- 1Department of Laboratory Medicine, The First Affiliated Hospital of Nanjing Medical University, Nanjing, China
- 2Branch of National Clinical Research Center for Laboratory Medicine, Nanjing, China
- 3Department of Breast and Thyroid Surgery, Huai’an First People’s Hospital, Nanjing Medical University, Huai’an, China
- 4Health Management Center, The First Affiliated Hospital with Nanjing Medical University, Nanjing, China
- 5Department of Breast Surgery, Women’s Hospital of Nanjing Medical University (Nanjing Women and Children’s Healthcare Hospital), Nanjing, China
Background: Mastitis is an inflammatory condition of the mammary gland, commonly observed in lactating and non-puerperal women, posing significant health and economic challenges. Lipopolysaccharide (LPS), a component of the outer membrane of Gram-negative bacteria, is a major inducer of mastitis. Ammonia, a key molecule in nitrogen metabolism, has been implicated in inflammatory pathways, yet its specific role in mastitis remains unclear. This study aims to investigate the mechanism by which ammonia influences the development of mastitis, particularly its effects on T cell activity and inflammatory factor expression.
Methods: qRT-PCR and ELISA were performed to measure the levels of IL-6, TNF, and IL-1β in the breast tissue of mice with LPS-induced mastitis, with or without ammonia treatment. HE staining was used to evaluate the degree of inflammation in the mammary tissue. FACS analysis was employed to assess the percentage, viability, and proliferation of immune cells in the breast tissue. CRISPR-Cas9 technology was used to knockout the SLC4A11 gene in T cells.
Results: Ammonia treatment significantly alleviated LPS-induced mastitis by reducing inflammation and inflammatory factor levels. It also decreased the percentage of CD4+ and CD8+ T cells, inhibited T cell viability and proliferation, and reduced pro-inflammatory cytokine expression (TNF and IFN-γ). Knockdown of the ammonia transporter Slc4a11 in T cells exacerbated mastitis, suggesting that Slc4a11 regulates T cell activity and inflammation during the progression of mastitis.
Conclusion: In summary, these findings highlight the critical role of ammonia and its transporter Slc4a11 in LPS-induced mastitis, providing potential therapeutic targets for future interventions.
1 Introduction
Mastitis is a prevalent disease characterized by inflammation and damage in mammary glands, imposing substantial economic burdens on the health of women (1). The primary causative factor of mastitis is bacterial infection, with LPS from Gram-negative bacteria such as Escherichia coli playing a pivotal role in initiating the inflammatory response (2). LPS, acting as a potent immune stimulant, triggers a cascade of immune responses when it interacts with toll-like receptor 4 (TLR4) on mammary epithelial cells and immune cells (3). This interaction leads to the activation of nuclear factor kappa B (NF-κB), resulting in the production of pro-inflammatory cytokines such as tumor necrosis factor-alpha (TNF-α) and interleukin-1 beta (IL-1β) (4). The ensuing inflammation causes tissue damage and can lead to a compromised blood-milk barrier, further exacerbating the condition.
Ammonia, a highly reactive nitrogenous compound, is generated through the catabolism of proteins and other nitrogen-containing substances (5). While it is well-known for its ability to exacerbate oxidative stress by promoting the production of reactive oxygen species (ROS) and compromising antioxidant defenses, ammonia may also play regulatory roles in inflammation (6). For instance, low concentrations of ammonia have been shown to enhance the bactericidal activity of neutrophils, which serve as a crucial first line of defense against bacterial infections (7). Moreover, ammonia metabolism may be implicated in the resolution of inflammation, as ammonia can act as a substrate for the production of nitric oxide (NO), a molecule with dual roles in inflammation—exhibiting both pro-inflammatory and anti-inflammatory properties depending on its concentration and context (8).
In terms of immune cell function, ammonia has been shown to influence the activity of various immune cells, such as macrophages, dendritic cells, and T cells (9). For instance, it can impair macrophage phagocytosis and cytokine production, alter dendritic cell maturation and antigen presentation, and suppress T cell proliferation and cytokine secretion (10).
While the direct link between ammonia and mastitis has not been extensively studied, it is plausible to consider the potential impact of ammonia metabolism in the context of mastitis. By targeting the ammonia metabolism pathway, it could be possible to slow down the growth of inflammatory or pathogenic cells within the breast tissue. For instance, inhibiting glutamate dehydrogenase (GDH) activity has been shown to alleviate tumor growth in mouse models of breast cancer, suggesting that similar approaches could be explored for mastitis (11).
In this study, we focus on the role of ammonia in the development of mastitis. By studying the effect of ammonia on the mouse model of mastitis, the mechanism of ammonia in the development of mastitis can be revealed. Our study uses a variety of research methods, including cell separation, flow cytometry analysis, ELISA, qRT-PCR, and CRISPR-Cas9. We showed that ammonia could inhibit LPS-induced mastitis, reduce T cell activity, and decrease the expression of inflammatory factors.
2 Methods and materials
2.1 Reagents
The list below details the flow cytometry antibodies that were employed (all sourced from BD Biosciences, unless specified otherwise): PE-conjugated antibodies to mouse TNF (MP6-XT22, 554419), Alexa Fluor 700-conjugated antibodies to mouse CD4 (RM4-5, 557956), PerCP-CyTM5.5-conjugated antibodies to mouse CD8α(53-6.7, 551162), FITC-conjugated antibodies to mouse CD45(30-F11, 553079), Fixable Viability Stain 780 (565388), Alexa Fluor 647-conjugated antibodies to mouse IFN-γ(XMG1.2, 557735), DNasel (Sigma-Aldrich, DN25), Collagenase,type IV(Sigma-Aldrich, C5138), RBC lysis buffer(beyotime, C3702), NH4Cl(Sigma-Aldrich, 213330).
2.2 Mice model
The 6 weeks-old wild-type C57BL/6 mice were purchased from GemPharmatech Co Ltd (Nanjing, China). The mice were housed in a controlled barrier environment, and all experimental procedures were executed in strict compliance with the ethical standards set by the Ethical Review Committee for Laboratory Animal Welfare at Nanjing Medical University. Throughout the study, the mice were maintained under a 12h light/dark cycle at a temperature range of 22-24°C, with ad libitum access to food and water.
For the induction of mastitis, Eight-week-old C57BL/6 mice were injected LPS directly into the fourth pair of mammary gland tissues. After injection, the mice were divided into two treatment groups: a control group receiving a vehicle (Vehicle) and an experimental group treated with ammonium chloride (NH4Cl, referred to as Ammonia). At 72 hours following the administration of anesthesia with isoflurane, the mice underwent retro-orbital blood sampling (100ul) and sacrificed for mastitis tissues under sterile operating conditions for subsequent processing.
