- 1Parasitology Division, Council of Scientific and Industrial Research–Central Drug Research Institute, Lucknow, India
- 2Department of Medicine, Institute of Medical Sciences, Banaras Hindu University, Varanasi, India
- 3Molecular and Structural Biology Division, Council of Scientific and Industrial Research–Central Drug Research Institute, Lucknow, India
Our prior studies demonstrated that cellular response of T helper 1 (Th1) type was generated by a soluble antigenic fraction (ranging from 89.9 to 97.1 kDa) of Leishmania donovani promastigote, in treated Leishmania patients as well as hamsters and showed significant prophylactic potential against experimental visceral leishmaniasis (VL). Eighteen Th1 stimulatory proteins were identified through proteomic analysis of this subfraction, out of which 15 were developed as recombinant proteins. In the present work, we have evaluated these 15 recombinant proteins simultaneously for their comparative cellular responses in treated Leishmania patients and hamsters. Six proteins viz. elongation factor-2, enolase, aldolase, triose phosphate isomerase, protein disulfide isomerase, and p45 emerged as most immunogenic as they produced a significant lymphoproliferative response, nitric oxide generation and Th1 cytokine response in PBMCs and lymphocytes of treated Leishmania patients and hamsters respectively. The results suggested that these proteins may be exploited for developing a successful poly-protein and/or poly-epitope vaccine against VL.
Introduction
Visceral leishmaniasis (VL), a life-threatening systemic disease also referred as black sickness or kala-azar, is caused by Leishmania (L.) donovani and L. infantum (chagasi) and is transmitted to the human host via the bite of infected female dipteran vector, sandfly. This disease is widespread in the Indian subcontinent, East Africa, Mediterranean basin, Central and South America. About 90% of the annual 100 000 cases of VL are from India, Sudan, Brazil, Ethiopia, Bangladesh, and Nepal. Human migration and environmental changes lead to further expansion of the geographical range of this disease which ultimately affects the epidemiological triad (Desjeux, 2004). In India, Bihar serves as the major epicenter of this disease as it covers 80% of VL cases (Hasker et al., 2012). Available chemotherapeutics proved to be inadequate to curb this disease due to its toxicity and are also not cost effective (Coler et al., 2015). Current kala-azar control roadmap in the Indian subcontinent is threatened by the occurrence of relapse cases (Croft et al., 2006; Srivastava et al., 2011; Garcia-Hernandez et al., 2012; Mohapatra, 2014). Immune response, in particular, cell-mediated immune (CMI) response, is severely compromised during active VL, therefore, its up-regulation is indispensable for the parasite’s clearance (Stanley and Engwerda, 2007). A suitable vaccine against VL offers a viable alternative keeping in view the fact that Leishmania-infected individuals generate life-long immunity. Also, considering the existence of 80–90% of subclinical or asymptomatic Leishmania cases (endemic healthy contacts) as well as post kala-azar dermal leishmanoid (PKDL) cases, a safe and effective vaccine will be critical if the success of recent VL control efforts in Indian subcontinent is to be sustained (Engwerda and Matlashewski, 2015).
For the reduction of parasitic burden in infected individuals, generation of interleukin (IL)-2, interferon (IFN)-γ and tumor necrosis factor (TNF)-α, indicative of T helper 1 (Th1) type response is essential. Also, there requires a balance between proinflammatory IFN-γ/TNF-α and regulatory IL-10 cytokines (Coler et al., 2015). Leishmanial antigens with predominant Th1 type response in infected rodent models have been recognized as ‘potential protective antigens’ and, therefore, promising vaccine candidates. Based on this, several antigens were evaluated which shows mild to moderate protection in different animal models (mice and hamster) and human subjects as reviewed by Joshi et al. (2014). Moreover, current strategies for vaccine development have advanced toward immunologically vital miniature antigenic regions, i.e., the epitopes identified from potential native or recombinant proteins as they are competent enough to generate protective immunity against infectious organisms. Furthermore, designing of vaccines containing multiple epitopes derived from different antigens, i.e., poly-epitope vaccines fortify the immune response via targeting multiple antigenic regions. Also, due to the genetic polymorphism of the mammalian immune system, a multi-component vaccine thought to elicit a better protective immune response (Goto et al., 2011).
Our earlier studies, using classical activity based fractionation and sub-fractionation of the soluble proteins from an Indian L. donovani promastigote, led to the identification of a potential sub-fraction (89.9–97.1 kDa) which induced Th1 type cellular response in peripheral blood mononuclear cells (PBMCs)/lymphocytes of treated Leishmania patients and hamsters. Furthermore, this fraction also offered significant prophylactic efficacy in hamsters against Leishmania challenge (Garg et al., 2006; Kumari et al., 2008a,b). Subsequently, 18 Th1 stimulatory proteins were identified through proteomic characterization of this subfraction (Kumari et al., 2008b). Of these, 15 could be developed as recombinant proteins, some of which were subjected to biochemical and immunological characterization and were assessed for their suitability as prophylactic vaccine in hamster model (Kushawaha et al., 2011, 2012a,b; Gupta et al., 2012, 2014; Jaiswal et al., 2014; Khare et al., 2014; Baharia et al., 2015). In the present communication, all the recombinant proteins were evaluated simultaneously for their comparative immunogenicity (cellular responses) in PBMCs and lymphocytes of treated Leishmania patients as well as hamsters in order to identify the most potent ones which can be further taken up for developing potential poly-protein and/or poly-epitope vaccine against VL.
Materials and Methods
Host and Parasite
Golden hamsters (Mesocricetus auratus, 50–55 g) housed in the climatically controlled room, served as a suitable experimental host. The care and use of hamsters were monitored by institutional animal ethics committee (IAEC) which also approved animal experimentation protocols (Approval No. 150/09/Para/IAEC dated 23.10.09) following guidelines of committee for the purpose of control and supervision of experiments on animals (CPCSEA). L. donovani strain (MHOM/IN/80/Dd8) was procured as promastigotes from American type culture collection (ATCC, Manassas, VA, USA) and was maintained in vitro following the protocol of Garg et al. (2005). Parasite’s virulence was maintained by serial passaging of amastigote in hamsters (Dube et al., 2005).
