- 1Division of Public Health, Infectious Diseases and Occupational Medicine, Department of Medicine, Mayo Clinic, Rochester, MN, United States
- 2William J. von Liebig Center for Transplantation and Clinical Regeneration, Mayo Clinic, Rochester, MN, United States
While the general population regained a certain level of normalcy with the end of the global health emergency, the risk of contracting COVID-19 with a severe outcome is still a major concern for people with compromised immunity. This paper reviews the impact of COVID-19 on people with immunocompromised status, identifies the gaps in the current management landscape, and proposes actions to address this unmet need. Observational studies have demonstrated that people with immune dysfunction have a higher risk of COVID-19–related hospitalization and death, despite vaccination, than the general population. More research is needed to define the optimal prevention and treatment strategies that are specific to people with immunocompromised status, including novel vaccination strategies, monoclonal antibodies that provide passive immunity and complement suboptimal vaccination responses, and improved and safer antiviral treatment for COVID-19. Preventive measures beyond vaccination alone are urgently needed to protect this vulnerable population.
Introduction
Rapid medical advances and increased scientific understanding have led to improvements in the management of COVID-19; however, people with compromised immunity remain at increased risk of contracting SARS-CoV-2 and experiencing severe outcomes, including hospitalization and death (1). This heterogenous population comprises approximately 6.6% of US adults (2), and includes people with solid and hematologic cancers, advanced HIV, primary immunodeficiencies, and those taking immunosuppressive drugs for transplantation or autoimmune diseases (3–6). Despite vaccination, people with compromised immunity have been disproportionately affected by the COVID-19 pandemic, with negative consequences in terms of individual and societal costs (1, 7, 8). In addition to immunocompromised status, these individuals often have advanced age and other comorbidities that increase their risk for poor outcomes (7–11).
Data are lacking to guide optimal prevention and treatment strategies for COVID-19 in immunocompromised individuals. Furthermore, there is a need for individualized therapy because of the broad range of immune response abnormalities and their underlying illnesses. This paper reviews the impact of COVID-19 on people with immunocompromised status, identifies the gaps in the current management landscape, and proposes actions to address this unmet need.
Impact of COVID-19 on people with immunocompromised status
Since its start, the COVID-19 pandemic has caused more than 7 million deaths worldwide (12). Despite the availability of effective vaccines and treatments, SARS-CoV-2 continues to take a toll. In the United States alone, the Centers for Disease Control and Prevention (CDC) estimated that COVID-19 led to approximately 911,000 new hospital admissions and 74,000 attributable deaths in 2023 (13).
Clinical impact: increased disease severity and mortality
Three large observational studies attempted to quantify the increased risk and severity of COVID-19 in immunocompromised populations, COVID-19–Associated Hospitalization Surveillance Network (COVID-NET), Emerging Populations and Outcomes associated with COVID-19-Health Conditions (EPOCH-US), and INFORM (1, 7, 8).
COVID-NET monitored cases from March 1, 2020, through February 28, 2022, and included a representative sample of 22,345 adults aged ≥18 years in the United States hospitalized with COVID-19. Data from this population-based active surveillance suggested that immunocompromised people were overrepresented, accounting for 12% of adult hospitalizations (8). Furthermore, among vaccinated adults, those with immunocompromised status had higher risks of intensive care unit (ICU) admission (adjusted odds ratio 1.40) and in-hospital death (1.87) compared with those with competent immune function.
The EPOCH-US study included a cohort of over 16 million people registered in the Healthcare Integrated Research Database from April 1, 2018, through March 31, 2022. Of this cohort, 3% (n=458,049) were identified as immunocompromised (1). Overall, 13.5% (n=61,865) of this immunocompromised cohort developed COVID-19, with the largest prevalence among people with end-stage renal disease (20%), followed by primary immunodeficiency, hematopoietic stem cell transplant and solid organ transplant recipients (all 16%), immunosuppressive treatment (14%), and hematologic or solid tumor malignancy (9%). Of the immunocompromised cohort who developed COVID-19, 23.5% had hospitalizations associated with their first COVID-19 diagnosis, with a mean length of stay of 15.4 days. The mean cost for these events was estimated at nearly $1 billion US dollars in 2021, with a mean cost of $64,029 per patient.
INFORM, an ongoing retrospective cohort and electronic health data study in England (7), compared COVID-19–related outcomes (hospitalization, ICU admission, and death) among different groups of immunocompromised individuals and the general population. The study population comprised a random sample of 25% of all individuals aged ≥12 years (almost 12 million) registered in the National Health Service databases on January 1, 2022. Of this sample, 4% (470,910) were immunocompromised. Initial results from January 1 through December 31, 2022, showed that immunocompromised individuals disproportionately accounted for approximately 25% of COVID-related outcomes, including 22% (4585/20,910) of COVID-19 hospitalizations, 28% (125/440) of ICU admissions, and 24% (1145/4810) of deaths. Among the highly vaccinated population (≥3 doses of a COVID-19 vaccine), immunocompromised individuals accounted for approximately 25% of COVID-19 hospitalizations, 36% of ICU admissions, and 25% of deaths. In this group, the risks of COVID-19 hospitalization and ICU admission (incidence rate ratios adjusted for age, sex, and number of comorbidities) were 2.17 (95% CI 2.09–2.26) and 4.66 (95% CI 3.56–6.11), respectively. Individuals with the highest risk for COVID-19 hospitalization were solid organ and hematopoietic stem cell transplant recipients and those undergoing treatment for hematological malignancy.
Indirect and non-clinical impact
Contracting COVID-19 can have additional negative consequences, including interrupted treatment for cancer or other underlying disease, potential adverse drug-drug interactions (DDIs) with COVID-19 treatments, loss of work productivity, social isolation, and emotional and financial burden (Figure 1). Furthermore, the initial infection could lead to a syndrome called “long COVID” with negative consequences in a person’s quality of life (14).
