- 1Human Immunology Laboratory, Acibadem University School of Medicine, Istanbul, Türkiye
- 2Jackson Laboratory for Genomic Medicine, Farmington, CT, United States
Mucosal-associated invariant T (MAIT) cells play diverse roles in cancer, infectious diseases, and immunotherapy. This review explores their intricate involvement in cancer, from early detection to their dual functions in promoting inflammation and mediating anti-tumor responses. Within the solid tumor microenvironment (TME), MAIT cells can acquire an ‘exhausted’ state and secrete tumor-promoting cytokines. On the other hand, MAIT cells are highly cytotoxic, and there is evidence that they may have an anti-tumor immune response. The frequency of MAIT cells and their subsets has also been shown to have prognostic value in several cancer types. Recent innovative approaches, such as programming MAIT cells with chimeric antigen receptors (CARs), provide a novel and exciting approach to utilizing these cells in cell-based cancer immunotherapy. Because MAIT cells have a restricted T cell receptor (TCR) and recognize a common antigen, this also mitigates potential graft-versus-host disease (GVHD) and opens the possibility of using allogeneic MAIT cells as off-the-shelf cell therapies in cancer. Additionally, we outline the interactions of MAIT cells with the microbiome and their critical role in infectious diseases and how this may impact the tumor responses of these cells. Understanding these complex roles can lead to novel therapeutic strategies harnessing the targeting capabilities of MAIT cells.
1 Introduction to MAIT cells
Mucosal-associated invariant T (MAIT) cells represent a conserved arm of the immune system, bridging the innate and adaptive responses that play a crucial role in the body’s defense against infectious diseases, particularly at mucosal sites (1–4). They are distinguished by their specialized semi-invariant T cell receptors (TCRs), primarily composed of Vα7.2-Jα33 in humans, paired with a limited set of β-chains, which recognize riboflavin (vitamin B2) metabolites, produced by bacteria and fungi, presented by the MR1 molecule on the surface of infected cells (3–7). This unique antigen recognition mechanism allows MAIT cells to respond to a wide range of microbial infections, as these metabolite antigens are conserved across many bacterial and fungal species, not found in human cells (1, 6, 8–12). MAIT cells are also involved in tissue repair and regeneration, privileged interaction between defined members of the microbiota, which sequentially controls both tissue-imprinting and subsequent responses to injury (13, 14).
Upon encountering these microbial metabolites, MAIT cells are quickly activated and initiate a potent immune response through two distinct pathways: TCR-dependent (MR1-dependent) and TCR-independent (MR1-independent) mechanisms (Figure 1A). In the TCR-dependent pathway, they swiftly secrete a variety of pro-inflammatory cytokines, such as IFN-γ, TNF, IL-17, and express cytotoxic molecules like granzyme B and perforin. This enables them to recruit other cells to the site of inflammation such as neutrophils and modulate others such as dendritic cells and macrophages, thus influencing the broader immune response (1, 6, 8, 15–17). Additionally, their activation is augmented by cytokines such as IL-18, IL-23, and IL-1β, as well as TLR agonists and bacterial products (16, 18–21). This multi-signal approach ensures robust MAIT cell activation and proliferation, integral to their role in the immune response.
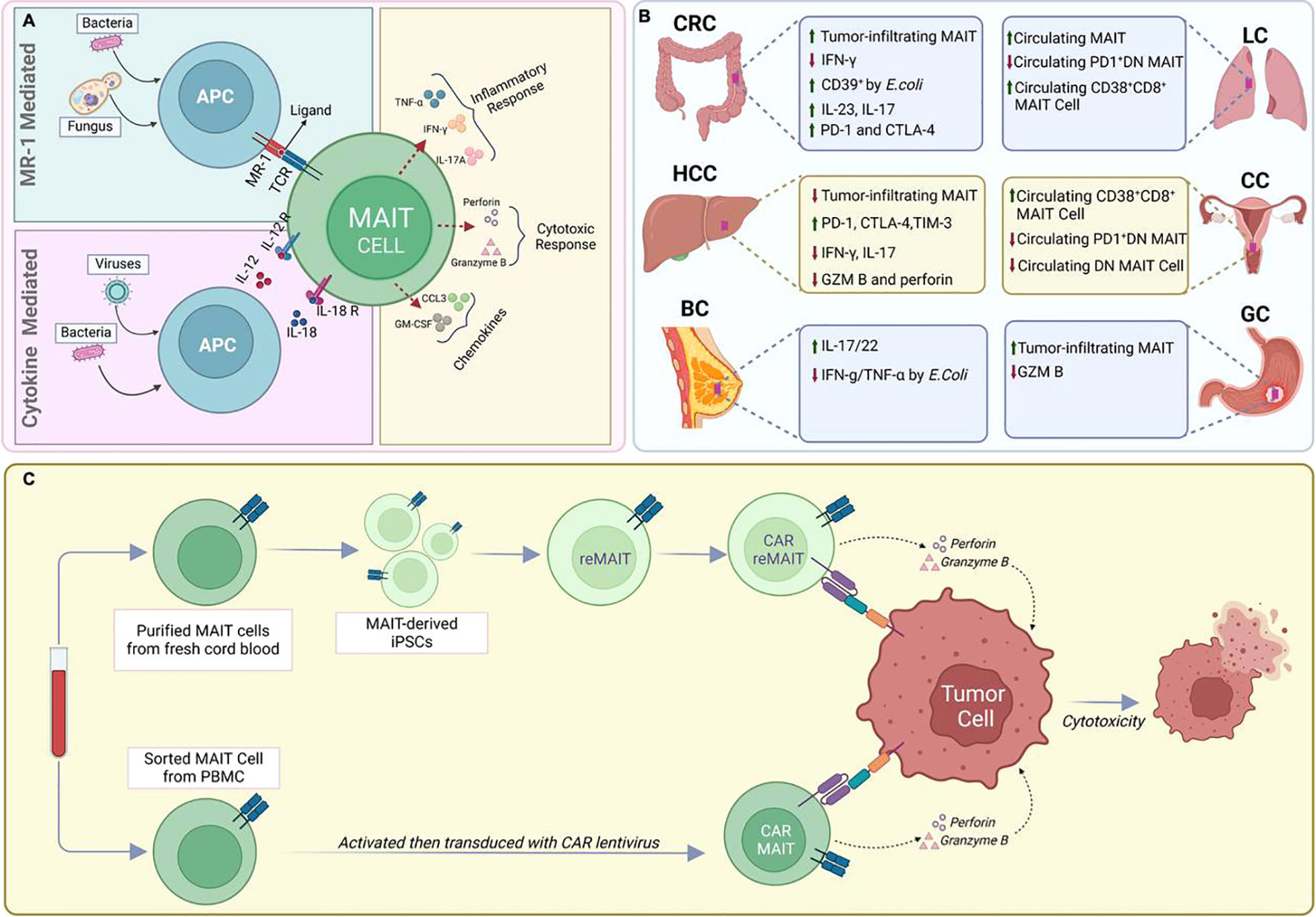
Figure 1 MAIT cells in infectious diseases and Cancer. (A). Recognition of viruses or bacteria by MAIT cells (B) Functional and phenotypic features of tumor-infiltrating MAIT cells. (C) CAR-T cell engineering of natural or IPS-derived human MAIT cells against cancer.
MAIT cells can also be activated in an TCR-independent (MR1-independent) manner by cytokines released in response to viral infections or by other immune cells. IL-12 and IL-18 are key players in this cytokine-driven pathway, and additional cytokines like IL-7, IL-15, and type-I IFNs also play a role (16, 22–24). The activation mediated by cytokines could offer an additional level of protection against viral infections. This is particularly relevant because these infections do not generate the riboflavin byproducts required for activation through the MR1 pathway (16, 24).
2 MAIT cells during infectious diseases
MAIT cells play a crucial role in the immune response to infections (Figure 1A). They have been shown to directly clear multidrug-resistant bacteria and overcome mechanisms of antimicrobial resistance (25–29). Studies have also supported the protective role of MAIT cells in the antimicrobial immune response, as suggested by mouse models where the deletion of MR1, and hence MAIT cells, rendered mice more susceptible to bacterial infections (10, 30). Moreover, activated MAIT cells produce diverse cytokines and cytotoxic effector molecules and accumulate at the site of infection, demonstrating their protective role against various bacterial infections (31–33), including tuberculosis (26, 34), Salmonella (8, 35), Legionella (36, 37), Francisella tularensis (36, 38), klebsiella pneumoniae (39) and clostridium difficile (40). Zhao et al. demonstrated that systemic infection of mice with Francisella tularensis live vaccine strain (LVS) led to substantial expansion of MAIT cells in organs and blood. The study revealed a Th1-like MAIT-1 phenotype, featuring specific transcription factors and cytokine profiles, pivotal for controlling bacterial replication (41). Following infection resolution, Zhao et al. observed a transition of expanded MAIT cells into stable memory-like populations, suggesting a potential foundation for a Francisella tularensis vaccine (41).
