- 1Department of Rehabilitation Medicine, Shanghai Fourth People’s Hospital Affiliated to Tongji University School of Medicine, Shanghai, China
- 2School of Chinese Medicine, Hong Kong Baptist University, Hong Kong, Hong Kong SAR, China
- 3Department of Ophthalmology, Chengdu Pidu District Hospital of Traditional Chinese Medicine, Chengdu, China
- 4School of Health Preservation and Rehabilitation, Chengdu University of Traditional Chinese Medicine, Chengdu, China
- 5Department of Biochemistry and Biophysics, School of Basic Medical Sciences, Peking University, Beijing, China
The immunotherapy for gastrointestinal tumors, as a significant research direction in the field of oncology treatment in recent years, has garnered extensive attention due to its potential therapeutic efficacy and promising clinical application prospects. Recent advances in immunotherapy notwithstanding, challenges persist, such as side effects, the complexity of the tumor immune microenvironment, variable patient responses, and drug resistance. Consequently, there is a pressing need to explore novel adjunctive therapeutic modalities. β-glucan, an immunomodulatory agent, has exhibited promising anti-tumor efficacy in preclinical studies involving colorectal cancer, pancreatic cancer, and gastric cancer, while also mitigating the adverse reactions associated with chemotherapy and enhancing patients’ quality of life. However, further clinical and fundamental research is warranted to comprehensively evaluate its therapeutic potential and underlying biological mechanisms. In the future, β-glucan holds promise as an adjunctive treatment for gastrointestinal tumors, potentially bringing significant benefits to patients.
1 Introduction
In addition to severe gastrointestinal dysfunction, gastrointestinal tumor patients often present with clinical symptoms such as fatigue (24.9%), numbness or tingling sensation (17.2%), abdominal distension (17.2%), dry mouth (15.9%), memory difficulties (11.8%), and severe pain (32%, 47/145), these high symptom burdens may be correlated with a decreased quality of life among gastrointestinal tumor survivors, and the significant economic burden resulting from cancer treatment is also associated with a lower quality of life (1). Currently, the main clinical treatments for gastrointestinal tumors are surgery and radiotherapy/chemotherapy. However, these therapies often result in adverse reactions such as leukopenia, thrombocytopenia, anorexia, anemia, diarrhea, liver damage, mucositis, and dysphagia (2, 3). Tumor immunotherapy is an essential adjuvant therapy following tumor surgery, chemotherapy, and radiotherapy, with the advantages of superior specificity, broad anti-tumor spectrum, and mild toxic side effects (4–6). In recent years, tumor immunotherapy has gradually been applied in the treatment of gastrointestinal tumors, representing a therapeutic approach that activates or enhances the patient’s immune system to attack and eliminate tumor cells (7). Despite significant progress achieved in recent years, it still faces multiple challenges, including: 1) the presence of certain side effects such as diarrhea, fatigue, rash, and elevated lipase levels (8); 2) the complexity of the tumor immune microenvironment, which can affect the effectiveness of immunotherapy (7); 3) the heterogeneity in the response of different patients to specific immunotherapy due to individual differences (9, 10); 4) the development of resistance and low response rates to immune checkpoint inhibitors, resulting from specific genetic mutations or upregulated gene expression in tumor cells or immune cells (7, 11). Therefore, we need to explore novel adjunctive therapies for clinical treatment of gastrointestinal tumors.
β-Glucan is widely used as a natural modulator of biological effects in the study of tumor immunotherapy. In recent years, relevant studies have confirmed that (12), as an immune modulator, β-glucan has shown positive effects in inhibiting tumor cell proliferation and activating anti-tumor immune responses (13–16). Meanwhile, due to its stable structure, it is also used as a component of oral anti-tumor drug delivery carriers (17).
The most common β-glucans used in clinical applications include yeast glucan, shiitake mushroom polysaccharides, fission mushroom polysaccharides, and oat glucan;The source, structure, water solubility and molecular weight of these different β-glucans influence the intensity and type of immune response induced by them (18). β-glucans can be categorized into particulate and soluble glucans based on their water solubility. It has been shown that particulate β-glucan can activate dendritic cells (DCs) and macrophages through the dectin-1 pathway (19, 20). However, soluble glucans can also enhance macrophage proliferation and dectin-1 expression by inducing granulocyte-macrophage colony stimulating factor (GM-CSF) production in macrophages via dectin-1-independent ERK and p38 MAPK pathways, and ultimately exert antitumor effects by mediating TNF-α production via the Syk pathway (21). β-Glucan emerges as a promising biological effector modulator, exhibiting both safety and efficacy in modulating innate and adaptive immune responses (22). It triggers a cascade of immune defense reactions by interacting with surface receptors of diverse immune cells, indicating its potential as a therapeutic agent in immunomodulatory strategies (23).
Gastrointestinal tumors are becoming one of the deadliest diseases worldwide. β-Glucan, a powerful immunomodulator, has exhibited remarkable anti-tumor efficacy in preclinical investigations targeting colorectal, pancreatic, and gastric cancers (14, 24, 25). Notably, its high stability underscores its significance as a carrier for targeted drugs specifically designed to combat gastric cancer (17). Clinical studies further validate its ability to enhance the 5-year survival rate in patients with hepatocellular carcinoma, gastric cancer, and colorectal cancer, while simultaneously reducing the rate of recurrence (26–28). Moreover, β-glucan alleviates adverse reactions associated with chemotherapy, including nausea, abdominal discomfort, mucositis, diarrhea, and leukopenia/thrombocytopenia (29), ultimately leading to an enhancement in patients’ overall quality of life (30). In the future, with the advancement of clinical research and technological innovations, β-glucan holds the promise of emerging as a significant adjunctive therapeutic option for patients with gastrointestinal tumors.
2 Classification and sources of β-glucans
β-Glucan, a versatile polysaccharide, exhibits a wide range of biological functions and is found ubiquitously in diverse biological entities, including Cereals, fungi, algae and bacteria, primarily in the forms of (1 → 3) (1 → 6)-β-D-glucan, (1 → 3) (1 → 4)-β-D-glucan and (1 → 3)-β-D-glucan, in addition, studies have shown that the branching derived from the glycosidic chain core varies among different species (Table 1). Cereals constitute the primary source of β-glucans. Specifically, wheat typically contains approximately 1% β-glucans, oats range from 3% to 7%, and barley exhibits a β-glucan content spanning from 5% to 11%, indicating their significant presence in these cereal grains (48). Naturally occurring β-glucans derived from cereals exhibit a distinctive structural motif that primarily comprises β-(1→3) and (1→4) glycosidic linkages, which underlie their unique chemical constitution and diverse physicochemical properties. These β-glucans in cereals are linear homopolysaccharides composed of D-glucopyranosyl residues linked through β-(1→3) and β-(1→4) glycosidic bonds (49).In contrast to cereal-derived β-glucans, β-glucans isolated from fungi and bacteria possess a distinct structural composition, primarily characterized by β-(1→3) and (1→6) glycosidic linkages. In these microorganisms, β-glucans consist of a linear backbone composed of glucose residues interconnected via β-(1→3) linkages, often adorned with glucose side-chains of varying lengths, which are attached through β-(1→6) linkages (50). On average, β-(1→6) substitutions occur at intervals of every two to three β-(1→3) main chain residues.
3 Biosynthetic Pathways of β-glucan
Recently, Pallinti Purushotham et al. (51) have identified the catalytic activity and protein structural characteristics of barley (1, 3; 1, 4)-β-glucan synthase (CslF6) through cryo-electron microscopy and biochemical experiments, revealing the detailed mechanism of CslF6-catalyzed (1, 3; 1, 4)-β-glucan biosynthesis. Specifically, the C6 hydroxyl groups of consecutive (1, 3)- and (1, 4)-β-linked glycosyl units point in approximately the same direction during translocation, while consecutive (1, 4)-β-linked glycosyl units rotate approximately 180°. A conserved “ switch motif “ at the entrance of the transmembrane (TM) channel serves as a crucial element for CslF6 enzymatic activity. This motif recognizes specific features of the translocating glucan, interacts with the penultimate glucosyl unit, leading to the formation of a new cellotriosyl unit (DP3) (51). The “switch motif” can also interact with the antepenultimate glucosyl unit, triggering the repositioning of the glucan acceptor, ultimately resulting in the formation of a new cellotetraosyl unit (DP4) (51). This interaction with the penultimate and antepenultimate glucosyl units ultimately generates (1, 3; 1, 4)-β-glucan containing DP3 and DP4 (Figure 1).
