- Department of Plant protection, Faculty of Agriculture, Bu-Ali Sina University, Hamedan, Iran
Endophytic fungi have been recognized as a potential source of bioactive secondary metabolites. The endophytic Trichoderma species were isolated from Vinca plants (Vinca major, Vinca herbacea, and Vinca minor), found in Iran and screened for antimicrobial and anti-proliferative activity. Based on morphological and phylogenetic analyses, four fungal species were identified: T. asperellum, T. brevicompactum, T. koningiopsis, and T. longibrachiatum. In addition, endophytic fungi bioactivity of methanol and ethyl acetate extracts (7.8–250 μgml−1) were assessed against a panel of pathogenic fungi and bacteria and IC80 was calculated. Data showed that both methanol and ethyl acetate extracts from all endophytic isolates had significant cytotoxic effects against the model target fungus Pyricularia oryzae. Further research indicated that they had significant antimicrobial bioactivity against the human pathogenic bacteria Staphylococcus aureus and Escherichia coli, and plant pathogenic bacteria Ralstonia solanacearum and Clavibacter michiganensis as well. According to the bioactivity results, crude ethyl acetate extract of T. koningiopsis VM115 isolate was determined for TLC and GC-MS analysis. An antifungal compound was isolated from ethyl acetate extract of T. koningiopsis VM115 based on bioassay guided fractionation. The 1H-NMR and 13C-NMR spectroscopic data showed that the compound was trichodermin, which exhibited strong fungicidal effects against P. oryzae, Aspergillus fumigatus, and Botrytis cinera with MICs of 31.25 μg ml−1 through in vitro antifungal tests. GC-MS analysis identified six classes of volatile compound produced by T. koningiopsis VM115 (alcohols, esters, pyrones (lactones), acids, furanes and lipids). 6-n-pentyl-6H-pyran-2-one (6PP) was identified as one of the most abundant metabolites in this research. These results indicate that the fungal endophytes from Vinca plants had antibacterial and cytotoxic activities; evidence that endophytes are a good source of biological activity and compounds. This work is the first report of Trichodermin production by T. koningiopsis species.
Introduction
Although a large number of secondary metabolites are produced by endophytic fungi, which are non-essential, this can serve as an ecological advantage to them in certain environments. Pharmaceutically useful compounds, pigments, plant growth regulators, and mycotoxins are included in the mentioned metabolites (Keller et al., 2005). Thus, identification of these components and optimization of fungal growth conditions can help to achieve maximum production of secondary metabolites. The fungal genus Trichoderma contains some of the most potent biocontrol agents in use today (Harman et al., 2004; Ming et al., 2012). Moreover, some of its taxa have been shown to occur as endophytes, especially in tropical arboreous vegetation, the strains of which have often high antagonistic activities against their pathogens (Fravel, 1988). Trichoderma species are generally regarded as saprophytic fungi; as such they have minimal nutritional needs and produce and secrete a plethora of secondary metabolites into their vicinity. Though these compounds often appear in an organism with obscure or unknown functions, they can have medical, agricultural, and industrial applications, thus being important for humans. Trichoderma spp. displays antimicrobial activity against many important bacteria, yeasts, and filamentous fungi (Vizcaíno et al., 2005), in which numerous and varied secondary metabolites, such as peptaibols, gliotoxin, gliovirin, polyketides, pyrones, and terpenes may be involved (Schnürer et al., 1999; Vinale et al., 2008). Structural consideration of the Trichoderma antibiotic molecules present in nature has identified two main types (Sivasithamparam and Ghisalberti, 1998; Reino et al., 2008): volatile metabolites and low molecular weights like simple aromatic compounds, some polyketides including pyrones, isocyanates, butenolides, and volatile terpenes, all of which are rather non-polar substances of considerable vapor pressures; and polar metabolites of high molecular weights, which may induce direct interactions between Trichoderma spp. and their antagonists in the same way as gliovirin, peptaibols, and diketopiperazine-like gliotoxin. As a natural product, terpenes constitute the largest group of secondary metabolites with important pharmacological activities such as antiviral, antibacterial, antimalarial, and anti-inflammatory actions, inhibition of cholesterol synthesis, and anticancer activity. A large series of these compounds are produced by the filamentous fungi like Trichoderma (Degenkolb et al., 2008; Reino et al., 2008; Korpi et al., 2009; Cardoza et al., 2011; Mukherjee et al., 2012). Sesquiterpenes from Trichoderma have demonstrated antibacterial, antifungal and neuroleptic activities (Bennett and Klich, 2003). One particular group of sesquiterpenes includes fungal toxins known as trichothecenes. There are different species of Trichoderma producing bioactive compound that act as a mycotoxin such as Trichothecene. It is a sesquiterpenoid derived secondary metabolite synthesized mainly by Fusarium, other fungal genera such as Trichoderma, Trichothecium, Stachybotrys (Wilkins et al., 2003; Shentu et al., 2014; Frisvad and Thrane, 2004). It is known that these sesquiterpenoid compounds are also harmful to plants and animals that feed on infected fodder. Also, Trichothecenes in Trichoderma, trichodermin, and harzianum A, have been reported by only some species (T. arundinaceum and T. brevicompactum), (Corley et al., 1994; Cardoza et al., 2011). Trichodermin displays antifungal and anti-yeast activities as well as phytotoxicity (Nielsen, 2003; Rocha et al., 2005; Jin et al., 2007; Tijerino et al., 2011). Reino et al. (2008) have reviewed many volatile secondary metabolites that can be potentially produced by Trichoderma spp. Volatile secondary metabolites have demonstrated a key role in mycoparasitism of Trichoderma and its interaction with plants (Vinale et al., 2008). Trichoderma species are known to produce more than 40 different metabolites besides many important secondary metabolites like mycotoxins, which induce antimicrobial activities (Sivasithamparam and Ghisalberti, 1998). As a well-described volatile product, 6-pentyl-a-pyrone (6-PAP) represents antimicrobial and herbicidal activities through a secondary metabolism in Trichoderma (Galindo et al., 2004). These metabolites have been utilized in different biological processes, including the bio-control of microorganisms with their living environments. They can be produced to induce a competition between species by mediating resistance against predators, parasites, and diseases, and facilitating reproductive processes (Sivasithamparam and Ghisalberti, 1998).
About 7 species originate from Vinca (Apocynaceae) genus worldwide. It has been represented by Vinca herbacea Waldst and Kit as a native plant with two other cultivated species, Vinca minor L. and Vinca major L., in Iran (Rechinger, 1974; Mozaffarian, 2006). In central and southern Europe, as well as southwest of Asia, V. minor L. (lesser periwinkle) has been a native plant, while being cultivated in the US and other countries as a ground cover. V. major, with common names such as big leaf periwinkle, large periwinkle, greater periwinkle, and blue periwinkle; it is a flowering plant species native to western Mediterranean. V. herbacea, which is commonly called herbaceous periwinkle, is a native plant capable of flowering in the east and southeast parts of Europe. It is distributed from Austria toward Greece at the south and Crimea at the east; it is also found in north western Asia, in the Caucasus and Alborz mountain ranges. These plants have been traditionally applied worldwide to treat various ailments throughout the ages. The plants bear active phyto constituents and exhibit varied pharmacological activities such as anti-cancer, anti-diabetic, anti-oxidant, anti-hypertensive, anti-microbial, and cytotoxic activities (Kral, 2012).
The goal of this study was to screen for antimicrobial and anti-proliferative activity in endophytic fungi of Trichoderma species isolated from the surface of sterilized leaves and stems of three Vinca plants found in Iran; V. major, V. herbacea, and V. minor. Tests were done for significant anti-proliferative and antibacterial capabilities of endophytic Trichoderma species from Vinca plants. The aim was to identify secondary metabolites produced by superior isolates, using thin layer chromatography (TLC), gas chromatography combined with mass spectrometry (GC-MS), MS, 1H-NMR (Nuclear Magnetic Resonance), 13C-NMR. Being identified as trichodermin by using spectroscopic data, antifungal compounds were isolated through bioassay-guided fractionation.
Materials and Methods
General Procedures Based on Experiments
Column Chromatographies (CCs) of silica gel G (200–300 mesh, Merck.) and Sephadex LH-20 (Merck.) and TLC of silica gel GF254 (10–40 lm, Merck.) were performed. Distillation of all the solvents was done before use. NMR spectra and ESI-MS were obtained using a spectrometer (Bruker AM-400) and Finnigan LCQ-Advantage (m/z), respectively. The chemical shifts d (ppm) rel. to Me4Si, and coupling constants J (Hz.) were then conducted. All the other chemicals under study were of analytical grades.
Sampled Locations
Samples were collected from five distinct provinces of Iran, representing the versatility of the country, i.e., Esfahan (South of Iran), Mazandaran (North of Iran), Hamedan (West of Iran), Tehran (Center of Iran). Plant temple (stem, leaf) were collected from three species V. major, V. herbacea, and V. minor as listed in Table 1.
