- 1Laboratorio de Diagnóstico y Biotecnología, ADL Diagnostic Chile SpA, Puerto Montt, Chile
- 2Escuela de Bioquímica, Facultad de Ciencias, Universidad Austral de Chile, Valdivia, Chile
Antibiotic resistance is a major public health concern due to its association with the loss of efficacy of antimicrobial therapies. Horizontal transfer events may play a significant role in the dissemination of resistant bacterial phenotypes, being mobilizable plasmids a well-known mechanism. In this study, we aimed to gain insights into the genetics underlying the development of antibiotic resistance by Piscirickettsia salmonis isolates, a bacterial fish pathogen and causative agent of salmonid piscirickettsiosis, and the main target of antibiotics used in Chilean salmon farming. We provide experimental evidence that the plasmid p3PS10, which harbors multidrug resistance genes for chloramphenicol (cat2), tetracyclines [tet(31)], aminoglycosides (sat1 and aadA1), and sulfonamides (sul2), is carried by a group of P. salmonis isolates exhibiting a markedly reduced susceptibility to oxytetracycline in vitro (128–256 μg/mL of minimal inhibitory concentration, MIC). Antibiotic susceptibility analysis extended to those antibiotics showed that MIC of chloramphenicol, streptomycin, and sulfamethoxazole/trimethoprim were high, but the MIC of florfenicol remained at the wild-type level. By means of molecular cloning, we demonstrate that those genes encoding putative resistance markers are indeed functional. Interestingly, mating assays clearly show that p3PS10 is able to be transferred into and replicate in different hosts, thereby conferring phenotypes similar to those found in the original host. According to epidemiological data, this strain is distributed across aquaculture settings in southern Chile and is likely to be responsible for oxytetracycline treatment failures. This work demonstrates that P. salmonis is more versatile than it was thought, capable of horizontally transferring DNA, and probably playing a role as a vector of resistance traits among the seawater bacterial population. However, the low transmission frequency of p3PS10 suggests a negligible chance of resistance markers being spread to human pathogens.
Introduction
Mammal husbandry and aquaculture are human activities that demand antimicrobials at a large scale, not only as a veterinary tool for disease management, but also to meet animal welfare directives. The environment surrounding these production settings, especially sediments, contains residues of antibiotics, yielding favorable conditions for the selection and emergence of resistant types. In this regard, it has been suggested that the environment plays an important role as a reservoir of resistant bacteria and genes that might transform susceptible cells into resistant pathogenic bacteria (Berendonk et al., 2015). The salmon industry in Chile is threatened by this situation. Several studies have accounted for the presence of environmental non-wild-type bacterial strains and resistance markers near Chilean marine aquacultures (Miranda and Zemelman, 2002; Miranda et al., 2003; Miranda and Rojas, 2007; Buschmann et al., 2012; Shah et al., 2014). Therefore, it has been hypothesized that aquaculture settings may serve as a source of antibiotic resistance genes (ARG), thereby enhancing the likelihood of growing resistance to antibiotics in human and animal pathogens (Cabello et al., 2016).
Piscirickettsiosis, also named salmonid rickettsial septicemia or SRS, is a severe bacterial disease affecting reared salmonids worldwide. The incidence of the disease is particularly high in southern Chile, where it is triggered by notably virulent strains of Piscirickettsia salmonis. In this country, economic losses attributed to SRS have been estimated at more than half a billion dollars annually (Maisey et al., 2016). P. salmonis is a Gram-negative, facultative intracellular pathogen that has shown remarkable resilience to be controlled by means of conventional strategies (Rozas and Enríquez, 2014). Attempts to limit outbreaks and pathogen dissemination with antimicrobials have probably led to the emergence of isolates with reduced susceptibility to different therapeutic agents (Henríquez et al., 2015). This issue represents an important concern since only a few active principles are authorized for SRS treatments, with florfenicol (FFC) and oxytetracycline (OTC) being the main drugs used (SERNAPESCA, 2016).
Thus far, it is known that the P. salmonis genome encompasses a chromosome of about 3.2 Mb and at least three plasmids of medium size (Pulgar et al., 2015). Comparative studies have shown that P. salmonis essential genes are encoded by the chromosome, including those necessary for virulence (Bravo and Martinez, 2016). However, accessory genes contained in additional replicons such as plasmids carried by P. salmonis field isolates have not yet been studied in detail. As recently reported, our laboratory accomplished the sequencing of P. salmonis AY3800B (Bohle et al., 2017). This isolate is representative of a genovariant that displays a phenotype associated with high in vitro minimal inhibitory concentrations (MIC) of OTC (128–256 μg/mL). Such high in vitro OTC MIC levels will probably lead to treatment failures as these greatly exceed the maximum plasma levels achieved when salmonids are medicated with OTC (Elema et al., 1996). During sequence analysis, a plasmid designated as p3PS10 showed to be unique to AY3800B. Interestingly, this plasmid encodes several antibiotic resistance markers, along with a cluster of type 4 secretion system (T4SS) genes probably related to the ability of self-transference. In this study, we address the functional characterization of the first episome isolated from P. salmonis and discuss the implications of its potential transmissibility to other bacteria.
Materials and Methods
Bacterial Strains, Growth Conditions and Nucleic Acid Purification
Bacterial strains and plasmids used in this study are listed in Table 1. Escherichia coli strains were grown on standard trypticase soy broth (TSB, BD) at 37°C. For P. salmonis growth, plates filled with nutrient-rich medium ADL-PSA were left for 5 days at 18°C (Henríquez et al., 2015). Bacteria were subsequently used for genomic DNA purification with the GeneJet Genomic DNA Purification Kit according to the manufacturer's instructions (Thermo Fisher Scientific). DNA concentration and quality were assessed by means of a fluorometric method (Qubit, Thermo Fisher Scientific) and agarose gel electrophoresis, respectively. Plasmid miniprep purifications were carried out using a commercial kit (Quiaprep, Qiagen). To collect enough material for restriction digestion analysis, a midiprep was subsequently done (Heilig et al., 2001). Plasmids precipitated with isopropanol and pelleted by centrifugation were finally purified with a commercial kit (GeneJet). Internal procedure PGSe-03 “Management of biological and toxic waste,” which is in compliance with the Chilean directive D.S. 148 “Sanitary Regulation for Hazardous Wastes Management,” was followed for the management of biological residues.
