- 1Klinik für Anästhesiologie, Intensivmedizin und Schmerztherapie, Universitätsklinikum Knappschaftskrankenhaus Bochum, Bochum, Germany
- 2Medical Proteome Analysis, Center for Proteindiagnostics (PRODI), Ruhr-University Bochum, Bochum, Germany
- 3Medizinisches Proteom-Center, Ruhr-University Bochum, Bochum, Germany
- 4Department of Molecular and Medical Virology, Ruhr-University Bochum, Bochum, Germany
- 5Leibniz-Institut für Virologie, Hamburg, Germany
- 6Center for Translational Medicine, Medical Clinic I, Marien Hospital Herne, University Hospital of the Ruhr-University Bochum, Herne, Germany
- 7Klinik für Anästhesiologie und Operative Intensivstation, Universitätsklinikum Bonn, Bonn, Germany
- 8Klinik für Anästhesiologie, operative Intensivmedizin und Schmerztherapie, Universitätsklinikum Münster, Münster, Germany
- 9Zentrum für Künstliche Intelligenz, Medizininformatik und Datenwissenschaften, Bochum, Germany
Introduction: Repair of mitochondrial damage seems pivotal for clinical recovery and determining outcome in patients with critical COVID-19. However, reliable biomarkers for non-invasively assessing mitochondrial repair in peripheral blood of critically ill COVID-19 patients are currently lacking. Accordingly, we sought to assess different surrogates of mitochondrial repair in peripheral blood and correlate these measurements with clinical outcome in patients with critical COVID-19.
Methods: In this prospective multicentric cohort study, 88 critically ill COVID-19 patients were enrolled across three German intensive care units. Gene products of mitochondrial quality control (MFN2, PINK, TFAM, TFB2M) and the mtDNA copy number were measured in peripheral blood mononuclear cells. Furthermore, the protein interactions between TFAM and TFB2M were quantified. Patients were stratified regarding 30-day mortality.
Results: Transcript levels of the assessed mRNA markers of mitochondrial quality control were not associated with clinical outcome. In contrast, more than 10.7 protein interactions per cell were associated with a 74% 30-day survival (37 out of 50), while 10.7 or fewer protein interactions per cell were associated with a 32% 30-day survival (12 out of 38; p < 0.001). Furthermore, multivariable Cox regression analysis revealed TFAM-TFB2M protein interaction as an independent predictor for 30-day survival (HR: 3.2; 95% CI: 1.6 to 6.5; p < 0.001).
Discussion: Our findings indicate that TFAM-TFB2M protein interactions, identified as a novel biomarker, are strongly and independently associated with 30-day survival in critical COVID-19. Therefore, our data suggest a significant impact of mitochondrial repair and quality control on clinical outcome in critical COVID-19.
1 Introduction
An infection with the SARS-CoV-2 virus can result in COVID-19 with a varying severity of clinical manifestations, from mild to critical illness. Especially critical COVID-19 requires intensive care treatment, potentially leading to long-term sequelae and death (1, 2). The predominant manifestation of critical COVID-19 is respiratory failure, septic shock, and multiple organ dysfunction, mimicking the characteristics of bacterial sepsis (3, 4). In both bacterial sepsis and critical COVID-19, multi-organ failure is affected by mitochondrial dysfunction (4, 5). Here, previous studies have demonstrated that SARS-CoV-2 induces mitochondrial structural changes and damage, resulting in mitochondrial dysfunction (6–10). Accordingly, mitochondrial repair via processes of mitochondrial quality control (MQC), seems pivotal for functional recovery likely impacting clinical outcome in COVID-19 (11–14).
Previous studies in sepsis patients already demonstrated that messenger RNA (mRNA) transcripts in peripheral blood mononuclear cells (PBMCs) related to MQC, especially mitochondrial biogenesis, represent potential biomarker candidates, which were able to display clinical outcome in septic patients (15). A further promising candidate to assess mitochondrial repair in patients suffering from COVID-19 is the interaction between mitochondrial transcription factor A (TFAM) and mitochondrial transcription factor B2 (TFB2M) (16). TFAM and TFB2M are crucial components of the mitochondrial transcription initiation complex, essentially promoting mitochondrial biogenesis (17, 18). In bacterial sepsis, a reduced number of protein interactions between TFAM and TFB2M measured in PBMCs was associated with inefficient mitochondrial recovery and increased mortality in sepsis (19). To determine the complex formation between TFAM and TFB2M in critical COVID-19, a well-established assay, called proximity ligation assay (PLA), was performed (35). The PLA is a fluorescence-based method that is used to measure protein-protein interactions or protein modifications in situ. PLA signals are only detected when two proteins are in close proximity to one another, which is therefore a measure of the ability of two proteins to interact.
Thus, the objective of this study is to investigate different mRNA transcripts related to mitochondrial repair and quality control including the protein interaction between TFAM and TFB2M targeting mitochondrial biogenesis, as predictors for clinical outcome in patients with critical COVID-19.
2 Study design and methods
2.1 Study design and population
This multicentric prospective cohort study was conducted on three intensive care units (ICUs) of German university hospitals from September 2020 to December 2021 as part of CovidDataNet.NRW (German Clinical Trial Registry No. DRKS00026184). The study was approved by the Ethics Committee of the Medical Faculty of the Ruhr-University Bochum (registration number #5047-14), and subsequently by the local ethics committees of each participating center. Written informed consent was secured from all participants or their legal representatives prior to enrolment. This study was conducted in strict accordance with the Declaration of Helsinki, guidelines for good clinical practice, and relevant local legal regulations.
Patients with critical COVID-19 were considered eligible if the infection with SARS-CoV-2 was verified using polymerase chain reaction (PCR) assays and if they fulfilled the criteria for critical course of COVID-19 defined according to the World Health Organization as a disease with the confirmation of one (or more) of the following symptoms:
● Respiratory distress ≥ 30 breaths per minute.
● Oxygen saturation ≤ 93% at rest under ambient air or mandatory oxygen.
● Oxygenation index ≤ 300 mmHg [arterial oxygen partial pressure (paO2)/fractional inspired oxygen (FiO2)].
● Presence of respiratory failure, septic shock, and/or multiple organ dysfunction.
Furthermore, the initial blood sampling was required to be completed within the first 24 hours after the diagnosis of sepsis. The exclusion criteria were age under 18 years, pregnancy, existing anemia, a diagnosed mitochondrial disorder, usage of medications identified as mitochondrial toxins, and rejection of the study by the patient or legal representatives. In total 88 adult patients diagnosed with critical COVID-19 were enrolled between September 2021 and December 2022.
2.2 Procedures/exposure
2.2.1 Sample preparation
Whole blood samples were collected in sodium EDTA tubes (BD Biosciences, Heidelberg, Germany) on the day of enrollment. PBMCs and plasma fractions were isolated using Ficoll density gradient centrifugation (GE Healthcare Europe, Freiburg, Germany). For microscopy the cells were counted, spun onto microscope slides using a cytospin (Cellspin II, Tharmac, Wiesbaden, Germany) and then fixed with 4% formaldehyde solution (Sigma-Aldrich). PBMCs, dissolved in Bambanker freezing medium (Nippon Genetics, Düren, Germany), and plasma were stored at -80°C.