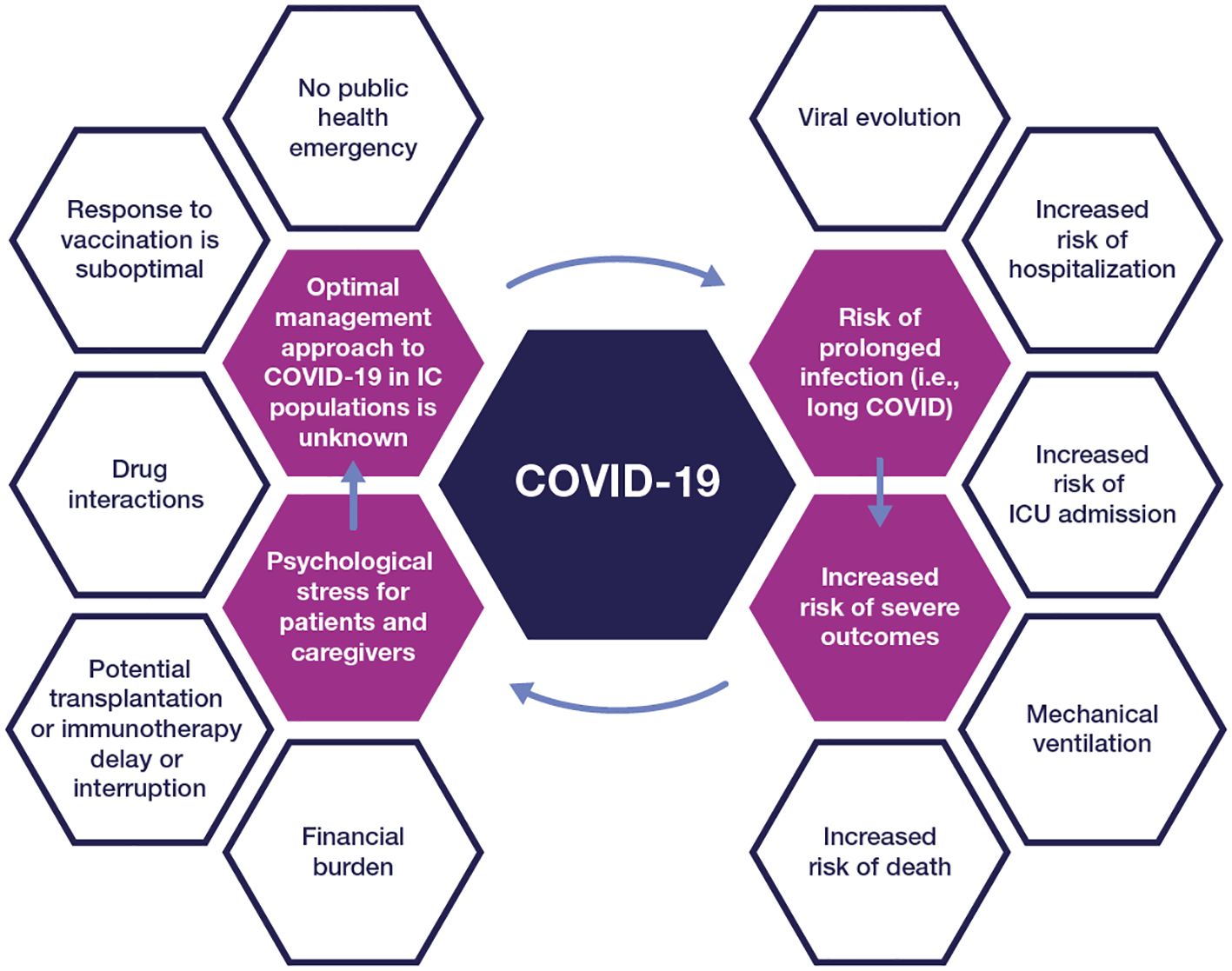
Figure 1 The impact of COVID-19 on people with compromised immunity IC, immunocompromised; ICU, intensive care unit.
The emotional and psychological impact of COVID-19 on immunocompromised people, their families, and caregivers cannot be underestimated. A survey conducted at the beginning of the pandemic revealed high levels of fear/anxiety among 70% of adults with primary immunodeficiencies (15). These individuals were most afraid of exposure from contact with strangers, especially in public places. Qualitative analyses conducted among parents of children with cancer (16) revealed similar negative sentiments associated with COVID-19, while healthcare providers struggled with responsibility around inadvertently transmitting COVID-19 to their immunocompromised patients (17).
Implications of viral persistence and evolution
Immunocompromised individuals with COVID-19 may suffer from persistent infection with prolonged viral shedding (18), and even viral rebound following treatment with antivirals (19), which carries significant public health implications. In a comprehensive review, DeWolf and colleagues (4) summarized the current understanding of how altered host immunity in individuals with cancer and other immunocompromising conditions impacts the prevention, clinical course, and long-term sequelae of SARS-CoV-2 infection. Multiple mechanisms contribute to the increased vulnerability of this population, depending on the type and severity of immunosuppression, including compromised epithelial barrier, impaired innate and adaptive T-cell immune responses, and altered tissue-resident immune cells (4). While antibody responses have been the primary clinical marker to prevent infection, T-cell responses also play a valuable role in controlling infection. Overall, the data suggest that neutralizing antibodies, CD4 T cells, and CD8 T cells work together in controlling COVID-19, and a deficiency in any one of the three immune measures is a risk factor for severe outcomes (20–23).
While immunocompetent individuals typically recover from COVID-19 within 5–7 days, immunocompromised people are at risk of prolonged infection due to slower clearance of the virus. People with hematologic malignancies and transplant recipients may shed viable virus for a median of 4 weeks (24–26). Moreover, SARS-CoV-2 may persist for an even longer period of time with proven infectivity (>8 months) (27), or progress into a chronic infection (long COVID-19) (28), increasing the opportunity for the emergence of mutant variants (29–31). A detailed analysis of an immunocompromised cohort with COVID-19 (18) showed that SARS-CoV-2 clearance and evolution varied by type and severity of immunosuppressive condition; suppression of both B- and T-cell responses resulted in the highest risk of persistent infection. Expectedly, individuals with hematological malignancies or hematopoietic stem cell transplantation were at increased risk of delayed viral clearance. Next-generation sequencing of available viral samples revealed that 39% of participants in the immunocompromised group had nucleotide changes in the spike protein vs. 12% of participants in the non-immunocompromised group, highlighting their potential as a source for mutant variants.
Indeed, the continuous rapid evolution of SARS-CoV-2 has had a substantial impact on the prevention and treatment of COVID-19. It has been challenging to predict how the virus may mutate, not only because of its unique biological signature as an RNA virus, but also because of its interactions with human and animal hosts (32, 33). Emergent variants have had different transmission patterns, virulence, and incubation periods (32, 34). Infections caused by Omicron variants are known to lead to less severe disease (35), but the short incubation period compared with previous variants (Omicron variant: 3.42 days [95% CI 2.88–3.96] vs. Alpha variant: 5.0 days [95% CI 4.94–5.06]) (36) could have contributed to faster and more widespread transmission. The JN.1 variant became dominant across the globe, with only one additional receptor-binding domain (RBD) mutation compared to its predecessor, BA.2.86 (37). Moreover, mutations at the N-terminal and RBD of the spike protein have led to a reduction or loss of the neutralizing activity of monoclonal antibodies (mAbs) against Omicron and its subvariants (38).