PCR Amplification, Molecular Cloning and Restriction Analysis
Cloning of putative resistance markers was done using the PCR2.1 TOPO TA Cloning Kit (Invitrogen). PCR fragments comprising putative native promoter and coding sequences (CDS) were amplified with primers listed in Table 2. The PCR mix contained 2.5 μL of buffer, 1.0 μL of 50 mM MgCl2, 0.2 μM of each primer and 1 U of Taq Platinum in a final volume of 25 μL. PCR conditions were as follows: initial denaturation at 95°C for 5 min, followed by 30 cycles of denaturation at 95°C for 15 s, annealing at 60°C for 15 s, extension at 72°C for 15 s, with a final extension at 72°C for 5 min. Primers p3PS10_F and R and conditions similar to these described above were used to verify the presence of p3PS10 in selected isolates of our P. salmonis strain collection. For restriction analysis, 0.5 μg of plasmid DNA were digested with EcoRI or BamHI for 1 h at 37°C. Samples were separated by 1% agarose gel electrophoresis at 110 V for 30 min.
Plasmid Copy Number Determination
qPCR-based parameters were applied for the calculation of p3PS10 plasmid copy numbers (PCN; Skulj et al., 2008). Briefly, aliquots of broth cultures of AY3800B, E. coli p3PS10, and E. coli pOTC were each collected after 24, 48, 72, 96, and 120 h of cultivation at 18°C for P. salmonis or 37°C for E. coli. Bacterial disruption was accomplished by heating the samples to 95°C for 10 min in PCR water. qPCR were done using the primer pairs tetR_F and R, BC_F and R, and the corresponding probes tetR_P and BC_P. For E. coli strains, primers and probe targeting the rpoD gene as a single-copy chromosomal marker were used. PCR conditions were those recommended for TaqMan Gene Expression Master Mix (Applied Biosystems): a holding stage at 50°C for 2 min, enzyme activation at 95°C for 10 min, followed by 40 cycles of 95°C for 15 s and 60°C for 1 min. Calibration curves were obtained using log10 dilutions of ultramers tetR_U, rpoD_U and BC_U (Table 2). Efficiencies of PCR reactions were taken into account for PCN calculations derived from plasmid/chromosomal quotients as described by Skulj and colleagues.
Whole-Genome Sequencing and Annotation
The genomes of OTC-resistant AY3864B, AY6297B, and AY6532B P. salmonis LF-89-like isolates were sequenced using Pacific Bioscience (PacBio) chemistry. For AY3864B, Illumina technology (HiSeq 2000 platform, RRID:SCR_010233) was utilized additionally. Libraries were prepared using the kits recommended by the manufacturers: DNA Polymerase Binding Kit P6 v2 for PacBio and Nextera for Illumina. Sequencing was performed at Macrogen Inc., Seoul, South Korea. Long reads were de novo assembled with SMRT Analysis version 2.3.0.1 (PacBio, RRID:SCR_002942) to yield the full sequence of each replicon. Subsequently, read correction was done by mapping Illumina paired-end short reads against the PacBio assembly with CLC Genomic Workbench v6.5 software (CLC bio, RRID:SCR_011853). Annotation was accomplished with the NCBI Prokaryotic Genome Annotation Pipeline using the best-placed reference protein set as the annotation method implemented in GeneMarkS+ revision 3.0 software (RRID:SCR_011930). Sequencing statistics and accession numbers for the sequences deposited in GenBank are found in Supplementary Table 1.
Sequence Analysis
Comparative analyses were carried out with CLC software after the p3PS10 sequence was retrieved from GenBank under the accession number CP013819. Standard algorithms BLASTN and BLASTX at NCBI were also used for sequence analyses. Refinement of antibiotic resistance marker prediction was done with tools to be encountered in the Comprehensive Antibiotic Research Database, CARD1 (McArthur et al., 2013). This analysis was extended to the entire genome of AY3800B and the reference LF-89 strains (Pulgar et al., 2015; Bohle et al., 2017). Primer design was done with the Primer3 webtool2
Antibiotic Susceptibility Profiling
Due to the lack of standardized procedures for the determination of MIC for aquatic microorganisms like P. salmonis, we carried out a protocol previously established in our laboratory to this end (Henríquez et al., 2015). This assay relies on the use of PSB medium at 18°C, which supports the growth of P. salmonis. This medium is equivalent to the one recommended by the Clinical Laboratory Standards Institute (CLSI) to perform MIC assays for P. salmonis (Yañez et al., 2012). Stock solutions of chloramphenicol (CFC), FFC, OTC, streptomycin (STR), and sulfamethoxazole/trimethoprim 19:1 (SXT) (all purchased from Sigma-Aldrich) were prepared following the procedure described in the guidelines (CLSI, 2014a). Solvents for the dilution of antibiotics corresponded to those described in the CLSI document VET03/VET04_S2 (CLSI, 2014b). 96-well microplates were filled with twofold serial dilutions of 0.06–1,024 μg/mL of each antibiotic, then inoculated with approximately 5 × 105 colony forming units/well. MIC were determined with a microplate reader after 96–120 h, considering the lowest concentration of antibiotics that impeded bacterial growth. For the assessment of MIC for E. coli, Mueller-Hinton broth with cation-adjusted medium (BD) at 22°C was used along with E. coli ATCC 25922 as control strain.
