- 1Division of Hematology/Oncology, Department of Medicine, University of California, San Francisco, San Francisco, CA, United States
- 2Kaiser Permanente San Francisco Medical Center, San Francisco, CA, United States
- 3Center for Tobacco Control Research and Education, University of California, San Francisco, San Francisco, CA, United States
- 4Helen Diller Family Comprehensive Cancer Center, University of California, San Francisco, San Francisco, CA, United States
- 5Division of Cardiology, Department of Medicine, University of California, San Francisco, San Francisco, CA, United States
- 6Fred Hutchinson Cancer Center, Seattle, WA, United States
Electronic cigarettes (e-cigarettes) are thought to pose low risk of cancer because the components of e-cigarette liquid are not carcinogens. We analyzed the effects of the two major components, PG/VG and nicotine, on tumor development in preclinical models. We found that PG/VG promoted tumor cell migration in migration assays and contributed to more aggressive, metastatic, and immunosuppressive tumors in vivo, aggravated by the presence of nicotine. Whole body exposure of mice to PG/VG and nicotine rendered animals more susceptible to developing tumors with high frequencies of infiltrating proinflammatory macrophages expressing IL-6 and TNFα. Moreover, tumor-infiltrating and circulating T cells in e-cigarette exposed mice showed increased levels of immune checkpoints including CTLA4 and PD-1. Treatment with anti-CTLA4 antibody was able to abrogate metastasis with no detrimental effects on its ability to induce tumor regression in exposed mice. These findings suggest that the major components used in e-cigarette fluid can impact tumor development through induced immunosuppression.
Introduction
E-cigarettes deliver nicotine to users by aerosolizing a solution of propylene glycol, glycerin, nicotine and flavoring agents. While e-cigarette use has been linked to cardiovascular, metabolic, pulmonary, oral and other diseases in people, the effects of e-cigarettes on cancer in people is limited (1). Because they do not burn tobacco, e-cigarettes generate much lower levels of combustion-related carcinogens than cigarettes do (2). It is also widely noted that nicotine is not a carcinogen. Propylene glycol and vegetable glycerin (PG/VG) (3) are seen as benign because they are widely used as humectants in food and are generally recognized as safe for ingestion. As a result, e-cigarettes are widely assumed to impose minimal cancer risks (4). Indeed, e-cigarettes have been promoted as a harm reduction alternative to cigarettes among smokers with cancer (5–7).
While not a carcinogen, nicotine has been associated with tumorigenesis in a number of malignancies, mainly through engagement of nicotinic acetylcholine receptors (8, 9). Nicotine can also induce malignant cell cycle transformation through a number of pathways (10–12), including alteration of p53 function (13). Nicotine also has immunomodulatory effects, including suppression of T cell proliferation in vitro (14), engagement of inhibitory pathways in the form of immune checkpoints like PD-1, and blunting of downstream IL-2 signaling in CD8+ T cells (15). Additionally, nicotine has been shown to induce immunosuppressive tumor microenvironments by means of proinflammatory cytokine upregulation (16) or recruitment of protumoral myeloid populations such as M2 macrophages or N2 neutrophils, thereby promoting metastasis and hampering antitumor immunity both in cancer- and immune-intrinsic manners (17–19).
PG/VG, which is not a carcinogen, induces proinflammatory cytokines and extracellular matrix components that contribute to tumor angiogenesis, an effect increased by nicotine (20). In vivo data shows that PG/VG caused T cell immunosuppression and proinflammatory cytokine release, with and additive effect from nicotine (21). Based on these effects, one would expect that exposure to a mixture of PG/VG and nicotine would promote the growth and metastasis of established tumors. Indeed, Pham et al. found that e-cigarette exposure promoted breast cancer and lung metastasis in mice (19). The present study expands this work to assess the in vitro and in vivo effects of exposure of cancer cells to PG/VG and nicotine. We found that exposure of tumor cells to PG/VG and nicotine increased metastases. We also found an immunosuppressive effect upon e-cigarette exposure in the myeloid and lymphoid tumor-infiltrating compartments, including induction of exhaustion markers on T cells. Finally, we show that these exhaustion markers are functional as the tumors could respond to immune checkpoint blockade (ICB). In sum, although not carcinogens, PG/VG and nicotine promote the progression of established cancers.
Materials and methods
E-cigarette liquids
E-cigarette liquids were prepared the same for in vitro exposure of cell cultures and in vivo whole-body exposure of mice. The PG/VG solution was prepared by mixing PG (99.5% USP grade, CAS 7–55-6, density 1.26 g/ml) and VG (99.7% USP grade, CAS 56–85-1, density 1.04 g/ml) (both from MyFreedomSmokes, https://myfreedomsmokes.shop/) at a 1:1 volume ratio. Nicotine (N3876, Sigma) was added to the PG/VG mix to the desired experimental concentration (0, 6 and 36 mg/ml). All solutions were sterile-filtered through 0.45 μm filters before exposure to cells or mice.
Preconditioning of tumor cells+ in culture prior to subcutaneous challenge was performed for 14 days at 37 C in 6-well plates, with control groups being incubated only in glucose, pyruvate-supplemented Dulbecco’s Modified Eagle Medium (DMEM) containing 10% fetal bovine serum for the same amount of time. Conditioned media were replaced every 2 days.
In vitro scratch assays
To assess the impact of PG/VG and nicotine on tumor cell migration, scratch assays were performed by adapting a protocol described elsewhere (22). Briefly, freshly passaged colorectal carcinoma MC38 cells were rested overnight at 37C. Then, 7 x 104 cells were seeded into each side of 2-well coculture inserts containing a ‘scratch’ or cell-free gap (80209, Ibidi) and incubated at 37C overnight. Then, after checking that a monolayer was formed inside the inserts, the inserts were removed with sterilized forceps, cells washed once with Phosphate Buffered Saline (PBS), then culture media added containing PG/VG and nicotine, and plates incubated at 37C. PG/VG alone was dissolved in PBS at concentrations ranging from 2.5 to 20 μM. In wells exposed to PG/VG + nicotine, PG/VG was kept at 2.5 μM for all nicotine concentrations. Cell migration was then monitored by time lapse microscopy (Incucyte). Migration rates were quantified through Incucyte built-in confluency calculator as percentage of scratch area -defined by a diameter between the fronts of each monolayer- covered by cells. After 7 days in culture, viable cells were quantified in a Vi-CELL counter (Beckman Coulter).
Animal studies, cell lines and in vivo imaging
8- to 10-week-old male wildtype C57BL/6J mice (000664, Jackson) were used in mouse experiments. All cell lines used in this study were certified yearly by the STR Profiling method available through ATCC at https://www.atcc.org/services/cell-authentication/mouse-cell-str-testing. Latest certification date was January 2023. We conducted both systemic and subcutaneous in vivo tumor studies. In systemic studies, tumor cells are injected via tail vein into bloodstream. They home to lungs to generate ‘systemic tumors’. In subcutaneous studies, tumor cells are injected intradermally on the flank of mice, where they settle and form a solid tumor mass. For subcutaneous in vivo tumor studies, 0.25 million untreated or pretreated murine melanoma B16, 0.5 million colorectal carcinoma MC38, or 1 million prostate adenocarcinoma TRAMP-C2 cells were injected on the right flank of mice and tumors were measured twice a week with a digital caliper (Fisher). Tumor volume was obtained with the formula V = L (length) × W (width) × W × π/6, where L was the higher measure, and W was the lower measure. Endpoint volume was set at 2,000 mm3.
For systemic tumor experiments, 5 x 105 luciferase-expressing murine colorectal carcinoma MC38 cells (kindly donated by Jeff Bluestone) were injected intravenously through the tail vein of each animal (n=5) on day 0. In preconditioning experiments, cells were harvested from 6-well plates after the 14-day preconditioning plus 1–2 post-thaw passages. In whole body exposure experiments, cells were harvested after 1–2 post-thaw passages. In both cases, cells were counted with a Vi-CELL cell counter (Beckman Coulter) at a 1:60 dilution and prepared at 5M cells/ml in DPBS, 100 µl of which were used for intravenous injections per animal. Tumor progression was monitored using an IVIS Spectrum in vivo imaging device (Xenogen). Briefly, animals were injected i.p. with 0.2 ml of DPBS containing 3 mg D-Luciferin (88294, Thermo) and were imaged after a 3-minute incubation. Images were processed and bioluminescence radiance was quantified using Living Image version 4.7.4.20726.
In immune checkpoint blockade (ICB) studies, animals were implanted with 0.5 million MC38 cells, were randomized into treatment groups and were treated intraperitoneally on days 3, 6 and 9 with 200 μg isotype control (IgG2k/a, BioXCell) or anti-CTLA4 (clone UC-10, BioXCell).
All mice were maintained at the UCSF vivarium and received food and water ad libitum.
Whole body e-cigarette exposure
As described previously (23), to assess the impact of inhaled e-cigarette aerosol in the tumorigenesis of systemically implanted tumors, mice were exposed in a chamber to e-cigarette mixes containing PG/VG and 0, 6 or 36 mg/ml nicotine for 1h daily (5 days/week) for 4 weeks, a scheme that has been already reported to show differences in vivo (24). In particular, 36 mg/ml yielded nicotine concentrations similar to that of a standard cigarette smoking model (23). E-cigarette liquids were aerosolized with an atomizer designed for vaping oils (1.8 Ω cotton coil, Aspire) using a Gram Universal Vaping Machine (Gram Research) at 9.4 watts. It was operated with Gram VM software, version 4.15.25. Coil power was set at 4V. Puff volume was 80 ml, drawn over 4 seconds into a syringe through an electronically controlled three-way valve, then injected to vaping chamber over 2 seconds. In order to fill the vaping chamber with aerosol, 10 puffs were initially injected over approximately one minute, followed by 110 puffs over one hour. The chamber was evacuated at a constant rate of 2.0 liters/minute during the exposure using a calibrated flowmeter (Dwyer, Michigan City, IN, US) to draw in a mixture of fresh aerosol and room air. Upon completion of 110 puffs, the vacuum outflow speed was increased to clear the chamber over a period of 5 minutes. After exposure, mice were removed from the chamber. After 4 weeks, whole-body exposure was ceased and 5 x 105 Luc-MC38 cells were injected intravenously onto mice and tumor growth was monitored by in vivo bioluminescence as described above. Air-exposed animals were included as control.
