- 1Renal Division, Department of Medicine, Peking University First Hospital, Peking University Institute of Nephrology, Beijing, China
- 2Capital Institute of Pediatrics, Peking University Teaching Hospital, Beijing, China
Kawasaki disease (KD) is a relatively common autoimmune disease of childhood, characterized by systemic vasculitis and involvement of the cardiovascular system, particularly the coronary artery. Progressive inflammatory cascades and vascular injury are regarded as two major processes underlying KD. Although it is regarded as a self-limiting disease, some children exhibit resistance to intravenous immunoglobulin (IVIG) treatment, which can lead to the development of life-threatening coronary artery aneurysms that persist into adulthood. Pyroptosis, a special inflammatory cell death pattern, results in the intense release of inflammatory mediators and injuries of tissues such as endothelial cell damage. Evidence from in vitro studies and animal models suggests that pyroptosis and associated inflammatory cascades may play a significant role in KD. Here, we highlight the latest insights into pyroptosis in KD and explore the potential therapeutic interventions that target pyroptosis.
Introduction
Kawasaki disease (KD) was first reported by Japanese pediatrician, Dr. Tomisaku Kawasaki in 1967, and is also referred to as mucocutaneous lymph node syndrome (1, 2). It is distinguished by a set of defining clinical features, including persistent fever, conjunctival congestion, a distinctive strawberry-like tongue, a characteristic rash, changes in the peripheral limbs, and non-suppurative cervical lymphadenopathy (3) (Table 1). It is recognized as the leading cause of acquired heart disease in children, particularly those under the age of 5 years old (4). The incidence rate of KD exhibits variability across different racial groups and geographical locations. Notably, in certain areas with higher prevalence, such as Japan, the incidence rate among children under the age of 5 is particularly significant, reaching as high as 1 in 100 (5). Since the onset of the severe acute respiratory syndrome coronavirus type 2 (SARS-CoV-2) pandemic, the epidemiological landscape of Kawasaki disease has experienced substantial shifts across various regions. Amidst the rigorous implementation of epidemic prevention strategies, there has been a marked reduction in the incidence of Kawasaki disease, with decreases ranging from approximately 20% to 40% (6, 7). Despite its classification as an autoimmune disease, no conventional autoantigen has been found until now in KD, likely due to the complexity of the inflammatory cascades involved. The application of intravenous immunoglobulin (IVIG) has dramatically improved the outcome of patients with KD, however, a notable proportion of patients (4%) still progress to develop coronary artery aneurysms (8, 9).
Nevertheless, a limited understanding of the pathophysiology of KD has impeded the identification of the etiological events that lead to vasculitis and cardiovascular involvement. Inflammation and endothelial cell injury are two critical processes in the underlying pathological mechanisms (10). The infiltration of immune cells, particularly in the coronary arteries, is a common feature during the acute phase of KD (11, 12). Monocytes/macrophages and associated pro-inflammatory cytokines, such as interleukin-1 beta (IL-1β), play a significant role in vascular endothelial damage. Evidence from both in vitro and in vivo studies underscore the central role of IL-1β in KD and its related complications, strongly suggesting the involvement of pyroptosis in the pathological process of KD (10, 13, 14).
Recent findings have highlighted that children from certain ethnic backgrounds are displaying the characteristic symptoms of KD, such as strawberry tongue, rash, and conjunctival congestion, subsequent to exposure to SARS-CoV-2 and Yersinia pestis (15, 16). These microorganisms trigger a swift cell-damaging process known as pyroptosis, characterized by the activation of the inflammasome and the subsequent action of the gasdermin family of proteins, which function as pore-forming proteins (17–20). Morphologically, cells undergoing pyroptosis are distinguished by features such as cell swelling, the formation of large bubbles, and eventual rupture resulting in lytic cell death. Notably, patients with KD exhibit higher expression of the NLR family pyrin (PYD) domain-containing 3 (NLRP3) inflammasome, caspase-1, gasdermin D (GSDMD), and downstream proinflammatory cytokines such as IL-1β compared to healthy controls (21).
Here, we outline the pathophysiology of pyroptosis in KD and discuss the potential therapeutic strategies targeting pyroptosis for the treatment of KD.
Mechanisms of pyroptosis
Pyroptosis is a form of inflammatory necrosis initiated by the formation of an inflammasome (17, 18). The canonical activation of the inflammasome relies on NOD-like receptors and non-NOD receptors, which function as sensor proteins. Together with adaptor proteins and the protease caspase-1, the sensor proteins constitute the inflammasome complex, enabling the cleavage of procaspase-1 into active caspase-1. Active caspase-1 then cleaves GSDMD to generate N-terminal GSDMD (N-GSDMD), which then inserts into the membrane and oligomerizes, forming pores that ultimately lead to cell rupture. Additionally, active caspase-1 can also cleave IL-1β and IL-18, releasing them through these pores. Intercellular lipopolysaccharide (LPS) can trigger non-canonical inflammasome-induced pyroptosis, where caspase-11 is activated, leading to the cleavage of GSDMD, IL-1β, and IL-18. The upregulation of GSDMD and IL-1β in the serum of patients with KD indicates a significant role for pyroptosis in its pathogenesis(21, 22). Furthermore, both macrophages and endothelial cells exhibit a pyroptosis-associated pattern in KD, underscoring a crucial role of pyroptosis in inflammation and endothelial cell injury (Figure 1).
