- 1Jiangsu Key Laboratory of Sericultural Biology and Biotechnology, School of Biotechnology, Jiangsu University of Science and Technology, Zhenjiang, China
- 2The Key Laboratory of Silkworm and Mulberry Genetic Improvement, Ministry of Agriculture, Sericultural Research Institute, Chinese Academy of Agricultural Science, Zhenjiang, China
- 3School of Pharmaceutical Sciences, Wenzhou Medical University, Wenzhou, China
- 4Nursing School, Zhenjiang College, Zhenjiang, China
Vine tea (Ampelopsis grossedentata) is a widely used Chinese herb with a long history of application in southern China, known for its notable anti-infective, antibacterial and immunomodulatory pharmacological properties. It has potential for application in aquaculture as an inexpensive and readily available dietary supplement, promoting growth, antioxidant activity, and immune regulation in fish. However, there have been very few studies investigating the effects of vine tea on fish miRNAs. Loach is an economically important freshwater fish species, highly valued for its delicious flavor, but research on its miRNA relatively limited. To examine immune-related miRNAs in loach and to further determine the antibacterial immune mechanisms of vine tea, we performed small RNA sequencing analysis of loach liver tissue before and after treatment with vine tea extract. The results showed that vine tea could affect the antibacterial immune activity of loach through the miRNA regulation. A total of 25 differentially expressed miRNAs were identified in liver tissues, and KEGG pathway analysis revealed that most of these miRNAs were involved in innate immune responses such as autophagy, lysosomes, endocytosis, and pattern receptor signaling pathways. To the best of our knowledge, this is the first study to profile miRNA expression in loach after treatment with vine tea extract. This work deepens the understanding of the role of loach miRNAs in the immune system and opens new prospects for application of vine tea in aquaculture.
1 Introduction
MicroRNAs (miRNAs) are ~22-nucleotide highly conserved endogenous RNAs that govern gene expression post-transcriptionally across diverse living organisms (1, 2). Single-stranded miRNAs are often completely or incompletely complementarily paired with the 3’ untranslated region of the target mRNA, which leads to miRNA degradation or blocks the translation process of miRNA (3). The first miRNA (Lin-4) was discovered in Caenorhabditis elegans in 1993 (4), since then thousands of miRNAs have been extensively studied in animals, plants and viruses. Previous studies have shown that miRNAs regulate of a variety of biological processes in vivo, including development tissue, signal transduction, proliferation, apoptosis, differentiation, immune response, inflammation, and others (5–7). Given their importance, a large number of miRNAs have been identified by high-throughput sequencing and bioinformatics in fish, such as zebrafish (Danio rerio), grass carp (Ctenopharyngodon idellus), yellow croaker (Pseudosciaena crocea), tilapia (Oreochromis niloticus), mandarin fish (Siniperca chuatsi), and Blunt snout bream (Megalobrama amblycephala), etc. (8). Multiple studies show that miRNAs play crucial roles in fish physiology, nutrition, immune response, and immune escape (9). For example, miR-146a (10), miR1338-5p (11), miR-122 (12), and miR-181b-5p (13) regulate long-chain fatty acid synthesis and are involved in cholesterol metabolism, glycolipid metabolism, and musculogenesis, etc.; miR-146a (14), miR-3570 (15), miR-192 (16), miR-200a-3p (17)and miR-210 (18) play roles in antiviral and antibacterial immunity as well as promoting the immune response in fish. However, the miRNA network regulating fish immunity in aquaculture remains poorly understood, requiring further functional and mechanistic studies.
The loach (Misgurnus anguillicaudatus) is a Cobitidae family in the Cypriniformes order and is widely distributed throughout eastern Asia, including China, Japan, Korea and other countries (19, 20). Known for its high protein and low-fat contents, it is an excellent freshwater aquaculture species favored by consumers (21). Beyond its economic and nutritional value, it also serves as an important experimental animal model in research on growth performance, immune function, and other aspects. During culture, factors, including water quality, temperature and stocking density suppress immune function of loach, increasing their susceptibility to proto-microbial infections, specifically from Gram-negative bacteria. Aeromonas hydrophila, a Gram-negative, rod-shaped, highly virulent bacterium (22), is commonly occur in freshwater aquaculture and can cause ulcers, ascites, septicaemia, erythema, and liver damage in a wide range of fish species (23–25), leading to high mortality rates and significant economic losses, challenges that also influence the loach aquaculture industry.
In aquaculture, antibiotics are commonly used treat bacterial infections, however, their misuse not only leads to the emergence of drug resistance in pathogenic bacteria, but also threatens the ecological environment and public health (26, 27). In addition, aquaculture is often challenged by immunosuppression due to high-density farming, environmental stressors, and frequent pathogen exposure, leading to higher disease susceptibility and mortality rates. These challenges have created an urgent need for natural dietary supplements that can strengthen aquaculture immunity and reduce dependence on antibiotics (28). There are several lines of evidence that phytobiotics may be the future trend for antibiotic replacement in aquaculture, and they contain bioactive compounds with immune-enhancing and bactericidal properties (29). However, studies on plant-derived drugs related to antibacterial infection of loach and miRNA of loach were scare.
Vine tea (Ampelopsis grossedentata) is a precious traditional Chinese herb used as both medicine and food. It is a vine plant in the Ampelopsis genus of the Vitaceae family, widely distributed in southern regions (30–32). Vine tea has gained increasing attention due to its rich content, particularly its leaves, which are known as the ‘king of flavonoids’ they contain dihydromyricetin, providing various health benefits such as hypolipidemic, hypotensive, anti-inflammatory, antioxidant, antimicrobial, and immune boosting effects making it a popular health tea drink (33–35). Recent pharmacological research has shown that dihydromyricetin improves immune function via modulating cytokine production, lowering oxidative stress, and altering inflammatory pathways, including NF-κB signaling pathway (36, 37). This is because, Chinese government allowed to use its leaves as a feed ingredient for poultry and livestock, thereby improving the health of the organism and increasing the level of nutrition (38). In aquaculture and farm animals, Supplementation with vine tea extracts is a promising technique for improving fish health by increasing innate and adaptive immune responses, antioxidant defenses, and gut microbiome balance. Such properties suggest that vine tea could be used as functional feed additive to promote more sustainable and resilient aquaculture practices (39). For example, a previous study shown that the addition of moderate amounts of vine tea extracts to feeds promotes growth, induce antioxidant activity, and boost immunity in fish, supporting their healthy culture (40). However, further research is required to confirm the potential therapeutic role of vine tea in protecting fish against bacterial infection.
MiRNAs have been widely studied in fish nutrition and immune regulation (41), but no studies have been published on the influence of vine tea on miRNA expression profiles in fish. In light of this, we pre-added vine tea extract to the feed and investigated the dynamics of the miRNA transcriptome in the liver of loach following A. hydrophila infection. This study will, for the first time, demonstrate moderating effect of vine tea on fish after bacterial infection using comparative miRNA analysis, and investigate the immunomodulatory mechanism of vine tea. Our results contribute to better understanding of the role of miRNAs in loach and highlight the potential of vine tea extract as a feed additive for aquaculture.
2 Materials and methods
2.1 Feed and bacteria preparation
The vine tea extract was dissolved, and the solution was sprayed on the feed to achieve a final concentration of 0.01 kg vine tea/kg. The feed was air-dried and sealed and stored at 4°C. A. hydrophila was the strain available in the laboratory. A. hydrophila at -80°C was taken and added to fresh medium, recovered overnight and the concentration was determined, before the bacterial solution was stored at 4°C for subsequent use.