The BA.2.87.1 variant is now being closely tracked because it has over 30 changes in the spike protein of the virus when compared to XBB.1.5, the variant that the 2023–2024 vaccine was designed to protect against (39). The potential public health impact of these variants on the durability of vaccine and therapeutic coverage make it essential to address most, if not all, of the reservoirs of virus mutagenesis.
Gaps in the current management landscape for immunocompromised people
Vaccination response is suboptimal
Immunocompromised people respond to COVID-19 vaccination to different degrees but generally have a suboptimal response to vaccination and, consequently, less protection against severe outcomes (40). This is due to multiple factors, including reduced T-cell–specific or humoral-specific responses, which leads to low seroconversion rates post-vaccination, generation of antibodies with low neutralization activity against SARS-CoV-2 (41–43), and a short duration of protection. Studies have shown that although alterations in the B-cell compartment correlate with decreased humoral responses to COVID-19 vaccines, some degree of T-cell–mediated protection from severe disease might be conserved (23, 44, 45). A meta-analysis of 82 studies (46) showed that after one vaccine dose, people with hematological cancers, immune-mediated inflammatory disorders, and solid cancers were half less likely to seroconvert, while transplant recipients were 16 times less likely to seroconvert (risk ratio 0.06 [95% CI 0.04–0.09]) compared with immunocompetent controls. After a second dose, seroconversion remained least likely in transplant recipients. Parker et al. (47) identified 23 studies (2 clinical trials and 21 observational studies) reporting on vaccination outcomes for 1722 people with immunocompromised status. For participants with responses after the standard primary series, the median antibody response rate increased modestly from 41% (IQR 23–58) to 67% (55–69), while for low or non-responders, the median antibody response rate increased to 44% (32–55).
Waning of vaccine-induced protection is a major concern. Vaccine effectiveness decreases among individuals with comorbidities and those aged ≥55 years (48); this begins as early as the first month after full vaccination (defined as one or two vaccine doses, depending on vaccine type) (49). In immunocompromised individuals, the impact of waning is even more evident. During the Omicron period, vaccine effectiveness (defined as protection against COVID-19 requiring hospital admission following three doses of mRNA vaccines) in immunocompromised people waned to 48% (40–55%) by months four to five (50). By comparison, effectiveness in immunocompetent individuals decreased to 71% (68–74%). For the 2023–2024 (monovalent XBB.1.5) COVID-19 vaccine, effectiveness among adults aged ≥18 years with immunocompromising conditions was 38% in the 7–59 days after receipt of an updated vaccine dose and 34% in the 60–119 days after receipt of an updated dose (51).
The public health emergency has ended
In May 2023, COVID-19 stopped being regarded as a global health emergency (52). The non-pharmacological interventions that had a positive impact on the course of the disease, such as free at-home rapid diagnostic tools, social distancing, and wearing masks, became less frequently implemented (53).
Additionally, uptake of the recommended vaccine boosters has decreased. As of March 31, 2024, only 23% (95% CI 22.1–23.1%) of adults in the United States reported having received an updated 2023–2024 COVID-19 vaccine (54). In particular, data from the VISION Network showed that only 18% of adults with immunocompromising conditions had received the updated COVID-19 vaccine (51).
Prevention with mAbs has not always been available in the United States
A preventive strategy briefly available during the pandemic was the use of mAbs for pre- and post-exposure prophylaxis. This approach of passive transfer of immunity offered a more immediate and reliable level of neutralizing titers (55), especially for people who are unable to develop their own endogenous antibodies from vaccination or natural infection. In December 2021, the US Food and Drug Administration (FDA) granted emergency use authorization for tixagevimab-cilgavimab as pre-exposure prophylaxis in adults and children (≥12 years, weighing ≥40 kg) (56, 57). Real-world evidence studies (58–60) demonstrated the benefits of mAb prophylaxis in people with immunocompromised status. However, in January 2023, the emergency use authorization was retracted in the United States because of loss of neutralization activity against the Omicron XBB and subsequent variants (61, 62). Following that, until recently, there were no authorized mAbs for pre-exposure or post-exposure prophylaxis of COVID-19. On March 22, 2024, the US FDA authorized the emergency use of pemivibart (formerly VYD222) for pre-exposure prophylaxis among adolescents and adults with moderate-to-severe immune compromised status. The emergency use authorization was based on the totality of scientific evidence available, including demonstration of in vitro neutralizing activity against major SARS-CoV-2 variants, such as JN.1 (63), and immunobridging data from the ongoing CANOPY clinical trial (NCT06039449). Anaphylaxis was seen in four trial participants.
Treatment with mAbs is not currently available in the United States
Therapeutic mAbs (e.g., bamlanivimab, bamlanivimab-etesevimab, casirivimab-imdevimab, sotrovimab, and bebtelovimab) are no longer authorized for the treatment of COVID-19 because of the loss of neutralizing activity against Omicron subvariants (64–66). This has limited the treatment options for some immunocompromised people who have impaired ability to mount an immune response to natural infection, especially those with CD20 depletion (67). Currently, there are limited data on potential future mAb therapies and their capacity to reduce the risk of disease progression in people with immunocompromised status.
Box 1. Call to action: proposed strategies and considerations to improve COVID-19 outcomes for people with compromised immunity.
Current treatment regimens have limitations
Antiviral drugs available to treat high-risk individuals include remdesivir, nirmatrelvir/ritonavir, and molnupiravir (68). However, clinicians need to consider many factors when prescribing one of the three options. For example, nirmatrelvir/ritonavir and molnupiravir treatment must be initiated early (<5 days after diagnosis) (69, 70). Additionally, the use of ritonavir-boosted nirmatrelvir is associated with myriad of potential DDIs (71). Interestingly, there is reluctance among physicians in prescribing this antiviral combination drug for the immunocompromised population (72). Barriers to antiviral use among eligible patients with COVID-19 also include access, logistics, and lack of perceived effectiveness. Remdesivir is administered intravenously over a three-day treatment course (73), which could pose logistical challenges to the care team, while molnupiravir may not be as effective in reducing COVID-19 hospitalizations in patients at high risk of severe outcomes (74).
Another issue with antivirals for treatment of COVID-19 is the phenomenon of virologic rebound, characterized by recurrence of symptoms and reversion to SARS-CoV-2 test positivity after initial recovery with treatment. Estimates of viral rebound have varied; however, in a recent multicenter observational study of 127 participants (19), virologic rebound with shedding of replication-competent virus occurred in approximately 21% of people taking a 5-day course of nirmatrelvir-ritonavir and 2% of those who were not using therapy.
