- 1College of Food Science and Technology, Shanghai Ocean University, Shanghai, China
- 2Shanghai Engineering Research Center of Aquatic-Product Processing and Preservation, Shanghai, China
- 3Laboratory of Quality and Safety Risk Assessment for Aquatic Product on Storage and Preservation (Shanghai), Ministry of Agriculture Shanghai, Shanghai, China
Acidic electrolyzed water (AEW), a novel non-thermal sterilization technology, is widely used in the food industry. In this study, we firstly investigated the effect of AEW as a new pressure transmitting medium for high hydrostatic pressure (AEW-HHP) processing on microorganisms inactivation on shelled fresh shrimp. The optimal conditions of AEW-HHP for Vibrio parahaemolyticus inactivation on sterile shelled fresh shrimp were obtained using response surface methodology: NaCl concentration to electrolysis 1.5 g/L, treatment pressure 400 MPa, treatment time 10 min. Under the optimal conditions mentioned above, AEW dramatically enhanced the efficiency of HHP for inactivating V. parahaemolyticus and Listeria monocytogenes on artificially contaminated shelled fresh shrimp, and the log reductions were up to 6.08 and 5.71 log10 CFU/g respectively, while the common HHP could only inactivate the two pathogens up to 4.74 and 4.31 log10 CFU/g respectively. Meanwhile, scanning electron microscopy (SEM) showed the same phenomenon. For the naturally contaminated shelled fresh shrimp, AEW-HHP could also significantly reduce the micro flora when examined using plate count and PCR-DGGE. There were also no significant changes, histologically, in the muscle tissues of shrimps undergoing the AEW-HHP treatment. In summary, using AEW as a new transmitting medium for HHP processing is an innovative non thermal technology for improving the food safety of shrimp and other aquatic products.
Introduction
Shrimp is one of the most popular and important seafood worldwide because of its delicacy and abundant nutrients (Norhana et al., 2010). In China, consumption of shrimp, especially shelled fresh shrimp, has increased annually (Wang et al., 2013). However, shrimp products are usually contaminated with numerous spoilage organisms and pathogens (McCarthy, 1997; Liu et al., 2006; Xie et al., 2012). These bacteria were regarded as the crucial influencing factors for the quality and safety of shrimp, and could easily increase the possibilities of spoilage and infection risk (Nirmal and Benjakul, 2010). Therefore, development of an efficient and international accepted intervention method for eliminating these microorganisms in shrimp products would be of great value to the seafood industry and for improving public health (Lenz et al., 2015; Sevenich et al., 2015).
A variety of intervention methods have been established for controlling spoilage or pathogenic bacteria in food, such as high hydrostatic pressure, electrolyzed oxidizing water, ultrasound, irradiation, some chemicals or essential oils (McCarthy, 1997; Andrews et al., 2000; Mahmoud, 2009; Chen et al., 2011; Ma and Su, 2011; Prakash et al., 2012). High hydrostatic pressure (HHP), a non-thermal food sterilization and preservation technology, could reduce microorganisms without damaging the sensory quality of the products (Rendueles et al., 2011; Belletti et al., 2013a; Lorido et al., 2015). In a typical HHP procedure, the food products are sealed and placed into a steel chamber containing a pressure transmitting medium, and pumps are used to create pressure. The effect of HHP for food sterilization and preservation could be influenced by the treatment pressure, treatment time and pressure transmitting medium (Georget et al., 2015). Traditionally, common water is often used as the pressure transmitting medium for HHP (Rubio et al., 2013; Omer et al., 2015; Ma and Mu, 2016), however some researches also suggested that change of pressure transmitting fluids might improve the inactivation effect of HHP (Balasubramanian and Balasubramaniam, 2003; Georget et al., 2015).
In recent years, acidic electrolyzed water (AEW) has been regarded as an effective sanitizer and widely used in the food industries of many countries (Smigic et al., 2009; Wang et al., 2014). By electrolysis in the specific device, a diluted sodium chloride solution dissociates into AEW, which has a low pH of 2–3, a high oxidation-reduction potential (ORP) of > 1100 mV, and an active chlorine content (ACC) of 10–90 ppm (Hricova et al., 2008). In contrast with conventional sanitizer, AEW not only has better bactericidal effect, but also less adverse impact on public health and the environment (Katayose et al., 2007; Huang et al., 2008; Xie et al., 2012). Previous research has proven that AEW in combination with other intervention methods could significantly enhance their inactivation efficiency (Liu R. et al., 2013). However, no studies have reported that AEW could be used as a new transmitting medium in HHP. Also, the impact of AEW on the inactivation efficiency of HHP is unknown.
This study firstly developed a novel sterilization and preservation technology by using the HHP combined with AEW as a new transmitting medium (AEW-HHP). A response surface (RS) model was applied to quantify the influence of AEW on high hydrostatic pressure processing for reducing pathogenic bacteria on shelled fresh shrimp. And the preservation effect of this new technology was evaluated by the reduction of indigenous microflora and the change of muscle fiber of shelled fresh shrimp. This novel sterilization and preservation technology could provide a powerful tool for eliminating pathogenic microorganisms and improving the seafood quality and safety.
Materials and Methods
Bacterial Strain and Culture Preparation
A four-strain cocktail of V. parahaemolyticus strains (ATCC 17802; ATCC 33847; F 18, Litopenaeus vannamei isolate; F 36, serotype O3: K6) and a two-strain cocktail of L. monocytogenes (ATCC 19115; ATCC 19116) were used in the study. Each strain for V. parahaemolyticus (stored in 25% glycerol at -80°C) was separately cultured in tryptic soy broth (TSB, Beijing Land Bridge Technology Company Ltd., Beijing, China) plus 3% NaCl and incubated at 37°C for 18 h. Other strains of L. monocytogenes were grown in TSB at 37°C for 18–20 h. Enriched cultures were pooled into a sterile centrifuge tube and centrifuged at 3000 g, 25°C for 10 min (Centrifuge 5417R, eppendorf, Germany). Pelleted cells were re-suspended in sterile peptone water (PW; 0.85% NaCl, 0.1% peptone) to obtain a multi-strain cocktail of ~ 9 log10 CFU/mL of V. parahaemolyticus and L. monocytogenes, respectively.
Preparation and Inoculation of Shrimp Samples
Fresh shrimps (Litopenaeus vannamei) were purchased alive from a local market in Shanghai, PR China before each experiment and transported to the laboratory in plastic bags containing pond water sparged with oxygen to keep the shrimps viable. At the laboratory the shrimps were rinsed with running tap water, a protocol described in GB/T 4789.7-2008 (Code of National Standard of China, 2008). The shrimps were beheaded, peeled, and sterilized under UV light for 30 min. Shelled fresh shrimp samples (7 ± 1 g per shrimp) were soaked into 200 mL of suspensions containing four V. parahaemolyticus strains (or two L. monocytogenes strains) for 30 min, and then transferred to plastic plates for 30 min to allow for bacterial attachment. The above protocol was carried out at room temperature (23 ± 2°C). The inoculated shrimps were used for the subsequent treatments.
