- 1Institute of Marine Biology, Ocean College, Zhejiang University, Hangzhou, China
- 2Wuxi Dongfang Environmental Engineering Design and Research Institute, Wuxi, China
- 3Zhoushan Municipal Center for Disease Control and Prevention, Zhoushan, China
- 4Institute of Environment Resources and Soil Fertilizer, Zhejiang Academy of Agricultural Sciences, Hangzhou, China
Herein, we investigated the chemical, electrochemical, and spectroscopic characteristics of humic acids (HAs) extracted from sediments of different origin [Ling Qiao river, Xi Xi wetland, Qi Zhen lake (QZ), and Hu Zhou pond in Zhejiang province, China], paying particular attention to their role in the enhancement of nitrate and FeOOH reduction. Notably, the highest C/N ratio (16.16), O/C ratio (1.89), and Fe content (11.57 g kg-1 sample) were observed for HAs extracted from QZ sediment. Cyclic voltammetry analyses confirmed that all HAs contained redox-active groups and exhibited redox potentials between -0.36 and -0.28 V vs. the standard hydrogen electrode. All HAs showed similar Fourier transform infrared spectra with variable absorption intensity, the spectra verified the presence of aromatic C=C, C–H, and C=O of quinone ketones group in HAs. Electron spin resonance suggested that quinone moieties within HAs are the redox-active centers. All HAs promoted the microbial reduction of nitrate and amorphous FeOOH by Shewanella oneidensis strain MR-1, achieving high nitrate reduction extents of 79–98.4%, compared to the biotic and abiotic control values of 29.6 and 0.006%, respectively. The corresponding extents of Fe(II) production equaled 43.25–60.5%, exceeding those of biotic and abiotic controls (28.5 and 0.005%, respectively). In addition to the highest C/N, O/C ratio, and Fe content, HA extracted from QZ sediment also exhibited the highest nitrate and FeOOH reduction performances. Although the proportion of organic redox-active carbon is small, the potential electron-mediating ability is not ignorable. HAs are redox active for enhancing microbial reduction of nitrate and amorphous FeOOH regardless of the location or texture of parent sediments, implying their great potential for acting as redox mediator in enhancing multiple microbial reduction, thereby affecting various biogeochemical processes (i.e., iron cycle, nitrogen cycle, etc.) as well as in situ remediation in anaerobic environment.
Introduction
Humic substances (HSs) are redox-active organic materials widely present in natural environment, e.g., in soils, sediments, peatlands, and wetlands (Lovley et al., 1996). According to their solubility, HSs can be divided into three groups (Aiken et al., 1985), namely humin, humic acids (HAs), and fulvic acids. Among them, HAs are a prominent fraction of HSs, significantly affecting the distribution, sorption, transport, and fate of environmental pollutants (Scott et al., 1998; Gu and Chen, 2003; Guo et al., 2018), e.g., the production and toxicity of trace metal contaminants, disinfection byproduct formation, adsorption and dissolution of hydrophobic organic pollutants, and redox reactions in soils.
Dissimilatory reduction is known to comprise two different processes, denoted as denitrification and reduction to (DNRA). The latter process, also known as ammonification or fermentative reduction, has recently attracted increased attention due to its significance for nitrogen cycling in various ecosystems, achieving greater N retention than denitrification, since the produced is more available for plant and microbial uptake but less prone to losses via leaching or volatilization compared with N2 produced by denitrification (Rütting et al., 2011). Dissimilatory microbial reduction of Fe(III) to Fe(II) plays a key role in environmental iron cycling and influences a number of biochemical processes such as the exchange of nutrients and trace metals between aquatic systems and sediments (Wang et al., 2017). Thus, both DNRA and FeOOH reduction significantly affect organic matter exchange and geochemical cycling in anoxic systems.
Reduction reactions under anaerobic conditions are commonly limited by the rate of electron transfer, which can be accelerated by the use of redox mediators (RMs), with certain reactions simply not taking place in their absence (Zhang and Katayama, 2012; Zhang et al., 2017). Over the past two decades, HSs and their quinoid model, anthraquinone-2,6-disulfonate (AQDS), have been reported to act as RMs for the reduction of several substrates (Gu and Chen, 2003; Costa et al., 2010; Zhang D.D. et al., 2015; Wang et al., 2017; Guo et al., 2018), e.g., chlorinated compounds, nitrate, azo dyes, and oxidized metal ions such as Fe(III), Cr(VI), and U(VI).
Humic acids have been hypothesized to contain redox-active functional groups such as quinones and have the ability to transfer electrons, accepting those generated by microbial lactate oxidation and donating them to nitrate or Fe(III)-containing minerals, thus accelerating the reduction of nitrate and FeOOH (Lovley et al., 1996; Wang et al., 2017). Although HAs (extracted from soils/surface waters) and their quinoid analogs (e.g., AQDS) have been extensively characterized, the redox characterization of HAs extracted from different sediments and their functions as RMs for microbial nitrate and Fe reduction has been underexplored. Moreover, owing to the complexity and heterogeneity of HAs, their structures are difficult to determine by conventional methods. Therefore, to better understand the reactivity of HAs originating from different sediments, these species were herein characterized by chemical, electro(chemical), and spectroscopic methods, and their effects on nitrate and FeOOH reduction by model dissimilatory metal-reducing bacteria (Shewanella oneidensis MR-1) were also determined, shedding light on the underlying reasons of different electron-mediating efficiencies of HAs on microbial metabolic processes.
Materials and Methods
Sediment Samples
Sediment samples were obtained from four different locations [Ling Qiao river (LQ) (29° 51′ 59″ N, 121° 33′ 35″ E), Xi Xi wetland (XX) (30° 15′ 27″ N, 120° 3′ 25″ E), Qi Zhen lake (QZ) (30° 18′ 28″ N, 120° 5′ 3″ E), and Hu Zhou pond (HZ) (30° 46′ 38″ N, 120° 9′ 5″ E)] in Zhejiang province, China. LQ represents an area with pollution, there are many factories around it, such as detergent factories, paper mills, etc. XX is inside the nature reserve area with a lot of vegetation (such as herbs and shrubs). QZ represents an area with low pollution, which is located in Zijingang campus of Zhejiang University. HZ sediment was sampled from aquaculture pond cultured with black carp. The collected samples were air-dried and stored at room temperature prior to use. Further description of physicochemical characteristics of the sampling sites and interstitial water in the sediments is shown in Supplementary Table S1 of Supplementary Material.
Extraction and Purification of HAs
Humic acids were extracted from air-dried sediment samples as described previously (Zhang and Katayama, 2012). Briefly, 500 g of sediments treated with 2 vol% HF (Schmidt et al., 1997) was shaken with 0.1 M NaOH at 150-170 rpm for 24 h and centrifuged, with the supernatant collected as the extract. The above process was repeated at least eight times until the dark color of the extract was not obvious. The collected alkaline extract was acidified to pH < 2 with 2 M HCl and coagulated in the dark overnight, with the produced precipitate (HAs) subsequently collected by centrifugation and subjected to repeated alkali and acid treatment to remove the residual inorganic particulate matter. Finally, the obtained HAs were rinsed with HF once and then repeatedly rinsed with distilled water and freeze-dried. All the solutions and distilled water used during the extraction were flushed with N2, and all the centrifugation steps were carried out at 5000 × g for 10 min. Samples extracted from sediment collected from the LQ, XX, QZ, and HZ were denoted as LQ-HA, XX-HA, QZ-HA, and HZ-HA, respectively.
Four HAs stock solutions were separately prepared by dissolving HAs in deionized water containing 2 ml of 0.1 M NaOH solution under nitrogen, with the pH subsequently adjusted to 7.2 with 1 M HCl. A standard commercial (Aldrich) HA stock solution was also prepared in the same way. Thus the obtained stock solution was deoxygenated by purging with N2 and used immediately (Zhang and Katayama, 2012).