Soluble L. donovani (SLD) Promastigote Antigen and Recombinant Proteins
The preparation of SLD antigen from metacyclic promastigotes was done as per the method of Gupta et al. (2007) and stored at -80°C till further use. Fifteen recombinant Th1 stimulatory proteins utilized in this study namely elongation factor-2 (elF-2), p45, aldolase (Aldo), enolase (Eno), triosephosphate isomerase (TPI), protein disulfide isomerase (PDI), cofactor-independent phosphoglycerate mutase (iPGM), adenosyl homocysteinase (ADHT), calreticulin (Cal), heat shock proteins (Hsp) – Hsp70 and Hsp83, trypanothione reductase (TPR), NAD-dependent deacetylase SIR2 homolog (NAD) and two hypothetical proteins (Hypo 1 and 2) have been successfully cloned, expressed and purified. Sequences of all the 15 proteins have been submitted to the national center for biotechnology information (NCBI) as depicted in Table 1.
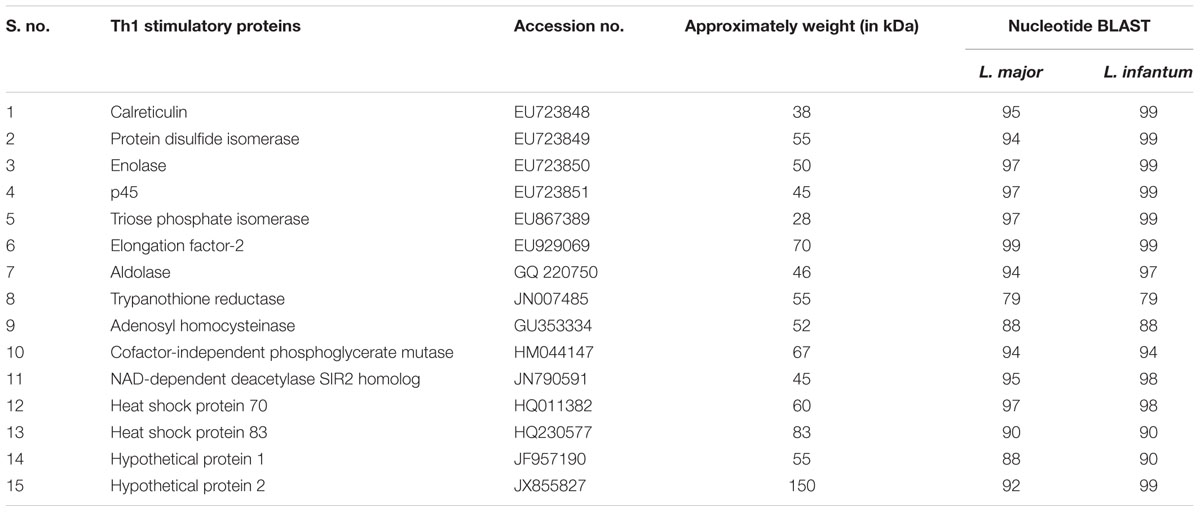
TABLE 1. List of recombinant proteins with their accession no. submitted to the national center for biotechnology information (NCBI).
Isolation of Lymph Node Cells from Treated Leishmania-Infected Hamsters
A total of 40 hamsters were utilized in this study. A 0.1 ml of inoculum containing 107 amastigotes/ml was injected intracardially in 30 hamsters while rest of the 10 animals were kept as uninfected control. The magnitude of infection in infected animals were assessed 1 month later by performing a splenic biopsy. Miltefosine (Synphabase, Switzerland), an oral antileishmanial drug, was administered in 15 out of 30 infected hamsters @ 40 mg/kg bodyweight daily for 5 days. Remaining 15 animals were kept as infected control. The method of Garg et al. (2005) was used for isolation of mononuclear lymph node cells.
Isolation of PBMCs from Treated VL Patients
Human ethics committee of the kala-azar medical research center (KMRC), Muzaffarpur approved the protocols and study on human subjects belonging to hyper-endemic areas of Bihar (Approval No EC-KAMRC/Vaccine/VL/2007-01). A written informed consent was also taken from all of them before their enrollment to this study. All the study subjects, as shown in Table 2, were examined for leishmanial as well as other possible infections by a local clinician. Venous blood samples were collected from one to 6-months-old follow up ambisome or amphotericin B treated VL patients (confirmed by physical examination and clinical symptoms) as well as from healthy contacts (neither showed any clinical symptoms nor received any treatment for kala-azar). PBMCs were isolated from the collected venous blood following the method of Garg et al. (2005).
Lymphocyte Proliferation and Nitric Oxide Assay
A 0.1 ml of cell suspension (PBMCs from treated patients/healthy contacts and mononuclear cells from treated/uninfected hamsters) in complete RPMI-1640 (Sigma-Aldrich, USA) containing 1×106 cells/ml was seeded in 96-well flat-bottom tissue culture plates (Nunc, Denmark). Each of the 15 recombinant proteins viz. rLdPDI, rLdTPI, rLdelF-2, rLdp45, rLdAldo, rLdEno, rLdiPGM, rLdADHT, rLdCal, rLdHsp70, rLdHsp83, rLdTPR, rLdNAD and the two hypothetical proteins- rLdHypo1 and rLdHypo2 as well as SLD (10 μg/ml/well) were used separately in triplicate. Phytohemagglutinin (PHA, 10 μg/ml, Sigma-Aldrich, USA) for Patient’s PBMCs and concanavalin A (ConA, 10 μg/ml, Sigma-Aldrich, USA) for hamster’s mononuclear cells were used as standard mitogens. Wells without stimulants served as blank controls. Cells were maintained in a CO2 incubator (37°C with 5% CO2) for 3 days in case of mitogens and for 5 days in case of recombinant proteins. 50 μl of XTT (Biological Industries, Israel) was added to 100 μl of culture supernatant around 18 h prior to termination of the experiment. The absorbance of the samples, at 480 nm with 650 nm as a reference wavelength, was measured in a SPECTRAmax PLUS 384 microplate reader (Molecular Devices, USA).
