- 1Department of Animal Sciences, College of Food Agriculture and Environmental Sciences, The Ohio State University, Wooster, OH, United States
- 2Department of Animal Production, Faculty of Agriculture, Cairo University, Giza, Egypt
- 3Prestage Department of Poultry Science, College of Agriculture and Life Sciences, North Carolina State University, Raleigh, NC, United States
- 4Food For Health Discovery Theme, The Ohio State University, Columbus, OH, United States
Intraepithelial lymphocytes (IEL) reside in the epithelium at the interface between the contents of the intestinal lumen and the sterile environment of the lamina propria. Because of this strategic location, IEL play a crucial role in various immunological processes, ranging from pathogen control to tissue stability. In mice and humans, IEL exhibit high diversity, categorized into induced IEL (conventional CD4 and CD8αβ T cells) and natural IEL (TCRαβCD8αα, TCRγδ, and TCRneg IEL). In chickens, however, the subpopulations of IEL and their functions in enteric diseases remain unclear. Thus, we conducted this study to investigate the role of IEL populations during necrotic enteritis (NE) in chickens. At 14 days of age, sixty-three Specific-pathogen-free (SPF) birds were randomly assigned to three treatments: Control (sham challenge), Eimeria maxima challenge (EM), and Eimeria maxima + Clostridium Perfringens (C. Perfringens) co-challenge (EM/CP). The EM and EM/CP birds were infected with Eimeria maxima at day 14 of age, and EM/CP birds were additionally orally inoculated with C. perfringens at days 18 and 19 of age. Birds were weighed at days 18, 20, and 26 of age to assess body weight gain (BWG). At 20 days of age (1 day-post C. perfringens infection; dpi), and 26 days of age (7 dpi), 7 birds per treatment were euthanized, and jejunum was harvested for gross lesion scores, IEL isolation, and gene expression. The EM/CP birds exhibited subclinical NE disease, lower BWG and shorter colon length. The Most changes in the IEL populations were observed at 1 dpi. The EM/CP group showed substantial increases in the total number of natural IEL subsets, including TCRαβ+CD4-CD8-, TCRαβ+CD8αα+, TCRγδ+, TCRneg and innate CD8α (iCD8α) cells by at least two-fold. However, by 7 dpi, only the number of TCRαβ+CD4-CD8- and TCRαβ+CD8αα+ IEL maintained their increase in the EM/CP group. The EM/CP group had significantly higher expression of proinflammatory cytokines (IL-1β and IFN-γ) and Osteopontin (OPN) in the jejunum at 1 dpi. These findings suggest that natural IEL with innate and innate-like functions might play a critical role in the host response during subclinical NE, potentially conferring protection against C. perfringens infection.
1 Introduction
Necrotic Enteritis (NE) presents a significant challenge for the poultry industry, resulting in substantial financial losses of approximately $6 billion annually (1). This disease can manifest in two forms: clinical infection, causing high mortality rates, and subclinical infection, leading to reduced performance (2). Both forms negatively impact the health and profitability of birds in the poultry industry. In the USA, Antibiotic Growth Promoters (AGPs) have been routinely used in poultry operations since 1951 to control NE and other enteric infections (3). However, due to the concerns about antibiotic resistance and the emergence of superbugs, numerous countries, particularly Europe have imposed restriction on AGP usage in poultry (4). These restrictions have resulted in a resurgence of NE and have promoted numerous research efforts to identify feasible alternatives for its control (5–7). Research into antibiotic alternatives has yielded potential strategies for NE control, although none have been as effective as AGPs (6). Therefore, a more comprehensive understanding of various components within the chicken enteric immune system and their interactions with C. perfringes is needed. One promising avenue is studying intraepithelial lymphocytes (IEL) response to NE. IEL are located within the intestinal epithelium and represent one of the first lines of defense in encountering enteric infections (8).
Our current knowledge regarding the role of IEL primarily derives from studies conducted in mice and humans, with limited research focused on chickens. These cells have been recognized as an essential arm of mucosal immunity in the intestine, playing a crucial role in maintaining intestinal homeostasis by tolerating food particles and microbiota while eliminating pathogens (9). The IEL populations are composed of diverse subsets of immune cells, with the majority of being T cells (90%) expressing either TCRαβ or TCRγδ receptors (8, 9). These T cells can be further categorized into induced and natural IEL (8). Induced TCR+ IEL, including conventional CD4 and CD8αβ T cells, are activated in the periphery upon encountering their specific foreign antigens prior to entering the IEL populations. In contrast, natural IEL (TCRαβ+CD8αα+ and TCRγδ+ subsets) migrate directly to the intestinal epithelium upon their generation (10). The frequencies of T lineage IEL vary across the small intestine and colon in both humans and mice (9–11). In humans, there is a higher frequency of TCRαβ+ IEL compared to TCRγδ+ IEL in the small intestine and colon (12, 13). Conversely, the mouse small intestine has nearly equal proportions of both populations, with a shift toward TCRαβ+ IEL in the colon. In both human and mouse, the colon maintains a higher proportion of TCRαβ+CD4+ IEL than the small intestine (13). However, the opposite is observed for TCRαβ+CD8αβ+ IEL. In mice, 20-50% of TCRαβ+ IEL in the intestine can be TCRαβ+CD8αα+, while in humans, this population constitutes less than 1% of TCRαβ+ IEL (11). Finally, approximately 10% of IEL are non-T cells known as TCRneg IELs, which include innate lymphoid-like cells (14–16), intracellular CD3, and iCD8α (17, 18).
In the chickens, the presence of IEL was first reported by Lawn and his colleagues in 1988, using light and electron microscopy (19). Their findings indicated that the majority of chicken IEL are T-lineage lymphocytes. Subsequent later studies, while confirming the majority of IEL are T cells, demonstrated that natural killer (NK) cells also make up a significant portion of IEL (20, 21). Moreover, the role of IEL subsets mediating chicken responses to enteric diseases was limited to CD4+, CD8+, and TCRγδ+ T cells, which increased in frequency and changed their gene expression profiles during Eimeria infection (22–24). However, the response of subsets to NE has not been investigated. Therefore, the main aim of this study is to characterize IEL subsets using flow cytometry during C. perfringens-induced NE in SPF chickens. We employed a co-infection approach involving Eimeria maxima followed by C. perfringens to induce NE. Eimeria parasites are recognized as the primary predisposing factor for NE due to their ability to disrupt the integrity of the intestinal epithelium (25, 26). This disruption leads to the secretion of mucus and leakage of nutrients into the intestinal lumen, creating an environment that attracts C. perfringens to the small intestine and facilitates colonization (27, 28). Our results indicate that the numbers of natural IEL subsets, including TCRαβ+CD4-CD8-, TCRαβ+CD8αα+, TCRγδ+, TCRneg, and iCD8α+, increased during an early C. perfringens infection. Additionally, C. perfringens infection induced expression of OPN, IL-1β, and IFN-γ genes at 1 dpi (day-post C. perfringens infection).
2 Materials and methods
2.1 Ethics statement
The animal in the research trial was approved by The Ohio State University’s Institutional Animal Care and Use Committee (IACUC 2022A00000026) and conducted in compliance with the guidelines and regulations.
2.2 Husbandry, experimental design, and performance
Sixty-three SPF birds were placed in a single-floor pen with a sawdust litter in an environmentally controlled house (Supplementary Figure 1). The birds were divided into three groups (control, EM, and EM/CP) of 21 birds each and placed in three separate pens. On day 14 of age, the control group underwent a mock challenge with phosphate buffer saline (PBS, Quality Biological), while the EM and EM/CP groups were orally challenged with 5x103 sporulated oocysts/ml/bird of Eimeria maxima (provided generously by Dr. Lisa Beilke). On days 18 and 19 of age, the EM/CP group was orally inoculated with C. perfringens strain type A (CP1 [netB-] (29), provided by Dr. Lisa Beilke) at a concentration of 1x108 CFU/ml, while the EM group was administered PBS. Monitoring for signs of illness, mortality, and body weight was carried out starting from day 18 of age and continued throughout the duration of the experiment. Body weight gain (BWG) was calculated from days 18 to 20 and days 18 to 26 of age. At day 20 (1dpi) and 26 (7dpi) of age, 7 birds per group were euthanized through CO2 asphyxiation for sample collections.
2.3 Lesion scores and intestinal length
At 1 dpi, the jejunum-ileum junction was examined for NE lesions using 0-4 scale system (30), where 0 denotes no gross lesions, 1 signifies a thin-walled or friable intestine, 2 indicates focal necrosis or ulceration, 3 means large patches of necrosis, and 4 represents a severe, extensive necrosis. Additionally, the lengths of the duodenum, jejunum, ileum, and colon were individually measured for each bird.
2.4 IEL isolation and flowcytometry
At 1 and 7 dpi, approximately 10 cm segment of the jejunum was subjected to mechanical disruption, following a previously established protocol (31). In brief, the intestinal tissues were thoroughly rinsed with PBS and longitudinally opened to remove mucus and fecal matters. The tissue was cut into approximately 1 cm pieces and agitated in PBS supplemented with 5% chicken serum (Sigma-Aldrich, St. Louis, MO, USA), 2 mM EDTA (Quality Biological, Gaithersburg, MD, USA), and 2 mM dithiothreitol (Sigma-Aldrich, USA) at 150 rpm, 37°C for 45 minutes. Subsequently, the supernatant was passed through a gauze column, and the IEL fraction was enriched from the supernatants using a discontinuous 40/70% Percoll density gradient (Cytiva, Marlborough, MA, USA). The recovered cells were incubated for 5 minutes with ACK buffer (Quality Biological, USA) to lyse red blood cells and resuspended in a staining buffer. The number of live cells was counted using the trypan blue exclusion method. Then, the immune cells were stained with fluorochrome-conjugated anti-chicken CD45 SPRD (LT40), CD4 PE-CY7 (CT-4), CD3 AF547 (CT-3), TCRγδ FITC (TCR-1), CD8α AF700 (CT-8), and CD8β PE (EP42) antibodies (Southern Biotech, Birmingham, AL, USA). To differentiate between live and dead cells, we further stained cells with ghost viability dye-Red 510 (Tonbo Biosciences, San Diego, CA, USA). The frequency of stained cells was acquired using a flow cytometer BD FACSCanto II (BD Biosciences, Franklin Lakes, NJ, USA), and data analysis was carried out using FlowJo v10.8.1 software (BD Biosciences, USA). Figure 1 shows the gating strategy for IEL subsets. Data was reported as the number of cells per gram of tissue.