Preparation of Acidic Electrolyzed Water (AEW)
AEW with different physicochemical characteristics was prepared by electrolyzing different concentrations of sodium chloride (NaCl concentration to electrolysis; Table 1) using an AEW generator (FW-200, AMANO, Japan). The generator was allowed to run for 15 min with the amperage setting as 10 A before collecting water. The pH and ORP were determined using a pH/ORP meter (model pH 430, Corning Inc., NY). The ACC in AEW was determined by a colorimetric method using a digital chlorine test kit (RC-2Z, Kasahara Chemical Instruments Corp., Saitama, Japan). All measurements were carried out in triplicate. The AEW had a range of pH 2.28–2.39, ORP 1077–1168 mV, and ACC 36–73 mg/L.
High Hydrostatic Pressure (HHP) Treatment
A high pressure food processor (HPP.L2-600/2, Tianjin Tyson Miaotuo Biological Engineering Technology Company Ltd., Tianjin, China) was used in the present study. The come-up rate was approximately 240 MPa/min and the pressure release time was almost immediate. Samples were packaged in sterile plastic bags and treated under the desired levels 200, 300, or 400 MPa, for treatment time 5, 10, or 15 min. Common water or AEW was used as the transmitting medium for HHP.
Bacterial Enumeration
The bacterial enumeration method used in this study was the plating counting method according to previous studies (Belletti et al., 2013a; Wang et al., 2014). Briefly, shrimp samples were homogenized for 2 min in a stomacher (BagMixer400VW, Interscience, France). Then, a direct-plating procedure was used for the enumeration of V. parahaemolyticus, L. monocytogenes and indigenous microflora on shrimp samples. TCBS ager for V. parahaemolyticus, PALCAM agar (Beijing Land Bridge Technology Company Ltd., Beijing, China) for L. monocytogenes and Trypticase Soy Agar (TSA; Beijing Land Bridge Technology Company Ltd., Beijing, China) for indigenous microflora was used, respectively. The detection limit of the direct-plating was approximately 2 log10 CFU/g. Colonies were counted after the plates were incubated at 37°C for 24 h. Three replicates at each sampling time were done.
Response Surface Model Development and Validation
RS methodology based on the Box-Behnken experimental design (BBD) was used to describe the relationship and interactions between three technological variables (Table 1) on the inactivation of V. parahaemolyticus. Design Expert package (Version 8.0.6, Stat-Ease Inc., Minneapolis, USA) was used to design the treatment conditions (Table 2), for regression analysis and to generate the second order polynomial model. The shrimp samples were treated under different conditions (Table 2), and the response value was expressed as the Log reductions after the treatments.
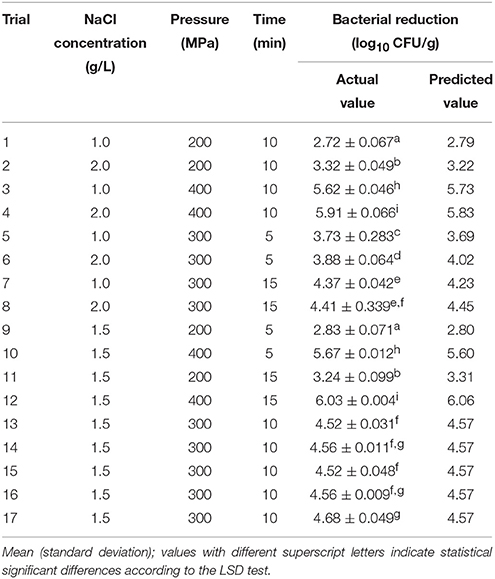
Table 2. Actual and predicted reduction of V. parahaemolyticus on shrimps by response surface model according to the Box-Behnken experimental design arrangement.
Analysis of the Variance (ANOVA) and the corresponding post-hoc contrasts were performed to compare the results of the different experimental conditions. The statistical significance and goodness of fit of the model were evaluated using the determination coefficients (R2), the P-values from the Fisher F-test and the Lack of Fit test. The accuracy of the RS model describing the inactivation of V. parahaemolyticus was evaluated by the following criteria: the accuracy factor (Af, Equation 1), bias factor (Bf, Equation 2) and the root-mean-squares error (RMSE, Equation 3; Zurera-Cosano et al., 2006; Dong et al., 2007):
where obs was observed values, pred was predicted values by RS model, and the n stands for the number of observations. Ideally, predictive models would have Af = Bf = 1. The response surface graphs and contour plots were used to describe the individual effects and interactions of the variables.
To validate the model, eight additional trials with random combinations of four variables were conducted (Table 3). The method of bacterial inactivation on shrimps was the same as explained previously for the inactivation treatments. The selected parameters were within the original range of the experimental design but not included in the establishment of the model. The observed results were used to evaluate performance of the model by the Af (Equation 1) and the Bf (Equation 2) as proposed by Ross (1996).
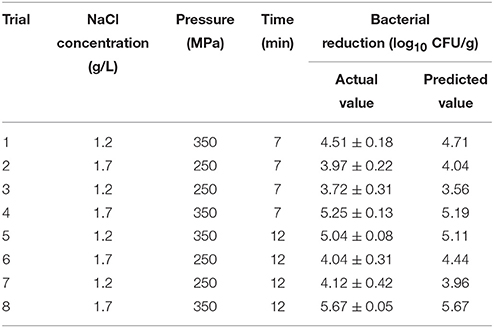
Table 3. Actual and predicted reduction values for inactivation of V. parahaemolyticus on shelled fresh shrimp under the additional random eight conditions.
Application of AEW-HHP Treatment on Artificially Contaminated Shelled Fresh Shrimp
The optimal conditions of AEW-HHP for inactivation of bacteria on artificially contaminated shrimp were obtained by RS methodology. Based on the optimal conditions, artificially contaminated shelled fresh shrimp samples with V. parahaemolyticus or L. monocytogenes were treated by AEW-HHP. The treatment conditions were set as NaCl concentration to electrolysis 1.5 g/L, treatment time 10 min and treatment pressure 200, 300, and 400 MPa. Bacterial reduction was determined by plating as described above.
Observation by Scanning Electron Microscopy (SEM)
After AEW-HHP treatment, bacteria on artificially contaminated shelled fresh shrimp were observed by SEM. The preparation and observation of samples were according to the conventional method (Shen and Zhiguo, 2008). In brief, the treated artificially contaminated shrimp samples were fixed with 2.5% glutaraldehyde, then dehydrated in graded ethanol series, coated with gold-palladium (Ion Sputter and Carbon Coating Unit E-1045, Hitachi Company, Japan) and observed under a scanning electron microscope (NOVA NanoSEM230, FEI Company, USA).
Application of AEW-HHP Treatment on Naturally Contaminated Shelled Fresh Shrimp
To further verify the inactivation efficacy, the AEW-HHP treatment was applied to eliminate the indigenous microflora on naturally shelled fresh shrimp. The treatment conditions were set as NaCl concentration to electrolysis 1.5 g/L, treatment time 10 min and treatment pressure 200, 300, and 400 MPa. The bacterial enumeration of indigenous microflora was carried out using TSA. The diversity of bacteria in shrimp was analyzed by PCR-DGGE, and the quality of shrimp was evaluated by histological analysis.