2.2 Animal trial and sample collection
The loaches for this experiment were obtained from a river fresh market in Wenzhou City, Zhejiang Province, China. The loach was transported to an aquarium and reared at room temperature of 25°C for 12 h light/dark cycle. Feed regular feed twice a day for a few days. After one week of domestication, 40 healthy loach samples (mean weight 10 ± 0.5 g) were randomly divided into two groups. The treatment group (AG+) was fed with 2.5 g of mixed feed with vine tea extract at a final concentration of 1 mg/ml, while the control group (AG-) group was fed with equal amount of normal feed. A. hydrophila suspension was added to the treatment and control groups, respectively, after 3 hours to maintain the bacterial concentration in the water column at 1 × 105 CFU/ml. Each treatment group included 20 loaches to ensure sufficient biological replication. Twenty-four hours after bacterial infestation, three loaches from each of the treatment and control groups were selected for execution, and liver tissues were collected and stored at -80°C after liquid nitrogen flash freezing until total RNA was extracted for small RNA sequencing. All experimental procedures were conducted following standardized protocols to ensure consistency and reproducibility.
2.3 Construction and sequencing of two small RNA libraries
Total RNA was extracted from liver tissues of the treatment and control groups using Trizol reagent (Japan, TAKARA) according to the manufacturer’s instructions. RNA integrity was assessed by RNA Integrity Number (RIN) values in an Agilent 2100 Bioanalyzer (Agilent Technologies Inc, California, USA). RNA concentration and purity of were determined by measuring OD260 and OD260/OD280 using a NanoDrop 2000 Spectrophotometer (Thermo Scientific, Waltham, Massachusetts, USA). Libraries were prepared following the procedure of the NEB Next Multiplex Small RNA Library Prep Set for Illumina kit (New England Biolabs Inc, USA). Briefly, RNA were ligated to 5’ and 3’ sequencing junctions using ligase, then reverse transcribed into cDNA using RT primers. The cDNA was subsequently amplified by PCR with the adaptor specific primers. The purified PCR products were separated by 15% PAGE gel electrophoresis to construct small RNA library. Libraries concentrations were detected using an Agilent 2100 Bioanalyzer, and the quality of the libraries was checked using an Agilent High Sensitivity DNA Kit (Agilent Technologies Inc, USA). Finally, Single-End mode sequencing was performed on an Illumina sequencer, following the procedure of Personal Biotechnology Company Limited (China, Shanghai).
2.4 Sequencing reads analysis
Raw reads generated by high-throughput sequencing were filtered using an internally developed script to remove reads containing splices and low quality reads (average sequencing quality < 20). In addition, reads containing poly - N were removed, resulting in clean data. The number of clean reads with sequence lengths between 18 nt and 36 nt was then counted for each of the two libraries. After de-duplication of identical sequences within individual samples, the unique reads were blasted against the Rfam database (http://sanger.ac.uk/pub/databases/Rfam/) to remove four known types of ncRNAs, including rRNA, tRNA, snRNA and snoRNA. Reads that were not annotated as the above ncRNAs were subsequently aligned to mature miRNA sequences from all animals listed in miRBase database 22.0 (http://www.mirbase.org/) to identify conserved miRNAs, allowing up to two mismatch.
2.5 Differential analysis of miRNAs
Based on the number of sequences mapped to conserved miRNAs, the reads counts of statistically measured miRNAs were used to represent miRNA expression levels in each sample. To identify differentially expressed miRNAs between treatment and control groups, miRNAs counts were standardized. Differential expression analysis was performed using DESeq software, with the criterion for differential expression defined as an absolute log2 fold change (treatment group/control group) greater than 1. In addition, a P-value < 0.05 considered statistically significant.
2.6 Prediction and enrichment analysis of target genes
To further investigate the potential function of significantly differentially expressed miRNAs after vine tea extract treatment, target gene prediction was performed using miranda software. The 3’UTR sequences of homologous species genes were used as custom targets, 25 differentially expressed miRNAs were used as custom miRNAs. The miRNA threshold was set at a free energy of less than -20 kcal/mol. Enrichment analysis of the predicted target genes was performed with Gene Ontology (GO). First, all the predicted target genes were mapped to each GO term, the number of target genes in each term was counted, and P-value (the criterion for significant enrichment is P-value<0.05) were calculated by the hypergeometric distribution method, with the whole genome as the background. GO terms with P-values < 0.05 were considered significantly enriched. In addition, Kyoto Encyclopedia of Genes and Genomes (KEGG) enrichment analysis was conducted to better understand the biological functions of the target genes and to identify significantly enriched pathways.
2.7 Validation of differentially expressed miRNAs
To validate the differentially expressed miRNAs identified by high-throughput sequencing, six miRNAs were randomly selected for real-time fluorescence quantitative PCR (RT-qPCR) analysis. Forward primers based on mature miRNA sequence were designed by Primer5 software, and a universal reverse primer was used. Details of the forward primers and corresponding mature miRNAs are shown in Table 1. RNA reverse transcription was performed using the same total RNA samples as those used for library construction, following the instructions of the miRNA 1st Strand cDNA Synthesis Kit (by slicing A) (Vazyme, Nanjing, China). Subsequently, the qPCR reaction mixture was prepared according to the instructions of the TransStart® Top Green qPCR SuperMix (+Dyei) (TransGen, Beijing) kit, including 1μL template cDNA, 0.5 μL of each primer (10 μmol·L-1), 10uL Top Green qPCR SuperMix and 8uL of RNase-free water. Finally, the reaction program was set up on a QuantStudio™ Real-Time PCR System (ABI, USA): 94°C for 30 s, 94°C for 5 s, 55°C for 15 s, 72°C for 10 s, and 40 cycles. The relative expression levels of miRNAs were assessed by the 2-ΔΔCt method, and β-actin gene was used as the internal control for normalization. All experiments for each miRNA were performed in triplicate.
3 Results
3.1 Summary of miRNA sequencing data
Liver tissues of pre-treated with vine tea extract before A. hydrophila (AG+) and tissues directly infected without pre-treatment (AG-) were used as samples for miRNA library construction and deep sequencing on Illumina platform. A summary of the two libraries is presented in Table 2. Sequencing generated 16,239,217 and 19,036,596 raw reads from the AG- and AG+ liver samples, respectively. After filtering of raw reads, including adaptor removal and mass clipping, a total of 14,660,513 and 17,102,503 clean reads (≥ 18 nt in length) were obtained for subsequent analysis. After removing duplicate identical sequences within each libraries, 350,436 and 343,509 unique reads were identified, respectively. The length distribution of the clean reads in the two libraries is shown in Figure 1, with the majority of reads distributed between 20–23 nt consistent with known size range of functional s RNAs (usually 20 ~ 24 nt) (42).
The read length distribution were broadly similar between the two libraries, with 22nt reads showing the highest abundance, accounting for 34.80% and 36.41% of the clean reads in the AG– and AG+ libraries, respectively, followed by reads 21, 23 and 20nt in length. The clean reads were then mapped to the Rfam and miRBase databases, resulting in 13,872,720 and 16,260,602 annotated reads, corresponding to 94.63% and 95.08% of the clean reads from the AG- and AG+ libraries, respectively (Figure 2). These results indicated that most reads were successfully annotated to non-coding RNAs (rRNA, tRNA, snRNA, and snoRNA) or miRNAs, confirming the high quality of the sequencing data. In addition, the number of unique reads annotated to the small RNA database was 109,066 and 92,816 for the AG– and AG+ libraries respectively.