Mating Assays
Donors P. salmonis AY3800B and p3PS10-lacking P. salmonis (PM15972A1, PM32597B1, PM63907), as well as E. coli TOP10 recipient strains were grown to the early exponential phase of their respective growth curves. Aliquots of 1.0 mL (OD600 = 1.0) were mixed, centrifuged at 5,000 rpm for 5 min, and resuspended in 0.1 mL of saline before being spread onto a non-selective ADL-PSA plate in the absence of antibiotic. Plates were incubated at 18°C for 24 h. Bacteria were collected by scraping plates and were transferred to 1.0 mL of saline solution, spinned down for resuspension in 0.1 mL of saline and finally spread onto ADL-PSA or TSA plates under selective conditions depending on the recipient. P. salmonis transconjugants were recovered after 7 days at 18 or 22°C, and E. coli transconjugants after 24 h at 22°C. In the case of P. salmonis mating, transconjugants were selected on PSA agar plates plus OTC (50 μg/mL), in some instances after their additional supplementation with oxolinic acid (5 μg/mL). In all experiments performed, donor and recipient cells streaked on plates selective for transconjugants were used as controls. Transconjugant genotypes were confirmed by PCR. Transfer frequencies were measured as the ratio of transconjugants to recipient cells assuming similar numbers of donor and recipient cells.
Results
p3PS10 Is a Multidrug Resistance, IncP-Related, Low-Copy-Number Plasmid
Plasmid p3PS10 is predicted to encode 52 CDS, which are listed in Table 3. According to their hypothetical functions, the entire sequence can be split into three sections: replication/maintenance, transmissibility and antibiotic resistance determinants (Figure 1A). Interestingly, a pair of tet(31) tetracycline (TET) resistance genes encoding a transcriptional regulator and an efflux pump protein were found, resembling a canonical array for TET-resistance cassettes (Thaker et al., 2009). In close proximity to the tet element we found a gene encoding dihydropteroate synthase (sul2), an enzyme associated with reduced susceptibility to sulfonamides, and an acetyltransferase encoded by cat2, which likely confers resistance to CFC (Sköld, 2000; Schwarz et al., 2004). Moreover, integron-associated acetyltransferase sat1, along with an adenylyltransferase (aadA1) related to aminoglycoside resistance were identified (Ramírez et al., 2010). All these CDS were detected within an 11-kb locus (Figure 1B). This region has a complex organization including integron and transposon arrangements. Restriction digestion of p3PS10 with EcoRI or BamHI yielded fragments of 6.2 and 2.4 kb, suggesting that the size of the antibiotic resistance locus was accurately estimated from the sequence assembly (Figure 1C). Comparative analysis performed with CARD matched with all the mentioned markers, but also identified five additional chromosomal targets for CFC resistance in sequences of both the wild-type LF-89 and AY3800B strains (Supplementary Table 2).
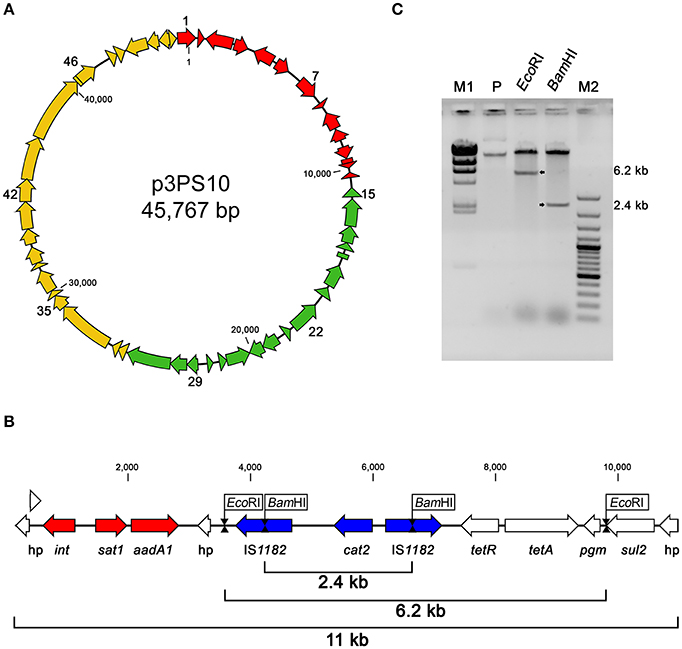
Figure 1. (A) Genetic organization of plasmid p3PS10. In red, CDS for putative antibiotic resistance markers. Green arrows represent CDS putatively involved in replication/maintenance. Yellow arrows indicate CDS related to plasmid mobilization. More details on the annotation are found in Table 3. (B) Detailed organization of the 11-kb multidrug resistance locus showing integron (red) and transposon (blue) structures. (C) Restriction analysis of p3PS10 (inverted colors). Fragments released after BamHI and EcoRI digestion are shown in the corresponding lanes (arrows), while the P lane shows the linear and supercoiled forms present in the purified material. M1, Lambda DNA/HindIII ladder; M2, GeneRuler 100 bp Plus DNA ladder (both from Thermo Fisher Scientific).
Conventionally, the determination of the plasmid incompatibility (Inc) group is based on the amino acid sequence of the replication protein Rep, a key component of plasmid replication systems (Shintani et al., 2015). Comparative analyses of the rep sequence of p3PS10 (WP_012850373) showed a perfect match with the corresponding rep gene of pEIB202, a plasmid that has been classified as a member of the IncP group (Wang et al., 2009). Since components of the partition system of p3PS10 are also related to the IncP group (Siddique and Figurski, 2002), we postulate that p3PS10 belongs to this same Inc group.
Concerning the PCN of p3PS10, we predict the value to be low and rest our appraisal on the yield obtained by midipreps (Supplementary Figure 1). Indeed, qPCR-based calculations indicated an average PCN of 3-5 in P. salmonis cells (Figure 2). This low PCN was also observed in the heterologous host E. coli p3PS10, but not in the control strain E. coli pOTC, which carries a high-copy-number pCR2.1 derivative. In this strain, PCN of pOTC exceeded 1,000, as can be expected for this type of plasmid (Invitrogen). Strikingly, PCN of p3PS10 remained constant even after 120 h (~12 generations) of culture in the absence of selective pressure, irrespective of the host. The same observation was made after 60 generations of cultivation (not shown). In contrast, PCN of pOTC started to decline after 48 h in medium without antibiotic.