Bacterial Diversity Analysis Using PCR-DGGE
PCR-DGGE analysis was used to assess the changes in the diversity of bacteria on naturally contaminated shrimps. DNA was extracted according to the method described by Ampe et al. (1999). Briefly, all samples were homogenized for 2 min in the stomacher. The resulting suspension (1 mL) was centrifuged (12,000 g, 2 min), and then the supernatant was discarded. The obtained pellet was used to extract DNA using DNA extraction kit (TIANamp bacteria DNA kit, Tiangen Biotech CoLtd., Beijing, PR China). One microliter of the DNA sample was used as template for the PCR. The primers V3-2 (5′-ATTACCGCGGCTGCTGG-3′) and V3-3 incorporated a 40-bp GC clamp (5′-CGCCCGCCGCGCGCGGCGGGCG GGGCGGGGGCACGGGGGGCCTACGGGAGGCAGCAG-3′) which was used to amplify the hypervariable V3 region of the 16S rDNA (Muyzer et al., 1993). The V3 region is a good choice in terms of length and species-species heterogeneity (Wang et al., 2015). The PCR mix was composed of 25 μL of Premix Ex Taq (Takara, Otsu, Japan), 19 μL of ddH2O, 1 μL of each primer, and 2 μL of DNA template. The reactions were performed on a thermal cycler (Mastercycler pro S; Eppendorf AG, Germany). The PCR program was performed according to Muyzer et al. (1993) with slight modifications as follows: initial denaturation at 95°C for 3 min; 25 cycles of denaturation at 95°C for 1 min, annealing at 55°C for 1 min and extension at 72°C for 30 s; final extension at 72°C for 5 min. The amplified products were separated with 1% (m/v) agarose gel electrophoresis and visualized under UV light.
DGGE was performed with BioRad DCode™ Universal Mutation Detection System (BioRad Laboratories, USA) using 20 μL of the purified and concentrated PCR product. The PCR products were applied to 8% (m/v) polyacrylamide gels in 1 × TAE buffer which contained a 40–55% urea-formamide denaturing gradient. Electrophoresis was conducted at 100 V for 10 h under a constant temperature (60°C). The DGGE gels were stained with SYBR green I and visualized under UV light (Lin et al., 2013). All determinations were carried out in duplicate.
Scanned images of the DGGE gels were analyzed with Image Lab (Bio-Rad, USA). Shannon index was calculated by DGGE banding pattern analysis. Shannon index of bacterial diversity, H′, was obtained by Equation (4). Pi was calculated as Equation (5).
where Pi is the importance probability of the bands in a gel lane, ni is the height of a peak and N is the sum of all the peak heights of the bands in the densitometric profile (Ogino et al., 2001).
Histological Analysis
The histological analysis was performed according to Liu D. et al. (2013) with some modifications. The second uromere of shrimp samples was cut, and fixed in 4% formalin for 24 h. The tissue was dehydrated with a graded series of ethanol (70, 80, 90, 95, and 100%), and then transferred to xylene and embedded in paraffin for 1–2 h. The tissue sections of 7 μm were obtained using a RM2235 microtome (Leica Microsystems CMS GmbH, Wetzlar, Germany). The sections were transferred onto glass slides after reshaped in water, dried, deparaffinized, rehydrated, stained (hematoxylin-eosin staining), and then photo-graphed. All determinations were carried out in triplicate.
An image analysis of each picture was made according to the methods of Díaz-Tenorio et al. (2007). Blank area represents the area of gaps between muscle fibers. The percentage of blank areas in each picture was calculated. Results were expressed as percentages of total analyzed area.
Statistical Analysis
All values were expresses as the mean ± standard deviation (SD). Statistical analysis was performed by using SPSS statistical package 17.0 (SPSS Inc., Chicago, USA). One way analysis of variance was conducted to compare the effects under different conditions. The least significant difference (LSD) test was used to determine differences at α = 0.05.
Results
Optimal Conditions of AEW-HHP for V. parahaemolyticus Inactivation
The initial load on shelled fresh shrimp was approximately 7.1 log10 CFU/g. Seventeen trials under different combined conditions (NaCl concentration to electrolysis, treatment pressure and treatment time) were designed by BBD. Table 2 shows the results of Log reductions of V. parahaemolyticus on shelled fresh shrimp under different conditions. The actual values of Log reductions ranged from 2.72 to 6.03 log10 CFU/g.
A backward stepwise regression was carried out using Design Expert package to develop a RS model based on Table 2, and the established model was as follows:
where R was response value (log10 CFU/g); X1, X2, and X3 represent variables, including NaCl concentration to electrolysis, treatment pressure and treatment time, respectively. The results of the ANOVA for quadratic model indicated the goodness of fit of the regression equation: the R2 and Adj. R2 were 0.994 and 0.986, respectively. The value of the Adj.R2 indicated a high degree of correlation between the observed and predicted values, which suggested that only 1.6% of the total variation cannot be explained by the current model (Wang et al., 2014). RMSE also provided a measure of the goodness-of-fit of the model to the data used to produce it (Wang et al., 2014) and its value was 0.29 indicating that the RS model fitted well with the observed data. The statistical significance and adequacy of the model were determined using the Fisher F-test and Lack of Fit test (Table 4). F-value of 129.49 and probability value (p < 0.0001) indicated that the differences between different treatments were highly significant. The model is adequate since the Lack of Fit test (p > 0.05) was not significant (Wang et al., 2014).
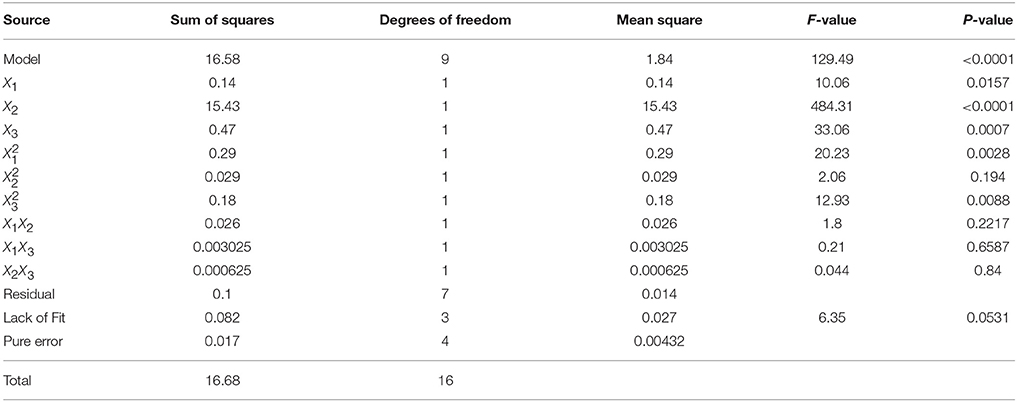
Table 4. Analysis of variance (ANOVA) for response surface model of V. parahaemolyticus inactivation on shelled fresh shrimp.
The response surface plot in Figure 1A describes the inactivation effect of NaCl concentration and treatment pressure at the treatment time of 10 min. The population of V. parahaemolyticus on shelled fresh shrimp significantly reduced (p < 0.0001) as the concentration of NaCl and pressure increased. The change of NaCl concentration played a similar role with treatment time in V. parahaemolyticus inactivation, and the bacteria were less sensitive to both NaCl concentration and time than pressure (Figures 1B,C). The estimated optimal treatment conditions were 1.5 g/L NaCl concentration to electrolysis at 400 MPa for 10 min, leading to a maximum bacterial reduction of 6.08 log10 CFU/g.
