- 1The Department of Biomedical and Clinical Sciences (BKV), Linköping University, Linköping, Sweden
- 2Clinical Genomics Linköping, Linköping University, Linköping, Sweden
- 3Department of Hand Surgery and Plastic Surgery and Burns, University Hospital, Linköping, Sweden
Management of difficult-to-heal skin wounds presents a significant clinical challenge, particularly when associated with inflammatory skin disorders. The differentiation of stem cells into keratinocyte-like cells has been explored as a potential regenerative therapy. Ras-related protein (Rab) 7, a key regulator of membrane trafficking, influences the activity of several growth factors. In this study, the competitive Rab7 inhibitor, CID-1067700, was investigated for the differentiation of adipose-derived stem cell into keratinocyte-like cells. This treatment upregulated several epidermal markers, including P63, cytokeratin 5 and 14 and filaggrin, while downregulated the stem cell marker, vimentin. Microarray data showed upregulation of the anti-inflammatory gene HMOX-1, coupled with the downregulation of various inflammation-related pathways, such as TNF, chemokine, AGE-RAGE and IL-17 signalling cascades, as well as cytokine-cytokine receptor interaction pathways. Gene set enrichment analysis predicted Ehmt2, an epigenetic regulator, to be the top activated upstream regulator, enhancing the transcriptional activity. Protein array analysis showed reduced secretion of several inflammatory cytokines, including IL-1α, IL-8, IL-17A, and IL-32, while enhancing the secretion of the epidermal growth factor. Our findings provide preliminary evidence that CID-1067700, as an additive to the differentiation media, not only enhances stem cell differentiation into keratinocyte-like cells, but also improves their anti-inflammatory properties. These combined regenerative and anti-inflammatory properties may offer significant therapeutic potential for patients with chronic skin wounds, particularly those with underlying inflammatory conditions.
1 Introduction
The skin functions as a multi-layered barrier against environmental hazards and plays a crucial role in various physiological processes, including thermoregulation and vitamin D synthesis. Despite its remarkable regenerative capacity, the skin remains vulnerable to injury and disease, with chronic skin wounds representing a significant clinical challenge. These wounds deviate from the normal healing process, persist in the inflammatory phase and fail to heal within the typical timeframe of three months (1). The impaired healing observed in chronic wounds is primarily attributed to dysregulated inflammatory responses, characterized by an imbalance between pro- inflammatory and anti-inflammatory cytokines (2). Furthermore, elevated levels of reactive oxygen species (3) and proteases (4) exacerbate cellular damage and degrade extracellular matrix, thereby further impeding the wound healing process.
Chronic wounds are frequently associated with an underlying condition, such as diabetes, venous insufficiency, and arterial insufficiency (5, 6). Notably, over 20% of chronic wounds exhibit inflammatory or autoimmune components, complicating wound management and significantly increasing patient morbidity. This leads to doubling of the failure rate for standard wound management procedures (7). Systemic autoimmune diseases are frequently associated with lower leg ulcers, which further challenges the therapeutic interventions and prolongs the healing process. For example, rheumatoid arthritis is linked with leg ulcers in more than 26% of the patients on long-term follow-up (8). Similarly, scleroderma is associated with lower leg ulcers in 4% of patients, with 70% presented bilaterally (9). In cases of pyoderma gangrenosum, surgical intervention can exacerbate skin wounds associated with ruptured bullae (7). The classical treatment for these conditions is long-term corticosteroids, which are known to impair skin wound healing (10).
In developed nations, the prevalence of chronic wounds ranges from 1% to 2% of the general population, with incidence rates exceeding 5% among the elderly (11). The economic burden of chronic wounds is substantial, with healthcare expenditures exceeding €16.8 billion in Germany, SEK 23 billion in Sweden, £8.8 billion in the UK, and $145 billion in US (12–14). Moreover, these wounds profoundly impact the patients’ quality of life, causing chronic pain, depression, anxiety, and social isolation (1).
Traditional treatments for chronic wounds, such as skin grafts, often prove insufficient and may result in complications at the donor site (15). The advancements in biomaterial production have led the development of novel wound dressings which can offer help for some patients. Unfortunately, there are challenges associated with these dressings, including incorporation in the wound, hypersensitivity to animal-derived components, cost and availability (16). Stem cell-based therapy has emerged as a promising approach in wound repair, offering anti-inflammatory properties and an ability to accelerate the healing process (17). Adipose-derived stem cells (ASCs) demonstrate significant potential for treating inflammatory and autoimmune skin conditions due to their anti-inflammatory paracrine secretion and regenerative capabilities (18). The therapeutic potential of ASCs in managing chronic wounds is particularly noteworthy. Studies have shown ASCs ability to transdifferentiate into various cell lineages, including keratinocyte-like cells (KLCs) in vitro (19–21). Cellular differentiation is known to induce dynamic changes in gene expression, protein secretion, and physical properties, including membrane trafficking. The latter has emerged as a critical regulator governing stem cell differentiation and fate acquisition, mediated through pivotal signalling pathways (22). Ras-related protein 7 (Rab7) is a master regulator of membrane trafficking and plays essential roles in endosome maturation, vesicle transport from early to late endosome, lysosome biogenesis, and autophagy via its GTPase activity (23, 24). Thus, Rab7 influences the activity, localization, and degradation of signalling receptors and ligands involved in key developmental pathways, including fibroblast growth factor (25), epidermal growth factor (26), and ligands from the Wnt (27), Notch (28) and Hedgehog (29) pathways. Interestingly, Rab7 influences various signalling pathways that are activated during inflammation. For instance, it can affect the NF-κB pathway, which is a key regulator of inflammatory responses (30).
Our unpublished data identified Rab GDP dissociation inhibitor alpha as a key secretion by keratinocytes that could be responsible for enhancing the differentiation of ASCs into KLCs. As Rab7 is a known target for this inhibitor, we hypothesized that inhibiting Rab7 with a small molecule could enhance the differentiation of ASCs into KLCs. Thus, this study aimed to investigate the molecular mechanisms underlying Rab7-mediated stem cell differentiation into keratinocyte-like cells, through comprehensive molecular analyses as well as protein profiling. We hypothesized that Rab7 inhibition enhances ASC differentiation into KLCs with anti-inflammatory properties via altered membrane trafficking.
2 Materials and methods
2.1 Cell culture
The adipose-derived mesenchymal stem cell (ASCs) line, ASC52telo (SCRC-4000, ATCC) immortalized with human telomerase reverse transcriptase (hTERT), were selected for their consistent stem cell properties and reproducibility. This cell line is widely used in literature for modelling primary stem cells and showed comparable findings to primary cells in terms of biology, metabolism, differentiation and even gene expression pattern (21, 31–33). ASCs were expanded in Dulbecco’s Modified Eagle Medium (DMEM; Thermo Fisher, Cat. 31885049) supplemented with 10% fetal bovine serum (FBS, Thermo Fisher, Cat. 10500064) and 1% penicillin/streptomycin, in a humidified incubator at 37°C with 5% CO₂.
2.2 Cell viability assay
The cytotoxicity of CID-106770 (Sigma-Aldrich, Cat. SML0545) was examined using the 3-(4,5-Dimethylthiazol-2-yl)-2,5-Diphenyltetrazolium Bromide (MTT) assay. Briefly, 3 × 103 cells in 200 μl of DMEM were seeded onto 96-well culture plates and the concentrations of 0, 20, 40, 60, 80, and 100 μM of CID-106770 were applied for 24 or 72 hours. A vehicle control of cells treated with 0.1% DMSO (Sigma-Aldrich, Cat. 472301) were also included in the experiment. The MTT solution (5 mg/ml; Sigma-Aldrich, Cat No. M5655) was added to each well, and the cells were incubated at 37°C for 4 hours. The formazan crystals were dissolved in 200 μl DMSO, and the absorbance was measured at 570 nm (Spectra Max plus 384, Molecular Devices). The normalized absorbance of each treatment was divided by the absorbance of untreated cells to calculate the fold change of cell survival relative to untreated control.
2.3 Cell differentiation and treatment
To induce the differentiation of ASCs into KLCs, the cells were cultured in a differentiation media (DM) consisted of EpiLife™ Medium (ThermoFisher, Cat. MEPI500CA) supplemented with EpiLife™ Defined Growth Supplement (EDGS, ThermoFisher, Cat. S0125) and 1% penicillin/streptomycin, along with previously published differentiation-inducing additives summarized in Table 1 (34). The cells were cultured on 2 μg/cm² of collagen type I from rat tail (Fisher Scientific, Cat. 10114532). The study included three experimental groups: a control group with cells cultured in DM, a vehicle control group with cells treated with DM and 0.1% DMSO, and a treatment group that received DM and Rab7 inhibitor, CID-1067700 at 40 μM. The differentiation media, along with the respective additives, was changed twice weekly, for ten days (Figure 1).
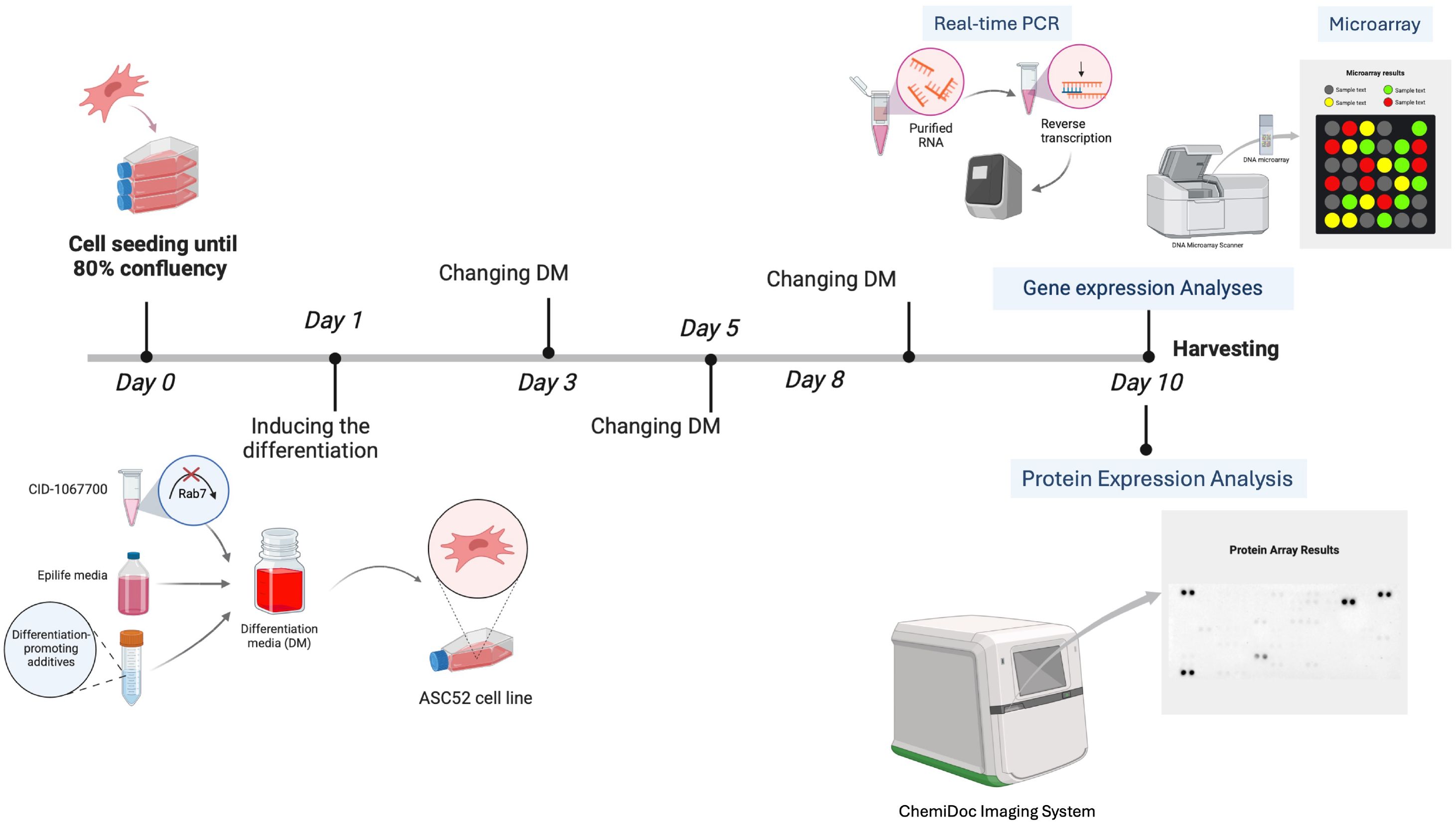
Figure 1. A simplified illustration of the experimental design. Figure created with BioRender.com.
