- 1School of Traditional Chinese and Western Medicine, Gansu University of Chinese Medicine, Lanzhou, China
- 2Department of Pulmonary and Critical Care Medicine, Affiliated Hospital of North Sichuan Medical College, Nanchong, China
- 3Department of Thoracic Surgery, The First Hospital of Lanzhou University, Lanzhou, China
- 4Department of Thoracic Surgery, Shanghai Pulmonary Hospital, Shanghai, China
- 5Department of Thoracic Surgery and Minimally Invasive Thoracic Surgery Unit (UCTMI), Coruña University Hospital, A Coruña, Spain
- 6Shanghai Engineering Research Center of Lung Transplantation, Shanghai, China
Background: Ferroptosis, an iron-dependent form of regulated cell death, has garnered significant attention as a potential therapeutic target in oncology due to its unique mechanism involving lipid peroxidation and reactive oxygen species accumulation. In non-small cell lung cancer (NSCLC), ferroptosis offers promising strategies to overcome drug resistance and enhance the efficacy of existing therapies. While the literature on ferroptosis in NSCLC has expanded rapidly over the past decade, a comprehensive understanding of its research trends, global collaboration patterns, and emerging hotspots remains lacking.
Objective: This study employs bibliometric and visualized analysis to systematically evaluate global research trends, influential contributors, and thematic evolution in ferroptosis research for NSCLC. The findings aim to guide future investigations and promote interdisciplinary collaborations.
Methods: Data were extracted from the Web of Science Core Collection on December 24, 2024. Bibliometric tools including VOSviewer, CiteSpace, and GraphPad Prism were used to analyze publication trends, citation patterns, collaborative networks, and research hotspots. Key indicators such as publication output, geographic contributions, institutional performance, and keyword co-occurrence were visualized to elucidate the field’s development.
Results: A total of 964 publications from 52 countries and regions were analyzed, with China and the United States emerging as the most influential contributors. Chinese institutions such as Fudan University and Central South University led in publication output, while US-based authors had the highest citation impact. Research hotspots included ferroptosis mechanisms, biomarkers, oxidative stress, immunotherapy, and drug resistance. Keyword and citation analyses reveal an increasing emphasis on integrating ferroptosis inducers with immune checkpoint inhibitors and leveraging nanomedicine for targeted therapy.
Conclusion: This bibliometric analysis highlights the rapid expansion of ferroptosis research in NSCLC, revealing key contributors, global trends, and emerging areas of focus. The integration of ferroptosis with immunotherapy and precision medicine holds immense promise for advancing NSCLC treatment. Future research should prioritize international collaboration, explore resistance mechanisms, and harness advanced technologies such as nanomedicine and artificial intelligence to maximize therapeutic potential.
1 Introduction
Ferroptosis, a recently identified form of regulated cell death characterized by iron-dependent lipid peroxidation, has emerged as a promising area of investigation in oncology (1). Unlike apoptosis, necrosis, and autophagy, ferroptosis is distinguished by its reliance on intracellular iron and the accumulation of reactive oxygen species (ROS) derived from lipid peroxides, making it a unique mechanism of cell death (2, 3). In cancer research, ferroptosis has garnered increasing attention due to its potential to overcome drug resistance and enhance the efficacy of existing treatments (4, 5). Lung cancer, a leading cause of cancer-related mortality worldwide, comprises two major types: small cell lung cancer (SCLC) and non-small cell lung cancer (NSCLC), with the latter accounting for approximately 85% of cases (6, 7). Despite advancements in targeted therapies and immunotherapies, NSCLC remains a clinical challenge, with high rates of recurrence and metastasis contributing to poor prognoses in many patients (8, 9).
Recent studies have highlighted the role of ferroptosis in regulating lung cancer progression through mechanisms involving oxidative stress, iron metabolism, and interactions within the tumor microenvironment (10). Notably, ferroptosis intersects with cancer immunology by triggering immunogenic cell death, which activates antitumor immunity, and by altering the tumor microenvironment to enhance immunotherapy efficacy. By inducing ferroptosis, researchers aim to develop novel therapeutic strategies that target resistant tumor cells, enhance the cytotoxic effects of chemotherapy and radiotherapy, and modulate immune responses (10, 11). The combination of ferroptosis inducers with immune checkpoint inhibitors has shown promising preclinical results in NSCLC models, indicating that ferroptosis could enhance NSCLC immunotherapy strategies (12, 13). However, the interplay between ferroptosis and cancer biology remains complex, with several unresolved questions regarding its regulatory pathways, tumor-specific effects, and potential side effects in clinical applications.
Bibliometric analysis provides a systematic approach to evaluating the progress and dynamics of research fields by analyzing publication data, including authorship, collaboration networks, citation patterns, and keyword clusters (14). As a quantitative and visual tool, bibliometric analysis allows researchers to identify influential studies, emerging hotspots, and global trends, offering valuable insights for guiding future research. While the literature on ferroptosis in lung cancer has expanded rapidly over the past decade, a comprehensive bibliometric review of this field has yet to be conducted.
This study aims to address this gap by systematically analyzing publications related to ferroptosis in NSCLC. By examining trends in publication output, geographic distribution, institutional collaborations, and research hotspots, we seek to provide a comprehensive overview of the field’s development. By providing a detailed overview of the current state of ferroptosis research in lung cancer, this study seeks to inform interdisciplinary collaborations, foster innovation, and contribute to the translation of ferroptosis-based therapies into clinical practice.
2 Materials and methods
2.1 Data acquisition
Web of Science Core Collection (WoSCC), as a high-quality digital literature resource database encompassing various fields, has been widely accepted by researchers and is considered the optimal choice for conducting bibliometric analysis (14, 15). WoSCC was selected as the primary data source due to its extensive coverage of high-impact, peer-reviewed journals, which ensures the inclusion of rigorously vetted research in ferroptosis and NSCLC. This focus on high-quality publications aligns with the study’s goal of providing a reliable and comprehensive analysis of the field. On December 24, 2024, we conducted a search for all articles related to ferroptosis in lung cancer within WoSCC, using the following search query: (((((((((((TS=("Carcinoma, Non-Small-Cell Lung")) OR TS=("Carcinoma, Non Small Cell Lung")) OR TS=("Carcinomas, Non-Small-Cell Lung")) OR TS=("Lung Carcinoma, Non-Small-Cell")) OR TS=("Lung Carcinomas, Non-Small-Cell")) OR TS=("Non-Small-Cell Lung Carcinomas")) OR TS=("Carcinoma, Non-Small Cell Lung")) OR TS=("Non-Small Cell Lung Cancer")) OR TS=("Non-Small-Cell Lung Carcinoma")) OR TS=("Non Small Cell Lung Carcinoma")) OR TS=("Nonsmall Cell Lung Cancer")) OR TS=("Non-Small Cell Lung Carcinoma")) OR TS=(NSCLC) AND TS=(Ferroptosis). The literature selection for this study was based on the following inclusion criteria: (1) full-text publications related to ferroptosis in lung cancer; (2) articles and review manuscripts written in English. The exclusion criteria were as follows: (1) subjects unrelated to ferroptosis in lung cancer; (2) articles in the form of conference abstracts, news, brief reports, etc. The pure text version of the selected papers was exported. The research flow chart is shown in Figure 1.
2.2 Data analysis and visualization
This study utilized several software tools for data analysis and visualization, including GraphPad Prism (version 8.0.2), CiteSpace (6.2.4R 64-bit Advanced Edition), and VOSviewer (version 1.6.18), to comprehensively analyze the annual publication volume, national publication trends, and various proportions, as well as to generate scientific knowledge maps. GraphPad Prism v8.0.2 was used to calculate and display the annual publication volume, publication trends, and research proportions across different regions/countries (16). CiteSpace, developed by Professor Chaomei Chen, is a literature analysis software mainly used for visualizing and constructing co-citation networks (17). By creating an experimental framework, CiteSpace aids researchers in identifying emerging concepts within a specific field and assessing existing research techniques. Additionally, CiteSpace is capable of revealing frontier issues and development trends in research, as well as forecasting future academic progress. VOSviewer, a free Java-based software developed by Waltman et al., is designed for analyzing large-scale bibliometric data and visualizing the results (18). The software offers several visualization modes, including network visualization, overlay visualization, and density visualization, which assist users in effectively identifying collaboration patterns, co-occurring terms, and research hotspots in the literature. In VOSviewer, the minimum threshold for keyword co-occurrence was set to 8, with the default 'association strength' algorithm applied, the layout configured to its default values, a cluster resolution of 1, and the 'merge small clusters' option selected. In CiteSpace, the analysis spanned 2015 to 2024, with a time slice of 1 year per segment, using the default term source and analyzing one item at a time; the selection criteria utilized the g-index with a k-value of 25, while other parameters remained at their default settings. For clustering, an 'all in one' approach was adopted using the 'cluster' option, with layout and style optimized for clarity, and clustering was based on 'Keywords' as labels, employing the log-likelihood ratio (LLR) method. For citation burst analysis, the γ value was set to 0.1, the minimum duration to 1 year, with all other settings kept default, and the top 50 burst keywords were selected for further examination. These tools provided multidimensional support for data analysis and visualization in this study, helping to identify and assess the patterns, trends, and collaborations within the literature, as well as revealing the key issues and future directions of research in the field. This study followed the guidelines for reporting bibliometric reviews of biomedical literature (BIBLIO) (19).
