- 1College of Basic Medical Sciences, Shanxi University of Chinese Medicine, Jinzhong, China
- 2Basic Laboratory of Integrated Traditional Chinese and Western Medicine, Shanxi University of Chinese Medicine, Jinzhong, China
Hepatocellular carcinoma (HCC) is a prevalent malignancy, often associated with compromised immune function in affected patients. This can be attributed to the secretion of specific factors by liver cancer cells, which hinder the immune response and lead to a state of immune suppression. Polysaccharides derived from traditional Chinese medicine (TCM) are valuable constituents known for their immunomodulatory properties. This review aims to look into the immunomodulatory effects of TCM polysaccharides on HCC. The immunomodulatory effects of TCM polysaccharides are primarily manifested through the activation of effector T lymphocytes, dendritic cells, NK cells, and macrophages against hepatocellular carcinoma (HCC) both in vivo and in vitro settings. Furthermore, TCM polysaccharides have demonstrated remarkable adjuvant antitumor immunomodulatory effects on HCC in clinical settings. Therefore, the utilization of TCM polysaccharides holds promising potential for the development of novel therapeutic agents or adjuvants with advantageous immunomodulatory properties for HCC.
1 Introduction
According to the data provided by the World Health Organization (WHO), hepatocellular carcinoma (HCC) is the world’s sixth most common malignant tumor and the third leading cause of cancer death worldwide in 2020 (1). It is characterized by a short duration of illness and is difficult to heal, and the invasion, metastasis, and spread of HCC cells are the reasons for the high mortality rate of this disease (2). The conventional treatment of HCC is still mainly surgical resection, supplemented by drug therapy, but the disadvantage of surgical treatment of HCC is its high risk and challenging nature (3). The recurrence rate of surgical treatment is as high as 70% within 5 years (4). The effectiveness of traditional therapies for HCC is not satisfactory, so it is increasingly urgent to find new therapeutic methods for HCC. The early research on HCC mainly focuses on the tumor cells themselves. Still, with the deepening of the research, the focus has shifted to the surrounding tissue environment of the tumor. Especially, it has been reported that the occurrence, progression, and recurrence of HCC are closely related to its immune microenvironment (5–7).
The immune system has the functions of immune surveillance, defense, and regulation, and is composed of immune organs, immune cells, and immune active substances. The reason why tumors can escape the immune surveillance of the body is principally because they can produce excessive immunosuppressive factors, resulting in poor antitumor immune function in patients, which is not enough to produce a strong antitumor immune response to clear tumor cells (8–10). Therefore, the immunosuppressive microenvironment of HCC can provide a favorable condition for tumor growth, and immunotherapy is one of the effective methods to inhibit HCC growth (11).
Since the Japanese scholar Haoro Chihara first discovered in 1968 that Lentinan polysaccharide regulates tumor immune response, an upsurge in the research on the regulation of tumor immune response by Traditional Chinese Medicine (TCM) polysaccharides is booming (12). Polysaccharide is a linear or branched polymeric sugar composed of more than 10 monosaccharides connected by a glycosidic bond, which can be expressed by the general formula (C6H10O5) n (13). TCM polysaccharide is an effective polysaccharide extracted from a single Chinese medicine by modern scientific and technological methods. It mainly consists of glucose (Glu), rhamnose (Rha), mannose (Man), galactose (Gal), xylose (Xyl), arabinose (Ara), ribose (Rib), fructose (Fru), sucrose (Suc), fucose (Fuc), and other monosaccharide components (14, 15), which has anti-aging, anti-tumor, immune regulation and other functions (16–19). A large number of clinical trials and experimental studies have proved that some TCM polysaccharides (especially those extracted from the TCM with possible anti-tumor effect) have a certain function of regulating tumor immune response, with mild or no toxic and side effects at effective doses. For example, Oenothera biennis Polysaccharide (Evening primrose Polysaccharide) not only has a comparable antitumor effect to 5-Fluorouracil (5-FU) but has protective effects on liver and kidney function which is reflected in the low levels of alanine aminotransferase (ALT), aspartate aminotransferase (AST) and alkalines phosphatases (ALP), creatinine and urea in serum. Nonetheless, 5-FU administration to H22 tumor-bearing mice could not reverse the hepatic and renal damage, behaving a high level of ALT, AST, ALP, creatinine, and urea (20). However, TCM polysaccharides have large molecular weights and complex chemical structures, and the interaction mechanism between TCM polysaccharides and the immune system is still unclear.
In this article, the immunomodulatory effects of TCM polysaccharides on HCC, including their roles in regulating tumor adaptive immune response and innate immune response, were reviewed. We aim to provide a scientific basis for the development of novel therapeutic agents or adjuvants for this malignant tumor with beneficial immunomodulatory properties.
2 Role of TCM polysaccharides played in regulating tumor innate immune response
There are many types of cells of the myeloid lineage involved in innate immunity, including dendritic cells (DCs), natural killer (NK) cells, macrophages, monocytes, polymorphonuclear cells, mast cells, and so on. The roles of innate immunity in antitumor responses are related to that innate immune cells can detect tumors via some receptors, induce and amplify adaptive immune responses (such as that DCs are required for T-cell activity against tumors as the antigen-presenting cells), and exert direct effector responses (such as phagocytosis for macrophages and polymorphonuclear cells, and natural cytotoxicity for NK cells) (21). Toll-like receptors (TLRs) and some other pattern recognition receptors (PRRs) or integrin receptors are common receptors that play a crucial part in the innate immune system and may the critical targets for activating the antitumor response of the innate immune cells by TCM polysaccharide (Figure 1).
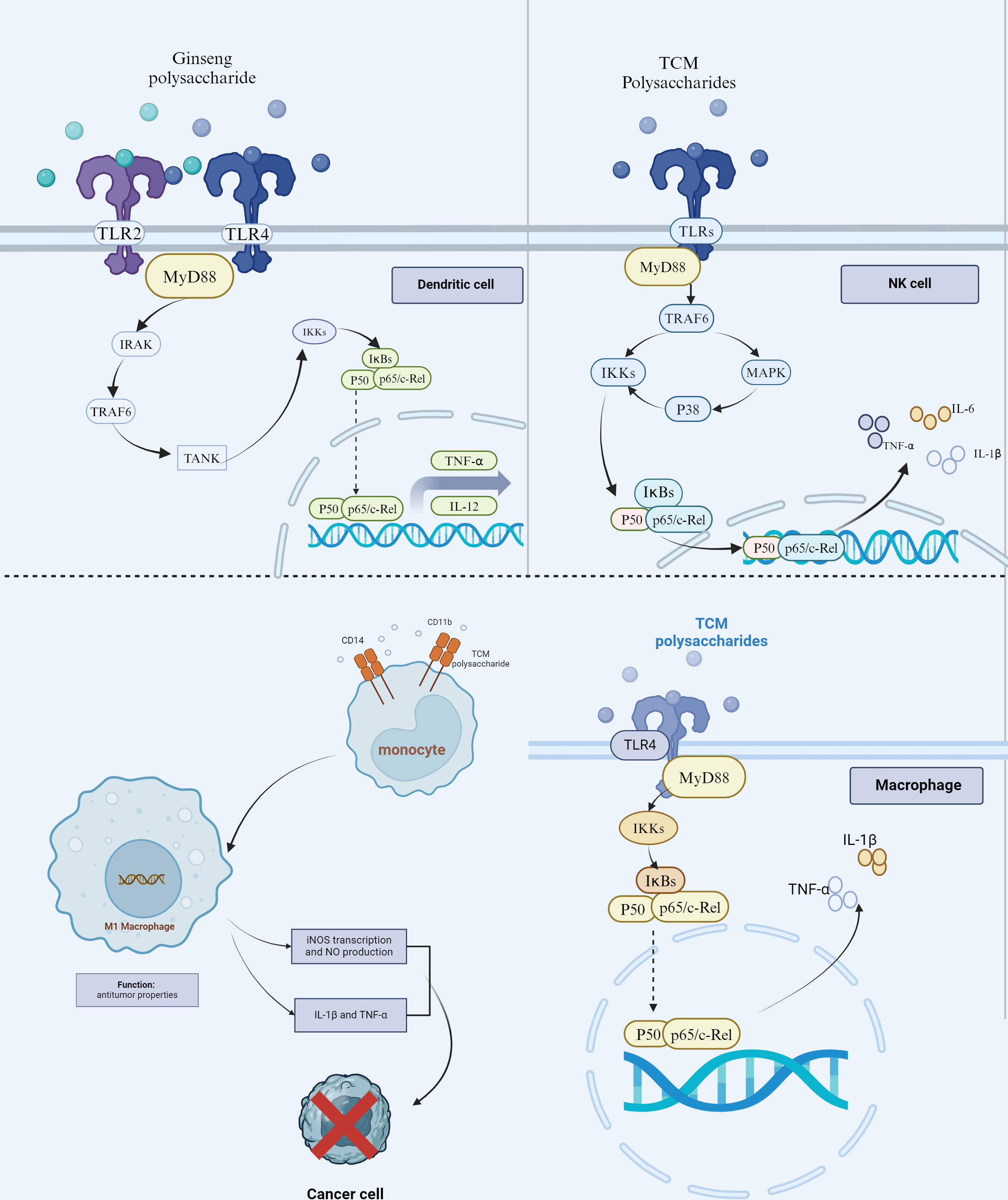
Figure 1 TCM polysaccharides’ role in regulating tumor innate immune response. TCM polysaccharides (such as Ginseng polysaccharides) can trigger the DCs to secrete the TNF-α and IL-12 through the typical NF-κB signaling pathway by binding to TLR4/TLR2. Meanwhile, TCM Polysaccharides promote NK cells to kill tumor cells by directly recognizing the TLRs-MyD88 complex and eventually activating the MAPK and NF- κ B signaling pathway to produce TNF-α, IL-1β, and IL-6. TCM Polysaccharides (such as Astragalus membranaceus Polysaccharide) enhance the differentiation of monocytes into M1 macrophages through the combination with CD11 and CD14/TLR-4 complex. The elevated transcription of iNOS and expression of NO, TNF-α, and IL-1β is helpful in inhibiting tumor growth. TCM Polysaccharides can also activate the macrophages directly by binding to the TLR4.
2.1 TCM polysaccharides involved in immune regulation of DCs
DCs are the initiators of the immune response (22). It has a strong antigen-phagocytosis in the immature, while a strong antigen-presenting function after maturity. DCs can stimulate the activation of initial T lymphocytes and play a key role in inducing anti-tumor immune response (23, 24). Studies have found that although there was sufficient DCs infiltration in the tumor microenvironment, these cells lose their due function of presenting tumor antigen and activating T lymphocytes, as well as are prone to apoptosis (25). Recently, studies have shown that promoting the maturation of DCs and enhancing their antigen-presenting effect can play a significant role in tumor treatment and vaccine preparation (26, 27).
TCM polysaccharides, such as Ginseng Polysaccharide (28), Rehmannia Polysaccharide (29), and Laminaria Polysaccharide (30), could also possess significant pharmacological effects against HCC. Ginseng Polysaccharide (31), Rehmannia Polysaccharide (32), and Laminaria Polysaccharide (33) can promote the maturation of bone marrow DCs (BMDCs), which were extracted from the femurs and tibias of female C57BL/6 mice, by down-regulating the activity of acid phosphoenzyme. After being treated with these TCM polysaccharides, the matured BMDCs manifested decreased phagocytosis activity and increased antigen-presenting ability. Furthermore, the decreased number of lysosomes, upregulated expression of membrane molecules at the protein level (including CD80, CD83, CD86, CD40, and MHC II), enriched secretion of interleukin (IL)-12 and tumor necrosis factor (TNF)-α could also be detected. All these pharmacologic effects of TCM polysaccharide together induced the T lymphocytes to be active to produce a powerful anti-tumor immune response at last.