2.4 Gene expression analysis
2.4.1 RNA extraction
Total RNA was extracted using the RNeasy Plus Mini kit (Qiagen, Cat. 74134), according to the manufacturer’s instructions. RNA was quantified using a Nanodrop spectrophotometer (ThermoFisher, Cat. 701-058112) and reverse transcribed with the Maxima™ First Strand cDNA Synthesis Kit (ThermoFisher, Cat. K1642).
2.4.2 Microarray analysis
RNA samples were assessed for size and integrity using the Agilent 2100 Bioanalyzer (Agilent Technologies). Samples with RNA Integrity Number (RIN) ≥ 9.6 were hybridized with the Human Clariom™ S microarray kit (Thermo Fisher Scientific, Cat. 902926), following the manufacturer’s recommended protocol. Post-hybridization, the arrays were washed and stained using the GeneChip™ Expression Wash, Stain, and Scan Kit (Thermo Fisher Scientific, Cat. 900720) and the GeneChiptm Fluidics Station 450 (Thermo Fisher Scientific) to remove non-specific binding and enhance signal detection. Subsequently, the arrays were scanned with the GeneChip Scanner 3000 7G (Affymetrix, Santa Clara). Data pre-processing, quality control, and the calculation of log2 fold-changes between sample expression values were performed using the Transcriptome Analysis Console Software (Affymetrix). Differentially expressed genes (DEGs) were identified based on a log2 fold change value |FC| of ≥ 1.5 and an adjusted false discovery rate (FDR) p-value < 0.05.
2.4.3 Bioinformatic analysis
Pathway enrichment analysis was conducted on all statistically significant DEGs using iPathwayGuide™ (Advaita Bioinformatics, MI, USA). The gene interactions and pathways were computed with reference to the Kyoto Encyclopaedia of Genes and Genomes (KEGG) database (www.genome.jp/kegg). In addition to pathway enrichment analysis, the most significantly enriched gene ontology (GO) biological processes associated with DEGs were evaluated using the iPathwayGuide software. Only differentially expressed genes exhibiting a fold change of ≥ |1.5| and a false discovery rate (FDR) of ≤0.05 were included in the analysis.
2.4.4 Real-time Polymerase chain reaction
Real-time polymerase chain reaction (qPCR) was performed using the 7500 Real-Time PCR System (Applied Biosystems, Cat. 4351104) and the PowerUp™ SYBR™ Green Master Mix (Fisher Scientific, Cat. 15390929), following the manufacturer’s instructions. The primer sequences are listed in Table 2. The following thermal cycling conditions were used: UDG (uracil-DNA glycosylase) activation at 50°C for 2 minutes, polymerase activation at 95°C for 2 minutes, followed by 40 cycles of denaturation at 95°C for 3 seconds and annealing/extension at 60°C for 30 seconds. Gene expression levels were normalized to GAPDH and calculated relative to the control group using the 2-∆∆Cq method.
2.5 Proteome profiling
Cell culture supernatant was collected on day 10 of differentiation. The differential protein expression between the control and treatment group was assessed using the Proteome Profiler™ Human XL Cytokine Array (R&D Systems, Cat. ARY022B), according to the manufacturer’s protocol. Briefly, the array membranes were incubated with the blocking buffer for one hour on a rocking platform, followed by overnight incubation at 4°C with 500 μL of cell culture supernatant. The arrays were then incubated with a cocktail of biotinylated detection antibodies for one hour, followed by chemiluminescent detection using Streptavidin-HRP. The membranes were imaged using the ChemiDoc™ MP imaging system (Bio-Rad, Cat. 12003154), and the pixel intensities were analysed using Fiji (35).
2.6 Surface protein characterization
Immunofluorescence staining was conducted to assess the differentiation of ASCs into KLCs based on their expression of epithelial differentiation markers. The cells were fixed with 4% ice-cold methanol for 20 minutes and permeabilized using 0.5% Triton X-100 for 15 minutes. Nonspecific binding was blocked using 3% bovine serum albumin (Sigma-Aldrich, Cat. A9418). The cells were incubated with the primary antibodies (listed in Table 3) overnight at 4°C and with the fluorescently labelled secondary antibodies for one hour at room temperature. Nuclear counterstaining was performed using NucBlue™ Fixed Cell ReadyProbes™ Reagent (DAPI, Fisher Scientific, Cat. R37606) for 2 minutes, followed by rinsing with PBS. The cells were visualized at 20x magnification using a fluorescent inverted microscope (DMi8, Leica), and image analysis was conducted using Fiji (35) by dividing the fluorescence intensity of positive cells by the total number of cells. Data are reported as a fold change in fluorescence intensity unit per cell (FU/cell) and relative to the cells treated with differentiation media only.
2.7 Statistical analysis
The data are presented as mean ± standard deviation (SD) of at least three experimental replicates, unless stated otherwise. Statistical analysis was conducted using IBM SPSS Statistics software, version 29. Statistical differences were determined by independent samples t-test or one-way analysis of variance (ANOVA), followed by Tukey’s post hoc test for cases with equal variance or Dunnett’s T3 test for cases with unequal variance. The homogeneity of variances was confirmed using Levene’s Test. p-value < 0.05 was considered statistically significant.
3 Results
3.1 CID-106770 did not affect the cell viability at the studied concentrations
The potential cytotoxic effect of CID-106770 on ASCs viability was evaluated after 24-hour and 72-hour. None of the tested concentrations (20, 40, 60, 80, and 100 μM) of CID-106770 or the vehicle control (0.1% DMSO) exhibited a significant cytotoxic effect on ASCs cell viability when compared to untreated cells (Figure 2A). CID-1067700 was selected at 40 μM for further experiments as this concentration was demonstrated in literature to efficiently inhibit Rab7 activity, while maintaining cell viability and minimizing cytotoxicity (36–38).
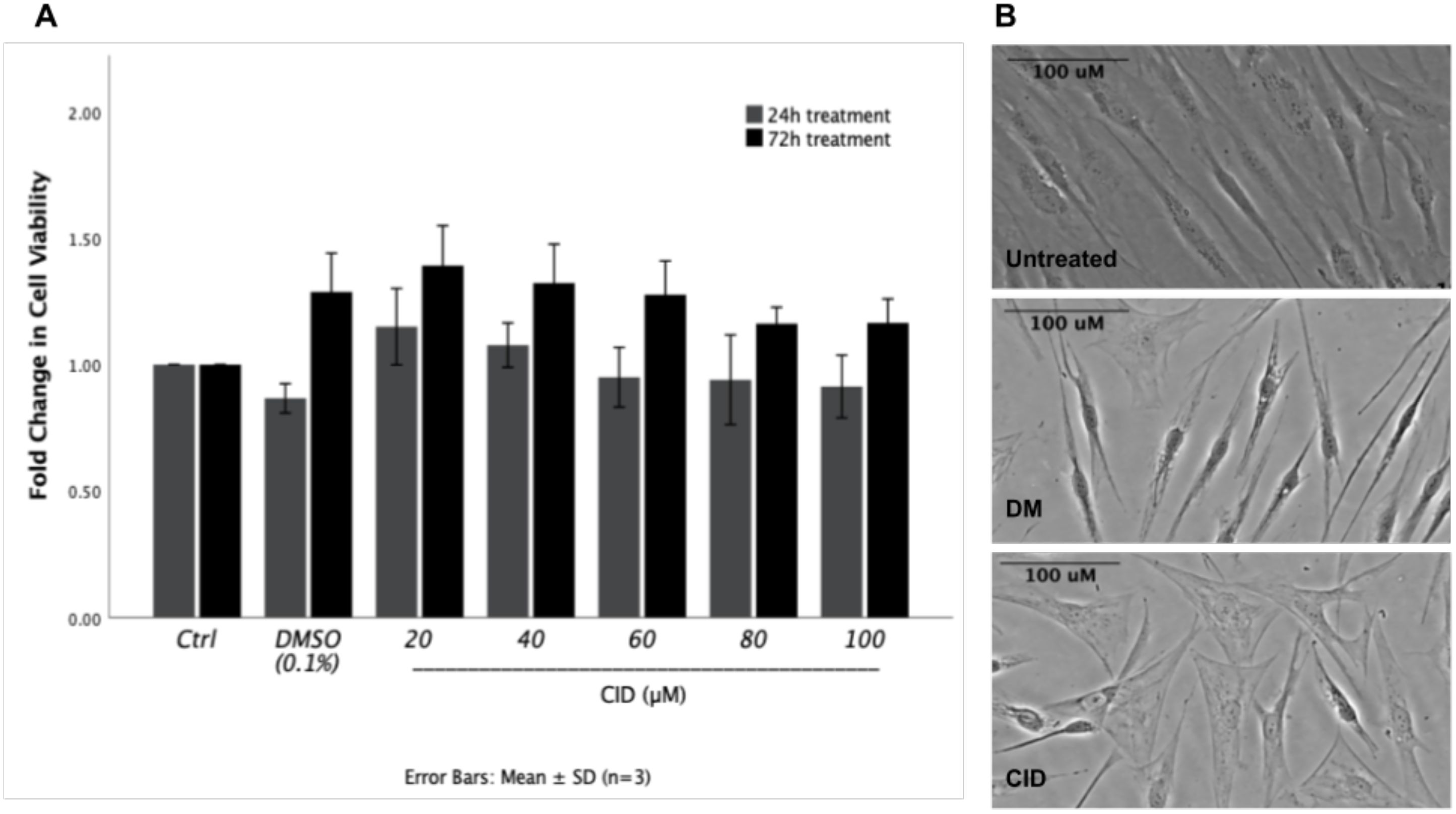
Figure 2. Effect of Rab7 inhibition on the viability and morphology of ASCs. (A) Viability of ASCs after treatment with different concentrations of CID-1067700 at 24 and 72 hours. No significant changes in cell viability were observed. (B) Representative bright-field images illustrating the morphological changes in ASCs after 10 days of treatment with differentiation media (DM) with and without 40 μM CID-1067700, in comparison to undifferentiated ASCs. Scale bars = 100 μm.
3.2 ASCs change of morphology upon treatment
Morphological changes were observed in ASCs after 10 days of treatment with differentiation media supplemented with CID-1067700. Untreated ASCs displayed typical fibroblast-like morphological characteristics. However, after 10 days of differentiation, cells treated with CID-1067700 showed high number of flattened cells (Figure 2B).
3.3 Rab7 inhibitor induced transcriptomic changes in ASCs
Microarray gene expression profiling was conducted to elucidate the genes and regulatory pathways influenced by Rab7 inhibition in ASCs. Principal Component Analysis (PCA) (Figure 3A) and Heatmap analysis (Supplementary Figure S1) of the transcriptomic profiles revealed that the vehicle group (DMSO) and the differentiation media control group (DM) were clustered together, indicating a negligible effect of the vehicle. Therefore, subsequent analyses focused on comparing the differences between CID-treated ASCs and those treated with DM. Out of 21,448 studied genes,134 DEGs were identified in ASCs treated with CID versus DM (Supplementary Table 1). Among these DEGs, 107 were downregulated and 27 were upregulated (Figure 3B).