2.3 Magnetic-activated cell sorting cell separation
For isolation of mouse T cells from spleen, CD3+ cells were enriched from spleens by using a CD3 MicroBead Kit, according to the manufacturer’s instructions (Miltenyi Biotec). Enriched CD3+ cells were stained with CD3 antibodies and detected by the flow cytometer. The purity of T cells was > 95%.
2.4 H&E staining
Mammary gland samples from mice were submerged in a 10% phosphate-buffered formalin solution for overnight fixation. Subsequently, the samples underwent a dehydration process prior to being embedded in paraffin at 58°C for an extended period. Once solidified, the paraffin-embedded tissues were sectioned into 5–10 μm thick slices. These sections were rehydrated through a series of ethanol washes and stained with a hematoxylin solution composed of 0.1% hematoxylin, 5% potassium sulfate (Kal [SO4]2), and 0.02% potassium iodate (KIO3). Following the primary staining, the sections were counterstained with a 1% eosin solution. Dehydration and clearing were performed using xylene, and the slides were mounted with a neutral gum medium. Imaging of the H&E-stained sections was conducted using a Motic slide scanner (Motic, EasyScan NFC, Xiamen).
2.5 Enzyme-linked immunosorbent assay
C57BL/6 mice at eight weeks of age received an in situ injection of LPS into the fourth pair of mammary gland tissues. After 24 hours, the mice were anesthetized using isoflurane, and 100 microliters of blood were collected via the retro-orbital route. The blood samples were then centrifuged at 3000 revolutions per minute (rpm) for 5 minutes to separate plasma. The concentrations of IL-6, TNF-α, and IL-1β in the plasma were quantified using specific ELISA kits (R & D systems), following the manufacturer’s guidelines.
2.6 Flow cytometry analysis
To isolate immune cells associated with inflammation, the mice were humanely euthanized, and their mammary glands were extracted and minced into small pieces. These pieces were enzymatically digested in RPMI 1640 medium (Gibco) containing 1 mg/ml collagenase type IV and 0.1 mg/ml DNase I for 2 hours in a 37°C shaking incubator at 200 revolutions per minute (r.p.m.). The resulting cell suspension was centrifuged over a Percoll density gradient (GE Healthcare) to separate mononuclear cells at the 40% to 80% interface. These cells were further processed by filtration through a 70 μm cell strainer. Spleens were also processed by crushing through a 40 μm cell strainer, followed by red blood cell lysis using an RBC lysis buffer. For intracellular cytokine staining, cells were stimulated with 50 ng/ml phorbol 12-myristate 13-acetate (PMA, Sigma-Aldrich), 1 μg/ml ionomycin (Sigma-Aldrich), and 2.5 μg/ml monensin at 37°C in RPMI 1640 medium supplemented with 10% fetal bovine serum (FBS) for 4 hours. Cells were then labeled with surface antibodies, fixed with a Fixation/Permeabilization buffer for 40 minutes, and subsequently stained with intracellular antibodies.
2.7 Quantitative real-time polymerase chain reaction
Total RNA was extracted from mouse mammary tissues using TRIzol reagent (Invitrogen) and reverse transcribed into complementary DNA (cDNA) using HiScript II Q Select RT SuperMix for qPCR (Vazyme, Cat: R232-01). qRT-PCR was performed on the ABI 7500 Thermocycler (Applied Biosystems) in 20 μl reaction volumes, which included the cDNA, specific primers, and AceQ qPCR SYBR Green Master Mix (Vazyme, Cat: Q121-02).
2.8 CRISPR-Cas 9
Cells were seeded at a density of 105 cells/well in a 24-well plate one day prior to transfection. Two 1.5 mL EP centrifuge tubes, labeled as tube A and tube B, were prepared. In tube A, 25 µL of Opti-MEM (Cat# 11095080, Thermo Fisher), 36 pmol of Cas9 protein (Cat# Z03469, GenScript), and 36 pmol of EasyEditsgRNA were combined and incubated for 15 min at room temperature. Meanwhile, tube B received 25 µL of Opti-MEM and 1.5 µL of Lipofectamine 3000 (Cat# L3000015, Thermo Fisher), mixed gently, and incubated at room temperature for 10 min. After incubation, the contents of tubes A and B were gently combined and incubated at room temperature for an additional 10 min. The resulting transfection mixture (50 µL per well) was added to the cells in the 24-well plate and incubated at 37°C. Cells were harvested 72 h post-transfection for subsequent processing.
2.9 Adoptive transfer
T cells were purified from spleen using T cell isolation kit (Miltenyi Biotec, Cat# 130-094-973). Then we employed CRISPR-Cas9 technology to specifically knockout the SLC4A11 gene in T cells. The 1*106 gRNA-Ctrl and gRNA-Slc4a11 T cells in the presence of anti-CD3 Ab (2 μg/mL) and anti-CD28 Ab (5 μg/mL) were respectively intravenous injected into Rag1-/- mice, followed by construction of mastitis model.
2.10 Activation of T cell
The 1×105 gRNA-Ctrl and gRNA-Slc4a11 T cells were incubated in 96-well plate. T cells were activated with anti-CD3 Ab (2 μg/mL) and anti-CD28 Ab (5 μg/mL) in RPMI 1640 included murine IL-2(5ng/ml).
2.11 Statistical analysis
The data were analyzed by GraphPad Prism 10.0 software and are presented as the mean ± SEM. The statistics were analyzed by using an unpaired t test for two groups and one-way ANOVA for multiple groups. P values were provided as *, P < 0.05; **, P < 0.01; and ***, P < 0.001.
3 Results
3.1 Ammonia inhibits LPS-induced mastitis
To investigate the role of ammonia in the development of mastitis, we first established a mouse mastitis model by administering LPS (0.1 mg/ml). Histological examination via hematoxylin and eosin (HE) staining revealed that, compared to the control group, ammonia treatment significantly alleviated inflammation in the mammary glands of mice (Figure 1A). Further analysis using quantitative PCR (qPCR) demonstrated that the mRNA expression levels of key inflammatory factors, including TNF-α, IL-6, and IL-1β, were significantly reduced in the ammonia treatment group (Figure 1B). Consistent with these findings, enzyme-linked immunosorbent assay (ELISA) experiments showed that the concentrations of TNF-α, IL-6, and IL-1β were also markedly decreased in the ammonia treatment group (Figure 1C). Additionally, flow cytometry analysis revealed that the percentages of CD45-positive immune cells, as well as CD4-positive and CD8-positive T cells, were significantly lower in the ammonia treatment group (Figures 1D, E).
