- 1State Key Laboratory for Biology of Plant Diseases and Insect Pests, Institute of Plant Protection, Chinese Academy of Agricultural Sciences, Beijing, China
- 2Department of Biology, Carthage College, Kenosha, WI, United States
- 3Department of Biological Sciences, University of Wisconsin-Milwaukee, Milwaukee, WI, United States
Xanthomonas oryzae pv. oryzae (Xoo) causes bacterial leaf blight of rice, one of the most devastating bacterial diseases of this staple crop worldwide. Xoo produces a range of virulence-related factors to facilitate its pathogenesis in rice, however, the regulatory mechanisms of Xoo virulence expression have been not fully elucidated. Recent studies have revealed that virulence factor production is regulated via cyclic dimeric guanosine monophosphate (c-di-GMP) signaling pathway that is well-conserved in Xoo and other Xanthomonas species. A set of GGDEF, EAL, HD-GYP, and PilZ domain proteins with diverse signal sensory domains for c-di-GMP synthesis, hydrolysis, and binding is encoded in the Xoo genome. Bioinformatic, genetic, and biochemical analysis has identified an array of diguanylate cyclases (DGCs) and phosphodiesterases (PDEs), as well as degenerate GGDEF/EAL, PilZ domain proteins along with a transcription regulator. These signaling components have been characterized to regulate various bacterial cellular processes, such as virulence, exopolysaccharide (EPS) production, biofilm formation, motility, and adaptation at the transcriptional, post-translational, and protein-protein interaction levels. This review summarized the recent progress in understanding the importance and complexity of c-di-GMP signaling in regulating bacterial virulence expression, highlighting the identified key signal elements and orthologs found in Xanthomonads, discussing the diverse functions of GGDEF/EAL/HD-GYP domains, existence of a complicated multifactorial network between DGCs, PDEs, and effectors, and further exploration of the new c-di-GMP receptor domains. These findings and knowledge lay the groundwork for future experimentation to further elucidate c-di-GMP regulatory circuits involved in regulation of bacterial pathogenesis.
Introduction
The Gram-negative plant pathogen Xanthomonas oryzae pv. oryzae (Xoo) causes bacterial leaf blight disease of rice, one of the most devastating bacterial diseases of this staple crop in the world, leading to yield losses of rice production up to 20–50% under disease-favorable conditions (Mew et al., 1993; Niño-Liu et al., 2006). Xoo has become an ideal model to study the molecular mechanisms of bacterial pathogenesis in monocot plants (Niño-Liu et al., 2006; He et al., 2007; Salzberg et al., 2008; White and Yang, 2009). Typically, Xoo infects rice leaves through wounds or hydathodes, and then invades xylem vessels leading to systemic infection (Mew et al., 1993). Like many other Gram-negative bacteria, Xoo also possesses a type III secretion system (T3SS) to inject and deliver effectors into rice cells, some of which are critical determinants of pathogenesis during Xoo–rice interaction (Tsuge et al., 2006, 2014; White and Yang, 2009). Furthermore, diverse regulators including transcriptional factors, two-component transduction systems (TCS), and diffusible signal factor (DSF) pathways control the expression of virulence factors in a cooperative manner and contribute significantly to the virulence of Xoo in planta (Jha et al., 2007; Das et al., 2009; White and Yang, 2009; He et al., 2010; Yang et al., 2012; Tian et al., 2015; Li H. Y. et al., 2018). However, the regulatory mechanisms of bacterial virulence expression have been not fully understood in Xoo.
Pathogenicity of Xoo was immediately initiated in rice-Xoo interactions, while the expressions of T3SS-related hrp and c-di-GMP metabolic enzyme and receptor genes were significantly changed. For example, hrpG and hrpX were upregulated in 5 min after interacting with rice leaves, while 5 genes encoding c-di-GMP metabolic enzymes were firstly down-regulated, and then up-regulated. Absolute expression level of c-di-GMP receptor Clp was high, but its fold changes in expression were low, suggesting that Clp activity might be transcriptionally and/or post-translationally controlled via its binding to c-di-GMP. These studies indicate that both hrp-encoded T3SS and c-di-GMP signaling pathways play the important roles in interaction of Xoo to host rice (Kim et al., 2016). Xoo strains PXO99 and PXO86 from Philippines and GD1358 from China exhibited different virulence patterns in 30 rice varieties. Transcriptional expression profiling demonstrated that expression patterns of virulence-related genes including avrXA21, hrpG and the gum cluster in GD1358 were differential from those in PXO99 and PXO86. Thus, Xoo strains from the different regions exhibited distinctly different expression patterns of putative virulence-related genes (Zhang F. et al., 2013). A range of additional virulence-related factors, including extracellular polysaccharide (EPS) synthesis, biofilm formation, motility, extracellular cellulase, xylanase, catalase, and adhesin, are produced by Xoo to facilitate its pathogenesis (Ray et al., 2000; Jha et al., 2007; Das et al., 2009; White and Yang, 2009). Further insight into signaling pathways involved in regulation of virulence will contribute to the understanding of Xoo pathogenesis, and effectively allow the development of prevention strategies to facilitate new methods of control of pathogenic infection in rice.
