- 1Department of Clinical Microbiology, Christian Medical College, Vellore, India
- 2Department of Microbiology, Molecular Genetics and Immunology, University of Kansas Medical Centre, Kansas, KS, USA
- 3Department of Haematology, Christian Medical College, Vellore, India
- 4Department of Nephrology, Christian Medical College, Vellore, India
Colistin has long been a reserve drug used for the treatment of carbapenem resistant Klebsiella pneumoniae. Carbapenem resistance in K. pneumoniae has been increasing and is as high as 44% in India. Although a reserve agent, with rise in rates of resistance to carbapenems, the usage of colistin has increased over the years leading to slow emergence of resistance. Colistin resistance is mainly mediated by the alteration in the LPS of bacterial outer membrane with the addition of L-Ara4-N and PEtN molecules. These alterations are mediated by mutations in several genes involved in lipidA modifications and most commonly mutations in mgrB gene has been reported. Recently there is emergence of plasmid mediated resistance due to mcr-1 and mcr-2 genes which poses a threat for the rapid global spread. This study aims at characterizing eight colistin resistant K. pneumoniae from bacteremia by whole genome sequencing. Eight K. pneumoniae were isolated from blood culture during 2013 and 2014 at the Department of Clinical Microbiology, Christian Medical College, India. Antimicrobial susceptibility testing was performed and minimum inhibitory concentration (MIC) was determined for colistin and polymyxin B by broth-micro dilution method. Whole genome sequencing was performed using Ion Torrent and the genome of all eight isolates was analyzed. The eight isolates were resistant to all the antimicrobials expect tigecycline. MIC of colistin and polymyxin B were ranged from 4 to 1024 μg/ml and 0.5 to 2048 μg/ml respectively. Multiple mutations were observed in the chromosomal genes involved in lipid A modifications. mcr-1 and mcr-2 gene was absent in all the isolates. The most significant were mutations in mgrB gene. Among the eight isolates, four, three and one were belonged to sequence types ST 231, ST14 and ST147 respectively. Seven isolates had blaOXA−48 like, one co-expressed blaNDM−1 and blaOXA−48 like genes leading to carbapenem resistance. Overall, multiple numbers of alterations have been observed. This includes silent mutations, point mutations, insertions and/or deletions. Mutations in mgrB gene is responsible for resistance to colistin in this study. Due to emergence of resistance to reserve drugs, there is a need for combination therapies for carbapenem resistant K. pneumoniae and colistin must be judiciously used.
Introduction
Klebsiella pneumoniae is a gram negative bacteria and is a common cause of severe community and hospital acquired infections. It readily colonizes human mucosal surfaces especially the gastrointestinal (GI) tract and oropharynx (Bagley, 1985; Dao et al., 2014; Rock et al., 2014). In immunocompromised hosts, it can invade other sites leading to severe infections (Paczosa and Mecsas, 2016). In India, carbapenems are commonly used for treatment of K. pneumoniae due to high rates of ESBL producers (Ah et al., 2014). Increasing carbapenem resistance has resulted in colistin (polymyxin E) and tigecycline being used as last resort drugs for treatment of multidrug resistant (MDR) isolates (Carmeli et al., 2010).
Polymyxins are cationic polypeptides with five different compounds, known as polymyxin A–E (Storm et al., 1977). However, only polymyxin B and polymyxin E (colistin) are used in clinical practice to treat bacterial infections. When initially introduced, polymyxins were not widely used due to their adverse effects of nephrotoxicity and neurotoxicity (Falagas and Kasiakou, 2006). However, with the rise of carbapenem resistant organisms, polymyxins have gained attention as one of the few agents available for treatment (Falagas et al., 2005). Polymyxins are positively charged antimicrobial peptide molecules, which act by biding to negatively charged phosphate groups in the lipidA moiety of lipopolysaccharides (LPS). This causes disruption and loss of cell membrane integrity, leading to cell death (Yahav et al., 2012).
Due to its rising and sometimes inappropriate use, colistin resistance is increasing. Resistance to colistin is mainly mediated by modification of the LPS moiety with the addition of positively charged L-Ara4-N and PEtN molecules (Falagas et al., 2010). This modification decreases the negative charge of the outer membrane, reducing its interaction with colistin (Velkov et al., 2013). It is mainly due to mutations in the two component regulatory systems. The most common mutations are in mgrB, phoP/phoQ, pmrA, pmrB, pmrC, and crrABC (Cheng et al., 2010; Poirel et al., 2015; Wright et al., 2015).
Recently, plasmid mediated colistin resistance named mcr-1 (plasmid Mediated Colistin Resistance) encoding for phosphoethanolamine transferase has been reported in Escherichia coli and K. pneumoniae from China (Liu et al., 2016). Following this, mcr-1.2, a variant of mcr-1, was identified in KPC producing K. pneumoniae in Italy (Di Pilato et al., 2016) followed by the mcr-2 gene reported from Belgium, This had 76% identity with the mcr-1 gene previously reported (Xavier et al., 2016). The increasing number of resistance mechanisms reported in such a short time period is alarming.