(Electro)chemical Analyses
The C, H, N, and S contents of HAs were determined using a Yanaco MT-5 CHN-corder (Yanaco New Science Inc., Kyoto, Japan) with a high-temperature combustion method. Ash contents were determined by combustion of dried HAs at 600°C for 5 h. Oxygen contents were calculated by the mass difference. Metal contents were determined by inductively coupled plasma atomic emission spectroscopy (Optima 3300DV, PerkinElmer, Yokohama, Japan) after perchloric acid and nitric acid digestion, the procedure of acid digestion is described as follows: 0.3 g of each HA sample was accurately weighted and placed at 50 ml Erlenmeyer flask, then digested with about 8.0 ml of mixed acid solution (the volume ratio of nitric acid to perchloric acid was 4:1) on an electrothermal board at 120°C to keep micro-boiling state. When the brown smoke disappeared and the sample was nearly transparent, continue heating to near dryness, cooling, finally diluted to 20 ml with 2% nitric acid.
Cyclic voltammetry (CV) measurements were performed using a potentiostat (HSV-110; Hokuto Denko Inc., Osaka, Japan) comprising twisted Pt (0.8 mm × 1 m, Nilaco, Tokyo, Japan) as working and counter electrodes and a Ag/AgCl reference electrode (6 mm × 15 cm, Fusheng Analytical Instrument Co., Shanghai, China). To perform these measurements, HA samples (0.25 g) were suspended in 1 mM NaClO4 in dimethyl sulfoxide (DMSO, 50 ml) as an electrolyte (Nurmi and Tratnyek, 2002), and CV curves were recorded at a scan rate of 10 mV s-1 within a potential range of -2.0 to 0.0 V vs. Ag/AgCl. All test solutions were purged with high-purity N2 for 15 min before each set of scans, and background scans were performed before every measurement.
Fourier transform infrared (FTIR) spectra of HAs were recorded on a JASCO FT/IR-6 100 spectrometer (JASCO, Tokyo, Japan) in the range of 400–4000 cm-1 at a resolution of 4 cm-1 using eight scans. The KBr pellet technique was applied, and the recorded spectra were background-corrected using pure KBr and ambient air as blanks (Francioso et al., 1998).
Electron spin resonance (ESR) was used to measure the organic radical contents of HSs (Roden et al., 2010). The signal intensity of the ESR radicals of each HA was examined. The pH of HA was adjusted using either 0.1 M HCl or 0.1 M NaOH to pH 3 or 11, and freeze-dried. The ESR spectra were recorded at room temperature using a Bruker ESRA-300 spectrometer (Berlin-Adlershof, Germany), operating at 9.85 GHz with a 100 kHz modulation frequency of the steady magnetic field. The freeze-dried HA samples were measured in quartz glass sample tubes with an inner diameter of 1 mm. Other spectrometric conditions for the measurements were as follows: magnetic field centered at 3500 G, 6.37 mW microwave power, 20.5 s sweep time, and 0.08 s time constant.
Bacterial Culturing and Growth Conditions
The model dissimilatory metal-reducing bacteria (S. oneidensis strain MR-1) purchased from China Center for Type Culture Collection was used for microbial reduction experiments. The above bacteria were aerobically inoculated in a Luria-Bertani medium (10 g l-1 NaCl, 5 g l-1 yeast extract, 10 g l-1 tryptone) for 12 h in a shaker at 150-170 rpm, 30°C, being subsequently harvested by 3 × 10-min centrifugation at 8000 ×g and 4°C in phosphate buffer shortly before reaching the exponential growth phase. Washed late-log-phase cultures (2 × 107 cells ml-1) of MR-1 were incubated in anaerobic HA medium, which was prepared in 50-ml bottles containing 20 ml of mineral medium (Zhang et al., 2014), 0.2-μm-filter-sterilized vitamin solution, 125 mg l-1 HA solution, and 10 mM formate. The mineral medium comprised (per liter) 1.0 g of NH4Cl, 0.05 g of CaCl2⋅2H2O, 0.1 g of MgCl2⋅6H2O, 0.4 g of K2HPO4, 1 ml of trace element SL-10 solution, 1 ml of Se/W solution, 15 mM 3-(N-morpholino)propanesulfonic acid (MOPS) buffer (pH 7.2), and 50 mg resazurin-Na.
Nitrate (5 mM) or amorphous FeOOH (4 mM) was added as electron acceptors, and the bottles were sealed with Teflon-coated butyl rubber stoppers and aluminum seals and autoclaved at 121°C for 30 min. The cultures were statically incubated in the dark at 30°C. A 4-mM solution of amorphous FeOOH was prepared as described elsewhere (Lovley et al., 1996). Herein, the nitrate- and iron-reducing cultures are referred to as -HA and Fe-HA cultures, respectively. For all conditions, triplicate cultures were prepared in addition to an autoclaved control (abiotic control) and an HA-free control (biotic control), and each culture was measured three times.
In the above cultures, was quantified by ultraviolet spectrophotometry (Cawse, 1967), and was quantified by Nessler’s reagent colorimetric method (Lee and Takahashi, 1966). The concentration of Fe(II) was measured spectrophotometrically using 1,10-phenanthroline (Jones et al., 1981).
Results
Chemical Characterization
The yield (by weight), nonmetalloid (C, H, N, S, O) element contents and ash contents of HAs are shown in Table 1. Specifically, the above yields varied from 1.64 to 15.04%, whereas the elemental compositions (containing ash) varied in the range of 8.26–15.27% for C, 1.30–2.89% for H, 0.65–1.21% for N, 0.35–0.93% for S, and 12.62–25.41% for O, the ash contents of HAs varied from 55.1 to 76.2%. C/N, H/C, and O/C atomic ratios were calculated as 12.88–16.16, 1.89–3.45, and 1.15–1.89, respectively, differing widely for different HAs.
Table 2 shows the metal (Fe, Cu, Mn, Zn, Cd, Cr, Pb) element contents of HAs. All HAs contained large amounts of Fe (9.82–11.57 mg per g HA) and Cu (0.58–1.46 mg per g HA), while the concentrations of Mn, Zn, and Cr equaled 0.07–0.14, 0.07–0.18, and 0.09–0.11 mg per g HA, respectively. The concentration of Cd was below the detection limit, except for QZ-HA (0.001 mg per g HA), similarly to that of Pb, except for LQ-HA (0.003 mg per g HA).
Electrochemical Characterization
To assess the redox-active moieties of HAs, CV experiments were carried out using DMSO as a solvent to increase the electrode reactivity of HAs, since HSs generally produce CV curves with little or no useful structure due to lack of electrode activity (Sawyer et al., 1995).
Redox couples were observed for all HA samples (Figure 1), and the corresponding redox potentials were estimated from CV curves recorded in DMSO. The potentials obtained in DMSO (vs. Ag/AgCl) were approximately 0.45 V higher than those in H2O [vs. the standard hydrogen electrode (SHE)] (Nurmi and Tratnyek, 2002), with the validity of this correction confirmed by our previous research on humins (Zhang and Katayama, 2012). The redox potentials of LQ-HA, XX-HA, QZ-HA, and HZ-HA were estimated as -0.3, -0.32, -0.28, and -0.36 V (vs. SHE), respectively.