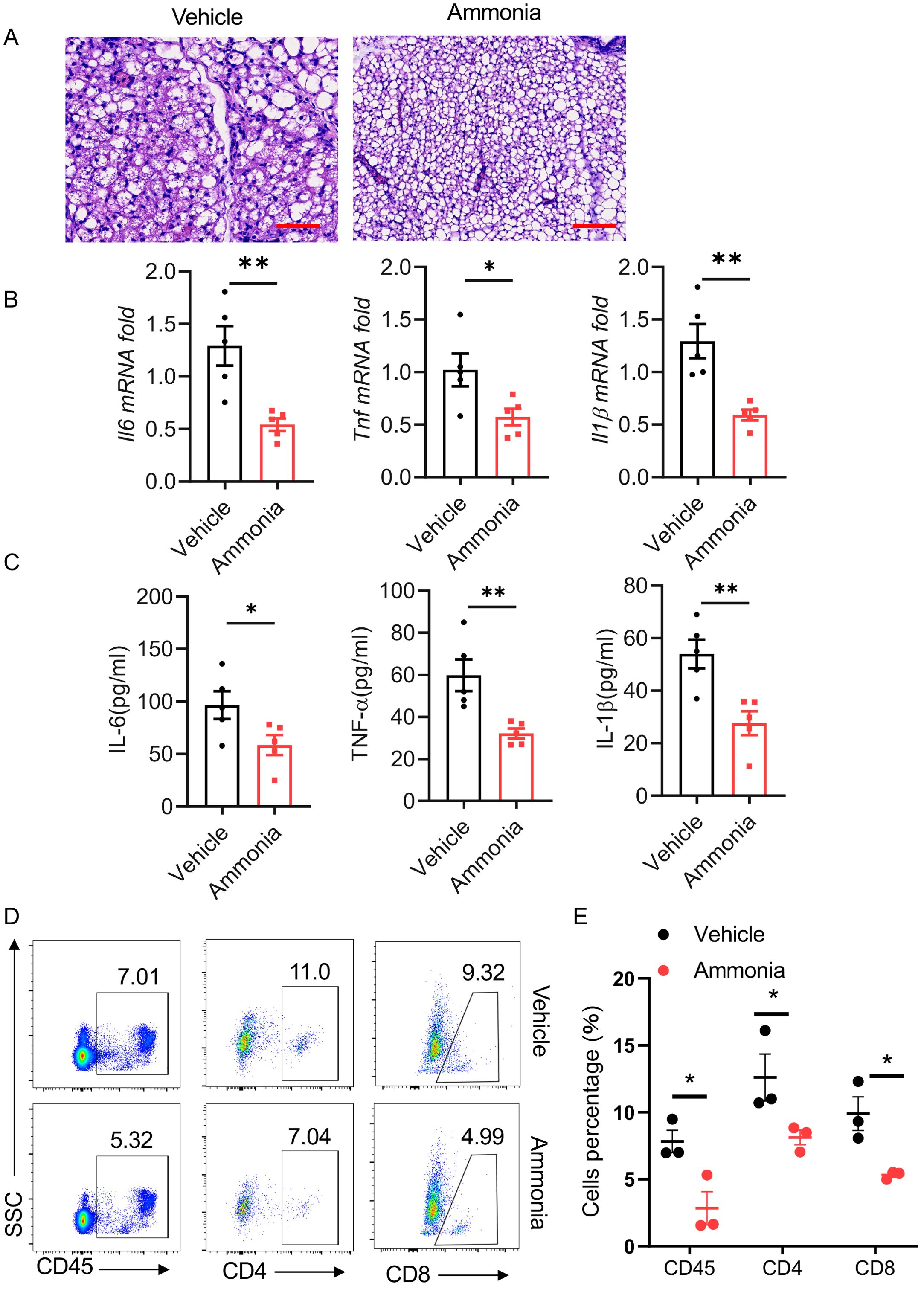
Figure 1. Ammonia inhibits LPS-induced mastitis. (A) Histological analysis of breast tissue stained with H&E. The left panel shows the vehicle control group, and the right panel represents the ammonia treatment group (Scale bar: 50 μm). (B) Quantitative analysis of mRNA expression levels of inflammatory cytokines (Il6, Tnf, Il1β) in breast tissue. (C) Concentrations of IL-6, TNF-α, and IL-1β in serums measured by ELISA. (D) Flow cytometric analysis of CD45+ immune cells, CD4+ T cells and CD8+ T cells in breast tissue. (E) The percentages of CD45+ immune cells, CD4+ T cells and CD8+ T cells in the control group and ammonia treatment group. Data are presented as mean ± SEM. *p < 0.05, **p < 0.01.
In summary, our findings demonstrate that ammonia treatment effectively mitigates LPS-induced inflammation and modulates the immune cell composition within breast tissue.
3.2 Ammonia reduces T cell viability in LPS-induced mastitis
To further elucidate the impact of ammonia on T cell activity in mastitis, we conducted flow cytometric analyses on mammary tissue samples from two distinct groups. The findings indicated that the percentage of T cell death was significantly elevated in the ammonia treatment group relative to the control group (Figures 2A, B). This observation implies a substantial dampening of T cell activity, which is indicative of an anti-inflammatory effect. We then extended our analysis to separately examine the activity of CD4-positive and CD8-positive T cells using flow cytometry. The results demonstrated that ammonia treatment led to a significant decrease in the proliferation of both CD4-positive and CD8-positive T cells (Figure 2C). This reduction in T cell proliferation was accompanied by alleviation of inflammation and exhibited a clear dose-dependent relationship (Figures 2D, E).