Similarly, nitric oxide (NO) generation was estimated by co-culturing J774A.1 macrophages with 100 μl supernatant of stimulated mononuclear cells for 24 h and then by adding an equal amount of Griess reagent (Sigma-Aldrich, USA; Garg et al., 2005). The absorbance of the reaction was measured at 540 nm (Ding et al., 1988). Lipopolysaccharide (LPS, 10 μg/ml, Sigma-Aldrich, USA) was used as standard NO producer.
Estimation of Th1/Th2 Cytokines in Culture Supernatant of PBMCs of Treated Patients/Healthy Contacts
Peripheral blood mononuclear cells (1 × 106 cells/ml) from treated human patients as well as healthy contacts were plated in 96-well culture plates (Nunc, Denmark). Recombinant proteins at a concentration of 10 μg/ml were added to triplicate wells and incubated for 5 days. The levels of IFN-γ, IL-12, TNF-α, IL-10, IL-4, and TGF-β (in pg/ml) were estimated in the culture supernatant by ELISA (enzyme-linked immunosorbent assay) kit (BD OptEIA, Pharmingen).
Statistical Analysis
Two sets of independent experiments (each containing 3–5 animals) were performed. One-way ANOVA was applied in cell-proliferation assay and NO estimation by comparing recombinant proteins group with SLD in treated hamsters as well as in human patients using GraphPad Prism 6.01 (GraphPad Software, San Diego, CA, USA). Results were expressed as mean ± SE. Significance was considered when the p-values were <0.05.
Results
Recombinant Th1 Stimulatory Proteins Induce Strong Lymphoproliferative Response in Treated Leishmania Hamsters and NO Production in J774 Macrophages
The cellular response generated by lymphocytes isolated from lymph nodes of treated hamsters as assessed by XTT against SLD and 15 recombinant proteins have been shown in Figure 1A. Significantly, a higher proliferative response against Con A was observed in treated hamsters indicating the procedural sensitivity (data not shown). Ten recombinant proteins viz. rLdEno, rLdPDI, rLdTPI, rLdelF-2, rLdp45, rLdAldo, rLdADHT, rHsp83 and the two hypothetical proteins- rLdHypo1 and rLdHypo2 showed significantly higher stimulation in lymphocytes isolated from treated hamsters (mean OD ± SE 3.510 ± 0.04988, 3.468 ± 0.02080, 3.420 ± 0.05009, 3.650 ± 0.04775, 3.415 ± 0.03242, 3.458 ± 0.05535, 3.303 ± 0.1672, 3.272 ± 0.06071, 3.418 ± 0.03132, and 3.343 ± 0.1215) than SLD (mean OD ± SE 2.346 ± 0.2640). Rest of the proteins has shown lesser proliferative response than SLD which was not statistically significant.
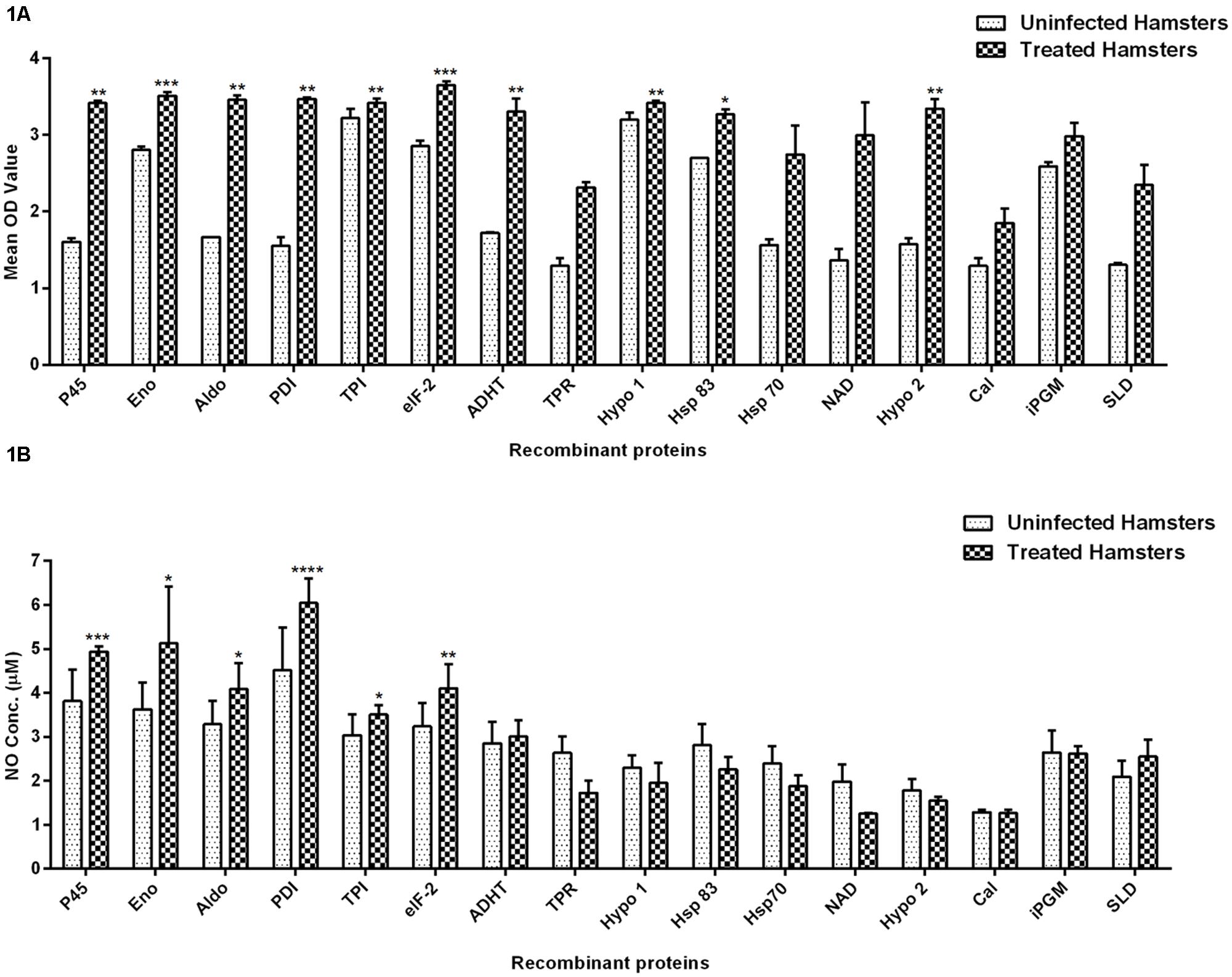
FIGURE 1. Lymphoproliferative response and NO production. (A) LTT response in lymph node cells of treated Leishmania-infected hamsters in response to 15 recombinant proteins and SLD (10 μg/ml each) and (B) Nitric oxide production measured by Griess reagent in supernatants collected from J774 A.1 macrophage cultures 24 h after incubation. Each bar represents the pooled data (mean ± SE). Significance values indicate the difference in stimulation between the SLD and the recombinant proteins (*p < 0.05, **p < 0.01, ***p < 0.001, and ****p < 0.0001).