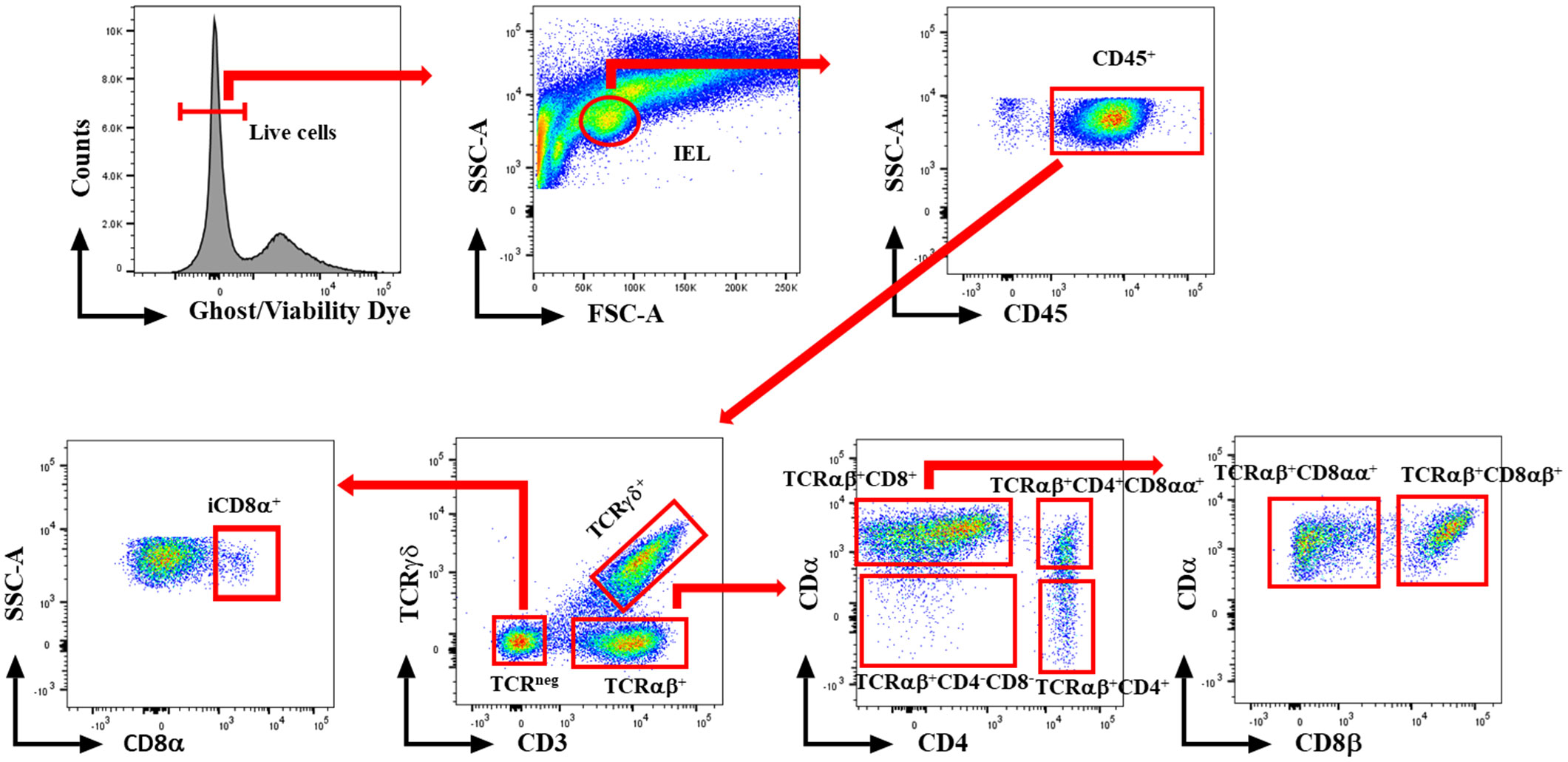
Figure 1 Gating strategy for flowcytometric analysis of IEL populations in jejunum of chickens. Cells were stained with ghost Red 510 and anti-chicken CD45 SPRD, CD4 PE-CY7, CD3 AF547, TCRγδ FITC, CD8α AF700, and CD8β PE antibodies. Gates were created in FlowJo.
2.5 RNA isolation and gene expression
At 1 and 7dpi, about 1 cm from the distal portion of the jejunum tissues were collected in RNA later and stored in -80° C until further analysis. RNA was isolated from jejunum tissues using the Monarch® Total RNA Miniprep Kit (New England Biolabs®, Ipswich, MA, USA) according to the standard protocol. Subsequently, cDNA was synthesized from the RNA using the LunaScript® RT SuperMix Kit (New England Biolabs®, USA). Quantitative real-time PCR was then conducted on a Bio-Rad CFX connect machine using Luna® Universal qPCR Master Mix (New England Biolabs®, USA) for OPN, IL-1β, IFN-γ, TGF-β, and TNF-α genes. The primer sequences are shown in Table 1. The cycle threshold (Ct) for each gene was normalized to the housekeeping gene, GAPDH. Relative fold change was calculated in comparison to the control group using the 2- ΔΔCt method (32).
2.6 Statistical analysis
Data analysis was performed using GraphPad PRISM v10.0.3 software (GraphPad, Boston, MA, USA). Flow cytometric data and lesion scores underwent analysis through a one-way non-parametric test (Kruskal-Wallis), followed by Dunn’s test. For BWGs, intestinal length, and gene expression, One-way ANOVA was conducted, followed by the Dunnett test. Multiple comparison tests were employed to distinguish means between groups. The results were expressed as mean ± standard error of the mean (SEM), with statistical significance set at P < 0.05.
3 Results
3.1 Emeria Maxima and C. perfringens co-infection induced mild NE disease
Throughout the course of C. perfringens infection, no instances of mortality or morbidity were recorded in any of the groups. Body weights were documented for each group on days 18, 20, and 26 of age. Body weight gain (BWG) was calculated for two intervals (18-20 and 18-26). In both intervals, the EM/CP group exhibited significantly lower BWG (P < 0.01) compared to the non-infected control group (Figure 2A). However, BWG was comparable between the EM/CP and EM groups. Only at 18-20 interval, the EM showed significantly reduced BWG (P < 0.01) compared to the control group. At 1 dpi, the EM/CP group displayed the highest lesion scores (score 1) in the jejunum-ileum junction compared to the control and EM groups (Figure 2B).
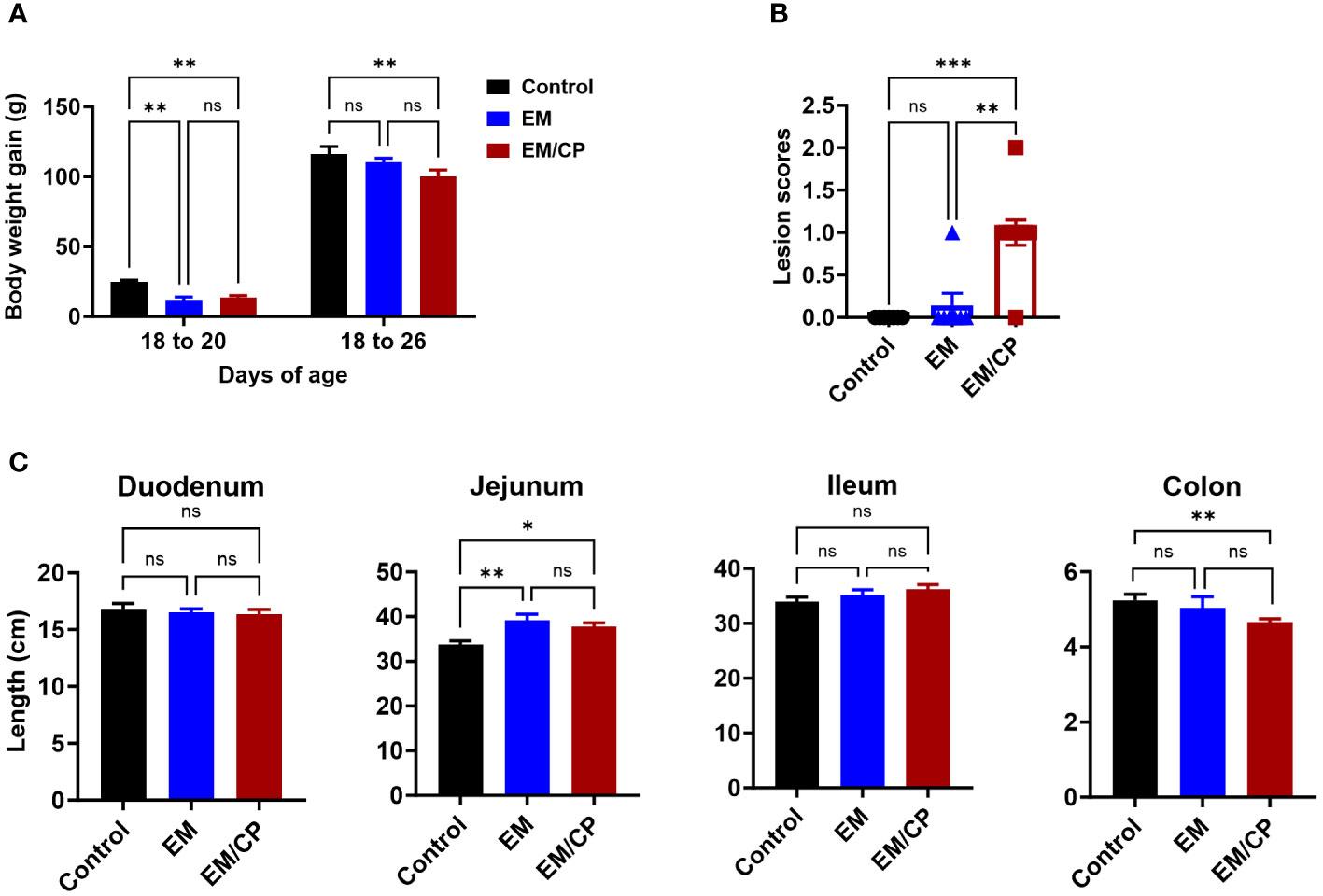
Figure 2 Eimeria challenge followed by C. Perfringes infection induced mild NE disease. (A) Body weight gain per gram from day 18-20 and 18-26 of age. One-way ANOVA and Dunnett tests. (B) Gross lesion scores of jejunal-ileum junctions at 1 dpi (day 20 of age). Control group received PBS. EM group infected with Eimeria maxima; at day 14 of age. EM/CP group infected with Eimeria maxima at day 14 of age and C. perfringens at days 18 and 19 of age. One-way Kruskal-Wallis and Dunn’s tests. Each dot represents an individual bird. (C) Length of intestinal sections in cm at 1 dpi (day 20 of age). One-way ANOVA and Dunnett tests. ns, non-significance, *P<0.05; **P<0.01; ***P<0.001.