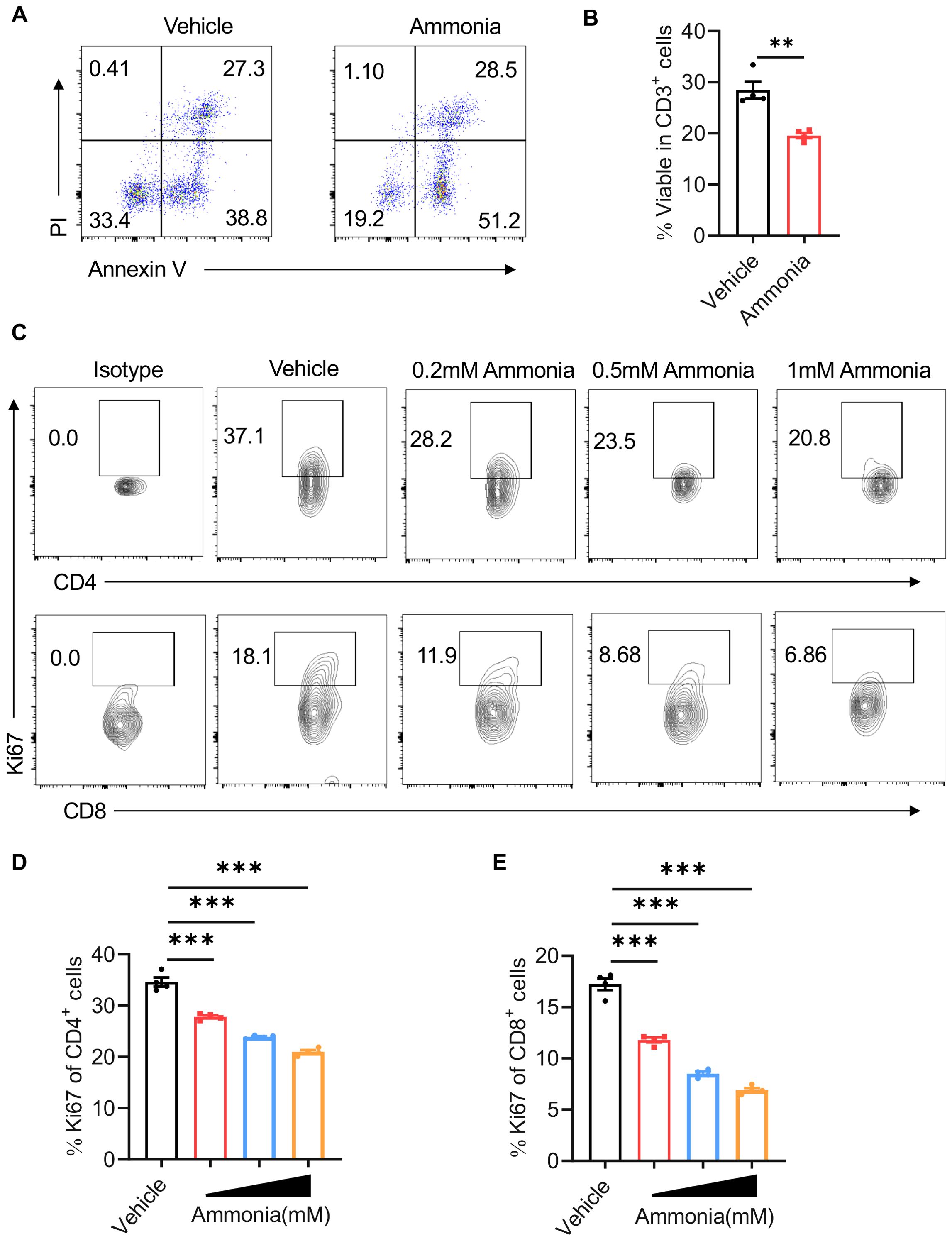
Figure 2. Ammonia reduces T cell viability in LPS-induced mastitis. (A) Flow cytometric analysis of PI+ Annexin V+ of CD3+ cells in breast tissue. (B) The percentages of viable CD3+ cells. (C) Flow cytometric analysis of CD4+ Ki67+ and CD8+ Ki67+ T cells at different concentrations of ammonia (0.2mM, 0.5mM, 1mM). (D) Quantification of Ki67+ cells within CD4+ T cells. (E) Quantification of Ki67+ cells within CD8+ T cells. Data are presented as mean ± SEM. **p < 0.01, ***<0.001.
Collectively, these findings indicate that ammonia treatment significantly diminishes the viability and proliferation of both CD4+ and CD8+ T cells in LPS-induced mastitis, thereby contributing to the observed anti-inflammatory effects.
3.3 Ammonia reduced LPS-induced mastitis through T cells
Recent studies have highlighted that the accumulation of ammonia is a critical mechanism underlying the impaired pro-inflammatory function of effector T cells (12). In our investigation into the role of T cells in the anti-inflammatory effects of ammonia on mastitis, we observed that, compared to the control group, the activity of CD4-positive T cells was significantly diminished in the ammonia-treated group. This was accompanied by marked reductions in the expression levels of key inflammatory markers, including TNF and IFNγ (Figures 3A-D). Similarly, ammonia treatment also led to a notable decline in the activity of CD8-positive T cells, with a corresponding decrease in the expression of their related inflammatory indicators (Figures 3E-H).
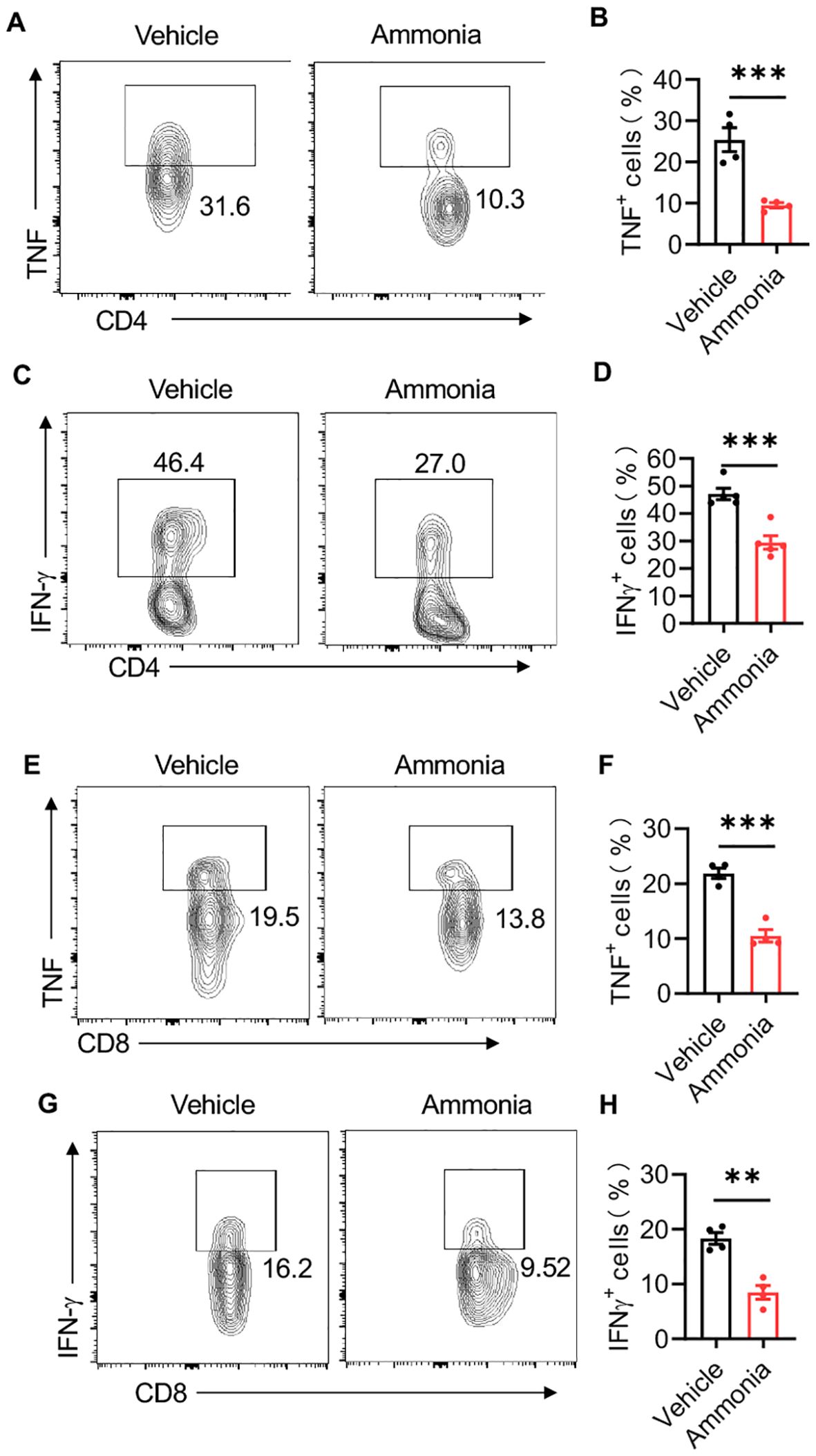