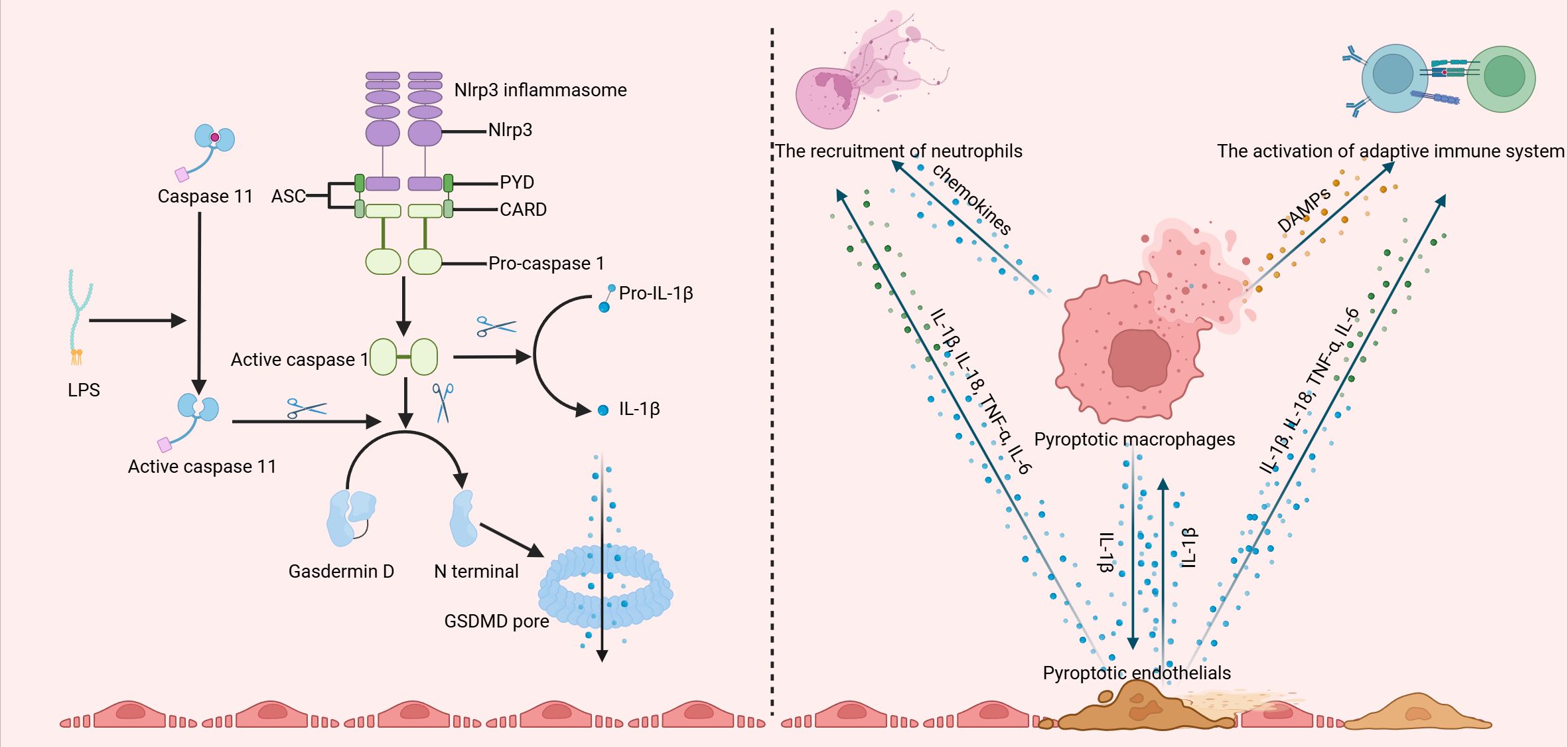
Figure 1. The canonical activation of the inflammasome involves the assembly of the NLRP3 inflammasome, which facilitates the cleavage of procaspase-1 into its active form, caspase-1. Active caspase-1 then cleaves gasdermin D (GSDMD), producing the N-terminal fragment of GSDMD (N-GSDMD). This fragment subsequently inserts into the membrane and oligomerizes, forming pores that ultimately result in cell lysis. Additionally, active caspase-1 is capable of cleaving IL-1β, allowing its release through these pores. Moreover, intracellular lipopolysaccharide (LPS) can activate a non-canonical inflammasome pathway, triggering caspase-11 and leading to the cleavage of GSDMD. Both macrophages and endothelial cells display a pyroptosis-associated phenotype in Kawasaki disease (KD), interacting with one another and contributing to the infiltration of neutrophils and T and B cells. (Created with BioRender.com).
Pyroptosis and macrophage-mediated inflammation in KD
Unlike silent apoptosis, pyroptosis is characterized by the abundant release of damage-associated molecular patterns (DAMP) and inflammatory cytokines, such as high mobility group protein B1 (HMGB1) and IL-1β. The inflammation in KD is intricate, involving the activation of immune cells that infiltrate the arterial wall, leading to necrotizing arteritis. Notably, in some severe cases among children, KD has been associated with macrophage activation syndrome, marked by significantly elevated levels of pyroptosis-related proteins, including apoptosis-associated speck-like protein containing CARD (ASC), caspase-1, IL-1β, IL-18, GSDMD, and LDH in their serum (Table 2) (23). Many studies have emphasized the crucial role of monocytes and macrophages in the release of cytokines and the resultant destructive inflammation in patients with KD (12, 24). Here, we propose the potential involvement of macrophage pyroptosis in KD, which may contribute to the development of the inflammatory microenvironment.