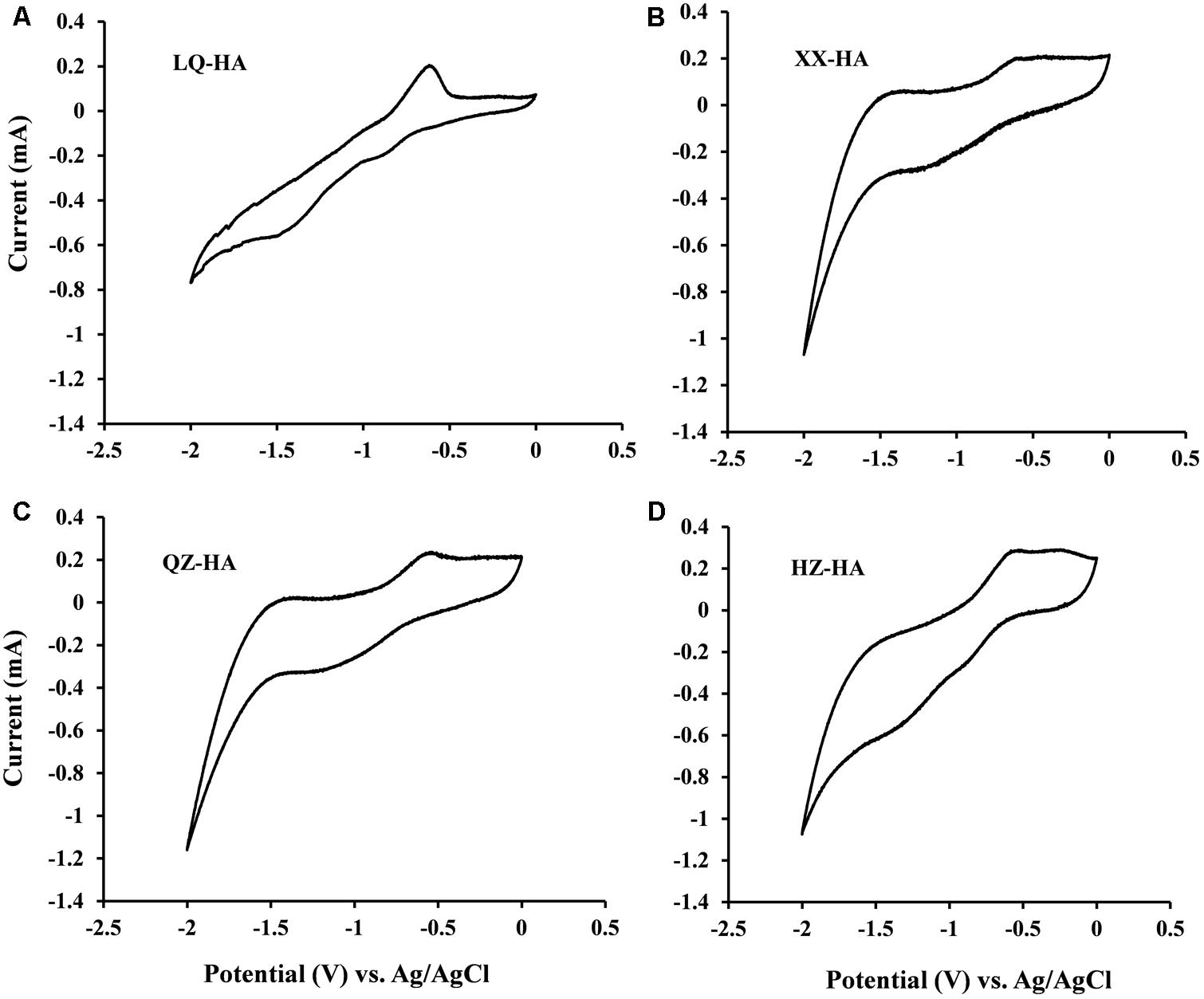
FIGURE 1. Cyclic voltammograms of different HAs [LQ-HA (A), XX-HA (B), QZ-HA (C), HZ-HA (D)] dissolved in DMSO. Abbreviated names of HAs are the same as those presented in Table 1.
Spectroscopic Characterization
Fourier transform infrared spectra are shown in Figure 2. Interpretation of the spectra were based on those literatures (Francioso et al., 1998; Senesi et al., 2003; Hesse et al., 2005; Tatzber et al., 2007).
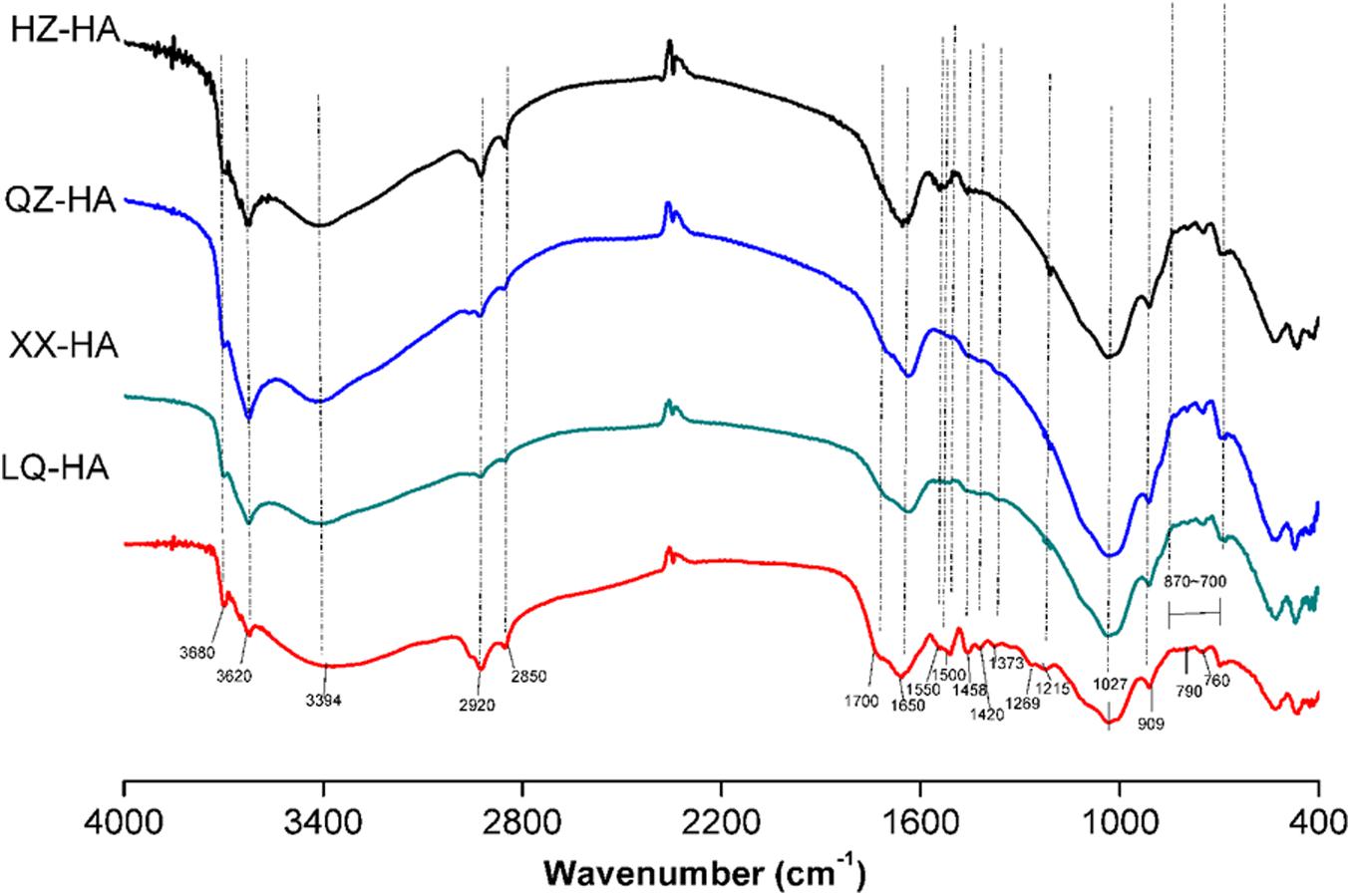
FIGURE 2. Fourier transform infrared (FTIR) spectra of HAs extracted from four different sediments. Abbreviated names of HAs are the same as those presented in Table 1.