The emergence of colistin resistance is a serious cause for concern for both clinicians and patients, particularly in countries with high rates of carbapenem resistant Enterobacteriaceae such as China, India, and Greece. Hence, it is necessary to perform surveillance studies for colistin resistance organisms in Indian hospitals. In this study, we report eight colistin resistant K. pneumoniae isolated from bloodstream infections from Christian Medical College, Vellore, India. Whole genome sequencing was employed to study the mechanisms of colistin resistance such as chromosomal genes and plasmid mediated mcr-1 and mcr-2 genes.
Materials and Methods
Bacterial Strains
A total of eight clinical isolates of colistin resistant (CR) K. pneumoniae (CRKP1–CRKP8) were included in this study. These CR-K. pneumoniae were isolated in blood culture from patients admitted at Christian Medical College, Vellore, South India between 2013 and 2015. All the eight isolates were identified up-to species level as per standard biochemical tests (Versalovic et al., 2011) and by Vitek2 (bioMerieux)
Demographic and Clinical Details of Patients
These were obtained from electronic medical records available in the hospital intranet.
Antimicrobial Susceptibility Testing
The Kirby Bauer disk diffusion method was performed to ascertain antimicrobial susceptibility to commonly used agents. This included ceftazidime (30 μg), cefotaxime (30 μg), cefoxitin (30 μg), cefepime (30 μg), piperacillin/tazobactam (100/10 μg), imipenem (10 μg), meropenem (10 μg), ciprofloxacin (5 μg), gentamicin (10 μg), netilmicin (30 μg), amikacin (30 μg), polymyxin B (300 units), and tigecycline (15 μg). The susceptibility results were interpreted as per CLSI (2013, 2014, 2015) guidelines, respectively (CLSI M100S-23; CLSI M100S-24; CLSI M100S-25). Tigecycline results were interpreted according to FDA criteria (http://www.accessdata.fda.gov/drugsatfda_docs/label/2009/021821s016lbl.pdf).
The minimum inhibitory concentration (MICs) were determined by broth micro dilution method (BMD) for both polymyxin agents, colistin and polymyxin B. The results were interpreted as per EUCAST guidelines (EUCAST, 2013, 2014, 2015, respectively), (EUCAST susceptibility breakpoint v 3.0, v 4.0 and v 5.0). E. coli ATCC 25922 was used as a quality control.
Carbapenemase Detection by CarbaNP and Multiplex PCR
The CarbaNP test was performed for all isolates by the previously described method (Nordmann et al., 2012). K. pneumoniae ATCC BAA 1705 and K. pneumoniae ATCC BAA 1706 were used as positive and negative controls, respectively.
For molecular testing, all the isolates were grown overnight on blood agar and DNA was extracted using Qiagen kit as per manufacturer's protocol (Qiagen Bacterial DNA mini kit). The presence of carbapenemase genes such as blaSPM, blaIMP, blaVIM, blaNDM, blaKPC, and blaOXA−48 like genes were determined by conventional multiplex PCR as described previously (Anandan et al., 2015). The products were analyzed on 2% agarose gel stained with ethidium bromide. Respective positive controls were used for each run (Courtesy: IHMA, Inc., USA).
Molecular Characterization of Colistin Resistance
PCR and Sequencing for LPS Modifications Genes
Genes involved in LPS modification including mgrB, phoP/phoQ, and pmrA/pmrB/pmrD were amplified and sequenced as previously described (Jayol et al., 2015; Wright et al., 2015).
Whole Genome Sequencing (WGS) for CR- K. pneumoniae
WGS was performed using the Ion Torrent PGM platform using 400 bp read chemistry. Sequencing was performed as per the protocol recommended by Life Technologies. Raw reads were assembled de novo using Assembler SPAdes software v4.4.0.1 in Torrent suite server version 4.4.3. The genome was annotated using the PATRIC (Pathosystems Resource Integration Center -https://www.patricbrc.org) and RAST (Rapid Annotations using Subsystems Technology) databases. Upon annotation, antimicrobial resistance genes were found as per ARDB and CARD, respectively. These genes were further taken for mutational analysis. The whole genome sequences were deposited at GenBank under the following accession numbers: CRKP1 (MOXM00000000); CRKP2 (MIEJ00000000); CRKP3 (MPCT00000000); CRKP4 (MDZG00000000); CRKP5 (MOXL00000000); CRKP6 (MEBR00000000); CRKP7 (MOXN00000000); CRKP8 (LZYN00000000).
Molecular Typing of CR K. pneumoniae by MLST
The MLST database at the Center of Genomic Epidemiology was used to identify the sequence types (ST) of the study isolates using WGS data (https://cge.cbs.dtu.dk/services/MLST/).
eBURST
The program eBURST v 3.0 was used to identify clonal complexes.