3 Results
3.1 Global trend in publication outputs and citations
The analysis revealed that, as of December 24, 2024, a total of 964 publications related to ferroptosis in lung cancer were indexed in the WoSCC database, comprising 757 original articles and 207 reviews. These studies were contributed by researchers from 52 countries and regions, 1,157 institutions, and 5,198 authors. As shown in Figure 2, the annual publication output and citation frequency of related studies from 2015 to 2024 exhibited a clear growth trend, which can be divided into three phases: before 2017, the annual output remained below 10 publications; since 2018, the annual output exceeded 10 publications, marking a steady increase; and after 2021, the annual output surpassed 100 publications for the first time, reaching 117, and continued to grow significantly, peaking at 362 publications in 2024. This rapid growth in publication volume indicates that ferroptosis in lung cancer is likely to remain a prominent and active area of research in the foreseeable future.
3.2 Distribution of countries/regions
A total of 52 countries and regions have conducted research on the application of ferroptosis in lung cancer. Figures 3A, B display the annual publication output of the top 10 countries over the past decade. The top five countries in this field are China, the United States, Germany, Japan, and South Korea. China accounts for 84.13% of the total publications, significantly outpacing all other countries. Among the top 10 countries/regions in terms of publication output, China’s papers have been cited 20,915 times (Table 1), far exceeding all other nations. Its citation/publication ratio (25.79) ranks fourth globally, indicating that the quality of its publications is generally high. The United States ranks second in publication output (93 papers) and second in citations (7,721), with the highest citation/publication ratio (83.02), indicating a strong citation impact. The collaboration network, shown in Figure 3C, illustrates close cooperation between China and the United States, the two countries with the highest publication output. The United States has strong collaborations with the United Kingdom, Italy, and Spain, while China collaborates more closely with Germany, India, and South Korea. China not only leads in publication volume and citation frequency but also demonstrates exceptional centrality (0.53) in the global scientific collaboration network, indicating its dominant position in this field.
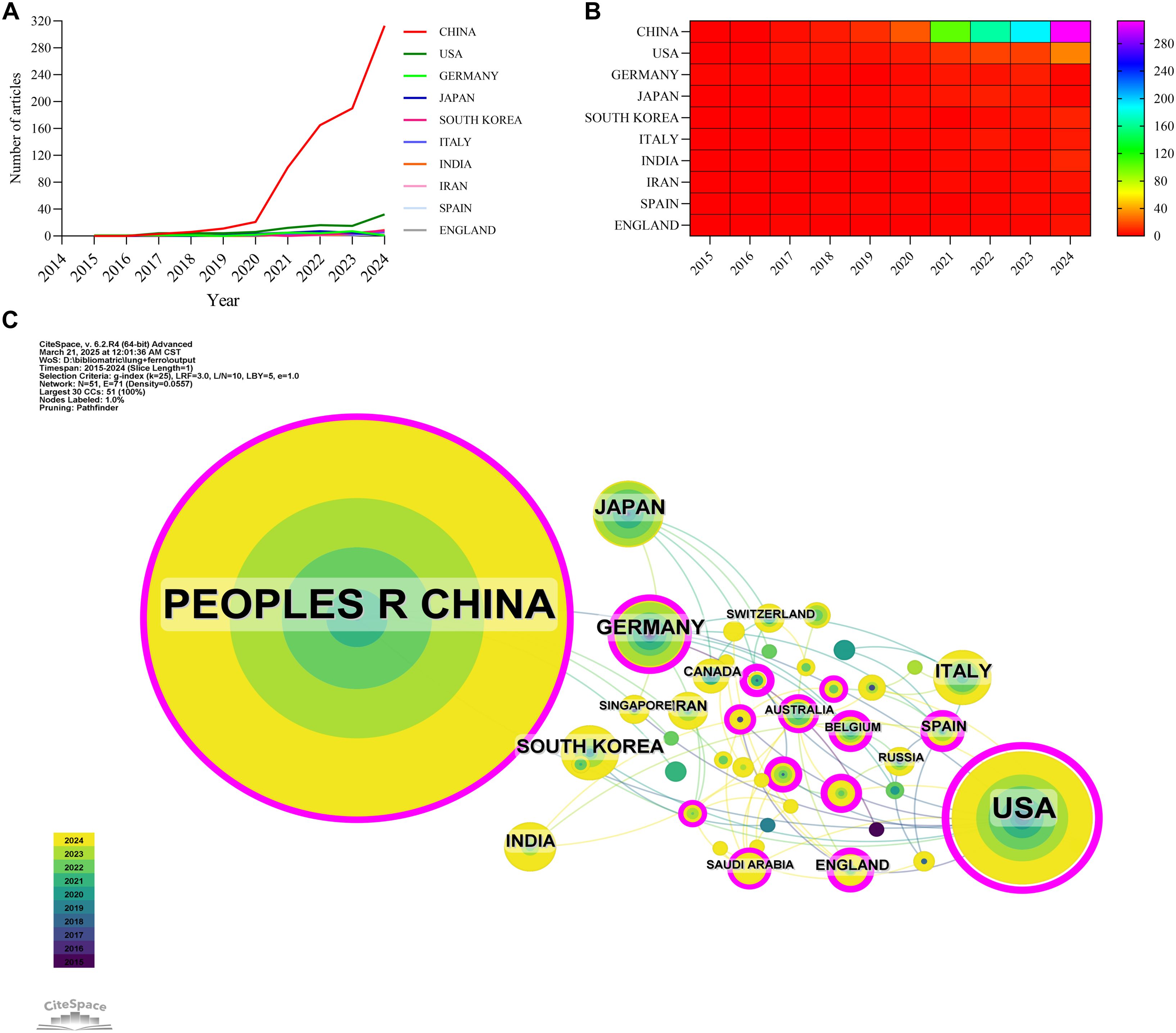
Figure 3. Country/region collaboration network of research on ferroptosis for non-small cell lung cancer. (A) Line graph of national publications. (B) Heat map of national publications. (C) Networks of country cooperation, node size represents the volume of publications and the line color represents the year of collaboration.
3.3 Institutions
A total of 1,157 institutions have systematically published research related to ferroptosis in lung cancer. The top ten institutions in terms of publication output are all from China (Table 2, Figure 4). Fudan University leads with the highest number of publications (44 papers, 2,040 citations, an average of 46.36 citations per paper). Central South University ranks second (42 papers, 2,067 citations, an average of 49.21 citations per paper), followed by Shanghai Jiao Tong University (41 papers, 922 citations, an average of 22.49 citations per paper) in third place, and the Chinese Academy of Sciences (39 papers, 1,922 citations, an average of 49.28 citations per paper) in fourth place. Further analysis revealed that both domestic and international institutions tend to collaborate more frequently with institutions within their own countries. This underscores the need to strengthen international collaborations and break down academic barriers.
3.4 Journals
Tables 3 and 4 list the top 10 journals in terms of both publication output and citation frequency. Frontiers in Pharmacology (28 papers, 2.90%) is the most prolific journal in this field, followed by Frontiers in Oncology (26 papers, 2.70%), Cell Death & Disease (22 papers, 2.28%), and Frontiers in Cell and Developmental Biology (18 papers, 1.87%). Among the top ten most prolific journals, Cell Death & Disease has the highest impact factor (IF) of 8.1. All of the journals listed fall within the Q1 or Q2 quartiles. The elevated proportion of Q1 journals could be attributed to the field's inclination toward high-impact, stringently peer-reviewed publications, which correspond closely with the research topic's significance and interdisciplinary character. The journal impact is determined by its citation frequency, indicating the significant influence the journal has on the scientific community. According to the density map of the journals, publications in this field primarily fall into four categories: oncology, immunology, pharmacology, and comprehensive (Figure 5A).
