- 1Department of Neurology, Houston Methodist Research Institute, Houston, TX, United States
- 2College of Medicine, Texas A&M University, Bryan, TX, United States
- 3Department of Neurology, Weill Cornell Medical College, New York, NY, United States
S100A4 is a Ca2+-binding protein involved in multiple chronic inflammatory and neoplastic conditions. This review focuses on recent advances in the understanding of S100A4 function in immune cells, comparing and contrasting S100A4 regulation of immune responses in cancer and chronic inflammatory diseases. We provide evidence that S100A4 regulation of immune cell function has a profound role in promoting the pathogenesis of cancer and pro-inflammatory conditions. Finally, we discuss relevant future directions to target S100A4 therapeutically in different disease states.
1 Introduction
The S100 family of proteins contains at least 24 small, Ca2+-binding proteins that perform a wide range of functions throughout the cell (1, 2). Both the structure and function of S100 proteins are regulated by Ca2+ binding via elongation factor (EF)-hand domains (1). S100 proteins act as Ca2+ sensors that respond quickly to changes in Ca2+ levels, leading to conformational changes and protein binding that modulate the activity of target proteins. In humans, S100 family genes primarily cluster on chromosome 1q21.3, with some members distributed across chromosomes 4, 5, 21, and X (3). Expression of S100 proteins is highly regulated by oxidative stress, hypoxia, cytokines, growth factors, and toll-like receptor (TLR) ligands, which highlights their importance in maintaining immune homeostasis (2). Amongst them, S100A4 stands out as a member that is strongly implicated in a wide range of pathologies ranging from cancer to chronic inflammatory conditions.
S100A4 is among the most extensively studied member of this family due to its strong association with inflammation, cancer, and neurodegeneration (4–6). S100A4 is also known as fibroblast specific protein-1 (FSP-1), among other names (7), as a gene highly expressed in breast cancer associated fibroblasts. Obviously, this is a misnomer as S100A4 is expressed in many other cell types. S100A4 expression has been associated with chronic inflammatory conditions such as fibrosis (8), rheumatoid arthritis (RA) (9, 10), allergic asthma (11–13) and dermatitis (14). In the context of cancer, S100A4 has been shown to promote metastasis, the epithelial-mesenchymal transition, and immune suppression (5, 15, 16). While many studies have focused on S100A4 function within cancer cells to promote cancer progression (4, 5) and cancer stem cell function (16, 17), a growing body of evidence indicates that S100A4 signaling serves multifaceted roles in the innate and adaptive immune systems. In other words, S100A4 is important in not only the normal immune response but also the pathogenesis and progression of dysregulated inflammatory conditions.
S100A4 is a highly conserved, 101-amino-acid polypeptide, with a C-terminal canonical EF-hand motif and an N-terminal pseudo-EF-hand motif. Ca2+ binding to S100A4 promotes its dimerization, which is dependent on the F72, Y75, F78, and L79 residues in helix IV of the canonical EF motif (18). Additionally, S100A4 can form oligomers, including heterodimers with other S100 proteins (19). The dimeric and oligomeric forms of S100A4 have non-overlapping roles. For example, oligomeric, but not dimeric, S100A4 stimulates neuronal differentiation and neurite outgrowth (20) as well as monocyte activity (21). Conversely, dimeric, but not oligomeric, S100A4 interacts with binding partner myosin-IIA (22).
S100A4 protein has been observed in the nucleus, cytoplasm, and extracellular space, and it functions both intracellularly and extracellularly (6). In the cytoplasm, S100A4 regulates cell motility and invasion by binding to non-muscle myosin IIA, rhotekin, tropomyosin, and F-actin (2, 23–26). In the nucleus, S100A4 binds to the C-terminal transactivation domain of p53 to modulate its pro-apoptotic function and promotes p53 degradation (27). In addition, S100A4 regulates expression of transcription factors that control the proneural-mesenchymal transition in glioma stem cells (16). Extracellularly, S100A4 has cytokine-like function and can promote inflammation, angiogenesis, and extracellular matrix remodeling (6). Externalization of S100A4 is typically associated with stress and pathological conditions, but this mechanism remains elusive because S100A4 lacks a signal peptide for secretion (6). Recent studies show that S100A4 may be released via exosomes (28–30). Another possibility is that S100A4 is partially released from dying cells to promote its pathological effects. For example, a recent study demonstrated that DNA-bound S100A4 was released from dying cancer cells to promote metastatic function in neighboring cancer cells via the RAGE pathway (31).
S100A4 is also considered a damage-associated molecular pattern (DAMP) family member. When outside the cell, S100A4 binds to its receptors RAGE (32), TLR4 (9, 21, 29), Embigin (33), Annexin A2, and IL-10R (34) to activate predominantly pro-inflammatory pathways, such as JAK/STAT, MAPK, and NF-κB. Hence, both intracellular and extracellular S100A4 signaling play major roles in regulation of the immune system. This review focuses on the biology of S100A4 in various immune cell subtypes and examines S100A4 activities in the contexts of immune responses in cancer, autoimmunity, and fibrosis.
2 S100A4 function in myeloid cells
2.1 Monocytes/macrophages
S100A4 expression affects macrophage function in several different ways. First, S100A4 has a direct role in regulating cell motility via interaction with myosin-IIA (23, 26), and macrophages isolated from S100a4 -/- mice have impaired ability to migrate to inflammation sites in a thioglycolate-induced model of peritonitis (23). This impairment is attributed to deficient CSF1R signaling and over-assembly of non-muscle myosin IIA in S100a4 -/- macrophages (23). Second, S100A4 can modulate cell invasion, and S100a4 -/- macrophages were shown to regulate cell invasion independently of myosin IIA via control of podosome rosettes and microtubule acetylation (24). Finally, S100A4 activates RAGE-dependent NF-κβ signaling in RAW 264.7 macrophages (35).
2.1.1 S100A4 regulates macrophage polarization and immunosuppression in cancer
In the context of cancer, S100A4 plays an important role in polarizing macrophages towards a pro-tumor, M2-like, alternatively activated state (36). Immunosuppressive, tumor-associated macrophages (TAMs) across human cancer types express high levels of S100A4 (15, 37–39). In TAMs from human liver cancer patients, S100A4 was identified as a key gene associated with macrophage polarization and infiltration (38, 39). In mouse models of glioma, we showed that S100a4 -/- TAMs have enhanced phagocytic activity compared with wild-type TAMs (15). Mechanistically, S100A4 expression in macrophages can induce expression of M2-like immunosuppressive surface and functional markers CD206, Arginase-1 (Arg-1), PD-L1, and TGF-β in a PPAR-γ-dependent manner (36) (Figure 1a). Additionally, S100A4 promotes fatty acid uptake in macrophages via induction of CD36, a fatty acid transporter (36) (Figure 1a). Multiple groups have shown that fatty acid metabolism regulates TAM differentiation and activation (40, 41). S100A4 may likely regulate macrophage polarization towards the M2-like state in concert with CD36-induced fatty acid metabolism (40, 41) (Figure 1a).
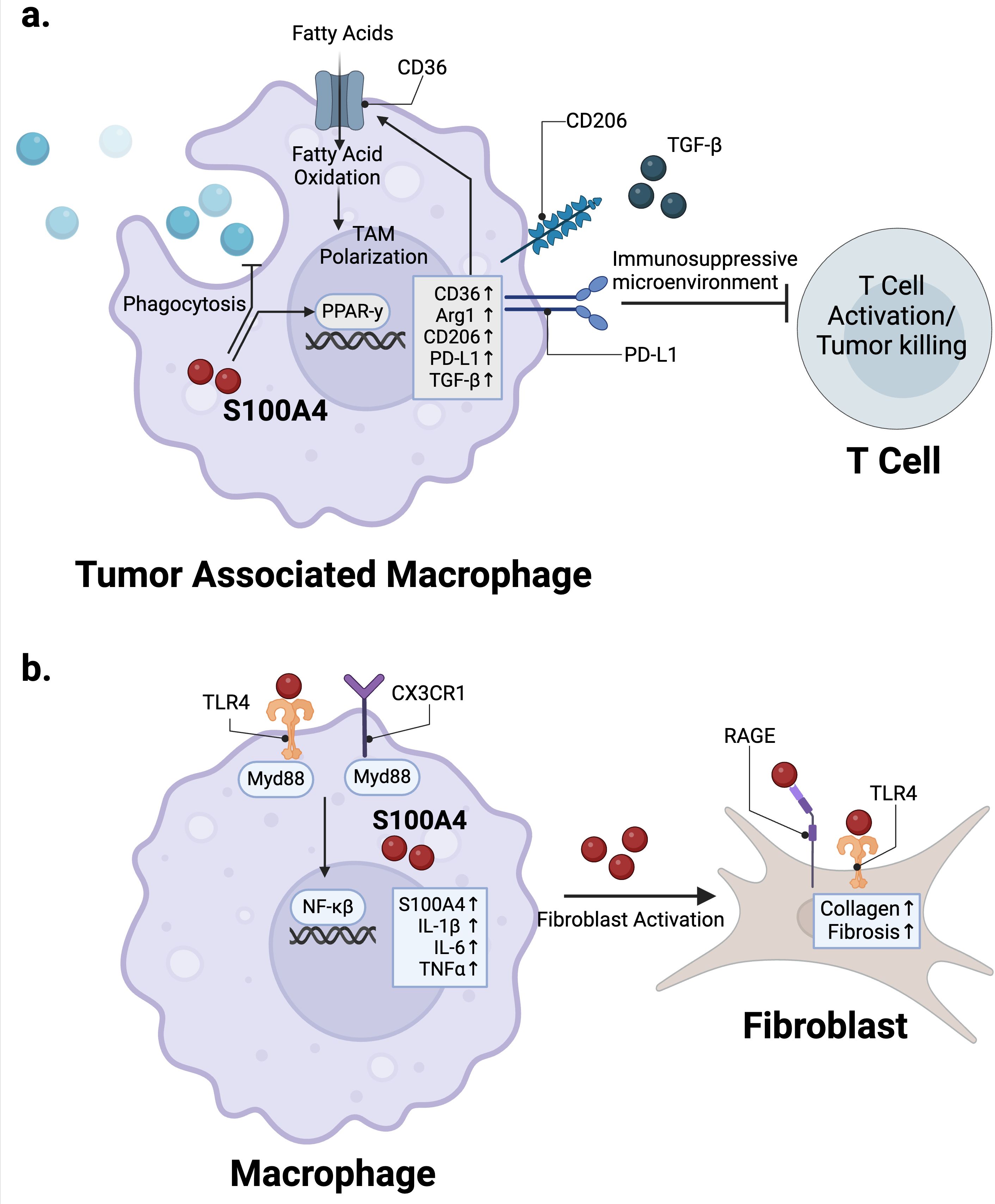
Figure 1. S100A4 regulation of macrophages in cancer and fibrosis. (a) S100A4 expression in TAMs activates PPAR-γ signaling to promote expression of immunosuppressive markers such as Arg1, CD206, PD-L1, as well as CD36, which upregulates fatty acid metabolism to promote TAM polarization. (b) TLR4 and CX3CR1 signaling in macrophages upregulate S100A4 expression, which then activates fibroblasts via RAGE and TLR4 in the context of fibrosis. Created in BioRender. Wong, T. (2025) https://BioRender.com/r88b394.
S100A4 can also play an indirect role in promoting TAM differentiation and activation. To demonstrate this, Prasmickaite et al. conducted in vitro studies by co-culturing THP-1 monocytes with human breast cancer cell lines, as well as studies in primary cultures. They demonstrated that extracellular S100A4 promotes secretion of pro-inflammatory cytokines by breast cancer cells, which triggers THP-1 monocyte-to-macrophage differentiation (42). THP-1 TAMs conditioned in S100A4+ breast cancer cell culture media in turn promote mesenchymal transition of breast cancer cells (42). The authors noted that extracellular S100A4 alone also played a role in macrophage differentiation (42). In a separate study, co-transplantation experiments in vivo with THP-1 and 4T1 cells showed that S100a4 WT, but not S100a4 knockout (KO), THP-1 TAMs promote tumor growth in vivo (36).
2.1.2 S100A4 regulates macrophage-mediated fibrosis and chronic inflammation
S100A4 plays a major role in regulating macrophage function in the context of chronic inflammatory conditions such as fibrosis and RA. Multiple studies have shown that S100A4 expression in macrophages has a pro-fibrotic role (43–46). The role for S100A4 in promoting fibrosis has been recently reviewed by others (8). In brief, evidence suggests that S100A4 signaling via RAGE and/or TLR4 activates the TGF-β/SMAD pathway in fibroblasts to promote fibrosis (47, 48) (Figure 1b). Accordingly, targeting S100A4 either genetically or therapeutically via antibodies and small molecular inhibitors attenuates fibrosis in multiple mouse fibrosis models and human disease models (46, 49–51). An underappreciated facet of this biology is that the source of S100A4 within fibrotic tissues appears to be primarily from bone marrow-derived or tissue-resident macrophages, not from fibroblasts, in contrast to its common name FSP1 (fibroblast specific protein-1) (43–46) (Figure 1b). This has been demonstrated by multiplex immunofluorescence (IF) and flow cytometry analyses in mouse models as well as tissue samples from patients with liver, lung, and kidney fibrosis, where S100A4+ cells co-express macrophage markers such as F4/80, and CD68 (43–45, 50). Studies from mouse models and human liver fibrosis patients indicate that macrophages are the predominant S100A4 expressing population (44, 46, 52). Secreted S100A4 activates hepatic stellate cells, a pericyte-like population of cells with a major role in promoting fibrosis (53, 54), to increase αSMA and collagen production in a c-Myb-dependent manner (46). In mouse models of liver fibrosis, S100A4+ macrophages/Kupffer cells show increased expression of pro-inflammatory cytokines such as TNFα, IL-1β and IL-6, as well as molecules and cytokines classically associated with immunosuppression and the M2-like alternatively activated state, such as IL-10 and COX2 (44) (Figure 1b). S100A4 expression in mouse liver macrophages was shown to be driven by the CX3CR1/Myd88/NF-κβ signaling axis, and targeting this pathway alleviated liver fibrosis in a high-fat diet-driven mouse model of metabolic dysfunction-associated fatty liver disease (MAFLD) in vivo (55) (Figure 1b). Downregulation of METTL14, a methyltransferase decreased in livers of patients with MAFLD and in mouse models of the disease, promotes this pathological S100A4+ macrophage population.