Zheng et al. provide a comprehensive review on MAIT cells in gastrointestinal bacterial infections, revealing their intricate roles. They emphasize recent findings, showcasing the dynamic nature of MAIT cell responses, which vary based on etiological agents and anatomical locations (42). MAIT cells can also improve bacterial control during chronic infection and reduce bacterial loads through IL-17A-dependent mechanisms (26, 43).
MAIT cells have also been implicated in the immune response during viral infections such as COVID-19 and HIV infections. In the context of HIV infection, MAIT cells are depleted early on, but they retain functional cytokine expression, suggesting a potential role in controlling microbial translocation in the gut during HIV infection (34, 44). Additionally, MAIT cells have been shown to respond to and suppress HIV-1, suggesting an antiviral protective role in vivo (45). In the context of COVID-19, MAIT cells have been found to be highly activated and functionally impaired in COVID-19 patients and that their numbers decline sharply in the circulation (46, 47). Additionally, MAIT cell alterations markedly correlated with disease severity and patient mortality, suggesting a negative role for MAIT cells in severe COVID-19 infection, where their activation and Granzyme B production are at the highest levels (48). MAIT cells play a significant role in hepatitis infections, particularly in chronic liver diseases such as hepatitis B and hepatitis C. Studies have indicated that MAIT cells are involved in chronic hepatitis, with a decrease in their numbers independent of the cause (49). Furthermore, MAIT cells have been found to be severely reduced and exhausted in individuals with chronic hepatitis B virus (HBV) infection, suggesting a potential impact on the immune response in these patients (50)
MAIT cells were also investigated for their role in severe influenza infection. Analysis of patients with avian H7N9 influenza pneumonia revealed higher CD161+Vα7.2+ MAIT cell counts in survivors, suggesting a potential protective function. In vitro studies with influenza A virus (IAV)-infected lung epithelial cells and peripheral blood mononuclear cells demonstrated MAIT cell activation, characterized by upregulation of IFNγ and granzyme B. Notably, IL-18, produced by IAV-exposed CD14+ monocytes, was identified as a key factor in MAIT cell cytokine production. This IL-18-dependent activation suggests a protective role for MAIT cells in influenza and potentially in other inflammatory conditions involving IL-18 production (51).
Several authors have previously meticulously compiled comprehensive tables summarizing the multifaceted roles of MAIT cells in various infections (3, 52, 53).
3 Multifaceted impact of MAIT cells in cancer
MAIT cells have also been reported to serve important but diverse roles in human cancer by their dual roles in promoting inflammation and mediating anti-tumor responses, indicating their multifaceted impact on cancer (Figure 1B) (54–58). Associations have been determined between MAIT cell frequency, circulating inflammatory markers, and clinical parameters to elucidate the role of MAIT cells in inflammation-driven cancer, indicating their potential relevance in cancer progression (59, 60).
In multiple cancers, including glioma and others, Kubica et al. found that MR1 is overexpressed, yet its impact on overall survival varies (61). Specifically, in glioma, high MR1 levels correlate with poorer outcomes (61). However, the link between MR1 expression and survival is complex and not universally clear across cancer types since he correlation between MR1 levels and MAIT cell infiltration differs among cancers (52). Beyond solid tumors, MR1 expression is also observed in multiple myeloma cell lines, which can present the vitamin-B derivative ligand to MAIT cells, which in turn can induce cytotoxic activity towards these myeloma cells (62).
The ability of MAIT cells to exert cytotoxic effects and secrete inflammatory cytokines situates them at a crossroads of tumor biology, where they can potentially influence tumor growth, progression, and response to treatment (55, 58, 63, 64). However, the role of MAIT cells in cancer is not straightforward (52). Petley et al. showed that MAIT cells enhance NK cell mediated anti-tumor immunity by inducing an IFN- γ transcriptome in NK cells followed by activation (58). As such, it is worth noting that MAIT cells can play a supplementary rather than a primary role in NK cell-mediated anti-tumor activity. Additionally, Gentles et al. demonstrated that more CD8+CD161+ T cells, including MAIT cells, in tumors are linked to better outcomes in various cancers, suggesting a potential role for these cells in clinical benefits, supporting the theme of anti-tumor effects (65). Additionally, MAIT cells have been shown to influence the tumor microenvironment and could be relevant in cancer immunotherapy, although their exact role remains uncertain (57, 58, 63).
Conversely, other studies have highlighted the tumor-promoting functions of MAIT cells. For example, they can suppress T and NK cells and blocking the MR1 protein may offer a new strategy for cancer immunotherapy (57). In non-small cell lung cancer patients, MAIT cells exhibited an exhausted tumor-promoting phenotype and were found to predict the response to anti-PD-1 immunotherapy (66). Negative impact of IL-17 producing MAIT cells on cancer progression has also been observed, suggesting a potential detrimental role in cancers such as breast cancer (63). MAIT cells can also impair anti-tumor immunity by expressing PD-1 ligands, which can in turn lead to conventional T cell exhaustion and reduced anti-tumor responses in prostate cancer (67).
The paradoxical behavior of MAIT cells that encompasses both the promotion of tumor growth and the execution of anti-tumor activities is possibly result of their ability to secrete a wide range of cytokines and cytotoxic molecules, influenced by the signals they receive from the tumor microenvironment (TME) (57–59, 68–71). On the one hand, MAIT cells have been observed to produce pro-inflammatory cytokines such as IL-17 and IL-13 within the TME (59, 72, 73). IL-17 is known to be involved in chronic inflammation and has been linked to the promotion of angiogenesis and tumor growth (74, 75). It can enhance the survival and proliferation of cancer cells, and its presence in the TME is often associated with a poor prognosis in several cancers (74–77) Similarly, IL-13 has been implicated in tumor progression and metastasis (73, 78). It contributes to an immunosuppressive TME by inhibiting the activity of cytotoxic cells and promoting the survival and proliferation of tumor cells (73, 78).
MAIT cells are also primarily found in mucosal tissues such as the gut, lungs, and cervix, and are thought to play a crucial role in the immune response in these tissues. As such, they could particularly migrate into tumor areas within these areas, including colorectal and cervical cancers (79). Their prevalence in these tissues positions them as potential early detectors and responders in the development of cancer, serving as the first line of defense against abnormal cellular changes that may lead to cancer (79).
The potential migration of MAIT cells into tumor areas within mucosal tissues has also been supported by the observation that circulating MAIT cells are reduced in mucosa-associated cancer patients, possibly because of migration to these areas (68). MAIT cells were also shown to accumulate in colon adenocarcinomas, indicating their potential to promote local immune responses to tumors, although factors in the tumor microenvironment may act to reduce MAIT cell IFN-γ production (79). Moreover, MAIT cells have been found to display hallmarks of bacterial antigen recognition in colorectal cancer (56). It was also suggested that MAIT cells in colorectal cancer, when activated, can be potential sources of stimulation in the tumor microenvironment (55).
In cervical cancer there also seems to be a link between the number of MAIT cells and myeloid-derived suppressor cells (MDSCs). This connection suggests that MAIT cells, through the release of IL-17 and other cytokines, could be involved in recruiting MDSCs to the tumor site, potentially aiding in tumor growth and progression (54; Lu et al., 2020). Increased CD8+, CD4+, and highly activated CD38+CD8+MAIT cells in cervical cancer patients’ peripheral blood compared to healthy donors, along with reduced PD1+ double negative (DN) MAIT cells were also observed (Lu et al., 2020). Importantly, higher levels of circulating PD1+ DN MAIT cells correlated with improved progression-free survival in cervical cancer patients (Lu et al., 2020).
Several studies that have also focused on MAIT cell frequencies as prognostic factors in these cancers. In colorectal cancer (CRC) it was observed that MAIT cell numbers in the periphery decrease, possibly because these cells migrate preferentially to the cancerous areas (59, 70). This pattern of MAIT cell redistribution has also been observed in patients with gastric cancer (GC) and cervical cancer, indicating a similar trend in these types of cancer as well (64, 80). Whereas, increased circulating MAIT cells appear to be correlated with lung cancer progression, further suggesting their potential role in tumor areas within the lungs (71). To provide a concise overview of changes in MAIT cell function across various solid tumors, a summary is presented in Table 1. These clinical observations highlight the potential prognostic potential of MAIT cells in cancer.
Overall, these findings collectively suggest that MAIT cells can display contrasting roles in controlling anti-tumor immune responses depending on their activation status, may have complex and diverse roles in cancer progression and potential as therapeutic targets in cancer treatment (58). However, their exact impact on tumor progression and therapy remains a complex and evolving area of study.
4 Impact of tumor microenvironment on MAIT cell immune responses
As discussed above, the signals received by MAIT cells, such as cytokines, cell-cell interactions, and metabolic cues, can sway them towards either promoting tumor growth or exerting anti-tumor effects (Figure 1B). Understanding these signals and the conditions under which they operate is crucial for harnessing the therapeutic potential of MAIT cells in cancer (25, 56, 67, 68, 70, 73, 87).