2.2.2 Quantitative polymerase chain reaction
To investigate, gene products associated with MQC in critical COVID-19, quantitative polymerase chain reaction (qPCR) was performed. mRNA transcripts of mitofusion 2 (MFN2), PTEN-induced kinase 1 (PINK1), TFAM and TFB2M as well as the copy number of mitochondrial DNA (mtDNA) were assessed. Therefore, total DNA and total RNA were extracted from PBMCs using the QIAamp DNA and RNeasy kits according to the manufacturer’s instructions (QIAGEN, Hilden, Germany). The synthesis of complementary DNA (cDNA)-synthesis was performed using the High-Capacity cDNA Kit (Applied Biosystems, Waltham, MA, USA). PCR was conducted in technical duplicates using GoTaq® qPCR Master Mix (Promega, Walldorf, Germany) and specific primers (Table 1) on a CFX Connect Real-Time System (Bio-Rad Laboratories, Feldkirchen, Germany). The relative mRNA expression level and mtDNA copy number quantification was performed as previously described (16, 19).
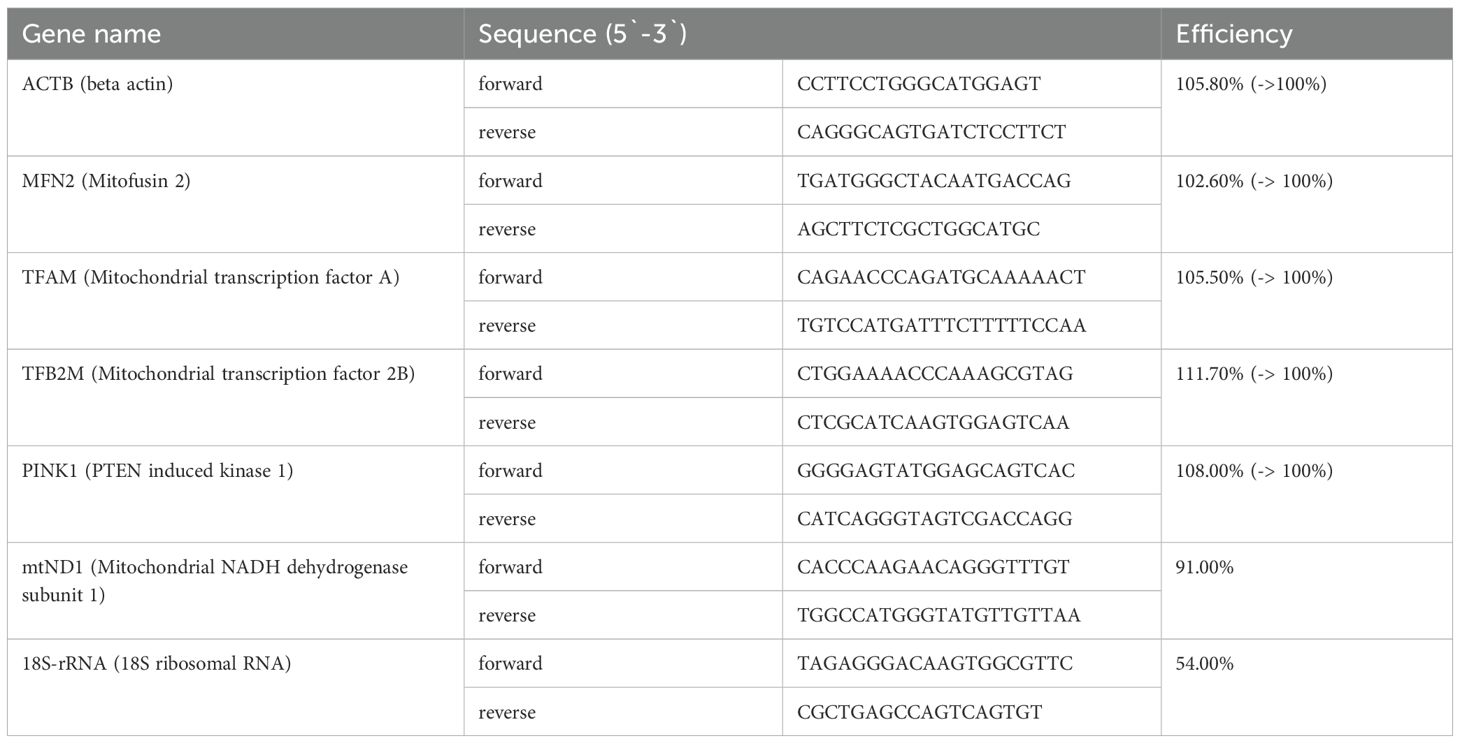
Table 1. Primer sequences of selected genes products (from Integrated DNA Technologies) associated with mitochondrial function and vitality as well as primer sequences of control gene products.
2.2.3 Proximity ligation assay
The PLA is used to measure protein-protein interactions in situ. If two proteins interact with each other, signals occur in the subsequent PLA reaction. Only when two proteins are in close proximity to one another signals in the form of individual dots could be detected. Therefore, PLA signals per cell are a measure of the ability of two proteins to interact. To analyze the protein-protein interaction of TFAM and TFB2M, a proximity ligation assay (PLA) was performed as previously described [16]. Slides were re-hydrated, permeabilized and primary antibodies against TFAM (1:50, sc-376672, Santa Cruz Biotechnology, Heidelberg, Germany) and TFB2M (1:100, 46-481, ProSci Incorporated, Poway, CA, USA) were incubated overnight at 4°C. After additional washing steps, an incubation with secondary antibodies (anti-mouse and anti-rabbit, NaveniFlex 100 MR, Navinci Diagnostics, Uppsala, Sweden) was following for 1 hour at 37°C. The subsequent PLA protocol was performed according to the manufacturer’s recommendations (Navinci Diagnostics). PBMCs were imaged using Zeiss Software ZEN for a Zeiss Apotome microscope (Zeiss, Oberkochen, Germany). Images were analyzed using ImageJ (Version 1.8.0_345) and CellProfiler (Version 4.2.3.) to quantify the average PLA signal per cell. For an appropriate analysis, at least 50 PBMCs were needed per patient. Out of 95 samples, 88 were analyzed.
2.3 Data collection and variables
Data were sourced from electronic health records, transferred to a central database, and verified at each study site. The dataset was validated by an independent intensive care specialist and a statistician for accuracy. Any discrepancies were addressed by local teams during data clearance.
2.4 Outcomes
For the evaluation of the association with clinical outcome, we stratified sepsis patients into two clinical outcome groups defined as “30-day survivors” (n = 49) and “30-day non-survivors” (n = 39). Given the hypothesis that the severity of COVID-19 upon ICU admission is associated with the degree of mitochondrial dysfunction at that time, we evaluated the Sepsis-related organ failure assessment score (SOFA score), using the protocol described by Lambden and colleagues (20), on the first day simultaneously with blood collection.
2.5 Statistical analysis
Severity scores for diseases were expressed as the median and the interquartile range (IQR). Variables on an interval scale with normal distribution were depicted as mean ± standard deviation (SD), alongside median and IQR (25th to 75th percentiles) as appropriate. Categorical variables were presented as counts and percentages. Confidence intervals (CIs) were established at a 95% level. All p-values are nominal, two-sided, and were considered significant at a predefined level of less than 0.05. The normality of the distribution within each group was assessed using the Shapiro-Wilk test. Consequently, differences between two normally distributed groups were analyzed using the unpaired Student’s t-test, while differences between two groups not following a normal distribution were evaluated using the Mann-Whitney U test.