Tissue processing
Lungs and spleens from surviving whole body exposure animals were surgically removed from three mice with sterilized equipment 22 days after tumor injection. These mice were not considered for survival analysis. Lungs were weighed and perfused with PBS. Metastatic nodules were counted. Then, they were mechanically dissociated with scalpel blades and digested to single cell suspensions by incubation for 1h at 37C in tumor digestion media containing DMEM, 10%FBS, 2 mg/ml Collagenase IV (C5138, Sigma-Aldrich) and DNAse I (D5025, Sigma-Aldrich). Spleens were mechanically dissociated through a 70 μm filter into a 50 ml conical tube with cold PBS. Lung and spleen lysates were filtered through a 100 μm filter into 50 ml conical tubes and filled with cold PBS, followed by centrifugation at 450 g for 5 minutes at 4C. Supernatants were discarded and pellets were resuspended in 5 ml ACK Lysing Buffer (118–156-101, Quality Biological), mixed well and kept on ice for 5 minutes. Lysis was stopped by filling the tubes with cold PBS. Samples were centrifuged again and finally resuspended in 1 ml cold PBS. Viable cells for downstream use were counted in a Vi-CELL cell counter (Beckman Coulter) at a 1:60 dilution.
Flow cytometry
Single cell suspensions were first incubated with 1:100 Zombie NIR (L34976, Life Technologies) for 10 minutes in the dark at room temperature. After washing, surface staining including 1:50 Fc Block (70–0161-U500, Tonbo Biosciences) was performed for 30 minutes on ice. Cells were fixed using the eBioscience FoxP3 kit (00–5523-00, Life Technologies) and intracellular staining was added to samples for 30 minutes on ice before final wash with FACS Buffer (PBS containing 2% FBS and 1mM EDTA). Samples were run on a LSR Fortessa X-50 (Becton Dickinson). Data were analyzed by FlowJo 10.7 (Tree-Star). A detailed list of mouse antibodies used can be found in Supplementary Table 1.
For exhaustion marker co-expression, Boolean gating of CTLA4, TIM3, LAG-3 and PD-1 expression was applied on gated splenic CD8+ T cells at FlowJo.
Data analysis and statistics
Most results were analyzed using regression with PG/VG concentration or presence (coded 0/1 for no/yes) and nicotine concentration (in mg/mL) or presence (coded 0/1) using Stata 15 mixed. Tumor growth curves were also analyzed on ln(tumor volume) using mixed, including PG/VG and nicotine interactions with time (to allow for slope changes) and nesting days with mouse to account for repeated measures. A quadratic term for time (days2 was included the regressions to allow for curvature over time to improve residual patterns). Estimates were computed using restricted maximum likelihood (REML) and unstructured covariance matrices.
The presence of metastases was analyzed for all cell types using a single logistic regression including variables for PG/VG (0/1), nicotine concentration divided by 36 mg/mL (so 1 corresponded to 36 mg/mL), and two effects-coded dummy variables to allow for differences in cell type.
Metastases rates in anti-CTLA4 experiments were analyzed by Fisher Exact tests.
Kaplan-Meier survival curves were analyzed using Mantel-Cox log rank tests in GraphPad Prism 10.1.2, first comparing all groups, then only comparing the PG/VG and nicotine groups.
Ethics statement
All experiments involving animals performed in this study were reviewed and approved by the Institutional Animal Care and Use Committee (IACUC) at UCSF under protocol number AN202446.
Data access statement
All data presented in this manuscript and its related Supplementary files are available from the corresponding author upon reasonable request.
Results
PG/VG promotes tumor cell motility while nicotine attenuates tumor cell duplication in vitro
To test whether e-cigarette PG/VG and nicotine affect tumor cell migration in vitro, we adapted a protocol developed by Liang and colleagues (22) and exposed adjacent monolayers of colorectal MC38 tumor cells, separated by a cell-free gap or ‘scratch area’, to e-cigarette chemicals, and quantified cell invasion into the scratch area over time (Figure 1A, Supplementary Figure S1 and Table 1). Exposure to PG/VG showed a significant dose-dependent increase in the ability of tumor cells to occupy the scratch area after 24h, independent of the presence of nicotine (Figures 1B, C). After 7 days of culture, PG/VG without nicotine also showed a significant dose-dependent increase in the number of tumor cells. However, the addition of nicotine led to reduced absolute number of viable tumor cells (Figure 1D and Supplementary Figure S1). These results indicate that PG/VG can be a promoter of tumor cell proliferation and invasiveness in vitro, with nicotine partially blunting proliferation while not affecting tumor cell motility.
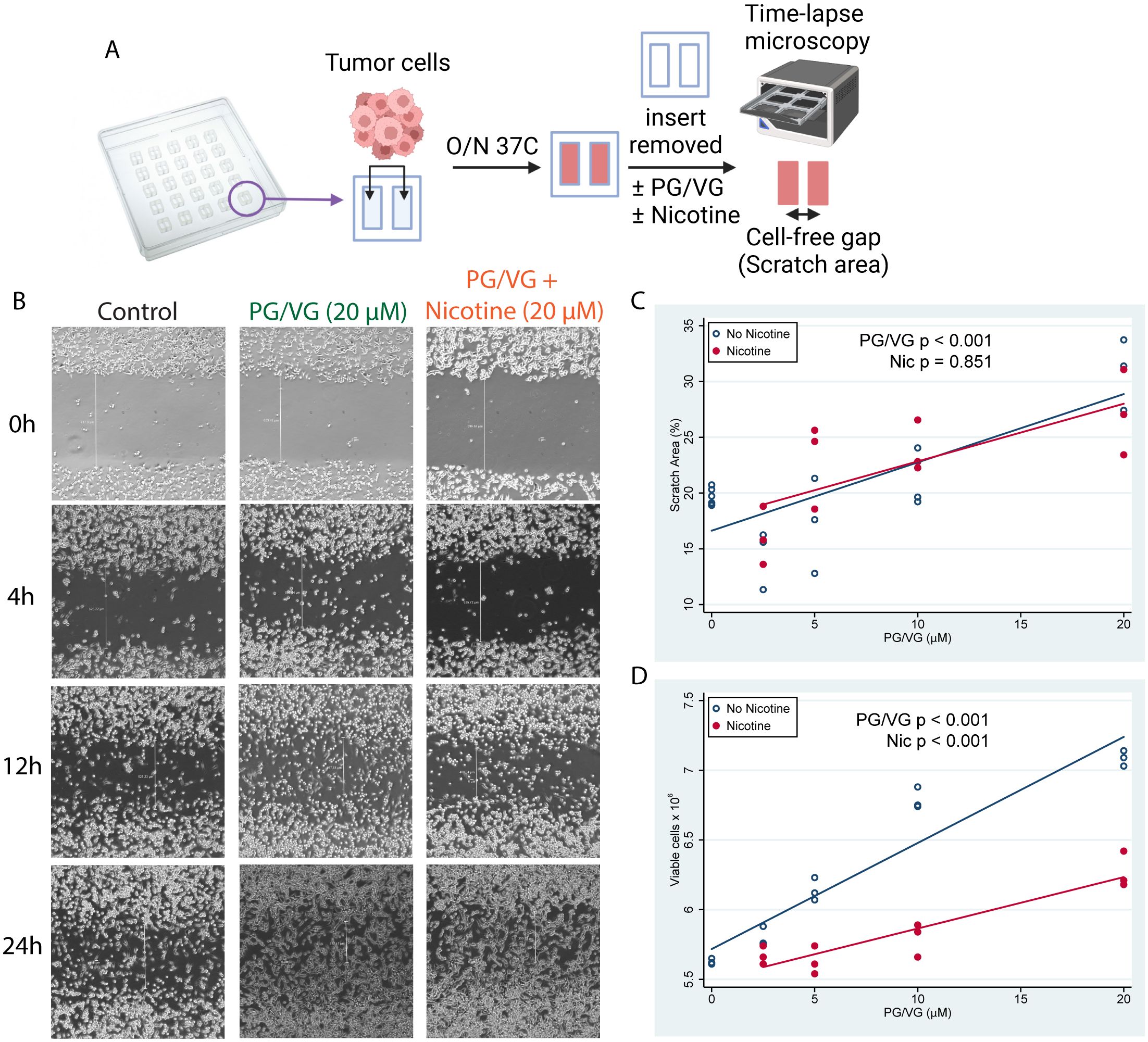
Figure 1. E-cigarette chemicals promote tumor cell migration in vitro. (A) Experimental design for cell scratch assays. Briefly, tumor cells were seeded into inserts containing a cell-free gap (scratch area) between two sides and were cultured overnight in the presence of concentrations of PG/VG from 0 to 20 µM with or without nicotine. Then, inserts were removed and invasion of the scratch area was monitored by time-lapse microscopy. (B) Representative microscope images (40X augmentation) taken at 0, 4, 12 and 24h from 1 of 3 experiments are shown. Vertical white lines show distance from cell fronts in μm, used to calculate percentage of covered scratch areas. (C) Percent of scratch area covered at 24h after insert removal fell by 0.58 (95% CI 0.39, 0.77) %/µM PG/VG (p<.001; Table 1) but was not affected by the presence of nicotine (p=.851). We also ran the model including the PG/VG x nicotine interaction and found no significant interaction (p=0.638). (D) Viable cell counts after 7 days increased by 0.060 (0.048, 0.071) x106 cells/µM PG/VG (p<.001; Table 1) and dropped by -0.56 (-0.72, -0.39) x 106 in the presence of nicotine (p<0.001). We also ran the model including the PG/VG x nicotine interaction and found a significant interaction (P<0.001). The PG/VG effect was about the same 0.076 (0.064, 0.088) (p<.001), whereas the nicotine main effect dropped to -0.22 ± .11 (-0.42, -0.02) (p=.029) with the interaction term being -.039 (-0.057, -0.021) (p<.001).
PG/VG and nicotine exposure leads to increased metastases in vivo
To test the relevance of our findings in vivo, we implanted three tumor cell lines (melanoma B16, colorectal MC38, and prostate TRAMP-C2) that had been previously incubated with PG/VG or nicotine into mice subcutaneously and monitored tumor growth (Figure 2A, B). Unlike the observed in vitro effects on tumor cell migration, there was no observable impact in endpoint primary tumor volumes in any of the models (Figures 2C, Table 2). Interestingly, PG/VG, but not nicotine, significantly increased tumor growth rate in the B16 melanoma model, consistent with the increased proliferation seen in vitro, with bigger differences over time (Supplementary Figure S2). We found peritoneal metastases occurring in 20% (6/30, B16), 50% (14/28, MC38) and 40% (12/30, TRAMP-C2) of mice harboring PG/VG+nicotine-exposed tumors, as well as in 20% (3/15, B16), 40% (6/15, MC38) and 28.6% (4/14, TRAMP-C2) for mice harboring PG/VG-exposed tumors, in striking contrast with the absent (0/15, B16 and MC38) or rare metastases (1/15, TRAMP-C2) unexposed tumors (Figure 2D). Our statistical analysis showed significant and independent effects of both PG/VG and nicotine on the increased occurrence of metastases with PG/VG having a bigger effect (Figure 2D). These findings are consistent with the ability of PG/VG to promote tumor cell invasiveness in vitro, but also highlight the effects e-cigarette components can have in tumor dissemination.