3.2 Differential expression of miRNAs
To identify conserved miRNAs in the AG- and AG+ libraries, data from both libraries were compared with mature miRNAs from all animals in the miRBase database. The results demonstrated that 924 and 982 conserved miRNAs were screened in the AG- and AG+, respectively, with 807 miRNAs were shared between the two libraries (Table 2, Additional File 1). Examination of the expression profiles of these miRNAs revealed that read counts varied widely, ranging from 0 to millions, indicating substantial differences in expression levels among different miRNAs. For example, manu-undef-553 exhibited the highest expression in both samples, with an average of 6,650,403 reads, followed by manu-mir-122-3 (1,603,475 reads) and manu-undef-668 (576,365 reads) (Additional File 2). To explore the anti-infection mechanisms in loach after vine tea pretreatment, it was crucial to screen differentially expressed miRNAs. In this study, analysis of miRNA expression levels between the AG- and AG+ groups revealed that 25 miRNAs were significantly differentially expressed (Figure 3). Among the 12 up-regulated miRNAs, t 2 were specific to the AG+ group, while among the 13 down-regulated miRNAs, 1 was specific to the AG- (Table 3). The number of reads for these differentially expressed miRNAs ranged from 0-24837, which is much lower than the expression of the highly abundant miRNAs mentioned above; they may play an important regulatory role in the immune response to A. hydrophila infection.
3.3 Validation of differentially expressed miRNAs by RT-qPCR
RT - qPCR was used to validate the differentially expressed miRNAs identified by high-throughput sequencing. Six immune-related miRNAs were selected for validation, include four up-regulated miRNAs (manu-mir-194-3, manu-undef-1012, manu-undef-834 and manu-undef-882) and two down-regulated miRNAs (manu-mir-19–2 and manu-mir-130-16). The results revealed that the expression patterns of these six miRNAs in the qRT-PCR assay were consistent with those observed in the high-throughput sequencing analyses (Figure 4). Although the relative expression levels used differed between the two methods, the trends in miRNAs expression were largely similar. These findings further confirmed the reliability and accuracy of the high-throughput sequencing data.
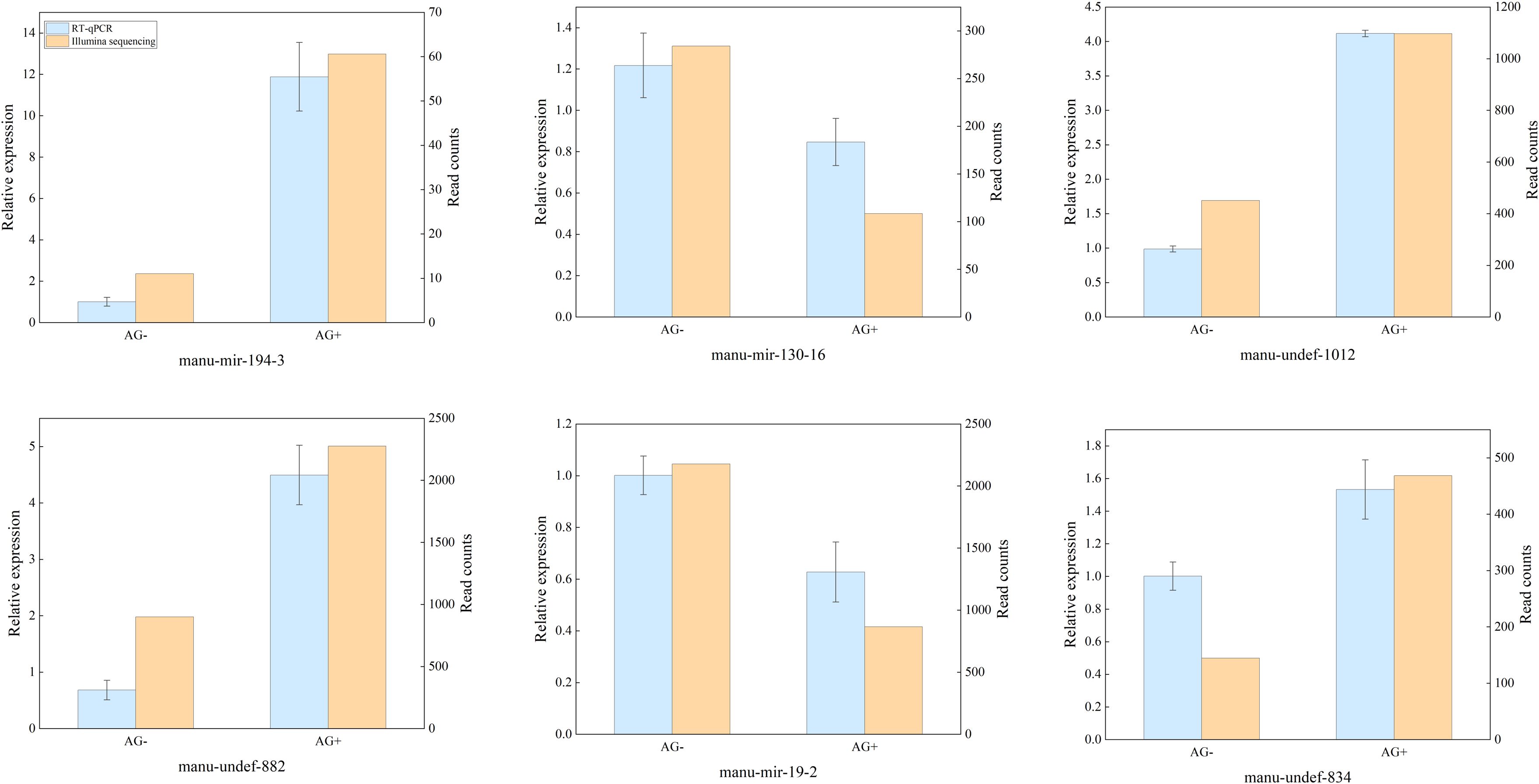
Figure 4. Comparison of relative expression levels between high-throughput sequencing and RT-qPCR results.
3.4 Targets prediction and functional analysis of miRNAs
The identification of target genes is essential for elucidating miRNA function. In this study, target prediction for the 25 differentially expressed miRNAs in loach liver was performed using miRanda software. Thousands of putative target genes were obtained through non-reference genome comparison, and multiple target sites were found on some target genes. The complexity of miRNA-mRNA interaction networks suggests that individual miRNAs can simultaneously regulate multiple target miRNAs, and that single mRNAs can, in turn, be regulated by multiple miRNAs. To infer the roles these differentially expressed miRNAs in the host, functional annotation and pathway enrichment analyses were performed on the predicted target genes.
GO enrichment and KEGG pathway analysis were performed on the predicted target genes to identify the functions of the differentially expressed miRNAs. As shown in Figure 5, the GO annotations for target genes were distributed across three main categories: molecular function, biological process and cellular component. In terms of cell components, the predicted target genes were mainly clustered in the nucleus, organelles, and cytoplasm. For biological processes, the most enriched terms include catabolic process, biosynthetic process and cellular stress response, with cellular stress being closely related to immune response. Regarding molecular functions, the target genes were primarily involved in translocation, binding, and catalytic activities.