Within the TME, MAIT cells can undergo significant phenotypic changes that alter their functionality. One of the most critical transformations is the development of an ‘exhausted’ phenotype. This state of exhaustion is characterized by a reduced capacity to proliferate, produce cytokines, and mediate cytotoxicity, alongside an increased expression of inhibitory receptors such as PD-1, CTLA-4, and TIM-3. Such phenotypic changes indicate that MAIT cells may contribute to an immunosuppressive tumor microenvironment, potentially aiding in cancer progression (25, 56, 67, 70, 87).
The path to exhaustion typically begins with chronic antigen stimulation and persistent inflammation within the TME (59, 70). As MAIT cells encounter an ongoing barrage of signals - from cancer cell antigens presented by MR1 to inflammatory cytokines and chemokines - they initially respond robustly. However, this sustained activation can lead to a state of overstimulation, may lead to the previously mentioned functional dysregulation (25, 56, 67, 70, 87).
The implications of MAIT cell exhaustion for cancer progression are manifold. Exhausted MAIT cells may contribute to an immunosuppressive TME, allowing cancer cells to evade immune detection and destruction. They may also promote the recruitment and function of regulatory cells, such as regulatory T cells (Tregs) and myeloid-derived suppressor cells (MDSCs), which further inhibit the anti-tumor response. In addition, these exhausted MAIT cells can secrete cytokines that support tumor survival, angiogenesis, and metastasis, thus inadvertently aiding cancer progression.
Furthermore, the exhausted phenotype is often associated with a reduced production of IFN-γ, IL-17, granzyme B, and perforin by MAIT cells (88, 89). This not only diminishes the direct anti-tumor activity of MAIT cells but also impacts the broader anti-tumor immune response, as these molecules play crucial roles in orchestrating and executing effective anti-cancer immunity (82, 83, 87–89).
In hepatocellular carcinoma (HCC), tumor tissue localized MAIT cells also exhibited exhausted phenotype with upregulation of inhibitory immune molecules (PD-1, CTLA-4, and TIM-3) and secreted lower quantities of effector molecules (e.g., IFN-g, granzyme B, and perforin) (87). As suggested by this immunosuppressive phenotype, high MAIT cell infiltration into HCC solid tumors was correlated with adverse prognosis (87). Furthermore, these cells often express pro-tumor cytokines like IL-8, possibly contributing to the recruitment of immunosuppressive MDSCs to the tumor site. The presence of such immunosuppressive MAIT cells in HCC has been associated with a negative impact on patient prognosis (87, 90).
Thus, the presence, functional state, and interactions of MAIT cells with other immune cells within the TME can provide valuable insights into disease progression and patient outcomes. However, the dualistic nature of MAIT cells and their varying roles in different cancer types or even within the same cancer type but at different stages or different patients underscore the need for a deeper understanding of their biology. Understanding these mechanisms is crucial because it could lead to novel therapeutic approaches that leverage MAIT cells’ natural targeting abilities including genetically engineering MAIT cells to enhance cancer treatment.
5 Role of MAIT cells in cancer treatment
The role of MAIT cells in positive contribution to anti-tumor immune responses is also an area of ongoing investigation (66) especially against solid tumors (65, 91). MAIT cells are capable of exerting anti-tumor effects, predominantly through the action of cytotoxic molecules (92). They can also release granzyme B and perforin, which are essential for the direct killing of target cells (23, 93). The anti-tumor activity of MAIT cells is further enhanced by their ability to produce IFN-γ, a cytokine critical for immune surveillance and the activation of other immune cells, including macrophages and dendritic cells. IFN-γ can enhance the antigen-presenting capabilities of these cells, leading to a more effective anti-tumor immune response (23, 32, 94, 95).
As discussed above, MAIT cells express immune checkpoint receptors and possess cytotoxic functions, making them attractive in tumor immunology research. Innate-like T cell subsets, including MAIT cells, have shown potential in targeting the immunosuppressive TME composed of tumor-associated macrophages (TAMs) and MDSCs (32, 63, 70). Interestingly, Biasi et al. (2021) found that detecting circulating MAIT cells could identify patients’ positive responses to anti-PD1 therapy, suggesting their promise as a biomarker for treatment effectiveness (96). Additionally, the frequency of circulating MAIT cells was observed to influence treatment response and survival in melanoma patients undergoing anti-PD1 therapy, emphasizing a potential role for MAIT cells in shaping treatment outcomes (97).
While the current understanding of how MAIT cells are activated within tumors is predominantly focused on their response to microbial metabolites (59) the possibility that they could also be triggered by antigens originating from the tumor itself has not been ruled out (55, 63). If such tumor-derived antigens that activate MAIT cells are identified, they could serve as precise targets for new immunotherapies, potentially leading to more effective cancer treatments.
Besides solid tumors, the involvement of mucosa-associated invariant T (MAIT) cells in hematological malignancies and transplantation immunity has also been a subject of interest in recent research. MAIT cells may also play multifunction role in hematological malignancies and transplantation immunity, highlighting their potential role as an immunomodulatory factor (98).
However, experimental evidence indicates that MAIT cells can retain their anti-tumor functions even when MR1 is knocked out from the tumor cells, suggesting alternative pathways through which MAIT cells can exert their effects. The nuances of MR1 expression and the conditions that influence the anti-tumor activities of MAIT cells are still under investigation. Discrepancies in MR1 expression across various tumor cell lines and the resulting differences in MAIT cell response underscore the complexity of the immune system’s interaction with cancer. Utilization of 3D bioprinted tumor models to study the effect of localization and activation of MAIT cells within the 3D tumor tissue model could help elucidate and these complex interactions (99).
Priya and Brutkiewicz et al. use an artificial antigen-presenting cell (aAPC) comprised of latex beads coated with an MR1 tetramer complex loaded with antigen (Ag) and anti-CD28 antibody, they successfully addressed MAIT cell expansion limitations (100). Furthermore, they demonstrated that aAPC-expanded MAIT cells retained functionality, preserved their original phenotype, and displayed both proinflammatory cytokine secretion and cytotoxicity against GBM cell lines (100). These findings strongly support the potential of aAPC-generated MAIT cells as a promising and efficient option for adoptive immunotherapy against tumors (100).
Recent advancements in immunotherapy have highlighted chimeric antigen receptor (CAR)-based therapies, with CAR-T cells showing promise in targeting hematological malignancies (Figure 1C) (52, 101). However, these therapies have significant limitations, such as severe side effects, and are less effective against solid tumors. Additionally, CAR-T therapies require autologous cells, which are costly and time-consuming to produce (102).
Utilizing MAIT cells engineered with CAR molecules (CAR-MAIT) could be a novel approach that may address some of these challenges. Recently, we developed such CAR-MAIT cells to target lymphomas and breast cancer cells (101). We had found that these engineered cells demonstrate robust cytotoxicity against CD19+ lymphomas and Her2-expressing breast cancer cell lines, while releasing lower levels of inflammatory cytokines, potentially leading to fewer adverse reactions like cytokine release syndrome (101). This approach also avoids the MHC class I restriction, enabling the development of ‘off-the-shelf’ therapies. Importantly, we were able to expand large numbers of these MAIT cells from PBMCs of healthy donors (typically more than 100-fold). Given that MAIT cell proportions can be as high as 10-20% of CD8 T cells in some donors, we believe that generating abundant cells for off-the-shelf therapeutic applications is likely achievable (101). Furthermore, MAIT cells may continue to expand in vivo, as they will still recognize and be activated by their common cognate antigen, thus requiring fewer cell transfers to patients.
Efforts to address the suboptimal ex vivo expansion efficiency of Mucosal-Associated Invariant T (MAIT) cells from healthy donor peripheral blood led to the development of pluripotent stem cell (PSC)-derived MAIT-like cells (Figure 1C) (103). Wakao et al. pioneered the reprogramming of cord blood (CB) MAIT cells into induced pluripotent stem cells (iPSCs) using a Sendai viral vector (103). The resulting MAIT-iPSC clones demonstrated pluripotency through various tests and were differentiated into MAIT-like lymphocytes using a two-step protocol (103). More recently, it was proposed that iPSC-derived Mucosal-Associated Invariant T (MAIT) cells in tumor immune cell therapy or prophylaxis may prevent relapse (104). While phenotypic and functional characteristics of reprogrammed MAIT cells do not fully mirror those of PBMC-derived MAIT cells (103), this innovative approach further aims to address the limitations associated with the ex vivo expansion of MAIT cells, such as the restricted numbers obtained after expansion compared to the ex vivo expansion of all T cells. Together, the ability to efficiently expand MAIT cells ex vivo while maintaining a functional phenotype may facilitate the development of new MAIT cell–based tumor immunotherapies.