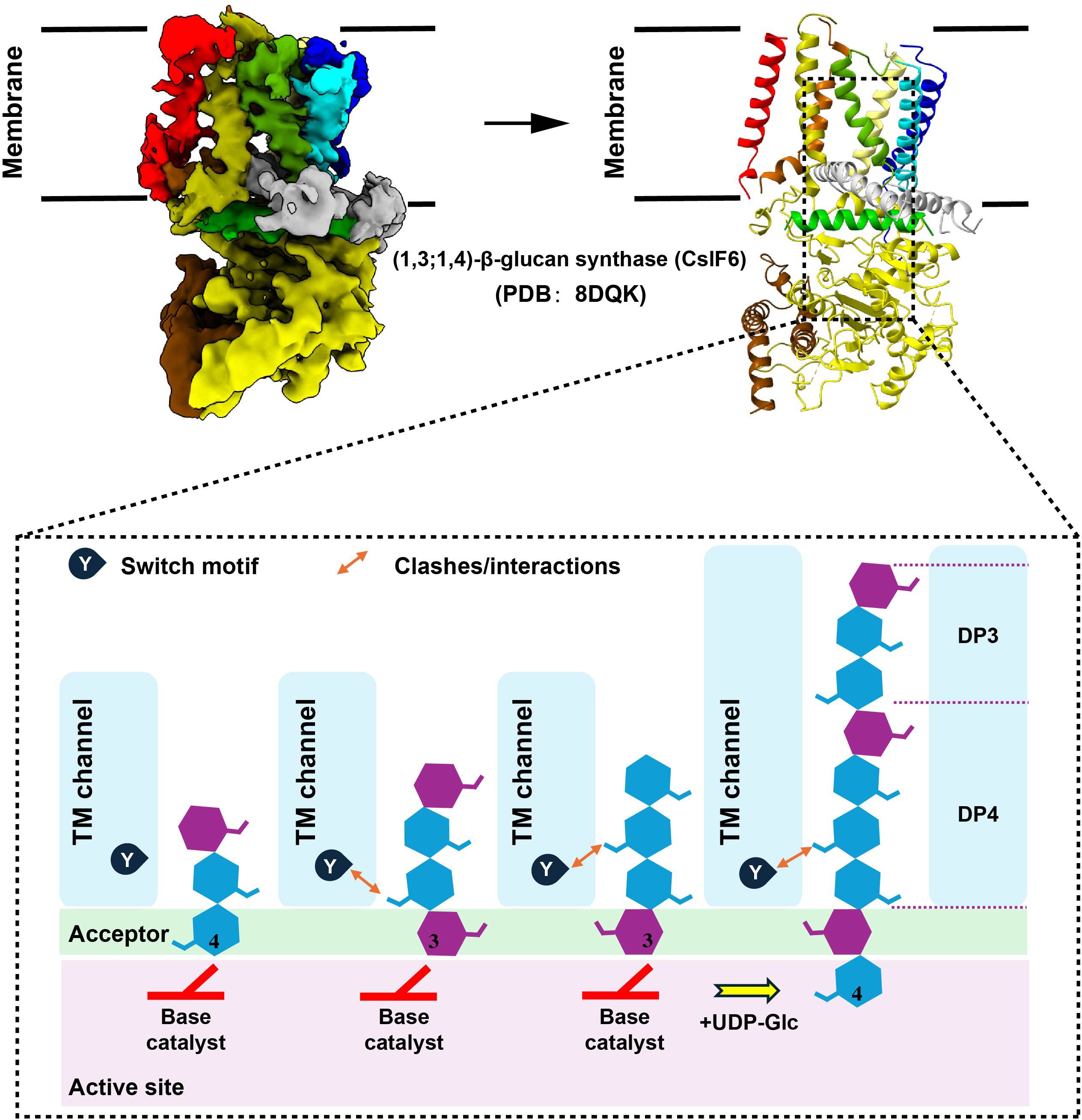
Figure 1 Model of (1, 3; 1, 4)-β-glucan biosynthesis. To generate figure using the raw data from the PDB database (PDB: 8DQK), cartoon representations of the protein structures were created using PyMOL. The graphical approach for illustrating the synthetic mechanism is referenced from DOI: 10.1126/sciadv.add1596.
In the biosynthetic pathway of β-1,3-glucan, the pivotal membrane-integral Glucan Synthetase (GS) complex (Figure 2A), comprised of a fks/gsc-encoded catalytic subunit and a rho1-encoded regulatory subunit, holds paramount significance (52). Recent studies have made groundbreaking progress by utilizing cryo-electron microscopy (Cryo-EM) to elucidate the structure of fungal 1,3-β-glucan synthase (Fks1) (53, 54) (Figure 2B). This significant advancement has profoundly contributed to our understanding of the β-1,3-glucan synthesis mechanism. Specifically, the Fks1 catalytic subunit necessitates the soluble GTPase Rho1 as its regulatory partner, enabling uridine diphosphate glucose (UDP-Glc) to serve as the sugar donor for the catalysis of β(1→3) glycosidic bonds in 1,3-β-glucan, thereby promoting the translocation of glucan chains from the intracellular milieu to the extracellular space (52, 53). The cryo-EM structure reveals the apo state, characterized by the flexibility of IF2 and IF3 and an unoccupied active site primed for substrate binding. In this state, the glucan transport channel remains closed, held in place by TM8. However, upon substrate binding and the subsequent chemical reaction, the GT domain undergoes a downward motion, expelling the formed glucan product out of the cell. Simultaneously, IF2 and IF3 play a role in stabilizing the glucan chain during its elongation. Notably, the movement of TM8 shifts outward, opening the channel for glucan release (53). (Figure 2C).
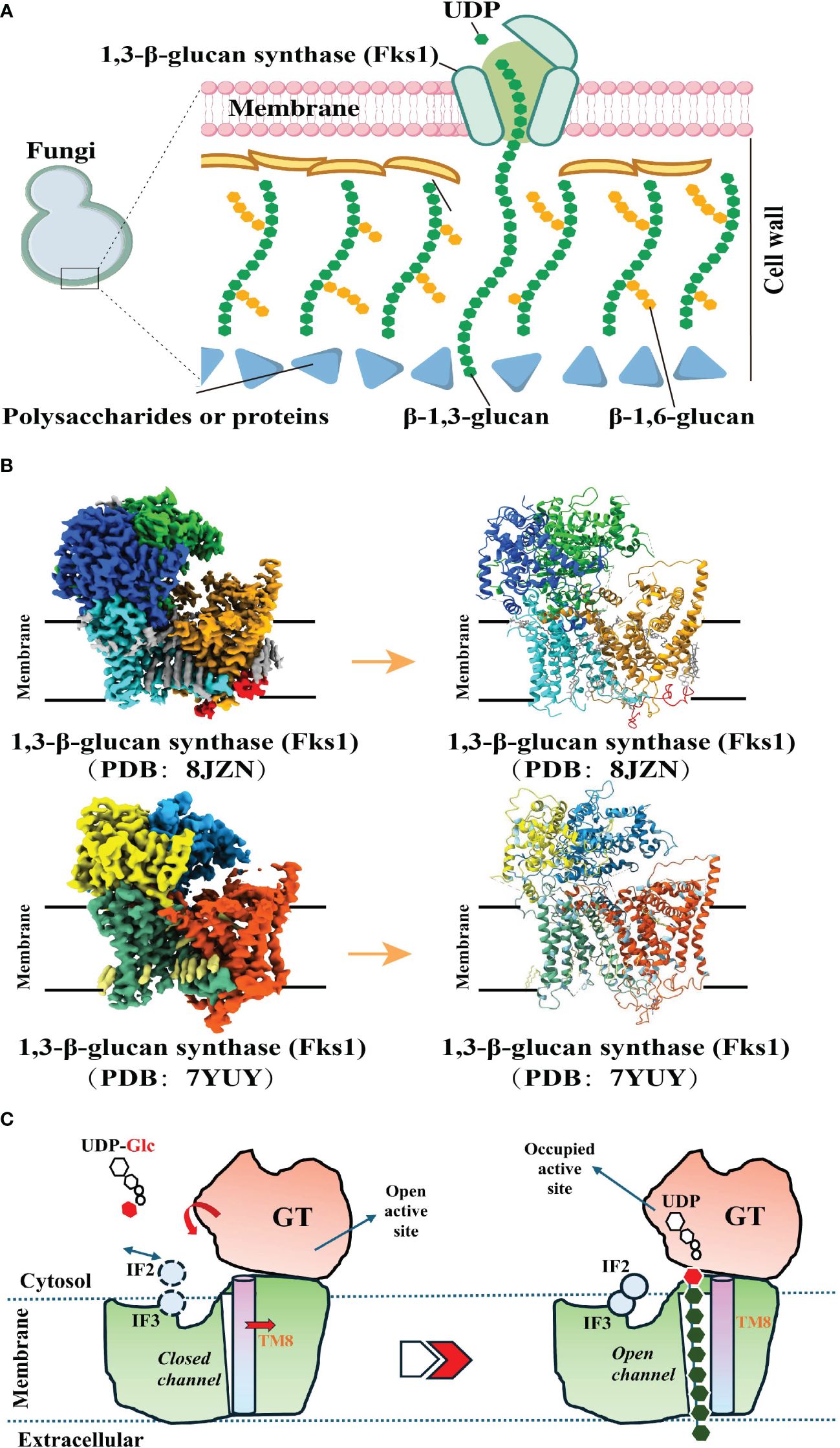
Figure 2 Biosynthetic Pathways of 1, 3-β-Glucan. (A). A model of the fungal cell wall depicts FKS1 utilizing UDP-Glc as a substrate to synthesize the crucial β-1,3-glucan component. (A) was created using Figdraw (https://www.figdraw.com. ID : TROPO6a95 d). (B). Cryo-EM map of FKS1 and the FKS1 structure in cartoon display. To generate figures using the raw data from the PDB database (PDB: 8JZN and PDB: 7YUY), cartoon representations of the protein structures were created using PyMOL. (C). Proposed catalytic mechanism of Fks1. The graphical approach for illustrating the synthetic mechanism is referenced from DOI: 10.1126/sciadv.adh7820 and DOI: 10.1038/s41586-023-05856-5.