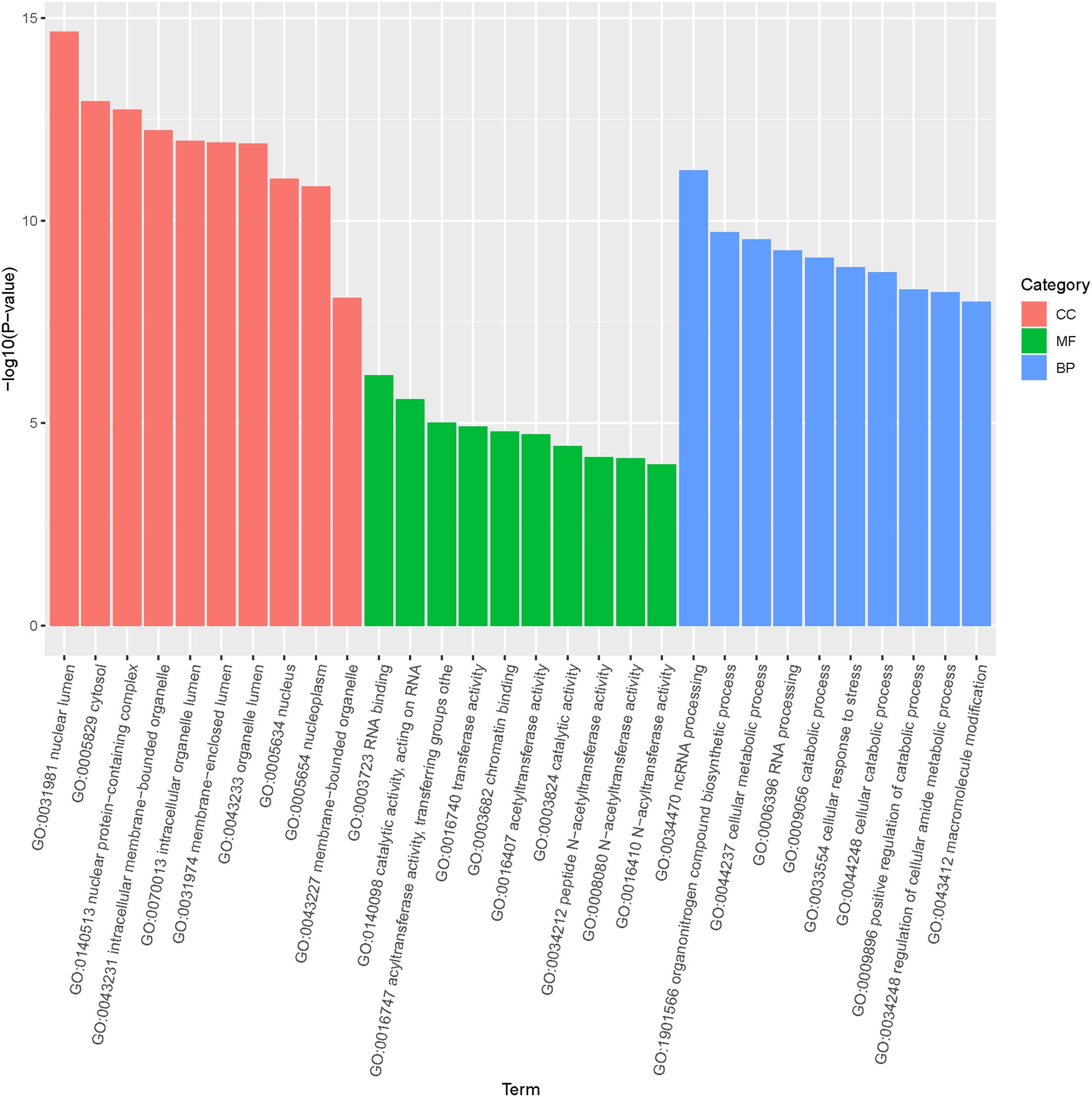
Figure 5. Gene ontology (GO) distribution of target genes of differentially expressed miRNAs in loach.
Furthermore, to identify the pathways regulated by miRNAs during bacterial infection in loach, KEGG pathway enrichment of the target genes was conducted using clusterProfiler software. The results showed a total of 160 enriched pathways, with the ‘MAPK signaling pathway’ showing the highest number of target genes (378 genes) (Additional File 3). Among the top 20 significantly enriched pathways, the target genes were mainly involved in lipid metabolism, amino acid metabolism, carbohydrate metabolism, and signal transduction-related pathways (Figure 6). Noteably, immune system-related pathways such as lysosomes, autophagy, and protein processing in the endoplasmic reticulum were also significantly enriched, implying that the majority of the differentially expressed miRNAs may exert their anti-infective effects by modulating immune-related pathways.
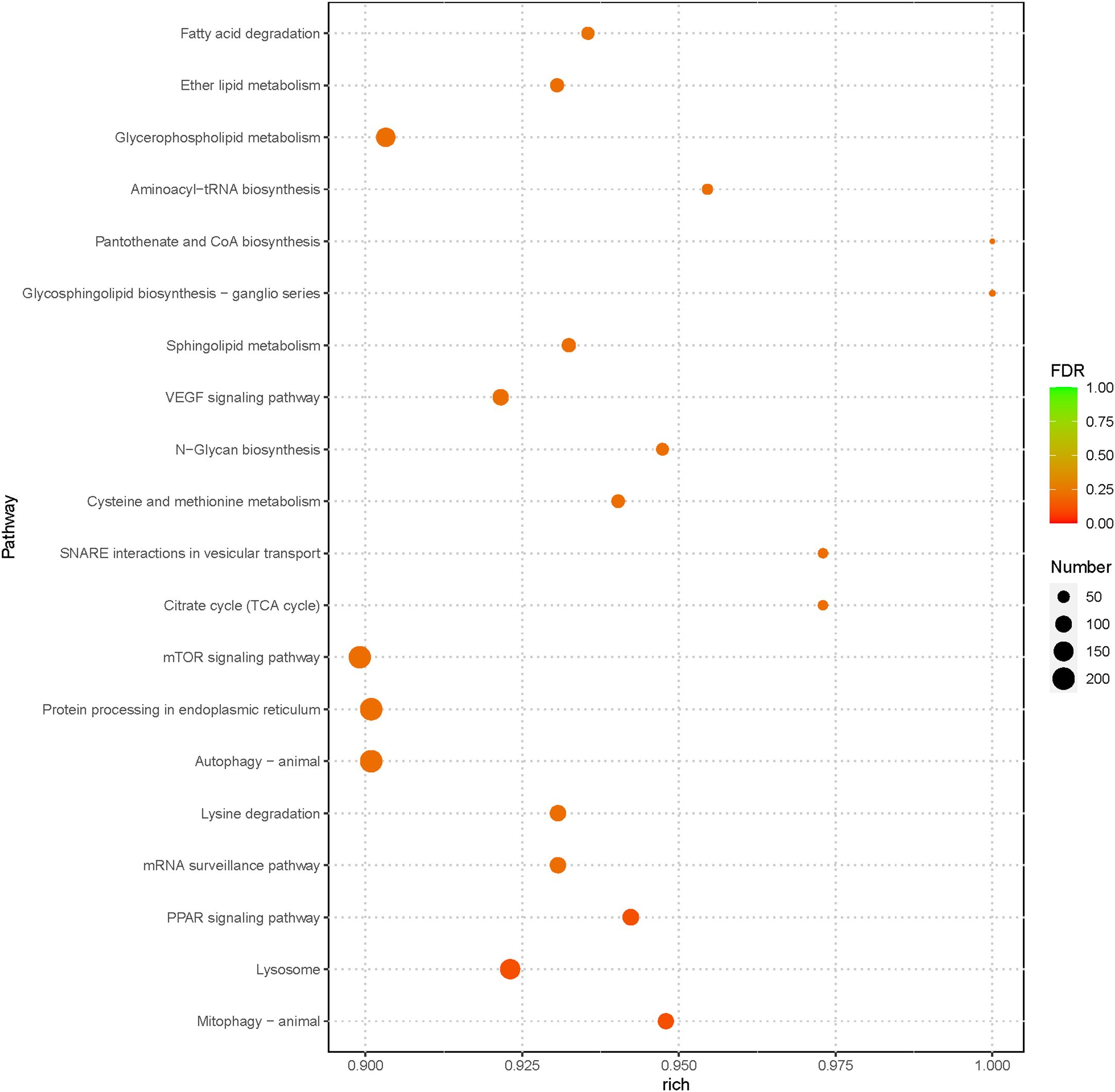
Figure 6. The 20 most enriched KEGG pathways enriched by the putative target genes of the differentially expressed miRNAs from AG- and AG+ samples.