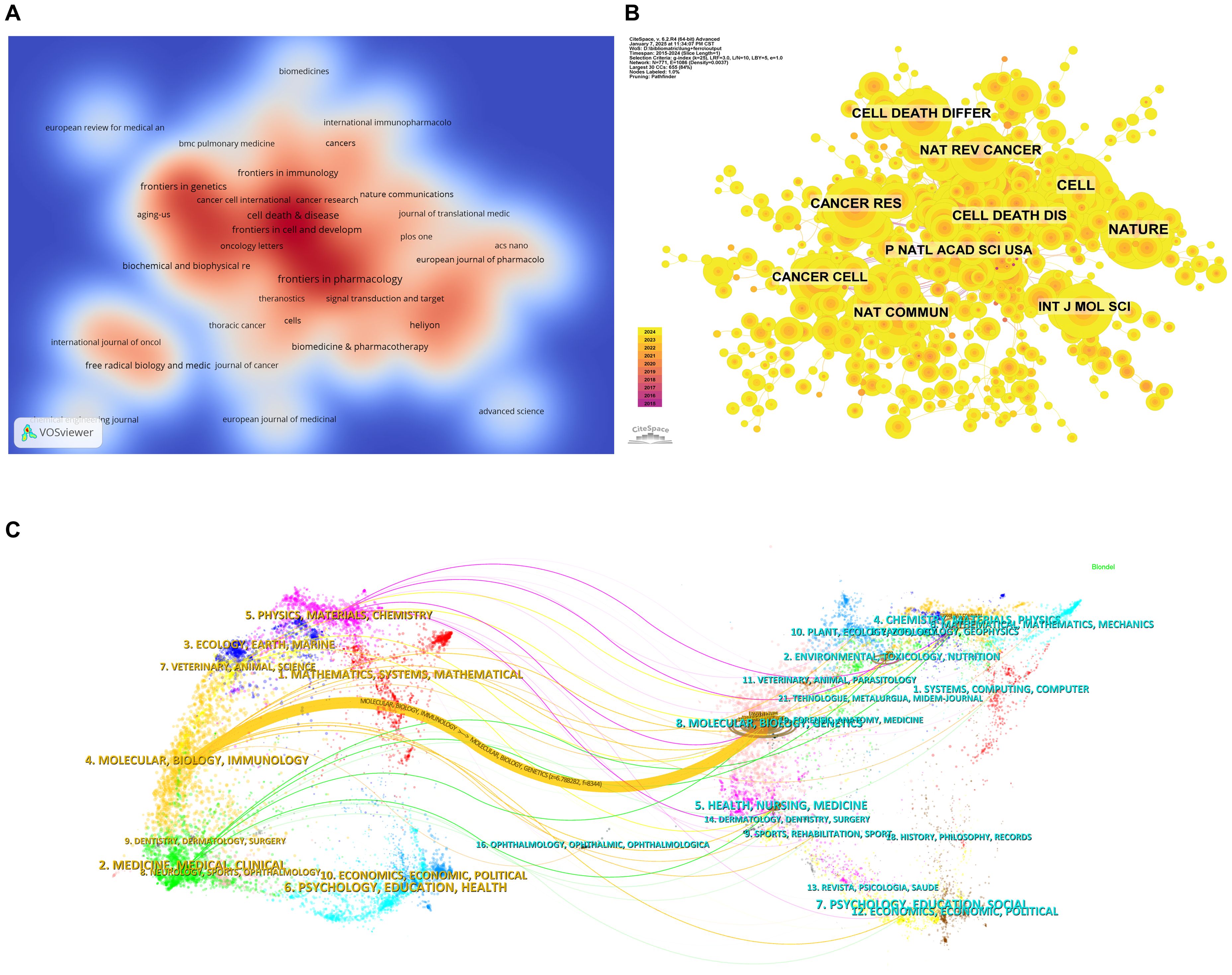
Figure 5. Analysis of journal sources. (A) Density web map of journal publications. (B) Co-citation network map of journals. (C) Dual map of journals.
Based on Figure 5B and Table 4, the most frequently co-cited journal is CELL (728 citations), followed by NATURE (666 citations) and Cell Death & Disease (562 citations). Among the top ten most co-cited journals, Nature Reviews Cancer (NAT REV CANCER) is the most highly cited (434 citations), with the highest IF (72.5) among the top ten. All co-cited journals fall within the Q1 quartile. The thematic distribution of academic publications is illustrated through the dual-map overlay (Figure 5C), where the colored trajectories represent citation links. The citing journals are on the left, and the cited journals are on the right. From the results, we identified a major citation pathway: research published in journals within the Molecular/Biology/Genetics domain is predominantly cited by research published in journals within the Molecular/Biology/Immunology domain.
3.5 Authors
Among all authors who have published research on ferroptosis in lung cancer, Table 5 lists the top 10 most prolific authors. These authors collectively contributed 87 papers, accounting for 7.04% of all publications in this field. Tao Yongguang authored the most papers (10), followed by Zhan Cheng (10), Liu Shuang (9), Wang Xiang (9), and Zhang Xiao (9). The collaborative network among authors was visualized using CiteSpace (Figure 6). Figure 7 and Table 5 present the top 10 most frequently co-cited and cited authors, respectively. A total of 86 authors were cited more than 50 times, highlighting their significant reputation and influence in the field. The largest nodes in the co-citation network correspond to the most frequently co-cited authors, including Dixon SJ (504 citations), Yang WS (343 citations), and Stockwell BR (310 citations). Tao Yongguang and Dixon SJ are pioneers in applied research and basic research, respectively. Tao's series of work focused on the therapeutic potential of ferroptosis in NSCLC, while Dixon's pioneering research first defined ferroptosis and laid the theoretical foundation for this field.
3.6 Citation and co-citation analysis
Citation analysis is a key method for assessing the relevance of academic papers, as it reflects both the influence of articles within a field and the research hotspots in that domain. Table 6 lists the top 10 most cited papers. The most cited paper, titled "CD8+ T cells regulate tumour ferroptosis during cancer immunotherapy," has been cited 161 times, elucidating the synergistic interplay between ferroptosis and antitumor immunity. This is followed by ""Ferroptosis: mechanisms, biology and role in disease," with 154 citations, and "Broadening horizons: the role of ferroptosis in cancer" with 152 citations, which collectively provide a systematic summary of the molecular mechanisms of ferroptosis, offering a theoretical framework for identifying ferroptosis-related targets (20–22).
To further explore the relationships among these citations, a co-citation network was constructed, using a one-year time slice for the period from 2015 to 2024. The co-citation network contains 681 nodes and 3,586 links (Figure 8A). Cluster and temporal analyses of the co-cited references revealed nine distinct clusters (Figures 8B,C). Early research hotspots were identified in the cancer cluster (Cluster 4). Mid-stage hotspots include overall survival (Cluster 1), survival (Cluster 7), and DNA damage and repair. Emerging hotspots and trends in this field are represented by clusters such as prognosis (Cluster 0), dihydroartemisinin (Cluster 2), NSCLC (Cluster 3), cuproptosis (Cluster 5), lung disease (Cluster 6), and butyrate (Cluster 9).