Cyclic dimeric guanosine monophosphate (c-di-GMP) is a widespread second messenger in pathogenic bacteria (Hengge, 2009). The importance of c-di-GMP involvement in the regulation of various biological processes, such as virulence, EPS production, biofilm formation, motility, and pathogen environment adaptation has been illustrated in several bacteria (Hengge, 2009, 2013; Jenal et al., 2017). Downstream effects of c-di-GMP are dose-dependent; lower concentrations of intracellular c-di-GMP promote virulence factor production and motility, while higher concentrations invariably facilitate biofilm formation in bacteria (Jenal et al., 2017). The opposing actions of diguanylate cyclase (DGC) and phosphodiesterase (PDE) enzymes control the synthesis and degradation of c-di-GMP, respectively (Schirmer and Jenal, 2009). Generally, DGCs contain a conserved GGDEF domain, while PDEs possess conserved EAL or HD-GYP domains (Dow et al., 2006; Schirmer, 2016; Römling et al., 2017). Besides containing GGDEF, EAL, or HD-GYP domains, these proteins possess additional signal sensory and recognition domains that can integrate diverse environmental signals to the c-di-GMP signaling pathway (Römling et al., 2013; Jenal et al., 2017).
c-di-GMP binding promotes changes in the structure, as well as the function of the receptor/effector. This interaction allows changes in gene expression or enzyme activity to occur (Chou and Galperin, 2016; Yang et al., 2017). Several c-di-GMP receptors have been characterized from various bacterial species, including PilZ domain proteins, transcriptional regulators, degenerate GGDEF or EAL domain proteins, polynucleotide phosphorylase (PNPase), riboswitches and kinases (Hengge, 2009, 2013; Wang et al., 2012; Römling et al., 2013; Chou and Galperin, 2016). Functionally, c-di-GMP receptors exert their regulatory functions at the transcriptional, translational, and post-translational levels (Hengge, 2009, 2013; Jenal et al., 2017). Identification of receptors/effectors is of scientific importance, providing the basis for elucidation of c-di-GMP signaling pathway regulation of downstream functions.
Due to the economic importance of bacterial blight of rice and the excellent model of bacterial pathogens in monocot plants, recent studies on Xoo and close Xanthomonas species have revealed the importance and complexity of c-di-GMP signaling pathways in regulating virulence. The array of key components involved in c-di-GMP signal metabolism, reception, transduction and phenotypic regulation has been functionally identified and characterized, and regulatory circuits of virulence at the transcriptional, post-translational, and protein-protein interaction levels have been demonstrated. This review summarized the recent progress in understanding c-di-GMP signaling pathways and regulation of virulence in Xoo, all while relating those findings to other close Xanthomonas spp. including X. oryzae pv. oryzicola (Xoc), X. campestris pv. campestris (Xcc), and X. axonopodis pv. citri (Xac), highlighting the identified key signal elements and orthologs, discussing diverse functions of GGDEF/EAL/HD-GYP domains, existence of a complicated multifactorial network between DGCs, PDEs and effectors, and further exploration of new c-di-GMP receptor domains. These findings and knowledge lay the groundwork for future experimentation to further elucidate c-di-GMP regulatory circuits involved in the regulation of bacterial pathogenesis.
Recognition of the GGDEF, EAL and HD-GYP Domain Proteins
In the genome of Xoo PXO99A, 26 genes were annotated to encode GGDEF, EAL, and HD-GYP domain proteins revealed by bioinformatic analysis, including 11 GGDEF domain standalone proteins (GdpX1-11), 2 EAL domain standalone protein (EdpX1-2), 10 GGDEF/EAL domain proteins (GEdpX1-10), and 3 HD-GYP domain proteins (HGdpX1-3) (Figure 1) (Yang et al., 2012; Xue et al., 2015). A 212-kb genomic duplication, including PXO_00964, PXO_00965, PXO_00967, PXO_01019 (pdeR), and PXO_01021 was identified in the genome of Xoo strain PXO99A (Salzberg et al., 2008; Yang et al., 2012). Since having two copies in the genome, two designations PXO_01019 and PXO_06147 of pdeR were given accordingly (Table 1).