Similarly in lung fibrosis, S100A4 is highly expressed by tissue resident alveolar macrophages and bone-marrow-derived, M2-like macrophages (43, 56). Multiplexed IF in MHV68 and bleomycin induced mouse models of lung fibrosis show that S100A4-positive macrophages co-express the M2-like marker Arg1 (43). In lung samples from human idiopathic pulmonary fibrosis (IPF) patients, S100A4-positive macrophages co-express CD163, another M2-like macrophage marker, suggesting S100A4 expression is in M2-like macrophages (43). Expression of S100A4 in alveolar macrophages was validated by immunohistochemistry staining in a separate cohort of IPF patients (56). Elevated levels of S100A4 in bronchoalveolar lavage fluid have been detected in IPF patients compared to controls, as well as non-specific interstitial pneumonia, hypersensitivity pneumonitis, and sarcoidosis patients (56).
Mouse alveolar macrophages grown in vitro and polarized by IL-4, but not by IFN-γ, increase S100A4 expression, which in turn activates lung fibroblasts and likely contributes to disease progression (43). Consistent with this, S100a4 -/- mice are protected from lung fibrosis (43, 50), including in a bleomycin-induced lung fibrosis model. In this model, protection from fibrosis was reversed through the adoptive transfer of S100A4 wild type (WT) macrophages in S100a4 -/- mice, demonstrating its causal role in this context (50). It is unclear whether S100A4 expression in macrophages regulates its polarization state, as is the case in TAMs, or if it is merely an effector molecule that drives pro-fibrotic states in lung fibroblasts. Nonetheless, S100A4 clearly plays a pathogenic role in these contexts and is a major target for treatment of these conditions.
S100A4 has also been well implicated in RA, with evidence to suggest an immunomodulatory role in monocytes/macrophages in this context (9, 10). Increased S100A4 levels in both plasma and synovial fluid of RA patients is associated with increased disease severity; high S100A4 levels in serum also correspond to poorer treatment response in RA patients (57). S100A4 activates TLR4 signaling in peripheral blood mononuclear cells (PBMCs) isolated from RA patients, leading to increased inflammatory response (9). This study did not identify which cell types are involved in this signaling, but TLR4 is predominantly expressed on cells of myeloid origin, such as monocytes, macrophages, and dendritic cells. Studies in the context of cancer associated myeloid derived suppressor cells (MDSCs) show that S100A4 directly bind to TLR4, suggesting a direct effect in RA as well (58). Furthermore, monocytes from healthy donors exposed to oligomeric S100A4 have enhanced response to lipopolysaccharide (LPS) stimulation, as characterized by increases in IL-1β, IL-6, and TNFα (21).
2.1.3 S100A4 and the interplay between cancer and fibrosis
Notably, there is a growing recognition of the interplay between S100A4, fibrosis, and cancer. Patients with fibrosis are at a higher risk for developing malignancies, and cancer-associated-fibroblasts (CAF) have been extensively studied to elucidate their role in regulating malignant transformation and progression (59–61). S100A4 has previously been implicated in regulation of CAFs (62, 63). S100A4 expressing CAFs secrete VEGF-A and Tenascin-C to promote metastatic colonization of the lung and liver in mouse breast and colorectal cancer models (62). In mouse models of breast cancer metastasis to the lung, macrophage-derived S100A4 promotes formation of the premetastatic niche via activation of lung fibroblasts (64). In human patients and mouse models of hepatocellular carcinoma (HCC), high S100A4 expression positively associates with liver fibrosis and HCC grade and severity (52).
S100A4-expressing macrophages can also be recruited to the liver to induce fibrosis as an adverse outcome in anti-tumor immunotherapy associated toxicity (65). CD137, also known as 4-1BB, is an inducible co-signaling receptor of the TNF receptor superfamily that has been extensively tested as an immunotherapy target in cancer (66–68), but clinical trials with agonistic anti-CD137 antibodies revealed severe dose-dependent hepatotoxicity (69). Studies in mice showed that agonistic anti-CD137 antibody treatment induces liver fibrosis by recruiting macrophages that secrete S100A4 (65). This promote accumulation of pathogenic CD8 T cells in the liver by prolonging their survival via activation of the Akt pathway (65). This phenotype was reversed in S100a4 -/- mice and S100A4-thymidine kinase transgenic mice, where S100A4-expressing cells can be depleted with ganciclovir treatment (65). Combination with antibody blockade of S100A4 also reduced liver fibrosis without inhibiting the anti-CD137 antibody anti-tumor response in the MC38 mouse colorectal cancer (CRC) model, suggesting that simultaneously targeting S100A4 may be viable to alleviate immunotherapy-associated liver toxicity in some contexts (65).
When fibrosis occurs within the TME in the context of cancer, S100A4 has immunomodulatory effect. CAFs can impair immune surveillance and reduce the efficacy of anti-tumor immunotherapy through the TGF-β signaling axis (70–73). This has been demonstrated in syngeneic mouse models of non-small cell lung cancer, metastatic colorectal cancer, and metastatic urothelial cancer (71–73). Additionally, CAFs can regulate TAM function to promote immunosuppression. In breast cancer mouse models, fibrosis regulated by TAMs in a TGF-β dependent manner increases arginine consumption and ornithine production, which reprogrammed CD8 T cells to a dysfunctional state, promoting immunosuppression and tumor progression (74). In contrast, direct depleting CAFs to target fibrosis in pancreatic cancer induces immunosuppression through increase accumulation of regulatory T cells (T-regs) that accelerates disease progression, suggesting a protective role of CAFs in some conditions (75). Macrophages are a central hub for the interplay between these pathological processes, and it will be important to decipher whether the functional state of macrophages may be regulated by S100A4 as both a mediator of macrophage polarization and an effector molecule to signal to other cells, such as fibroblasts.
In summary, these data support a working model where, across disease states such as cancer, fibrosis, and chronic inflammation, S100A4 regulates pathogenic macrophage polarization to an M2-like, alternatively activated state with immunosuppressive and profibrotic functions. In addition, S100A4 is an effector molecule that functions as a signal from macrophages to other stromal cells such as fibroblasts and T cells to promote disease progression.
2.2 Myeloid derived suppressor cells
MDSCs describe a heterogenous population of immature (MHCII-negative) myeloid cells that are defined functionally by their ability to suppress T cell activity (76). They accumulate in various pathological conditions, particularly in cancer and chronic inflammation, and contribute to disease progression and resistance to cancer treatments (76).
S100A4 binding to TLR4 regulates MDSCs in different disease states. In cancer, S100a4 -/- mice are partially protected from tumor growth due to loss of circulating MDSCs (58). Additionally, exogenous S100A4 protects MDSCs from 5-fluorouracil-induced apoptosis by activating TLR4-ERK1/2 signaling (58) (Figure 2). Consistent with this, a different study showed that extracellular S100A4 promotes MDSC expansion and suppression of T cell proliferation and activation in the context of acute myeloid leukemia (AML) (77). This study showed that S100A4 binds to GP130 on MDSCs to activate JAK2/STAT3 signaling (77). GP130 is a newly identified receptor of S100A4, and S100A4 mediated activation of the GP130/JAK2/STAT3 signaling pathway in MDSCs promotes expression of Arg1, TGF-β, and IL-10 (77).
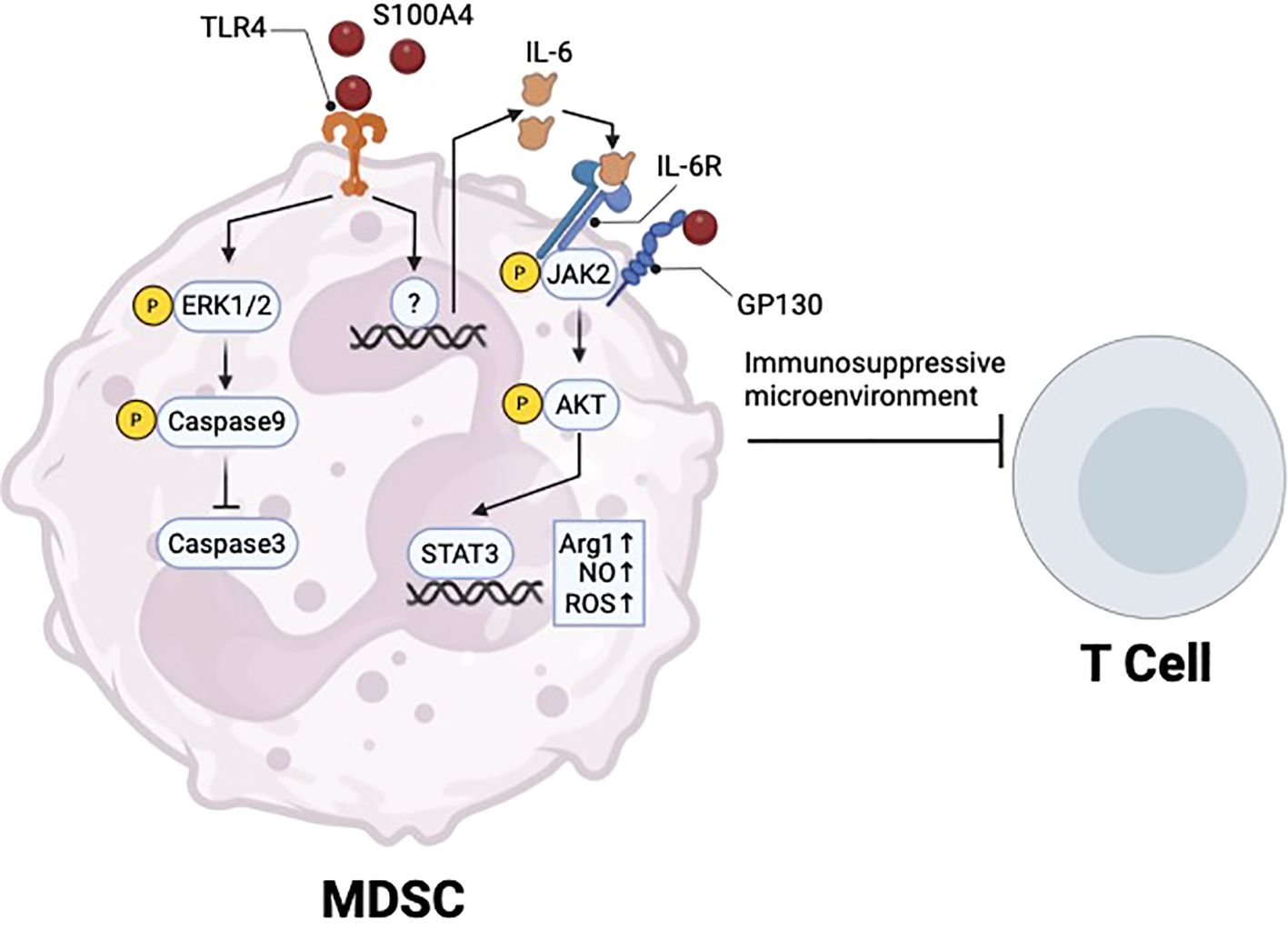
Figure 2. S100A4 regulation of MDSC survival and immunosuppressive function. Extracellular S100A4 binds to TLR4 to promote ERK1/2 signaling and prevent apoptosis, thus promoting MDSC survival in cancer. S100A4-TLR4 axis also upregulates IL-6, which can act through autocrine signaling to activate JAK2/STAT3, which promotes the immunosuppressive function of MDSCs. Created in BioRender. Wong, T. (2025) https://BioRender.com/f68w574.
In a separate study of Sjogren’s syndrome, a long-term autoimmune disease, S100A4 signaling via olfactory ecto-mesenchymal stem cell-derived (OE-MSC) exosomes promoted IL-6 production in MDSCs via TLR4 signaling, which led to activation of the JAK2/STAT3 pathway (29) (Figure 2). This led to upregulation of Arg1 expression, as well as nitric oxide (NO) and reactive oxygen species (ROS) production in MDSCs, to promote immunosuppressive function (29) (Figure 2). In vitro, blockade of TLR4 using a neutralizing antibody prevented IL-6 production in MDSCs, while blockade of MDSC-derived exosomal IL-6 by siRNA knockdown reversed immunosuppressive effects on CD4 T cell proliferation (29).