Further analysis involved determining the cut-off for TFAM-TFB2M interaction based on the Youden Index, to distinguish between high (>10.7 PLA signals per cell) and low (≤10.7 PLA signals per cell) protein activity. This was followed by Kaplan-Meier survival analysis and Cox regression models to evaluate the impact of mitochondrial protein interactions on the first day on survival over 30 days. Initially, a univariate Cox regression analysis was performed using TFAM-TFB2M protein interactions as variable, stratified by the Youden Index threshold. A multivariate Cox regression model was then performed, additionally considering adjustment for age and the SOFA score. Statistical analyses were carried out using SPSS software (version 28, IBM, Chicago, IL, USA), while GraphPad Prism 9 (GraphPad, San Diego, CA, USA) facilitated the creation of graphical representations.
3 Results
3.1 Patient characteristics
Ninety-five patients with severe COVID-19 on three different ICUs were enrolled in this study, but the final analysis was conducted on 88 patient (Table 2). The mean age was 58 (± 1.6) years and median SOFA score was 9 [5 to 12]. Further baseline characteristics are shown in Table 2.
3.2 30-day survivors versus 30-day non-survivors
First, we explored the role of different gene transcripts related to MQC. Here, mRNA-expression of MFN2, PINK, TFAM, and TFB2M along with the mtDNA copy number were assessed. However, no significant differences in gene expression were observed between the 30-day survivors and the 30-day non-survivors (each, p > 0.05; Figure 1).
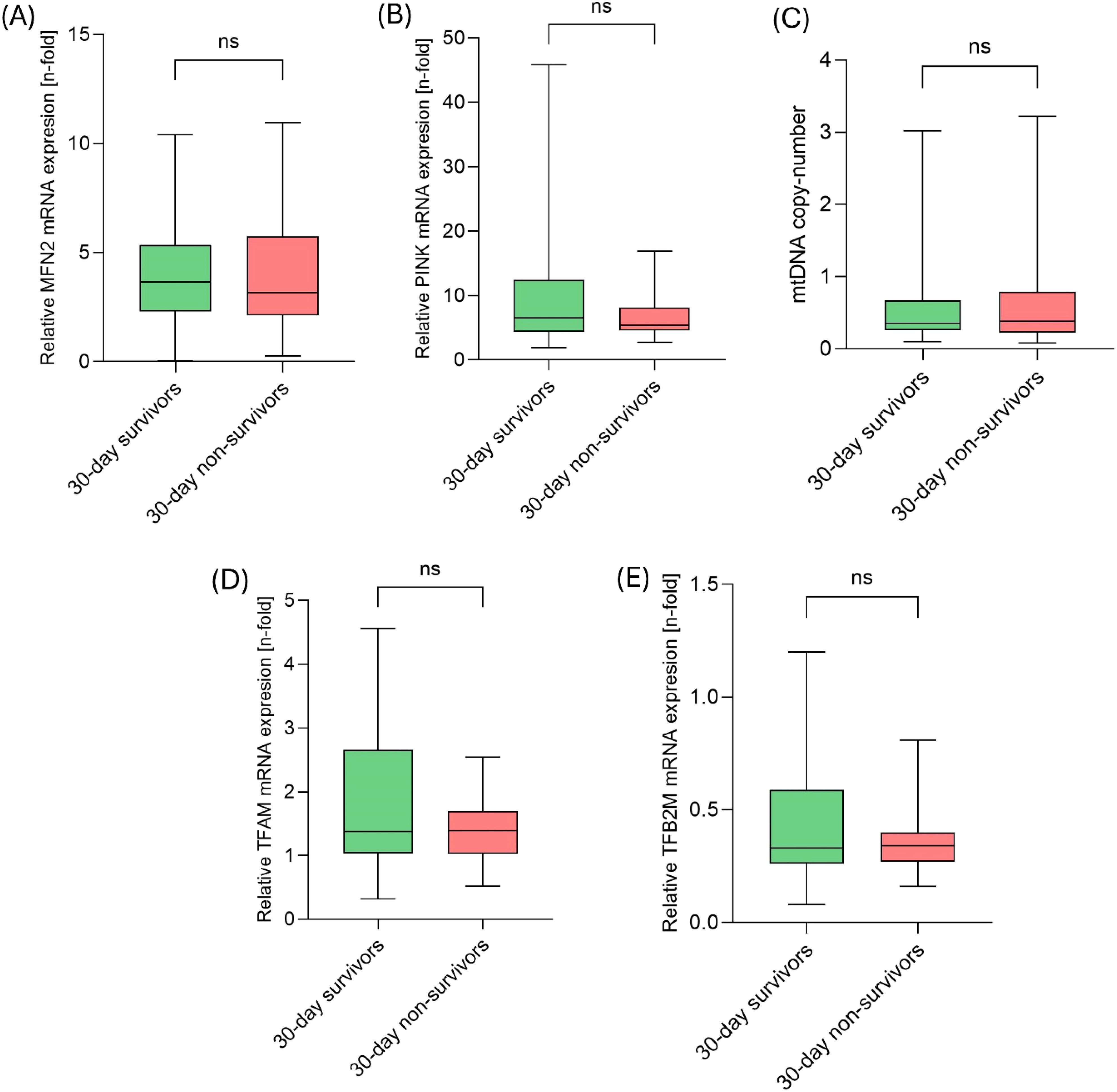
Figure 1. mRNA transcripts of MQC-related genes did not differ between 30-day survivors and 30-day non-survivors on day 1. (A) Comparison of relative MFN2 mRNA expression, (B) relative PINK mRNA expression, (C) mtDNA copy-number, (D) relative TFAM mRNA expression and (E) relative TFB2M mRNA expression between 30-day survivors and 30-day non-survivors.
In contrast, the mitochondrial protein interaction of TFAM with TFB2M within the mitochondrial transcription complex was increased on day 1 in 30-day survivors compared to 30-day non-survivors (p = 0.001; Figure 2). In detail, 44% higher quantity of PLA signals per cell were detected in 30-day survivors (15 signals per cell, IQR: 10.5 to 18.0) in comparison to 30-day non-survivors (8.7 signals per cell, IQR: 5.0 to 15.6).