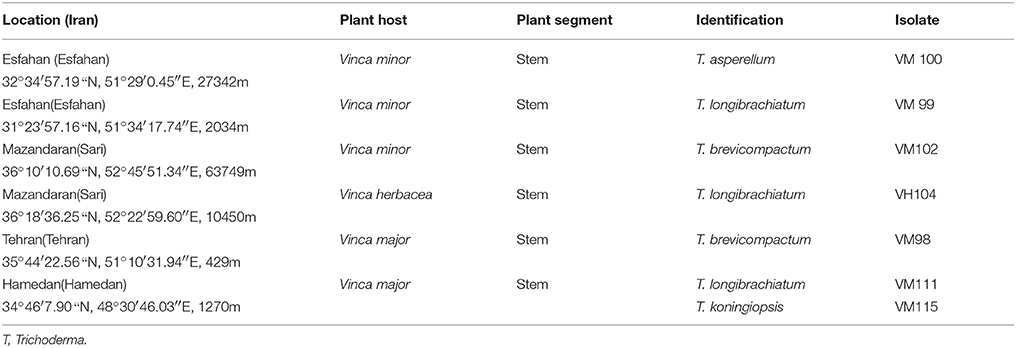
Table 1. Endophytic Trichoderma species isolates from each Vinca host plant species, according to sampling sites, plant tissue and fungal isolate.
Recovery and Identification of Endophytic Fungi
Fresh tissue was collected from Apocynaceae, in each locality from June to October, 2014. From each plant specimen, three randomly selected pieces were surface sterilized and in total 700 plant pieces (~0.5 × 0.5 × 1 cm from inner layers) were incubated on potato dextrose agar (PDA) and water agar (WA) culture media, at 26–28°C, for 2–12 weeks. Hyphal tips were isolated, purified, and maintained at 4°C. The endophytic isolates were identified by investigating their colony morphology and the mechanism of spore production on PCA (Potato Carrot Agar), after 7 days at 22–25°C, under 16/8-h light intervals. Fungal specimen was stained and studied under microscope, according to the reference (Bissett, 1984, 1991a,b, 1992; Gams and Bissett, 1998).
Identification Based on Molecular and Morphological Features
The cultures were grown on 2% Malt Extract Agar (MEA) and Potato Dextrose Agar (PDA) at 20°C under an ambient daylight condition or in a light/dark cycle of 12 h/12 h under fluorescent and near-UV light. To determine linear growth rates, fresh mycelial plugs were placed near the edges of PDA plates with a diameter of 9 cm and incubated at 20°C. The colony radius was measured at an interval of 24 h. Using the preparations performed in lactic acid, microscopic observations and measurements were conducted. Following maturing of the conidia within 4–7 days of incubation, observation of the structures and morphologies of macronematous conidiophores taken from the edges of conidiogenous fascicles or pustules was made. After 14 days, conidial morphology was recorded and measurements were done. Based on a comparison with the keys and descriptions presented in recent taxonomic literature, morphologies of the preliminary species were identified through observation (Bissett, 1984, 1991a,b,c, 1992; Gams and Bissett, 1998; Hoyos-Carvajal et al., 2009). Molecular identification was based on the fungal isolate growth in the test tubes containing PDB media at 28°C for 7 days. Using CinnaPure-DNA (Sinaclon, Iran), the genomic DNA was extracted after harvesting the mycelium. A region of nuclear rDNA, containing the internal transcribed spacer regions 1 and 2 and the 5.8S rDNA gene region and a fragment of tef1 was amplified by polymerase chain reaction (PCR) using the primer pair ITS1 (5′-TCCGTAGGTGAACCTGCGG-3′) and ITS4 (5′-TCCTCCGCTTA TTGATATGC-3′) and the primer pair tef1 fw (5′-GTGAGCGTGGTATCACCATCG-3′) and tef1 rev (5′-GCCATCCTTGGAGACCAGC-3′) was done by Kraus et al. (2004). The PCR products were sent to the Macrogen sequencing service (Macrogen Inc. Seoul, Korea) for direct sequencing of double strands of DNA.
Phylogenetic Analyses
Sequences were checked with BioEdit v. 7.0.9.0 (Hall, 2006). The ITS and EF1-α sequences of outgroup (Hypomyces subiculosus TFC 97-166) and additional isolate was retrieved from GenBank. Sequences were aligned with MUSCLE (Edgar, 2004). Manual adjustments were done if necessary after checking the alignments. Using the simple indel-coding implemented by GapCoder, the phylogenetic analyses were performed based on the phylogenetic information contained in the indels (gaps) (Young and Healy, 2003). Phylogenetic analyses were performed with PAUP v. 4.0b10 (Swofford, 2003) for neighbor-joining (NJ) and maximum-parsimony (MP) analyses as described by Abdollahzadeh et al. (2010, 2014). Bootstrap analysis was done with 1,000 replicates. The general time-reversible model of evolution (Rodriguez et al., 1990), including estimation of invariable sites and assuming a discrete gamma distribution with six rate categories (GTR+I+Γ) was used. A partition homogeneity test (PHT) was used to determine the congruence between the ITS and EF1-α datasets (Farris et al., 1995; Huelsenbeck et al., 1996; Abdollahzadeh et al., 2014). New sequences were deposited in GenBank (Table 2).
Metabolite Extraction
To cultivate the fungi on PDB, the selected endophyte cultures were inoculated in 250 mL Erlenmeyer flasks containing 100 mL of the medium. Each flask was incubated at 28°C for 2 weeks with periodical shaking at 150 rpm. After the incubation period, the fungal fermentation broth was homogenized by addition of 10% methanol. Methanol and ethyl acetate were used as organic solvents to extract the metabolite via the solvent extraction procedure. An equal volume of solvent was added to the filtrate, mixed well for 10 min and kept for 5 min until the two clear immiscible layers were formed. By using a separating funnel, separation of the upper layer of the solvent, which contained the extracted compounds, was done. To obtain the crude metabolite, the compound yielded by evaporating the solvent was dried in a rotator evaporator under vacuum (Bhardwaj et al., 2015; Sharma et al., 2016). A brown gum was presented after evaporation of the combined ethyl acetate and methanol extracts. The crude extract was then dissolved in dimethyl sulphoxide at 1 mgmL−1of concentration and was stored at 4°C for 24 h before injecting in to GC-MS and the secondary metabolites were kept at −20°C until they were needed for bioassays.
Anti-proliferative Activity
Anti-proliferative and cytotoxic bioactivity of methanol and ethyl acetate extracts was determined against the conidial germination of P. oryzae, as a model. P. oryzae conidial suspension (4 × 104 mL−1; 50 μL including 0.02% yeast extract) was seeded into each well of the 96-well microtiter plates. To obtain the final concentrations of 250, 125, 62.5, 31.25, 15.62, and 7.81 μg mL−1, each well received 50 μL of the sample extract in a serially dilution manner. The assay plates were incubated at 28°C for 16 h. For each sample extract, a microscopic observation was done on the germinations and sizes of the germ tubes that originated from the 75 conidia and the results were compared with the control group to determine MICs. The experiments were performed in triplicate (Kobayashi et al., 1996).
Cell Viability Assay
For the measurement of cell viability, tetrazolium salt MTT was applied to determine the fungal methanol and ethyl acetate extracts cytotoxicity (μg/mL−1) against P. oryzae conidia(Levitz and Diamond, 1985; Patel et al., 2013). Our methodology was based on a catalyzed reaction of the functional hyphae through hydrogenases, which led to the cleavage of the yellow tetrazolium salt MTT [3-(4,5-dimethylthiazol-2-yl)- 2,5-diphenyltetrazolium bromide] to MTT-formazan as its purple derivative. MTT-formazan can be quantified through spectrophotometry within 550 nm after being dissolved in isopropanol. A 50 μL of the conidial suspension of P. oryzae (7.5 × 104 mL−1) including a 0.02% yeast extract together with a 200-μl aliquot of conidial suspension was poured into each well of a 96-well plate to yield a final concentration of 15,000 conidia/well. An untreated conidial suspension of fungal extract extracted from P. oryzae was used as the control.
Minimal Inhibitory Concentration (MIC) and Minimum Bactericidal Concentration (MBC) Determination
Methanol and ethyl acetate extracts prepared from the endophytes were examined for their antimicrobial activity against Human pathogenic bacteria gram-positive Staphylococcus aureus PTCC (1189) and Gram-negative Escherichia coli PTCC (1399) and Plant pathogenic bacteria Gram-negative Ralstonia solanacearum and Gram-positive Clavibacter michiganensis (laboratory of bacteriology, Bu Ali Sina University). First, bacteria were grown to obtain 1 × 106 CFU mL−1. Then, micro broth dilution assays were performed as described for Anti proliferative Assays, but in nutrient broth (NB) medium. The experimental plates were incubated at 28°C for 16 h for Plant pathogenic bacteria and 37°C for 16 h for Human pathogenic bacteria. The growth of target bacteria was observed and compared with the control to determine the MIC and the minimum bactericidal concentration (MBC). The experiments were performed in triplicate. Upon obtaining the required data, the metabolite concentration required for 80% of in vitro inhibition was presented as IC80 value.