Further empirical studies for the mechanism of activating the BMDCs by Ginseng polysaccharide have been proceeded in vivo with male Kunming mice and in vitro with BMDCs and T cells isolated from the BALB/c mice, because Ginseng is one of the most commonly used Chinese medicinal materials for anti-HCC (34). Ginseng Polysaccharide can promote the maturity of BMDCs by TCM Polysaccharide binding to the PRRs, such as TLR 4, TLR 2, and mannose receptor (MR) because Ginseng Polysaccharide consists of different monosaccharide components, including glucose, mannose fractions, galactose, and galacturonic acid fractions, etc. The glucose and mannose fractions are prone to be identified by TLR2 and MR, while galactose and galacturonic acid fractions can be recognized by TLR4 (35, 36). Once binding to TLR4/TLR2, the MyD88-mediated NF-κB signaling pathway could be triggered by Ginseng polysaccharide, leading to the functional maturation of BMDCs and the secretion of the proinflammatory cytokines (including TNF-α and IL-12). Ginseng Polysaccharide-induced secretion of IL-12 and TNF-α protein from BMDCs can be inhibited by admiration of antibodies against TLR2 and TLR4 (37). Therefore, the TLR4/TLR2-MyD88-NF-κB signaling pathway may play a vital role in the DCs-mediated anti-HCC effect induced by Ginseng Polysaccharide.
2.2 TCM polysaccharides involved in immune regulation of NK cells
NK cells are derived from bone marrow lymphoid stem cells, which do not express specific antigen-recognition receptors (38). They can recognize themselves and non-themselves through surface activating receptors and inhibitory receptors without antigen pre-stimulation, and directly kill tumor cells and virus-infected target cells by secreting granzyme and perforin (39, 40). Nowadays, NK cells have been considered an attractive, but underexplored, therapeutic target in HCC because of their role in immunosurveillance and their ability to eliminate malignant tumor cells (41). It was found that polysaccharides from various TCMs can improve the activity of NK cells to kill tumor cells, such as Oenothera biennis Polysaccharide (20), Gynostemma pentaphyllum Polysaccharide (42), and Kaempferia galanga L Polysaccharide (43). In some in vivo studies with H22 tumor-bearing mice, these three TCM Polysaccharides all could effectively inhibit the solid tumor growth of H22 hepatocarcinoma transplanted in mice, as well as elevate the body weight, spleen/thymus indexes and splenocyte proliferation of mice, through promoting the activity of NK cells in tumor-bearing mice.
Like some pathogen-associated molecular patterns (such as peptidoglycan and Lipopolysaccharide), TCM Polysaccharides can activate NK cells to kill tumor cells by directly recognizing TLRs, because they always have the components of glucose, galactose, and galacturonic acid fractions. Once these components bind to TLRs expressed on the NK cell’s surface, the TLRs-MyD88 complex will be formed to promote the activity of downstream transcription factors, including mitogen-activated protein kinases (MAPKs) and NF-κB, and the phosphorylation of its downstream proteins. It has been reported that the TCM Polysaccharides could enhance NK cell cytotoxicity against tumor cells via TLR4/MAPKs/NF-κB pathway, promote the TLR4-dependent production of interferon (IFN)-γ, and upregulate the protein level expression of CD69 in spleen NK cells (44, 45).
Taken together, these findings indicate that TCM Polysaccharides (at least including Oenothera biennis polysaccharide, Gynostemma pentaphyllum Polysaccharide, and Kaempferia galanga L Polysaccharide) have anti-HCC activity partly via improving the immune responses of NK cells, and the TLRs on the surface of NK cells may be the important binding-sites.
2.3 TCM polysaccharides involved in immune regulation of tissue-resident macrophages
The tissue-resident macrophages, like Kupffer cells in the liver, are characterized by their persistence in adults and associated with specialized tissue cells stably and closely (46). They have a variety of PRRs, modulatory receptors, and cytokine receptors on their surface, which can directly kill targets (such as pathogens, intracellular parasitic bacteria, and tumor cells), participate in the inflammatory response, process, and present antigens to initiate adaptive immune responses and secrete cytokines to regulate the immune response (47). In response to different stimuli and signaling, macrophages with antitumor properties or with a tumor-promoting capacity are classified as M1-like or M2-like phenotype (48). Tumor cells take advantage of this feature to generate more M2 cells and thus promote their growth (48). Zebrafish inflammation model studies have proved that the immunomodulatory effect of TCM polysaccharides may be related to the regulation of macrophage phagocytosis and the enhancement of antigen-presenting function (49, 50). For instance, Platycodon grandiflorus Polysaccharide was found to markedly activate iNOS transcription and NO production in RAW 264.7 cells and mouse peritoneal macrophages isolated from wild-type C3H/HeN, and anti-CD14 or anti-CD 11b antibody decreased NO production after the administration of Platycodon grandiflorus Polysaccharide in spleen-resident macrophages in BDF1 mice (51, 52). CD14, as an anchoring protein and a ubiquitous PRR, is primarily understood to act as a co-receptor for TLRs to activate innate immunity responses to pathogens and tissue injury (53). As the α-chain of integrin receptor CD11b/CD18 (also known as αMβ2, Mac-1, and CR3), CD11b is highly expressed on the surface of innate immune cells, including macrophages and neutrophils. It is a new target for tumor immunotherapy. When CD11b activity is elevated, the number of M1 macrophages increases, while when CD11b becomes less, it leads to more M2 cell development (54). Therefore, it was indicated that CD14 and CD11b on the surface of macrophage may be the possible cellular binding sites of this TCM polysaccharide to exert anti-tumor effects. It has been proved that CD14 and CD 11b were the binding sites and effect targets of Platycodon grandiflorus Polysaccharide to macrophage, nevertheless, Platycodon grandiflorus Polysaccharide did not promote the proliferation of T cells, the expression of IL-2 in Th1 cells, or the expression of IL-4 in Th2 cells (52).
For another example, Astragalus membranaceus Polysaccharide could enhance the anti-tumor function of peritoneal macrophages isolated from BALB/c and C3H/HeJ mice by inducing them to produce cytokines (including TNF-α and IL-1β), and this effect can be eliminated in TLR-4 mutant C3H/HeJ mice or through the injection of TLR4 antibodies, proving that promotion the secretion of cytokines in macrophages by Astragalus membranaceus Polysaccharide is possibly related to the TLR4 binding (55).
When it comes to treating hepatocellular carcinoma, Astragalus membranaceus Polysaccharide could enhance the expression of M1 macrophage biomarkers (such as iNOS, IL-1β, and TNF-α) and M1 macrophage proportions, as well as could reduce the expression of M2 macrophage biomarkers (including IL-10, Arg-1) and M2 macrophage proportions in the tumor-associated macrophages (TAMs)/MHCC97H cells and TAMs/Huh7 cells co-cultured models. Moreover, the Astragalus membranaceus Polysaccharide-mediated elevation of the M1 phenotype of TAMs could significantly repress the ability of proliferation, migration, and invasion both in MHCC97H and Huh7 cells. On the other side of the coin, Astragalus membranaceus Polysaccharide could also enhance the M1/M2 macrophage ratio in the tumor tissues of Huh7-bearing BALB/c nude mice model and thus inhibit the growth of HCC in vivo (56). This anti-HCC effect of Astragalus membranaceus Polysaccharide may have a close relationship with the combination of CD11 and CD14/TLR-4 complex.
So to summarize, regulating the activity of innate immune cells, including DCs, NK cells, and macrophages through the membrane receptors such as TLRs, is one of the important mechanisms by which TCM polysaccharides exert the anti-HCC effect.
3 Role of TCM polysaccharides played in regulating tumor adaptive immune response
T lymphocytes and B lymphocytes are the main effector cells in the anti-tumor adaptive immune response (57, 58). However, the tumor itself can produce many immunosuppressive factors to repress the activation of effector T lymphocytes (Teffs) and B lymphocytes, thus, the immune microenvironment of the patients with tumors is in a state of immunosuppression (59, 60). Many TCM polysaccharides have strong activation of Teffs and B lymphocytes and can inhibit the growth of tumor cells (61). The molecular pathway of T lymphocytes activated by TCM polysaccharides is mainly through the T-cell receptor (TCR)/CD3 complex-mediated signaling pathway (including MAPK signaling pathway), while the regulatory effect on B lymphocytes activated by TCM polysaccharides is mainly bound to the IgM/CD79 complex receptor or TLR2/4 on the cell surface, and the MAPK and NF-κB signal transduction pathways are also involved in B lymphocytes activation (Figure 2).
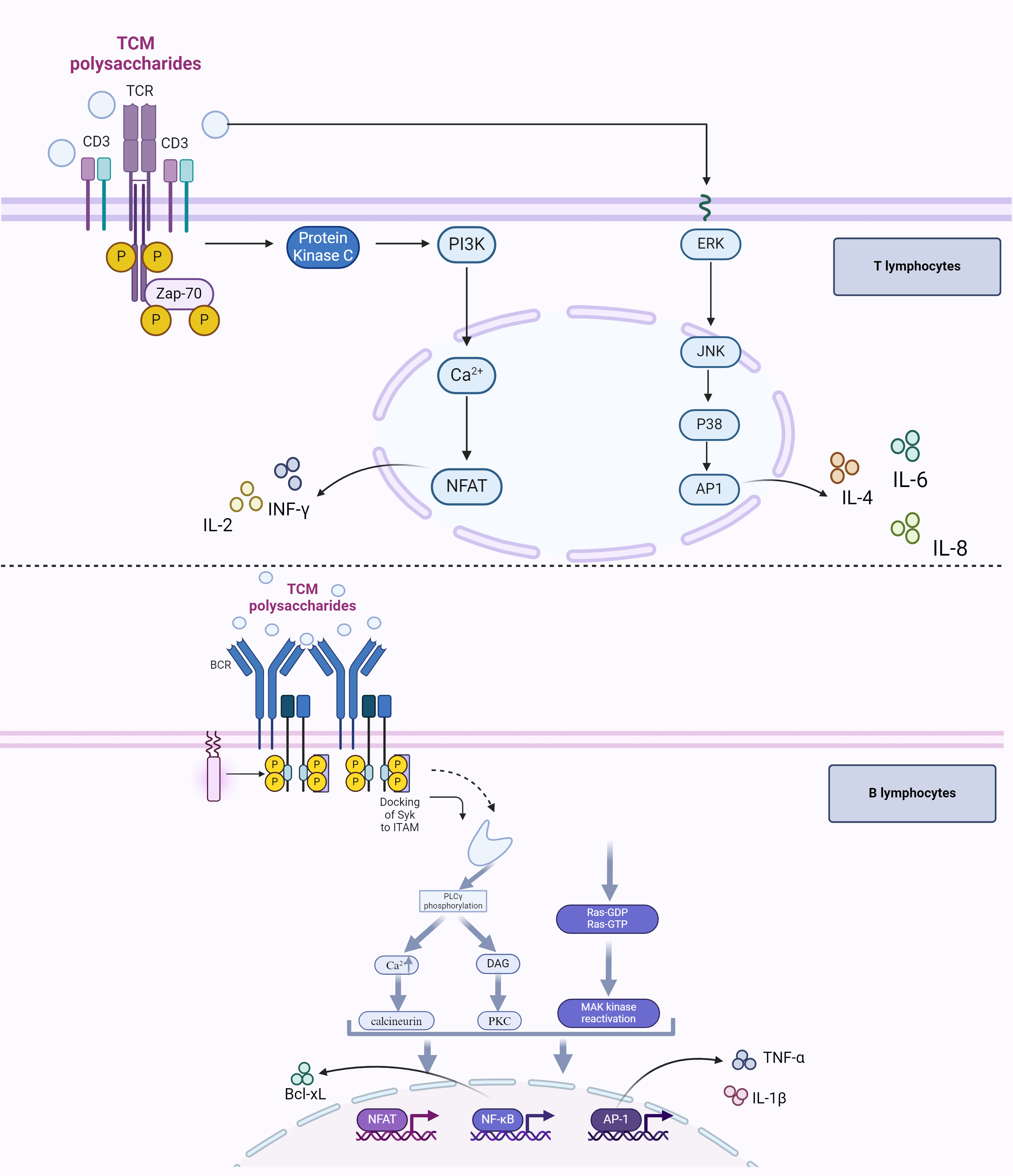
Figure 2 TCM polysaccharides’ role in regulating tumor adaptive immune response. Once recognized directly or indirectly by the TCR, TCM polysaccharides activate the PTK, and the latter plays an important role in antitumor by regulating the expression of cytokines, including IL-2, IL-4, IL-6, IL-8, and INF-γ, via PI3K or MAPK signaling pathway. B lymphocytes can also recognize TCM polysaccharides through mIg/CD79 complex or TLR 2/4 signaling cascades, which will lead to the elevation of nuclear translocation of NFAT/AP-1/NF-κB and regulate the expression of B lymphocyte-related genes, through the activation of the calcineurin/PKC/MAPK (ERK) signaling cascade.