One distinguishing characteristic that significantly contributes to their therapeutic appeal is their incapacity to induce graft-versus-host disease (GvHD) (105–107). This is attributed to their restriction by MR1 and their non-recognition of mismatched major histocompatibility complex (MHC) molecules and protein autoantigens (105). Rigorous assessments, including in vitro studies and xenogeneic GvHD mouse models, consistently affirm the GvHD-free safety profile of MAIT cells (108). Recent research endeavors have shed light on the multifaceted advantages of MAIT cells, extending beyond their GvHD-free safety profile. Notably, MAIT cells exhibit resistance to xenobiotics, adding an additional layer of appeal for their therapeutic application (2). Studies conducted by Bohineust et al. showcase that these engineered MAIT cells can engraft without eliciting GvHD in preclinical immunodeficient mouse models, presenting a distinct advantage over CD19-targeted CAR-T cells (109).
Furthermore, several studies demonstrated the potential for MAIT cells in solid tumor-targeting immunotherapies due to their natural ability to infiltrate tissue sites of chronic inflammation and their abundance in the tumor microenvironment (99, 101, 109, 110). In this tumor environment, MAIT cells can also act as innate T cells with the potential to bridge innate and adaptive immune immunity, and can rapidly exert their effector functions upon activation without the requirement for clonal expansion, similar to innate immune (111) (112). Upon activation, MAIT cells rapidly secrete cytokines and exert cytotoxic functions, making them highly relevant in tumor immunity (70). Indeed, such feature would be significant in that even in the case where tumors escape the attack from CAR-MAIT cells, they can still exert anti-tumor activity.
Overall, we think CAR-MAIT cells represent a significant advancement in our arsenal of immunotherapy. Their development could overcome the limitations of current CAR-T therapies and harness the immune system’s power more safely and effectively. Future studies, especially in vivo experiments will be needed to fully understand the therapeutic potential of CAR-MAIT cells.
6 MAIT cell and microbiome interplay in cancer
MAIT cells also play a crucial role in interacting with the microbiota and its products, protecting body barriers, and maintaining tissue homeostasis (113). MAIT cells have been found to be enriched in the human liver, which is constantly exposed to bacterial products from the intestine, indicating their role in the gut microbiota-liver axis (114, 115). Furthermore, MAIT cells have been shown to control barrier function and attenuate pathogenic T cell responses in the colon, suggesting their importance in maintaining gut integrity (116). The gut microbiome is crucial for the development and function of MAIT cells, and their association with the tumor microbiome supports the interest in manipulating the gut microbiome to reshape their response (56, 117).
Additionally, MAIT cells are thought to play a role in bacterial immunity, as they interact with metabolites synthesized by various yeast and bacteria (118). Moreover, MAIT cells have been proposed to play an important role in protecting mucosal surfaces against multiple bacterial pathogens (119). In contrast, studies have also revealed a deleterious role of MAIT cells in promoting inflammation, gut mucosa dysfunction, and gut microbiota alteration in obesity, indicating a complex role of MAIT cells in different contexts (3). Furthermore, the reduction and functional immaturity of MAIT cells in the peripheral blood of patients with primary Sjögren’s syndrome suggest a potential link between MAIT cells and autoimmune conditions (119).
The potential role of MAIT cells in the regulation of metabolic pathways and their crosstalk with the microbiota represents an exciting new line of research (120). In our studies focusing on activation of MAIT cells differing in their TCR Vbeta regions, we found that MAIT cells responded selectively to microorganisms that produce riboflavin metabolites, which are common across a variety of bacterial species within the microbiota (11). This suggests that MAIT cells play a role in fine-tuning the immune response to a broad spectrum of microbial inhabitants in the human body, including both commensal and pathogenic species. This ability to discriminate between different bacteria can be crucial for maintaining a balanced microbiome. The insights into how MAIT cells interact with the microbiome could have implications for understanding and treating diseases that are influenced by microbiota imbalances, such as inflammatory bowel disease, obesity, and certain types of cancer or immunotherapy as discussed below.
Growing evidence highlights a relationship between the human microbiome and cancer, particularly in the context of solid tumors. The composition of the microbiome has been found to offer prognostic insights and is implicated in the initiation, growth, and spread of tumors. Additionally, certain microbial signatures have been identified that may either contribute to tumor development or enhance the efficacy of immunotherapies (121–123).
MAIT cells, which have an affinity for microbe-rich environments and respond to microbial metabolites such as those from riboflavin metabolism, are poised to play a crucial role in the dialogue between the microbiome and the tumor microenvironment (52). They possess the unique ability to detect these metabolites presented by the MR1 protein on the surface of various cells, including tumor cells (6). Notably, Liu and Brutkiewicz et al. investigated the role of TLR9 activation in MR1-mediated bacterial antigen presentation and concluded that the activation of Toll-like Receptor 9 (TLR9) is crucial for Major Histocompatibility Complex Class I-Related gene (MR1)-mediated bacterial antigen presentation (124). Interestingly, studies have shown that melanoma cells can increase their expression of MR1 when exposed to the microbial metabolite 5-OP-RU, highlighting the potential for microbial components to modulate immune recognition (62).
Changes in the microbiota, termed dysbiosis, have been linked to tumor development and progression. Dysbiosis can lead to altered production of microbial metabolites, affecting the activation and function of MAIT cells. For example, a microbiota that favors inflammation may promote the secretion of pro-tumorigenic cytokines by MAIT cells, such as IL-17 and IL-13, contributing to tumor growth and progression (123). Conversely, certain microbial populations might induce anti-tumor responses by MAIT cells, enhancing their cytotoxic functions and the production of IFN-γ, which could suppress tumor growth. For instance, some studies suggest that specific bacterial strains can boost the efficacy of cancer immunotherapies, potentially through the activation of MAIT cells (125).
Moreover, the TME itself can alter the local microbiota, creating a feedback loop that further influences MAIT cell function (14, 126, 127). Tumor-induced changes in the local environment, such as hypoxia or altered nutrient availability, can select for microbial populations that either exacerbate or mitigate tumor progression (127).
The microbiota also plays a role in the modulation of the immune checkpoint molecules on MAIT cells. For example, certain bacteria might influence the expression of PD-1 on MAIT cells, affecting their state of exhaustion and ability to fight cancer cells (70, 128). Thus, given the central role of the microbiota in regulating immune responses, therapeutic strategies are being considered that could modulate the microbiota to enhance the anti-tumor activity of MAIT cells. These include the use of prebiotics, probiotics, or fecal microbiota transplants to reshape the microbial ecosystem in favor of a more robust anti-tumor immune response.
MAIT cells’ interactions with both cancer cells and the microbiome are integral to their dual role in tumor biology. Their capacity to respond to early cancerous changes, influenced by shifts in the microbiome, along with the ongoing research into their tumor recognition mechanisms, underscores the potential of MAIT cells as targets for innovative cancer therapies (56, 63).
7 Conclusions and future directions
In conclusion, current advances in MAIT cell immunobiology underscores the necessity to unravel the involvement and therapeutic potential of MAIT cells in in cancer biology, as well as to exploit the microbiome-MAIT cell interaction for the development of new cancer immunotherapies. The abundance of MAIT cells, ability to expand them in vitro with their antigen and possibility of generating these cells from iPSCs in humans presents an exciting avenue for future studies to develop them into CAR-MAIT cells. Other diverse avenues for future studies may include the exploration and development of immunotherapeutic strategies through utilization of MAIT cell anti-tumor recognition and functions and the potential use of small molecule or microbiome-derived therapeutics to stimulate their responses. These approaches highlight the promising prospects for advancing cancer treatment through understanding and developing MAIT cell-based therapeutics.
Author contributions
DU: Conceptualization, Writing – original draft, Writing – review & editing. MY: Writing – original draft, Writing – review & editing. OB: Visualization, Writing – review & editing.
Funding
The author(s) declare that no financial support was received for the research, authorship, and/or publication of this article.
Conflict of interest
The authors declare that the research was conducted in the absence of any commercial or financial relationships that could be construed as a potential conflict of interest.
Publisher’s note
All claims expressed in this article are solely those of the authors and do not necessarily represent those of their affiliated organizations, or those of the publisher, the editors and the reviewers. Any product that may be evaluated in this article, or claim that may be made by its manufacturer, is not guaranteed or endorsed by the publisher.
Abbreviations
MAIT, Mucosal-Associated Invariant T; PBMC, Peripheral Blood Mononuclear Cells; TCR, T Cell Receptor; MR1, Major Histocompatibility Complex Class I-Related Protein 1; TLR, Toll-Like Receptor; HIV, Human Immunodeficiency Virus; HBV, Hepatitis B Virus; NK, Natural Killer Cells; PD-1, Programmed Cell Death Protein 1; TME, Tumor Microenvironment; CTLA-4, Cytotoxic T-Lymphocyte-Associated Protein 4; TIM-3, T Cell Immunoglobulin and Mucin Domain 3; Treg, Regulatory T Cells; MDSC, Myeloid-Derived Suppressor Cells; aAPC, Artificial Antigen-Presenting Cells; GvHD: Graft-versus-Host Disease; iPSC, Induced Pluripotent Stem Cells; IFN, Interferon; TNF, Tumor Necrosis Factor; IL, Interleukin.
