- Faculty of Bioscience and Technology for Food, Agriculture and Environment, University of Teramo, Teramo, Italy
Food-associated microbes can reach the gut as viable cells and interact with the human host providing potential health benefits. In this study, we evaluated the impact on cell viability and the adhesion ability of 22 Lactobacillus plantarum strains, mainly isolated from fermented foods, on a Normal-derived Colon Mucosa cell line. Furthermore, due to the presence of mucus layer on the gut epithelium, we also investigated whether the mucin could affect the microbial adhesion property. Our results demonstrated that all the strains displayed a strong ability to adhere to host cells, showing a strain-dependent behavior with preference for cell edges, that resulted not to be affected by the presence of mucin. Based on interleukin-8 release of intestinal cells induced by some Lb. plantarum strains, our data suggest a potential cross-talk with the host immune system as unconventional property of these food-associated microbes.
Introduction
Based on the guidelines of the International Scientific Association for Probiotics and Prebiotics, fermented food-associated microbes can be defined as “active” strains exerting beneficial or health-promoting effects to the host (Hill et al., 2014). To provide health benefits, naturally occurring food-associated microbes must be metabolically stable and active, they have to survive in large numbers through the gastro-intestinal (GI) tract, persist in the gut and interact with the intestinal epithelium (David et al., 2014). Since many food-associated bacterial strains display probiotic properties (Derrien and van Hylckama Vlieg, 2015), the interaction between the gut epithelial cells and orally administrated bacteria is an emerging area of interest both for commercial actors as well as for researchers from different disciplines (Pathmakanthan et al., 2000; Stanton et al., 2001).
Several Lactobacillus plantarum strains, frequently isolated from fermented foods (Molin, 2001; Haza et al., 2004; Kakisu et al., 2013), have been characterized as having probiotic properties (Niedzielin and Kordecki, 2001; Stevenson et al., 2014).
In addition, it has been recently reported that Lb. plantarum associated with fermented foods share physiological properties with strains showing health-promoting activity and, consequently, fermented foods containing a high amount of live cells of that species could be considered beneficial as those containing Lb. plantarum strains with documented probiotic features (Marco et al., 2017). Additionally, selected strains belonging to this species were also recognized suitable as starters for production of probiotic dairy products due to their technological properties (Georgieva et al., 2009). Successful probiotic bacteria are usually able to colonize the intestine, at least temporarily, by adhering to the intestinal mucosa (Ouwehand et al., 1999). Therefore, the ability of probiotic organisms to adhere to the host GI mucosa is one of the main criteria in probiotic strain selection and a prerequisite for transient host colonization. A high efficiency of intestinal colonization has been recognized as a desirable feature that prolongs the time of bacterial beneficial effect on the host, by promoting the gut residence time and the interaction with host epithelial and immune cells (Lebeer et al., 2008; Kleerebezem et al., 2010; Juge, 2012). Moreover, the adhesion of bacteria to intestine is considered a crucial aspect especially in relation with the host immune system modulation (Schiffrin et al., 1997) as well as for the antagonistic activity against enteropathogens (Coconnier et al., 1993; Bernet et al., 1994).
Since it is difficult to assess this property in vivo, different in vitro models have been developed to evaluate bacterial adherence (Del Re et al., 2000; Collado et al., 2007; Laparra and Sanz, 2009). Human intestinal epithelial cell lines, such as Caco-2 and HT-29, have been widely used (Lee et al., 2000; Bianchi et al., 2004; Wang et al., 2009), in order to mimic in vitro the intestinal conditions. Despite the majority of investigation on probiotics have been carried out by using tumor-derived in vitro cell lines, currently the use of these models is under debate on scientific community due to the different associated phenotypes compared with the normal intestinal epithelial cells (Cencic and La Bonnardiere, 2002; Nissen et al., 2009). Indeed, many probiotic products are used by healthy consumers, presuming that probiotics assumption can preserve their health, and potentially reduce the long-term risk of many diseases. Actually, in the last years, intestinal epithelial cell lines having a healthy phenotype, have been used to investigate adhesion and immunomodulation properties of potential probiotics (Nissen et al., 2009; Cencic and Langerholc, 2010; Botta et al., 2014; Gorenjak et al., 2014), as well as anti-inflammatory effects of dietary adjuncts (Schneider et al., 2016).
In the intestine, the presence of mucus layer is a fundamental physical barrier that covers the intestinal cells and protects them from mechanical damage as well as from pathogenic bacterial invasion by modifying or inhibiting bacterial interaction with the mucosal surface. Mucus also provides a habitat and nutrients for some bacteria (Van Tassell and Miller, 2011), and is being considered an ecological niche for both commensal and potentially pathogenic microorganisms (Neutra and Forstner, 1988; Tuomola et al., 1999). Therefore, the bacterial capability to adhere to the mucus layer is considered a prerequisite for microbial persistence in the intestinal environment (Ouwehand et al., 2002). Indeed, due to the continuous renewal of mucus, only bacteria that are able to adhere to it can persist in the GI tract and potentially interact with the epithelium, in order to establish a host-microbe dialog (Papadimitriou et al., 2015). In this context, we should recall that the interaction of orally ingested microbes with the intestinal epithelium has just begun to be rigorously studied (Derrien and van Hylckama Vlieg, 2015; Marco et al., 2017).
The aim of this study was to explore a collection of food-associated Lb. plantarum strains, previously characterized for several functional properties (Prete et al., 2017), for their adhesion properties both in qualitative and quantitative ways through different in vitro cell adhesion assays, by using Normal-derived Colon Mucosa (NCM460) cells as intestinal mucosa in vitro model. Furthermore, due to the presence of mucus layer on the GI epithelium, we also investigated the adhesion property of selected Lb. plantarum strains, and whether the mucin might affect their growth in GI tract.
Finally, we selected some food-associated Lb. plantarum strains to determine their potential immunomodulatory aspects by evaluating interleukin-8 (IL-8) cytokine release in an in vitro inflammation model.
Materials and Methods
Bacterial Strains and Growth Conditions
Twenty-two Lb. plantarum strains, belonging to our laboratory collection at the University of Teramo, were investigated in this study (Table 1). Lb. plantarum ATCC®14917TM, Lb. plantarum WCFS1 and two commercial probiotic strains, Lb. plantarum IMC510® and Lb. plantarum IMC513® (Synbiotec®, Camerino, MC, Italy), were included in the study as reference strains (Table 1). Food-associated Lb. plantarum strains were previously isolated from different fermented foods (table olives, sourdough, and raw-milk cheeses). They were also genetically and phenotypically characterized for several properties including their ability to survive in the GI transit as well as their antigenotoxic activity (Prete et al., 2017). All the strains were grown in microaerophilic conditions at 37°C in MRS broth (Oxoid, Basingstoke, United Kingdom), and they were subcultured once before each experiments.
Intestinal Cell Culture
NCM460 cells (INCELL Corporation, LLC, San Antonio, TX, United States) were grown in INCELL’s enriched M3Base medium supplemented with 1% (v/v) Penicillin/Streptomycin 100X (Corning, NY, United States), 1% (v/v) Non-Essential Amino Acids 100X solution (Euroclone, Pero, Italy), and 10% (v/v) heated inactivated Fetal Bovine Serum (FBS; Corning, NY, United States). Cells were grown in culture dishes at 37°C in a 5% CO2 atmosphere, and seeded at 60–70% confluence (105 cells/well in 96-well plate; 3 × 106 cells/well in 6-well plate) for 24 h prior the co-incubation conditions.
Cell Viability Assay
The cellular metabolic activity was assessed with in vitro colorimetric 3-(4,5-dimethylthiazole-2- yl)-2,5-phenyl tetrazolium bromide (MTT) assay, slightly modified in order to evaluate the potential cytotoxic effect of the selected strains toward NCM460 human intestinal cells (Figure 1). Briefly, once NCM460 confluent monolayer was achieved, cell medium was removed and substituted with sterile Phosphate Buffer Saline (PBS), and bacteria (108 CFU/ml) were added to each well. 96-well plates were incubated for 4 and 24 h at 37°C in a 5% CO2 atmosphere. Before carrying out the MTT reduction assay, a bacterial lysis step was applied in each well in order to remove any live bacterial cells, thus minimizing interferences and avoiding misinterpretation of the experimental results. After incubation, PBS was removed and 10 μL of MTT (5 mg/mL) and 100 μL of fresh PBS were added to each well. The mixture was incubated for 3 h at 37°C in a 5% CO2 atmosphere. Then, supernatants were removed and the formazan crystals were dissolved adding 100 μL of acidified isopropanol. Once complete solubilisation of the purple formazan crystals was obtained, 96-well plate was shaken for 10 min and the OD was measured at 570 and 630 nm, by using an EnSpire multimode plate reader (PerkinElmer, Waltham, MA, United States). Microtiter plate also contained control wells (without bacteria) and blank wells (without cells) to provide blank measurement and to remove background noise for absorbance readings. Data were expressed as percentage of cellular metabolic activity by means of the following equation: [OD of tested sample (human cells with bacteria)/(OD of negative control (human cells w/o bacteria) × 100] - 100.