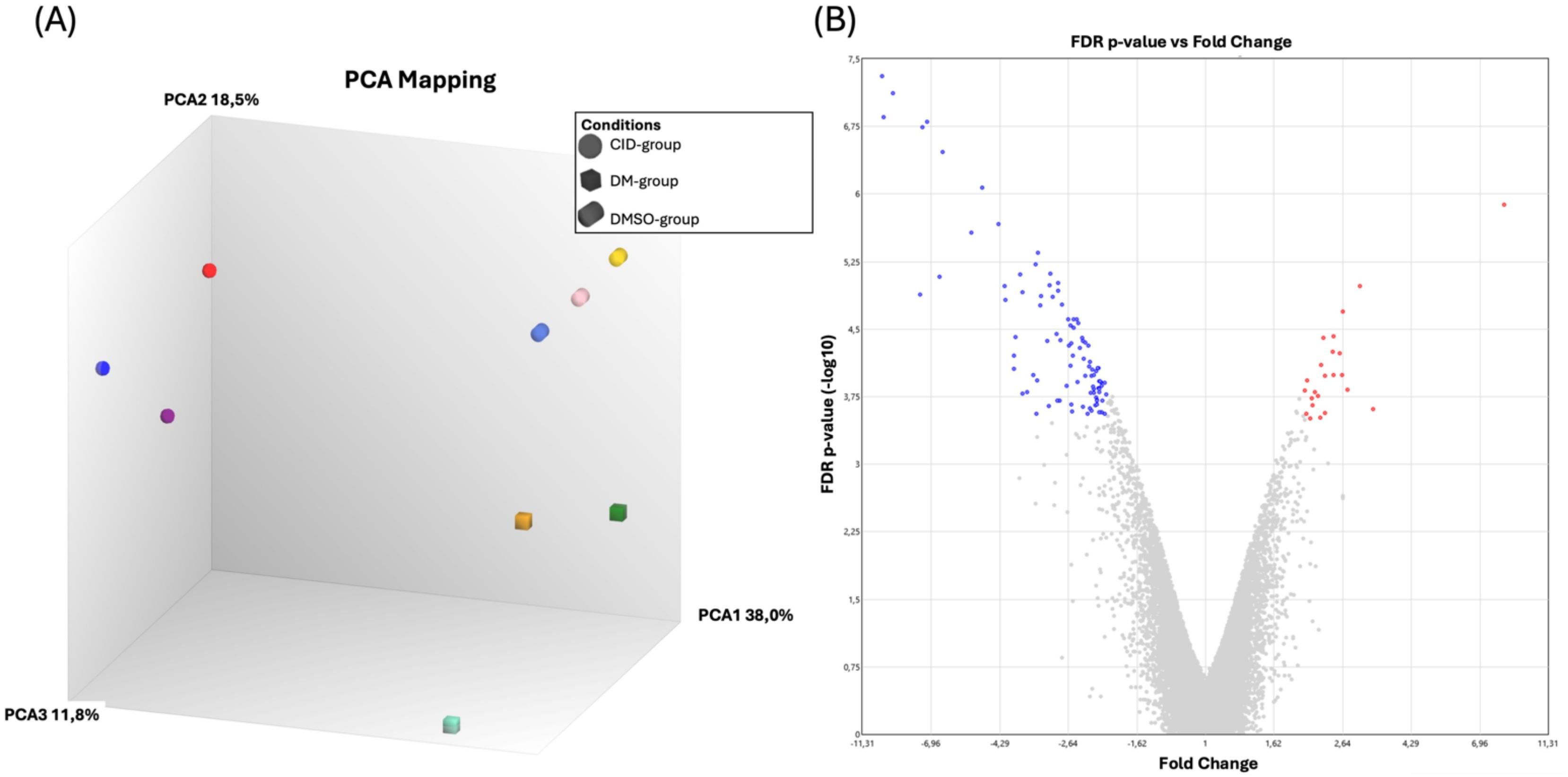
Figure 3. Rab7 inhibition leads to transcriptomic changes in ASCs. (A) Principal component analysis (PCA) of the transcriptomic profiles of cells treated as control (DM), with vehicle (DMSO), or with CID. PCA revealed clustering of the vehicle group with DM group, indicating a high degree of similarity between these two groups. (B) Volcano plot showed the differentially expressed genes (DEGs) in the CID-treated ASCs compared to those treated with DM. Downregulated genes are shown in blue, upregulated genes are in red, while insignificantly regulated genes are in grey.
3.4 Rab7 inhibition altered key signalling pathways and biological processes in ASCs
The identified DEGs were used as input for the iPathwayGuide software to determine significantly impacted pathways in response to CID-treatment. A total of 92 pathways were affected by CID treatment, encompassing: Chemokine Signalling Pathway with key genes such as VCAM1, CXCL1, and CXCL8 showing differential expression. This pathway is crucial for regulating immune cell migration and inflammatory responses. The Viral Protein Interaction with Cytokine and Cytokine Receptor Pathway also showed significant changes, with PIK3R3, CCL2, and COL1A1 genes being differentially expressed, indicating potential implications in immune response modulation and viral pathogenesis. Additionally, the TNF Signalling Pathway was notably impacted, with key genes including TNFSF10, CXCL6, and JAG1. This suggests a possible role in inflammation and apoptosis. The AGE-RAGE Signalling Pathway in Diabetic Complications was another critical pathway affected, with genes such as CXCL5, COL3A1, and TNFRSF11B showing differential expression, highlighting its importance in diabetic complications and cellular stress responses. The Cytokine-Cytokine Receptor Interaction Pathway was significantly affected, with IL1RL1, CXCL8, and PIK3R3 genes being key differentially expressed genes, underscoring its role in immune signalling and inflammation. Lastly, the IL-17 Signalling Pathway was significantly impacted, with CXCL1, CXCL6, and JAG1 genes showing differential expression, indicating its role in inflammatory and autoimmune responses (Figure 4A).
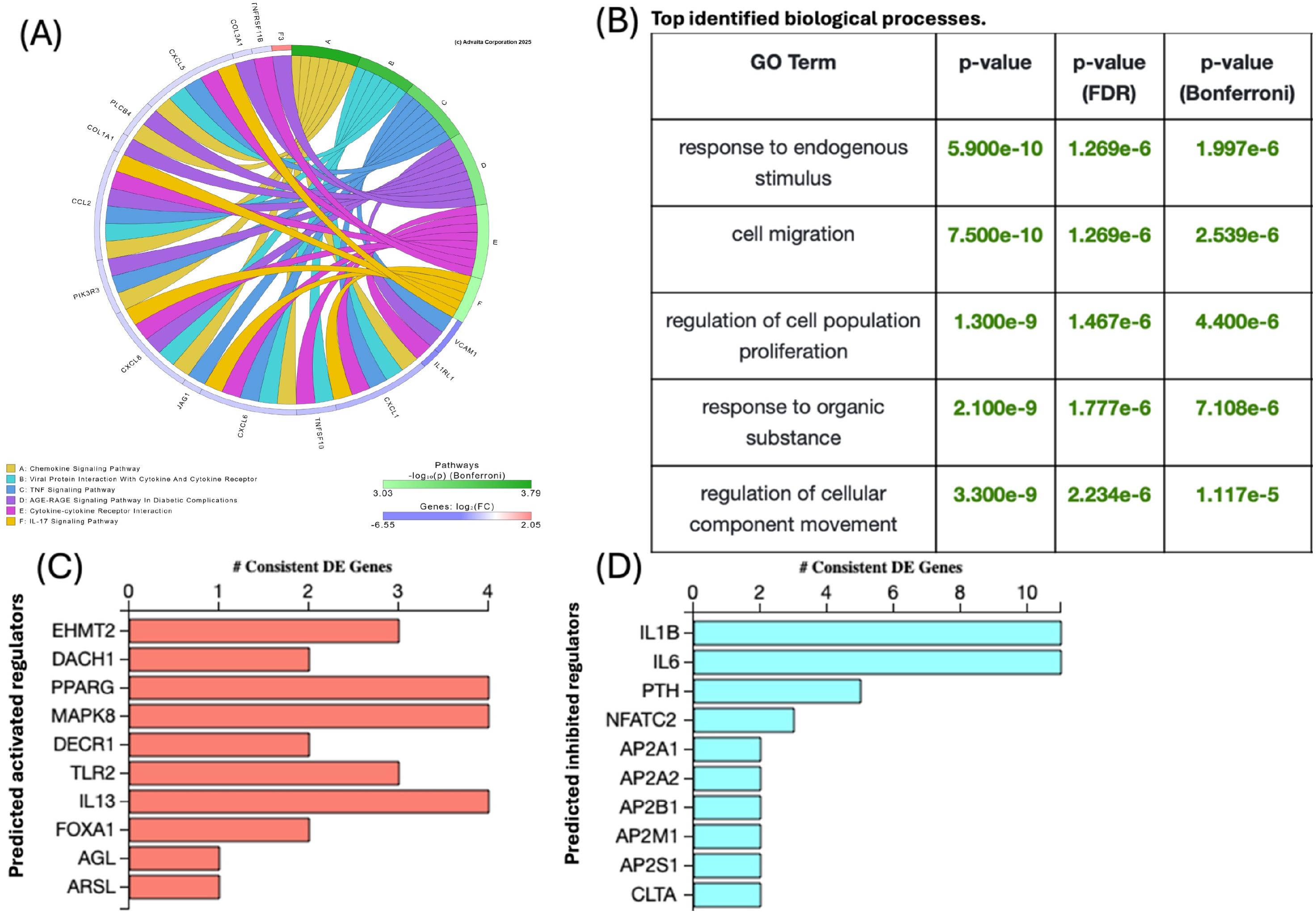
Figure 4. Impact of Rab7 inhibition on signalling pathways, biological processes, and upstream regulators in ASCs. (A) Chord Diagram of the top six significantly enriched pathways. (B) The top five enriched GO biological processes among the DEGs in the CID-treated ASCs. (C) Predicted activated or (D) inhibited upstream regulators for the DEGs in CID-treated ASCs compared to the control group. These predictions were made using the iPathwayGuide software.
We identified the top five biological processes significantly impacted by the differentially expressed genes in CID treated cells. These include the response to endogenous stimulus, cell migration, regulation of cell population proliferation, response to organic substance, and regulation of cellular (Figure 4B).
In this study, we identified several upstream regulators that are predicted to be either activated or inhibited, playing crucial roles in the differential expression of genes in CID treated cells. Among the activated regulators, EHMT2 (euchromatic histone lysine methyltransferase 2) stands out as a significant regulator. The differential expression of EHMT2 was consistent with its predicted activation, affecting key genes such as VCAM1, PDK4, and CXCL8. Other notable upstream regulators predicted to be activated include DACH1, PPARG, MAPK8, DECR1, TLR2, IL13, FOXA1, AGL, and ARSL. For instance, PPARG (peroxisome proliferator-activated receptor gamma) is involved in the regulation of adipogenesis and inflammation, while TLR2 (toll-like receptor 2) plays a critical role in the immune response to microbial infections. IL13 (interleukin 13) is a cytokine involved in the regulation of inflammatory responses, and FOXA1 is a transcription factor involved in gene expression during development (Figure 4C).
Conversely, several upstream regulators were predicted to be inhibited, including IL1B (interleukin 1 beta) and IL6 (interleukin 6), both key cytokines involved in the regulation of inflammatory responses and immune modulation (i.e., chronic inflammation and wound healing). Their predicted inhibition suggests a potential downregulation of inflammatory pathways, which could have implications for immune response and disease progression. Other inhibited regulators include PTH (parathyroid hormone), NFATC2 (nuclear factor of activated T-cells, cytoplasmic 2), and several adaptor proteins such as AP2A1, AP2A2, AP2B1, AP2M1, AP2S1, and CLTA. The inhibition of PTH suggests potential effects on calcium homeostasis and bone metabolism, while the inhibition of NFATC2 indicates possible impacts on T-cell activation and immune function (Figure 4D).
3.5 Differential gene expression was consistent by qPCR
To ensure the reliability of the microarray analysis, the expression levels of seven DEGs identified by the microarray analysis (COL81A, COL1A1, ITGB8, MGP, FGF7, VCAM1, and HMOX-1) were validated using qPCR. These genes were selected based on their relevance to keratinocyte functions or to validate their potential significance in ASCs differentiation. A strong correspondence between the microarray (Figure 5A) and the qPCR results (Figure 5B) was observed for all selected genes, confirming the reliability and accuracy of the microarray findings.