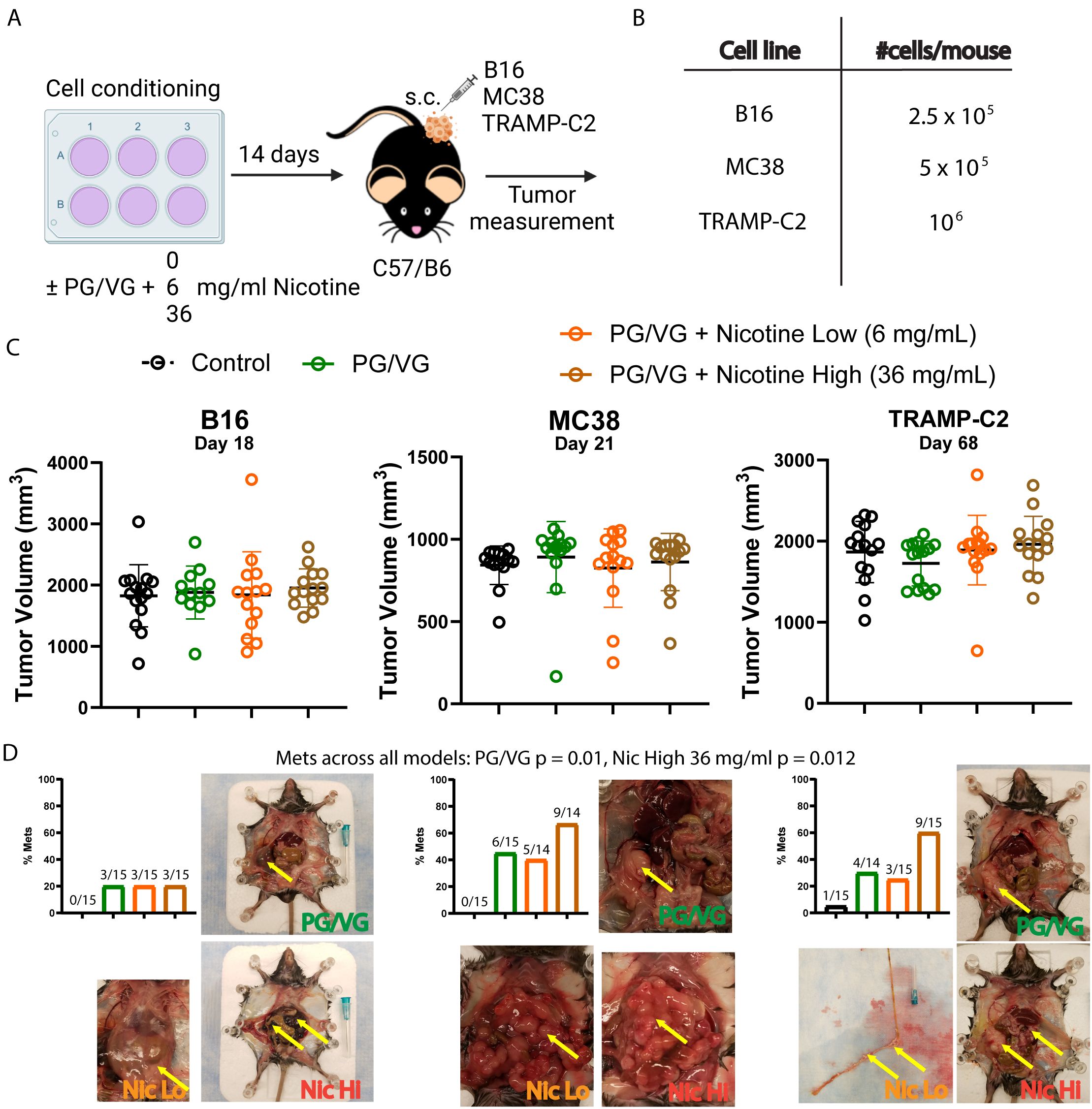
Figure 2. Preconditioning with PG/VG increases metastasis in different subcutaneous tumor models. (A) Experimental scheme to test the impact of PG/VG and nicotine on the outcome of subcutaneous tumors. Tumor cells were cultured in the presence of 2.5 μM PG/VG with concentrations of nicotine of 0, 6 and 36 mg/ml for 14 days. Then, preconditioned cells were implanted subcutaneously in the right flanks of wildtype C57/B6 mice (5 mice per experiment, n=3 experiments). Tumors were measured twice weekly and animals were inspected for metastases after euthanization. (B) Viable cells implanted per mouse for each tumor model: melanoma B16, colorectal MC38 or prostate TRAMP-C2. (C) Endpoint tumor volumes for B16, MC38 and TRAMP-C2 models. Error bars represent standard error mean (SEM). Neither PG/VG nor nicotine affected tumor volume (B16: PG/VG p=0.876, nicotine p=0.601; MC38: PG/VG p=0.740, nicotine p=0.914; TRAMP-C2, PG/VG p=0.500, nicotine p=0.130; Table 2). (D) Bar plots showing metastasis rates for each subcutaneous tumor model. Logistic regression showed increased odds of metastases associated with exposure to PG/VG (14.9; 95% CI 1.9–116.7; p=0.010) and nicotine (2.9; 1.3–6.9, p=0.012 at 36 mg/mL), controlling for tumor type. Representative pictures with peritoneal metastases are shown for mice exposed to PG/VG with or without nicotine for each tumor model. Control animals (black bars) were not exposed to PG/VG or nicotine. Yellow arrows indicate highly metastasized areas. Metastasis was extremely rare in mice not exposed to PG/VG or nicotine (0/15 in B16 and MC38, 1/15 in TRAMP-C2).
Tumor cell exposure to PG/VG leads to more aggressive systemic tumors without an additive effect from nicotine
We utilized the luciferase-expressing MC38 (Luc-MC38) colorectal cancer tumor cells to longitudinally monitor tumor progression by bioluminescence imaging (Figure 3A). The injected tumor cells deposit in the lungs and form tumoral niches, constituting a model for lung metastasis. By day 15 after tumor cell injection, PG/VG-exposed tumor cells showed significantly increased luminescence radiance compared to the control group, evidencing more tumor burden. The addition of nicotine did not show a statistically additive effect over PG/VG (Figures 3B, C and Supplementary Figure S3). Exposure to PG/VG was also significantly associated with decreased survival rates compared to unexposed mice, again with no additive effect of nicotine (Figure 3D). These results reinforce our previous findings involving the enhanced invasiveness of cells exposed to PG/VG.
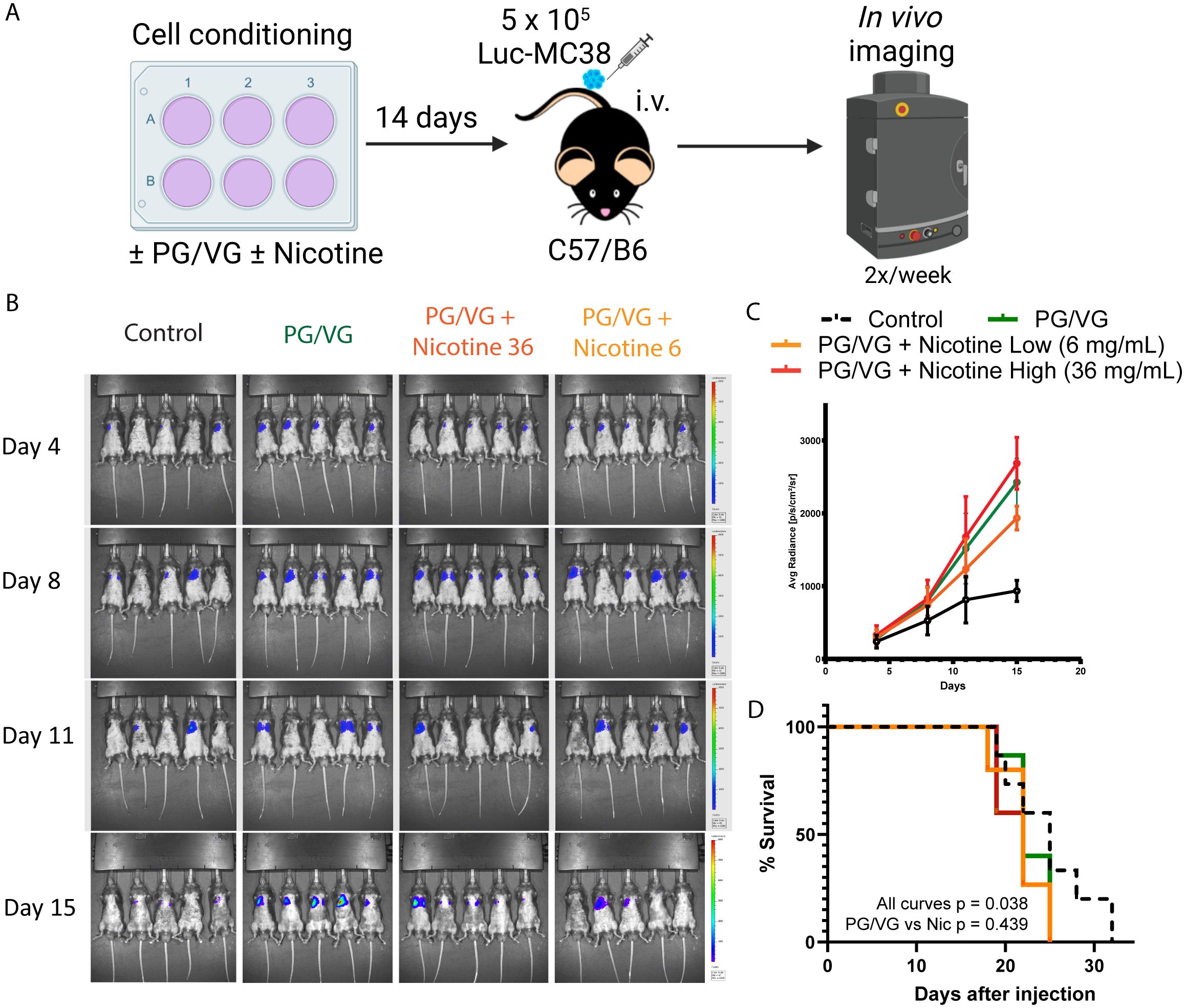
Figure 3. Accelerated tumor progression upon PG/VG preconditioning. (A) Experimental scheme to test the impact of PG/VG and nicotine in a model of disseminated cancer. Luciferase-expressing colorectal cancer MC38 (Luc-MC38) cells were cultured in the presence of PG/VG with concentrations of nicotine of 0, 6 and 36 mg/mL for 14 days. Then, 5 x 105 preconditioned Luc-MC38 cells were injected intravenously in the tail veins of wildtype C57/B6 mice (n=5 per experiment, n=3 experiments). Tumor bioluminescence was monitored twice weekly for 15 days. (B) Representative whole body bioluminescence images on days 4, 8, 11 and 15 post-implantation is shown. (C) Time-course measurement of tumor bioluminescence expressed as Average Radiance for mice involved in experiments described in (A). Error bars represent SEM. (D) Kaplan-Meier survival curves for the 3 experiments. There were significant differences between all four curves (p=0.038, by log rank test). There was no significant difference between the PG/VG, PG/VG plus low nicotine, and PG/VG plus high nicotine curves (p=0.439).