The research indicates that CSLD3 is a UDP-glucose-dependent β-1,4-glucan synthase (55). However, due to the lack of structural elucidation, the specific biosynthetic pathway involving this protein remains unreported. Although KRE6, SKN1, FfGS6, and GsmA have been proven to participate in β-1,6-glucan synthesis, the qualitative characterization of the activities of these β-1,6-glucan synthases has not been achieved, and their protein structures have yet to be successfully resolved. Consequently, the regulatory mechanisms underlying their function remain unclear (56–58).
4 Receptors that mediate the effects of β-glucan
The immune regulatory function of β-glucan depends on the complexity of their conformational changes (59). β-glucan can regulate innate immune cells by binding to pattern recognition receptors (PRR) on the surface, thereby exerting anti-tumor effects (60–63). β-glucan activate specific receptors or proteins, such as Dectin-1, toll-like receptors (TLR), complement receptor 3 (CR3), scavenger receptors (SR), and lactacyl ceramide (LacCer), triggering the secretion of cytokines, and subsequently activating other anti-tumor immune cells (64–66) (Figure 3A).
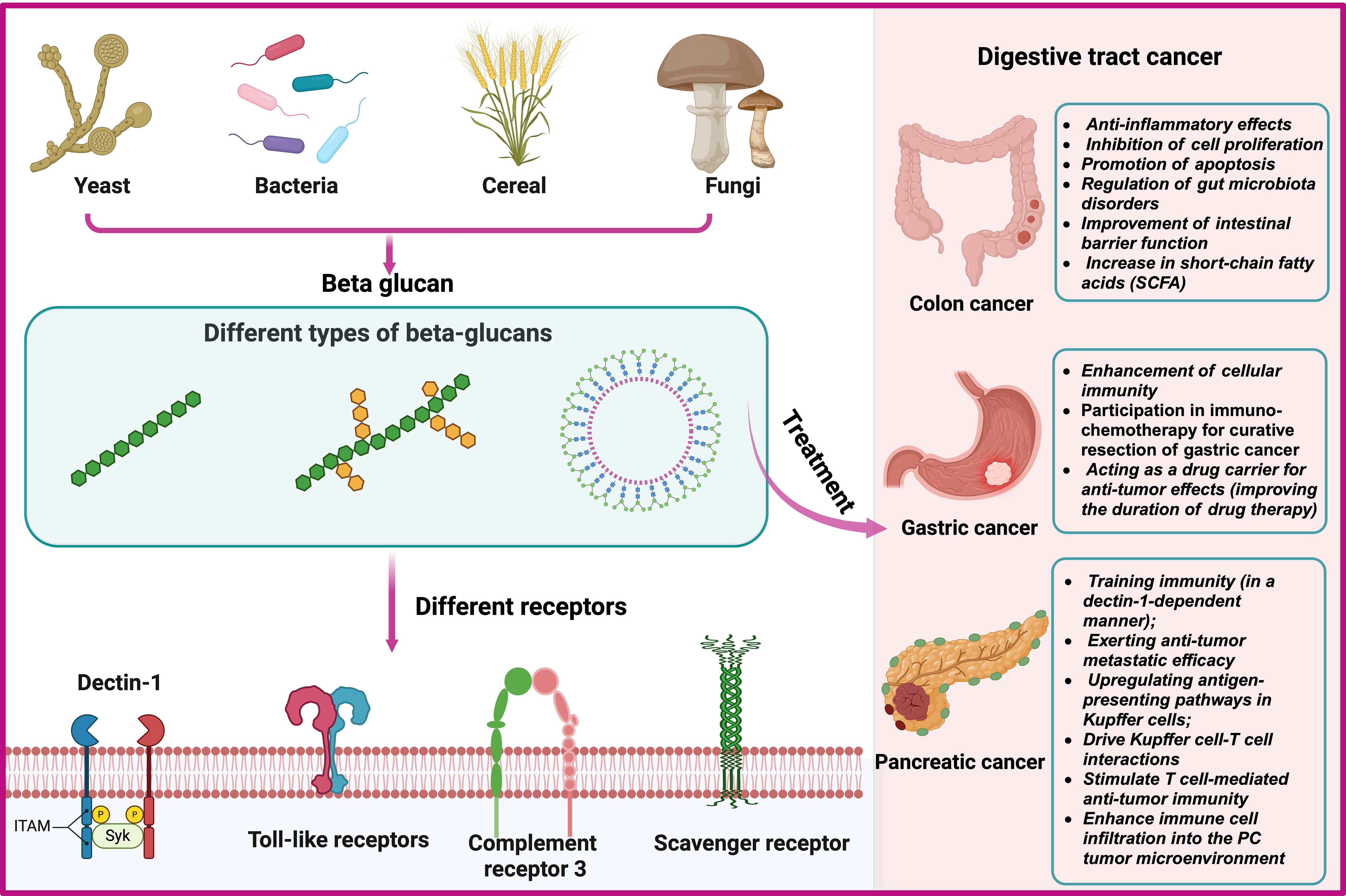
Figure 3 Sources, Classification and Interacting Receptors of Beta-Glucans and Biological Mechanisms for the Prevention and Treatment of Gastrointestinal Tumors.
5 The role and biological mechanism of β-glucan in the prevention and treatment of digestive system neoplasms
It is well known that β-glucan, as an immune modulator, can effectively activate the immune system (67, 68). It exerts anti-tumor effects by activating innate and adaptive immune responses (69–71). Recent studies have shown that β-glucan has anti-tumor effects in colorectal cancer (54), pancreatic cancer (24) and gastric cancer (25). Furthermore, in animal models of gastric cancer, β-glucan can serve as a drug carrier to enhance the retention time of drugs at the tumor site, further augmenting the anti-tumor effects (17, 72). The biological mechanism of its role is still being explored (Figure 3B).
