- 1Mohammed VI Faculty of Medicine, Mohammed VI University of Sciences and Health (UM6SS), Rabat, Morocco
- 2Laboratory of Neurooncology, Oncogenetic and Personalized Medicine, Faculty of Medicine, Mohammed VI University of Sciences and Health (UM6SS), Casablanca, Morocco
- 3Department of Radiotherapy, International University Hospital Cheikh Khalifa, Casablanca, Morocco
- 4Immunopathology-Immunotherapy-Immunomonitoring Laboratory, Faculty of Medicine, Mohammed VI University of Sciences and Health (UM6SS), Casablanca, Morocco
- 5Department of Gynecology and Obstetrics, Mohammed VI International University Hospital, Bouskoura, Morocco
- 6Laboratory of Immunogenetics and Human Pathologies, Faculty of Medicine and Pharmacy, Casablanca, Morocco
Locally advanced cervical cancer remains a significant therapeutic challenge, with high rates of recurrence and metastasis despite advances in chemoradiation. Immunotherapy, particularly immune checkpoint inhibitors targeting the PD-1/PD-L1 axis, has emerged as a promising strategy to enhance treatment efficacy. This review explores the integration of immunotherapy with standard chemoradiation, highlighting the potential of PD-1 inhibitors, such as pembrolizumab, in improving progression-free survival (PFS) among high-risk patients. Furthermore, the role of predictive biomarkers, including microsatellite instability (MSI) and tumor mutational burden (TMB), is examined to refine patient selection and personalize therapeutic approaches. Emerging strategies, including the use of nivolumab, ipilimumab, and maintenance immunotherapy, are also discussed. While preliminary clinical data are encouraging, further research is required to optimize treatment combinations, establish robust patient selection criteria, and enhance long-term outcomes in cervical cancer management.
1 Introduction
Cervical cancer, particularly in its locally advanced stages, remains one of the most challenging cancers to treat. While significant advances have been made in early detection and treatment modalities, the prognosis for patients with advanced cervical cancer remains poor (1, 2). The primary treatment for cervical cancer involves a combination of chemoradiation, which includes the use of cisplatin-based chemotherapy alongside external beam radiation (3, 4). Chemoradiation has proven effective in reducing tumor burden, improving survival rates, and in many cases, achieving remission. However, despite these advances, a significant proportion of patients still experience recurrence or metastasis. This underscores a crucial limitation of current therapeutic approaches and the need for more targeted and effective interventions to improve long-term outcomes for these patients.
The rationale for integrating immunotherapy in cervical cancer treatment regimens is deeply rooted in the intricate interactions between the host immune dynamics, human papilloma virus (HPV), and cervical cancer cells (5). Because of its viral origin, as most cases are driven by infection with HPV, cervical cancer exhibit a specific immune profile associated in 20% of cases with high tumor mutational burden (6). High-risk HPV types cause 90-100% of cervical cancer, HPV 16/18 causes 70% of cervical cancer, and HPV 16 alone causes 50% of cervical cancer (7). It is noteworthy that even if the majority of cervical cancer are caused by HPV, a considerable percentage of cervical cancer cases (range 8.4–13.9%) are HPV independent (8, 9). Furthermore, HPV independent cervical tumors are associated with advanced stages and worse clinical outcomes (10–15).
Persistent high-risk human papillomavirus (HPV) infection, particularly types 16 and 18, is the main driving force of cervical carcinogenesis. The viral oncoproteins E6 and E7 promote malignant transformation and immune evasion by inactivating the tumor suppressor proteins p53 and retinoblastoma (pRb) (16, 17). The tumor cells infected with high-risk HPV downmodulate the expression of MHC class I, which allows them to escape recognition by cytotoxic T-cells (18). Furthermore, it has been shown that PD-L1’s expression is regulated by E6 protein through a miR-143/HIF-1a axis (19).
The immunosuppressive microenvironment within cervical cancer, characterized by regulatory T cells (Tregs) and myeloid-derived suppressor cells (MDSCs), makes it an excellent target for immunotherapy (18). Clinical trials have demonstrated that immune checkpoint inhibitors (ICIs) can restore T-cell activity and enhance antitumor immunity by targeting PD-1/PD-L1 and CTLA-4 (20–24).
Recently immune signatures for treatment prediction and cervical cancer prognosis have been established (25–29). The potential response predictors identified include, PD-L1-immunoreactive (IR) area, PD-L2, CD8, FGF-basic, IL-7, IL-8, IL-12p40, IL-15, and TNF-alpha (30). It has been shown that impaired TILs and disturbed immune mediators are prominent contributor to therapeutic failure (30). Indeed, patients belonging to the responders group show increased TILs with functional polarization of CD4 T cell populations; Th1, Th2, Th17, and Treg. However, non-responders group exhibit elevated PD-1 scores, CD8+ and PD-L2+ TILs, CD68+ macrophages, and PD-L1 immune reactivity.
In this review we shed light on the potential of immunotherapy-based treatment in locally advanced cervical cancer. Additionally, we cover recent advances in identifying biomarkers for cervical cancer diagnosis and prognosis. We also present a succinct summary of emerging immunotherapy approaches. Overall, this review emphasizes the current standing of immunotherapy in locally advanced cervical cancer.
2 Immunotherapy: a promising strategy for cervical cancer treatment
In recent years, the incorporation of immunotherapy into treatment regimens has emerged as a promising strategy in the fight against cervical cancer (31). Immunotherapy aims to enhance the body’s natural immune response to cancer cells, which are often able to evade detection by the immune system. This evasion occurs through various mechanisms, including the upregulation of immune checkpoint pathways that suppress immune activity (32). The immune microenvironment in cervical cancer is shaped by the interplay between HPV-induced inflammation, immune suppression, and the tumor’s ability to evade immune surveillance (33, 34). This environment often includes the accumulation of regulatory T-cells (Tregs), myeloid-derived suppressor cells (MDSCs), and tumor-associated macrophages (TAMs), all of which contribute to the suppression of antitumor immune responses (35). The presence of these immunosuppressive cells within the TME limits the effectiveness of conventional therapies and presents a barrier to successful treatment.
One of the key immune checkpoints involved in this process is the PD-1/PD-L1 axis, which is upregulated in response to persistent HPV infection (36, 37). Tumor cells exploit this pathway to suppress T-cell activation, preventing the immune system from attacking the tumor effectively. By blocking PD-1 or PD-L1, immune checkpoint inhibitors can reverse this suppression, restoring the ability of T-cells to mount an effective anti-tumor response. Clinical studies have demonstrated that PD-1 inhibitors, either as monotherapies or in combination with other treatments, can significantly improve response rates and progression-free survival in patients with metastatic cervical cancer (38).
In addition to PD-1/PD-L1 inhibitors, other immunotherapy strategies, such as immune checkpoint inhibitors targeting CTLA-4, have also been explored in cervical cancer (39). While PD-1/PD-L1 inhibitors have garnered the most attention, the potential for combining different immune checkpoint inhibitors to overcome multiple mechanisms of immune evasion is an area of active research.
3 Combination of immunotherapy and chemoradiation: synergistic effects
Recent studies have suggested that combining immunotherapy with chemoradiation may enhance the overall anti-tumor immune response in cervical cancer. Cisplatin, the chemotherapy agent commonly used in chemoradiation regimens for cervical cancer, has been shown to have immunomodulatory effects. Cisplatin can increase the presentation of tumor antigens by promoting dendritic cell recruitment to the tumor site and enhancing the activation of CD8+ cytotoxic T-cells (40, 41). This immune activation occurs alongside the direct cytotoxic effects of cisplatin on tumor cells, which leads to tumor cell death and the release of tumor-associated antigens that can stimulate the immune system.
Similarly, radiation therapy, while primarily designed to kill tumor cells directly, also plays a role in shaping the immune response (42). Radiation has been shown to act as an immunomodulator by inducing a process known as “immunogenic cell death” (43). This process leads to the release of danger-associated molecular patterns (DAMPs) that alert the immune system to the presence of tumor cells. Radiation also promotes the infiltration of T-cells into the tumor and enhances the production of pro-inflammatory cytokines, which further stimulate the immune response (44). These effects make radiation therapy an effective complementary treatment to immunotherapy, especially when combined with immune checkpoint inhibitors. Radiation-SI: Un autre point important que nous devons discuter.
These findings suggest that combining immunotherapy with chemoradiation not only enhances the immune system’s ability to recognize and attack cancer cells but also improves the overall therapeutic efficacy of the treatment regimen.
4 Novel immunotherapy approaches in locally advanced cervical cancer setting
In cervical cancer, the rationale for the use of immunotherapy is highly compelling and finds its basis in the complex interactions between the immune system, HPV infection, and tumor cells (5). Indeed, the viral-induced cervical cancer present a specific immunologic profile associated with high tumor mutational burden in approximatively 20% of cases, increasing the neoantigen burden and enhancing the immunogenicity of the tumor (6). In addition, the high expression of ICIs (CTLA4, PD1, PDL1) and the presence of tumor-infiltrating lymphocytes (TILs), unveiled the significant immunogenic potential of cervical cancer settings (45, 46).
ICIs has become a central component of first-line treatment for recurrent or metastatic CC, and is under investigation in locally advanced disease with promising results from several phase III trials (47). However, other promising immunotherapeutic strategies are in earlier phases of development, including vaccines (48), tumor infiltrating lymphocytes (49–51), genetically engineered T cells targeting HPV-associated proteins (52–54), and antibody-drug conjugates (55) (Figure 1). All these strategies may synergize and change the standard of care for definitive management of the different settings of cervical cancer.