Adhesion Assays to Human Intestinal Cells
The microbial adhesion ability was assessed in both qualitative and quantitative ways through different in vitro cell adhesion assays. First, the qualitative microbial ability to adhere to NCM460 cells was assessed by an in vitro Gram-stained adhesion assay, modifying the protocol described by Kotzamanidis et al. (2010). Briefly, NCM460 monolayers were prepared on glass coverslips in 6-well tissue culture plates and were incubated at 37°C in a 5% CO2 atmosphere. Subsequently, overnight Lb. plantarum cultures (∼100 bacteria/cell) were added over the monolayer of NCM460 cells, the plates were placed at 37°C under 5% CO2 atmosphere and incubated for 90 min. The plates were then washed twice with PBS in order to remove unbound bacteria, fixed with methanol and stained with Gram staining method. The adherent lactobacilli were microscopically examined at different magnification levels. The microbial ability to adhere to NCM460 intestinal cells was confirmed by quantifying the percentage of adherent bacteria, according to the protocol described by Duary et al. (2011) with some modifications. Briefly, Lb. plantarum strains were added (∼100 bacteria/cell) to NCM460 confluent monolayers (105 cells/well seeded in 96-well plates 24 h prior the experiment) in PBS as assay medium, and then incubated for 1.5 h at 37°C in 5% CO2 atmosphere. After incubation, cells were washed with sterile PBS and lysed by addition of 0.25% Trypsin/EDTA solution (Corning, NY, United States). The remaining suspensions with adhering bacteria were serially diluted with saline solution, plated into MRS agar plates, and then incubated for 48 h at 37°C under microaerophilic conditions. Adhesion to NCM460 cells was calculated as percentage of adhered bacteria correlated to the initial number of bacteria added in each well.
Adhesion Test to Mucin
Bacterial ability to adhere to mucin layer was assessed according to Tallon et al. (2007) with minor modifications. Type III porcine gastric mucin (Sigma Aldrich, St Louis, MO, United States) was dissolved in PBS to a final concentration of 10 mg/ml and initially immobilized in 96-wells plate by overnight incubation at 4°C. After washing twice with PBS, Bovine Serum Albumin (BSA) solution (20 mg/ml) was added to each well in order to perform a saturation step for 4 h at 4°C.
A minimum of three replicates was used to estimate the adhesion of each strain. Lb. plantarum overnight cultures were centrifuged at 9000 × g for 15 min, and the bacterial cells were resuspended in sterile PBS and adjusted to the optical density (OD600) of 0.150. Each strain suspension was added to each well and incubated 1.5 h at 37°C. The supernatants were removed from each well and the plate was washed twice with sterile PBS to release unbound bacteria. Adhering bacteria were desorbed with 0.25% Trypsin/EDTA solution (Corning, NY, United States) for 2 min. One hundred microliters of the content of each well were used to do serial dilutions, and then plated on MRS agar plates. The concentration of Trypsin-EDTA solution was tested on all strains in order to avoid a negative effect on bacterial viability.
Mucin Growth Assay
Mucin influence on microbial growth was assessed for each strain by evaluating the growth in presence of Type III porcine gastric mucin (Sigma Aldrich, St Louis, MO, United States). Lb. plantarum cells growth on MRS broth supplemented with 1 mg/ml of Type III porcine gastric mucin, was monitored at OD600 using an EnSpire® multimode plate reader (PerkinElmer, Waltham, MA, United States). The plate reader was run in discontinuous mode, with absorbance readings performed in 60-min intervals and preceded by 30 s shaking at medium speed. Cultures were grown in three biologically independent replicates and the resulting data were expressed as mean ± SEM.
Evaluation of IL-8 Release by Enzyme-Linked Immunosorbent Assay
To evaluate the anti-inflammatory effects of Lb. plantarum on NCM460 cells, the IL-8 cytokine production was investigated using Human IL-8/CXCL8 kit from R&D Systems, (R&D Systems, Minneapolis, MN, United States), and analyzed using an EnSpire® multimode plate reader (PerkinElmer, Waltham, MA, United States). Briefly, NCM460 cells were pre-treated with Lb. plantarum cells suspensions (∼100 bacteria/cell) for 4 h and subsequently inflammation was induced for 24 h using a human cytokine mixture (IL-1β, TNF-α, and INF-γ) (Schneider et al., 2016). After centrifugation, the supernatants were collected and analyzed following the manufacturer’s instructions. Inflamed NCM460 cells without bacterial pre-treatment (control) and not inflamed NCM460 cells (NI-NCM460) as negative control, were included in the assay.
Statistical Analysis
Data were analyzed by means of Prism 7.0 program (GraphPad Software Inc., La Jolla, CA, United States) using the one-way analysis of variance (ANOVA) followed by Bonferroni’s post hoc analysis. ELISA data were assessed by Student’s t-test. A level of p < 0.05 was considered statistically significant.
Results
Microbial Impact on Human Cell Viability
The potential impact of 22 Lb. plantarum strains on human cell viability was assessed by the MTT colorimetric assay. In order to mimic the in vivo interaction between intestinal mucosa and ingested bacteria, the cellular metabolic activity of NCM460 cells was measured after two different co-incubation time, a short-term co-incubation (around 4 h) and a long-term co-incubation (24 h) that represents the persistence time of bacteria in the GI tract (Figure 2). Results from short-term incubation showed the same trend among all Lb. plantarum strains, which, however, did not result statistically significant, indicating that all Lb. plantarum strains are likely to leave the cellular metabolic activity of the NCM460 cells unchanged or even improved (Figure 2A).
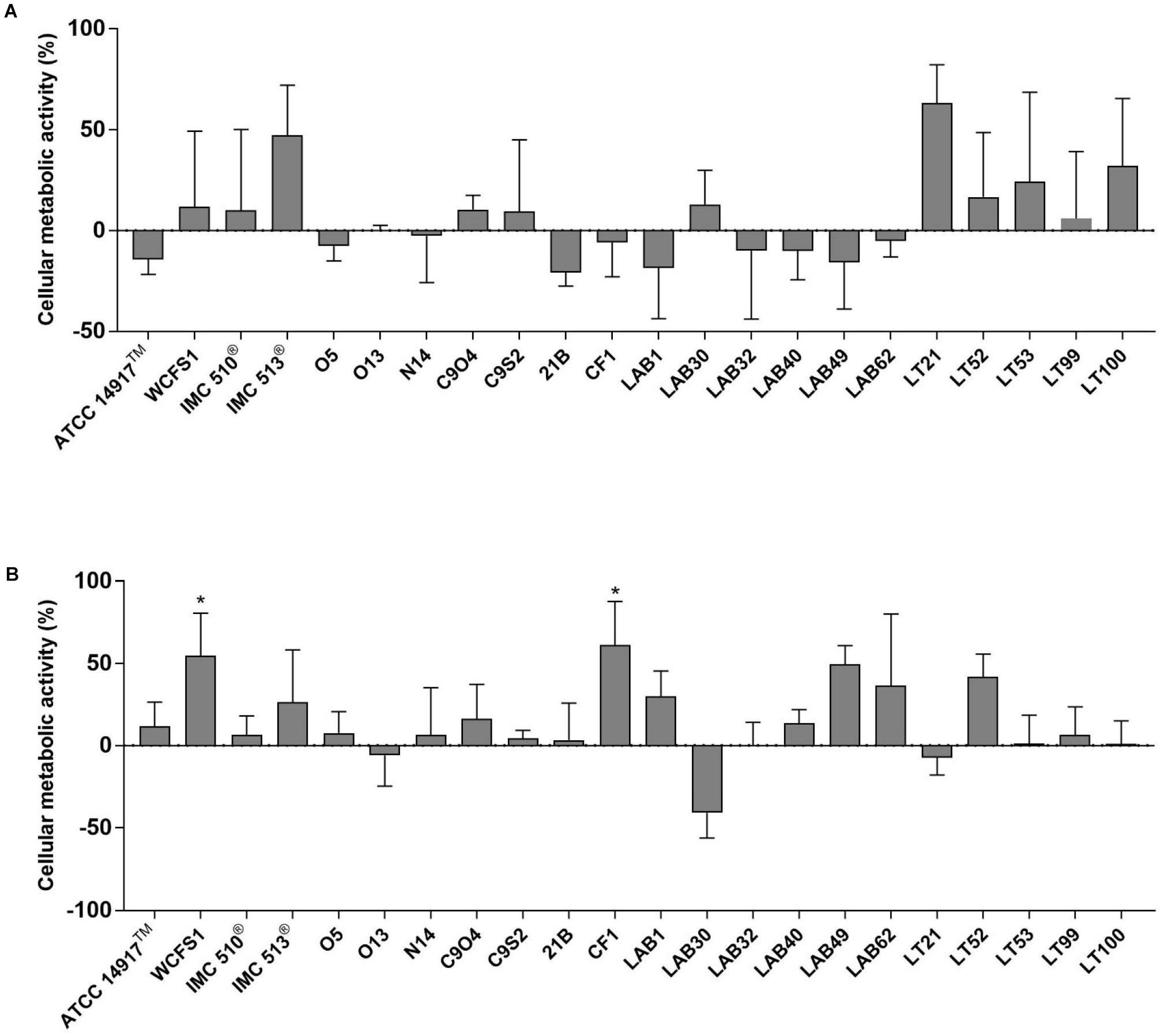
FIGURE 2. Evaluation of cellular metabolic activity in NCM460 cells incubated with Lactobacillus plantarum strains for 4 h (A) and 24 h (B). Data are shown as mean ratio (percentage – 100; ±SD) of absorbance in the samples well (OD of human cells with bacteria) and negative control (OD of human cells): (ODS/ODNC × 100) - 100.