5.1 The therapeutic effect and mechanism of β-glucan on colorectal cancer
In animal models of colorectal cancer, Liu N et al. found that β-glucan can exert the following biological functions, including: 1) anti-inflammatory effect; 2) inhibition of cell proliferation; 3) promotion of cell apoptosis; 4) regulation of intestinal flora disorder; 5) improvement of intestinal barrier function; 6) increase of short-chain fatty acids (SCFA), which can exert anti-tumor effects through synergistic effects of the above biological functions (14). Qi J et al. found that alternating intake of β-glucan in the diet of mice with colon cancer can reduce colon damage and mortality by reducing TNF-α levels, increasing the relative abundance of Parabacteroides, and downregulating three genes associated with inflammation and cancer (Hmgcs2, Fabp2, and Gpt) (73). IL-22 binding protein (IL-22BP) play an important role in inhibiting the development of intestinal tumors (74). In a mouse colorectal tumor model, low molecular weight β-glucan laminarin can exert its anti-colon tumor effect by regulating the Dectin-1-PGE2-IL-22BP signaling axis (75). Kim JH et al. found that low-molecular-weight Aureobasidium pullulans-fermented β-D-glucan (LMW-AP-FBG) can exert anti-tumor effects in mice with CT-26 colon cancer cells by reducing tumor proliferation, inducing apoptosis, and inhibiting inflammation (by inhibiting the NF-κB signaling pathway) (76). Kopiasz et al. found that low-molecular- weight β-glucan (derived from oats) can stimulate autophagy and increase apoptosis levels to positively affect early colorectal cancer rats (77). Sulaiman Binmama et al. found that in the mouse colon cancer model induced by azomethane (AOM) and injected subcutaneously with cancer cells, and found that S.cerevisiae isolated whole glucan particles (WGP) enhance the energy status of macrophages by activating Dectin-1, thereby inhibiting tumor growth (78). It is believed that S.cerevisiae or β-glucan can be used to prevent the occurrence of colon cancer. A recent study has elucidated the impact of a sustained 8-week dietary intake of low-molecular-weight oat β-glucan on the antioxidant capability, inflammatory markers, and colonic metabolomic profiles in a rat model of early colon cancer induced by azoxymethane (AOM). The findings reveal that β-glucan intervention significantly enhances total antioxidant status, diminishes superoxide dismutase (SOD) activity, and lowers thiobarbituric acid reactive substances (TBARS) levels. Notably, β-glucan supplementation was found to reduce proinflammatory cytokines including interleukin (IL-1α, IL-1β, IL-12 and C-reactive protein (CRP), while augmenting IL-10 concentrations. Moreover, metabolomic analyses have validated that oat β-glucan elevates the abundance of metabolites such as amino acids, purines, biotin, and folic acid. The researchers postulate that during the incipient stages of carcinogenesis, colonic metabolism undergoes profound alterations, and low-molecular-weight oat β-glucan emerges as a promising dietary supplement for modulating these mechanisms (79). Liu N and colleagues achieved precise fractionation of β-glucan isolated from lentils into three fractions with varying weight-average molecular weights (Mw) through ultrasonic degradation, and thoroughly evaluated their therapeutic potential in colorectal cancer. In cellular experiments, these β-glucan fractions exhibited remarkable inhibitory effects on colon cancer cell proliferation, induced apoptosis, and alleviated inflammatory responses. Moreover, in vivo investigations utilizing mouse models highlighted the superior anti-inflammatory and anti-colorectal cancer properties of low-molecular-weight β-glucan, which was attributed to its ability to restore the intestinal mucosal barrier, enhance the content of SCFAs, modulate the metabolic landscape of intestinal microbiota, and reshape the intestinal microbiota composition. These findings offer compelling scientific evidence for the utilization of β-glucan as a modulator of intestinal microbiota, representing a promising alternative therapeutic strategy for the clinical treatment of colon cancer (14). The impact of β-glucan on intestinal immune responses during colitis-associated colorectal cancer (CAC) remains unclear. Researchers have delved into the underlying mechanisms of β-glucan’s role in the pathogenesis of CAC. Following oral administration of β-glucan in a CAC mouse model, a significant increase in antitumor DCs within the tumor microenvironment was observed, leading to a subsequent augmentation of CD8+ T cells and associated cytokine production. Additionally, β-glucan enhanced resilience against chronic colitis by remodeling the inflammatory microenvironment. These data suggest that β-glucan can ameliorate experimental intestinal inflammation and delay the progression of CAC (80). Ji Hyeon Kim and colleagues evaluated the therapeutic potential of LMW-AP-FBGin BALB/c mice implanted with subcutaneous CT-26 colon cancer cells. Their study demonstrated that daily intraperitoneal injection of LMW-AP-FBG (5 mg/kg) over a two-week period significantly suppressed tumor growth by reducing tumor proliferation and inducing apoptosis. Moreover, the treatment reduced the viability of CT-26 cells in a dose-dependent manner, via mechanisms that involve the loss of mitochondrial transmembrane potential and the promotion of apoptosis. This investigation indicates that LMW-AP-FBG possesses anticancer properties both in vitro and in vivo (76). The above studies suggest that β-glucan plays a certain preventive and therapeutic role in preclinical models of colorectal cancer, and further in-depth study of its biological mechanism of action in the future will lay the foundation for its clinical translation.
5.2 Therapeutic effect and mechanism of β-glucan on pancreatic cancer
Research indicates that β-glucan, as an immune agonist, has the ability to train the body’s immune system and promote anti-tumor immune effects by training and enhancing the body’s immune function (81, 82). In the mouse pancreatic cancer (PC) model, β-glucan can induce training immunity in mouse pancreas and prolong the survival rate of PC; the combination therapy with irreversible electroporation (IRE) showed better anti-tumor effects in a PC mouse model. The biological effects of this combination therapy mainly include: 1) Enhancing immune cell infiltration into the PC tumor microenvironment; 2) Enhancing the training response of tumor infiltrating bone marrow cells. 3) Reducing local and distant tumor burden and prolong the survival rate of mouse in situ PC model (24). Due to the highly desmoplastic tumor microenvironment (TME) of pancreatic ductal adenocarcinoma (PDAC), its treatment prognosis is poor. Researchers have developed an oral β-glucan-functionalized zinc doxorubicin nanoparticle system (β-Glus-ZnD-NPs) for targeted PDAC therapy. This β-Glus-ZnD NPs system actively targets and traverses the folds of the small intestine, overcoming the intestinal epithelial barrier. Subsequently, the nanoparticles are phagocytosed by endogenous macrophages (β-Glus-ZnD@Mϕ), which are activated to produce matrix metalloproteinases that destroy the desmoplastic stromal barrier. These macrophages also differentiate into an M1-like phenotype, modulating the tumor microenvironment and recruiting effector T cells, ultimately inducing tumor cell apoptosis (83). The liver is the most common site of metastasis in PDAC. A study indicates that β-glucan can activate liver-resident macrophages (Kupffer cells) and prevent PDAC from metastasizing to the liver. β-glucan exerts its anti-tumor metastasis effect through the following mechanisms: 1) upregulating the antigen presentation pathway in Kupffer cells; 2) driving the interaction between Kupffer cells and T cells; 3) stimulating T cells -mediated anti-tumor immunity; 4) Kupffer cells and T cells coordinate the activation of macrophages (BMDM). At the same time, researchers also found that the combination of β-glucan treatment and anti-PD1 treatment can inhibit liver metastasis. Geller AE and other studies have found that β-glucan enters the pancreas in a dectin-1-dependent manner, and then β-glucan stimulates trained myeloid cells to flow into the pancreas to exert anti-tumor effects (84). Researchers used CCR2−/− mice to confirm that the peripheral immune cells that fight tumors are mainly trained CCR2+ myeloid cells, which recruit and infiltrate into pancreatic tumor cell areas through the CCL2-CCR2 signaling axis, and then exert their anti-tumor effects through phagocytosis and ROS-mediated cytotoxicity (84). To sum up, in the treatment of pancreatic cancer, a “cold tumor”, β-glucan primarily trains immune cells by acting as an immunostimulator, thereby mediating anti-tumor immune responses.
5.3 The therapeutic effect and mechanism of β-glucan on gastric cancer
Lentinan, a β-glucan extracted from mushrooms, has been proven to possess antitumor activity (25, 85). Researchers have confirmed its preventive and therapeutic effects on gastric cancer, and the mechanisms of its action include: 1) inhibiting the G2/M phase of the gastric cancer cell division cycle to exert anti-proliferative effects; 2) Lentinan can attenuate the wound healing, colony formation, and migration abilities of AGS cells; 3) Lentinan can increase the expression of phospho-p38, while reducing the expression of phospho-ERK1/2 and Mu-2-related death-inducing gene (MuD) proteins; 4) Lentinan induces the generation of reactive oxygen species (ROS), directly participating in cell death (25). In the treatment of gastric cancer, β-glucan can be used as a main component of drug carriers to jointly exert anti-tumor effects. To develop an oral drug that improves treatment efficacy by prolonging gastric treatment time, researchers have developed an oral delivery tool based on β-glucan, which can control the release of Bcl2 siRNA and 5-fluorouracil (5FU) payloads for more than 6 hours. By increasing their adsorption time in gastric mucus, Bcl2 siRNA can selectively knock out the Bcl2 gene to promote cell apoptosis and alleviate cancer (17). The team developed the therapeutic effect of oral administration of Navi/siRNA mediated by Glucan and Docosahexaenoic Acid (GADA) was studied in a mouse model of β-gastric cancer (72). The results showed that the mice treated with GADA/NAVI/siRNA had a higher inhibitory effect on Bcl2. In summary, in the treatment of gastric cancer, β-glucan not only plays a direct anti-tumor role, but also can be used as a major component of drug carriers to increase the adhesion properties of the carrier, thereby improving the duration and efficacy of drug treatment. These studies have opened up a new horizon for long-term oral drug therapy for gastric cancer.