Vaped PG/VG and nicotine lead to increased tumor progression
We performed whole-body exposure experiments in which mice inhaled aerosolized e-cigarette liquids 1 hour daily for 4 weeks. Following this exposure, we injected Luc-MC38 colorectal cancer cells intravenously (Figure 4A). In line with the preconditioning experiments, exposure to inhaled PG/VG led to a higher tumor burden as early as day 13 post tumor implantation. Nicotine did not modulate this effect (Figures 4B, C and Supplementary Figure S4, Table 1). Again, this increase in tumor aggressiveness translated into significantly diminished survival rates for mice exposed to PG/VG that was not affected by adding nicotine, with first deaths occurring as early as day 18 post implantation (Figure 4D). We harvested the lungs from mice for downstream analysis in each of these studies at day 22 after implantation for further characterization. We found significantly increased metastatic nodule counts in lungs from PG/VG- and nicotine-exposed mice, reaching over 200 nodes in a pair of lungs (Figures 4E, F). Nicotine exposure also led to significantly increased lung weight, but PG/VG alone had no apparent effect (Figure 4G and Supplementary Figure S4, Table 1).
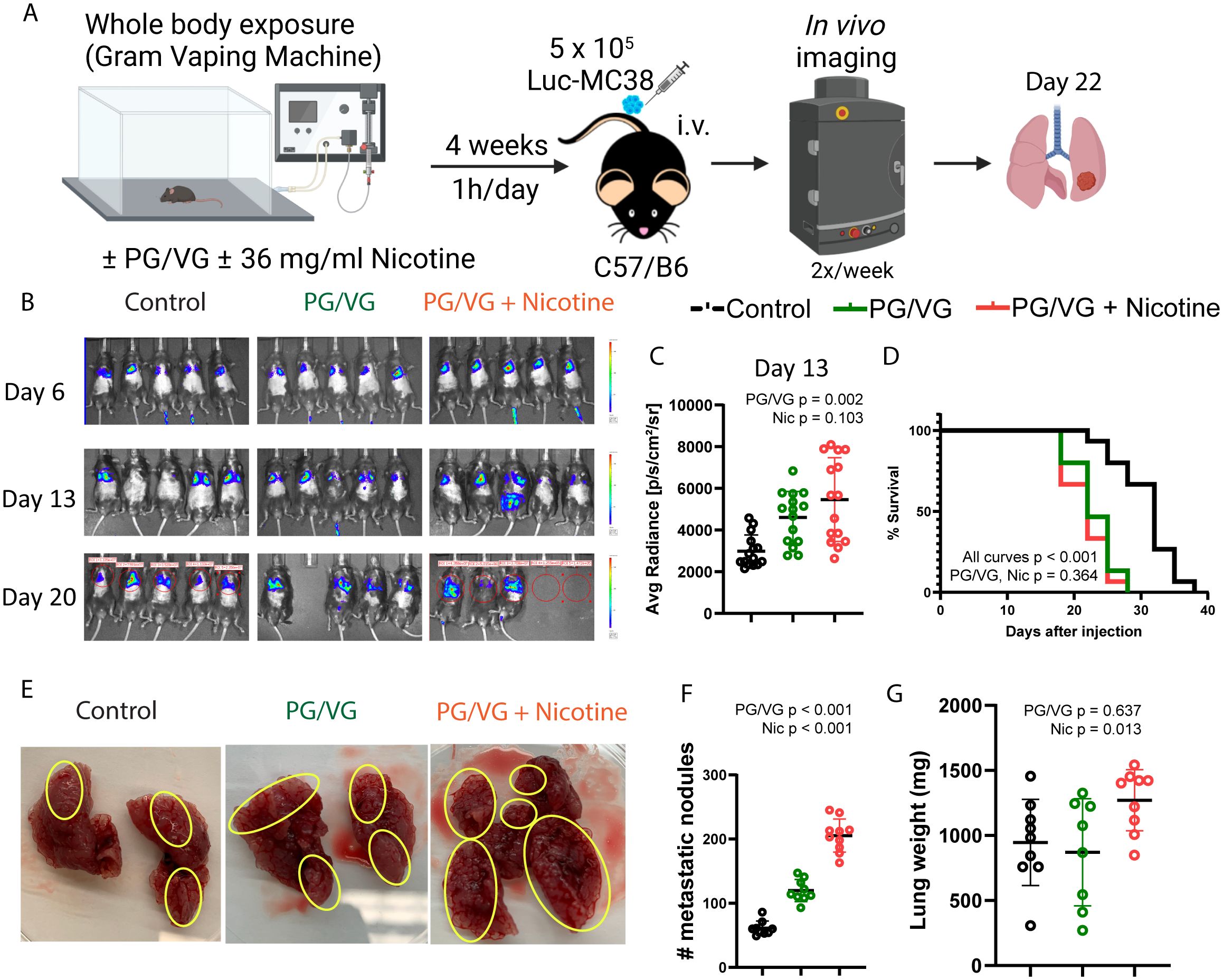
Figure 4. Whole body exposure of mice e-cigarette aerosol leads accelerated tumor growth and more aggressive metastasis. (A) Experimental scheme for whole body exposure experiments. Mice were exposed to e-cigarette aerosol with 2.5 µM PG/VG and 0 or 36 mg/ml nicotine for 1h daily for 4 weeks. Then, mice (n=5 per experiment, n=3 experiments) were challenged intravenously with 5 x 105 Luc-MC38 cells. Tumor bioluminescence was monitored twice weekly. Lungs from three mice per experimental condition were harvested on day 22 post-implantation, weighed, inspected for metastasis burden and immunophenotyped by flow cytometry. (B) Representative whole body bioluminescence images on days 6, 13 and 20 post-implantation is shown. (C) Tumor bioluminescence on day 13 after implantation expressed as average photon radiance was significantly increased by PG/VG (p=0.002; Table 1) but not nicotine (p=0.103). (D) Kaplan-Meier survival curves from whole body experiments described in (A). There were significant differences between all three curves (p,0.001, by log rank test). There was no significant difference between the PG/VG and PG/VG plus nicotine curves (p=0.364), suggesting that the survival was reduced by exposure to PG/VG with no additional effect of nicotine effect. (E) Representative images from surgically extracted lungs 22 days after tumor cell injection. Yellow circles indicate areas with metastatic MC38 nodes. (F) Metastatic node count from day 22 lungs increased significantly with exposure to PG/VG (p<0.001; Table 1), with a further increase when nicotine was added (p<0.001). (G) Lung weight on day 22 after tumor injection was not affected by PG/VG (p=0.637; Table 1), but increased with the addition of nicotine (p=0.013).
Vaped PG/VG and nicotine lead to immunosuppression
Because anti-tumor immunity is important in suppressing tumor progression, we performed flow cytometry on dissociated cells from the lungs at day 22 to assess how exposure modulates the tumor immune microenvironment. Lungs from nicotine-exposed mice possessed significantly increased macrophage infiltration, representing up to 70% of all CD45+ immune cells of (Figure 5A and Supplementary Figure S5, Table 1). Exposure to PG/VG aerosol also led to significantly decreased frequency of CD8+ T cells, which was minimally worsened with the addition of nicotine (Figure 5B and Supplementary Figure S5). Exposure to PG/VG aerosol also induced minimal IL-6 production in lung-infiltrating macrophages. We did not observe significant differences in macrophage TGFβ across groups (Supplementary Figure S5). However, lung-infiltrating macrophages from nicotine-exposed mice expressed significantly higher levels of IL-6 and TNFα (Figures 5C-F and Supplementary Figure S5), indicating that nicotine helps to drive inflammation within the TME. We also observed significantly higher TNFα levels in lung-infiltrating CD8+ T cells from nicotine-exposed mice. Combined with lack of proliferation (Ki67-) or reduced expression of canonical markers for antitumoral responses such as interferon gamma (IFNγ) or cytolytic Perforin (PRF1) in these CD8+ T cells, this suggests impaired antitumoral T cell fitness and contribution to protumoral inflammation (Supplementary Figure S5). Strikingly, exposure to PG/VG alone or in combination with nicotine led to significantly higher frequencies of exhausted PD-1-expressing, Ki67-negative, CD8+ T cells with impaired cytotoxicity (Figures 5G-J and Supplementary Figure S5). Notably, CD4+FoxP3+ T regulatory (Treg) frequencies did not vary significantly between groups, although an increasing trend was observed upon nicotine exposure (Supplementary Figure S5). Together, these findings indicate that PG/VG alone elicits immunosuppression, but when combined with nicotine, induces inflammation that may enhance tumor dissemination.