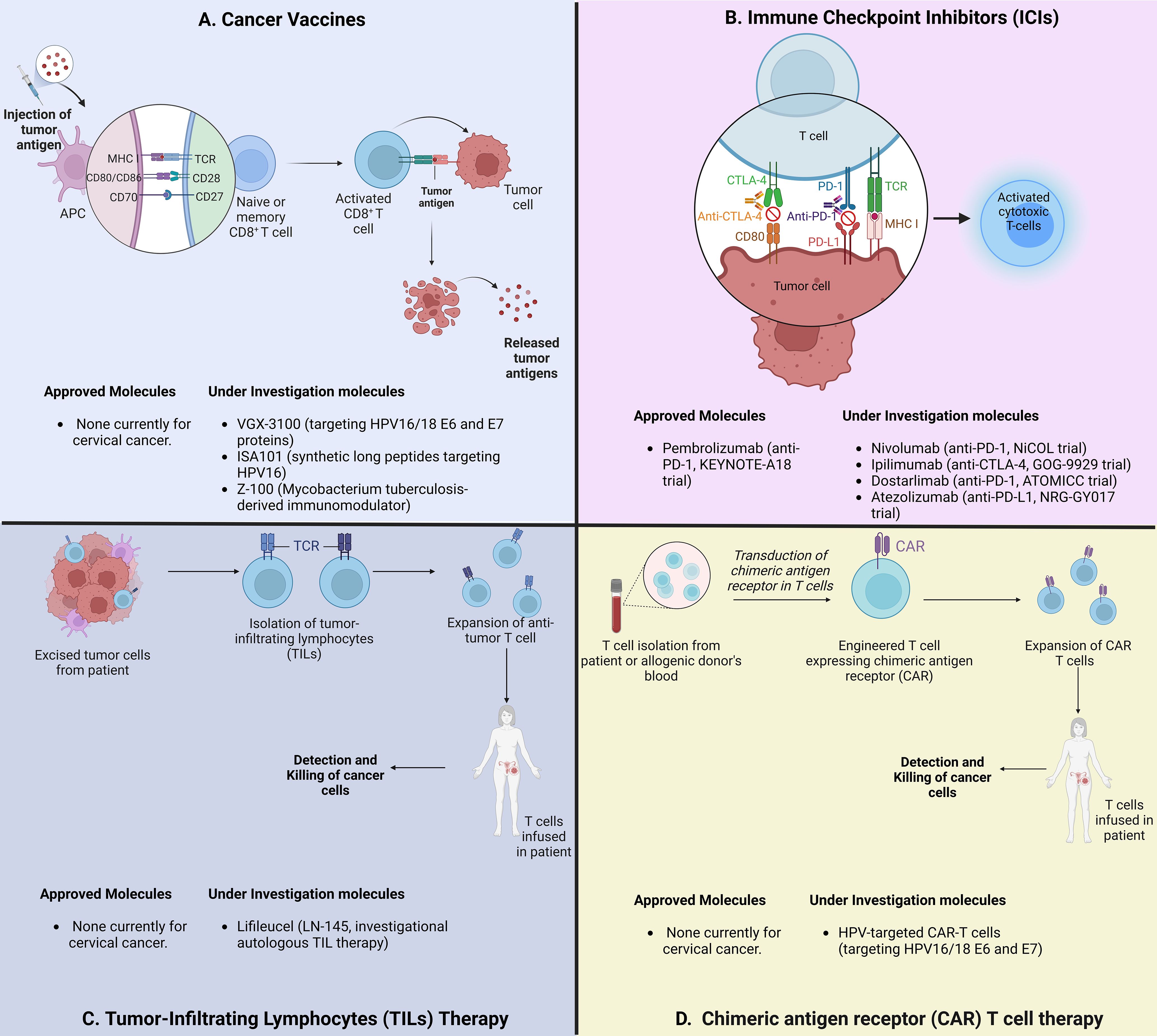
Figure 1. Schematic overview of immunotherapeutic strategies for locally advanced cervical cancer. (A) Strategies to induce and enhance cervical cancer-specific T Cells. VGX-3100: HPV16/18 vaccine; Z-100: Mycobacterium tuberculosis extract. (B) Strategies to block immune checkpoints and restore T-cell function. PD-1: Programmed cell Death protein 1; CTLA-4: Cytotoxic T Lymphocyte Associated Protein 4. (C) Strategies using adoptive cell therapy to augment TILs. (D) CAR-T cell therapies targeting HPV-associated proteins.
While ICIs represent the most widely studied immunotherapy, therapeutic vaccines and cell-based therapy approaches are under investigation in cervical cancer with encouraging results in pre-clinical trials (48) (Table 1). Although these therapies are principally being investigated in metastatic and recurrent diseases, ongoing studies are exploring their benefit in locally advanced cervical cancer (56). The well-known oncogenic properties of HPV and supportive post-infection microenvironment (PIM) created by HPV-infected cells, eagerly promoted investigations on therapeutic vaccines treatment. For cervical cancer, the majority of investigated therapeutic vaccines targeted the oncoproteins HPVs E6 and E7 (57).
Building on the positive outcome of two phase II studies in metastatic and recurrent settings, the phase III AIM2CERV clinical trial (NCT02853604) was tailored to determine the effectiveness of Axalimogene filolisbac after the completion of CCRT in high-risk locally advanced cervical cancer (FIGO stage I–II with positive pelvic nodes, stage III–IVA, and any stage with para-aortic nodes) (58, 59). Axalimogene filolisbac (ADXS-HPV, Princeton, NJ, USA) is a live attenuated, recombinant Listeria monocytogenes (Lm) bacterium bioengineered to secrete an antigen-adjuvant fusion protein that includes a truncated fragment of listeriolysin O (tLLO) fused to the full-length E7 peptide of HPV-16 (tLLO-HPV-16 E7) (58). The rapid uptake of ADXS-HPV by antigen presenting cells and secretion of the fusion HPV E7 protein, stimulate innate immunity, followed promptly by HPV-specific effector T-cells infiltration of the TME and tumor cell killing (60, 61). Although designed to target HPV type 16-associated cancers, results from ADXS-HPV preclinical and clinical studies showed immunogenic response against high-risk HPV other than type 16 (62). The AIM2CERV clinical trial has been terminated in 2019 and no results have been published yet.
PDS0101 is a multipeptide therapeutic vaccine targeting the E6 and E7 oncoproteins of high-risk HPV type 16. The coadministration of peptides with the nanoparticle R-DOTAP (Versamune) platform, induces type 1 interferons and enhances antigen cross-presentation. PDS0101 induced HPV-specific CD4+ and CD8+ T-cell immune responses and was well tolerated (63). The ongoing single-arm, phase II IMMUNOCERV trial (NCT04580771), focuses on evaluating a liposomal HPV-16 E6/E7 T-cell activating immunotherapy (PDS0101) approach combined with CCRT in advanced cervical cancer patients (64). The interim analysis demonstrated promising results on eight patients that completed the treatment, showing a complete response rate of 87.5% on PET at 3 months; the 1-year disease-free survival rate was 85.7%, and the 1-year overall survival 100%, associated with an acceptable toxicity profile.
Moreover, genomic profiling and radiomic studies to guide the response to immunotherapy in advanced cervical cancer are promising. Accumulated data confirm the dysregulation of molecular pathways like PI3K/AKT/mTOR, PIK3CA, STK11, and PTEN. In patients treated with anti-PD1 immunotherapy, ERBB3 mutation and a high TMB have been shown associated with prolonged survival, making them predictive biomarkers candidates in advanced cervical cancer (65, 66). Radiomic studies hold great potential to guide the response to different immunotherapy regimens, according to intrinsic tumor radiosensitivity (67, 68).
5 Challenges of predictive biomarkers for cervical carcinoma immunotherapy
Studies discussed above highlight that only a minority of patients with cervical cancer can benefit from the use of ICI therapy. Improving ICI efficacy can be achieved by identifying potential biomarkers for the assessment of the therapeutic effect. Several factors have been identified as predictive biomarkers for anti-PD1/PDL1 immunotherapy response, including PD-L1 expression in tumor cells and tumor-infiltrating lymphocytes, tumor mutational burden (TMB), microsatellite instability (MSI), and/or mismatch repair deficiency (MMR) (69–71).
Normal cervical tissue does not express PDL1 protein. However, in premalignant and malignant lesions the expression of PDL1 is reported to be in the range of 95% in cervical intraepithelial neoplasia (CIN) and 80% in cervical SCC (72). In cervical SCC, another study reported a low PDL1 expression rate of 24.9% (73). Cervical adenocarcinoma shows low rates of PDL1 expression with one study reporting PDL1 positivity in 14% of samples versus 54% in SCC (74). These discrepancies between studies may be attributed to variations in analysis and detection methods as well as the cut-off values used to define positive expression.
Based on the KEYNOTE-158 clinical trial findings, showing a clinical activity of pembrolizumab in 14% of PDL1-positive patients, the FDA approved pembrolizumab for cervical carcinomas with PDL1 positivity defined by a combined positive score (CPS) ≥ 1 assessed by the DAKO 22C3 assay (75, 76). The CPS calculates the ratio between all PD-L1-positive neoplastic cells, lymphocytes and macrophages, multiplied by 100 and divided by the total number of viable tumor cells (77). However, even if the use of CPS positivity help identifying patients who may respond to PD1/PDL1 blockade, many studies have reported responses in PDL1 negative tumors (78). The heterogeneity in PDL1 expression may contribute to variable results and undermine its reliability as a biomarker (74). More recent approaches for PD1/PDL1 assessment beyond IHC, include PDL1 copy number analysis and RNAish to detect PDL1 mRNA (79, 80).
Tumor mutational burden (TMB) is a surrogate biomarker of neo-antigens load and immunogenicity, with high TMB status associated with favorable response to ICIs (81). The TMB is calculated by assessing the number of nonsynonymous somatic mutations per mega-base (mb) (82–84). High TMB has been shown to be more significantly associated with response to PD-1 and PD-L1 immunotherapy than PD-1 or PD-L1 expression (85). While high TMB is relatively uncommon in cervical cancer, some studies have suggested that patients with high TMB may derive greater benefit from immune checkpoint inhibitors (86). In a study describing the distribution of TMB across a cohort of 284 cervical SCC cases, a TMB median of 5.4 mutations/mb have been calculated, with TMB > 20 mutations/mb in 6.7% cases (85). The potential for combining TMB with other biomarkers, such as PD-L1 expression, to guide treatment decisions is an exciting avenue for future research.