6 Application of β-glucans in the treatment of patients with gastrointestinal tumors
For patients with advanced gastrointestinal cancer, it is highly desirable to continue chemotherapy with minimal adverse reactions for an extended period. Previous clinical studies have indicated that the adjunctive use of β-glucan during chemotherapy or radiotherapy for hepatocellular carcinoma, gastric cancer, and colorectal cancer can increase the 5-year survival rate by 15% and reduce the recurrence rate by 43% (26–28). In Japan, two types of β-glucans, krestin and lentinan, are licensed as drugs for gastric cancer treatment (85). Among them, Krestin is extracted from Coriolus versicolor, and this preparation has been clinically used for surgical treatment of resectable gastric cancer (86–88). Okuno K et al. investigated the effects of an oral β-glucan immunomodulator, Lentinula edodes mycelia extract (LEM), on immune function and chemotherapy-induced adverse reactions in 1 patient with gastric cancer and 7 patients with colorectal cancer. After treatment with LEM (1800 mg/day for 28 days), 8 patients experienced reduced adverse reactions such as nausea and abdominal pain caused by chemotherapy. Additionally, LEM increased the production of interferon (IFN)-γ by CD4+, CD8+ T, and CD56+ NK/NKT cells. These results suggest that the concurrent use of LEM with chemotherapy can reduce the probability of adverse reactions caused by cancer chemotherapy in patients with advanced cancer (89).
Lentinan (LNT) is an immune adjuvant medicine for advanced gastric cancer in Japan, in a multicenter clinical study conducted in Japan, 71 patients with advanced colorectal cancer who met the inclusion criteria were enrolled. In this study, researchers employed superfine dispersed lentinan (SDL), an oral formulation of 1,3-β-glucan, as an adjunctive therapy to assess post-chemotherapy adverse reactions, quality of life (QOL), and the binding capacity of peripheral blood monocytes (PBMs) to lentinan (LNT). After receiving SDL treatment (15 mg/day for 84 days), QOL was significantly improved in the majority of patients. Notably, the PBM binding rate to LNT was significantly higher in the QOL-improved group compared to the non-improved group. These results demonstrate that SDL is both safe and effective in suppressing chemotherapy-induced adverse reactions and enhancing QOL in patients with advanced colorectal cancer. Furthermore, the binding capacity of PBMs to LNT may serve as a potential predictor of QOL improvement following SDL administration (30). In a retrospective study examining the effects of oral β-glucan administration on chemotherapy-induced adverse reactions in 62 patients with advanced colorectal tumors, significant reductions in chemotherapy-related adverse reactions such as oral mucositis and diarrhea were observed following treatment with β-glucan (50 mg/day for 7 days). Notably, there were no significant decreases in white blood cell count, neutrophil count, or platelet count following chemotherapy. These findings suggest that β-glucan may be utilized to alleviate adverse reactions following chemotherapy in patients with advanced colorectal cancer (29) In patients with KRAS-mutant colorectal cancer (CRC), monotherapy with cetuximab has been ineffective. Researchers have explored the combination of β-glucan (Imprime PGG) with cetuximab, owing to Imprime PGG’s ability to enhance the recognition of natural immune cells. The study revealed that the combination of Imprime PGG and cetuximab demonstrated convincing yet moderate clinical activity, providing evidence for the clinical efficacy of Imprime PGG when combined with complement-activating antibodies (90). In a clinical study, β-glucan was incubated with the blood of colorectal cancer patients, and it was found that after incubation with β-glucan, the DNA damage parameters in the blood significantly decreased, suggesting that β-glucan can significantly reduce DNA damage in colorectal cancer patients through antioxidant effects (91). In a phase I clinical trial evaluating β-glucan-based combination therapy for PDAC, researchers aimed to assess the therapeutic efficacy of BTH1704 (a monoclonal antibody targeting aberrantly glycosylated Mucin 1) and Imprime PGG (a glucan derived from yeast that is crucial in triggering leukocyte-mediated cytotoxic responses against tumor cells), combined with gemcitabine, in patients with advanced PDAC (NCT02132403).
7 Summary and prospect
β-Glucan, as an immunomodulator, has demonstrated promising anti-tumor effects in preclinical studies of colorectal cancer, pancreatic cancer, and gastric cancer (14, 24, 25). Notably, the high stability of β-glucan renders it a crucial component for serving as a carrier for targeted drugs against gastric cancer (17). In clinical studies, β-glucan has been shown to improve the 5-year survival rate of hepatocellular carcinoma, gastric cancer, and colorectal cancer, while reducing the recurrence rate (26–28). Additionally, it can mitigate adverse reactions caused by chemotherapy, such as nausea, abdominal pain, mucositis, diarrhea, and leukopenia/thrombocytopenia (29), leading to an improvement in patient quality of life (30). Furthermore, β-glucan has the potential to enhance the anti-tumor effects of anti-tumor immune agents (90). Given that β-glucan is mostly derived from edible mushrooms, its natural origin promotes the development of β-glucan-related drugs or clinical health products. In the future, β-glucan may serve as a combined treatment method with monoclonal antibodies to improve anti-tumor immune responses. Although β-glucan has been proven to exert certain therapeutic effects in the treatment of gastrointestinal tumors, most of the studies demonstrating its anti-tumor effects are preclinical. More clinical research is needed to evaluate its therapeutic efficacy in gastrointestinal tumors. Simultaneously, further basic research is required to explore the specific biological mechanisms of β-glucan in the prevention and treatment of gastrointestinal tumors. In the future, β-glucan may serve as an adjuvant therapy for gastrointestinal tumors, benefiting patients with such malignancies.
Author contributions
MW: Writing – original draft, Writing – review & editing. JP: Writing – original draft, Writing – review & editing. WX: Writing – review & editing. ZY: Writing – review & editing, Visualization. YZ: Writing – review & editing. JW: Writing – original draft, Writing – review & editing. AZ: Writing – review & editing.
Funding
The author(s) declare financial support was received for the research, authorship, and/or publication of this article. This work was supported by the Tongji University Affiliated Shanghai Fourth People’s Hospital Research Launch Special Fund (No.sykyqd0201), key clinical supportive specialist construction project of Hongkou District (No.HKLCFC202406), Basic Research Project of Discipline Boosting Program of the Fourth People’s Hospital Affiliated to Tongji University (NO.SY-XKZT-2023-1013) and the Tongji University Affiliated Shanghai Fourth People’s Hospital Talent Promotion Program Project (NO.SY-XKZT-2023-3005).
Acknowledgments
Figure 3 was drawn by Biorender (https://app.biorender.com).
Conflict of interest
The authors declare that the research was conducted in theabsence of any commercial or financial relationships that could be construed as a potential conflict of interest.
Publisher’s note
All claims expressed in this article are solely those of the authors and do not necessarily represent those of their affiliated organizations, or those of the publisher, the editors and the reviewers. Any product that may be evaluated in this article, or claim that may be made by its manufacturer, is not guaranteed or endorsed by the publisher.