Interestingly, exogenous S100A4 has been shown to promote IL-6 production in monocytes, while S100A4-positive macrophages in the liver were shown to have increased expression of IL-6 transcripts compared with S100A4-negative counterparts. IL-6 is a driver of M2-like polarization in macrophages via activation of JAK2/STAT3 signaling (78, 79). In addition to exogenous S100A4 signaling, single-cell RNA sequencing (scRNAseq) of tumor infiltrating immune cells in glioma patients showed that S100A4 is highly expressed in MDSCs and immunosuppressive macrophages (15). These observations suggest that exogenous and endogenous S100A4 expression is correlated with IL-6 expression in different myeloid cells and may underlie the role of S100A4 in immune suppressive myeloid cells (MDSC and M2-like macrophages) (21, 44).
2.3 Dendritic cells
While most studies investigating the role of S100A4 in immune cells has focused on macrophages and lymphocytes, some reports provide compelling evidence that S100A4 promotes adaptive immunity through DCs. Ovalbumin (OVA) is a model allergen commonly used to induce a Th2 polarized response (80). S100a4 -/- mice are protected from OVA-induced allergic dermatitis, characterized by reduced infiltration of eosinophils, T cells, neutrophils, and DCs at the challenge site, coupled with reduced serum OVA-specific antibodies and T cell memory responses (14). DCs isolated from S100a4 -/- mice had reduced capacity to stimulate T cell proliferation in vitro (14). This phenotype was partially abrogated by treatment with recombinant S100A4, suggesting that extracellular and intracellular S100A4 signaling in DCs may have non-redundant roles in promoting T cell activation/proliferation. A subsequent study confirmed these results, and further showed that adoptive transfer of WT DCs, but not S100a4 -/- DCs, into S100a4 -/- mice one day prior to immunization with OVA could restore humoral and cellular immune responses, implicating the importance of S100A4 in regulating adaptive immunity through DCs (81). Extracellular S100A4 signaling through RAGE and TLR4 was shown to potentiate activation of DCs by upregulating both expression of cytokines critical to adaptive immunity, such as IL-2 and IL-6, as well as co-stimulatory molecules, such as CD80, CD86, and CD40 in vitro (82, 83). S100A4 induced cytokine expression in DCs was partially mediated by NF-κβ signaling, while co-stimulatory molecules CD80 and CD86 were not, indicating that S100A4 acts through multiple downstream signaling pathways to regulate DC function (82) (Figure 3).
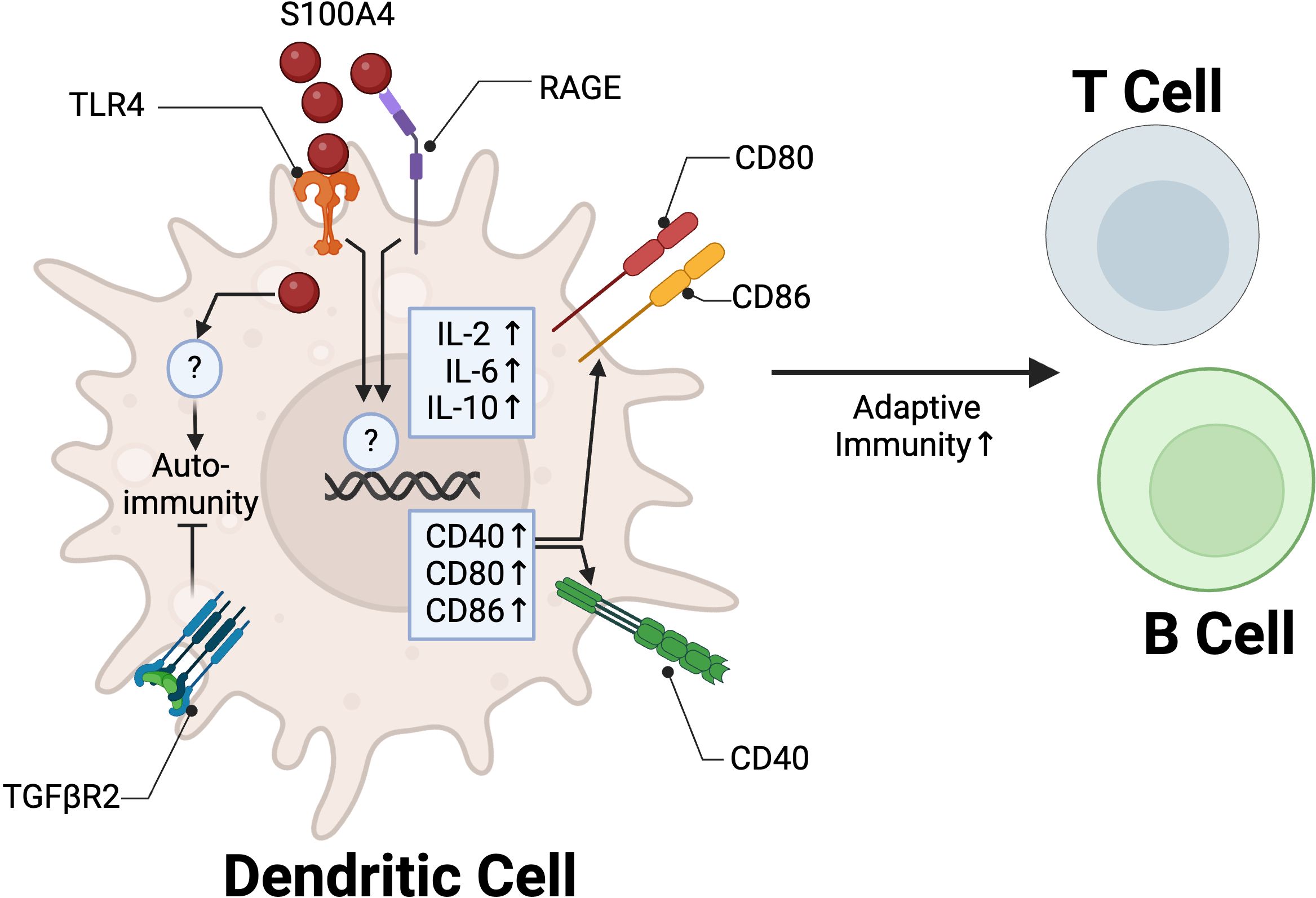
Figure 3. S100A4 regulation of DC function in adaptive immunity. In DCs, extracellular S100A4 binds to TLR4 and RAGE to promote adaptive immunity through upregulation of IL-2 and IL-6 and co-stimulatory molecules CD40, CD80, and CD86. TGFβR2 signaling in S100A4+ DCs is critical to promote tolerance and prevent autoimmune pancreatitis. Created in BioRender. Wong, T. (2025) https://BioRender.com/y63r777.
S100A4 is also involved in the tolerogenic function of DCs. Human monocyte-derived DC’s pulsed with soluble S100A4 were shown to increase CD4+ CD25+ FOXP3+ regulatory T cell proliferation in vitro (84). A separate study showed that TGF-β signaling may mediate immune tolerance in S100A4-expressing DCs. Mice with S100A4/FSP1 Cre-mediated conditional knockout of TGFβR2 (Tgfbr2fspKO) spontaneously develop autoimmune pancreatitis by 6 weeks of age. Adoptive transfer of Tgfbr2fspKO DCs, but not WT DCs, into 2-week-old syngeneic WT mice similarly led to induction of autoimmune pancreatitis (85) (Figure 3). The molecular mechanisms underlying the interplay between S100A4 and TGF-β in regulating DCs remain. These two players have been implicated in regulating fibroblast activity, with S100A4 as a downstream mediator of TGF-β signaling (48). Overall, these findings suggest that S100A4 plays an essential role in the regulation of adaptive immune responses through positive modulation of DC function to promote inflammation and immune tolerance.
3 S100A4 function in lymphocytes
S100A4 is expressed in T cells during lineage commitment and memory differentiation (86), as well as in pathological conditions of asthma (12), arthritis (87), chronic infection (88), and cancer (15). A S100a4 +/GFP mouse model suggested that during a steady state, S100A4 is primarily expressed in CD4 and CD8 memory T cells compared with naïve populations (86). This was also shown in humans, as bulk RNAseq of sorted human CD8 T cells showed that S100A4 expression was absent in naïve T cells but highly expressed in memory T cell subpopulations (89). Similarly, in pathological conditions such as cancer, viral infection, and chronic inflammation, S100A4 is expressed in activated T cells, T-regs, and exhausted CD8 T cells (15, 87, 88).
While S100A4 was shown to regulate macrophage motility and migration, there are mixed reports regarding T cell migration. S100A4 has been shown to be a chemoattractant for T cells in the context of breast cancer lung metastasis (90, 91), though the receptor mediating this interaction is unknown. S100a4-/- T cells had no change in migration in vitro towards the chemokine CXCL10 (14). Similarly, in mouse models of autoimmune colitis, S100a4-/- T cells sufficiently mediated local autoimmunity (86). In a mouse model of allergic dermatitis, CD8 but not CD4 T cell infiltration at the rechallenge site was decreased in S100a4 -/- mice compared to WT controls (14). In the context of experimental autoimmune encephalitis (EAE), a mouse model of multiple sclerosis driven by Th1 and Th17 response (92), global depletion of S100A4 is protective against EAE symptoms by decreasing microglia mediated neuroinflammation (93). However, while autoreactive CD4+ T cells in EAE upregulate S100A4 (94), adoptive transfer of S100a4-/- T cells are sufficient to drive disease progression into Rag2 -/- mice, indicating T cell recruitment to the central nervous system (CNS) and subsequent function is not impaired by loss of endogenous S100A4 signaling (86). It is worth noting that elevated S100A4 levels have been observed in cerebrospinal fluid of MS patients compared to controls (95); the role of S100A4 in the CNS has been reviewed elsewhere (96).
Reports for T cell activation and polarization are conflicting. Treatment of mouse primary T cells with extracellular S100A4 activates JAK/STAT pathway and reduces Th1 polarization while not affecting Th2 polarization, indicated by marker expression and decreased IFNγ and increased IL-10 secretion (90). In line with this, a recent study in mice administered with a M. tuberculosis vaccine demonstrated that adjuvant S100A4 protein treatment promotes Th1 and Th17 polarization (82, 83), although it is not clear whether this in vivo effect was mediated through direct activation of T cells or through antigen presenting cell (APC) modulation. While extracellular S100A4 appears to partially regulate T cell polarization, multiple studies report that depletion of endogenous S100A4 expression does not impact the capacity of CD4 T cells to differentiation into Th0, Th1, Th2, or Th17 effector cells in vitro under polarizing conditions (86). In a study using a model of bacterial infection with ActA-deficient OVA-transgenic L. Monocytogenes, S100a4 -/- mice showed neither deficiency in number of splenic OVA-specific effector CD8 T cells, nor a decrease in IFNγ production after OVA challenge (86). On the other hand, a different study showed that splenocytes from S100a4 -/- mice showed decreased IFNγ production post stimulation with an αCD3 antibody, supporting the idea that S100A4 is necessary for robust T cell activation (87). Overall, these studies highlight the context-dependent function of S100A4 in regulating T cell polarization and activation. (Figure 4a).