Colon length shortening is commonly associated with intestinal inflammation and is considered a biological marker in various colitis models in mice (33–36). Hence, we investigated whether NE led to alterations in the length of intestinal sections at 1 dpi (Figure 2C). The EM/CP and EM groups exhibited a significantly longer jejunum length and a shortened colon length compared to the control group (P<0.05, and P < 0.01, respectively). However, the jejunum and colon lengths were comparable in the EM/CP and EM groups. Additionally, there were no discernible changes in the lengths of the duodenum and ileum between the groups. These findings suggest that Emeria Maxima and C. perfringens co-infection induced a mild or subclinical NE disease under our experimental conditions.
3.2 Natural IEL increases during the early incidence of NE
To explore the involvement of Intraepithelial Lymphocyte (IEL) populations during NE disease, we examined the IEL subpopulations in jejunum tissues following C. perfringens infection at 1 and 7 dpi. Figure 1 outlines the gating strategy applied for flow cytometric analysis of IEL subsets. At 1 dpi, there were no alterations in the number of induced IEL populations (TCRαβ+CD8αβ+, TCRαβ+CD4+, TCRαβ+CD4+CD8αα+) across all groups (Figure 3). However, there were notable increases in the number of jejunal natural IEL subpopulations after C. perfringens infection at 1 dpi (Figures 4–6). The EM/CP groups demonstrated over a two-fold increase in the number of TCRαβ+CD8αα+ cells compared to the control and EM groups (Figure 4). The number of TCRαβ+ IEL lacking the expression of CD4 and CD8 receptors significantly (P<0.01) increased in the EM/CP group compared to the control group only.
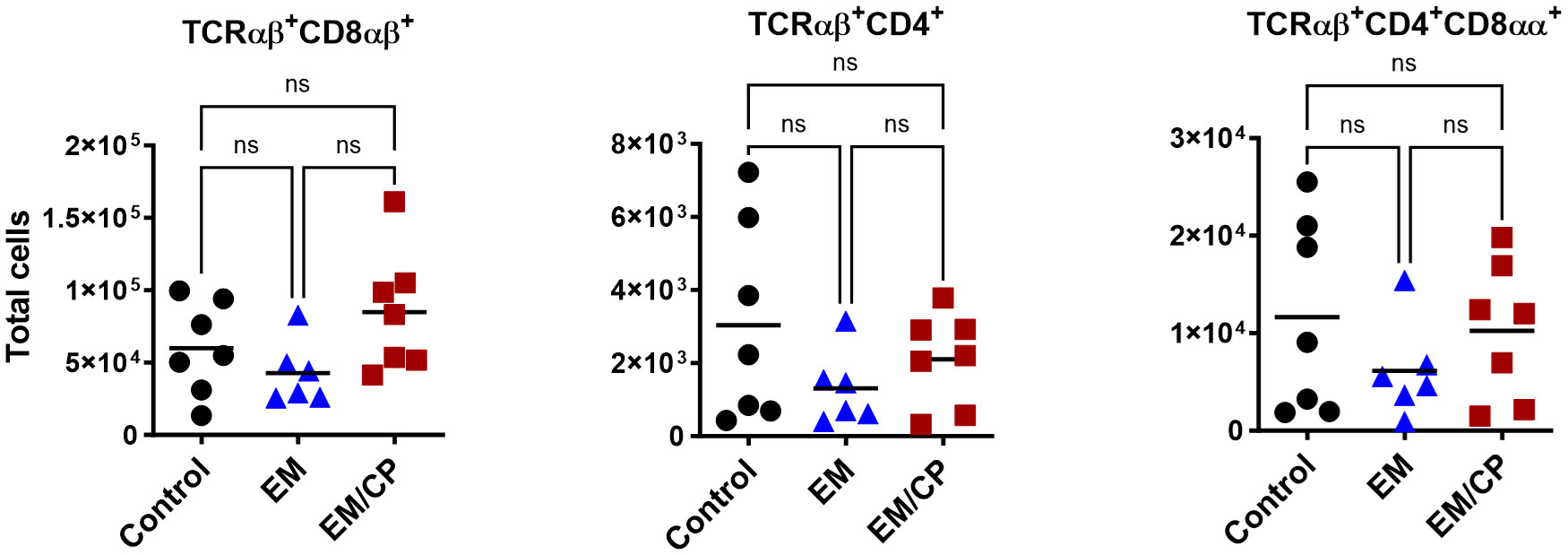
Figure 3 The Number of induced IEL was not impacted by NE disease at 1 dpi. Control group received PBS. EM group infected with Eimeria maxima; at day 14 of age. EM/CP group infected with Eimeria maxima at day 14 of age and C. perfringens at days 18 and 19 of age. TCRαβ+ cells are CD45+CD3+ TCRγδ-. Total cell number/gram of jejunum. One-way Kruskal-Wallis and Dunn’s tests. Each dot represents an individual bird. Black bar depicts mean value. ns, non-significance.
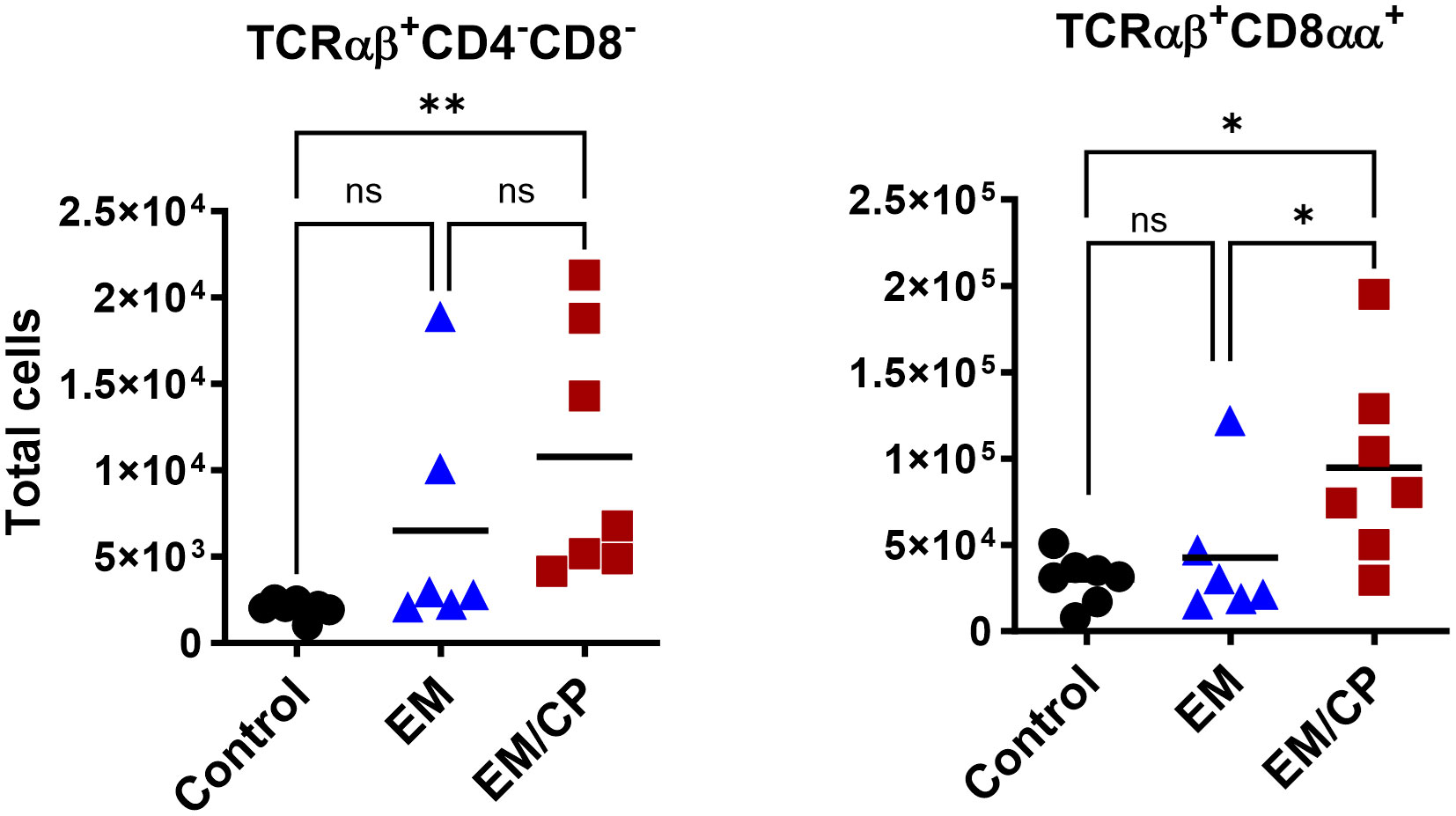
Figure 4 The number of natural TCRαβ+CD8αα+ IEL increased following NE disease at 1 dpi. Control group received PBS. EM group infected with Eimeria maxima; at day 14 of age. EM/CP group infected with Eimeria maxima at day 14 of age and C. perfringens at days 18 and 19 of age. TCRαβ+ cells are CD45+CD3+ TCRγδ-. Total cell number/gram of jejunum. One-way Kruskal-Wallis and Dunn’s tests. Each dot represents an individual bird. Black bar depicts mean value. ns, non-significance; *P<0.05; **P<0.01.