References
1. Ahn S, Jung H, Kim S, Shin SJ, Park CG, Chu SH. Quality of life among Korean gastrointestinal cancer survivors. Eur J Oncol nursing: Off J Eur Oncol Nurs Society. (2017) 30:15–21. doi: 10.1016/j.ejon.2017.07.002
2. Chen J, Guo Y, Fang M, Yuan Y, Zhu Y, Xin Y, et al. Neoadjuvant chemoradiotherapy for resectable gastric cancer: A meta-analysis. Front Oncol. (2022) 12:927119. doi: 10.3389/fonc.2022.927119
3. Lu H, Sun Y, Zhu Z, Yao J, Xu H, Huang R, et al. Effect of chemoradiotherapy on the survival of resectable gastric cancer patients: A systematic review and meta-analysis. Ann Surg Oncol. (2022) 29:6962–75. doi: 10.1245/s10434-022-12005-1
4. Weiss EM, Wunderlich R, Ebel N, Rubner Y, Schlücker E, Meyer-Pittroff R, et al. Selected anti-tumor vaccines merit a place in multimodal tumor therapies. Front Oncol. (2012) 2:132. doi: 10.3389/fonc.2012.00132
5. Drerup JM, Liu Y, Padron AS, Murthy K, Hurez V, Zhang B, et al. Immunotherapy for ovarian cancer. Curr Treat options Oncol. (2015) 16:317. doi: 10.1007/s11864-014-0317-1
6. Dharmadhikari N, Mehnert JM, Kaufman HL. Oncolytic virus immunotherapy for melanoma. Curr Treat options Oncol. (2015) 16:326. doi: 10.1007/s11864-014-0326-0
7. Hegde PS, Chen DS. Top 10 challenges in cancer immunotherapy. Immunity. (2020) 52:17–35. doi: 10.1016/j.immuni.2019.12.011
8. Overman MJ, McDermott R, Leach JL, Lonardi S, Lenz HJ, Morse MA, et al. Nivolumab in patients with metastatic DNA mismatch repair-deficient or microsatellite instability-high colorectal cancer (CheckMate 142): an open-label, multicentre, phase 2 study. Lancet Oncol. (2017) 18:1182–91. doi: 10.1016/S1470-2045(17)30422-9
9. Kim ST, Cristescu R, Bass AJ, Kim KM, Odegaard JI, Kim K, et al. Comprehensive molecular characterization of clinical responses to PD-1 inhibition in metastatic gastric cancer. Nat Med. (2018) 24:1449–58. doi: 10.1038/s41591-018-0101-z
10. Cristescu R, Lee J, Nebozhyn M, Kim KM, Ting JC, Wong SS, et al. Molecular analysis of gastric cancer identifies subtypes associated with distinct clinical outcomes. Nat Med. (2015) 21:449–56. doi: 10.1038/nm.3850
11. Cheng AL, Qin S, Ikeda M, Galle P, Ducreux M, Zhu A, et al. LBA3 - IMbrave150: Efficacy and safety results from a ph III study evaluating atezolizumab (atezo) + bevacizumab (bev) vs sorafenib (Sor) as first treatment (tx) for patients (pts) with unresectable hepatocellular carcinoma (HCC). Ann Oncol. (2019) 30:ix186–ix7. doi: 10.1093/annonc/mdz446.002
12. Wani SM, Gani A, Mir SA, Masoodi FA, Khanday FA. β-Glucan: A dual regulator of apoptosis and cell proliferation. Int J Biol macromolecules. (2021) 182:1229–37. doi: 10.1016/j.ijbiomac.2021.05.065
13. Ding C, Shrestha R, Zhu X, Geller AE, Wu S, Woeste MR, et al. Inducing trained immunity in pro-metastatic macrophages to control tumor metastasis. Nat Immunol. (2023) 24:239–54. doi: 10.1038/s41590-022-01388-8
14. Liu N, Zou S, Xie C, Meng Y, Xu X. Effect of the β-glucan from Lentinus edodes on colitis-associated colorectal cancer and gut microbiota. Carbohydr polymers. (2023) 316:121069. doi: 10.1016/j.carbpol.2023.121069
15. da Silva TP, Geraldelli D, Martins KO, Braga AJL, Rosa AP, Ferneda JMA, et al. Antioxidant, anti-inflammatory and beneficial metabolic effects of botryosphaeran [(1→3)(1→6)-β-d-glucan] are responsible for its anti-tumour activity in experimental non-obese and obese rats bearing Walker-256 tumours. Cell Biochem Funct. (2022) 40:213–27. doi: 10.1002/cbf.3690
16. Khan AA, Gani A, Khanday FA, Masoodi FA. Biological and pharmaceutical activities of mushroom β-glucan discussed as a potential functional food ingredient. Bioactive Carbohydrates Dietary Fibre. (2018) 16:1–13. doi: 10.1016/j.bcdf.2017.12.002
17. Afrin H, Esquivel SV, Kumar R, Zahid MI, Oporeza B, Rahman MF, et al. β-glucan-mediated oral codelivery of 5FU and bcl2 siRNA attenuates stomach cancer. ACS Appl materials interfaces. (2023) 15:32188–200. doi: 10.1021/acsami.3c03528
18. Synytsya A, Novák M. Structural diversity of fungal glucans. Carbohydr polymers. (2013) 92:792–809. doi: 10.1016/j.carbpol.2012.09.077
19. Qi C, Cai Y, Gunn L, Ding C, Li B, Kloecker G, et al. Differential pathways regulating innate and adaptive antitumor immune responses by particulate and soluble yeast-derived β-glucans. Blood. (2011) 117:6825–36. doi: 10.1182/blood-2011-02-339812
20. Goodridge HS, Reyes CN, Becker CA, Katsumoto TR, Ma J, Wolf AJ, et al. Activation of the innate immune receptor Dectin-1 upon formation of a 'phagocytic synapse'. Nature. (2011) 472:471–5. doi: 10.1038/nature10071
21. Masuda Y, Togo T, Mizuno S, Konishi M, Nanba H. Soluble β-glucan from Grifola frondosa induces proliferation and Dectin-1/Syk signaling in resident macrophages via the GM-CSF autocrine pathway. J leukocyte Biol. (2012) 91:547–56. doi: 10.1189/jlb.0711386
22. van Steenwijk HP, Bast A, de Boer A. Immunomodulating effects of fungal beta-glucans: from traditional use to medicine. Nutrients. (2021) 13(4):1333. doi: 10.3390/nu13041333
23. Jin Y, Li P, Wang F. β-glucans as potential immunoadjuvants: A review on the adjuvanticity, structure-activity relationship and receptor recognition properties. Vaccine. (2018) 36:5235–44. doi: 10.1016/j.vaccine.2018.07.038
24. Woeste MR, Shrestha R, Geller AE, Li S, Montoya-Durango D, Ding C, et al. Irreversible electroporation augments β-glucan induced trained innate immunity for the treatment of pancreatic ductal adenocarcinoma. J immunotherapy Cancer. (2023) 11:e006221. doi: 10.1136/jitc-2022-006221
25. Park GS, Shin J, Hong S, Gopal J, Oh J-W. Anticarcinogenic potential of the mushroom polysaccharide lentinan on gastric and colon cancer cells: Antiproliferative, antitumorigenic, Mu-2-related death-inducing gene, MUDENG ramifications. J Ind Eng Chem. (2024) 135:122–30. doi: 10.1016/j.jiec.2024.01.024
26. Ohwada S, Ikeya T, Yokomori T, Kusaba T, Roppongi T, Takahashi T, et al. Adjuvant immunochemotherapy with oral Tegafur/Uracil plus PSK in patients with stage II or III colorectal cancer: a randomised controlled study. Br J cancer. (2004) 90:1003–10. doi: 10.1038/sj.bjc.6601619
27. Oba K, Kobayashi M, Matsui T, Kodera Y, Sakamoto J. Individual patient based meta-analysis of lentinan for unresectable/recurrent gastric cancer. Anticancer Res. (2009) 29:2739–45.
28. Yang P, Liang M, Zhang Y, Shen B. Clinical application of a combination therapy of lentinan, multi-electrode RFA and TACE in HCC. Adv Ther. (2008) 25:787–94. doi: 10.1007/s12325-008-0079-x
29. Karaca H, Bozkurt O, Ozaslan E, Baldane S, Berk V, Inanc M, et al. Positive effects of oral β-glucan on mucositis and leukopenia in colorectal cancer patients receiving adjuvant FOLFOX-4 combination chemotherapy. Asian Pacific J Cancer prevention: APJCP. (2014) 15:3641–4. doi: 10.7314/APJCP.2014.15.8.3641
30. Hazama S, Watanabe S, Ohashi M, Yagi M, Suzuki M, Matsuda K, et al. Efficacy of orally administered superfine dispersed lentinan (beta-1,3-glucan) for the treatment of advanced colorectal cancer. Anticancer Res. (2009) 29:2611–7.
31. Perez Herrera M, Gao J, Vasanthan T, Temelli F, Henderson K. β-Glucan content, viscosity, and solubility of Canadian grown oat as influenced by cultivar and growing location. Can J Plant Science. (2016) 96:183–96. doi: 10.1139/cjps-2014-0440
32. Izydorczyk MS, Dexter JE. Barley β-glucans and arabinoxylans: Molecular structure, physicochemical properties, and uses in food products–a Review. Food Res Int. (2008) 41:850–68. doi: 10.1016/j.foodres.2008.04.001
33. Lazaridou A, Biliaderis CG, Izydorczyk MS. Cereal beta-glucans: structures, physical properties, and physiological functions. Funct Food carbohydrates. (2007) 1–72.