References
1. Martin E, Treiner E, Duban L, Guerri L, Laude H, Toly C, et al. Stepwise development of MAIT cells in mouse and human. PloS Biol. (2009) 7:e54. doi: 10.1371/journal.pbio.1000054
2. Dusseaux M, Martin E, Serriari N, Peguillet I, Premel V, Louis D, et al. Human MAIT cells are xenobiotic-resistant, tissue-targeted, CD161hi IL-17-secreting T cells. Blood. (2011) 117:1250–9. doi: 10.1182/blood-2010-08-303339
3. Toubal A, Nel I, Lotersztajn S, Lehuen A. Mucosal-associated invariant T cells and disease. Nat Rev Immunol. (2019) 19:643–57. doi: 10.1038/s41577-019-0191-y
4. Ioannidis M, Cerundolo V, Salio M. The immune modulating properties of mucosal-associated invariant T cells. Front Immunol. (2020) 11:1556. doi: 10.3389/fimmu.2020.01556
5. Treiner E, Duban L, Bahram S, Radosavljevic M, Wanner V, Tilloy F, et al. Selection of evolutionarily conserved mucosal-associated invariant T cells by MR1. Nature. (2003) 422:164–9. doi: 10.1038/nature01433
6. Kjer-Nielsen L, Patel O, Corbett AJ, Le Nours J, Meehan B, Liu L, et al. MR1 presents microbial vitamin B metabolites to MAIT cells. Nature. (2012) 491:717–23. doi: 10.1038/nature11605
7. Eckle SB, Corbett AJ, Keller AN, Chen Z, Godfrey DI, Liu L, et al. Recognition of vitamin B precursors and byproducts by mucosal associated invariant T cells. J Biol Chem. (2015) 290:30204–11. doi: 10.1074/jbc.R115.685990
8. Le Bourhis L, Martin E, Peguillet I, Guihot A, Froux N, Core M, et al. Antimicrobial activity of mucosal-associated invariant T cells. Nat Immunol. (2010) 11:701–8. doi: 10.1038/ni.1890
9. Lepore M, Kalinichenko A, Colone A, Paleja B, Singhal A, Tschumi A, et al. Parallel T-cell cloning and deep sequencing of human MAIT cells reveal stable oligoclonal TCRbeta repertoire. Nat Commun. (2014) 5:3866. doi: 10.1038/ncomms4866
10. Meermeier EW, Harriff MJ, Karamooz E, Lewinsohn DM. MAIT cells and microbial immunity. Immunol Cell Biol. (2018) 96:607–17. doi: 10.1111/imcb.12022
11. Tastan C, Karhan E, Zhou W, Fleming E, Voigt AY, Yao X, et al. Tuning of human MAIT cell activation by commensal bacteria species and MR1-dependent T-cell presentation. Mucosal Immunol. (2018) 11:1591–605. doi: 10.1038/s41385-018-0072-x
12. Garner LC, Amini A, FitzPatrick MEB, Lett MJ, Hess GF, Filipowicz Sinnreich M, et al. Single-cell analysis of human MAIT cell transcriptional, functional and clonal diversity. Nat Immunol. (2023) 24:1565–78. doi: 10.1038/s41590-023-01575-1
13. Constantinides MG, Link VM, Tamoutounour S, Wong AC, Perez-Chaparro PJ, Han SJ, et al. MAIT cells are imprinted by the microbiota in early life and promote tissue repair. Science. (2019) 366. doi: 10.1126/science.aax6624
14. Jabeen M, Hinks T. MAIT cells and the microbiome. Front Immunol. (2023) 14. doi: 10.3389/fimmu.2023.1127588
15. Meierovics AI, Cowley SC. MAIT cells promote inflammatory monocyte differentiation into dendritic cells during pulmonary intracellular infection. J Exp Med. (2016) 213:2793–809. doi: 10.1084/jem.20160637
16. Hinks TSC, Zhang XW. MAIT cell activation and functions. Front Immunol. (2020) 11:1014. doi: 10.3389/fimmu.2020.01014
17. Fan Q, Nan H, Li Z, Li BT, Zhang FZ, Bi LQ. New insights into MAIT cells in autoimmune diseases. Biomedicine Pharmacotherapy. (2023) 159. doi: 10.1016/j.biopha.2023.114250
18. Turtle CJ, Delrow JJ, Joslyn RC, Swanson HM, Basom R, Tabellini L, et al. Innate signals overcome acquired TCR signaling pathway regulation and govern the fate of human CD161hi CD8α+ Semi-invariant T cells. Blood. (2011) 118(10):2752–62. doi: 10.1182/blood-2011-02-334698
19. Hagel JP, Garner LC, Bilton M, Mehta H, Leng TQ, Hackstein CP, et al. Human MAIT cell activation in vitro. Mait Cells: Methods Protoc. (2020) 2098:97–124. doi: 10.1007/978-1-0716-0207-2_7
20. Tao HS, Pan Y, Chu S, Li L, Xie JH, Wang P, et al. Differential controls of MAIT cell effector polarization by mTORC1/mTORC2 via integrating cytokine and costimulatory signals. Nat Commun. (2021) 12. doi: 10.1038/s41467-021-22162-8
21. Li YR, Zhou K, Wilson M, Kramer A, Zhu Y, Dawson N, et al. Mucosal-associated invariant T cells for cancer immunotherapy. Mol Ther. (2023) 31:631–46. doi: 10.1016/j.ymthe.2022.11.019
22. Ussher JE, Bilton M, Attwod E, Shadwell J, Richardson R, de Lara C, et al. CD161++ CD8+ T cells, including the MAIT cell subset, are specifically activated by IL-12+IL-18 in a TCR-independent manner. Eur J Immunol. (2014) 44:195–203. doi: 10.1002/eji.201343509
23. Kurioka A, Ussher JE, Cosgrove C, Clough C, Fergusson JR, Smith K, et al. MAIT cells are licensed through granzyme exchange to kill bacterially sensitized targets. Mucosal Immunol. (2015) 8(2):429–40. doi: 10.1038/mi.2014.81
24. van Wilgenburg B, Scherwitzl I, Hutchinson EC, Leng T, Kurioka A, Kulicke C, et al. MAIT cells are activated during human viral infections. Nat Commun. (2016) 7:11653. doi: 10.1038/ncomms11653
25. Leeansyah E, Ganesh A, Quigley MF, Sonnerborg A, Andersson J, Hunt PW, et al. Activation, exhaustion, and persistent decline of the antimicrobial MR1-restricted MAIT-cell population in chronic HIV-1 infection. Blood. (2013) 121:1124–35. doi: 10.1182/blood-2012-07-445429
26. Sakai S, Kauffman KD, Oh S, Nelson CE, Barry CE 3rd, Barber DL. MAIT cell-directed therapy of Mycobacterium tuberculosis infection. Mucosal Immunol. (2021) 14:199–208. doi: 10.1038/s41385-020-0332-4
27. Chua WJ, Truscott SM, Eickhoff CS, Blazevic A, Hoft DF, Hansen TH. Polyclonal mucosa-associated invariant T cells have unique innate functions in bacterial infection. Infect Immun. (2012) 80:3256–67. doi: 10.1128/IAI.00279-12
28. Boulouis C, Sia WR, Gulam MY, Teo JQM, Png YT, Phan TK, et al. Human MAIT cell cytolytic effector proteins synergize to overcome carbapenem resistance in. PloS Biol. (2020) 18. doi: 10.1101/2020.01.16.908806
29. Wu SH, Yang X, Lou YL, Xiao XX. MAIT cells in bacterial infectious diseases: heroes, villains, or both?. Clin Exp Immunol. (2023). doi: 10.1093/cei/uxad102
30. van Wilgenburg B, Loh L, Chen ZJ, Pediongco TJ, Wang HM, Shi M, et al. MAIT cells contribute to protection against lethal influenza infection in vivo. Nat Commun. (2018) 9. doi: 10.1038/s41467-018-07207-9
31. Hartmann N, Harriff MJ, McMurtrey CP, Hildebrand WH, Lewinsohn DM, Kronenberg M. Role of MAIT cells in pulmonary bacterial infection. Mol Immunol. (2018) 101:155–59. doi: 10.1016/j.molimm.2018.06.270
32. Rudak PT, Choi JJ, Haeryfar SMM. MAIT cell-mediated cytotoxicity: roles in host defense and therapeutic potentials in infectious diseases and cancer. J Leukocyte Biol. (2018) 104(3):473–86. doi: 10.1002/JLB.4RI0118-023R
33. Nel I, Bertrand L, Toubal A, Lehuen A. MAIT cells, guardians of skin and mucosa?. Mucosal Immunol. (2021) 14:803–14. doi: 10.1038/s41385-021-00391-w
34. Fernandez CS, Amarasena T, Kelleher AD, Rossjohn J, McCluskey J, Godfrey DI, et al. MAIT cells are depleted early but retain functional cytokine expression in HIV infection. Immunol Cell Biol. (2015) 93:177–88. doi: 10.1038/icb.2014.91
35. Chen Z, Wang H, D'Souza C, Sun S, Kostenko L, Eckle SB, et al. Mucosal-associated invariant T-cell activation and accumulation after in vivo infection depends on microbial riboflavin synthesis and co-stimulatory signals. Mucosal Immunol. (2017) 10:58–68. doi: 10.1038/mi.2016.39