TLC Bioautography of the T. koningiopsis VM115
According to the information provided in Tables 3–5 between methanol and ethyl acetate extracts, crude ethyl acetate extract of T. koningiopsis VM115 isolate was used for TLC and GC-MS analysis. The ethyl acetate extract of each isolated fungus was introduced to silica gel plate (10 × 5 cm) by adding a fluorescent indicator, which was developed by placing it in a paper-linked filter in a glass chromatography tank containing 50 ml of an evenly mixed solution of CHCl3/ MeOH (v/v, 10: 1). As soon as the solvent height was observed to reach 9.5 cm, the plates were removed from the tank. The presence of UV-absorbing compounds was corroborated after seeing dark spots on the dried plates under a UV radiation of 254 nm. The spots were marked with a pencil. Then, using an aerosol spray, a suspension of 106 P. oryzae spores per ml was gently and evenly sprayed onto the plates to make it turn translucent. In a biological hood, the fungal suspension was prepared and sprayed on to the plates using gloves. Washing of the spray bottle with 70% ethanol and sterile distilled water was followed prior to use. The plates were placed in plastic bags in a light box at 28 ± 1°C for a 12-h photoperiod. Within 1 week, the plates were photographed under UV at 254 nm and the inhibition zones were observed as white circular areas of reduced densities or as the fungus non-growth area.
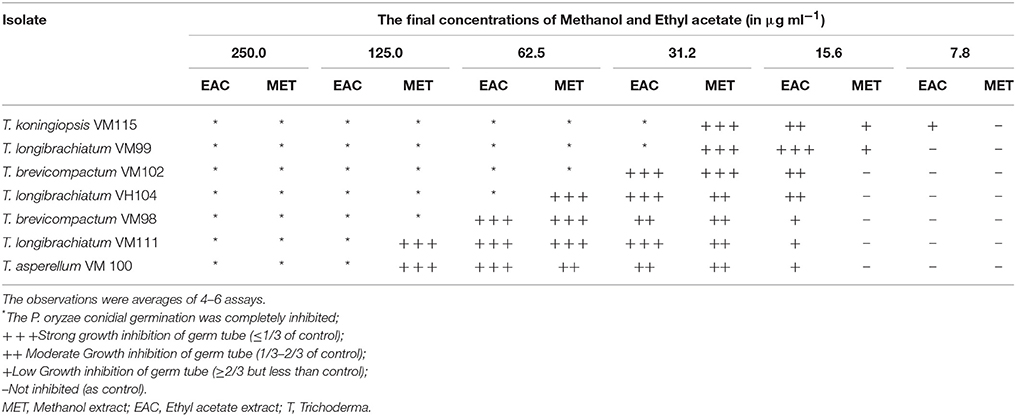
Table 3. Antiproliferative activities of (EAC and MET) metabolites from endophytic Trichoderma species against the conidia of Pyricularia oryzae the observations were averages of 4–6 assays.
Extraction and Fractionation of T. koningiopsis VM115 Culture
After obtained following the process described above, a culture filtrate of T. koningiopsis VM115 was extracted with ethyl acetate. A residue (20.0 g) was produced in vacuo evaporation of the organic solvent from the extract and dissolved in a lower amount (10 ml) of MeOH around 45°C. The resultant liquor was stored at −10°C overnight, followed by filtration to remove any waxy materials such as the precipitate. MeOH removal under reduced pressure provided a brown lump, and MeOH was added to it until it was completely dissolved. Acetone was added to the MeOH solution drop by drop with acetone; finally at 15% (v/v), it was kept overnight below −10°C to precipitate salts and saccharides. The obtained filtrate was concentrated in vacuo to render a residue (13 g), which was then exposed to column chromatography over Si gel column (100 g, 200–300 mesh) eluting with petroleum ether-acetone mixtures (v/v, 1: 0, 20: 1, 10: 1, 5: 1, 3: 1, 0: 1, 800 ml each) to provide five fractions. Fr.2 (1.9 g)active to the test fungi by TLC bioautography was rechromatographed over Si gel column only (50 g, 200–300 mesh), eluting with CHCl3-MeOH gradient (v/v, 100:1, 50:1, 20:1, 10:1, 400 ml each). Fr2 was separated further on a Sephadex LH-20 column, eluting with acetone to achieve eight sub fractions Fr2 (1–8). On a silica gel column (20 g), active Fr2 (3) was then purified and eluted with petroleum ether/ethyl acetate (20:1, v/v) to yield 1.045 g of active compound (1). Compound 1 was identified by spectral analyses including MS, 1H-NMR, 13C-NMR (Liu et al., 2006).
Antifungal Assay of T. koningiopsis VM115 Compound
Antifungal activities of compound 1 were examined in vitro by testing P. oryzae, Botrytis cinera and Aspergillus fumigatus through the method described in the literature (Barchiesi et al., 2000) based on ketoconazole co-assay as a positive reference. Each compound was isolated in triplicate.
GC-MS Conditions of T. koningiopsis VM115
An Agilent technologies 7890A gas chromatograph connected to a 5975 Cinert MSD was used to identify volatile and semi-volatile secondary metabolites from the crude extract of fungi isolate. Also, an HP-5MS fused silica capillary column was applied (Hewlett-Packard, 30 m × 0.25 mm i.d. 0.25 μm film, cross-linked to 5% phenyl methyl siloxane stationary phase). The entire system was checked using Chemstation software (Hewlett-Packard, version A.01.01). Electron impact mass spectra were recorded at 70 eV, and ultra-high pure HAE (99.999%) gas was used as the carrier gas at flow rate of 1 Ml min−1. The injection volume was found to be 1 μL, and all injections were done in a split-less mode. The injector and detector temperature settings were 250 and 280°C, respectively. Column oven temperature was set initially at 50°C for 5 min, then raised to 260°C (ramp: 4°C/min) and held for 5 min. The database of the National Institute of Standards and Technology (NIST) was applied to interpret mass spectrum of GC-MS with more than 62,000 patterns. Using the data obtained from NIST05 (National Institute of Standards and Technology, US), WILEY 8, and FFNSC1.3 (Flavor and Fragrance Natural and Synthetic Compounds) libraries, the existing bioactive compounds in the extracts were identified through a comparison of mass spectra. The molecular weights and structural components of the test materials were determined. Ultimately, most of the compounds of this fungus were identified and verified by comparing the standard GC/MS data with those of the fungal products.
Statistical Analysis
SAS (Ver. 9.1), statistical software was used to compare the means through the test of Least Significant Difference (LSD). The differences between the varied treatments were specified at 5% level (P = 0.05).
Results
Host and Fungi Identity and Phylogeny of Trichoderma Species Isolates
In total, seven endophytic Trichoderma fungal isolates were recovered from six different locations from 500 plant specimens from healthy above ground tissue (leaf, stem) of the Vinca major, Vinca herbacea, and Vinca minor (Apocynaceae family) (Table 1). Endophytes were classified according to morphological traits using the key in Gams and Bissett (1998) (Figures 1–4). By combining the 2 unlinked regions of the genes including ITS/ EF1-α, the phylogenetic analyses were conducted. The internal nodes were observed to highly support the phylogenies that resulted from the stable and reproducible ITS/ EF1-α. Production of the trees with similar topologies was represented by the individual congruent datasets since no significant divergence (P = 0.48) was seen through the partition homogeneity test in PAUP 4.0b10 (Swofford, 2003). Therefore, ITS and EF1-α datasets were combined for analysis. The combined ITS and EF1-α sequences for 17 ingroup and 1 outgroup taxa contained 1,574 characters including alignment gaps, of which 334 characters were excluded, 482 were constant, 557 were variable and parsimony-uninformative and 535 were parsimony-informative. A heuristic search of the remaining 535 parsimony-informative characters resulted in a single most parsimonious tree of 426 steps (CI = 0.76, HI = 0.23, RI = 0.86). Seven isolates from four provinces were sequenced for ITS and tef1 and species were identified according to a combination of morphologic and genotypic characters. The identification details of these isolations and their origins are shown in Table 1, in which 4 species are recognized: Trichoderma asperellum, Trichoderma brevicompactum, Trichoderma koningiopsis, Trichoderma longibrachiatum. Results of the phylogenetic analysis based on ITS and EF1-α sequences are shown in Figure 5. Overall, four fungal species were morphologically identified by ITS and EF1-α sequence analyses.