Nitric oxide generation by J774 A.1 was studied since it is known that NO-mediated macrophage effector mechanism is critical for reducing parasitic burden in the animal model. NO production was recorded after 24 h of incubation and was found to be significantly higher against six recombinant proteins viz. rLdPDI, rLdTPI, rLdelF-2, rLdp45, rLdAldo, and rLdEno in comparison to SLD as shown in Figure 1B. NO generation by LPS stimulated cells served as positive control while unstimulated cells are considered as a negative control (data not shown).
Recombinant Th1 Stimulatory Proteins Stimulate Significant Lymphoproliferative and Predominant Thl Cytokine Response in Treated VL Patients
Peripheral blood mononuclear cells of treated Leishmania patients were further utilized to validate the cellular responses observed in treated hamsters. There were marked differences in responses generated by individual donors in each study group. Figure 2 depicted the lymphocyte proliferation response generated by 15 recombinant proteins in comparison to SLD. Seven out of 15 proteins, i.e., rLdPDI, rLdTPI, rLdelF-2, rLdp45, rLdAldo, rLdEno, and rLdHsp70 showed significantly higher lymphoproliferative response (3.001 ± 0.08074, 3.179 ± 0.04249, 3.164 ± 0.02774, 3.158 ± 0.06383, 3.038 ± 0.03051, 3.098 ± 0.08782, and 3.044 ± 0.01308) in PBMCs from treated VL patients. The higher proliferative response against PHA indicated procedural sensitivity (data not shown).
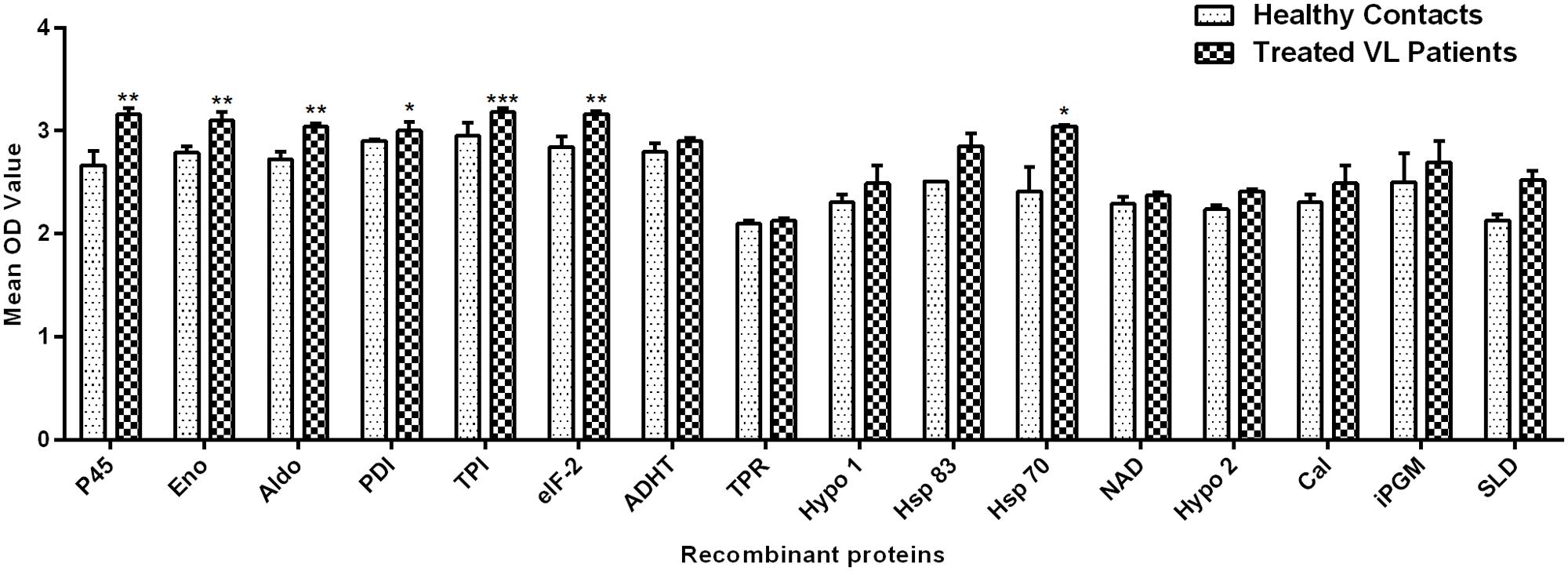
FIGURE 2. Lymphoproliferative response in PBMCs of treated VL patients in response to 15 recombinant proteins and SLD (10 μg/ml each). Each bar represents the pooled data (mean ± SE). Significance values indicate the difference in stimulation between the SLD and the recombinant proteins (*p < 0.05, **p < 0.01, and ***p < 0.001).
Cytokine responses of those recombinant proteins, that have shown optimal lymphoproliferative response and NO generation in both human PBMCs and hamster’s lymphocytes, i.e., rLdEno, rLdAld, rLdp45, rLdTPI, rLdPDI, and rLdelF-2, were measured in PBMCs of treated Leishmania patients as well as healthy contacts. The levels of IFN-γ, TNF-α, and IL-12 (Th1 cytokines) were found to be upregulated as compared to Th2 cytokines (IL-4, IL-10, and TGF-β) as shown in Table 3.