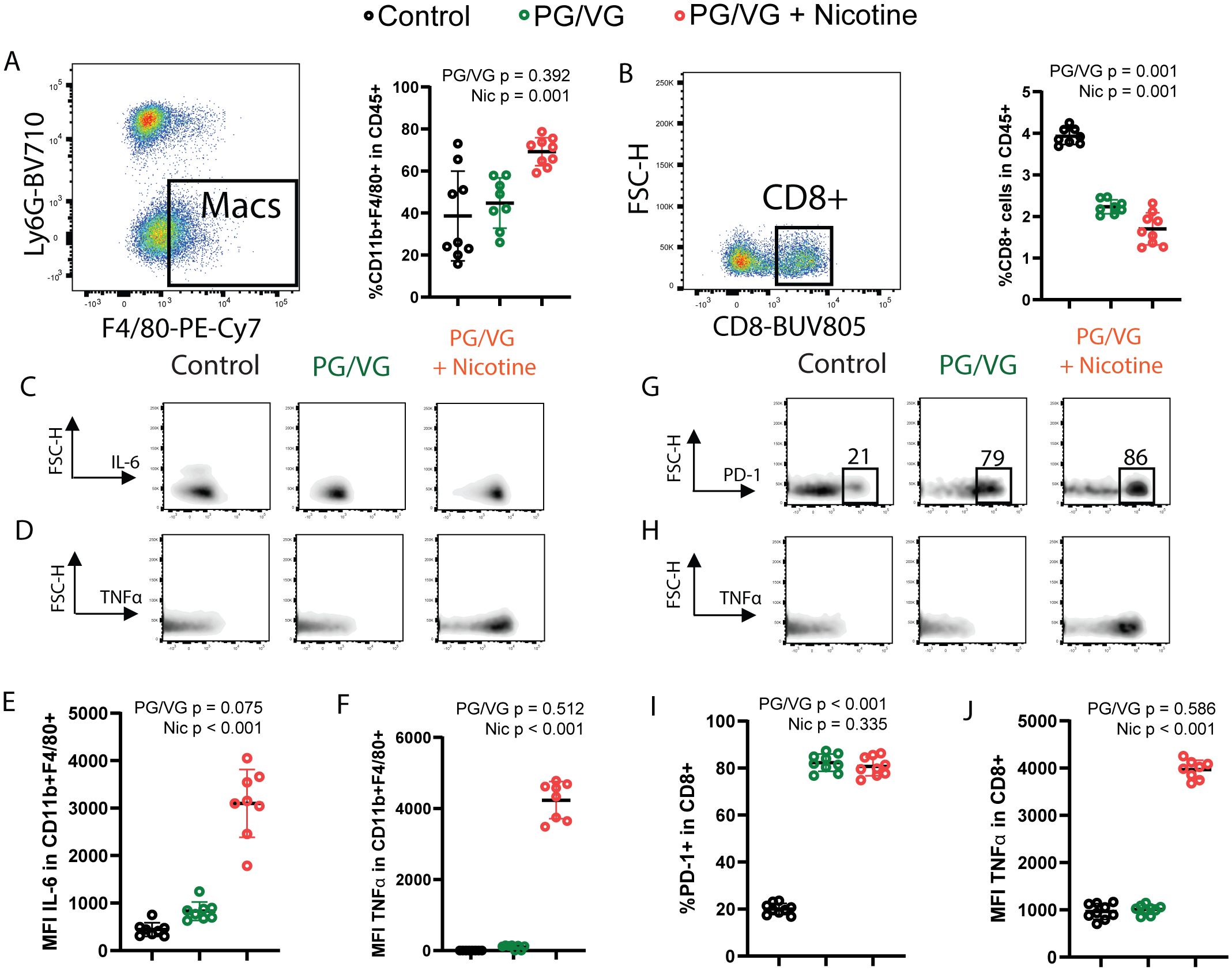
Figure 5. Whole body exposure of mice e-cigarette aerosol leads to increased myeloid and lymphoid immunosuppression in the tumor microenvironment. (A) Left, representative flow cytometry pseudocolor dot plot showing gating on lung-infiltrating macrophages from animals exposed to PG/VG with or without 36 mg/ml nicotine as described in Figure 4A. Right, lung-infiltrating macrophage frequency within gated live CD45+ immune cells was not affected by PG/VG (p=0.392; Table 1) but increased with the addition of nicotine (p=0.001). (B) Left, representative flow cytometry pseudocolor dot plot showing gating on lung-infiltrating CD8+ T cells. Right, lung-infiltrating CD8+ T cell frequency within gated live CD45+ immune cells fell significantly with exposure to PG/VG (p<0.001; Table 1) and about one-third more with the addition of nicotine (p<0.001). (C) Representative flow cytometry density plots showing IL-6 expression in gated lung-infiltrating macrophages. (D) Representative flow cytometry density plots showing TNFα expression in gated lung-infiltrating macrophages. (E) Mean fluorescence intensity of IL-6 in gated lung macrophages was not affected significantly by PG/VG (p=0.075; Table 1) but increased significantly with the addition of nicotine (p<0.001). (F) Mean fluorescence intensity of TNFα in gated lung macrophages was not affected significantly by PG/VG (p=0.512; Table 1) but increased significantly with the addition of nicotine (p<0.001). (G) Representative flow cytometry density plots showing PD-1 expression in gated lung-infiltrating CD8+ T cells. (H) Representative flow cytometry density plots showing TNFα expression in gated lung- infiltrating CD8+ T cells. (I) Percentage PD-1+ cells in gated lung CD8+ T cells was significantly higher in the presence of PG/VG (p<0.001; Table 1) but not affected by addition of nicotine (p=0.335). (J) Mean fluorescence intensity of TNFα in gated lung CD8+ T cells was not significantly different in the presence of PG/VG (p=0.586; Table 1) but significantly increased with the addition of nicotine (p<0.001).
Tumors exposed to PG/VG and nicotine are responsive to immune checkpoint inhibition
Given the observed impact of PG/VG and nicotine on the immune landscape of tumors, we analyzed the circulating T cell compartment for expression immune checkpoints to assess for systemic immune effects. Despite not showing differences in frequencies across groups (Supplementary Figure S6), splenic CD8+ T cells from mice exposed to PG/VG and nicotine expressed significantly higher levels of the immune checkpoints CTLA4, PD-1 and TIM3, while maintained comparable levels of LAG-3 to unexposed mice (Figure 6A). To assess the degree of exhaustion in these cells by co-expression of multiple immune checkpoints, we applied Boolean gating and found that 10.6% of PG/VG-exposed splenic CD8+ T cells showed co-expression of all 4 immune checkpoints, significantly doubling the levels on control CD8+ T cells (5.22%). Moreover, the presence of nicotine showed a significant additive effect, elevating circulating CTLA4+ PD-1+ TIM3+LAG-3+ CD8+ T cells to 17.34% within the CD8 compartment (Figures 6B, C), in line with the higher levels of exhaustion detected in the tumors.
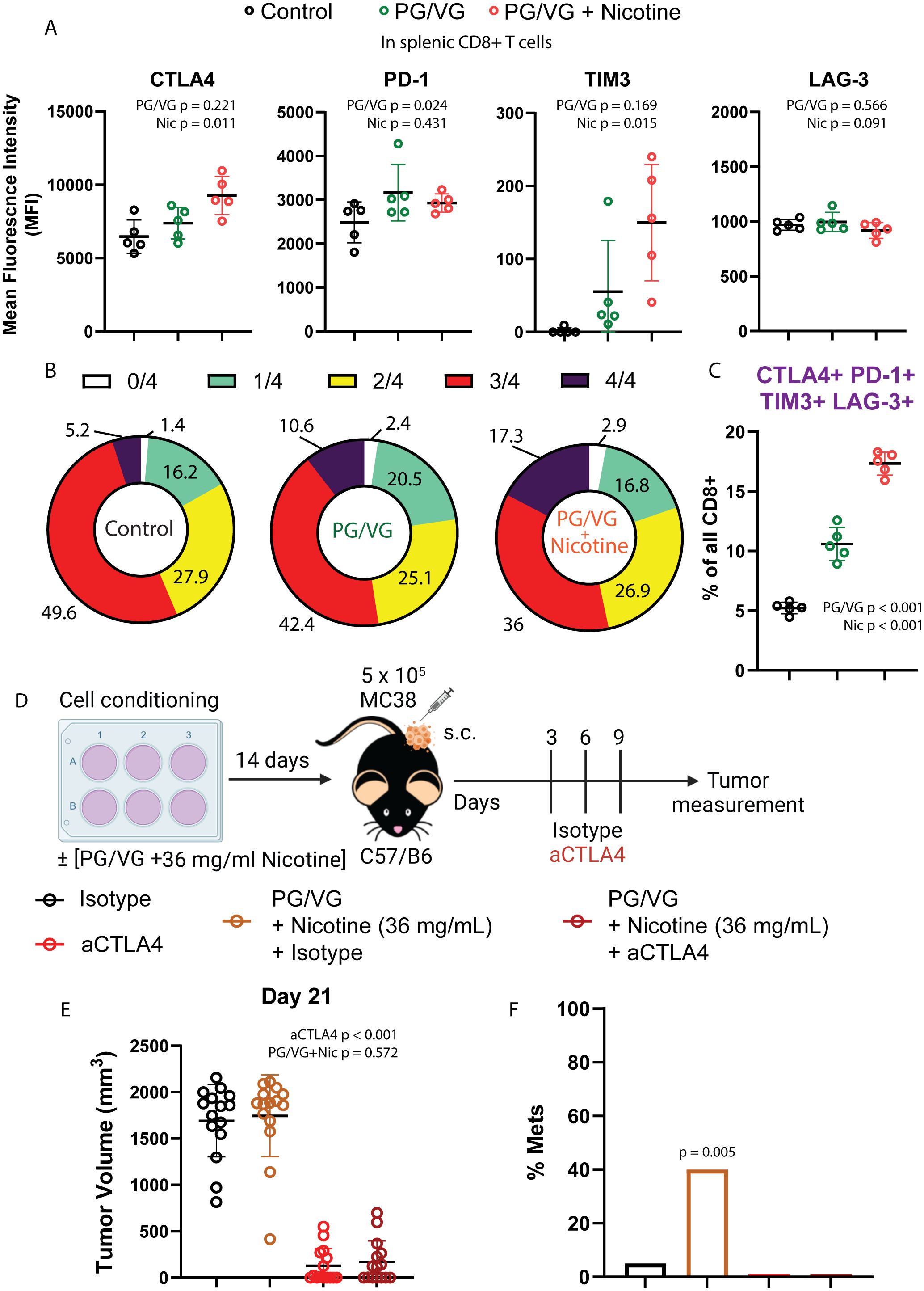
Figure 6. Tumors exposed to proinflammatory e-cigarette components are responsive to immune checkpoint blockade. (A) Mean fluorescence intensity of T cell immune checkpoints CTLA4, PD-1, TIM3 and LAG-3 on splenic CD8+ T cells from mice exposed to PG/VG with or without 36 ml/mg nicotine as described in Figure 4A. CTLA4 and TIM3 were significantly elevated only in the presence of nicotine (p=0.011, p=0.015 respectively). PD-1 was significantly elevated in the presence of PG/VG (p=0.024) with no nicotine effect (p=0.431). No significant differences were found for LAG-3 expression. Summarized results can be found in Table 1. (B) The frequency of immune checkpoint co-expression (none (0), any given one (1), any combination of 2 (2), any combination of 3 (3) or all markers (4)) is shown in splenic CD8+ T cells as assessed by flow cytometry Boolean gating. (C) Percentage of quadruple-positive CTLA4+TIM3+LAG-3+PD-1+ splenic CD8+ T cells. Significantly elevated quadruple-positive cells were found in the presence PG/VG (p<0.001, Table 1) with a significant additive nicotine effect (p<0.001). (D) Experimental scheme to test the effect of immune checkpoint inhibition in e-cigarette preconditioned subcutaneous tumors. After preconditioning MC38 cells in the presence of 2.5 μM PG/VG with or without 36 mg/ml nicotine for 14 days, mice (n=5 per experiment) were implanted with tumors subcutaneously. Starting 3 days after implantation., mice were treated with intraperitoneal anti-CTLA4 (aCTLA4) or the relevant isotype control (IgG2k/a) on days 3, 6 and 9 after implantation. (E) Endpoint tumor volumes. Error bars represent standard error mean (SEM). Treatment with anti-CTLA4 showed a significant effect on tumor volume (p<0.001; Table 2) but not observed for PG/VG+nicotine (p=0.572). No significant interaction between anti-CTLA4 treatment and presence of PG/VG+nicotine was observed (p=0.934). (F) Metastasis rates: 6/15 (40%) animals with PG/VG-preconditioned MC38 tumors showed peritoneal metastases, which were absent (0/15) in both anti-CTLA4-treated groups and nearly absent (1/15) in control mice. There was no significant difference in metastases among these three groups (p=0.762 by Fisher Exact Test (p=0.762) and a significant increase in the PG/VG+nicotine mice compared to the others (p=0.005 by Fisher Exact Test).