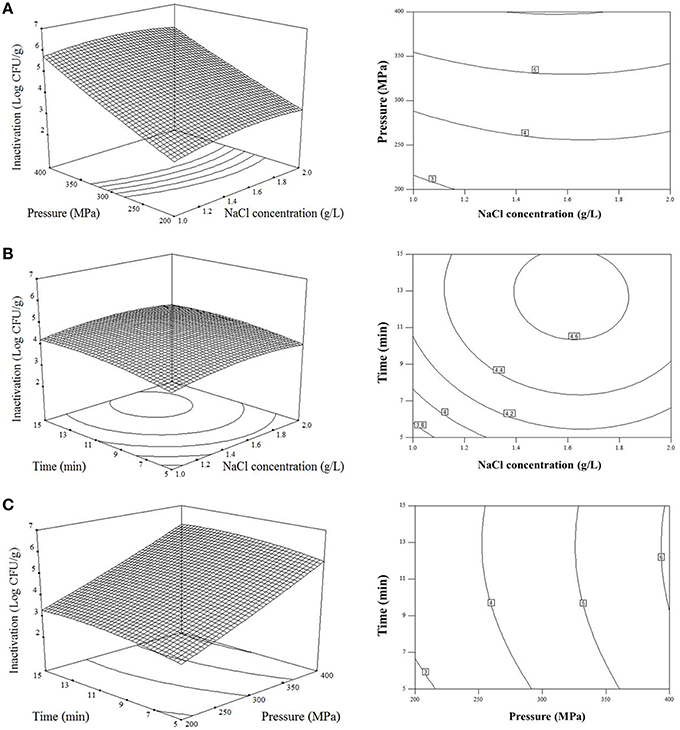
Figure 1. Response surface plots describing the effect of combined high hydrostatic pressure and AEW technological variables on inactivation of V. parahaemolyticus on shrimps. (A) Effects of NaCl concentration to electrolysis (X1) and pressure (X2) on reduction of V. parahaemolyticus with treatment time of 10 min; (B) Effects of NaCl concentration to electrolysis (X1) and treatment time (X3) on reduction of V. parahaemolyticus with pressure of 300 MPa; (C) Effects of pressure (X2) and treatment time (X3) on reduction of V. parahaemolyticus with electrolyzing NaCl concentration of 1.5 g/L.
Validation is an important step, which can assess the capacity of developed models (Bover-Cid et al., 2012). Therefore, an external validation was carried out. The results of additional eight independent experiments (Table 3) shown in Figure 2A were used to calculate the Af, Bf, and RSME based on Equations (1–3). The Af value was 1.03, revealing a merely 3% difference between the observations and predictions. The calculated Bf was 1.01, which lies in the acceptable range of 0.9–1.05 proposed by Ross (1996). RMSE (0.19) revealed that the predicted values from RS model fitted well with the observed data.
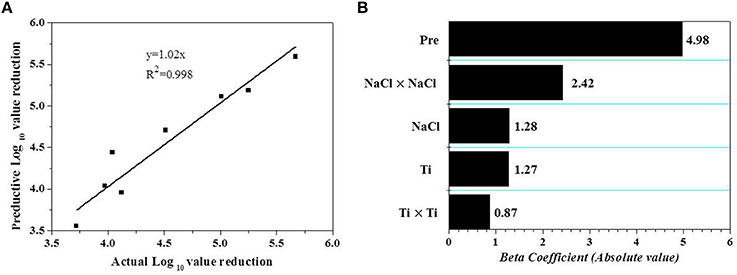
Figure 2. (A) Linear correlation between observed and predicted reductions of V. parahaemolyticus on shrimps under additional random 8 conditions within the range of the experimental domain. (B) Pareto chart of the effects of technological (independent) variables with statistical significance (p < 0.05) on the AEW-HHP inactivation of V. parahaemolyticus on shelled fresh shrimp. Beta coefficients correspond to the standardized coefficients estimated by the regression analysis. Pre and Ti are the abbreviation for pressure and time.
After the establishment of the model, a Pareto chart was used to investigate the relative contribution of each variable to the V. parahaemolyticus inactivation (Figure 2B). The result showed that the treatment pressure was the most important factor determining the effect of AEW-HHP on inactivation of V. parahaemolyticus on shelled fresh shrimp within the selected variables, and the impact of the independent variable and their interactions was ranked as X2 > > X1 = X3 > according to Figure 2B.
Effect of AEW-HHP on Artificially Contaminated shelled Fresh Shrimp
The initial microbial populations on shelled fresh shrimp, after inoculating and drying in a bio-safety cabinet for about 1 h, were 7.12 and 6.94 log10 CFU/g for V. parahaemolyticus and L. monocytogenes, respectively. Figure 3 shows the population reduction of V. parahaemolyticus and L. monocytogenes on shelled fresh shrimp after HHP and AEW-HHP at 200, 300, and 400 MPa for 10 min, respectively. There were significant differences between the microbial populations of AEW-HHP treated shrimp and those of HHP treated shrimp (p < 0.05). All results showed that AEW-HHP was more effective than HHP for inactivation of V. parahaemolyticus and L. monocytogenes, with a maximum reduction of about 6.08 and 5.71 log10 CFU/g at 400 MPa, respectively for AEW-HHP (Figure 3).
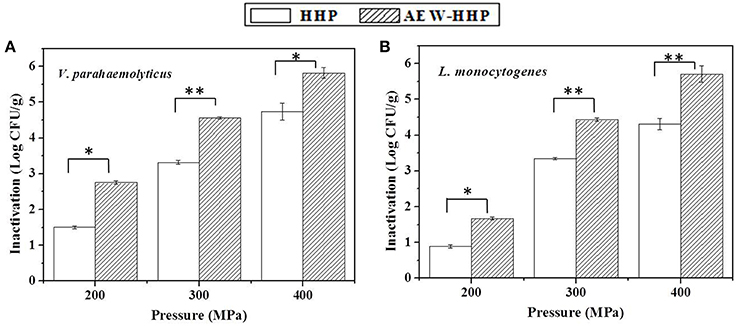
Figure 3. Population reduction of V. parahaemolyticus (A) and L. monocytogenes (B) on shelled fresh shrimp after HHP and AEW-HHP for 200, 300, and 400 MPa, respectively. Bars represent standard deviation (n = 3). Asterisks on the bars within the same pressure indicate significant differences (p < 0.05).
The impacts of AEW-HHP on the micromorphology of bacteria on shelled fresh shrimp were observed by SEM and the results presented in Figure 4. As shown in Figure 4, a large amount of V. parahaemolyticus and L. monocytogenes existed on the surface of the untreated shrimp samples as the control. There were still some bacterial populations on the HHP-treated shelled fresh shrimp surfaces (Figure 4), while very few microbial cells were found on the surfaces of shrimp samples after AEW-HHP treatment (Figures 4A,B). Therefore, these results showed that AEW-HHP had a remarkable efficacy on reducing the bacteria on shelled fresh shrimp.