Results
Demographic and Clinical Details of Patients
The demographic and clinical details of each patient were obtained from electronic medical records. The patients' ranged from 25 to 59 years in age. Five were male. Six patients were under the care of the hematology team, one was under the care of neurology, and one under nephrology. All but one patient was immunocompromised. K. pneumoniae sepsis was hospital acquired in all cases and the sources of infection were neutropenic sepsis (n = 4), pneumonia (n = 1), urosepsis (n = 1), intra-abdominal infection (n = 1), and line infection (n = 1). Meropenem was administered to all patients before K. pneumoniae was isolated and colistin was administered to five patients. Four patients expired within 30 days of infection and seven within 90 days of infection.
Antimicrobial Susceptibility Testing
All isolates were extremely drug resistant (XDR) as per the definition of Magiorakos et al. (2012) All isolates were resistant to cephalosporins, carbapenems, fluoroquinolones, aminoglycosides, and polymyxin B by disk diffusion but were susceptible to tigecycline. The MIC-values determined by BMD for colistin and polymyxin B ranged from 4 to 1024 and 0.5 to 2048 μg/ml, respectively. The MIC results of the eight isolates are summarized in Table 1.
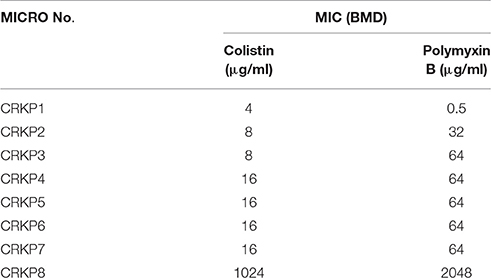
Table 1. Minimum inhibitory concentration (MIC) for colistin and polymyxin B by broth micro dilution (BMD) for K. pneumoniae.
Carbapenemase Detection
CarbaNP test was positive for all the eight isolates tested indicating the production of carbapenemases. Multiplex PCR for carbapenamase genes revealed the presence of blaOXA−48 like gene in seven isolates. One isolate co-expressed blaNDM−1 + OXA−232 genes.
Whole Genome Sequence Analysis
For whole genome sequencing analysis, PATRIC, and RAST databases have been used to analyse the annotated sequences (http://patricbrc.org/) (http://rast.nmpdr.org/). As these eight isolates were XDR strains, multiple resistance genes against various classes of antimicrobial agents have been identified. These are summarized in Table 2. For cephalosporin resistance, the most common resistance genes were blaTEM, blaSHV, and blaCTX−M−15. For carbapenem resistance, blaOXA−232, a variant of blaOXA−48 like gene was found in six of the study isolates, one isolate co-producing blaOXA−232 and blaNDM−1, while one isolate had blaOXA−181, also a variant of the blaOXA−48 like gene.
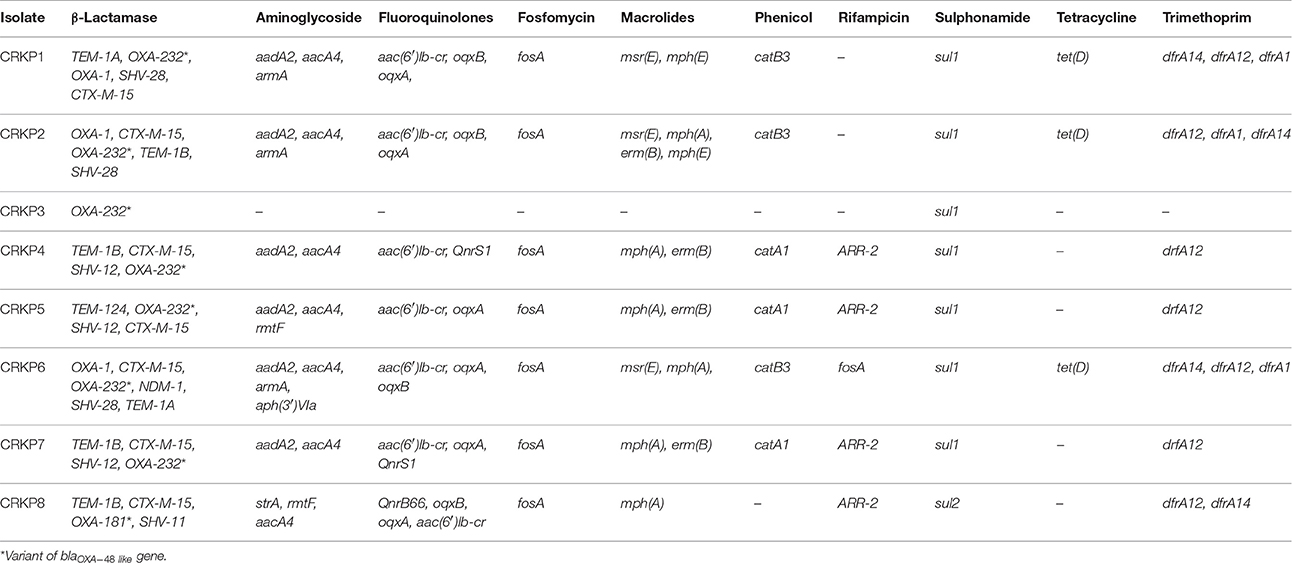
Table 2. Antimicrobial resistance genes identified against various antimicrobial agents in CR K. pneumoniae.