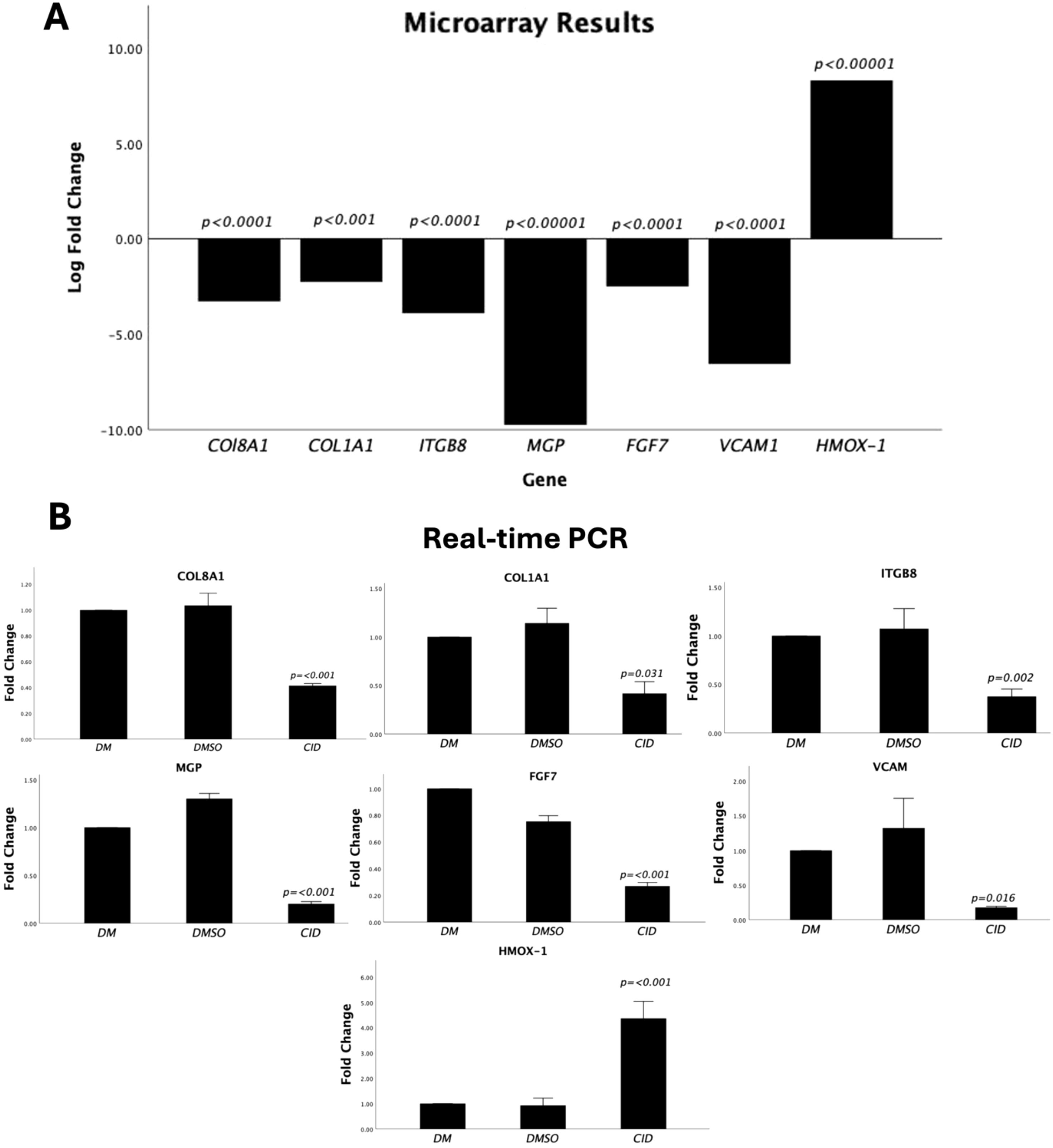
Figure 5. Validation of the expression levels of the differentially expressed genes identified by microarray with qPCR. (A) Bar chart displaying the relative expression levels of seven selected DEGs (COL81A, COL1A1, ITGB8, MGP, FGF7, VCAM1, and HMOX-1). Bars represent the log2-fold changes in gene expression in CID-1067700-treated ASCs (CID) compared to the control group (DM). (B) Validation of the expression levels of the selected genes by qPCR. The expression levels are normalized to GAPDH and calculated relative to the expression of ASCs treated with DM only using the 2-∆∆Cq method. Data are presented as mean ± standard deviation (SD) of three experimental replicates. The vehicle control was added to the qPCR to validate similarity with the negative control.
3.6 Rab7 inhibition induced proteomic changes in ASCs
The protein array revealed a decrease in the secretion levels of several proteins, including the inflammatory cytokines, IL-1α, IL-8, IL-17A, and IL-32, in CID-treated cells compared to the control group. These findings suggest that Rab7 inhibition may mitigate inflammatory responses in ASCs. Conversely, the treatment of CID resulted in an upregulation of epidermal growth factor (EGF), suggesting a potential enhancement in cellular mechanisms associated with wound healing and tissue regeneration (Figure 6).
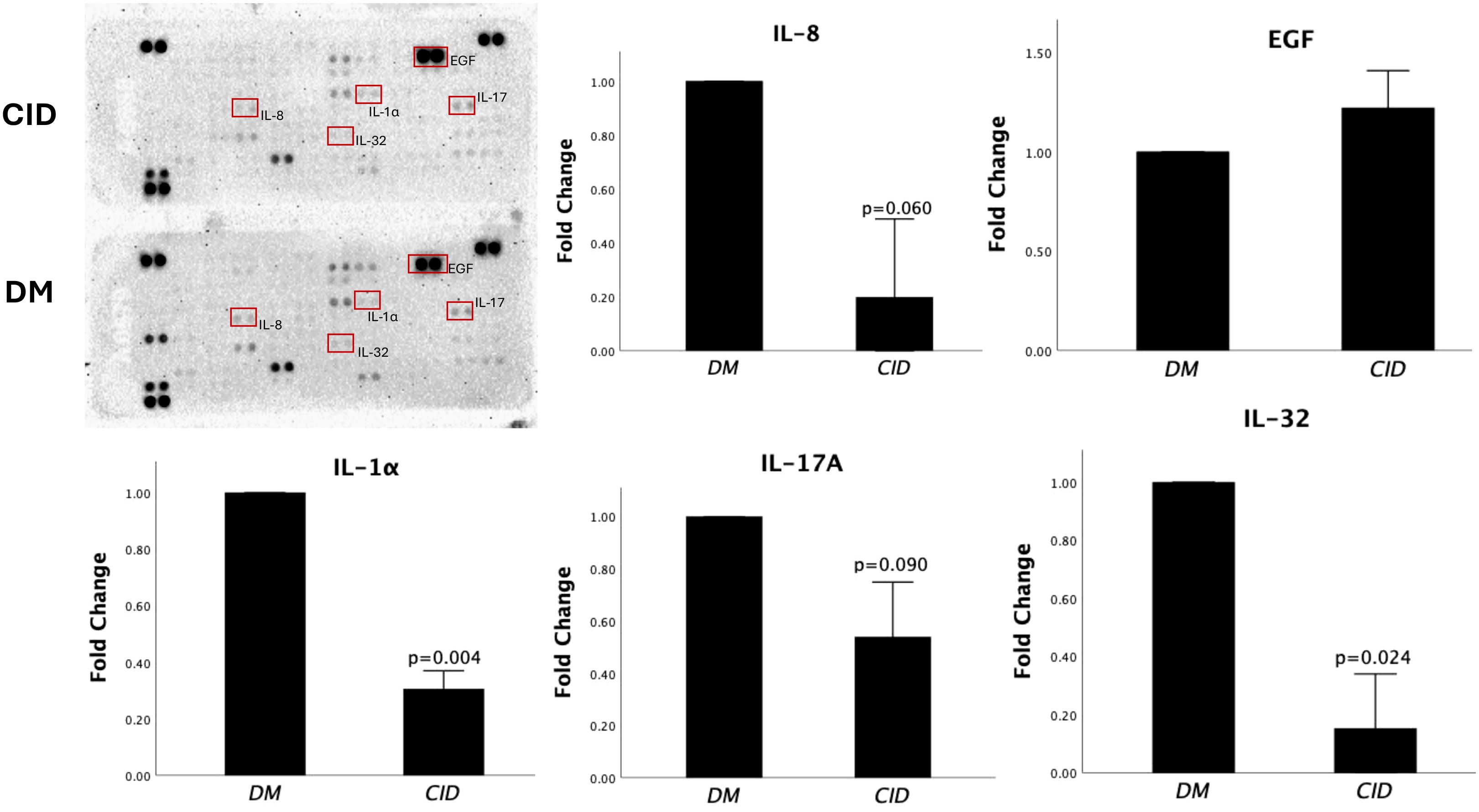
Figure 6. Rab7 Inhibition induces changes in the proteomic profile of ASCs. The upper left image shows the original scan of the protein array membrane. Noticeable reduction in the levels of inflammatory cytokines IL-1α, IL-8, IL-17A, and IL-32 were observed in CID-treated cells, whereas the expression level of EGF was increased. Bars represent the mean fold change in protein expression ± standard deviation (SD) of two experimental replicates in response to CID treatment compared to the control (DM).
3.7 Rab7 inhibition enhanced the expression of epithelial differentiation markers in ASCs
Immunofluorescence analysis revealed that Rab7 inhibition significantly increased the expression of several epithelial differentiation markers, including CK5 (p=0.024), CK14 (p=0.023), Filaggrin (p=0.006), and P63 (p=0.003) in ASCs treated with CID-1067700. The upregulation of CK5 and CK14 was validated further, using a conjugated antibody from a different provider (Supplementary Figure S2). These results indicate that a 10-day treatment with CID-1067700 not only induced the differentiation of ASCs into basal KLCs but also promoted their differentiation into differentiated KLCs, as supported by the presence of the intermediate differentiation marker, Filaggrin (Figure 7).
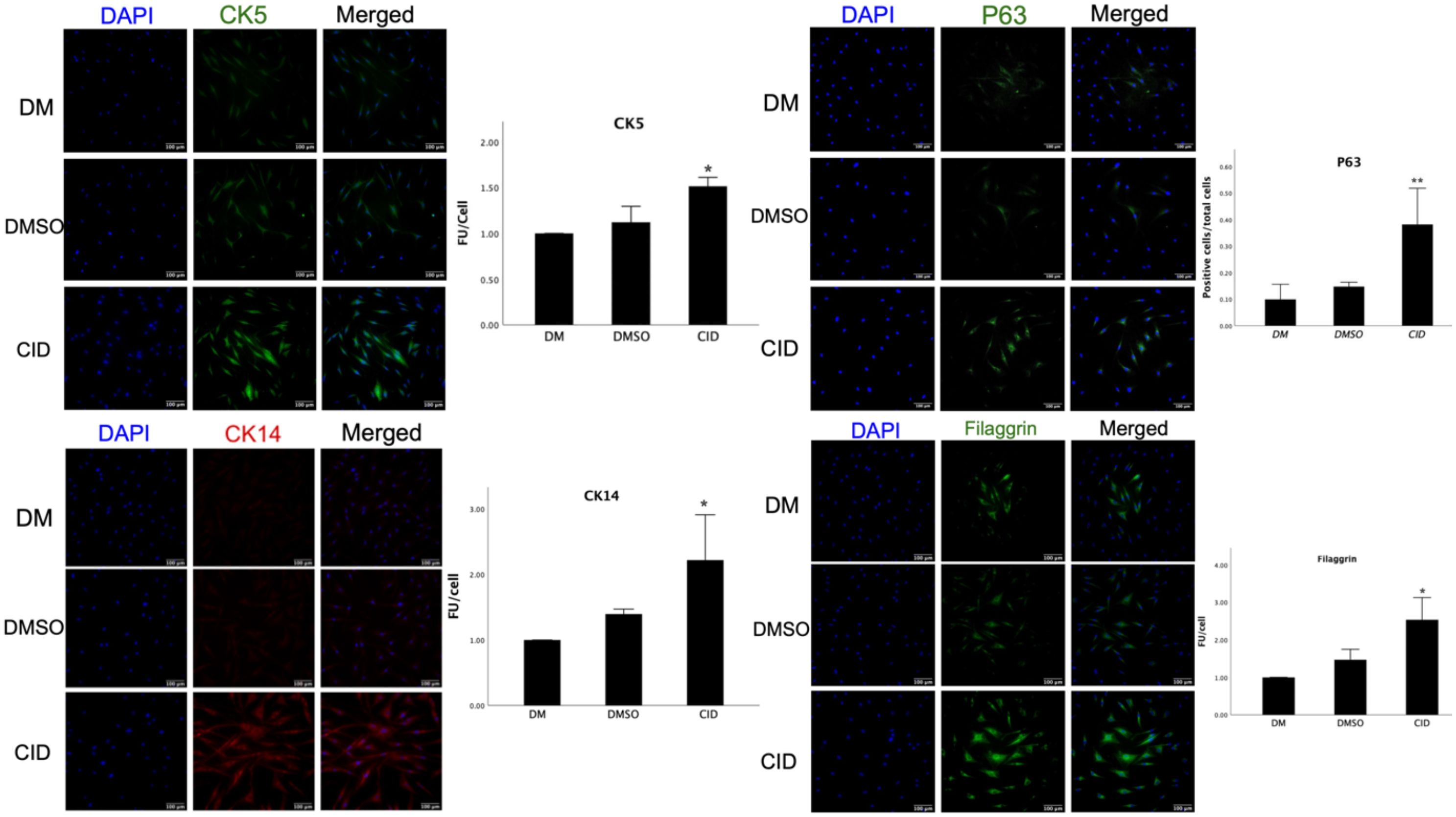
Figure 7. Rab7 Inhibition enhances the expression of epithelial differentiation markers in ASCs. The latter were cultured with differentiation media for ten days with and without CID-1067700, and immunocytochemistry analysis was performed to assess the expression of Cytokeratin 5 and 14, as well as Filaggrin and P63. Nuclei were counterstained with DAPI (blue). The bars indicate the mean fluorescence intensity unit (FU) per cell ± SD of 3 replicates. Asterisks represent significant difference compared to cells treated with differentiation media (DM) only (* p<0.5, ** p<0.01). Scale bars = 100 μm.