3.1 Direct and indirect recognition of TCM polysaccharides by T lymphocytes
It is well known that many polysaccharides can be treated by MHC Class II molecules and presented to T cells for indirect recognition by TCR. TCM Polysaccharides could be degraded into small molecular weight carbohydrates by the NO system via the endocytosis pathway, and these small molecular weight carbohydrates can be processed and presented by MHC Class II molecules and recognized by TCR molecules (62). Brian (63) has demonstrated by laser confocal method that the adhesion of antigen-presenting cells and T lymphocytes occurred after the addition of polysaccharide antigens, and the co-localization of MHC Class II molecules, TCR molecules, and polysaccharide antigens could also be detected simultaneously. Moreover, polysaccharides can also be directly recognized by T lymphocytes in two ways. One is that polysaccharides can be presented to γδ T cells by CD1 complex via intracellular pathways (64), and another is that polysaccharides (as a kind of hapten) can be simultaneously presented by MHC class II molecules and recognized by TCR molecules, in the form of glycoprotein or sugar linkage (65).
Once recognized directly or indirectly by the TCR, TCM polysaccharides will possess good immune-promoting effects by activating the protein tyrosine kinase (PTK) (66). PTK will upregulate the expression of genes involved in immune response (including IL-2, IL-4, IL-6, IL-8, and INF-γ) at both the transcriptional and translational levels through the PI3K or MAPK signaling pathway: (1) The protein kinase C (PKC) can be stimulated to migrate from cytoplasm to membrane by PTK via PI3K signaling pathway and result in the increase of Ca2+ concentration in cytoplasm. After being activated by the intracytoplasmic accumulation of Ca2+, calcineurin induces the nuclear translocation of the nuclear factor of activated T cells (NAFT) and elevates its transcription factor activity, leading to a high level of expression of IL-2 and INF-γ. (2) PTK can induce the nuclear translocation of activator protein 1 (AP1) via the MAPK signaling pathway (ERK/JNK/p38). As a transcription factor associated with immune regulation, AP1 in the nucleus can induce gene expression, such as IL-4, IL-6, and IL-8 (67, 68).
3.2 Recognition of TCM polysaccharides by B lymphocytes
3.2.1 Membrane immunoglobulin complex receptor-mediated recognition
The membrane immunoglobulin (mIg) receptor is the most important receptor and characteristic sign on the surface of B lymphocytes. It can form a mIg complex receptor with CD79b, which is known as the B cell receptor (BCR), to recognize antigens and regulate B lymphocyte activation. When mIg/CD79 binds to TCM polysaccharides, the calcineurin/PKC/MAPK (ERK) signaling cascade will be activated to induce the nuclear translocation of NFAT/AP-1/NF-κB, which will regulate the expression of B lymphocyte-related genes (69).
3.2.2 TLR 2/4 signaling cascades mediated recognition
TLR2/4 receptor also exists on the surface of B lymphocytes, and TCM polysaccharide can bind to the TLR2/4 receptor on the surface of B lymphocytes to regulate the transcription of related genes via the MAPK signaling pathway or NF-κB signaling pathway. Studies have shown that Acanthopanax Polysaccharide can significantly stimulate the proliferation of B lymphocytes in C3H/HeN mice by activating MAPKs (such as Erk1/2, p38, and JNK), and the transcription factor NF-B, but when cultured with TLR4 antibody or TLR2 antibody, the proliferation of B lymphocytes is significantly inhibited. These results indicated that Acanthopanax polysaccharide could bind to the TLR2/4 receptor on the surface of B lymphocytes to promote the proliferation of B lymphocytes (70). However, polymyxin B (PMB), a specific inhibitor of LPS, did not significantly affect the activities of Acanthopanax polysaccharide on B lymphocytes, which indicated that Acanthopanax polysaccharide could activate the TLR signaling cascades differently from LPS.
To summarize briefly, activating the T lymphocytes and B lymphocytes is another important mechanism for TCM polysaccharides to anti-HCC. The TCR, BCR, and TLRs are the main binding sites for TCM polysaccharides to induce the activation of immune cells in the adaptive immunity system.
4 Experimental and Clinical trials on HCC of TCM polysaccharides by modulating immune response
4.1 Experimental trial on HCC of TCM polysaccharides by modulating immune response
4.1.1 Trametes robiniophila Murr polysaccharide
Trametes robiniophila Murr is a kind of light brown agaric growing on the trunk of the Sophora tree, belonging to Agaricales and Basidiomycetes. It is also known as Huaier and has been used in traditional Chinese medicine for nearly 1,600 years and was first documented by Li Shizhen of the Ming Dynasty (71). In recent decades, Trametes robiniophila Murr has been discovered and used as a supplement in the treatment of cancer, especially has achieved satisfactory results for anti-HCC (72, 73). The accumulated evidence shows that the anti-tumor mechanism of Trametes robiniophila Murr is mainly to inhibit the proliferation of endothelial cells, interfere with the formation of tumor blood vessels, activate the immune system, induce the apoptosis of tumor cells, and inhibit the proliferation of tumor cells (74, 75). Trametes robiniophila Murr Polysaccharide, one homogenous polysaccharide purified from Huaier, exerted more potent antitumor activity in inhibiting HCC growth (76). In an HCC H22-based mice experiment, Trametes robiniophila Murr Polysaccharide significantly repressed the growth and the pulmonary metastasis of the transplanted H22 solid HCC in vivo. While prolonging the live time of mice bearing H22 tumors, Trametes robiniophila Murr Polysaccharide elevated the relative weight of immune organs (spleen and thymus) and lymphocyte proliferation reaction notably, including an increasing percentage of CD4+ T cells and natural killer (NK) cells, whereas a decreasing number of CD8+ T cells. Besides, the administration of Trametes robiniophila Murr Polysaccharide promoted the secretion of immune-stimulating serum cytokines (IL-2 and IFN-γ) but inhibited the production of immune-suppressing serum cytokines (IL-10) in H22-bearing mice (77). It suggested that Trametes robiniophila Murr Polysaccharide could exhibit prominent antitumor activities via enhancement of host immune system function, and could be developed individually as a potent immunological response modifier for HCC therapy.
4.1.2 Salvia miltiorrhiza polysaccharide
Salvia miltiorrhiza (also named Danshen) is the dried root of the Chinese medical plant Salvia miltiorrhiza Bunge (Labiatae), and in the clinical practice of TCM, it has long been used for clearing heat for detumescence, soothing the nerves and tranquilizing the mind (78, 79). In line with the Chinese medicine theory, Salvia miltiorrhiza was commonly used for the prevention and treatment of myocardial infarction, apoplexy, hepatitis, tumors, and immunological disorders (80, 81). At the same time, Salvia miltiorrhiza has shown a good application prospect for the treatment of liver fibrosis and HCC (82–84). Polysaccharide is one of the main immunomodulatory active components in the water extract of Salvia miltiorrhiza, and it can dose-dependently promote the proliferation of T lymphocytes and B lymphocytes of the cancer patients and significantly improve the cytotoxicity of T lymphocytes against cancer cells, as well as up-regulated the gene expression of cytokines including IL-4, IL-6, and IFN-γ (85, 86). It has been proved that Salvia miltiorrhiza Polysaccharide can suppress the proliferation of H22 cells on tumor-bearing mice in vivo, by alleviating the apoptosis of tumor transplantation-induced CD4+ T cell and dysregulating of serum cytokine profiles (such as prostaglandin E2), as well as elevating the cytotoxic activities of NK cells and CD8+ T cells (87). Moreover, Salvia miltiorrhiza Polysaccharide also substantially declined the intracellular cyclic adenosine monophosphate (cAMP) in CD4+ T cells, which subsequently elevated the protein levels of JAK3 and enhanced the STAT5 or STAT5 phosphorylation-dependent expression of anti-apoptotic genes (87). These works provided a new perspective for the application of Salvia miltiorrhiza against HCC and offered experimental evidence for using Salvia miltiorrhiza Polysaccharide as an adjunct reagent in the clinical treatment of HCC.
4.1.3 Ganoderma lucidum polysaccharide
As a versatile traditional Chinese herb and nutraceutical, Ganoderma lucidum has been applied to treat multiple diseases in the clinical practice of TCM, including digestive disorders, cardiovascular system disease, and nervous system disease (88–90). Among all of its extracts, Ganoderma lucidum Polysaccharide is the main bioactive component and possesses many therapeutic effects, such as antitumor, immunoregulatory, anti-oxidant, and anti-neurodegenerative activities (91–93). It has been reported that Ganoderma lucidum Polysaccharide is capable of suppressing the growth of tumors in hepatoma-bearing Kunming and BALB/c mice associated with an increase of the ratio of Teffs to Tregs, through increasing the secretion of IL-2 to eliminate the suppression effect of Treg on the proliferation of Teffs (94). The ability of Ganoderma lucidum Polysaccharide to suppress the proliferation of Treg is possibly related to inhibiting the gene transcription level expression of Foxp3 by elevating the expression of miR-125b, and in the hepatoma-bearing mice, miR-125b inhibitor abolished the effect of Ganoderma lucidum Polysaccharide on tumor growth (94).
4.1.4 Strongylocentrotus nudus eggs polysaccharide
Strongylocentrotus nudus is another kind of precious traditional Chinese medicine, which has the remarkable effect of anti-tumor (95). As a D-glucan containing an α-1, 4-linked backbone, and α-1, 3-linked branches, Strongylocentrotus nudus Egg Polysaccharide is extracted and purified from sea urchins’ eggs (96). Strongylocentrotus nudus Egg Polysaccharide is widely accepted as a bioactive anticancer compound with profound inhibitory effects on tumor growth closely related to its immunomodulatory biological activity (44, 97). Numerous pharmacological studies have indicated that Strongylocentrotus nudus egg polysaccharide is an anticancer candidate working by activating T lymphocytes and B lymphocytes (98, 99). In several experiments of a mouse model of H22 hepatocellular carcinoma, Strongylocentrotus nudus Egg Polysaccharide could inhibit the tumor growth of H22-bearing imprinting control region (ICR) mice and stimulate the proliferation of T lymphocytes and B lymphocytes in a dose-dependent manner, involving not only remarkably enhance the spleen and thymus indices, CD4+ and CD8+ T cell numbers as well as CTL activity, but it also elevated IgA, IgM and IgG levels in the serum. At the same time, the IL-2 and TNF-α secretion in serum also elevated after the admiration of Strongylocentrotus nudus Egg Polysaccharide (98, 100). Furthermore, Strongylocentrotus nudus Egg Polysaccharide could also induce the proliferation of splenocytes isolated from the ICR mice in vitro, and this effect could be inhibited by the TLR2 and TLR4 monoclonal antibodies, which indicated that Strongylocentrotus nudus Egg Polysaccharide may mediate splenocyte proliferation via TLR2 and TLR4 (100). These studies demonstrate that Strongylocentrotus nudus Egg Polysaccharide can effectively inhibit HCC via enhancement of adaptive immune system function, and it could be a potential therapeutic drug for hepatocarcinoma.