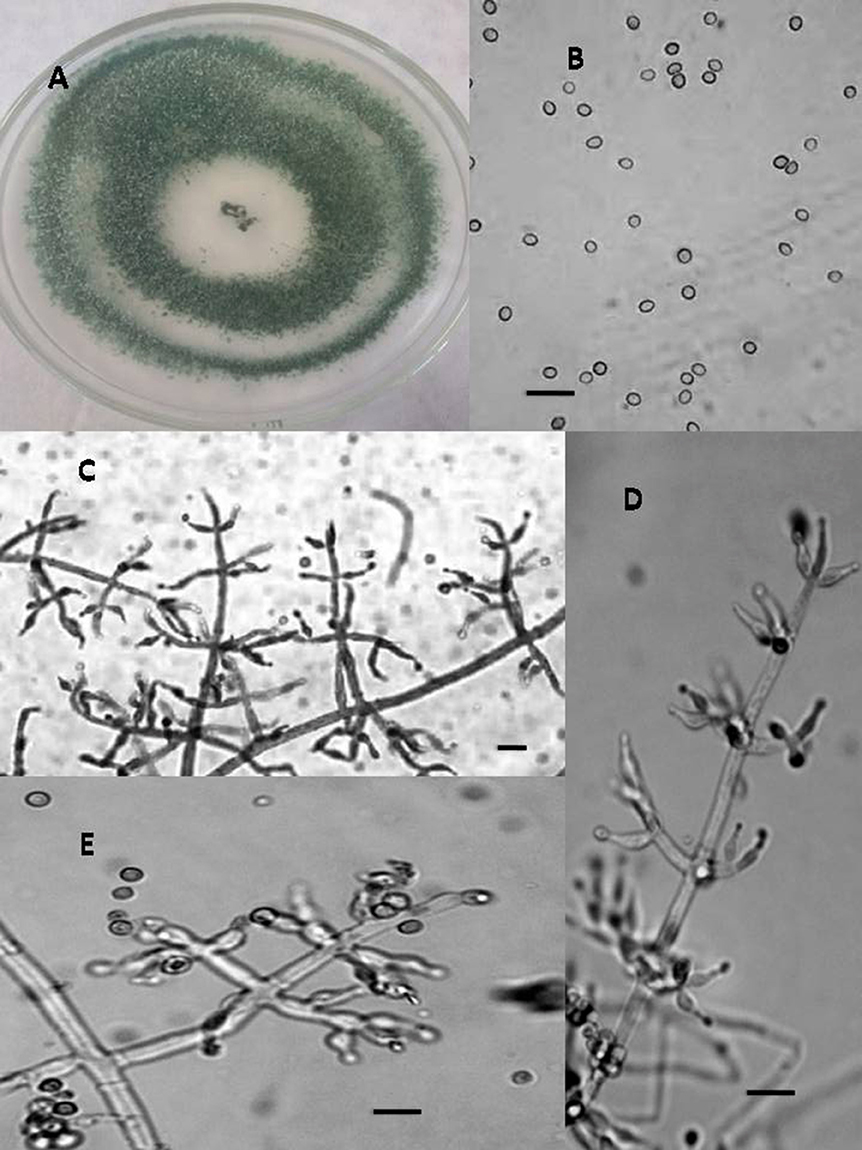
Figure 1. Morphology of T. asperellum Colony appearance on PCA (Potato Carrot Agar) (A); conidia (B); conidiophores (C–E), Scale Bar 10 μm.
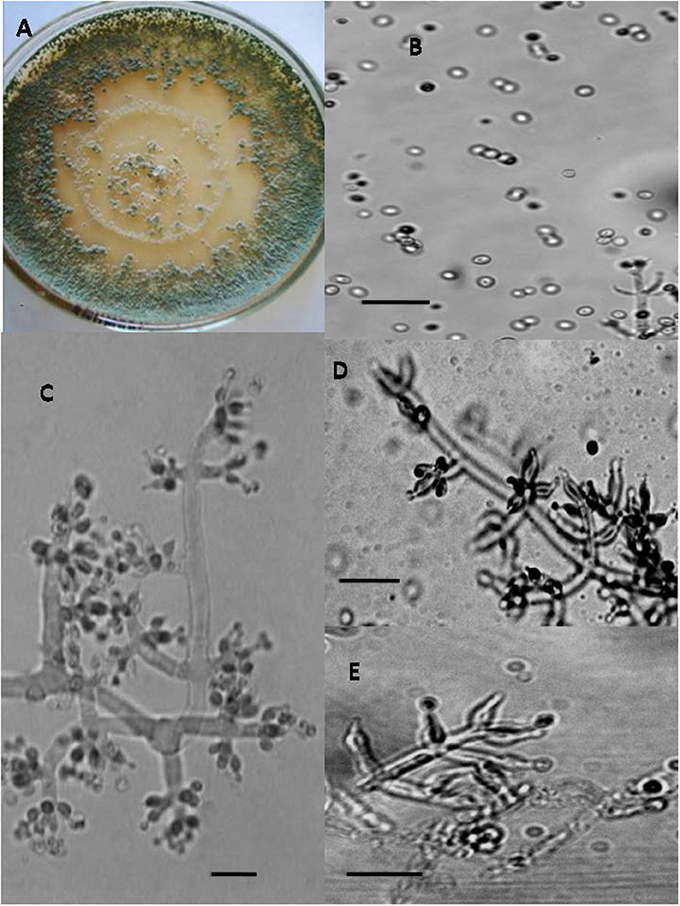
Figure 2. Morphology of T. brevicompactum Colony appearance on PCA (Potato Carrot Agar) (A); conidia (B); conidiophores (C–E), Scale Bar 10 μm.
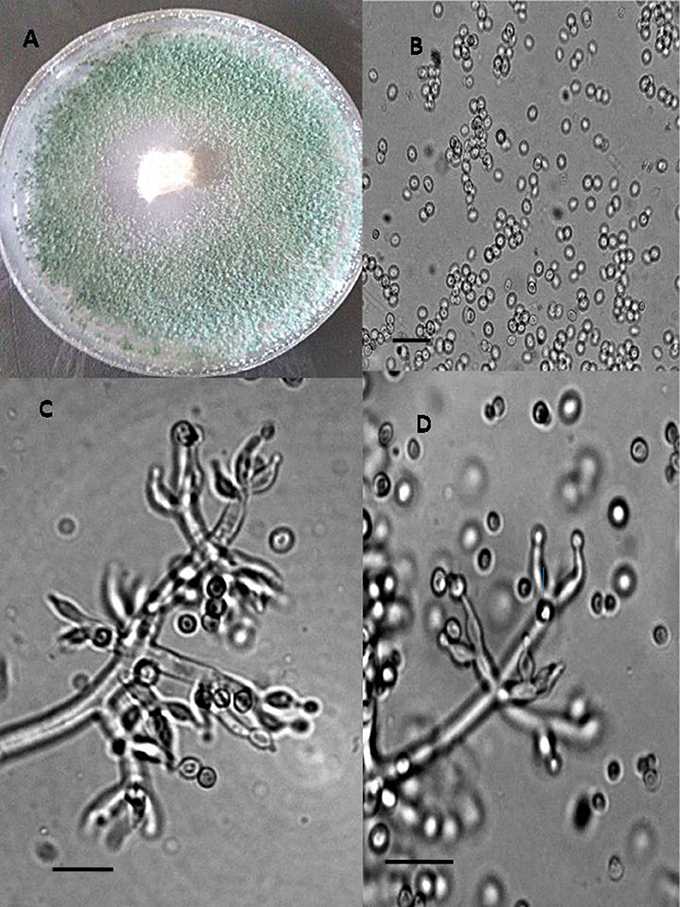
Figure 3. Morphology of T. koningiopsis Colony appearance on PCA (Potato Carrot Agar) (A); conidia (B); conidiophores (C,D), Scale Bar 10 μm.
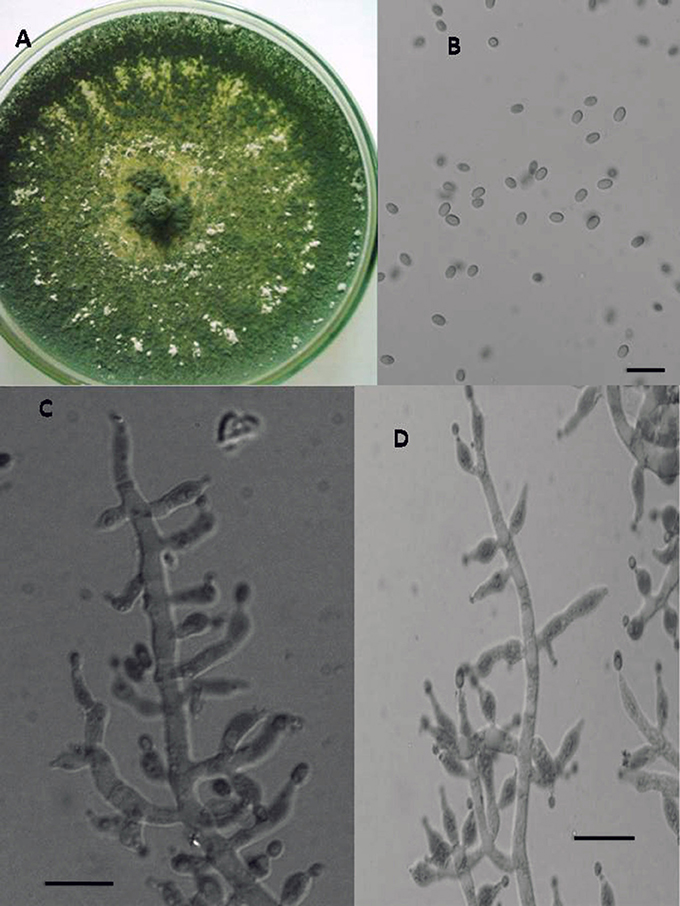
Figure 4. Morphology of T. longibrachiatum Colony appearance on PCA (Potato Carrot Agar) (A); conidia (B); conidiophores (C,D), Scale Bar 10 μm.