Biomarker analysis of the basket study KEYNOTE 158, based on the companion diagnostic FoundationOne CDx assay, accelerated approval of pembrolizumab in patients with high TMB (≥ 10 mutations/mb) (6). the FoundationOne CDx panel detects substitutions, insertions and deletions, and copy number alterations in 324 genes, select gene rearrangements, and genomic signatures including microsatellite instability and tumor mutation burden (TMB) in patients with advanced or recurrent solid tumors (87). TMB holds significant promise as a predictive biomarker of therapeutic response immunotherapy. Further studies are needed to standardize and harmonize TMB assessment across assays, and confirm its predictive efficacy in diverse settings.
Microsatellite instability (MSI) or mismatch repair deficiency (MMR-d) have been linked to exceptional benefit from ICIs. MSI-high tumors are known to exhibit a higher mutational burden, leading to the production of more neoantigens, which can enhance the immune response to the tumor (88). Although MSI-high tumors are relatively rare in cervical cancer, they have been associated with better responses to immunotherapy in other cancer types, such as colorectal cancer (89, 90). MSI and MMR-d are agnostic indication for the use of pembrolizumab in refractory tumors. In cervical SCC, MSI-high was found in 11.8% (89, 91). Many MSI markers are routinely used to identify MSI-High cancers, with the principal aim of treating patients and offering tailored therapeutic approach. However, the MSI testing is limited to certain types of cancer such as endometrial and colorectal cancer. There is an urgent need to invest in gynecological cancers-specific MSI panels which for more accurate and effective screening (92). A comprehensive characterization of MMR protein expression in cervical cancer confirms that MMR-D is rare compared to neuroendocrine tumors. In a recent study, Van Den Berg et al., propose MSH-2-low and not MMR-D as biomarker of ICI response in cervical cancer due to its association with high mutational burden, immune activation, and RAD50 frameshift mutations (93). In cervical cancer, the identification of MSI-high tumors may offer an opportunity for personalized treatment strategies that incorporate immune checkpoint inhibitors.
A deep understanding of the complex relationship between PD1/PDL1 expression, TMB and MSI status, is a prerequisite to improve the use of immunotherapy in cervical cancer patients.
Tumor-draining lymph nodes (TDLNs) located along the lymphatic drainage pathway of primary tumors, serve as a repository of anti-tumor immune cells and are the primary sites at which anti-tumor lymphocytes are primed to tumor-specific antigens (94). Thus, TDLNs can mirror the immune dynamics of battle between immune and tumor cells. In cervical cancer, immune profiling by flow cytometry of pelvic TDLN revealed predominant and elevated PD-1 expression on effector T-cell subsets. In addition, elevated levels of CD8+ FoxP3+ CD25+ effector T cells were identified as a potential biomarker for predicting response to PD-1 blockade that merits prompt evaluation (95). Gaining knowledge of TDLN-immune biomarkers provides attractive means for improving the evaluation system and developing new avenue of patient stratification, timing of immunotherapy, and efficacy outcomes. Clinical studies should focus on investigating changes in TDLNs during ICIs treatment, including alterations in size, immune cell profiles, and cell function. Additionally, there is a need to enhance diagnostic tools for assessing TDLNs (96).
Given the high financial cost of using predictive biomarker tests in routine practices, prognostic scores based on routine laboratory data represent a promising breakthrough. For example, the Lung Immune Prognostic Index (LIPI) score based on neutrophil, lymphocyte, and LDH levels have been identified as a reliable tool to guide immunotherapy treatment decisions (97).
The identification and validation of cervical cancer biomarkers can be of great importance in designing adaptive clinical trials (98). Unlike non-adaptive trial designs, adaptive designs are flexible using accumulating data based on interim analysis to modify the ongoing trial without affecting the integrity and validity of the trial (99). Recently, several biomarkers were used to guide adaptive trial designs, including the marker-stratified design, marker-strategy design, enrichment design, basket design, N-of-1 design and master protocol design (99).
Finally, effective prevention of cervical cancer lies on early screening. While traditional cervical cytology remains the gold standard for cervical cancer screening, this method is time-consuming, subjective, has limited and highly variable sensitivity, and relies on the expertise and experience of pathologists (100). The emergence of Artificial intelligence (AI) screening systems holds promise in enhancing cervical cancer imaging diagnostics and predicting clinical responses (101). Compared to cytology, AI screening enhances the speed, accuracy, and reliability of cancer detection. AI-enhanced screening relies on consistency of cytopathological results, improving sensitivity, and reducing the risk of misdiagnosis (102, 103). In a study reported by Zhu et al., an AI-aided comprehensive cervical diagnostic system (AIATBS) has been developed (104). This system integrated five AI models; YOLOv3 for object detection, Xception and DenseNet-50 for target classification, U-net for nucleus segmentation, and XGBoost model for final slide-level diagnostic decisions. Although AIATBS system applicability and robustness for routine assistive diagnostic screening was demonstrated, it actually increases the complexity of related-screening processes (101). Rahaman et al., developed a classification of cervical cytology using a deep learning-based hybrid deep feature fusion (HDFF) technique (105). However, the classification focuses only on squamous epithelial cells. Recently, Wang et al., have created an AI-assisted cervical cancer screening (AICCS) by investigating the whole-slide images (WSIs) of cervical cytology (101). Even if the use of AI can enhance the quality of medical services, several limitations need to be considered, mainly limited operator experience, skill levels of cytology preparers, and ethical considerations (101).
6 Discussion
The combination of immune checkpoint inhibitors with chemoradiation (CRT) has emerged as a promising therapeutic approach, fueled by the successes observed in malignancies such as non-small cell lung cancer (NSCLC) and small cell lung cancer (SCLC). Notably, durvalumab, an anti-PD-L1 inhibitor, demonstrated significant improvements in both overall survival (OS) and progression-free survival (PFS) following CRT in the PACIFIC and ADRIATIC trials (106, 107), which established its therapeutic potential in lung cancer. This success paved the way for its investigation in cervical cancer, with the CALLA trial marking the first Phase 3 study to evaluate the combination of immunotherapy and CRT for untreated locally advanced cervical cancer (108) (Table 2). Published in The Lancet, the CALLA trial enrolled a diverse cohort of patients from 105 hospitals across 15 countries, including those with squamous, adenocarcinoma, and adenosquamous histologies. The inclusion criteria encompassed patients with FIGO stages IB2–IIB (with lymph node involvement) or stage ≥III. Participants were randomized to receive either durvalumab (1500 mg every 4 weeks) or placebo alongside standard CRT, which consisted of external beam radiation (45 Gy) with weekly cisplatin or carboplatin, followed by image-guided brachytherapy. The Table 2 summarizes key clinical trials using Immunotherapeutic strategies in Cervical cancer.
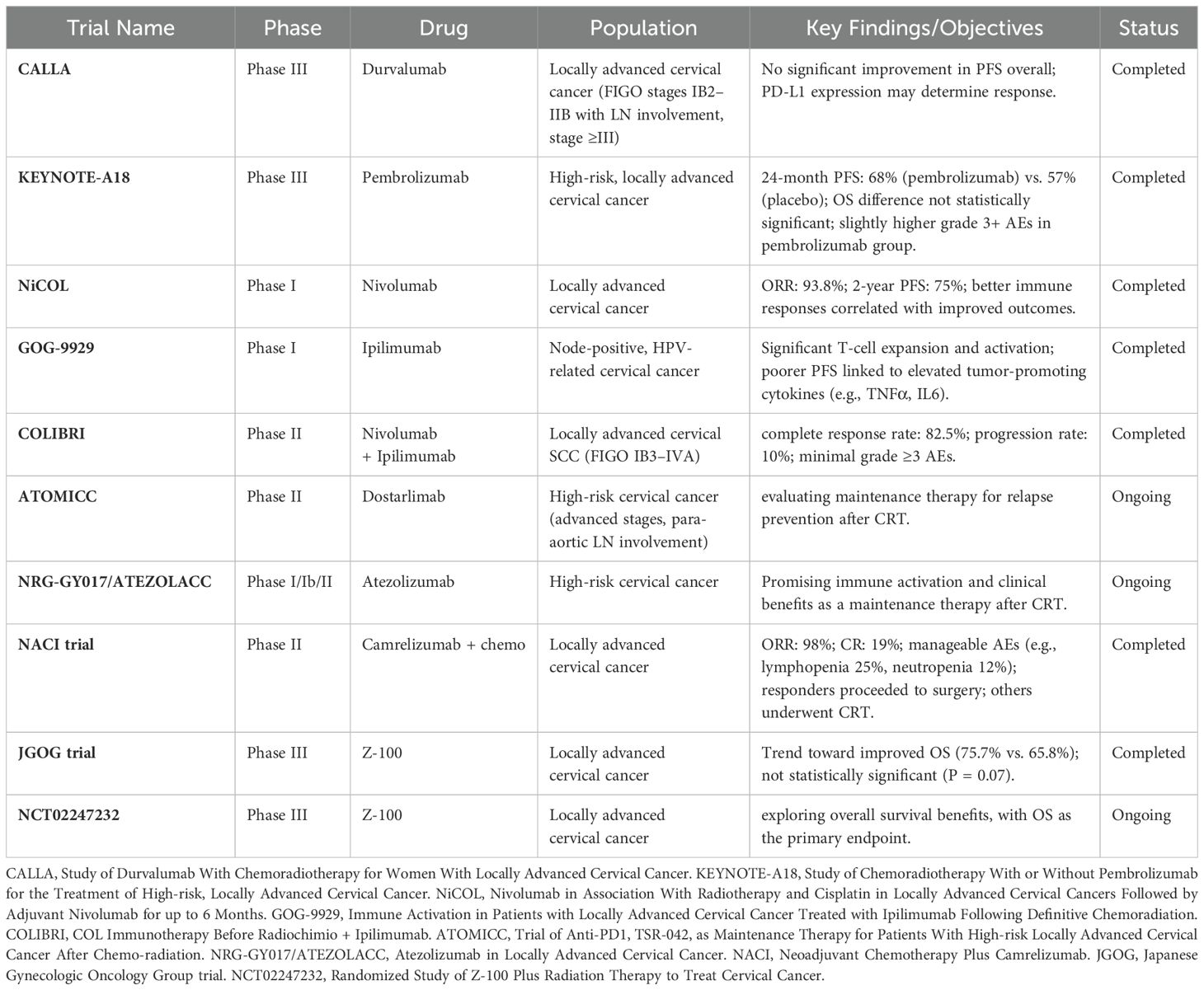
Table 2. Summary of completed and ongoing clinical trials using Immunotherapeutic strategies in Locally advanced Cervical Cancer
Despite the promising theoretical foundation for durvalumab’s efficacy, the trial ultimately failed to demonstrate a significant improvement in PFS in the overall patient population. Although the combination of durvalumab and CRT was well tolerated, a deeper analysis revealed that PD-L1 expression might play a crucial role in determining which patients are more likely to benefit from this treatment. In contrast, the ENGOT-cx11/GOG-3047/KEYNOTE-A18 trial, which investigated pembrolizumab (200 mg for 5 cycles, followed by 400 mg for 15 cycles) in combination with CRT, yielded more promising results (109). This Phase 3 trial enrolled a cohort of 1,060 high-risk, locally advanced cervical cancer patients across 176 centers in 30 countries. The study demonstrated a 24-month PFS rate of 68% in the pembrolizumab arm, compared to 57% in the placebo group (HR = 0.70, p = 0.0020), although the OS difference at 24 months (87% vs. 81%) did not reach statistical significance. Importantly, adverse events of grade 3 or higher were more common in the pembrolizumab group (75% vs. 69%), suggesting that while the therapy has potential, close monitoring of adverse effects is warranted. Despite the modest impact on OS, these results highlight pembrolizumab’s potential to improve PFS in high-risk cervical cancer patients, positioning it as a key candidate for further clinical exploration. This combination has already been incorporated into the updated 2025 National Comprehensive Cancer Network (NCCN) guidelines, where pembrolizumab is recommended as part of the standard treatment regimen for FIGO 2014 and 2018 stage III–IVA cervical cancer. However, it should be noted that this approach has not yet been adopted by the European Society for Medical Oncology (ESMO) or the European Society of Gynecological Oncology, in collaboration with the European Society for Radiotherapy and Oncology and the European Society of Pathology (ESGO/ESTRO/ESP) (110, 111).