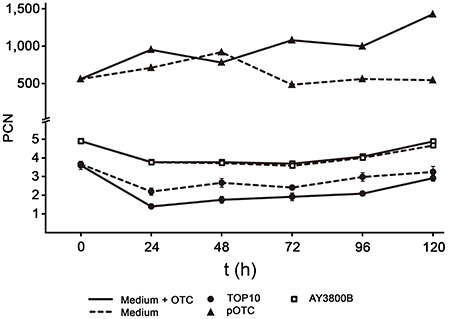
Figure 2. PCN for p3PS10 in AY3800B (unfilled squares) and E. coli TOP10 (black circles) host strains cultured in PSB or TSB medium supplemented (continuous line) or not (dashed line) with 50 μg/mL of OTC, respectively. E. coli pOTC, which is carrying a pCR2.1 derivative, is shown as control (black triangles).
The Plasmid Is Able to be Transferred Into and Replicate in Homo- and Heterologous Hosts
Bioinformatic analyses disclosed that p3PS10 encodes a conjugative T4SS gene cluster, which may permit its transmission to different hosts. This possibility was tested in mating assays. These experiments revealed that the donor strain AY3800B is able to transfer the plasmid into homologous hosts. In fact, our results show that the plasmid was mobilized between P. salmonis isolates displaying different antibiotic susceptibility patterns (Table 4). Remarkably, p3PS10 was able to replicate within an EM-90-like isolate, representative of a genogroup that has only shown wild-type susceptibility for the major classes of antibiotics utilized for SRS treatment (Saavedra et al., 2016). We calculated the frequencies of plasmid transference and confirmed that such events are rare when donor and recipient derive from different genetic backgrounds, e.g., when LF-89-like AY3800B was paired with EM-90-like PM15972A1. In contrast, transfer frequencies were one or even two orders of magnitude higher for mating pairs with similar genetic backgrounds: in AY3800B-PM63907 and AY3800B-PM32597B1 mating assays, donors and recipients belonged to the LF-89 genogroup (Otterlei et al., 2016). The plasmid was also transferred to the heterologous recipient E. coli. In this species, the formation of transconjugants occurred at a frequency similar to that observed in P. salmonis mating pairs with the LF-89 background. Antibiotic-resistant colonies were considered as true transconjugants since no spontaneous mutants with reduced susceptibility to either CFC, OTC, SXT, or STR were detected in recipient backgrounds.
Antibiotic Resistance Genes Carried by the Plasmid Are Functional
Based on our results of sequence analyses and the detection of multiple resistance traits, we decided to characterize the susceptibility of p3PS10-carrying P. salmonis AY3800B to OTC, FFC, CFC, STR, and SXT by means of MIC assays (Table 5). The comparison of MIC values for AY3800B with those obtained for the wild-type P. salmonis LF-89 reference strain showed that the AY3800B strain was indeed less susceptible to OTC, CFC, and STR, while MIC for SXT were high in both strains. Interestingly, the E. coli TOP10 derivative resulting from the transference of p3PS10 exhibited MIC values comparable to those measured for the donor strain AY3800B, which indicates the functionality of resistance markers carried by the plasmid. To confirm this hypothesis, resistance markers for OTC and CFC were cloned into E. coli TOP10. For both antibiotics tested, the phenotypic traits of the modified strains were consistent with the bioinformatically predicted function of the corresponding CDS found in p3PS10. In fact, MIC of OTC and CFC were 1,024 and 512 μg/mL in E. coli pOTC and E. coli pCFC, respectively, which are values similar to those obtained in the E. coli p3PS10 strain. The fact that E. coli pCFC did not lose susceptibility to FFC, a halogenated CFC derivative, after the transfer of p3PS10, suggests that the reduced susceptibility to CFC is mediated by an acetyltransferase selective for this drug. Due to the intrinsically high in vitro MIC of STR for E. coli TOP10, we chose to clone the predicted resistance marker for STR into the STR-susceptible E. coli DH5α. This way, we were able to show the functionality of this marker, too. With regards to susceptibility to SXT, P. salmonis isolates proved to be resistant to this compound irrespective of the presence of p3PS10. Nevertheless, cloning of sulfonamide resistance determinant sul2 into E. coli TOP10 yielded E. coli pSUL2, which displayed a significantly decreased susceptibility to SXT.
p3PS10 Might Have Been Acquired in a Horizontal Gene Transfer Event
The sequencing of P. salmonis AY3864B and AY6297B showed that both isolates harbor an identical p3PS10 plasmid that has been given different names during automatic annotation (p3PS11 and p4PS8, respectively), whereas P. salmonis AY6532B carries p4PS9, a smaller plasmid encompassing 20.4 kb and lacking transfer genes, sulfonamide and TET resistance markers (Supplementary Figure 2, Supplementary Table 3). BLASTN analysis disclosed that 35 kb of the backbone of p3PS10 matched with an identity of more than 99% to the sequence of the 43.7-kb plasmid pEIB202 found in the Gram-negative bacterium Edwarsiella tarda strain EIB202, the causative agent of the fish disease edwardsiellosis (Wang et al., 2009). Both p3PS10 and pEIB202 show similar patterns of genetic organization and comprise sequences encoding multidrug resistance markers, genes required for replication, and a set of T4SS genes. Nevertheless, the 11-kb locus of p3PS10 that harbors resistance genes clearly distinguishes this plasmid from pEIB202. The sequence of this locus showed to be partially homologous to a segment of 3.5 kb found in the 48-kb plasmid pRAS2 (accession number AJ250203). pRAS2 was isolated from Aeromonas salmonicida subsp. salmonicida 1682/92, and constitutes an episome conferring STR, sulfonamide, and TET resistances (L'Abee-Lund and Sorum, 2000).