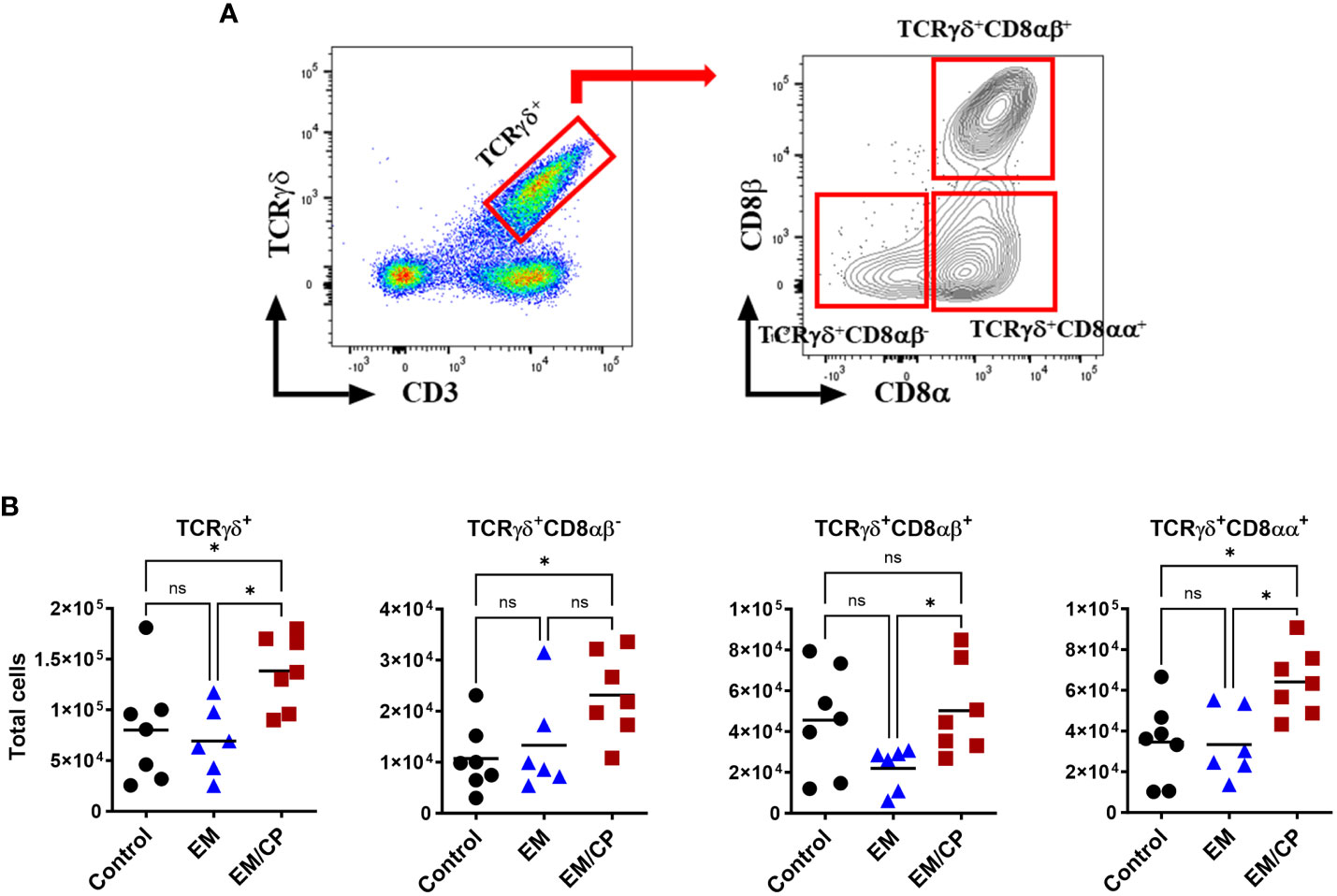
Figure 5 The number of natural TCRγδ IEL subsets increased following NE disease at 1 dpi. (A) Gating strategy used to differentiate TCRγδ IEL subpopulations. (B) The number of TCRγδ IEL subsets/gram of jejunum. Control group received PBS. EM group infected with Eimeria maxima; at day 14 of age. EM/CP group infected with Eimeria maxima at day 14 of age and C. perfringens at days 18 and 19 of age. TCRγδ+ cells are CD45+CD3+ TCRγδ+. One-way Kruskal-Wallis and Dunn’s tests. Each dot represents an individual bird. Black bar depicts mean value. ns, non-significance; *P<0.05.
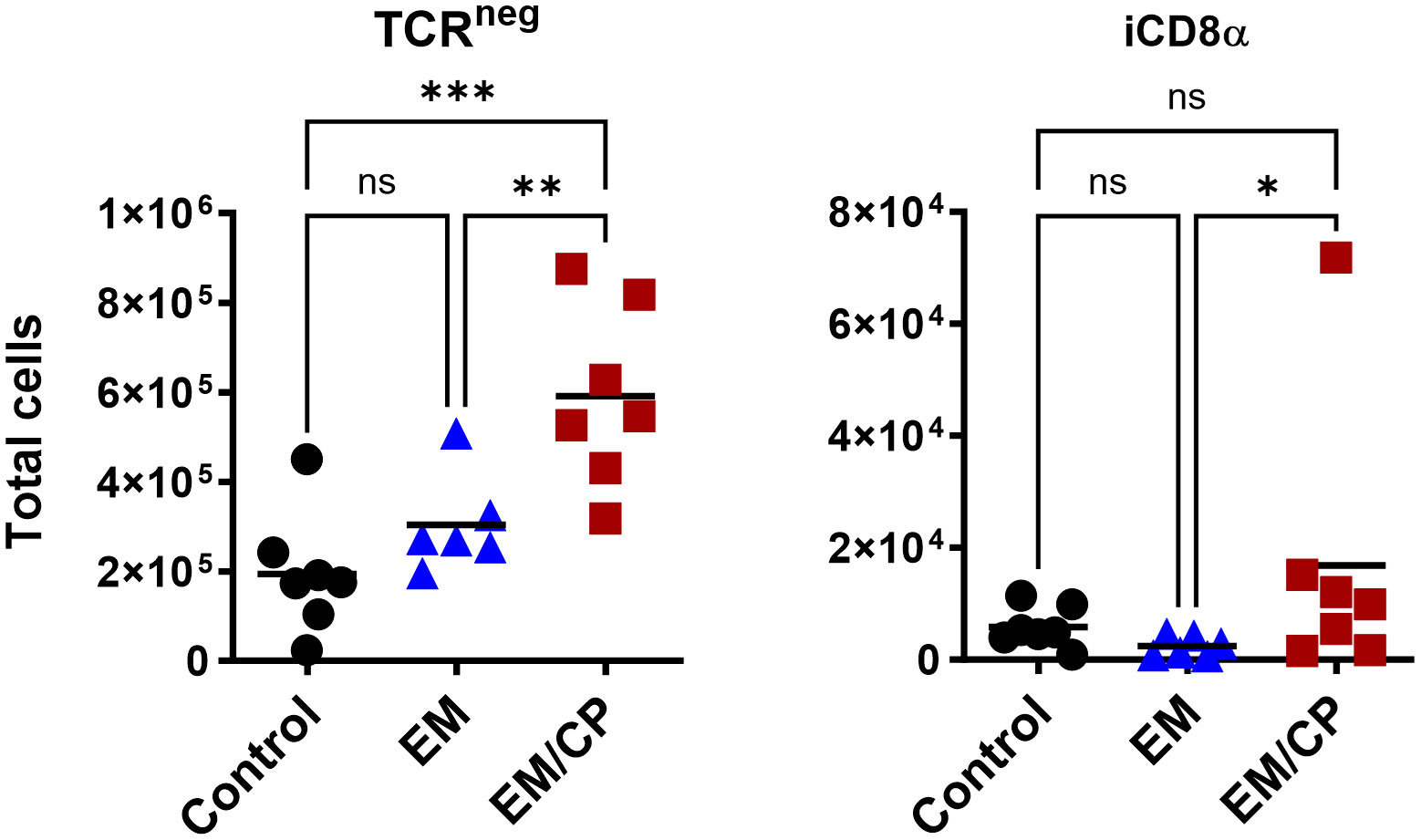
Figure 6 The number of natural TCRneg IEL induced following NE disease at 1 dpi. Control group received PBS. EM group infected with Eimeria maxima; at day 14 of age. EM/CP group infected with Eimeria maxima at day 14 of age and C. perfringens at days 18 and 19 of age. TCRneg cells are CD45+CD3-. Total cell number in jejunum/gram. One-way Kruskal-Wallis and Dunn’s tests. Each dot represents an individual bird. Black bar depicts mean value. ns, non-significance; *P<0.05; *P<0.05; **P<0.01; ***P<0.001.