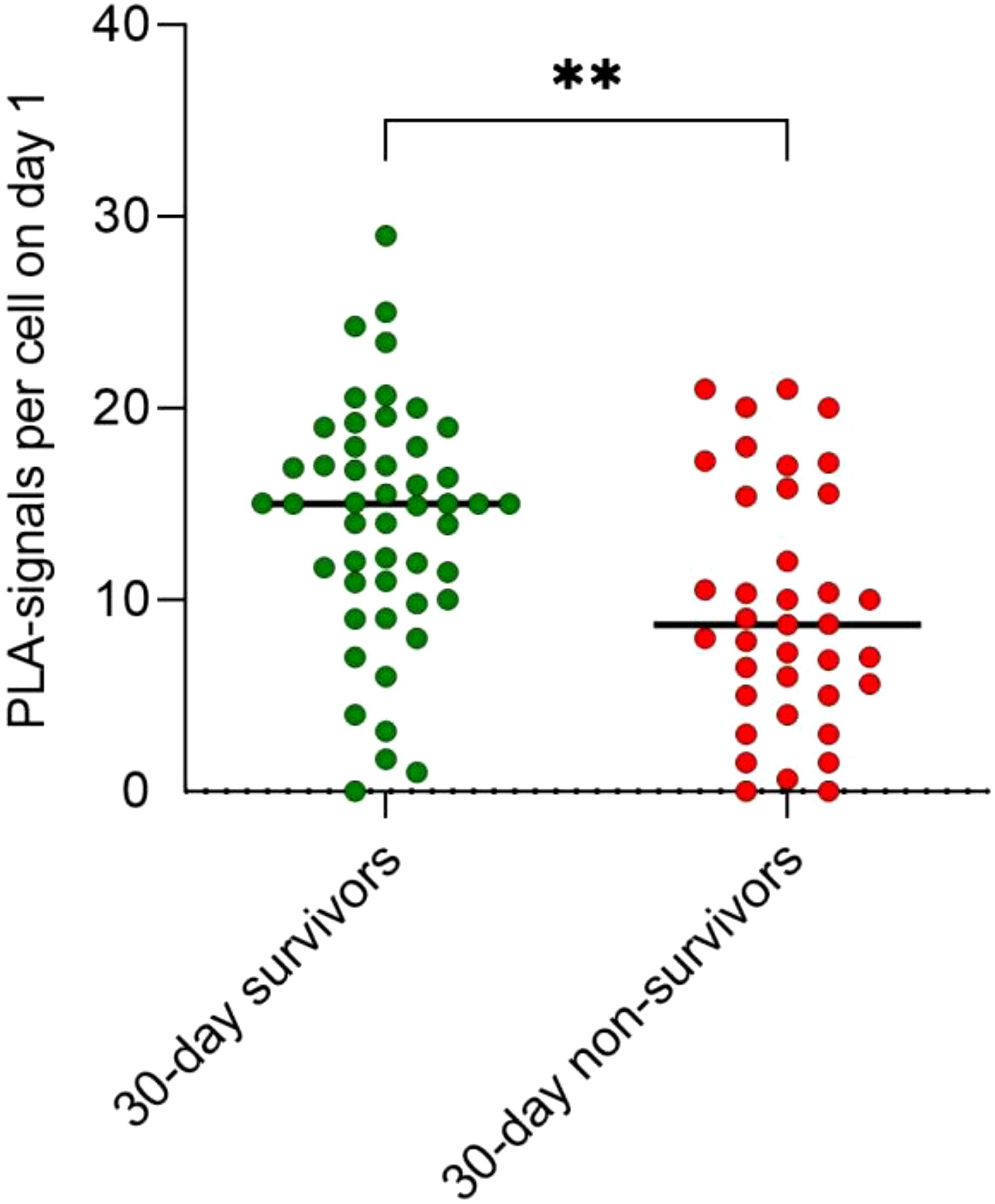
Figure 2. Interactions of TFAM with TFB2M in the human mitochondrial transcription initiation complex increases in 30-day survivors compared to 30-day non-survivors (** p = 0.0017).
3.3 Outcome of COVID-19
The interaction between TFAM and TFB2M was measured using proximity ligation assay (PLA) and the average PLA signal per cell was determined for each patient. There were differences in the average PLA signal per cell between the individual patients (Figure 3A). Therefore, a cut-off for TFAM-TFB2M protein interactions on day 1 regarding prediction of 30-day mortality was calculated using the Youden index. The area under the receiver operating characteristic curve was 0.72 (95% CI: 0.59 to 0.84; p = 0.002), with a sensitivity of 75% and a specificity of 67%. Correspondingly, more than 10.7 PLA signals per cell (high activity of mitochondrial biogenesis) were associated with a 74% survival rate at 30 days (37 of 50; Figure 4) and less than 10.7 PLA signals per cell (reduced activity of mitochondrial biogenesis) were related with a 32% survival rate at 30 days (12 of 38; p < 0.001; Figure 4). A multivariable Cox regression analysis revealed that a low amount of mitochondrial protein interactions of TFAM with TFB2M (≤ 10.7 PLA signals per cell) was associated with a more than 3-fold higher risk of death within 30 days after adjusting for age and SOFA score (hazard ratio: 3.3; 95% CI: 1.6 to 6.5, p < 0.001; Table 3). Thus, Cox regression analyses indicated the mitochondrial protein interactions of TFAM and TFB2M as a strong and independent prognostic factor for 30-day survival (Table 3).
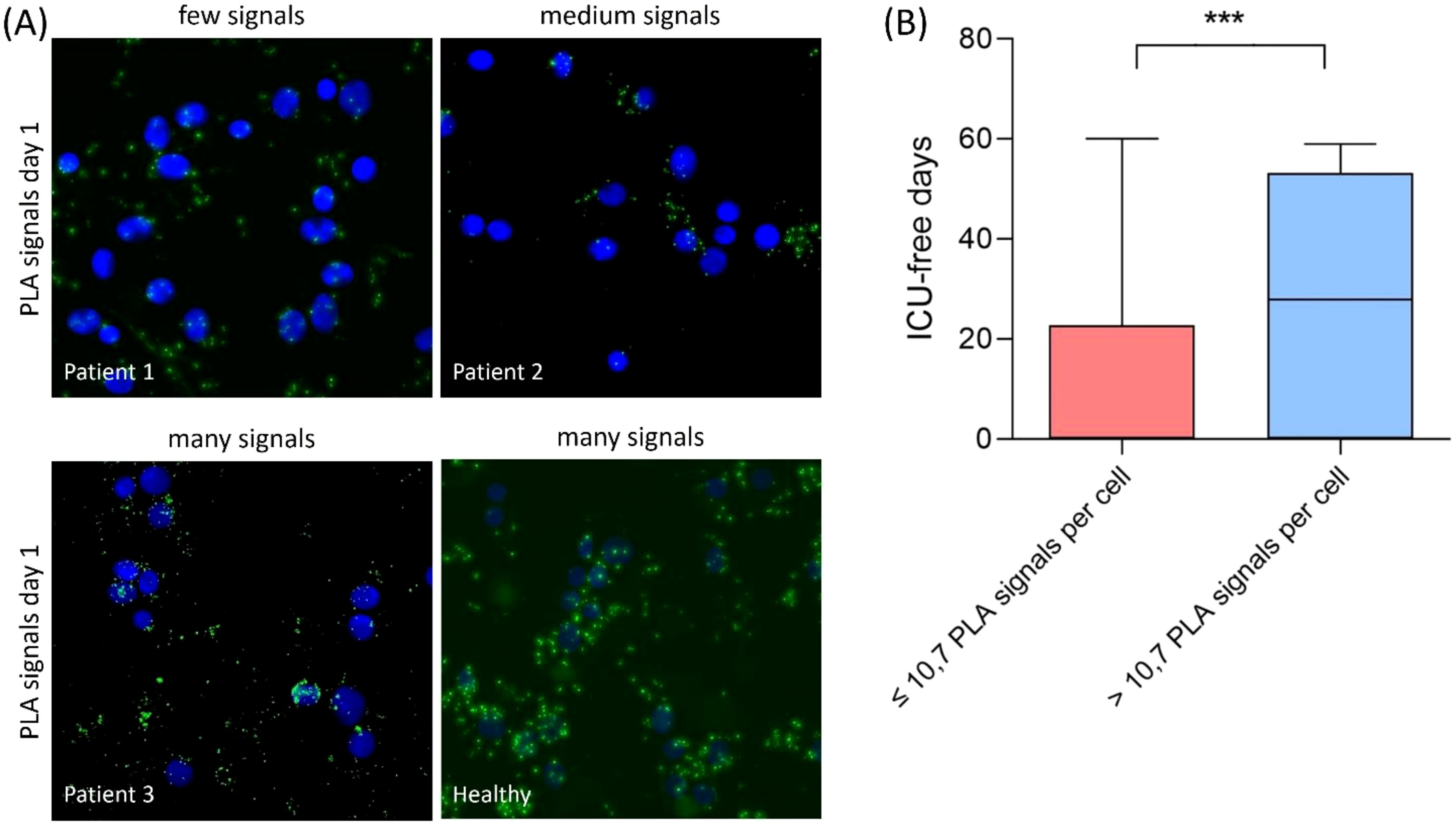
Figure 3. (A) Proximity ligation assay (PLA) in PBMCs of critical COVID-19 patients on day 1 and healthy control. PLA was conducted to assess the interaction between TFAM and TFB2M within the human mitochondrial transcription initiation complex, serving as an indicator of mitochondrial functionality and viability. Each green dot represents a signal for a TFAM/TFBM interaction. Nuclear staining with DAPI (blue) can be used to determine the average PLA signal per cell. The average PLA signal per cell was then associated with 30-day survival of critical COVID-19. (B) Patients with >10.7 PLA signals experience more ICU-free days over a 60-day period compared to patients with <10.7 PLA signals (*** p = 0.0008).