4.1.5 Radix Glycyrrhizae polysaccharide
Treg cells in the peripheral blood of tumor patients (including HCC) increased significantly and inhibited the function of dendritic cells (DCs) and Teffs through the secretion of IL-10 and transforming growth factor (TGF)-β, thus inhibiting the anti-tumor immune response of the body (101, 102). Increased levels of Treg cells have been detected in the peripheral blood, the primary tumor microenvironment, and the draining lymph nodes of cancer patients (103–105). Therefore, interventions targeting Treg cells have become the new strategy of many therapies for malignant tumors (106, 107). Radix Glycyrrhizae is a commonly used traditional Chinese herbal medicine with a wide range of pharmacological functions throughout Chinese history, and the polysaccharide is a major active compound extracted from it, which is cytotoxic to cancer cells (108, 109). An in vivo experimental study with H22 hepatocarcinoma-bearing BALB/c mice has shown that Radix Glycyrrhizae Polysaccharide could down-regulate the population of Treg cells and decrease the expression of Foxp3 mRNA and IL-10 mRNA in the lymph node. At the same time, Radix Glycyrrhizae Polysaccharide treatment could also influence the level of the Th1/Th2 cytokines in serum by decreasing the level of IL-10 and TGF-β and increasing the level of IL-2 and IL-12p70 (110). It was indicated that the mechanism of Radix Glycyrrhizae Polysaccharide on lowering the proportion of Treg cells may be related to the regulation of the expression of Foxp3 and balance of the secretion of Th1/Th2 cytokines.
4.2 Clinical trials on HCC of TCM polysaccharides by modulating immune response
The traditional clinical treatment of HCC is surgery, radiotherapy, and chemoradiotherapy. At present, immunotherapy has become one of the most promising areas in tumor therapy, and regulating the biological function of immune cells has been proven to be a powerful weapon to exert anti-HCC effects, and has been applied more and more in clinical practice (111, 112). As natural active macromolecules with a wide range of sources, TCM polysaccharides can achieve anti-tumor effects by enhancing immune regulation, inhibiting tumor growth, tumor cell invasion and metastasis, and the development of tumor microenvironment (TME). Compared with the traditional anti-tumor treatments, TCM polysaccharides show the advantages and characteristics of multi-pathway and multi-target action. At the same time, according to some clinical observational studies or randomized controlled trials (RCT), it can improve the sensitivity of tumor cells to radiotherapy and chemotherapy, reduce adverse reactions, reduce the risk of tumor resistance and recurrence, and play a positive role in HCC treatment (113). Therefore, the participation of TCM polysaccharides in the adjuvant treatment during or after tumor surgery or chemoradiotherapy has attracted more and more attention from researchers, and many TCM polysaccharides have been used in clinical adjuvant therapy on HCC to enhance clinical efficacy and reduce toxic side effects.
The most widely used TCM polysaccharide in the clinical treatment of HCC is Lentinan polysaccharide. The results of three RCT researches involving 374 HCC patients, which were conducted in Shandong Province, Henan Province, and Tianjin City in China, showed that compared with the patients that only treated with transcatheter arterial chemoembolization (TACE), administration of Lentinan polysaccharide after TACE could increase the tumor volume reduction rate, NK cell activity, CD4+ T cell level, CD4+/CD8+ ratio and the content of IL-2 in serum, as well as decrease the level of CD8+ T cells and the content soluble IL-2 receptor (sIL-2R) in serum. However, there was no statistical difference in alpha-fetoprotein (AFP) reduction rate between the two treatments (114, 115). Another meta-analysis involving 7 randomized controlled trials, a total of 577 HCC patients, showed that compared with TACE alone, Lentinan polysaccharide adjuvant TACE may improve the 1-year survival rate of HCC patients, and increase the number of CD4+ T cells and CD4+/CD8+ ratio in peripheral blood of patients (116). Moreover, intraperitoneal injection of Lentinan polysaccharide in the treatment of advanced HCC patients with ascites could also improve the symptom remission rate and Karnofsky Performance Status (KPS) score (117). All the results indicated that Lentinan polysaccharide has certain advantages in the therapy of HCC as an adjuvant by improving the immunity of the patients. In addition to positively regulating the activation of Teffs, Lentinan polysaccharide can also negatively regulate the proliferation of Treg cells in HCC patients. In a clinical observational trial involving 46 HCC patients, Lentinan polysaccharide could downregulate the proportion of Treg cells in the peripheral blood of the patients, and it would be more effective when administered immediately after TACE operation (118). These results suggested that Lentinan polysaccharide could significantly improve the immune status and promote the reconstruction of immune function of HCC patients after TACE operation, by reducing the toxic side effects of TACE therapy, inhibiting the growth of hepatocellular carcinoma cells and promoting the recognition of hepatocellular tumor antigen.
Overall, although the efficacy and the mechanism still need to be further evaluated and revealed, TCM polysaccharides do have very attractive potential in anti-HCC, according to the current studies. It is necessary to further improve the experimental design of laboratory studies and clinical trials, to evaluate the application prospect of TCM polysaccharides in the treatment of HCC more objectively.
5 Deficiency and prospects
The detailed information on the TCM polysaccharides involved in this review is exhibited in Table 1 (20, 119–129), and their application in treating HCC in animal experiments and clinical trials has been summarized in Table 2. And in Table 3, the mechanism of action of different TCM polysaccharides with different immune cells was also exhibited. Based on the current research status in this field, TCM Polysaccharide, as an adjunct therapeutic drug, may have a very broad application prospect in the clinical treatment of HCC. It is intriguing to speculate that TCM polysaccharides can directly activate innate immune response or adaptive immune response to anti-HCC by mediating TLR signaling because natural TCM has been safely used for a long period in many oriental countries. Moreover, they have not been associated with any detrimental tissue injuries, which can be caused by LPS, at their biologically effective concentrations.
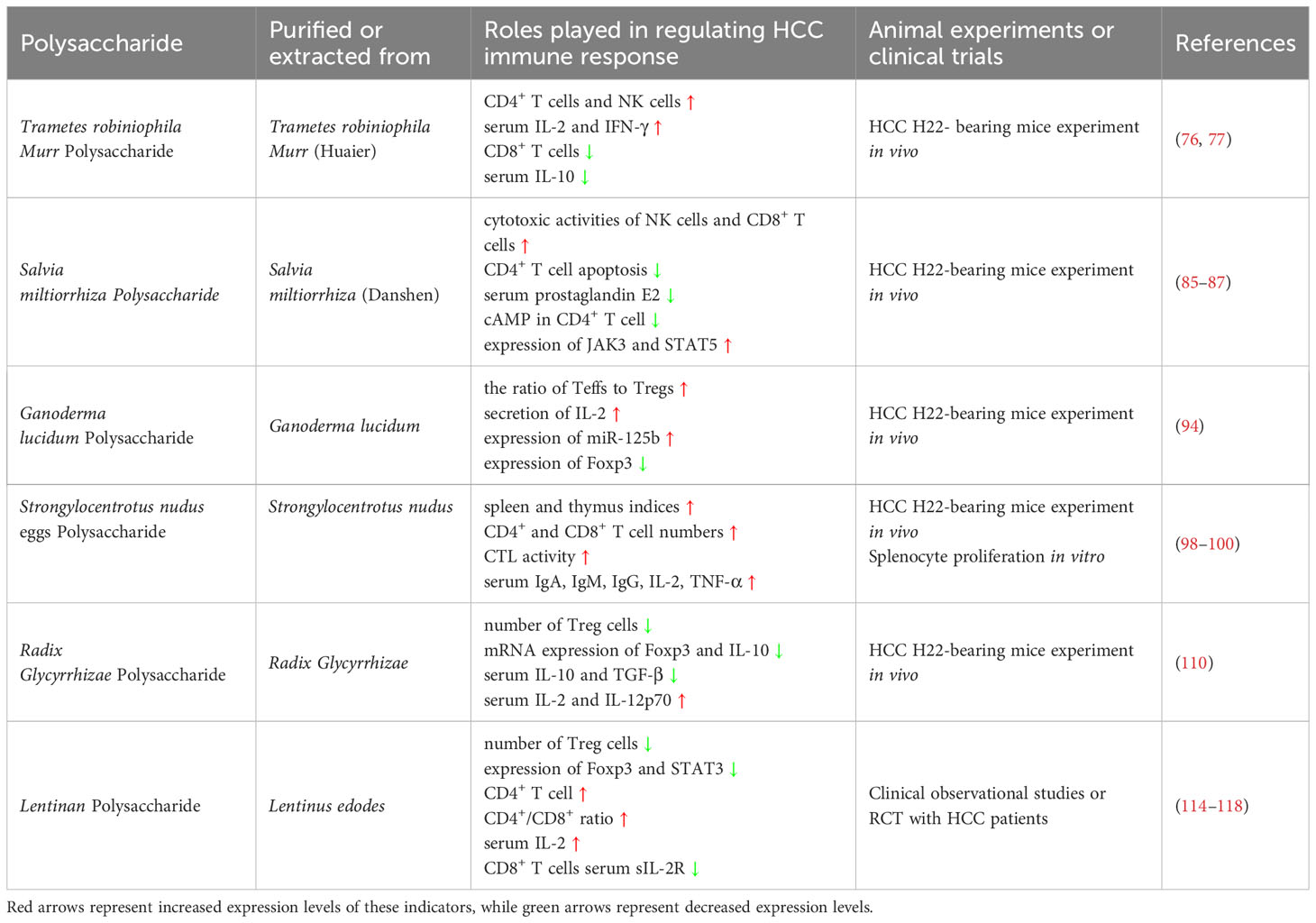
Table 2 The application of TCM Polysaccharide in treating HCC in animal experiments and clinical trials.