Macrophages exert a double-sided effect in patients with KD. Macrophages can scavenge pathogenic microorganisms, thereby protecting children from immune deregulation caused by infections (25). However, an excessive presence of pro-inflammatory macrophages can secret cytokines and matrix-degrading enzymes, contributing to inflammation infiltration and destabilization of vascular smooth muscle cells. Researchers have demonstrated the central role of macrophages among the immune cells in KD through single-cell RNA sequencing. They identified that monocytes/macrophages are the primary source of dysregulated immune gene expression in KD, including the upregulation of various cytokines and drug targets, such as IL1B and TNF (26).
Importantly, NLRP3 inflammasome activation in monocytes and macrophages has been observed in both in vitro and in vivo studies. Liu and colleagues were the first to report the activation of the NLRP3 inflammasome in peripheral blood mononuclear cells (PBMCs) from patients with KD (21). They found that NLRP3 and other pyroptosis-related genes were significantly upregulated in KD, particularly in patients with coronary artery lesions (CALs). Kuo et al. were the first to report the upregulation of pyroptosis-related caspases in leukocytes of KD, including caspase-1, -4, and -5 (27). Intriguingly, the levels of these pyroptosis-related caspases returned to normal after effective treatment with standard-of-care medications. These findings suggest that pyroptosis in peripheral leukocytes plays a critical role in the inflammatory pathogenesis of KD.
However, the susceptibility mechanisms of the activation of the NLRP3 inflammasome in KD remain unclear. Inositol-triphosphate 3-kinase C (ITPKC), a widely known KD-associated gene, has been shown to regulate NLRP3 by modulating intracellular calcium levels (28). Animal models based on Itpkc-deficient mice further support this perspective. Recently, Jin et al. revealed significant crosstalk between neutrophil extracellular traps (NETs) and PBMC pyroptosis (14). They found that elevated levels of NETs in KD patients were sufficient to induce the activation of the NLRP3 inflammasome via a ROS-dependent signaling pathway, resulting in the pyroptosis of PBMCs, particularly monocytes, and the release of inflammatory mediators such as IL-1β. These findings indicate a close correlation between pyroptosis and the inflammatory factors derived from monocytes and macrophages.
In vivo studies have demonstrated that pyroptosis plays a significant role in macrophage-mediated inflammation (Figure 2). Currently, there are three main types of animal models for KD, including Lactobacillus casei cell wall extract (LCWE), Candida albicans water-soluble fraction (CAWS), and nucleotide-binding oligomerization domain containing 1 (Nod1) ligand-induced mice (10). Among these, the LCWE-induced animal models are regarded to have pathological changes that most closely resemble those seen in children with KD (29). Moreover, both the NLRP3 inflammasome and the pyroptosis pathway are crucial in the progression of coronary lesions. In LCWE-induced animal models, increased macrophage-derived IL-1β maturation and secretion have been observed (30).
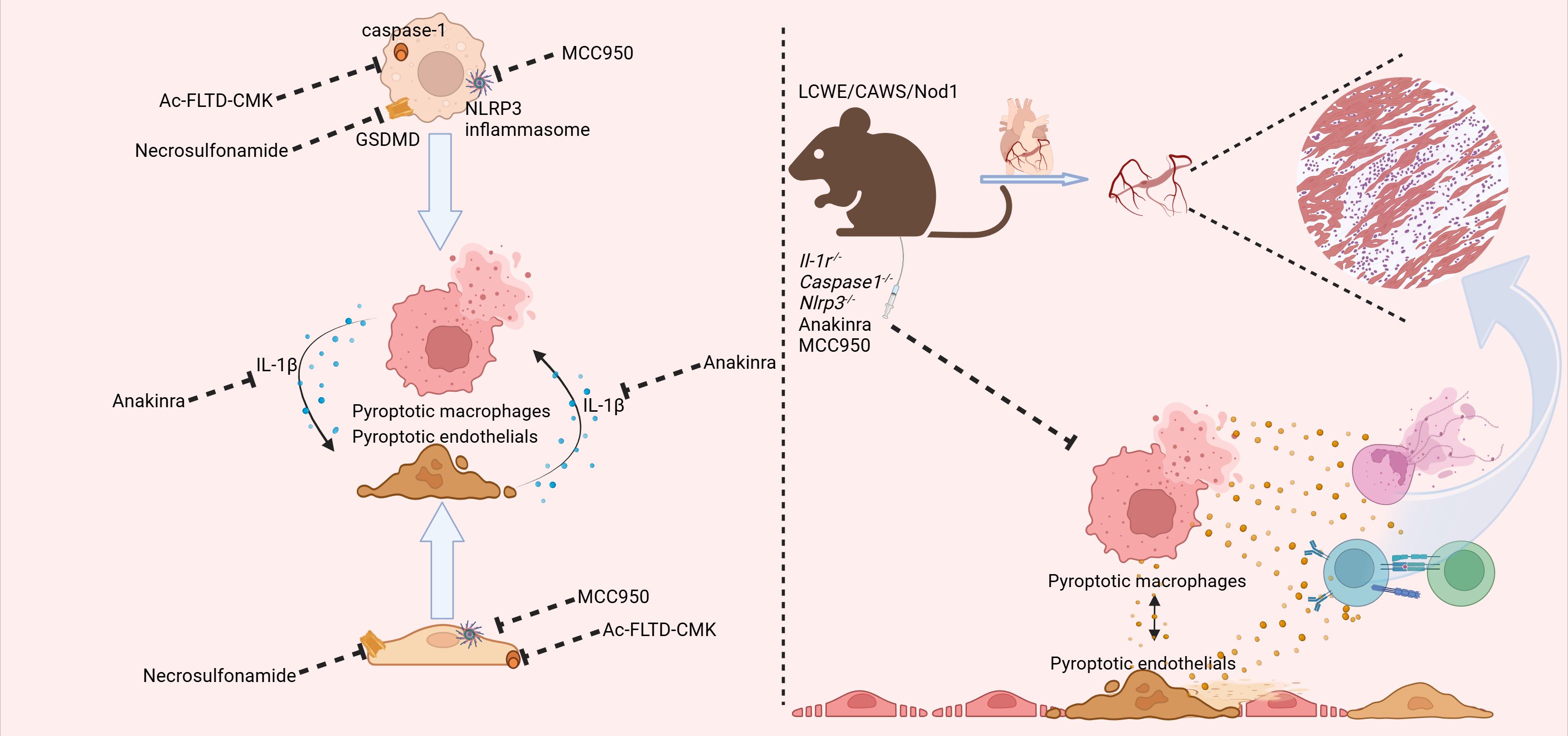
Figure 2. The findings from both in vivo and in vitro studies have converged to highlight the pivotal role of pyroptosis-related pathways in the pathogenesis of KD. In the realm of in vitro research, the employment of the caspase1 inhibitor N-acetyl-Phe-Leu-Thr-Asp-chloromethylketone (Ac-FLTD-CMK), the gasdermin D (GSDMD) inhibitor necrosulfamide, and the NLR family pyrin domain containing 3 (NLRP3) inhibitor MCC950 has collectively demonstrated the ability to suppress pyroptosis within macrophages and endothelial cells. This suppression, in turn, serves to temper the subsequent inflammatory responses. Additionally, the interleukin-1 receptor antagonist anakinra has been shown to inhibit the downstream effects of interleukin-1 beta (IL-1β), thereby dampening the amplification of the inflammatory cascade between macrophages and endothelial cells. In the context of in vivo experimentation, mice deficient in Il-1r, Caspase-1, and Nlrp3 exhibited a marked attenuation in the progression of coronary inflammation, as observed in KD mice. Moreover, both anakinra and MCC950 were found to exert similar protective effects, further corroborating the significance of targeting these pathways in the treatment of KD. (Created with BioRender.com).