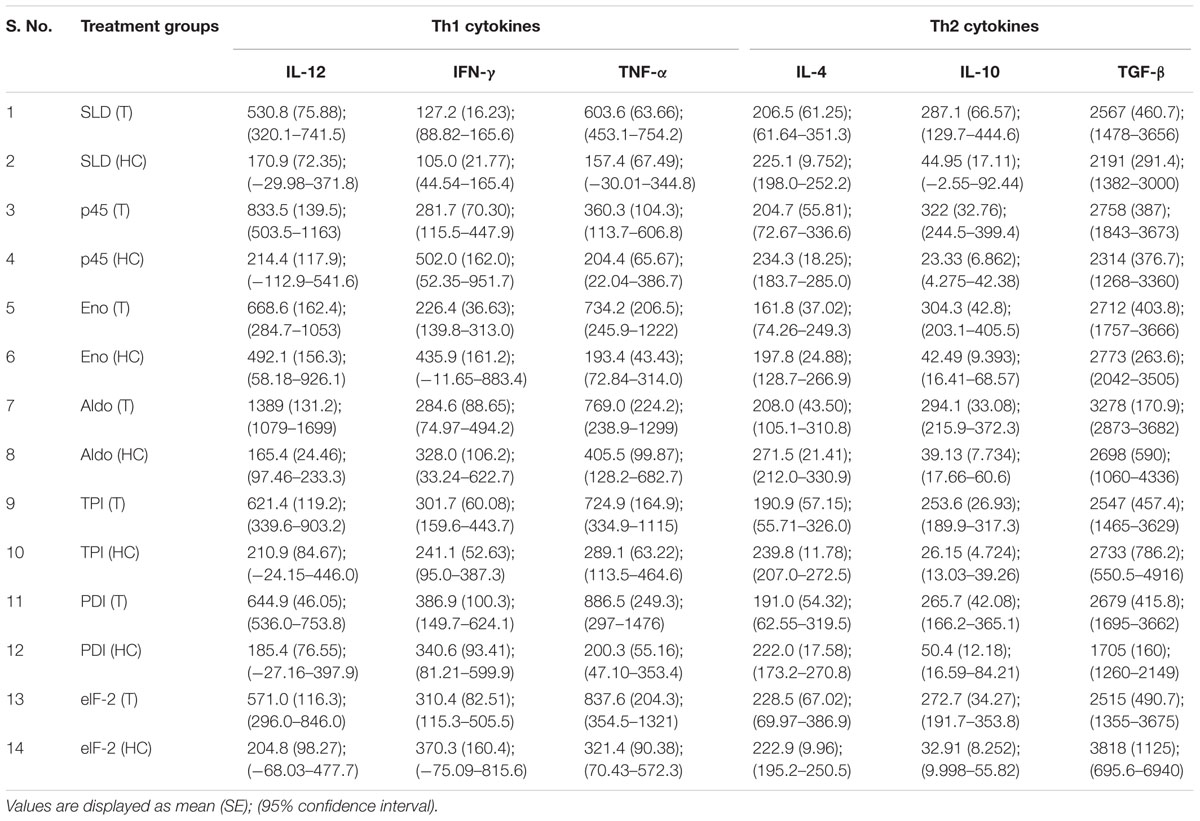
TABLE 3. Cytokine levels in recombinant proteins and SLD stimulated PBMCs from treated VL patients (T) and healthy contacts (HC).
Discussion
Successful recovery from VL due to L. donovani is associated with the development of lifelong immunity and resistance to reinfection. In view of this fact, that generation of a strong CMI response play a pivotal role in controlling VL, T-cell stimulatory antigens were thought to be good vaccine targets (Kamhawi et al., 2014). Exploration of such antigens from Indian L. donovani parasite resulted in the identification of F2 fraction (ranging from 89.9 to 97.1 kDa) that elicited cellular responses in PBMCs/lymphocytes of treated Leishmania patients/hamsters (Kumari et al., 2008a). Further, proteomic analysis of F2 fraction revealed 18 proteins (Kumari et al., 2008b) and out of these, 15 viz. elF-2, p45, Aldo, Eno, TPI, PDI, iPGM, ADHT, Cal, Hsp70, Hsp83, TPR, NAD, Hypo 1 and 2 were developed as recombinant ones. Most of these recombinant proteins were individually characterized biochemically and immunologically as potential vaccine candidates (Kushawaha et al., 2011, 2012a,b; Gupta et al., 2012, 2014; Jaiswal et al., 2014; Khare et al., 2014; Baharia et al., 2015). However, to select the most potential one(s) for further advancement toward the development of an effective poly-protein vaccine against VL, all these recombinant Th1 stimulatory proteins were simultaneously evaluated for their immunogenicity in PBMCs/lymphocytes of treated Leishmania patients/hamsters. The observation made herein indicated toward the potentiality of seven recombinant proteins viz. Eno, Aldo, p45, TPI, PDI, elF-2, and Hsp70 which was well-supported by the findings in other parasitic and infectious diseases highlighting them as a suitable vaccine candidates (Palm et al., 2003; Pal-Bhowmick et al., 2007; Avilan et al., 2011; Li et al., 2011; Chen et al., 2012; Matsubayashi et al., 2013; McNulty et al., 2013; Wang et al., 2013; Zinsser et al., 2013).
As stated earlier that due to the genetic polymorphism of the mammalian immune system, a multicomponent vaccine is considered to evoke a better protective immune response. Therefore, as a result, Q protein, Leish-111f, Leish-110f, KSAC, etc., came into existence that have been demonstrated to afford better protection against experimental VL (Molano et al., 2003; Parody et al., 2004; Coler et al., 2007; Trigo et al., 2010; Goto et al., 2011). Among these polyprotein vaccines, Leish-110f is under clinical trial in Indian population and the outcome of this vaccination trial is yet to be seen. However, since, the strains of L. donovani in East Africa and L. infantum in the Mediterranean Basin are markedly heterogeneous and genetically different from Indian strains (Abass et al., 2015) it is imperative to design and develop an indigenous polyprotein/polytope vaccine.