All HAs showed bands around 3680 and 3620 cm-1 as well as a broad peak around 3394 cm-1, attributed to O–H vibrations of carboxylic or phenolic groups and to N–H stretches of amides and amines. Peaks around 2920 and 2850 cm-1 were assigned to symmetric and asymmetric vibrations of aliphatic CH3 and CH2 groups. A small peak at 1700 cm-1 could be attributed to carbonyl vibrations of carboxyl groups, esters, aldehydes, and ketones. A pronounced peak at 1650 cm-1 was ascribed to C=C stretches of aromatic rings, C=O stretches of amide groups (amide I band), and C=O of quinone ketones, and asymmetrical C–O stretches of carboxyl groups, which are commonly observed for HAs (Zhang C. et al., 2015).
Ling Qiao river-HA also showed a high resolved fingerprint area (from 1600 to 900 cm-1), whereas these spectra were not obvious in other HAs probably because of the low proportion of the corresponding groups. As for these spectra, the peak at 1550 cm-1 was assigned to COO–, the peak at 1500 cm-1 was assigned to the N–H, C=N (amide II band), and C=C, the peak at 1458 cm-1 was assigned to aliphatic C–H, the peak at 1420 cm-1 could be attributed to C=N of primary amides (amide III band), the peak at 1373 cm-1 could be attributed to –CO–CH3 and possibly from nitrate, the peak at 1269 cm-1 could be attributed to C–O stretching of aryl ethers, the peak at 1215 cm-1 could be attributed to C–O and OH of COOH, C–O of aryl ethers and phenols, P–O–aryl and tertiary alcohols, and a strong band at 1027 cm-1 could be attributed to C–O stretching of alcohol, sulfoxides, carbohydrates, or polysaccharides-like substances or Si–O of silicates. The band at 909 cm-1 was assigned to an aromatic C–H deformation. Meanwhile, the bands from 870 to 700 cm-1 could be attributed to aromatic C–H, and less substituted rings appear at less wave numbers, the peak at 790 cm-1 could be attributed to benzene rings with two or three adjacent H and/or an isolated H, R2C=CRH groups and nitrates, the peak at 760 cm-1 could be attributed to sp3–CH2 and benzene rings with four and/or five adjacent H.
Electron spin resonance spectra of HAs are shown in Figure 3. The results showed that all of the spectra for HAs were devoid of hyperfine splitting. The spectroscopic splitting constants (g) were in the range of 2.0041–2.0046, HZ-HA showed an ESR signal at g = 2.0046, While XX-HA at 2.0044, QZ-HA at 2.0042, and LQ-HA at 2.0041, these values agrees with previously reported g-values for HSs with semi-quinones being the primary organic radicals (Scott et al., 1998). All HAs adjusted at pH 11 produced remarkable increase in radical signal compared with that of HAs at pH 3. The increase in ESR signal at high pH is typical for the semiquinone-type radicals, which supports the interpretation that quinone moieties were responsible for the observed signal increase (Paul et al., 2006; Zhang and Katayama, 2012). However, we cannot exclude the contribution of ESR signals from other origins such as thiols, nitrogen functional groups, or metal-organic complexes.
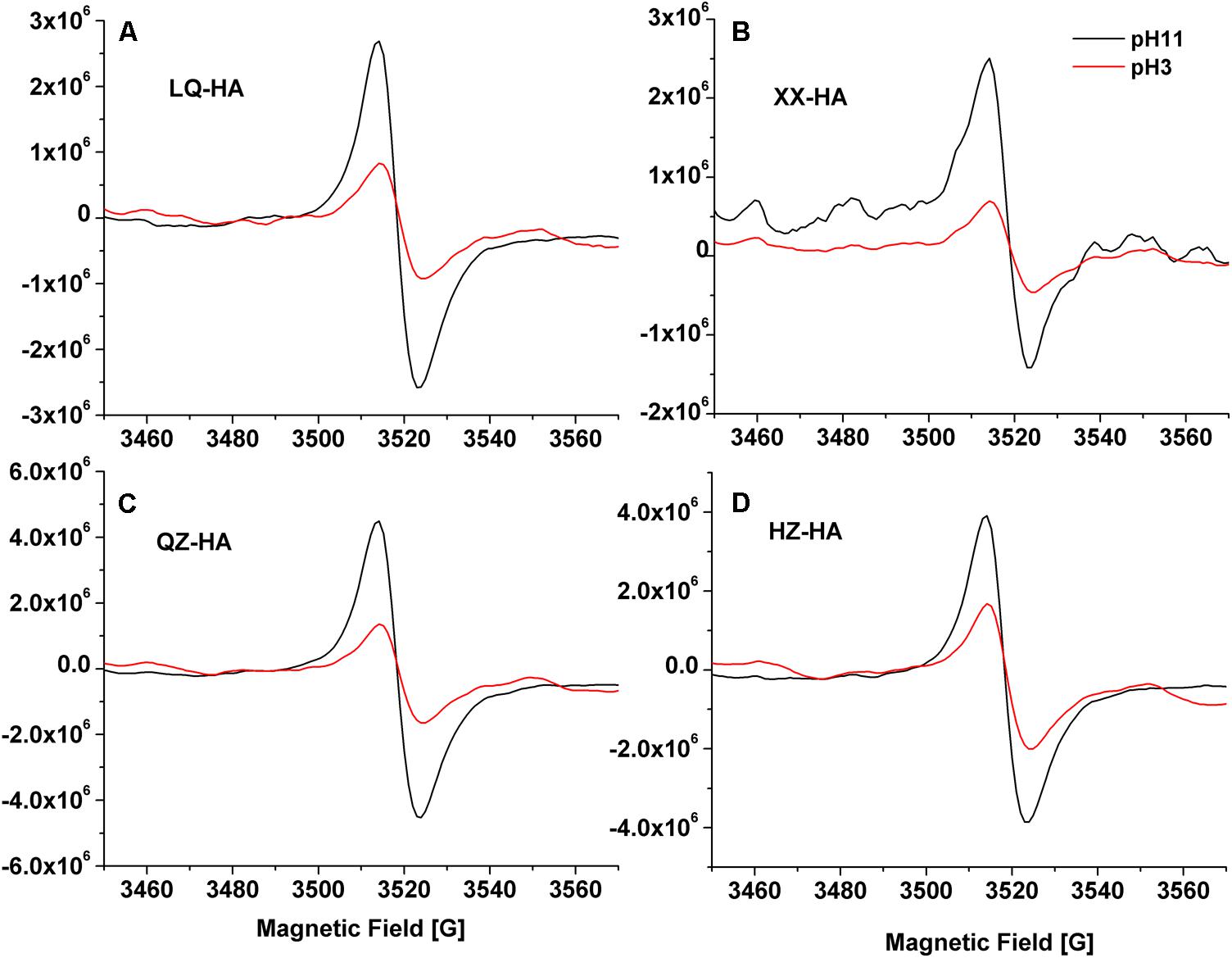
FIGURE 3. Electron Spin Resonance (ESR) spectra of different HAs [LQ-HA (A), XX-HA (B), QZ-HA (C), HZ-HA (D)] samples. Abbreviated names of HAs are the same as those presented in Table 1.
HA-Promoted Microbial Reduction of Nitrate
Figure 4 shows changes in the amounts of nitrate, nitrite, and ammonium observed during HA-promoted microbial reduction of 5 mM nitrate by MR-1. The biotic control corresponded to an HA-free culture, and the abiotic control was represented by an autoclave-sterilized culture. All HAs enhanced the microbial reduction of nitrate to different extents. Under nitrate-reducing conditions, the 5-mM nitrate was finally reduced to ammonium within 3 days (Figures 4A,C). The decrease of nitrate concentrations showed that the addition of HAs to the -HA culture triggered anaerobic microbial DNRA without a lag phase. Compared to the biotic and abiotic controls, nitrate was initially reduced to nitrite, with the increase of ammonium concentration being slow within the first 8 h. Subsequently, the reduction of the produced nitrite to ammonium resulted in a rapid concentration increase of the latter between 8 and 14 h, with a plateau reached after 72 h. Among the four HAs, QZ-HA showed the best performance for nitrate reduction, achieving a reduction extent of 98.4% (4.92 mM ammonium in the culture), followed by HZ-HA (4.47 mM, 89.4%), XX-HA (4.19 mM, 83.8%), and LQ-HA (3.95 mM, 79%). However, for the biotic control, only ∼33% of nitrate could be transformed into ammonium, with the rest mainly converted to nitrite and accumulated, i.e., only 1.48 mM ammonium and ∼2.7 mM nitrite were produced. In the abiotic control sample, only 0.05 mM nitrite and 0.03 mM ammonium were observed, with the reduction extent thus equaling 0.006% (Figure 4C).