Except few strains (e.g., Lb. plantarum O13, LAB30, and LT21), MTT data, obtained after 24 h incubation, showed an overall increasing effect in the metabolic activity of NCM460 cells (Figure 2B). Indeed, the majority of tested Lb. plantarum strains induced an increase in the metabolic activity of NCM460 cells, ranging from 12% (Lb. plantarum ATCC®14917TM) to 55% (Lb. plantarum WCFS1) and 61% for Lb. plantarum CF1, thus showing statistically higher values than the negative control (p < 0.05).
Adhesion Efficiency of Lb. plantarum to Human Intestinal Cells
The adhesion efficiency of 22 Lb. plantarum strains was also investigated on NCM460 cell line by two independent methods: (i) direct microscopic examination after Gram differential staining and (ii) bacterial enumeration by plating on MRS agar (Figures 3, 4). Microscopical observations showed an overall high adhesion capability of all Lb. plantarum strains on NCM460 cell line, albeit with different adhesion behavior. Interestingly, a comparative evaluation with optical microscope showed a bacterial preference in adhering around NCM460 cell edges (Figures 3A–D). In particular, Figure 3 shows how Lb. plantarum aggregate close to the cells by bordering them and/or directly above the cell surface, without adhering to the plate. Indeed, the most adhesive Lb. plantarum strains adhered not only to cells edges, but also colonized the entire NCM460 monolayer (Figures 3E,F). The qualitative tests, with regard to adhesion efficiency observed microscopically, were further validated by enumerating adherent bacteria with a classical culture-dependent method. The adhesion efficiency was calculated as percentage of adhesion values compared to the initial bacterial seeded in each well and assumed to be equal to 100%, confirming the high colonization efficiency, previously observed by microscopy assay. Indeed, all Lb. plantarum strains were able to adhere to NCM460, with an adhesion percentage ranging from 77 to 98% (Figure 4). These results underline that the majority of Lb. plantarum strains isolated from foods display an adhesion efficiency similar to that of Lb. plantarum from human sources, revealing thus a promising colonization ability to the human epithelium.
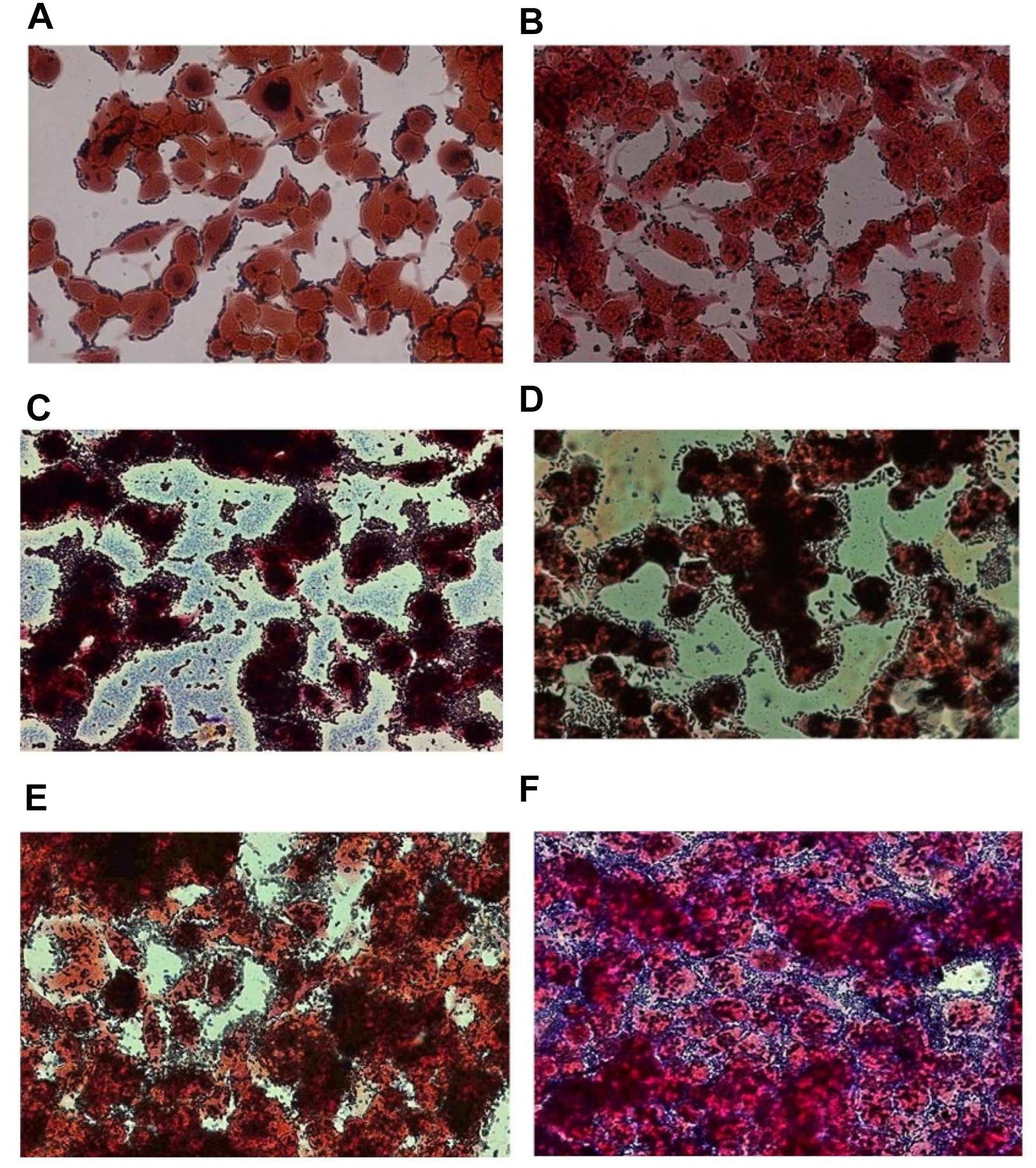
FIGURE 3. Adhesion of representative Lb. plantarum strains on NCM460 cell cultures observed by microscope (40×) after Gram staining, displaying different adhesion attitude: preference in adhering to cell edges or monolayer colonization. (A) Lb. plantarum IMC510®, probiotic strain; (B) Food-borne Lb. plantarum O5, isolated from table olives; (C) Food-borne Lb. plantarum LAB40, isolated from raw-milk cheeses; (D) Food-borne Lb. plantarum 21B, isolated from sourdough; (E) Food-borne Lb. plantarum LAB62, isolated from raw-milk cheeses; and (F) Food-borne Lb. plantarum C9S2, isolated from table olives.