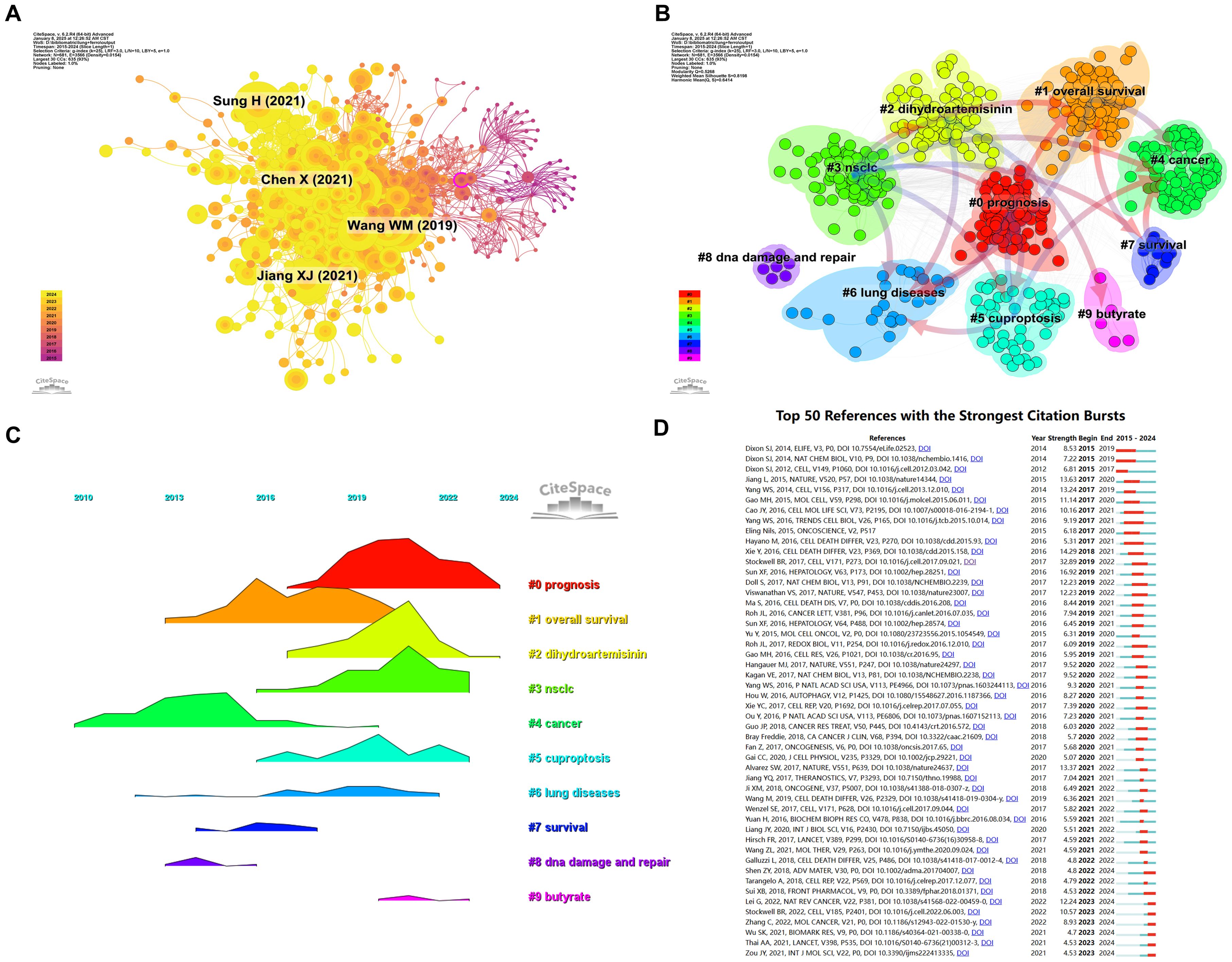
Figure 8. Analysis cited references and co-cited references. (A) Co-cited network of literature. (B) Clustering of co-cited literature. (C) Peak map of co-cited literature. (D) Bursting map of cited literature.
Figure 8D illustrates the top 50 references with the strongest citation bursts. The most prominent citation burst is associated with the 2014 paper titled "Pharmacological inhibition of cystine-glutamate exchange induces endoplasmic reticulum stress and ferroptosis", which exhibited the strongest burst strength of 8.53, reflecting its lasting influence on the study of ferroptosis mechanisms (23). While recent burst literature (such as Lei G, 2022, Nat Rev Cancer) points to the latest progress in ferroptosis as a vulnerability in cancer treatment (24). Among the 50 references with the strongest citation bursts, 46 were published between 2015 and 2024, indicating that these papers have been frequently cited over the past decade. Notably, 8 of these references are currently in their peak citation period, suggesting that ferroptosis-related research in lung cancer will continue to attract significant attention in the future.
3.7 Keywords and hotspots
Keyword analysis provides a rapid understanding of the status and developmental trends within a research field. Based on the co-occurrence analysis of keywords in VOSviewer, the most frequently appearing keyword is ferroptosis (681 occurrences), followed by apoptosis (143), cell death (142), lung cancer (140), and death (109) (Table 7, Figures 9A, B). After removing irrelevant keywords, a network comprising 169 keywords with at least 8 occurrences was constructed, revealing six distinct clusters. Cluster 1 (red) includes 51 keywords, such as ferroptosis, immunotherapy, prognosis, biomarker, and metastasis. Cluster 2 (green) contains 33 keywords, including lung cancer, oxidative stress, autophagy, drug resistance, and tumor suppressor. Cluster 3 (blue) consists of 31 keywords, including iron, GPX4, cisplatin, ROS, and iron metabolism. Cluster 4 (yellow) includes 25 keywords, such as apoptosis, angiogenesis, p53, and invasion. Cluster 5 (purple) comprises 24 keywords, including artemisinin, chemotherapy, drug delivery, and nanomedicine. Finally, Cluster 6 (cyan) contains 5 keywords, including EGFR and toxicity.
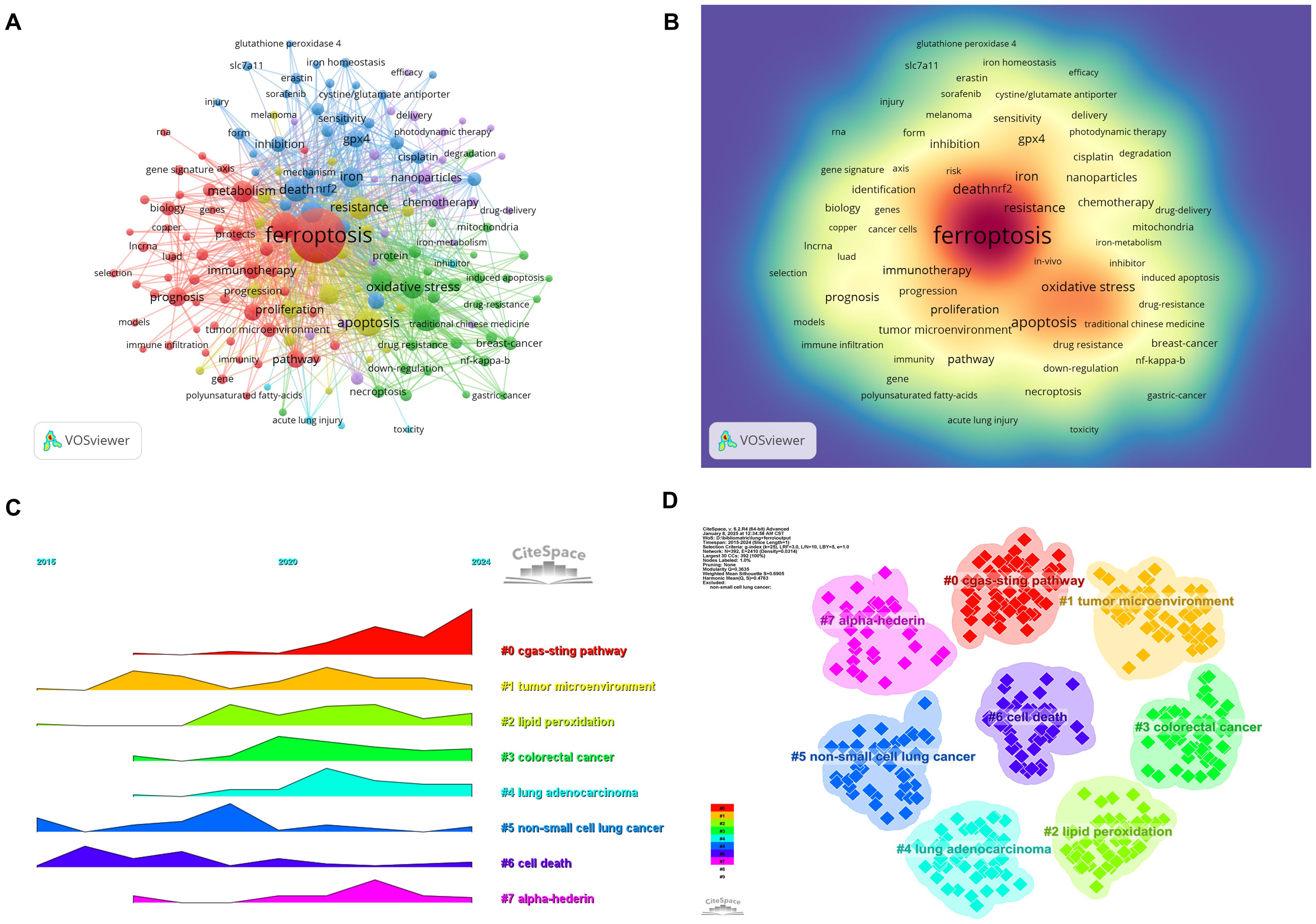
Figure 9. Analysis of keywords associated with ferroptosis in non-small cell lung cancer. (A) Network map of high-frequency keywords. (B) Density map of keywords. (C) Peak map of keyword clustering. (D) Clustering map of keywords.