3.8 Rab7 inhibition modulates the mRNA expression of mesenchymal and epithelial markers in ASCs
The mRNA expression levels of vimentin, an intermediate filament protein predominantly produced by mesenchymal stem cells, as well as the epidermal markers, CK5, CK14, and Filaggrin, were analysed using Real-time PCR. The results indicated that Rab7 inhibition in ASCs led to a significant reduction in vimentin expression (p=0.031) and an increase in Filaggrin expression (p=0.028) as illustrated in Figures 8A, B. However, a decrease in the expression of CK5 (p=0.009) and CK14 (p=<0.001) was observed in response to Rab7 inhibition in ASCs cells (Figures 8C, D).
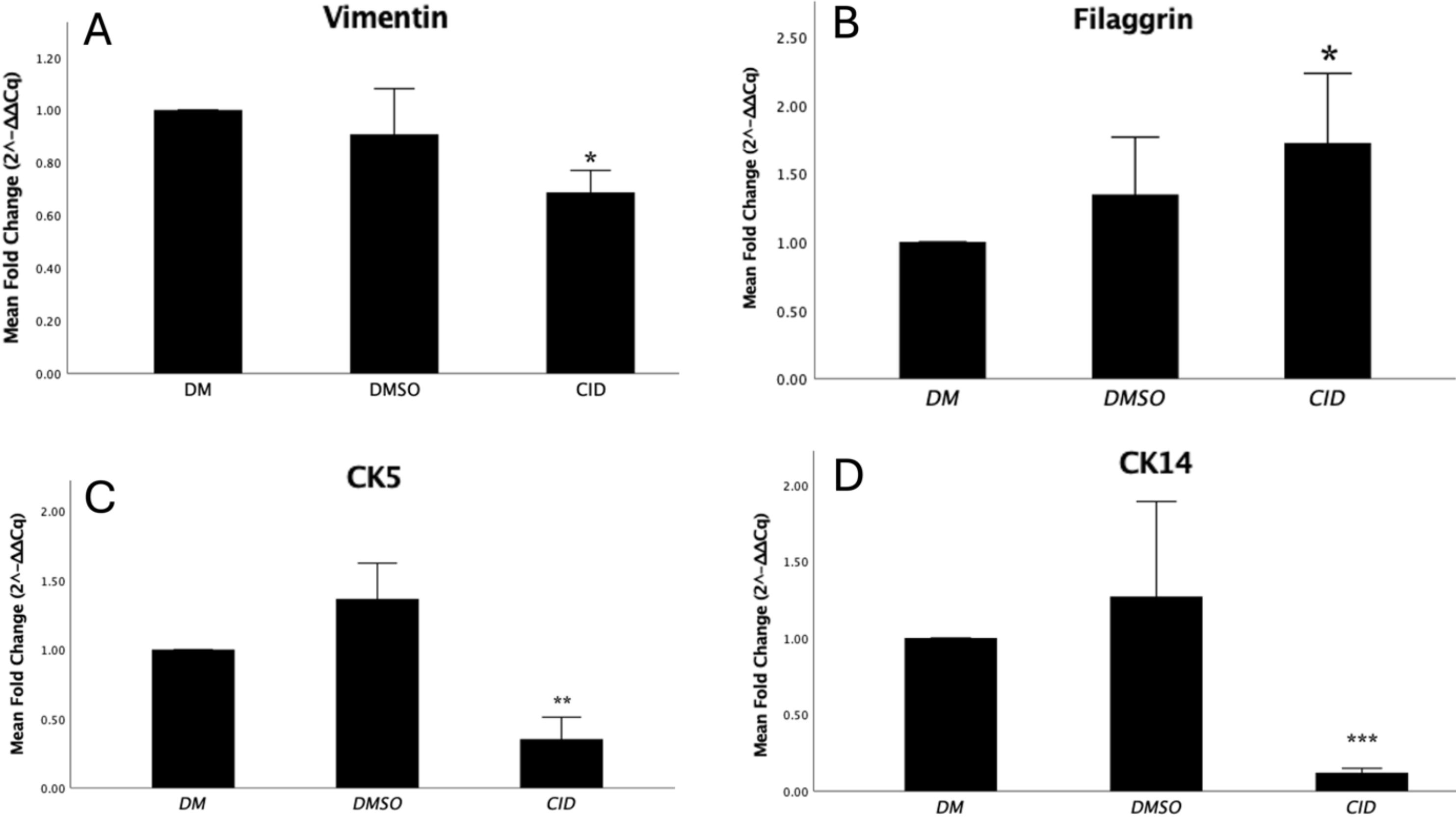
Figure 8. Effect of Rab7 inhibition on the expression of keratinocyte markers in ASCs cells. The gene expression of (A) Vimentin, (B) Filaggrin, (C) Cytokeratin 5, and (D) Cytokeratin 14 was measured by Real-time PCR. The expression levels were normalized to GAPDH expression and calculated relative to cells treated with differentiation media (DM) alone. Asterisks indicate a significant difference compared to cells treated with DM (* p<0.05, ** p<0.01, *** p<0.001).
4 Discussion
The skin has a vast natural regenerative capacity, which is vital to maintain the protectory function against the daily environmental and mechanical challenges. Upon injury, keratinocytes initiate an immunological cascade by secreting a repertoire of cytokines, chemokines, and antimicrobial peptides to stimulate the innate immune response (39, 40). This pro-inflammatory environment facilitates immune cell recruitment and pathogen elimination, which primes the wound bed for subsequent healing processes (41). Moreover, keratinocytes possess remarkable ability to resolve inflammation, fostering the transition from the inflammatory to the proliferative phase of wound healing, and promoting tissue repair (42). However, in the context of chronic wounds, this tightly regulated process is disrupted, leading to prolonged inflammation and tissue degradation. As keratinocytes become arrested in a chronic inflammation state, they exhibit compromised antimicrobial defence capabilities and fail to activate requisite signalling pathways essential for healing progression (6, 43).
Rab GTPases, the largest family of GTPases, are indispensable regulators of membrane trafficking due to their pivotal role in vesicle formation, transport, and fusion in endocytosis, exocytosis, and autophagy (24). Each Ras-related protein (Rab) governs specific steps of vesicular transport, exhibiting precise localization to distinct intracellular compartments. Moreover, several Rab proteins exert control over the degradation and activity of signalling receptors and ligands across developmental signalling pathways (44–46). Rab7 emerges as a key regulator, exerting profound influence over critical developmental signalling pathways. Rab7 regulates the endocytic trafficking of Wnt ligands, receptors, and co-receptors, thereby influencing their stability, availability, and signalling efficacy. Similar effect exerts on the Notch receptors and ligands. Notch is a conserved determinant of cell fate, differentiation, and tissue development (27, 28, 47). This protein plays a role in skin development and regeneration influenced by mitochondrial reactive oxygen species (48). Rab7 extends its regulatory role to the fibroblast growth factor (FGF) signalling pathway, modulating the trafficking and degradation of FGF receptors (FGFRs) and their ligands, thereby dictating the spatial and temporal dynamics of FGF signalling. Rab7 also regulates Hedgehog Signalling Pathway, a critical pathway for embryonic development, tissue patterning, and organogenesis. Furthermore, the impact of Rab7 encompasses melanosome maturation and transport, regulating the trafficking of melanosomes within melanocytes, the specialized pigment-producing cells in the skin (49, 50). These cumulative insights underscore the role of Rab7 in modulating the activation, availability, and efficacy of key developmental signalling pathways. Thus, Rab7 exerts profound influence over cell fate determination, tissue morphogenesis, and organ development. Despite the critical role of membrane trafficking in steering stem cell differentiation, existing differentiation protocols predominantly emphasize targeting signalling pathways and overlook the intracellular vesicle trafficking as a complementary approach for modulating stem cell fate.
In this study, the potential influence of Rab7 in enhancing the differentiation ability of ASCs into KLCs was explored. ASCs were cultured in differentiation media, which has previously been shown to promote the differentiation into KLCs (34). The cells were cultured in the presence or absence of CID-1067700, a competitive Rab7 inhibitor, and the differentiation process was assessed based on the cell morphology and their expression of keratinocyte markers, including Cytokeratin 5 (CK5), Cytokeratin 14 (CK14), P63, and Filaggrin.
Morphological observations revealed that Rab7 inhibition induced a noticeable flattening of ASCs after ten days of treatment. Such morphology suggests that the cells were undergoing the differentiation process, as keratinocytes adopt a flattened shape upon differentiation to provide structural rigidity and mechanical strength, which are key characteristic of the skin epithelium (51). CK5 and CK14 were utilised as early differentiation markers due to their expression in the basal layer of the epidermis. In contrast, filaggrin, is an intermediate marker of epidermal differentiation primarily expressed in well-differentiated keratinized epithelial cells, and it plays a crucial role in regulating epidermal homeostasis (51).
This study revealed that Rab7 inhibitor increased the protein expression of all these markers as early as ten days in culture. However, the mRNA expression of the basal keratinocyte markers CK5 and CK14 was decreased following Rab7 inhibition. This observation suggests that the Rab7 inhibitor effectively promotes the differentiation of adipose-derived stem cells into keratinocyte-like cells. This aligns with the role of Rab7 in regulating membrane trafficking and autophagy, processes crucial for cellular differentiation (37). However, the decrease in mRNA expression of basal keratinocyte markers CK5 and CK14 indicates a complex regulatory mechanism at play. One possible explanation is that Rab7 inhibition may lead to post-transcriptional modifications or stabilization of these proteins, resulting in higher protein levels despite lower mRNA expression. This phenomenon can occur due to enhanced protein stability or reduced degradation, which is often observed in differentiated cells where protein turnover rates are lower (52). Additionally, Rab7’s involvement in autophagy and endocytic pathways might influence the cellular environment, promoting differentiation signals that enhance protein expression while simultaneously downregulating mRNA synthesis as the cells transition through the differentiation state (37). This dual effect underscores the importance of Rab7 in coordinating the balance between stem cell maintenance and differentiation, highlighting its potential as a therapeutic target for enhancing stem cell-based regenerative therapies. Furthermore, we suggest that Rab7 inhibition may facilitate ASC progression through the differentiation process, as basal keratinocytes typically undergo both morphological and biochemical changes during differentiation. These changes include the production of flattened cells and changes in the pattern of keratin expression (53). For example, basal keratinocytes express CK5 and CK14 (54, 55). As they progress into the suprabasal compartment, the transcription of the basal keratins (CK5 and CK14) is downregulated and the transcription of a new set of differentiation state–specific markers, such as filaggrin, is concomitantly induced (54–56). Filaggrin, produced by spinous keratinocytes in the granular layer, facilitates the packing of keratin filaments during terminal differentiation of keratinocytes (57). The upregulation of filaggrin accompanied by the downregulation of CK5 and CK14 gene expression supports this explanation. For further validation, future studies should examine the temporal changes in mRNA expression of CK5 and CK14 in ASCs treated with the Rab7 inhibitor at various stages of differentiation. Time-course experiments would help elucidate whether Rab7 inhibition accelerates the downregulation of basal keratins and the upregulation of terminal differentiation markers.