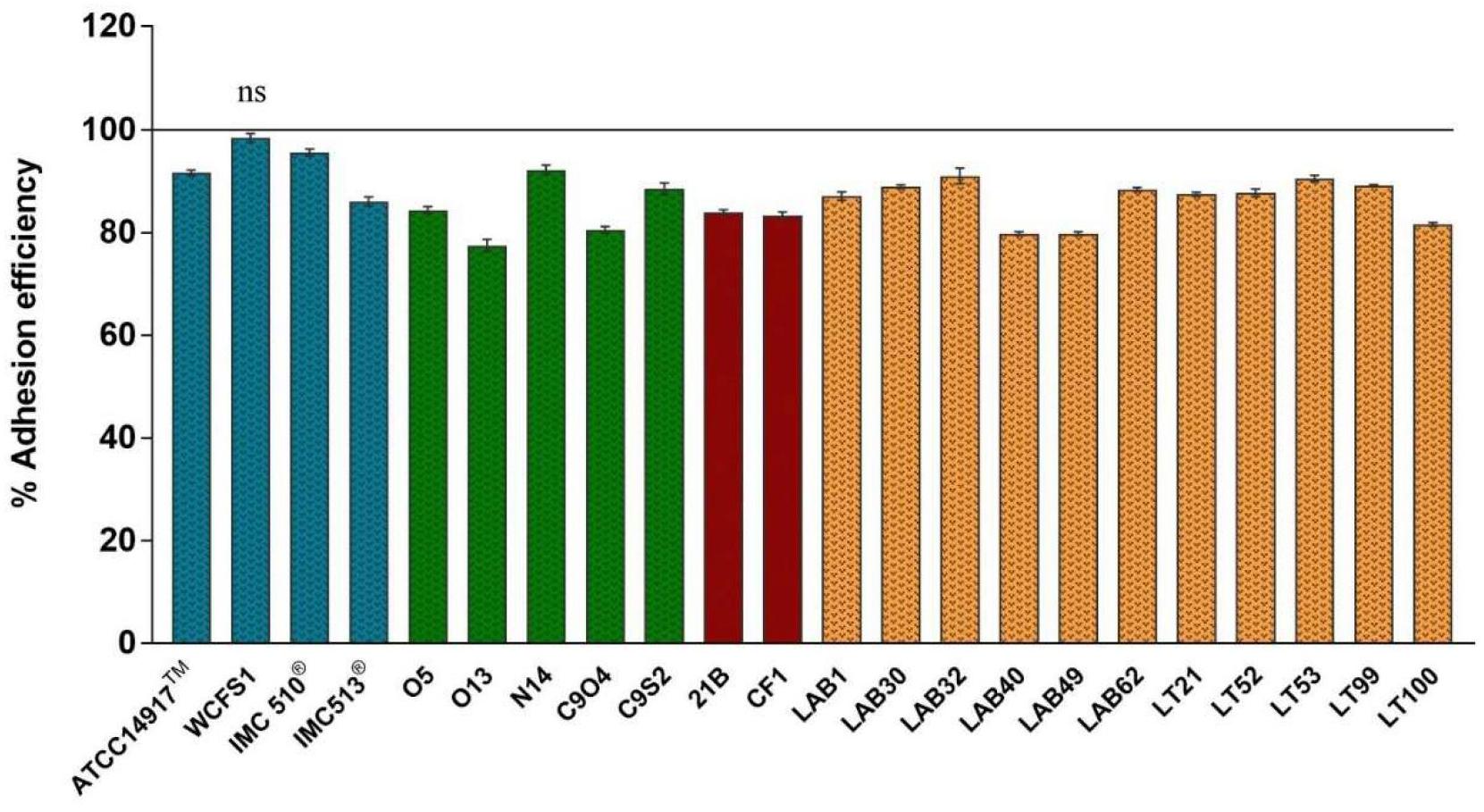
FIGURE 4. Adhesion efficiency of Lb. plantarum strains to NCM460 cells. Values are expressed as mean ± SEM. p < 0.0001 versus control; ns = not statistically significant; and 100% = initial bacterial number.
Lactobacillus plantarum Adhesion and Growth on Mucin
Due to the presence of mucus layer on the GI epithelium, we also investigated whether the mucin can affect the microbial adhesion property or it might have any impact on Lb. plantarum growth. Porcine gastric mucin is routinely used as a model for its human equivalent in different studies concerning to bacterial degradation of this substrate (Derrien et al., 2008; Ruas-Madiedo et al., 2008; Turroni et al., 2010) as well as in bacterial adherence studies (Tallon et al., 2007; Jensen et al., 2014). Therefore, we tested all 22 Lb. plantarum strains for adhesion to porcine gastric mucin, showing an overlapping with the results previously obtained with human NCM460 cells. The box plot showed an overall high adhesion capability among Lb. plantarum species, as shown in Figure 5. Results confirmed a percentage of adhesion range, from 79% (Lb. plantarum N14) to 92% (Lb. plantarum LAB1) similar to that found in the adhesion assay with NCM460 cells. Both probiotic strains, Lb. plantarum IMC510® and Lb. plantarum IMC513®, besides Lb. plantarum WCFS1, were also able to adhere to mucin at a level of approximately 5 × 106 CFU/well. Interestingly, the majority of the Lb. plantarum strains showed such high adhesion properties, and strains isolated from different fermented foods were shown to adhere at the same level with that of probiotics. As shown in Figure 6, adhesion efficiency to bind to the mucin was strain-dependent as observed in the NCM460 adhesion assays. Finally, the growth of all Lb. plantarum strains, both from human and food sources, showed to be affected in presence of porcine mucin (Figure 7); in particular, the majority of the strains reveal an increased growth, being probably able to use mucin as a carbon source. Therefore, the combinations of our adhesion results suggested the potential ability of Lb. plantarum species to successfully colonize in vivo the intestinal mucosa.
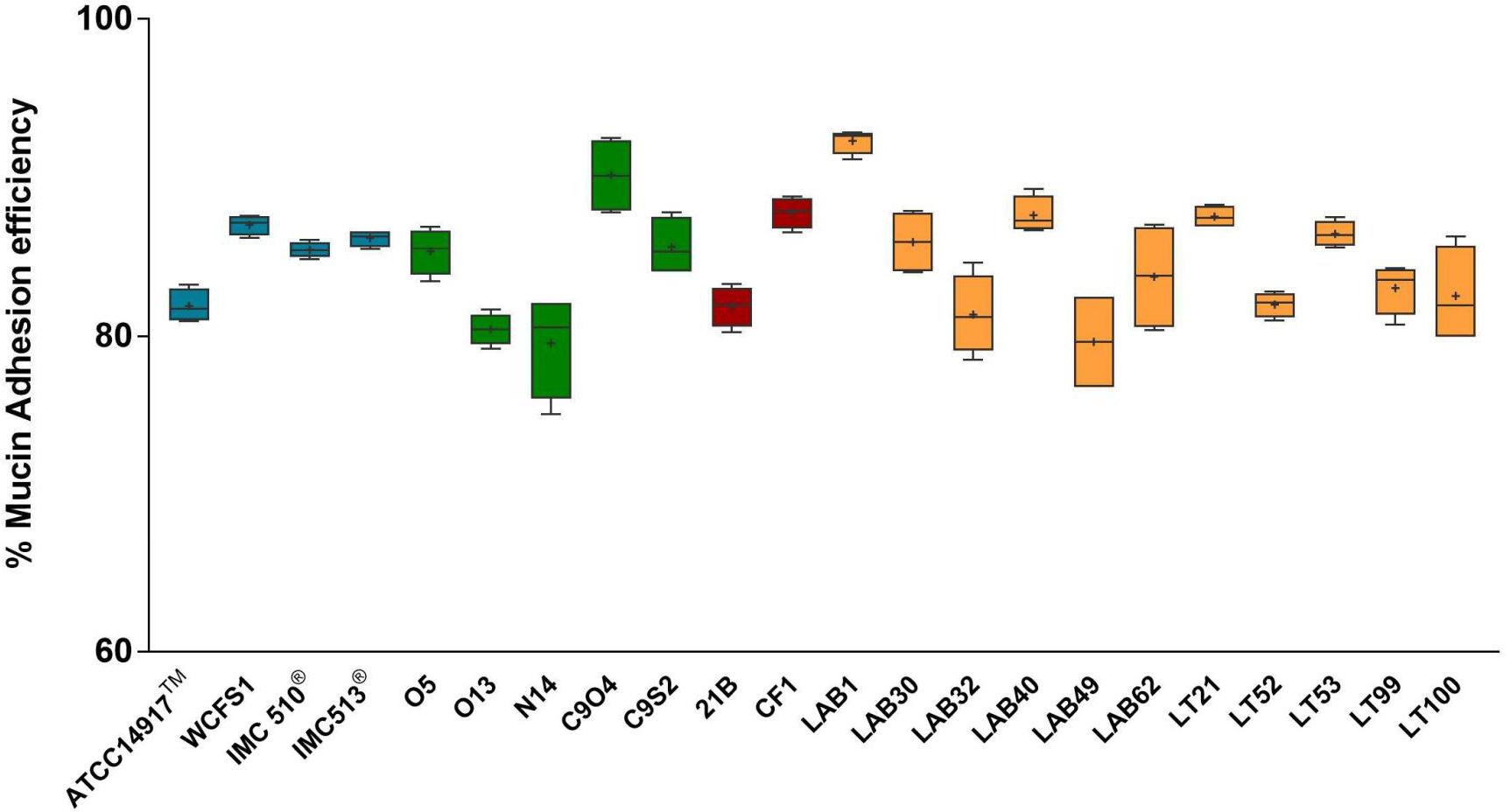
FIGURE 5. Mucin adhesion efficiency of Lb. plantarum strains. Box and whisker plots with median, 25–75th percentiles, range and mean as “+” p < 0.0001 versus control.