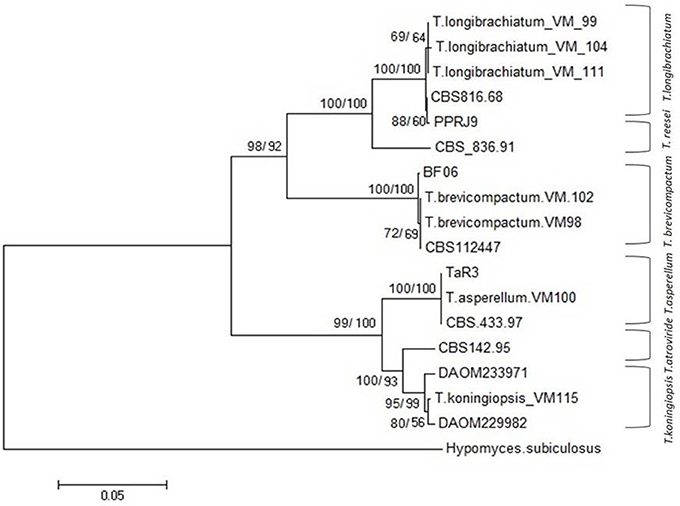
Figure 5. NJ tree based on combined dataset of ITS and EF1-α sequences. Bootstrap values. 50% (1,000 replicates) shown above branches [those of parsimony analysis (1,000 replicates) are shown on the right side Slash]. Scale bar indicates nucleotide substitution in NJ analysis. Hypomyces subiculosus is outgroup. T, Trichoderma.
Anti-proliferative Bioactivity of Methanol and Ethyl Acetate Extracts
To primarily screen the antitumor activities, P. oryzae fungus was used as a model target (Kobayashi et al., 1996; Dong et al., 2008; Xu et al., 2009). Accordingly, conidial germination and development of germ tube from P. oryzae was adapted for evaluating anti-proliferative activity of methanol and ethyl acetate extracts Trichoderma metabolites. The results presented in Table 3 demonstrate significant bioactivity for all Apocynaceae endophytic Trichoderma isolates. These findings indicate that the ethyl acetate extracts from endophytic Trichoderma isolates showed higher anti-proliferative effects compared to methanol extracts. Notably, the isolate T. koningiopsis VM115 showed the most significant bioactivity among all isolates. T. koningiopsis VM115 showed that conidial germination was completely inhibited at 250–31.2 μg mL−1 and inhibition of germ tube elongation at a concentration of 31.2–7.8 μg mL−1.
Cell Viability Assay
The results of the Cell viability of the extracts of Trichoderma isolates are shown in Figure 6. All Trichoderma isolates metabolites exhibited significant activity against P. oryzae conidia after treatment with ethyl acetate extracts and methanol extracts with an IC50 value at a range of 7.8–31.2 μg mL−1 and 15.6–62.5 μg mL−1, respectively. Also ethyl acetate extracts from all endophytic isolates showed higher cytotoxic effects compared to methanol extracts. In total, ethyl acetate extracts of T. koningiopsis VM115 isolate was the most cytotoxic at 7.8 μg mL−1. However, no inhibitions were found in the untreated P. oryzae conidial suspension. The results of cell viability assay and antiproliferative activity indicated that significant cell growth inhibition for all Apocynaceae endophytic Trichoderma isolates. The data elicited strong likelihood of cell growth inhibition and cytotoxic effects by T. koningiopsis VM115 isolate.
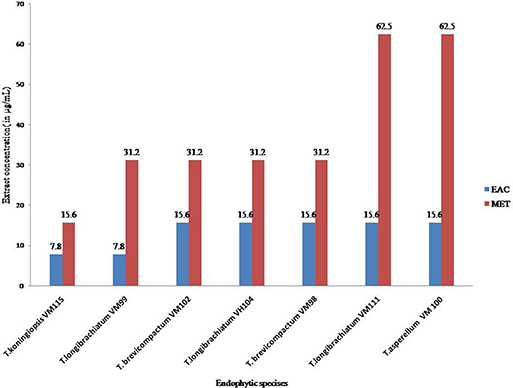
Figure 6. Cell viability assays of (EAC and MET) metabolites from endophytic species. Data (significant at P B 0.05) were obtained from three replicates. Data are reported as IC50 values. MET, Methanol extract; EAC, Ethyl acetate extract.
Bacteriostatic and Bactericidal Bioactivity of Fungal Crude Extract
All endophytic isolates were screened for antibacterial activity using two human pathogens (S. aureus PTCC and E. coli PTCC) and two plant pathogens (Ral. solanacearum and Cl. michiganensis) as targets. All Trichoderma isolate metabolites showed bacteriostatic and bactericidal activity against the two Gram-negative and two Gram-positive bacteria targets (Table 4). Ethyl acetate extracts were bacteriostatic with an IC80 value at a range of 7.8–15.6 μg mL−1 and bactericidal with an IC80 value at a range of 15.6–62.5 μg mL−1. The methanol extracts were bacteriostatic with an IC80 value at a range of 7.8–62.5 μg mL−1 and bactericidal with an IC80 value at a range of 31.2–62.5 μg mL−1. Also, T. koningiopsis VM115 and T. longibrachiatum VM 99 were more influential against Gram-negative and Gram-positive bacteria. Ethyl acetate extract had higher-level activity than other extracts, compared with bioactivity between methanol and ethyl acetate extract.
Identifications of Metabolites
According to the information provided in Tables 3–5 between ethyl acetate and methanol extracts, crude ethyl acetate extract of T. koningiopsis VM115 isolate was used for TLC and GC-MS analysis. The one known antifungal metabolite purified from the ethyl acetate extract T. koningiopsis VM115 was identified as compound 1 (Godtfredsen and Vangedal, 1964) via spectral analyses, such as MS, 1H-NMR, 13C-NMR (Figure 7).
Trichodermin (4b-acetoxy-12, 13-epoxytrichothec-9-ene) = colorless oil. 1H NMR (CDCl3, 400 MHz) δ: 5.48 (1H, dd, J = 7.8, 3.6 Hz, H-4), 5.31 (1H, d, J = 5.4 Hz, H-10), 3.71 (1H, d, J = 5.2 Hz, H-2), 3.50 (1H, d, J = 5.4 Hz, H-11), 3.02 (1H, d, J = 4.0 Hz, H-13a), 2.73 (2H, m, H-13b, H-3a), 2.46 (1H, dd, J = 15.4, 7.8 Hz, H-3b), 1.81–1.97 (3H, m, H-7a, H-8a, H-8b), 1.62 (3H, s, H-16), 1.33 (1H, brd, J = 12.1 Hz, H-7b), 0.83 (3H, s, H-15), 0.61 (3H, s, H-14). 13C-NMR (CDCl3,100 MHz) δ: 170.6 (s, C-1'), 139.8 (s, C-9), 118.3 (d, C-10), 78.8 (d, C-2), 74.7 (d, C-4), 70.1 (d, C-11), 65.2 (s, C-12), 48.6 (s, C-5), 47.5 (t, C-13), 40.1 (s, C-6), 36.3 (t, C-3), 27.7 (t, C-8), 24.1 (t, C-7), 23.0 (q, C-16), 20.9 (q, C-2'), 15.7 (q, C-15), 5.5 (q, C-14).
ESI-MS: 315 [M + Na]+, 607 [2 M + Na]+, 251 (M+H)+.
Antifungal Assay of Trichodermin
T. koningiopsis VM115 compound was bio assayed against pathogenic and model fungi B. cinera, A. fumigatus, and P. oryzae by the serial dilution method (Table 5). Having an MIC close to that of ketoconazole as a positive control, compound 1 was seen to be involved in a strong bioactivity against the test fungi.
GC-MS Analysis
Crude ethyl acetate extract of T. koningiopsis VM115 isolate was used for GC-MS analysis. The mass spectra of GC-MS-MS were interpreted by the database of National Institute of Standards and Technology (NIST) with more 62,000 patterns. The chromatogram anticipated the presence of many compounds and they were identified according to peak area, the retention time, and molecular formula. The retention time and abundance of the compounds under the described conditions in GC-MS section are shown in Table 6; the identification of these metabolites revealed that endophytic T. koningiopsis VM115 has the capacity to produce bioactive compounds. Collectively, each of the seven classes of volatile compounds was generated by T. koningiopsis VM115 [alcohols, esters, monoterpene, pyrones (lactones), acids, furanes, and lipids] (Table 6).The most abundant compound was 6-pentyl-alpha-pyrone (6-PP) with a 20.442 min retention time, based on the total area of the GC analysis, originally specified by Collins and Halim (1972), and indicated as one of the important bioactive compounds from T. harzianum and T. koningii species as reviewed by Hanson (2005).
Discussion
It has become evident in the last two decades that all healthy plants on earth harbor endophytic microorganisms (Strobel and Daisy, 2003). Much investigation has been done to explore biodiversity and bioactivity of fungal and bacterial endophytic microorganisms associated with numerous host plant species (Strobel and Daisy, 2003; Aly et al., 2010; Kusari et al., 2012, 2013). The present study investigated biodiversity and bioactivity of Trichoderma endophytic species in plants of the Vinca plants growing in Iran. Tests were conducted on 7 isolates from five provinces in Iran; Esfahan, Mazandaran, Hamedan, and Tehran. A wide variety of species was demonstrated by the results: T. asperellum (1 isolate), T. brevicompactum (2), T. koningiopsis (1), and T. longibrachiatum (3). Endophytic T. longibrachiatum showed ubiquitous dispersion in all sampling locations and on all three plants, and T. brevicompactum was isolated from V. minor and V. major but T. asperellum, and T. koningiopsis isolates were isolated from only one of the plants (Table 1). Our findings provide further indication that endophytic Trichoderma fungal species isolates exhibit significant anti-proliferative, cytotoxic and antimicrobial activity. Moreover, in general, ethyl acetate extract metabolite from endophytic isolates showed higher cytotoxic and antibacterial activity than methanol extracts. Also, varied secondary metabolites with robust antifungal and antibiotic activities are produced by Trichoderma species (Siddiquee et al., 2012). Moreover, amongst all, bioactivity of ethyl acetate and methanol extract metabolites from T. koningiopsis VM115 were superior compared to the others. This was demonstrated against P. oryzae (Table 3 and Figure 6). Abdulmyanova et al. (2015) evaluated ethyl acetate (EtAc) extracts of endophytes from V. minor and V. erecta. They demonstrated that these extracts have potential cytotoxic activity on three cancer cells, indicating the presence of cytotoxic compounds in these extracts that were exerted against the survival and growth of the model bacteria (Table 4). This result might indicate the presence of a series of compounds in T. koningiopsis VM115 that make it compete with or attack antagonist cells in a mixed population within environmental niches. This may suggest that the endophytic species of Trichoderma have evolutionary, selective, and protective roles when living inside their host plants.