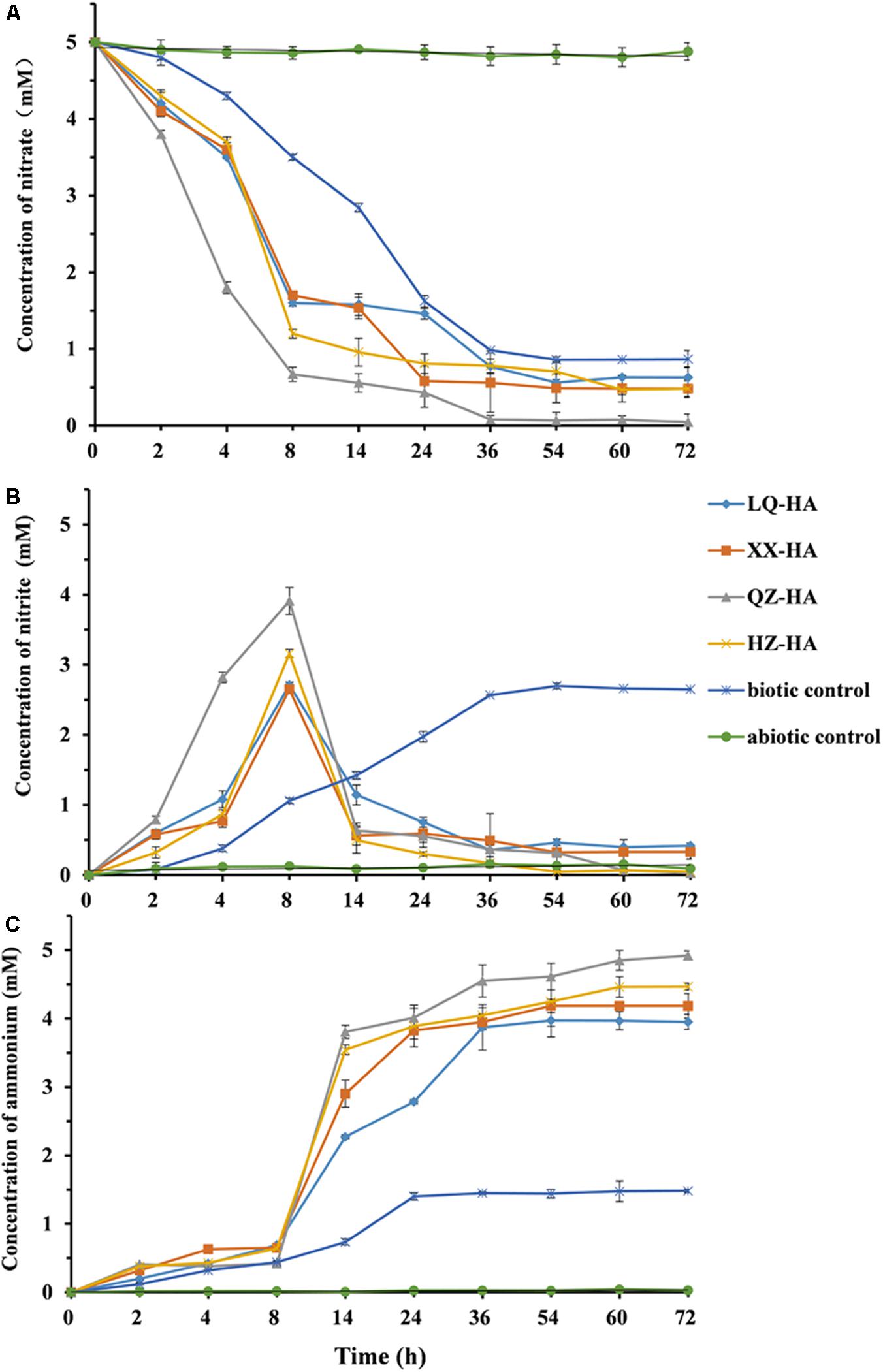
FIGURE 4. The changes in the concentration of nitrate (A), nitrite (B), ammonium (C) left in the culture within 72 h, in which microbial reduction were conducted by MR-1 in the presence of HAs of different origins (LQ-HA, XX-HA, QZ-HA, and HZ-HA) when 5 mM nitrate was added as the electron acceptor in each culture. Data show the mean values of triplicate cultures, and vertical bars show the difference in triplicate cultures.
Figures 4B,C show that the nitrite concentration reached its maximum after 8 h, with the highest reaction extents observed for QZ-HA, and those of other cultures being similar to each other. In the time window of 8–72 h, -HA cultures (except for the biotic control) mainly featured the reduction of nitrite to ammonium, with the highest reaction extent observed for QZ-HA, followed by HZ-HA, XX-HA, and LQ-HA in decreasing order. For the biotic control, the concentration of nitrite stabilized at 2.6 mM after 36 h, and that of ammonium stabilized at 1.45 mM after 24 h (Figures 4B,C). No nitrate consumption or nitrite and ammonium production was observed for all abiotic controls.
The observed changes in amounts of nitrate, nitrite, and ammonium as mediated by standard HA during microbial reduction of nitrate (5 mM) by MR-1 were showed in Supplementary Figure S1 of Supplementary Material.
HA-Promoted Microbial Reduction of FeOOH
Figure 5 shows the results of dissimilatory Fe(III) reduction by MR-1 in the presence of different HAs when 4 mM FeOOH was added as electron acceptor. In the Fe-HA culture, Fe(III) was reduced to Fe(II), with all HAs enhancing this process to variable extents within 156 h; as a result, 1.73–2.42 mM Fe(II) (reduction extent = 43.25–60.5%) was eventually produced in the presence of HAs. Conversely, in the HA-free biotic control, only 1.14 mM Fe(II) was detected, corresponding to a reduction extent of 28.5%, with the respective values for the abiotic control equaling 0.02 mM and 0.005%. The QZ-HA culture showed the best reduction performance, achieving a reduction extent of 60.5% [2.42 mM Fe(II)], followed by XX-HA (47.5%, 1.90 mM), HZ-HA (46.25%, 1.85 mM), and LQ-HA (43.25%, 1.73 mM), with the above three cultures showing very similar performances. Importantly, the HA-promoted microbial reduction of Fe(III) started with a lag phase of 4–48 h, during which the concentration of Fe(II) increased very slowly, followed by a rapid concentration increase after 48 h and culminating in a plateau after 112–156 h. The above trend was similar to that observed for the biotic control, whereas there is no production of Fe(II) in the abiotic control. The results of dissimilatory Fe(III) reduction of 4 mM FeOOH by MR-1 in the presence of standard HA were shown in Supplementary Figure S2 of Supplementary Material.