3.5 Immune-related signaling pathways enriched for target genes
A number of studies have reported that fish miRNAs play a crucial role in immunity against pathogens by effectively regulating the immune response. In this study, vine tea extract, as a potential exogenous immunomodulator, induced changes in the expression levels of immune-related miRNAs in loach. KEGG pathway enrichment analysis showed that the target genes of differentially expressed miRNAs were enriched in multiple immune-related signaling pathways (Figure 7). Specifically, 200 and 365 target genes were annotated in the autophagy and endocytosis pathways, respectively. These pathways are regulated by the majority of differentially expressed miRNAs and represent fundamental physiological processes by which organisms respond to infectious pathogens, serving as prerequisites for the activation of subsequent immune responses. Moreover, 178, 215, 108, and 76 candidate genes were involved in the C-type lectin receptor signaling pathway, NOD-like receptor signaling pathway, Toll-like receptor signaling pathway, and RIG-I-like receptor signaling pathway, respectively. These pattern recognition receptors enable the host sense bacterial invasion and mediates the innate immune response. Collectively, these results support the hypothesis that vine tea extract pretreatment regulates the response of loach to bacterial infection by modulation of miRNA expression.
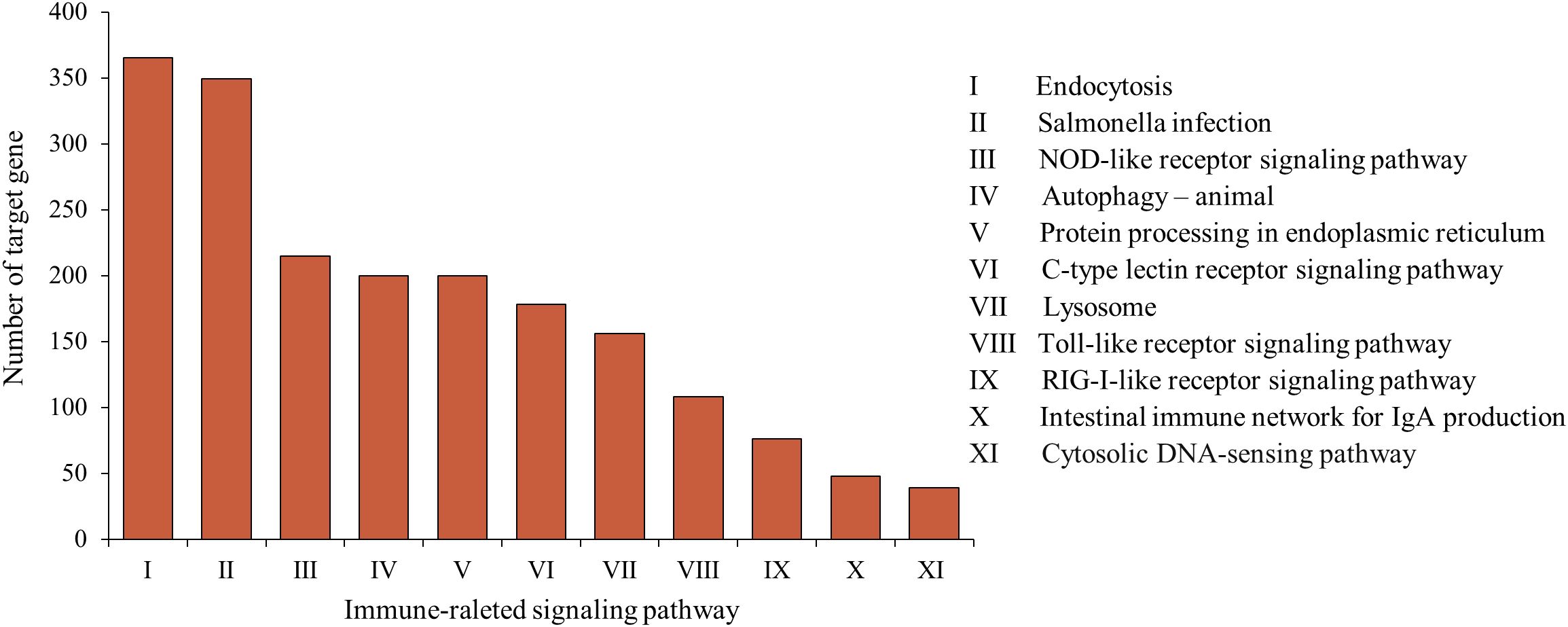
Figure 7. The distribution of genes regulated by these differently expressed miRNAs in immune-related signaling pathway.
4 Discussion
Vine tea, a traditional Chinese medicinal plant used as a tea-like beverage in folklore, is rich in a variety of nutrients such as flavonoids, polysaccharides, amino acids and trace elements, etc. Previous studies have shown that vine tea and its extracts contain significant anti-infective bioactivities, including antiviral, anti-bacterial, anti-inflammatory, and immune-regulating activities (43, 44). To the best of our knowledge, there are no reports the application vine tea extracts in aquaculture. In fish, vine tea extract may serve as potential phytobiotics for the prevention and treatment of bacterial infections, thereby reducing mortality and economic losses. Many studies have shown that miRNAs play a critical roles in the immune response to bacterial infections in fish. Therefore, using loach as an experimental animal, we aimed to identify miRNAs and analyze miRNA expression profiles by high-throughput sequencing to understand how vine tea extract modulates immune responses during bacterial infections. In this study, livers from loach before and after treatment with vine tea extract, followed by infection with A. hydrophila were collected. Two small RNA libraries were constructed and sequenced using high-throughput deep sequencing technology. A total of 1099 conserved miRNAs were identified by removing non-coding RNAs and repetitive sequences. Differentially expressed miRNAs were then subjected to target gene prediction and functional analysis.
The reads distributions of both libraries indicate that the sequences with the highest abundance are 22 nt in length, which is the typical length of animal miRNAs. This is consistent with the miRNAs length distributions observed in other fish species, such as Siniperca chuatsi (45), Miichthys miiuy (46), Ctenopharyngodon Idella (47), cophthalmus maximus (48), all which show a main peak centered at 22nt centered. Sice the whole genome sequence of loach is not yet available in the miRBase database, the high-throughput sequencing results were compared with mature miRNA sequences from other animal species to identify conserved miRNAs. A total of 1099 miRNAs were finally identified, originating from different species. Analysis of miRNA expression profiles revealed a wide range in reads counts, from as low as 0 to as high as 6,712,291, indicating substantial differences in expression levels among different miRNAs. The most highly expressed miRNA showed similarity to miR-122-5p in rabbits. Rabbit miR-122-5p (ocu-miR-122-5p) has been demonstrated to control gene associated with acute liver failure (ALF) (49). Further investigation is required to determine whether this miRNA also plays a biological role in regulating liver inflammatory response in loach. Since no reference genome was available for alignment and no novel miRNA prediction analyses was performed, some data potentially relevant to the purpose of the study may have been overlooked.
Immunity-associated miRNAs have been identified in several fish model and non-model organisms, where they play diverse roles in the immune response, either promoting the immune response or participate in immune escape mechanisms. In this study, a total of 25 significantly differentially expressed miRNAs were identified when comparing the loach groups treated and untreated with vine tea extract. To verify the reliability of high-throughput sequencing results, RT-qPCR were performed on six miRNAs: manu-mir-194-3, manu-undef-1012, manu-undef-834, manu-undef-882, manu-mir-19-2, and manu-mir-130-16. The qPCR results that were consistent with deep-sequencing analysis. Functional enrichment analysis of the target genes regulated by these differentially expressed miRNAs indicated that multiple miRNAs are involved in signaling pathways associated with the innate immune response. Notably, many of these immune pathways are mediated through the targeting of pattern recognition receptors and downstream signaling factors.
