- 1College of Animal Science and Veterinary Medicine, Henan Agricultural University, Zhengzhou, China
- 2Henan Key Laboratory of Innovation and Utilization of Grassland Resources, Zhengzhou, China
Dietary fiber (DF) is increasingly thought to regulate diversity of piglet gut microbiota to alleviate weaning stress in piglets. This study was conducted to investigate the effects of DF on growth performance of piglets and composition of their gut microbiota, as well as the interaction between gut microbiota and short-chain fatty acids (SCFAs) in piglets. A total of 840 piglets were allocated to three dietary treatments consisting of a control group (CG), an alfalfa meal group (AG), and a commodity concentrated fiber group (OG) in a 30-day feeding trial. Gut mucosa and feces samples were used to determine bacterial community diversity by 16S rRNA gene amplicon sequencing. Fiber treatment had a positive effect on growth performance and metabolism of SCFAs in piglets, in particular, compared with CG, the diarrhea rate was significantly decreased, and the content of propionic acid (PA) in the cecum was markedly increased in AG. The Shannon indices of the jejunum microbiota in AG were higher than CG. At the genus level, compared to CG, in the duodenum, the relative abundance of Paenibacillus in AG and OG was higher; in the jejunum, the relative abundances of Bacillus, Oceanobacillus, Paenibacillus, Lactococcus, Enterococcus, and Exiguobacterium were higher, whereas the relative abundance of Mycoplasma was lower in AG; in the cecum, there was also lower relative abundance of Helicobacter in AG and OG, and furthermore, the relative abundance of Faecalibacterium in OG was higher than in CG and AG. Spearman correlation analysis showed that Pseudobutyrivibrio was positively correlated with acetic acid, PA, and butyric acid (BA), while Bacteroides and Anaerotruncus were negatively correlated with PA and BA. In addition, microbiota analyses among different intestine segments showed distinct differences in microbiota between the proximal and distal intestines. Bacteria in the proximal segments were mainly Firmicutes, while bacteria in the distal segments were mainly Bacteroidetes and Firmicutes. Overall, these findings suggested that DF treatment could reduce the diarrhea rate of piglets and had beneficial effects on gut health, which might be attributed to the alteration in gut microbiota induced by DF and the interaction of the gut microbiota with SCFAs.
Introduction
The high mortality and low growth performance of piglets during the weaning period seriously affects production efficiency in the pig industry. Stress caused by changes in environment and feed as well as the immaturity of the digestive and immune systems results in a decrease in feed intake of piglets and also digestive disorders, leading to increased incidence of diarrhea in piglets (Lallès et al., 2007). Heo et al. (2013) reported that the occurrence of pathogenic diarrhea in piglets was mainly due to the release of endotoxin by Escherichia coli that adhered to gut epithelial cells. Breeders usually feed piglets with digestible diets, such as milk by-products, animal protein, and cooked rice, to prevent indigestible nutrients which are exploited by pathogens in hindgut (Mateos et al., 2006). Antibiotics have been recognized as one of the most successful therapies in medicine, and are administered in both human and veterinary medicine. However, the use of antibiotics is now debatable due to the growing number of antibiotic-resistant bacteria within human and animal gut microbiota. The application of antibiotics as part of the feed not only increases the cost of farming, but also fails to achieve the desired growth performance during the weaning period (Berrocoso et al., 2013). Therefore, there is an urgent need to find alternatives to antibiotics to both maintain piglet health at the critical weaning period and preserve public health.
At present, breeders are trying to relieve weaning stress of piglets through dietary fiber (DF). Studies have shown that DF influences the physicochemical properties of chyme (Canibe and Bach Knudsen, 2002), improving the morphology and micro-ecological environment of the gut, stimulating the secretion of digestive enzymes (Gerritsen et al., 2012), and promoting the development and integrity of the digestive tract mucosa (Liu et al., 2012). It has also been confirmed that DF contributes to the development, health, and the micro-ecological balance of piglet gut (Molist et al., 2010). Several studies have indicated that the effect of fiber on piglet diarrhea depends on the amount of fiber added and its physicochemical properties. Li and Zhang (2006) increased the content of crude fiber (CF) to 5.3% in the piglet diet by adding alfalfa meal and found that the fiber could reduce the diarrhea rate of piglets by affecting water absorption in the small intestine and inhibiting the growth of pathogens. Gut microbiota, serving as an important barrier in the host, play crucial roles in animal health and growth, including digestion and fermentation of carbohydrates, production of vitamins, maintenance of normal functions of the intestinal villi, regulation of the immune responses, and protection from pathogenic bacteria; however, gut microbiota also have effects on the liver, brain, and even on the metabolism of fat and muscle tissue, finally influencing the whole host metabolic network of nutrient and energy (Schroeder and Bäckhed, 2016). DF cannot be digested by host digestive enzymes but can be degraded into monosaccharides and short-chain fatty acids (SCFAs) by gut microbiota (Gresse et al., 2017). As the major energy source for hindgut microbiota, DF is believed to have significant effects on the composition and diversity of microbiota (Filippo and Hartl, 2010; Heinritz et al., 2016). Given that there are few studies evaluating the microbial mechanism of DF on weaning piglet health, the current research was undertaken to investigate the effects of different DF on growth performance, the composition and metabolites of gut and feces microbiota (GFM), as well as the metabolic interaction between hindgut microbiota and SCFAs in weaning piglets. This would provide some microbial mechanistic insights into the application of different DF on weaning piglet health.
Materials and Methods
Experimental Design and Sampling
All experimental procedures in this study were approved by the Institutional Animal Ethics Committee of Henan Agricultural University. A total of 840 piglets (Duroc × Landrace × Large White, age 35 days) with body weights of 9.26 ± 0.17 kg were randomly allotted to three treatments, with each treatment comprising four replicated pens of 70 piglets. The mixed groups of male and female piglets were housed in slatted floor indoor pens with access to feed and water ad libitum for 30 days under standard management conditions. All piglets were supplied with feed formulated to meet NRC (2012) recommendations. Experimental diets for each treatment were as follows: control group (CG) with corn and soybean-meal diet in which 2.27% CF was included; alfalfa meal group (AG) with diets in which CG diet was partly replaced with 5% alfalfa meal and the CF content was 3.27%; commodity concentrated fiber group (OG) with diets in which CG diet was partly replaced with 2% commodity concentrated fiber and the CF content was 3.27%. The detailed ingredient composition and nutrient content of the investigated diets are presented in Table 1. The average daily feed intake (ADFI), average daily gain (ADG), feed to gain (F:G), mortality, and diarrhea rate were recorded.
At the end of the experiment, one piglet in each replicate was selected for sampling/processing. Feces samples were obtained using sterilized equipment and were frozen in liquid nitrogen. After fasting overnight, the gastrointestinal tract of each piglet was removed immediately after slaughter and segments (duodenum, jejunum, ileum, cecum, and colon) were identified and ligated before separation. Sterile cold phosphate buffer was used to wash the mucosa. Subsequently, mucosal samples from the duodenum, jejunum, ileum, cecum, and colon were collected by scraping the mucosa with a sterile glass microscope slide. At the same time, samples of chyme from cecum and colon sections were also collected. All samples were stored in sterile cryopreservation tubes and immediately frozen at -80°C until further analysis. Then, three repeats were randomly selected from each treatment to be sequenced, one individual per replicate collecting six sample types (duodenum, jejunum, ileum, cecum, colon, and feces).
DNA Extraction and 16S rRNA Gene Sequencing
Microbial DNA was extracted from the digestive tract (duodenum, jejunum, ileum, cecum, and colon) mucosa and feces samples using an E.Z.N.A®. Soil DNA Kit (Omega Bio-tek, Norcross, GA, United States) according to manufacturer’s protocols. The final DNA concentration and purity were determined by NanoDrop 2000 UV–vis spectrophotometer (Thermo Scientific, Wilmington, NC, United States), and DNA quality was checked by 1% agarose gel electrophoresis (Yang et al., 2015). The V3–V4 hypervariable regions of the bacterial 16S rRNA gene were amplified with primers 338F (5′-ACTCCTACGGGAGGCAGCAG-3′) and 806R (5′-GGACTACHVGGGTWTCTAAT-3′) by PCR (GeneAmp 9700, ABI, United States; Singh et al., 2015) using the following program: 3 min denaturation at 95°C; 27 cycles of 30 s at 95°C, 30 s annealing at 55°C, and 45 s elongation at 72°C; and a final extension at 72°C for 10 min. PCR reactions were performed in triplicate with each 20 μL reaction mixture containing 4 μL of 5× FastPfu Buffer, 2 μL of 2.5 mM dNTPs, 0.8 μL of each primer (5 μM), 0.4 μL FastPfu Polymerase, and 10 ng template DNA. The resulting PCR products were extracted from a 2% agarose gel and further purified using the AxyPrep DNA Gel Extraction Kit (Axygen Biosciences, Union City, CA, United States) and quantified using QuantiFluorTM-ST (Promega, United States) according to the manufacturer’s protocol.
GenBank Accession Number
Purified amplicons were pooled in equimolar amounts and paired-end sequenced (2 × 300), on an Illumina MiSeq platform (Illumina, San Diego, CA, United States) according to standard protocols, by Majorbio Bio-Pharm Technology Co. Ltd. (Shanghai, China). The raw reads were deposited into the NCBI Sequence Read Archive (SRA) database under accession number SRP 121201.