In mice, TCRγδ T cells constitute a small fraction of circulating lymphocytes in the blood and peripheral tissues (37), and these cells make up about 40-70% of the total IEL population, with 80% of TCRγδ cells expressing CD8αα homodimers (38). Chickens, on the other hand, have a substantial fraction of TCRγδ T cells in the blood and various tissues, including the intestine (39). In the current study, the total number of TCRγδ cells was significantly (P<0.05) increased by twofold in the EM/CP group compared to the other two groups (Figure 5). Because intestinal TCRγδ T cells in chickens express CD8αα homodimers or CD8αβ heterodimers (40), we further analyzed the TCRγδ+ IEL subpopulations in the jejunum based on their expression of CDαα and/or CDαβ receptors. The EM/CP group had more TCRγδ+CD8αα+ and TCRγδ+CD8αβ- cells compared to the non-infected control group, while their numbers of TCRγδ+CD8αα+ and TCRγδ+CD8αβ+ cells were increased compared to the EM group. Then, we examined a fraction of non-T cell IEL that do not express CD3 receptor, TCRneg IEL (Figure 6). There was a significant surge in the number of TCRneg IEL in jejunum following C. perfringens infection compared to the other groups. Among TCRneg cells, the number of iCD8α+ cells increased in the EM/CP group compared to the EM group but not to the control group at 1dpi.
At 7dpi, there were no changes in the number of most IEL subpopulations in the jejunum, except for TCRαβ+CD4-CD8- and TCRαβ+CD8αα+ cells (Figure 7). The EM/CP group maintained a significant greater number of these cells compared to the control group. These results indicate that natural IEL might play a critical intestinal defense during the early stage of NE.
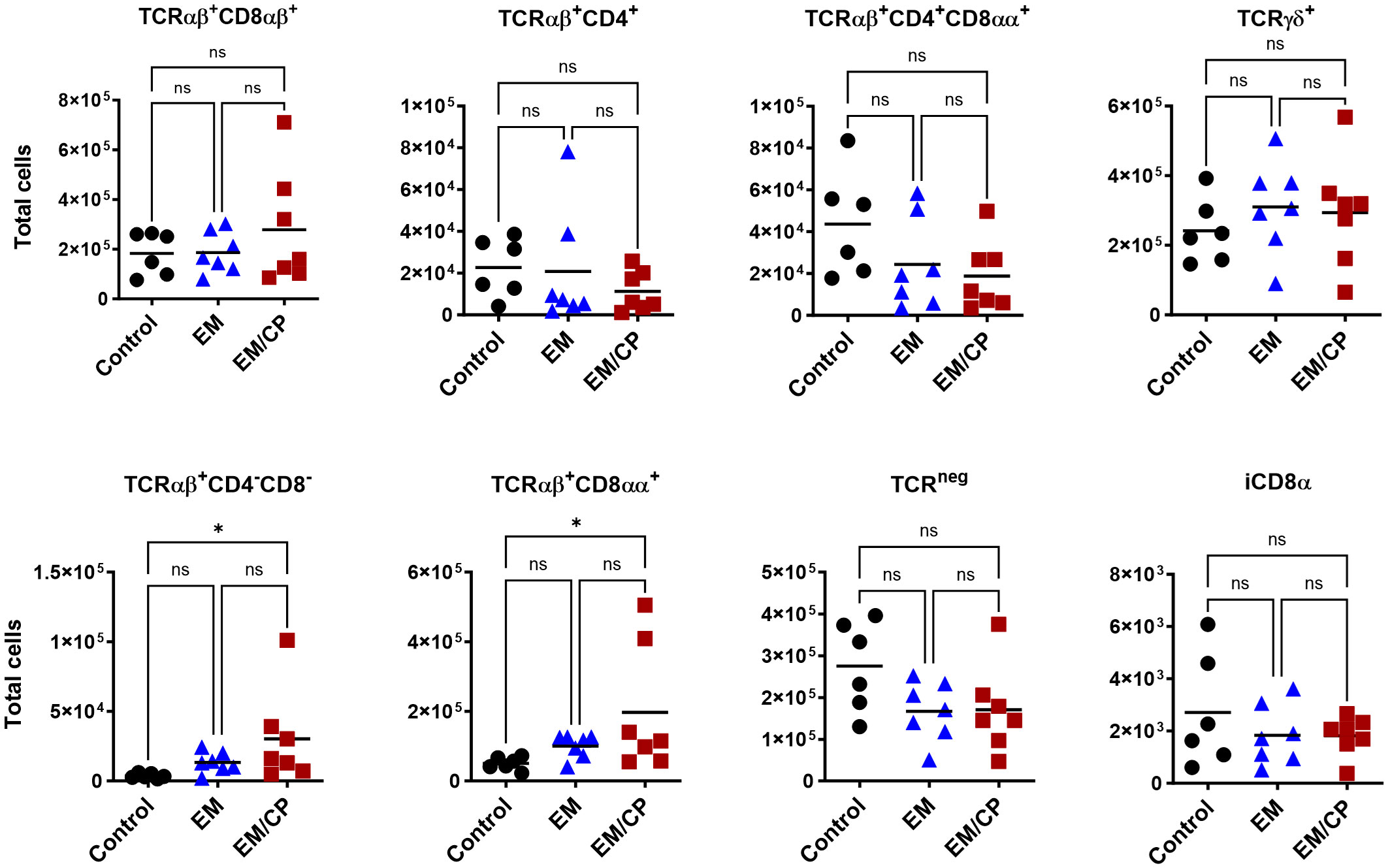
Figure 7 The changes in IEL subsets in the jejunum at 7dpi, were limited to TCRαβ+CD4-C8- and TCRαβ+CD8αα+ cells. Control group received PBS. EM group infected with Eimeria maxima; at day 14 of age. EM/CP group infected with Eimeria maxima at day 14 of age and C. perfringens at days 18 and 19 of age. Total cell number/gram of tissue. One-way Kruskal-Wallis and Dunn’s tests. Each dot represents an individual bird. Black bar depicts mean value. ns, non-significance; *P<0.05.
3.3 OPN expression in the jejunum is associated with early C. perfringens infection
OPN is a pleiotropic cytokine encoded by the Spp-1 gene and plays a crucial role in various biological processes. Our previous findings in mice indicate that OPN is primarily secreted by iCD8α cells in the intestine, and together, they serve as important mediators of IEL homeostasis by providing survival and proliferation signals for most IEL populations (31, 41). Observing an increase in the number of iCD8α cells in the jejunum after C. perfringens infection at 1 dpi, we investigated whether the expression of the OPN gene is associated with the rise in the number of iCD8α cells. At 1 dpi, the expression of OPN mRNA in the jejunum of the EM/CP group was significantly upregulated by 15 and 2 folds compared to the control (P<0.001), and the EM groups (P<0.05), respectively (Figure 8). There was no change in the expression of OPN between groups at 7 dpi. Additionally, we assessed the expression of different inflammatory and anti-inflammatory cytokines. Similar to the OPN pattern, the expressions of IFN-γ, and IL-1β genes were significantly upregulated in the EM/CP group compared to the control (P<0.0001) and EM (P<0.05) groups at 1 dpi, but not at 7 dpi (Figure 8). In addition, The EM group displayed significant upregulation of IFN-γ and IL-1β genes at 1 dpi only. On the other hand, there were no changes in the expression of TGF-β and TNF-α genes between groups at all time points.
