconiti Lateralis Praeparata on treating abdominal pain. Pharmacol Clinics Chin Materia Med (2019) 35(03):105–10.
108. Doheny D, Manore SG, Wong GL, Lo HW. Hedgehog signaling and truncated GLI1 in cancer. Cells (2020) 9(9):2114. doi: 10.3390/cells9092114
109. Li B, Wang W, Li Z, Chen Z, Zhi X, Xu J, et al. MicroRNA-148a-3p enhances cisplatin cytotoxicity in gastric cancer through mitochondrial fission induction and cyto-protective autophagy suppression. Cancer Lett (2017) 410:212–27. doi: 10.1016/j.canlet.2017.09.035
110. Li Yi-fang ZA, Hu Jian-peng WR, Yue W. Effect and mechanism of modified Gualou Xiebai Banxia Decoction on chronic atrophic gastritis in rats. Chin J Clin Pharmacol (2021) 37(16):2201–5.
111. Chen Lu YJ, Lv Jun-hui SH, Li Jun-cai LH. Mechanism of weiweikang in treating chronic atrophic gastritis by affecting hedgehog pathway. J Basic Chin Med (2019) 25(12):1668–72.
112. Li Sihan LX, Tian Lin LM, Minghan H. Effect of spleen-fortifying, fire-clearing and collateral-unblocking medicinals on Sonic Hedgehog signaling pathway in rats with chronic atrophic gastritis. J Beijing Univ Traditional Chin Med (2021) 44(02):143–51.
113. Chu A, Yu X, Guo Q, Li Q, Sun M, Yuan Y, et al. H. pylori slyD, a novel virulence factor, is associated with Wnt pathway protein expression during gastric disease progression. Microb Pathog (2020) 148:104428. doi: 10.1016/j.micpath.2020.104428
114. Liu C, Li Y, Semenov M, Han C, Baeg GH, Tan Y, et al. Control of beta-catenin phosphorylation/degradation by a dual-kinase mechanism. Cell (2002) 108(6):837–47. doi: 10.1016/S0092-8674(02)00685-2
115. Zhao Y, Li B, Wang G, Ge S, Lan X, Xu G, et al. Dendrobium officinale Polysaccharides Inhibit 1-Methyl-2-Nitro-1-Nitrosoguanidine Induced Precancerous Lesions of Gastric Cancer in Rats through Regulating Wnt/β-Catenin Pathway and Altering Serum Endogenous Metabolites. Molecules (2019) 24(14). doi: 10.3390/molecules24142660
116. Yan Z, Xu T, Xu Y, Chen W, An Z, Zhu F. Jianpiyiqi formula ameliorates chronic atrophic gastritis in rats by modulating the Wnt/β-catenin signaling pathway. Exp Ther Med (2021) 22(2):878. doi: 10.3892/etm.2021.10310
117. Zhang P, Yang M, Zhang Y, Xiao S, Lai X, Tan A, et al. Dissecting the single-cell transcriptome network underlying gastric premalignant lesions and early gastric cancer. Cell Rep (2019) 27(6):1934–47.e5. doi: 10.1016/j.celrep.2019.04.052
118. Oya Y, Hayakawa Y, Koike K. Tumor microenvironment in gastric cancers. Cancer Sci (2020) 111(8):2696–707. doi: 10.1111/cas.14521
119. Chen X, Wang Z, Deng R, Yan H, Liu X, Kang R. Intervertebral disc degeneration and inflammatory microenvironment: expression, pathology, and therapeutic strategies. Inflammation Res (2023) 72(9):1811–28. doi: 10.1007/s00011-023-01784-2
Keywords: gastric precancerous lesions, inflammatory microenvironment, gastric cancer, antioxidants, TCM
Citation: Zhang S, Shen Y, Liu H, Zhu D, Fang J, Pan H and Liu W (2023) Inflammatory microenvironment in gastric premalignant lesions: implication and application. Front. Immunol. 14:1297101. doi: 10.3389/fimmu.2023.1297101
Received: 19 September 2023; Accepted: 26 October 2023;
Published: 15 November 2023.
Edited by:
Ling Wang, South China University of Technology, ChinaReviewed by:
Han Wang, Case Western Reserve University, United StatesQing Nian, Sichuan Academy of Medical Sciences and Sichuan Provincial People’s Hospital, China
Rong Yan, Shanghai University of Traditional Chinese Medicine, China
Copyright © 2023 Zhang, Shen, Liu, Zhu, Fang, Pan and Liu. This is an open-access article distributed under the terms of the Creative Commons Attribution License (CC BY). The use, distribution or reproduction in other forums is permitted, provided the original author(s) and the copyright owner(s) are credited and that the original publication in this journal is cited, in accordance with accepted academic practice. No use, distribution or reproduction is permitted which does not comply with these terms.
*Correspondence: Huafeng Pan, Z3pwaGZAZ3p1Y20uZWR1LmNu; Wei Liu, bGl1d2VpQGd6dWNtLmVkdS5jbg==