Bioinformatics Analysis of Sequencing Data
Raw fastq files were demultiplexed, quality-filtered by Trimmomatic, and merged by FLASH with the following criteria: (i) reads were truncated at any site receiving an average quality score < 20 over a 50-bp sliding window; (ii) primers were exactly matched allowing two nucleotide mismatching, and reads containing ambiguous bases were removed; and (iii) sequences whose overlap was longer than 10 bp were merged according to their overlap sequence. Operational taxonomic units (OTUs) were clustered with 97% similarity cutoff using UPARSE (version 7.11), and chimeric sequences were identified and removed using UCHIME. The taxonomy of each 16S rRNA gene sequence was analyzed by RDP Classifier algorithm2 against the Silva (SSU123) 16S rRNA database using a confidence threshold of 70%. Biodiversity of the samples was calculated with ACE, Chao1, and Shannon indices (Schloss et al., 2009). The one-way analysis of molecular variance (AMOVA) method was used to identify differences between groups (Schloss et al., 2009). The shifts in the relative abundance of the bacterial phyla were displayed by a heatmap (Kolde, 2015), which was modeled with vegan package in R. Based on OTUs, Weighted-unifrac principal coordinate analysis (PCoA; Lozupone et al., 2011) and Bray–Curtis sample hierarchical cluster analysis were used to summarize the composition of gut microbiota in different parts of the digestive tract (Jiang et al., 2013). To determine the effect of posterior segment microbiota interacting with SCFAs, redundancy analysis (RDA) was performed at the genus level using the R language vegan packet (RDA 2014; Mu et al., 2017).
Determination of SCFAs
The amount of SCFAs in cecum and colon contents were determined using gas chromatography (GC) according to the method of Alfa et al. (2017). The samples on a HP-88 column (100 m long × 0.25 mm diameter and 0.2 μm film thickness) were separated by using a TRACETM 1310 GC with flame ionization detector (FID). The temperature program was 70°C for 1 min, then raised to 180°C at 25°C/min and held for 1 min, then raised to 200°C at 10°C/min and held for 1 min, then raised to 220°C at 2°C/min and held for 10 min, and finally raised to 240°C at 20°C/min and held for 6 min. Samples were run with a 20:1 split ratio and a 1.3 mL/min column flow. Hydrogen was used as the carrier gas. The temperatures of the injector and detector were 270 and 290°C, respectively.
Statistical Analysis
Statistical analyses were performed with the software SPSS 18.0 (IBM, New York, NY, United States). Data were evaluated by one-way ANOVA, and the differences between the means were assessed using Duncan’s test. P < 0.05 was considered statistically significant.
Results
Growth Performance and SCFA Fermentation
The effect of different DF on piglet growth performance is presented in Table 2. There were no differences in ADFI, ADG, and F:G among treatments (P > 0.05). Compared with CG treatment, the mortality rate of piglets in AG had a decreasing trend (P > 0.05), while the diarrhea rate decreased significantly (P < 0.05). The diarrhea rate of piglets in OG also had a decreasing trend (P > 0.05) compared with CG treatment.
Different DF greatly influences the amount of SCFAs in the cecum of piglets (Figure 1A). Compared with CG treatment, there was an increasing trend in the amounts of acetic acid (AA) and butyric acid (BA) in AG and OG, and the amounts of propionic acid (PA) and isovaleric acid (ISOVA) in AG significantly increased (P < 0.05). Furthermore, the amounts of valeric acid (VA) and isobutyric acid (ISOBA) in AG significantly increased compared to both CG and OG (P < 0.05). There were no significant differences observed in the amounts of SCFAs in the colon of piglets (P > 0.05; Figure 1B).
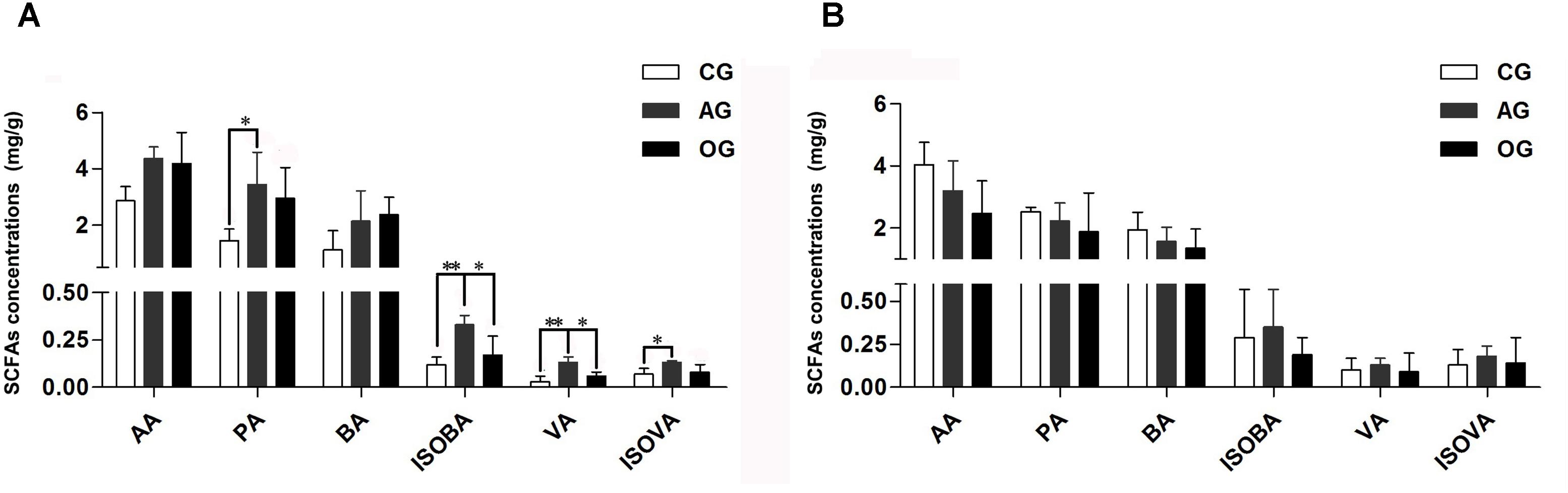
FIGURE 1. Effects of different DF on SCFAs fermentation of piglets. (A) SCFAs only cecum samples. (B) SCFAs only colon samples. AA, acetic acid; PA, propionic acid; BA, butyric acid; ISOBA, isobutyric acid; VA, valeric acid; ISOVA, isovaleric acid. ∗0.01 < P < = 0.05, ∗∗0.001 < P < = 0.01, ∗∗∗P < = 0.001.
Characterization of Microbiota Across the Gut and Feces
After removing incorrect and chimeric sequences, 2,033,322 sequencing reads were generated from the 54 samples. On average, 37,654 sequences per sample were obtained, with an average length of 443 bp. Using the criterion of 97% sequence similarity at the species level, 1751 OTUs were identified, all of which belonged to the bacteria domain according to Greengenes classification. Finally, on average, 417 ± 116 OTUs (Good’s coverage) per sample were identified. Rarefaction curves, Shannon curves, and Chao 1 curves were employed to analyze the richness and diversity of the microbiota community, as well as reflecting the data volume and sequencing depth (Supplementary Figure S1).
Diversity and Composition of Gut Microbiota According to DF Treatment
From the analyses of the effects of different DF on the richness and diversity of GFM in piglets, there were no significant differences in Chao 1 indices of GFM (P > 0.05; Supplementary Figure S2). The Shannon indices of the jejunum microbiota (J) in AG increased significantly compared with CG treatment (P = 0.031), but no significant differences were observed in the other GFM (P > 0.05; Figure 2).