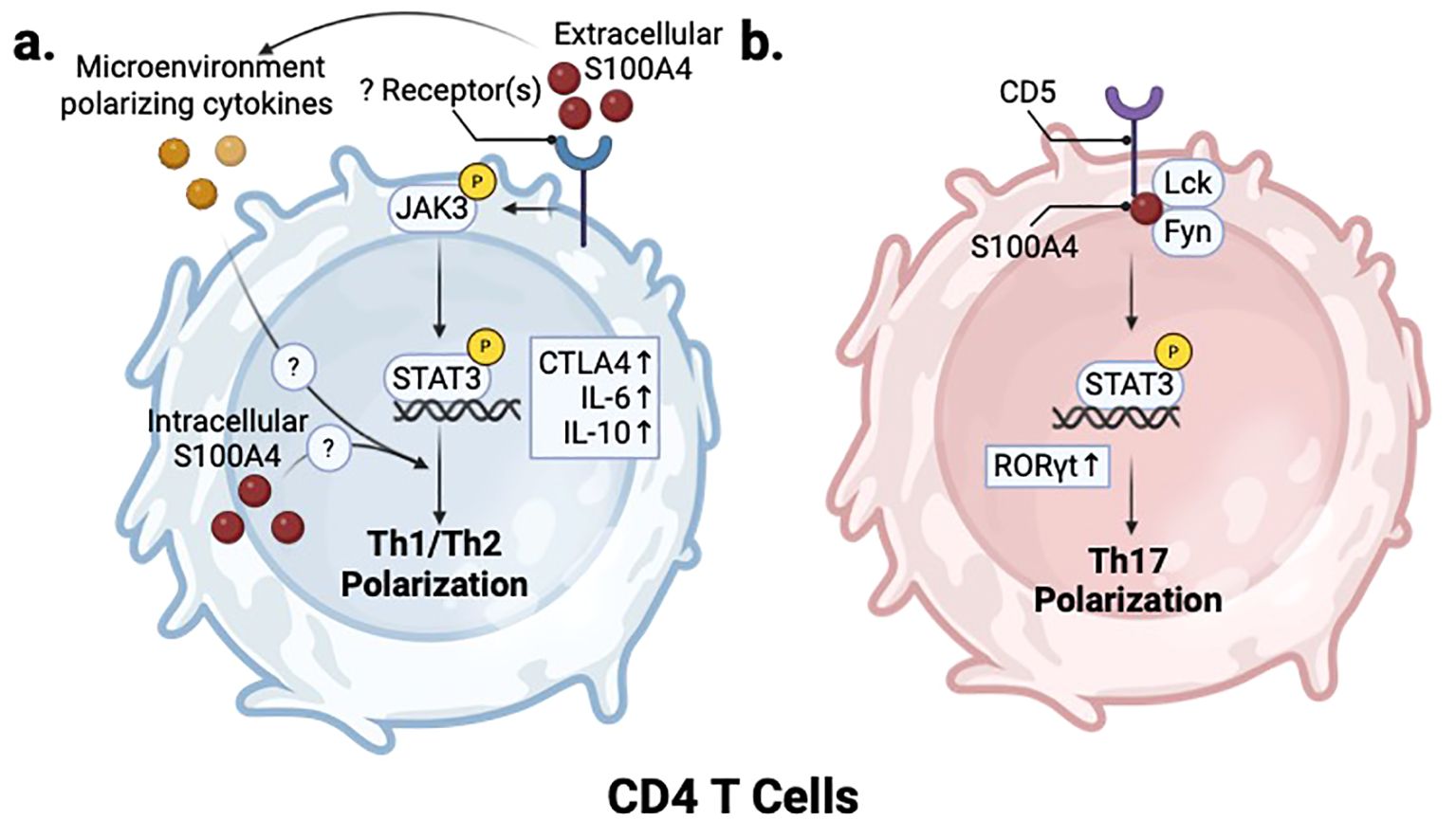
Figure 4. S100A4 regulates T helper cell polarization. (a) In CD4 T cells, S100A4-mediated activation of JAK/STAT signaling through an unknown receptor also inhibits Th1 and promotes Th2 polarization. (b) In Th17 cells, S100A4 regulates Lck/Fyn activity in CD5 signaling to promote RORγt expression and induce Th17 polarization. Created in BioRender. Wong, T. (2025) https://BioRender.com/v93n538.
Another study shows that S100A4 also regulates CD8 T cell survival through the Akt pathway (65). Studies with the mouse CD8 lymphoid cell line CTLL-2 showed that extracellular S100A4 treatment in vitro significantly reduces apoptosis by IL-2 deprivation. This effect could be reversed with an S100A4 blocking antibody and with an Akt inhibitor (65).
3.1 S100A4 regulates T cell function to promote cancer progression and metastasis
S100A4 expression in cancer is highly associated with T cell exhaustion and immunosuppression. Through single cell RNA-sequencing of tissues from glioma patients, we showed that S100A4 is highly expressed in exhausted, tumor-infiltrating CD8 T cells and T-regs (15). In addition, we showed that S100A4 expression regulates T cell immunomodulatory function in cancer. Using an S100a4-GFP knock-in reporter mouse with syngeneic mouse glioma models, we previously showed that GFP+ glioma infiltrating CD4 T cells from S100a4 -/- mice had reduced ability to inhibit T cell proliferation in vitro compared with their counterparts from S100a4 GFP/+ mice (15). However, it is unknown if this is due to secretion and extracellular signaling of S100A4 or via intracellular S100A4 signaling. In a separate study with human PBMCs in vitro, extracellular S100A4 alone was sufficient to enhance T cell immunosuppression by inducing IL-10 and IDO production in T cells (97). Taken together, these results suggest that both intracellular and extracellular S100A4 have immunomodulatory function in T cells.
S100A4 can also promote cancer metastasis by enhancing premetastatic niche formation. T cells play a complex role in regulating the pre-metastatic niche, and their pro- or anti-tumor effects are dependent on cell type and functional state (98). CD4 T cells can play a pro-metastatic role by modulating pro-tumor macrophages via IL-4 signaling or by differentiating into immunosuppressive regulatory T cells that inhibit cytotoxic T cell proliferation (99–101). Using the MMTV-PyMT spontaneous metastatic breast cancer mouse model, one study showed that S100A4 secretion by fibroblasts promotes T cell accumulation in pulmonary metastatic sites to promote metastasis to the lung (91). Antibody blockade of S100A4 in the same mouse model also reduced both primary tumor growth and metastatic outgrowth, with reduced T cell recruitment in the pre-metastatic niche (90). Consistently, S100a4 -/- mice are protected from both primary tumor growth and metastasis (5, 15, 58, 102). In vitro stimulation of T cells with S100A4 revealed increased secretion of cytokines including CCL24 and g-CSF. This was confirmed in vivo in interstitial fluid of T cell-infiltrated lungs in tumor-bearing mice (91) (Figure 5a). g-CSF is a major regulator of neutrophil production and function (103), and several studies have indicated that immunosuppressive neutrophils promote breast cancer lung metastasis by suppressing cytotoxic T cell function (104–107). Consistent with this, we recently reported that a S100A4 blocking antibody (S100A4-11) treatments inhibits neutrophil infiltration in lungs of breast tumor bearing mice, preventing premetastatic niche formation (108). Notably, we did not observe a significant change in T cell numbers but did observe that TIGIT mediated suppression of T and NK cells is reversed in S100A4-11 treated lungs (108).
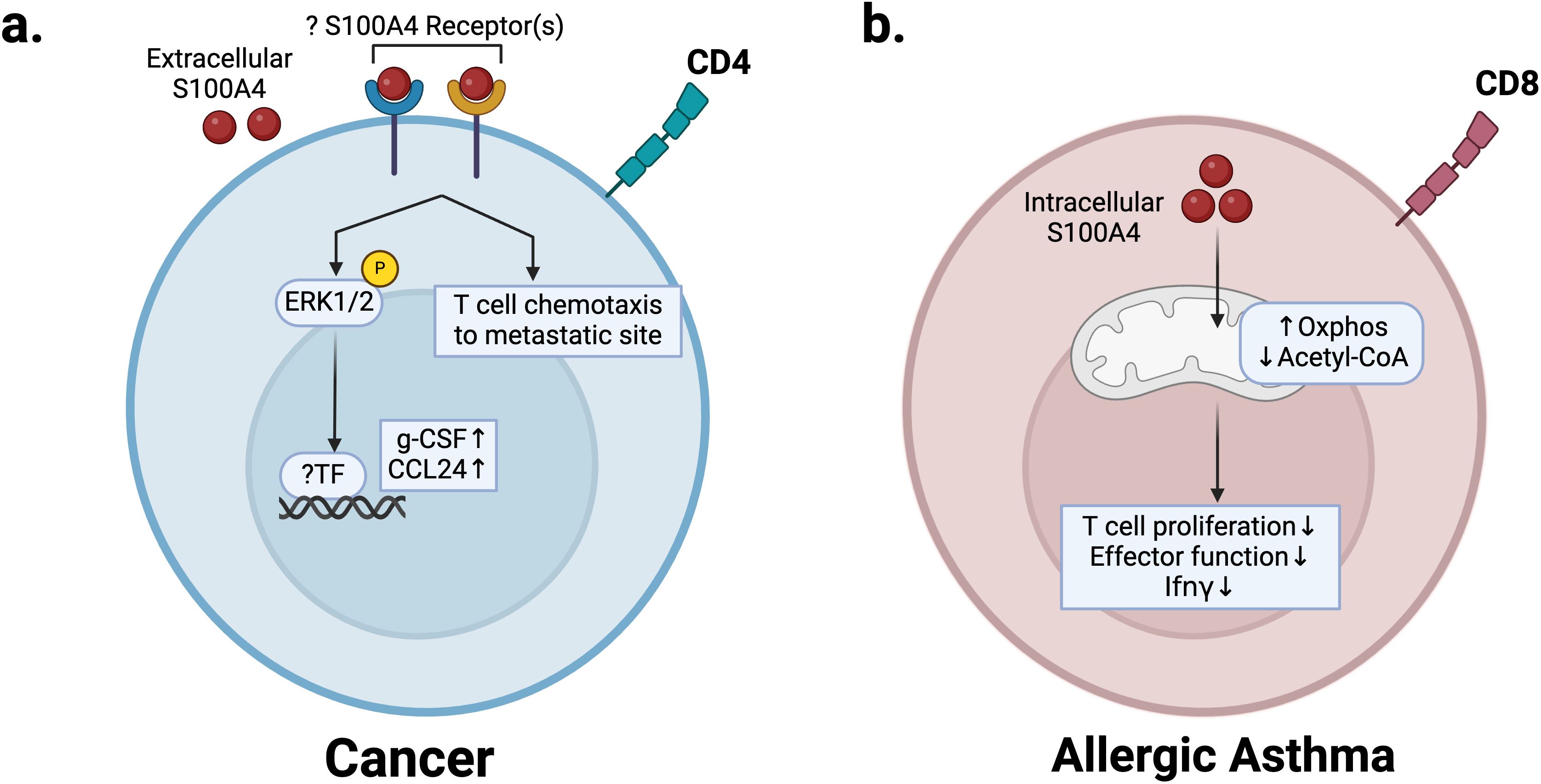
Figure 5. S100A4 regulation of T cells in cancer and allergic asthma disease states. (a) In the premetastatic niche, extracellular S100A4 regulates recruitment of T cells and production of pro-inflammatory cytokines g-CSF and CCL24 to promote neutrophil infiltration and metastasis. (b) In allergic asthma, S100A4 expression in CD8 T cells promotes mitochondrial metabolism, which reduces acetyl-CoA levels, prevents IFNγ production, and decreases effector function, thus promoting pathogenesis. Created in BioRender. Wong, T. (2025) https://BioRender.com/q01y039.
3.2 S100A4 regulates T cell polarization to promote pathogenesis in chronic inflammatory disease
Allergic asthma is a chronic inflammatory disease of the airways driven by Th2 response and eosinophils (109). S100A4 can regulate the T cell-mediated immune response in allergic asthma (12). S100A4 expression in CD8 T cells is increased in mouse models of allergic asthma and leads to CD8 effector memory T cell dysfunction (12). Mechanistically, S100A4 expression upregulates mitochondrial metabolism in CD8 T cells to decrease acetyl-CoA levels, thus impairing effector gene transcription (Figure 5b). This leads to dysfunction of effector memory T cells with high S100A4 expression (S100A4-hi), characterized by impaired IFNγ production (12) (Figure 5b). Under conditions of OVA-induced allergic asthma, S100A4-hi CD8 T cells had increased memory development and proliferation compared with S100A4-low-expressing (S100A4-low) counterparts and drove disease progression by inhibiting IFNγ production. S100A4-low, IFNγ-producing CD8 T cells were shown to be protective via adoptive transfer (12). Decreased IFNγ signaling due to apoptosis of S100A4-low IFNγ producing CD8 T cells and persistence of S100A4-hi CD8 T cells drives an increase in Th2 cytokines in the lungs of asthmatic mice, which is reversed in S100a4 -/- mice (12).
S100A4 also plays a role in the development of Th17 cells in the context of RA by regulating the CD5-STAT3-IRF4-RORγT axis (Figure 4b). An imbalance between FOXP3+ regulatory T cells and RORγT+ Th17 cells promotes the pathogenesis of RA (110). In a methylated bovine serum albumin (mBSA) immunized mouse model of RA, inflamed synovial fluids of S100a4 -/- mice showed higher levels of T-regs and lower levels of RORγT+ Th17 cells than their wildtype counterparts. S100A4 deficiency in mice led to a reduced proportion of IL-17α-producing CD4 T cells within the spleen, which correlated with decreased pSTAT3 and RORγT mRNA, two major regulators of Th17 development. In addition, decreased CD5 expression and activity in CD4 T cells were noted in S100a4 -/- helper T cells (87). In vitro immunoprecipitation experiments using Jurkat cells revealed that S100A4 associates in complex with Src-family kinases Lck and Fyn (87). CD5 was shown to bind directly to the EF2 domain of S100A4 (87) (Figure 4b). Importantly, S100A4 modulates CD5 downstream signaling by regulating Lck/Fyn phosphorylation of the CD5 cytoplasmic domain, thus promoting downstream activation of STAT3 to promote Th17 lineage differentiation (87) (Figure 4b). CD5 is an inhibitory receptor that downregulates TCR signaling on T cells, and plays a role in Th17 development by promoting IL23 receptor expression and STAT3 activation (111). The purported interaction between S100A4 and CD5 may have implications in other disease contexts, such as cancer, given the ability of CD5 to downregulate T cell response (112, 113).
In line with this, studies using mouse models of chronic viral infection show that S100A4 expression is upregulated in exhausted CD8 T cells (88, 89), suggesting that S100A4 expression may be a signature of exhausted CD8 T cells across different pathological conditions.
In conclusion, these results highlight how S100A4 expression in T cells can polarize T cells into pathogenesis-promoting states, consistent across chronic-inflammatory diseases and cancer.