In addition to pembrolizumab, other immune checkpoint inhibitors are emerging as promising options in the treatment of cervical cancer. Nivolumab, an anti-PD-1 antibody, was evaluated in the NiCOL Phase 1 trial in combination with CRT (112). This trial included 16 patients with locally advanced cervical cancer, revealing an overall response rate (ORR) of 93.8%, alongside a two-year PFS rate of 75%. Immune response analysis from the study revealed that patients with better PFS exhibited stronger immune cell infiltrates and more robust immune cell interactions, whereas those with progressive disease had higher levels of PD-L1 expression and increased regulatory T cell populations. These findings suggest that nivolumab may be particularly beneficial for patients with an activated anti-tumor immune response, further supporting its potential in the treatment of cervical cancer.
Furthermore, the combination of ipilimumab, an anti-CTLA-4 antibody, with CRT has also shown promise in early-phase trials. The GOG-9929 Phase I trial (113), which tested this combination in 21 patients with node-positive, HPV-related cervical cancer, demonstrated significant T-cell population expansion and upregulation of activation markers, such as ICOS and PD-1, particularly enhancing HPV-specific T-cell responses in HPV18+ tumors. However, elevated levels of tumor-promoting cytokines, including TNFα and IL6, were associated with poorer PFS, emphasizing the need for precise modulation of immune responses to optimize clinical outcomes. In a related Phase II neoadjuvant study, the COLIBRI trial combined nivolumab and ipilimumab in locally advanced cervical squamous cell carcinoma (FIGO stages IB3–IVA), resulting in impressive outcomes such as significant CD8+ T-cell infiltration, high complete response rates (82.5%), and low progression rates (10%), particularly in stage III-C disease (114). These promising results suggest that dual immune checkpoint blockade may enhance anti-tumor immunity in high-risk cervical cancer patients.
In parallel, maintenance immunotherapy strategies are under active investigation as a means to prolong PFS and reduce the risk of recurrence. The ongoing ATOMICC trial is assessing dostarlimab, an anti-PD-1 checkpoint inhibitor, as a maintenance therapy for patients who achieved partial or complete responses following CRT (115). This Phase II trial focuses on high-risk cervical cancer patients, particularly those with advanced FIGO stages or para-aortic lymph node involvement, to determine whether dostarlimab can effectively reduce relapse rates. Likewise, atezolizumab, a PD-L1 inhibitor, is being explored in combination with CRT in several trials, including the NRG-GY017 Phase I/Ib and ATEZOLACC Phase II studies, which have demonstrated promising immune activation and potential clinical benefits. These findings position atezolizumab as a potentially valuable maintenance option in cervical cancer.
Neoadjuvant and adjuvant approaches, such as the combination of Camrelizumab, an anti-PD-1 monoclonal antibody, with chemotherapy, are also being explored. A Phase II trial conducted across multiple centers in China reported an ORR of 98%, with 19% of patients achieving a complete response (116). Patients who responded to this chemo-immunotherapy combination proceeded to surgery, while non-responders underwent CRT. While the treatment was generally well tolerated, the most common grade 3–4 adverse events were lymphopenia (25%) and neutropenia (12%), underscoring the need for careful management of immune-related toxicities. Additionally, Z-100, an extract, derived from Mycobacterium tuberculosis, which restores the Th1 immune response (117), has been evaluated in a Phase III trial by the Japanese Gynecologic Oncology Group. Although the trial did not reach statistical significance, there was a trend toward improved overall survival in the Z-100 group (75.7%) compared to the placebo group (65.8%), indicating its potential to enhance the efficacy of radiotherapy (118). Ongoing trials, such as NCT02247232, are expected to provide further insights, with overall survival as the primary endpoint.
The ICIs’ mechanism of action is through the reactivation of anti-tumor T-cells, resulting in a new tumor cytoxicity profile with immunotherapy-related adverse effects significantly different from those of conventional chemotherapy. In a recent retrospective study, Shehaj et al., evaluated the occurrence and type of immune-associated side effects in a cohort of 61 patients with gynecological malignancies who received ICIs; anti–PD-1 antibodies (pembrolizumab, dostarlimab, durvalumab) or the anti-PD-L1 antibody atezolizumab (119). The key finding is that ICIs therapy was well tolerated, with anti-PD-(L)1 antibodies tending to be better tolerated (120). The duration of ICI therapy was reported as the only significant factor influencing the incidence of adverse events (119).
Feng et al., showed that adverse events did not differ between the first-line ICIs plus platinum and paclitaxel group versus first-line platinum and paclitaxel group in advanced and recurrent cervical cancer patients (121). The common reported adverse effects of Pembrolizumab are tolerable liver dysfunction, hypothyroidism, neutropenia, anemia, decreased appetite, fatigue, and fever. Most of them improved after therapy discontinuation (122). A few cases of cutaneous adverse events were reported associated with pembrolizumab combination chemotherapy in patients with metastatic or recurrent cervical cancer (123). Adverse events related to nivolumab and atezolizumab, PD-1/PD-L1 inhibitors still at I/II phase clinical trial, are unavailable (122).
The high cost of immunotherapies constitute a financial burden, limiting patients’ access to these treatments (124). Immunotherapy cost-effectiveness vary significantly among countries and depends on the treatment indication and the use of biomarkers, such as PDL1 (125). Till now, no clear evidence regarding the cost-effectiveness of anti PD-L1 and PD-1 for treating LACC have been presented, and available data are not in favor of the cost-effectiveness of anti PD-L1 in LACC (126). Only one cost-effectiveness analysis for the treatment of patients with cervical cancers was conducted through a partitioned survival model indicating that pembrolizumab was not cost-effective versus the placebo (127). The cost is a major barrier to immunotherapy accessibility in low- and middle-income countries. Studies investigating the most cost-effective dosing strategies for ICIs are urgently needed (124). Furthermore, healthcare systems must focus on improving prevention programs, which offer the most cost-effective strategy for the control of cervical cancer.
Finally, patient selection for immunotherapy approaches remains challenging. Further research and new guidelines are necessary to address the best personalized therapeutic options based on histological subtype, PD-L1 status, and patient’s relapse history as they are no longer ICI-naïve. Thus, research on predictive biomarkers using innovative approaches, has to be strengthened. The focus should also be on the best sequencing immunotherapy strategies: neo-adjuvant, concomitant, maintenance, or a combination; monotherapy or dual immune checkpoint inhibitors; and the combination of chemotherapy with immunotherapy. Furthermore, with the increased use of immunotherapies, how to overcome resistance is a major concern. The presence of primary mechanisms of resistance deprive patients of immunotherapy benefits. Deciphering the intricate TME and its dynamics will enable a better patient’s stratification and thus more precise and effective immunotherapy.
7 Conclusion
Immune checkpoint inhibitors represent a paradigm shift in the treatment of locally advanced cervical cancer. While some studies highlight the complexities of patient selection, others reinforce the potential of checkpoint blockade in enhancing PFS. As additional data emerge, optimizing patient stratification through biomarkers, refining combination strategies, and addressing immune-related toxicities will be critical in integrating immunotherapy into standard cervical cancer treatment protocols. Furthermore, the development of novel agents, exploration of synergistic therapeutic combinations, and ongoing refinement of treatment sequencing will play a pivotal role in maximizing clinical benefits. Future research should focus on identifying predictive biomarkers, improving patient selection criteria, and mitigating adverse effects to ensure that immunotherapy is both effective and tolerable for a broader patient population.
Author contributions
FK: Conceptualization, Data curation, Formal Analysis, Investigation, Methodology, Resources, Software, Visualization, Writing – original draft, Writing – review & editing. AG: Data curation, Methodology, Validation, Writing – review & editing. HI: Data curation, Formal Analysis, Visualization, Writing – original draft. HM: Formal Analysis, Software, Visualization, Writing – review & editing. BG: Conceptualization, Data curation, Methodology, Validation, Visualization, Writing – review & editing, Writing – original draft. AB: Funding acquisition, Methodology, Project administration, Supervision, Validation, Writing – review & editing.
Funding
The author(s) declare that financial support was received for the research and/or publication of this article. This work was supported by the Moroccan Ministry of Higher Education, Research and innovation through a “PPR1” project; by the Moroccan Ministry of Higher Education, Research and innovation and the Digital Development Agency “ADD” through an “Al-khawarizmi” project; and by an intra-Africa Academic Mobility Grant provided by the EUROPEAN COMMISSION, ref number 624289-PANAF-1-2020-1-KEPANAFMOBAF.