36. Meierovics A, Yankelevich WJ, Cowley SC. MAIT cells are critical for optimal mucosal immune responses during in vivo pulmonary bacterial infection. Proc Natl Acad Sci U.S.A. (2013) 110:E3119–28. doi: 10.1073/pnas.1302799110
37. Wang H, D'Souza C, Lim XY, Kostenko L, Pediongco TJ, Eckle SBG, et al. MAIT cells protect against pulmonary Legionella longbeachae infection. Nat Commun. (2018) 9:3350. doi: 10.1038/s41467-018-05202-8
38. Shibata K, Shimizu T, Nakahara M, Ito E, Legoux F, Fujii S, et al. The intracellular pathogen Francisella tularensis escapes from adaptive immunity by metabolic adaptation. Life Sci Alliance. (2022) 5. doi: 10.26508/lsa.202201441
39. Georgel P, Radosavljevic M, Macquin C, Bahram S. The non-conventional MHC class I MR1 molecule controls infection by Klebsiella pneumoniae in mice. Mol Immunol. (2011) 48:769–75. doi: 10.1016/j.molimm.2010.12.002
40. Smith AD, Foss ED, Zhang I, Hastie JL, Giordano NP, Gasparyan L, et al. Microbiota of MR1 deficient mice confer resistance against Clostridium difficile infection. PloS One. (2019) 14:e0223025. doi: 10.1371/journal.pone.0223025
41. Zhao Z, Wang H, Shi M, Zhu T, Pediongco T, Lim XY, et al. Francisella tularensis induces Th1 like MAIT cells conferring protection against systemic and local infection. Nat Commun. (2021) 12:4355. doi: 10.1038/s41467-021-24570-2
42. Zheng Y, Han F, Ho A, Xue Y, Wu Z, Chen X, et al. Role of MAIT cells in gastrointestinal tract bacterial infections in humans: More than a gut feeling. Mucosal Immunol. (2023) 16:740–52. doi: 10.1016/j.mucimm.2023.06.005
43. Sakai S, Lora NE, Kauffman KD, Dorosky DE, Oh S, Namasivayam S, et al. Functional inactivation of pulmonary MAIT cells following 5-OP-RU treatment of non-human primates. Mucosal Immunol. (2021) 14:1055–66. doi: 10.1038/s41385-021-00425-3
44. Barber-Axthelm IM, Kent SJ, Juno JA. Understanding the role of mucosal-associated invariant T-cells in non-human primate models of HIV infection. Front Immunol. (2020) 11:2038. doi: 10.3389/fimmu.2020.02038
45. Phetsouphanh C, Phalora P, Hackstein CP, Thornhill J, Munier CML, Meyerowitz J, et al. Human MAIT cells respond to and suppress HIV-1. Elife. (2021) 10. doi: 10.7554/eLife.50324
46. Parrot T, Gorin JB, Ponzetta A, Maleki KT, Kammann T, Emgard J, et al. MAIT cell activation and dynamics associated with COVID-19 disease severity. Sci Immunol. (2020) 5. doi: 10.1101/2020.08.27.20182550
47. Yang Q, Wen Y, Qi F, Gao X, Chen W, Xu G, et al. Suppressive monocytes impair MAIT cells response via IL-10 in patients with severe COVID-19. J Immunol. (2021) 207:1848–56. doi: 10.4049/jimmunol.2100228
48. Flament H, Rouland M, Beaudoin L, Toubal A, Bertrand L, Lebourgeois S, et al. Outcome of SARS-CoV-2 infection is linked to MAIT cell activation and cytotoxicity. Nat Immunol. (2021) 22:322–35. doi: 10.1038/s41590-021-00870-z
49. Tan HY, Yong YK, Ng CS, Vimali J, Mohamed R, Murugesan A, et al. Mucosal-associated invariant T cells: diplomatic front-runners in the fight against hepatitis B virus infection. Crit Rev Immunol. (2021) 41:1–16. doi: 10.1615/CritRevImmunol.v41.i5
50. Huang W, He W, Shi X, Ye Q, He X, Dou L, et al. Mucosal-associated invariant T-cells are severely reduced and exhausted in humans with chronic HBV infection. J Viral Hepat. (2020) 27:1096–107. doi: 10.1111/jvh.13341
51. Loh L, Wang Z, Sant S, Koutsakos M, Jegaskanda S, Corbett AJ, et al. Human mucosal-associated invariant T cells contribute to antiviral influenza immunity via IL-18-dependent activation. Proc Natl Acad Sci U.S.A. (2016) 113:10133–8. doi: 10.1073/pnas.1610750113
52. Godfrey DI, Koay HF, McCluskey J, Gherardin NA. The biology and functional importance of MAIT cells. Nat Immunol. (2019) 20:1110–28. doi: 10.1038/s41590-019-0444-8
53. Joyce S, Okoye GD, Driver JP. Die Kampfe und schlachten-the struggles and battles of innate-like effector T lymphocytes with microbes. Front Immunol. (2023) 14:1117825. doi: 10.3389/fimmu.2023.1117825
54. Haeryfar SMM, Shaler CR, Rudak PT. Mucosa-associated invariant T cells in malignancies: a faithful friend or formidable foe? Cancer Immunol Immunother. 67(12):1885–96. doi: 10.1007/s00262-018-2132-1
55. Berzins SP, Wallace ME, Kannourakis G, Kelly J. A role for MAIT cells in colorectal cancer. Front Immunol. (2020) 11:949. doi: 10.3389/fimmu.2020.00949
56. Li S, Simoni Y, Becht E, Loh CY, Li N, Lachance D, et al. Human tumor-infiltrating MAIT cells display hallmarks of bacterial antigen recognition in colorectal cancer. Cell Rep Med. (2020) 1:100039. doi: 10.1016/j.xcrm.2020.100039
57. Yan J, Allen S, McDonald E, Das I, Mak JYW, Liu L, et al. MAIT cells promote tumor initiation, growth, and metastases via tumor MR1. Cancer Discov. (2020) 10:124–41. doi: 10.1158/2159-8290.CD-19-0569
58. Petley EV, Koay HF, Henderson MA, Sek K, Todd KL, Keam SP, et al. MAIT cells regulate NK cell-mediated tumor immunity. Nat Commun. (2021) 12:4746 doi: 10.1038/s41467-021-25009-4
59. Ling L, Lin Y, Zheng W, Hong S, Tang X, Zhao P, et al. Circulating and tumor-infiltrating mucosal associated invariant T (MAIT) cells in colorectal cancer patients. Sci Rep. (2016) 6:20358. doi: 10.1038/srep20358
60. Melo AM, O'Brien AM, Phelan JJ, Kennedy SA, Wood NAW, Veerapen N, et al. Mucosal-associated invariant T cells display diminished effector capacity in oesophageal adenocarcinoma. Front Immunol. (2019) 10:1580. doi: 10.3389/fimmu.2019.01580
61. Kubica P, Lara-Velazquez M, Bam M, Siraj S, Ong I, Liu P, et al. MR1 overexpression correlates with poor clinical prognosis in glioma patients. Neurooncol Adv. (2021) 3:vdab034. doi: 10.1093/noajnl/vdab034
62. Gherardin NA, Loh L, Admojo L, Davenport AJ, Richardson K, Rogers A, et al. Enumeration, functional responses and cytotoxic capacity of MAIT cells in newly diagnosed and relapsed multiple myeloma. Sci Rep. (2018) 8:4159. doi: 10.1038/s41598-018-22130-1
63. Cogswell DT, Gapin L, Tobin HM, McCarter MD, Tobin RP. MAIT cells: partners or enemies in cancer immunotherapy?. Cancers (Basel). (2021) 13. doi: 10.3390/cancers13071502
64. Shao C, Zhu C, Zhu Y, Hao J, Li Y, Hu H, et al. Decrease of peripheral blood mucosal-associated invariant T cells and impaired serum Granzyme-B production in patients with gastric cancer. Cell Biosci. (2021) 11:12. doi: 10.1186/s13578-020-00518-9
65. Gentles AJ, Newman AM, Liu CL, Bratman SV, Feng W, Kim D, et al. The prognostic landscape of genes and infiltrating immune cells across human cancers. Nat Med. (2015) 21:938–45. doi: 10.1038/nm.3909
66. Shi L, Lu J, Zhong D, Song M, Liu J, You W, et al. Clinicopathological and predictive value of MAIT cells in non-small cell lung cancer for immunotherapy. J Immunother Cancer. (2023) 11. doi: 10.1136/jitc-2022-005902
67. Jarvis EM, Collings S, Authier-Hall A, Dasyam N, Luey B, Nacey J, et al. Mucosal-associated invariant T (MAIT) cell dysfunction and PD-1 expression in prostate cancer: implications for immunotherapy. Front Immunol. (2021) 12:748741. doi: 10.3389/fimmu.2021.748741
68. Won EJ, Ju JK, Cho Y-N, Jin H-M, Park K-J, Kim T-J, et al. Clinical relevance of circulating mucosal-associated invariant T cell levels and their anti-cancer activity in patients with mucosal-associated cancer. Oncotarget. (2016). doi: 10.18632/oncotarget.v7i46