Figure 3. Ammonia reduces LPS-induced mastitis through T cells. (A, C) Flow cytometric analysis of TNF+ and IFNγ+ cells within CD4+ T cells in breast tissue. (B, D) The percentages of TNF+ and IFNγ+ cells within CD4+ T cells in the control group and ammonia treatment group. (E, G) Flow cytometric analysis of TNF+ and IFNγ+ cells within CD8+ T cells. (F, H) The percentages of TNF+ and IFNγ+ cells within CD8+ T cells in the control group and ammonia treatment group. Data are presented as mean ± SEM. **p < 0.01, ***<0.001.
Overall, the results demonstrate that ammonia treatment significantly inhibits the activation of CD4+ and CD8+ T cells, as shown by the decreased proportions of TNF+ and IFNγ+ cells. This highlights the potential of ammonia as an anti-inflammatory mediator in LPS-induced mastitis.
3.4 Downregulated the expression of Slc4a11 promotes LPS-induced mastitis
To elucidate the potential mechanisms underlying ammonia’s ability to suppress mastitis progression, we focused on the ammonia transport-related gene SLC4A11. We then validated this hypothesis through quantitative PCR (qPCR) analysis. The results revealed that the mRNA expression level of SLC4A11 was significantly upregulated in the ammonia treatment group compared to the control group (Figure 4A).
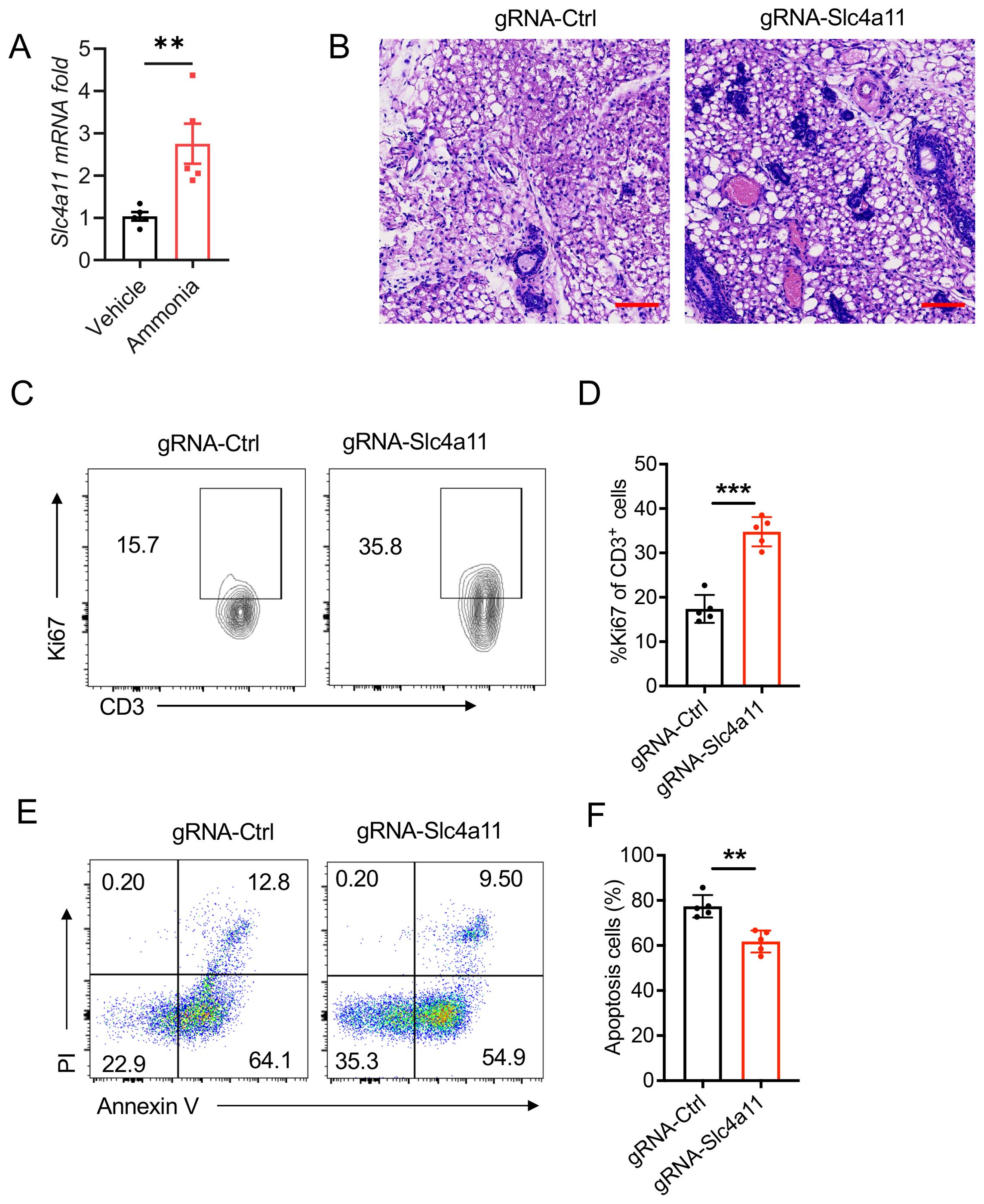
Figure 4. Downregulated the expression of Slc4a11 promotes LPS-induced mastitis. (A) Quantitative analysis of Slc4a11 mRNA expression levels in T cells. (B) Histological analysis of breast tissue stained with H&E (Scale bar: 50 μm). The left panel displays the control group treated with gRNA-Ctrl and the right panel shows the group treated with gRNA targeting Slc4a11 (gRNA-Slc4a11). (C, D) Flow cytometric analysis of CD3+ Ki67+ T cells in the gRNA-Slc4a11 group compared to the gRNA-Ctrl group. (E, F) The percentages of apoptosis cells in the gRNA-Slc4a11 group compared to the gRNA-Ctrl group. Data are presented as mean ± SEM. **p < 0.01, ***<0.001.