4 S100A4 in immune modulation of cancer
To this point, we have focused on the roles of S100A4 in regulating different immune cell types in normal and pathological contexts such as cancer. However, it is important to also consider that S100A4 is expressed in multiple cell types, including in cancer cells. Secretion of S100A4 from any of these cells can affect multiple downstream cell types including immune cells, thereby impacting the overall immune response. Thus, it is important to understand the overarching immunomodulatory impact of S100A4 within the tumor microenvironment (TME), which has important implications for immunomodulatory therapies. Many studies have focused on S100A4’s ability to promote the progression of cancer and metastasis through regulation of cancer cell motility, cancer cell survival, angiogenesis, cancer stem cell stemness, the epithelial-to-mesenchymal transition, and formation of the premetastatic niche (5, 16, 31, 114–116); however, a growing body of research also implicates S100A4 in immunomodulation of the TME to promote a pro-tumor response (15, 35, 36, 52, 58, 65, 91, 117).
Across cancers, S100A4 expression is associated with poor survival and has a negative impact on the anti-tumor immune response (15, 38, 39, 118), but the mechanism by which S100A4 modulates this response may vary in different cancers. In gliomas, which are characterized by limited T cell infiltration and abundance of immunosuppressive myeloid cells that contribute to therapy resistance (119), depleting S100A4 in stromal cells is sufficient to reprogram the immune microenvironment and increase CD4 and CD8 T cell infiltration, enhance macrophage phagocytosis, and result in significant survival extension (15). In HCC, depleting S100A4 in stromal cells reduces tumor stemness properties and inflammation-associated pathological features (52, 120). Like in liver fibrosis, the majority of S100A4-expressing stromal cells in HCC are of myeloid origin. In colitis-associated CRC mouse models, S100A4 genetic depletion in stromal cells or antibody blockade reduces CRC tumorigenesis (35). This reduction in CRC tumorigenesis is mediated by reduced macrophage and neutrophil infiltration in the colon, resulting in reduced inflammatory response. Decreased S100A4/RAGE-dependent NF-κβ signaling in macrophages also mediates this inflammatory response (35). In mouse models of breast cancer, S100A4 promotes the recruitment of pro-tumor Th2 polarized CD4 T cells to both the primary tumor and metastatic sites to promote disease progression (91, 108, 121), and antibody blockade of S100A4 reduces CD3+ T cell infiltration in the primary tumor and lung metastasis (90). Similarly, T cell accumulation was also reduced by genetic depletion of S100a4 in stromal cells in MMTV-PyMT and CSML100 tumor bearing mice (90, 91). In a syngeneic mouse model of prostate cancer, a S100A4 blocking antibody reduced the overall number of intratumor CD3+ T cells while increasing the number of intratumor CD209+ dendritic cells (117). There was a corresponding decrease in circulatory type 2 cytokines, including IL-4, IL-5, IL-6, and IL-13, as well as CCL5, suggesting that S100A4 blockade reverses the Th1/Th2 imbalance of CD4 T cells that occurs in these tumors (117). These findings align with S100A4’s purported role in altering the Th1/Th2 ratio by inhibiting Th1 polarization (Figure 4a).
In summary, S100A4 is a promising target in cancer therapy, as depletion of S100A4 reprograms the TME to a more anti-tumor environment. However, molecular mechanisms of S100A4 function in these aforementioned settings need further elucidation. Furthermore, additional studies are necessary to determine how and when targeting S100A4 in cancer synergizes with current, standard-of-care chemo, radiation, targeted, or immunotherapies such as anti-PD-1, anti-PD-L1, and anti-CTLA4, or CAR-T and other cellular therapies.
5 S100A4 inhibitors
Given its major role in promoting the progression of cancer, fibrosis, and chronic inflammatory conditions, there are ongoing efforts to develop inhibitors to target S100A4 signaling. Multiple S100A4 blocking antibodies have been reported and evaluated in preclinical models of breast cancer, prostate cancer, HCC, CRC, and skin fibrosis (49, 52, 65, 90, 117, 121). Treatment using the 6B12 antibody in mice decreased metastatic burden but did not change primary tumor size in the CSML100 breast cancer model (121). In the MMTV-PyMT spontaneous metastatic breast cancer mouse model, 6B12 and S100A4-11 antibody treatments reduced both number of metastases and metastatic burden at endpoint (90, 108). Within the premetastatic niche, 6B12 treatment reduced fibronectin deposition, g-CSF production, and T cell recruitment (90). In 4T1 breast model, S100A4-11 antibody also significantly reduced lung metastasis by inhibiting neutrophil infiltration and TIGIT signaling to NK and T cells in the lung to form premetastatic niche (108). Similarly, in a syngeneic mouse prostate cancer model, 6B12 treatment reduced the rate of tumor growth, indicating antibody drugs that target S100A4 hold great promise (117).
While there is no FDA-approved anti-S100A4 antibody therapy, a Phase 1 clinical trial has been successfully completed (NCT05965089). The 6B12 antibody and its humanized counterpart AX-202/CAL101 has also been tested in mouse models of skin fibrosis as well as human systemic sclerosis (SSc) patient skin samples (49, 51). 6B12 treatment showed decreased dermal thickness, myofibroblast count, and collagen deposition in a mouse model of fibrosis. Bulk RNAseq analysis of skin from control and treated animals showed that 6B12 antibody treatment resulted in downregulation of genes associated with inflammation as well as T cell chemotaxis and activation. These results suggest a reduction in inflammatory processes that characterize skin fibrosis. In human disease models, SSc patient derived fibroblasts treated with AX-202/CAL101 exhibited reduced profibrotic gene and protein expression compared to controls (122). Similarly, treatment of human SSc patient precision cut skin samples with AX-202/CAL101 resulted in downregulation of proinflammatory and profibrotic transcriptional pathways as measured by bulk RNAseq (51). Recently, a phase 1 study assessing AX-202/CAL101 in psoriasis patients (NCT05965089) was completed, which showed favorable safety profile. A phase 2 study in patients with IPF will begin recruitment in 2025 (press release from Calluna Pharma).
In the context of allergic disease, 6B12 antibody was also tested in mouse models of asthma and in PBMCs from human allergic rhinitis patients (11, 14). In OVA-sensitized and challenged mice, 6B12 treatment reduced airway hyperresponsiveness, inflammatory cell infiltrates, and release of inflammatory cytokines in bronchioalveolar fluid (11). In PBMCs from allergic rhinitis patients, 6B12 treatment reduced secretion of IL-5 and IL-13, a key cytokine involved in the pathogenesis of allergic rhinitis (14).
Niclosamide and trifluoperazine (TFP) have been identified as non-specific small molecule inhibitors of S100A4 through drug screens of luciferase reporters and S100A4/myosin IIA interaction, respectively (22, 43, 123–129). Niclosamide is an FDA-approved anti-helminthic and has been studied for repurposing and preclinical validation for different diseases such as fibrosis, RA, and asthma due to its anti-inflammatory properties (130, 131). As a possible S100A4-targeting therapeutic, niclosamide was first identified through a high-throughput screen with HCT116 human CRC cell line using a S100A4-promoter-driven luciferase reporter assay (125). Mechanistically, niclosamide inhibits an upstream regulator of S100A4 transcription, the Wnt signaling transcription effector complex CTNNB1/TCF complex (125). However, it is unclear how much of these changes can be attributed to S100A4 inhibition directly and how much other Wnt signaling targets. There are currently several clinical trials investigating niclosamide safety and efficacy in the treatment of cancer (132).
TFP is another FDA-approved, first-generation, anti-psychotic and a member of the phenothiazine family. TFP was identified through a biosensor-based assay as an inhibitor of S100A4/myosin-IIA interaction (133). Compared with other phenothiazines, TFP showed the highest ability to block S100A4 mediated depolymerization of myosin-IIA filaments (22). A crystal structure of S100A4 bound to TFP indicated that TFP disrupts the interaction between S100A4 and myosin-IIA by sequestering S100A4 into S100A4/TFP oligomers (22). TFP has been investigated as an anti-cancer therapeutic because of its function as a calmodulin (CaM) modulator, preventing calcium binding to CaM and increasing cytosolic calcium levels (134, 135). In vitro TFP treatment shows efficacy against human triple-negative breast cancer and glioblastoma (GBM) cell lines. In vivo, TFP suppresses tumor growth in GBM xenograft models (123, 134, 135), though it is unclear whether this is associated with its modulation of S100A4 function. TFP also has immune modulatory function, inhibiting pro-inflammatory cytokine release in vitro and in vivo in the context of sepsis (136). Despite these findings, there have been no further studies assessing TFP in therapeutic targeting of S100A4.
Given S100A4’s diverse function both intracellularly and extracellularly, antibody treatment likely provides a safe way to block S100A4 extracellular signaling to its receptors TLR4 and RAGE, but this will not affect intracellular function of S100A4. In contrast, small molecule inhibitors can likely target both intracellular and extracellular S100A4 function but lack the specificity of antibody treatments. Thus, rational strategies based on S100A4 biology in various contexts are necessary when deciding how best to target S100A4.
6 Concluding remarks
Review of the recent literature shows that S100A4 function in the immune system plays several key roles in the progression of cancer, fibrosis, and autoimmune diseases (Table 1). While much has been revealed of its importance in macrophages, T cells, MDSCs, and DCs, the molecular mechanisms through which S100A4 acts in each cell type is largely unknown. Given its ability to function both extracellularly as a DAMP and cytokine, as well as intracellularly in both the cytoplasm and at the nucleus, cell type specific and mechanistic studies are necessary to determine the specific mechanisms in different diseases.
S100A4 was initially nominated as a therapeutic target in the context of cancer metastasis. The field has since evolved in its understanding of how S100A4 promotes multiple aspects of cancer progression through immune modulation and cancer cell aggressiveness. In addition, S100A4 has emerged as a therapeutic target in fibrosis, again as an immune modulator. S100A4 involvement in these pathological processes is intimately intertwined with its role in regulating systemic and local immune response. Of particular importance is distinguishing the extracellular and intracellular functions of S100A4, as the former is much more readily targetable via antibody blocking, while the latter may require small molecule inhibitors or engineered biologics. Notably, S100a4 -/- mice are viable and fertile, indicating that it is a non-essential gene and potential side effects anticipated from S100A4 inhibitors is minimal. In line with this, the Phase1 trial by Calluna reported favorable safety profile and no serious adverse events. In addition, it will be important to target S100A4 in a cell-type and context-dependent manner, given its differing effects between immune cells and in different diseases.
Crucially, many knowledge gaps remain in our mechanistic understanding of how S100A4 expression and secretion in various cell types promote disease states. Most prior in vivo studies regarding S100A4 function have been performed with germline knockout of S100a4 in mice. It is difficult to delineate which of the interpreted in vivo phenotypes are a direct result of S100A4 function in specific immune cells or are secondary effects of S100A4 deficiency in another immune or stromal cell, including fibroblasts and mesenchymal cells. Using conditional deletion with lineages specific Cre-mice in combination with single-cell methodologies can help to resolve these questions. In addition, while we know that both intracellular and extracellular S100A4 play important roles in these processes, little is known about which specific S100A4 targets, receptors, and downstream signaling pathways mediate these processes in different disease states and cell types. It will be critical to elucidate which S100A4 receptors regulate different immune cell function in various disease contexts, allowing for more targeted approaches for disease modulation.
In conclusion, unraveling the complex interactions between S100A4 and the immune system can open new avenues for therapeutic interventions in cancer, fibrosis, and pro-inflammatory conditions.
Author contributions
TW: Conceptualization, Investigation, Supervision, Visualization, Writing – original draft, Writing – review & editing. RK: Writing – original draft, Writing – review & editing. KY: Conceptualization, Funding acquisition, Project administration, Resources, Supervision, Visualization, Writing – review & editing.
Funding
The author(s) declare that financial support was received for the research, authorship, and/or publication of this article. This study was supported by funding from NIH 1R01NS121405-01 to KY.
Acknowledgments
We would like to acknowledge the efforts of Amy Taylor in assisting with our literature search, and Michelle Swick for editorial assistance. The figures in the article were created with BioRender.
Conflict of interest
KY and TW are inventors of an S100A4 blocking antibody.
The remaining author declare that the research was conducted in the absence of any commercial or financial relationships that could be construed as a potential conflict of interest.
Generative AI statement
The author(s) declare that no Generative AI was used in the creation of this manuscript.
Publisher’s note
All claims expressed in this article are solely those of the authors and do not necessarily represent those of their affiliated organizations, or those of the publisher, the editors and the reviewers. Any product that may be evaluated in this article, or claim that may be made by its manufacturer, is not guaranteed or endorsed by the publisher.