Microarray analysis revealed substantial changes in gene expression profiles, with 134 differentially expressed genes (DEGs) identified in CID-treated ASCs. Pathway enrichment analysis highlighted the significant impact of Rab7 inhibition on various immune-related pathways. Notably, the chemokine signalling pathway, TNF signalling pathway, cytokine-cytokine receptor interactions, and IL-17 signalling pathway were among the pathways affected by CID treatment. These pathways play critical roles in inflammation and immune response, the two critical processes implicated in the pathogenesis of chronic skin wounds (6). These findings indicates that Rab7 inhibition does not only promote keratinocyte differentiation but may also modulate immune functions.
Prediction analysis using the iPathwayGuide software predicted the euchromatin histone lysine methyltransferase 2 (Ehmt2) as the top activated upstream regulator in CID-treated ASCs. Ehmt2 is known for its role in epigenetic regulation as well as modulating critical biological processes, such as cell cycle regulation, lineage commitment, and differentiation (58). As a repressor, Ehmt2, in conjunction with the Ehmt1/2 complex, serves as a central regulator of nuclear epigenetic processes essential for proper developmental progression from early embryonic stages to terminal differentiation (59–61). Epigenetic regulation is well known to be involved in stem cell differentiation into several lineages (62–65). Ehmt2 has been implicated in the terminal commitment of various lineages, including blood, cardiac, retinal, neural, muscle, and germline lineages (66–72). Moreover, Ehmt2 exert a transcriptional co-activator function, distinct from its classical role in methylation (73). Ehmt2 is also indispensable for the activation of critical genes involved in early development, such as p21 and β-Globin as well as Nfe2 and Runx2 (58, 74). The latter is known to promote the differentiation of ASCs to the epidermal lineage (75). These effects are expected to influence the wound healing process, although we believe that such corelation is understudied. The role of epigenetics in wound healing has been extensively reviewed. The migrating cells at the wound edge were shown to change their epigenetic signature to allow migration and wound closure. The involvement of histone methyltransferases, such as Ezh1 and Ezh2, was crucial for this purpose (76). Furthermore, histone methylation was vital for the determination of tissue macrophage properties as well as peripheral blood mononuclear cells, affecting the wound transition from the inflammatory phase (77). However, the role of Ehmt2 in wound repair needs to be experimentally verified to confirm the potential involvement in the healing process and skin regeneration. Knocking down Ehmt2 in ASC52 cell line can characterize its role in epidermal differentiation in the presence and absence of Rab7 inhibitor.
Furthermore, the protein array data revealed upregulation of EGF in the CID-treated ASCs, indicating a beneficial shift towards enhanced keratinocyte differentiation and wound healing. EGF is a critical regulator of cell proliferation and differentiation, particularly within the epidermis. By promoting keratinocyte migration and proliferation, EGF plays an important role in the re-epithelialization process during wound healing (78, 79). The observed increase in EGF levels in Rab7-inhibited ASCs suggests a potential mechanism for the enhancement of KLCs differentiation and indicates a potential role in accelerating wound healing.
Conversely, the inflammatory cytokines IL-1β and IL-6 were predicted as the top inhibited upstream regulators. This aligns with the observed downregulation of several inflammatory cytokines, including IL-1α, IL-8, IL-17A, and IL-32, in the protein array analysis of the supernatant from CID-treated cell culture. The reduction in these cytokines indicates a potent anti-inflammatory effect of Rab7 inhibition in ASCs, which could be beneficial in managing chronic wounds characterized by persistent inflammation. Recent study by Sonmez Kaplan et al. (80) showed that stimulation with these inflammatory cytokines significantly inhibited the differentiation of dental pulp stem cells into osteogenic, chondrogenic, and adipogenic lineages. Moreover, IL-1β-induced spheres displayed enhanced stemness and drug resistance in colon cancer cells, accompanied by epithelial-mesenchymal transition (80). These findings elucidate the interplay between inflammatory cytokines and stem cell behaviour, highlighting their negative regulatory roles in stem cell differentiation. This represents another advantage for Rab-7 inhibitor as an additive to stem cell differentiation programs and opens a new paradigm for research.
The decrease in pro-inflammatory cytokines observed in this study is of particular interest because of their established roles in wound healing. Elevated levels of IL-17A are commonly associated with chronic wounds. An obvious example is IL-17A rich wound fluid isolated from venous ulcers and lesional tissues of patients with pyoderma gangrenosum (81, 82). Furthermore, IL-17A exhibits synergistic effects with other pro-inflammatory cytokines such as TNF-α, IL-1, and IL-6, exacerbating the inflammatory response and contributing to wound chronicity (83, 84). The dysregulation of chemokine signalling can lead to excessive influx of immune cells into the skin, leading to inflammation and tissue damage (85). Similarly, dysregulated cytokine-cytokine receptor interactions can promote inflammatory responses, immune cell activation, and tissue damage (86). Furthermore, advanced glycation end products (AGEs) and their receptor (RAGE) are implicated in diabetic complications, including diabetic foot ulcers (DFU). Elevated AGE-RAGE levels induce the production of pro-inflammatory cytokines and reactive oxygen species, thereby contributing to the prolonged inflammatory phase and delayed wound healing (87).
Targeting Rab7 holds a great potential for treating chronic skin wounds for several reasons. Firstly, the upregulation of keratinocyte markers indicates that ASCs can differentiate into keratinocytes, which may improve the skin barrier (88). This could lead to the development of topical treatments or cell-based therapies that promote keratinocyte formation and improve skin integrity. Secondly, Rab7 inhibition may attenuate the inflammatory environment in wounds associated with autoimmune diseases through the downregulation of inflammation-related pathways. This could help balance the immune system dysregulation and promote wound healing, both by promoting the natural healing mechanism and adding KLCs as cell-based therapy (6). The clinical translation can also include the production of new anti-inflammatory treatments that specifically target Rab7, potentially reducing the need for systemic immunosuppressive therapies and their associated side effects. Finally, by understanding the specific genes and pathways inhibited by Rab7, a more personalized treatment approach can be developed, tailored to individual patients’ molecular characteristics, which potentially improves treatment responses and outcomes. The insights gained from studying Rab7 could inspire new therapeutic strategies, such as the use of gene editing technologies (e.g., CRISPR) to modulate Rab7 activity in chronic wounds.
However, it’s essential to acknowledge the limitations of our study, including the use of a cell line rather than primary cells. While the ASC52telo cell line offers practical advantages in experimental settings, including reproducibility, scalability, and the absence of donor variability, they may not fully replicate the heterogeneity and physiological context of primary cells. Additionally, these cells could be expected to exhibit potential alterations in gene expression and epigenetic profiles due to the introduction of hTERT during their immortalization process, which may influence their differentiation potential and responses to external stimuli. In fact, ASC52telo cells have been demonstrated to retain key characteristics of primary ASCs, making them a valuable model for controlled mechanistic studies, such as those investigating signalling pathways and differentiation dynamics (21, 31, 33). Prior studies have successfully used these cells to validate platforms for stem cell culture and expansion under defined, Xeno-free, and serum-free conditions, highlighting their suitability for stem cell modelling. These studies also emphasize the compatibility of ASC52telo cells with microcarrier-based systems and their ability to reproduce typical features of primary ASCs, making them a robust model for investigating transcriptional dynamics in controlled environments (e.g., bioreactors), as well as other tissue engineering applications (31). Additionally, ASC52telo cells have been featured in research exploring signalling pathways and cellular differentiation processes, further validating their utility in understanding molecular mechanisms and transcriptional regulation in stem cell biology (32). Nonetheless, to enhance the translational relevance of our findings and address potential biases introduced using an immortalized cell line, future studies should integrate primary ASCs to confirm findings and assess inter-individual variability. Incorporating organoid models or in vivo approaches would further contextualize these results within a physiologically relevant environment. Investigating the application method in animal models would be of special interest for clinical application. Systemic application versus local application as a solution or in combination with biomaterial, can provide an insight regarding the local effect on the wound as well as the possible side effects. Nevertheless, 2.5 mg/kg of CID were injected intraperitoneally in rats without reported side effects (89). Another limitation of this study is the reliance on immunofluorescence as the sole proteomic evidence of stem cell differentiation into the epithelial lineage. Characterizing differentiation using Western blotting, protein arrays, or other proteomic techniques would provide further confirmation of the differentiation status. Other Rab7 inhibitors, such as VP 3.15., can be evaluated in the future for further validation of the cause-effect. Moreover, functional assays focusing on Rab7 inhibition’s mechanistic effects on stem cell differentiation and inflammation would complement transcriptomic and proteomic analyses, providing a comprehensive understanding of the observed phenomena, including wound healing assay.
5 Conclusion
The current study highlights novel regulatory mechanisms governing stem cell differentiation and inflammation in the context of skin regeneration. The identification of Rab7 and Ehmt2 as key regulators of ASCs differentiation opens new avenues for therapeutic intervention, offering potential targets for developing innovative treatments aimed at enhancing tissue repair and regeneration. Further investigation into the potential crosstalk between Rab7, Ehmt2, and inflammatory cytokines holds promise for uncovering novel therapeutic targets and advancing the field of regenerative medicine.
Data availability statement
The datasets presented in this study can be found in online repositories. The names of the repository/repositories and accession number(s) can be found below: https://www.ncbi.nlm.nih.gov/geo/, GSE275489.
Author contributions
RA: Investigation, Methodology, Formal analysis, Writing – original draft, Writing – review & editing. MT: Formal analysis, Writing – review & editing, Investigation, Methodology, Validation, Writing – original draft. ME: Formal analysis, Conceptualization, Funding acquisition, Resources, Writing – review & editing. AE-S: Formal analysis, Writing – review & editing, Conceptualization, Project administration, Supervision.
Funding
The author(s) declare that financial support was received for the research and/or publication of this article. The authors would like to thank the Hand and Plastic Surgery Department, Linköping University Hospital, Region Östergötland, Sweden and The Centre for Advanced Medical Product, Sweden for supporting this study.
Acknowledgments
The authors would like to acknowledge the Core Facility at the Faculty of Medicine and Health Sciences, Linköping University for the valuable technical support. The authors are also grateful for the kind help of Dr. Hady Shahin.
Conflict of interest
The authors declare that the research was conducted in the absence of any commercial or financial relationships that could be construed as a potential conflict of interest.
Generative AI statement
The author(s) declare that Generative AI was used in the creation of this manuscript. For linguistic revision.
Publisher’s note
All claims expressed in this article are solely those of the authors and do not necessarily represent those of their affiliated organizations, or those of the publisher, the editors and the reviewers. Any product that may be evaluated in this article, or claim that may be made by its manufacturer, is not guaranteed or endorsed by the publisher.
Supplementary material
The Supplementary Material for this article can be found online at: https://www.frontiersin.org/articles/10.3389/fimmu.2025.1503007/full#supplementary-material.
Supplementary Figure 1 | Rab7 inhibition induces transcriptomic changes in ASCs. Heatmap analysis displaying the differentially expressed genes (DEGs) in ASCs following Rab7 inhibition. Samples with relatively high expression of a given gene are shown in red, while those with relatively low expression are shown in blue. Clustering of the samples is illustrated by the dendrogram on top.
Supplementary Figure 2 | Immunofluorescence analysis was repeated using conjugated primary antibodies from a different vendor to further confirm the expression of cytokeratin 5 and 14, which are (A) CoraLite®555 Cytokeratin 5 antibody (1:200, Proteintech, Cat. CL555-28506) and (B) CoraLite® Plus 488 Cytokeratin 14 antibody (1:200, Proteintech, Cat. CL488-10143). Data are presented as mean fluorescence intensity unit (FU) per cell ± SD of two replicates, five fields were analysed in each replicate and p < 0.05 was considered significant. Scale bars = 200 μm. The results are consistent with previous findings, confirming the upregulation of CK5 and CK14 in response to CID.