Subsequently, we employed CRISPR-Cas9 technology to specifically knockout the SLC4A11 gene in T cells. These modified T cells were then adoptively transferred into the mammary tissue. HE staining demonstrated that deletion of SLC4A11 in T cells led to a significant exacerbation of mastitis severity (Figure 4B). Furthermore, the knockout of the Slc4a11 gene leads to an increased proportion of T cells expressing Ki67 (Figures 4C, D) and increased percentages of living cells (Figures 4E, F) after the adoptive transfer.
3.5 Downregulated the expression of Slc4a11 promotes LPS-induced mastitis through T cells
To determine whether Slc4a11 regulates the progression of mastitis through modulation of T cells, we employed CRISPR-Cas9 technology to knock down Slc4a11 expression specifically in T cells. Compared to the control group, the knockdown of Slc4a11 expression resulted in a significant increase in the expression levels of key inflammatory markers, including TNF and IFNγ (Figures 5A-D). Similarly, in CD8-positive T cells, the expression levels of TNF and IFNγ were also markedly elevated following Slc4a11 knockdown (Figures 5E-H).
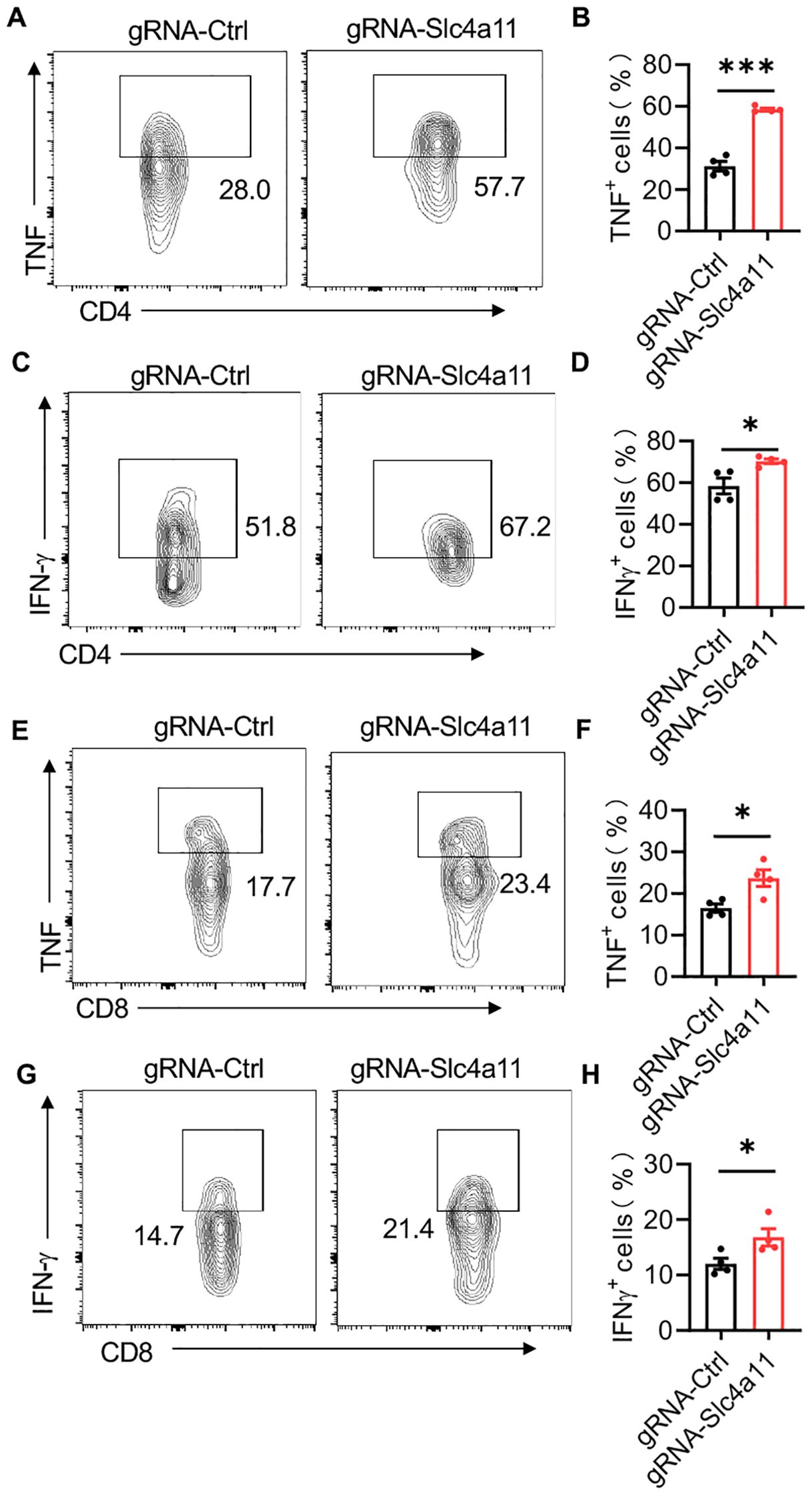
Figure 5. Slc4a11 Downregulation Enhances T Cell-Mediated Inflammation in LPS-Induced Mastitis. (A, C) Flow cytometric analysis of TNF+ and IFNγ+ cells within CD4+ T cells. (B, D) The percentages of TNF+ and IFNγ+ cells within CD4+ T cells in the gRNA-Slc4a11 group compared to the gRNA-Ctrl group. (E, G) Flow cytometric analysis of TNF+ and IFNγ+ cells within CD8+ T cells. (F, H) The percentages of TNF+ and IFNγ+ cells within CD8+ T cells in the gRNA-Slc4a11 group compared to the gRNA-Ctrl group. Data are presented as mean ± SEM. *p < 0.05, ***<0.001.