To examine whether these immune checkpoint are limiting antitumor responses, we tested the sensitivity of e-cigarette-exposed subcutaneous tumors to immunotherapy in the MC38 model (25) by treating mice with the immune checkpoint inhibitor anti-CTLA4 (Figure 6D). In this model, anti-CTLA4 therapy prevented tumor growth independently of the presence of PG/VG + 36 mg/mL nicotine (Figure 6E and Supplementary Figure S6, Table 2). Notably, anti-CTLA4 treatment completely prevented the appearance of peritoneal metastases in e-cigarette-exposed animals (Figure 6F). These findings show that CTLA-4 represents a functional checkpoint in this setting.
Discussion
Even though neither PG/VG nor nicotine are carcinogens (i.e., tumor initiators), we found both in vitro and in vivo, that exposure to these compounds promotes tumor growth and occurrence of metastasis across multiple preclinical tumor models. PG/VG stimulates these processes in a dose-dependent manner and nicotine often, but not always, amplifies these effects.
Our results are consistent with the one earlier study examining the effect of e-cigarette exposure on tumor growth and metastasis, which examined the effect on breast cancer tumor grown and lung metastasis in mice (19). Like us, Pham et al. used a mixture of 50:50 PG/VG and nicotine (24 mg/mL) and identified the protumoral role of tumor-infiltrating suppressive macrophages upon their CCR5:CCL5 crosstalk with tumor cells, enhanced by exposure to a mixture of PG/VG and nicotine. They did not separate the effects of PG/VG and nicotine and did not characterize the lymphoid compartment infiltrating the breast and metastatic tumor models. The present study validates the proinflammatory macrophage infiltration described by Pham and colleagues as well as the other reports showing the association between e-cigarettes and IL-6 upregulation in humans and mice (20, 23, 26, 27). This study goes beyond the existing literature to identify important pro-metastatic roles for PG/VG and nicotine, as well as their involvement in immunosuppression both locally within the tumor and systemically in circulating lymphocytes.
PG/VG is not inert. Rather, PG/VG contributes to in vitro tumor cell invasion, as well as in vivo metastasis and systemic tumor aggressiveness. Biological effects of PG/VG have already been described including upregulation of extracellular matrix components (20), increased pulmonary and airway mucus (28–30), increased oxidation and DNA damage (31, 32), and metabolic alterations that compromise airway epithelial barriers (33). We also found that PG/VG exposure induced a reduction in CD8 T cells, a cell type critical for mediating anti-tumor immunity, providing a potential mechanism for enhanced tumor growth. This result is consistent with Sciezska and colleagues’ finding of impaired lung immunosurveillance in the form of decreased CD8+ T cells in lungs from animals exposed to PG/VG (34). We also found that CD8+ infiltrating PG/VG-exposed tumors showed increased levels of the immune checkpoints, further hampering effective antitumoral responses and clearance of cancerous cells. Together, these data support the notion that PG/VG can render exposed hosts more vulnerable to immunological challenges, such as viral infections (23, 35) or the development or dissemination of tumors, in addition to promoting tumor-intrinsic mechanisms leading to changes in tumor cell survival and invasion. Future studies diving into the molecular aspects of such changes could help better understand the formation of premetastatic niches.
While nicotine does not contribute to tumor initiation in our studies, it can make existing tumors worse. Nicotine affects several biological pathways, including cancer-relevant ones (10–12, 36–38). In our study, nicotine’s presence in vivo led to immune-intrinsic pro-tumoral changes, ranging from increasing macrophage- and T cell-induced immunosuppressive inflammation within the tumor microenvironment, decreasing CD8+ T cell infiltration and proliferation or increasing T cell exhaustion. Our immunological findings are consistent with previous immunoinhibitory roles reported for e-cigarette nicotine in vitro (14) and in vivo (15, 23, 34). One of such effects might be the observed upregulation of TNFα in immunosuppressive, tumor-infiltrating macrophages, previously reported in the context of chronic e-cigarette exposure (39, 40). The lack of differences in TGFβ might be explained by its more critical role in forming the metastatic niche and its expression from lung epithelial cells rather than macrophages (30). On the other hand, impaired anti-tumoral CD8+ T cell responses from PBMCs derived from smoker subjects have been shown in humanized tumor xenografts (15); while we observed a modest effect in Treg infiltration, we demonstrated that induction of inhibitory immune checkpoints such as PD-1, TIM3, LAG-3 or CTLA-4 on T cells occurs in syngeneic models upon e-cigarette exposure. Furthermore, we demonstrate that expression of these checkpoints is consequential, since treatment with anti-CTLA-4 antibodies can induce tumor regression in PG/VG plus nicotine-exposed animals. While Madison and colleagues found no correlation between the presence of nicotine and innate immune disruption caused by e-cigarettes (41), our data strongly suggest nicotine can impair adaptive immune responses.
These findings suggest the need to study cell-cell interactions to identify additional specific biological, metabolic pathways or critical infiltrating cell populations both to understand mechanisms of action and identify potential therapeutic or preventive interventions. Given the reported relevance for both lymphoid (14, 15, 21, 23, 34) and myeloid (19, 42) populations in antitumor responses in the presence of e-cigarette components, reinforced by the findings in this study, more preclinical studies involving exposure to PG/VG, nicotine and other e-cigarette components with targeted depletion of immune populations could be very informative to identify key targetable cell subsets, pathways or molecules.
This work is subject to several limitations. Firstly, the exclusive use of male mice in our experiments does not allow assessment of gender-based differences in the effect of e-cigarette exposure. Complementary studies in female mice will ascertain the extent of such differences. Because it is not possible to aerosolize nicotine without a humectant (in this case PG/VG), in most of the experiments we exposed the cells or animals to PG/VG or PG/VG plus nicotine. This arrangement allows us to draw conclusions about PG/VG and the marginal effect of adding nicotine, but limits conclusions about nicotine alone. The PG: VG ratio in commercially available e-cigarettes is not regulated and varies widely (43–45). We studied a previously reported relevant mixture of 1:1 PG/VG and nicotine, not whole aerosol generated by commercial e-cigarettes, which also includes flavoring and other components, as well as a range of PG: VG ratios. Future work could try to disentangle the roles of VG and PG separately. Finally, while we report the response of mouse cancers to relatively short exposures to PG/VG and nicotine, the specific relevance to chronic human exposure to these e-cigarette components, together with other components of e-cigarettes such as flavors, remains to be determined.
In 2012, the Food and Drug Administration established a list of Harmful and Potentially Harmful Constituents in Tobacco Products and Tobacco Smoke (46) (HPHC), which is dominated by toxicants in cigarette smoke. The HPHC list provides key metrics for assessing the harmfulness of tobacco products. In 2019 the FDA proposed adding 19 compounds to the HPHC list, including propylene glycol and glycerol, to reflect compounds in e-cigarettes (47). While e-cigarette components may induce lower carcinogenesis (although there is little data beyond lower biomarkers of exposure), there are many indirect effects that must be considered when assessing their health impact, including promotion of metastasis or immunosuppressive infiltration documented here. The results presented in this study underscore the consideration of propylene glycol as a harmful component given its widespread use in e-cigarettes as well as heated tobacco products (48).
Our results demonstrate new potential risks associated with e-cigarettes. Future assessments of the safely of e-cigarettes should include not only incidence of cancer, but also acceleration of cancers caused by other agents.
Data availability statement
The original contributions presented in the study are included in the article/Supplementary Material. Further inquiries can be directed to the corresponding author.
Ethics statement
The animal study was approved by Institutional Animal Care and Use Committee (IACUC). The study was conducted in accordance with the local legislation and institutional requirements.
Author contributions
MA-B: Conceptualization, Data curation, Formal analysis, Investigation, Methodology, Visualization, Writing – original draft, Writing – review & editing. CP: Conceptualization, Data curation, Investigation, Methodology, Writing – review & editing. PC: Data curation, Methodology, Writing – review & editing. AC: Data curation, Methodology, Writing – review & editing. YL: Data curation, Methodology, Writing – review & editing. AS: Data curation, Writing – review & editing. JG: Resources, Writing – review & editing. SG: Conceptualization, Formal analysis, Funding acquisition, Investigation, Supervision, Visualization, Writing – original draft, Writing – review & editing. LF: Conceptualization, Funding acquisition, Supervision, Writing – review & editing.