However, there are still shortcomings in the research, and the clinical application prospects can be further improved from the following aspects: (1) Most of the current reports on the regulation of anti-tumor immunity by TCM polysaccharides focus on the effects on the number, phenotype, activity and signaling pathway of single immune cells, but ignore that the interaction of multiple cells in the tumor immune microenvironment also play a synergistic role in anti-tumor (130). The complexity and diversity of TCM polysaccharide structure make it have the characteristics of multi-pathway and multi-target action, which is particularly important for the prevention and treatment of systemic diseases, especially cancer, through the overall regulation of immune cells in the immune system (131). Therefore, it is one of the directions to break through the current research bottleneck to carry out research work from micro to macro, from single cell to cell network. (2) As bioactive macromolecules, TCM polysaccharides need to bind to pattern-recognition receptors on the cell membrane of immune cells to initiate immune regulation and cellular immune response. Different immune cells have different receptors, and polysaccharides from different TCM sources can bind to different receptors (35, 54, 132). Which receptor on the surface of immune cells is involved in TCM polysaccharide recognition and binding, as well as the induced cell cascade reaction has been a hot topic of research. However, the current research mainly focuses on the changes in signal molecules after polysaccharide action. Further studies on the types and functions of polysaccharide receptors can be used as another research direction to reveal the mechanism of specific targeting of TCM polysaccharides against immune cells to regulate and control the growth of HCC. (3) Intestinal microbiota is highly associated with the occurrence and development of HCC (133). The characteristic microbiota of the intestinal tract is significantly correlated with the function of immune cells in the host tumor immune microenvironment, and the dysbiosis of gut microbiota could increase bacterial translocation, which suppresses the activation of antigen-presenting cells such as DCs and macrophages and then leading to the declined IFN-γ/TNF-α production from T cell subsets to kill the tumor cells (134). Therefore, Intestinal microbiota can regulate the immune microenvironment of tumors and affect the development of tumors. TCM Polysaccharides can regulate the tumor immune microenvironment and play an anti-tumor role as prebiotics to maintain the diversity of intestinal microorganisms in the intestinal tract by proliferating the growth of beneficial bacteria and inhibiting the growth of pathogenic bacteria, thus activating the immune cells such as intestinal DCs and inducing the expansion of germinal centers (GC) to trigger the differentiation of the B cells (135). Moreover, the anti-tumor effects of TCM Polysaccharides probably also have a close relationship with the fecal short-chain fatty acid (SCFA) (136). SCFAs, including acetate, butyrate, and propionate, are the key productions from indigestible polysaccharides by bacterial fermentation (137). SCFAs can reduce the incidence of tumors by acting on B cells to secrete antibodies and increasing the level of aldehyde dehydrogenase in DCs to convert vitamin A to retinoic acid to activate the plasma cells (138, 139). Therefore, the research on “TCM polysaccharides-intestinal microbiota-tumor immune microenvironment-immune cells” can better explore the anti-tumor mechanism of TCM polysaccharides. (4) The biological activity of TCM polysaccharides is closely related to its primary and advanced structure, but the study of its structure-activity relationship is still a shortcoming in this field (140). Therefore, it is of great significance to further break through the key technical barriers of TCM polysaccharide preparation and characterization for the innovation and transformation of TCM polysaccharides and anti-tumor precision medicine. (5) We can also focus on the combined application of TCM polysaccharides with chemotherapy or immunotherapy drugs, the use of TCM polysaccharides as drug delivery carriers, and the construction of tumor-targeting ligands to regulate the tumor immune microenvironment and other research directions. (6) Clinical studies on the treatment of HCC by TCM polysaccharides are mainly carried out in China at present, and the research subjects are all Chinese patients (114–118), which may affect the conclusion of this study. At the same time, none of the literature mentioned the distribution hiding, blind method, loss of access, and intentionality analysis. It is suggested that a multi-center randomized controlled double-blind trial with a large sample should be carried out with a reasonable design and strict implementation to further verify the efficacy of TCM polysaccharides in treating HCC. In terms of methodology, full randomization and implementation of assignment concealment should be used to report trials according to consolidated standards of reporting trials (CONSORT) criteria, while long-term follow-up should be conducted including endpoint indicators (such as survival rate and median survival) and negative results reporting, to draw more reliable conclusions for clinical guidance.
6 Conclusion
TCM polysaccharides have a very broad application prospect in the research field of anti-HCC by regulating the innate and adaptive immune response. It is a key issue to accelerate and clarify the mechanism of TCM polysaccharides in regulating the immune regulation of HCC, which can provide a more comprehensive theoretical basis for the clinical application of TCM polysaccharides in tumor therapy and has important academic value and practical significance.
Author contributions
YL: Writing – original draft, Writing – review & editing. JW: Writing – original draft. HH: Writing – review & editing.
Funding
The author(s) declare that financial support was received for the research, authorship, and/or publication of this article. This study was funded by Construction project of high-level Traditional Chinese Medicine Key Discipline of National Administration of Traditional Chinese Medicine (zyyzdxk-2023022); Basic research of integrated Chinese and Western medicine on rheumatic immune diseases; Science and Technology Innovation Project of Shanxi University of Chinese Medicine (Grant Number: 2022TD2003); Key team of scientific and technological innovation talents of Shanxi Province with integrated Traditional Chinese and Western Medicine for preventing and treating rheumatological diseases (Grant Number: 202204051002033); Special project of scientific and technological cooperation and exchange in Shanxi Province (Grant Number: 202104041101013); Basic Laboratory of Integrated Traditional Chinese and Western Medicine, Shanxi University of Chinese Medicine.
Conflict of interest
The authors declare that the research was conducted in the absence of any commercial or financial relationships that could be construed as a potential conflict of interest.
Publisher’s note
All claims expressed in this article are solely those of the authors and do not necessarily represent those of their affiliated organizations, or those of the publisher, the editors and the reviewers. Any product that may be evaluated in this article, or claim that may be made by its manufacturer, is not guaranteed or endorsed by the publisher.
References
1. Kamal MA, Mandour YM, Abd El-Aziz MK, Stein U, El Tayebi HM. Small molecule inhibitors for hepatocellular carcinoma: advances and challenges. Molecules (Basel Switzerland). (2022) 27:5537. doi: 10.3390/molecules27175537.
2. Kim SW, Lee JM, Kim JH, Park SJ, Yoon JH, Joo I. Clinical feasibility of radiofrequency ablation using novel adjustable separable electrodes with a multipurpose needle for treating small hepatocellular carcinomas: a prospective single-center study. Int J Hyperthermia: Off J Eur Soc For Hyperthermic Oncology North Am Hyperthermia Group. (2023) 40:2235102. doi: 10.1080/02656736.2023.2235102.
3. Cho Y, Choi JW, Kwon H, Kim KY, Lee BC, Chu HH, et al. Transarterial chemoembolization for hepatocellular carcinoma: 2023 expert consensus-based practical recommendations of the Korean liver cancer association. Korean J Radiol. (2023) 24:606–25. doi: 10.3348/kjr.2023.0385.
4. Muñoz-Martínez S, Sapena V, García-Criado Á, Darnell A, Forner A, Belmonte E, et al. Risk of treatment failure and death after ablation in hepatocellular carcinoma patients-A multiparametric prediction. Cancers. (2023) 15:3269. doi: 10.3390/cancers15133269.
5. Donne R, Lujambio A. The liver cancer immune microenvironment: Therapeutic implications for hepatocellular carcinoma. Hepatol (Baltimore Md.). (2023) 77:1773–96. doi: 10.1002/hep.32740.
6. Llovet JM, Castet F, Heikenwalder M, Maini MK, Mazzaferro V, Pinato DJ, et al. Immunotherapies for hepatocellular carcinoma [J]. Nat Rev Clin Oncol. (2022) 19:151–72. doi: 10.1038/s41571-021-00573-2.
7. Li Z, Wang Y, Xing R, Zeng H, Yu X-J, Zhang Y-J, et al. Cholesterol efflux drives the generation of immunosuppressive macrophages to promote the progression of human hepatocellular carcinoma. Cancer Immunol Res. (2023) 11:1400–13. doi: 10.1158/2326-6066.CIR-22-0907.
8. Volponi C, Gazzillo A, Bonavita E. The tumor microenvironment of hepatocellular carcinoma: untying an intricate immunological network. Cancers. (2022) 14:6151. doi: 10.3390/cancers14246151.
9. Montella L, Sarno F, Ambrosino A, Facchini S, D’Antò M, Laterza MM, et al. The role of immunotherapy in a tolerogenic environment: current and future perspectives for hepatocellular carcinoma. Cells. (2021) 10:1909. doi: 10.3390/cells10081909.
10. Lee HL, Jang JW, Lee SW, Yoo SH, Kwon JH, Nam SW, et al. Inflammatory cytokines and change of Th1/Th2 balance as prognostic indicators for hepatocellular carcinoma in patients treated with transarterial chemoembolization. Sci Rep. (2019) 9:3260. doi: 10.1038/s41598-019-40078-8.
11. Lu Y, Sun Q, Guan Q, Zhang Z, He Q, He J, et al. The XOR-IDH3α axis controls macrophage polarization in hepatocellular carcinoma. J Hepatol. (2023) 79:1172–84. doi: 10.1016/j.jhep.2023.06.022.
12. Ikekawa T, Nakanishi M, Uehara N, Chihara G, Fukuoka F. Antitumor action of some Basidiomycetes, especially Phllinus linteus. Gan. (1968) 59:155–7.
13. Xie L, Yan H, Han L, Cui L, Hussain H, Feng Q, et al. Structural characterization and anti-inflammatory activity of neutral polysaccharides from American ginseng. Int J Biol Macromolecules. (2023) 248:125586. doi: 10.1016/j.ijbiomac.2023.125586.
14. Fang J, Li Y-X, Luo H-Y, Zhang W-H, Chan K-C, Chan Y-M, et al. Impacts of sulfur fumigation on the chemistry and immunomodulatory activity of polysaccharides in ginseng. Int J Biol Macromolecules. (2023) 247:125843. doi: 10.1016/j.ijbiomac.2023.125843.
15. Zhao W, Duan C, Liu Y, Lu G, Lyu Q, Liu X, et al. Modulating effects of Astragalus polysaccharide on immune disorders via gut microbiota and the TLR4/NF-κB pathway in rats with syndrome of dampness stagnancy due to spleen deficiency. J Zhejiang University. Science. B. (2023) 24:650–62. doi: 10.1631/jzus.B2200491.
16. Tuo W, Wang S, Shi Y, Cao W, Liu Y, Su Y, et al. Angelica sinensis polysaccharide extends lifespan and ameliorates aging-related diseases via insulin and TOR signaling pathways, and antioxidant ability in Drosophila. Int J Biol Macromolecules. (2023) 241:124639. doi: 10.1016/j.ijbiomac.2023.124639.
17. Zhan X, Xu X, Zhang P, Wang X, Hu Z, Zhao W, et al. Crude polysaccharide from Danggui Buxue decoction enhanced the anti-tumor effect of gemcitabine by remodeling tumor-associated macrophages. Int J Biol Macromolecules. (2023) 242:125063. doi: 10.1016/j.ijbiomac.2023.125063.
18. Liu X, Wu X, Wang S, Zhao Z, Jian C, Li M, et al. Microbiome and metabolome integrally reveal the anti-depression effects of Cistanche deserticola polysaccharides from the perspective of gut homeostasis. Int J Biol Macromolecules. (2023) 245:125542. doi: 10.1016/j.ijbiomac.2023.125542.
19. Feng Y, Wu C, Chen H, Zheng T, Ye H, Wang J, et al. Rhubarb polysaccharide and berberine co-assembled nanoparticles ameliorate ulcerative colitis by regulating the intestinal flora. Front In Pharmacol. (2023) 14:1184183. doi: 10.3389/fphar.2023.1184183.
20. Zeng G, Ju Y, Shen H, Zhou N, Huang L. Immunopontentiating activities of the purified polysaccharide from evening primrose in H22 tumor-bearing mice. Int J Biol Macromolecules. (2013) 52:280–5. doi: 10.1016/j.ijbiomac.2012.10.005.
21. Demaria O, Cornen S, Daëron M, Morel Y, Medzhitov R, Vivier E. Harnessing innate immunity in cancer therapy. Nature. (2019) 574:45–56. doi: 10.1038/s41586-019-1593-5.
22. Pirillo C, Al Khalidi S, Sims A, Devlin R, Zhao H, Pinto R, et al. Cotransfer of antigen and contextual information harmonizes peripheral and lymph node conventional dendritic cell activation. Sci Immunol. (2023) 8:eadg8249. doi: 10.1126/sciimmunol.adg8249.
23. Wculek SK, Cueto FJ, Mujal AM, Melero I, Krummel MF, Sancho D. Dendritic cells in cancer immunology and immunotherapy [J]. Nat Rev Immunol. (2020) 20:7–24. doi: 10.1038/s41577-019-0210-z.
24. Shui Y, Hu X, Hirano H, Tsukamoto H, Guo W-Z, Hasumi K, et al. Combined phospholipids adjuvant augments anti-tumor immune responses through activated tumor-associated dendritic cells. Neoplasia (New York N.Y.). (2023) 39:100893. doi: 10.1016/j.neo.2023.100893.