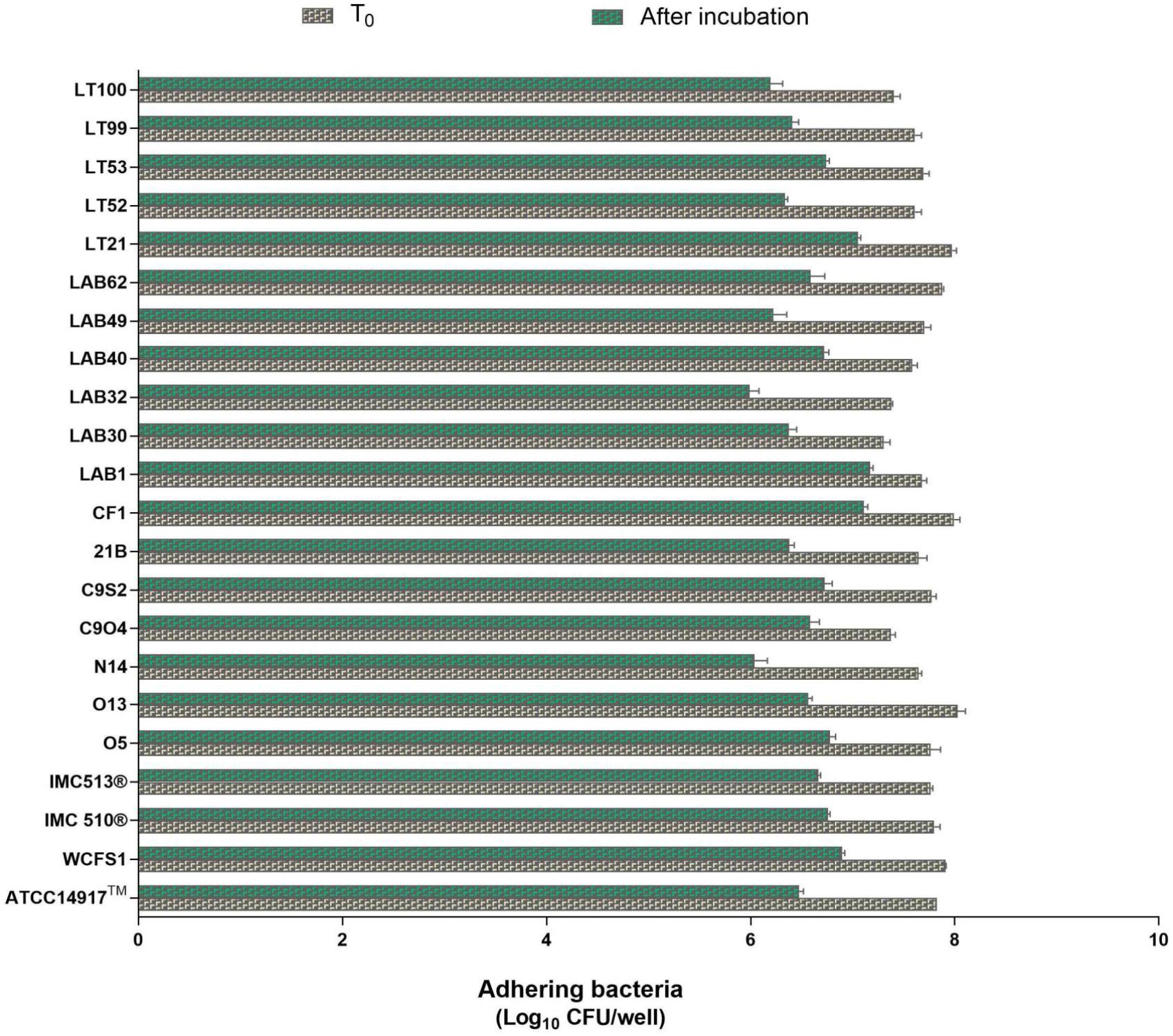
FIGURE 6. Enumeration of adhering Lb. plantarum cultures to mucin. Values are expressed as mean ± SEM from three biological replicates and are reported as log10 CFU/well. A level of p < 0.0001 was considered statistically significant.
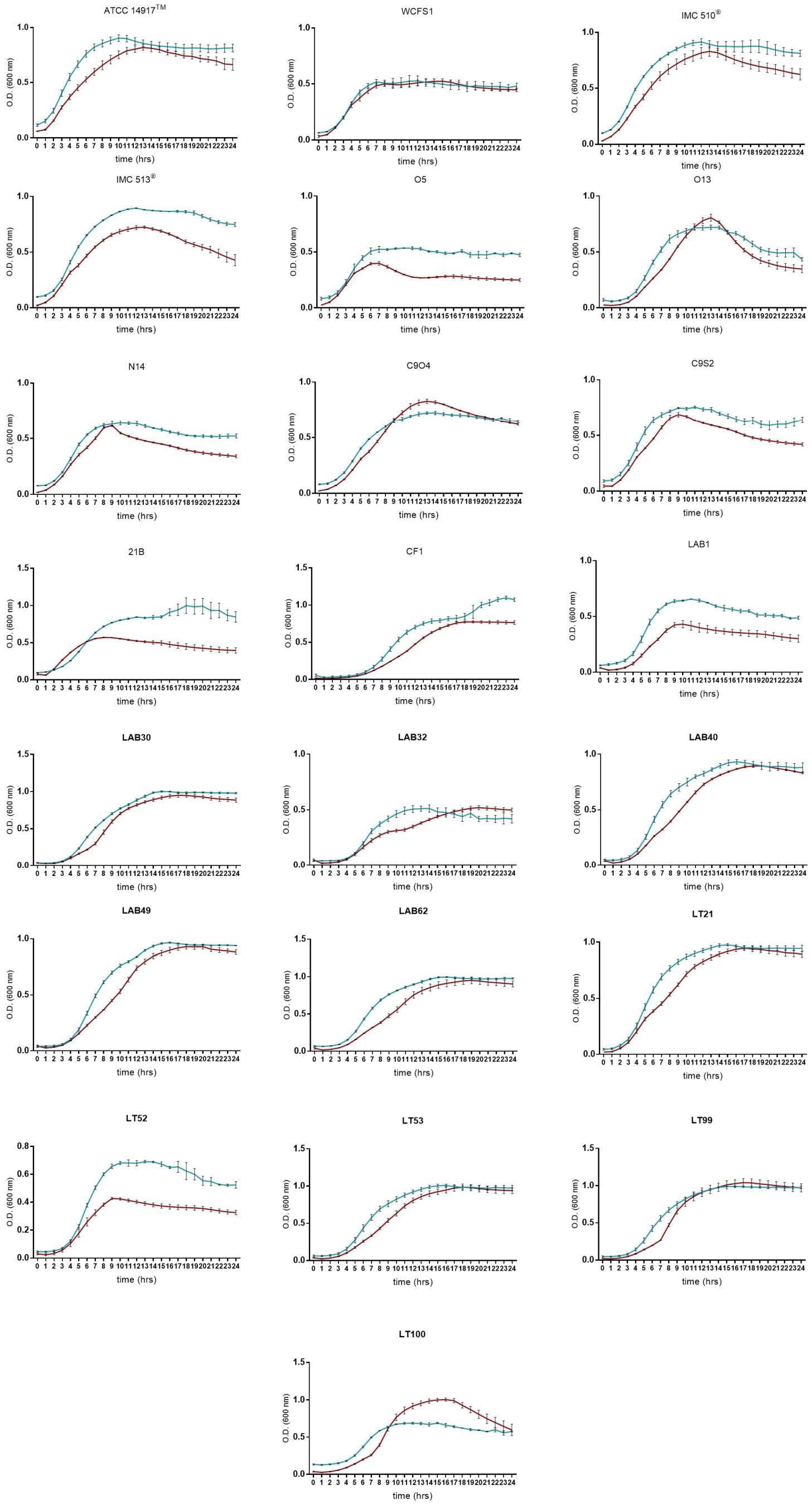
FIGURE 7. Lactobacillus plantarum growth curves in presence of porcine mucin (dark red line means MRS broth, blue lines means MRS broth with porcine mucin (0.1% w/v). Data are reported as mean values ± SEM from three biological replicates.
Lactobacillus plantarum Modulation of IL-8 Release on Inflamed Human Intestinal Cells
Based on the high adhesion efficiency, highlighting how food-associated Lb. plantarum strains could share physiological attitudes with human-derived and/or probiotic strains, we tested the potential immunomodulatory impact of five Lb. plantarum strains (O5, C9S2, CF1, LAB62, and LT52) isolated from table olives, sourdough and raw-milk cheeses, besides two strains from human sources (WCFS1 and IMC513®) on NCM460 cells. In particular, we investigated IL-8 release in an in vitro inflammation model. Lb. plantarum strains treatment led to a reduction of IL-8 levels compared to control, as reported in Figure 8. Indeed, statistical analysis underlined that Lb. plantarum C9S2, CF1, and LAB62, were able to significantly reduce IL-8 levels (inhibition percentage: 56.18, 75.56, and 57.51%, respectively) (Figure 8).