Funding
The author(s) declare financial support was received for the research, authorship, and/or publication of this article. This study was funded in part by cooperative agreement U54HL147127 from the National Heart, Lung, and Blood Institute and the Food and Drug Administration Center for Tobacco Products and the UCSF Cancer Center, the DRC Center Grant NIH P30 DK063720, and NIH grant R35CA253175. We thank the UCSF TCORS working group for valuable feedback and discussion. We thank Mike Klodnicki for assistance in mounting and servicing the Gram vaping machine.
Acknowledgments
We thank the UCSF Parnassus Flow Cytometry Core PFCC (RRID: SCR_018206) for assistance generating Flow Cytometry data. We thank the UCSF Preclinical Imaging Core for technical help. We also thank BioRender for digital image support. The content is solely the responsibility of the authors and does not necessarily represent the official views of NIH or the Food and Drug Administration. The funding agencies played no role in study design, collection, analysis, and interpretation of data, writing the report, or the decision to submit for publication.
Conflict of interest
LF received research funding from Abbvie, Bavarian Nordic, Bristol-Myers Squibb, Dendreon, Janssen, Merck, Nektar, Roche/Genentech and Parker Institute; served as a consultant to Abbvie, Actym, Amgen, Astra Zeneca, Bioatla, Bolt, Bristol Myer Squibb, Crescendo, Daiichi Sankyo, Immunogenesis, Innovent, Merck, NGMBio, Nutcracker, RAPT, Senti, Sutro, and Roche/Genentech; and has ownership interests in Actym, Bioatla, Bolt, Immunogenesis, Nutcracker, RAPT, and Senti, unrelated to the work here. SAG served as a consultant to the World Health Organization for work unrelated to this project.
The remaining authors declare that the research was conducted in the absence of any commercial or financial relationships that could be construed as a potential conflict of interest.
Publisher’s note
All claims expressed in this article are solely those of the authors and do not necessarily represent those of their affiliated organizations, or those of the publisher, the editors and the reviewers. Any product that may be evaluated in this article, or claim that may be made by its manufacturer, is not guaranteed or endorsed by the publisher.
Supplementary material
The Supplementary Material for this article can be found online at: https://www.frontiersin.org/articles/10.3389/fimmu.2024.1444020/full#supplementary-material
Supplementary Figure 1 | E-cigarette chemicals promote tumor cell migration in vitro. (A) Percent of scratch area covered by MC38 cells at 0, 4, 12 and 24h after insert removal in cell migration assays. P=PG/VG at 2.5 µM. (B) Viable cells on days 3, 5 and 7 after cell seeding.
Supplementary Figure 2 | PG/VG or nicotine impact primary growth for B16 but not M38 and TRAMP-C2 tumors. (A) Tumor growth analysis over time for experiments described in Figure 5. At any given time, tumor volume is larger in B16 (melanoma) tumors in animals exposed to PG/VG (ln(tumor volume) increased by 4.22 (95%CI 2.95, 5.50), p<0.001; Table 2), with a slower rate of grown (ln(tumor volume)/day -0.25 (-0.32, -0.17), p<0.001). Nicotine did not significantly affect tumor volume (p=0.169 for offset, p=0.093 for change in growth rate). Neither PG/VG nor nicotine was significantly associated with changes in tumor growth for MC38 (colorectal) or TRAMP-C2 (prostate) tumors. Lines represent fit curves. Error bars represent SEM. (B) Raw tumor volume measurements for subcutaneous B16, MC38 and TRAMP-C2 implantation experiments described in Figure 5A. Error bars represent SEM.
Supplementary Figure 3 | PG/VG tumor cell preconditioning induces more aggressive Luc-MC38 tumors. (A) Tumor bioluminescence on day 15 after implantation expressed as average photon radiance. Tumor bioluminescence was significantly increased in cells preconditioned with PG/VG (p<.001; Table 1). Adding nicotine had no additional effect (p=0.187).
Supplementary Figure 4 | Whole body exposure of mice e-cigarette aerosol leads accelerated tumor growth and more aggressive metastasis. (A) Whole body exposure machine set up for experiments described in Figure 2. Briefly, vaped e-cigarette liquids were injected into an exposure chamber containing live mice continuously for 1h, 5 days a week. (B) Time-course measurement of tumor bioluminescence expressed as Average Radiance for mice involved in experiments described in Figure 2. Error bars represent SEM. (C) Representative pictures of harvested lungs from unchallenged mice (left) or e-cigarette-exposed mice (right).
Supplementary Figure 5 | Whole body exposure of mice e-cigarette aerosol leads to increased myeloid and lymphoid immunosuppression in the tumor microenvironment. (A) Flow cytometry gating strategy used for phenotyping of Luc-MC38 tumor immune infiltrates harvested from lungs of mice involved in experiments described in Figure 2. (B) Left, offset histograms representing normalized modal frequencies for markers Ki67, IFNγ and PRF1 in gated tumor-infiltrating CD8+ T cells. Right, percentages of Ki67+, IFNγ+ and PRF1+ T cells within the CD8+ compartment. (C) Mean fluorescence intensity of TGFβ in gated lung macrophages was not affected significantly (p=0.5257). (D) Percentage CD4+FoxP3+ cells in gated CD45+ cells. No significant differences were observed (p=0.1597).
Supplementary Figure 6 | Tumors exposed to proinflammatory e-cigarette components are responsive to immune checkpoint blockade. (A) Splenic CD8+ T cell frequencies within gated live CD45+ immune cells in mice involved in whole body exposure experiments. (B) Representative flow cytometry histograms showing normalized modal frequencies for CTLA4, PD-1, TIM3 and LAG-3 expression in gated splenic CD8+ T cells. (C) Tumor volume curves for n=3 experiments described in Figure 4. Red arrows indicate Isotype control or anti-CTLA4 treatment. Black lines represent fit curves. Error bars represent SEM.
Supplementary Table 1 | Antibodies used for flow cytometry.
Supplementary Table 2 | Source data for Figures 1–6.
Supplementary Table 3 | Occurrence of metastases in Figure 2D.
Supplementary Table 4 | Tumor growth curves in Figure S2.
Abbreviations
CTLA4, Cytotoxic T-lymphocyte associated protein 4; FDA, Food and Drug Association; HPHC, Harmful and Potentially Harmful Constituents; ICB, Immune checkpoint blockade; IL, Interleukin; LAG-3, Lymphocyte-activation gene 3; nAChR, Nicotinic acetylcolin receptor; PBMC, Peripheral blood mononuclear cells; PD-1, Programmed cell death protein 1; PG/VG, Propylene glycol/vegetable glycerin; PRF1, Perforin; TIM3, T-cell immunoglobulin and mucin domain 3; TME, Tumor microenvironment.
References
1. Glantz SA, Nguyen N, Oliveira da Silva A. A population-based review of odds of various diseases in e-cigarette and dual use. N Engl J Med Evid. (2024) 3. doi: 10.1056/EVIDoa2300229
2. Committee on the Review of the Health Effects of Electronic Nicotine Delivery Systems, Board on Population Health and Public Health Practice, Health and Medicine Division, National Academies of Sciences, Engineering, and Medicine. Public health consequences of E-cigarettes. Stratton K, Kwan LY, Eaton DL, editors. Washington, D.C: National Academies Press (2018). Available at: https://www.nap.edu/catalog/24952.
3. Broderick JJ, Brumburgh F, Krum JK, Laughlin JL. Recent progress in the consideration of flavc/ring ingredients under the food additives amendment. Food Technol. (1965).
5. Dautzenberg B, Garelik D. Patients with lung cancer: Are electronic cigarettes harmful or useful? Lung Cancer. (2017) 105:42–8. doi: 10.1016/j.lungcan.2016.05.011
6. Harrow S, Bauld L, Macphee J, Ford A, Sinclair L, Mckell J, et al. P2.10–03 feasibility and acceptability of E-cigarettes as an aid to quitting smoking in lung cancer patients: A pilot study. J Thorac Oncol. (2018) 13:S774. doi: 10.1016/j.jtho.2018.08.1332
7. Ford A, Sinclair L, Mckell J, Harrow S, Macphee J, Morrison A, et al. Feasibility and acceptability of e-cigarettes as an aid to quitting smoking among lung cancer patients: a pilot study. Tob Induc Dis. (2018) 16:195–196. Available at: http://www.journalssystem.com/tid/Feasibility-and-acceptability-of-e-cigarettes-as-an-aid-to-quitting-smoking-among,84428,0,2.html
8. Sanner T, Grimsrud TK. Nicotine: carcinogenicity and effects on response to cancer treatment – A review. Front Oncol. (2015) 5:196/abstract. doi: 10.3389/fonc.2015.00196/abstract
9. Mravec B, Tibensky M, Horvathova L, Babal P. E-cigarettes and cancer risk. Cancer Prev Res (Phila Pa). (2020) 13:137–44. doi: 10.1158/1940-6207.CAPR-19-0346
10. Schaal C, Chellappan SP. Nicotine-mediated cell proliferation and tumor progression in smoking-related cancers. Mol Cancer Res. (2014) 12:14–23. doi: 10.1158/1541-7786.MCR-13-0541
11. Schuller HM. Is cancer triggered by altered signalling of nicotinic acetylcholine receptors? Nat Rev Cancer. (2009) 9:195–205. doi: 10.1038/nrc2590
12. Taly A, Corringer PJ, Guedin D, Lestage P, Changeux JP. Nicotinic receptors: allosteric transitions and therapeutic targets in the nervous system. Nat Rev Drug Discovery. (2009) 8:733–50. doi: 10.1038/nrd2927
13. Zhang Q, Ganapathy S, Avraham H, Nishioka T, Chen C. Nicotine exposure potentiates lung tumorigenesis by perturbing cellular surveillance. Br J Cancer. (2020) 122:904–11. doi: 10.1038/s41416-020-0730-0