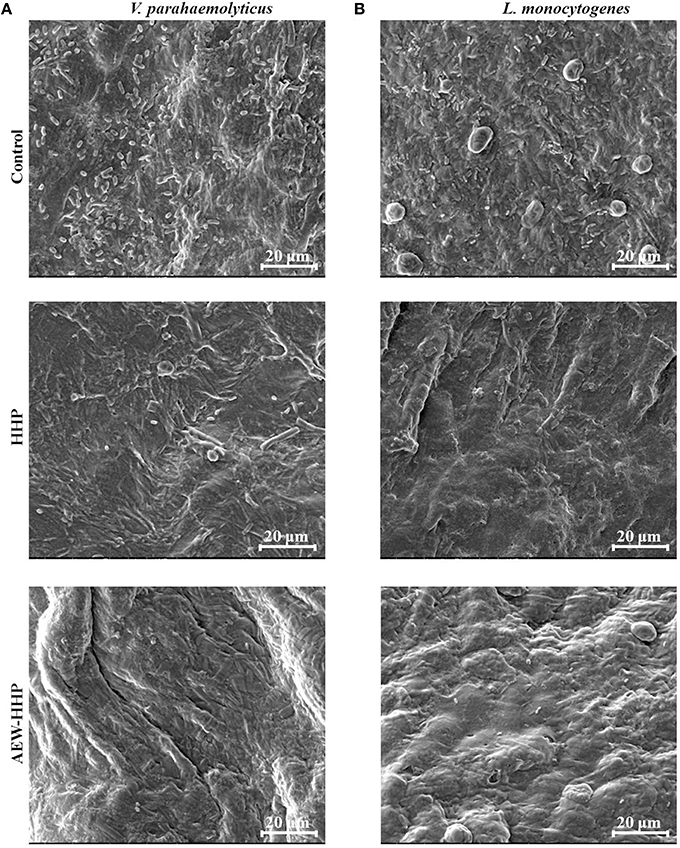
Figure 4. Scanning electron microscopy (SEM) graphs of microbial populations on shelled fresh shrimp inoculated with V. parahaemolyticus (A) and L. monocytogenes (B) after different treatments. All treatments and determinations were performed in triplicate. The treatments are as follows: untreated as control; HHP treated (NaCl concentration to electrolysis 0 g/L, treatment pressure 400 MPa, treatment time 10 min); AEW-HHP treated (NaCl concentration to electrolysis 1.5 g/L, treatment pressure 400 MPa, treatment time 10 min).
Effect of AEW-HHP on Indigenous Microflora on Shelled Fresh Shrimp
Efficacy of AEW-HHP in reducing indigenous microflora on shelled fresh shrimp is reported in Figure 5A. The initial microbial population of total aerobic bacteria in untreated fresh shelled shrimp was 6.72 log10 CFU/g. The micro flora decreased 0.98, 3.31, and 4.29 log10 CFU/g for 200, 300, and 400 MPa, respectively after HHP. In comparison with HHP, AEW-HHP treatment had obviously better disinfection efficacy. AEW-HHP at 200, 300, and 400 MPa could effectively reduce the microbial population by up to 1.47, 4.33, and 5.66 log10 CFU/g, respectively. There were significant differences between the microbial populations of AEW-HHP treated shrimp and those of HHP treated shrimp (p < 0.05).
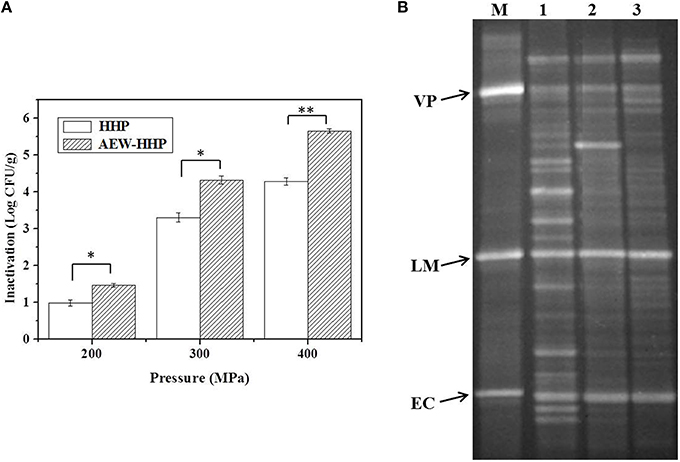
Figure 5. Population reduction of natural micro flora (A) and PCR-DGGE fingerprints of bacterial flora (B) on shelled fresh shrimp after HHP and AEW-HHP treatment. Bars represent standard deviation (n = 3). Asterisks on the bars within the same pressure indicate significant differences (p < 0.05). M, marker; 1, untreated; 2, HHP treated; 3, AEW-HHP treated; VP, V. parahaemolyticus; LM, L. monocytogenes; EC, Escherichia coli.
PCR-DGGE method was carried out to investigate the effect of HHP and AEW-HHP on the diversity of microbial communities in shrimp. The results of PCR-DGGE are shown in Figure 5B. DGGE fingerprints clearly indicated that bacterial diversity in shrimp could be reduced by both HHP and AEW-HHP treatment, while AEW-HHP treatment displayed a better effectiveness than HHP. The Shannon index H′ of untreated shrimp samples was 2.883, and this value changed to 2.418 by HHP treatment. Surprisingly, the Shannon index H′ was decreased to 2.064 when AEW-HHP was applied to treat the shrimp samples. Moreover, the average similarity coefficient of DGGE fingerprints of microbial communities treated with AEW-HHP (0.567) was lower than that with HHP (0.716). Therefore, these results directly demonstrated that AEW-HHP had a stronger efficacy on reducing the diversity of microbial communities in shelled fresh shrimp.
Effect of AEW-HHP on Muscle Fiber of Shelled Fresh Shrimp
Figure 6 shows the light microscopic images of muscle fibers in shelled fresh shrimp treated with AEW-HHP and HHP. In the control group, the muscle fibers were tightly attached to each other and gaps between muscle fibers were much smaller with its percentage being 0.532 ± 0.043. During the subsequent treatment, the percentage of blank area was 0.478 ± 0.016 and 0.494 ± 0.022 with HHP (Figure 6B) and AEW-HHP (Figure 6C), respectively. Based on the microscopic histological analysis, AEW-HHP treatment not only could reduce natural micro flora on shelled fresh shrimp, but also had no impact on the muscle tissue of shrimp when compared with the control group (p > 0.05).
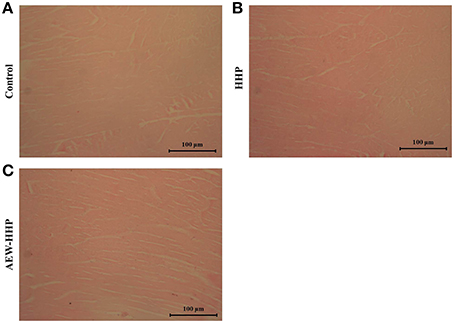
Figure 6. Light microscopy observations on longitudinal sections of shrimp untreated as control (A), HHP treated (B), and AEW-HHP treated (C).
Discussion
An effective sterilization and preservation technology is a prerequisite for food safety and quality in the food industry (Heinz and Buckow, 2010; Balasubramaniam et al., 2015). Developing a food preservation technology, which could markedly induce microbial inactivation and improve safety of the processed products, is necessary in the manufacturing process of food industries (Belletti et al., 2013b; Reineke et al., 2015). In the present study, we succeeded to indicate that AEW could be regarded as a new transmitting medium instead of common water for significantly improving the sterilizing effect of HHP on shelled fresh shrimp. This novel approach has great application prospect in the food industry for its excellent performance.