Additionally, these biological processes were found to be highly dependent on the activation of the NLRP3 inflammasome and caspase-1 in macrophages. Both caspase-1-deficient and IL-1 receptor-deficient mice exhibited reduced coronary lesions in response to LCWE, further suggesting the potential role of macrophage pyroptosis in KD (12, 31). Subsequent investigation revealed that deficiency in caspase-1 and NLRP3 in macrophages could inhibit LCWE-induced abdominal aortic aneurysm formation. Furthermore, in addition to IL-1β, IL-1α also contributed to the pathogenesis of KD, highlighting the significance of macrophage pyroptosis and its crucial role in KD. Notably, pyroptosis-derived IL-1β also played an essential role in the development of coronary injury in a CAWS-induced KD mouse model (32). Takahashi and colleagues found that CAWS induced the expression of Dectin-2 in macrophages, which activates the NF-κB pathway, leading to the assembly of the inflammasome and the subsequent cleavage of caspase-1 (33). Both IL-1β and NLRP3 deficiency could protect mice from the induction of vasculitis in these models. In the Nod1 ligand mouse model, a significant infiltration of macrophages into the coronary arteries was observed (34, 35). Furthermore, specific depletion of macrophages significantly attenuated Nod1 ligand-induced vasculitis. Surprisingly, the concentration of circulating IL-1β was significantly higher and correlated with macrophage-mediated inflammation (36).
In all three models, the activation of pyroptosis-related pathways in macrophages played a crucial role. Nevertheless, few research studies have investigated the role of GSDMD, the executor of pyroptosis, in these animal models. More in-depth investigation on macrophage pyroptosis is warranted in the future.
Pyroptosis and endothelial cell injuries in KD
The injury of endothelial cells is one of the hallmark pathophysiological processes in KD. In addition to the previously discussed relationship between pyroptosis and macrophage-mediated inflammation, pyroptosis occurring in endothelial cells can directly lead to their destruction, contributing to the formation of coronary aneurysm. Jia et al. were the first to demonstrate that KD serum induces macrophages to trigger pyroptosis in human umbilical vein endothelial cells (HUVECs), featured by the activation of the NLRP3 inflammasome and the cleavage of GSDMD and IL-1β (13). Notably, GSDMD-related pyroptosis inhibitors such as necrosulfonamide significantly mitigated the release of IL-1β induced by endothelial cell pyroptosis.
Furthermore, subsequent experiments confirmed that the HMGB1/RAGE/cathepsin B signaling pathway is involved in activating the NLRP3 inflammasome in endothelial cells, leading to pyroptosis. These in vitro studies clearly demonstrated that endothelial cells can undergo pyroptosis in the context of KD. Subsequently, the authors found that inhibiting the NLRP3 inflammasome attenuated the pyroptosis of endothelial cells in CAWS-induced animal models. Thus, endothelial pyroptosis may help explain the endothelial injury observed in coronary arteries in KD. Furthermore, in the Nod1 ligand mouse model, delivery of the Nod1 ligand specifically activates vascular cells, such as endothelial cells, leading to elevated production of IL-1β. This, in turn, results in the recruitment of inflammatory cells and the expansion of the inflammatory cascade, suggesting a close correlation between pyroptosis and endothelial cells (36, 37).
In fact, animal models with endothelial cell conditional knockout of pyroptosis-related proteins are needed to accurately illustrate the role of pyroptosis in endothelial cell injuries in KD. Moreover, direct immunohistochemical staining of the pyroptosis pathway in endothelial cells from patients with KD is also needed. Therefore, further improvements in this area of research are necessary.