The use of convalescent plasma with high titers of anti–SARS-CoV-2 antibodies appeared to be a safe and effective way to treat severe COVID-19 at the onset of the pandemic. Although meta-analysis studies concluded that convalescent plasma was not associated with lower all-cause mortality or improved disease progression (75, 76), some National Institutes of Health panel members recommend the use of high-titer COVID-19 convalescent plasma, with or without antiviral therapy, for immunocompromised patients with prolonged, symptomatic disease (77). However, this treatment option is not widely available because of limited supply.
Call to action: immunocompromised people need optimized prevention and treatment strategies
The limited options available to immunocompromised people for the prevention and treatment of COVID-19 represent a gap in the public health and therapeutic landscape. Large randomized clinical trials that led to the authorization and use of existing therapeutic agents often excluded immunocompromised participants, hence their benefits have not been rigorously tested in this group of vulnerable people (78). A definitive approach for the management of COVID-19 in people with immunocompromising conditions is missing. Given the lack of clear evidence-based studies, the current clinical approach has been extrapolated from larger trials focusing on non-immunocompromised individuals and case studies. A series of potential strategies that could improve outcomes for immunocompromised people are proposed (Box 1).
Optimized vaccination strategies
Vaccination remains the cornerstone of prevention; however, since vaccination response is dependent on the individual’s immune system, an optimized strategy is needed for the immunosuppressed population. Depending on the COVID-19 vaccination history, different vaccination plans are subsequently recommended. People with immunocompromised status usually receive additional COVID-19 doses to boost their immune response at two or more months after the last recommended COVID-19 vaccine. The CDC developed specific guidance on COVID-19 vaccines for people who are moderately or severely immunocompromised (79), which are further endorsed by the National Institutes of Health (68). Improving the durability of vaccine response should also be prioritized. Considering that administration of the updated 2023–2024 COVID-19 vaccine resulted in only modest effectiveness (80), more research is needed in terms of dosing and frequency. Since there are different levels of immune compromise status and vaccine responses, it is suggested that dosing and frequency be individualized, as guided by underlying immune status and vaccine response.
mAbs that complement suboptimal vaccine responses
There is an urgent need for the development of mAbs as prophylaxis for COVID-19 in people with immunocompromising conditions. This strategy is intended to provide passive immunity that complements the suboptimal vaccine response. Several mAbs are being studied in response to the emerging variants; two such long-acting mAbs (81, 82) are being evaluated for pre-exposure prophylaxis of COVID-19 in immunocompromised participants. Novel mAbs should be broad spectrum with a prolonged half-life and potential to treat emerging variants. New technologies that could predict emerging variants should be sought to guide the development of mAbs with a durable therapeutic lifespan.
Improved antiviral treatment
Oral drugs are a preferred convenient approach to treatment, but they should be highly effective and without DDI potential. In addition, further consideration of the dose, timing, and duration of treatment with antiviral drugs is needed to inform optimal use and reduce the potential for viral rebound and infectivity. Various combination antiviral-antiviral drug or antiviral-antibody treatments have been explored. Small studies in B-cell–depleted patients with persistent SARS-CoV-2 infection showed better outcomes with combination therapy than monotherapy (83, 84). Mikulska et al. (85) investigated the combination therapy of remdesivir plus nirmatrelvir/ritonavir, or molnupiravir in case of renal failure, with mAbs in 22 immunocompromised patients with prolonged/relapsed COVID-19. The group observed significant increases in the rate of virological response when the combination treatment included mAbs, both at early stage (P=0.032) and after 30 days of treatment (P=0.046). However, controlled clinical trials are needed to advance these observations beyond case series and provide solid evidence for optimized antiviral treatment strategies.
Targeted population-based health emergency centered around immunocompromised people
Healthcare systems, policymakers, and regulators need to work together and develop population-based health measures that focus on people with compromised immunity. Harm-reduction measures to prevent the spread of SARS-CoV-2 to the highest-risk individuals, such as masking and adherence to the CDC isolation guidelines, are known to be effective. A declaration of health emergency that is centered around immunosuppressed people will allow for more funding to develop new vaccines, diagnostics, and therapeutics for this vulnerable population.
Conclusions
After more than four years since the start of the COVID-19 pandemic, SARS-CoV-2 has moved into an “endemic phase,” with periodic surges of cases throughout the year. Most people have either been infected or vaccinated, thereby having some level of protective immunity from severe disease. However, immune protection wanes over time, and this leads to a rise of cases and exposes vulnerable and immunosuppressed people to infection. This perspective provided a review of the gaps in the management of COVID-19 among immunosuppressed individuals. A call to optimize prevention efforts through vaccination and passive immunity transfer is highlighted. Likewise, improvement in treatment strategies, such as development of novel, safer drugs, and the exploration of combination therapies, is needed. Such efforts to optimize the care of immunosuppressed individuals should be supported by public agencies in partnership with the healthcare industry and institutions.
Data availability statement
The original contributions presented in the study are included in the article. Further inquiries can be directed to the corresponding author.
Author contributions
RR: Conceptualization, Investigation, Writing – original draft, Writing – review & editing.
Funding
The author declares no financial support was received for research, authorship, and or publication of this article. The article processing fee was paid by Invivyd, Inc.
Acknowledgments
Editorial assistance was provided by Georgiana Manica, PhD, and Jean Turner of Parexel, and was funded by Invivyd, Inc. Pamela Hawn, PharmD, of Invivyd Inc, contributed to the conception of and editorial review of the paper.
Conflict of interest
RR has received research grant funds to the institution from Gilead, Roche, and Regeneron (all projects completed), fees as member of a DSMB for Novartis (services completed) and Endpoint Adjudication Committee for Allovir (services completed), and consultancy fees from Invivyd, Inc for previously completed services.
Publisher’s note
All claims expressed in this article are solely those of the authors and do not necessarily represent those of their affiliated organizations, or those of the publisher, the editors and the reviewers. Any product that may be evaluated in this article, or claim that may be made by its manufacturer, is not guaranteed or endorsed by the publisher.