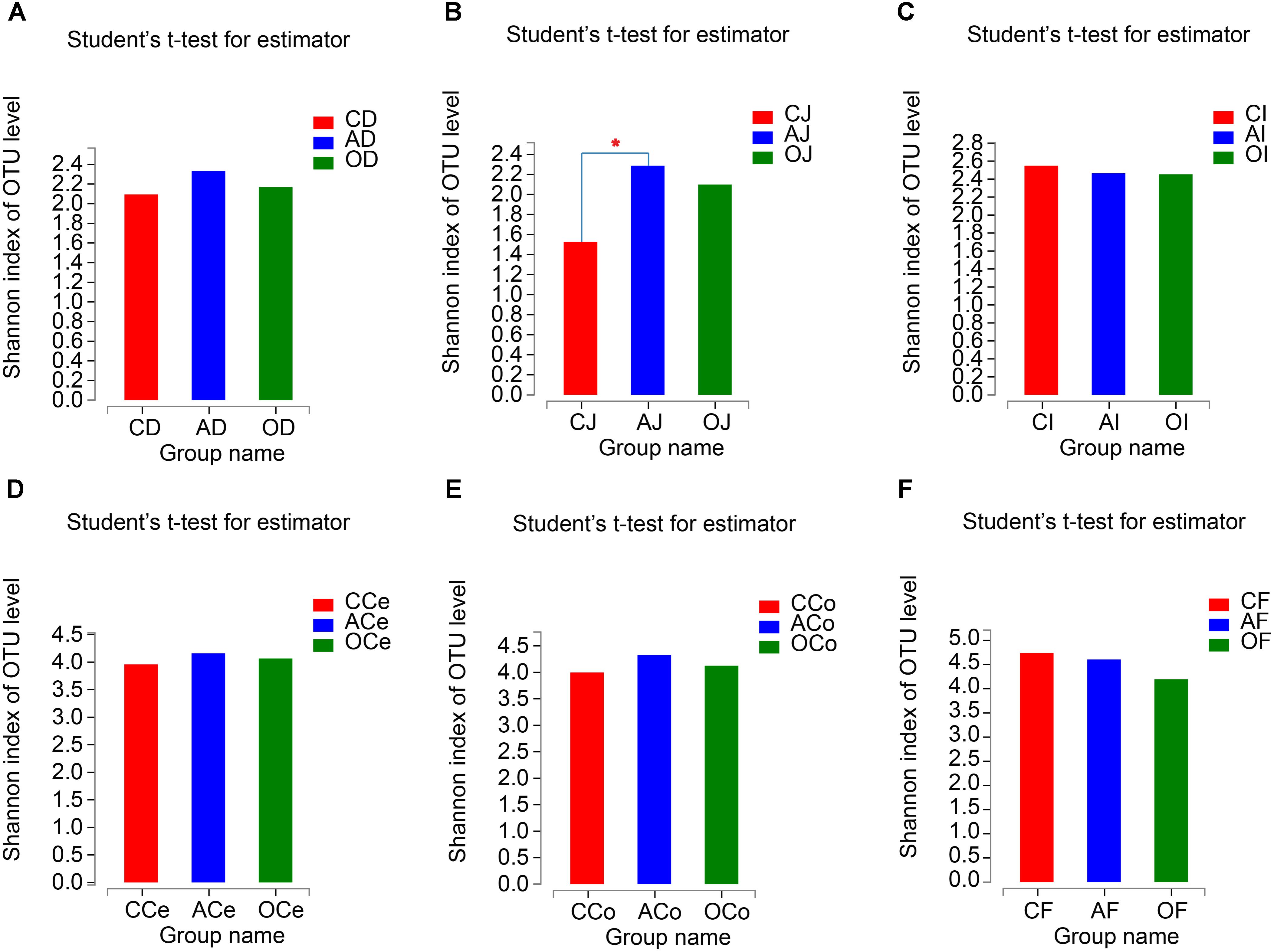
FIGURE 2. The Shannon Diversity Index Analyses of Microbiota Community. (A) Duodenum samples. (B) Jejunum samples. (C) Ileum samples. (D) Cecum samples. (E) Colon samples. (F) Feces samples. CD, AD, and OD: duodenum mucosal microbiota of control group, alfalfa meal group and commodity concentrated fiber group, respectively. CJ, AJ, and OJ: jejunum mucosal microbiota of control group, alfalfa meal group and commodity concentrated fiber group, respectively. CI, AI, and OI: ileum mucosal microbiota of control group, alfalfa meal group and commodity concentrated fiber group, respectively. CCe, ACe, and OCe: cecum mucosal microbiota of control group, alfalfa meal group and commodity concentrated fiber group, respectively. CCo, ACo, and OCo: colon mucosal microbiota of control group, alfalfa meal group and commodity concentrated fiber group, respectively. CF, AF, and OF: feces mucosal microbiota of control group, alfalfa meal group and commodity concentrated fiber group, respectively. ∗0.01 < P < = 0.05, ∗∗0.001 < P < = 0.01, ∗∗∗P < = 0.001.
At the phylum level (Supplementary Figure S3), the most abundant bacteria were Firmicutes, Bacteroidetes, Tenericutes, Proteobacteria, Actinobacteria, and Spirochaetes across GFM, with the different phyla occupying different dominant positions across GFM. In the microbiota of the duodenum (D), jejunum (J), and ileum (I), Firmicutes, Proteobacteria, and Tenericutes were the dominant phyla. Among these bacteria, Firmicutes represented a ratio of 60–90%. Bacteroidetes, Firmicutes, and Proteobacteria were the dominant phyla in cecum (Ce) and colon (Co) microbiota where Bacteroidetes and Firmicutes occupied 30–50%, respectively. The effects of different DF on the microbiota community across GFM at the phylum level are presented in Figure 3. The relative abundance of Firmicutes in jejunum microbiota of the AG (AJ) increased significantly compared with that in the CG (CJ) and the commodity concentrated fiber group (OJ) (P = 0.002 and P = 0.035, respectively), while the relative abundance of Tenericutes in AJ decreased significantly compared with that in CJ (P = 0.007). No differences were observed in the microbiota community of D, I, Ce, Co, and F among treatments (P > 0.05).
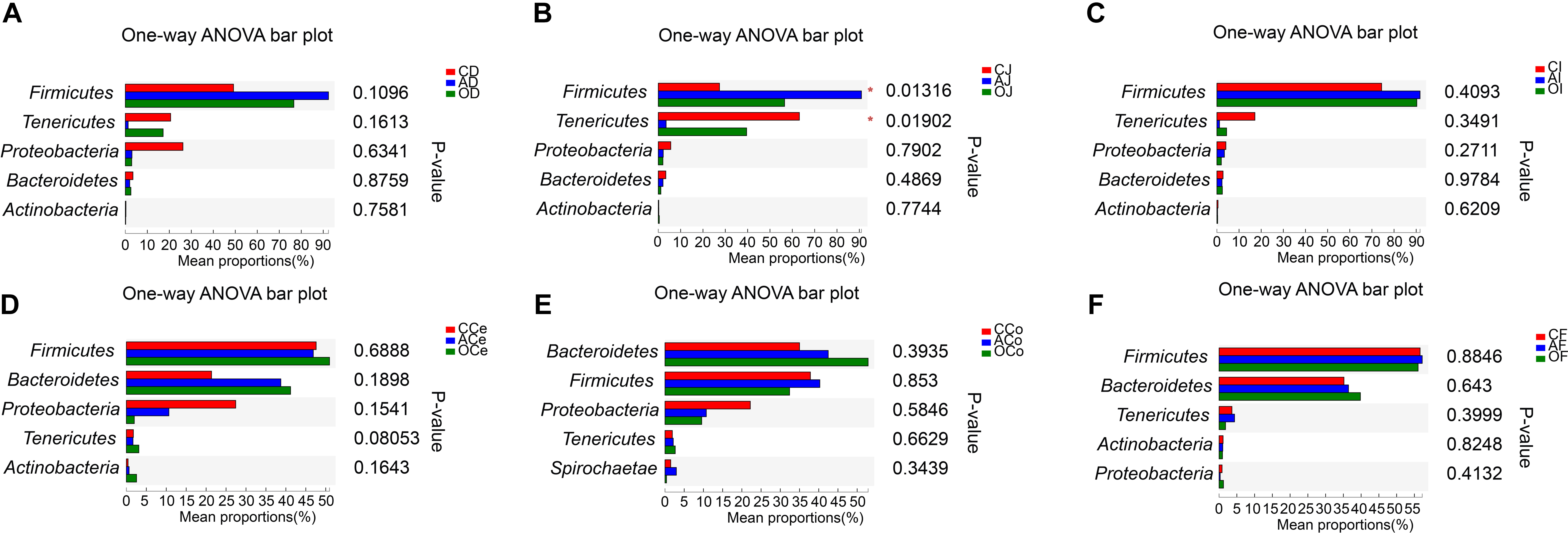
FIGURE 3. The Composition Analysis of Microbiota Community at the Phyla Level. (A) Duodenum samples. (B) Jejunum samples. (C) Ileum samples. (D) Cecum samples. (E) Colon samples. (F) Feces samples. CD, AD, and OD: duodenum mucosal microbiota of control group, alfalfa meal group and commodity concentrated fiber group, respectively. CJ, AJ, and OJ: jejunum mucosal microbiota of control group, alfalfa meal group and commodity concentrated fiber group, respectively. CI, AI, and OI: ileum mucosal microbiota of control group, alfalfa meal group and commodity concentrated fiber group, respectively. CCe, ACe, and OCe: cecum mucosal microbiota of control group, alfalfa meal group and commodity concentrated fiber group, respectively. CCo, ACo, and OCo: colon mucosal microbiota of control group, alfalfa meal group and commodity concentrated fiber group, respectively. CF, AF, and OF: feces mucosal microbiota of control group, alfalfa meal group and commodity concentrated fiber group, respectively. ∗0.01 < P < = 0.05, ∗∗0.001 < P < = 0.01, ∗∗∗P < = 0.001.