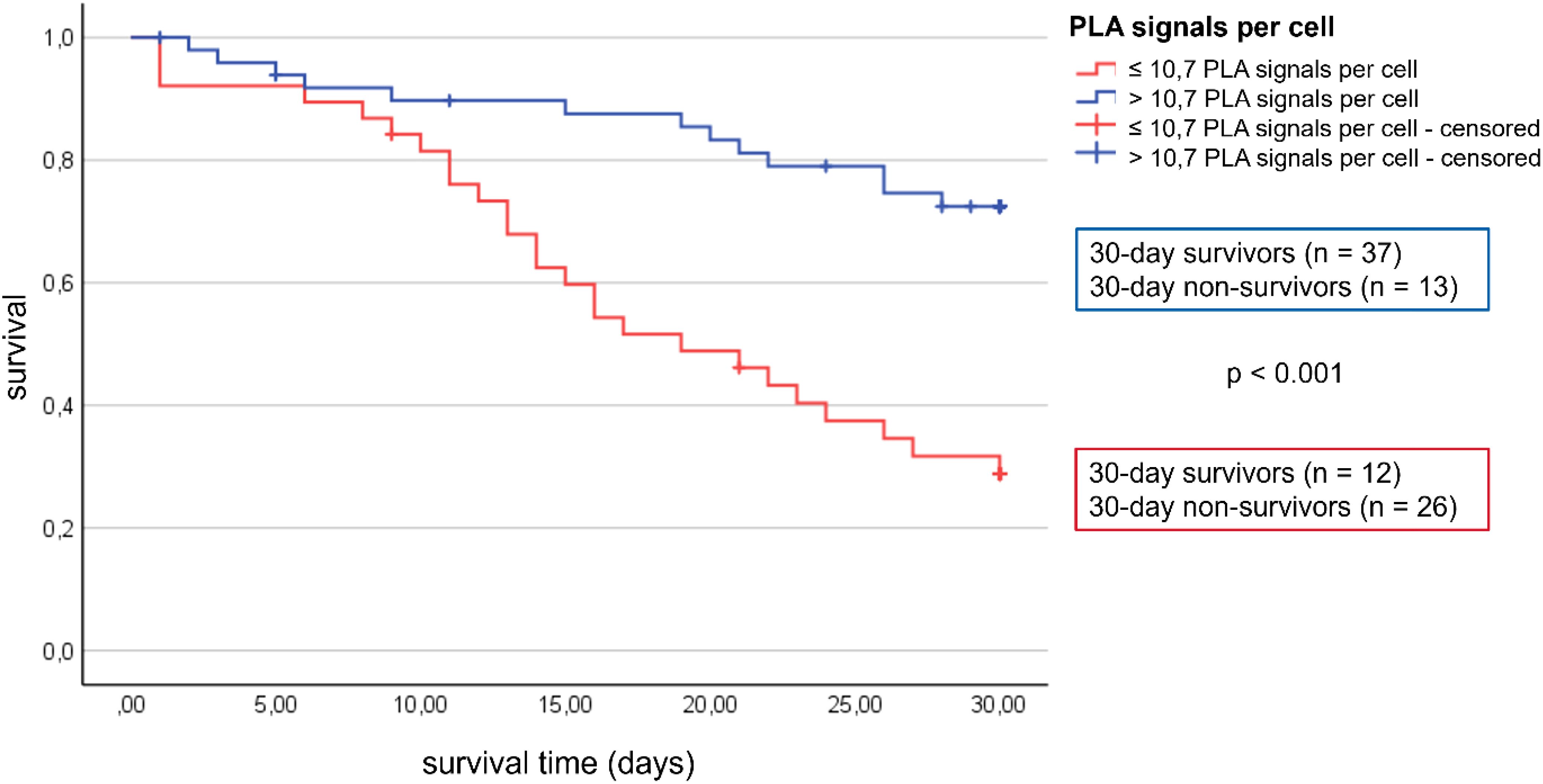
Figure 4. Patients with >10.7 PLA signals have a higher 30-day survival rate than patients with <10.7 PLA signals.
Additionally, we observed that the group with reduced TFAM-TFB2M interactions (≤10.7 PLA signals per cell) had a median of 0 ICU-free days [IQR: 0 to 3] over a 60-day period, in contrast to 28 ICU-free days [IQR: 4 to 25] for the group with increased TFAM-TFB2M interactions (> 10.7 PLA signals per cell; p < 0.001; Figure 3B).
4 Discussion
Our study unveils mitochondrial protein interactions as a novel biomarker in critical COVID-19. Specifically, we shed light on the promising role of mitochondrial biogenesis assessed via the interaction between TFAM and TFB2M in peripheral blood of critical COVID-19 patients. Remarkably, compared to mRNA transcripts of MQC-related genes and the mtDNA copy number, the TFAM-TFB2M mitochondrial protein interaction exhibited superior capability in reflecting disease severity and was correlated with clinical outcomes. Specifically, enhanced mitochondrial biogenesis, indicated by elevated TFAM and TFB2M interactions on day 1, was associated with a 74% survival rate at 30 days and a greater number of ICU-free days. In contrast, patients with decreased interactions of TFAM and TFB2M had more than 3-fold higher risk to die within the first 30 days. These results highlight the promising potential of TFAM-TFB2M protein interactions as a new and clinically useful biomarker for mitochondrial dysfunction in severe COVID-19.