Conflict of interest
The authors declare that the research was conducted in the absence of any commercial or financial relationships that could be construed as a potential conflict of interest.
Generative AI statement
The author(s) declare that no Generative AI was used in the creation of this manuscript.
Publisher’s note
All claims expressed in this article are solely those of the authors and do not necessarily represent those of their affiliated organizations, or those of the publisher, the editors and the reviewers. Any product that may be evaluated in this article, or claim that may be made by its manufacturer, is not guaranteed or endorsed by the publisher.
Abbreviations
CRT, Chemo-Radiation; CTLA-4, Cytotoxic T-Lymphocyte associated Antigen 4; DAMPs, Danger-Associated Molecular Patterns; ESGO, European Society of Gynaecological Oncology; ESP, European Society of Pathology; ESTRO, European Society for Radiotherapy and Oncology; HPV, Human Papillomavirus; MSI, Micro-Satellite Instability; TMB, Tumor Mutational Burden; MDSCs, Myeloid-Derived Suppressor Cells; NCCN, National Comprehensive Cancer Network; NSCLC, Non-Small Cell Lung Cancer; ORR, Overall Response Rate; PFS, Progression-Free Survival; PD-1, Programmed cell Death protein 1; PD-L1, Programmed cell Death ligand 1; SCLC, Small Cell Lung Cancer; TAMs, Tumor-Associated Macrophages; Tregs, Regulatory T-cells.
References
1. Wipperman J, Neil T, Williams T. Cervical cancer: evaluation and management. Am Fam Phys. (2018) 97:449–54.
2. Burmeister CA, Khan SF, Schäfer G, Mbatani N, Adams T, Moodley J, et al. Cervical cancer therapies: Current challenges and future perspectives. Tumour Virus Res. (2022) 13:200238. doi: 10.1016/j.tvr.2022.200238
3. Green JA, Kirwan JM, Tierney JF, Symonds P, Fresco L, Collingwood M, et al. Survival and recurrence after concomitant chemotherapy and radiotherapy for cancer of the uterine cervix: a systematic review and meta-analysis. Lancet. (2001) 358:781–6. doi: 10.1016/S0140-6736(01)05965-7
4. Fachini AMD, Zuliani AC, Sarian LO, Teixeira JC, Esteves SCB, Da Costa MaChado H, et al. Long-term outcomes of concomitant cisplatin plus radiotherapy versus radiotherapy alone in patients with stage IIIB squamous cervical cancer: A randomized controlled trial. Gynecol Oncol. (2021) 160:379–83. doi: 10.1016/j.ygyno.2020.11.029
5. Duranti S, Pietragalla A, Daniele G, Nero C, Ciccarone F, Scambia G, et al. Role of immune checkpoint inhibitors in cervical cancer: from preclinical to clinical data. Cancers. (2021) 13:2089. doi: 10.3390/cancers13092089
6. Marabelle A, Fakih M, Lopez J, Shah M, Shapira-Frommer R, Nakagawa K, et al. Association of tumour mutational burden with outcomes in patients with advanced solid tumours treated with pembrolizumab: prospective biomarker analysis of the multicohort, open-label, phase 2 KEYNOTE-158 study. Lancet Oncol. (2020) 21:1353–65. doi: 10.1016/S1470-2045(20)30445-9
7. Raju K. Virus and cervical cancer: role and implication: A review. BioMed Res Ther. (2014) 2:7. doi: 10.7603/s40730-015-0007-z
8. Fernandes A, Viveros-Carreño D, Hoegl J, Ávila M, Pareja R. Human papillomavirus-independent cervical cancer. Int J Gynecol Cancer. (2022) 32:1–7. doi: 10.1136/ijgc-2021-003014
9. Guan P, Howell-Jones R, Li N, Bruni L, De Sanjosé S, Franceschi S, et al. Human papillomavirus types in 115,789 HPV-positive women: A meta-analysis from cervical infection to cancer. Intl J Cancer. (2012) 131:2349–59. doi: 10.1002/ijc.27485
10. Banister CE, Liu C, Pirisi L, Creek KE, Buckhaults PJ. Identification and characterization of HPV-independent cervical cancers. Oncotarget. (2017) 8:13375–86. doi: 10.18632/oncotarget.14533
11. Hang D, Jia M, Ma H, Zhou J, Feng X, Lyu Z, et al. Independent prognostic role of human papillomavirus genotype in cervical cancer. BMC Infect Dis. (2017) 17:391. doi: 10.1186/s12879-017-2465-y
12. Chong GO, Lee YH, Han HS, Lee HJ, Park JY, Hong DG, et al. Prognostic value of pre-treatment human papilloma virus DNA status in cervical cancer. Gynecol Oncol. (2018) 148:97–102. doi: 10.1016/j.ygyno.2017.11.003
13. Nicolás I, Marimon L, Barnadas E, Saco A, Rodríguez-Carunchio L, Fusté P, et al. HPV-negative tumors of the uterine cervix. Modern Pathol. (2019) 32:1189–96. doi: 10.1038/s41379-019-0249-1
14. Da Mata S, Ferreira J, Nicolás I, Esteves S, Esteves G, Lérias S, et al. P16 and HPV genotype significance in HPV-associated cervical cancer—A large cohort of two tertiary referral centers. IJMS. (2021) 22:2294. doi: 10.3390/ijms22052294
15. Lei J, Arroyo-Mühr LS, Lagheden C, Eklund C, Nordqvist Kleppe S, Elfström M, et al. Human papillomavirus infection determines prognosis in cervical cancer. JCO. (2022) 40:1522–8. doi: 10.1200/JCO.21.01930
16. Bhattacharjee R, Das SS, Biswal SS, Nath A, Das D, Basu A, et al. Mechanistic role of HPV-associated early proteins in cervical cancer: Molecular pathways and targeted therapeutic strategies. Crit Rev Oncology/Hematol. (2022) 174:103675. doi: 10.1016/j.critrevonc.2022.103675
17. Balasubramaniam SD, Balakrishnan V, Oon CE, Kaur G. Key molecular events in cervical cancer development. Medicina. (2019) 55:384. doi: 10.3390/medicina55070384
18. Bashaw AA, Leggatt GR, Chandra J, Tuong ZK, Frazer IH. Modulation of antigen presenting cell functions during chronic HPV infection. Papillomavirus Res. (2017) 4:58–65. doi: 10.1016/j.pvr.2017.08.002
19. Konstantopoulos G, Leventakou D, Saltiel D-R, Zervoudi E, Logotheti E, Pettas S, et al. HPV16 E6 Oncogene Contributes to Cancer Immune Evasion by Regulating PD-L1 Expression through a miR-143/HIF-1a Pathway. Viruses. (2024) 16:113. doi: 10.3390/v16010113
20. Sharma P, Allison JP. Immune checkpoint targeting in cancer therapy: toward combination strategies with curative potential. Cell. (2015) 161:205–14. doi: 10.1016/j.cell.2015.03.030
21. Topalian SL, Hodi FS, Brahmer JR, Gettinger SN, Smith DC, McDermott DF, et al. Safety, activity, and immune correlates of anti–PD-1 antibody in cancer. N Engl J Med. (2012) 366:2443–54. doi: 10.1056/NEJMoa1200690
22. Pardoll DM. The blockade of immune checkpoints in cancer immunotherapy. Nat Rev Cancer. (2012) 12:252–64. doi: 10.1038/nrc3239
23. Ribas A, Wolchok JD. Cancer immunotherapy using checkpoint blockade. Science. (2018) 359:1350–5. doi: 10.1126/science.aar4060
24. Liu X, Wang S, Lv H, Chen E, Yan L, Yu J. Advances in the relationship of immune checkpoint inhibitors and DNA damage repair. Curr Res Trans Med. (2025) 73:103494. doi: 10.1016/j.retram.2025.103494
25. Chen Q, Zhai B, Li J, Wang H, Liu Z, Shi R, et al. Systemic immune-inflammatory index predict short-term outcome in recurrent/metastatic and locally advanced cervical cancer patients treated with PD-1 inhibitor. Sci Rep. (2024) 14:31528. doi: 10.1038/s41598-024-82976-6
26. Su R, Jin C, Bu H, Xiang J, Zhou L, Jin C. Development and validation of an immune-related prognostic signature in cervical cancer. Front Oncol. (2022) 12:861392. doi: 10.3389/fonc.2022.861392
27. Liu J, Wu Z, Wang Y, Nie S, Sun R, Yang J, et al. A prognostic signature based on immune-related genes for cervical squamous cell carcinoma and endocervical adenocarcinoma. Int Immunopharmacol. (2020) 88:106884. doi: 10.1016/j.intimp.2020.106884
28. Lukovic J, Pintilie M, Han K, Fyles AW, Bruce JP, Quevedo R, et al. An immune gene expression risk score for distant metastases after radiotherapy for cervical cancer. Clin Cancer Res. (2024) 30:1200–7. doi: 10.1158/1078-0432.CCR-23-2085
29. Palaia I, Tomao F, Di Pinto A, Pernazza A, Santangelo G, D’Alessandris N, et al. Response to neoadjuvant chemotherapy in locally advanced cervical cancer: the role of immune-related factors. In Vivo. (2021) 35:1277–83. doi: 10.21873/invivo.12379
30. Rocha Martins P, Luciano Pereira Morais K, De Lima Galdino NA, Jacauna A, Paula SOC, Magalhães WCS, et al. Linking tumor immune infiltrate and systemic immune mediators to treatment response and prognosis in advanced cervical cancer. Sci Rep. (2023) 13:22634. doi: 10.1038/s41598-023-49441-2
31. Ling SP, Ming LC, Dhaliwal JS, Gupta M, Ardianto C, Goh KW, et al. Role of immunotherapy in the treatment of cancer: A systematic review. Cancers. (2022) 14:5205. doi: 10.3390/cancers14215205