Supplementary Table 1 | List of the differentially regulated genes.
References
1. Lindholm C and Searle R. Wound management for the 21st century: combining effectiveness and efficiency. Int Wound J. (2016) 13 Suppl 2:5–15. doi: 10.1111/iwj.12623
2. Mahmoud NN, Hamad K, Al Shibitini A, Juma S, Sharifi S, Gould L, et al. Investigating inflammatory markers in wound healing: understanding implications and identifying artifacts. ACS Pharmacol Transl Sci. (2024) 7:18–27. doi: 10.1021/acsptsci.3c00336
3. Dunnill C, Patton T, Brennan J, Barrett J, Dryden M, Cooke J, et al. Reactive oxygen species (ROS) and wound healing: the functional role of ROS and emerging ROS-modulating technologies for augmentation of the healing process. Int Wound J. (2017) 14:89–96. doi: 10.1111/iwj.12557
4. McCarty SM and Percival SL. Proteases and delayed wound healing. Adv Wound Care (New Rochelle). (2013) 2:438–47. doi: 10.1089/wound.2012.0370
5. Eming SA, Martin P, and Tomic-Canic M. Wound repair and regeneration: mechanisms, signaling, and translation. Sci Transl Med. (2014) 6:265sr266. doi: 10.1126/scitranslmed.3009337
6. Zhao R, Liang H, Clarke E, Jackson C, and Xue M. Inflammation in chronic wounds. Int J Mol Sci. (2016) 17. doi: 10.3390/ijms17122085
7. Shanmugam VK, Angra D, Rahimi H, and McNish S. Vasculitic and autoimmune wounds. J Vasc Surg Venous Lymphat Disord. (2017) 5:280–92. doi: 10.1016/j.jvsv.2016.09.006
8. Shanmugam VK, DeMaria DM, and Attinger CE. Lower extremity ulcers in rheumatoid arthritis: features and response to immunosuppression. Clin Rheumatol. (2011) 30:849–53. doi: 10.1007/s10067-011-1710-9
9. Shanmugam VK, Price P, Attinger CE, and Steen VD. Lower extremity ulcers in systemic sclerosis: features and response to therapy. Int J Rheumatol. (2010) 2010. doi: 10.1155/2010/747946
10. Wang AS, Armstrong EJ, and Armstrong AW. Corticosteroids and wound healing: clinical considerations in the perioperative period. Am J Surg. (2013) 206:410–7. doi: 10.1016/j.amjsurg.2012.11.018
11. Ongarora BG. Recent technological advances in the management of chronic wounds: A literature review. Health Sci Rep. (2022) 5:e641. doi: 10.1002/hsr2.641
12. Harding K and Queen D. Estimating the cost of wounds for the United Kingdom and its countries. Int Wound J. (2024) 21:e14616. doi: 10.1111/iwj.14616
13. Queen D and Harding K. What’s the true costs of wounds faced by different healthcare systems around the world? Int Wound J. (2023) 20:3935–8. doi: 10.1111/iwj.14491
14. Queen D and Harding K. Estimating the cost of wounds both nationally and regionally within the top 10 highest spenders. Int Wound J. (2024) 21:e14709. doi: 10.1111/iwj.14709
15. Przekora A. A concise review on tissue engineered artificial skin grafts for chronic wound treatment: can we reconstruct functional skin tissue in vitro? Cells. (2020) 9. doi: 10.3390/cells9071622
16. Napavichayanun S and Aramwit P. Effect of animal products and extracts on wound healing promotion in topical applications: a review. J Biomater Sci Polym Ed. (2017) 28:703–29. doi: 10.1080/09205063.2017.1301772
17. Bian D, Wu Y, Song G, Azizi R, and Zamani A. The application of mesenchymal stromal cells (MSCs) and their derivative exosome in skin wound healing: a comprehensive review. Stem Cell Res Ther. (2022) 13:24. doi: 10.1186/s13287-021-02697-9
18. Yang J, Xiao M, Ma K, Li H, Ran M, Yang S, et al. Therapeutic effects of mesenchymal stem cells and their derivatives in common skin inflammatory diseases: Atopic dermatitis and psoriasis. Front Immunol. (2023) 14:1092668. doi: 10.3389/fimmu.2023.1092668
19. El-Serafi AT, El-Serafi IT, Elmasry M, Steinvall I, and Sjoberg F. Skin regeneration in three dimensions, current status, challenges and opportunities. Differentiation. (2017) 96:26–9. doi: 10.1016/j.diff.2017.06.002
20. Gimble JM, Ray SP, Zanata F, Wu X, Wade J, Khoobehi K, et al. Adipose derived cells and tissues for regenerative medicine. ACS Biomater Sci Eng. (2017) 3:1477–82. doi: 10.1021/acsbiomaterials.6b00261
21. Shahin H, Abdallah S, Das J, He W, El-Serafi I, Steinvall I, et al. miRNome and proteome profiling of human keratinocytes and adipose derived stem cells proposed miRNA-mediated regulations of epidermal growth factor and interleukin 1-alpha. Int J Mol Sci. (2023) 24. doi: 10.3390/ijms24054956
22. Li JH, Trivedi V, and Diz-Muñoz A. Understanding the interplay of membrane trafficking, cell surface mechanics, and stem cell differentiation. Semin Cell Dev Biol. (2023) 133:123–34. doi: 10.1016/j.semcdb.2022.05.010
23. Guerra F and Bucci C. Multiple roles of the small GTPase rab7. Cells. (2016) 5. doi: 10.3390/cells5030034
24. Wandinger-Ness A and Zerial M. Rab proteins and the compartmentalization of the endosomal system. Cold Spring Harb Perspect Biol. (2014) 6:a022616. doi: 10.1101/cshperspect.a022616
25. Kreis J, Camuto CM, Elsner CC, Vogel S, and Vick P. FGF-mediated establishment of left-right asymmetry requires Rab7 function in the dorsal mesoderm in Xenopus. Front Cell Dev Biol. (2022) 10:981762. doi: 10.3389/fcell.2022.981762
26. Ceresa BP and Bahr SJ. rab7 activity affects epidermal growth factor:epidermal growth factor receptor degradation by regulating endocytic trafficking from the late endosome. J Biol Chem. (2006) 281:1099–106. doi: 10.1074/jbc.M504175200
27. Kawamura N, Takaoka K, Hamada H, Hadjantonakis AK, Sun-Wada GH, and Wada Y. Rab7-mediated endocytosis establishes patterning of wnt activity through inactivation of dkk antagonism. Cell Rep. (2020) 31:107733. doi: 10.1016/j.celrep.2020.107733
28. Yamamoto S, Charng WL, and Bellen HJ. Endocytosis and intracellular trafficking of Notch and its ligands. Curr Top Dev Biol. (2010) 92:165–200. doi: 10.1016/S0070-2153(10)92005-X
29. Briscoe J and Therond PP. The mechanisms of Hedgehog signalling and its roles in development and disease. Nat Rev Mol Cell Biol. (2013) 14:416–29. doi: 10.1038/nrm3598
30. Prashar A, Schnettger L, Bernard EM, and Gutierrez MG. Rab GTPases in immunity and inflammation. Front Cell Infect Microbiol. (2017) 7:435. doi: 10.3389/fcimb.2017.00435
31. Muoio F, Panella S, Lindner M, Jossen V, Harder Y, Moccetti T, et al. Development of a biodegradable microcarrier for the cultivation of human adipose stem cells (hASCs) with a defined xeno- and serum-free medium. Appl Sci. (2021) 11:925. doi: 10.3390/app11030925
32. Primak A, Kalinina N, Skryabina M, Usachev V, Chechekhin V, Vigovskiy M, et al. Novel immortalized human multipotent mesenchymal stromal cell line for studying hormonal signaling. Int J Mol Sci. (2024) 25(4), 2421. doi: 10.3390/ijms25042421
33. Shahin H, Belcastro L, Das J, Perdiki Grigoriadi M, Saager RB, Steinvall I, et al. MicroRNA-155 mediates multiple gene regulations pertinent to the role of human adipose-derived mesenchymal stem cells in skin regeneration. Front Bioeng Biotechnol. (2024) 12:1328504. doi: 10.3389/fbioe.2024.1328504
34. Petry L, Kippenberger S, Meissner M, Kleemann J, Kaufmann R, Rieger UM, et al. Directing adipose-derived stem cells into keratinocyte-like cells: impact of medium composition and culture condition. J Eur Acad Dermatol Venereol. (2018) 32:2010–9. doi: 10.1111/jdv.15010
35. Schindelin J, Arganda-Carreras I, Frise E, Kaynig V, Longair M, Pietzsch T, et al. Fiji: an open-source platform for biological-image analysis. Nat Methods. (2012) 9:676–82. doi: 10.1038/nmeth.2019
36. Ke J, Pan J, Lin H, Huang S, Zhang J, Wang C, et al. Targeting rab7-rilp mediated microlipophagy alleviates lipid toxicity in diabetic cardiomyopathy. Adv Sci (Weinh). (2024) 11:e2401676. doi: 10.1002/advs.202401676
37. Lam T, Kulp DV, Wang R, Lou Z, Taylor J, Rivera CE, et al. Small molecule inhibition of rab7 impairs B cell class switching and plasma cell survival to dampen the autoantibody response in murine lupus. J Immunol. (2016) 197:3792–805. doi: 10.4049/jimmunol.1601427
38. Yan H, Fernandez M, Wang J, Wu S, Wang R, Lou Z, et al. B cell endosomal RAB7 promotes TRAF6 K63 polyubiquitination and NF-kappaB activation for antibody class-switching. J Immunol. (2020) 204:1146–57. doi: 10.4049/jimmunol.1901170
39. El-Serafi AT, El-Serafi I, Steinvall I, Sjoberg F, and Elmasry M. A systematic review of keratinocyte secretions: A regenerative perspective. Int J Mol Sci. (2022) 23(14), 7934. doi: 10.3390/ijms23147934
40. Tüzün Y, Antonov M, Dolar N, and Wolf R. Keratinocyte cytokine and chemokine receptors. Dermatol Clin. (2007) 25:467–476, vii. doi: 10.1016/j.det.2007.06.003
41. Chen YE, Fischbach MA, and Belkaid Y. Skin microbiota-host interactions. Nature. (2018) 553:427–36. doi: 10.1038/nature25177
42. Piipponen M, Li D, and Landén NX. The immune functions of keratinocytes in skin wound healing. Int J Mol Sci. (2020) 21(22), 8790. doi: 10.3390/ijms21228790
43. Landén NX, Li D, and Ståhle M. Transition from inflammation to proliferation: a critical step during wound healing. Cell Mol Life Sci. (2016) 73:3861–85. doi: 10.1007/s00018-016-2268-0
44. Nassari S, Del Olmo T, and Jean S. Rabs in signaling and embryonic development. Int J Mol Sci. (2020) 21(3), 1064. doi: 10.3390/ijms21031064
45. Stypulkowski E, Feng Q, Joseph I, Farrell V, Flores J, Yu S, et al. Rab8 attenuates Wnt signaling and is required for mesenchymal differentiation into adipocytes. J Biol Chem. (2021) 296:100488. doi: 10.1016/j.jbc.2021.100488
46. Xu S, Liu Y, Meng Q, and Wang B. Rab34 small GTPase is required for Hedgehog signaling and an early step of ciliary vesicle formation in mouse. J Cell Sci. (2018) 131(21), jcs213710. doi: 10.1242/jcs.213710
47. Alghazali R, Nugud A, and El-Serafi A. Glycan modifications as regulators of stem cell fate. Biol (Basel). (2024) 13(2), 76. doi: 10.3390/biology13020076
48. Nugud A, Sandeep D, and El-Serafi AT. Two faces of the coin: Minireview for dissecting the role of reactive oxygen species in stem cell potency and lineage commitment. J Adv Res. (2018) 14:73–9. doi: 10.1016/j.jare.2018.05.012
49. Gomez PF, Luo D, Hirosaki K, Shinoda K, Yamashita T, Suzuki J, et al. Identification of rab7 as a melanosome-associated protein involved in the intracellular transport of tyrosinase-related protein 1. J Invest Dermatol. (2001) 117:81–90. doi: 10.1046/j.0022-202x.2001.01402.x
50. Jordens I, Westbroek W, Marsman M, Rocha N, Mommaas M, Huizing M, et al. Rab7 and Rab27a control two motor protein activities involved in melanosomal transport. Pigment Cell Res. (2006) 19:412–23. doi: 10.1111/j.1600-0749.2006.00329.x
51. Lee J, Lee P, and Wu X. Molecular and cytoskeletal regulations in epidermal development. Semin Cell Dev Biol. (2017) 69:18–25. doi: 10.1016/j.semcdb.2017.05.018
52. Modica G and Lefrancois S. Post-translational modifications: How to modulate Rab7 functions. Small GTPases. (2020) 11(3):167–73. doi: 10.1080/21541248.2017.1387686
53. Fuchs E. Epidermal differentiation and keratin gene expression. J Cell Sci Suppl. (1993) 17:197–208. doi: 10.1242/jcs.1993.supplement_17.28
54. Fuchs E and Green H. Changes in keratin gene expression during terminal differentiation of the keratinocyte. Cell. (1980) 19:1033–42. doi: 10.1016/0092-8674(80)90094-x
55. Moll R, Franke WW, Schiller DL, Geiger B, and Krepler R. The catalog of human cytokeratins: patterns of expression in normal epithelia, tumors and cultured cells. Cell. (1982) 31:11–24. doi: 10.1016/0092-8674(82)90400-7
56. Bose A, Teh MT, Hutchison IL, Wan H, Leigh IM, and Waseem A. Two mechanisms regulate keratin K15 expression in keratinocytes: role of PKC/AP-1 and FOXM1 mediated signalling. PloS One. (2012) 7:e38599. doi: 10.1371/journal.pone.0038599
57. Kuechle MK, Presland RB, Lewis SP, Fleckman P, and Dale BA. Inducible expression of filaggrin increases keratinocyte susceptibility to apoptotic cell death. Cell Death Differ. (2000) 7:566–73. doi: 10.1038/sj.cdd.4400687
58. Deimling SJ, Olsen JB, and Tropepe V. The expanding role of the Ehmt2/G9a complex in neurodevelopment. Neurogenesis (Austin). (2017) 4:e1316888. doi: 10.1080/23262133.2017.1316888
59. Meng TG, Lei WL, Lu X, Liu XY, Ma XS, Nie XQ, et al. Maternal EHMT2 is essential for homologous chromosome segregation by regulating. Int J Biol Sci. (2022) 18:4513–31. doi: 10.7150/ijbs.75298
60. Pal A, Leung JY, Ang GCK, Rao VK, Pignata L, Lim HJ, et al. EHMT2 epigenetically suppresses Wnt signaling and is a potential target in embryonal rhabdomyosarcoma. Elife. (2020) 9, e57683. doi: 10.7554/eLife.57683
61. Zylicz JJ, Borensztein M, Wong FC, Huang Y, Lee C, Dietmann S, et al. G9a regulates temporal preimplantation developmental program and lineage segregation in blastocyst. Elife. (2018) 7, e33361. doi: 10.7554/eLife.33361
62. El-Serafi AT. Epigenetic modifiers and stem cell differentiation. In: Hayat M, editor. Stem cells and cancer stem cells, vol. 8. Dordrecht: Springer (2012). p. 147–54.