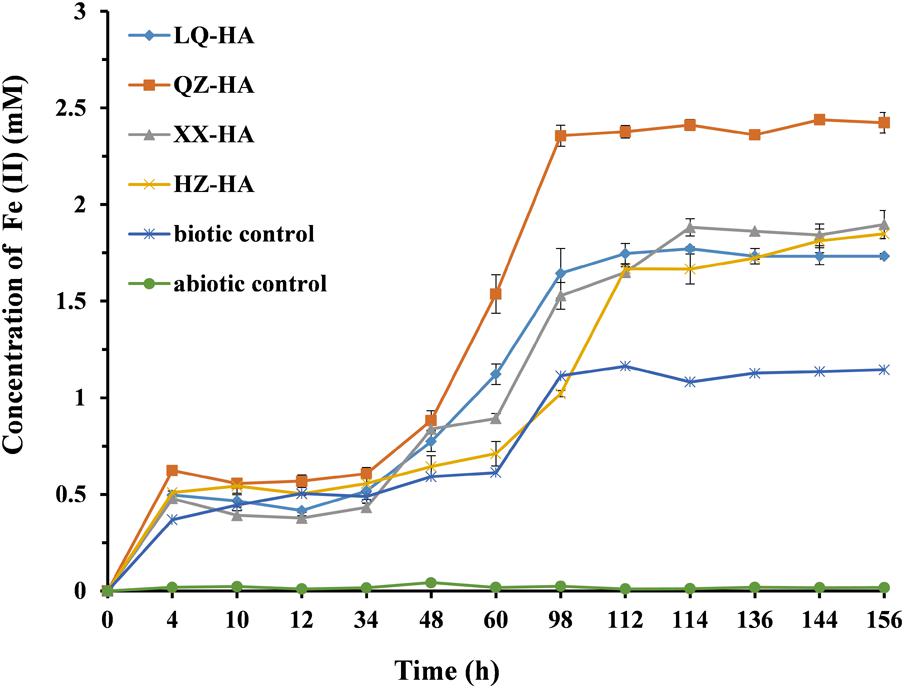
FIGURE 5. Production of total Fe(II) during the microbial reduction of FeOOH conducted by MR-1 in the presence of HAs of different origins (LQ-HA, XX-HA, QZ-HA, and HZ-HA) within 156 h. Data show the mean values of triplicate cultures, and vertical bars show the difference in triplicate cultures.
Discussion
Herein, we proved that HAs obtained from four different sediments (LQ-HA, XX-HA, QZ-HA, and HZ-HA) could stably enhance the microbial reduction of nitrate and amorphous FeOOH, with the best performance observed for QZ-HA. The results of elemental analysis, CV, FTIR spectroscopy, and ESR characterization of HAs were in agreement with each other.
Humic acids isolated from sediments of different origins exhibited variable yields and elemental compositions, in agreement with the fact that HSs of different origin show heterogeneity in their compositional and structural characteristics (Thomsen et al., 2002). The properties of HAs in sediments depend on microbial activity, sediment texture, environment productivity, and the extent of bioturbation and accumulation influence on surface sediments (Aiken et al., 1985). Considering atomic ratios, the data in Table 1 show that the C/N, H/C, and O/C ratio differs widely for different HAs. The C/N, H/C, and O/C are often used to identify HSs from different sources and to follow their structure changes in different environment (Steelink, 1985; He et al., 2008). The C/N ratio depends on the sources of HSs in natural systems, e.g., nonvascular aquatic plants exhibit high C/N ratios, typically between 2.0 and 10.0, whereas vascular terrestrial plants feature C/N ratios of 20 and higher (He et al., 2008), the C/N ratios in Table 1 ranged from 12.88 to 16.16, indicating that these HSs originated primarily from aquatic plants. The determined C/N values agreed with literature data (7.9–16.2) for sedimentary HAs (Filip et al., 1988), equaling 16.16 for QZ-HA and 12.88 for XX-HA. High C/N ratios also suggest a high degree of condensation, high stability, and an extended degree of organic matter humification (Lu et al., 2000), additionally indicating the predominance of polysaccharides (e.g., lignin) humification (Anuradha et al., 2011). QZ-HA, featuring the highest C/N ratio, also showed the highest nitrate and FeOOH reduction extents. The low C contents of four HAs in this study compared to those literatures (Steelink, 1985; Tatzber et al., 2007) might be attributable to the texture of the sediments with high organometallic compounds or other unknown contents as suggested by the obtained results in Table 1 (high ash contents contained in HAs) and in Table 2 (high Fe contents).
The value of H/C ratio can also be considered as a source indicator of organic matter. The H/C values in all HAs are greater than one, which supports the findings of previous studies on HAs (Guo et al., 2018), reflecting that these HSs probably originate from algal or organic matter rather than vascular plant material (Bourbonniere and Meyers, 1983). The O/C ratio is considered as an indicator of oxygen-containing functional group (e.g., carboxylic acid) of HSs, high O/C ratio indicates high carboxyl-C and carbonyl-C composition (Lu et al., 2000). QZ-HA, featuring the highest O/C ratio, may contain the highest oxygen-containing functional group, which contributed to the highest nitrate and FeOOH reduction extents.
The metal content analysis of HAs showed that the level of Fe was positively correlated with extents of DNRA and amorphous FeOOH reduction. QZ-HA showed the best performance in these reactions, which indicated that the inherent redox functionalities of HAs from Qi Zhen lake sediment may be more active and can more effectively facilitate the electron-mediating process for microbial reduction. LQ-HA featured the lowest reduction extent and contained the lowest amount of Fe (9.82 g kg-1 sample). Previously, an iron-rich HS was shown to exhibit a stable electron-mediating ability for the microbial reductive dechlorination of pentachlorophenol under anaerobic conditions (Zhang and Katayama, 2012), and a complex synthetized from HA and FeSO4 was also shown to exhibit stable redox activity (Zhang et al., 2014). Moreover, immobilized Fe–HA complexes were also demonstrated to act as RMs for iopromide removal from anaerobic sludge (Cruz-Zavala et al., 2016). Therefore, the high Fe content of QZ-HA might contribute to its performance as a RM.
Cyclic voltammetry analyses (Figure 1) showed that all HA samples possessed redox-active moieties and exhibited redox potentials between -0.36 and -0.28 V vs. SHE, demonstrating that these moieties belong to the same group despite their heterogeneous properties. The response of quinone moieties on Pt electrodes is known to be improved in DMSO due to its strong chemisorption on Pt (Nurmi and Tratnyek, 2002). Therefore, the improved CV signals of HAs in DMSO were attributed to the presence of quinone-like structures, which have been extensively documented as redox-active moieties (Ratasuk and Nanny, 2007; Uchimiya and Stone, 2009).
Fourier transform infrared analysis revealed that all investigated HAs exhibited similar spectra with different absorbance intensities, indicating that they possessed similar structures and contained the same classes of functional groups regardless of their origin (Figure 2). Notably, among all samples, the pronounced band around 1650 cm-1, which was ascribed to C=C stretches of aromatic rings, C=O stretches of amide groups (amide I band) and C=O of quinone ketones, and asymmetrical C–O stretches of carboxyl groups, the bands from 870 to 700 cm-1 could be attributed to aromatic C–H. These groups are commonly observed for HAs (Senesi et al., 2003; Hesse et al., 2005), they have been reported to be redox-active and might be involved in the action of HAs as RMs (Zhang et al., 2014; Guo et al., 2018). The increase in ESR signals at different pH suggested the presence of semiquinone-type radicals (Scott et al., 1998; Paul et al., 2006; Roden et al., 2010).
Shewanella oneidensis is a model dissimilatory iron-reducing bacterium which plays an important role in the biogeochemical cycling of elements and bioremediation because of its diverse respiratory capabilities. The results in Figures 4, 5 together with Supplementary Figures S1, S2 in the Supplementary Material provide the evidence that dissimilatory nitrate reduction and iron reduction in a pure culture of S. oneidensis strain MR1 were enhanced by HAs. During the DNRA process enhanced by HAs, ammonium which is more bio-available was produced, and nitrite accumulation was not observed, this may be beneficial for reducing nitrate leaching and N2O emissions in aquatic ecosystems (Rütting et al., 2011). Therefore, HAs in sediments enhanced the recycling of nitrogen used to form ammonium, which also represents in situ remediation ability in aquatic ecosystems through microbial redox reactions (Guo et al., 2018). Humic RMs were also reported for the enhancement of denitrification (Xu et al., 2015; Xiao et al., 2016), further study should also be carried out to investigate the effects of HSs as an RM on other microbial metabolic routes in the nitrogen cycle (i.e., nitrogen fixation and ammoniation, which will be helpful for further understanding on the influence of HSs to the total nitrogen cycle in aquatic ecosystems through microbial metabolisms).