Since, L. donovani infection in Syrian golden hamster closely mimics systemic infection in human, producing similar clinicopathological symptoms, the animal represents as an ideal model for progressive VL (Garg and Dube, 2006). Therefore, the cellular immune response of these recombinant proteins was studied in lymphocytes of treated Leishmania-infected hamsters and was further validated in PBMCs of treated VL patients and healthy contacts.
A range of factors may contribute to the immunogenicity of proteins in VL patients but a major driver for immunogenicity is likely to be the T-cells. Therefore, T-cell function is analyzed by LTT as it can indicate toward T-cell activation. In this study 10 out of 15 proteins viz. Eno, Aldo, p45, TPI, PDI, elF-2, Hsp83, ADHT, and Hypo 1 and 2 showed a better proliferative response in lymphocytes of treated hamster. Further, since NO is up-regulated by IFN-γ, in the absence of commercially available cytokine reagents for hamsters, the same was estimated indirectly using NO assay. Herein, six out of above stated 10 proteins with the better lymphoproliferative response, i.e., Eno, Aldo, p45, TPI, PDI, and elF-2 have shown optimally good NO response indicating toward their being highly immunogenic.
However, among all the 15 recombinant proteins, only seven proteins viz. Eno, Aldo, p45, TPI, PDI, elF-2, and Hsp70 exhibited good T-cell reactivity in PBMCs of treated VL patients and healthy contacts endorsing the observations made in lymphocytes of treated hamster. These six proteins, when further evaluated for their cytokine response in PBMCs, generated Th1 type immune response which was characterized by high production of IFN-γ, IL-12 and TNF-α against the recombinant proteins as compared to SLD and a low production of IL-4 and IL-10 which was inferior to SLD. The LTT response, as well as the cytokine levels in both the study groups, was comparable, suggesting that the exposed population to Leishmania infection, develop a strong protective immunity as was also observed by Tripathi et al. (2006).
Conclusion
This study shows that PBMCs and lymphocytes from treated VL patients and hamsters were able to recognize six potential Th1 stimulatory recombinant Leishmania proteins with the production of Th1 type cytokines that are associated with Leishmania killing. These findings lead to the first step of selecting superior vaccine targets. Now these proteins can be further subjected to the identification of potential antigenic peptides (epitope) using various immunoinformatic tools that can be better utilized for designing poly-epitope vaccine in order to fortify host immunity against dreaded kala-azar disease. This new vaccine approach to treat VL expands host protective immune responses, making host produce beneficial T-cells that alleviate disease symptoms more quickly.
Author Contributions
SJ, NKY, KR, CDPT, AKJ, PK, RT, RKB, SD, RG, and PKK purified recombinant proteins. SJ, NKY, KR, CDPT performed the human and animal experiments. SS provides human samples. SJ and AD analyzed the data and wrote the manuscript. SJ, AS, and AD conceived the study.
Funding
This work was financially supported by Department of Biotechnology, New Delhi (BT/PR11782/MED/29/113/2009; Dated: 15.09.2009).
Conflict of Interest Statement
The authors declare that the research was conducted in the absence of any commercial or financial relationships that could be construed as a potential conflict of interest.
Acknowledgments
We express our sincere gratitude to the Director, CSIR-CDRI for providing necessary facilities for conducting these experiments and acknowledge financial assistance (in the form of fellowships) from CSIR, UGC and ICMR. This manuscript bears CDRI communication no. 9187.
References
Abass, E., Kang, C., Martinkovic, F., Semiao-Santos, S. J., Sundar, S., Walden, P., et al. (2015). Heterogeneity of Leishmania donovani parasites complicates diagnosis of visceral leishmaniasis: comparison of different serological tests in three endemic regions. PLoS ONE 10:e0116408. doi: 10.1371/journal.pone.0116408
Avilan, L., Gualdron-Lopez, M., Quinones, W., Gonzalez-Gonzalez, L., Hannaert, V., Michels, P. A., et al. (2011). Enolase: a key player in the metabolism and a probable virulence factor of trypanosomatid parasites-perspectives for its use as a therapeutic target. Enzyme Res. 2011:932549. doi: 10.4061/2011/932549
Baharia, R. K., Tandon, R., Sharma, T., Suthar, M. K., Das, S., Siddiqi, M. I., et al. (2015). Recombinant NAD-dependent SIR-2 protein of Leishmania donovani: immunobiochemical characterization as a potential vaccine against visceral leishmaniasis. PLoS Negl. Trop. Dis. 9:e0003557. doi: 10.1371/journal.pntd.0003557
Chen, N., Yuan, Z. G., Xu, M. J., Zhou, D. H., Zhang, X. X., Zhang, Y. Z., et al. (2012). Ascaris suum enolase is a potential vaccine candidate against ascariasis. Vaccine 30, 3478–3482. doi: 10.1016/j.vaccine.2012.02.075
Coler, R. N., Duthie, M. S., Hofmeyer, K. A., Guderian, J., Jayashankar, L., Vergara, J., et al. (2015). From mouse to man: safety, immunogenicity and efficacy of a candidate leishmaniasis vaccine LEISH-F3+GLA-SE. Clin. Transl. Immunol. 4:e35. doi: 10.1038/cti.2015.6
Coler, R. N., Goto, Y., Bogatzki, L., Raman, V., and Reed, S. G. (2007). Leish-111f, a recombinant polyprotein vaccine that protects against visceral Leishmaniasis by elicitation of CD4+ T cells. Infect. Immun. 75, 4648–4654. doi: 10.1128/IAI.00394-07
Croft, S. L., Sundar, S., and Fairlamb, A. H. (2006). Drug resistance in leishmaniasis. Clin. Microbiol. Rev. 19, 111–126. doi: 10.1128/CMR.19.1.111-126.2006
Desjeux, P. (2004). Leishmaniasis: current situation and new perspectives. Comp. Immunol. Microbiol. Infect. Dis. 27, 305–318. doi: 10.1016/j.cimid.2004.03.004
Ding, A. H., Nathan, C. F., and Stuehr, D. J. (1988). Release of reactive nitrogen intermediates and reactive oxygen intermediates from mouse peritoneal macrophages. Comparison of activating cytokines and evidence for independent production. J. Immunol. 141, 2407–2412.