To study the similarity between p3PS10 and known plasmids at the level of amino acid sequences, BLASTX searches were carried out using the sequence of the 11-kb locus of p3PS10. We could identify several components conserved across different bacterial species, e.g., those genes encoding a class 2 integron-associated integrase, aminoglycoside resistance proteins, and a pair of IS1182 transposases flanking the cat2 gene (Figure 1B). The class 2 integron sequence conferred resistance to STR and was almost identical to the one detected in the cholera agent Vibrio cholerae VC97 (accession number GU570569; da Fonseca et al., 2011). Furthermore, the transposon-like structure in p3PS10 proved to be identical to a locus found in a plasmid carried by a CFC-resistant Photobacterium damselae subsp. piscicida strain (accession number AB453229), causative agent of pseudotuberculosis in fish (del Castillo et al., 2013). The 2-kb sequence encoding the tet-resistance determinant carried by p3PS10 was found in several aquatic pathogens, such as in A. salmonicida and Vibrio splendidus. Somewhat surprisingly, this sequence was also identified in the genome of Gallibacterium anatis strain 12656-12, a bacterium causing septicemia in chicken (Kudirkiene et al., 2013). The tet-resistance element was highly prevalent in a collection of G. anatis field isolates resistant to tetracycline, which have been gathered in Mexico and Denmark (Bojesen et al., 2011). We investigated the prevalence of the tet element in a group of representative isolates from our P. salmonis collection (Figure 3). As expected, those isolates carrying tet(31) were associated with OTC treatment failures observed in the field (Supplementary Table 4). These isolates were recovered from SRS outbreaks that took place in the south of Región de Aysén, in the period 2013-2015 (Supplementary Figure 3). This restricted dissemination pattern contrasts with the wide distribution reported for non-wild-type isolates for quinolones and FFC (Henríquez et al., 2015).
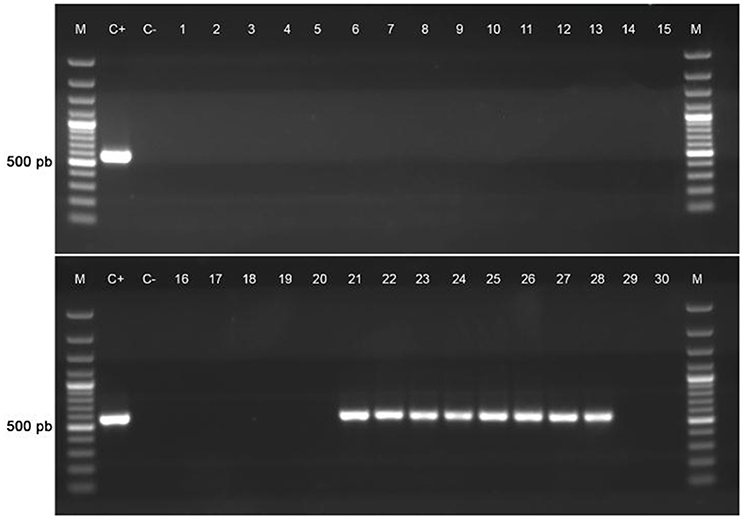
Figure 3. Screening for the tet(31) element in a group of representative P. salmonis isolates. Epidemiological data of isolates can be reviewed in Supplementary Table 4.
Discussion
The use of antibiotics in Chilean aquaculture is largely linked to the trend in productivity observed in the salmon industry. In the period 2014–2015, more than 500 metric tons/year were used to combat SRS outbreaks, with FFC and OTC being the active principles most frequently applied in field therapies (SERNAPESCA, 2015, 2016). Owing to a coordinated effort to reduce the use of antimicrobial drugs in the Chilean salmon industry, this amount dropped by roughly 30% the following year, reaching 382 tons in 2016 (SERNAPESCA, 2017). However, even in this scenario, the probability of the emergence and spread of resistances within the bacterial population surrounding salmon farms is still high. Additionally, in recent years, a continuous trend toward the preferential consumption of FFC was recorded and the relative share of this drug augmented to 81% and 90% in 2015 and 2016, respectively (SERNAPESCA, 2015, 2016). This situation predicts a reduction in the efficacy of therapies in forthcoming years due to the selection of strains with reduced susceptibilities to FFC. As it can be assumed from our results, the transference of p3PS10 to P. salmonis strains with a reduced susceptibility to FFC could trigger the worst-case scenario for the veterinary management of piscirickettsiosis outbreaks: so far, P. salmonis with a reduced susceptibility to FFC can be controlled applying OTC, but that would no longer be the case. In fact, P. salmonis isolates with non-wild-type traits for quinolone and FFC have already been reported (Henríquez et al., 2015; Saavedra et al., 2016). Therefore, the transmissibility and the replicative capacity of p3PS10 in homo- and heterologous hosts demonstrated in this study do not only point out the possibility of the emergence of P. salmonis strains displaying additional resistances, but also warn against the formation of other multidrug-resistant pathogens. An interesting point was the difference in p3PS10 transfer frequencies between P. salmonis isolates from identical and distinct genetic backgrounds. In our experiments, the transfer from LF-89-like to EM-90-like isolates occurred at a lower frequency, possibly because the latter group is refractory to the uptake of DNA. The fact that our actual collection of over 600 field isolates, with more than 50% of them being EM-90-like specimens, is lacking non-wild types belonging to this genogroup seems to support this theory. More research is needed on additional EM-90-like isolates to prove – or refute –this hypothesis.
This study further adds to our understanding of clinically important traits of P. salmonis. It is also the first description of antimicrobial resistances encoded by and mobilized in a plasmid of this pathogen. The medium-sized plasmid p3PS10 apparently replicates in a broad-host-range manner, while being preserved as a low-copy-number element, which reduces the fitness burden on its bacterial host. Intriguingly, the plasmid is maintained even in the absence of selective pressure. This finding strongly suggests that it carries a functional toxin-antitoxin (TA) addiction module, a genetic element whose expression facilitates the postsegregational killing of plasmid-less daughter cells in order to conserve the episome through generations (Unterholzner et al., 2013). TA modules were reported to be transcriptionally active in the LF-89 strain (Gómez et al., 2011), which makes it seem likely that similar preserving strategies are pursued by other plasmids found in P. salmonis. The significance of this mechanism lies in the persistence of resistance traits in the bacterial community even when the selective pressure has been removed from the environment.