One important question is why the TFAM-TFB2M mitochondrial protein interaction stands out as a novel biomarker superiorly reflecting clinical outcome of critical COVID-19, compared to other mRNA-based biomarkers, which also target processes of MQC. MQC involves balancing mitochondrial synthesis, remodeling, and degradation to repair damage effectively. Failure in these MQC processes correlates with worse outcomes (21). Studies highlight the role of mitochondrial biogenesis in recovery from COVID-19, positioning MQC as a critical tool for evaluating and predicting recovery from organ failure (14, 15). In this context, several recent studies detected a higher amount of cell-free mtDNA (cf-mtDNA) in patients with severe COVID-19 compared to asymptomatic COVID-19 patients, indicating that elevated mtDNA levels may be correlated with COVID-19 poor outcomes (21, 22). These results are contrary to our findings, as in our study, we did not find a difference in mtDNA copy number between survivors and non-survivors of COVID-19. However, this is mainly explained by the fact, that both Edinger et al. and Hepokowski et al. quantified mtDNA in plasma, i.e. cf-mtDNA, whereas our measurements of mtDNA copy numbers were performed on whole blood, mainly encompassing the mtDNA content of cells (21, 22). In this context, assessing cf-mtDNA is a surrogate for the associated tissue damage rather than a marker of MQC (23, 24). Of note Valdes and colleagues indeed demonstrated a higher amount of mtDNA copy number in whole blood, so focusing MQC, of COVID-19 patients with mild to moderate symptoms compared to critically ill COVID-19 patients and non-survivors (12). Although we cannot confirm this finding, the cellular mtDNA content in blood cells may also represent a biomarker for mitochondrial repair in critical COVID-19.
Regarding MQC-related genes, we observed that mRNA transcripts of these genes are not associated with outcome of our critically ill COVID-19 patients. Here, numerous studies have demonstrated upregulation of gene transcripts involved in MQC in COVID-19 (25, 26). Shokora et al. examined the mRNA expression of TFAM in COVID-19 and demonstrated elevated levels of TFAM gene expression in COVID-19 patients compared to controls (27), which is at least counterintuitive that patients with an enhanced mitochondrial biogenesis suffer from severe infection. In our previous study, we observed a protein maldistribution of TFAM in septic patients as potential explanation (16). Here, an increased amount of TFAM was detected in the cytosol whereas a decreased concentration of TFAM was found in the mitochondria (16). In this context, it is important to note that TFAM is expressed in the nucleus, translated in the cytosol, and subsequently actively transported to the mitochondria (28, 29). Thus, an elevated cytosolic mRNA and protein concentration of TFAM, not necessarily transposes in effective mitochondrial biogenesis during severe bacterial and viral infections, especially in sepsis. This protein maldistribution of TFAM could explain the observed diverge between TFAM mRNA expression and TFAM protein activity in sepsis (19) and now in this study in patients with COVID-19 and also likely include other nuclear-encoded mitochondrial proteins. Consequently, assessing TFAM’s functional activity provides more advanced “down-stream” approach for predicting clinical recovery. Here, our study introduces a new method to evaluate the interaction between the mitochondrial proteins TFAM and TFB2M, overcoming the limitations of current mRNA and protein biomarkers in COVID-19. Interestingly, our previous multicentric study could already confirm the TFAM-TFB2M interaction as a new biomarker, indicating mitochondrial recovery, in bacterial sepsis (19). Here, it was determined that this interaction serves as a precise indicator of disease severity and predicts clinical outcomes of septic patients as well. Thus, the mitochondrial biogenesis seems to be pivotal for recovery from bacterial sepsis as well as from critical COVID-19.
Since mitochondrial biogenesis seems to indicate disease severity and reflect organ dysfunction, an additional assessment of the affected organs would have been desirable. Thus, skeletal muscle biopsy studies have significantly contributed to our understanding of mitochondrial structure and function changes during sepsis and COVID-19 (28–31). However, these examinations are challenging due to the invasiveness of muscle biopsies and the time and effort required. Consequently, there is a growing interest in noninvasive tests using peripheral blood to provide information about a patient’s overall mitochondrial health. Assessing highly aerobic human PBMCs is particularly suitable for reflecting the mitochondrial function of other aerobic, adenosine triphosphate (ATP)-consuming organs such as the brain, heart, kidneys, liver, and lungs, which are susceptible to mitochondrial damage (15, 32). Therefore, the mitochondria of PBMCs likely serve as a useful proxy for the overall mitochondrial state in COVID-19 (9, 33, 34). Of note, the protein interaction between TFAM and TFB2M is just one promising example among many significant protein interactions involved in severe infections like critical COVID-19. Strikingly, our study demonstrates that assessing protein interactions can address major limitations of mRNA or protein biomarkers. Consequently, our work represents a crucial milestone that paves the way for innovative biomarkers in critical COVID-19. By using a proximity ligation assay to examine the interaction between TFAM and TFB2M, we were able to assess the mitochondrial activity of these proteins in situ with remarkable reliability (35).
Furthermore, despite the notable differences between bacterial sepsis and COVID-19, such as the immune response, we have been able to illuminate key shared pathophysiological mechanisms, for instance mitochondrial dysfunction and potential mislocalization of TFAM, underlying both conditions. Thus, organ dysfunction, characterized by mitochondrial dysfunction, and mitochondrial biogenesis, as hallmark of mitochondrial recovery, might represent a common pathomechanistical final stage of both, bacterial sepsis and COVID-19.
The limitations of this study are as follows: First, our study may introduce a selection bias, as we solely recruited on university ICUs and there potentially selected particular vulnerable patients. Second, we just examine the novel biomarker at one timepoint. Further studies are needed to investigate the TFAM-TFB2M-interatction during the course of COVID-19. Third, we did not differentiate among PBMC subpopulations. Thus, we cannot dismiss the possibility of varying contributions from different cell populations, potentially due to differences in mitochondrial content. Fourth, various factors impact on TFAM-TFB2M protein interactions, such as changes in mtDNA copy number, mitochondrial membrane potential, or import disorders of the transcription factors, which could impede accurate biological interpretations (36). Further studies will be needed to validate our findings and elaborate on the pathomechanistical role of mitochondrial biogenesis in COVID-19.
In conclusion, our findings highlight the importance of the TFAM-TFB2M protein interaction as a strong and independent predictor of 30-day survival in critical COVID-19. Strikingly, this potential novel biomarker targeting mitochondrial biogenesis is measurable in peripheral blood and does not require any invasive diagnostic procedure. In addition, with these findings we could elucidate a common pathomechanistical final stage of both, bacterial sepsis and COVID-19.
Data availability statement
The original contributions presented in the study are included in the article/supplementary material. Further inquiries can be directed to the corresponding author/s.
Ethics statement
The author(s) declare that financial support was received for the research and/or publication of this article. The studies involving humans were approved by Medical Faculty of the Ruhr-University Bochum (registration number #5047-14) Universitätsstraße 150. 44801 Bochum, Germany. The studies were conducted in accordance with the local legislation and institutional requirements. The participants provided their written informed consent to participate in this study.