Dube, A., Singh, N., and Sundar, S. (2005). Refractoriness to the treatment of sodium stibogluconate in Indian kala-azar field isolates persist in in vitro and in vivo experimental models. Parasitol. Res. 96, 216–223. doi: 10.1007/s00436-005-1339-1
Engwerda, C. R., and Matlashewski, G. (2015). Development of Leishmania vaccines in the era of visceral leishmaniasis elimination. Trans. R. Soc. Trop. Med. Hyg. 109, 423–424. doi: 10.1093/trstmh/trv039
Garcia-Hernandez, R., Manzano, J. I., Castanys, S., and Gamarro, F. (2012). Leishmania donovani develops resistance to drug combinations. PLoS Negl. Trop. Dis. 6:e1974. doi: 10.1371/journal.pntd.0001974
Garg, R., and Dube, A. (2006). Animal models for vaccine studies for visceral leishmaniasis. Indian J. Med. Res. 123, 439–454.
Garg, R., Gupta, S. K., Tripathi, P., Hajela, K., Sundar, S., Naik, S., et al. (2006). Leishmania donovani: identification of stimulatory soluble antigenic proteins using cured human and hamster lymphocytes for their prophylactic potential against visceral leishmaniasis. Vaccine 24, 2900–2909. doi: 10.1016/j.vaccine.2005.12.053
Garg, R., Gupta, S. K., Tripathi, P., Naik, S., Sundar, S., and Dube, A. (2005). Immunostimulatory cellular responses of cured Leishmania-infected patients and hamsters against the integral membrane proteins and non-membranous soluble proteins of a recent clinical isolate of Leishmania donovani. Clin. Exp. Immunol. 140, 149–156. doi: 10.1111/j.1365-2249.2005.02745.x
Goto, Y., Bhatia, A., Raman, V. S., Liang, H., Mohamath, R., Picone, A. F., et al. (2011). KSAC, the first defined polyprotein vaccine candidate for visceral leishmaniasis. Clin. Vaccine Immunol. 18, 1118–1124. doi: 10.1128/CVI.05024-11
Gupta, R., Kumar, V., Kushawaha, P. K., Tripathi, C. P., Joshi, S., Sahasrabuddhe, A. A., et al. (2014). Characterization of glycolytic enzymes–rAldolase and rEnolase of Leishmania donovani, identified as Th1 stimulatory proteins, for their immunogenicity and immunoprophylactic efficacies against experimental visceral leishmaniasis. PLoS ONE 9:e86073. doi: 10.1371/journal.pone.0086073
Gupta, R., Kushawaha, P. K., Tripathi, C. D., Sundar, S., and Dube, A. (2012). A novel recombinant Leishmania donovani p45, a partial coding region of methionine aminopeptidase, generates protective immunity by inducing a Th1 stimu latory response against experimental visceral leishmaniasis. Int. J. Parasitol. 42, 429–435. doi: 10.1016/j.ijpara.2012.02.013
Gupta, S. K., Sisodia, B. S., Sinha, S., Hajela, K., Naik, S., Shasany, A. K., et al. (2007). Proteomic approach for identification and characterization of novel immunostimulatory proteins from soluble antigens of Leishmania donovani promastigotes. Proteomics 7, 816–823. doi: 10.1002/pmic.200600725
Hasker, E., Singh, S. P., Malaviya, P., Picado, A., Gidwani, K., Singh, R. P., et al. (2012). Visceral leishmaniasis in rural bihar, India. Emerg. Infect. Dis. 18, 1662–1664. doi: 10.3201/eid1810.111083
Jaiswal, A. K., Khare, P., Joshi, S., Kushawaha, P. K., Sundar, S., and Dube, A. (2014). Th1 stimulatory proteins of Leishmania donovani: comparative cellular and protective responses of rTriose phosphate isomerase, rProtein disulfide isomerase and rElongation factor-2 in combination with rHSP70 against visceral leishmaniasis. PLoS ONE 9:e108556. doi: 10.1371/journal.pone.0108556
Joshi, S., Rawat, K., Yadav, N. K., Kumar, V., Siddiqi, M. I., and Dube, A. (2014). Visceral leishmaniasis: advancements in vaccine development via classical and molecular approaches. Front. Immunol. 5:380. doi: 10.3389/fimmu.2014.00380
Kamhawi, S., Oliveira, F., and Valenzuela, J. G. (2014). Using humans to make a human leishmaniasis vaccine. Sci. Transl. Med. 6:234fs218. doi: 10.1126/scitranslmed.3009118
Khare, P., Jaiswal, A. K., Tripathi, C. D., Joshi, S., Sundar, S., and Dube, A. (2014). Efficacy of Leishmania donovani trypanothione reductase, identified as a potent Th1 stimulatory protein, for its immunogenicity and prophylactic potential against experimental visceral leishmaniasis. Parasitol. Res. 113, 851–862. doi: 10.1007/s00436-013-3716-5
Kumari, S., Samant, M., Khare, P., Sundar, S., Sinha, S., and Dube, A. (2008a). Induction of Th1-type cellular responses in cured/exposed Leishmania-infected patients and hamsters against polyproteins of soluble Leishmania donovani promastigotes ranging from 89.9 to 97.1 kDa. Vaccine 26, 4813–4818. doi: 10.1016/j.vaccine.2008.06.102
Kumari, S., Samant, M., Misra, P., Khare, P., Sisodia, B., Shasany, A. K., et al. (2008b). Th1-stimulatory polyproteins of soluble Leishmania donovani promastigotes ranging from 89.9 to 97.1 kDa offers long-lasting protection against experimental visceral leishmaniasis. Vaccine 26, 5700–5711. doi: 10.1016/j.vaccine.2008.08.021
Kushawaha, P. K., Gupta, R., Sundar, S., Sahasrabuddhe, A. A., and Dube, A. (2011). Elongation factor-2, a Th1 stimulatory protein of Leishmania donovani, generates strong IFN-gamma and IL-12 response in cured Leishmania-infected patients/hamsters and protects hamsters against Leishmania challenge. J. Immunol. 187, 6417–6427. doi: 10.4049/jimmunol.1102081