References
1. Ketkar A, Willey V, Pollack M, Glasser L, Dobie C, Wenziger C, et al. Assessing the risk and costs of COVID-19 in immunocompromised populations in a large United States commercial insurance health plan: the EPOCH-US Study. Curr Med Res Opin. (2023) 39:1103–18. doi: 10.1080/03007995.2023.2233819
2. Martinson ML, Lapham J. Prevalence of immunosuppression among US adults. JAMA. (2024) 331:880–2. doi: 10.1001/jama.2023.28019
3. Belsky JA, Tullius BP, Lamb MG, Sayegh R, Stanek JR, Auletta JJ. COVID-19 in immunocompromised patients: a systematic review of cancer, hematopoietic cell and solid organ transplant patients. J Infect. (2021) 82:329–38. doi: 10.1016/j.jinf.2021.01.022
4. DeWolf S, Laracy JC, Perales MA, Kamboj M, van den Brink MRM, Vardhana S. SARS-CoV-2 in immunocompromised individuals. Immunity. (2022) 55:1779–98. doi: 10.1016/j.immuni.2022.09.006
5. Tangye SG, Covid Human Genetic Effort consortium. Impact of SARS-CoV-2 infection and COVID-19 on patients with inborn errors of immunity. J Allergy Clin Immunol. (2023) 151:818–31. doi: 10.1016/j.jaci.2022.11.010
6. Wallace BI, Kenney B, Malani PN, Clauw DJ, Nallamothu BK, Waljee AK. Prevalence of immunosuppressive drug use among commercially insured US adults, 2018-2019. JAMA Netw Open. (2021) 4:e214920. doi: 10.1001/jamanetworkopen.2021.4920
7. Evans RA, Dube S, Lu Y, Yates M, Arnetorp S, Barnes E, et al. Impact of COVID-19 on immunocompromised populations during the Omicron era: insights from the observational population-based INFORM study. Lancet Reg Health Eur. (2023) 35:100747. doi: 10.1016/j.lanepe.2023.100747
8. Singson JRC, Kirley PD, Pham H, Rothrock G, Armistead I, Meek J, et al. Factors associated with severe outcomes among immunocompromised adults hospitalized for COVID-19 - COVID-NET, 10 States, March 2020-February 2022. MMWR Morb Mortal Wkly Rep. (2022) 71:878–84. doi: 10.15585/mmwr.mm7127a3
9. Grifoni A, Alonzi T, Alter G, Noonan DM, Landay AL, Albini A, et al. Impact of aging on immunity in the context of COVID-19, HIV, and tuberculosis. Front Immunol. (2023) 14:1146704. doi: 10.3389/fimmu.2023.1146704
10. Kim L, Garg S, O'Halloran A, Whitaker M, Pham H, Anderson EJ, et al. Risk factors for intensive care unit admission and in-hospital mortality among hospitalized adults identified through the US Coronavirus Disease 2019 (COVID-19)-Associated Hospitalization Surveillance Network (COVID-NET). Clin Infect Dis. (2021) 72:e206–e14. doi: 10.1093/cid/ciaa1012
11. Rydyznski Moderbacher C, Ramirez SI, Dan JM, Grifoni A, Hastie KM, Weiskopf D, et al. Antigen-specific adaptive immunity to SARS-coV-2 in acute COVID-19 and associations with age and disease severity. Cell. (2020) 183:996–1012 e19. doi: 10.1016/j.cell.2020.09.038
12. World Health Organization. WHO coronavirus (COVID-19) dashboard (2023). Available online at: https://covid19.who.int/ (Accessed April 1, 2024).
13. Centers for Disease Control and Prevention. COVID data tracker (2023). Available online at: https://covid.cdc.gov/covid-data-tracker/#datatracker-home (Accessed April 1, 2024).
14. Davis HE, McCorkell L, Vogel JM, Topol EJ. Long COVID: major findings, mechanisms and recommendations. Nat Rev Microbiol. (2023) 21:133–46. doi: 10.1038/s41579-022-00846-2
15. Napiórkowska-Baran K, Rosada T, Więsik-Szewczyk E, Ziętkiewicz M, Matyja-Bednarczyk A, Baranowska K, et al. A multicenter survey on the aspects of everyday life in adult patients with primary antibody deficiencies treated with immunoglobulin G replacement during the COVID-19 pandemic. Int J Immunopathol Pharmacol. (2021) 35:20587384211044344. doi: 10.1177/20587384211044344
16. Sutherland-Foggio MS, Stanek CJ, Buff K, Nahata L, Foster-Akard T, Gerhardt CA, et al. The experiences of families of children with cancer during the COVID-19 pandemic: a qualitative exploration. Palliat Support Care. (2023) 22:1–8. doi: 10.1017/s1478951523001098
17. Marks IR, O'Neill J, Gillam L, McCarthy MC. Ethical challenges faced by healthcare workers in pediatric oncology care during the COVID-19 pandemic in Australia. Pediatr Blood Cancer. (2023) 70:e30114. doi: 10.1002/pbc.30114
18. Li Y, Choudhary MC, Regan J, Boucau J, Nathan A, Speidel T, et al. SARS-CoV-2 viral clearance and evolution varies by type and severity of immunodeficiency. Sci Transl Med. (2024) 16:eadk1599. doi: 10.1126/scitranslmed.adk1599
19. Edelstein GE, Boucau J, Uddin R, Marino C, Liew MY, Barry M, et al. SARS-CoV-2 virologic rebound with nirmatrelvir-ritonavir therapy: an observational study. Ann Intern Med. (2023) 176:1577–85. doi: 10.7326/m23-1756
20. Petrone L, Sette A, de Vries RD, Goletti D. The importance of measuring SARS-CoV-2-specific T-cell responses in an ongoing pandemic. Pathogens. (2023) 12:862. doi: 10.3390/pathogens12070862
21. Picchianti-Diamanti A, Navarra A, Aiello A, Lagana B, Cuzzi G, Salmi A, et al. Older age, a high titre of neutralising antibodies and therapy with conventional DMARDs are associated with protection from breakthrough infection in rheumatoid arthritis patients after the booster dose of anti-SARS-CoV-2 vaccine. Vaccines (Basel). (2023) 11:1684. doi: 10.3390/vaccines11111684
22. Sette A, Sidney J, Crotty S. T cell responses to SARS-CoV-2. Annu Rev Immunol. (2023) 41:343–73. doi: 10.1146/annurev-immunol-101721-061120
23. Tarke A, Coelho CH, Zhang Z, Dan JM, Yu ED, Methot N, et al. SARS-CoV-2 vaccination induces immunological T cell memory able to cross-recognize variants from Alpha to Omicron. Cell. (2022) 185:847–59 e11. doi: 10.1016/j.cell.2022.01.015
24. Boucau J, Marino C, Regan J, Uddin R, Choudhary MC, Flynn JP, et al. Duration of shedding of culturable virus in SARS-CoV-2 Omicron (BA.1) infection. N Engl J Med. (2022) 387:275–7. doi: 10.1056/NEJMc2202092
25. Kang SW, Kim JW, Kim JY, Lim SY, Jang CY, Chang E, et al. Characteristics and risk factors of prolonged viable virus shedding in immunocompromised patients with COVID-19: a prospective cohort study. J Infect. (2023) 86:412–4. doi: 10.1016/j.jinf.2023.01.024
26. Kang SW, Kim JW, Kim JY, Lim SY, Jang CY, Chang E, et al. Virological characteristics and the rapid antigen test as deisolation criteria in immunocompromised patients with COVID-19: A prospective cohort study. J Med Virol. (2023) 95:e29228. doi: 10.1002/jmv.29228