Trichothecenes comprise a group of sesquiterpenes, which are structurally characterized with a 12, 13-epoxy-trichothec-9-ene moiety. Interestingly, the nucleus of this sesquiterpene has been reported to appear in fungal cultures containing Fusarium, Myrothecium, Stachyobotrs, and Trichoderma (Ueno, 1985; Abbas et al., 2002; Liu et al., 2006). Biologically, it has been reported that macrocyclic trichothecenes have antimalarial (Zhang et al., 2002), antiviral (Garcia et al., 2002), antifungal and antibacterial (Wagenaar and Clardy, 2001), and insecticidal activities (Cole and Cox, 1981) besides being phytotoxic and cytotoxic (Abbas et al., 2002) to animals. In the current research, T. koningiopsis VM115 as a trichothecene was seen to have antifungal materials in its ethyl acetate extract with a comparable MIC with that of ketoconazole as a positive control (Table 5). This compound with fungicidal activity-Trichodermin (4b-hydroxy-12, 13-epoxytrichothec-9-ene) (Godtfredsen and Vangedal, 1964; Yang et al., 2010) as a member of 4β-aceoxy-12, 13-epoxytrichothecene family A belongs to the class of trichothecenes, a group of sesquiterpene toxins. The mechanism of action for this class of toxins is mainly protein biosynthesis inhibition by preventing peptidyl transferase activity, although it was initially thought to be potentially useful in anticancer therapeutics. Isolation of Trichodermin from a few species of Trichoderma, such as T. brevicompactum and T. viride, T. Longibrachiatum, and T. harzianum has been carried out and it includes the first reported Trichodermin production of T. koningiopsis (Godtfredsen and Vangedal, 1964; Watts et al., 1988; Nielsen et al., 1998, 2005; Reino et al., 2008; Yang et al., 2010; Tijerino et al., 2011).
A multifaceted interaction occurs between filamentous fungi and their living environments mainly with the help of volatile metabolites. Secondary metabolism of fungi may have a role in plant defense, when the mixture of volatile compounds was broken down into several classes of compound, the same inhibitory effects were not achieved. This suggests that it is the suite of volatile compounds that contributes to antifungal activity (Strobel et al., 2001; Strobel, 2003). Along with providing defense against pathogens of their host; certain endophytic fungi may aid plant survival in certain habitats. No volatile compounds have been identified to be solely involved in such bio-control activities though some are known to be associated with the antagonistic ability of Trichoderma species (Siddiquee et al., 2012). The most abundant metabolite originally characterized by Collins and Halim (1972) was identified to be 6-pentyl-alpha-pyronein this research.6-pentyl-2H-pyran-2-one (6-pentyl–prone) as a metabolite is responsible for the coconut aroma released from axenic ally grown colonies. It is commonly purified from the culture filtrates of different Trichoderma species, such as T. viride, T. atroviride, T. harzianum, and T. koningii. Both in vivo and in vitro antifungal activities against several plant pathogenic fungi have been shown by 6PP. Moreover, biosynthesis of this metabolite has been shown to have a strong relationship with the bio-control ability of the producing microbe (Kobayashi et al., 1996, 2004; Vinale et al., 2014). Many important components were produced by T. koningiopsis VM115. Fatty acids and hydrocarbons were produced by T. koningiopsis VM115. Fatty acids are organic acids with antibacterial and antifungal activities (Pohl et al., 2011). Ethanoic acid, hexadecanoic acid, and butanoic acid (as an unsaturated fatty acid) produced by T. koningiopsis VM115. These compounds were reported in the T. viride by Gershon and Shanks (1978). Hydrocarbons such as hexadecane were also produced. Previously, butyrolactone was isolated from Aspergillus terreus (Arai et al., 1982). Studies of endophytic fungal diversity have mainly determined relationships among endophytic fungi as well as their host plants, by looking for natural bioactive compounds obtained from the endophytic fungi. Endophytic fungi were shown to effectively yield many vital bioactive compounds with antimicrobial, insecticidal, cytotoxic, antioxidant, and anticancer activities. Trichoderma sp. is the most prominent genus among the mycoflora. All of the isolates of endophytic fungi exhibited significant antiproliferativeand antimicrobial activity on selected test organisms. TLC guided fractionation and MS, 1H-NMR, 13C-NMR data showed that trichodermin compound was isolated from T. koningiopsis VM115, which demonstrated strong fungicidal effects against selected test organisms through in vitro antifungal tests. Also, GC-MS analysis determined six classes of volatile compound produced by T. koningiopsis VM115. 6-n-pentyl-6H-pyran-2-one (6PP) was identified as one of the most abundant metabolites in this research. These results indicate that the fungal endophytes from Vinca plants had antibacterial and cytotoxic activities; evidence that endophytes are a good source of biological activity and compounds. These are very promising for application in agriculture and medicine. It should be significant for us to screen for antibacterial activities on fungal endophytes from Vinca plants. Endophytes were found to be a good source for compounds with biological activities as evidenced by the cytotoxic and antibacterial activities of the fungal endophytes obtained from Apocynaceae. Therefore, Trichoderma endophytic species are promising sources of new and natural bioactive metabolites that provide a great potential for further research.
Author Contributions
Both authors carried out the molecular genetic studies, participated in the sequence alignment and drafted the manuscript. Both authors carried out the antiproliferative, cytotoxic, and antimicrobial activities and TLC, GC-MS, MS, 1H-NMR, 13C-NMR analysis. Both authors participated in the sequence alignment. Both authors participated in the design of the study and performed the statistical analysis. Both authors conceived of the study, and participated in its design and coordination. All authors read and approved the final manuscript.
Conflict of Interest Statement
The authors declare that the research was conducted in the absence of any commercial or financial relationships that could be construed as a potential conflict of interest.
Acknowledgments
This project is supported by Bu Ali Sina University, Hamedan, Iran. Authors are thankful to Dr. Sonbol Nazeri, Dr. Gholam Khodakaramian, and Fatemeh Ghobadi (MSc) for their generous gifts of target fungi and bacteria at Bu-Ali Sina University, Hamedan, Iran and also thank Dr. Ali Pakdin and DR. Mohammadali Tajick at Sari agriculture and natural resource University, Mazandaran, Iran, for their technical assistance.
References
Abbas, H. K., Johnsona, B. B., Shierb, W. T., Takc, H., Jarvisc, B. B., and Boyette, C. D. (2002). Phytotoxicity and mammalian cytotoxicity of macrocyclic trichothecene mycotoxins from Myrothecium verrucaria. Phytochemistry 59, 309–313. doi: 10.1016/S0031-9422(01)00464-2
Abdollahzadeh, J., Javadi, A., Mohammadi Goltapeh, E., Zare, R., and Phillips, A. J. L. (2010). Phylogeny and morphology of four new species of Lasiodiplodia from Iran. Persoonia 25, 1–10. doi: 10.3767/003158510X524150
Abdollahzadeh, J., Javadi, A., Zare, R., and Phillips, A. J. L. (2014). A phylogenetic study of Dothiorella and Spencermartinsia species associated with woody plants in Iran, New Zealand, Portugal and Spain. Persoonia 32, 1–12. doi: 10.3767/003158514X678606
Abdulmyanova, L. I., Teomashko, N. N., Terentyeva, E. O., Ruzieva, D. M., Sattarova, R. S., Azimova, S. S., et al. (2015). Cytotoxic activity of fungal endophytes from Vinca, L. Int. J. Curr. Microbiol. App. Sci. 4, 321–329.
Aly, A. H., Debbab, A., Kjer, J., and Proksch, P. (2010). Fungal endophytes from higher plants: a prolific source of phytochemicals and other bioactive natural products. Fungal Divers 41, 1–16 doi: 10.1007/s13225-010-0034-4
Arai, K., Yoshimura, T., Itatani, Y., and Yamamoto, Y. (1982). Metabolic products of Aspergillus terreus. VIII. 1) Astepyrone: a novel metabolite of the strain IFO 4100. Chem. Pharmaceut. Bull. 1982, 31, 925–933. doi: 10.1248/cpb.31.925
Barchiesi, F., Arzeni, D., Fothergill, A. W., Di Francesco, L. F., Caselli, F., Rinaldi, M. G., et al. (2000). In vitro activities of the new antifungal triazole SCH 56592 against common and emerging yeast pathogens. Antimicrob. Agents Chemother. 44, 226–229. doi: 10.1128/AAC.44.1.226-229.2000
Bennett, J. W., and Klich, M. (2003). Mycotoxins. Clin. Microbiol. Rev. 16, 497–516. doi: 10.1128/CMR.16.3.497-516.2003
Bhardwaj, A., Sharma, D., Jodan, N., and Agrawal, P. K. (2015). Antimicrobial and phytochemical screening of endophytic fungi isolated from spikes of Pinus roxburghii. Arch. Clin. Microbiol. 6, 1–9.