Pattern recognition receptors (PRRs) are a class of biomolecules that recognize pathogen-associated molecular patterns (PAMPs) or damage-associated molecular patterns (DAMPs), and there are four classes of CLRs, NLRs, TLRs and RLRs (50). When PRR is activated by PAMP or DAMP, it transduces signals to the intracellular compartment and activates transcription factors localized in the cytoplasm, which enter the nucleus and activate the transcription of relevant genes, thereby releasing cytokines, interferons or other signaling molecules to rapidly mediate the natural immune response (51). In the study of immune regulation by fish miRNAs, there are many reports of PRRs-mediated immune responses. For instance, Xu et al. found that some genes in the TLR signaling pathway are negatively regulated by differentially expressed miRNAs, which facilitates the activation of transcription factors such as AP - 1, IRF5, NF - kB, and IRF3, and thus enhances host immune responses to clear infectious pathogens (46). In addition, Sun et al. reported that, under poly(I:C) stimulation, miiuy croaker miR-210 could participate in the regulation of the RLR signaling pathway by targeting the DUBA, which could protect the host from viral infection (18). In this research, the functions of the target genes of differentially expressed miRNAs were mainly involved in innate immune-related pathways such as the RIG-I signaling pathway and the TLR signaling pathway, which is similar to previous studies. Interestingly, Xiao et al. demonstrated that vine tea extract shows strong antibacterial activity, with its mechanism potentially related to suppression of the tricarboxylic acid cycle pathway (51). Our finding that the tricarboxylic acid cycle pathway is indeed significantly regulated by differentially expressed miRNAs implies that miRNAs also mediate other non-immune pathways that regulate antibacterial effects.
Beyond this, 25 differentially expressed miRNAs in response to vine tea administration, many of which are closely related to immune regulation. While most of these miRNAs remain uncharacterized, some have been previously demonstrated in other animal species, offering valuable insights into their potential biological roles. For examples, miR-194-3p has been described to function as a tumor suppressor through targeting genes involved in migration and cell proliferation, including MMP9. In addition to its anti-tumor role, it also governs immune responses via suppressing the NF-κB pathway and impacts autophagy via targeting SIRT1, thereby influencing viral replication (52). Likewise, miR-19, a member of the well-studied miR-17–92 cluster, plays a dual biological role in boosting cell proliferation and controlling immune responses and apoptosis (53). Extending this functional diversity, miR-31 has been implicated in the modulation of integrin expression, which influence migration and cell adhesion; notably, it can function either as an oncogene or a tumor suppressor depending on the cellular context (54). In the context of host-pathogen interactions, miR-130-3p has been demonstrated to inhibit antibacterial immune responses in teleost fish through targeting NOD1, thereby facilitating bacterial invasion (55). Complementing this, miR-203 also participates to immune regulation in fish via targeting IRAK4, which leads to the attenuation of inflammatory responses against Gram-negative bacterial infections (56). Overall, these results suggest that the differentially expressed miRNAs found in response to vine tea may play multifaceted biological roles in immune modulation, with potential implications for host defense and disease susceptibility.
Although this study offers new insights into the immunomodulatory impacts of vine tea supplementation and found many differentially expressed miRNAs, there are some limitations that should be acknowledged. First, the biological roles of the miRNAs identified in this study were inferred by literature comparisons and bioinformatic predictions; experimental confirmation, including gene expression analysis, miRNA inhibitor/mimic experiments studies, is essential to verify their direct contribution in immune modulation. Second, this study determined the impacts of vine tea supplementation over a short experimental period. The long-term effects of vine tea on health of fish, robustness of immunity, and resistance to diseases remain largely unknown and warrant further studies. Future studies should focus on confirming the crucial miRNA and mRNA interactions, evaluating the dose-dependent influences of vine tea, and performing extended feeding trials to assess the sustainability of its application in aquaculture.
Taken together, in terms of mitigating the threat of bacterial infectious diseases to the loach farming industry, we hope to find a cheap, accessible, effective and environmentally friendly way to do so. We therefore analyzed the expression profiles of bacterial infected loaches with vine tea extract treatment and identified 25 significantly differentially expressed miRNAs. Moreover, the reliability of these miRNA expression profiles was verified by RT - q PCR. Functional annotation of the predicted target genes of differentially expressed miRNAs revealed that the functions of these targets were mainly associated with immune response and antibacterial response. But, to fully elaborate the biological roles of these miRNAs, future studies should focus on experimental confirmation by gene expression analysis, interaction of miRNA-target research, and functional analyses by miRNA inhibitors or mimics. In addition, long-term feeding trials are required to determine the application, optimal dosage, and economic viability of vine tea supplementation in aquaculture operations. It is the first time on the response of miRNA in loach to vine tea extract. Our study deepens our understanding of the function of miRNAs in loach as same as provides a new direction for investigating the application of the antibacterial and immunomodulatory effects of vine tea.
Data availability statement
The datasets presented in this study can be found in online repositories. The names of the repository/repositories and accession number(s) can be found below: https://www.ncbi.nlm.nih.gov/genbank/, SRR29790697.
Ethics statement
Animal experiments were approved by the Research and Animal Ethics Committee of Wenzhou Medical University in accordance with the “Experimental Animal Law”. The study was conducted in accordance with the local legislation and institutional requirements.
Author contributions
WL: Conceptualization, Investigation, Writing – original draft. YQ: Conceptualization, Investigation, Software, Writing – original draft. SP: Formal analysis, Writing – original draft. AW: Methodology, Writing – original draft. XW: Conceptualization, Software, Writing – original draft. DL: Formal analysis, Methodology, Software, Writing – original draft. LD: Funding acquisition, Project administration, Resources, Software, Writing – original draft, Writing – review & editing.
Funding
The author(s) declare that financial support was received for the research and/or publication of this article. This research was funded by the Postgraduate Research Innovation Program of Jiangsu Province (KYCX24_4147). We appreciate the funding for the present study by Wenzhou Key Laboratory of Research and Transformation of Chinese Medicine.
Conflict of interest
The authors declare that the research was conducted in the absence of any commercial or financial relationships that could be construed as a potential conflict of interest.
Generative AI statement
The author(s) declare that no Generative AI was used in the creation of this manuscript.
Publisher’s note
All claims expressed in this article are solely those of the authors and do not necessarily represent those of their affiliated organizations, or those of the publisher, the editors and the reviewers. Any product that may be evaluated in this article, or claim that may be made by its manufacturer, is not guaranteed or endorsed by the publisher.