Author contributions
BW: Writing – original draft, Writing – review & editing. PT: Writing – original draft, Writing – review & editing. LP: Writing – original draft, Writing – review & editing. KR: Writing – original draft, Writing – review & editing. BK: Writing – original draft, Writing – review & editing. FW: Writing – original draft, Writing – review & editing. BD: Writing – original draft, Writing – review & editing. ME: Writing – original draft, Writing – review & editing. BS: Writing – original draft, Writing – review & editing. SP: Writing – original draft, Writing – review & editing. NB: Writing – original draft, Writing – review & editing. MaA: Writing – original draft, Writing – review & editing. CP: Writing – original draft, Writing – review & editing. SE: Writing – original draft, Writing – review & editing. CW: Writing – original draft, Writing – review & editing. AZ: Writing – original draft, Writing – review & editing. TG: Writing – original draft, Writing – review & editing. AnW: Writing – original draft, Writing – review & editing. MU: Writing – original draft, Writing – review & editing. HW: Writing – original draft, Writing – review & editing. AlW: Writing – original draft, Writing – review & editing. LB: Writing – original draft, Writing – review & editing. MiA: Writing – original draft, Writing – review & editing. DZ: Writing – original draft, Writing – review & editing. TR: Writing – original draft, Writing – review & editing.
Funding
The author(s) declare that financial support was received for the research and/or publication of this article. The study was funded by the Deutsche Forschungsgemeinschaft (DFG) grant (RA 3613/1-1) and by institutional grants from the University Hospital Knappschaftskrankenhaus Bochum. Furthermore the CovidDataNet.NRW was funded by the Ministerium für Wirtschaft, Innovation, Digitalisierung und Energie (MWIDE), Federal State of North Rhine-Westphalia.
Conflict of interest
Authors TR and BK disclosed and issued patent application by their employer in context with the content of this manuscript.
The remaining authors declare that the research was conducted in the absence of any commercial or financial relationships that could be construed as a potential conflict of interest.
Publisher’s note
All claims expressed in this article are solely those of the authors and do not necessarily represent those of their affiliated organizations, or those of the publisher, the editors and the reviewers. Any product that may be evaluated in this article, or claim that may be made by its manufacturer, is not guaranteed or endorsed by the publisher.
References
1. Zhou F, Yu T, Du R, Fan G, Liu Y, Liu Z, et al. Clinical course and risk factors for mortality of adult inpatients with COVID-19 in Wuhan, China: a retrospective cohort study. Lancet. (2020) 395:1054–62. doi: 10.1016/S0140-6736(20)30566-3
2. Huang C, Wang Y, Li X, Ren L, Zhao J, Hu Y, et al. Clinical features of patients infected with 2019 novel coronavirus in Wuhan, China. Lancet. (2020) 395:497–506. doi: 10.1016/S0140-6736(20)30183-5
3. Gupta A, Madhavan MV, Sehgal K, Nair N, Mahajan S, Sehrawat TS, et al. Extrapulmonary manifestations of COVID-19. Nat Med. (2020) 26:1017–32. doi: 10.1038/s41591-020-0968-3
4. Fredriksson K, Tjader I, Keller P, Petrovic N, Ahlman B, Scheele C, et al. Dysregulation of mitochondrial dynamics and the muscle transcriptome in ICU patients suffering from sepsis induced multiple organ failure. PloS One. (2008) 3:e3686. doi: 10.1371/journal.pone.0003686
5. Soria-Castro E, Soto ME, Guarner-Lans V, Rojas G, Perezpena-Diazconti M, Criales-Vera SA, et al. The kidnapping of mitochondrial function associated with the SARS-CoV-2 infection. Histol Histopathol. (2021) 36:947–65. doi: 10.14670/HH-18-354
6. Faizan MI, Chaudhuri R, Sagar S, Albogami S, Chaudhary N, Azmi I, et al. NSP4 and ORF9b of SARS-coV-2 induce pro-inflammatory mitochondrial DNA release in inner membrane-derived vesicles. Cells. (2022) 11(19). doi: 10.3390/cells11192969
7. Leopold V, Chouchane O, Butler JM, Schuurman AR, Michels EHA, de Brabander J, et al. Platelets of COVID-19 patients display mitochondrial dysfunction, oxidative stress, and energy metabolism failure compatible with cell death. Res Pract Thromb Haemost. (2023) 7:102213. doi: 10.1016/j.rpth.2023.102213
8. Mo Y, To KK, Zhou R, Liu L, Cao T, Huang H, et al. Mitochondrial dysfunction associates with acute T lymphocytopenia and impaired functionality in COVID-19 patients. Front Immunol. (2021) 12:799896. doi: 10.3389/fimmu.2021.799896
9. Ramachandran K, Maity S, Muthukumar AR, Kandala S, Tomar D, Abd El-Aziz TM, et al. SARS-CoV-2 infection enhances mitochondrial PTP complex activity to perturb cardiac energetics. iScience. (2022) 25:103722.
10. Shang C, Liu Z, Zhu Y, Lu J, Ge C, Zhang C, et al. SARS-coV-2 causes mitochondrial dysfunction and mitophagy impairment. Front Microbiol. (2021) 12:780768. doi: 10.3389/fmicb.2021.780768
11. Turton N, Millichap L, and Hargreaves IP. Potential biomarkers of mitochondrial dysfunction associated with COVID-19 infection. Adv Exp Med Biol. (2023) 1412:211–24. doi: 10.1007/978-3-031-28012-2_11
12. Valdes-Aguayo JJ, Garza-Veloz I, Vargas-Rodriguez JR, Martinez-Vazquez MC, Avila-Carrasco L, Bernal-Silva S, et al. Peripheral blood mitochondrial DNA levels were modulated by SARS-coV-2 infection severity and its lessening was associated with mortality among hospitalized patients with COVID-19. Front Cell Infect Microbiol. (2021) 11:754708. doi: 10.3389/fcimb.2021.754708
13. Shoraka S, Samarasinghe AE, Ghaemi A, and Mohebbi SR. Host mitochondria: more than an organelle in SARS-CoV-2 infection. Front Cell Infect Microbiol. (2023) 13:1228275. doi: 10.3389/fcimb.2023.1228275
14. Scozzi D, Cano M, Ma L, Zhou D, Zhu JH, O’Halloran JA, et al. Circulating mitochondrial DNA is an early indicator of severe illness and mortality from COVID-19. JCI Insight. (2021) 6(4). doi: 10.1172/jci.insight.143299
15. Kraft BD, Chen L, Suliman HB, Piantadosi CA, and Welty-Wolf KE. Peripheral blood mononuclear cells demonstrate mitochondrial damage clearance during sepsis. Crit Care Med. (2019) 47:651–8. doi: 10.1097/CCM.0000000000003681
16. Rahmel T, Marko B, Nowak H, Bergmann L, Thon P, Rump K, et al. Mitochondrial dysfunction in sepsis is associated with diminished intramitochondrial TFAM despite its increased cellular expression. Sci Rep. (2020) 10:21029. doi: 10.1038/s41598-020-78195-4