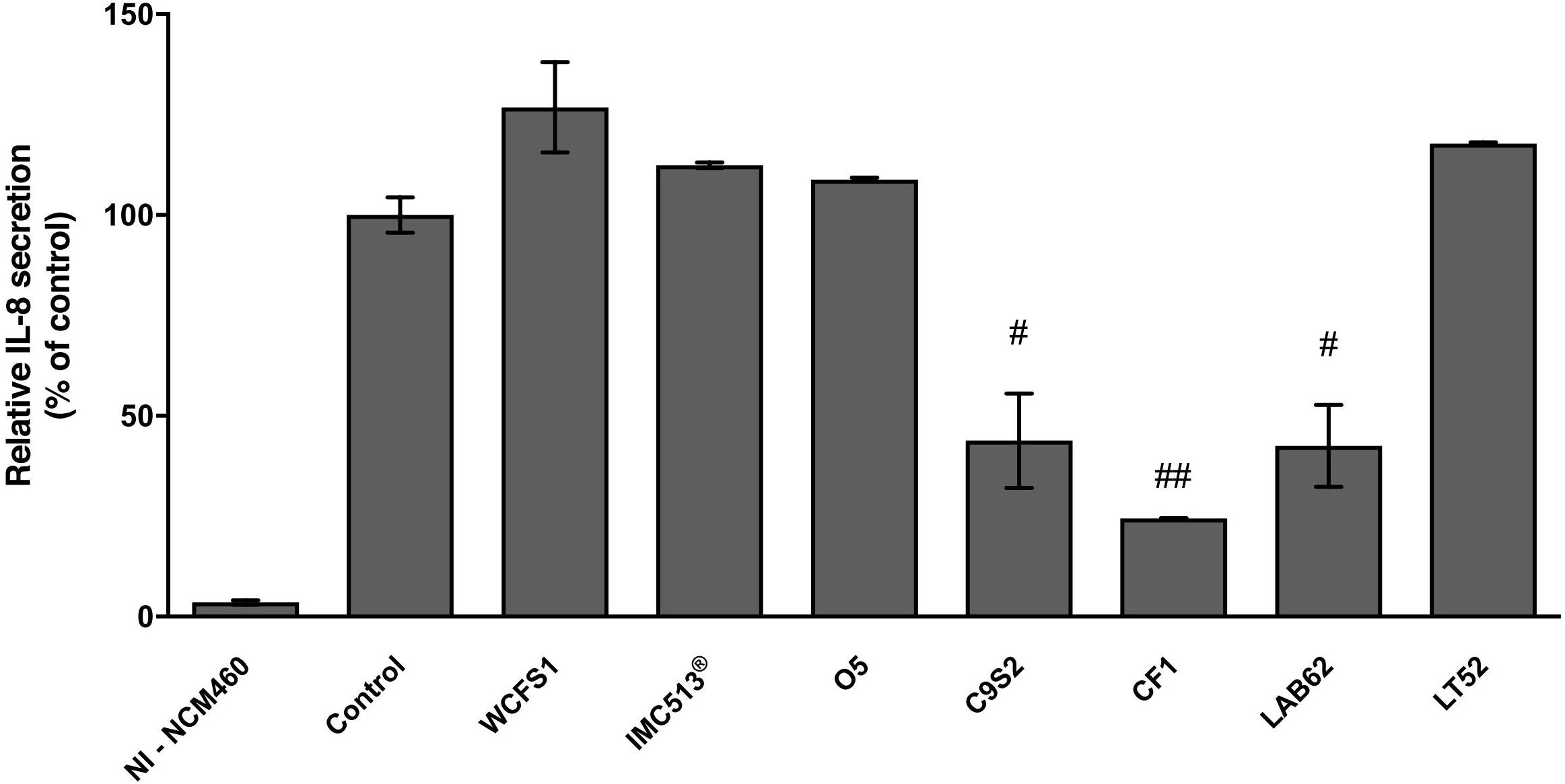
FIGURE 8. Lactobacillus plantarum modulation of IL-8 release on inflamed NCM460 cells. Values are expressed as mean ± SEM. ##p < 0.005 and #p < 0.05 versus inflamed control.
Discussion
Food-borne Lb. plantarum strains were previously isolated from different fermented foods (table olives, sourdough, and raw-milk cheeses) and characterized for the resistance to GI transit and other functional properties, such as antigenotoxic activity (Prete et al., 2017).
In line with probiotic characterization and in the context of strain safety, the non-cytotoxic effect of Lb. plantarum strains toward NCM460 human cells was confirmed by in vitro MTT assay. Contact of bacteria with cells in both short-term (4 h) and long-term (24 h) incubation time did not result in a significant decrease of cell viability, with the exception of few strains, while the preponderance of Lb. plantarum strains did not change the cellular metabolic activity of NCM460 cells. According to the defined priority scale for probiotics selection (FAO/WHO, 2006; Morelli, 2007), one of the main evaluation criteria is the adhesion efficiency to the intestinal epithelium aimed to guaranteeing the persistence in the gut environment and thus to conferring beneficial effects to human health. This criterion, together with the ability to endure GI conditions, was used to select strains with confirmed in vivo probiotic traits (Dunne et al., 2001) and which are currently active ingredients of probiotic products. The results obtained in this study showed a marked adhesion efficiency of Lb. plantarum strains to both mucus and intestinal cells with a clear preference for adherence to cell edges. However, considerable variability in the adhesive capacity was found within the same species, confirming how this attitude is strictly strain-dependent and influences the potential probiotic activities of each strain (Ivec et al., 2007; Tallon et al., 2007). Moreover, as previously reported (Molin, 2001; Klarin et al., 2005), the enormous adhesive capacity of Lb. plantarum 299v demonstrated to be a fundamental feature for the functional performance of many probiotics activities, including modulation of the host immune system. Indeed, bacterial adhesion is a complex interaction that involve a physical contact between both the bacterial cell and the mucosal surfaces (Duary et al., 2011; Papadimitriou et al., 2015). In particular, a fundamental role in the microbial adhesion mechanisms to some components of the host (such as mucus and collagen), as well as in survival and in the adaptation to the GI tract of Lb. plantarum, is played by some proteins and enzymes produced by this species, such as hydrolases and trans-glycosylases (Corsetti et al., 2015). In addition, the presence of mucus layer, as a protective barrier in the GI tract, plays a crucial role in the bacterial adhesion mechanism and several data literature report that some lactobacilli are not able to bind to the mucus layer (Jonsson et al., 2001; Duary et al., 2011; Jensen et al., 2014). Moreover, lactobacilli showing high adhesion properties to intestinal cells do not always display the same capability to bind to the mucin (Markowicz and Schmidt, 2015). Therefore, we investigated all the strains to assess the potential impact of mucin on adhesion efficiency and to identify their ability to grow in a medium containing mucin (Van Tassell and Miller, 2011). Our results support previous experimental evidences confirming the high efficiency of this species to adapt to the GI tract of healthy subjects (Siezen and van Hylckama Vlieg, 2011).
Based on these results, some representative food-associated Lb. plantarum strains were tested for their immunomodulatory properties by evaluating the release of a pro-inflammatory cytokine (IL-8). We investigated whether adhesive interaction of Lb. plantarum strains could modulate NCM460 IL-8 release after inflammation induced by a cytokine mixture (IL-1β, TNF-α, and INF-γ) that mimics inflammatory response in cell models (Mueller et al., 2013; Schneider et al., 2016). Therefore, among all the potential induced cytokines that NCM460 cells can release, we focused on IL-8 that is considered one of the most effective neutrophil activator, widely used as an inflammatory marker in intestinal cell lines (Noh et al., 2015; Schneider et al., 2016). Similar to previous studies, the treatment of intestinal cells with inflammatory cytokines led to a clear increase in IL-8 secretion (Figure 8; Reilly et al., 2007; Ren et al., 2013; Schneider et al., 2016). IL-8 release resulted to be significantly reduced in inflamed NCM460 cells after pre-treatment with food-related Lb. plantarum C9S2, CF1, and LAB62 in line with other studies with different in vitro cell models (McCracken et al., 2002; Ren et al., 2013; Noh et al., 2015). On the other hand, the human-derived Lb. plantarum WCFS1 and IMC513® did not affect IL-8 secretion. Moreover, this preliminary investigation showed a promising ability of the selected strains to interact with eukaryotic cells and to modulate in a strain-dependent manner IL-8 secretion levels.
Conclusion
Overall, food-associated Lb. plantarum strains displayed a similar behavior to those from human sources, supporting the adhesion efficiency as microbial prerequisite to exert health benefits in the host and revealing a promising interaction with host cells. In particular, the reduction of the IL-8 release by some food-related Lb. plantarum strains, even more than strains from human source, suggests potential cross-talk with the host immune system as unconventional property of food-associated microbes useful to develop novel functional foods with specific probiotic label.
Author Contributions
NB and AC designed the study. RP and NG-G performed the experiments. NB, AC, RP, and NG-G analyzed the data, discussed the results, and drafted the manuscript. All authors read and approved the final manuscript.
Funding
This work was supported by Italian Ministry of University and Research (PRIN Project 20152LFKAT to AC), and has received financial support from the European Union’s Horizon 2020 research and innovation program under the Marie Skłodowska-Curie grant agreement 713714 ESR 07 (to NG-G).
Conflict of Interest Statement
The authors declare that the research was conducted in the absence of any commercial or financial relationships that could be construed as a potential conflict of interest.
Acknowledgments
The authors gratefully acknowledge Prof. A. Cresci (Synbiotec, Camerino, Italy) to kindly provide us Lb. plantarum probiotic strains (IMC510® and IMC513®), and Prof. M. Maccarrone (University Campus Bio-Medico of Rome) for his expert manuscript revision.