A temporal visualization using CiteSpace (Figures 9C, D) was generated to illustrate the evolution of research hotspots over time. The analysis revealed that the cGAS-STING pathway, tumor microenvironment, lipid peroxidation, colorectal cancer, lung adenocarcinoma, non-small cell lung cancer, cell death, and alpha hederin are current research hotspots. Among the 434 strongest burst keywords identified in this field, we focused on the top 50 with the strongest citation bursts (Figure 10), which represent the current research priorities and potential future directions in this domain.
4 Discussion
4.1 General information
This bibliometric analysis, diverging from previous pan-cancer bibliometric studies on ferroptosis, centers on NSCLC, offering systematic evaluation of the exponential increase in ferroptosis-related lung cancer research over the past decade, particularly emphasizing the growing integration of ferroptosis with immunotherapy to guide precision medicine advancements. The increasing annual publication output and citation frequency since 2018 reflect a rising global interest in ferroptosis as a promising therapeutic target (25). The growing prominence of ferroptosis aligns with advancements in understanding its unique mechanisms, such as iron-dependent lipid peroxidation, and its potential applications in overcoming therapeutic resistance (21, 26).
The significant contributions of China and the United States to the volume and impact of publications indicate their leadership in ferroptosis research. China, with its extensive research output, has established itself as a dominant force, while the United States leads in citation impact, demonstrating high-quality contributions. This divergence underscores the need for increased international collaborations to enhance both the quantity and quality of research outputs globally (27).
4.2 Institutional and author contributions
Institutional analysis reveals that Chinese universities, particularly Fudan University, Central South University, and Shanghai Jiao Tong University, are at the forefront of ferroptosis research, contributing significantly to the field’s publication output. China accounts for 84.13% of total publications, demonstrating its dominance in research volume. However, collaboration networks show a marked preference for domestic partnerships, which may limit the potential for global knowledge sharing. In contrast, the United States, despite a lower publication volume, achieves a significantly higher citation rate (83.02 vs. 25.79), indicating a stronger emphasis on high-quality, impactful research. This disparity may stem from differences in research priorities and funding mechanisms: the United States tends to prioritize original, foundational studies that often lead to paradigm shifts, while China’s rapid publication output may reflect a focus on applied research and translational studies. Additionally, US institutions, such as the University of Texas and Memorial Sloan Kettering Cancer Center, exhibit stronger international partnerships, publishing frequently in high-impact, English-language journals that are widely cited globally, whereas Chinese institutions collaborate more within domestic networks, potentially influenced by language preferences, data sharing policies, and funding constraints (28).
Prominent authors such as Tao Yongguang, Dixon SJ, and Stockwell BR have significantly shaped the field, with research focusing on ferroptosis mechanisms, biomarkers, and therapeutic applications (3, 29). Dixon SJ’s seminal work on the discovery of ferroptosis has catalyzed numerous follow-up studies, positioning him as a key figure in the field, while Stockwell BR’s contributions to understanding ferroptosis regulation have influenced subsequent therapeutic developments (30). The strong influence of these authors underscores the importance of foundational research in driving innovation and collaboration. However, the preference for domestic collaboration in China may be attributed to several factors, including funding mechanisms that incentivize domestic programs, stringent data privacy regulations limiting the exchange of clinical and genomic data critical for ferroptosis research, and challenges related to intellectual property protection. Language barriers and differing research priorities further exacerbate this trend, as US-based research benefits from broader international visibility.
To address these challenges and bridge research gaps, strengthening international collaborations, particularly between leading institutions in China and the United States, is essential. Establishing transnational research alliances could facilitate data sharing, harmonize research protocols, and provide funding for joint projects. Open data platforms compliant with privacy regulations would enable researchers to share datasets securely, while academic exchange program could foster mutual understanding and trust among international teams. Additionally, addressing language barriers through translation services and promoting English as a common scientific language could enhance communication. By promoting these initiatives, the global impact of ferroptosis research could be improved, fostering innovation and accelerating the translation of foundational discoveries into clinical advancements.
4.3 Research hotspots and frontiers
Keyword co-occurrence and co-citation analyses reveal distinct clusters representing the major research hotspots in ferroptosis and lung cancer. These include ferroptosis mechanisms, oxidative stress, biomarkers, immunotherapy, and drug resistance. Notably, immunotherapy has emerged as a significant trend, with preclinical studies highlighting the potential of combining ferroptosis inducers with immune checkpoint inhibitors (ICIs) to enhance anti-tumor efficacy (10, 31). Such combinations have shown promise in overcoming immune evasion in NSCLC, underscoring ferroptosis as a complementary strategy in modern oncology immunotherapy (32).
Biomarker identification is another critical research frontier. Markers such as GPX4 and lipid peroxidation pathways are pivotal for predicting ferroptosis susceptibility and tailoring therapeutic interventions (33–35). Advances in nanomedicine, including the development of drug delivery systems targeting tumor-specific ferroptosis pathways, further enhance the precision and efficacy of these treatments (36, 37). Additionally, the cGAS-STING pathway and tumor microenvironment modulation have gained attention for their roles in linking ferroptosis to immune response and inflammation (38).
Temporal analysis of research trends highlights the evolving focus of ferroptosis studies. Early research emphasized fundamental mechanisms and the role of iron metabolism in ferroptosis (39). Mid-stage studies explored therapeutic applications, including chemotherapy and radiotherapy combinations (24, 30). More recently, research has shifted toward integrating precision medicine approaches, such as genomic profiling and personalized therapies, into ferroptosis-based interventions (40–42). This progression reflects the dynamic nature of the field and its responsiveness to technological and clinical advancements.
4.4 Ferroptosis mechanisms: an in-depth exploration in lung cancer
Ferroptosis, characterized by iron-dependent lipid peroxidation, is intricately linked to lung cancer progression, particularly in NSCLC. A hallmark of ferroptosis is dysregulated iron metabolism, which contributes to ROS production through the fenton reaction (26). In NSCLC, elevated expression of transferrin receptor 1 (TFR1) and reduced ferroportin levels drive iron accumulation, creating a pro-ferroptotic environment (43). Furthermore, tumor cells often exhibit increased ferritin levels, which confer chemoresistance (44, 45). Strategies targeting ferritinophagy or enhancing iron uptake have shown promise in sensitizing lung cancer cells to ferroptosis. Lipid peroxidation, mediated by enzymes like ACSL4 and lipoxygenases, plays a central role in ferroptosis by oxidizing polyunsaturated fatty acids (PUFAs) in membrane phospholipids (46). The overexpression of ACSL4 in NSCLC enhances ferroptosis susceptibility, while increased mono-unsaturated fatty acid levels confer resistance, highlighting the therapeutic potential of modulating lipid composition in tumors (47).
The glutathione (GSH)-GPX4 axis is another critical regulatory pathway in ferroptosis, with GPX4 reducing lipid hydroperoxides to non-toxic alcohols in a GSH-dependent manner (48). NSCLC cells often rely heavily on this axis to counteract oxidative damage. Inhibitors of GPX4, such as RSL3, and agents targeting cystine uptake, like erastin, disrupt this pathway, leading to ferroptotic cell death (49). The tumor microenvironment (TME) further modulates ferroptosis (50). Hypoxia, commonly observed in lung tumors, inhibits ROS generation, reducing ferroptosis sensitivity. Conversely, reoxygenation or pharmacological normalization of the TME restores ferroptosis susceptibility. The interplay between ferroptosis and immune responses is particularly noteworthy (51). Ferroptotic cancer cells release damage-associated molecular patterns (DAMPs), which activate anti-tumor immunity (52). Combining ferroptosis inducers with immune checkpoint inhibitors has shown synergistic effects in preclinical NSCLC models, highlighting the potential for integrated therapeutic strategies.
Recent advances in genomics and epigenetics provide further insights into ferroptosis regulation in lung cancer. Mutations in key genes, such as KRAS and TP53, alter ferroptosis sensitivity (53). KRAS mutations increase ROS production and ferroptotic vulnerability, while TP53 enhances ferroptosis through the regulation of cystine uptake and glutaminolysis (54). Epigenetic modifications, such as DNA methylation and histone acetylation, also influence ferroptosis-related gene expression (55). Clinically, ferroptosis inducers combined with chemotherapy or immunotherapy offer a promising avenue for overcoming resistance and improving therapeutic efficacy. Nanomedicine, including nanoparticle-based delivery systems, provides a targeted approach to maximize the benefits of ferroptosis inducers while minimizing off-target effects (56).