34. Rakszegi M, Lovegrove A, Balla K, Láng L, Bedő Z, Veisz O, et al. Effect of heat and drought stress on the structure and composition of arabinoxylan and β-glucan in wheat grain. Carbohydr polymers. (2014) 102:557–65. doi: 10.1016/j.carbpol.2013.12.005
35. Noss I, Doekes G, Thorne PS, Heederik DJ, Wouters IM. Comparison of the potency of a variety of β-glucans to induce cytokine production in human whole blood. Innate immunity. (2013) 19:10–9. doi: 10.1177/1753425912447129
36. Błaszczyk K, Wilczak J, Harasym J, Gudej S, Suchecka D, Królikowski T, et al. Impact of low and high molecular weight oat beta-glucan on oxidative stress and antioxidant defense in spleen of rats with LPS induced enteritis. Food Hydrocolloids. (2015) 51:272–80. doi: 10.1016/j.foodhyd.2015.05.025
37. Vetvicka V, Vetvickova J. Immune-enhancing effects of Maitake (Grifola frondosa) and Shiitake (Lentinula edodes) extracts. Ann Trans Med. (2014) 2:14. doi: 10.3978/j.issn.2305-5839.2014.01.05
38. Sasaki T, Takasuka N. Further study of the structure of lentinan, an anti-tumor polysaccharide from Lentinus edodes. Carbohydr Res. (1976) 47:99–104. doi: 10.1016/S0008-6215(00)83552-1
39. Wakshull E, Brunke-Reese D, Lindermuth J, Fisette L, Nathans RS, Crowley JJ, et al. PGG-glucan, a soluble beta-(1,3)-glucan, enhances the oxidative burst response, microbicidal activity, and activates an NF-kappa B-like factor in human PMN: evidence for a glycosphingolipid beta-(1,3)-glucan receptor. Immunopharmacology. (1999) 41:89–107. doi: 10.1016/S0162-3109(98)00059-9
40. Fisher M, Yang LX. Anticancer effects and mechanisms of polysaccharide-K (PSK): implications of cancer immunotherapy. Anticancer Res. (2002) 22:1737–54.
41. Bae AH, Lee SW, Ikeda M, Sano M, Shinkai S, Sakurai K. Rod-like architecture and helicity of the poly(C)/schizophyllan complex observed by AFM and SEM. Carbohydr Res. (2004) 339:251–8. doi: 10.1016/j.carres.2003.09.032
42. Mueller A, Raptis J, Rice PJ, Kalbfleisch JH, Stout RD, Ensley HE, et al. The influence of glucan polymer structure and solution conformation on binding to (1–>3)-beta-D-glucan receptors in a human monocyte-like cell line. Glycobiology. (2000) 10:339–46. doi: 10.1093/glycob/10.4.339
43. Bacon JS, Farmer VC, Jones D, Taylor IF. The glucan components of the cell wall of baker's yeast (Saccharomyces cerevisiae) considered in relation to its ultrastructure. Biochem J. (1969) 114:557–67. doi: 10.1042/bj1140557
44. Aimanianda V, Clavaud C, Simenel C, Fontaine T, Delepierre M, Latgé JP. Cell wall beta-(1,6)-glucan of Saccharomyces cerevisiae: structural characterization and in situ synthesis. J Biol Chem. (2009) 284:13401–12. doi: 10.1074/jbc.M807667200
45. Golian M, Chlebová Z, Žiarovská J, Benzová L, Urbanová L, Hovaňáková L, et al. Analysis of biochemical and genetic variability of pleurotus ostreatus based on the β-glucans and CDDP markers. . J fungi (Basel Switzerland). (2022) 8:563. doi: 10.3390/jof8060563
46. Bobadilla F, Rodriguez-Tirado C, Imarai M, Galotto MJ, Andersson R. Soluble β-1,3/1,6-glucan in seaweed from the southern hemisphere and its immunomodulatory effect. Carbohydr polymers. (2013) 92:241–8. doi: 10.1016/j.carbpol.2012.09.071
47. Barsanti L, Vismara R, Passarelli V, Gualtieri P. Paramylon (β-1,3-glucan) content in wild type and WZSL mutant of Euglena gracilis. Effects of growth conditions. J Appl Phycology. (2001) 13:59–65. doi: 10.1023/A:1008105416065
48. Skendi A, Biliaderis CG, Lazaridou A, Izydorczyk MS. Structure and rheological properties of water soluble β-glucans from oat cultivars of Avena sativa and Avena bysantina. J Cereal Science. (2003) 38:15–31. doi: 10.1016/S0733-5210(02)00137-6
49. Tohamy AA, El-Ghor AA, El-Nahas SM, Noshy MM. Beta-glucan inhibits the genotoxicity of cyclophosphamide, adriamycin and cisplatin. Mutat Res. (2003) 541:45–53. doi: 10.1016/S1383-5718(03)00184-0
50. Zhu F, Du B, Xu B. A critical review on production and industrial applications of beta-glucans. Food Hydrocolloids. (2016) 52:275–88. doi: 10.1016/j.foodhyd.2015.07.003
51. Purushotham P, Ho R, Yu L, Fincher GB, Bulone V, Zimmer J. Mechanism of mixed-linkage glucan biosynthesis by barley cellulose synthase-like CslF6 (1,3,1,4)-β-glucan synthase. Sci Adv. (2022) 8:eadd1596. doi: 10.1126/sciadv.add1596
52. Douglas CM. Fungal beta(1,3)-D-glucan synthesis. Med mycology. (2001) 39 Suppl 1:55–66. doi: 10.1080/mmy.39.1.55.66
53. Zhao CR, You ZL, Chen DD, Hang J, Wang ZB, Ji M, et al. Structure of a fungal 1,3-β-glucan synthase. Sci advances. (2023) 9:eadh7820. doi: 10.1126/sciadv.adh7820
54. Hu X, Yang P, Chai C, Liu J, Sun H, Wu Y, et al. Structural and mechanistic insights into fungal β-1,3-glucan synthase FKS1. Nature. (2023) 616:190–8. doi: 10.1038/s41586-023-05856-5
55. Yang J, Bak G, Burgin T, Barnes WJ, Mayes HB, Peña MJ, et al. Biochemical and Genetic Analysis Identify CSLD3 as a beta-1,4-Glucan Synthase That Functions during Plant Cell Wall Synthesis. Plant Cell. (2020) 32:1749–67. doi: 10.1105/tpc.19.00637
56. Han Q, Wang N, Yao G, Mu C, Wang Y, Sang J. Blocking β-1,6-glucan synthesis by deleting KRE6 and SKN1 attenuates the virulence of Candida albicans. Mol Microbiol. (2019) 111:604–20. doi: 10.1111/mmi.14176
57. Liu Y, Ma X, Long Y, Yao S, Wei C, Han X, et al. Effects of β-1,6-glucan synthase gene (FfGS6) overexpression on stress response and fruit body development in flammulina filiformis. Genes. (2022) 13:1753. doi: 10.3390/genes13101753
58. Zhou X, He J, Wang L, Wang Y, Du G, Kang Z. Metabolic engineering of saccharomyces cerevisiae to improve glucan biosynthesis. J Microbiol Biotechnol. (2019) 29:758–64. doi: 10.4014/jmb.1812.12049
59. Bohn JA, BeMiller JN. (1→3)-β-d-Glucans as biological response modifiers: a review of structure-functional activity relationships. Carbohydr Polymers. (1995) 28:3–14. doi: 10.1016/0144-8617(95)00076-3
60. Vetvicka V, Dvorak B, Vetvickova J, Richter J, Krizan J, Sima P, et al. Orally administered marine (1–>3)-beta-D-glucan Phycarine stimulates both humoral and cellular immunity. Int J Biol macromolecules. (2007) 40:291–8. doi: 10.1016/j.ijbiomac.2006.08.009
61. Song K, Xu L, Zhang W, Cai Y, Jang B, Oh J, et al. Laminarin promotes anti-cancer immunity by the maturation of dendritic cells. Oncotarget. (2017) 8:38554–67. doi: 10.18632/oncotarget.v8i24
62. Lee DH, Kim HW. Innate immunity induced by fungal β-glucans via dectin-1 signaling pathway. Int J medicinal mushrooms. (2014) 16:1–16. doi: 10.1615/IntJMedMushr.v16.i1
63. Javmen A, Nemeikaitė-Čėnienė A, Bratchikov M, Grigiškis S, Grigas F, Jonauskienė I, et al. β-glucan from saccharomyces cerevisiae induces IFN-γ Production in vivo in BALB/c mice. In Vivo (Athens Greece). (2015) 29:359–63.