17. Campbell CT, Kolesar JE, and Kaufman BA. Mitochondrial transcription factor A regulates mitochondrial transcription initiation, DNA packaging, and genome copy number. Biochim Biophys Acta. (2012) 1819:921–9. doi: 10.1016/j.bbagrm.2012.03.002
18. Kasashima K, Sumitani M, and Endo H. Human mitochondrial transcription factor A is required for the segregation of mitochondrial DNA in cultured cells. Exp Cell Res. (2011) 317:210–20. doi: 10.1016/j.yexcr.2010.10.008
19. Thon P, Trübner E, Zimmer F, Palmowski L, Ehrentraut SF, Putensen C, et al. Protein interaction assessing mitochondrial biogenesis as a next generation biomarker in sepsis: A prospective cohort study. CHEST Crit Care. (2024) 2(2). doi: 10.1016/j.chstcc.2024.100065
20. Lambden S, Laterre PF, Levy MM, and Francois B. The SOFA score-development, utility and challenges of accurate assessment in clinical trials. Crit Care. (2019) 23:374. doi: 10.1186/s13054-019-2663-7
21. Edinger F, Edinger S, Koch C, Markmann M, Hecker M, Sander M, et al. Peak Plasma Levels of mtDNA Serve as a Predictive Biomarker for COVID-19 in-Hospital Mortality. J Clin Med. (2022) 11(23). doi: 10.3390/jcm11237161
22. Hepokoski ML, Odish M, Lam MT, Coufal NG, Rolfsen ML, Shadel GS, et al. Absolute quantification of plasma mitochondrial DNA by droplet digital PCR marks COVID-19 severity over time during intensive care unit admissions. Am J Physiol Lung Cell Mol Physiol. (2022) 323(1):L84–92. doi: 10.1152/ajplung.00128.2022
23. Wei Z, Kwan BC, Chow KM, Cheng PM, Luk CC, Lai KB, et al. Urinary mitochondrial DNA level as a biomarker of tissue injury in non-diabetic chronic kidney diseases. BMC Nephrol. (2018) 19:367. doi: 10.1186/s12882-018-1178-9
24. Tumburu L, Ghosh-Choudhary S, Seifuddin FT, Barbu EA, Yang S, Ahmad MM, et al. Circulating mitochondrial DNA is a proinflammatory DAMP in sickle cell disease. Blood. (2021) 137:3116–26. doi: 10.1182/blood.2020009063
25. Shao H, Lan D, Duan Z, Liu Z, Min J, Zhang L, et al. Upregulation of mitochondrial gene expression in PBMC from convalescent SARS patients. J Clin Immunol. (2006) 26:546–54. doi: 10.1007/s10875-006-9046-y
26. Duan C, Ma R, Zeng X, Chen B, Hou D, Liu R, et al. SARS-coV-2 achieves immune escape by destroying mitochondrial quality: comprehensive analysis of the cellular landscapes of lung and blood specimens from patients with COVID-19. Front Immunol. (2022) 13:946731. doi: 10.3389/fimmu.2022.946731
27. Shoraka S, Mohebbi SR, Hosseini SM, and Zali MR. Comparison of plasma mitochondrial DNA copy number in asymptomatic and symptomatic COVID-19 patients. Front Microbiol. (2023) 14:1256042. doi: 10.3389/fmicb.2023.1256042
28. Brealey D, Brand M, Hargreaves I, Heales S, Land J, Smolenski R, et al. Association between mitochondrial dysfunction and severity and outcome of septic shock. Lancet. (2002) 360:219–23. doi: 10.1016/S0140-6736(02)09459-X
29. Carre JE, Orban JC, Re L, Felsmann K, Iffert W, Bauer M, et al. Survival in critical illness is associated with early activation of mitochondrial biogenesis. Am J Respir Crit Care Med. (2010) 182:745–51. doi: 10.1164/rccm.201003-0326OC
30. Fredriksson K, Hammarqvist F, Strigard K, Hultenby K, Ljungqvist O, Wernerman J, et al. Derangements in mitochondrial metabolism in intercostal and leg muscle of critically ill patients with sepsis-induced multiple organ failure. Am J Physiol Endocrinol Metab. (2006) 291:E1044–50. doi: 10.1152/ajpendo.00218.2006
31. Dodig D, Tarnopolsky MA, Margeta M, Gordon K, Fritzler MJ, and Lu JQ. COVID-19-associated critical illness myopathy with direct viral effects. Ann Neurol. (2022) 91:568–74. doi: 10.1002/ana.26318
32. Kramer PA, Ravi S, Chacko B, Johnson MS, and Darley-Usmar VM. A review of the mitochondrial and glycolytic metabolism in human platelets and leukocytes: implications for their use as bioenergetic biomarkers. Redox Biol. (2014) 2:206–10. doi: 10.1016/j.redox.2013.12.026
33. Tian M, Liu W, Li X, Zhao P, Shereen MA, Zhu C, et al. HIF-1alpha promotes SARS-CoV-2 infection and aggravates inflammatory responses to COVID-19. Signal Transduct Target Ther. (2021) 6:308. doi: 10.1038/s41392-021-00726-w
34. Streng L, de Wijs CJ, Raat NJH, Specht PAC, Sneiders D, van der Kaaij M, et al. In vivo and ex vivo mitochondrial function in COVID-19 patients on the intensive care unit. Biomedicines. (2022) 10. doi: 10.3390/biomedicines10071746
35. Koos B, Andersson L, Clausson CM, Grannas K, Klaesson A, Cane G, et al. Analysis of protein interactions in situ by proximity ligation assays. Curr Top Microbiol Immunol. (2014) 377:111–26. doi: 10.1007/82_2013_334
Keywords: COVID-19, mitochondrial dysfunction, TFAM, mitochondrial biogenesis, protein interaction, proximity ligation assay
Citation: Westhus B, Thon P, Palmowski L, Rump K, Koos B, Wiebel F, Dyck B, Eisenacher M, Sitek B, Pfaender S, Babel N, Anft M, Putensen C, Ehrentraut SF, Weisheit C, Zarbock A, von Groote T, Witowski A, Unterberg M, Nowak H, Wolf A, Bergmann L, Adamzik M, Ziehe D and Rahmel T (2025) The protein interaction of mitochondrial transcription factors A and B2 is associated with 30-day survival in critical COVID-19. Front. Immunol. 16:1445403. doi: 10.3389/fimmu.2025.1445403
Received: 12 June 2024; Accepted: 31 March 2025;
Published: 16 May 2025.
Edited by:
Pascal Pineau, Institut Pasteur, FranceReviewed by:
Stelvio Tonello, University of Eastern Piedmont, ItalyShahrzad Shoraka, Shahid Beheshti University, Iran
Copyright © 2025 Westhus, Thon, Palmowski, Rump, Koos, Wiebel, Dyck, Eisenacher, Sitek, Pfaender, Babel, Anft, Putensen, Ehrentraut, Weisheit, Zarbock, von Groote, Witowski, Unterberg, Nowak, Wolf, Bergmann, Adamzik, Ziehe and Rahmel. This is an open-access article distributed under the terms of the Creative Commons Attribution License (CC BY). The use, distribution or reproduction in other forums is permitted, provided the original author(s) and the copyright owner(s) are credited and that the original publication in this journal is cited, in accordance with accepted academic practice. No use, distribution or reproduction is permitted which does not comply with these terms.
*Correspondence: Dominik Ziehe, RG9taW5pay5aaWVoZUBydWhyLXVuaS1ib2NodW0uZGU=
†These authors have contributed equally to this work