For colistin resistance, RAST classified 11 genes involved in lipid A modification with L-Ara4N pathway. These included ugd, arnA_DH, arnA_FT, arnB, arnC, arnT, pmrJ, pmrL, pmrD, pmrM, and pmrG. In addition, 10 genes were classified under genes involved in Lipid A modifications. These include pagP,pagL, lpxO, phoQ, phoP, pmrA, pmrB, eptA, eptB, and yijP. Of these 21 genes, a few gene sequences could not be retrieved from the annotated genomes of the study isolates from RAST/PATRIC databases and hence analysis was not done. However, for the available genes, sequences were retrieved and analyzed for mutations. Upon analysis, multiple mutations were observed in various genes. In seven isolates, there was a change from arginine to alanine at position 114 of phoP. In one isolate, there was a change from arginine to alanine in position 128. In phoQ, five isolates had a substitution of glycine for aspartic acid at position 146 and three isolates had the same substitution at position 150. The mutations in other genes such as pmrB, pmrC, phoB, arnB, arnC, arnT, arnA_FT, and pagP are listed in Table 3 and have not been reported in other studies to date. No mutations were found in pmrA, eptB, and phoR genes in any of the isolates analyzed. mcr-1 and mcr-2 genes were absent in all the study isolates.
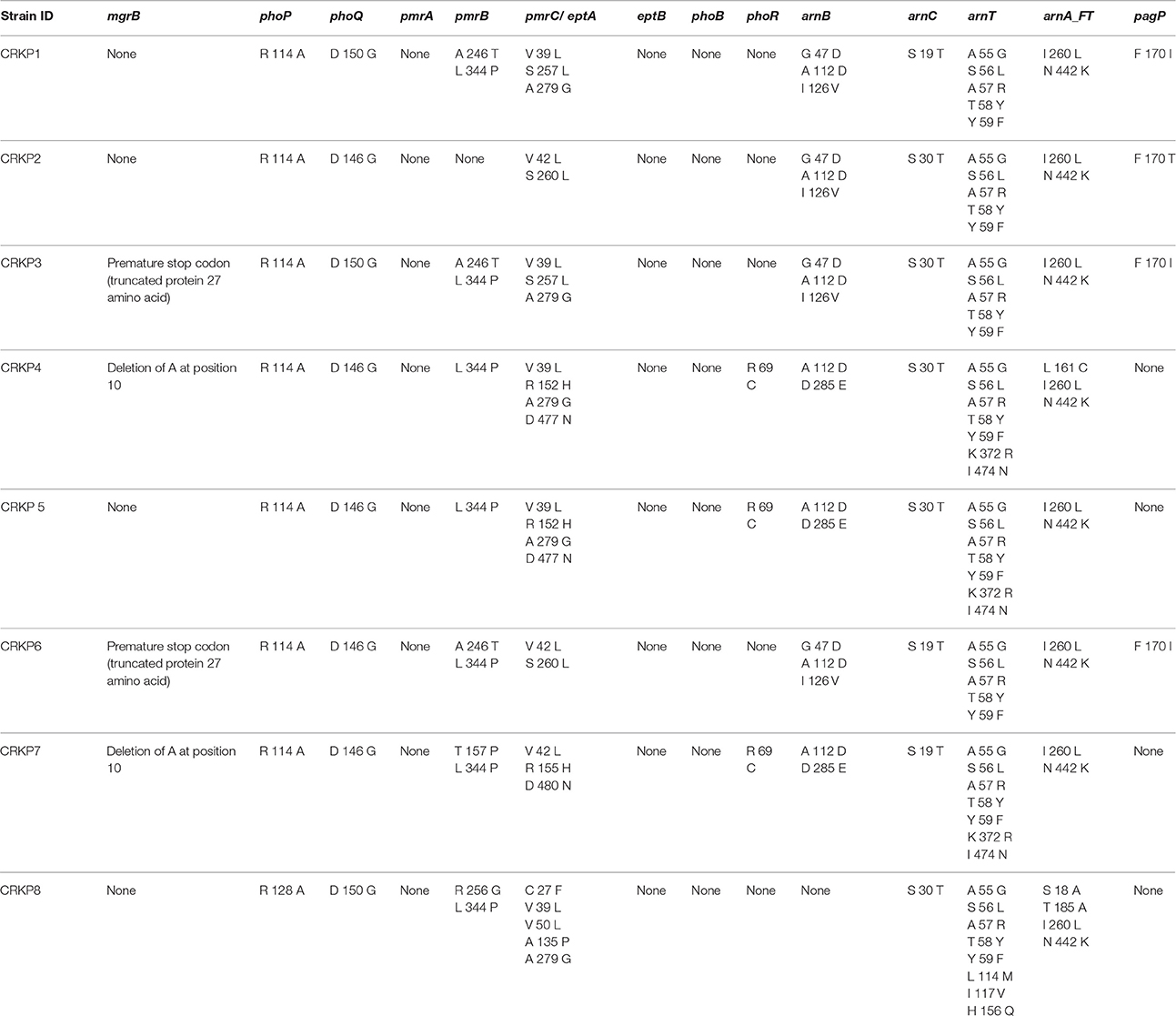
Table 3. Cumulative results of amino acid substitutions in various genes contributing to colistin resistance found upon WGS analysis of CR—K. pneumoniae.