References
1. Sreejit S, Flynn MC, Patil M, Krishnamurthy P, Murphy AJ, Nagareddy P. S100 family proteins in inflammation and beyond. Adv Clin Chem. (2020) 98:173–231. doi: 10.1016/bs.acc.2020.02.006
2. Donato R, Cannon BR, Sorci G, Riuzzi F, Hsu K, Weber DJ, et al. Functions of S100 proteins. Curr Mol Med. (2012) 13:24–57. doi: 10.2174/1566524011307010024
3. Bresnick AR, Weber DJ, Zimmer DB. S100 proteins in cancer. Nat Rev Cancer. (2015) 15:96–109. doi: 10.1038/nrc3893
4. Boye K, Mælandsmo GM. S100a4 and metastasis: A small actor playing many roles. Am J Pathol. (2010) 176:528–35. doi: 10.2353/ajpath.2010.090526
5. Fei F, Qu J, Zhang M, Li Y, Zhang S. S100a4 in cancer progression and metastasis: A systematic review. Oncotarget. (2017) 8:73219–39. doi: 10.18632/oncotarget.18016
6. Ambartsumian N, Klingelhöfer J, Grigorian M. The multifaceted S100a4 protein in cancer and inflammation. Methods Mol Biol. (2019) 1929:339–65. doi: 10.1007/978-1-4939-9030-6_22
7. Struz F, Okada H, Lo C, Danoff TM, Carone R, Tomaszewski J, et al. Identification and characterization of a fibroblast marker: fsp1. J Cell Biol. (1995) 130:393–405. doi: 10.1083/jcb.130.2.393
8. O’Reilly S. S100a4 a classical damp as a therapeutic target in fibrosis. Matrix Biol. (2024) 127:1–7. doi: 10.1016/j.matbio.2024.01.002
9. Cerezo LA, Remáková M, Tomčik M, Gay S, Neidhart M, Lukanidin E, et al. The metastasis-associated protein S100a4 promotes the inflammatory response of mononuclear cells via the tlr4 signalling pathway in rheumatoid arthritis. Rheumatol (Oxford). (2014) 53:1520–6. doi: 10.1093/rheumatology/keu031
10. Klingelhöfer J, Senolt L, Baslund B, Nielsen GH, Skibshøj I, Pavelka K, et al. Up-regulation of metastasis-promoting S100a4 (Mts-1) in rheumatoid arthritis: putative involvement in the pathogenesis of rheumatoid arthritis. Arthritis Rheum. (2007) 56:779–89. doi: 10.1002/art.22398
11. Liu S, Liu M, Zhong J, Chen S, Wang Z, Gao X, et al. Anti-S100a4 antibody administration alleviates bronchial epithelial-mesenchymal transition in asthmatic mice. Open Med (Warsaw Poland). (2023) 18. doi: 10.1515/med-2022-0622
12. Zhang H, Liu S, Li Y, Li J, Ni C, Yang M, et al. Dysfunction of S100a4(+) effector memory cd8(+) T cells aggravates asthma. Eur J Immunol. (2022) 52:978–93. doi: 10.1002/eji.202149572
13. Wu T, Ma L, Jin X, He J, Chen K, Zhang D, et al. S100a4 is critical for a mouse model of allergic asthma by impacting mast cell activation. Front Immunol. (2021) 12:692733. doi: 10.3389/fimmu.2021.692733
14. Bruhn S, Fang Y, Barrenäs F, Gustafsson M, Zhang H, Konstantinell A, et al. A generally applicable translational strategy identifies S100a4 as a candidate gene in allergy. Sci Trans Med. (2014) 6. doi: 10.1126/scitranslmed.3007410
15. Abdelfattah N, Kumar P, Wang C, Leu JS, Flynn WF, Gao R, et al. Single-cell analysis of human glioma and immune cells identifies S100a4 as an immunotherapy target. Nat Commun. (2022) 13:767. doi: 10.1038/s41467-022-28372-y
16. Chow K-H, Park HJ, George J, Yamamoto K, Gallup AD, Graber JH, et al. S100a4 is a biomarker and regulator of glioma stem cells that is critical for mesenchymal transition in glioblastoma. Cancer Res. (2017) 77:5360–73. doi: 10.1158/0008-5472.CAN-17-1294
17. Lo JF, Yu CC, Chiou SH, Huang CY, Jan CI, Lin SC, et al. The epithelial-mesenchymal transition mediator S100a4 maintains cancer-initiating cells in head and neck cancers. Cancer Res. (2011) 71:1912–23. doi: 10.1158/0008-5472.CAN-10-2350
18. Tarabykina S, Scott DJ, Herzyk P, Hill TJ, Tame JRH, Kriajevska M, et al. The dimerization interface of the metastasis-associated protein S100a4 (Mts1). J Biol Chem. (2001) 276:24212–22. doi: 10.1074/jbc.M009477200
19. Pathuri P, Vogeley L, Luecke H. Crystal structure of metastasis-associated protein S100a4 in the active, calcium-bound form. J Mol Biol. (2008) 383:62–77. doi: 10.1016/j.jmb.2008.04.076
20. Novitskaya V, Grigorian M, Kriajevska M, Tarabykina S, Bronstein I, Berezin V, et al. Oligomeric forms of the metastasis-related mts1 (S100a4) protein stimulate neuronal differentiation in cultures of rat hippocampal neurons *. J Biol Chem. (2000) 275:41278–86. doi: 10.1074/jbc.M007058200
21. Neidhart M, Pajak A, Laskari K, Riksen NP, Joosten LAB, Netea MG, et al. Oligomeric S100a4 is associated with monocyte innate immune memory and bypass of tolerance to subsequent stimulation with lipopolysaccharides. Front Immunol. (2019) 10:791. doi: 10.3389/fimmu.2019.00791
22. Malashkevich VN, Dulyaninova N, Ramagopal UA, Liriano MA, Varney KM, Knight D, et al. Phenothiazines inhibit S100a4 function by inducing protein oligomerization. Proc Natl Acad Sci USA. (2010) 107:8605–10. doi: 10.1073/pnas.0913660107
23. Li ZH, Dulyaninova NG, House RP, Almo SC, Bresnick AR. S100a4 regulates macrophage chemotaxis. Mol Biol Cell. (2010) 21:2598–610. doi: 10.1091/mbc.e09-07-0609
24. Dulyaninova NG, Ruiz PD, Gamble MJ, Backer JM, Bresnick AR. S100a4 regulates macrophage invasion by distinct myosin-dependent and myosin-independent mechanisms. Mol Biol Cell. (2018) 29:632–42. doi: 10.1091/mbc.E17-07-0460
25. Chen M, Bresnick AR, O’Connor KL. Coupling S100a4 to rhotekin alters rho signaling output in breast cancer cells. Oncogene. (2013) 32:3754–64. doi: 10.1038/onc.2012.383
26. Li Z-H, Bresnick AR. The S100a4 metastasis factor regulates cellular motility via a direct interaction with myosin-iia. Cancer Res. (2006) 66:5173–80. doi: 10.1158/0008-5472.CAN-05-3087
27. Orre LM, Panizza E, Kaminskyy VO, Vernet E, Gräslund T, Zhivotovsky B, et al. S100a4 interacts with P53 in the nucleus and promotes P53 degradation. Oncogene. (2013) 32:5531–40. doi: 10.1038/onc.2013.213
28. Wu X, Zhang H, Jiang G, Peng M, Li C, Lu J, et al. Exosome-transmitted S100a4 induces immunosuppression and non-small cell lung cancer development by activating stat3. Clin Exp Immunol. (2022) 210:309–20. doi: 10.1093/cei/uxac102
29. Rui K, Hong Y, Zhu Q, Shi X, Xiao F, Fu H, et al. Olfactory ecto-mesenchymal stem cell-derived exosomes ameliorate murine sjögren’s syndrome by modulating the function of myeloid-derived suppressor cells. Cell Mol Immunol. (2021) 18:440–51. doi: 10.1038/s41423-020-00587-3
30. Sun H, Wang C, Hu B, Gao X, Zou T, Luo Q, et al. Exosomal S100a4 derived from highly metastatic hepatocellular carcinoma cells promotes metastasis by activating stat3. Signal Transduction Targeted Ther. (2021) 6. doi: 10.1038/s41392-021-00579-3
31. Park W-Y, Gray JM, Holewinski RJ, Andresson T, So JY, Carmona-Rivera C, et al. Apoptosis-induced nuclear expulsion in tumor cells drives S100a4-mediated metastatic outgrowth through the rage pathway. Nat Cancer. (2023) 4:419–35. doi: 10.1038/s43018-023-00524-z
32. Yammani RR, Carlson CS, Bresnick AR, Loeser RF. Increase in production of matrix metalloproteinase 13 by human articular chondrocytes due to stimulation with S100a4: role of the receptor for advanced glycation end products. Arthritis rheumatism. (2006) 54:2901–11. doi: 10.1002/art.22042
33. Ruma IMW, Kinoshita R, Tomonobu N, Inoue Y, Kondo E, Yamauchi A, et al. Embigin promotes prostate cancer progression by S100a4-dependent and-independent mechanisms. Cancers. (2018) 10. doi: 10.3390/cancers10070239
34. Dmytriyeva O, Pankratova S, Owczarek S, Sonn K, Soroka V, Ridley CM, et al. The metastasis-promoting S100a4 protein confers neuroprotection in brain injury. Nat Commun. (2012) 3. doi: 10.1038/ncomms2202
35. Zhang J, Hou S, Gu J, Tian T, Yuan Q, Jia J, et al. S100a4 promotes colon inflammation and colitis-associated colon tumorigenesis. OncoImmunology. (2018) 7(8):393–405. doi: 10.1080/2162402X.2018.1461301
36. Liu S, Zhang H, Li Y, Zhang Y, Bian Y, Zeng Y, et al. S100a4 enhances protumor macrophage polarization by control of ppar-γ-dependent induction of fatty acid oxidation. J Immunother Cancer. (2021) 9. doi: 10.1136/jitc-2021-002548
37. Coulton A, Murai J, Qian D, Thakkar K, Lewis CE, Litchfield K, et al. Using a pan-cancer atlas to investigate tumour associated macrophages as regulators of immunotherapy response. Nat Commun. (2024) 15. doi: 10.1038/s41467-024-49885-8
38. Ding Z, Deng Z, Li H. Single-cell transcriptome analysis reveals the key genes associated with macrophage polarization in liver cancer. Hepatol Commun. (2023) 7. doi: 10.1097/hc9.0000000000000304
39. Kazakova E, Rakina M, Sudarskikh T, Iamshchikov P, Tarasova A, Tashireva L, et al. Angiogenesis regulators S100a4, sparc and spp1 correlate with macrophage infiltration and are prognostic biomarkers in colon and rectal cancers. Front Oncol. (2023) 13:1058337. doi: 10.3389/fonc.2023.1058337
40. Su P, Wang Q, Bi E, Ma X, Liu L, Yang M, et al. Enhanced lipid accumulation and metabolism are required for the differentiation and activation of tumor-associated macrophages. Cancer Res. (2020) 80:1438–50. doi: 10.1158/0008-5472.CAN-19-2994
41. Wu H, Han Y, Sillke YR, Deng H, Siddiqui S, Treese C, et al. Lipid droplet-dependent fatty acid metabolism controls the immune suppressive phenotype of tumor-associated macrophages. EMBO Mol Med. (2019) 11. doi: 10.15252/emmm.201910698
42. Prasmickaite L, Tenstad EM, Pettersen S, Jabeen S, Egeland EV, Nord S, et al. Basal-like breast cancer engages tumor-supportive macrophages via secreted factors induced by extracellular S100a4. Mol Oncol. (2018) 12:1540–58. doi: 10.1002/1878-0261.12319
43. Zhang W, Ohno S, Steer B, Klee S, Staab-Weijnitz CA, Wagner D, et al. S100a4 is secreted by alternatively activated alveolar macrophages and promotes activation of lung fibroblasts in pulmonary fibrosis. Front Immunol. (2018) 9:1216. doi: 10.3389/fimmu.2018.01216
44. Österreicher CH, Penz-Österreicher M, Grivennikov SI, Guma M, Koltsova EK, Datz C, et al. Fibroblast-specific protein 1 identifies an inflammatory subpopulation of macrophages in the liver. Proc Natl Acad Sci U.S.A. (2011) 108:308–13. doi: 10.1073/pnas.1017547108
45. Lin S-L, Kisseleva T, Brenner DA, Duffield JS. Pericytes and perivascular fibroblasts are the primary source of collagen-producing cells in obstructive fibrosis of the kidney. Am J Pathol. (2008) 173:1617–27. doi: 10.2353/ajpath.2008.080433
46. Chen L, Li J, Zhang J, Dai C, Liu X, Wang J, et al. S100a4 promotes liver fibrosis via activation of hepatic stellate cells. J Hepatol. (2015) 62:156–64. doi: 10.1016/j.jhep.2014.07.035
47. Bhattacharyya S, Kelley K, Melichian DS, Tamaki Z, Fang F, Su Y, et al. Toll-like receptor 4 signaling augments transforming growth factor-β Responses. Am J Pathol. (2013) 182:192–205. doi: 10.1016/j.ajpath.2012.09.007
48. Tomcik M, Palumbo-Zerr K, Zerr P, Avouac J, Dees C, Sumova B, et al. S100a4 amplifies tgf-β-induced fibroblast activation in systemic sclerosis. Ann Rheumatic Dis. (2015) 74:1748–55. doi: 10.1136/annrheumdis-2013-204516
49. Švec X, Štorkánová H, Trinh-Minh T, Tran MC, Štorkánová L, Hulejová H, et al. S100a4-neutralizing monoclonal antibody 6b12 counteracts the established experimental skin fibrosis induced by bleomycin. Rheumatol (Oxford). (2024) 63:817–25. doi: 10.1093/rheumatology/kead295