64. Brown GD, Gordon S. Immune recognition of fungal beta-glucans. Cell Microbiol. (2005) 7:471–9. doi: 10.1111/cmi.2005.7.issue-4
65. Brown GD, Gordon S. Immune recognition. A New receptor beta-glucans. Nature. (2001) 413:36–7. doi: 10.1038/35092620
66. Noorbakhsh Varnosfaderani SM, Ebrahimzadeh F, Akbari Oryani M, Khalili S, Almasi F, Mosaddeghi Heris R, et al. Potential promising anticancer applications of β-glucans: a review. Bioscience Rep. (2024) 44:BSR20231686. doi: 10.1042/BSR20231686
67. Meng X, Liang H, Luo L. Antitumor polysaccharides from mushrooms: a review on the structural characteristics, antitumor mechanisms and immunomodulating activities. Carbohydr Res. (2016) 424:30–41. doi: 10.1016/j.carres.2016.02.008
68. Lam K-L, Chi-Keung Cheung P. Non-digestible long chain beta-glucans as novel prebiotics. Bioactive Carbohydrates Dietary Fibre. (2013) 2:45–64. doi: 10.1016/j.bcdf.2013.09.001
69. Geller A, Shrestha R, Yan J. Yeast-derived β-glucan in cancer: novel uses of a traditional therapeutic. Int J Mol Sci. (2019) 20:3618. doi: 10.3390/ijms20153618
70. Aleem E. β-Glucans and their applications in cancer therapy: focus on human studies. Anti-cancer Agents medicinal Chem. (2013) 13:709–19. doi: 10.2174/1871520611313050005
71. Borchers AT, Keen CL, Gershwin ME. Mushrooms, tumors, and immunity: an update. Exp Biol Med (Maywood NJ). (2004) 229:393–406. doi: 10.1177/153537020422900507
72. Kumar R, Afrin H, Bhatt HN, Beaven E, Gangavarap A, Esquivel SV, et al. Mucoadhesive carrier-mediated oral co-delivery of bcl2 inhibitors improves gastric cancer treatment. ACS Appl materials interfaces. (2024) 16:305–17. doi: 10.1021/acsami.3c15226
73. Qi J, Yu J, Li Y, Luo J, Zhang C, Ou S, et al. Alternating consumption of β-glucan and quercetin reduces mortality in mice with colorectal cancer. Food Sci Nutr. (2019) 7:3273–85. doi: 10.1002/fsn3.1187
74. Huber S, Gagliani N, Zenewicz LA, Huber FJ, Bosurgi L, Hu B, et al. IL-22BP is regulated by the inflammasome and modulates tumorigenesis in the intestine. Nature. (2012) 491:259–63. doi: 10.1038/nature11535
75. Tang C, Sun H, Kadoki M, Han W, Ye X, Makusheva Y, et al. Blocking Dectin-1 prevents colorectal tumorigenesis by suppressing prostaglandin E2 production in myeloid-derived suppressor cells and enhancing IL-22 binding protein expression. Nat Commun. (2023) 14:1493. doi: 10.1038/s41467-023-37229-x
76. Kim JH, Seo J, No H, Kuge T, Mori T, Kimoto H, et al. Low-molecular-weight β-1,3-1,6-glucan derived from aureobasidium pullulans exhibits anticancer activity by inducing apoptosis in colorectal cancer cells. Biomedicines. (2023) 11. doi: 10.3390/biomedicines11020529
77. Kopiasz Ł, Dziendzikowska K, Oczkowski M, Harasym J, Gromadzka-Ostrowska J. Low-molar-mass oat beta-glucan impacts autophagy and apoptosis in early stages of induced colorectal carcinogenesis in rats. Int J Biol macromolecules. (2024) 254:127832. doi: 10.1016/j.ijbiomac.2023.127832
78. Binmama S, Dang CP, Visitchanakun P, Hiengrach P, Somboonna N, Cheibchalard T, et al. Beta-Glucan from S. cerevisiae Protected AOM-Induced Colon Cancer in cGAS-Deficient Mice Partly through Dectin-1-Manipulated Macrophage Cell Energy. Int J Mol Sci. (2022) 23:10951. doi: 10.3390/ijms231810951
79. Wilczak J, Prostek A, Dziendzikowska K, Gajewska M, Kopiasz Ł, Harasym J, et al. Beta-glucan as a metabolic regulator in early stage of colorectal cancer-A model study on azoxymethane-treated rats. Oat. Int J Mol Sci. (2024) 25:4635. doi: 10.3390/ijms25094635
80. Xie Y, Shao F, Duan X, Ding J, Ning Y, Sun X, et al. Whole β-glucan particle attenuates AOM/DSS-induced colorectal tumorigenesis in mice via inhibition of intestinal inflammation. Front Pharmacol. (2023) 14:1017475. doi: 10.3389/fphar.2023.1017475
81. Alexander MP, Fiering SN, Ostroff GR, Cramer RA, Mullins DW. Beta-glucan-induced inflammatory monocytes mediate antitumor efficacy in the murine lung. Cancer immunology immunotherapy: CII. (2018) 67:1731–42. doi: 10.1007/s00262-018-2234-9
82. Hersh EM, Gutterman JU, Mavligit GM. BCG as adjuvant immunotherapy for neoplasia. Annu Rev Med. (1977) 28:489–515. doi: 10.1146/annurev.me.28.020177.002421
83. Chen KH, Nguyen N, Huang TY, Lin YJ, Yu YT, Song HL, et al. Macrophage-hitchhiked orally administered β-glucans-functionalized nanoparticles as "Precision-guided stealth missiles" for targeted pancreatic cancer therapy. Advanced materials (Deerfield Beach Fla). (2023) 35:e2304735. doi: 10.1002/adma.202304735
84. Geller AE, Shrestha R, Woeste MR, Guo H, Hu X, Ding C, et al. The induction of peripheral trained immunity in the pancreas incites anti-tumor activity to control pancreatic cancer progression. Nat Commun. (2022) 13:759. doi: 10.1038/s41467-022-28407-4
85. Ina K, Kataoka T, Ando T. The use of lentinan for treating gastric cancer. Anti-cancer Agents medicinal Chem. (2013) 13:681–8. doi: 10.2174/1871520611313050002
86. Nakazato H, Koike A, Saji S, Ogawa N, Sakamoto J. Efficacy of immunochemotherapy as adjuvant treatment after curative resection of gastric cancer. Study Group Immunochemotherapy PSK Gastric Cancer. Lancet (London England). (1994) 343:1122–6. doi: 10.1016/S0140-6736(94)90233-X
87. Toge T, Yamaguchi Y. Protein-bound polysaccharide increases survival in resected gastric cancer cases stratified with a preoperative granulocyte and lymphocyte count. Oncol Rep. (2000) 7:1157–61. doi: 10.3892/or
88. Oba K, Teramukai S, Kobayashi M, Matsui T, Kodera Y, Sakamoto J. Efficacy of adjuvant immunochemotherapy with polysaccharide K for patients with curative resections of gastric cancer. Cancer immunology immunotherapy: CII. (2007) 56:905–11. doi: 10.1007/s00262-006-0248-1
89. Okuno K, Uno K. Efficacy of orally administered Lentinula edodes mycelia extract for advanced gastrointestinal cancer patients undergoing cancer chemotherapy: a pilot study. Asian Pacific J Cancer prevention: APJCP. (2011) 12:1671–4.
90. Segal NH, Gada P, Senzer N, Gargano MA, Patchen ML, Saltz LB. A phase II efficacy and safety, open-label, multicenter study of imprime PGG injection in combination with cetuximab in patients with stage IV KRAS-mutant colorectal cancer. Clin colorectal cancer. (2016) 15:222–7. doi: 10.1016/j.clcc.2016.02.013
Keywords: beta glucan, β-glucan receptor, mechanism, immunotherapy, digestive tract tumors
Citation: Wang M, Pan J, Xiang W, You Z, Zhang Y, Wang J and Zhang A (2024) β-glucan: a potent adjuvant in immunotherapy for digestive tract tumors. Front. Immunol. 15:1424261. doi: 10.3389/fimmu.2024.1424261
Received: 27 April 2024; Accepted: 27 June 2024;
Published: 19 July 2024.
Edited by:
Jeongmin Song, Cornell University, United StatesReviewed by:
Safaet Alam, Bangladesh Council of Scientific and Industrial Research (BCSIR), BangladeshNisha Kannan, Cornell University, United States
Sohyoung Lee, Cornell University, United States
Copyright © 2024 Wang, Pan, Xiang, You, Zhang, Wang and Zhang. This is an open-access article distributed under the terms of the Creative Commons Attribution License (CC BY). The use, distribution or reproduction in other forums is permitted, provided the original author(s) and the copyright owner(s) are credited and that the original publication in this journal is cited, in accordance with accepted academic practice. No use, distribution or reproduction is permitted which does not comply with these terms.
*Correspondence: Junyu Wang, d2p5MDMxNTA2MThAMTYzLmNvbQ==; Anren Zhang, YW5yZW4wMTI0QDE2My5jb20=
†These authors have contributed equally to this work