27. Sepulcri C, Dentone C, Mikulska M, Bruzzone B, Lai A, Fenoglio D, et al. The longest persistence of viable SARS-CoV-2 with recurrence of viremia and relapsing symptomatic COVID-19 in an immunocompromised patient-a case study. Open Forum Infect Dis. (2021) 8:ofab217. doi: 10.1093/ofid/ofab217
28. Dioverti V, Salto-Alejandre S, Haidar G. Immunocompromised patients with protracted COVID-19: a review of "long persisters". Curr Transplant Rep. (2022) 9:209–18. doi: 10.1007/s40472-022-00385-y
29. Avanzato VA, Matson MJ, Seifert SN, Pryce R, Williamson BN, Anzick SL, et al. Case study: prolonged infectious SARS-CoV-2 shedding from an asymptomatic immunocompromised individual with cancer. Cell. (2020) 183:1901–12.e9. doi: 10.1016/j.cell.2020.10.049
30. Choi B, Choudhary MC, Regan J, Sparks JA, Padera RF, Qiu X, et al. Persistence and evolution of SARS-CoV-2 in an immunocompromised host. N Engl J Med. (2020) 383:2291–3. doi: 10.1056/NEJMc2031364
31. Corey L, Beyrer C, Cohen MS, Michael NL, Bedford T, Rolland M. SARS-CoV-2 variants in patients with immunosuppression. N Engl J Med. (2021) 385:562–6. doi: 10.1056/NEJMsb2104756
32. Markov PV, Ghafari M, Beer M, Lythgoe K, Simmonds P, Stilianakis NI, et al. The evolution of SARS-coV-2. Nat Rev Microbiol. (2023) 21:361–79. doi: 10.1038/s41579-023-00878-2
33. Si Y, Wu W, Xue X, Sun X, Qin Y, Li Y, et al. The evolution of SARS-CoV-2 and the COVID-19 pandemic. PeerJ. (2023) 11:e15990. doi: 10.7717/peerj.15990
34. Carabelli AM, Peacock TP, Thorne LG, Harvey WT, Hughes J, Peacock SJ, et al. SARS-CoV-2 variant biology: immune escape, transmission and fitness. Nat Rev Microbiol. (2023) 21:162–77. doi: 10.1038/s41579-022-00841-7
35. Wrenn JO, Pakala SB, Vestal G, Shilts MH, Brown HM, Bowen SM, et al. COVID-19 severity from Omicron and Delta SARS-CoV-2 variants. Influenza Other Respir Viruses. (2022) 16:832–6. doi: 10.1111/irv.12982
36. Wu Y, Kang L, Guo Z, Liu J, Liu M, Liang W. Incubation period of COVID-19 caused by unique SARS-CoV-2 strains: a systematic review and meta-analysis. JAMA Netw Open. (2022) 5:e2228008. doi: 10.1001/jamanetworkopen.2022.28008
37. Yang S, Yu Y, Xu Y, Jian F, Song W, Yisimayi A, et al. Fast evolution of SARS-CoV-2 BA.2.86 to JN.1 under heavy immune pressure. Lancet Infect Dis. (2024) 24:e70–e2. doi: 10.1016/s1473-3099(23)00744-2
38. Pather S, Madhi SA, Cowling BJ, Moss P, Kamil JP, Ciesek S, et al. SARS-CoV-2 Omicron variants: burden of disease, impact on vaccine effectiveness and need for variant-adapted vaccines. Front Immunol. (2023) 14:1130539. doi: 10.3389/fimmu.2023.1130539
39. Centers for Disease Control and Prevention. CDC tracks new SARS-CoV-2 variant, BA.2.87.1 (2024). Available online at: https://www.cdc.gov/respiratory-viruses/whats-new/covid-19-variant-update-2024-02-09.html (Accessed April 1, 2024).
40. Link-Gelles R, Weber ZA, Reese SE, Payne AB, Gaglani M, Adams K, et al. Estimates of bivalent mRNA vaccine durability in preventing COVID-19-associated hospitalization and critical illness among adults with and without immunocompromising conditions - VISION Network, September 2022-April 2023. MMWR Morb Mortal Wkly Rep. (2023) 72:579–88. doi: 10.15585/mmwr.mm7221a3
41. Agha ME, Blake M, Chilleo C, Wells A, Haidar G. Suboptimal response to coronavirus disease 2019 messenger RNA vaccines in patients with hematologic malignancies: a need for vigilance in the postmasking era. Open Forum Infect Dis. (2021) 8:ofab353. doi: 10.1093/ofid/ofab353
42. Cheung MW, Dayam RM, Shapiro JR, Law JC, Chao GYC, Pereira D, et al. Third and fourth vaccine doses broaden and prolong immunity to SARS-CoV-2 in adult patients with immune-mediated inflammatory diseases. J Immunol. (2023) 211:351–64. doi: 10.4049/jimmunol.2300190
43. Tartof SY, Slezak JM, Fischer H, Hong V, Ackerson BK, Ranasinghe ON, et al. Effectiveness of mRNA BNT162b2 COVID-19 vaccine up to 6 months in a large integrated health system in the USA: a retrospective cohort study. Lancet. (2021) 398:1407–16. doi: 10.1016/s0140-6736(21)02183-8
44. Ruggieri S, Aiello A, Tortorella C, Navarra A, Vanini V, Meschi S, et al. Dynamic evolution of humoral and T-cell specific immune response to COVID-19 mRNA vaccine in patients with multiple sclerosis followed until the booster dose. Int J Mol Sci. (2023) 24:8525. doi: 10.3390/ijms24108525
45. Tortorella C, Aiello A, Gasperini C, Agrati C, Castilletti C, Ruggieri S, et al. Humoral- and T-cell-specific immune responses to SARS-CoV-2 mRNA vaccination in patients with MS using different disease-modifying therapies. Neurology. (2022) 98:e541–e54. doi: 10.1212/WNL.0000000000013108
46. Lee A, Wong SY, Chai LYA, Lee SC, Lee MX, Muthiah MD, et al. Efficacy of covid-19 vaccines in immunocompromised patients: systematic review and meta-analysis. BMJ. (2022) 376:e068632. doi: 10.1136/bmj-2021-068632
47. Parker EPK, Desai S, Marti M, Nohynek H, Kaslow DC, Kochhar S, et al. Response to additional COVID-19 vaccine doses in people who are immunocompromised: a rapid review. Lancet Glob Health. (2022) 10:e326–e8. doi: 10.1016/s2214-109x(21)00593-3
48. Menni C, May A, Polidori L, Louca P, Wolf J, Capdevila J, et al. COVID-19 vaccine waning and effectiveness and side-effects of boosters: a prospective community study from the ZOE COVID Study. Lancet Infect Dis. (2022) 22:1002–10. doi: 10.1016/s1473-3099(22)00146-3
49. Addo IY, Dadzie FA, Okeke SR, Boadi C, Boadu EF. Duration of immunity following full vaccination against SARS-CoV-2: a systematic review. Arch Public Health. (2022) 80:200. doi: 10.1186/s13690-022-00935-x
50. Ferdinands JM, Rao S, Dixon BE, Mitchell PK, DeSilva MB, Irving SA, et al. Waning of vaccine effectiveness against moderate and severe covid-19 among adults in the US from the VISION network: test negative, case-control study. BMJ. (2022) 379:e072141. doi: 10.1136/bmj-2022-072141
51. Link-Gelles R, Rowley EAK, DeSilva MB, Dascomb K, Irving SA, Klein NP, et al. Interim effectiveness of updated 2023-2024 (monovalent XBB.1.5) COVID-19 vaccines against COVID-19-associated hospitalization among adults aged ≥ 18 years with immunocompromising conditions - VISION Network, September 2023-February 2024. MMWR Morb Mortal Wkly Rep. (2024) 73:271–6. doi: 10.15585/mmwr.mm7312a5
52. World Health Organization. WHO Director-General's opening remarks at the media briefing – 5 May 2023 (2023). Available online at: https://www.who.int/news-room/speeches/item/who-director-general-s-opening-remarks-at-the-media-briefing---5-may-2023 (Accessed April 1, 2024).