References
Bernet, M. F., Brassart, D., Neeser, J. R., and Servin, A. L. (1994). Lactobacillus acidophilus LA1 binds to cultured human intestinal cell lines and inhibits cell attachment and cell invasion by enterovirulent bacteria. Gut 35, 483–489. doi: 10.1136/gut.35.4.483
Bianchi, M. A., Del Rio, D., Pellegrini, N., Sansebastiano, G., Neviani, E., and Brighenti, F. (2004). A fluorescence-based method for the detection of adhesive properties of lactic acid bacteria to Caco-2 cells. Lett. Appl. Microbiol. 39, 301–305. doi: 10.1111/j.1472-765X.2004.01589.x
Botta, C., Langerholc, T., Cencic, A., and Cocolin, L. (2014). In vitro selection and characterization of new probiotic candidates from table olive microbiota. PLoS One 9:e94457. doi: 10.1371/journal.pone.0094457
Cencic, A., and La Bonnardiere, C. (2002). Trophoblastic interferon-gamma: current knowledge and possible role(s) in early pig pregnancy. Vet. Res. 33, 139–157. doi: 10.1051/vetres:2002003
Cencic, A., and Langerholc, T. (2010). Functional cell models of the gut and their applications in food microbiology - a review. Int. J. Food Microbiol. 141, S4–S14. doi: 10.1016/j.ijfoodmicro.2010.03.026
Coconnier, M. H., Bernet, M. F., Kerneis, S., Chauviere, G., Fourniat, J., and Servin, A. L. (1993). Inhibition of adhesion of enteroinvasive pathogens to human intestinal Caco-2 cells by Lactobacillus acidophilus strain LB decreases bacterial invasion. FEMS Microbiol. Lett. 110, 299–306. doi: 10.1111/j.1574-6968.1993.tb06339.x
Collado, M. C., Meriluoto, J., and Salminen, S. (2007). In vitro analysis of probiotic strain combinations to inhibit pathogen adhesion to human intestinal mucus. Food Res. Int. 40, 629–636. doi: 10.1016/j.foodres.2006.11.007
Corsetti, A., Ciarrocchi, A., and Prete, R. (2015). Lactic Acid Bacteria: Lactobacillus spp.: Lactobacillus plantarum. Reference Module in Food Science. New York, NY: Elsevier, 1–8. doi: 10.1016/B978-0-08-100596-5.00856-8
David, L. A., Maurice, C. F., Carmody, R. N., Gootenberg, D. B., Button, J. E., Wolfe, B. E., et al. (2014). Diet rapidly and reproducibly alters the human gut microbiome. Nature 505, 559–563. doi: 10.1038/nature12820
Del Re, B., Sgorbati, B., Miglioli, M., and Palenzona, D. (2000). Adhesion, auto aggregation and hydrophobicity of 13 strains of Bifidobacterium longum. Lett. Appl. Microbiol. 31, 438–442. doi: 10.1046/j.1365-2672.2000.00845.x
Derrien, M., Collado, M. C., Ben-Amor, K., Salminen, S., and de Vos, W. M. (2008). The mucin degrader Akkermansia muciniphila is an abundant resident of the human intestinal tract. Appl. Environ. Microbiol. 74, 1646–1648. doi: 10.1128/AEM.01226-07
Derrien, M., and van Hylckama Vlieg, J. E. T. (2015). Fate, activity, and impact of ingested bacteria within the human gut microbiota. Trends Microbiol. 23, 354–366. doi: 10.1016/j.tim.2015.03.002
Duary, R. K., Rajput, Y. S., Batish, V. K., and Grover, S. (2011). Assessing the adhesion of putative indigenous probiotic lactobacilli to human colonic epithelial cells. Indian J. Med. Res. 134, 664–671. doi: 10.4103/0971-5916.90992
Dunne, C., O’Mahony, L., Murphy, L., Thornton, G., Morrissey, D., O’Halloran, S., et al. (2001). In vitro selection criteria for probiotic bacteria on human origin: correlation with in vivo findings. Am. J. Clin. Nutr. 73, 386–392. doi: 10.1093/ajcn/73.2.386s
FAO/WHO (2006). Probiotics in Food. Health and Nutritional Properties and Guidelines for Evaluation: FAO Food and Nutrition. Rome: FAO.
Georgieva, R., Iliev, I., Haertle, T., Chobert, J. M., Ivanova, I., and Danova, S. (2009). Technological properties of candidate probiotic Lactobacillus plantarum strains. Int. Dairy J. 19, 696–702. doi: 10.1016/j.idairyj.2009.06.006
Gorenjak, M., Gradišnik, L., Trapečar, M., Pistello, M., Kozmus, C. P., Škorjanc, D., et al. (2014). Improvement of lipid profile by probiotic/protective cultures: study in a non-carcinogenic small intestinal cell model. New Microbiol. 37, 51–64.
Haza, A. I., Zabala, A., and Morales, P. (2004). Protective effect and cytokine production of a Lactobacillus plantarum strain isolated from ewes’ milk cheese. Int. Dairy J. 14, 29–38. doi: 10.1016/S0958-6946(03)00146-8
Hill, C., Guarner, F., Reid, G., Gibson, G. R., Merenstein, D. J., Pot, B., et al. (2014). Expert consensus document: the international scientific association for probiotics and prebiotics consensus statement on the scope and appropriate use of the term probiotic. Nat. Rev. Gastroenterol. Hepatol. 11, 506–514. doi: 10.1038/nrgastro.2014.66
Ivec, M., Botìc, T., Koren, S., Jakobsen, M., Weingartl, H., and Cencic, A. (2007). Interactions of macrophages with probiotic bacteria lead to increased antiviral response against vesicular stomatitis virus. Antiviral Res. 75, 266–274. doi: 10.1016/j.antiviral.2007.03.013
Jensen, H., Roos, S., Jonsson, H., Rud, I., Grimmer, S., van Pijkeren, J. P., et al. (2014). Role of Lactobacillus reuteri cell and mucus-binding protein a (CmbA) in adhesion to intestinal epithelial cells and mucus in vitro. Microbiology 160, 671–681. doi: 10.1099/mic.0.073551-0
Jonsson, H., Strom, E., and Roos, S. (2001). Addition of mucin to the growth medium triggers mucus-binding activity in different strains of Lactobacillus reuteri in vitro. FEMS Microbiol. Lett. 204, 19–22. doi: 10.1111/j.1574-6968.2001.tb10855.x
Juge, N. (2012). Microbial adhesins to gastrointestinal mucus. Trends Microbiol. 20, 30–39. doi: 10.1016/j.tim.2011.10.001
Kakisu, E., Bolla, P., Abraham, A. G., de Urraza, P., and De Antoni, G. L. (2013). Lactobacillus plantarum isolated from kefir: protection of cultured Hep-2 cells against Shigella invasion. Int. Dairy J. 33, 22–26. doi: 10.1016/j.idairyj.2013.06.004
Klarin, B., Johansson, M. L., Molin, G., Larsson, A., and Jeppsson, B. (2005). Adhesion of the probiotic bacterium Lactobacillus plantarum 299v onto the gut mucosa in critically ill patients: a randomised open trial. Crit. Care 9:R285. doi: 10.1186/cc3522
Kleerebezem, M., Hols, P., Bernard, E., Rolain, T., Zhou, M., Siezen, R. J., et al. (2010). The extracellular biology of the lactobacilli. FEMS Microbiol. Rev. 34, 199–230. doi: 10.1111/j.1574-6976.2010.00208.x
Kotzamanidis, C., Kourelis, A., Litopoulou-Tzanetaki, E., Tzanetakis, N., and Yiangou, M. (2010). Evaluation of adhesion capacity, cell surface traits and immunomodulatory activity of presumptive probiotic Lactobacillus strains. Int. J. Food Microbiol. 140, 154–163. doi: 10.1016/j.ijfoodmicro.2010.04.004
Laparra, J. M., and Sanz, Y. (2009). Comparison of in vitro models to study bacterial adhesion to the intestinal epithelium. Lett. Appl. Microbiol. 49, 695–701. doi: 10.1111/j.1472-765X.2009.02729.x
Lebeer, S., Vanderleyden, J., and De Keersmaecker, S. C. J. (2008). Genes 2 and molecules of lactobacilli supporting probiotic action. Microbiol. Mol. Biol. Rev. 72, 728–764. doi: 10.1128/MMBR.00017-08
Lee, Y. K., Lim, C. Y., Teng, W., Ouwehand, A. C., Tuomola, E. M., and Salminen, S. (2000). Quantitative approach in the study of adhesion of lactic acid bacteria to intestinal cells and their competition with enterobacteria. Appl. Environ. Microbiol. 66, 3692–3697. doi: 10.1128/AEM.66.9.3692-3697.2000
Marco, M. L., Heeney, D., Binda, S., Cifelli, C. J., Cotter, P. D., Foligne, B., et al. (2017). Health benefits of fermented foods: microbiota and beyond. Curr. Opin. Biotechnol. 44, 94–102. doi: 10.1016/j.copbio.2016.11.010
Markowicz, C., and Schmidt, M. T. (2015). Lactobacillus strains belonging to casei group display various adherence to enterocytes and mucus. Acta Sci. Pol. Technol. Aliment. 14, 247–255. doi: 10.17306/G.AFS.2015.3.26
McCracken, V. J., Chun, T., Baldeón, M. E., Ahrnè, S., Molin, G., and Mackie, R. I. (2002). TNF-alpha sensitizes HT-29 colonic epithelial cells to intestinal lactobacilli. Exp. Biol. Med. 227, 665–670. doi: 10.1177/153537020222700817
Molin, G. (2001). Probiotics in foods not containing milk or milk constituents, with special reference to Lactobacillus plantarum 299v. Am. J. Clin. Nutr. 73(2 Suppl.), 380S–385S. doi: 10.1093/ajcn/73.2.380s
Morelli, L. (2007). In vitro assessment of probiotic bacteria: from survival to functionality. Int. Dairy J. 17, 1278–1283. doi: 10.1016/j.idairyj.2007.01.015
Mueller, D., Triebel, S., Rudakovski, O., and Richling, E. (2013). Influence of triterpenoids present in apple peel on inflammatory gene expression associated with inflammatory bowel disease (IBD). Food Chem. 139, 339–346. doi: 10.1016/j.foodchem.2013.01.101
Neutra, M. R., and Forstner, J. F. (1988). “Gastrointestinal mucus: synthesis, secretion and function,” in Physiology of the Gastrointestinal Tract, ed. L. R. Johnson (New York, NY: Raven Press), 975–1009.