32. Xie Y, Kong W, Zhao X, Zhang H, Luo D, Chen S. Immune checkpoint inhibitors in cervical cancer: Current status and research progress. Front Oncol. (2022) 12:984896. doi: 10.3389/fonc.2022.984896
33. Monk BJ, Enomoto T, Kast WM, McCormack M, Tan DSP, Wu X, et al. Integration of immunotherapy into treatment of cervical cancer: Recent data and ongoing trials. Cancer Treat Rev. (2022) 106:102385. doi: 10.1016/j.ctrv.2022.102385
34. Stefanoudakis D, Karopoulou E, Matsas A, Katsampoula GA, Tsarna E, Stamoula E, et al. Immunotherapy in cervical and endometrial cancer: current landscape and future directions. Life. (2024) 14:344. doi: 10.3390/life14030344
35. Li Y, Gao X, Huang Y, Zhu X, Chen Y, Xue L, et al. Tumor microenvironment promotes lymphatic metastasis of cervical cancer: its mechanisms and clinical implications. Front Oncol. (2023) 13:1114042. doi: 10.3389/fonc.2023.1114042
36. Kamali AN, Bautista JM, Eisenhut M, Hamedifar H. Immune checkpoints and cancer immunotherapies: insights into newly potential receptors and ligands. Ther Adv Vaccines Immunother. (2023) 11:25151355231192043. doi: 10.1177/25151355231192043
37. Gaikwad S, Agrawal MY, Kaushik I, Ramachandran S, Srivastava SK. Immune checkpoint proteins: Signaling mechanisms and molecular interactions in cancer immunotherapy. Semin Cancer Biol. (2022) 86:137–50. doi: 10.1016/j.semcancer.2022.03.014
38. Yadav D, Kwak M, Chauhan PS, Puranik N, Lee PCW, Jin J-O. Cancer immunotherapy by immune checkpoint blockade and its advanced application using bio-nanomaterials. Semin Cancer Biol. (2022) 86:909–22. doi: 10.1016/j.semcancer.2022.02.016
39. Karpathiou G, Chauleur C, Mobarki M, Peoc’h M. The immune checkpoints CTLA-4 and PD-L1 in carcinomas of the uterine cervix. Pathol - Res Pract. (2020) 216:152782. doi: 10.1016/j.prp.2019.152782
40. Kroon P, Frijlink E, Iglesias-Guimarais V, Volkov A, Van Buuren MM, Schumacher TN, et al. Radiotherapy and cisplatin increase immunotherapy efficacy by enabling local and systemic intratumoral T-cell activity. Cancer Immunol Res. (2019) 7:670–82. doi: 10.1158/2326-6066.CIR-18-0654
41. Zhou Z, Zhao Y, Chen S, Cui G, Fu W, Li S, et al. Cisplatin promotes the efficacy of immune checkpoint inhibitor therapy by inducing ferroptosis and activating neutrophils. Front Pharmacol. (2022) 13:870178. doi: 10.3389/fphar.2022.870178
42. Boopathi E, Den RB, Thangavel C. Innate immune system in the context of radiation therapy for cancer. Cancers. (2023) 15:3972. doi: 10.3390/cancers15153972
43. Monjazeb AM, Schalper KA, Villarroel-Espindola F, Nguyen A, Shiao SL, Young K. Effects of radiation on the tumor microenvironment. Semin Radiat Oncol. (2020) 30:145–57. doi: 10.1016/j.semradonc.2019.12.004
44. Liu S, Wang W, Hu S, Jia B, Tuo B, Sun H, et al. Radiotherapy remodels the tumor microenvironment for enhancing immunotherapeutic sensitivity. Cell Death Dis. (2023) 14:679. doi: 10.1038/s41419-023-06211-2
45. Yang W, Lu Y, Yang Y, Kang J, Jin Y, Wang H. Expressions of programmed death (PD)-1 and PD-1 ligand (PD-L1) in cervical intraepithelial neoplasia and cervical squamous cell carcinomas are of prognostic value and associated with human papillomavirus status. J Obstet Gynaecol. (2017) 43:1602–12. doi: 10.1111/jog.13411
46. D’Alessandris N, Palaia I, Pernazza A, Tomao F, Di Pinto A, Musacchio L, et al. PD-L1 expression is associated with tumor infiltrating lymphocytes that predict response to NACT in squamous cell cervical cancer. Virchows Arch. (2021) 478:517–25. doi: 10.1007/s00428-020-02922-5
47. Sherer MV, Kotha NV, Williamson C, Mayadev J. Advances in immunotherapy for cervical cancer: recent developments and future directions. Int J Gynecol Cancer. (2022) 32:281–7. doi: 10.1136/ijgc-2021-002492
48. Smalley Rumfield C, Roller N, Pellom ST, Schlom J, Jochems C. Therapeutic vaccines for HPV-associated Malignancies. ITT. (2020) 9:167–200. doi: 10.2147/ITT.S273327
49. Van Poelgeest MIE, Visconti VV, Aghai Z, Van Ham VJ, Heusinkveld M, Zandvliet ML, et al. Potential use of lymph node-derived HPV-specific T cells for adoptive cell therapy of cervical cancer. Cancer Immunol Immunother. (2016) 65:1451–63. doi: 10.1007/s00262-016-1892-8
50. Stevanović S, Draper LM, Langhan MM, Campbell TE, Kwong ML, Wunderlich JR, et al. Complete regression of metastatic cervical cancer after treatment with human papillomavirus–targeted tumor-infiltrating T cells. JCO. (2015) 33:1543–50. doi: 10.1200/JCO.2014.58.9093
51. Stevanović S, Helman SR, Wunderlich JR, Langhan MM, Doran SL, Kwong MLM, et al. A phase II study of tumor-infiltrating lymphocyte therapy for human papillomavirus–associated epithelial cancers. Clin Cancer Res. (2019) 25:1486–93. doi: 10.1158/1078-0432.CCR-18-2722
52. Doran SL, Stevanović S, Adhikary S, Gartner JJ, Jia L, Kwong MLM, et al. T-cell receptor gene therapy for human papillomavirus–associated epithelial cancers: A first-in-human, phase I/II study. JCO. (2019) 37:2759–68. doi: 10.1200/JCO.18.02424
53. Jin J, Gkitsas N, Fellowes VS, Ren J, Feldman SA, Hinrichs CS, et al. Enhanced clinical-scale manufacturing of TCR transduced T-cells using closed culture system modules. J Transl Med. (2018) 16:13. doi: 10.1186/s12967-018-1384-z
54. Nagarsheth NB, Norberg SM, Sinkoe AL, Adhikary S, Meyer TJ, Lack JB, et al. TCR-engineered T cells targeting E7 for patients with metastatic HPV-associated epithelial cancers. Nat Med. (2021) 27:419–25. doi: 10.1038/s41591-020-01225-1
55. Coleman RL, Lorusso D, Gennigens C, González-Martín A, Randall L, Cibula D, et al. Efficacy and safety of tisotumab vedotin in previously treated recurrent or metastatic cervical cancer (innovaTV 204/GOG-3023/ENGOT-cx6): a multicentre, open-label, single-arm, phase 2 study. Lancet Oncol. (2021) 22:609–19. doi: 10.1016/S1470-2045(21)00056-5
56. Massobrio R, Bianco L, Campigotto B, Attianese D, Maisto E, Pascotto M, et al. New frontiers in locally advanced cervical cancer treatment. JCM. (2024) 13:4458. doi: 10.3390/jcm13154458
57. Yuan Y, Cai X, Shen F, Ma F. HPV post-infection microenvironment and cervical cancer. Cancer Lett. (2021) 497:243–54. doi: 10.1016/j.canlet.2020.10.034
58. Huh WK, Brady WE, Fracasso PM, Dizon DS, Powell MA, Monk BJ, et al. Phase II study of axalimogene filolisbac (ADXS-HPV) for platinum-refractory cervical carcinoma: An NRG oncology/gynecologic oncology group study. Gynecol Oncol. (2020) 158:562–9. doi: 10.1016/j.ygyno.2020.06.493
59. Petit RG, Mehta A, Jain M, Gupta S, Nagarkar R, Kumar V, et al. ADXS11–001 immunotherapy targeting HPV-E7: final results from a Phase II study in Indian women with recurrent cervical cancer. J Immunother Cancer. (2014) 2:P92, 2051–1426-2-S3-P92. doi: 10.1186/2051-1426-2-S3-P92
60. Miles B, Safran HP, Monk BJ. Therapeutic options for treatment of human papillomavirus-associated cancers - novel immunologic vaccines: ADXS11–001. Gynaecol Oncol Res Pract. (2017) 4:10. doi: 10.1186/s40661-017-0047-8
61. Krupar R, Imai N, Miles B, Genden E, Misiukiewicz K, Saenger Y, et al. Abstract LB-095: HPV E7 antigen-expressing Listeria-based immunotherapy (ADXS11-001) prior to robotic surgery for HPV-positive oropharyngeal cancer enhances HPV-specific T cell immunity. Cancer Res. (2016) 76:LB–095-LB-095. doi: 10.1158/1538-7445.AM2016-LB-095
62. Maciag PC, Radulovic S, Rothman J. The first clinical use of a live-attenuated Listeria monocytogenes vaccine: A Phase I safety study of Lm-LLO-E7 in patients with advanced carcinoma of the cervix. Vaccine. (2009) 27:3975–83. doi: 10.1016/j.vaccine.2009.04.041
63. Floudas CS, Goswami M, Donahue RN, Pastor DM, Redman JM, Brownell I, et al. Novel combination immunotherapy and clinical activity in patients with HPV-associated cancers: A nonrandomized clinical trial. JAMA Oncol. (2025) 11(4):394–9. doi: 10.1001/jamaoncol.2024.6998
64. Yoshida-Court K, Gjyshi O, O’Hara M, Lin L, Jhingran A, Joyner M, et al. 674 IMMUNOCERV, an ongoing phase II trial combining PDS0101, an HPV-specific T cell immunotherapy, with chemotherapy and radiation for treatment of locally advanced cervical cancers. J Immunother Cancer. (2022) 10. doi: 10.1136/jitc-2022-SITC2022.0674
65. Zhou Y, Rassy E, Coutte A, Achkar S, Espenel S, Genestie C, et al. Current standards in the management of early and locally advanced cervical cancer: update on the benefit of neoadjuvant/adjuvant strategies. Cancers. (2022) 14:2449. doi: 10.3390/cancers14102449