25. Xiao Z, Wang R, Wang X, Yang H, Dong J, He X, et al. Impaired function of dendritic cells within the tumor microenvironment. Front Immunol. (2023) 14:1213629. doi: 10.3389/fimmu.2023.1213629.
26. Mestrallet G, Sone K, Bhardwaj N. Strategies to overcome DC dysregulation in the tumor microenvironment. Front Immunol. (2022) 13:980709. doi: 10.3389/fimmu.2022.980709.
27. Zhu S, Yang N, Wu J, Wang X, Wang W, Liu Y-J, et al. Tumor microenvironment-related dendritic cell deficiency: a target to enhance tumor immunotherapy. Pharmacol Res. (2020) 159:104980. doi: 10.1016/j.phrs.2020.104980.
28. Jia H, Zhao B, Zhang F, Santhanam RK, Wang X, Lu J. Extraction, structural characterization, and anti-hepatocellular carcinoma activity of polysaccharides from panax ginseng meyer. Front In Oncol. (2021) 11:785455. doi: 10.3389/fonc.2021.785455.
29. Chao JCJ, Chiang S-W, Wang C-C, Tsai Y-H, Wu M-S. Hot water-extracted Lycium barbarum and Rehmannia glutinosa inhibit proliferation and induce apoptosis of hepatocellular carcinoma cells. World J Gastroenterol. (2006) 12:4478–84. doi: 10.3748/wjg.v12.i28.4478.
30. Tian L, Li C-M, Li Y-F, Huang T-M, Chao N-X, Luo G-R, et al. Laminarin from seaweed (Laminaria japonica) inhibits hepatocellular carcinoma through upregulating senescence marker protein-30. Cancer Biotherapy Radiopharmaceuticals. (2020) 35:277–83. doi: 10.1089/cbr.2019.3179.
31. Wang Z, Meng J, Xia Y, Meng Y, Du L, Zhang Z, et al. Maturation of murine bone marrow dendritic cells induced by acidic Ginseng polysaccharides. Int J Biol Macromolecules. (2013) 53:93–100. doi: 10.1016/j.ijbiomac.2012.11.009.
32. Zhang Z, Meng Y, Guo Y, He X, Liu Q, Wang X, et al. Rehmannia glutinosa polysaccharide induces maturation of murine bone marrow derived Dendritic cells (BMDCs). Int J Biol Macromolecules. (2013) 54:136–43. doi: 10.1016/j.ijbiomac.2012.12.005.
33. Meng J, Cao Y, Meng Y, Luo H, Gao X, Shan F. Maturation of mouse bone marrow dendritic cells (BMDCs) induced by Laminaria japonica polysaccharides (LJP). Int J Biol Macromolecules. (2014) 69:388–92. doi: 10.1016/j.ijbiomac.2014.05.018.
34. Liu S, Yang Y, Qu Y, Guo X, Yang X, Cui X, et al. Structural characterization of a novel polysaccharide from Panax notoginseng residue and its immunomodulatory activity on bone marrow dendritic cells. Int J Biol Macromolecules. (2020) 161:797–809. doi: 10.1016/j.ijbiomac.2020.06.117.
35. Liu C, Choi MW, Xue X, Cheung PCK. Immunomodulatory effect of structurally characterized mushroom sclerotial polysaccharides isolated from polyporus rhinocerus on bone marrow dendritic cells. J Agric Food Chem. (2019) 67:12137–43. doi: 10.1021/acs.jafc.9b03294.
36. Zhang X, Qi C, Guo Y, Zhou W, Zhang Y. Toll-like receptor 4-related immunostimulatory polysaccharides: Primary structure, activity relationships, and possible interaction models. Carbohydr Polymers. (2016) 149:186–206. doi: 10.1016/j.carbpol.2016.04.097.
37. Du J, Chen X, Wang C, Sun H. Pathway analysis of global gene expression change in dendritic cells induced by the polysaccharide from the roots of Actinidia eriantha. J Ethnopharmacology. (2018) 214:141–52. doi: 10.1016/j.jep.2017.12.009.
38. Hongo D, Tang X, Baker J, Engleman EG, Strober S. Requirement for interactions of natural killer T cells and myeloid-derived suppressor cells for transplantation tolerance. Am J Transplantation: Off J Am Soc Transplant Am Soc Transplant Surgeons. (2014) 14:2467–77. doi: 10.1111/ajt.12914.
39. Li S, Du H, Dn G, Li X, Zao X, Ye Y. Integrated analysis of single-cell and bulk RNA-sequencing reveals tumor heterogeneity and a signature based on NK cell marker genes for predicting prognosis in hepatocellular carcinoma. Front Pharmacol. (2023) 14:1200114. doi: 10.3389/fphar.2023.1200114.
40. Asl NS, Behfar M, Amiri RS, Mohseni R, Azimi M, Firouzi J, et al. Intra-lesion injection of activated Natural Killer (NK) cells in recurrent Malignant brain tumors. Int Immunopharmacol. (2023) 120:110345. doi: 10.1016/j.intimp.2023.110345.
41. Curio S, Belz GT. The unique role of innate lymphoid cells in cancer and the hepatic microenvironment. Cell Mol Immunol. (2022) 19:1012–29. doi: 10.1038/s41423-022-00901-1.
42. Liu J, Zhang L, Ren Y, Gao Y, Kang L, Qiao Q. Anticancer and immunoregulatory activity of Gynostemma pentaphyllum polysaccharides in H22 tumor-bearing mice. Int J Biol Macromolecules. (2014) 69:1–4. doi: 10.1016/j.ijbiomac.2014.05.014.
43. Yang X, Ji H, Feng Y, Yu J, Liu A. Structural characterization and antitumor activity of polysaccharides from kaempferia galanga L. Oxid Med Cell Longevity. (2018) 2018:9579262. doi: 10.1155/2018/9579262.
44. Xie X, Ma L, Zhou Y, Shen W, Xu D, Dou J, et al. Polysaccharide enhanced NK cell cytotoxicity against pancreatic cancer via TLR4/MAPKs/NF-κB pathway in vitro/vivo. Carbohydr Polymers. (2019) 225:115223. doi: 10.1016/j.carbpol.2019.115223.
45. Xu L, Zhang W, Zeng L, Jin J-O. Rehmannia glutinosa polysaccharide induced an anti-cancer effect by activating natural killer cells. Int J Biol Macromolecules. (2017) 105:680–5. doi: 10.1016/j.ijbiomac.2017.07.090.
46. Lazarov T, Juarez-Carreño S, Cox N, Geissmann F. Physiology and diseases of tissue-resident macrophages. Nature. (2023) 618:698–707. doi: 10.1038/s41586-023-06002-x.
47. Shabir K, Gharanei S, Orton S, Patel V, Chauhan P, Karteris E, et al. Asprosin exerts pro-inflammatory effects in THP-1 macrophages mediated via the toll-like receptor 4 (TLR4) pathway. Int J Mol Sci. (2022) 24:227. doi: 10.3390/ijms24010227.
48. Arvanitakis K, Koletsa T, Mitroulis I, Germanidis G. Tumor-associated macrophages in hepatocellular carcinoma pathogenesis, prognosis and therapy. Cancers (Basel). (2022) 14:226. doi: 10.3390/cancers14010226.
49. Sha W, Zhao B, Wei H, Yang Y, Yin H, Gao J, et al. Astragalus polysaccharide ameliorates vascular endothelial dysfunction by stimulating macrophage M2 polarization via potentiating Nrf2/HO-1 signaling pathway. Phytomedicine: Int J Phytotherapy Phytopharmacology. (2023) 112:154667. doi: 10.1016/j.phymed.2023.154667.
50. Lu Z, Cao H, Liu D, Zheng Y, Tian C, Liu S, et al. Optimal combination of anti-inflammatory components from Chinese medicinal formula Liang-Ge-San. J Ethnopharmacology. (2021) 269:113747. doi: 10.1016/j.jep.2020.113747.
51. Yoon YD, Han SB, Kang JS, Lee CW, Park SK, Lee HS, et al. Toll-like receptor 4-dependent activation of macrophages by polysaccharide isolated from the radix of Platycodon grandiflorum. Int Immunopharmacol. (2003) 3:1873–82. doi: 10.1016/j.intimp.2003.09.005.
52. Han SB, Park SH, Lee KH, Lee CW, Lee SH, Kim HC, et al. Polysaccharide isolated from the radix of Platycodon grandiflorum selectively activates B cells and macrophages but not T cells. Int Immunopharmacol. (2001) 1:1969–78. doi: 10.1016/S1567-5769(01)00124-2.
53. Sharygin D, Koniaris LG, Wells C, Zimmers TA, Hamidi T. Role of CD14 in human disease. Immunology. (2023) 169:260–70. doi: 10.1111/imm.13634.
54. Schmid MC, Khan SQ, Kaneda MM, Pathria P, Shepard R, Louis TL, et al. Integrin CD11b activation drives anti-tumor innate immunity. Nat Commun. (2018) 9:5379. doi: 10.1038/s41467-018-07387-4.
55. Shao B-M, Xu W, Dai H, Tu P, Li Z, Gao X-M. A study on the immune receptors for polysaccharides from the roots of Astragalus membranaceus, a Chinese medicinal herb. Biochem Biophys Res Commun. (2004) 320:1103–11. doi: 10.1016/j.bbrc.2004.06.065.
56. Li C, Pan X-Y, Ma M, Zhao J, Zhao F, Lv Y-P. Astragalus polysacharin inhibits hepatocellular carcinoma-like phenotypes in a murine HCC model through repression of M2 polarization of tumor-associated macrophages. Pharm Biol. (2021) 59:1533–9. doi: 10.1080/13880209.2021.1991384.
57. Shi Y. PLAN B for immunotherapy: Promoting and leveraging anti-tumor B cell immunity. J Controlled Release: Off J Controlled Release Soc. (2021) 339:156–63. doi: 10.1016/j.jconrel.2021.09.028.
58. Cieniewicz B, Bhatta A, Torabi D, Baichoo P, Saxton M, Arballo A, et al. Chimeric TIM-4 receptor-modified T cells targeting phosphatidylserine mediates both cytotoxic anti-tumor responses and phagocytic uptake of tumor-associated antigen for T cell cross-presentation. Mol Therapy: J Am Soc Gene Ther. (2023) 31:2132–53. doi: 10.1016/j.ymthe.2023.05.009.
59. Bargiela D, Cunha PP, Veliça P, Foskolou IP, Barbieri L, Rundqvist H, et al. Vitamin B6 metabolism determines T cell anti-tumor responses. Front In Immunol. (2022) 13:837669. doi: 10.3389/fimmu.2022.837669.
60. Katakai T. Yin and yang roles of B lymphocytes in solid tumors: Balance between antitumor immunity and immune tolerance/immunosuppression in tumor-draining lymph nodes. Front In Oncol. (2023) 13:1088129. doi: 10.3389/fonc.2023.1088129.
61. Liu T, Li Q, Xu X, Li G, Tian C, Zhang T. Molecular mechanisms of anti-cancer bioactivities of seaweed polysaccharides. Chin Herbal Medicines. (2022) 14:528–34. doi: 10.1016/j.chmed.2022.02.003.
62. Galaine J, Kellermann G, Guillaume Y, Boidot R, Picard E, Loyon R, et al. Heparan sulfate proteoglycans promote telomerase internalization and MHC class II presentation on dendritic cells. J Immunol (Baltimore Md.: 1950). (2016) 197:1597–608. doi: 10.4049/jimmunol.1502633.
63. Cobb BA, Wang Q, Tzianabos AO, Kasper DL. Polysaccharide processing and presentation by the MHCII pathway. Cell. (2004) 117:677–87. doi: 10.1016/j.cell.2004.05.001.