Potential interventions targeted pyroptosis
Although the use of high-dose IVIG together with aspirin has significantly reduced the risk of developing coronary artery lesions in patients with KD, approximately 20% of patients still exhibit a poor response to the above treatment and remain at higher risk for coronary artery complications (9). Given the crucial role of pyroptosis in the progression of KD, targeting this process may represent a promising treatment strategy, particularly for those with IVIG-resistant KD (Table 2).
IL-1β and IL-18 are important inflammatory factors in the pyroptosis pathway. Many studies have demonstrated that blocking the IL-1β pathway may serve as a valid therapeutic option for KD. Insights from mouse models suggested that IL-1α, IL-1β, and their receptors are essential for the development of KD-associated vasculitis (12, 30, 31, 38). Anakinra, a recombinant human interleukin-1 receptor (IL-1R) antagonist, has previously been used for the treatment of macrophage activation syndrome. Notably, a growing body of case reports highlights the successful use of anakinra in patients with IVIG-resistant KD (39–42). In a study conducted by Isabelle et al., 11 patients with KD who did not respond to immunoglobulin were treated with anakinra, resulting in significant improvements in their body temperature, systemic inflammatory markers, and coronary artery dilation (43). An open-label Phase IIa clinical trial further evaluated the safety of anakinra in patients with IVIG-resistant KD (44). The results from a Phase I/IIa trial by Jin et al. also confirmed that anakinra is safe for clinical use in both infants and children with KD and coronary artery aneurysms (45). Based on these findings, we believe that anakinra may have therapeutic effects in patients with refractory KD, particularly in severe complications such as Kawasaki shock syndrome. It could also help alleviate coronary artery dilation and aneurysms or prevent their deterioration. As a result, anakinra is currently being evaluated in clinical trials, and future randomized controlled trials with longer durations and larger sample sizes are necessary to verify its safety and effectiveness. While elevated levels of IL-18 in the serum of patients with KD have been observed, no animal or clinical studies targeting IL-18 have been performed (46). Recombinant human IL-18 binding protein, a natural IL-18 inhibitor, has shown promise in many inflammation-associated diseases and could potentially be beneficial in the treatment of KD (47).
The activation of NLRP3 is a pivotal event in the pyroptosis pathway. Theoretically, directly targeting NLRP3 with small molecule inhibitors could offer additional therapeutic benefits for KD by blocking downstream effects of pyroptosis, including the release of cellular contents and the production of IL-1β and IL-18. For example, MCC590, an inhibitor of NLRP3, has been shown to significantly alleviate the coronary artery inflammation induced by CAWS (33). Nevertheless, it is important to note that all studies to date have been conducted in animal models, with no clinical trial yet performed. In addition to the NLRP3 inflammasome, other components of the pyroptosis pathway warrant attention. Mice with a caspase-1 deficiency were protected from LCWE-induced coronary artery lesions. N-acetyl-Phe-Leu-Thr-Asp-chloromethylketone (Ac-FLTD-CMK), a carefully designed selective inhibitor of caspase-1, -4, -5, and -11, has been proven to effectively alleviate pyroptosis in endothelial cells in vitro (13). However, there is a paucity of research examining the effects of targeting caspases on KD in in vivo studies. Similarly, necrosulfonamide, a small molecular inhibitor of GSDMD, has demonstrated the ability to attenuate cell death and reduce IL-1β release in in vitro studies (13). Thus, more in-depth studies are needed to systematically explore the effects of targeting caspases and GSDMD in vivo, progressing from animal models to clinical trials.
Conclusion
Pyroptosis is a distinct inflammatory death pattern, characterized by the release of inflammatory cytokines and rupture of membranes. Existing literature indicates that pyroptosis in various cell types, including macrophages and endothelial cells, plays a significant role in the progression of KD. In this review, we elucidate the molecular mechanisms underlying pyroptosis in KD and present new insights into pyroptosis-targeting therapeutic strategies. Our goal is to enhance the efficacy of current KD therapies, particularly for patients who are resistant to intravenous immunoglobulin therapy.
Author contributions
X-YH: Writing – original draft, Writing – review & editing. H-RQ: Writing – review & editing.
Funding
The author(s) declare that no financial support was received for the research and/or publication of this article.
Conflict of interest
The authors declare that the research was conducted in the absence of any commercial or financial relationships that could be construed as a potential conflict of interest.
Generative AI statement
The author(s) declare that no Generative AI was used in the creation of this manuscript.
Publisher’s note
All claims expressed in this article are solely those of the authors and do not necessarily represent those of their affiliated organizations, or those of the publisher, the editors and the reviewers. Any product that may be evaluated in this article, or claim that may be made by its manufacturer, is not guaranteed or endorsed by the publisher.