50. Li Y, Bao J, Bian Y, Erben U, Wang P, Song K, et al. S100a4+ Macrophages are necessary for pulmonary fibrosis by activating lung fibroblasts. Front Immunol. (2018) 9:1776. doi: 10.3389/fimmu.2018.01776
51. Trinh-Minh T, Györfi A-H, Tomcik M, Tran-Manh C, Zhou X, Dickel N, et al. Effect of anti-S100a4 monoclonal antibody treatment on experimental skin fibrosis and systemic sclerosis–specific transcriptional signatures in human skin. Arthritis Rheumatol. (2024) 76:783–95. doi: 10.1002/art.42781
52. Li Y, Wang J, Song K, Liu S, Zhang H, Wang F, et al. S100a4 promotes hepatocellular carcinogenesis by intensifying fibrosis-associated cancer cell stemness. Oncoimmunology. (2020) 9:1725355. doi: 10.1080/2162402X.2020.1725355
53. Mederacke I, Hsu CC, Troeger JS, Huebener P, Mu X, Dapito DH, et al. Fate tracing reveals hepatic stellate cells as dominant contributors to liver fibrosis independent of its aetiology. Nat Commun. (2013) 4. doi: 10.1038/ncomms3823
54. Higashi T, Friedman SL, Hoshida Y. Hepatic stellate cells as key target in liver fibrosis. Advanced Drug Delivery Rev. (2017) 121:27–42. doi: 10.1016/j.addr.2017.05.007
55. Wang YF, Zhang WL, Li ZX, Liu Y, Tan J, Yin HZ, et al. Mettl14 downregulation drives S100a4(+) monocyte-derived macrophages via myd88/nf-κb pathway to promote mafld progression. Signal Transduct Target Ther. (2024) 9:91. doi: 10.1038/s41392-024-01797-1
56. Lee J-U, Chang HS, Shim E-Y, Park J-S, Koh E-S, Shin H-K, et al. The S100 calcium-binding protein A4 level is elevated in the lungs of patients with idiopathic pulmonary fibrosis. Respir Med. (2020) 171. doi: 10.1016/j.rmed.2020.105945
57. Šenolt L, Grigorian M, Lukanidin E, Simmen B, Michel BA, Pavelka K, et al. S100a4 is expressed at site of invasion in rheumatoid arthritis synovium and modulates production of matrix metalloproteinases. Ann Rheumatic Dis. (2006) 65:1645–48. doi: 10.1136/ard.2005.047704
58. Li Q, Dai C, Xue R, Wang P, Chen L, Han Y, et al. S100a4 protects myeloid-derived suppressor cells from intrinsic apoptosis via tlr4-erk1/2 signaling. Front Immunol. (2018) 9:388. doi: 10.3389/fimmu.2018.00388
59. Piersma B, Hayward MK, Weaver VM. Fibrosis and cancer: A strained relationship. Biochim Biophys Acta Rev Cancer. (2020) 1873. doi: 10.1016/j.bbcan.2020.188356
60. Winkler J, Abisoye-Ogunniyan A, Metcalf KJ, Werb Z, Winkler J, Abisoye-Ogunniyan A, et al. Concepts of extracellular matrix remodelling in tumour progression and metastasis. Nat Commun. (2020) 11. doi: 10.1038/s41467-020-18794-x
61. Sugimoto H, Mundel TM, Kieran MW, Kalluri R. Identification of fibroblast heterogeneity in the tumor microenvironment. Cancer Biol Ther. (2006) 5:1640–46. doi: 10.4161/cbt.5.12.3354
62. O’Connell JT, Sugimoto H, Cooke VG, MacDonald BA, Mehta AI, LeBleu VS, et al. Vegf-a and tenascin-C produced by S100a4+ Stromal cells are important for metastatic colonization. Proc Natl Acad Sci USA. (2011) 108:16002–7. doi: 10.1073/pnas.1109493108
63. Bartoschek M, Oskolkov N, Bocci M, Lövrot J, Larsson C, Sommarin M, et al. Spatially and functionally distinct subclasses of breast cancer-associated fibroblasts revealed by single cell rna sequencing. Nat Commun. (2018) 9. doi: 10.1038/s41467-018-07582-3
64. Qi Y, Zhao T, Li R, Han M, Guo X. Macrophage-secreted S100a4 supports breast cancer metastasis by remodeling the extracellular matrix in the premetastatic niche. BioMed Res Int. (2022) 2022(9895504):1–14. doi: 10.1155/2022/9895504
65. Zhang J, Song K, Wang J, Li Y, Liu S, Dai C, et al. S100a4 blockage alleviates agonistic anti-cd137 antibody-induced liver pathology without disruption of antitumor immunity. Oncoimmunology. (2018) 7. doi: 10.1080/2162402X.2017.1296996
66. Ye Q, Song D-G, Poussin M, Yamamoto T, Best A, Li C, et al. Cd137 accurately identifies and enriches for naturally-occurring tumor-reactive T cells in tumor. Clin Cancer research: an Off J Am Assoc Cancer Res. (2014) 20:44–55. doi: 10.1158/1078-0432.CCR-13-0945
67. Narazaki H, Zhu Y, Luo L, Zhu G, Chen L. Cd137 agonist antibody prevents cancer recurrence: contribution of cd137 on both hematopoietic and nonhematopoietic cells. Blood. (2010) 115:1941–8. doi: 10.1182/blood-2008-12-192591
68. Geuijen C, Tacken P, Wang L-C, Klooster R, van Loo PF, Zhou J, et al. A human cd137×Pd-L1 bispecific antibody promotes anti-tumor immunity via context-dependent T cell costimulation and checkpoint blockade. Nat Commun. (2021) 12. doi: 10.1038/s41467-021-24767-5
69. Segal NH, Logan TF, Hodi FS, McDermott D, Melero I, Hamid O, et al. Results from an integrated safety analysis of urelumab, an agonist anti-cd137 monoclonal antibody. Clin Cancer Res. (2017) 23:1929–36. doi: 10.1158/1078-0432.CCR-16-1272
70. Jiang H, Hegde S, DeNardo DG. Tumor-associated fibrosis as a regulator of tumor immunity and response to immunotherapy. Cancer Immunology Immunotherapy: CII. (2017) 66:1037–48. doi: 10.1007/s00262-017-2003-1
71. Herzog BH, Baer JM, Borcherding N, Kingston NL, Belle JI, Knolhoff BL, et al. Tumor-associated fibrosis impairs immune surveillance and response to immune checkpoint blockade in non–small cell lung cancer. Sci Trans Med. (2023) 15. doi: 10.1126/scitranslmed.adh8005
72. Mariathasan S, Turley SJ, Nickles D, Castiglioni A, Yuen K, Wang Y, et al. Tgfβ Attenuates tumour response to pd-L1 blockade by contributing to exclusion of T cells. Nature. (2018) 554:544–8. doi: 10.1038/nature25501
73. Tauriello DVF, Palomo-Ponce S, Stork D, Berenguer-Llergo A, Badia-Ramentol J, Iglesias M, et al. Tgfβ Drives immune evasion in genetically reconstituted colon cancer metastasis. Nature. (2018) 554:538–43. doi: 10.1038/nature25492
74. Tharp KM, Kersten K, Maller O, Timblin GA, Stashko C, Canale FP, et al. Tumor-associated macrophages restrict cd8+ T cell function through collagen deposition and metabolic reprogramming of the breast cancer microenvironment. Nat Cancer. (2024) 5:1045–62. doi: 10.1038/s43018-024-00775-4
75. Özdemir BC, Pentcheva-Hoang T, Carstens JL, Zheng X, Wu C-C, Simpson T, et al. Depletion of carcinoma-associated fibroblasts and fibrosis induces immunosuppression and accelerates pancreas cancer with diminished survival. Cancer Cell. (2014) 25:719–34. doi: 10.1016/j.ccr.2014.04.005
76. Hegde S, Leader AM, Merad M. Mdsc: markers, development, states, and unaddressed complexity. Immunity. (2021) 54:875–84. doi: 10.1016/j.immuni.2021.04.004
77. Peng Y, Zhang J, Zhang T, Wang C, Bai J, Li Y, et al. S100a4 mediates the accumulation and functions of myeloid-derived suppressor cells via gp130/jak2/stat3 signaling in acute myeloid leukemia. Biochim Biophys Acta (BBA) - Mol Basis Dis. (2025) 1871. doi: 10.1016/j.bbadis.2024.167498
78. Chen S, Saeed AF, Liu Q, Jiang Q, Xu H, Xiao GG, et al. Macrophages in immunoregulation and therapeutics. Signal Transduction Targeted Ther. (2023) 8. doi: 10.1038/s41392-023-01452-1
79. Fu X-L, Duan W, Su C-Y, Mao F-Y, Lv Y-P, Teng Y-S, et al. Interleukin 6 induces M2 macrophage differentiation by stat3 activation that correlates with gastric cancer progression. Cancer Immunology Immunotherapy: CII. (2017) 66:1597–608. doi: 10.1007/s00262-017-2052-5
80. Haspeslagh E, Debeuf N, Hammad H, Lambrecht BN. Murine models of allergic asthma. Inflammation. (2017) 1559:121–36. doi: 10.1007/978-1-4939-6786-5_10
81. Sun J-B, Holmgren J, Larena M, Terrinoni M, Fang Y, Bresnick AR, et al. Deficiency in calcium-binding protein S100a4 impairs the adjuvant action of cholera toxin. Front Immunol. (2017) 8:1119. doi: 10.3389/fimmu.2017.01119
82. Sen Chaudhuri A, Yeh YW, Zewdie O, Li NS, Sun JB, Jin T, et al. S100a4 exerts robust mucosal adjuvant activity for co-administered antigens in mice. Mucosal Immunol. (2022) 15:1028–39. doi: 10.1038/s41385-022-00535-6
83. Abil OZ, Liu S, Yeh Y-W, Wu Y, Chaudhuri AS, Li NS, et al. A mucosal vaccine formulation against tuberculosis by exploiting the adjuvant activity of S100a4—a damage-associated molecular pattern molecule. Vaccine. (2024) 42. doi: 10.1016/j.vaccine.2024.07.052
84. Lotfi R, Wiegmann DS, Asseck L, Erle A, Yildiz T, Jahrsdörfer B, et al. Necrosis-associated factors (Damps) including S100a4 used to pulse dendritic cells (Dcs) induce regulatory T cells. J Cell Sci Ther. (2012) 3. doi: 10.4172/2157-7013.1000134
85. Boomershine CS, Chamberlain A, Kendall P, Afshar-Sharif AR, Huang H, Washington MK, et al. Autoimmune pancreatitis results from loss of tgfbeta signalling in S100a4-positive dendritic cells. Gut. (2009) 58:1267–74. doi: 10.1136/gut.2008.170779
86. Weatherly K, Bettonville M, Torres D, Kohler A, Goriely S, Braun MY. Functional profile of S100a4-deficient T cells. Immunity Inflammation Dis. (2015) 3:431–44. doi: 10.1002/iid3.85
87. Brisslert M, Bian L, Svensson MND, Santos RF, Jonsson I-M, Barsukov I, et al. S100a4 regulates the src-tyrosine kinase dependent differentiation of th17 cells in rheumatoid arthritis. Biochim Biophys Acta. (2014) 1842:2049–59. doi: 10.1016/j.bbadis.2014.07.003
88. Wherry EJ, Ha S-J, Kaech SM, Haining WN, Sarkar S, Kalia V, et al. Molecular signature of cd8+ T cell exhaustion during chronic viral infection. Immunity. (2007) 27:670–84. doi: 10.1016/j.immuni.2007.09.006
89. Haining WN, Ebert BL, Subrmanian A, Wherry EJ, Eichbaum Q, Evans JW, et al. Identification of an evolutionarily conserved transcriptional signature of cd8 memory differentiation that is shared by T and B cells. J Immunol (Baltimore Md: 1950). (2008) 181:1859–68. doi: 10.4049/jimmunol.181.3.1859
90. Grum-Schwensen B, Klingelhöfer J, Beck M, Bonefeld CM, Hamerlik P, Guldberg P, et al. S100a4-neutralizing antibody suppresses spontaneous tumor progression, pre-metastatic niche formation and alters T-cell polarization balance. BMC Cancer. (2015) 15:44. doi: 10.1186/s12885-015-1034-2
91. Grum-Schwensen B, Klingelhöfer J, Grigorian M, Almholt K, Nielsen BS, Lukanidin E, et al. Lung metastasis fails in mmtv-pymt oncomice lacking S100a4 due to a T-cell deficiency in primary tumors. Cancer Res. (2010) 70:936–47. doi: 10.1158/0008-5472.Can-09-3220
92. Jäger A, Dardalhon V, Sobel RA, Bettelli E, Kuchroo VK. Th1, th17 and th9 effector cells induce experimental autoimmune encephalomyelitis with different pathological phenotypes. J Immunol (Baltimore Md: 1950). (2009) 183:7169–77. doi: 10.4049/jimmunol.0901906
93. Jingjing H, Tongqian W, Shirong Y, Lan M, Jing L, Shihui M, et al. S100a4 promotes experimental autoimmune encephalomyelitis by impacting microglial inflammation through tlr4/nf-κb signaling pathway. Int Immunopharmacol. (2024) 142. doi: 10.1016/j.intimp.2024.112849
94. Turvey ME, Koudelka T, Comerford I, Greer JM, Carroll W, Bernard CC, et al. Quantitative proteome profiling of cns-infiltrating autoreactive cd4+ Cells reveals selective changes during experimental autoimmune encephalomyelitis. J Proteome Res. (2014) 13:3655–70. doi: 10.1021/pr500158r