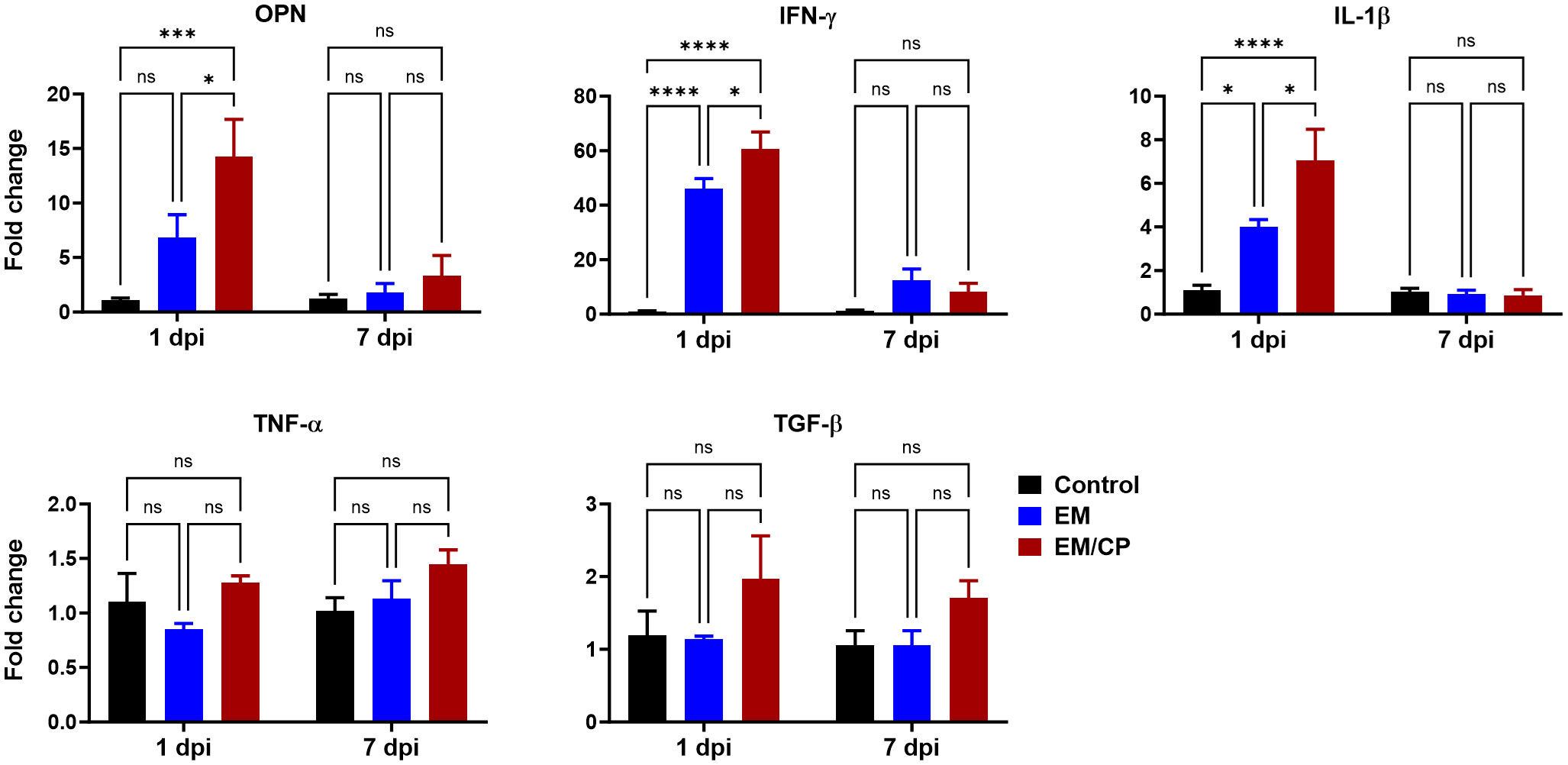
Figure 8 Gene expression of selected genes in jejunum following NE disease at 1dpi and 7dpi. Control group received PBS. EM group infected with Eimeria maxima; at day 14 of age. EM/CP group infected with Eimeria maxima at day 14 of age and C. perfringens at days 18 and 19 of age. OPN, osteopontin or Spp-1; IL-1β, interleukin 1beta; IFN-γ, interferon gamma; TNF-α, tumor necrosis factor alpha; TGF-β, tumor growth factor beta. One-way ANOVA and Dunnett tests. Fold change presented as mean ± SEM. ns, non-significance; *P<0.05; ***P<0.01; ****P<0.001.
4 Discussion
C. perfringens is an opportunistic bacterium known to trigger NE outbreaks in broiler chickens, particularly in the presence of predisposing factors such as stress, immunosuppression, an imbalanced diet, and intestinal damage caused by Eimeria infection (26). The outcomes of NE pathogenicity can manifest as either clinical infection, characterized by a high mortality rate and severe intestinal damage, or subclinical infection, associated with low mortality, mild intestinal damage, malnutrition, and reduced body weight gain (30, 42). In our experimental conditions, C. perfringens infection induced subclinical NE disease, as evidenced by the absence of mortality, reduced BWGs, and mild intestinal damage (average lesion scores = 1; Figure 2). Although the EM/CP group exhibited the lowest BWGs, these gains were not statistically significant compared to EM group. This lack of significance could be attributed to factors such as the chicken background (SPF layer chicks in this study), their age, and virulence of C. perfringens strain (25). At 14 days of age, birds might be more susceptible to EM infection with 5x103 oocysts, which conceals the differences in BWGs between EM/CP and EM groups (43). The signs of subclinical NE were accompanied with upregulation of proinflammatory cytokine genes IL-1β and IFN-γ, but not TNF-α at 1 dpi, which is necessary for the activation of innate immune cells such as macrophages (Figure 8). It is common in murine models of colitis that intestinal inflammation alters colon length (33–36). Our data indicated that C. perfringens infection shortened the colon length at 1 dpi (Figure 2C), suggesting potential colonic inflammation. Interestingly, mice infected with porcine C. perfringens displayed a shortened colon length in an infectious dose-dependent manner (44).
Intestinal IEL which are interspersed at the basolateral side of epithelial cells, represent the primary immunological defense against microbial invaders. To deepen our understanding of host-pathogen interactions during NE disease, we performed a flow cytometric analysis of IEL compartments in the jejunum following C. perfringens infection at 1 and 7 dpi. During the early stage of infection at 1 dpi, the EM/CP-infected group exhibited substantial numbers of natural IEL subpopulations, including TCRαβ+CD4-CD8-, TCRαβ+CD8αα+, TCRγδ+, and TCRneg cells in the jejunum compared to the control and EM groups (Figures 4–6). The first three cell types are subsets of T cells known as “innate-like T cells,” capable of interacting with non-classical MHC molecules and, therefore, do not require antigen presentation for their functions (45, 46). The TCRαβ+CD4-CD8- cells generated in the thymus, migrate directly to the intestinal epithelium, where they gain expression of CD8αα homodimers to become TCRαβ+CD8αα+IEL (47–49). TCRαβ+CD8αα+ cells are exclusively part of the IEL compartment, constituting 20-50% and less than 1% of T-cell IEL in mice and humans, respectively (11, 50). Our current study is the first to report the presence of TCRαβ+CD8αα+ IEL in chickens, which constituted 20-30% of total T cell IEL. While the exact role of TCRαβ+CD8αα+ IEL in mucosal immunity is not fully understood, there is some evidence suggesting they have immune regulatory functions through the expression of TGF-β3, lymphocytes activation gene-3, and fibrinogen-like protein 2 (51). Therefore, the TCRαβ+CD8αα+ IEL protect immune compromised mice against colitis development following the adoptive transfer of CD4+CD45RBhi T cells (52). The robust increase in the number of natural TCRαβ+CD8αα+ IEL in EM/CP groups at 1 dpi and their potential regulatory function, might explain relatively low lesion scores and mild signs of NE disease.
Another significant alteration in natural IEL was the increase in the number of TCRγδ+ cells following C. perfringens infection at 1 dpi (Figure 5). Previous research has reported a high frequency of TCRγδ+ cells in the cecal tonsils following infection with a virulent-C. perfringens strain, suggesting these cells play a crucial role in mediating anti-C. perfringens immunity in chickens (53). Furthermore, ex-vivo stimulation of the small intestinal TCRγδ+ cells with C. perfringens increased the frequency of these cells (54). In the current study, the majority of jejunal TCRγδ+ IEL are expressing either CD8αα homodimers or CDαβ heterodimers, identifying two-distinct subsets of IEL (40). In contrast to mice and humans, TCRγδ+CD8αβ+ cells are particularly interesting because they are unique to the chicken IEL compartment, and their role in mucosal immunity is yet to be elucidated. Our data indicated an induction of TCRγδ+CD8αα+ IEL in EM/CP groups compared to all other groups, and the number of TCRγδ+CD8αβ+ IEL increased in the EM/CP group compared to the EM group at 1 dpi. The main function of TCRγδ+ IEL is to protect the intestinal epithelium by inhibiting an early invasion of resident and pathogenic microorganisms and maintaining intestinal homeostasis by limiting excessive inflammation and tissue damage during infection (55, 56). TCRγδ+ IEL produce a variety of effector molecules such as pro-inflammatory cytokines (IFN-γ and TNF-α), anti-inflammatory cytokines (TGF-β and IL-10), wound healing factors (TGF-β, prothymosin β4, and keratinocyte growth factor), cytotoxic enzymes (granzymes), and antimicrobial peptides (11). In Salmonella-infected chickens, both TCRγδ+CD8αα+ and TCRγδ+CD8αβ+ cells express IFN-γ mRNA, suggesting these cells can promote the phagocytic capability of macrophage to clear infection (57). Therefore, the upregulation of INF-γ expression (Figure 8) and augmentation of TCRγδ+ subsets in the jejunum of EM/CP group imply the essential role of TCRγδ+ IEL in enhancing the bactericidal function of macrophages at the early infection stage.
The third cell group of natural IEL that experienced a significant increase following C. perfringens infection at 1 dpi, was TCRneg cells (Figure 6). These cells belong to the innate immune cells and are not fully characterized in chickens. Previous studies have identified NK cells as a distinct TCRneg IEL population in chickens during embryonic and early life (20, 21). In mice and humans, several subsets of TCRneg IELs have been identified and characterized with vital functions in the mucosal immunity (8). TCRneg IEL are composed of innate lymphoid cells expressing NK receptors (NKP46, NK1.1, and NKP44) (15–17), and lymphocytes expressing intracellular CD3γ. The later lymphocytes are further divided according to the expression of CDαα into intracellular CD3 (CD8α−) and iCD8α (CD8α+) (18, 19). The iCD8α cells produce a diverse array of effector molecules, such as monocyte chemotactic protein 1 (MCP-1), IFN-γ, and OPN, and possess antigen processing and presentation, cytotoxicity, and phagocytosis, highlighting their crucial role during early immune responses (19). Our results indicate, for the first time, that chickens harbor iCD8α cells in the intestine and their number increased following C. perfringens infection (Figure 6), suggesting that these cells might confer protection against C. perfringens, similar to their protective role against Citrobacter rodentium infection in mice (19).