References
1. Kawasaki T, Kosaki F, Okawa S, Shigematsu I, Yanagawa H. A new infantile acute febrile mucocutaneous lymph node syndrome (MLNS) prevailing in Japan. Pediatrics. (1974) 54:271–6. doi: 10.1542/peds.54.3.271
2. McCrindle BW, Rowley AH, Newburger JW, Burns JC, Bolger AF, Gewitz M, et al. Diagnosis, treatment, and long-term management of kawasaki disease: A scientific statement for health professionals from the american heart association. Circulation. (2017) 135:e927–e99. doi: 10.1161/CIR.0000000000000484
3. Jone PN, Tremoulet A, Choueiter N, Dominguez SR, Harahsheh AS, Mitani Y, et al. Update on diagnosis and management of kawasaki disease: A scientific statement from the american heart association. Circulation. (2024) 150:e481–500. doi: 10.1161/CIR.0000000000001295
4. Singh S, Vignesh P, Burgner D. The epidemiology of Kawasaki disease: a global update. Arch Dis childhood. (2015) 100:1084–8. doi: 10.1136/archdischild-2014-307536
5. Son MBF, Newburger JW. Kawasaki disease. Pediatr review. (2018) 39:78–90. doi: 10.1542/pir.2016-0182
6. Son MBF, Newburger JW. SARS-coV-2-related mitigation measures and insights into kawasaki disease. JAMA pediatrics. (2022) 176:1167–8. doi: 10.1001/jamapediatrics.2022.3765
7. Vasudeva R, Poku FA, Thommana M, Parmar G, Umscheid J, Parmar N, et al. Trends and resource utilization in kawasaki disease hospitalizations in the United States, 2008-2017. Hosp pediatrics. (2022) 12:257–66. doi: 10.1542/hpeds.2021-006142
8. Tremoulet AH, Best BM, Song S, Wang S, Corinaldesi E, Eichenfield JR, et al. Resistance to intravenous immunoglobulin in children with Kawasaki disease. J pediatrics. (2008) 153:117–21. doi: 10.1016/j.jpeds.2007.12.021
9. Makino N, Nakamura Y, Yashiro M, Kosami K, Matsubara Y, Ae R, et al. Nationwide epidemiologic survey of Kawasaki disease in Japan, 2015-2016. Pediatr international: Off J Japan Pediatr Society. (2019) 61:397–403. doi: 10.1111/ped.2019.61.issue-4
10. Noval Rivas M, Arditi M. Kawasaki disease: pathophysiology and insights from mouse models. Nat Rev Rheumatol. (2020) 16:391–405. doi: 10.1038/s41584-020-0426-0
11. Leung DY, Cotran RS, Kurt-Jones E, Burns JC, Newburger JW, Pober JS. Endothelial cell activation and high interleukin-1 secretion in the pathogenesis of acute Kawasaki disease. Lancet (London England). (1989) 2:1298–302. doi: 10.1016/S0140-6736(89)91910-7
12. Lee Y, Schulte DJ, Shimada K, Chen S, Crother TR, Chiba N, et al. Interleukin-1β is crucial for the induction of coronary artery inflammation in a mouse model of Kawasaki disease. Circulation. (2012) 125:1542–50. doi: 10.1161/CIRCULATIONAHA.111.072769
13. Jia C, Zhang J, Chen H, Zhuge Y, Chen H, Qian F, et al. Endothelial cell pyroptosis plays an important role in Kawasaki disease via HMGB1/RAGE/cathespin B signaling pathway and NLRP3 inflammasome activation. Cell Death disease. (2019) 10:778. doi: 10.1038/s41419-019-2021-3
14. Jin J, Zhao Y, Fang Y, Pan Y, Wang P, Fan Z, et al. Neutrophil extracellular traps promote the activation of the NLRP3 inflammasome and PBMCs pyroptosis via the ROS-dependent signaling pathway in Kawasaki disease. Int immunopharmacology. (2025) 145:113783. doi: 10.1016/j.intimp.2024.113783
15. Godfred-Cato S, Bryant B, Leung J, Oster ME, Conklin L, Abrams J, et al. COVID-19-associated multisystem inflammatory syndrome in children - United States, march-july 2020. MMWR Morbidity mortality weekly Rep. (2020) 69:1074–80. doi: 10.15585/mmwr.mm6932e2
16. Feeney CC, Ajagbe OA, Suryadevara M. Yersinia enterocolitica infection presenting as incomplete kawasaki disease: 2 cases and a review of the literature. J Pediatr Infect Dis Society. (2021) 10:217–9. doi: 10.1093/jpids/piaa016
17. Yu P, Zhang X, Liu N, Tang L, Peng C, Chen X. Pyroptosis: mechanisms and diseases. Signal transduction targeted Ther. (2021) 6:128. doi: 10.1038/s41392-021-00507-5
18. Shi J, Gao W, Shao F. Pyroptosis: gasdermin-mediated programmed necrotic cell death. Trends Biochem Sci. (2017) 42:245–54. doi: 10.1016/j.tibs.2016.10.004
19. Hara T, Yamamura K, Sakai Y. The up-to-date pathophysiology of Kawasaki disease. Clin Trans Immunol. (2021) 10:e1284. doi: 10.1002/cti2.v10.5
20. Shahi A, Afzali S, Firoozi Z, Mohaghegh P, Moravej A, Hosseinipour A, et al. Potential roles of NLRP3 inflammasome in the pathogenesis of Kawasaki disease. J Cell Physiol. (2023) 238:513–32. doi: 10.1002/jcp.v238.3
21. Liu LP, Yuan YH, He XH, Chen M, Peng DX, Xu W, et al. Expression of Nod-like receptor protein 3 inflammasome in peripheral blood mononuclear cells of children with Kawasaki disease in the acute stage. Zhongguo dang dai er ke za zhi = Chin J Contemp pediatrics. (2019) 21:992–7. doi: 10.7499/j.issn.1008-8830.2019.10.008