Dissimilatory reduction of FeOOH, one of the most predominant terminal electron acceptors of extracellular respiration, plays an important role in the iron biogeochemical processes (Lovley, 1993), and influences the fate of various inorganic and organic environmental pollutants (Li et al., 2010; Zhou et al., 2016). Fe(III) was used as an electron acceptor during dissimilatory iron reduction by iron-reducing bacterium, the produced Fe(II) could couple the oxidation of organic pollutants (i.e., DDT) (Li et al., 2010) and heavy metals [i.e., Mn(IV), Cr(VI), and Hg(II)] (Richter et al., 2012), then organic pollutants were degraded and heavy metals were reduced to a lower valence state or co-precipitated with Fe(II). Notably, the presence of HAs greatly accelerate degradation of organic pollutants and the detoxification of toxic metals, thereby reducing their toxicity. HAs were suggested to be versatile RM, with quinone considered as main redox active groups, which could donate electrons to iron-reducing bacterium utilizing different terminal electron acceptors in microbial respiration (Wolf et al., 2009; Zhang et al., 2014; Wang et al., 2017; Guo et al., 2018).
The above characterization of HAs in this study suggested that the quinone structure in the organic fraction of HAs functioned as RM for the reduction of nitrate and FeOOH, this is consistent with the previous studies (Roden et al., 2010; Zhang C. et al., 2015; Wang et al., 2017). Contributions of other types of electron-mediating moieties such as metal-organic complexes (i.e., ferriporphyrin) could not be excluded, considering the large proportion of Fe. Further study is required to explore the electron-mediating nature of HAs.
Conclusion
All HAs extracted from different sediments were shown to enhance the microbial reduction of nitrate and FeOOH by S. oneidensis MR-1, with both reactions best promoted by QZ-HA, characterized by high C/N, O/C, and Fe ratios. CV analyses confirmed the presence of redox-active moieties in all HAs, and FTIR spectra suggested the aromatic C=C, C–H, and C=O of quinone ketones group in HAs, while ESR spectra verified that quinone moieties within HAs are the redox-active centers. Although the proportion of redox-active carbon is very small, the potential electron-mediating ability is not negligible. This study provided deeper understanding on the role of HAs participating in various biogeochemical processes (i.e., iron cycle, nitrogen cycle, etc.), which also hold great promise for the in situ bioremediation.
Author Contributions
C-FZ and D-DZ conceived and designed the study, and critically revised the manuscript. NZ performed the experiments, analyzed the data, and drafted the manuscript, H-DJ, X-WY, Z-CZ, and S-MY contributed reagents/materials/instruments, study implementation, and manuscript revision. All authors read and approved the final manuscript.
Funding
This work was supported by the Natural Science Foundation of China (Grant Nos. 31400096 and 41701346) and the Fundamental Research Funds for the Central Universities (Grant No. 2017QNA4045).
Conflict of Interest Statement
The authors declare that the research was conducted in the absence of any commercial or financial relationships that could be construed as a potential conflict of interest.
Supplementary Material
The Supplementary Material for this article can be found online at: https://www.frontiersin.org/articles/10.3389/fmicb.2018.01225/full#supplementary-material
References
Aiken, G. R., McKnight, D. M., Wershaw, R. L., and Maccarthy, P. (1985). Humic Substances in Soil, Sediment, and Water: Geochemistry, Isolation and Characterization. New York: John Wiley & Sons.
Anuradha, V., Nair, S., and Kumar, N. (2011). Humic acids from the sediments of three ecologically different estuarine systems-a comparison. Int. J. Environ. Sci. 2, 174–184. doi: 10.6088/ijes.00202010018
Bourbonniere, R. A., and Meyers, P. A. (1983). Characterization of sedimentary humic matter by alkaline hydrolysis. Org. Geochem. 5, 131–142. doi: 10.1016/0146-6380(83)90023-2
Cawse, P. (1967). The determination of nitrate in soil solutions by ultraviolet spectrophotometry. Analyst 92, 311–315. doi: 10.1039/AN9679200311
Costa, M. C., Mota, S., Nascimento, R. F., and Santos, A. B. D. (2010). Anthraquinone-2,6-disulfonate (AQDS) as a catalyst to enhance the reductive decolourisation of the azo dyes reactive red 2 and congo red under anaerobic conditions. Bioresour. Technol. 101, 105–110. doi: 10.1016/j.biortech.2009.08.015
Cruz-Zavala, A. S., Pat-Espadas, A. M., Rangel-Mendez, J. R., Chazaro-Ruiz, L. F., Ascacio-Valdes, J. A., Aguilar, C. N., et al. (2016). Immobilization of metal–humic acid complexes in anaerobic granular sludge for their application as solid-phase redox mediators in the biotransformation of iopromide in UASB reactors. Bioresour. Technol. 207, 39–45. doi: 10.1016/j.biortech.2016.01.125
Filip, Z., Alberts, J. J., Cheshire, M. V., Goodman, B. A., and Bacon, J. R. (1988). Comparison of salt marsh humic acid with humiclike substances from the indigenous plant species Spartina alterniflora (Loisel). Sci. Total Environ. 71, 157–172. doi: 10.1016/0048-9697(88)90164-7
Francioso, O., Ciavatta, C., Gessa, C., Tugnoli, V., and Sanchez-Cortes, S. (1998). Spectroscopic characterization of pyrophosphate incorporation during extraction of peat humic acids. Soil Sci. Soc. Am. J. 62, 181–187. doi: 10.2136/sssaj1998.03615995006200010024x
Gu, B., and Chen, J. (2003). Enhanced microbial reduction of Cr(VI) and U(VI) by different natural organic matter fractions. Geochim. Cosmochim. Acta 67, 3575–3582. doi: 10.1016/S0016-7037(03)00162-5
Guo, P., Zhang, C., Wang, Y., Yu, X., Zhang, Z., and Zhang, D. (2018). Effect of long-term fertilization on humic redox mediators in multiple microbial redox reactions. Environ. Pollut. 234, 107–114. doi: 10.1016/j.envpol.2017.10.106
He, M. C., Shi, Y. H., and Lin, C. Y. (2008). Characterization of humic acids extracted from the sediments of the various rivers and lakes in China. J. Environ. Sci. 20, 1294–1299. doi: 10.1016/S1001-0742(08)62224-X
Hesse, M., Meier, H., and Zeeh, B. (2005). Spektroskopische Methoden in der Organischen Chemie. Stuttgart: Thieme. doi: 10.1055/b-002-46985
Jones, D. R., Jansheski, W. C., and Goldman, D. S. (1981). Spectrophotometric determination of reduced and total iron in glass with 1, 10-phenanthroline. Anal. Chem. 53, 923–924. doi: 10.1021/ac00229a049
Lee, Y. P., and Takahashi, T. (1966). An improved colorimetric determination of amino acids with the use of ninhydrin. Anal. Biochem. 14, 71–77. doi: 10.1016/0003-2697(66)90057-1
Li, F., Li, X., Zhou, S., Zhuang, L., Cao, F., Huang, D., et al. (2010). Enhanced reductive dechlorination of DDT in an anaerobic system of dissimilatory iron-reducing bacteria and iron oxide. Environ. Pollut. 158, 1733–1740. doi: 10.1016/j.envpol.2009.11.020
Lovley, D. R. (1993). Dissimilatory metal reduction. Annu. Rev. Microbiol. 47, 263–290. doi: 10.1146/annurev.mi.47.100193.001403
Lovley, D. R., Coates, J. D., BluntHarris, E. L., Phillips, E. J. P., and Woodward, J. C. (1996). Humic substances as electron acceptors for microbial respiration. Nature 382, 445–448. doi: 10.1038/382445a0
Lu, X., Hanna, J., and Johnson, W. (2000). Source indicators of humic substances: an elemental composition, solid state 13 C CP/MAS NMR and Py-GC/MS study. Appl. Geochem. 15, 1019–1033. doi: 10.1016/S0883-2927(99)00103-1
Nurmi, J. T., and Tratnyek, P. G. (2002). Electrochemical properties of natural organic matter (NOM), fractions of NOM, and model biogeochemical electron shuttles. Environ. Sci. Technol. 36, 617–624. doi: 10.1021/es0110731
Paul, A., Stosser, R., Zehl, A., Zwirnmann, E., Vogt, R. D., and Steinberg, C. E. W. (2006). Nature and abundance of organic radicals in natural organic matter: effect of pH and irradiation. Environ. Sci. Technol. 40, 5897–5903. doi: 10.1021/es060742d
Ratasuk, N., and Nanny, M. A. (2007). Characterization and quantification of reversible redox sites in humic substances. Environ. Sci. Technol. 41, 7844–7850. doi: 10.1021/es071389u
Richter, K., Schicklberger, M., and Gescher, J. (2012). Dissimilatory reduction of extracellular electron acceptors in anaerobic respiration. Appl. Environ. Microbiol. 78, 913–921. doi: 10.1128/AEM.06803-11
Roden, E. E., Kappler, A., Bauer, I., Jiang, J., Paul, A., Stoesser, R., et al. (2010). Extracellular electron transfer through microbial reduction of solid-phase humic substances. Nat. Geosci. 3, 417–421. doi: 10.1038/ngeo870
Rütting, T., Boeckx, P., Müller, C., and Klemedtsson, L. (2011). Assessment of the importance of dissimilatory nitrate reduction to ammonium for the terrestrial nitrogen cycle. Biogeosciences 8, 1779–1791. doi: 10.5194/bg-8-1779-2011
Sawyer, D. T., Sobkowiak, A., and Roberts, J. L. (1995). Electrochemistry for Chemists. New York, NY: John Wiley & Sons.