Supplementary material
The Supplementary Material for this article can be found online at: https://www.frontiersin.org/articles/10.3389/fimmu.2025.1584985/full#supplementary-material
References
2. Shivdasani RA. MicroRNAs: regulators of gene expression and cell differentiation. Blood. (2006) 108:3646–53. doi: 10.1182/blood-2006-01-030015
3. Fabian MR, Sonenberg N, and Filipowicz W. Regulation of mRNA Translation and Stability by microRNAs. Annu Rev Biochem. (2010) 79:351–79. doi: 10.1146/annurev-biochem-060308-103103
4. Lee RC, Feinbaum RL, Ambros V, and The C. elegans heterochronic gene lin-4 encodes small RNAs with antisense complementarity to lin-14. Cell. (1993) 75:843–54. doi: 10.1016/0092-8674(93)90529-Y
5. Kloosterman WP and Plasterk RHA. The diverse functions of microRNAs in animal development and disease. Dev Cell. (2006) 11:441–50. doi: 10.1016/j.devcel.2006.09.009
6. Stern-Ginossar N, Elefant N, Zimmermann A, Wolf DG, Saleh N, Biton M, et al. Host immune system gene targeting by a viral miRNA. Science. (2007) 317:376–81. doi: 10.1126/science.1140956
7. Wu M-Y and Lu J-H. Autophagy and macrophage functions: inflammatory response and phagocytosis. Cells. (2019) 9:70. doi: 10.3390/cells9010070
8. Yuhong J, Leilei T, Fuyun Z, Hongyang J, Xiaowen L, Liying Y, et al. Identification and characterization of immune-related microRNAs in blunt snout bream, Megalobrama amblycephala. Fish Shellfish Immunol. (2016) 49:470–92. doi: 10.1016/j.fsi.2015.12.013
9. Tang X, Fu J, Yao Y, and Xu M. Identification and characterization of immune-related microRNAs in hybrid snakehead (Channa maculata♀ × Channa argus♂) after treated by EChinacea purpurea (Linn. ) Moench Fish Shellfish Immunol. (2023) 135:108653. doi: 10.1016/j.fsi.2023.108653
10. Qi P, Guo B, Zhu A, Wu C, and Liu C. Identification and comparative analysis of the Pseudosciaena crocea microRNA transcriptome response to poly(I:C) infection using a deep sequencing approach. Fish Shellfish Immunol. (2014) 39:483–91. doi: 10.1016/j.fsi.2014.06.009
11. Qiang J, Bao JW, Li HX, Chen DJ, He J, Tao YF, et al. miR-1338-5p Modulates Growth Hormone Secretion and Glucose Utilization by Regulating ghitm in Genetically Improved Farmed Tilapia (GIFT, Oreochromis niloticus). Front Physiol. (2017) 8:998. doi: 10.3389/fphys.2017.00998
12. Qiang J, Tao YF, Bao JW, Chen DJ, Li HX, He J, et al. High Fat Diet-Induced miR-122 Regulates Lipid Metabolism and Fat Deposition in Genetically Improved Farmed Tilapia (GIFT, Oreochromis niloticus) Liver. Front Physiol. (2018) 9:1422. doi: 10.3389/fphys.2018.01422
13. Zhao Z, Yu X, Jia J, Yang G, Sun C, and Li W. miR-181b-5p may regulate muscle growth in tilapia by targeting myostatin b. Front Endocrinol. (2019) 10:812. doi: 10.3389/fendo.2019.00812
14. Ni S, Yu Y, Wei J, Zhou L, Wei S, Yan Y, et al. MicroRNA-146a promotes red spotted grouper nervous necrosis virus (RGNNV) replication by targeting TRAF6 in orange spotted grouper, Epinephelus coioides. Fish Shellfish Immunol. (2018) 72:9–13. doi: 10.1016/j.fsi.2017.10.020
15. Xu T, Chu Q, Cui J, and Bi D. Inducible microRNA-3570 feedback inhibits the RIG-I-dependent innate immune response to rhabdovirus in teleost fish by targeting MAVS/IPS-1. J Virol. (2018) 92:e01594–17. doi: 10.1128/JVI.01594-17
16. Chu Q and Xu T. miR-192 targeting IL-1RI regulates the immune response in miiuy croaker after pathogen infection in vitro and in vivo. Fish Shellfish Immunol. (2016) 54:537–43. doi: 10.1016/j.fsi.2016.05.007
17. Wang Y, Xu G, Han J, and Xu T. miR-200a-3p regulates TLR1 expression in bacterial challenged miiuy croaker. Dev Comp Immunol. (2016) 63:181–6. doi: 10.1016/j.dci.2016.06.004
18. Sun Y, Han J, Chu Q, Liu X, and Xu T. microRNA-210 participates in regulating RIG-I signaling pathway via targeting DUBA in miiuy croaker after poly(I:C) stimulation. Fish Shellfish Immunol. (2018) 77:1–7. doi: 10.1016/j.fsi.2018.02.003
19. Zhao Z, He R, Chu P, Cai H, Shen H, Zhao Z, et al. YBX has functional roles in CpG-ODN against cold stress and bacterial infection of Misgurnus anguillicaudatus. Fish Shellfish Immunol. (2021) 118:72–84. doi: 10.1016/j.fsi.2021.08.018
20. Xu Y, Yu Y, Zhang X, Huang Z, Li H, Dong S, et al. Molecular characterization and expression analysis of complement component 3 in dojo loach (Misgurnus anguillicaudatus). Fish Shellfish Immunol. (2018) 72:484–93. doi: 10.1016/j.fsi.2017.11.022
21. Wang J, Fu G, Li W, Shi Y, Pang J, Wang Q, et al. The effects of two free-floating plants (Eichhornia crassipes and Pistia stratiotes) on the burrow morphology and water quality characteristics of pond loach (Misgurnus anguillicaudatus) habitat. Aquaculture Fisheries. (2018) 3:22–9. doi: 10.1016/j.aaf.2017.12.001
22. Saraceni PR, Romero A, Figueras A, and Novoa B. Establishment of Infection Models in Zebrafish Larvae (Danio rerio) to Study the Pathogenesis of Aeromonas hydrophila. Front Microbiol. (2016) 7:1219. doi: 10.3389/fmicb.2016.01219
23. Abdul Kari Z, Wee W, Mohamad Sukri SA, Che Harun H, Hanif Reduan MF, Irwan Khoo M, et al. Role of phytobiotics in relieving the impacts of Aeromonas hydrophila infection on aquatic animals: A mini-review. Front Vet Sci. (2022) 9doi: 10.3389/fvets.2022
24. Li J, Fan Q, Cai H, Deng J, Ming F, Li J, et al. Identification of RBP4 from bighead carp (Hypophthalmichthys nobilis)/silver carp (Hypophthalmichthys molitrix) and effects of CpG ODN on RBP4 expression under A. hydrophila challenge. Fish Shellfish Immunol. (2020) 100:476–88. doi: 10.1016/j.fsi.2020
25. Ofek T, Izhaki I, and Halpern M. Aeromonas hydrophila infection in tilapia triggers changes in the microbiota composition of fish internal organs. FEMS Microbiol Ecol. (2023) 99:fiad137. doi: 10.1093/femsec/fiad137
26. Lee SW, Sim KY, Wendy W, and Zulhisyam AK. Peperomia pellucida leaf extract as immunostimulator in controlling motile aeromonad septicemia due to Aeromonas hydrophila in red hybrid tilapia, Oreochromis spp. farming. Vet World. (2016) 9:231–4. doi: 10.14202/vetworld.2016.231-234
27. Abdul Kari Z, Wee W, Mohamad Sukri SA, Che Harun H, Hanif Reduan MF, Irwan Khoo M, et al. Role of phytobiotics in relieving the impacts of Aeromonas hydrophila infection on aquatic animals: A mini-review. Front Vet Sci. (2022) 9 doi: 10.3389/fvets.2022
28. Wang B, Thompson KD, Wangkahart E, Yamkasem J, Bondad-Reantaso MG, Tattiyapong P, et al. Strategies to enhance tilapia immunity to improve their health in aquaculture. Rev Aquac. (2023) 15:41–56. doi: 10.1111/raq.12731
29. Abd El-latif AM, El-Gawad EAA, Soror EI, Shourbela RM, and Zahran E. Dietary supplementation with miswak (Salvadora persica) improves the health status of Nile tilapia and protects against Aeromonas hydrophila infection. Aquaculture Rep. (2021) 19:100594. doi: 10.1016/j.aqrep.2021
30. Luo Q-J, Zhou W-C, Liu X-Y, Li Y-J, Xie Q-L, Wang B, et al. Chemical Constituents and α-Glucosidase Inhibitory, Antioxidant and Hepatoprotective Activities of Ampelopsis grossedentata. Molecules (2023) 28(24):7956. doi: 10.3390/molecules28247956