66. Huang X, He M, Peng H, Tong C, Liu Z, Zhang X, et al. Genomic profiling of advanced cervical cancer to predict response to programmed death-1 inhibitor combination therapy: a secondary analysis of the CLAP trial. J Immunother Cancer. (2021) 9:e002223. doi: 10.1136/jitc-2020-002223
67. Schernberg A, Bockel S, Annede P, Fumagalli I, Escande A, Mignot F, et al. Tumor shrinkage during chemoradiation in locally advanced cervical cancer patients: prognostic significance, and impact for image-guided adaptive brachytherapy. Int J Radiat OncologyBiologyPhys. (2018) 102:362–72. doi: 10.1016/j.ijrobp.2018.06.014
68. Reuzé S, Orlhac F, Chargari C, Nioche C, Limkin E, Riet F, et al. Prediction of cervical cancer recurrence using textural features extracted from 18F-FDG PET images acquired with different scanners. Oncotarget. (2017) 8:43169–79. doi: 10.18632/oncotarget.17856
69. Chardin L, Leary A. Immunotherapy in ovarian cancer: thinking beyond PD-1/PD-L1. Front Oncol. (2021) 11:795547. doi: 10.3389/fonc.2021.795547
70. Zhu J, Yan L, Wang Q. Efficacy of PD-1/PD-L1 inhibitors in ovarian cancer: a single-arm meta-analysis. J Ovarian Res. (2021) 14:112. doi: 10.1186/s13048-021-00862-5
71. Dumitru A, Dobrica E-C, Croitoru A, Cretoiu SM, Gaspar BS. Focus on PD-1/PD-L1 as a therapeutic target in ovarian cancer. IJMS. (2022) 23:12067. doi: 10.3390/ijms232012067
72. Mezache L, Paniccia B, Nyinawabera A, Nuovo GJ. Enhanced expression of PD L1 in cervical intraepithelial neoplasia and cervical cancers. Modern Pathol. (2015) 28:1594–602. doi: 10.1038/modpathol.2015.108
73. Koncar RF, Feldman R, Bahassi EM, Hashemi Sadraei N. Comparative molecular profiling of HPV-induced squamous cell carcinomas. Cancer Med. (2017) 6:1673–85. doi: 10.1002/cam4.1108
74. Heeren AM, Punt S, Bleeker MC, Gaarenstroom KN, van der Velden J, Kenter GG, et al. Prognostic effect of different PD-L1 expression patterns in squamous cell carcinoma and adenocarcinoma of the cervix. Modern Pathol. (2016) 29:753–63. doi: 10.1038/modpathol.2016.64
75. Chung HC, Ros W, Delord J-P, Perets R, Italiano A, Shapira-Frommer R, et al. Efficacy and safety of pembrolizumab in previously treated advanced cervical cancer: results from the phase II KEYNOTE-158 study. JCO. (2019) 37:1470–8. doi: 10.1200/JCO.18.01265
76. Huang RSP, Haberberger J, Murugesan K, Danziger N, Hiemenz M, Severson E, et al. Clinicopathologic and genomic characterization of PD-L1-positive uterine cervical carcinoma. Modern Pathol. (2021) 34:1425–33. doi: 10.1038/s41379-021-00780-3
77. Frederick J, Guerrero L, Evans T, Barreto J, Kulangara K. Abstract 749: Pathology training for combined positive score algorithm for the assessment of PD-L1 in human cancer tissues. Cancer Res. (2020) 80:749–9. doi: 10.1158/1538-7445.AM2020-749
78. Naumann RW, Hollebecque A, Meyer T, Devlin M-J, Oaknin A, Kerger J, et al. Safety and efficacy of nivolumab monotherapy in recurrent or metastatic cervical, vaginal, or vulvar carcinoma: results from the phase I/II checkMate 358 trial. J Clin Oncol. (2019) 37:2825–34. doi: 10.1200/JCO.19.00739
79. Goodman AM, Piccioni D, Kato S, Boichard A, Wang H-Y, Frampton G, et al. Prevalence of PDL1 amplification and preliminary response to immune checkpoint blockade in solid tumors. JAMA Oncol. (2018) 4:1237. doi: 10.1001/jamaoncol.2018.1701
80. Rotman J, Otter LASD, Bleeker MCG, Samuels SS, Heeren AM, Roemer MGM, et al. PD-L1 and PD-L2 expression in cervical cancer: regulation and biomarker potential. Front Immunol. (2020) 11:596825. doi: 10.3389/fimmu.2020.596825
81. Budczies J, Kazdal D, Menzel M, Beck S, Kluck K, Altbürger C, et al. Tumour mutational burden: clinical utility, challenges and emerging improvements. Nat Rev Clin Oncol. (2024) 21:725–42. doi: 10.1038/s41571-024-00932-9
82. Cao J, Yang X, Chen S, Wang J, Fan X, Fu S, et al. The predictive efficacy of tumor mutation burden in immunotherapy across multiple cancer types: A meta-analysis and bioinformatics analysis. Trans Oncol. (2022) 20:101375. doi: 10.1016/j.tranon.2022.101375
83. Wood MA, Weeder BR, David JK, Nellore A, Thompson RF. Burden of tumor mutations, neoepitopes, and other variants are weak predictors of cancer immunotherapy response and overall survival. Genome Med. (2020) 12:33. doi: 10.1186/s13073-020-00729-2
84. Xu Z, Dai J, Wang D, Lu H, Dai H, Ye H, et al. Assessment of tumor mutation burden calculation from gene panel sequencing data. OTT. (2019) 12:3401–9. doi: 10.2147/OTT.S196638
85. Chalmers ZR, Connelly CF, Fabrizio D, Gay L, Ali SM, Ennis R, et al. Analysis of 100,000 human cancer genomes reveals the landscape of tumor mutational burden. Genome Med. (2017) 9:34. doi: 10.1186/s13073-017-0424-2
86. Ota N, Yoshimoto Y, Darwis NDM, Sato H, Ando K, Oike T, et al. High tumor mutational burden predicts worse prognosis for cervical cancer treated with radiotherapy. Jpn J Radiol. (2022) 40:534–41. doi: 10.1007/s11604-021-01230-5
87. Takeda M, Takahama T, Sakai K, Shimizu S, Watanabe S, Kawakami H, et al. Clinical application of the foundationOne CDx assay to therapeutic decision-making for patients with advanced solid tumors. Oncol. (2021) 26:e588–96. doi: 10.1002/onco.13639
88. Gandini A, Puglisi S, Pirrone C, Martelli V, Catalano F, Nardin S, et al. The role of immunotherapy in microsatellites stable metastatic colorectal cancer: state of the art and future perspectives. Front Oncol. (2023) 13:1161048. doi: 10.3389/fonc.2023.1161048
89. Wong Y-F, Cheung T-H, Poon K-Y, Wang VW, Li JCB, Lo KW-K, et al. The role of microsatellite instability in cervical intraepithelial neoplasia and squamous cell carcinoma of the cervix. Gynecol Oncol. (2003) 89:434–9. doi: 10.1016/S0090-8258(03)00134-3
90. Noh JJ, Kim MK, Choi MC, Lee J-W, Park H, Jung SG, et al. Frequency of mismatch repair deficiency/high microsatellite instability and its role as a predictive biomarker of response to immune checkpoint inhibitors in gynecologic cancers. Cancer Res Treat. (2022) 54:1200–8. doi: 10.4143/crt.2021.828
91. Vanderwalde A, Spetzler D, Xiao N, Gatalica Z, Marshall J. Microsatellite instability status determined by next-generation sequencing and compared with PD -L1 and tumor mutational burden in 11,348 patients. Cancer Med. (2018) 7:746–56. doi: 10.1002/cam4.1372
92. Deshpande M, Romanski PA, Rosenwaks Z, Gerhardt J. Gynecological cancers caused by deficient mismatch repair and microsatellite instability. Cancers. (2020) 12:3319. doi: 10.3390/cancers12113319
93. Van Den Berg MC, Berg HF, Stokowy T, Hoivik EA, Woie K, Engerud H, et al. Expression patterns of mismatch repair proteins in cervical cancer uncover independent prognostic value of MSH-2. Int J Gynecol Cancer. (2024) 34:993–1000. doi: 10.1136/ijgc-2024-005377
94. Okamura K, Nagayama S, Tate T, Chan HT, Kiyotani K, Nakamura Y. Lymphocytes in tumor-draining lymph nodes co-cultured with autologous tumor cells for adoptive cell therapy. J Transl Med. (2022) 20:241. doi: 10.1186/s12967-022-03444-1
95. Heeren AM, Rotman J, Stam AGM, Pocorni N, Gassama AA, Samuels S, et al. Efficacy of PD-1 blockade in cervical cancer is related to a CD8+FoxP3+CD25+ T-cell subset with operational effector functions despite high immune checkpoint levels. J Immunother Cancer. (2019) 7:43. doi: 10.1186/s40425-019-0526-z
96. Delclaux I, Ventre KS, Jones D, Lund AW. The tumor-draining lymph node as a reservoir for systemic immune surveillance. Trends Cancer. (2024) 10:28–37. doi: 10.1016/j.trecan.2023.09.006
97. Hendriks LE, Rouleau E, Besse B. Clinical utility of tumor mutational burden in patients with non-small cell lung cancer treated with immunotherapy. Transl Lung Cancer Res. (2018) 7:647–60. doi: 10.21037/tlcr.2018.09.22
98. Zang Y, Lee JJ. Adaptive clinical trial designs in oncology. Chin Clin Oncol. (2014) 3:49. doi: 10.3978/j.issn.2304-3865.2014.06.04
99. Antoniou M, Jorgensen AL, Kolamunnage-Dona R. Biomarker-guided adaptive trial designs in phase II and phase III: A methodological review. PloS One. (2016) 11:e0149803. doi: 10.1371/journal.pone.0149803
100. Ramírez AT, Valls J, Baena A, Rojas FD, Ramírez K, Álvarez R, et al. Performance of cervical cytology and HPV testing for primary cervical cancer screening in Latin America: an analysis within the ESTAMPA study. Lancet Reg Health - Am. (2023) 26:100593. doi: 10.1016/j.lana.2023.100593