64. Sullivan BA, Kronenberg M. TCR-mediated recognition of glycolipid CD1 complexes. Curr Topics In Microbiol Immunol. (2007) 314:165–93. doi: 10.1007/978-3-540-69511-0_7
65. Cobb BA, Kasper DL. Coming of age: carbohydrates and immunity. Eur J Immunol. (2005) 35:352–6. doi: 10.1002/eji.200425889.
66. Abram CL, Lowell CA. The expanding role for ITAM-based signaling pathways in immune cells. Science’s STKE: Signal Transduction Knowledge Environ. (2007) 2007:re2. doi: 10.1126/stke.3772007re2.
67. Chen Z, Kwong Huat Tan B, Chan SH. Activation of T lymphocytes by polysaccharide-protein complex from Lycium barbarum L. Int Immunopharmacol. (2008) 8:1663–71. doi: 10.1016/j.intimp.2008.07.019.
68. Tingjun H, Rongliang Z. Promotion of Sophora subprosrate polysaccharide on nitric oxide and interleukin-2 production in murine T lymphocytes: implicated Ca2+ and protein kinase C. Int Immunopharmacol. (2004) 4:109–18. doi: 10.1016/j.intimp.2003.11.001.
69. Schamel WW, Reth M. Monomeric and oligomeric complexes of the B cell antigen receptor. Immunity. (2000) 13:5–14. doi: 10.1016/S1074-7613(00)00003-0.
70. Han SB, Yoon YD, Ahn HJ, Lee HS, Lee CW, Yoon WK, et al. Toll-like receptor-mediated activation of B cells and macrophages by polysaccharide isolated from cell culture of Acanthopanax senticosus. Int Immunopharmacol. (2003) 3:1301–12. doi: 10.1016/S1567-5769(03)00118-8.
71. Long H, Wu Z. Immunoregulatory effects of Huaier (Trametes robiniophila Murr) and relevant clinical applications. Front In Immunol. (2023) 14:1147098. doi: 10.3389/fimmu.2023.1147098.
72. Song X, Li Y, Zhang H, Yang Q. The anticancer effect of Huaier (Review). Oncol Rep. (2015) 34:12–21. doi: 10.3892/or.2015.3950.
73. Li K, Xiao K, Zhu S, Wang Y, Wang W. Chinese herbal medicine for primary liver cancer therapy: perspectives and challenges. Front In Pharmacol. (2022) 13:889799. doi: 10.3389/fphar.2022.889799.
74. Pan J, Yang C, Jiang Z, Huang J. Trametes robiniophila Murr: a traditional Chinese medicine with potent anti-tumor effects. Cancer Manage Res. (2019) 11:1541–9. doi: 10.2147/CMAR.
75. Shi K, Bi Y, Zeng X, Wang X. Effects of adjuvant huaier granule therapy on survival rate of patients with hepatocellular carcinoma. Front In Pharmacol. (2023) 14:1163304. doi: 10.3389/fphar.2023.1163304.
76. Yang A, Fan H, Zhao Y, Chen X, Zhu Z, Zha X, et al. An immune-stimulating proteoglycan from the medicinal mushroom Huaier up-regulates NF-κB and MAPK signaling via Toll-like receptor 4. J Biol Chem. (2019) 294:2628–41. doi: 10.1074/jbc.RA118.005477.
77. Li C, Wu X, Zhang H, Yang G, Hao M, Sheng S, et al. A Huaier polysaccharide inhibits hepatocellular carcinoma growth and metastasis. Tumour Biology: J Int Soc For Oncodevelopmental Biol Med. (2015) 36:1739–45. doi: 10.1007/s13277-014-2775-2.
78. Qiao Z, Ma J, Liu H. Evaluation of the antioxidant potential of Salvia miltiorrhiza ethanol extract in a rat model of ischemia-reperfusion injury [J]. Molecules (Basel Switzerland). (2011) 16:10002–12. doi: 10.3390/molecules161210002.
79. Li Y, Qiao L, Chen C, Wang Z, Fu X. Comparative study of Danshen and Siwu decoction based on the molecular structures of the components and predicted targets. BMC Complementary Med Therapies. (2021) 21:42. doi: 10.1186/s12906-021-03209-1.
80. Xie F, Zhang B, Dai S, Jin B, Zhang T, Dong F. Efficacy and safety of Salvia miltiorrhiza (Salvia miltiorrhiza Bunge) and ligustrazine injection in the adjuvant treatment of early-stage diabetic kidney disease: A systematic review and meta-analysis. J Ethnopharmacology. (2021) 281:114346. doi: 10.1016/j.jep.2021.114346.
81. Panossian A, Abdelfatah S, Efferth T. Network pharmacology of ginseng (Part III): antitumor potential of a fixed combination of red ginseng and red sage as determined by transcriptomics. Pharm (Basel Switzerland). (2022) 15:1345. doi: 10.3390/ph15111345.
82. Wu C, Chen W, Fang M, Boye A, Tao X, Xu Y, et al. Compound Astragalus and Salvia miltiorrhiza extract inhibits hepatocellular carcinoma progression via miR-145/miR-21 mediated Smad3 phosphorylation. J Ethnopharmacology. (2019) 231:98–112. doi: 10.1016/j.jep.2018.11.007.
83. Han S, Bi S, Guo T, Sun D, Zou Y, Wang L, et al. Nano co-delivery of Plumbagin and Dihydrotanshinone I reverses immunosuppressive TME of liver cancer. J Control Release. (2022) 348:250–63. doi: 10.1016/j.jconrel.2022.05.057.
84. Xiang M, Su H, Hu Y, Hu Y, Yang T, Shu G. Chemical composition of total flavonoids from Salvia chinensia Benth and their pro-apoptotic effect on hepatocellular carcinoma cells: potential roles of suppressing cellular NF-κB signaling. Food Chem Toxicology: an Int J Published For Br Ind Biol Res Assoc. (2013) 62:420–6. doi: 10.1016/j.fct.2013.09.008.
85. Chen Y, Li H, Li M, Niu S, Wang J, Shao H, et al. Salvia miltiorrhiza polysaccharide activates T Lymphocytes of cancer patients through activation of TLRs mediated -MAPK and -NF-κB signaling pathways. J Ethnopharmacology. (2017) 200:165–73. doi: 10.1016/j.jep.2017.02.029.
86. Wong C-K, Bao Y-X, Wong EL-Y, Leung P-C, Fung KP, Lam CWK. Immunomodulatory activities of Yunzhi and Danshen in post-treatment breast cancer patients. Am J Chin Med. (2005) 33:381–95. doi: 10.1142/S0192415X05002990.
87. Shu G, Zhao W, Yue L, Su H, Xiang M. Antitumor immunostimulatory activity of polysaccharides from Salvia chinensis Benth. J Ethnopharmacology. (2015) 168:237–47. doi: 10.1016/j.jep.2015.03.065.
88. Seweryn E, Ziała A, Gamian A. Health-promoting of polysaccharides extracted from ganoderma lucidum. Nutrients. (2021) 13:2725. doi: 10.3390/nu13082725.
89. Lin D, Zhang Y, Wang S, Zhang H, Gao C, Lu F, et al. Ganoderma lucidum polysaccharide peptides GL-PPSQ2 alleviate intestinal ischemia-reperfusion injury via inhibiting cytotoxic neutrophil extracellular traps. Int J Biol Macromolecules. (2023) 244:125370. doi: 10.1016/j.ijbiomac.2023.125370.
90. Liu X, Yang L, Li G, Jiang Y, Zhang G, Ling J. A novel promising neuroprotective agent: Ganoderma lucidum polysaccharide. Int J Biol Macromolecules. (2023) 229:168–80. doi: 10.1016/j.ijbiomac.2022.12.276.
91. Luo H, Tan D, Peng B, Zhang S, Vong CT, Yang Z, et al. The pharmacological rationales and molecular mechanisms of ganoderma lucidum polysaccharides for the therapeutic applications of multiple diseases. Am J Chin Med. (2022) 50:53–90. doi: 10.1142/S0192415X22500033.
92. Zhang Y, Song S, Li H, Wang X, Song L, Xue J. Polysaccharide from Ganoderma lucidum alleviates cognitive impairment in a mouse model of chronic cerebral hypoperfusion by regulating CD4+CD25+Foxp3+ regulatory T cells. Food Funct. (2022) 13:1941–52. doi: 10.1039/D1FO03698J.
93. Huang Q, Wang L, Zhang L, Hu B, Wang Q, Liang L. Antioxidant Properties of Triterpenoids Isolated from Bagasse-Cultivated Lingzhi or Reishi Medicinal Mushroom, Ganoderma lucidum (Agaricomycetes), at Different Developmental Stages. Int J Medicinal Mushrooms. (2022) 24:41–51. doi: 10.1615/IntJMedMushrooms.v24.i7.
94. Li A, Shuai X, Jia Z, Li H, Liang X, Su D, et al. Ganoderma lucidum polysaccharide extract inhibits hepatocellular carcinoma growth by downregulating regulatory T cells accumulation and function by inducing microRNA-125b. J Trans Med. (2015) 13:100. doi: 10.1186/s12967-015-0465-5.
95. Wang C, Li M, Li L, Shen X, Liu Y, Wang S. Strongylocentrotus nudos Egg Polysaccharide induces autophagy and apoptosis in leukaemia cells by regulating mitochondrial function. J Cell Mol Med. (2021) 25:272–83. doi: 10.1111/jcmm.15995.
96. Xing H, Zhu X, Liao J, Kong Y, Lu Y, Zhao D, et al. Pharmacokinetic study of Strongylocentrotus nudus egg polysaccharide in rats and beagles using a (3)H-labeling method. Front Pharmacol. (2023) 14:1109084. doi: 10.3389/fphar.2023.1109084.
97. Ke M, Wang H, Zhang M, Tian Y, Wang Y, Li B, et al. The anti-lung cancer activity of SEP is mediated by the activation and cytotoxicity of NK cells via TLR2/4 in vivo [J]. Biochem Pharmacol. (2014) 89:119–30. doi: 10.1016/j.bcp.2014.02.024.
98. Wang M, Wang H, Tang Y, Kang D, Gao Y, Ke M, et al. Effective inhibition of a Strongylocentrotus nudus eggs polysaccharide against hepatocellular carcinoma is mediated via immunoregulation in vivo. Immunol Lett. (2011) 141:74–82. doi: 10.1016/j.imlet.2011.08.001.
99. Liu C, Xi T, Lin Q, Xing Y, Ye L, Luo X, et al. Immunomodulatory activity of polysaccharides isolated from Strongylocentrotus nudus eggs. Int Immunopharmacol. (2008) 8:1835–41. doi: 10.1016/j.intimp.2008.09.005.
100. Zhang M, Liu Y, Li J, Ke M, Yu J, Dou J, et al. A polysaccharide component from Strongylocentrotus nudus eggs inhibited hepatocellular carcinoma in mice by activating T lymphocytes. Oncol Lett. (2017) 13:1847–55. doi: 10.3892/ol.2017.5624.
101. Li A, Ji B, Yang Y, Ye B, Zhu Q, Hu X, et al. Single-cell RNA sequencing highlights the role of PVR/PVRL2 in the immunosuppressive tumour microenvironment in hepatocellular carcinoma. Front Immunol. (2023) 14:1164448. doi: 10.3389/fimmu.2023.1164448.
102. Liang X, Gao H, Xiao J, Han S, He J, Yuan R, et al. Abrine, an IDO1 inhibitor, suppresses the immune escape and enhances the immunotherapy of anti-PD-1 antibody in hepatocellular carcinoma. Front In Immunol. (2023) 14:1185985. doi: 10.3389/fimmu.2023.1185985.
103. Ménétrier-Caux C, Gobert M, Caux C. Differences in tumor regulatory T-cell localization and activation status impact patient outcome. Cancer Res. (2009) 69:7895–8. doi: 10.1158/0008-5472.CAN-09-1642.