69. Mo J, Li Z, Gao Z, Wu J, Bao Y. The study of mucosal-associated invariant T cells in colon cancer and roles in immune activities. Oncotargets Ther. (2021) 2021(14):5263–73. doi: 10.2147/OTT.S332822
70. Rodin W, Sundstrom P, Ahlmanner F, Szeponik L, Zajt KK, Wettergren Y, et al. Exhaustion in tumor-infiltrating Mucosal-Associated Invariant T (MAIT) cells from colon cancer patients. Cancer Immunol Immunother. (2021) 70:3461–75. doi: 10.1007/s00262-021-02939-y
71. Zhang Q, Li P, Zhou W, Fang S, Wang J. Participation of increased circulating MAIT cells in lung cancer: a pilot study. J Cancer. (2022) 13:1623–29. doi: 10.7150/jca.69415
72. Shaler CR, Tun-Abraham ME, Skaro AI, Khazaie K, Corbett AJ, Mele T, et al. Mucosa-associated invariant T cells infiltrate hepatic metastases in patients with colorectal carcinoma but are rendered dysfunctional within and adjacent to tumor microenvironment. Cancer Immunol Immunother. (2017) 66:1563–75. doi: 10.1007/s00262-017-2050-7
73. Kelly J, Minoda Y, Meredith T, Cameron G, Philipp MS, Pellicci DG, et al. Chronically stimulated human MAIT cells are unexpectedly potent IL-13 producers. Immunol Cell Biol. (2019) 97:689–99. doi: 10.1111/imcb.12281
74. Qian X, Chen H, Wu X, Hu L, Huang Q, Jin Y. Interleukin-17 acts as double-edged sword in anti-tumor immunity and tumorigenesis. Cytokine. (2017) 89:34–44. doi: 10.1016/j.cyto.2015.09.011
75. Zhao J, Chen X, Herjan T, Li X. The role of interleukin-17 in tumor development and progression. J Exp Med. (2020) 217. doi: 10.1084/jem.20190297
76. Wu F, Xu J, Huang Q, Han J, Duan L, Fan J, et al. The role of interleukin-17 in lung cancer. Mediators Inflammation. (2016) 2016:8494079. doi: 10.1155/2016/8494079
77. Hurtado CG, Wan F, Housseau F, Sears CL. Roles for interleukin 17 and adaptive immunity in pathogenesis of colorectal cancer. Gastroenterology. (2018) 155:1706–15. doi: 10.1053/j.gastro.2018.08.056
78. Li X, Liu MK, Shi Q, Fang Y, Fu D, Shen ZX, et al. Elevated serum IL-13 level is associated with increased Treg cells in tumor microenvironment and disease progression of diffuse large B-cell lymphoma. Hematological Oncol. (2023) 41:230–38. doi: 10.1002/hon.2993
79. Sundström P, Ahlmanner F, Akéus P, Sundquist M, Alsén S, Yrlid U, et al. Human mucosa-associated invariant T cells accumulate in colon adenocarcinomas but produce reduced amounts of IFN-γ. J Immunol. (2015) 195(7):3472–81. doi: 10.4049/jimmunol.1500258
80. Huang WC, Hsiao YC, Wu CC, Hsu YT, Chang CL. Less circulating mucosal-associated invariant T cells in patients with cervical cancer. Taiwan J Obstet Gynecol. (2019) 58:117–21. doi: 10.1016/j.tjog.2018.11.022
81. Grivennikov SI, Wang K, Mucida D, Stewart CA, Schnabl B, Jauch D, et al. Adenoma-linked barrier defects and microbial products drive IL-23/IL-17-mediated tumour growth. Nature. (2012) 491:254–8. doi: 10.1038/nature11465
82. Zabijak L, Attencourt C, Guignant C, Chatelain D, Marcelo P, Marolleau J-P, et al. Increased tumor infiltration by mucosal-associated invariant T cells correlates with poor survival in colorectal cancer patients. Cancer Immunol Immunotherapy. (2015) 64:1601–8. doi: 10.1007/s00262-015-1764-7
83. Sundstrom P, Ahlmanner F, Akeus P, Sundquist M, Alsen S, Yrlid U, et al. Human mucosa-associated invariant T cells accumulate in colon adenocarcinomas but produce reduced amounts of IFN-γ. J Immunol. (2015) 195:3472–81. doi: 10.4049/jimmunol.1500258
84. Lu Z, Zhu M, Marley JL, Bi K, Wang K, Zhai M, et al. The combined action of monocytic myeloid-derived suppressor cells and mucosal-associated invariant T cells promotes the progression of cervical cancer. Int J Cancer. 148(6):1499–507. doi: 10.1002/ijc.33411
85. Zumwalde NA, Haag JD, Gould MN, Gumperz JE. Mucosal associated invariant T cells from human breast ducts mediate a Th17-skewed response to bacterially exposed breast carcinoma cells. Breast Cancer Res. (2018) 20:111. doi: 10.1186/s13058-018-1036-5
86. Zheng C, Zheng L, Yoo JK, Guo H, Zhang Y, Guo X, et al. Landscape of infiltrating T cells in liver cancer revealed by single-cell sequencing. Cell. (2017) 169:1342–56.e16. doi: 10.1016/j.cell.2017.05.035
87. Duan M, Goswami S, Shi JY, Wu LJ, Wang XY, Ma JQ, et al. Activated and exhausted MAIT cells foster disease progression and indicate poor outcome in hepatocellular carcinoma. Clin Cancer Res. (2019) 25:3304–16. doi: 10.1158/1078-0432.CCR-18-3040
88. Wherry EJ, Kurachi M. Molecular and cellular insights into T cell exhaustion. Nat Rev Immunol. (2015) 15:486–99. doi: 10.1038/nri3862
89. McLane LM, Abdel-Hakeem MS, Wherry EJ. CD8 T cell exhaustion during chronic viral infection and cancer. Annu Rev Immunol. (2019) 37:457–495. doi: 10.1146/annurev-immunol-041015-055318
90. Tobin RP, Jordan KR, Kapoor P, Spongberg E, Davis D, Vorwald VM, et al. IL-6 and IL-8 are linked with myeloid-derived suppressor cell accumulation and correlate with poor clinical outcomes in melanoma patients. Front Oncol. (2019) 9:1223. doi: 10.3389/fonc.2019.01223
91. Kurioka A, Cosgrove C, Simoni Y, Wilgenburg Bv, Geremia A, Björkander S, et al. CD161 defines a functionally distinct subset of pro-inflammatory natural killer cells. Front Immunol. (2018) 9. doi: 10.3389/fimmu.2018.00486
92. Väyrynen JP, Kantola T, Klintrup K, Bloigu R, Karhu T, Mäkelä J, et al. The relationships between serum cytokine levels and tumor infiltrating immune cells and their clinical significance in colorectal cancer. Int J Cancer. (2016). 139(1):112–21. doi: 10.1002/ijc.30040
93. Lamichhane R, Schneider M, Sara M, de la Harpe SM, Harrop TWR, Hannaway RF, et al. TCR- or cytokine-activated CD8+ Mucosal-associated invariant T cells are rapid polyfunctional effectors that can coordinate immune responses. Cell Rep. (2019). doi: 10.1101/600189
94. Mori L, Lepore M, De Libero G. The immunology of CD1- and MR1-restricted T cells. Annu Rev Immunol. (2016) 34:479–510. doi: 10.1146/annurev-immunol-032414-112008
95. Salio M, Gasser O, Lopez CG, Martens AWJ, Veerapen N, Gileadi U, et al. Activation of human mucosal-associated invariant T cells induces CD40L-dependent maturation of monocyte-derived and primary dendritic cells. J Immunol. (2017) 199(8):2631–8. doi: 10.4049/jimmunol.1700615
96. De Biasi S, Gibellini L, Lo Tartaro D, Puccio S, Rabacchi C, Mazza EMC, et al. Circulating mucosal-associated invariant T cells identify patients responding to anti-PD-1 therapy. Nat Commun. 12(1):1669. doi: 10.1038/s41467-021-21928-4
97. Vorwald VM, Davis DM, Van Gulick RJ, Torphy RJ, Borgers JS, Klarquist J, et al. Circulating CD8(+) mucosal-associated invariant T cells correlate with improved treatment responses and overall survival in anti-PD-1-treated melanoma patients. Clin Transl Immunol. (2022) 11:e1367. doi: 10.1002/cti2.1367
98. Gao MG, Zhao XS. Mining the multifunction of mucosal-associated invariant T cells in hematological Malignancies and transplantation immunity: A promising hexagon soldier in immunomodulatory. Front Immunol. (2022) 13:931764. doi: 10.3389/fimmu.2022.931764
99. Dey M, Kim MH, Nagamine M, Karhan E, Kozhaya L, Dogan M, et al. Biofabrication of 3D breast cancer models for dissecting the cytotoxic response of human T cells expressing engineered MAIT cell receptors. Biofabrication. (2022) 14. doi: 10.1101/2022.01.29.478309
100. Priya R, Brutkiewicz RR. MR1 tetramer-based artificial APCs expand MAIT cells from human peripheral blood that effectively kill glioblastoma cells. Immunohorizons. (2021) 5:500–11. doi: 10.4049/immunohorizons.2100003