53. Yetmar ZA, Razonable RR. No patient left behind: a multilayered approach to mitigate COVID-19 in transplant recipients. Transpl Infect Dis. (2023) 25:e13956. doi: 10.1111/tid.13956
54. Centers for Disease Control and Prevention. Weekly COVID-19 vaccination dashboard (2024). Available online at: https://www.cdc.gov/vaccines/imz-managers/coverage/covidvaxview/interactive/vaccination-dashboard.html (Accessed April 1, 2024).
55. Corti D, Purcell LA, Snell G, Veesler D. Tackling COVID-19 with neutralizing monoclonal antibodies. Cell. (2021) 184:3086–108. doi: 10.1016/j.cell.2021.05.005
56. US Food and Drug Administration. Coronavirus (COVID-19) update: FDA authorizes new long-acting monoclonal antibodies for pre-exposure prevention of COVID-19 in certain individuals (2021). Available online at: https://www.fda.gov/news-events/press-announcements/coronavirus-covid-19-update-fda-authorizes-new-long-acting-monoclonal-antibodies-pre-exposure (Accessed April 1, 2024).
57. Levin MJ, Ustianowski A, De Wit S, Launay O, Avila M, Templeton A, et al. Intramuscular AZD7442 (tixagevimab-cilgavimab) for prevention of covid-19. N Engl J Med. (2022) 386:2188–200. doi: 10.1056/NEJMoa2116620
58. Al Jurdi A, Morena L, Cote M, Bethea E, Azzi J, Riella LV. Tixagevimab/cilgavimab pre-exposure prophylaxis is associated with lower breakthrough infection risk in vaccinated solid organ transplant recipients during the omicron wave. Am J Transplant. (2022) 22:3130–6. doi: 10.1111/ajt.17128
59. Jondreville L, D'Aveni M, Labussière-Wallet H, Le Bourgeois A, Villate A, Berceanu A, et al. Pre-exposure prophylaxis with tixagevimab/cilgavimab (AZD7442) prevents severe SARS-CoV-2 infection in recipients of allogeneic hematopoietic stem cell transplantation during the Omicron wave: a multicentric retrospective study of SFGM-TC. J Hematol Oncol. (2022) 15:169. doi: 10.1186/s13045-022-01387-0
60. Nguyen Y, Flahault A, Chavarot N, Melenotte C, Cheminant M, Deschamps P, et al. Pre-exposure prophylaxis with tixagevimab and cilgavimab (Evusheld) for COVID-19 among 1112 severely immunocompromised patients. Clin Microbiol Infect. (2022) 28:1654.e1–.e4. doi: 10.1016/j.cmi.2022.07.015
61. US Food and Drug Administration. FDA announces Evusheld is not currently authorized for emergency use in the U.S (2023). Available online at: https://www.fda.gov/drugs/drug-safety-and-availability/fda-announces-evusheld-not-currently-authorized-emergency-use-us (Accessed April 1, 2024).
62. Imai M, Ito M, Kiso M, Yamayoshi S, Uraki R, Fukushi S, et al. Efficacy of antiviral agents against Omicron subvariants BQ.1.1 and XBB. N Engl J Med. (2023) 388:89–91. doi: 10.1056/NEJMc2214302
63. Invivyd. Fact sheet for healthcare providers: emergency use authorization of Pemgarda (Pemivibart) (2024). Available online at: https://invivyd.com/wp-content/uploads/2024/03/EUA-122-PEMGARDA-Healthcare-Providers-Fact-Sheet-FINAL-v1.0-22Mar2024.pdf (Accessed April 1, 2024).
64. US Food and Drug Administration. FDA announces bebtelovimab is not currently authorized in any US region (2022). Available online at: https://www.fda.gov/drugs/drug-safety-and-availability/fda-announces-bebtelovimab-not-currently-authorized-any-us-region (Accessed April 1, 2024).
65. US Food and Drug Administration. Coronavirus (COVID-19) update: FDA limits use of certain monoclonal antibodies to treat COVID-19 due to the Omicron variant (2022). Available online at: https://content.govdelivery.com/accounts/USFDA/bulletins/3075786 (Accessed April 1, 2024).
66. US Food and Drug Administration. FDA updates sotrovimab emergency use authorization (2022). Available online at: https://www.fda.gov/drugs/drug-safety-and-availability/fda-updates-sotrovimab-emergency-use-authorization (Accessed April 1, 2024).
67. Kasten MJ, Lahr BD, Parisapogu A, Yetmar ZA, O'Horo JC, Orenstein R, et al. COVID-19 outcome is not affected by anti-CD20 or high-titer convalescent plasma in immunosuppressed patients. Sci Rep. (2023) 13:21249. doi: 10.1038/s41598-023-48145-x
68. National Institutes of Health. Special considerations in people who are immunocompromised (2023). Available online at: https://www.covid19treatmentguidelines.nih.gov/special-populations/immunocompromised/ (Accessed April 1, 2024).