31. Li Y, Kumar PS, Tan S, Huang C, Xiang Z, Qiu J, et al. Anticancer and antibacterial flavonoids from the callus of Ampelopsis grossedentata; a new weapon to mitigate the proliferation of cancer cells and bacteria. RSC Adv. (2022) 12:24130–8. doi: 10.1039/D2RA03437A
32. Yin H, Yan Q, Y, and Tang H. Dihydromyricetin nanoparticles alleviate lipopolysaccharide-induced acute kidney injury by decreasing inammation and cell apoptosis via the TLR4/NF-κB pathway. J Funct Biomater. (2024) 15(9):249. doi: 10.3390/jfb15090249
33. Carneiro RCV, Ye L, Baek N, Teixeira GHA, and O’Keefe SF. Vine tea (Ampelopsis grossedentata): A review of chemical composition, functional properties, and potential food applications. J Funct Foods. (2021) 76:104317. doi: 10.1016/j.jff.2020.104317
34. Wang Z, Jiang Q, Li P, Shi P, Liu C, Wang W, et al. The water extract of ampelopsis grossedentata alleviates oxidative stress and intestinal inflammation. Antioxidants (Basel). (2023) 12(3):547. doi: 10.3390/antiox12030547
35. Xie K, He X, Chen K, Chen J, Sakao K, and Hou D-X. Antioxidant Properties of a Traditional Vine Tea, Ampelopsis grossedentata. Antioxidants (Basel). (2019) 8(8):295. doi: 10.3390/antiox8080295
36. Liu D, Mao Y, Ding L, and Zeng X. Dihydromyricetin: A review on identification and quantification methods, biological activities, chemical stability, metabolism and approaches to enhance its bioavailability. Trends Food Sci Technol. (2019) 91:586–97. doi: 10.1016/j.tifs.2019.07.038
37. He C, Chen Y, Xie J, Luo M, Fisher D, Hien NTT, et al. Dihydromyricetin: an emerging compound with comprehensive effects on multiple systems. Front Pharmacol. (2025) 15:1488003. doi: 10.3389/fphar.2024.1488003
38. Huang T, Wang X, Yang Q, Peng S, and Peng M. Effects of dietary supplementation with Ampelopsis grossedentata extract on production performance and body health of hens. Trop Anim Health Prod. (2022) 54:45. doi: 10.1007/s11250-022-03044-7
39. Liu X, Zhang F, Li M, Li R, Zhang Z, Xu J, et al. Supplementation of Ampelopsis grossedentata extract contributes to the improvement of intestinal health in swine. Front Vet Sci. (2024) 11:1417309. doi: 10.3389/fvets.2024.1417309
40. Yang Q, Wang Y, Li G, Huang X, Zheng L, Peng M, et al. Effect of dietary supplementation Ampelopsis grossedentata extract on growth performance and muscle nutrition of Megalobrama hoffmanni by gut bacterial mediation. Heliyon. (2024) 10:e29008. doi: 10.1016/j.heliyon.2024
41. Zhou W, Xie Y, Li Y, Xie M, Zhang Z, Yang Y, et al. Research progress on the regulation of nutrition and immunity by microRNAs in fish. Fish Shellfish Immunol. (2021) 113:1–8. doi: 10.1016/j.fsi.2021.03.011
42. Wei B, Cai T, Zhang R, Li A, Huo N, Li S, et al. Novel microRNAs uncovered by deep sequencing of small RNA transcriptomes in bread wheat (Triticum aestivum L.) and Brachypodium distachyon (L.) Beauv. Funct Integr Genomics. (2009) 9:499–511. doi: 10.1007/s10142-009-0128-9
43. Chervet A, Neme R, Decombat C, Longechamp L, Habanjar O, Rousset A, et al. Exploring the therapeutic potential of Ampopsis grossedentata leaf extract as an anti-inflammatory and antioxidant agent in human immune cells. Int J Mol Sci. (2023) 25(1):416. doi: 10.3390/ijms25010416
44. Yang BH, Li B, and Ma JL. Research progress of medicinal plant Ampelopsis grossedentata. J Dalian Univ Nationalities. (2023) 25:199–203. doi: 10.13744/j.cnki.cn21-1431/g4.2023.03.001
45. Zhou Y, Wang Y-Y, Fu H-C, and Huang H-Z. MicroRNA expression and analysis of immune-related putative target genes in ISKNV-infected spleen of mandarin fish (Siniperca chuatsi). Aquaculture. (2022) 547:737450. doi: 10.1016/j
46. Xu G, Han J, and Xu T. Comparative analysis of the small RNA transcriptomes of miiuy croaker revealed microRNA-mediated regulation of TLR signaling pathway response to Vibrio Anguillarum infection. Fish Shellfish Immunol. (2016) 52:248–57. doi: 10.1016/j.fsi.2016.03.011
47. Xu X, Shen Y, Fu J, Lu L, and Li J. Next-generation sequencing identified microRNAs that associate with motile aeromonad septicemia in grass carp. Fish Shellfish Immunol. (2015) 45:94–103. doi: 10.1016/j.fsi.2015.02.008
48. Gao C, Cai X, Fu Q, Yang N, Song L, Su B, et al. Dynamics of MiRNA Transcriptome in Turbot (Scophthalmus maximus L.) Intestine Following Vibrio Anguillarum Infection. Mar Biotechnol. (2019) 21:550–64. doi: 10.1007/s10126-019-09903-z
49. Hukowska-Szematowicz B, Maciejak-Jastrzębska A, Blatkiewicz M, Maciak K, Góra M, Janiszewska J, et al. Changes in microRNA expression during rabbit hemorrhagic disease virus (RHDV) infection. Viruses. (2020) 12:965. doi: 10.3390/v12090965
50. Li D and Wu M. Pattern recognition receptors in health and diseases. Sig Transduct Target Ther. (2021) 6:291. doi: 10.1038/s41392-021-00687-0
51. Xiao X-N, Wang F, Yuan Y-T, Liu J, Liu Y-Z, and Yi X. Antibacterial Activity and Mode of Action of Dihydromyricetin from Ampelopsis grossedentata Leaves against Food-Borne Bacteria. Molecules. (2019) 24:2831. doi: 10.3390/molecules24152831
52. Huang H, Liao D, Zhou G, He B, Pu R, and Cui Y.A. MicroRNA-194-3p impacts autophagy and represses rotavirus replication via targeting silent information regulator 1. Virol J. (2023) 20:210. doi: 10.1186/s12985-023-02175-z
53. Li X, Teng C, Ma J, Fu N, Wang L, Wen J, et al. miR-19 family: A promising biomarker and therapeutic target in heart, vessels and neuron. s. Life Sci. (2019) 232:116651. doi: 10.1016/j.lfs.2019.116651
54. Augoff K, Das M, Bialkowska K, McCue B, Plow EF, and Sossey AK. miR-31 is a broad regulator of b1-integrin expression and function in cancer cells. Mol Cancer Res. (2011) 9(11):1500–8. doi: 10.1158/1541-7786.MCR-11-0311
55. Zheng W, Su H, Lv X, Xin S, and Xu T. Exon-Intron Circular RNA circRNF217 Promotes Innate Immunity and Antibacterial Activity in Teleost Fish by Reducing miR-130-3p Function. J Immunol. (2022) 208(5):1099–1114. doi: 10.4049/jimmunol.2100890
Keywords: microRNA, Ampelopsis grossedentata, loach, pathogen, innate immune
Citation: Lai W, Qiu Y, Pan S, Wan A, Wang X, Lv D and Dai L (2025) Alleviating effect of vine tea on Aeromonas hydrophila infection revealed by small RNA transcriptome analyses of loach liver. Front. Immunol. 16:1584985. doi: 10.3389/fimmu.2025.1584985
Received: 28 February 2025; Accepted: 30 April 2025;
Published: 21 May 2025.
Edited by:
Saima Kausar, University of Agriculture, Faisalabad, PakistanReviewed by:
Qiu-Ning Liu, Yancheng Teachers University, ChinaGuan-Jun Yang, Ningbo University, China
Congfen Zhang, Chengdu University, China
Copyright © 2025 Lai, Qiu, Pan, Wan, Wang, Lv and Dai. This is an open-access article distributed under the terms of the Creative Commons Attribution License (CC BY). The use, distribution or reproduction in other forums is permitted, provided the original author(s) and the copyright owner(s) are credited and that the original publication in this journal is cited, in accordance with accepted academic practice. No use, distribution or reproduction is permitted which does not comply with these terms.
*Correspondence: Xueyang Wang, eHVleWFuZ3dhbmdAanVzdC5lZHUuY24=; Dingding Lv, bGRkNDIyQDE2My5jb20=; Lishang Dai, bGlzaGFuZzIwMTZAd211LmVkdS5jbg==
†These authors have contributed equally to this work