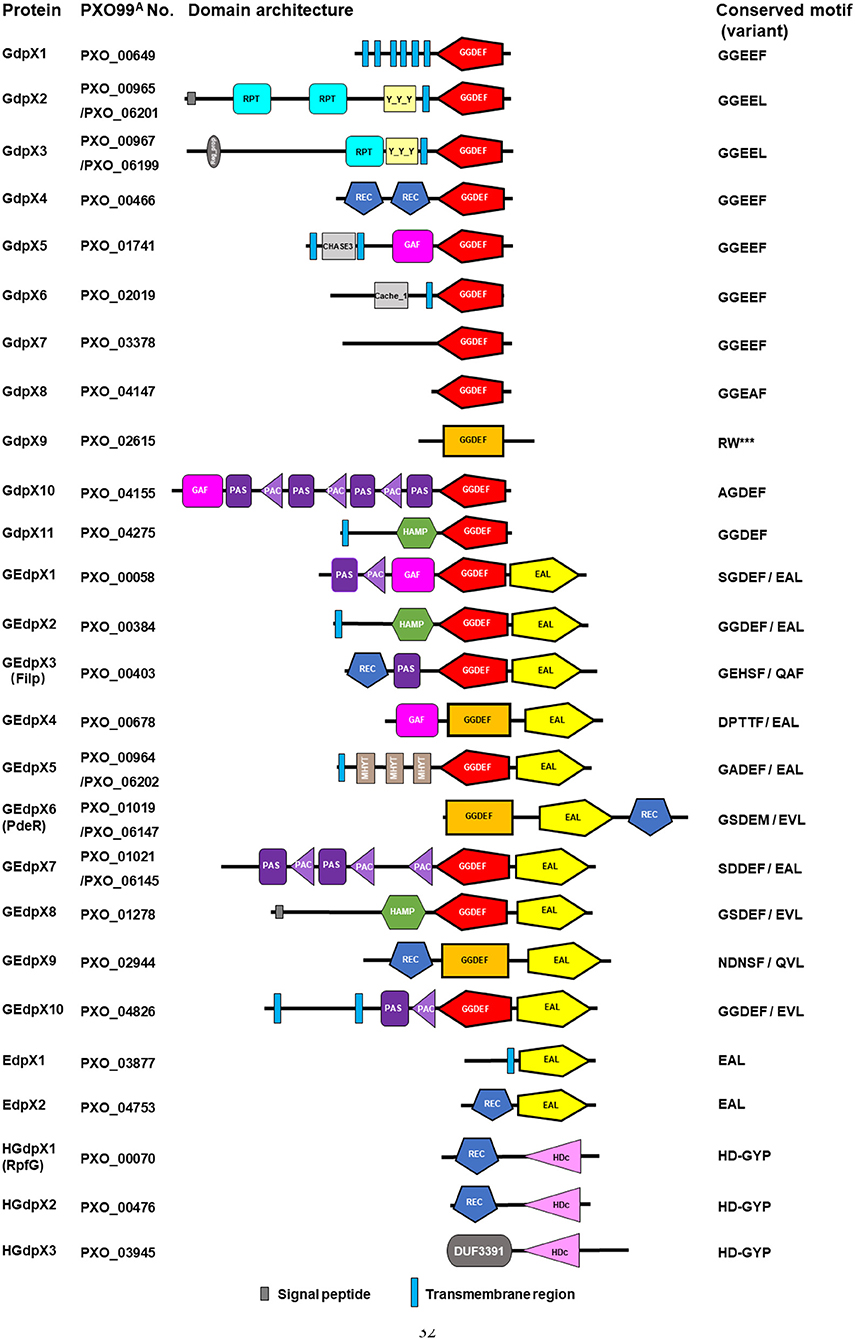
Figure 1. Identification of GGDEF, EAL and HD-GYP domain architecture and conserved motif in Xanthomonas oryzae pv. oryzae. Simple Modular Architecture Research Tool (SMART) (http://smart.embl-heidelberg.de/) was used to analyze the domains. The GGDEF domains implicated in c-di-GMP synthesis were shown in red, while the degenerate GGDEF domains in rectangle were predicted by SMART only with the Pfam database and displayed in orange. The EAL and HD-GYP domains implicated in c-di-GMP hydrolysis are illustrated in yellow and pink, respectively. ***means amino acid deficiency when compared with homologous proteins.