Sequencing data suggest that p3PS10 has been horizontally acquired. The GC content of the plasmid discloses a genomic signature distant from its host P. salmonis (55 and 39%, respectively), while its mobilization was confirmed by mating assays. Despite the overwhelming sequence identity between the backbones of plasmids found in fish pathogens P. salmonis and E. tarda, genetic divergence was evident regarding the locus encoding multidrug resistance. This finding may be explained by the composite structure of the 11-kb locus, which probably consists of recombination-prone, independently mobilizable DNA fragments, a feature that can be extended to the tet-resistance cassette found in p3PS10. A homologous tet(31) element was found in pRAS2, an episome of A. salmonicida, a Gram-negative bacterium that causes furunculosis in salmonids. pRAS2 and its relative pRAS1 have been related to resistances to TET as well as trimethoprim and sulfonamides, and such resistances are exhibited by a series of A. salmonicida isolates recovered in Norwegian fish farms in the late 1980s (L'Abee-Lund and Sorum, 2000; Sørum et al., 2003). It is equally conceivable that p3PS10 has been acquired from environmental bacteria. In fact, Miranda et al. (2003) described environmental microorganisms able to tolerate OTC at concentrations ranging between 128 and 2,048 μg/mL. These MIC values are comparable to those described in the present study. However, the sequences of these tet genes do not match with that of tet(31), which was found in p3PS10 and its relatives. The fact that the P. salmonis tet-resistance determinant shows a high degree of sequence identity with aquatic and terrestrial pathogens such as G. anatis reflects the ubiquity of such resistance elements, providing an example of the flux of ARG between distinct environments.
According to our results, the mechanism underlying OTC resistance examined by us is different from that recently described by Contreras-Lynch et al. (2017). In the cited work, P. salmonis with reduced susceptibility to OTC were defined as those isolates tolerating OTC concentrations of >0.5 μg/mL, and MIC values of only 1.0–4.0 μg/mL were obtained for representatives of this category. By contrast, the group of isolates studied here exhibited significantly higher MIC to OTC and these MIC correlated with resistance from a clinical point of view. One possible explanation for these differences is that alternative mechanisms operate in those non-wild types reported by Contreras-Lynch and colleagues, such as the TET efflux pumps identified in a previous study (Cartes et al., 2016).
The phenotype linked to the resistance markers for CFC and SXT, as predicted after antibiotic resistance ontology analyses performed with CARD, deserves special attention. According to the results of these analyses, both the genomes of P. salmonis LF-89 and AY3800B contain five copies of chromosomal genes encoding CFC resistance-related efflux pumps, and AY3800B additionally carries cat2. However, and regardless of the redundancy, it seems that only the specific acetyltransferase encoded by cat2 in p3PS10 confers resistance to CFC. This conclusion is based on MIC values obtained for CFC-susceptible P. salmonis and resistant E. coli pCFC strains and stresses the need for a careful interpretation of the bioinformatic prediction. Concerning the resistance to sulfonamides, the prediction did not result as straightforward. The putative resistance marker sul2 was identified in the SXT-resistant AY3800B strain, but a similar phenotype was also displayed by the sul2-lacking LF-89 background. These findings reflect a lower sensitivity of CARD analysis for this trait. Thus, the genetic background of reduced susceptibility to SXT, as exhibited by all P. salmonis strains used in this study, requires further examination.
In summary, our results shed light on the plasmid biology and the genetic basis of antibiotic resistance in P. salmonis. This pathogen can be considered a dissemination vector for ARG in the seawater ecosystem, a role played by this insidious bacterium that has been underestimated so far. However, the low transfer frequency detected for p3PS10 makes its dissemination directly to human pathogens a rather unlikely event. The plasmid described in this work may serve as a valuable genetic tool for upcoming basic research on piscirickettsiosis, but also for future biotechnological applications focused on the control of this important fish pathogen.
Author Contributions
JS carried out PCR assays, molecular cloning, mating assays, and helped with the preparation of the figures. MG phenotypically characterized bacterial strains and performed PCN experiments. JV-G accomplished the restriction analyses and assisted with mating assays. HB designed qPCR primers and probes, assembled and annotated the sequencing data, and carried out CARD analyses. PB aided to obtain financial support and to draft the manuscript. MM conceived the study, performed sequence analyses and wrote the manuscript. All authors read and approved the final version of the text.
Funding
This work was funded by project 14IDL2-30005 of The Chilean Economic Development Agency, CORFO.
Conflict of Interest Statement
The authors declare that the research was conducted in the absence of any commercial or financial relationships that could be construed as a potential conflict of interest.
Acknowledgments
The authors gratefully acknowledge the edition of the manuscript by Dr. Melanie Kaiser. They are also thankful to ADL's veterinary team represented by Alexis Cancino and Andrés Riofrío, who collected field data on SRS outbreaks in collaborative salmon farms.
Supplementary Material
The Supplementary Material for this article can be found online at: https://www.frontiersin.org/articles/10.3389/fmicb.2018.00923/full#supplementary-material
Footnotes
References
Berendonk, T. U., Manaia, C. M., Merlin, C., Fatta-Kassinos, D., Cytryn, E., Walsh, F., et al. (2015). Tackling antibiotic resistance: the environmental framework. Nat. Rev. Microbiol. 13, 310–317. doi: 10.1038/nrmicro3439
Bohle, H., Henríquez, P., Grothusen, H., Navas, E., Bustamante, F., Bustos, P., et al. (2017). The Genome sequence of an oxytetracycline-resistant isolate of the fish pathogen Piscirickettsia salmonis harbors a multidrug resistance plasmid. Genome Announc. 5, e01571–e01516. doi: 10.1128/genomeA.01571-16
Bohle, H., Henríquez, P., Grothusen, H., Navas, E., Sandoval, A., Bustamante, F., et al. (2014). Comparative genome analysis of two isolates of the fish pathogen Piscirickettsia salmonis from different hosts reveals major differences in virulence-associated secretion systems. Genome Announc. 2:e01219-14. doi: 10.1128/genomeA.01219-14
Bojesen, A. M., Bager, R. J., Ifrah, D., and Aarestrup, F. M. (2011). The rarely reported tet(31) tetracycline resistance determinant is common in Gallibacterium anatis. Vet. Microbiol. 149, 497–499. doi: 10.1016/j.vetmic.2010.11.015
Bravo, C., and Martinez, V. (2016). Whole-genome comparative analysis of the pathogen Piscirickettsia salmonis. Vet. Microbiol. 196, 36–43. doi: 10.1016/j.vetmic.2016.10.015
Buschmann, A. H., Tomova, A., Lopez, A., Maldonado, M. A., Henriquez, L. A., Ivanova, et al. (2012). Salmon aquaculture and antimicrobial resistance in the marine environment. PLoS ONE 7:e42724. doi: 10.1371/journal.pone.0042724
Cabello, F. C., Godfrey, H. P., Buschmann, A. H., and Dolz, H. (2016). Aquaculture as yet another environmental gateway to the development and globalisation of antimicrobial resistance. Lancet Infect. Dis. 7, e127–e133. doi: 10.1016/S1473-3099(16)00100-6
Cartes, C., Isla, A., Lagos, F., Castro, D., Muñoz, M., Yañez, A., et al. (2016). Search and analysis of genes involved in antibiotic resistance in Chilean strains of Piscirickettsia salmonis. J. Fish Dis. 40, 1025–1039. doi: 10.1111/jfd.12579
CLSI (2014a). Clinical and Laboratory Standards Institute. Methods for Broth Dilution Susceptibility Testing of Bacteria Isolated From Aquatic Animals. Wayne, PA: Clinical and Laboratory Standards Institute.