Bissett, J. (1984). A revision of the genus Trichoderma I. Sect. Longibrachiatum sect. nov. Can. J. Bot. 62, 924–931. doi: 10.1139/b84-131
Bissett, J. (1991a). A revision of the genus Trichoderma II. In frageneric classification. Can. J. Bot. 69, 2357–2372. doi: 10.1139/b91-297
Bissett, J. (1991b). A revision of the genus Trichoderma. III. Sect. Pachybasium. Can. J. Bot. 69, 2373–2417. doi: 10.1139/b91-298
Bissett, J. (1991c). A revision of the genus Trichoderma. IV. Additional notes on section Longibrachiatum. Can. J. Bot. 69, 2418–2420. doi: 10.1139/b91-299
Cardoza, R. E., Malmierca, M. G., Hermosa, M. R., Alexander, N. J., McCormick, S. P., Proctor, R. H., et al. (2011). Identification of loci and functional characterization of trichothecene biosynthetic genes in the filamentous fungus Trichoderma. Appl. Environ. Microbiol. 77, 4867–4877. doi: 10.1128/AEM.00595-11
Cole, R. J., and Cox, R. H. (1981). Handbook of Toxic Fungal Metabolites I. New York, NY: Academic Press.
Collins, R. P., and Halim, A. F. (1972). Characterization of the major aroma constituent of the fungus Trichoderma virens (Pers.) J. Agricult. Food Chem. 20, 437–438. doi: 10.1021/jf60180a010
Corley, D. G., Miller-Wideman, M., and Durley, R. C. (1994). Isolation and structure of harzianum A: A new trichothecene from Trichoderma harzianum. J. Nat. Prod. 57, 442–425. doi: 10.1021/np50105a019
Degenkolb, T., von Döhren, H., Nielsen, K. F., Samuels, G. J., and Brückner, H. (2008). Recent advances and future prospects in peptaibiotics, hydrophobin, and mycotoxin research, and their importance for chemotaxonomy of Trichoderma and Hypocrea. Chem. Biodiv. 5, 671–680. doi: 10.1002/cbdv.200890064
Dong, Q. F., Wang, J. L., Zhang, S. F., Wang, Z., Zhang, C. X., Gao, H., et al. (2008). Antifungal activity of crude extracts and fat-soluble constituents of Holotrichia diomphalia larvae. Bioresource Technol. 99, 8521–8523. doi: 10.1016/j.biortech.2008.03.010
Edgar, R. C. (2004). MUSCLE: multiple sequence alignment with high accuracy and high throughput. Nucleic Acids Res. 32, 1792–1797. doi: 10.1093/nar/gkh340
Farris, J. S., Kallersjo, M., Kluge, A. G., and Bult, C. (1995). Testing significance of incongruence. Cladistics 10, 315–319 doi: 10.1111/j.1096-0031.1994.tb00181.x
Fravel, D. R. (1988). Role of antibiosis in the biocontrol of plant diseases. Annu. Rev. Phytopathol. 26, 75–91. doi: 10.1146/annurev.py.26.090188.000451
Frisvad, J. C., and Thrane, U. (2002). “Mycotoxin production by common filamentous fungi.” in Introduction to Food- and Airborne Fungi, 6th Edn., eds R. A. Samson, E. S. Hoekstra, J. C. Frisvad, and O. Filtenborg (Utrecht: Centraalbureau voor Schimmelcultures), 321–331.
Galindo, E., Flores, C., Larralde-Corona, P., Corkidi-Blanco, G., Rocha-Valadez, J. A., and Serrano-Carreo'n, L. (2004). Production of 6-pentyl-alpha-pyrone by Trichoderma harzianum cultured in unbaffled and baffled shake flasks. Biochem. Eng. J. 18, 1–8. doi: 10.1016/S1369-703X(03)00115-3
Gams, W., and Bissett, J. (1998). “Morphology and identification of Trichoderma,” in Trichoderma and Gliocladium. Basic Biology, Taxonomy and Genetics, eds C. P. Kubicek and G. E. Harman (London: Taylor & Francis Ltd), 34.
Garcia, C. C., Rosso, M. L., Bertoni, M. D., Maier, M. S., and Damonte, E. B. (2002). Evaluation of the antiviral activity against junin virus of macrocyclic trichothecenes produced by the hypocrealean epibiont of Baccharis coridifolia. Planta Med. 68, 209–212 doi: 10.1055/s-2002-23134
Gershon, H., and Shanks, L. (1978). “Chapter 6: Antifungal activity of fatty acids and derivatives: structure-activity relationships,” in The Pharmacological Effect of Lipids, ed J. J. Kabara (Champaign, IL: American Oil Chemists' Society), 51–63.
Godtfredsen, W. O., and Vangedal, S. (1964). Trichodermin, a new antibiotic related to trichothecin. Proc. Chem. Soc. 6, 188–189.
Hall, T. (2006). Bioedit 7.5.0.3. Department of Microbiology, North Carolina State University. Available online at: http://www.mbio.ncsu.edu/BioEdit/Bioedit.html.
Hanson, J. R. (2005). The chemistry of the bio-control agent, Trichoderma harzianum. Sci. Prog. 88, 237–248. doi: 10.3184/003685005783238372
Harman, G. E., Howell, C. R., Viterbo, A., Chet, I., and Lorito, M. (2004). Trichoderma species–opportunistic avirulent plant symbionts. Nat. Rev. Microbiol. 2, 43–56 doi: 10.1038/nrmicro797
Hoyos-Carvajal, L., Orduz, S., and Bissett, J. (2009). Genetic and metabolic biodiversity of Trichoderma from Colombia and adjacent neotropic regions. Fungal Genet. Biol. 46, 615–631. doi: 10.1016/j.fgb.2009.04.006
Huelsenbeck, J. P., Bull, J. J., and Cunningham, C. V. (1996). Combining data in phylogenetic analysis. Trends Ecol. Evol. 11, 152–158. doi: 10.1016/0169-5347(96)10006-9
Jin, H. Z., Lee, J. H., Zhang, W. D., Lee, H. B., Hong, Y. S., Kim, Y. H., et al. (2007). Harzianums A and B produced by a fungal strain, Hypocrea sp F000527, and their cytotoxicity against tumor cell lines. J. Asian Nat. Prod. Res. 9, 203–207. doi: 10.1080/10286020500531977
Keller, N. P., Turner, G., and Bennett, J. W. (2005). Fungal secondary metabolism-from biochemistry to genomics. Nat. Rev. Microbiol. 3, 937–947. doi: 10.1038/nrmicro1286
Kobayashi, H., Namikoshi, M., Yoshimoto, T., and Yokochi, T. (1996). A screening method for antimitotic and antifungal substances using conidia of Pyricularia oryzae, modification and application tropical marine fungi. J. Antibiot. 49:873. doi: 10.7164/antibiotics.49.873
Kobayashi, M., Kanasaki, R., Ezaki, M., Sakamoto, K., Takase, S., Fujie, A., et al. (2004). FR227244, a novel antifungal antibiotic from Myrothecium cinctum No. 002 I. Taxonomy, fermentation, isolation and physio-chemical properties. J. Antibiot. 57, 780–787. doi: 10.7164/antibiotics.57.780
Korpi, A., Jarnberg, J., and Pasanen, A. L. (2009). Microbial volatile organic compounds. Crit. Rev. Toxicol. 39, 139–193. doi: 10.1080/10408440802291497
Kral, R. (2012). Annotated Checklist of the Vascular Plants of Alabama. Texas, TX: Botanical Research Institute of Texas 3(2).A. 1890. 1–112. Copyright 2011
Kraus, G. F., Druzhinina, I., Gams, W., Bissett, J., Zafari, D., Szakacs, G., et al. (2004). Trichoderma brevicompactum sp. nov. Mycologia 96, 1059–1073. doi: 10.2307/3762089
Kusari, S., Hertweck, C., and Spiteller, M. (2012). Chemical ecology of endophytic fungi: origins of secondary metabolites. Chem. Biol. 19, 792–798 doi: 10.1016/j.chembiol.2012.06.004
Kusari, S., Pandey, S. P., and Spiteller, M. (2013). Untapped mutualistic paradigms linking host plant and endophytic fungal production of similar bioactive secondary metabolites. Phytochemistry 91, 81–87. doi: 10.1016/j.phytochem.2012.07.021
Levitz, S. M., and Diamond, R. D. (1985). A rapid colorimetric assay of fungal viability with the tetrazolium salt MTT. J. Infect. Dis. 152, 938–945 doi: 10.1093/infdis/152.5.938
Liu, J. Y., Huang, L. L., Ye, Y. H., Zou, W. X., Guo, Z. J., and Tan, R. X. (2006). Antifungal and new metabolites of Myrothecium sp. Z16, a fungus associated with white croaker Argyromosum argentatus. J. Appl. Microbiol. 100, 195–202. doi: 10.1111/j.1365-2672.2005.02760.x
Ming, Q., Han, T., Li, W., Zhang, Q., Zhang, H., Zheng, C., et al. (2012). Tanshinone II A and tanshinone I production by Trichoderma atroviride D16, an endophytic fungus in Salvia miltiorrhiza. Phytomedicine 19, 330–333 doi: 10.1016/j.phymed.2011.09.076
Mukherjee, P. K., Horwitz, B. A., and Kenerley, C. M. (2012). Secondary metabolism in Trichoderma – a genomic Perspective. Microbiology 158, 35–45. doi: 10.1099/mic.0.053629-0
Nielsen, K. F. (2003). Review: Mycotoxin production by indoor molds. Fungal Genet. Biol. 39, 103–101. doi: 10.1016/S1087-1845(03)00026-4
Nielsen, K. F., Fenhan, T. G., Zafari, D., and Thrane, U. (2005). Trichothecene production by Trichoderma brevicompactum. J. Agric. Food Chem. 53, 8190–8196 doi: 10.1021/jf051279b
Nielsen, K. F., Hansen, M., Larsen, T. O., and Thrane, U. (1998). Production of trichothecene mycotoxins on water damaged gypsum boards in Danish buildings. Int. Biodeter. Biodegr. 42, 1–7. doi: 10.1016/S0964-8305(98)00035-3
Patel, N., Oudemans, P. V., Hillman, B. I., and Kobayashi, D. Y. (2013). Use of the tetrazolium salt MTT to measure cell viability effects of the bacterial antagonist Lysobacter enzymogenes on the filamentous fungus Cryphonectria parasitica. Antonie Van Leeuwenhoek 103, 1271–1280. doi: 10.1007/s10482-013-9907-3
Pohl, C. H., Johan, L. F., Kock, J. L. F., and Thibane, V. S. (2011). Antifungal Free Fatty Acids: A Review. Science Against Microbial Pathogens: Communicating Current Research and Technological Advances. Badajoz: Formatex Research Center.