Schmidt, M., Knicker, H., Hatcher, P. G., and Kogel-Knabner, I. (1997). Improvement of 13C and 15N CPMAS NMR spectra of bulk soils, particle size fractions and organic material by treatment with 10% hydrofluoric acid. Eur. J. Soil Sci. 48, 319–328. doi: 10.1111/j.1365-2389.1997.tb00552.x
Scott, D. T., McKnight, D. M., Blunt-Harris, E. L., Kolesar, S. E., and Lovley, D. R. (1998). Quinone moieties act as electron acceptors in the reduction of humic substances by humics-reducing microorganisms. Environ. Sci. Technol. 32, 2984–2989. doi: 10.1021/es980272q
Senesi, N., D’Orazio, V., and Ricca, G. (2003). Humic acids in the first generation of eurosoils. Geoderma 116, 325–344. doi: 10.1016/S0016-7061(03)00107-1
Steelink, C. (1985). “Implications of elemental characteristics of humic substances,” in Humic Substances in Soil, Sediment, and Water, eds G. R. Aiken, D. M. McKnight, R. L. Wershow, and P. MacCarthy (New York, NY: John Wiley & Sons).
Tatzber, M., Stemmer, M., and Spiegel, H. (2007). FTIR-spectroscopic characterization of humic acids and humin fractions obtained by advanced NaOH, Na4P2O7, and Na2CO3 extraction procedures. J. Plant Nutr. Soil. Sci. 170, 522–529. doi: 10.1002/jpln.200622082
Thomsen, M., Lassen, P., Dobel, S., Hansen, P. E., Carlsen, L., and Mogensen, B. B. (2002). Characterisation of humic materials of different origin: a multivariate approach for quantifying the latent properties of dissolved organic matter. Chemosphere 49, 1327–1337. doi: 10.1016/S0045-6535(02)00335-1
Uchimiya, M., and Stone, A. T. (2009). Reversible redox chemistry of quinones: impact on biogeochemical cycles. Chemosphere 77, 451–458. doi: 10.1016/j.chemosphere.2009.07.025
Wang, X.-N., Sun, G.-X., Li, X.-M., Clarke, T. A., and Zhu, Y.-G. (2017). Electron shuttle-mediated microbial Fe (III) reduction under alkaline conditions. J. Soil Sediment. 18, 159–168. doi: 10.1007/s11368-017-1736-y
Wolf, M., Kappler, A., Jiang, J., and Meckenstock, R. U. (2009). Effects of humic substances and quinones at low concentrations on ferrihydrite reduction by Geobacter metallireducens. Environ. Sci. Technol. 43, 5679–5685. doi: 10.1021/es803647r
Xiao, Z., Awata, T., Zhang, D., Zhang, C., Li, Z., and Katayama, A. (2016). Enhanced denitrification of Pseudomonas stutzeri by a bioelectrochemical system assisted with solid-phase humin. J. Biosci. Bioeng. 122, 85–91. doi: 10.1016/j.jbiosc.2015.11.004
Xu, Q., Guo, J., Niu, C., Lian, J., Hou, Z., Guo, Y., et al. (2015). The denitrification characteristics of novel functional biocarriers immobilised by non-dissolved redox mediators. Biochem. Eng. J. 95, 98–103. doi: 10.1016/j.bej.2014.12.004
Zhang, C., and Katayama, A. (2012). Humin as an electron mediator for microbial reductive dehalogenation. Environ. Sci. Technol. 46, 6575–6583. doi: 10.1021/es3002025
Zhang, C., Zhang, D., Li, Z., Akatsuka, T., Yang, S., Suzuki, D., et al. (2014). Insoluble Fe-humic acid complex as a solid-phase electron mediator for microbial reductive dechlorination. Environ. Sci. Technol. 48, 6318–6325. doi: 10.1021/es501056n
Zhang, C., Zhang, D., Xiao, Z., Li, Z., Suzuki, D., and Katayama, A. (2015). Characterization of humins from different natural sources and the effect on microbial reductive dechlorination of pentachlorophenol. Chemosphere 131, 110–116. doi: 10.1016/j.chemosphere.2015.02.043
Zhang, D. D., Zhang, C. F., Xiao, Z. X., Suzuki, D., and Katayama, A. (2015). Humin as an electron donor for enhancement of multiple microbial reduction reactions with different redox potentials in a consortium. J. Biosci. Bioeng. 119, 188–194. doi: 10.1016/j.jbiosc.2014.07.010
Zhang, D., Zhang, N., Yu, X., Zhang, Z., Yang, S., and Zhang, C. (2017). Effect of humins from different sediments on microbial degradation of 2,2’,4,4’,5,5’-hexachlorobiphenyl (pcb153), and their polyphasic characterization. RSC Adv. 7, 6849–6855. doi: 10.1039/C6RA25934K
Keywords: humic acid, electron mediator, elemental composition, spectroscopic analysis, nitrate reduction, FeOOH reduction, redox activity
Citation: Zhang N, Zhang D-D, Ji H-D, Yu X-W, Zhang Z-C, Yang S-M and Zhang C-F (2018) Redox Structures of Humic Acids Derived From Different Sediments and Their Effects on Microbial Reduction Reactions. Front. Microbiol. 9:1225. doi: 10.3389/fmicb.2018.01225
Received: 24 November 2017; Accepted: 22 May 2018;
Published: 08 June 2018.
Edited by:
Qiang Wang, Institute of Hydrobiology (CAS), ChinaReviewed by:
Miguel Angel Sanchez-Monedero, Consejo Superior de Investigaciones Científicas (CSIC), SpainMichael R. Twiss, Clarkson University, United States
Copyright © 2018 Zhang, Zhang, Ji, Yu, Zhang, Yang and Zhang. This is an open-access article distributed under the terms of the Creative Commons Attribution License (CC BY). The use, distribution or reproduction in other forums is permitted, provided the original author(s) and the copyright owner are credited and that the original publication in this journal is cited, in accordance with accepted academic practice. No use, distribution or reproduction is permitted which does not comply with these terms.
*Correspondence: Chun-Fang Zhang, zhangcf@zju.edu.cn
†These authors have contributed equally to this work.