Targeted Gene Sequencing Analysis of Mutations in Genes Involved in LPS Modifications
Of the eight isolates screened for mutations, CRKP3 and CRKP6 had a premature stop codon in mgrB gene resulting in a truncated protein of 27 amino acids. Whereas, a frame shift mutation in CRKP4 and deletion of nucleotide A at 10th position of mgrB gene in CRKP7 was noted, this could be responsible for the resistant phenotype. Three isolates, CRKP1, CRKP2, and CRKP5 did not have any mutations in the mgrB gene. Sequencing of two component systems phoP/phoQ and pmrA/pmrB and pmrD found multiple silent mutations. Since targeted sequencing results did not reveal much information, WGS data analysis was performed to further understand the additional resistance mechanisms.
Molecular Typing of CR K. pneumoniae by MLST
MLST database available at https://cge.cbs.dtu.dk/services/MLST/ by the Center of Genomic Epidemiology was used to identify the sequence type (ST) of the study isolates using WGS data. Of the eight CR-K. pneumoniae, three different sequence types were identified (ST231, ST14, and ST147). Four isolates belonged to ST231 (CRKP3, CRKP4, CRKP6, and CRKP7), three to ST14 (CRKP1, CRKP2, and CRKP5) and one to ST147 (CRKP8).
eBURST
eBURST analysis found no clustering of colistin resistant isolates into a clonal complex. The result is depicted in Figure 1.
Discussion
Antimicrobial resistance is on the rise globally. The limited treatment options available for carbapenem resistant K. pneumoniae have resulted in increasing use of colistin, despite its adverse effects. Colistin resistance is now emerging, leaving clinicians with very few options to treat these extensively drug resistant bacteria.
The main challenge in screening colistin resistance lies in determining polymyxin susceptibility (Balaji et al., 2011). Polymyxins are large cationic peptide molecules. They do not diffuse through agar well, hence disk diffusion is neither reliable nor recommended. A reliable screening test is awaited. Other susceptibility methods produce variable and discrepant results. Further, polymyxin B and colistin produce discrepant results (Hindler and Humphries, 2013; Humphries, 2015). Colistin susceptibility testing by disk diffusion has resulted in very major errors of up to 32% when compared with broth or agar dilution methods for colistin (Tan and Ng, 2006; van der Heijden et al., 2007). E-test may also produce discrepant results, hence broth microdilution is considered the reference method (Tan et al., 2007).
From this study results, the MIC was found to be two to eight fold higher for polymyxin B when compared to colistin by BMD method. Such discordant results could be due to the non-reliability of MIC testing for polymyxin B. These findings concur with previous studies where the MIC was found to be higher for polymyxin B than colistin (van der Heijden et al., 2007).
A number of studies have reported discrepant results when testing polymyxin B and colistin susceptibility by disk diffusion and MIC methods (Behera et al., 2010). Similar observations were noted in this study; all eight CR-K. pneumoniae were found to be resistant on BMD testing with variable MIC values for polymyxin B and colistin as shown in Table 1. Detection of molecular resistance mechanisms are based on preliminary screening with polymyxins. This emphasizes the need for a reliable screening test.
mgrB is the commonest chromosomal gene mutation involving modification of LPS which includes insertional inactivation causing colistin resistance. The second commonest cause is multiple mutations in the pmrAB and phoPQ, the two component systems involved in the LPS modifications.
Of the genes analyzed, mutations in mgrB with truncation to 27 amino acids concurs with previous studies (Poirel et al., 2015). The T157P mutation in pmrB has also been previously identified (Jayol et al., 2014, 2015; Olaitan et al., 2014). Multiple new mutations were identified in various genes in this study. These include mutations in phoP (R114A), phoQ (D146G), pmrB (L334P, A246T), pmrC (V39L, R152H, A279G, D477N, D480N, S260L, C27F, V50L, A235P), phoR (R69C), arnB (A112D, D285E, G47D, I126V), arnC (S30T), arnA_FT (I260L, N442K, L161C, S18A, T185A), and pagP (F170I). However, the significance of these mutations and their contribution to colistin resistance needs to be studied further.
One isolate (CRKP8) had very high MICs of 1024 μg/ml and 2048 μg/ml by BMD for colistin and polymyxin B respectively. This is a huge variation in MIC between two different methodologies.
The clonality distribution of colistin resistant K. pneumoniae is of great interest. Worldwide, sequence types (ST) associated with colistin resistant K. pneumoniae are ST258, ST512, and ST147 (Mammina et al., 2012; Cannatelli et al., 2014). However, in our study it was ST231, ST14 and ST147. Our study agrees with Jayol et al who reported ST14 K. pneumoniae producing blaOXA−48 like (Jayol et al., 2014, 2015). However, in this study, the resistance mechanisms were mgrB mediated rather than due to mutation in pmrB. One of the study isolates belonging to ST14 (CRKP6) was a blaNDM−1 producer. Isolates with mutated and/or truncated mgrB were of different sequence types. This further indicates that resistance determinants are irrespective of the sequence types.