At the genus level (Supplementary Figure S4), the relative abundance of the microbiota community across GFM also varied. A total of 15 bacterial genera were detected in D, J, and I where the relative abundance of these bacteria represented more than 1%; Bacillus, Mycoplasma, Oceanobacillus, and Lactococcus were the dominant genera. Moreover, 55 bacterial genera were detected in Ce, Co, and F where the relative abundance of these bacteria represented more than 1%. Among these bacteria, Prevotella_9, Bacillus, Prevotellaceae_NK3B31_group, and Alloprevotella were the dominant genera. The effects of different DF on the microbiota community at the genus level across GFM are presented in Figure 4. Different DF significantly affected the relative abundance of different bacterial genera across GFM. In the duodenum, the relative abundance of Paenibacillus in the AG (AD) and the commodity concentrated fiber group (OD) increased significantly compared with that in the CG (CD; P = 0.009 and P = 0.046, respectively). In the jejunum, different DF greatly influenced the relative abundance of Bacillus, Oceanobacillus, Paenibacillus, Lactococcus, Enterococcus, Exiguobacterium, and Mycoplasma; all these bacteria except for Mycoplasma presented higher relative abundance in AJ than that in CJ (P = 0.003, P = 0.003, P = 0.014, P < 0.001, P = 0.034, and P = 0.001, respectively). The relative abundances of Bacillus, Oceanobacillus, Paenibacillus, and Exiguobacterium in AJ were also much higher than those in OJ (P = 0.046, P = 0.040, P = 0.012, and P = 0.049, respectively). In addition, the relative abundances of Paenibacillus and Exiguobacterium in OJ were significantly higher than that in CJ (P = 0.048 and P = 0.028, respectively). However, the relative abundance of Mycoplasma in AJ decreased significantly compared with that in CJ (P = 0.007). In the cecum, the relative abundance of Helicobacter in the AG (ACe) and the commodity concentrated fiber group (OCe) decreased significantly compared with that in the CG (CCe) (both P < 0.001). Furthermore, the relative abundance of Faecalibacterium in OCe was much higher than that in CCe and ACe (P = 0.003 and P = 0.004, respectively).
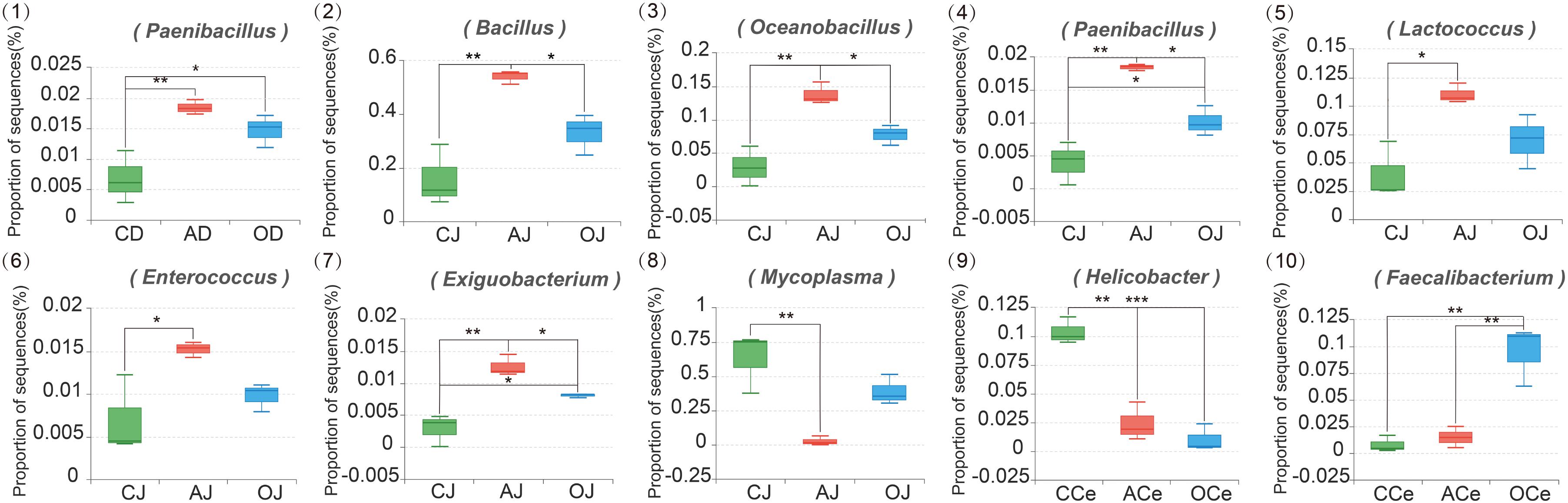
FIGURE 4. The Composition Analysis of Microbiota Community at the Genus Level. CD, AD, and OD: duodenum mucosal microbiota of control group, alfalfa meal group, and commodity concentrated fiber group, respectively. CJ, AJ, and OJ: jejunum mucosal microbiota of control group, alfalfa meal group, and commodity concentrated fiber group, respectively. CI, AI, and OI: ileum mucosal microbiota of control group, alfalfa meal group, and commodity concentrated fiber group, respectively. CCe, ACe, and OCe: cecum mucosal microbiota of control group, alfalfa meal group, and commodity concentrated fiber group, respectively. CCo, ACo, and OCo: colon mucosal microbiota of control group, alfalfa meal group, and commodity concentrated fiber group, respectively. CF, AF, and OF: feces mucosal microbiota of control group, alfalfa meal group, and commodity concentrated fiber group, respectively. ∗0.01 < P < = 0.05, ∗∗0.001 < P < = 0.01, ∗∗∗P < = 0.001.
To present the composition of the bacterial community directly, the relative abundance of the microbiota community was depicted by color intensity, and the community compositions were further clustered according to the similarity of species or relative abundance of samples, resulting in the heatmap presented in Figure 5. The microbiota in D, J, and I clustered together, as did the microbiota in Ce, Co, and F. It was observed that Firmicutes played a predominant role in D, J, and I while Bacteroidetes and Firmicutes were prevalent in Ce, Co, and F. In addition, the heatmap indicated that the diversity of microflora in Ce, Co, and F was much more varied than that in D, J, and I.
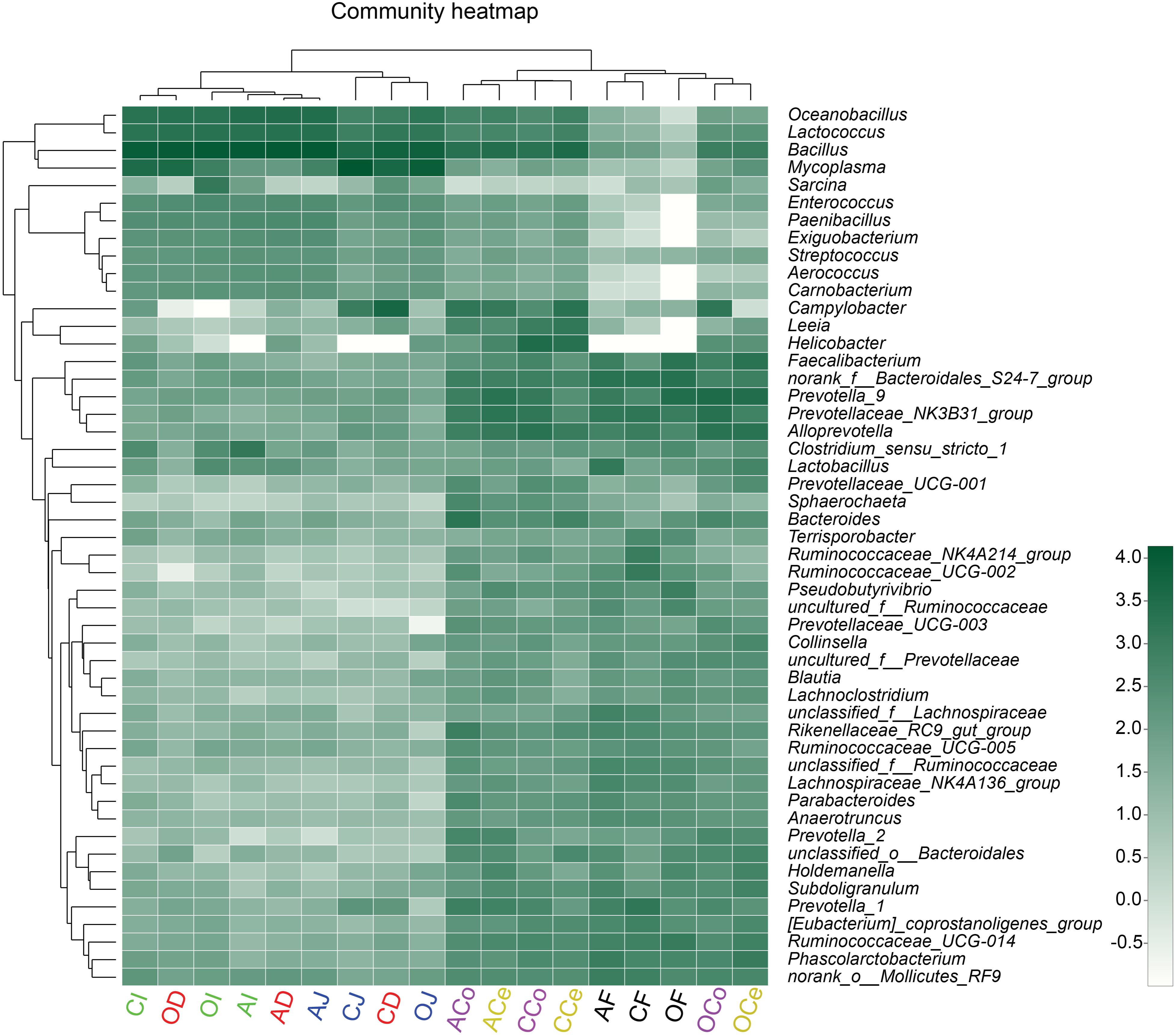
FIGURE 5. Heatmap of the microbiota composition in the gut and feces microbiota at the genus level. The relationship among species is determined by the complete clustering method with Bray–Curtis distance. The top tree showed the clustering relationship of samples. In the heatmap, green color means higher relative abundance whereas white color signifies lower relative abundance. CD, AD, and OD: duodenum mucosal microbiota of control group, alfalfa meal group, and commodity concentrated fiber group, respectively. CJ, AJ, and OJ: jejunum mucosal microbiota of control group, alfalfa meal group, and commodity concentrated fiber group, respectively. CI, AI, and OI: ileum mucosal microbiota of control group, alfalfa meal group, and commodity concentrated fiber group, respectively. CCe, ACe, and OCe: cecum mucosal microbiota of control group, alfalfa meal group, and commodity concentrated fiber group, respectively. CCo, ACo, and OCo: colon mucosal microbiota of control group, alfalfa meal group, and commodity concentrated fiber group, respectively. CF, AF, and OF: feces mucosal microbiota of control group, alfalfa meal group, and commodity concentrated fiber group, respectively.