69. Johnson MG, Strizki JM, Brown ML, Wan H, Shamsuddin HH, Ramgopal M, et al. Molnupiravir for the treatment of COVID-19 in immunocompromised participants: efficacy, safety, and virology results from the phase 3 randomized, placebo-controlled MOVe-OUT trial. Infection. (2023) 51:1273–84. doi: 10.1007/s15010-022-01959-9
70. Sun F, Lin Y, Wang X, Gao Y, Ye S. Paxlovid in patients who are immunocompromised and hospitalised with SARS-CoV-2 infection. Lancet Infect Dis. (2022) 22:1279. doi: 10.1016/s1473-3099(22)00430-3
71. Fishbane S, Hirsch JS, Nair V. Special considerations for paxlovid treatment among transplant recipients with SARS-CoV-2 infection. Am J Kidney Dis. (2022) 79:480–2. doi: 10.1053/j.ajkd.2022.01.001
72. Lahouati M, Cazanave C, Labadie A, Gohier P, Guirlé L, Desclaux A, et al. Outcomes of targeted treatment in immunocompromised patients with asymptomatic or mild COVID-19: a retrospective study. Sci Rep. (2023) 13:15357. doi: 10.1038/s41598-023-42727-5
73. Infectious Diseases Society of America. IDSA guidelines on the treatment and management of patients with COVID-19 (2023). Available online at: https://www.idsociety.org/COVID19guidelines#Recommendations16-18:Remdesivir (Accessed April 1, 2024).
74. Butler CC, Hobbs FDR, Gbinigie OA, Rahman NM, Hayward G, Richards DB, et al. Molnupiravir plus usual care versus usual care alone as early treatment for adults with COVID-19 at increased risk of adverse outcomes (PANORAMIC): an open-label, platform-adaptive randomised controlled trial. Lancet. (2023) 401:281–93. doi: 10.1016/s0140-6736(22)02597-1
75. Jorda A, Kussmann M, Kolenchery N, Siller-Matula JM, Zeitlinger M, Jilma B, et al. Convalescent plasma treatment in patients with Covid-19: a systematic review and meta-analysis. Front Immunol. (2022) 13:817829. doi: 10.3389/fimmu.2022.817829
76. Mihalek N, Radovanović D, Barak O, Čolović P, Huber M, Erdoes G. Convalescent plasma and all-cause mortality of COVID-19 patients: systematic review and meta-analysis. Sci Rep. (2023) 13:12904. doi: 10.1038/s41598-023-40009-8
77. National Institutes of Health. COVID-19 convalescent plasma (2023). Available online at: https://www.covid19treatmentguidelines.nih.gov/therapies/antivirals-including-antibody-products/covid-19-convalescent-plasma/ (Accessed April 1, 2024).
78. Shoham S, Batista C, Ben Amor Y, Ergonul O, Hassanain M, Hotez P, et al. Vaccines and therapeutics for immunocompromised patients with COVID-19. EClinicalMedicine. (2023) 59:101965. doi: 10.1016/j.eclinm.2023.101965
79. Centers for Disease Control and Prevention. COVID-19 vaccines for people who are moderately or severely immunocompromised (2023). Available online at: https://www.cdc.gov/coronavirus/2019-ncov/vaccines/recommendations/immuno.html (Accessed April 1, 2024).
80. Link-Gelles R, Ciesla AA, Mak J, Miller JD, Silk BJ, Lambrou AS, et al. Early estimates of updated 2023-2024 (monovalent XBB.1.5) COVID-19 vaccine effectiveness against symptomatic SARS-CoV-2 infection attributable to co-circulating Omicron variants among immunocompetent adults - increasing community access to testing program, United States, September 2023-January 2024. MMWR Morb Mortal Wkly Rep. (2024) 73:77–83. doi: 10.15585/mmwr.mm7304a2
81. ClinicalTrials.gov. Study understanding pre-exposure prophylaxis of novel antibodies (SUPERNOVA) sub-study: study understanding pre-exposure prophylaxis of novel antibodies (SUPERNOVA) sub-study (SUPERNOVA) (2023). Available online at: https://classic.clinicaltrials.gov/ct2/show/NCT05648110 (Accessed April 1, 2024).
82. ClinicalTrials.gov. A study to investigate the prevention of COVID-19 with VYD222 in adults with immune compromise and in participants aged 12 years or older who are at risk of exposure to SARS-CoV-2 (2023). Available online at: https://classic.clinicaltrials.gov/ct2/show/NCT06039449 (Accessed April 1, 2024).
83. D'Abramo A, Vita S, Beccacece A, Navarra A, Pisapia R, Fusco FM, et al. B-cell-depleted patients with persistent SARS-CoV-2 infection: combination therapy or monotherapy? A real-world experience. Front Med (Lausanne). (2024) 11:1344267. doi: 10.3389/fmed.2024.1344267
84. Vita S, D'Abramo A, Coppola A, Farroni C, Iori AP, Faraglia F, et al. Combined antiviral therapy as effective and feasible option in allogenic hematopoietic stem cell transplantation during SARS-COV-2 infection: a case report. Front Oncol. (2024) 14:1290614. doi: 10.3389/fonc.2024.1290614
85. Mikulska M, Sepulcri C, Dentone C, Magne F, Balletto E, Baldi F, et al. Triple combination therapy with 2 antivirals and monoclonal antibodies for persistent or relapsed severe acute respiratory syndrome coronavirus 2 infection in immunocompromised patients. Clin Infect Dis. (2023) 77:280–6. doi: 10.1093/cid/ciad181
Keywords: COVID-19, immunocompromised, SARS-CoV-2, prevention, treatment
Citation: Razonable RR (2024) Protecting the vulnerable: addressing the COVID-19 care needs of people with compromised immunity. Front. Immunol. 15:1397040. doi: 10.3389/fimmu.2024.1397040
Received: 06 March 2024; Accepted: 08 April 2024;
Published: 02 May 2024.
Edited by:
Javier Carbone, Gregorio Marañón Hospital, SpainReviewed by:
Delia Goletti, National Institute for Infectious Diseases “Lazzaro Spallanzani” IRCCS, ItalyCopyright © 2024 Razonable. This is an open-access article distributed under the terms of the Creative Commons Attribution License (CC BY). The use, distribution or reproduction in other forums is permitted, provided the original author(s) and the copyright owner(s) are credited and that the original publication in this journal is cited, in accordance with accepted academic practice. No use, distribution or reproduction is permitted which does not comply with these terms.
*Correspondence: Raymund R. Razonable, UmF6b25hYmxlLlJheW11bmRAbWF5by5lZHU=