Interestingly, isolates CRKP3 and CRKP6, both of which had a mutation in mgrB gene resulting in a truncated product, were not of the same clone. However, isolate CRKP4 and CRKP7 which had a frame shift mutation in mgrB gene, both belonged to ST231.
Conclusion
Inappropriate usage of colistin in the clinical setting should be avoided, as selection pressure may contribute to colistin resistance due to mutations. Further, use of colistin in agricultural practices should be forbidden to prevent the further spread of resistance. The global demand for colistin in agriculture is estimated to reach 16,500 tons by 2021 (QYResearch Medical Research Centre, 2015). India is also one of the leading producers of colistin for veterinary use (Liu et al., 2016). In this study, multiple mutations in the genes coding for LPS modification have been observed. These include silent mutations, point mutations, insertions, and/or deletions. Mutation profiles were observed to be divergent. This signifies the fact that clinical isolates do develop mutations for survival. Altogether, mutations in the mgrB gene were found to be the most common, followed by mutations in the other genes such as pmrB, pmrC, arnB, and arnAFT. This observation emphasizes that colistin resistance is multifactorial. However, the significant effect of these mutations observed in this study needs to be validated. Therefore, further studies should be focused on the role of these genes in pathways involving LPS modifications conferring resistance to colistin.
Ethics Statement
Ethical approval was obtained for the study from the Ethical Committee of Christian Medical College, Vellore, India.
Author Contributions
AP, CS, FI: lab methods, data analysis, manuscript writing. BV, IB: study design and manuscript writing. LN, BG, SV: study design and data collection.
Funding
Fluid Research Grant of Christian Medical College, Vellore, India.
Conflict of Interest Statement
The authors declare that the research was conducted in the absence of any commercial or financial relationships that could be construed as a potential conflict of interest.
References
Ah, Y. M., Kim, A. J., and Lee, J. Y. (2014). Colistin resistance in Klebsiella pneumoniae. Int. J. Antimicrob. Agents 44, 8–15. doi: 10.1016/j.ijantimicag.2014.02.016
Anandan, S., Damodaran, S., Gopi, R., Bakthavatchalam, Y. D., and Veeraraghavan, B. (2015). Rapid screening for carbapenem resistant organisms: current results and future approaches. J. Clin. Diagn. Res. 9, DM01. doi: 10.7860/JCDR/2015/14246.6530
Bagley, S. T. (1985). Habitat association of Klebsiella species. Infect. Control 6, 52–58. doi: 10.1017/S0195941700062603
Balaji, V., Jeremiah, S. S., and Baliga, P. R. (2011). Polymyxins: antimicrobial susceptibility concerns and therapeutic options. Indian J. Med. Microbiol. 29, 230. doi: 10.4103/0255-0857.83905
Behera, B., Mathur, P., Das, A., Kapil, A., Gupta, B., Bhoi, S., et al. (2010). Evaluation of susceptibility testing methods for polymyxin. Int. J. Infect. Dis. 14, e596–e601. doi: 10.1016/j.ijid.2009.09.001
Cannatelli, A., Di Pilato, V., Giani, T., Arena, F., Ambretti, S., Gaibani, P., et al. (2014). In vivo evolution to colistin resistance by PmrB sensor kinase mutation in KPC-producing Klebsiella pneumoniae is associated with low-dosage colistin treatment. Antimicrob. Agents Chemother. 58, 4399–4403. doi: 10.1128/AAC.02555-14
Carmeli, Y., Akova, M., Cornaglia, G., Diakos, G. L., Garau, J., Harbarth, S., et al. (2010). Controlling the spread of carbapenemase-producing gram-negatives: therapeutic approach and infection control. Clin. Microbiol. Infect. 16, 102–111. doi: 10.1111/j.1469-0691.2009.03115.x
Cheng, H. Y., Chen, Y. F., and Peng, H. L. (2010). Molecular characterization of the PhoPQ-PmrD-PmrAB mediated pathway regulating polymyxin B resistance in Klebsiella pneumoniae CG43. J. Biomed. Sci. 17, 1. doi: 10.1186/1423-0127-17-60
Clinical Laboratory Standards Institute (CLSI) (2013). Performance Standards for Antimicrobial Susceptibility Testing; Twenty Third Informational Supplement. Wayne, PA: Clinical and Laboratory Standards Institute. CLSI document M100-S23.
Clinical Laboratory Standards Institute (CLSI) (2014). Performance Standards for Antimicrobial Susceptibility Testing; Twenty Fourth Informational Supplement. Wayne, PA: Clinical and Laboratory Standards Institute. CLSI document M100-S24.
Clinical Laboratory Standards Institute (CLSI) (2015). Performance Standards for Antimicrobial Susceptibility testing; Twenty Fifth Informational Supplement. Wayne, PA: Clinical and Laboratory Standards Institute. CLSI document M100-S25.