The optimal conditions of AEW-HHP for V. parahaemolyticus inactivation on shelled fresh shrimp were obtained by using a response surface methodology. The results of the model showed that 1.5 g/L was the optimal NaCl concentration to produce the AEW, and the optimal treatment pressure and treatment time were determined as 400 MPa and 10 min respectively. Under the optimized treatment conditions, this method could effectively reduce V. parahaemolyticus on the shelled fresh shrimp by 6.08 log10 CFU/g. The response surface model developed in this study gave strong evidence that AEW combined with HHP could effectively eliminate V. parahaemolyticus in seafood, and also this model could be applied in the future for assessing the infection risk reduction of V. parahaemolyticus (Espinosa et al., 2012; Praveen et al., 2013).
In order to further verify the sterilization performance of this AEW-HHP method for other bacteria, L. monocytogenes and indigenous microflora on shelled fresh shrimp were investigated in this study. Due to the fact that the optimal conditions for V. parahaemolyticus inactivation may not be appropriate for other microorganisms, we evaluated the reduction of L. monocytogenes and indigenous microflora on shrimp samples at 200, 300, and 400 MPa (Figures 3, 5A). Compared with common water as the pressure transmitting medium, AEW-HHP dramatically enhanced the inactivation effect for V. parahaemolyticus inactivation from 4.74 up to 6.08 log10 CFU/g. For L. monocytogenes, AEW-HHP could reduce 5.71 log10 CFU/g, while HHP could only reduce 4.31 log10 CFU/g. A similar result was observed for indigenous microflora on shelled fresh shrimp, with AEW-HHP showing an additional reduction of 1.37 log10 CFU/g. The fact that the effect of AEW-HHP was remarkably higher than that of HHP might be explained by the synergy between bactericidal techniques. As an effective sterilizing agent, AEW not only was widely used to eliminate a variety of pathogens in food products (Xie et al., 2012; Wang et al., 2014), but also has been proven to be a reliable assistant for improving the inactivation efficiency of other intervention methods (Liu R. et al., 2013; Jemni et al., 2014; Ding et al., 2015). This study firstly revealed the synergy between AEW and HHP, and further researches will be needed to investigate the mechanisms of synergy.
The microbial populations on shrimp were further observed by SEM (Figure 4). SEM has been proven as a useful tool to provide the intuitive evidences for demonstrating the survival or death of microbial populations after treatment (Hao et al., 2015; Hossain et al., 2015). In this study, the results of SEM were in agreement with those of plate counting; reduced microbial populations were found on the surfaces of inoculated V. parahaemolyticus and L. monocytogenes shrimp samples treated by AEW-HHP in comparison with HHP treatment. Although SEM is not a quantitative technique, it provided more comprehensive information and clearly displayed the survival situation of bacteria on shrimp after AEW-HHP treatment.
Besides traditional methods, molecular techniques were also employed in this study to evaluate the efficiency of AEW-HHP. DGGE fingerprints directly demonstrated that AEW-HHP had a stronger efficacy on reducing the diversity of microbial communities on shrimp than conventional HHP (Figure 5B). Previous studies have shown that microbial communities were associated with food spoilage (Sullam et al., 2012; Chaillou et al., 2015). The ability of reducing microbial diversity on shrimp indicated that this novel method could be used for prevention of seafood spoilage. Furthermore, the muscle tissues of untreated, HHP treated and AEW-HHP treated shrimp samples were compared by microscopic histological analysis. The change of muscle tissue has been demonstrated as an important index for sensory quality of food (Lin et al., 2013; Wang et al., 2015). The results showed that AEW-HHP treatment has no impact on the muscle tissue of shrimp when compared with the untreated and HHP treated groups. That indicated that this method could be regarded as an emerging preservation technology with limited effects on the sensory quality of food.
To summarize, various results of this study have demonstrated that AEW could be used as a new transmitting medium for improvement of sterilization and preservation performance of HHP processing. The AEW-HHP method could serve as a potential sterilization and preservation technology to reduce the risk of microbial infections and ensure the aquatic products safety. Hence, the AEW-HHP approach developed in this study could provide an effective tool for improving the seafood safety and protecting public health.
Author Contributions
YZ and YP designed and drafted the work, and agreed to be accountable for all aspects of the work in ensuring that questions related to the accuracy, and final approval of the version to be published. SD, ZZ, LX, and YL did the work of acquisition, analysis and interpretation of data for the work, and revising it critically for important intellectual content, and integrity of any part of the work are appropriately investigated and resolved, and final approval of the version to be published.
Conflict of Interest Statement
The authors declare that the research was conducted in the absence of any commercial or financial relationships that could be construed as a potential conflict of interest.
Acknowledgments
This research was supported by the National Natural Science Foundation of China (31271870, 31571917), the project of Science and Technology Commission of Shanghai Municipality (14DZ1205100, 14320502100), Key Project of Shanghai Agriculture Prosperity through Science and Technology (2014, 3–5 and 2015, 4–8), Shanghai Engineering Research Center of Aquatic-Product Processing & Preservation (11DZ2280300). We would like to thank Fei Zhao, Aijing Zhao, and Jiangping Sun for their assistance in performing the experiments.
References
Ampe, F., Ben, Omar, N., Moizan, C., Wacher, C., and Guyot, J. P. (1999). Polyphasic study of the spatial distribution of microorganisms in Mexican pozol, a fermented maize dough, demonstrates the need for cultivation-independent methods to investigate traditional fermentations. Appl. Environ. Microbiol. 65, 5464–5473.
Andrews, L. S., Park, D. L., and Chen, Y. P. (2000). Low temperature pasteurization to reduce the risk of Vibrio infections from raw shell-stock oysters. Food Addit. Contam. 19, 787–791. doi: 10.1080/026520300415336
Balasubramanian, S., and Balasubramaniam, V. M. (2003). Compression heating influence of pressure transmitting fluids on bacteria inactivation during high pressure processing. Food Res. Int. 36, 661–668. doi: 10.1016/S0963-9969(03)00014-0
Balasubramaniam, V. M., Martínez-Monteagudo, S. I., and Gupta, R. (2015). Principles and Application of high pressure-based technologies in the food industry. Annu. Rev. Food Sci. Technol. 6, 435–462. doi: 10.1146/annurev-food-022814-015539
Belletti, N., Garriga, M., Aymerich, T., and Bover-Cid, S. (2013a). High pressure inactivation of a virulent Enterococcus faecalis on dry-cured ham: modeling the effect of processing parameters. Innov. Food Sci. Emerg. 18, 43–47. doi: 10.1016/j.ifset.2013.01.006
Belletti, N., Garriga, M., Aymerich, T., and Bover-Cid, S. (2013b). Inactivation of Serratia liquefaciens on dry-cured ham by high pressure processing. Food Microbiol. 35, 34–37. doi: 10.1016/j.fm.2013.03.001
Bover-Cid, S., Belletti, N., Garriga, M., and Aymerich, T. (2012). Response surface methodology to investigate the effect of high pressure processing on Salmonella inactivation on dry-cured ham. Food Res. Int. 45, 1111–1117. doi: 10.1016/j.foodres.2011.05.004
Chaillou, S., Chaulot-Talmon, A., Caekebeke, H., Cardinal, M., Christieans, S., Denis, C., et al. (2015). Origin and ecological selection of core and food-specific bacterial communities associated with meat and seafood spoilage. Isme J. 9, 1105–1118. doi: 10.1038/ismej.2014.202
Chen, Z., Zhu, C., and Han, Z. (2011). Effects of aqueous chlorine dioxide treatment on nutritional components and shelf-life of mulberry fruit (Morus alba L.). J. Biosci. Bioeng. 111, 675–681. doi: 10.1016/j.jbiosc.2011.01.010
Code of National Standard of China (2008). Microbiological Examination of Food Hygiene-Examination of Vibrio parahaemolyticus. Code of National Standard of China GB/T 4789.7-2008.