CLSI (2014b). Clinical and Laboratory Standards Institute. Performance Standards for Antimicrobial Susceptibility Testing of Bacteria Isolated From Aquatic Animals, Second Informational Supplement. Wayne, PA: Clinical and Laboratory Standards Institute.
Contreras-Lynch, S., Smith, P., Olmos, P., Loy, M. E., Finnegan, W., and Miranda, C. D. (2017). A novel and validated protocol for performing MIC tests to determine the susceptibility of Piscirickettsia salmonis isolates to florfenicol and oxytetracycline. Front. Microbiol. 8:1255. doi: 10.3389/fmicb.2017.01255
da Fonseca, ÉL., dos Santos Freitas, F., and Vicente, A. C. (2011). Pc promoter from class 2 integrons and the cassette transcription pattern it evokes. J. Antimicrob. Chemother. 66, 797–801. doi: 10.1093/jac/dkr011
del Castillo, C. S., Jang, H. B., Hikima, J., Jung, T. S., Morii, H., Hirono, I., et al. (2013). Comparative analysis and distribution of pP9014, a novel drug resistance IncP-1 plasmid from Photobacterium damselae subsp. piscicida. Int. J. Antimicrob. Agents 42, 10–18. doi: 10.1016/j.ijantimicag.2013.02.027
Elema, M. O., Hoff, K. A., and Kristensen, H. G. (1996). Bioavailability of oxytetracycline from medicated feed administered to Atlantic salmon (Salmo salar L.) in seawater. Aquaculture 143, 7–14. doi: 10.1016/0044-8486(96)01253-7
Fryer, J. L., Lannan, C. N., Giovannoni, S. J., and Wood, N. D. (1992). Piscirickettsia salmonis gen. nov., sp. nov., the causative agent of an epizootic disease in salmonid fishes. Int. J. Syst. Bacteriol. 42, 120–126. doi: 10.1099/00207713-42-1-120
Gómez, F. A., Cárdenas, C., Henríquez, V., and Marshall, S. H. (2011). Characterization of a functional toxin-antitoxin module in the genome of the fish pathogen Piscirickettsia salmonis. FEMS Microbiol. Lett. 317, 83–92. doi: 10.1111/j.1574-6968.2011.02218.x
Heilig, J. S., Elbing, K. L., and Brent, R. (2001). Large-scale preparation of plasmid DNA. Curr. Protoc. Mol. Biol. Chapter 1:Unit1 7. doi: 10.1002/0471142727.mb0107s41
Henríquez, P., Kaiser, M., Bohle, H., Bustos, P., and Mancilla, M. (2015). Comprehensive antibiotic susceptibility profiling of Chilean Piscirickettsia salmonis field isolates. J. Fish Dis. 39, 441–448. doi: 10.1111/jfd.12427
Kudirkiene, E., Christensen, H., and Bojesen, A. M. (2013). Draft genome sequence of Gallibacterium anatis bv. haemolytica 12656-12 Liver, an isolate obtained from the liver of a septicemic chicken. Genome Announc. 1, e00810–e00813. doi: 10.1128/genomeA.00810-13
L'Abee-Lund, T. M., and Sorum, H. (2000). Functional Tn5393-like transposon in the R plasmid pRAS2 from the fish pathogen Aeromonas salmonicida subspecies salmonicida isolated in Norway. Appl. Environ. Microbiol. 66, 5533–5535. doi: 10.1128/AEM.66.12.5533-5535.2000
Maisey, K., Montero, R., and Christodoulides, M. (2016). Vaccines for piscirickettsiosis (salmonid rickettsial septicaemia, SRS): the Chile perspective. Expert. Rev. Vaccines 16, 215–228. doi: 10.1080/14760584.2017.1244483
McArthur, A. G., Waglechner, N., Nizam, F., Yan, A., Azad, M. A., Baylay, A. J., et al. (2013). The comprehensive antibiotic resistance database. Antimicrob. Agents Chemother. 57, 3348–3357. doi: 10.1128/AAC.00419-13
Miranda, C. D., Kehrenberg, C., Ulep, C., Schwarz, S., and Roberts, M. C. (2003). Diversity of tetracycline resistance genes in bacteria from Chilean salmon farms. Antimicrob. Agents Chemother. 47, 883–888. doi: 10.1128/AAC.47.3.883-888.2003
Miranda, C. D., and Rojas, R. (2007). Occurrence of florfenicol resistance in bacteria associated with two Chilean salmon farms with different history of antibacterial usage. Aquaculture 266, 39–46. doi: 10.1016/j.aquaculture.2007.02.007
Miranda, C. D., and Zemelman, R. (2002). Bacterial resistance to oxytetracycline in Chilean salmon farming. Aquaculture 212, 31–47. doi: 10.1016/S0044-8486(02)00124-2
Otterlei, A., Brevik, O. J., Jensen, D., Duesund, H., Sommerset, I., Frost, P., et al. (2016). Phenotypic and genetic characterization of Piscirickettsia salmonis from Chilean and Canadian salmonids. BMC Vet. Res. 12:55. doi: 10.1186/s12917-016-0681-0
Pulgar, R., Travisany, D., Zúñiga, A., Maass, A., and Cambiazo, V. (2015). Complete genome sequence of Piscirickettsia salmonis LF-89 (ATCC VR-1361) a major pathogen of farmed salmonid fish. J. Biotechnol. 212, 30–31. doi: 10.1016/j.jbiotec.2015.07.017
Ramírez, M. S., Pineiro, S., Group, A. I. S., and Centron, D. (2010). Novel insights about class 2 integrons from experimental and genomic epidemiology. Antimicrob. Agents Chemother. 54, 699–706. doi: 10.1128/AAC.01392-08