4.5 Clinical translation of ferroptosis mechanisms
The translation of ferroptosis mechanisms into clinical strategies represents a promising frontier in NSCLC treatment. Preclinical studies have demonstrated the efficacy of ferroptosis inducers, such as erastin and RSL3, in sensitizing NSCLC cells to chemotherapy and radiotherapy (57). Notably, the combination of ferroptosis inducers with ICIs has shown synergistic effects in preclinical models, enhancing anti-tumor immunity and overcoming immune evasion. Recent studies have reported that ferroptotic cancer cells release DAMPs, which activate dendritic cells and promote T cell-mediated anti-tumor responses (58).
Despite the promising preclinical results, the clinical translation of ferroptosis research faces several challenges. First, the lack of validated biomarkers for ferroptosis susceptibility poses a significant barrier to patient stratification and treatment optimization. While GPX4 and ACSL4 have emerged as potential biomarkers, their clinical utility remains to be confirmed in large-scale studies. Second, the potential off-target effects of ferroptosis inducers, such as systemic toxicity and damage to normal tissues, raise concerns about their safety in clinical applications. Developing targeted delivery systems, such as nanoparticle-based carriers, could mitigate these risks by selectively delivering ferroptosis inducers to tumor sites. Third, the complex interplay between ferroptosis and the TME complicates its clinical application. For example, hypoxia and nutrient deprivation in the TME can reduce ferroptosis sensitivity, necessitating strategies to modulate the TME for enhanced therapeutic efficacy. Finally, regulatory and logistical challenges, such as the design of robust clinical trials and the establishment of standardized protocols, must be addressed to accelerate the translation of ferroptosis research into clinical practice (59).
4.6 Future research trends
The future of ferroptosis research in lung cancer is centered on unraveling its complex molecular mechanisms and integrating this knowledge into precision medicine. While significant strides have been made, further exploration of ferroptosis' interplay with other cell death pathways, such as apoptosis and autophagy, remains essential (60). Investigating the role of ferroptosis within the TME, including its effects on immune cells and stromal interactions, can unveil strategies to modulate the TME for enhanced therapeutic efficacy. Moreover, advances in genomic profiling have identified key biomarkers that influence ferroptosis sensitivity (61). Integrating these biomarkers into clinical workflows will enable the stratification of patients and the personalization of ferroptosis-based therapies. Predictive models that combine genomic, transcriptomic, and metabolic data hold promise for optimizing patient selection and treatment strategies. Nanotechnology-based drug delivery systems, including lipid nanoparticles and polymeric micelles, offer targeted delivery of ferroptosis inducers to tumor sites, minimizing systemic toxicity while enhancing efficacy (62). These systems can be further developed to co-deliver ferroptosis inducers alongside chemotherapeutic agents or immune checkpoint inhibitors, providing a synergistic, multidimensional approach to lung cancer treatment. Additionally, overcoming resistance mechanisms, such as tumor adaptations that evade ferroptosis by altering antioxidant pathways or lipid metabolism, will be critical. Finally, the application of artificial intelligence in ferroptosis research promises to accelerate drug discovery, optimize treatment protocols, and uncover novel therapeutic targets, paving the way for innovative and personalized treatment strategies that improve outcomes for lung cancer patients (63).
4.6 Limitations
This study represents a pioneering effort to analyze global research trends and potential frontiers in ferroptosis research for lung cancer through a bibliometric approach. However, certain limitations should be noted. The analysis was based exclusively on English-language articles and reviews retrieved from the WoSCC. This reliance on a single database may have excluded relevant studies indexed in other databases such as Scopus or PubMed, or publications in other languages, thereby potentially limiting the comprehensiveness of the findings. Future studies should consider integrating data from multiple databases to ensure a more comprehensive analysis. Additionally, the selection of keywords was guided by quantitative thresholds, it is important to acknowledge that the process may still involve some subjectivity, particularly in the exclusion of irrelevant terms. Another issue is that articles are influenced by their publication duration; recently published high-quality studies may have been underrepresented due to lower citation frequencies. Furthermore, bibliometric metrics, such as citation counts, reflect visibility rather than the intrinsic quality or clinical relevance of research, highlighting the need for more nuanced evaluation methods. Despite these constraints, the trends and insights elucidated in this study provide a robust foundation for understanding the current research landscape and guiding future investigations in this rapidly evolving field.
5 Conclusion
This study provides a comprehensive bibliometric and visualized analysis of ferroptosis research in NSCLC, highlighting global trends, collaboration patterns, and emerging research directions. Over the past decade, ferroptosis has become an increasingly prominent area of study, with significant contributions from China and the United States. Research hotspots include ferroptosis mechanisms, oxidative stress, immunotherapy, biomarkers, and nanomedicine, with recent trends emphasizing the integration of ferroptosis inducers with immune checkpoint inhibitors and advanced drug delivery systems. Our findings underscore the importance of ferroptosis in overcoming therapeutic resistance and advancing precision medicine for NSCLC. Future research should focus on unraveling the molecular mechanisms of ferroptosis, exploring its interplay with the tumor microenvironment, and addressing resistance mechanisms. International collaborations and the application of cutting-edge technologies such as nanomedicine and artificial intelligence are critical for maximizing the therapeutic potential of ferroptosis in lung cancer. Despite its limitations, this study offers valuable insights into the evolving landscape of ferroptosis research and its translational potential for improving patient outcomes in NSCLC.
Data availability statement
The original contributions presented in the study are included in the article/supplementary material. Further inquiries can be directed to the corresponding authors.
Author contributions
WZ: Conceptualization, Data curation, Formal Analysis, Methodology, Resources, Validation, Writing – original draft, Writing – review & editing. JG: Investigation, Methodology, Validation, Writing – original draft. YC: Conceptualization, Formal Analysis, Methodology, Writing – original draft. GJ: Conceptualization, Formal Analysis, Investigation, Methodology, Writing – original draft. DG-R: Conceptualization, Methodology, Validation, Writing – original draft. MM: Conceptualization, Project administration, Supervision, Validation, Writing – original draft, Writing – review & editing. CC: Conceptualization, Supervision, Validation, Visualization, Writing – original draft, Writing – review & editing.
Funding
The author(s) declare that financial support was received for the research and/or publication of this article. This work was funded by the Key R&D Program - International Cooperation Fields(24YFWA011).
Acknowledgments
We appreciate the data availability that was present via the Web of Science Core Ensemble Data, as well as the cooperation of all authors.
Conflict of interest
The authors declare that the research was conducted in the absence of any commercial or financial relationships that could be construed as a potential conflict of interest.
Generative AI statement
The author(s) declare that no Generative AI was used in the creation of this manuscript.
Publisher’s note
All claims expressed in this article are solely those of the authors and do not necessarily represent those of their affiliated organizations, or those of the publisher, the editors and the reviewers. Any product that may be evaluated in this article, or claim that may be made by its manufacturer, is not guaranteed or endorsed by the publisher.