22. Si F, Lu Y, Wen Y, Chen T, Zhang Y, Yang Y. Cathelicidin (LL-37) causes expression of inflammatory factors in coronary artery endothelial cells of Kawasaki disease by activating TLR4-NF-κB-NLRP3 signaling. Immunity Inflammation disease. (2023) 11:e1032. doi: 10.1002/iid3.v11.9
23. Wen YN, Chen J, Liu F, Ding Y, Yin W. Clinical features of Kawasaki disease complicated by macrophage activation syndrome: an analysis of 27 cases. Zhongguo dang dai er ke za zhi = Chin J Contemp pediatrics. (2023) 25:572–8. doi: 10.7499/j.issn.1008-8830.2302015
24. Sun Y, Tao Y, Geng Z, Zheng F, Wang Y, Wang Y, et al. The activation of CaN/NFAT signaling pathway in macrophages aggravated Lactobacillus casei cell wall extract-induced Kawasaki disease vasculitis. Cytokine. (2023) 169:156304. doi: 10.1016/j.cyto.2023.156304
25. Shapouri-Moghaddam A, Mohammadian S, Vazini H, Taghadosi M, Esmaeili SA, Mardani F, et al. Macrophage plasticity, polarization, and function in health and disease. J Cell Physiol. (2018) 233:6425–40. doi: 10.1002/jcp.v233.9
26. Wang Z, Xie L, Ding G, Song S, Chen L, Li G, et al. Single-cell RNA sequencing of peripheral blood mononuclear cells from acute Kawasaki disease patients. Nat Commun. (2021) 12:5444. doi: 10.1038/s41467-021-25771-5
27. Kuo KC, Yang YL, Lo MH, Cai XY, Guo MM, Kuo HC, et al. Increased expression of pyroptosis in leukocytes of patients with kawasaki disease. Diagnostics (Basel Switzerland). (2021) 11. doi: 10.3390/diagnostics11112035
28. Alphonse MP, Duong TT, Shumitzu C, Hoang TL, McCrindle BW, Franco A, et al. Inositol-triphosphate 3-kinase C mediates inflammasome activation and treatment response in kawasaki disease. J Immunol (Baltimore Md: 1950). (2016) 197:3481–9. doi: 10.4049/jimmunol.1600388
29. Lehman TJ, Walker SM, Mahnovski V, McCurdy D. Coronary arteritis in mice following the systemic injection of group B Lactobacillus casei cell walls in aqueous suspension. Arthritis rheumatism. (1985) 28:652–9. doi: 10.1002/art.1780280609
30. Wakita D, Kurashima Y, Crother TR, Noval Rivas M, Lee Y, Chen S, et al. Role of interleukin-1 signaling in a mouse model of kawasaki disease-associated abdominal aortic aneurysm. Arteriosclerosis thrombosis Vasc Biol. (2016) 36:886–97. doi: 10.1161/ATVBAHA.115.307072
31. Gorelik M, Lee Y, Abe M, Andrews T, Davis L, Patterson J, et al. IL-1 receptor antagonist, anakinra, prevents myocardial dysfunction in a mouse model of Kawasaki disease vasculitis and myocarditis. Clin Exp Immunol. (2019) 198:101–10. doi: 10.1111/cei.13314
32. Murata H. Experimental candida-induced arteritis in mice. Relation to arteritis in the mucocutaneous lymph node syndrome. Microbiol Immunol. (1979) 23:825–31. doi: 10.1111/j.1348-0421.1979.tb02815.x
33. Anzai F, Watanabe S, Kimura H, Kamata R, Karasawa T, Komada T, et al. Crucial role of NLRP3 inflammasome in a murine model of Kawasaki disease. J Mol Cell Cardiol. (2020) 138:185–96. doi: 10.1016/j.yjmcc.2019.11.158
34. Motomura Y, Kanno S, Asano K, Tanaka M, Hasegawa Y, Katagiri H, et al. Identification of pathogenic cardiac CD11c+ Macrophages in nod1-mediated acute coronary arteritis. Arteriosclerosis thrombosis Vasc Biol. (2015) 35:1423–33. doi: 10.1161/ATVBAHA.114.304846
35. Nishio H, Kanno S, Onoyama S, Ikeda K, Tanaka T, Kusuhara K, et al. Nod1 ligands induce site-specific vascular inflammation. Arteriosclerosis thrombosis Vasc Biol. (2011) 31:1093–9. doi: 10.1161/ATVBAHA.110.216325
36. Ohashi R, Fukazawa R, Watanabe M, Hashimoto K, Suzuki N, Nagi-Miura N, et al. Characterization of a murine model with arteritis induced by Nod1 ligand, FK565: A comparative study with a CAWS-induced model. Modern Rheumatol. (2017) 27:1024–30. doi: 10.1080/14397595.2017.1287150
37. Yang Y, Wang N, Wang Z, Zhao M, Chen L, Shi Z. Protective role of forsythoside B in Kawasaki disease-induced cardiac injury: Inhibition of pyroptosis via the SIRT1-NF-κB-p65 signaling pathway. Chemico-biological interactions. (2024) 392:110953. doi: 10.1016/j.cbi.2024.110953
38. Lee Y, Wakita D, Dagvadorj J, Shimada K, Chen S, Huang G, et al. IL-1 signaling is critically required in stromal cells in kawasaki disease vasculitis mouse model: role of both IL-1α and IL-1β. Arteriosclerosis thrombosis Vasc Biol. (2015) 35:2605–16. doi: 10.1161/ATVBAHA.115.306475
39. Shafferman A, Birmingham JD, Cron RQ. High dose Anakinra for treatment of severe neonatal Kawasaki disease: a case report. Pediatr Rheumatol Online J. (2014) 12:26. doi: 10.1186/1546-0096-12-26
40. Guillaume MP, Reumaux H, Dubos F. Usefulness and safety of anakinra in refractory Kawasaki disease complicated by coronary artery aneurysm. Cardiol young. (2018) 28:739–42. doi: 10.1017/S1047951117002864