Diversity and Composition of Gut Microbiota Across the GFM
From the richness and diversity analyses of microbiota community across the GFM of piglets, the Shannon and Chao 1 indices of proximal intestine (D, J, and I) microbiota decreased significantly compared with those of distal intestine and feces (Ce, Co, and F) microbiota (Supplementary Figure S5). Analysis of differences among the microbiota community across GFM at the phylum and genus level are presented in Supplementary Figure S6. There were differences among GFM; in particular, there were significant differences between small and large intestine microbiota. At the phylum level, compared to distal intestine, the relative abundance of Firmicutes in the proximal intestine was higher, while the relative abundance of Bacteroidetes was lower. At the genus level, compared to the distal intestine, the relative abundances of Bacillus, Mycoplasma, Oceanobacillus, and Lactococcus in the proximal intestine were higher, while the relative abundances of Prevotella_9, Prevotellaceae_NK3B31_group, Alloprevotella, anorank_f_Bacteroidales_S24-7_group, Faecalibacterium, Prevotella_1, Ruminococcaceae_UCG-014, norank_o_Mollicutes_RF9, and Phascolarctobacterium were lower. To further study similarities and differences among GFM, cluster tree and PCoA analysis was conducted (Supplementary Figure S7). It was clearly observed that the samples of the proximal intestine were distinct from those in the distal intestine.
Association and Model Predictive Analysis
Redundancy analysis was conducted based on all samples of Ce and Co and their environmental factors (SCFAs, pH; Figures 6A,B). The correlation between microbiota distribution and environmental factors were as follows: BA > PA > AA > pH > VA > ISOVA > ISOBA in Ce, and BA > AA > PA > pH > ISOBA > VA > ISOVA in Co. In addition, RDA indicated that there was positive correlation among BA, PA, and AA, and negative correlation between pH and these three major SCFAs. Moreover, the correlation among these three major SCFAs is relatively tight in both Ce and Co. Correlation analysis was conducted between the top 50 bacterial genera and the environmental factors, and is directly reflected by a heatmap (Figure 6C). The threshold |R| > 0.4 is considered as having correlation. The results indicated that Bacteroides is positively correlated with pH, while Pseudobutyrivibrio, Megamonas, and Lactobacillus are negatively correlated with pH. Pseudobutyrivibrio is positively correlated with AA, while uncultured_f_Ruminococcaceae and unclassified_f_Ruminococcaceae are negatively correlated with this SCFA. Pseudobutyrivibrio is also positively correlated with PA and BA, but Anaerotruncus and Bacteroides are negatively correlated with them. Prevotella_1, Ruminococcaceae_UCG-005, Prevotellaceae_NK3B31_group, Anaerovibrio, and Lachnospiraceae_NK4A136_group are positively correlated with ISOBA.Prevotella_1, Prevotellaceae_NK3B31_group, and Prevotellaceae_UCG-003 are positively correlated with VA. Prevotellaceae_NK3B31_group is positively correlated with ISOVA, but Faecalibacterium, Phascolarctobacterium, Lactobacillus, and Megamonas are negatively correlated with this SCFA.
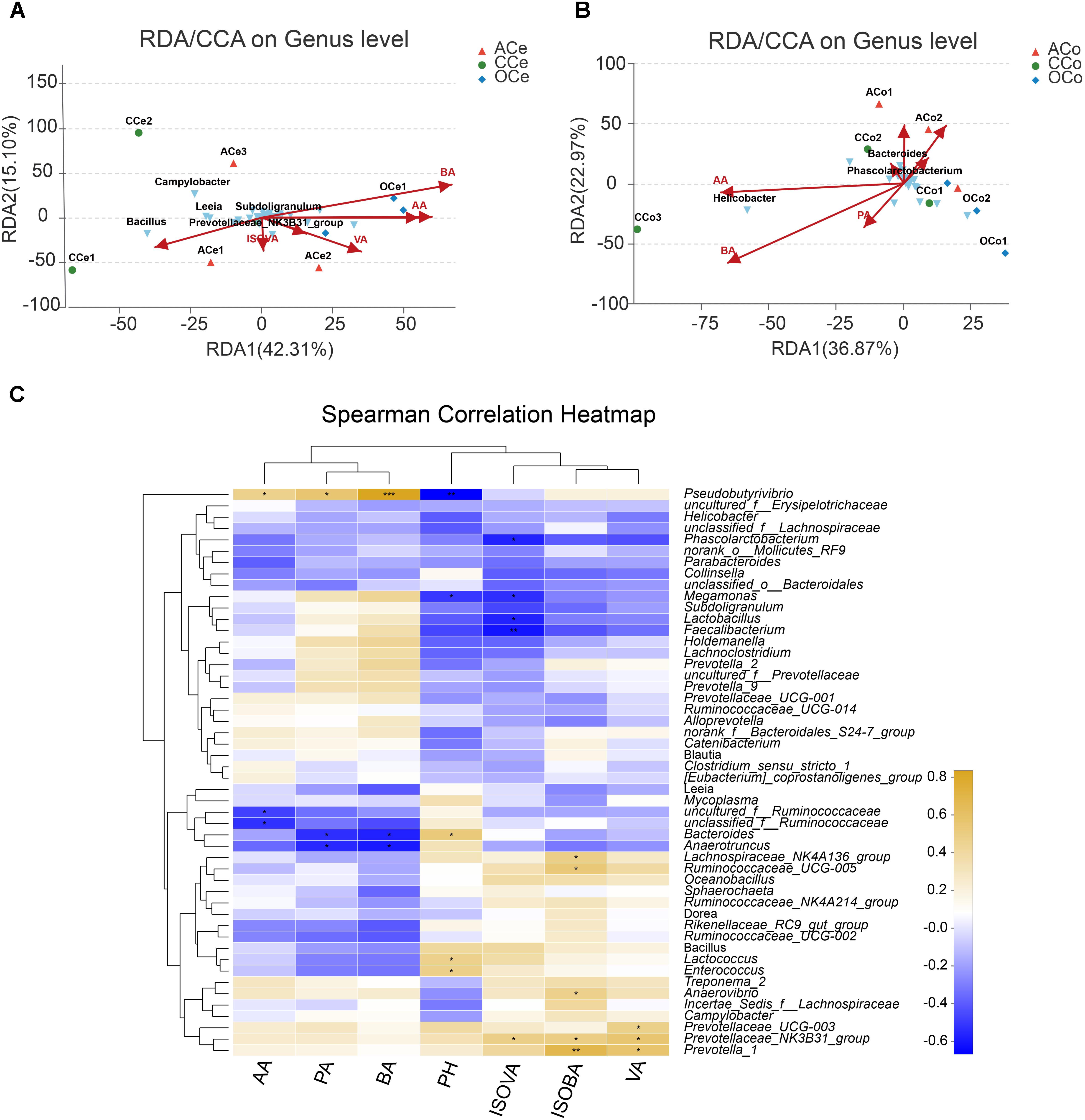
FIGURE 6. Association and model predictive analysis. (A) RDA targeting only cecum samples. (B) RDA targeting only colon samples. (C) Heatmap of the correlation analysis was conducted between the top 50 bacterial genera and the environmental factors. AA, acetic acid; PA, propionic acid; BA, butyric acid; ISOBA, isobutyric acid; VA, valeric acid; ISOVA, isovaleric acid. ∗0.01 < P < = 0.05, ∗∗0.001 < P < = 0.01, ∗∗∗P < = 0.001.