Our previous results in mice indicated that iCD8α cells through OPN mediate the homeostasis of IEL subpopulations by promoting their survival in a CD44-dependent manner, proliferation, and migration to intestinal epithelium (31). For example, OPN-knockout mice exhibit a reduction in the numbers of TCRαβ+CD4+, TCRαβ+CD4+CD8αα+, TCRαβ+CD8αβ+, TCRαβ+CD8αα+, and TCRγδ+ IEL in the small intestine and colon compared to wild-type mice. Moreover, OPN sustains proper expression of Foxp3 on regulatory T cells in the intestine and therefore, protect against colitis (31). In the present study, induction of subclinical NE was associated with upregulation of OPN mRNA level and high number of iCD8α IEL in the jejunum (Figure 8). These findings underscore the importance of iCD8a cells and OPN in intestinal health and warrant further investigation of their roles during mucosal immune responses.
In summary, in this report, we provide evidence supporting the immunological significance of natural IEL subsets, specifically TCRγδ+, TCRαβ+CD8αα+, and TCRneg, including iCD8a cells, in the early immune response of chickens to NE. Furthermore, we report, for the first time, that chickens harbor fractions of TCRαβ+CD8αα+ and iCD8a cells in IEL compartments, suggesting their potential role in conferring protection against C. perfringens infection. However, the precise functions and mechanisms of these IEL populations in the context of intestinal inflammation in chickens warrant further investigation.
Data availability statement
The raw data supporting the conclusions of this article will be made available by the authors, without undue reservation.
Ethics statement
The animal study was approved by The Ohio State University’s Institutional Animal Care and Use Committee. The study was conducted in accordance with the local legislation and institutional requirements.
Author contributions
SM: Data curation, Investigation, Methodology, Writing – original draft. SH: Data curation, Investigation, Methodology, Resources, Writing – review & editing. BS: Data curation, Investigation, Methodology, Writing – review & editing. LB: Methodology, Writing – review & editing. AN: Conceptualization, Formal Analysis, Funding acquisition, Investigation, Methodology, Project administration, Resources, Software, Supervision, Validation, Visualization, Writing – review & editing.
Funding
The author(s) declare financial support was received for the research, authorship, and/or publication of this article. This research was supported with start-up funds for AN from the Ohio State University. This research was partially supported by the Egyptian Ministry of Higher Education Scholarship for SH.
Conflict of interest
The authors declare that the research was conducted in the absence of any commercial or financial relationships that could be construed as a potential conflict of interest.
Publisher’s note
All claims expressed in this article are solely those of the authors and do not necessarily represent those of their affiliated organizations, or those of the publisher, the editors and the reviewers. Any product that may be evaluated in this article, or claim that may be made by its manufacturer, is not guaranteed or endorsed by the publisher.
Supplementary material
The Supplementary Material for this article can be found online at: https://www.frontiersin.org/articles/10.3389/fimmu.2024.1354701/full#supplementary-material
References
1. Keyburn A, Wade B. The true cost of necrotic enteritis. Poultry World. (2015). https://www.poultryworld.net/poultry/the-true-cost-of-necrotic-enteritis/.
2. To H, Suzuki T, Kawahara F, Uetsuka K, Nagai S, Nunoya T. Experimental induction of necrotic enteritis in chickens by a netB-positive Japanese isolate of Clostridium perfringens. J Veterinary Med Sci. (2017) 79:350–8. doi: 10.1292/jvms.16-0500
3. McDevitt RM, Brooker JD, Acamovic T, Sparks NHC. Necrotic enteritis; a continuing challenge for the poultry industry. World’s Poultry Sci J. (2006) 62:221–47. doi: 10.1079/WPS200593
4. Martin MJ, Thottathil SE, Newman TB. Antibiotics overuse in animal agriculture: A call to action for health care providers. Am J Public Health. (2015) 105:2409–10. doi: 10.2105/AJPH.2015.302870
5. Hughes L, Hermans P, Morgan K. Risk factors for the use of prescription antibiotics on UK broiler farms. J Antimicrobial Chemotherapy. (2008) 61:947–52. doi: 10.1093/jac/dkn017
6. Emami NK, Dalloul RA. Centennial Review: Recent developments in host-pathogen interactions during necrotic enteritis in poultry. Poultry Sci. (2021) 100:101330. doi: 10.1016/j.psj.2021.101330
7. Feng X, Li T, Zhu H, Liu L, Bi S, Chen X, et al. Effects of challenge with Clostridium perfringens, Eimeria and both on ileal microbiota of yellow feather broilers. Front Microbiol. (2022) 13:1063578. doi: 10.3389/fmicb.2022.1063578
8. Olivares-Villagómez D, Van Kaer L. Intestinal intraepithelial lymphocytes: sentinels of the mucosal barrier. Trends Immunol. (2018) 39:264–75. doi: 10.1016/j.it.2017.11.003
9. Cheroutre H, Lambolez F, Mucida D. The light and dark sides of intestinal intraepithelial lymphocytes. Nat Rev Immunol. (2011) 11:445–56. doi: 10.1038/nri3007
10. Abadie V, Discepolo V, Jabri B. Intraepithelial lymphocytes in celiac disease immunopathology. Semin Immunopathol. (2012) 34:551–66. doi: 10.1007/s00281-012-0316-x
11. Van Kaer L, Olivares-Villagómez D. Development, homeostasis, and functions of intestinal intraepithelial lymphocytes. J Immunol. (2018) 200:2235–44. doi: 10.4049/jimmunol.1701704
12. Boll G, Rudolphi A, Spiess S, Reimann J. Regional specialization of intraepithelial T cells in the murine small and large intestine. Scand J Immunol. (1995) 41:103–13. doi: 10.1111/j.1365-3083.1995.tb03541.x
13. Mowat AM, Agace WW. Regional specialization within the intestinal immune system. Nat Rev Immunol. (2014) 14:667–85. doi: 10.1038/nri3738
14. Fuchs A, Vermi W, Lee JS, Lonardi S, Gilfillan S, Newberry RD, et al. Intraepithelial type 1 innate lymphoid cells are a unique subset of IL-12- and IL-15-responsive IFN-γ-producing cells. Immunity. (2013) 38:769–81. doi: 10.1016/j.immuni.2013.02.010
15. Talayero P, Mancebo E, Calvo-Pulido J, Rodríguez-Muñoz S, Bernardo I, Laguna-Goya R, et al. Innate lymphoid cells groups 1 and 3 in the epithelial compartment of functional human intestinal allografts. Am J Transplant. (2016) 16:72–82. doi: 10.1111/ajt.13435(4)
16. Van Acker A, Gronke K, Biswas A, Martens L, Saeys Y, Filtjens J, et al. A murine intestinal intraepithelial NKp46-negative innate lymphoid cell population characterized by group 1 properties. Cell Rep. (2017) 19:1431–43. doi: 10.1016/j.celrep.2017.04.068(5)
17. Ettersperger J, Montcuquet N, Malamut G, Guegan N, Lopez-Lastra S, Gayraud S, et al. Interleukin-15-dependent T-cell-like innate intraepithelial lymphocytes (6) develop in the intestine and transform into lymphomas in celiac disease. Immunity. (2016) 45:610–25. doi: 10.1016/j.immuni.2016.07.018
18. Van Kaer L, Scott Algood HM, Singh K, Parekh VV, Greer MJ, Piazuelo MB, et al. CD8αα innate-type lymphocytes in the intestinal epithelium mediate mucosal immunity. Immunity. (2014) 41:451–64. doi: 10.1016/j.immuni.2014.08.010(7
19. Lawn AM, Rose ME, Bradley JW, Rennie MC. Lymphocytes of the intestinal mucosa of chickens. Cell Tissue Res. (1988) 251:189–95. doi: 10.1007/BF00215464
20. Göbel TW, Kaspers B, Stangassinger M. NK and T cells constitute two major, functionally distinct intestinal epithelial lymphocyte subsets in the chicken. Int Immunol. (2001) 13:757–62. doi: 10.1093/intimm/13.6.757
21. Meijerink N, Van Haarlem DA, Velkers FC, Stegeman AJ, Rutten VPMG, Jansen CA. Analysis of chicken intestinal natural killer cells, a major IEL subset during embryonic and early life. Dev Comp Immunol. (2021) 114:103857. doi: 10.1016/j.dci.2020.103857
22. Hong YH, Lillehoj HS, Lillehoj EP, Lee SH. Changes in immune-related gene expression and intestinal lymphocyte subpopulations following Eimeria maxima infection of chickens. Vet Immunol Immunopathol. (2006) 114:259–72. doi: 10.1016/j.vetimm.2006.08.006
23. Choi KD, Lillehoj HS, Zalenga DS. Changes in local IFN-gamma and TGF-beta4 mRNA expression and intraepithelial lymphocytes following Eimeria acervulina infection. Vet Immunol Immunopathol. (1999) 71:263–75. doi: 10.1016/s0165-2427(99)00103-8
24. Kim CH, Lillehoj HS, Bliss TW, Keeler CL, Hong YH, Park DW, et al. Construction and application of an avian intestinal intraepithelial lymphocyte cDNA microarray (AVIELA) for gene expression profiling during Eimeria maxima infection. Vet Immunol Immunopathol. (2008) 124:341–54. doi: 10.1016/j.vetimm.2008.04.013