Dao, T. T., Liebenthal, D., Tran, T. K., Vu, B. N. T., Nguyen, D. N. T., Tran, H. K. T., et al. (2014). Klebsiella pneumoniae oropharyngeal carriage in rural and urban Vietnam and the effect of alcohol consumption. PLoS ONE 9:e91999. doi: 10.1371/journal.pone.0091999
Di Pilato, V., Arena, F., Tascini, C., Cannatelli, A., De Angelis, L. H., Fortunato, S., et al. (2016). MCR-1.2, a New mcr variant carried on a transferable plasmid from a colistin-resistant KPC carbapenemase-producing Klebsiella pneumoniae strain of sequence type 512. Antimicrob. Agents Chemother. 60, 5612–5615. doi: 10.1128/AAC.01075-16
Falagas, M. E., and Kasiakou, S. K. (2006). Toxicity of polymyxins: a systematic review of the evidence from old and recent studies. Crit. Care 10, 1. doi: 10.1186/cc3995
Falagas, M. E., Kasiakou, S. K., and Saravolatz, L. D. (2005). Colistin: the revival of polymyxins for the management of multidrug-resistant gram-negative bacterial infections. Clin. Infect. Dis. 40, 1333–1341. doi: 10.1086/429323
Falagas, M. E., Rafailidis, P. I., and Matthaiou, D. K. (2010). Resistance to polymyxins: mechanisms, frequency and treatment options. Drug Resist. Updat. 13, 132–138. doi: 10.1016/j.drup.2010.05.002
Hindler, J. A., and Humphries, R. M. (2013). Colistin MIC variability by method for contemporary clinical isolates of multidrug-resistant Gram-negative bacilli. J. Clin. Microbiol. 51, 1678–1684. doi: 10.1128/JCM.03385-12
Humphries, R. M. (2015). Susceptibility testing of the polymyxins: where are we now? Pharmacotherapy 35, 22–27. doi: 10.1002/phar.1505
Jayol, A., Poirel, L., Brink, A., Villegas, M. V., Yilmaz, M., and Nordmann, P. (2014). Resistance to colistin associated with a single amino acid change in protein PmrB among Klebsiella pneumoniae isolates of worldwide origin. Antimicrob. Agents Chemother. 58, 4762–4766. doi: 10.1128/AAC.00084-14
Jayol, A., Poirel, L., Villegas, M. V., and Nordmann, P. (2015). Modulation of mgrB gene expression as a source of colistin resistance in Klebsiella oxytoca. Int. J. Antimicrob. Agents 46, 108–110. doi: 10.1016/j.ijantimicag.2015.02.015
Liu, Y. Y., Wang, Y., Walsh, T. R., Yi, L. X., Zhang, R., Spencer, J., et al. (2016). Emergence of plasmid-mediated colistin resistance mechanism MCR-1 in animals and human beings in China: a microbiological and molecular biological study. Lancet Infect. Dis. 16, 161–168. doi: 10.1016/S1473-3099(15)00424-7
Magiorakos, A. P., Srinivasan, A., Carey, R. B., Carmeli, Y., Falagas, M. E., Giske, C. G., et al. (2012). Multidrug-resistant, extensively drug-resistant and pandrug-resistant bacteria: an international expert proposal for interim standard definitions for acquired resistance. Clin. Microbiol. Infect. 18, 268–281. doi: 10.1111/j.1469-0691.2011.03570.x
Mammina, C., Bonura, C., Di Bernardo, F., Aleo, A., Fasciana, T., Sodano, C., et al. (2012). Ongoing spread of colistin-resistant Klebsiella pneumoniae in different wards of an acute general hospital, Italy, June to December 2011. Euro Surveill. 17:20248.