Rechinger, K. H. (ed.). (1974). “No. 103: Apocynaceae,” in Flora Iranica (Graz: Akademische Druck u Verlagsanstalt−11), 54.
Reino, J. L., Guerrero, R. F., Herna'ndez-Gala'n, R., and Collado, I. G. (2008). Secondary metabolites from species of the biocontrol agent Trichoderma. Phytochem. Rev. 7, 89–123. doi: 10.1007/s11101-006-9032-2
Rodriguez, F., Oliver, J. F., Marin, A., and Medina, J. R. (1990). The general stochastic model of nucleotide substitutions. J. Theoret. Biol. 142, 485–501. doi: 10.1016/S0022-5193(05)80104-3
Rocha, O., Ansari, K., and Doohan, F. M. (2005). Effects of trichothecene mycotoxins on eukaryotic cells: a review. Food Addit. Contam. 22, 369–378. doi: 10.1080/02652030500058403
Schnürer, J., Olsson, J., and Börjesson, T. (1999). Fungal volatiles as indicators of food and feeds spoilage. Fung. Genet. Biol. 27, 209–217. doi: 10.1006/fgbi.1999.1139
Sharma, D., Pramanik, A., and Agrawal, P. K. (2016). Evaluation of bioactive secondary metabolites from endophytic fungus Pestalotiopsis neglecta BAB-5510 isolated from leaves of Cupressus torulosa D. Don. 3 Biotech 6:210. doi: 10.1007/s13205-016-0518-3
Shentu, X. P., Liu, W. P., Zhan, X. H., Xu, Y. P., and Xu, J. F. (2014). Transcriptome sequencing and gene expression analysis of Trichoderma brevicompactum under different culture conditions. PLoS ONE 9:e94203. doi: 10.1371/journal.pone.0094203
Siddiquee, S., Cheong, B. E., Taslima, K., Kausar, H., and Hasan, M. M. (2012). Separation and identification of volatile compounds from liquid cultures of Trichoderma harzianum by GC-MS using three different capillary columns. J. Chromatogr. Sci. 50, 358–367. doi: 10.1093/chromsci/bms012
Sivasithamparam, K., and Ghisalberti, E. L. (1998). “Secondary metabolism in Trichoderma and Gliocladium,” in Trichoderma and Gliocladium: Basic Biology, Taxonomy, and Genetics Vol. 1, eds C. P. Kubicek and G. E. Harman (London: Francis and Taylor Ltd), 139–191.
Strobel, G. A. (2003). Endophytes as sources of bioactive products. Microbes Infect. 5, 535–544. doi: 10.1016/S1286-4579(03)00073-X
Strobel, G., and Daisy, B. (2003). Bioprospecting for microbial endophytes and their natural products. Microbiol. Mol. Biol. Rev. 67, 491–502. doi: 10.1128/MMBR.67.4.491-502.2003
Strobel, G., Dirksie, E., and Sears, J. (2001). Markworth C Volatile antimicrobials from Muscodor albus, a novel endophytic fungus. Microbiology 147, 2943–2950. doi: 10.1099/00221287-147-11-2943
Swofford, D. L. (2003). PAUP* 4.0b10: Phylogenetic Analysis Using Parsimony (*and Other Methods). Sunderland, MA: Sinauer Associates.
Tijerino, A., Cardoza, R. E., Moraga, J., Malmierca, M. G., Vicente, F., Aleu, J., et al. (2011). Overexpression of trichodiene synthase gene tri5 increases trichodermin production and antimicrobial activity in Trichoderma brevicompactum. Fungal Genet. Biol. 48, 285–296. doi: 10.1016/j.fgb.2010.11.012
Ueno, Y. (1985). The toxicology of mycotoxins. Crit. Rev. Toxicol. 14, 99–132. doi: 10.3109/10408448509089851
Vinale, F., Sivasithamparam, K., Ghisalberti, E. L., Marra, R., Barbetti, M. J., and Li, H. (2008). A novel role for Trichoderma secondary metabolites in the interactions with plants. Physiol. Mol. Plant Pathol. 72, 80–86. doi: 10.1016/j.pmpp.2008.05.005
Vinale, F., Sivasithamparam, K., Ghisalberti, E. L., Woo, S. L., Nigro, M., Marra, R., et al. (2014). Trichoderma secondary metabolites active on plants and fungal pathogens. Open Mycol. J. 8(Suppl. 1, M5), 127–139. doi: 10.2174/1874437001408010127
Vizcaíno, J. A., Sanz, L., and Basilio, A. (2005). Screening of antimicrobial activities in Trichoderma isolates representing three Trichoderma sections. Mycol. Res. 109, 1397–1406. doi: 10.1017/S0953756205003898
Wagenaar, M. M., and Clardy, J. (2001). Two new roridins isolated from Myrothecium sp. J Antibiot. 54, 517–520. doi: 10.7164/antibiotics.54.517
Watts, R., Dahiya, J., Chandhary, K., and Tauro, P. (1988). Isolation and characterization of a new antifungal metabolite of Trichoderma reesei. Plant Soil 107, 81–84. doi: 10.1007/BF02371547
Wilkins, K., Nielsen, K. F., and Din, S. U. (2003). Patterns of volatile metabolites and nonvolatile trichothecenes produced by isolates of Stachybotrys, Fusarium, Trichoderma, Trichothecium and Memnoniella. Environ. Sci. Pollut. Res. 10, 162–166. doi: 10.1065/espr2002.05.118
Xu, L. L., Han, T., Wu, J. Z., Zhang, Q. Y., Zhang, H., Huang, B. K., et al. (2009). Comparative research of chemical constituents, antifungal and antitumor properties of ether extracts of Panax ginseng and its endophytic fungus. Phytomedicine 16, 609–616. doi: 10.1016/j.phymed.2009.03.014
Yang, Z. S., Li, G. H., Zhao, P. J., Zheng, X., Luo, S. L., Li, L., et al. (2010). Nematicidal activity of Trichoderma spp. and isolationof an active compound. World J. Microbiol. Biotechnol. 26, 2297–2302. doi: 10.1007/s11274-010-0410-y
Young, N. D., and Healy, J. (2003). GapCoder automates the use of indel characters in phylogenetic analysis. BMC Bioinformatics 4:6. doi: 10.1186/1471-2105-4-6
Keywords: endophytic Trichoderma species, trichodermin, volatile compounds, antimicrobial, anti-proliferative
Citation: Leylaie S and Zafari D (2018) Antiproliferative and Antimicrobial Activities of Secondary Metabolites and Phylogenetic Study of Endophytic Trichoderma Species From Vinca Plants. Front. Microbiol. 9:1484. doi: 10.3389/fmicb.2018.01484
Received: 13 July 2017; Accepted: 14 June 2018;
Published: 11 July 2018.
Edited by:
Edvaldo Antonio Ribeiro Rosa, Pontifícia Universidade Católica do Paraná, BrazilReviewed by:
Osmar Nascimento Silva, Universidade Católica Dom Bosco, BrazilFohad Mabood Husain, King Saud University, Saudi Arabia
Copyright © 2018 Leylaie and Zafari. This is an open-access article distributed under the terms of the Creative Commons Attribution License (CC BY). The use, distribution or reproduction in other forums is permitted, provided the original author(s) and the copyright owner(s) are credited and that the original publication in this journal is cited, in accordance with accepted academic practice. No use, distribution or reproduction is permitted which does not comply with these terms.
*Correspondence: Doustmorad Zafari, ZF96YWZhcmlAeWFob28uY29t