Discussion
Attempts have been made to add fiber to the diet of weaning piglets to make the piglets adapt quickly to the thicker feed during the growing season (Molist et al., 2014). However, some of the results concerning the effects of adding fiber to diets on growth performance in piglets were inconsistent (Freire et al., 2000; Hogberg and Lindberg, 2004; Jeaurond et al., 2008). Lindberg (2014) also confirmed that the type and origin of the fiber determined the effect of the fiber on the swine diet. In our study, the addition of fiber from different sources to piglet diets had no effects on ADFI, ADG, and F:G in piglets. However, compared to the CG, the diarrhea rate of piglets fed with alfalfa meal significantly reduced. From the perspective of fermentability of the fiber, alfalfa meal is rich in insoluble fiber (cellulose) but also has a little soluble fiber (pectins) (Brambillasca et al., 2015). Similarly, the commercial fiber used in this study is an insoluble, slowly fermented fiber. Gerritsen et al. (2012) reported that the addition of 15% insoluble non-starch polysaccharides (NSP) to the low-protein diet of piglets had no effect on the performance of piglets, but promoted development of the intestine and affected the colon microbiota. In addition, previous studies have shown that insoluble NSP can reduce the residence time of chyme in the gastrointestinal tract and alleviate the anorexia and digestive disorders of piglets caused by gut stasis, thereby reducing diarrhea in piglets (Molist et al., 2012). In the current study, the PA content in the cecum was markedly increased by alfalfa meal treatment, demonstrating that the fiber in the alfalfa meal appears to have a prebiotic effect. The main fermentation products of DF in the hindgut are SCFAs. Among them, AA, PA, and BA are the most abundant, comprising 90–95% of SCFAs present in the hindgut (Ríos-Covián et al., 2016). Fermentable fibers such as oligosaccharides and soluble NSP are usually fermented in the ileum, while the slowly fermented insoluble NSP can provide a growth medium for the microbiota until the end of the large intestine. It is well known that SCFAs play an important role in maintaining the morphology and function of epithelial cells. Butyrate is metabolized by hindgut cells as the main energy source, and the rest can be transported to the liver and used in different biosynthetic pathways. Propionate is mainly involved in the process of gluconeogenesis (Zhang Q. et al., 2016), while acetate and butyrate are predominantly involved in lipid biosynthesis (den Besten et al., 2013; Ríos-Covián et al., 2016). In addition, SCFAs act as specific G protein-coupled receptor (GPR) signaling molecules and are involved in the regulation of glucose and lipid metabolism (den Besten et al., 2013). Furthermore, Shibata et al. (2017) reported that SCFAs can decrease pH of the gut and inhibit the colonization and growth of some pathogens such as E. coli and Salmonella. The findings in the present study indicated that alfalfa meal treatment could generate more SCFAs and promote gut health of the piglets, which are closely correlated with the decreased diarrhea rate of piglets in the alfalfa meal treatment.
With the rapid development of sequencing technology in recent years, more researchers are using Illumina MiSeq sequencing which is lower in cost and has a higher sequencing depth and coverage than 454 pyrosequencing (Fouhy et al., 2015). In this study, we employed Illumina MiSeq sequencing to investigate the effects of different DF on the richness and diversity across GFM, and found that different DF had varied influences on microbiota of the same intestinal segment. In general, Chao1 and Shannon indices of GFM showed an increasing trend under the fiber treatment. In particular, the Shannon index of the jejunum microbiota was significantly increased by alfalfa meal treatment, indicating that fiber treatment could increase the richness and diversity of GFM, and subsequently promote the health of piglets. Gresse et al. (2017) also reported that the addition of a certain amount of fiber to the basal diet of piglets could increase the diversity of GFM.
Dietary fiber plays an important role in maintaining gut microbiota balance and gut health. As Castillo et al. (2007) reported, the host gut microbiota vary in response to the composition of DF due to the specific substrate preference of the bacteria. Therefore, the composition of gut microbiota could be regulated by supplementation with specific DF. Zhang D. et al. (2016) have shown that fiber from alfalfa sources in piglet diets can regulate gut microbiota and promote gut health. In this study, analysis of microbiota data at phylum level showed that alfalfa meal treatment significantly increased the relative abundance of Firmicutes and decreased the relative abundance of Tenericutes in the jejunum. These changes seemed to have a positive effect on gut health of piglets. This may be explained by the fact that Firmicutes mainly includes beneficial bacteria, while Tenericutes mainly includes harmful bacteria. Further analysis at the genus level indicated that DF treatment significantly increased the relative abundance of Paenibacillus, Bacillus, Oceanobacillus, Lactococcus, Enterococcus, Exiguobacterium, and Faecalibacterium, while significantly decreasing the relative abundance of Helicobacter and Mycoplasma. Numerous studies have reported on the role of these bacterial genera in gut health. Members of the genus Paenibacillus are able to produce antibacterial and antifungal compounds against plant and animal pathogens (Youngryun et al., 2000). Bacillus, Lactococcus, and Enterococcus faecalis have beneficial effects on reducing mortality, forming antimicrobial compounds, inhibiting the growth of pathogens, and regulating activation of the immune system (Lee and Kim, 2011; Hu et al., 2015). Faecalibacterium is involved in butyrate synthesis, which is the main nutrient for the regeneration and repair of gut epithelial cells (Lopezsiles et al., 2017). However, infection with Helicobacter is one of the major inducers of chronic active gastritis and peptic ulcer (Heimesaat et al., 2014). The family Mycoplasmataceae is known to infect pigs (Deblanc et al., 2016). In this study, there was a high relative abundance of Mycoplasma sualvi and Helicobacter in the pigs consuming control feed, which might result in gut inflammation, and subsequently piglet diarrhea. In conclusion, alteration in gut microbiota induced by DF treatment resulted in an improvement in gut health, potentially explaining the reduction of piglet diarrhea.
Interestingly, DF could regulate the gut microbiota on one hand, while on the other hand, some bacteria were also involved in the metabolic processing of DF. In the current study, the amounts of Exiguobacterium and Paenibacillus in fiber treatment significantly increased. Exiguobacterium plays key roles in the fermentation of cellulose and the transglycosylation of sugars because of its high β-glucosidase activity (Gao et al., 2015). Paenibacillus sp. HY-8 has high activity of GH11β-1,4-xylanase and extracellular endo-β-1,4-mannanase, promoting the degradation of xylan and mannan (Heo et al., 2006; Kim et al., 2017). Similarly, Zhao et al. (2018) found that high insoluble DF increased the relative abundance of fiber-degrading bacteria in pig feces. Further study on the correlation analysis of fermentation products of DF (SCFAs) and microbiota could provide additional insights into their interactions.
From the perspective of the diversity of microbiota in different parts of the intestines, this study indicated that the gut microbiota varied greatly between proximal and distal intestines, but the difference between adjacent intestinal segments was relatively small. In the proximal intestine, the predominant microbiota was Firmicutes, mainly containing Bacillus, Mycoplasma, Oceanobacillus, Lactococcus, and other organisms. For the distal intestine, the prevailing microbiota were Bacteroidetes and Firmicutes, includingPrevotella_9, Prevotellaceae_NK3B31_group, Alloprevotella,anorank_f_Bacteroidales_S24-7_group, Faecalibacterium, Prevotella_1, Ruminococcaceae_UCG-014, norank_o_Mollicutes_RF9, Phascolarctobacterium, and so on. These results were consistent with the distribution of gut microbiota of other species (Mu et al., 2017). The observed differences in microbiota between proximal and distal intestines were closely related to the function and environment of different intestine segments. The function of the proximal segment is mainly digestion and absorption of protein, fat, and starch, while the hindgut is a strictly anaerobic environment; therefore, the majority of bacteria colonizing the posterior segment are strict anaerobes and facultative anaerobes. DF can be decomposed by microbiota fermentation in the hindgut to generate SCFAs as energy for epithelial cells, which was in agreement with the increase of SCFAs in the cecum and colon.
The micro-ecosystem of the posterior segment of the pig gut is very complicated as approximately 400–500 species and about 1012 microbiota per gram of intestine exist in this segment (Castillo et al., 2007). To date, studies have mainly focused on the species and characteristics of porcine posterior hyphae-degrading bacteria (Shuchi et al., 2013; Christopherson et al., 2014), and there is limited research on the correlation between hindgut fiber-degrading bacteria and SCFAs generated by fiber fermentation. Taking into account that specific microbiota in the gut may be involved in the digestion and metabolism of the fiber, further investigations were performed in the current study to analyze the metabolic interaction of gut microbiota with fermentation products of DF in the hindgut. Using RDA/CCA analysis, AA, PA, and BA were significantly associated with cecum and colon microbiota. Furthermore, Spearman correlation analysis on the top 50 microbiota genera and the environmental factors in the hindgut showed that the microbiota bacteria with positive effects on SCFAs were Pseudobutyrivibrio, Ruminococcaceae_UCG-005, Prevotellaceae_NK3B31_group, Lachnospiraceae_NK4A136_group, Anaerovibrio, Prevotellaceae_ UCG-003, and so on, while the microbiota negatively correlated with SCFAs were Bacteroides and Anaerotruncus. Previous research found that Bacteroides are the most prevalent anaerobic bacteria in the hindgut. Bacteroides fragilis comprises only 2% of the total Bacteroides in the gut but causes more than 70% of Bacteroides infections. In contrast, Pseudobutyrivibrio decomposes various carbohydrates, mainly generating BA as well as formic acid and lactic acid (Zhang et al., 2017). The metabolic process of the family Ruminococcaceae is a typical type of mixed fermentation, producing AA, formic acid, and a small amount of lactic acid (Biddle et al., 2013). The main fermentation products generated by Prevotella are AA, succinic acid, a small amount of ISOBA, ISOVA, and lactic acid (Kovatcheva-Datchary et al., 2015). All of these investigations in our study suggested that specific bacteria in the hindgut were involved in the process of digestion and metabolism of the DF, subsequently affecting generation of SCFAs and inhibiting colonization of some harmful bacteria, and finally possibly influencing the whole network of nutrient and energy metabolism, which was in agreement with the reports of Lin et al. (2017). However, the interaction of gut microbiota with SCFAs and their response mechanism to DF requires further study.