25. Prescott JF, Smyth JA, Shojadoost B, Vince A. Experimental reproduction of necrotic enteritis in chickens: a review. Avian Pathol. (2016) 45:317–22. doi: 10.1080/03079457.2016.1141345
26. Collier CT, Hofacre CL, Payne AM, Anderson DB, Kaiser P, Mackie RI, et al. Coccidia-induced mucogenesis promotes the onset of necrotic enteritis by supporting Clostridium perfringens growth. Veterinary Immunol Immunopathology. (2008) 122:104–15. doi: 10.1016/j.vetimm.2007.10.014
27. Miska KB, Fetterer RH. The mRNA expression of amino acid and sugar transporters, aminopeptidase, as well as the di- and tri-peptide transporter PepT1 in the intestines of Eimeria infected broiler chickens. Poult Sci. (2017) 96:465–73. doi: 10.3382/ps/pew303
28. Su S, Miska KB, Fetterer RH, Jenkins MC, Wong EA. Expression of digestive enzymes and nutrient transporters in Eimeria-challenged broilers. Exp Parasitol. (2015) 150:13–21. doi: 10.1016/j.exppara.2015.01.003
29. Wilson KM, Chasser KM, Duff AF, Briggs WN, Latorre JD, Barta JR, et al. Comparison of multiple methods for induction of necrotic enteritis in broilers. I. J Appl Poultry Res. (2018) 27:577–89. doi: 10.3382/japr/pfy033
30. Shojadoost B, Vince AR, Prescott JF. The successful experimental induction of necrotic enteritis in chickens by Clostridium perfringens: a critical review. Vet Res. (2012) 43:74. doi: 10.1186/1297-9716-43-74
31. Nazmi A, Greer MJ, Hoek KL, Piazuelo MB, Weitkamp J-H, Olivares-Villagómez D. Osteopontin and iCD8α Cells promote intestinal intraepithelial lymphocyte homeostasis. J Immunol. (2020) 204:1968–81. doi: 10.4049/jimmunol.1901168
32. Livak KJ, Schmittgen TD. Analysis of relative gene expression data using real-time quantitative PCR and the 2–ΔΔCT method. Methods. (2001) 25:402–8. doi: 10.1006/meth.2001.1262
33. Chassaing B, Aitken JD, Malleshappa M, Vijay-Kumar M. Dextran Sulfate Sodium (DSS)-Induced Colitis in Mice. Current Protocols in Immunology, (Wiley) (2014) 104:15.25.1–15.25.14. doi: 10.1002/0471142735.im1525s104
34. Jayawardena D, Tyagi S, Nazmi A, Olivares- Villagómez D, Dudeja PK. Ion transport basis of diarrhea in a mouse model of adoptive T cell transfer colitis. Dig Dis Sci. (2020) 65:1700–9. doi: 10.1007/s10620-019-05945-4
35. Ko S-J, Bu Y, Bae J, Bang Y, Kim J, Lee H, et al. Protective effect of Laminaria japonica with probiotics on murine colitis. Mediators Inflammation. (2014) 2014:417814. doi: 10.1155/2014/417814
36. Bhinder G, Sham HP, Chan JM, Morampudi V, Jacobson K, Vallance BA. The citrobacter rodentium mouse model: studying pathogen and host contributions to infectious colitis. J Vis Exp. (2013) 72:50222. doi: 10.3791/50222
37. Nielsen MM, Witherden DA, Havran WL. γδ T cells in homeostasis and host defence of epithelial barrier tissues. Nat Rev Immunol. (2017) 17:733–45. doi: 10.1038/nri.2017.101
38. Guy-Grand D, Rocha B, Mintz P, Malassis-Seris M, Selz F, Malissen B, et al. Different use of T cell receptor transducing modules in two populations of gut intraepithelial lymphocytes are related to distinct pathways of T cell differentiation. J Exp Med. (1994) 180:673–9. doi: 10.1084/jem.180.2.673
39. Sowder JT, Chen CL, Ager LL, Chan MM, Cooper MD. A large subpopulation of avian T cells express a homologue of the mammalian T gamma/delta receptor. J Exp Med. (1988) 167:315–22. doi: 10.1084/jem.167.2.315
40. Tregaskes CA, Kong FK, Paramithiotis E, Chen CL, Ratcliffe MJ, Davison TF, et al. Identification and analysis of the expression of CD8 alpha beta and CD8 alpha alpha isoforms in chickens reveals a major TCR-gamma delta CD8 alpha beta subset of intestinal intraepithelial lymphocytes. J Immunol. (1995) 154:4485–94. doi: 10.4049/jimmunol.154.9.4485
41. Nazmi A, Hoek KL, Greer MJ, Piazuelo MB, Minato N, Olivares-Villagómez D. Innate CD8αα+ cells promote ILC1-like intraepithelial lymphocyte homeostasis and intestinal inflammation. PloS One. (2019) 14:e0215883. doi: 10.1371/journal.pone.0215883
42. Gholamiandehkordi AR, Timbermont L, Lanckriet A, Van Den Broeck W, Pedersen K, Dewulf J, et al. Quantification of gut lesions in a subclinical necrotic enteritis model. Avian Pathol. (2007) 36:375–82. doi: 10.1080/03079450701589118
43. Lu M, Li RW, Zhao H, Yan X, Lillehoj HS, Sun Z, et al. Effects of Eimeria maxima and Clostridium perfringens infections on cecal microbial composition and the possible correlation with body weight gain in broiler chickens. Res Veterinary Sci. (2020) 132:142–9. doi: 10.1016/j.rvsc.2020.05.013
44. Jiang Z, Su W, Wen C, Li W, Zhang Y, Gong T, et al. Effect of porcine clostridium perfringens on intestinal barrier, immunity, and quantitative analysis of intestinal bacterial communities in mice. Front Vet Sci. (2022) 9:881878. doi: 10.3389/fvets.2022.881878
45. Molgora M, Colonna M. Innate-like T cells: A promising asset in anti-cancer immunity. Cancer Cell. (2022) 40:714–6. doi: 10.1016/j.ccell.2022.05.017
46. Dadi S, Chhangawala S, Whitlock BM, Franklin RA, Luo CT, Oh SA, et al. Cancer immunosurveillance by tissue-resident innate lymphoid cells and innate-like T cells. Cell. (2016) 164:365–77. doi: 10.1016/j.cell.2016.01.002
47. Gangadharan D, Lambolez F, Attinger A, Wang-Zhu Y, Sullivan BA, Cheroutre H. Identification of pre- and postselection TCRalphabeta+ intraepithelial lymphocyte precursors in the thymus. Immunity. (2006) 25:631–41. doi: 10.1016/j.immuni.2006.08.018
48. McDonald BD, Bunker JJ, Ishizuka IE, Jabri B, Bendelac A. Elevated T cell receptor signaling identifies a thymic precursor to the TCRαβ(+)CD4(-)CD8β(-) intraepithelial lymphocyte lineage. Immunity. (2014) 41:219–29. doi: 10.1016/j.immuni.2014.07.008
49. Ruscher R, Kummer RL, Lee YJ, Jameson SC, Hogquist KA. CD8αα intraepithelial lymphocytes arise from two main thymic precursors. Nat Immunol. (2017) 18:771–9. doi: 10.1038/ni.3751
50. Nazmi A, McClanahan KG, Olivares-Villagomez D. Unconventional intestinal intraepithelial lymphocytes in health and disease. Crit Rev Immunol. (2021) 41:23–38. doi: 10.1615/CritRevImmunol.2021039957
51. Denning TL, Granger SW, Mucida D, Graddy R, Leclercq G, Zhang W, et al. Mouse TCRalphabeta+CD8alphaalpha intraepithelial lymphocytes express genes that down-regulate their antigen reactivity and suppress immune responses. J Immunol. (2007) 178:4230–9. doi: 10.4049/jimmunol.178.7.4230
52. Poussier P, Ning T, Banerjee D, Julius M. A unique subset of self-specific intraintestinal T cells maintains gut integrity. J Exp Med. (2002) 195:1491–7. doi: 10.1084/jem.20011793
53. Kulkarni RR, Gaghan C, Mohammed J, Sharif S, Taha-Abdelaziz K. Cellular immune responses in lymphoid tissues of broiler chickens experimentally infected with necrotic enteritis–producing clostridium perfringens strains. Avian Dis. (2023) 67:186–196. doi: 10.1637/aviandiseases-D-23-00012
54. Boodhoo N, Shojadoost B, Alizadeh M, Kulkarni RR, Sharif S. Ex Vivo Differential Responsiveness to Clostridium perfringens and Lactococcus lactis by Avian Small Intestine Macrophages and T Cells. Front Immunol. (2022) 13:807343 (22. doi: 10.3389/fimmu.2022.807343
55. Ismail AS, Behrendt CL, Hooper LV. Reciprocal interactions between commensal bacteria and gamma delta intraepithelial lymphocytes during mucosal injury. J Immunol. (2009) 182:3047–54. doi: 10.4049/jimmunol.0802705
56. Ismail AS, Severson KM, Vaishnava S, Behrendt CL, Yu X, Benjamin JL, et al. Gammadelta intraepithelial lymphocytes are essential mediators of host-microbial homeostasis at the intestinal mucosal surface. Proc Natl Acad Sci U.S.A. (2011) 108:8743–8. doi: 10.1073/pnas.1019574108
Keywords: necrotic enteritis, Clostridium perfringens, mucosal immunity, intraepithelial lymphocytes, gene expression, intestine, chickens
Citation: Majeed S, Hamad SK, Shah BR, Bielke L and Nazmi A (2024) Natural intraepithelial lymphocyte populations rise during necrotic enteritis in chickens. Front. Immunol. 15:1354701. doi: 10.3389/fimmu.2024.1354701
Received: 12 December 2023; Accepted: 06 February 2024;
Published: 22 February 2024.
Edited by:
Rami A. Dalloul, University of Georgia, United StatesReviewed by:
Susana Magadan, University of Vigo, SpainKyung-Woo Lee, Konkuk University, Republic of Korea
Charles Z. Li, Agricultural Research Service (USDA), United States
Copyright © 2024 Majeed, Hamad, Shah, Bielke and Nazmi. This is an open-access article distributed under the terms of the Creative Commons Attribution License (CC BY). The use, distribution or reproduction in other forums is permitted, provided the original author(s) and the copyright owner(s) are credited and that the original publication in this journal is cited, in accordance with accepted academic practice. No use, distribution or reproduction is permitted which does not comply with these terms.
*Correspondence: Ali Nazmi, bmF6bWkuMUBvc3UuZWR1