101. Wang J, Yu Y, Tan Y, Wan H, Zheng N, He Z, et al. Artificial intelligence enables precision diagnosis of cervical cytology grades and cervical cancer. Nat Commun. (2024) 15:4369. doi: 10.1038/s41467-024-48705-3
102. Bao H, Bi H, Zhang X, Zhao Y, Dong Y, Luo X, et al. Artificial intelligence-assisted cytology for detection of cervical intraepithelial neoplasia or invasive cancer: A multicenter, clinical-based, observational study. Gynecol Oncol. (2020) 159:171–8. doi: 10.1016/j.ygyno.2020.07.099
103. Sørbye SW, Suhrke P, Revå BW, Berland J, Maurseth RJ, Al-Shibli K. Accuracy of cervical cytology: comparison of diagnoses of 100 Pap smears read by four pathologists at three hospitals in Norway. BMC Clin Pathol. (2017) 17:18. doi: 10.1186/s12907-017-0058-8
104. Zhu X, Li X, Ong K, Zhang W, Li W, Li L, et al. Hybrid AI-assistive diagnostic model permits rapid TBS classification of cervical liquid-based thin-layer cell smears. Nat Commun. (2021) 12:3541. doi: 10.1038/s41467-021-23913-3
105. Rahaman MM, Li C, Yao Y, Kulwa F, Wu X, Li X, et al. DeepCervix: A deep learning-based framework for the classification of cervical cells using hybrid deep feature fusion techniques. Comput Biol Med. (2021) 136:104649. doi: 10.1016/j.compbiomed.2021.104649
106. Spigel DR, Faivre-Finn C, Gray JE, Vicente D, Planchard D, Paz-Ares L, et al. Five-year survival outcomes from the PACIFIC trial: durvalumab after chemoradiotherapy in stage III non–small-cell lung cancer. JCO. (2022) 40:1301–11. doi: 10.1200/JCO.21.01308
107. Cheng Y, Spigel DR, Cho BC, Laktionov KK, Fang J, Chen Y, et al. Durvalumab after chemoradiotherapy in limited-stage small-cell lung cancer. N Engl J Med. (2024) 391:1313–27. doi: 10.1056/NEJMoa2404873
108. Monk BJ, Toita T, Wu X, Vázquez Limón JC, Tarnawski R, Mandai M, et al. Durvalumab versus placebo with chemoradiotherapy for locally advanced cervical cancer (CALLA): a randomised, double-blind, phase 3 trial. Lancet Oncol. (2023) 24:1334–48. doi: 10.1016/S1470-2045(23)00479-5
109. Lorusso D, Xiang Y, Hasegawa K, Scambia G, Leiva M, Ramos-Elias P, et al. Pembrolizumab or placebo with chemoradiotherapy followed by pembrolizumab or placebo for newly diagnosed, high-risk, locally advanced cervical cancer (ENGOT-cx11/GOG-3047/KEYNOTE-A18): a randomised, double-blind, phase 3 clinical trial. Lancet. (2024) 403:1341–50. doi: 10.1016/S0140-6736(24)00317-9
110. Marth C, Landoni F, Mahner S, McCormack M, Gonzalez-Martin A, Colombo N. Cervical cancer: ESMO Clinical Practice Guidelines for diagnosis, treatment and follow-up. Ann Oncol. (2017) 28:iv72–83. doi: 10.1093/annonc/mdx220
111. Cibula D, Raspollini MR, Planchamp F, Centeno C, Chargari C, Felix A, et al. ESGO/ESTRO/ESP Guidelines for the management of patients with cervical cancer – Update 2023*. Int J Gynecol Cancer. (2023) 33:649–66. doi: 10.1136/ijgc-2023-004429
112. Rodrigues M, Vanoni G, Loap P, Dubot C, Timperi E, Minsat M, et al. Nivolumab plus chemoradiotherapy in locally-advanced cervical cancer: the NICOL phase 1 trial. Nat Commun. (2023) 14:3698. doi: 10.1038/s41467-023-39383-8
113. Da Silva DM, Enserro DM, Mayadev JS, Skeate JG, Matsuo K, Pham HQ, et al. Immune activation in patients with locally advanced cervical cancer treated with ipilimumab following definitive chemoradiation (GOG-9929). Clin Cancer Res. (2020) 26:5621–30. doi: 10.1158/1078-0432.CCR-20-0776
114. Ray-Coquard IL, Kaminsky-Forrett M-C, Joly F, Montané L, Bello Roufai D, Savoye AM, et al. 819TiP COLIBRI trial (GINECO-CE108b): A multicenter, window study evaluating immune impact and safety of nivolumab in combination with ipilimumab before initial radio-chemotherapy (RTCT) treatment for locally advanced cervix cancer. Ann Oncol. (2021) 32:S770. doi: 10.1016/j.annonc.2021.08.1261
115. Garcia-Duran C, Grau F, Villacampa G, Oaknin A. ATOMICC trial: a randomized, open-label, phase II trial of anti-PD1, dostarlimab, as maintenance therapy for patients with high-risk locally advanced cervical cancer after chemoradiation. Int J Gynecol Cancer. (2022) 32:1196–200. doi: 10.1136/ijgc-2022-003370
116. Li K, Chen J, Hu Y, Wang Y-Z, Shen Y, Chen G, et al. Neoadjuvant chemotherapy plus camrelizumab for locally advanced cervical cancer (NACI study): a multicentre, single-arm, phase 2 trial. Lancet Oncol. (2024) 25:76–85. doi: 10.1016/S1470-2045(23)00531-4
117. Oka H, Emori Y, Sasaki H, Shiraishi Y, Yoshinaga K, Kurimoto T. Anti-Tumor Mechanism of Z-100, an Immunomodulatory Arabinomannan Extracted from Mycobacterium tuberculosis Strain Aoyama B, on Pulmonary Metastases of B16F10 Melanoma: Restoration of Helper T Cell Responses via Suppression of Glucocorticoid-Genesis. Microbiol Immunol. (2002) 46:343–51. doi: 10.1111/j.1348-0421.2002.tb02705.x
118. Sugiyama T, Fujiwara K, Ohashi Y, Yokota H, Hatae M, Ohno T, et al. Phase III placebo-controlled double-blind randomized trial of radiotherapy for stage IIB–IVA cervical cancer with or without immunomodulator Z-100: a JGOG study. Ann Oncol. (2014) 25:1011–7. doi: 10.1093/annonc/mdu057
119. Shehaj I, Schröder M, Linz VC, Krajnak S, Almstedt K, Stewen K, et al. Occurrence and management of immunotherapy-associated adverse events in patients with gynecological cancers. Cancers. (2024) 16:1371. doi: 10.3390/cancers16071371
120. Martins F, Sofiya L, Sykiotis GP, Lamine F, Maillard M, Fraga M, et al. Adverse effects of immune-checkpoint inhibitors: epidemiology, management and surveillance. Nat Rev Clin Oncol. (2019) 16:563–80. doi: 10.1038/s41571-019-0218-0
121. Feng L, Shi Q, Wang S, Zhao Y, Wu H, Wei L, et al. The outcome of advanced and recurrent cervical cancer patients treated with first-line platinum and paclitaxel with or without indication for immune checkpoint inhibitors: the comparative study. BMC Cancer. (2024) 24:1267. doi: 10.1186/s12885-024-12989-x
122. Qi L, Li N, Lin A, Wang X, Cong J. Efficacy and safety of pembrolizumab on cervical cancer: A systematic review and single-arm meta-analysis. Front Oncol. (2022) 12:910486. doi: 10.3389/fonc.2022.910486
123. Adachi T, Matsui T, Okata-Karigane U, Takahashi C, Tahara U, Hyodo M, et al. Delayed and immediate cutaneous adverse events during pembrolizumab combination chemotherapy against cervical cancer: Case series. J Dermatol. (2025) 52:132–7. doi: 10.1111/1346-8138.17521
124. Malmberg R, Zietse M, Dumoulin DW, Hendrikx JJMA, Aerts JGJV, van der Veldt AAM, et al. Alternative dosing strategies for immune checkpoint inhibitors to improve cost-effectiveness: a special focus on nivolumab and pembrolizumab. Lancet Oncol. (2022) 23:e552–61. doi: 10.1016/S1470-2045(22)00554-X
125. Green AK. Challenges in assessing the cost-effectiveness of cancer immunotherapy. JAMA Netw Open. (2021) 4:e2034020. doi: 10.1001/jamanetworkopen.2020.34020
126. Mucherino S, Lorenzoni V, Triulzi I, Del Re M, Orlando V, Capuano A, et al. Cost-effectiveness of treatment optimisation with biomarkers for immunotherapy in solid tumours: A systematic review. Cancers. (2024) 16:995. doi: 10.3390/cancers16050995
Keywords: cervical cancer, chemoradiation, immunotherapy, immune checkpoint inhibitors, clinical trial, biomarkers, personalized treatment
Citation: Kouhen F, El Ghanmi A, Inghaoun H, Miftah H, Ghazi B and Badou A (2025) The promise of PD1/PDL1 targeted immunotherapy in locally advanced cervical cancer: a game-changer for patients outcome? Front. Immunol. 16:1573576. doi: 10.3389/fimmu.2025.1573576
Received: 09 February 2025; Accepted: 14 April 2025;
Published: 13 May 2025.
Edited by:
Vinay Kumar, The Pennsylvania State University, United StatesReviewed by:
Nidhi Sharma, The University of Chicago, United StatesLopamudra Das Ghosh, Texas A and M University, United States
Copyright © 2025 Kouhen, El Ghanmi, Inghaoun, Miftah, Ghazi and Badou. This is an open-access article distributed under the terms of the Creative Commons Attribution License (CC BY). The use, distribution or reproduction in other forums is permitted, provided the original author(s) and the copyright owner(s) are credited and that the original publication in this journal is cited, in accordance with accepted academic practice. No use, distribution or reproduction is permitted which does not comply with these terms.
*Correspondence: Abdallah Badou, YWJkYWxsYWguYmFkb3VAdW5pdmgyYy5tYQ==