14. Kalra R, Singh SP, Pena-Philippides JC, Langley RJ, Razani-Boroujerdi S, Sopori ML. Immunosuppressive and anti-inflammatory effects of nicotine administered by patch in an animal model. Clin Vaccine Immunol. (2004) 11:563–8. doi: 10.1128/CDLI.11.3.563-568.2004
15. Cheng CC, Lin HC, Chiang YW, Chang J, Sie ZL, Yang BL, et al. Nicotine exhausts CD8+ T cells against tumor cells through increasing miR-629–5p to repress IL2RB-mediated granzyme B expression. Cancer Immunol Immunother. (2021) 70:1351–64. doi: 10.1007/s00262-020-02770-x
16. Underwood PW, Zhang DY, Cameron ME, Gerber MH, Delitto D, Maduka MU, et al. Nicotine induces IL-8 secretion from pancreatic cancer stroma and worsens cancer-induced cachexia. Cancers. (2020) 12:329. doi: 10.3390/cancers12020329
17. Tyagi A, Sharma S, Wu K, Wu SY, Xing F, Liu Y, et al. Nicotine promotes breast cancer metastasis by stimulating N2 neutrophils and generating pre-metastatic niche in lung. Nat Commun. (2021) 12:474. doi: 10.1038/s41467-020-20733-9
18. Tyagi A, Wu SY, Sharma S, Wu K, Zhao D, Deshpande R, et al. Exosomal miR-4466 from nicotine-activated neutrophils promotes tumor cell stemness and metabolism in lung cancer metastasis. Oncogene. (2022) 41:3079–92. doi: 10.1038/s41388-022-02322-w
19. Pham K, Huynh D, Le L, Delitto D, Yang L, Huang J, et al. E-cigarette promotes breast carcinoma progression and lung metastasis: Macrophage-tumor cells crosstalk and the role of CCL5 and VCAM-1. Cancer Lett. (2020) 491:132–45. doi: 10.1016/j.canlet.2020.08.010
20. Beklen A, Uckan D. Electronic cigarette liquid substances propylene glycol and vegetable glycerin induce an inflammatory response in gingival epithelial cells. Hum Exp Toxicol. (2021) 40:25–34. doi: 10.1177/0960327120943934
21. Muthumalage T, Rahman I. Pulmonary immune response regulation, genotoxicity, and metabolic reprogramming by menthol- and tobacco-flavored e-cigarette exposures in mice. Toxicol Sci. (2023) 193:146–65. doi: 10.1093/toxsci/kfad033
22. Liang CC, Park AY, Guan JL. In vitro scratch assay: a convenient and inexpensive method for analysis of cell migration in vitro. Nat Protoc. (2007) 2:329–33. doi: 10.1038/nprot.2007.30
23. Maishan M, Sarma A, Chun LF, Caldera S, Fang X, Abbott J, et al. Aerosolized nicotine from e-cigarettes alters gene expression, increases lung protein permeability, and impairs viral clearance in murine influenza infection. Front Immunol. (2023) 14:1076772. doi: 10.3389/fimmu.2023.1076772
24. Glynos C, Bibli SI, Katsaounou P, Pavlidou A, Magkou C, Karavana V, et al. Comparison of the effects of e-cigarette vapor with cigarette smoke on lung function and inflammation in mice. Am J Physiol-Lung Cell Mol Physiol. (2018) 315:L662–72. doi: 10.1152/ajplung.00389.2017
25. Efremova M, Rieder D, Klepsch V, Charoentong P, Finotello F, Hackl H, et al. Targeting immune checkpoints potentiates immunoediting and changes the dynamics of tumor evolution. Nat Commun. (2018) 9:32. doi: 10.1038/s41467-017-02424-0
26. Sayed IM, Masso-Silva JA, Mittal A, Patel A, Lin E, Moshensky A, et al. Inflammatory phenotype modulation in the respiratory tract and systemic circulation of e-cigarette users: a pilot study. Am J Physiol-Lung Cell Mol Physiol. (2021) 321:L1134–46. doi: 10.1152/ajplung.00363.2021
27. Song MA, Mori KM, McElroy JP, Freudenheim JL, Weng DY, Reisinger SA, et al. Accelerated epigenetic age, inflammation, and gene expression in lung: comparisons of smokers and vapers with non-smokers. Clin Epigenetics. (2023) 15:160. doi: 10.1186/s13148-023-01577-8
28. Ghosh A, Coakley RC, Mascenik T, Rowell TR, Davis ES, Rogers K, et al. Chronic E-cigarette exposure alters the human bronchial epithelial proteome. Am J Respir Crit Care Med. (2018) 198:67–76. doi: 10.1164/rccm.201710-2033OC
29. Kim MD, Chung S, Baumlin N, Qian J, Montgomery RN, Sabater J, et al. The combination of propylene glycol and vegetable glycerin e-cigarette aerosols induces airway inflammation and mucus hyperconcentration. Sci Rep. (2024) 14:1942. doi: 10.1038/s41598-024-52317-8
30. Kim MD, Chung S, Dennis JS, Yoshida M, Aguiar C, Aller SP, et al. Vegetable glycerin e-cigarette aerosols cause airway inflammation and ion channel dysfunction. Front Pharmacol. (2022) 13:1012723. doi: 10.3389/fphar.2022.1012723
31. Canistro D, Vivarelli F, Cirillo S, Babot Marquillas C, Buschini A, Lazzaretti M, et al. E-cigarettes induce toxicological effects that can raise the cancer risk. Sci Rep. (2017) 7:2028. doi: 10.1038/s41598-017-02317-8
32. Tang Ms, Wu XR, Lee HW, Xia Y, Deng FM, Moreira AL, et al. Electronic-cigarette smoke induces lung adenocarcinoma and bladder urothelial hyperplasia in mice. Proc Natl Acad Sci. (2019) 116:21727–31. doi: 10.1073/pnas.1911321116
33. Woodall M, Jacob J, Kalsi KK, Schroeder V, Davis E, Kenyon B, et al. E-cigarette constituents propylene glycol and vegetable glycerin decrease glucose uptake and its metabolism in airway epithelial cells in vitro. Am J Physiol-Lung Cell Mol Physiol. (2020) 319:L957–67. doi: 10.1152/ajplung.00123.2020
34. Scieszka DP, Garland D, Hunter R, Herbert G, Lucas S, Jin Y, et al. Multi-omic assessment shows dysregulation of pulmonary and systemic immunity to e-cigarette exposure. Respir Res. (2023) 24:138. doi: 10.1186/s12931-023-02441-2
35. Sharma A, Lee J, Fonseca AG, Moshensky A, Kothari T, Sayed IM, et al. E-cigarettes compromise the gut barrier and trigger inflammation. iScience. (2021) 24:102035. doi: 10.1016/j.isci.2021.102035
36. Chu M, Guo J, Chen CY. Long-term exposure to nicotine, via ras pathway, induces cyclin D1 to stimulate G 1 cell cycle transition. J Biol Chem. (2005) 280:6369–79. doi: 10.1074/jbc.M408947200
37. Chang YW, Singh KP. Nicotine-induced oxidative stress contributes to EMT and stemness during neoplastic transformation through epigenetic modifications in human kidney epithelial cells. Toxicol Appl Pharmacol. (2019) 374:65–76. doi: 10.1016/j.taap.2019.04.023
38. Nishioka T, Kim HS, Luo LY, Huang Y, Guo J, Yan Chen C. Sensitization of epithelial growth factor receptors by nicotine exposure to promote breast cancer cell growth. Breast Cancer Res. (2011) 13:R113. doi: 10.1186/bcr3055
39. Ghosh A, Coakley RD, Ghio AJ, Muhlebach MS, Esther CR, Alexis NE, et al. Chronic E-cigarette use increases neutrophil elastase and matrix metalloprotease levels in the lung. Am J Respir Crit Care Med. (2019) 200:1392–401. doi: 10.1164/rccm.201903-0615OC
40. Brown DM, Donaldson K, Borm PJ, Schins RP, Dehnhardt M, Gilmour P, et al. Calcium and ROS-mediated activation of transcription factors and TNF-α cytokine gene expression in macrophages exposed to ultrafine particles. Am J Physiol-Lung Cell Mol Physiol. (2004) 286:L344–53. doi: 10.1152/ajplung.00139.2003
41. Madison MC, Landers CT, Gu BH, Chang CY, Tung HY, You R, et al. Electronic cigarettes disrupt lung lipid homeostasis and innate immunity independent of nicotine. J Clin Invest. (2019) 129:4290–304. doi: 10.1172/JCI128531
42. Scott A, Lugg ST, Aldridge K, Lewis KE, Bowden A, Mahida RY, et al. Pro-inflammatory effects of e-cigarette vapour condensate on human alveolar macrophages. Thorax. (2018) 73:1161–9. doi: 10.1136/thoraxjnl-2018-211663
43. EL-Hellani A, Salman R, El-Hage R, Talih S, Malek N, Baalbaki R, et al. Nicotine and carbonyl emissions from popular electronic cigarette products: correlation to liquid composition and design characteristics. Nicotine Tob Res. (2016) 20:ntw280. doi: 10.1093/ntr/ntw280
44. Lee YJ, Na CJ, Botao L, Kim KH, Son YS. Quantitative insights into major constituents contained in or released by electronic cigarettes: Propylene glycol, vegetable glycerin, and nicotine. Sci Total Environ. (2020) 703:134567. doi: 10.1016/j.scitotenv.2019.134567
45. Richardson PIC, Burke A, Gotts N, Goodacre R. Quantifying PG : VG ratio and nicotine content in commercially available e-liquids using handheld Raman spectroscopy. Analyst. (2023) 148:4002–11. doi: 10.1039/D3AN00888F
46. Kux L. Harmful and potentially harmful constituents in tobacco products and tobacco smoke; established list. Food Drug Administration. (2012).
47. Schiller LJ. Harmful and Potentially Harmful Constituents in Tobacco Products; Established List; Proposed Additions; Request for Comments. Maryland, USA: Food and Drug Administration (2019).
Keywords: electronic cigarettes, metastasis, whole body exposure, immunosuppression, immune checkpoint blockade
Citation: Arias-Badia M, Pai C-CS, Chen P, Chang A, Lwin YM, Srinath A, Gotts JE, Glantz SA and Fong L (2024) E-cigarette exposure disrupts antitumor immunity and promotes metastasis. Front. Immunol. 15:1444020. doi: 10.3389/fimmu.2024.1444020
Received: 04 June 2024; Accepted: 29 July 2024;
Published: 16 August 2024.
Edited by:
Elena Voronov, Ben-Gurion University of the Negev, IsraelReviewed by:
Chi-Heng Wu, Dren Bio, Inc., United StatesMichal Amit Rahat, Technion-Israel Institute of Technology, Israel
Copyright © 2024 Arias-Badia, Pai, Chen, Chang, Lwin, Srinath, Gotts, Glantz and Fong. This is an open-access article distributed under the terms of the Creative Commons Attribution License (CC BY). The use, distribution or reproduction in other forums is permitted, provided the original author(s) and the copyright owner(s) are credited and that the original publication in this journal is cited, in accordance with accepted academic practice. No use, distribution or reproduction is permitted which does not comply with these terms.
*Correspondence: Lawrence Fong, bGF3cmVuY2UuZm9uZ0BmcmVkaHV0Y2gub3Jn
†These authors have contributed equally to this work and share senior authorship