Rozas, M., and Enríquez, R. (2014). Piscirickettsiosis and Piscirickettsia salmonis in fish: a review. J. Fish Dis. 37, 163–188. doi: 10.1111/jfd.12211
Saavedra, J., Hernández, N., Osses, A., Castillo, A., Cancino, A., Grothusen, H., et al. (2016). Prevalence, geographic distribution and phenotypic differences of Piscirickettsia salmonis EM-90-like isolates. J. Fish Dis. 40, 1055–1063. doi: 10.1111/jfd.12581
Schwarz, S., Kehrenberg, C., Doublet, B., and Cloeckaert, A. (2004). Molecular basis of bacterial resistance to chloramphenicol and florfenicol. FEMS Microbiol. Rev. 28, 519–542. doi: 10.1016/j.femsre.2004.04.001
SERNAPESCA (2015). Informe Sobre Uso de Antimicrobianos en la Salmonicultura Nacional 2014. Valparaíso. Available online at: http://www.sernapesca.cl
SERNAPESCA (2016). Informe Sobre Uso de Antimicrobianos en la Salmonicultura Nacional 2015. Valparaíso. Available online at: http://www.sernapesca.cl
SERNAPESCA (2017). Informe Sobre Uso de Antimicrobianos en la Salmonicultura Nacional 2016. Valparaíso. Available online at: http://www.sernapesca.cl
Shah, S. Q., Cabello, F. C., L'abee-Lund, T. M., Tomova, A., Godfrey, H. P., Buschmann, A. H., et al. (2014). Antimicrobial resistance and antimicrobial resistance genes in marine bacteria from salmon aquaculture and non-aquaculture sites. Environ. Microbiol. 16, 1310–1320. doi: 10.1111/1462-2920.12421
Shintani, M., Sanchez, Z. K., and Kimbara, K. (2015). Genomics of microbial plasmids: classification and identification based on replication and transfer systems and host taxonomy. Front. Microbiol. 6:242. doi: 10.3389/fmicb.2015.00242
Siddique, A., and Figurski, D. H. (2002). The active partition gene incC of IncP plasmids is required for stable maintenance in a broad range of hosts. J. Bacteriol. 184, 1788–1793. doi: 10.1128/JB.184.6.1788-1793.2002
Sköld, O. (2000). Sulfonamide resistance: mechanisms and trends. Drug Resist. Updat. 3, 155–160. doi: 10.1054/drup.2000.0146
Skulj, M., Okrslar, V., Jalen, S., Jevsevar, S., Slanc, P., Strukelj, B., et al. (2008). Improved determination of plasmid copy number using quantitative real-time PCR for monitoring fermentation processes. Microb. Cell Fact. 7:6. doi: 10.1186/1475-2859-7-6
Sørum, H., L'abee-Lund, T. M., Solberg, A., and Wold, A. (2003). Integron-containing IncU R plasmids pRAS1 and pAr-32 from the fish pathogen Aeromonas salmonicida. Antimicrob. Agents Chemother. 47, 1285–1290. doi: 10.1128/AAC.47.4.1285-1290.2003
Thaker, M., Spanogiannopoulos, P., and Wright, G. D. (2009). The tetracycline resistome. Cell. Mol. Life Sci. 67, 419–431. doi: 10.1007/s00018-009-0172-6
Unterholzner, S. J., Poppenberger, B., and Rozhon, W. (2013). Toxin-antitoxin systems: biology, identification, and application. Mob. Genet. Elements 3:e26219. doi: 10.4161/mge.26219
Wang, Q., Yang, M., Xiao, J., Wu, H., Wang, X., Lv, Y., et al. (2009). Genome sequence of the versatile fish pathogen Edwardsiella tarda provides insights into its adaptation to broad host ranges and intracellular niches. PLoS ONE 4:e7646. doi: 10.1371/journal.pone.0007646
Keywords: Piscirickettsia salmonis, piscirickettsiosis, antibiotic resistance, tetracycline, plasmid
Citation: Saavedra J, Grandón M, Villalobos-González J, Bohle H, Bustos P and Mancilla M (2018) Isolation, Functional Characterization and Transmissibility of p3PS10, a Multidrug Resistance Plasmid of the Fish Pathogen Piscirickettsia salmonis. Front. Microbiol. 9:923. doi: 10.3389/fmicb.2018.00923
Received: 31 October 2017; Accepted: 20 April 2018;
Published: 08 May 2018.
Edited by:
Jaime Romero, Universidad de Chile, ChileReviewed by:
David Verner-Jeffreys, Centre for Environment, Fisheries and Aquaculture Science (CEFAS), United KingdomClaudio D. Miranda, Universidad Católica del Norte, Chile
Copyright © 2018 Saavedra, Grandón, Villalobos-González, Bohle, Bustos and Mancilla. This is an open-access article distributed under the terms of the Creative Commons Attribution License (CC BY). The use, distribution or reproduction in other forums is permitted, provided the original author(s) and the copyright owner are credited and that the original publication in this journal is cited, in accordance with accepted academic practice. No use, distribution or reproduction is permitted which does not comply with these terms.
*Correspondence: Marcos Mancilla, bW1hbmNpbGxhQGFkbGRpYWdub3N0aWMuY2w=
†These authors have contributed equally to this work.