104. Zhou L, Wang J, Lyu S-C, Pan L-C, Shi X-J, Du G-S, et al. PD-L1+NEUT, foxp3+Treg, and NLR as new prognostic marker with low survival benefits value in hepatocellular carcinoma. Technol In Cancer Res Treat. (2021) 20:15330338211045820. doi: 10.1177/15330338211045820.
105. Han SJ, Jain P, Gilad Y, Xia Y, Sung N, Park MJ, et al. Steroid receptor coactivator 3 is a key modulator of regulatory T cell-mediated tumor evasion. Proc Natl Acad Sci United States America. (2023) 120:e2221707120. doi: 10.1073/pnas.2221707120.
106. Geng C-A, Chen F-Y, Zheng J-B, Liao P, Li T-Z, Zhang X-M, et al. Rubiginosin B selectively inhibits Treg cell differentiation and enhances anti-tumor immune responses by targeting calcineurin-NFAT signaling pathway. Phytomedicine. (2023) 116:154898. doi: 10.1016/j.phymed.2023.154898.
107. Yi C, Chen L, Lin Z, Liu L, Shao W, Zhang R, et al. Lenvatinib targets FGF receptor 4 to enhance antitumor immune response of anti-programmed cell death-1 in HCC. Hepatol (Baltimore Md.). (2021) 74:2544–60. doi: 10.1002/hep.31921.
108. He L, Kang Q, Zhang Y, Chen M, Wang Z, Wu Y, et al. Glycyrrhizae Radix et Rhizoma: The popular occurrence of herbal medicine applied in classical prescriptions. Phytother Res. (2023) 37:3135–60. doi: 10.1002/ptr.7869.
109. Basar N, Oridupa OA, Ritchie KJ, Nahar L, Osman NMM, Stafford A, et al. Comparative cytotoxicity of glycyrrhiza glabra roots from different geographical origins against immortal human keratinocyte (HaCaT), lung adenocarcinoma (A549) and liver carcinoma (HepG2) cells. Phytotherapy Research: PTR. (2015) 29:944–8. doi: 10.1002/ptr.5329.
110. He X, Li X, Liu B, Xu L, Zhao H, Lu A. Down-regulation of Treg cells and up-regulation of TH1/TH2 cytokine ratio were induced by polysaccharide from Radix Glycyrrhizae in H22 hepatocarcinoma bearing mice. Molecules (Basel Switzerland). (2011) 16:8343–52. doi: 10.3390/molecules16108343.
111. Huang Y, Zhang Y, Zhang M, Zhao K, Feng L, Guan J, et al. Combined immunotherapy for hepatocellular carcinoma: how to maximize immune checkpoint blockade synergic anti-tumor effect. Crit Rev In Oncology/hematology. (2023) 189:104070. doi: 10.1016/j.critrevonc.2023.104070.
112. Sové RJ, Verma BK, Wang H, Ho WJ, Yarchoan M, Popel AS. Virtual clinical trials of anti-PD-1 and anti-CTLA-4 immunotherapy in advanced hepatocellular carcinoma using a quantitative systems pharmacology model. J For Immunotherapy Cancer. (2022) 10:e005414. doi: 10.1136/jitc-2022-005414.
113. Bing YZ YH, Li JM. Clinical observation of Lentinan polysaccharide in treating hepatocellular carcinoma. Chin J Clin Oncol. (1999) 26:393–4.
114. Zhao XQ TY, Wang P, Zhang ZM. Treatment of advanced hepatocellular carcinoma with Lentinan polysaccharide combined with TACE. Shandong Med J. (2005) 45:37–8.
115. Nan J XC. Effects of Lentinan polysaccharide combined with chemotherapy on liver function, T cell subsets, IL-12, sIL-2R in patients with HCC. Chin J Integrated Traditional Western Med Liver Dis. (2018) 28:153–5.
116. Qiao B LY, Ze YG, Zhaxi BBa, Zhao YL, Gong P. Meta-analysis of lentinan adjuvant transcatheter arterial chemoembolization on treatment patients with primary liver cancer. Tibetan Med. (2017) 38:3–7.
117. Xu S. Efficacy evaluation of peritoneal infusion of Lentinan polysaccharide in improving ascites in patients with advanced hepatocellular carcinoma. Anti-Infection Pharm. (2016) 13:442–3.
118. Qing-lan Y. Lentinan peripheral blood CD4 + CD25 + regulatory T cells before and after TACE of primary liver cancer [J]. China Pract Med. (2012) 23:21–2.
119. Fang L, Zhang Y, Wang Q, Zang Y, Li Z, Duan Z, et al. A polysaccharide from Huaier ameliorates cisplatin nephrotoxicity by decreasing oxidative stress and apoptosis via PI3K/AKT signaling. Int J Biol Macromol. (2019) 139:932–43. doi: 10.1016/j.ijbiomac.2019.07.219.
120. Luo L, Xue J, Shao Z, Zhou Z, Tang W, Liu J, et al. Recent developments in Salvia miltiorrhiza polysaccharides: Isolation, purification, structural characteristics and biological activities. Front Pharmacol. (2023) 14:1139201. doi: 10.3389/fphar.2023.1139201.
121. Ai-Lati A, Liu S, Ji Z, Zhang H, Mao J. Structure and bioactivities of a polysaccharide isolated from Ganoderma lucidum in submerged fermentation. Bioengineered. (2017) 8:565–71. doi: 10.1080/21655979.2017.1283459.
122. Zhou M, Zhi J, Zhi J, Xiong Z, Wu F, Lu Y, et al. Polysaccharide from Strongylocentrotus nudus eggs regulates intestinal epithelial autophagy through CD36/PI3K-Akt pathway to ameliorate inflammatory bowel disease. Int J Biol Macromol. (2023) 244:125373. doi: 10.1016/j.ijbiomac.2023.125373.
123. Li X, Zhang H, Xu H. Analysis of chemical components of shiitake polysaccharides and its anti-fatigue effect under vibration. Int J Biol Macromol. (2009) 45:377–80. doi: 10.1016/j.ijbiomac.2009.07.005.
124. Zhang CH, Yu Y, Liang YZ, Chen XQ. Purification, partial characterization and antioxidant activity of polysaccharides from Glycyrrhiza uralensis [J]. Int J Biol Macromol. (2015) 79:681–6. doi: 10.1016/j.ijbiomac.2015.05.060.
125. Wang N, Wang X, He M, Zheng W, Qi D, Zhang Y, et al. Ginseng polysaccharides: A potential neuroprotective agent. J Ginseng Res. (2021) 45:211–7. doi: 10.1016/j.jgr.2020.09.002.
126. Bian Z, Zhang R, Zhang X, Zhang J, Xu L, Zhu L, et al. Extraction, structure and bioactivities of polysaccharides from Rehmannia glutinosa: A review. J Ethnopharmacol. (2023) 305:116132. doi: 10.1016/j.jep.2022.116132.
127. Chen J, Yang J, Du H, Aslam M, Wang W, Chen W, et al. Laminarin, a major polysaccharide in stramenopiles. Mar Drugs. (2021) 19:576. doi: 10.3390/md19100576.
128. Ma JQ, Dong AB, Xia HY, Wen SY. Preparation methods, structural characteristics, and biological activity of polysaccharides from Platycodon grandifloras. Int J Biol Macromol. (2023) 258:129106. doi: 10.1016/j.ijbiomac.2023.129106
129. Jiang Y, Qi X, Gao K, Liu W, Li N, Cheng N, et al. Relationship between molecular weight, monosaccharide composition and immunobiologic activity of Astragalus polysaccharides. Glycoconj J. (2016) 33:755–61. doi: 10.1007/s10719-016-9669-z.
130. Lv B, Wang Y, Ma D, Cheng W, Liu J, Yong T, et al. Immunotherapy: reshape the tumor immune microenvironment. Front Immunol. (2022) 13:844142. doi: 10.3389/fimmu.2022.844142.
131. Xue H, Li P, Bian J, Gao Y, Sang Y, Tan J. Extraction, purification, structure, modification, and biological activity of traditional Chinese medicine polysaccharides: A review. Front Nutr. (2022) 9:1005181. doi: 10.3389/fnut.2022.1005181.
132. Fossum E, Tesfaye DY, Bobic S, Gudjonsson A, Braathen R, Lahoud MH, et al. Targeting antigens to different receptors on conventional type 1 dendritic cells impacts the immune response. J Immunol. (2020) 205:661–73. doi: 10.4049/jimmunol.1901119.
133. Hu C, Xu B, Wang X, Wan WH, Lu J, Kong D, et al. Gut microbiota-derived short-chain fatty acids regulate group 3 innate lymphoid cells in HCC. Hepatology. (2023) 77:48–64. doi: 10.1002/hep.32449.
134. Behary J, Amorim N, Jiang XT, Raposo A, Gong L, McGovern E, et al. Gut microbiota impact on the peripheral immune response in non-alcoholic fatty liver disease related hepatocellular carcinoma. Nat Commun. (2021) 12:187. doi: 10.1038/s41467-020-20422-7.
135. Tang C, Ding R, Sun J, Liu J, Kan J, Jin C. The impacts of natural polysaccharides on intestinal microbiota and immune responses - a review. Food Funct. (2019) 10:2290–312. doi: 10.1039/C8FO01946K.
136. Yuan X, Xue J, Tan Y, Yang Q, Qin Z, Bao X, et al. Albuca bracteate polysaccharides synergistically enhance the anti-tumor efficacy of 5-fluorouracil against colorectal cancer by modulating β-catenin signaling and intestinal flora. Front Pharmacol. (2021) 12:736627. doi: 10.3389/fphar.2021.736627.
137. Shibata N, Kunisawa J, Kiyono H. Dietary and microbial metabolites in the regulation of host immunity. Front Microbiol. (2017) 8:2171. doi: 10.3389/fmicb.2017.02171.
138. Postler TS, Ghosh S. Understanding the holobiont: how microbial metabolites affect human health and shape the immune system. Cell Metab. (2017) 26:110–30. doi: 10.1016/j.cmet.2017.05.008.
139. Wu W, Sun M, Chen F, Cao AT, Liu H, Zhao Y, et al. Microbiota metabolite short-chain fatty acid acetate promotes intestinal IgA response to microbiota which is mediated by GPR43. Mucosal Immunol. (2017) 10:946–56. doi: 10.1038/mi.2016.114.
Keywords: hepatocellular carcinoma, traditional Chinese medicine, polysaccharides, antitumor immunostimulatory, Toll like receptor, mannose receptor
Citation: Liu Y, Wu J and Hao H (2024) Antitumor immunostimulatory activity of the traditional Chinese medicine polysaccharide on hepatocellular carcinoma. Front. Immunol. 15:1369110. doi: 10.3389/fimmu.2024.1369110
Received: 11 January 2024; Accepted: 09 February 2024;
Published: 22 February 2024.
Edited by:
Tiezheng Hou, University College London, United KingdomReviewed by:
Parichart Hongsing, Mae Fah Luang University, ThailandQuan Liu, Southern University of Science and Technology, China
Chenghai Liu, Shanghai University of Traditional Chinese Medicine, China
Xiao Wei Han, Liaoning University of Traditional Chinese Medicine, China
Junfeng Zhang, Nanjing University of Chinese Medicine, China
Copyright © 2024 Liu, Wu and Hao. This is an open-access article distributed under the terms of the Creative Commons Attribution License (CC BY). The use, distribution or reproduction in other forums is permitted, provided the original author(s) and the copyright owner(s) are credited and that the original publication in this journal is cited, in accordance with accepted academic practice. No use, distribution or reproduction is permitted which does not comply with these terms.
*Correspondence: Huiqin Hao, hhq@sxtcm.edu.cn