These findings suggest that downregulation of Slc4a11 enhances the activation of both CD4+ and CD8+ T cells, as evidenced by the increased proportions of TNF+ and IFNγ+ cells. This indicates that Slc4a11 plays a crucial role in modulating T cell responses and thereby influencing the inflammatory process in LPS-induced mastitis.
4 Discussion
Our study provides evidence that ammonia plays a significant role in modulating T cell activity and thereby influencing the progression of mastitis. This aligns with recent research showing that SLC4A11 mediates conductive H+(OH-) transport, which is stimulated by raising the extracellular pH (pHe); similarly, ammonia-induced whole cell currents were also stimulated by an increase in pHe (13–15). This suggests that SLC4A11 could be a key player in the modulation of T cell activity in mastitis.
SLC4A11, a member of the SLC4 family of transporters, is known for its role in transporting dicarboxylate and bicarbonate anions (16, 17). In the context of mastitis—an inflammatory condition of the mammary glands—SLC4A11 may contribute to the pathogenesis by influencing the transport of these ions, thereby affecting cellular pH and potentially modulating the inflammatory response. The precise mechanisms by which SLC4A11 operates in mastitis are not fully understood but likely involve multiple pathways. One possibility is that SLC4A11 helps maintain cellular homeostasis by regulating the transport of ammonia, a byproduct of protein metabolism that can be toxic at high concentrations (18). In conditions like mastitis, where inflammation is heightened, the role of SLC4A11 in managing ammonia levels could be particularly crucial.
Furthermore, SLC4A11 has been implicated in the regulation of cellular energy metabolism and may influence ATP production in mitochondria (19, 20). This function could be highly relevant in mastitis, as cellular energy metabolism is often altered during inflammation and may affect the ability of cells to respond to inflammatory stimuli (21). Additionally, SLC4A11 has been identified as a candidate gene associated with resistance to mastitis in dairy cows (22), suggesting that genetic variations in this gene could influence an animal’s susceptibility to the disease.
The modulation of ammonia levels and the targeting of Slc4a11 offer a potential therapeutic strategy for the treatment of mastitis. Recent studies suggest that periductal mastitis is an inflammatory disease related to bacterial infection and consequent immune responses, which supports the idea that targeting the immune response, such as T cell activity, could be beneficial (23–25). Our study adds to this body of work by demonstrating that ammonia, through its interaction with Slc4a11, can inhibit the activity of pro-inflammatory T cells, providing a means to dampen the inflammatory response and protect against tissue damage associated with mastitis.
While our study provides novel insights into the role of ammonia and Slc4a11 in mastitis, there are limitations that warrant further investigation. The controversy surrounding the exact mode of ammonia transport by SLC4A11 necessitates further research (20, 26, 27). Future studies should aim to validate our findings in clinical samples and investigate the detailed molecular mechanisms by which ammonia and Slc4A11 interact to regulate T cell activity and inflammation. Additionally, understanding the long-term effects of ammonia treatment on immune function and mastitis recurrence will be crucial for the development of safe and effective therapeutic strategies.
5 Conclusion
In conclusion, our study sheds light on the intricate relationship between ammonia, T cell activity, and mastitis progression. The findings suggest that targeting the ammonia-Slc4a11 axis may offer a promising approach for the management of mastitis, warranting further research into its therapeutic potential. By building on the recent literature, we can better understand the complex interactions at play and develop more targeted treatments for mastitis.
Data availability statement
The raw data supporting the conclusions of this article will be made available by the authors, without undue reservation.
Ethics statement
The animal study was approved by The Ethical Review Committee for Laboratory Animal Welfare at Nanjing Medical University. The study was conducted in accordance with the local legislation and institutional requirements.
Author contributions
LZ: Conceptualization, Formal analysis, Investigation, Writing – review & editing. YuW: Data curation, Formal analysis, Writing – original draft. ZL: Writing – original draft. PX: Resources, Software, Writing – original draft. YaW: Project administration, Writing – original draft. HG: Methodology, Writing – original draft. HY: Supervision, Validation, Writing – review & editing.
Funding
The author(s) declare that financial support was received for the research and/or publication of this article. This work was supported by the following grants: Jiangsu Province Maternal and Child Health Care Association Research Project (no. FYX202348 to Yuqing Wu; no. FYX202347 to Lei Zhu).
Conflict of interest
The authors declare that the research was conducted in the absence of any commercial or financial relationships that could be construed as a potential conflict of interest.
Generative AI statement
The author(s) declare that no Generative AI was used in the creation of this manuscript.
Publisher’s note
All claims expressed in this article are solely those of the authors and do not necessarily represent those of their affiliated organizations, or those of the publisher, the editors and the reviewers. Any product that may be evaluated in this article, or claim that may be made by its manufacturer, is not guaranteed or endorsed by the publisher.