Díaz-Tenorio, L. M., García-Carreño, F. L., and Pacheco-Aguilar, R. (2007). Comparison of freezing and thawing treatments on muscle properties of whiteleg shrimp (Litopenaeus vannamei). J. Food Biochem. 31, 563–576. doi: 10.1111/j.1745-4514.2007.00130.x
Ding, T., Ge, Z., Shi, J., Xu, Y. T., Jones, C. L., and Liu, D. H. (2015). Impact of slightly acidic electrolyzed water (SAEW) and ultrasound on microbial loads and quality of fresh fruits. LWT-Food Sci. Technol. 60, 1195–1199. doi: 10.1016/j.lwt.2014.09.012
Dong, Q. L., Tu, K., Guo, L. Y., Li, H. W., and Zhao, Y. (2007). Response surface model for prediction of growth parameters from spores of Clostridium sporogenes under different experimental conditions. Food Microbiol. 24, 624–632. doi: 10.1016/j.fm.2006.12.003
Espinosa, A. C., Jesudhasan, P., Arredondo, R., Cepeda, M., Mazari-Hiriart, M., Mena, K. D., et al. (2012). Quantifying the reduction in potential health risks by determining the sensitivity of poliovirus type 1 chat strain and rotavirus SA-11 to electron beam irradiation of iceberg lettuce and spinach. Appl. Environ. Microbiol. 78, 988. doi: 10.1128/AEM.06927-11
Georget, E., Sevenich, R., Reineke, K., Mathy, A., Heinz, V., Callanan, M., et al. (2015). Inactivation of microorganisms by high isostatic pressure processing in complex matrices: a review. Innov. Food Sci. Emerg. 27, 1–14. doi: 10.1016/j.ifset.2014.10.015
Hao, J., Li, H., Wan, Y., and Liu, H. (2015). Combined effect of acidic electrolyzed water (AcEW) and alkaline electrolyzed water (AlEW) on the microbial reduction of fresh-cut cilantro. Food Control 50, 699–704. doi: 10.1016/j.foodcont.2014.09.027
Heinz, V., and Buckow, R. (2010). Food preservation by high pressure. J. Verbrauch. Lebensm. 5, 73–81. doi: 10.1007/s00003-009-0311-x
Hossain, M. S., Rahman, N. N. N. A., Balakrishnan, V., Alkarkhi, A. F. M., Rajion, Z. A., and Kadir, M. O. A. (2015). Optimizing supercritical carbon dioxide in the inactivation of bacteria in clinical solid waste by using response surface methodology. Waste Manage. 38, 462–473 doi: 10.1016/j.wasman.2015.01.003
Hricova, D., Stephan, R., and Zweifel, C. (2008). Electrolyzed water and its application in the food industry. J. Food Prot. 71, 1934–1947. doi: 10.5167/uzh-4971
Huang, Y. R., Hung, Y. C., Hsu, S. Y., Huang, Y. W., and Hwang, D. F. (2008). Application of electrolyzed water in the food industry. Food Control 19, 329–345. doi: 10.1016/j.foodcont.2007.08.012
Jemni, M., Gomez, P. A., Souza, M., Chaira, N., Ferchichi, A., Otón, M., et al. (2014). Combined effect of UV-C, ozone and electrolyzed water for keeping overall quality of date palm. LWT-Food Sci. Technol. 59, 649–655. doi: 10.1016/j.lwt.2014.07.016
Katayose, M., Yoshida, K., Achiwa, N., and Eguchi, M. (2007). Safety of electrolyzed seawater for use in aquaculture. Aquaculture 264, 119–129. doi: 10.1016/j.aquaculture.2006.08.050
Lenz, C. A., Reineke, K., Knorr, D., and Voge, R. F. (2015). High pressure thermal inactivation of Clostridium botulinum type E endospores-kinetic modeling and mechanistic insights. Front. Microbiol. 6:652. doi: 10.3389/fmicb.2015.00652
Lin, T., Wang, J. J., Li, J. B., Liao, C., Pan, Y. J., and Zhao, Y. (2013). Use of acidic electrolyzed water ice for preserving the quality of shrimp. J. Agric. Food Chem. 61, 8695–8702. doi: 10.1021/jf4019933
Liu, C. C., Duan, J. Y., and Su, Y. C. (2006). Efficiency of electrolyzed oxidizing water on reducing Listeria monocytogenes contamination on seafood processing gloves. Int. J. Food Microbiol. 110, 149–154. doi: 10.1016/j.ijfoodmicro.2006.02.004
Liu, D., Liang, L., Xia, W., Regenstein, J. M., and Zhou, P. (2013). Biochemical and physical changes of grass carp (Ctenopharyngodon idella) fillets stored at - 3 and 0°C. Food Chem. 140, 105–114. doi: 10.1016/j.foodchem.2013.02.034
Liu, R., He, X., Shi, J., Nirasawa, S., Tatsumi, E., Li, L., et al. (2013). The effect of electrolyzed water on decontamination, germination and γ-aminobutyric acid accumulation of brown rice. Food Control 33, 1–5. doi: 10.1016/j.foodcont.2013.02.008
Lorido, L., Estevez, M., Ventanas, J., and Ventanas, S. (2015). Comparative study between Serrano and Iberian dry-cured hams in relation to the application of high hydrostatic pressure and temporal sensory perceptions. LWT-Food Sci. Technol. 64, 1234–1242. doi: 10.1016/j.lwt.2015.07.029
Mahmoud, B. S. M. (2009). Effect of X-ray treatments on inoculated Escherichia coli O157: H7, Salmonella enterica, Shigella flexneri and Vibrio parahaemolyticus in ready-to-eat shrimp. Food Microbiol. 26, 860–864. doi: 10.1016/j.fm.2009.05.013
Ma, L., and Su, Y. C. (2011). Validation of high pressure processing for inactivating Vibrio parahaemolyticus in Pacific oysters (Crassostrea gigas). Int. J. Food Microbiol. 144, 469–474. doi: 10.1016/j.ijfoodmicro.2010.10.037
Ma, M., and Mu, T. (2016). Modification of deoiled cumin dietary fiber with laccase and cellulose under high hydrostatic pressure. Carbohyd. Polym. 136, 87–94. doi: 10.1016/j.carbpol.2015.09.030
McCarthy, S. A. (1997). Incidence and survival of Listeria monocytogenes in ready-to-eat seafood products. J. Food Protect. 60, 372–376.