95. Fissolo N, Matute-Blanch C, Osman M, Costa C, Pinteac R, Miró B, et al. Csf serpina3 levels are elevated in patients with progressive ms. Neurol Neuroimmunology Neuroinflamm. (2021) 8. doi: 10.1212/NXI.0000000000000941
96. D’Ambrosi N, Milani M, Apolloni S. S100a4 in the physiology and pathology of the central and peripheral nervous system. Cells. (2021) 10. doi: 10.3390/cells10040798
97. Eisenbacher JL, Schrezenmeier H, Jahrsdörfer B, Kaltenmeier C, Rojewski MT, Yildiz T, et al. S100a4 and uric acid promote mesenchymal stromal cell induction of il-10+/ido+ Lymphocytes. J Immunol. (2014) 192:6102–10. doi: 10.4049/jimmunol.1303144
98. Patras L, Shaashua L, Matei I, Lyden D. Immune determinants of the pre-metastatic niche. Cancer Cell. (2023) 41:546–72. doi: 10.1016/j.ccell.2023.02.018
99. DeNardo DG, Barreto JB, Andreu P, Vasquez L, Tawfik D, Kolhatkar N, et al. Cd4+ T cells regulate pulmonary metastasis of mammary carcinomas by enhancing protumor properties of macrophages. Cancer Cell. (2009) 16:91–102. doi: 10.1016/j.ccr.2009.06.018
100. Viguier M, Lemaître F, Verola O, Cho M-S, Gorochov G, Dubertret L, et al. Foxp3 expressing cd4+Cd25high regulatory T cells are overrepresented in human metastatic melanoma lymph nodes and inhibit the function of infiltrating T cells. J Immunol. (2004) 173:1444–53. doi: 10.4049/jimmunol.173.2.1444
101. Olkhanud PB, Damdinsuren B, Bodogai M, Gress RE, Sen R, Wejksza K, et al. Tumor-evoked regulatory B cells promote breast cancer metastasis by converting resting cd4+ T cells to T-regulatory cells. Cancer Res. (2011) 71:3505–15. doi: 10.1158/0008-5472.CAN-10-4316
102. Grum-Schwensen B, Klingelhofer Jr, Berg CH, El-Naaman C, Grigorian M, Lukanidin E, et al. Suppression of tumor development and metastasis formation in mice lacking the S100a4(Mts1) gene. Cancer Res. (2005) 65:3772–80. doi: 10.1158/0008-5472.CAN-04-4510
103. Ioannis K, Eralda S, Egal ESA, Beswick E. G-csf in tumors: aggressiveness, tumor microenvironment and immune cell regulation. Cytokine. (2021) 142. doi: 10.1016/j.cyto.2021.155479
104. Gong Z, Li Q, Shi J, Li P, Hua L, Shultz LD, et al. Immunosuppressive reprogramming of neutrophils by lung mesenchymal cells promotes breast cancer metastasis. Sci Immunol. (2023) 8. doi: 10.1126/sciimmunol.add5204
105. Coffelt SB, Kersten K, Doornebal CW, Weiden J, Vrijland K, Hau C-S, et al. Il17-producing γδ T cells and neutrophils conspire to promote breast cancer metastasis. Nature. (2015) 522:345–8. doi: 10.1038/nature14282
106. Li P, Lu M, Shi J, Hua L, Gong Z, Li Q, et al. Dual roles of neutrophils in metastatic colonization are governed by the host nk cell status. Nat Commun. (2020) 11. doi: 10.1038/s41467-020-18125-0
107. Kowanetz M, Wu X, Lee J, Tan M, Hagenbeek T, Qu X, et al. Granulocyte-colony stimulating factor promotes lung metastasis through mobilization of ly6g+Ly6c+ Granulocytes. Proc Natl Acad Sci USA. (2010) 107:21248–55. doi: 10.1073/pnas.1015855107
108. Leu J-S, Deng H, Tran HN, Wei X, Hashiomoto C, Leonard F, et al. Combined inhibition of S100a4 and tigit suppresses late-stage breast cancer metastasis to the lung by activating T and nk cells. bioRxiv. (2024). doi: 10.1101/2024.11.24.624743
109. Locksley RM. Asthma and allergic inflammation. Cell. (2010) 140:777–83. doi: 10.1016/j.cell.2010.03.004
110. Niu Q, Cai B, Huang Z-c, Shi Y-y, Wang L-l. Disturbed th17/treg balance in patients with rheumatoid arthritis. Rheumatol Int. (2012) 32:2731–36. doi: 10.1007/s00296-011-1984-x
111. Wit Jd, Souwer Y, Beelen A, Groot Rd, Muller FJM, Bos HK, et al. Cd5 costimulation induces stable th17 development by promoting il-23r expression and sustained stat3 activation. Blood. (2011) 118:6107–14. doi: 10.1182/blood-2011-05-352682
112. Bhandoola A, Bosselut R, Yu Q, Cowan ML, Feigenbaum L, Love PE, et al. Cd5-mediated inhibition of tcr signaling during intrathymic selection and development does not require the cd5 extracellular domain. Eur J Immunol. (2002) 32:1811–7. doi: 10.1002/1521-4141(200206)32:6<1811::AID-IMMU1811>3.0.CO;2-G
113. Patel RP, Ghilardi G, Zhang Y, Chiang Y-H, Xie W, Guruprasad P, et al. Cd5 deletion enhances the antitumor activity of adoptive T cell therapies. Sci Immunol. (2024) 9. doi: 10.1126/sciimmunol.adn6509
114. Forst B, Hansen MT, Klingelhöfer J, Møller HD, Nielsen GH, Grum-Schwensen B, et al. Metastasis-inducing S100a4 and rantes cooperate in promoting tumor progression in mice. PloS One. (2010) 5:e10374. doi: 10.1371/journal.pone.0010374
115. Li A, Zhu L, Lei N, Wan J, Duan X, Liu S, et al. S100a4-dependent glycolysis promotes lymphatic vessel sprouting in tumor. Angiogenesis. (2022) 26:19–36. doi: 10.1007/s10456-022-09845-6
116. Semov A, Moreno MJ, Onichtchenko A, Abulrob A, Ball M, Ekiel I, et al. Metastasis-associated protein S100a4 induces angiogenesis through interaction with annexin ii and accelerated plasmin formation. J Biol Chem. (2005) 280:20833–41. doi: 10.1074/jbc.M412653200
117. Ganaie AA, Mansini AP, Hussain T, Rao A, Siddique HR, Shabaneh A, et al. Anti-S100a4 antibody therapy is efficient in treating aggressive prostate cancer and reversing immunosuppression: serum and biopsy S100a4 as a clinical predictor. Mol Cancer Ther. (2020) 19:2598–611. doi: 10.1158/1535-7163.Mct-20-0410
118. Stein U, Burock S, Herrmann P, Wendler I, Niederstrasser M, Wernecke K-D, et al. Diagnostic and prognostic value of metastasis inducer S100a4 transcripts in plasma of colon, rectal, and gastric cancer patients. J Mol Diagnostics. (2011) 13:189–98. doi: 10.1016/j.jmoldx.2010.10.002
119. Piao Y, Liang J, Holmes L, Henry V, Sulman E, de Groot JF. Acquired resistance to anti-vegf therapy in glioblastoma is associated with a mesenchymal transition. Clin Cancer Res. (2013) 19:4392–403. doi: 10.1158/1078-0432.CCR-12-1557
120. Jiao J, González Á, Stevenson HL, Gagea M, Sugimoto H, Kalluri R, et al. Depletion of S100a4+ Stromal cells does not prevent hcc development but reduces the stem cell-like phenotype of the tumors. Exp Mol Med. (2018) 50. doi: 10.1038/emm.2017.175
121. Klingelhöfer J, Grum-Schwensen B, Beck MK, Knudsen RS, Grigorian M, Lukanidin E, et al. Anti-S100a4 antibody suppresses metastasis formation by blocking stroma cell invasion. Neoplasia. (2012) 14:1260–8. doi: 10.1593/neo.121554
122. Denton CP, Xu S, Zhang F, Maclean RH, Clark KEN, Borchert S, et al. Clinical and pathogenic significance of S100a4 overexpression in systemic sclerosis. Ann Rheumatic Dis. (2023) 82:1205–17. doi: 10.1136/ard-2023-223862
123. Bhat K, Saki M, Vlashi E, Cheng F, Duhachek-Muggy S, Alli C, et al. The dopamine receptor antagonist trifluoperazine prevents phenotype conversion and improves survival in mouse models of glioblastoma. Proc Natl Acad Sci USA. (2020) 117:11085–96. doi: 10.1073/pnas.1920154117
124. Kortüm B, Radhakrishnan H, Zincke F, Sachse C, Burock S, Keilholz U, et al. Combinatorial treatment with statins and niclosamide prevents crc dissemination by unhinging the macc1-β-catenin-S100a4 axis of metastasis. Oncogene. (2022) 41:4446–58. doi: 10.1038/s41388-022-02407-6
125. Sack U, Walther W, Scudiero D, Selby M, Kobelt D, Lemm M, et al. Novel effect of antihelminthic niclosamide on S100a4-mediated metastatic progression in colon cancer. JNCI: J Natl Cancer Institute. (2011) 103:1018–36. doi: 10.1093/jnci/djr190
126. Wen J, Jiao B, Tran M, Wang Y, Wen J, Jiao B, et al. Pharmacological inhibition of S100a4 attenuates fibroblast activation and renal fibrosis. Cells. (2022) 11:2762. doi: 10.3390/cells11172762
127. Stewart RL, Carpenter BL, West DS, Knifley T, Liu L, Wang C, et al. S100a4 drives non-small cell lung cancer invasion, associates with poor prognosis, and is effectively targeted by the fda-approved anti-helminthic agent niclosamide. Oncotarget. (2016) 7:34630–42. doi: 10.18632/oncotarget.8969
128. Serrano A, Apolloni S, Rossi S, Lattante S, Sabatelli M, Peric M, et al. The S100a4 transcriptional inhibitor niclosamide reduces pro-inflammatory and migratory phenotypes of microglia: implications for amyotrophic lateral sclerosis. Cells. (2019) 8. doi: 10.3390/cells8101261
129. Milani M, Mammarella E, Rossi S, Miele C, Lattante S, Sabatelli M, et al. Targeting S100a4 with niclosamide attenuates inflammatory and profibrotic pathways in models of amyotrophic lateral sclerosis. J Neuroinflamm. (2021) 18. doi: 10.1186/s12974-021-02184-1
130. Cabrita I, Benedetto R, Schreiber R, Kunzelmann K. Niclosamide repurposed for the treatment of inflammatory airway disease. JCI Insight. (2019) 4. doi: 10.1172/jci.insight.128414
131. Liang L, Huang M, Xiao Y, Zen S, Lao M, Zou Y, et al. Inhibitory effects of niclosamide on inflammation and migration of fibroblast-like synoviocytes from patients with rheumatoid arthritis. Inflammation Res. (2015) 64:225–33. doi: 10.1007/s00011-015-0801-5
132. Jiang H, Li AM, Ye J. Frontiers | the magic bullet: niclosamide. Front Oncol. (2022) 12:1004978. doi: 10.3389/fonc.2022.1004978
133. Garrett SC, Hodgson L, Rybin A, Toutchkine A, Hahn KM, Lawrence DS, et al. A biosensor of S100a4 metastasis factor activation: inhibitor screening and cellular activation dynamics. Biochemistry. (2008) 47:986–96. doi: 10.1021/bi7021624
134. Kang S, Hong J, Lee JM, Moon HE, Jeon B, Choi J, et al. Trifluoperazine, a well-known antipsychotic, inhibits glioblastoma invasion by binding to calmodulin and disinhibiting calcium release channel ip3r. Mol Cancer Ther. (2017) 16:217–27. doi: 10.1158/1535-7163.MCT-16-0169-T
135. Fancy RM, Kim H, Napier T, Buchsbaum DJ, Zinn KR, Song Y. Calmodulin antagonist enhances dr5-mediated apoptotic signaling in tra-8 resistant triple negative breast cancer cells. J Cell Biochem. (2018) 119:6216–30. doi: 10.1002/jcb.26848
Keywords: S100A4, FSP-1, immune suppression, cancer, fibrosis, chronic inflammation, macrophages, T cells
Citation: Wong T, Kang R and Yun K (2025) The multi-faceted immune modulatory role of S100A4 in cancer and chronic inflammatory disease. Front. Immunol. 16:1525567. doi: 10.3389/fimmu.2025.1525567
Received: 09 November 2024; Accepted: 10 February 2025;
Published: 26 February 2025.
Edited by:
Maurizio Chiriva-Internati, University of Texas MD Anderson Cancer Center, United StatesReviewed by:
Zou Xiang, Hong Kong Polytechnic University, Hong Kong SAR, ChinaRicardo Pujol Borrell, Autonomous University of Barcelona, Spain
Copyright © 2025 Wong, Kang and Yun. This is an open-access article distributed under the terms of the Creative Commons Attribution License (CC BY). The use, distribution or reproduction in other forums is permitted, provided the original author(s) and the copyright owner(s) are credited and that the original publication in this journal is cited, in accordance with accepted academic practice. No use, distribution or reproduction is permitted which does not comply with these terms.
*Correspondence: Kyuson Yun, a3l1bkBob3VzdG9ubWV0aG9kaXN0Lm9yZw==