Conclusion
Diets containing alfalfa meal and commodity concentrated fiber had positive effects on growth performance and SCFAs metabolism of piglets. In particular, the diarrhea rate of piglets was significantly decreased, and the content of PA in the cecum was markedly increased by AG treatment. Furthermore, DF had beneficial effects on bacterial richness and diversity, as well as gut microbiota composition. Piglets with fiber treatment had greater proportions of beneficial bacteria and fiber-degrading bacteria, whereas harmful bacteria were significantly decreased. Further investigation indicated that there were close metabolic interactions between hindgut microbiota and SCFAs. These results suggested that DF reduced the diarrhea rate of piglets and had prebiotic effects on gut health, which might be attributed to the alteration in gut microbiota induced by DF and their interaction with SCFAs. In addition, the microbiota composition of gut and feces samples in piglets was systematically analyzed to provide theoretical support for the findings of microbiota differences between the proximal and distal intestines. These findings will facilitate the development of strategies for the regulation of gut microbiota through DF to improve piglet health and productivity.
Ethics Statement
This study was performed strictly according to the recommendations of the Guide for the Care and Use of Laboratory Animals of the Ministry of Health, China. The research protocol was reviewed and approved by the Research Ethics Committee of Henan Agricultural University. Permission was obtained from the farm owners before the samples were collected. Not involved in these situations.
Author Contributions
YS conceived and designed the experiments. BL, WW, and XZ performed the experiments and wrote the paper. XS and JX analyzed the data. DL, YC, and CW contributed reagents, materials, and analysis tools.
Funding
Financial support for this research was provided by the Earmarked Fund for Modern Agro-industry Technology Research System of China (CARS-34) and 948 Project of Ministry of Agriculture of China (2015-Z46).
Conflict of Interest Statement
The authors declare that the research was conducted in the absence of any commercial or financial relationships that could be construed as a potential conflict of interest.
Supplementary Material
The Supplementary Material for this article can be found online at: https://www.frontiersin.org/articles/10.3389/fmicb.2018.02344/full#supplementary-material
Footnotes
References
Alfa, M. J., Strang, D., Tappia, P. S., Graham, M., Van, D. G., Forbes, J. D., et al. (2017). A randomized trial to determine the impact of a digestion resistant starch composition on the gut microbiome in older and mid-age adults. Clin. Nutr. 37, 797–807. doi: 10.1016/j.clnu.2017.03.025
Berrocoso, J. D., Saldaña, B., Serrano, M. P., Cámara, L., Ibáñez, M. A., and Mateos, G. G. (2013). Influence of crude protein content, ingredient complexity, feed form, and duration of feeding of the Phase I diets on productive performance and nutrient digestibility of Iberian pigs. J. Anim. Sci. 91, 1237–1246. doi: 10.2527/jas.2012-5448
Biddle, A., Stewart, L., Blanchard, J., and Leschine, S. (2013). Untangling the genetic basis of fibrolytic specialization by Lachnospiraceae and Ruminococcaceae in diverse gut communities. Diversity 5, 627–640. doi: 10.3390/d5030627
Brambillasca, S., Zunino, P., and Cajarville, C. (2015). Addition of inulin, alfalfa and citrus pulp in diets for piglets: influence on nutritional and faecal parameters, intestinal organs, and colonic fermentation and bacterial populations. Livest. Sci. 178, 243–250. doi: 10.1016/j.livsci.2015.06.003
Canibe, N., and Bach Knudsen, K. E. (2002). Degradation and physicochemical changes of barley and pea fibre along the gastrointestinal tract of pigs. J. Sci. Food Agric. 82, 27–39. doi: 10.1002/jsfa.985
Castillo, M., Martín-Orúe, S. M., Nofrarías, M., Manzanilla, E. G., and Gasa, J. (2007). Changes in caecal microbiota and mucosal morphology of weaned pigs. Vet. Microbiol. 124, 239–247. doi: 10.1016/j.vetmic.2007.04.026
Christopherson, M. R., Dawson, J. A., Stevenson, D. M., Cunningham, A. C., Bramhacharya, S., Weimer, P. J., et al. (2014). Unique aspects of fiber degradation by the ruminal ethanologen Ruminococcus albus 7 revealed by physiological and transcriptomic analysis. BMC Genomics 15:1066. doi: 10.1186/1471-2164-15-1066
Deblanc, C., Delgadoortega, M., Gorin, S., Berri, M., Paboeuf, F., Berthon, P., et al. (2016). Mycoplasma hyopneumoniae does not affect the interferon-related anti-viral response but predisposes the pig to a higher inflammation following swine influenza virus infection. J. Gen. Virol. 97, 2501–2515. doi: 10.1099/jgv.0.000573
den Besten, G., Lange, K., Havinga, R., van Dijk, T. H., Gerding, A., Van, E. K., et al. (2013). Gut-derived short-chain fatty acids are vividly assimilated into host carbohydrates and lipids. Am. J. Physiol. Gastrointest. Liver Physiol. 305, G900–G910. doi: 10.1152/ajpgi.00265.2013
Filippo, C. D., and Hartl, D. L. (2010). Impact of diet in shaping gut microbiota revealed by a comparative study in children from Europe and rural Africa. Proc. Natl. Acad. Sci. U.S.A. 107, 14691–14696. doi: 10.1073/pnas.1005963107
Fouhy, F., Deane, J., Rea, M. C., O’Sullivan,Ó, Ross, R. P., O’Callaghan, G., et al. (2015). The effects of freezing on faecal microbiota as determined using miseq sequencing and culture-based investigations. PLoS One 10:e0119355. doi: 10.1371/journal.pone.0119355
Freire, J. P. B., Guerreiro, A. J. G., Cunha, L. F., and Aumaitre, A. (2000). Effect of dietary fibre source on total tract digestibility, caecum volatile fatty acids and digestive transit time in the weaned piglet. Anim. Feed Sci. Technol. 87, 71–83. doi: 10.1016/S0377-8401(00)00183-8
Gao, L., Chen, L., Huang, Q., Meng, L., Zhong, R., Liu, C., et al. (2015). Effect of dietary fiber type on intestinal nutrient digestibility and hindgut fermentation of diets fed to finishing pigs. Livest. Sci. 174, 53–58. doi: 10.1016/j.livsci.2015.01.002
Gerritsen, R., van der Aar, P., and Molist, F. (2012). Insoluble nonstarch polysaccharides in diets for weaned piglets. J. Anim. Sci. 90(Suppl. 4), 318–320. doi: 10.2527/jas.53770
Gresse, R., Chaucheyrasdurand, F., Fleury, M. A., Van de Wiele, T., Forano, E., and Blanquetdiot, S. (2017). Gut microbiota dysbiosis in postweaning piglets: understanding the keys to health. Trends Microbiol. 25, 851–873. doi: 10.1016/j.tim.2017.05.004
Heimesaat, M. M., Fischer, A., Plickert, R., Wiedemann, T., Loddenkemper, C., Göbel, U. B., et al. (2014). Helicobacter pylori induced gastric immunopathology is associated with distinct microbiota changes in the large intestines of long-term infected mongolian gerbils. PLoS One 9:e100362. doi: 10.1371/journal.pone.0100362
Heinritz, S. N., Weiss, E., Eklund, M., Aumiller, T., Louis, S., Rings, A., et al. (2016). Intestinal microbiota and microbial metabolites are changed in a pig model fed a high-fat/low-fiber or a low-fat/high-fiber diet. PLoS One 11:e0154329. doi: 10.1371/journal.pone.0154329
Heo, J. M., Opapeju, F. O., Pluske, J. R., Kim, J. C., Hampson, D. J., and Nyachoti, C. M. (2013). Gastrointestinal health and function in weaned pigs: a review of feeding strategies to control post-weaning diarrhoea without using in-feed antimicrobial compounds. J. Anim. Physiol. Anim. Nutr. 97, 207–237. doi: 10.1111/j.1439-0396.2012.01284.x
Heo, S. Y., Kwak, J. Y., Oh, H. W., Park, D. S., Bae, K. S., Shin, D. H., et al. (2006). Characterization of an extracellular xylanase in Paenibacillus sp. hy-8 isolated from an herbivorous longicorn beetle. J. Microbiol. Biotechnol. 16, 1753–1759.