Muyzer, G., De Waal, E. C., and Uitterlinden, A. G. (1993). Profiling of complex microbial populations by denaturing gradient gel electrophoresis analysis of polymerase chain reaction-amplified genes coding for 16S rRNA. Appl. Environ. Microbiol. 59, 695–700.
Nirmal, N. P., and Benjakul, S. (2010). Effect of catechin and ferulic acid on melanosis and quality of Pacific white shrimp subjected to freeze-thawing prior refrigerated storage. Food Control 21, 1263–1271. doi: 10.1016/j.foodcont.2010.02.015
Norhana, W. M. N., Poole, E. S., Deeth, C. H., and Dykes, A. G. (2010). Prevalence, persistence and control of Salmonella and Listeria in shrimp and shrimp products: a review. Food Control 21, 343–361. doi: 10.1016/j.foodcont.2009.06.020
Ogino, A., Koshikawa, H., Nakahara, T., and Uchiyama, H. (2001). Succession of microbial communities during a biostimulation process as evaluated by DGGE and clone library analyses. J. Appl. Microbiol. 91, 625–635. doi: 10.1046/j.1365-2672.2001.01424.x
Omer, M. K., Prieto, B., Rendueles, E., Alvarez-Ordoñez, A., Lunde, K., Alvseike, O., et al. (2015). Microbiological, physicochemical and sensory parameters of dry fermented sausages manufactured with high hydrostatic pressure processed raw meat. Meat Sci. 108, 115–119. doi: 10.1016/j.meatsci.2015.05.002
Prakash, B., Singh, P., Kedia, A., and Dubey, N. K. (2012). Assessment of some essential oils as food preservatives based on antifungal, antiaflatoxin, antioxidant activities and in vivo efficacy in food system. Food Res. Int. 49, 201–208. doi: 10.1016/j.foodres.2012.08.020
Praveen, C., Dancho, B. A., Kingsley, D. H., Calci, K. R., Meade, G. K., Mena, K. D., et al. (2013). Susceptibility of murine norovirus and hepatitis A virus to electron beam irradiation in oysters and quantifying the reduction in potential infection risks. Appl. Environ. Microbiol. 79, 3796. doi: 10.1128/AEM.00347-13
Reineke, K., Schottroff, F., Meneses, N., and Knorr, D. (2015). Sterilization of liquid foods by pulsed electric fields-an innovative ultra-high temperature process. Front. Microbiol. 6:400. doi: 10.3389/fmicb.2015.00400
Rendueles, E., Omer, M. K., Alvseike, O., Alonso-Calleja, C., Capita, R., and Prieto, M. (2011). Microbiological food safety assessment of high hydrostatic pressure processing: a review. LWT - Food Sci. Tech. 44, 1251–1260. doi: 10.1016/j.lwt.2010.11.001
Ross, T. (1996). Indices for performance evaluation of predictive models in food microbiology. J. Appl. Bacteriol. 81, 501–508. doi: 10.1111/j.1365-2672.1996.tb03539.x
Rubio, R., Bover-Cid, S., Martin, B., Garriga, M., and Aymerich, T. (2013). Assessment of safe enterococci as bio-protective cultures in low-acid fermented sausages combined with high hydrostatic pressure. Food Microbiol. 33, 158–165. doi: 10.1016/j.fm.2012.09.012
Sevenich, R., Reineke, K., Hecht, P., Fröhling, A., Rauh, C., Schlüter, O., et al. (2015). Impact of different water activities (aw) adjusted by solutes on high pressure high temperature inactivation of Bacillus amyloliquefaciens spores. Front. Microbiol. 6:689. doi: 10.3389/fmicb.2015.00689
Shen, Q., and Zhiguo, L. (2008). Molecular Biological Techniques. Beijing: China Chemical Industry Press.
Smigic, N., Rajkovic, A., Antal, E., Medic, H., Lipnicka, B., Uyttendaele, M., et al. (2009). Treatment of Escherichia coli O157:H7 with lactic acid neutralized electrolyzed oxidizing water and chlorine dioxide followed by growth under sub-optimal conditions of temperature, pH and modified atmosphere. Food Microbiol. 26, 629–637. doi: 10.1016/j.fm.2009.04.010
Sullam, K. E., Essinger, S. D., Lozupone, C. A., O'Connor, M. P., Rosen, G. L., Knight, R., et al. (2012). Environmental and ecological factors that shape the gut bacterial communities of fish: a meta-analysis. Mol. Ecol. 21, 3363–3378. doi: 10.1111/j.1365-294X.2012.05552.x
Wang, J. J., Zhang, Z. H., Li, J. B., Lin, T., Pan, Y. J., and Zhao, Y. (2014). Modeling Vibrio parahaemolyticus inactivation by acidic electrolyzed water on cooked shrimp using response surface methodology. Food Control 36, 273– 279. doi: 10.1016/j.foodcont.2013.08.031
Wang, M., Wang, J. J., Sun, X. H., Pan, Y. J., and Zhao, Y. (2015). Preliminary mechanism of acidic electrolyzed water ice on improving the quality and safety of shrimp. Food Chem. 176, 333–341. doi: 10.1016/j.foodchem.2014.12.089
Wang, W., Li, M., Fang, W., Pradhan, A. K., and Li, Y. (2013). A predictive model for assessment of decontamination effects of lactic acid and chitosan used in combination on Vibrio parahaemolyticus in shrimps. Int. J. Food Microbiol. 167, 124–130. doi: 10.1016/j.ijfoodmicro.2013.07.012
Xie, J., Sun, X. H., Pan, Y. J., and Zhao, Y. (2012). Combining basic electrolyzed water pretreatment and mild heat greatly enhanced the efficacy of acidic electrolyzed water against Vibrio parahaemolyticus on shrimp. Food Control 23, 320–324. doi: 10.1016/j.foodcont.2011.07.019
Keywords: acidic electrolyzed water, high hydrostatic pressure processing, indigenous microflora, inactivation, aquatic products
Citation: Du S, Zhang Z, Xiao L, Lou Y, Pan Y and Zhao Y (2016) Acidic Electrolyzed Water as a Novel Transmitting Medium for High Hydrostatic Pressure Reduction of Bacterial Loads on Shelled Fresh Shrimp. Front. Microbiol. 7:305. doi: 10.3389/fmicb.2016.00305
Received: 21 December 2015; Accepted: 24 February 2016;
Published: 09 March 2016.
Edited by:
Amit Kumar Tyagi, The University of Texas MD Anderson Cancer Center, USAReviewed by:
Alberto Scoma, Gent University, BelgiumZhao Chen, Clemson University, USA
Avelino Alvarez-Ordóñez, Teagasc Food Research Centre, Ireland
Copyright © 2016 Du, Zhang, Xiao, Lou, Pan and Zhao. This is an open-access article distributed under the terms of the Creative Commons Attribution License (CC BY). The use, distribution or reproduction in other forums is permitted, provided the original author(s) or licensor are credited and that the original publication in this journal is cited, in accordance with accepted academic practice. No use, distribution or reproduction is permitted which does not comply with these terms.
*Correspondence: Yong Zhao, eXpoYW9Ac2hvdS5lZHUuY24=
†These authors have contributed equally to this work.