63. El-Serafi AT, Oreffo ROC, and Roach HI. Epigenetic modifiers influence lineage commitment of human bone marrow stromal cells: Differential effects of 5-aza-deoxycytidine and trichostatin A. Differentiation. (2011) 81:35–41. doi: 10.1016/j.diff.2010.09.183
64. El-Serafi AT, Sandeep D, Abdallah S, Lozansson Y, Hamad M, and Khan AA. Paradoxical effects of the epigenetic modifiers 5-aza-deoxycytidine and suberoylanilide hydroxamic acid on adipogenesis. Differentiation. (2019) 106:1–8. doi: 10.1016/j.diff.2019.02.003
65. Elsharkawi I, Parambath D, Saber-Ayad M, Khan AA, and El-Serafi AT. Exploring the effect of epigenetic modifiers on developing insulin-secreting cells. Hum Cell. (2020) 33:1–9. doi: 10.1007/s13577-019-00292-y
66. Chen X, Skutt-Kakaria K, Davison J, Ou YL, Choi E, Malik P, et al. G9a/GLP-dependent histone H3K9me2 patterning during human hematopoietic stem cell lineage commitment. Genes Dev. (2012) 26:2499–511. doi: 10.1101/gad.200329.112
67. Inagawa M, Nakajima K, Makino T, Ogawa S, Kojima M, Ito S, et al. Histone H3 lysine 9 methyltransferases, G9a and GLP are essential for cardiac morphogenesis. Mech Dev. (2013) 130:519–31. doi: 10.1016/j.mod.2013.07.002
68. Katoh K, Yamazaki R, Onishi A, Sanuki R, and Furukawa T. G9a histone methyltransferase activity in retinal progenitors is essential for proper differentiation and survival of mouse retinal cells. J Neurosci. (2012) 32:17658–70. doi: 10.1523/JNEUROSCI.1869-12.2012
69. Olsen JB, Wong L, Deimling S, Miles A, Guo H, Li Y, et al. G9a and ZNF644 physically associate to suppress progenitor gene expression during neurogenesis. Stem Cell Rep. (2016) 7:454–70. doi: 10.1016/j.stemcr.2016.06.012
70. Rao VK, Ow JR, Shankar SR, Bharathy N, Manikandan J, Wang Y, et al. G9a promotes proliferation and inhibits cell cycle exit during myogenic differentiation. Nucleic Acids Res. (2016) 44:8129–43. doi: 10.1093/nar/gkw483
71. Ugarte F, Sousae R, Cinquin B, Martin EW, Krietsch J, Sanchez G, et al. Progressive chromatin condensation and H3K9 methylation regulate the differentiation of embryonic and hematopoietic stem cells. Stem Cell Rep. (2015) 5:728–40. doi: 10.1016/j.stemcr.2015.09.009
72. Zylicz JJ, Dietmann S, Günesdogan U, Hackett JA, Cougot D, Lee C, et al. Chromatin dynamics and the role of G9a in gene regulation and enhancer silencing during early mouse development. Elife. (2015) 4, e09571. doi: 10.7554/eLife.09571
73. Lee DY, Northrop JP, Kuo MH, and Stallcup MR. Histone H3 lysine 9 methyltransferase G9a is a transcriptional coactivator for nuclear receptors. J Biol Chem. (2006) 281:8476–85. doi: 10.1074/jbc.M511093200
74. Chaturvedi CP, Hosey AM, Palii C, Perez-Iratxeta C, Nakatani Y, Ranish JA, et al. Dual role for the methyltransferase G9a in the maintenance of beta-globin gene transcription in adult erythroid cells. Proc Natl Acad Sci U S A. (2009) 106:18303–8. doi: 10.1073/pnas.0906769106
75. Li Q, Zhao H, Xia S, Wei H, Chen F, and Jin P. RUNX2 promotes epithelial differentiation of ADSCs and burn wound healing via targeting E-cadherin. Oncotarget. (2018) 9:2646–59. doi: 10.18632/oncotarget.23522
76. Zhang S and Duan E. Epigenetic regulations on skin wound healing: implications from current researches. Ann Transl Med. (2015) 3:227. doi: 10.3978/j.issn.2305-5839.2015.07.12
77. Yu H, Wang Y, Wang D, Yi Y, Liu Z, Wu M, et al. Landscape of the epigenetic regulation in wound healing. Front Physiol. (2022) 13:949498. doi: 10.3389/fphys.2022.949498
78. McCawley LJ, O’Brien P, and Hudson LG. Epidermal growth factor (EGF)- and scatter factor/hepatocyte growth factor (SF/HGF)- mediated keratinocyte migration is coincident with induction of matrix metalloproteinase (MMP)-9. J Cell Physiol. (1998) 176:255–65. doi: 10.1002/(SICI)1097-4652(199808)176:2<255::AID-JCP4>3.0.CO;2-N
79. Shin SH, Koh YG, Lee WG, Seok J, and Park KY. The use of epidermal growth factor in dermatological practice. Int Wound J. (2023) 20:2414–23. doi: 10.1111/iwj.14075
80. Sonmez Kaplan S, Sazak Ovecoglu H, Genc D, and Akkoc T. TNF-α, IL-1B and IL-6 affect the differentiation ability of dental pulp stem cells. BMC Health. (2023) 23:555. doi: 10.1186/s12903-023-03288-1
81. Ligi D, Mosti G, Croce L, Raffetto JD, and Mannello F. Chronic venous disease - Part I: Inflammatory biomarkers in wound healing. Biochim Biophys Acta. (2016) 1862:1964–74. doi: 10.1016/j.bbadis.2016.07.018
82. Marzano AV, Damiani G, Ceccherini I, Berti E, Gattorno M, and Cugno M. Autoinflammation in pyoderma gangrenosum and its syndromic form (pyoderma gangrenosum, acne and suppurative hidradenitis). Br J Dermatol. (2017) 176:1588–98. doi: 10.1111/bjd.15226
83. Goldberg M, Nadiv O, Luknar-Gabor N, Agar G, Beer Y, and Katz Y. Synergism between tumor necrosis factor alpha and interleukin-17 to induce IL-23 p19 expression in fibroblast-like synoviocytes. Mol Immunol. (2009) 46:1854–9. doi: 10.1016/j.molimm.2009.01.004
84. Hadian Y, Bagood MD, Dahle SE, Sood A, and Isseroff RR. Interleukin-17: potential target for chronic wounds. Mediators Inflammation. (2019) 2019:1297675. doi: 10.1155/2019/1297675
85. Apte A, Liechty KW, and Zgheib C. Immunomodulatory biomaterials on chemokine signaling in wound healing. Front Pharmacol. (2023) 14:1084948. doi: 10.3389/fphar.2023.1084948
86. Upadhyay PR, Seminario-Vidal L, Abe B, Ghobadi C, and Sims JT. Cytokines and epidermal lipid abnormalities in atopic dermatitis: A systematic review. Cells. (2023) 12(24), 2793. doi: 10.3390/cells12242793
87. Jin Y, Huang Y, Zeng G, Hu J, Li M, Tian M, et al. Advanced glycation end products regulate macrophage apoptosis and influence the healing of diabetic foot wound through miR-361-3p/CSF1R and PI3K/AKT pathway. Heliyon. (2024) 10:e24598. doi: 10.1016/j.heliyon.2024.e24598
88. Kim BE and Leung DYM. Significance of skin barrier dysfunction in atopic dermatitis. Allergy Asthma Immunol Res. (2018) 10:207–15. doi: 10.4168/aair.2018.10.3.207
Keywords: regenerative medicine, wound healing, stem Cells, CID-1067700, Rab7, differentiation, Ras pathway, skin
Citation: Alghazali R, Tabebi M, Elmasry M and El-Serafi A (2025) Rab7 inhibitor enhances stem cell differentiation into keratinocyte-like cells with anti-inflammatory properties. Front. Immunol. 16:1503007. doi: 10.3389/fimmu.2025.1503007
Received: 27 September 2024; Accepted: 29 April 2025;
Published: 26 May 2025.
Edited by:
Omar El Meligy, King Abdulaziz University, Saudi ArabiaReviewed by:
Wenjun Zhang, Indiana University Bloomington, United StatesFarhan Sachal Cyprian, Qatar University, Qatar
Copyright © 2025 Alghazali, Tabebi, Elmasry and El-Serafi. This is an open-access article distributed under the terms of the Creative Commons Attribution License (CC BY). The use, distribution or reproduction in other forums is permitted, provided the original author(s) and the copyright owner(s) are credited and that the original publication in this journal is cited, in accordance with accepted academic practice. No use, distribution or reproduction is permitted which does not comply with these terms.
*Correspondence: Ahmed El-Serafi, YWhtZWQuZWxzZXJhZnlAbGl1LnNl