Hogberg, A., and Lindberg, J. E. (2004). Influence of cereal non-starch polysaccharides and enzyme supplementation on digestion site and gut environment in weaned piglets. Anim. Feed Sci. Technol. 116, 113–128. doi: 10.1016/j.anifeedsci.2004.03.010
Hu, Y., Dun, Y., Li, S., Zhang, D., Peng, N., Zhao, S., et al. (2015). Dietary Enterococcus faecalis LAB31 improves growth performance, reduces diarrhea, and increases fecal lactobacillus number of weaned piglets. PLoS One 10:e0116635. doi: 10.1371/journal.pone.0116635
Jeaurond, E. A., Rademacher, M., Pluske, J. R., Zhu, C. H., and Cfm, D. L. (2008). Impact of feeding fermentable proteins and carbohydrates on growth performance, gut health and gastrointestinal function of newly weaned pigs. Can. J. Anim. Sci. 88, 271–281. doi: 10.4141/CJAS07062
Jiang, X. T., Peng, X., Deng, G. H., Sheng, H. F., Wang, Y., Zhou, H. W., et al. (2013). Illumina sequencing of 16S rRNA tag revealed spatial variations of bacterial communities in a mangrove wetland. Microb. Ecol. 66, 96–104. doi: 10.1007/s00248-013-0238-8
Kim, D. Y., Chung, C. W., Cho, H.-Y., Rhee, Y. H., Shin, D.-H., Son, K.-H., et al. (2017). Biocatalytic characterization of an endo-β-1,4-mannanase produced by Paenibacillus sp. strain HY-8. Biotechnol. Lett. 39, 149–155. doi: 10.1007/s10529-016-2228-7
Kovatcheva-Datchary, P., Nilsson, A., Akrami, R., Lee, Y. S., De, V. F., Arora, T., et al. (2015). Dietary fiber-induced improvement in glucose metabolism is associated with increased abundance of prevotella. Cell Metab. 22, 971–982. doi: 10.1016/j.cmet.2015.10.001
Lallès, J. P., Bosi, P., Smidt, H., and Stokes, C. R. (2007). Weaning — A challenge to gut physiologists. Livest. Sci. 108, 82–93. doi: 10.1016/j.livsci.2007.01.091
Lee, H., and Kim, H. Y. (2011). Lantibiotics, class I bacteriocins from the genus Bacillus. J. Microbiol. Biotechnol. 21, 229–235.
Li, Y. B., and Zhang, M. (2006). Effect of dietary fiber on performance andtrophic diarherra in piglets. Feed Ind. 5, 28–30.
Lin, D., Peters, B., Sinha, R., Goedert, J. J., Hayes, R., and Ahn, J. (2017). Association of dietary fiber intake and gut microbiota in healthy adults. J. Clin. Oncol. 35, 1569–1569. doi: 10.1200/JCO.2017.35.15_suppl.1569
Lindberg, J. E. (2014). Fiber effects in nutrition and gut health in pigs. J. Anim. Sci. Biotechnol. 5, 273–279. doi: 10.1186/2049-1891-5-15
Liu, H. Y., Lundh, T., Dicksved, J., and Lindberg, J. E. (2012). Expression of heat shock protein 27 in gut tissue of growing pigs fed diets without and with inclusion of chicory fiber. J. Anim. Sci. 90(Suppl. 4), 25–27. doi: 10.2527/jas.53724
Lopezsiles, M., Duncan, S. H., Garciagil, L. J., and Martinezmedina, M. (2017). Faecalibacterium prausnitzii: from microbiology to diagnostics and prognostics. ISME J. 11, 841–852. doi: 10.1038/ismej.2016.176
Lozupone, C., Lladser, M. E., Knights, D., Stombaugh, J., and Knight, R. (2011). UniFrac: an effective distance metric for microbial community comparison. ISME J. 5, 169–172. doi: 10.1038/ismej.2010.133
Mateos, G. G., Martin, F., Latorre, M. A., Vicente, B., and Lazaro, R. (2006). Inclusion of oat hulls in diets for young pigs based on cooked maize or cooked rice. Animalence 82, 57–63.
Molist, F., Manzanilla, E. G., Pérez, J. F., and Nyachoti, C. M. (2012). Coarse, but not finely ground, dietary fibre increases intestinal Firmicutes: bacteroidetes ratio and reduces diarrhoea induced by experimental infection in piglets. Br. J. Nutr. 108, 9–15. doi: 10.1017/S0007114511005216
Molist, F., Oostrum, M. V., Pérez, J. F., Mateos, G. G., Nyachoti, C. M., and van der Aar, P. J. (2014). Relevance of functional properties of dietary fibre in diets for weanling pigs. Anim. Feed Sci. Technol. 189, 1–10. doi: 10.1016/j.anifeedsci.2013.12.013
Molist, F., Segura, A. G. D., Pérez, J. F., Bhandari, S. K., Krause, D. O., and Nyachoti, C. M. (2010). Effect of wheat bran on the health and performance of weaned pigs challenged with Escherichia coli K88 +. Livest. Sci. 133, 214–217. doi: 10.1016/j.livsci.2010.06.067
Mu, C., Yang, Y., Su, Y., Zoetendal, E. G., and Zhu, W. (2017). Differences in microbiota membership along the gastrointestinal tract of piglets and their differential alterations following an early-life antibiotic intervention. Front. Microbiol. 8:797. doi: 10.3389/fmicb.2017.00797
Ríos-Covián, D., Ruasmadiedo, P., Margolles, A., Gueimonde, M., de los Reyesgavilán, C. G., and Salazar, N. (2016). Intestinal short chain fatty acids and their link with diet and human health. Front. Microbiol. 7:185. doi: 10.3389/fmicb.2016.00185
Schloss, P. D., Westcott, S. L., Ryabin, T., Hall, J. R., Hartmann, M., Hollister, E. B., et al. (2009). Introducing mothur: open-source, platform-independent, community-supported software for describing and comparing microbial communities. Appl. Environ. Microbiol. 75, 7537–7541. doi: 10.1128/AEM.01541-09
Schroeder, B. O., and Bäckhed, F. (2016). Signals from the gut microbiota to distant organs in physiology and disease. Nat. Med. 22, 1079–1089. doi: 10.1038/nm.4185
Shibata, N., Kunisawa, J., and Kiyono, H. (2017). Dietary and microbial metabolites in the regulation of host immunity. Front. Microbiol. 8:2171. doi: 10.3389/fmicb.2017.02171
Shuchi, S., Moholkar, V. S., and Arun, G. (2013). Isolation, identification, and characterization of a cellulolyticbacillus amyloliquefaciensstrain ss35 from rhinoceros dung. ISRN Microbiol. 2013:728134. doi: 10.1155/2013/728134
Singh, K. M., Jisha, T. K., Reddy, B., Parmar, N., Patel, A., Patel, A. K., et al. (2015). Microbial profiles of liquid and solid fraction associated biomaterial in buffalo rumen fed green and dry roughage diets by tagged 16S rRNA gene pyrosequencing. Mol. Biol. Rep. 42, 95–103. doi: 10.1007/s11033-014-3746-9
Yang, Y. X., Mu, C. L., Luo, Z., and Zhu, W. Y. (2015). Bromochloromethane, a methane analogue, affects the gut microbiota and metabolic profiles of the rat gastrointestinal tract. Appl. Environ. Microbiol. 82, 778–787. doi: 10.1128/AEM.03174-15
Youngryun, C., Chunghwan, K., Inhwan, H., and Jongsik, C. (2000). Paenibacillus koreensis sp. nov., a new species that produces an iturin-like antifungal compound. Int. J. Syst. Evol. Microbiol. 50(Pt 4), 1495–1500.
Zhang, D., Ji, H., Liu, H., Wang, S., Wang, J., and Wang, Y. (2016). Changes in the diversity and composition of gut microbiota of weaned piglets after oral administration of Lactobacillus or an antibiotic. Appl. Microbiol. Biotechnol. 100, 1–13. doi: 10.1007/s00253-016-7845-5
Zhang, Q., Koser, S. L., and Donkin, S. S. (2016). Propionate induces mRNA expression of gluconeogenic genes in bovine calf hepatocytes. J. Dairy Sci. 99, 3908–3915. doi: 10.3168/jds.2015-10312
Zhang, L., Chung, J., Jiang, Q., Sun, R., Zhang, J., Zhong, Y., et al. (2017). Characteristics of rumen microorganisms involved in anaerobic degradation of cellulose at various pH values. RSC Adv. 7, 40303–40310. doi: 10.1039/C7RA06588D
Keywords: dietary fiber, short-chain fatty acid, gut microbiota, diarrhea rate, piglets
Citation: Liu B, Wang W, Zhu X, Sun X, Xiao J, Li D, Cui Y, Wang C and Shi Y (2018) Response of Gut Microbiota to Dietary Fiber and Metabolic Interaction With SCFAs in Piglets. Front. Microbiol. 9:2344. doi: 10.3389/fmicb.2018.02344
Received: 17 May 2018; Accepted: 12 September 2018;
Published: 28 September 2018.
Edited by:
Benjamin P. Willing, University of Alberta, CanadaReviewed by:
Alinne Castro, Universidade Católica Dom Bosco (UCDB), BrazilLorena Ruiz, Instituto de Productos Lácteos de Asturias (IPLA), Spain
Copyright © 2018 Liu, Wang, Zhu, Sun, Xiao, Li, Cui, Wang and Shi. This is an open-access article distributed under the terms of the Creative Commons Attribution License (CC BY). The use, distribution or reproduction in other forums is permitted, provided the original author(s) and the copyright owner(s) are credited and that the original publication in this journal is cited, in accordance with accepted academic practice. No use, distribution or reproduction is permitted which does not comply with these terms.
*Correspondence: Yinghua Shi, YW5ueXN5aEBoZW5hdS5lZHUuY24=