References
1. Liu X, Zhang Y, Wu X, Xu F, Ma H, Wu M, et al. Targeting ferroptosis pathway to combat therapy resistance and metastasis of cancer. Front Pharmacol. (2022) 13:909821. doi: 10.3389/fphar.2022.909821
2. Dixon SJ, Lemberg KM, Lamprecht MR, Skouta R, Zaitsev EM, Gleason CE, et al. Ferroptosis: an iron-dependent form of nonapoptotic cell death. Cell. (2012) 149:1060–72. doi: 10.1016/j.cell.2012.03.042
3. Stockwell BR, Friedmann Angeli JP, Bayir H, Bush AL, Conrad M, Dixon SJ, et al. Ferroptosis: A regulated cell death nexus linking metabolism, redox biology, and disease. Cell. (2017) 171:273–85. doi: 10.1016/j.cell.2017.09.021
4. Qi R, Bai Y, Li K, Liu N, Xu Y, Dal E, et al. Cancer-associated fibroblasts suppress ferroptosis and induce gemcitabine resistance in pancreatic cancer cells by secreting exosome-derived ACSL4-targeting miRNAs. Drug resistance updates. (2023) 68:100960. doi: 10.1016/j.drup.2023.100960
5. Zhang C, Liu X, Jin S, Chen Y, and Guo R. Ferroptosis in cancer therapy: a novel approach to reversing drug resistance. Mol Cancer. (2022) 21:47. doi: 10.1186/s12943-022-01530-y
6. Bray F, Ferlay J, Soerjomataram I, Siegel RL, Torre LA, and Jemal A. Global cancer statistics 2018: GLOBOCAN estimates of incidence and mortality worldwide for 36 cancers in 185 countries. CA Cancer J Clin. (2018) 68:394–424. doi: 10.3322/caac.21492
7. Kocarnik JM, Compton K, Dean FE, Fu W, Gaw BL, Harvey JD, et al. Cancer incidence, mortality, years of life lost, years lived with disability, and disability-adjusted life years for 29 cancer groups from 2010 to 2019: A systematic analysis for the global burden of disease study 2019. JAMA Oncol. (2022) 8:420–44. doi: 10.1001/jamaoncol.2021.6987
8. Sun J, Wu S, Jin Z, Ren S, Cho , Zhu C, et al. Lymph node micrometastasis in non-small cell lung cancer. Biomed Pharmacother. (2022) 149:112817. doi: 10.1016/j.biopha.2022.112817
9. Yang S, Liu Y, Li MY, Ng CSH, Yang SL, Wang S, et al. FOXP3 promotes tumor growth and metastasis by activating Wnt/β-catenin signaling pathway and EMT in non-small cell lung cancer. Mol Cancer. (2017) 16:124. doi: 10.1186/s12943-017-0700-1
10. Zhao L, Zhou X, Xie F, Zhang L, Yan H, Huang J, et al. Ferroptosis in cancer and cancer immunotherapy. Cancer Commun (Lond Engl). (2022) 42:88–116. doi: 10.1002/cac2.12250
11. Yang F, Xiao Y, Ding JH, Jin X, Ma D, Li D, et al. Ferroptosis heterogeneity in triple-negative breast cancer reveals an innovative immunotherapy combination strategy. Cell Metab. (2023) 35:84–100.e108. doi: 10.1016/j.cmet.2022.09.021
12. Luo L and Xu G. Fascaplysin induces apoptosis and ferroptosis, and enhances anti-PD-1 immunotherapy in non-small cell lung cancer (NSCLC) by promoting PD-L1 expression. Int J Mol Sci. (2022) 23:1377435.449. doi: 10.3390/ijms232213774
13. Zhou C, Yu T, Zhu R, Lu J, Ouyang Zhang Z, et al. Timosaponin AIII promotes non-small-cell lung cancer ferroptosis through targeting and facilitating HSP90 mediated GPX4 ubiquitination and degradation. Int J Biol Sci. (2023) 19:1471–89. doi: 10.7150/ijbs.77979
14. Wei N, Xu Y, Li Y, Shi J, Zhang X, You Y, et al. A bibliometric analysis of T cell and atherosclerosis. Front Immunol. (2022) 13:948314. doi: 10.3389/fimmu.2022.948314
15. Jiang S, Liu Y, Zheng H, Zhang L, Zhao H, Sang X, et al. Evolutionary patterns and research frontiers in neoadjuvant immunotherapy: a bibliometric analysis. Int J Surg (London England). (2023) 109:2774–83. doi: 10.1097/S9.0000000000000492
16. Liu X and Li H. Global trends in research on aging associated with periodontitis from 2002 to 2023: a bibliometric analysis. Front endocrinology. (2024) 15:1374027. doi: 10.3389/fendo.2024.1374027
17. Chen C. Searching for intellectual turning points: progressive knowledge domain visualization. Proc Natl Acad Sci U S A. (2004) 101:5303–10. doi: 10.1073/pnas.0307513100
18. van Eck NJ and Waltman L. Software survey: VOSviewer, a computer program for bibliometric mapping. Scientometrics. (2010) 84:523–38. doi: 10.1007/s11192-009-0146-3
19. Montazeri A, Mohammadi S, Hesari PM, Ghaemi M, Riazi H, and Sheikhi-Mobarakeh Z. Preliminary guideline for reporting bibliometric reviews of the biomedical literature (BIBLIO): a minimum requirements. Systematic Rev. (2023) 12:239. doi: 10.1186/s13643-023-02410-2
20. Chen X, Kang R, Kroemer G, and Tang D. Broadening horizons: the role of ferroptosis in cancer. Nat Rev Clin oncology. (2021) 18:280–96. doi: 10.1038/s41571-020-00462-0
21. Jiang X, Stockwell BR, and Conrad M. Ferroptosis: mechanisms, biology and role in disease. Nat Rev Mol Cell Biol. (2021) 22:266–82. doi: 10.1038/s41580-020-00324-8
22. Wang W, Green M, Choi JE, Gijón M, Kennedy PD, Johnson JK, et al. CD8(+) T cells regulate tumour ferroptosis during cancer immunotherapy. Nature. (2019) 569:270–4. doi: 10.1038/s41586-019-1170-y
23. Dixon SJ, Patel DN, Welsch M, Skouta R, Lee ED, Hayano M, et al. Pharmacological inhibition of cystine-glutamate exchange induces endoplasmic reticulum stress and ferroptosis. eLife. (2014) 3:e02523. doi: 10.7554/eLife.02523
24. Lei G, Zhuang L, and Gan B. Targeting ferroptosis as a vulnerability in cancer. Nat Rev Cancer. (2022) 22:381–96. doi: 10.1038/s41568-022-00459-0
25. Zhang Y, Huang X, Qi B, Sun C, Sun K, Liu N, et al. Ferroptosis and musculoskeletal diseases: "Iron Maiden" cell death may be a promising therapeutic target. Front Immunol. (2022) 13:972753. doi: 10.3389/fimmu.2022.972753
26. Liang D, Minikes AM, and Jiang X. Ferroptosis at the intersection of lipid metabolism and cellular signaling. Mol Cell. (2022) 82:2215–27. doi: 10.1016/j.molcel.2022.03.022
27. Peng C, Kuang L, Zhao J, Ross AE, Wang Z, and Ciolino JB. Bibliometric and visualized analysis of ocular drug delivery from 2001 to 2020. J Controlled Release. (2022) 345:625–45. doi: 10.1016/j.jconrel.2022.03.031
28. Nedungadi P, Shah SM, Stokes MA, Kumar Nair V, Moorkoth A, and Raman R. Mapping autism's research landscape: trends in autism screening and its alignment with sustainable development goals. Front Psychiatry. (2023) 14:1294254. doi: 10.3389/fpsyt.2023.1294254
29. Wang H, Cheng Y, Mao C, Liu S, Xiao D, Huang J, et al. Emerging mechanisms and targeted therapy of ferroptosis in cancer. Mol Ther. (2021) 29:2185–208. doi: 10.1016/j.ymthe.2021.03.022
30. Stockwell BR. Ferroptosis turns 10: Emerging mechanisms, physiological functions, and therapeutic applications. Cell. (2022) 185:2401–21. doi: 10.1016/j.cell.2022.06.003
31. Gao W, Wang X, Zhou Y, Wang X, and Yu Y. Autophagy, ferroptosis, pyroptosis, and necroptosis in tumor immunotherapy. Signal Transduct Target Ther. (2022) 7:196. doi: 10.1038/s41392-022-01046-3
32. Yin J, Meng X, Peng L, Xie W, Liu X, He W, et al. Ferroptosis and cancer immunotherapy. Curr Mol Med. (2023) 23:401–9. doi: 10.217411566524022666220509124608
33. Liang D, Feng Y, Zandkarimi F, Wang H, Zhang Z, Kim J, et al. Ferroptosis surveillance independent of GPX4 and differentially regulated by sex hormones. Cell. (2023) 186:2748–2764.e2722. doi: 10.1016/j.cell.2023.05.003
34. Dodson M, Castro-Portuguez R, and Zhang DD. NRF2 plays a critical role in mitigating lipid peroxidation and ferroptosis. Redox Biol. (2019) 23:101107. doi: 10.1016/j.redox.2019.101107
35. Rochette L, Dogon G, Rigal E, Zeller M, Cottin Y, and Vergely C. Lipid peroxidation and iron metabolism: two corner stones in the homeostasis control of ferroptosis. Int J Mol Sci. (2022) 24. doi: 10.3390/ijms24010449