References
1. Blackmon MM, Nguyen H, Mukherji P. Acute Mastitis. (2024) Treasure Island (FL): StatPearls Publishing.
2. Johnzon CF, Dahlberg J, Gustafson AM, Waern I, Moazzami AA, Ostensson K, et al. The effect of lipopolysaccharide-induced experimental bovine mastitis on clinical parameters, inflammatory markers, and the metabolome: A kinetic approach. Front Immunol. (2018) 9:1487. doi: 10.3389/fimmu.2018.01487
3. Kawai T, Ikegawa M, Ori D, Akira S. Decoding Toll-like receptors: Recent insights and perspectives in innate immunity. Immunity. (2024) 57:649–73. doi: 10.1016/j.immuni.2024.03.004
4. Liu T, Zhang L, Joo D, Sun SC. NF-kappaB signaling in inflammation. Signal Transduct Target Ther. (2017) 2:17023. doi: 10.1038/sigtrans.2017.23
5. Kurmi K, Haigis MC. Nitrogen metabolism in cancer and immunity. Trends Cell Biol. (2020) 30:408–24. doi: 10.1016/j.tcb.2020.02.005
6. Huang Y, Mo S, Jin Y, Zheng Z, Wang H, Wu S, et al. Ammonia-induced excess ROS causes impairment and apoptosis in porcine IPEC-J2 intestinal epithelial cells. Ecotoxicol Environ Saf. (2022) 243:114006. doi: 10.1016/j.ecoenv.2022.114006
7. Sultana S, Foti A, Dahl JU. Bacterial defense systems against the neutrophilic oxidant hypochlorous acid. Infect Immun. (2020) 88:e00964-19. doi: 10.1128/IAI.00964-19
8. Sharma JN, Al-Omran A, Parvathy SS. Role of nitric oxide in inflammatory diseases. Inflammopharmacology. (2007) 15:252–9. doi: 10.1007/s10787-007-0013-x
9. Liu Y, Zhang X, Wang W, Liu T, Ren J, Chen S, et al. Ammonia promotes the proliferation of bone marrow-derived mesenchymal stem cells by regulating the Akt/mTOR/S6k pathway. Bone Res. (2022) 10:57. doi: 10.1038/s41413-022-00215-y
10. Bell HN, Huber AK, Singhal R, Korimerla N, Rebernick RJ, Kumar R, et al. Microenvironmental ammonia enhances T cell exhaustion in colorectal cancer. Cell Metab. (2023) 35:134–49. doi: 10.1016/j.cmet.2022.11.013
11. Spinelli JB, Yoon H, Ringel AE, Jeanfavre S, Clish CB, Haigis MC. Metabolic recycling of ammonia via glutamate dehydrogenase supports breast cancer biomass. Science. (2017) 358:941–6. doi: 10.1126/science.aam9305
12. Zhang H, Liu J, Yuan W, Zhang Q, Luo X, Li Y, et al. Ammonia-induced lysosomal and mitochondrial damage causes cell death of effector CD8(+) T cells. Nat Cell Biol. (2024) 26:1892–902. doi: 10.1038/s41556-024-01503-x
13. Kao L, Azimov R, Shao XM, Abuladze N, Newman D, Zhekova H, et al. SLC4A11 function: evidence for H(+)(OH(-)) and NH(3)-H(+) transport. Am J Physiol Cell Physiol. (2020) 318:C392–405. doi: 10.1152/ajpcell.00425.2019
14. Ferreira TB, Carrondo MJ, Alves PM. Effect of ammonia production on intracellular pH: Consequent effect on adenovirus vector production. J Biotechnol. (2007) 129:433–8. doi: 10.1016/j.jbiotec.2007.01.010
15. Kelly T, Kafitz KW, Roderigo C, Rose CR. Ammonium-evoked alterations in intracellular sodium and pH reduce glial glutamate transport activity. Glia. (2009) 57:921–34. doi: 10.1002/glia.20817
16. Shei W, Liu J, Htoon HM, Aung T, Vithana EN. Differential expression of the Slc4 bicarbonate transporter family in murine corneal endothelium and cell culture. Mol Vis. (2013) 19:1096–106.
17. Vilas GL, Loganathan SK, Liu J, Riau AK, Young JD, Mehta JS, et al. Transmembrane water-flux through SLC4A11: a route defective in genetic corneal diseases. Hum Mol Genet. (2013) 22:4579–90. doi: 10.1093/hmg/ddt307
18. Pizzagalli MD, Bensimon A, Superti-Furga G. A guide to plasma membrane solute carrier proteins. FEBS J. (2021) 288:2784–835. doi: 10.1111/febs.15531
19. Choi M, Bonanno JA. Mitochondrial targeting of the ammonia-sensitive uncoupler SLC4A11 by the chaperone-mediated carrier pathway in corneal endothelium. Invest Ophthalmol Vis Sci. (2021) 62:4. doi: 10.1167/iovs.62.12.4
20. Zhang W, Ogando DG, Bonanno JA, Obukhov AG. Human SLC4A11 is a novel NH3/H+ Co-transporter. J Biol Chem. (2015) 290:16894–905. doi: 10.1074/jbc.M114.627455
21. Bronzo V, Lopreiato V, Riva F, Amadori M, Curone G, Addis MF, et al. The role of innate immune response and microbiome in resilience of dairy cattle to disease: the mastitis model. Anim (Basel). (2020) 10:1397. doi: 10.3390/ani10081397
22. Fang L, Hou Y, An J, Li B, Song M, Wang X, et al. Genome-wide transcriptional and post-transcriptional regulation of innate immune and defense responses of bovine mammary gland to Staphylococcus aureus. Front Cell Infect Microbiol. (2016) 6:193. doi: 10.3389/fcimb.2016.00193
23. Xing M, Zhang S, Zha X, Zhang J. Current understanding and management of plasma cell mastitis: can we benefit from what we know? Breast Care (Basel). (2022) 17:321–9. doi: 10.1159/000517572
24. Pan N, Xiu L, Xu Y, Bao X, Liang Y, Zhang H, et al. Mammary gammadelta T cells promote IL-17A-mediated immunity against Staphylococcus aureus-induced mastitis in a microbiota-dependent manner. iScience. (2023) 26:108453. doi: 10.1016/j.isci.2023.108453
25. Liu L, Zhou F, Wang P, Yu L, Ma Z, Li Y, et al. Periductal mastitis: an inflammatory disease related to bacterial infection and consequent immune responses? Mediators Inflammation. (2017) 2017:5309081. doi: 10.1155/2017/5309081
26. Ogando DG, Choi M, Shyam R, Li S, Bonanno JA. Ammonia sensitive SLC4A11 mitochondrial uncoupling reduces glutamine induced oxidative stress. Redox Biol. (2019) 26:101260. doi: 10.1016/j.redox.2019.101260
Keywords: ammonia, SLC4A11, mastitis, T cell, LPS
Citation: Wu Y, Li Z, Xi P, Wang Y, Guo H, Yin H and Zhu L (2025) The ammonia-Slc4a11 axis in T cells alleviates LPS-induced mastitis. Front. Immunol. 16:1537483. doi: 10.3389/fimmu.2025.1537483
Received: 30 November 2024; Accepted: 10 April 2025;
Published: 30 April 2025.
Edited by:
Xuefeng Wang, Soochow University, ChinaReviewed by:
Xingxing Zhu, Mayo Clinic, United StatesHua Chen, Affiliated Kunshan Hospital of Jiangsu University, China
Tarik Safak, Kastamonu University, Türkiye
Copyright © 2025 Wu, Li, Xi, Wang, Guo, Yin and Zhu. This is an open-access article distributed under the terms of the Creative Commons Attribution License (CC BY). The use, distribution or reproduction in other forums is permitted, provided the original author(s) and the copyright owner(s) are credited and that the original publication in this journal is cited, in accordance with accepted academic practice. No use, distribution or reproduction is permitted which does not comply with these terms.
*Correspondence: Lei Zhu, bGVpemh1MTEyMG5qbXVAMTI2LmNvbQ==; Hong Yin, NzcwNzc5NzdAcXEuY29t
†These authors have contributed equally to this work