Kushawaha, P. K., Gupta, R., Tripathi, C. D., Khare, P., Jaiswal, A. K., Sundar, S., et al. (2012a). Leishmania donovani triose phosphate isomerase: a potential vaccine target against visceral leishmaniasis. PLoS ONE 7:e45766. doi: 10.1371/journal.pone.0045766
Kushawaha, P. K., Gupta, R., Tripathi, C. D., Sundar, S., and Dube, A. (2012b). Evaluation of Leishmania donovani protein disulfide isomerase as a potential immunogenic protein/vaccine candidate against visceral Leishmaniasis. PLoS ONE 7:e35670. doi: 10.1371/journal.pone.0035670
Li, W., Hu, X., Zhang, X., Ge, Y., Zhao, S., Hu, Y., et al. (2011). Immunisation with the glycolytic enzyme enolase confers effective protection against Candida albicans infection in mice. Vaccine 29, 5526–5533. doi: 10.1016/j.vaccine.2011.05.030
Matsubayashi, M., Teramoto-Kimata, I., Uni, S., Lillehoj, H. S., Matsuda, H., Furuya, M., et al. (2013). Elongation factor-1alpha is a novel protein associated with host cell invasion and a potential protective antigen of Cryptosporidium parvum. J. Biol. Chem. 288, 34111–34120. doi: 10.1074/jbc.M113.515544
McNulty, S., Colaco, C. A., Blandford, L. E., Bailey, C. R., Baschieri, S., and Todryk, S. (2013). Heat-shock proteins as dendritic cell-targeting vaccines–getting warmer. Immunology 139, 407–415. doi: 10.1111/imm.12104
Mohapatra, S. (2014). Drug resistance in leishmaniasis: newer developments. Trop. Parasitol. 4, 4–9. doi: 10.4103/2229-5070.129142
Molano, I., Alonso, M. G., Miron, C., Redondo, E., Requena, J. M., Soto, M., et al. (2003). A Leishmania infantum multi-component antigenic protein mixed with live BCG confers protection to dogs experimentally infected with L. infantum. Vet. Immunol. Immunopathol. 92, 1–13. doi: 10.1016/S0165-2427(02)00315-X
Pal-Bhowmick, I., Mehta, M., Coppens, I., Sharma, S., and Jarori, G. K. (2007). Protective properties and surface localization of Plasmodium falciparum enolase. Infect. Immun. 75, 5500–5508. doi: 10.1128/IAI.00551-07
Palm, J. E., Weiland, M. E., Griffiths, W. J., Ljungstrom, I., and Svard, S. G. (2003). Identification of immunoreactive proteins during acute human giardiasis. J. Infect. Dis. 187, 1849–1859. doi: 10.1086/375356
Parody, N., Soto, M., Requena, J. M., and Alonso, C. (2004). Adjuvant guided polarization of the immune humoral response against a protective multicomponent antigenic protein (Q) from Leishmania infantum. A CpG + Q mix protects Balb/c mice from infection. Parasite Immunol. 26, 283–293. doi: 10.1111/j.0141-9838.2004.00711.x
Srivastava, P., Prajapati, V. K., Rai, M., and Sundar, S. (2011). Unusual case of resistance to amphotericin B in visceral leishmaniasis in a region in India where leishmaniasis is not endemic. J. Clin. Microbiol. 49, 3088–3091. doi: 10.1128/JCM.00173-11
Stanley, A. C., and Engwerda, C. R. (2007). Balancing immunity and pathology in visceral leishmaniasis. Immunol. Cell Biol. 85, 138–147. doi: 10.1038/sj.icb7100011
Trigo, J., Abbehusen, M., Netto, E. M., Nakatani, M., Pedral-Sampaio, G., de Jesus, R. S., et al. (2010). Treatment of canine visceral leishmaniasis by the vaccine Leish-111f+MPL-SE. Vaccine 28, 3333–3340. doi: 10.1016/j.vaccine.2010.02.089
Tripathi, P., Ray, S., Sunder, S., Dube, A., and Naik, S. (2006). Identification of Leishmania donovani antigens stimulating cellular immune responses in exposed immune individuals. Clin. Exp. Immunol. 143, 380–388. doi: 10.1111/j.1365-2249.2005.03000.x
Wang, H. L., Li, Y. Q., Yin, L. T., Meng, X. L., Guo, M., Zhang, J. H., et al. (2013). Toxoplasma gondii protein disulfide isomerase (TgPDI) is a novel vaccine candidate against toxoplasmosis. PLoS ONE 8:e70884. doi: 10.1371/journal.pone.0070884
Keywords: Leishmania donovani, recombinant Th1 stimulatory proteins, immunogenicity, cellular response, lymphocytes, PBMCs, treated Leishmania-infected hamster, treated Leishmania patients
Citation: Joshi S, Yadav NK, Rawat K, Tripathi CDP, Jaiswal AK, Khare P, Tandon R, Baharia RK, Das S, Gupta R, Kushawaha PK, Sundar S, Sahasrabuddhe AA and Dube A (2016) Comparative Analysis of Cellular Immune Responses in Treated Leishmania Patients and Hamsters against Recombinant Th1 Stimulatory Proteins of Leishmania donovani. Front. Microbiol. 7:312. doi: 10.3389/fmicb.2016.00312
Received: 15 January 2016; Accepted: 26 February 2016;
Published: 22 March 2016.
Edited by:
Thomas Dandekar, Julius Maximilian University of Würzburg, GermanyReviewed by:
Magilé De La Caridad Fonseca, Institute of Tropical Medicine Pedro Kourí, CubaAlicia Ponte-Sucre, Universidad Central de Venezuela, Venezuela
Copyright © 2016 Joshi, Yadav, Rawat, Tripathi, Jaiswal, Khare, Tandon, Baharia, Das, Gupta, Kushawaha, Sundar, Sahasrabuddhe and Dube. This is an open-access article distributed under the terms of the Creative Commons Attribution License (CC BY). The use, distribution or reproduction in other forums is permitted, provided the original author(s) or licensor are credited and that the original publication in this journal is cited, in accordance with accepted academic practice. No use, distribution or reproduction is permitted which does not comply with these terms.
*Correspondence: Anuradha Dube, YV9kdWJlQGNkcmkucmVzLmlu