36. Huang M, Teng Q, Cao F, Huang J, and Pang J. Ferroptosis and ferroptosis-inducing nanomedicine as a promising weapon in combination therapy of prostate cancer. Biomater Sci. (2024) 12:1617–29. doi: 10.1039/d3bm01894f
37. Zhang M, Guo M, Gao Y, Wu C, Pan X, and Huang Z. Mechanisms and therapeutic targets of ferroptosis: Implications for nanomedicine design. J Pharmaceuti Anal. (2024) 14:100960. doi: 10.1016/j.jpha.2024.03.001
38. Chen Y, Fang ZM, Yi X, Wei X, and Jiang DS. The interaction between ferroptosis and inflammatory signaling pathways. Cell Death Dis. (2023) 14:205. doi: 10.1038/s41419-023-05716-0
39. Chen X, Yu C, Kang R, and Tang D. Iron metabolism in ferroptosis. Front Cell Dev Biol. (2020) 8:590226. doi: 10.3389/fcell.2020.590226
40. Berndt C, Alborzinia H, Amen VS, Ayton S, Barayeu U, Bartelt A, et al. Ferroptosis in health and disease. Redox Biol. (2024) 75:103211. doi: 10.1016/j.redox.2024.103211
41. Lei G, Zhuang L, and Gan B. The roles of ferroptosis in cancer: Tumor suppression, tumor microenvironment, and therapeutic interventions. Cancer Cell. (2024) 42:513–34. doi: 10.1016/j.ccell.2024.03.011
42. Zhou Q, Meng Y, Li D, Yao L, Le J, Liu Y, et al. Ferroptosis in cancer: From molecular mechanisms to therapeutic strategies. Signal Transduct Target Ther. (2024) 9:55. doi: 10.1038/s41392-024-01769-5
43. Cai S, Ding Z, Liu X, and Zeng J. Trabectedin induces ferroptosis via regulation of HIF-1α/IRP1/TFR1 and Keap1/Nrf2/GPX4 axis in non-small cell lung cancer cells. Chemico-Biol Interact. (2023) 369:110262. doi: 10.1016/j.cbi.2022.110262
44. Wang F, Deng G, Liang N, Hu P, Liu K, Liu T, et al. Serum ferritin level is an effective prognostic factor for lung cancer immunotherapy. Cancer Biol Ther. (2023) 24:2285367. doi: 10.1080/15384047.2023.2285367
45. Wu C, Zhong R, Wei T, Jin Y, He C, Li H, et al. Mechanism of targeting the mTOR pathway to regulate ferroptosis in NSCLC with different EGFR mutations. Oncol letters. (2024) 28:298. doi: 10.3892/01.2024.14431
46. Du L, Yang H, Ren Y, Ding Y, Xu Y, Zi X, et al. Inhibition of LSD1 induces ferroptosis through the ATF4-xCT pathway and shows enhanced anti-tumor effects with ferroptosis inducers in NSCLC. Cell Death Dis. (2023) 14:716. doi: 10.1038/s41419-023-06238-5
47. Jiang ML, Liu L, Wang Z, Yang X, Lin Z, Jiang R, et al. Kanglaite alleviates lung squamous cell carcinoma through ferroptosis. Int Immunopharmacol. (2025) 144:113616. doi: 10.1016/j.intimp.2024.113616
48. Wei Z, Zhou Z, Zhang Y, Wang J, Huang K, Ding Y, et al. PRKAA2 promotes tumor growth and inhibits ferroptosis through SLC7A11/GSH/GPX4 pathway in non-small cell lung cancer. Biotechnol Appl Biochem. (2024). doi: 10.1002/bab.2710
49. Peng S, Chen G, Yu KN, Feng Y, Zhao L, Yang M, et al. Synergism of non-thermal plasma and low concentration RSL3 triggers ferroptosis via promoting xCT lysosomal degradation through ROS/AMPK/mTOR axis in lung cancer cells. Cell communication signaling: CCS. (2024) 22:112. doi: 10.1186/s12964-023-01382-z
50. Cui K, Wang K, and Huang Z. Ferroptosis and the tumor microenvironment. J Exp Clin Cancer research: CR. (2024) 43:315. doi: 10.1186/s13046-024-03235-0
51. Yang P, Yang W, Wei Z, Li Y, Yang Y, and Wang J. Novel targets for gastric cancer: The tumor microenvironment (TME), N6-methyladenosine (m6A), pyroptosis, autophagy, ferroptosis and cuproptosis. Biomed Pharmacother. (2023) 163:114883. doi: 10.1016/j.biopha.2023.114883
52. Ramos S, Hartenian E, Santos JC, Walch P, and Broz P. NINJ1 induces plasma membrane rupture and release of damage-associated molecular pattern molecules during ferroptosis. EMBO J. (2024) 43:1164–86. doi: 10.1038/s44318-024-00055-y
53. Yan H, Talty R, Aladelokun O, Bosenberg M, and Johnson CH. Ferroptosis in colorectal cancer: a future target? Br J Cancer. (2023) 128:1439–51. doi: 10.1038/s41416-023-02149-6
54. Li J, Liu J, Zhou Z, Wu R, Chen X, Yu C, et al. Tumor-specific GPX4 degradation enhances ferroptosis-initiated antitumor immune response in mouse models of pancreatic cancer. Sci Trans Med. (2023) 15:eadg3049. doi: 10.1126/scitranslmed.adg3049
55. Wang Y, Hu J, Wu S, Fleishman JS, Li Y, Xu Y, et al. Targeting epigenetic and posttranslational modifications regulating ferroptosis for the treatment of diseases. Signal Transduct Target Ther. (2023) 8:449. doi: 10.1038/s41392-023-01720-0
56. Yi J, Minikes AM, and Jiang X. Aiming at cancer in vivo: ferroptosis-inducer delivered by nanoparticles. Cell Chem Biol. (2019) 26:621–2. doi: 10.1016/j.chembiol.2019.05.002
57. Kim JW, Min DW, Kim D, Kim J, Kim MJ, Lim H, et al. GPX4 overexpressed non-small cell lung cancer cells are sensitive to RSL3-induced ferroptosis. Sci Rep. (2023) 13:8872. doi: 10.1038/s41598-023-35978-9
58. Efimova I, Catanzaro E, van der Meeren L, Turubanova VD, Hammad H, Mishchenko TA, et al. Vaccination with early ferroptotic cancer cells induces efficient antitumor immunity. J Immunother Cancer. (2020) 8:e001369. doi: 10.1136/jitc-2020-001369
59. Gabert J, Beillard E, van der Velden VH, Bi W, Grimwade D, Pallisgaard N, et al. Standardization and quality control studies of 'real-time' quantitative reverse transcriptase polymerase chain reaction of fusion gene transcripts for residual disease detection in leukemia - a Europe Against Cancer program. Leukemia. (2003) 17:2318–57. doi: 10.1038/sj.leu.2403135
60. Tong X, Tang R, Xiao M, Xu J, Wang W, Zhang B, et al. Targeting cell death pathways for cancer therapy: recent developments in necroptosis, pyroptosis, ferroptosis, and cuproptosis research. J Hematol Oncol. (2022) 15:174. doi: 10.1186/s13045-022-01392-3
61. Jiang YZ, Ma D, Jin X, Xiao Y, Yu Y, Shi J, et al. Integrated multiomic profiling of breast cancer in the Chinese population reveals patient stratification and therapeutic vulnerabilities. Nat cancer. (2024) 5:673–90. doi: 10.1038/s43018-024-00725-0
62. Zhang J, Zhang S, Liu M, Yang Z, and Huang R. Research progress on ferroptosis and nanotechnology-based treatment in triple-negative breast cancer. Breast Cancer (Dove Med Press). (2024) 16:347–58. doi: 10.2147/BCTT.S475199
Keywords: ferroptosis, non-small cell lung cancer, bibliometrics, immunotherapy, biomarkers, drug resistance, nanomedicine
Citation: Zhang W, Gu J, Chen Y, Jiang G, Gonzalez-Rivas D, Ma M and Chen C (2025) Emerging research themes in ferroptosis research for non-small cell lung cancer: a bibliometric and visualized analysis. Front. Immunol. 16:1563108. doi: 10.3389/fimmu.2025.1563108
Received: 19 January 2025; Accepted: 28 April 2025;
Published: 16 May 2025.
Edited by:
Jinghua Pan, Jinan University, ChinaReviewed by:
Pingping Chen, University of Miami, Coral Gables, United StatesFeng Zhang, Nanjing University of Chinese Medicine, China
Ökkeş Zortuk, Ministry of Health, Türkiye
Yiqian Zhang, Tulane University, United States
Copyright © 2025 Zhang, Gu, Chen, Jiang, Gonzalez-Rivas, Ma and Chen. This is an open-access article distributed under the terms of the Creative Commons Attribution License (CC BY). The use, distribution or reproduction in other forums is permitted, provided the original author(s) and the copyright owner(s) are credited and that the original publication in this journal is cited, in accordance with accepted academic practice. No use, distribution or reproduction is permitted which does not comply with these terms.
*Correspondence: Chang Chen, Y2hlbnRob3JhY2ljQDE2My5jb20=; Minjie Ma, bHp1dHNtYUBvdXRsb29rLmNvbQ==
†These authors have contributed equally to this work