Nordmann, P., Poirel, L., and Dortet, L. (2012). Rapid detection of carbapenemase-producing Enterobacteriaceae. Emerging Infect. Dis. 18, 1503–1507. doi: 10.3201/eid1809.120355
Olaitan, A. O., Diene, S. M., Kempf, M., Berrazeg, M., Bakour, S., Gupta, S. K., et al. (2014). Worldwide emergence of colistin resistance in Klebsiella pneumoniae from healthy humans and patients in Lao PDR, Thailand, Israel, Nigeria and France owing to inactivation of the PhoP/PhoQ regulator mgrB: an epidemiological and molecular study. Int. J. Antimicrob. Agents 44, 500–507. doi: 10.1016/j.ijantimicag.2014.07.020
Paczosa, M. K., and Mecsas, J. (2016). Klebsiella pneumoniae: going on the offense with a strong defense. Microbiol. Mol. Biol. Rev. 80, 629–661. doi: 10.1128/MMBR.00078-15
Poirel, L., Jayol, A., Bontron, S., Villegas, M. V., Ozdamar, M., Türkoglu, S., et al. (2015). The mgrB gene as a key target for acquired resistance to colistin in Klebsiella pneumoniae. J. Antimicrob. Chemother. 70, 75–80. doi: 10.1093/jac/dku323
QYResearch Medical Research Centre (2015). The Global Polymyxin Industry Report 2015. Available online at: http://www.qyresearch.com
Rock, C., Thom, K. A., Masnick, M., Johnson, J. K., Harris, A. D., and Morgan, D. J. (2014). Frequency of Klebsiella pneumoniae Carbapenemase (KPC)–producing and Non-KPC-producing klebsiella species contamination of healthcare workers and the environment. Infect. Control Hosp. Epidemiol. 35, 426–429. doi: 10.1086/675598
Storm, D. R., Rosenthal, K. S., and Swanson, P. E. (1977). Polymyxin and related peptide antibiotics. Annu. Rev. Biochem. 46, 723–763. doi: 10.1146/annurev.bi.46.070177.003451
Tan, T. Y., and Ng, L. S. Y. (2006). Comparison of three standardized disc susceptibility testing methods for colistin. J. Antimicrob. Chemother. 58, 864–867. doi: 10.1093/jac/dkl330
Tan, T. Y., Ng, L. S. Y., Tan, E., and Huang, G. (2007). In vitro effect of minocycline and colistin combinations on imipenem-resistant Acinetobacter baumannii clinical isolates. J. Antimicrob. Chemother. 60, 421–423. doi: 10.1093/jac/dkm178
The European Committee on Antimicrobial Susceptibility Testing (EUCAST). (2013). Breakpoint Tables for Interpretation of MICs and Zone Diameters. Version 3.0. Available online at: http://www.eucast.org
The European Committee on Antimicrobial Susceptibility Testing (EUCAST) (2014). Breakpoint Tables for Interpretation of MICs and Zone Diameters. Version 4.0. Available online at: http://www.eucast.org
The European Committee on Antimicrobial Susceptibility Testing (EUCAST) (2015). Breakpoint Tables for Interpretation of MICs and Zone Diameters. Version 5.0. Available online at: http://www.eucast.org
van der Heijden, I. M., Levin, A. S., De Pedri, E. H., Fung, L., Rossi, F., Duboc, G., et al. (2007). Comparison of disc diffusion, Etest and broth microdilution for testing susceptibility of carbapenem-resistant P. aeruginosa to polymyxins. Ann. Clin. Microbiol. Antimicrob. 6:1. doi: 10.1186/1476-0711-6-1
Versalovic, J., Carroll, K. C., Funke, G., Jorgensen, J. H., Landry, M. L., and Warnock, D. W. (2011). Manual of Clinical Microbiology, 10th Edn. Washington, DC: American Society for Microbiology.
Velkov, T., Deris, Z. Z., Huang, J. X., Azad, M. A. K., Butler, M., Sivanesan, S., et al. (2013). Surface changes and polymyxin interactions with a resistant strain of Klebsiella pneumoniae. Innate Immun. 20, 350–363. doi: 10.1177/1753425913493337
Wright, M. S., Suzuki, Y., Jones, M. B., Marshall, S. H., Rudin, S. D., Van Duin, D., et al. (2015). Genomic and transcriptomic analyses of colistin-resistant clinical isolates of Klebsiella pneumoniae reveal multiple pathways of resistance. Antimicrob. Agents Chemother. 59, 536–543. doi: 10.1128/AAC.04037-14
Xavier, B. B., Lammens, C., Ruhal, R., Kumar-Singh, S., Butaye, P., Goossens, H., et al. (2016). Identification of a novel plasmid-mediated colistin-resistance gene, mcr-2, in Escherichia coli, Belgium. Euro Surveill. 21:30280. doi: 10.2807/1560-7917.ES.2016.21.27.30280
Keywords: colistin, polymyxin B, resistance, Klebsiella pneumoniae, whole genome sequencing
Citation: Pragasam AK, Shankar C, Veeraraghavan B, Biswas I, Nabarro LEB, Inbanathan FY, George B and Verghese S (2017) Molecular Mechanisms of Colistin Resistance in Klebsiella pneumoniae Causing Bacteremia from India—A First Report. Front. Microbiol. 7:2135. doi: 10.3389/fmicb.2016.02135
Received: 11 October 2016; Accepted: 19 December 2016;
Published: 09 January 2017.
Edited by:
Axel Cloeckaert, French National Institute for Agricultural Research (INRA), FranceReviewed by:
Séamus Fanning, University College Dublin, IrelandJuan Wang, University College Dublin, Ireland
Raffaele Zarrilli, University of Naples Federico II, Italy
Copyright © 2017 Pragasam, Shankar, Veeraraghavan, Biswas, Nabarro, Inbanathan, George and Verghese. This is an open-access article distributed under the terms of the Creative Commons Attribution License (CC BY). The use, distribution or reproduction in other forums is permitted, provided the original author(s) or licensor are credited and that the original publication in this journal is cited, in accordance with accepted academic practice. No use, distribution or reproduction is permitted which does not comply with these terms.
*Correspondence: Balaji Veeraraghavan, dmJhbGFqaUBjbWN2ZWxsb3JlLmFjLmlu