41. Sánchez-Manubens J, Gelman A, Franch N, Teodoro S, Palacios JR, Rudi N, et al. A child with resistant Kawasaki disease successfully treated with anakinra: a case report. BMC pediatrics. (2017) 17:102. doi: 10.1186/s12887-017-0852-6
42. Blonz G, Lacroix S, Benbrik N, Warin-Fresse K, Masseau A, Trewick D, et al. Severe late-onset kawasaki disease successfully treated with anakinra. J Clin rheumatology: Pract Rep rheumatic musculoskeletal diseases. (2020) 26:e42–e3. doi: 10.1097/RHU.0000000000000814
43. Kone-Paut I, Cimaz R, Herberg J, Bates O, Carbasse A, Saulnier JP, et al. The use of interleukin 1 receptor antagonist (anakinra) in Kawasaki disease: A retrospective cases series. Autoimmun Rev. (2018) 17:768–74. doi: 10.1016/j.autrev.2018.01.024
44. Koné-Paut I, Tellier S, Belot A, Brochard K, Guitton C, Marie I, et al. Phase II open label study of anakinra in intravenous immunoglobulin-resistant kawasaki disease. Arthritis Rheumatol (Hoboken NJ). (2021) 73:151–61. doi: 10.1002/art.41481
45. Yang J, Jain S, Capparelli EV, Best BM, Son MB, Baker A, et al. Anakinra treatment in patients with acute kawasaki disease with coronary artery aneurysms: A phase I/IIa trial. J pediatrics. (2022) 243:173–80.e8. doi: 10.1016/j.jpeds.2021.12.035
46. Alphonse MP, Duong TT, Tam S, Yeung RSM. Mercury increases IL-1β and IL-18 secretion and intensifies coronary arteritis in an animal model of Kawasaki disease. Front Immunol. (2023) 14:1126154. doi: 10.3389/fimmu.2023.1126154
47. Zhou T, Damsky W, Weizman OE, McGeary MK, Hartmann KP, Rosen CE, et al. IL-18BP is a secreted immune checkpoint and barrier to IL-18 immunotherapy. Nature. (2020) 583:609–14. doi: 10.1038/s41586-020-2422-6
48. Li M, Liu D, Cheng Z, Zhou X, Chen Z, Liu R, et al. Serum NLRP3: A potential marker for identifying high-risk coronary arterial aneurysm in children with Kawasaki disease. Cytokine. (2024) 180:156667. doi: 10.1016/j.cyto.2024.156667
49. Li S, Zhou Y, Wen Y, Wu Y, Liu F, Ding Y. Role of pyroptosis in IVIG-resistant kawasaki disease and the establishment of a new predictive model. J Inflammation Res. (2024) 17:10999–1008. doi: 10.2147/JIR.S490095
50. Krasovec S, Bezrodnik L, Gaillard MI, Carabajal P, Ginaca A, Vainstein E. Kawasaki disease. Immunol Eval 26 cases]. Medicina. (2001) 61:8–14.
51. Weng KP, Hsieh KS, Ho TY, Huang SH, Lai CR, Chiu YT, et al. IL-1B polymorphism in association with initial intravenous immunoglobulin treatment failure in Taiwanese children with Kawasaki disease. Circ journal: Off J Japanese Circ Society. (2010) 74:544–51. doi: 10.1253/circj.CJ-09-0664
52. Kim SK, Kang SW, Chung JH, Lee JS, Park HK, Yoon KL, et al. Coding single-nucleotide polymorphisms of interleukin-1 gene cluster are not associated with Kawasaki disease in the Korean population. Pediatr Cardiol. (2011) 32:381–5. doi: 10.1007/s00246-010-9858-7
53. Xie Y, Han B. Exploring the relationship between pyroptosis, infiltrating immune cells and Kawasaki disease with resistance to intravenous immunoglobulin (IVIG) via bioinformatic analysis. Immunobiology. (2022) 227:152261. doi: 10.1016/j.imbio.2022.152261
54. Porritt RA, Zemmour D, Abe M, Lee Y, Narayanan M, Carvalho TT, et al. NLRP3 inflammasome mediates immune-stromal interactions in vasculitis. Circ Res. (2021) 129:e183–200. doi: 10.1161/CIRCRESAHA.121.319153
55. Wang WT, He M, Shimizu C, Croker BA, Hoffman HM, Tremoulet AH, et al. Inflammasome activation in children with kawasaki disease and multisystem inflammatory syndrome. Arteriosclerosis thrombosis Vasc Biol. (2021) 41:2509–11. doi: 10.1161/ATVBAHA.121.316210
56. Huang YH, Lo MH, Cai XY, Kuo HC. Epigenetic hypomethylation and upregulation of NLRC4 and NLRP12 in Kawasaki disease. Oncotarget. (2018) 9:18939–48. doi: 10.18632/oncotarget.24851
Keywords: Kawasaki disease, pyroptosis, inflammation, mechanism, intervention
Citation: Han X-Y and Qi H-R (2025) Pyroptosis in Kawasaki disease: from mechanisms to targeted interventions. Front. Immunol. 16:1566985. doi: 10.3389/fimmu.2025.1566985
Received: 26 January 2025; Accepted: 26 March 2025;
Published: 16 April 2025.
Edited by:
Ilenia Di Cola, University of L’Aquila, ItalyReviewed by:
Madalina Adriana Bordea, University of Medicine and Pharmacy Iuliu Hatieganu, RomaniaCopyright © 2025 Han and Qi. This is an open-access article distributed under the terms of the Creative Commons Attribution License (CC BY). The use, distribution or reproduction in other forums is permitted, provided the original author(s) and the copyright owner(s) are credited and that the original publication in this journal is cited, in accordance with accepted academic practice. No use, distribution or reproduction is permitted which does not comply with these terms.
*Correspondence: Xiang-Yu Han, aHh5d2lzZUAxNjMuY29t
†These authors have contributed equally to this work