101. Dogan M, Karhan E, Kozhaya L, Placek L, Chen X, Yigit M, et al. Engineering human MAIT cells with chimeric antigen receptors for cancer immunotherapy. J Immunol. (2022) 209(8). doi: 10.4049/jimmunol.2100856
102. Sterner RC, Sterner RM. CAR-T cell therapy: current limitations and potential strategies. Blood Cancer J. (2021) 11:69. doi: 10.1038/s41408-021-00459-7
103. Wakao H, Yoshikiyo K, Koshimizu U, Furukawa T, Enomoto K, Matsunaga T, et al. Expansion of functional human mucosal-associated invariant T cells via reprogramming to pluripotency and redifferentiation. Cell Stem Cell. (2013) 12:546–58. doi: 10.1016/j.stem.2013.03.001
104. Sugimoto C, Fujita H, Wakao H. Harnessing the power of mucosal-associated invariant T (MAIT) cells in cancer cell therapy. Biomedicines. (2022) 10. doi: 10.3390/biomedicines10123160
105. Kurioka A, Jahun AS, Hannaway RF, Walker LJ, Fergusson JR, Sverremark-Ekstrom E, et al. Shared and distinct phenotypes and functions of human CD161++ Valpha7.2+ T cell subsets. Front Immunol. (2017) 8:1031. doi: 10.3389/fimmu.2017.01031
106. Li YR, Brown J, Yu Y, Lee D, Zhou K, Dunn ZS, et al. Targeting immunosuppressive tumor-associated macrophages using innate T cells for enhanced antitumor reactivity. Cancers (Basel). (2022) 14. doi: 10.3390/cancers14112749
107. Wang Z, Zhang S, Zhang X, Liu L, Zhou L, Shen Y, et al. Mucosal-associated invariant T cells predict increased acute graft-versus-host-disease incidence in patients receiving allogeneic hematopoietic stem cell transplantation. Cancer Cell Int. (2022) 22:297. doi: 10.1186/s12935-022-02703-x
108. Tourret M, Talvard-Balland N, Lambert M, Ben Youssef G, Chevalier MF, Bohineust A, et al. Human MAIT cells are devoid of alloreactive potential: prompting their use as universal cells for adoptive immune therapy. J Immunother Cancer. (2021) 9. doi: 10.1101/2021.04.29.21256184
109. Bohineust A, Tourret M, Derivry L, Caillat-Zucman S. Mucosal-associated invariant T (MAIT) cells, a new source of universal immune cells for chimeric antigen receptor (CAR)-cell therapy. Bull Cancer. (2021) 108:S92–5. doi: 10.1016/j.bulcan.2021.07.003
110. Labuz D, Cacioppo J, Li K, Aube J, Leung DT. Enhancing mucosal-associated invariant T cell function and expansion with human selective serum. Immunohorizons. (2023) 7:116–24. doi: 10.4049/immunohorizons.2200082
111. Wakao H, Sugimoto C, Kimura S, Wakao R. Mucosal-associated invariant T cells in regenerative medicine. Front Immunol. (2017) 8:1711. doi: 10.3389/fimmu.2017.01711
112. Garner LC, Klenerman P, Provine NM. Insights into mucosal-associated invariant T cell biology from studies of invariant natural killer T cells. Front Immunol. (2018) 9:1478. doi: 10.3389/fimmu.2018.01478
113. Mechelli R, Romano S, Romano C, Morena E, Buscarinu MC, Bigi R, et al. MAIT cells and microbiota in multiple sclerosis and other autoimmune diseases. Microorganisms. (2021) 9. doi: 10.3390/microorganisms9061132
114. Tang XZ, Jo J, Tan AT, Sandalova E, Chia A, Tan KC, et al. IL-7 licenses activation of human liver intrasinusoidal mucosal-associated invariant T cells. J Immunol. (2013) 190:3142–52. doi: 10.4049/jimmunol.1203218
115. Gebru YA, Choi MR, Raja G, Gupta H, Sharma SP, Choi YR, et al. Pathophysiological roles of mucosal-associated invariant T cells in the context of gut microbiota-liver axis. Microorganisms. (2021) 9. doi: 10.3390/microorganisms9020296
116. Varelias A, Gartlan KH, Wilkinson AN, Olver SD, Samson LD, Tey SK, et al. Expansion of IL-17A-secreting CD8(+) mucosa-associated invariant T cells in peripheral blood following stem cell mobilization. Blood Adv. (2019) 3:718–23. doi: 10.1182/bloodadvances.2018025601
117. Gibbs A, Healy K, Kaldhusdal V, Sundling C, Franzen-Boger M, Edfeldt G, et al. Preserved mucosal-associated invariant T cells in the cervical mucosa of HIV-infected women with dominant expression of the TRAV1-2-TRAJ20 T cell receptor alpha-chain. J Infect Dis. (2022) 226:1428–40. doi: 10.1093/infdis/jiac171
118. Koppejan H, Jansen DTSL, Hameetman M, Thomas R, Toes REM, van Gaalen FA. Altered composition and phenotype of mucosal-associated invariant T cells in early untreated rheumatoid arthritis. Arthritis Res Ther. (2019) 21:3. doi: 10.1186/s13075-018-1799-1
119. Wang H, Hogquist KA. How MAIT cells get their start. Nat Immunol. (2016) 17:1238–40. doi: 10.1038/ni.3584
120. Magalhaes I, Pingris K, Poitou C, Bessoles S, Venteclef N, Kiaf B, et al. Mucosal-associated invariant T cell alterations in obese and type 2 diabetic patients. J Clin Invest. (2015) 125:1752–62. doi: 10.1172/JCI78941
121. Elinav E, Garrett WS, Trinchieri G, Wargo J. The cancer microbiome. Nat Rev Cancer. (2019) 19:371–76. doi: 10.1038/s41568-019-0155-3
122. Doocey CM, Finn K, Murphy C, Guinane CM. The impact of the human microbiome in tumorigenesis, cancer progression, and biotherapeutic development. BMC Microbiol. (2022) 22:53. doi: 10.1186/s12866-022-02465-6
123. Sadrekarimi H, Gardanova ZR, Bakhshesh M, Ebrahimzadeh F, Yaseri AF, Thangavelu L, et al. Emerging role of human microbiome in cancer development and response to therapy: special focus on intestinal microflora. J Transl Med. (2022) 20:301. doi: 10.1186/s12967-022-03492-7
124. Liu J, Brutkiewicz RR. The Toll-like receptor 9 signalling pathway regulates MR1-mediated bacterial antigen presentation in B cells. Immunology. (2017) 152:232–42. doi: 10.1111/imm.12759
125. Yang W, Cong Y. Gut microbiota-derived metabolites in the regulation of host immune responses and immune-related inflammatory diseases. Cell Mol Immunol. (2021) 18:866–77. doi: 10.1038/s41423-021-00661-4
126. Qiu Q, Lin Y, Ma Y, Li X, Liang J, Chen Z, et al. Exploring the emerging role of the gut microbiota and tumor microenvironment in cancer immunotherapy. Front Immunol. (2020) 11:612202. doi: 10.3389/fimmu.2020.612202
127. Park EM, Chelvanambi M, Bhutiani N, Kroemer G, Zitvogel L, Wargo JA. Targeting the gut and tumor microbiota in cancer. Nat Med. (2022) 28:690–703. doi: 10.1038/s41591-022-01779-2
Keywords: MAIT cell, CAR-MAIT, immunotherapy, cancer, microbiome, peripheral blood mononuclear cells
Citation: Yigit M, Basoglu OF and Unutmaz D (2024) Mucosal-associated invariant T cells in cancer: dual roles, complex interactions and therapeutic potential. Front. Immunol. 15:1369236. doi: 10.3389/fimmu.2024.1369236
Received: 11 January 2024; Accepted: 26 February 2024;
Published: 13 March 2024.
Edited by:
Luc Van Kaer, Vanderbilt University Medical Center, United StatesReviewed by:
Hiroshi Wakao, Dokkyo Medical University, JapanSebastian Joyce, Vanderbilt University, United States
Randy Brutkiewicz, Indiana University Bloomington, United States
Copyright © 2024 Yigit, Basoglu and Unutmaz. This is an open-access article distributed under the terms of the Creative Commons Attribution License (CC BY). The use, distribution or reproduction in other forums is permitted, provided the original author(s) and the copyright owner(s) are credited and that the original publication in this journal is cited, in accordance with accepted academic practice. No use, distribution or reproduction is permitted which does not comply with these terms.
*Correspondence: Derya Unutmaz, ZGVyeWFAbWFjLmNvbQ==