Niedzielin, K., and Kordecki, H. A. (2001). Controlled double-blind, randomised study on the efficacy of Lactobacillus plantarum 299v in patients with irritable bowel syndrome. Eur. J. Gastroenterol. Hepatol. 13, 1143–1147. doi: 10.1097/00042737-200110000-00004
Nissen, L., Chingwaru, W., Sgorbati, B., Biavati, B., and Cencic, A. (2009). Gut health promoting activity of new putative probiotic/protective Lactobacillus spp. Strains: a functional study in the small intestinal cell model. Int. J. Food Microbiol. 135, 288–294. doi: 10.1016/j.ijfoodmicro.2009.08.027
Noh, S. Y., Kang, S. S., Yun, C. H., and Han, S. H. (2015). Lipoteichoic acid from Lactobacillus plantarum inhibits Pam2CSK4-induced IL-8 production in human intestinal epithelial cells. Mol. Immunol. 64, 183–189. doi: 10.1016/j.molimm.2014.11.014
Ouwehand, A. C., Isolauri, E., and Salminen, S. J. (2002). The role of intestinal microflora for the development of the immune system in early childhood. Eur. J. Nutr. 41, I32–I37.
Ouwehand, A. C., Niemi, P., and Salminen, S. J. (1999). The normal fecal microflora does not affect the adhesion of probiotic bacteria in vitro. FEMS Microbiol. Lett. 177, 35–38. doi: 10.1111/j.1574-6968.1999.tb13710.x
Papadimitriou, K., Zoumpopoulou, G., Foligne, B., Alexandraki, V., Kazou, M., Pot, B., et al. (2015). Discovering probiotic micro organisms: in vitro, in vivo, genetic and omics approaches. Front. Microbiol. 6:58. doi: 10.3389/fmicb.2015.00058
Pathmakanthan, S., Meance, S., and Edwards, C. A. (2000). Probiotics: a review of human studies to date and methodological approaches. Microb. Ecol. Health Dis. 2, 10–30. doi: 10.1080/089106000750060251
Prete, R., Tofalo, R., Federici, E., Ciarrocchi, A., Cenci, G., and Corsetti, A. (2017). Food-associated Lactobacillus plantarum and yeasts inhibit the genotoxic effect of 4-Nitroquinoline-1-Oxide. Front. Microbiol. 8:2349. doi: 10.3389/fmicb.2017.02349
Reilly, N., Poulin, V., Menconi, M., Onderdonk, A., Bengmark, S., and Hasselgren, P. O. (2007). Probiotics potentiate IL-6 production in IL-1beta-treated Caco-2 cells through a heat shock-dependent mechanism. Am. J. Phisiol. Regul. Integr. Comp. Phisiol. 293, R1169–R1179. doi: 10.1152/ajpregu.00770.2006
Ren, D. Y., Li, C., Quin, Y. Q., Yin, R. L., Du, S. W., Ye, F., et al. (2013). Lactobacilli reduce chemokine IL-8 production in response to TNF-alpha and Salmonella challenge of Caco-2 cells. Biomed. Res. Int. 2013:925219. doi: 10.1155/2013/925219
Ruas-Madiedo, P., Gueimonde, M., Fernández-García, M., de los Reyes-Gavilán, C. G., and Margolles, A. (2008). Mucin degradation by Bifidobacterium strains isolated from the human intestinal microbiota. Appl. Environ. Microbiol. 74, 1936–1940. doi: 10.1128/AEM.02509-07
Schiffrin, E. J., Brassart, D., Servin, A. L., Rochat, F., and Donnet-Hughes, A. (1997). Immune modulation of blood leukocytes in humans by lactic acid bacteria: criteria for strain selection. Am. J. Clin. Nutr. 66, 515S–520S. doi: 10.1093/ajcn/66.2.515S
Schneider, M., Efferth, T., and Abdel-Aziz, H. (2016). Anti-inflammatory effects of herbal preparations STW5 and STW5-II in cytokine-challenged normal human colon cells. Front. Pharmacol. 7:393. doi: 10.3389/fphar.2016.00393
Siezen, R., and van Hylckama Vlieg, J. E. (2011). Genomic diversity and versatility of Lactobacillus plantarum, a natural metabolic engineer. Microb. Cell Fact. 10(Suppl. 1):S3. doi: 10.1186/1475-2859-10-S1-S3
Stanton, C., Gardiner, G., Meehan, H., Collins, K., Fitzgerald, G., Lynch, P. B., et al. (2001). Market potential for probiotics. Am. J. Clin. Nutr. 73, 476S–483S. doi: 10.1093/ajcn/73.2.476s
Stevenson, C., Blaauw, R., Fredericks, E., Visser, J., and Roux, S. (2014). Randomized clinical trial: effect of Lactobacillus plantarum 299v on symptoms of irritable bowel syndrome. Nutrition 30, 1151–1157. doi: 10.1016/j.nut.2014.02.010
Tallon, R., Arias, S., Bressollier, P., and Urdaci, M. P. (2007). Strain - and matrix-dependent adhesion of Lactobacillus plantarum is mediated by proteinaceous bacterial compounds. J. Appl. Microbiol. 102, 442–451. doi: 10.1111/j.1365-2672.2006.03086.x
Tuomola, E. M., Ouwehand, A. C., and Salminen, S. J. (1999). Human ileostomy glycoproteins as a model for small intestinal mucus to investigate adhesion of probiotics. Lett. Appl. Microbiol. 28, 159–163. doi: 10.1046/j.1365-2672.1999.00518.x
Turroni, F., Bottacini, F., Foroni, E., Mulder, I., Kim, J. H., Zomer, A., et al. (2010). Genome analysis of Bifidobacterium bifidum PRL2010 reveals metabolic pathways for host-derived glycan foraging. Proc. Natl. Acad. Sci. U.S.A. 107, 19514–19519. doi: 10.1073/pnas.1011100107
Van Tassell, M. L., and Miller, M. J. (2011). Lactobacillus adhesion to mucus. Nutrients 3, 613–636. doi: 10.3390/nu3050613
Keywords: adhesion, IL-8 cytokine, intestinal epithelium, Lactobacillus plantarum, mucus
Citation: Garcia-Gonzalez N, Prete R, Battista N and Corsetti A (2018) Adhesion Properties of Food-Associated Lactobacillus plantarum Strains on Human Intestinal Epithelial Cells and Modulation of IL-8 Release. Front. Microbiol. 9:2392. doi: 10.3389/fmicb.2018.02392
Received: 23 May 2018; Accepted: 18 September 2018;
Published: 08 October 2018.
Edited by:
Francesca Patrignani, Università degli Studi di Bologna, ItalyReviewed by:
Jorge Reinheimer, National University of the Littoral, ArgentinaSylvain S. Kamdem, The University of Yaounde I, Cameroon
Copyright © 2018 Garcia-Gonzalez, Prete, Battista and Corsetti. This is an open-access article distributed under the terms of the Creative Commons Attribution License (CC BY). The use, distribution or reproduction in other forums is permitted, provided the original author(s) and the copyright owner(s) are credited and that the original publication in this journal is cited, in accordance with accepted academic practice. No use, distribution or reproduction is permitted which does not comply with these terms.
*Correspondence: Natalia Battista, bmJhdHRpc3RhQHVuaXRlLml0 Aldo Corsetti, YWNvcnNldHRpQHVuaXRlLml0
†Equally first authors