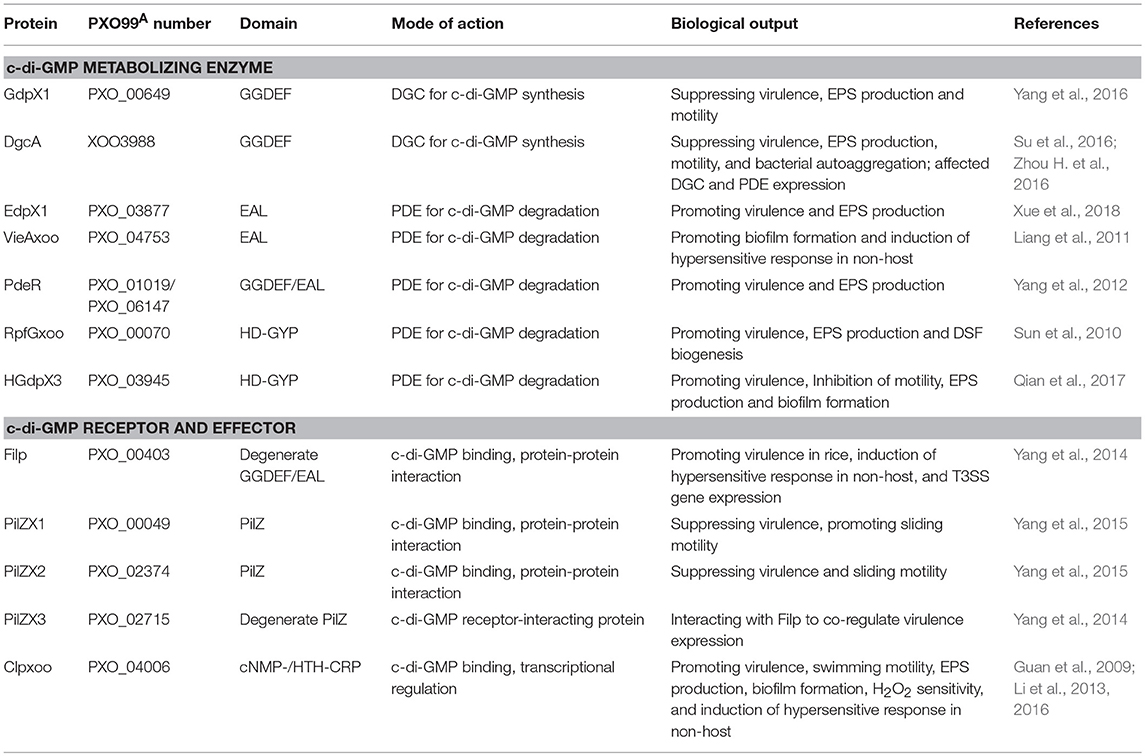
Table 1. Identification of c-di-GMP metabolizing enzymes, receptors, and effectors in Xanthomonas oryzae pv. Oryzae.
GGDEF domains are differentiated into three classes: enzymatically functional domains, enzymatically functional domains linked to an EAL domain, and enzymatically non-functional domains based on the domain homology and the signature/motif conservation (Römling et al., 2013; Römling and Galperin, 2017). Enzymatically functional domains consist of the GG(D/E)EF sequence motif, including the well-conserved D/E catalytic base and other residues involved in substrate binding and coordination of one of the two divalent cations. Enzymatically non-functional domains are usually characterized by a degenerate GGDEF motif, since any mutation within the motif abolishes the catalytic activity. In addition, the inhibitory site (I-site), designated by the central signature motif RXXD, is another functional feature that characterizes the activity profile (Römling et al., 2017). However, there are different studies indicating that several DGCs with some substitutions on the first amino acid of the canonical GG(D/E)EF motif are still functional in c-di-GMP synthesis (Pérez-Mendoza et al., 2011; Hunter et al., 2014; Baena et al., 2019). Sequence alignment analysis of 11 GdpXs showed the conserved GGDEF motifs in GdpX1, 4, 5, 6, 7, and 11, the conserved I-sites in GdpX2, 3, 4, 5, 6, 7, and 8, severely degenerate GGDEF motifs and I-sites in GdpX9 and GdpX10, and a degenerate GTP binding site in GdpX9 (Figure S1). Further sequence alignment analysis of an additional 10 GEdpXs indicated the conserved GGDEF motifs only in GEdpX2 and GEdpX10, and the degenerate I-sites in most of GEdpXs (Figure S2A). These findings suggest that most GdpXs and GEdpXs might be active and inactive DGCs, respectively. Furthermore, the proteins like GdpX10 and GEdpX1 with some substitutions on the first amino acid of the GG(D/E)EF motif might be DGC active.
EAL domains are divided into three classes: catalytically active, potentially catalytically active, and catalytically inactive. EAL domains can potentially possess enzymatic activity with deviation of residues from the conserved motif. Conserved EAL motifs and critical metal cation and substrate binding sites are vital for structure and function of the protein (Römling et al., 2017). Sequence alignment analysis indicated that EAL motifs and residues were conserved in GEdpXs, except for GEdpX3 (Filp) and GEdpX9 (PXO_02944) and all of EdpXs (Figures S2B, S3). Filp was experimentally confirmed to be a c-di-GMP receptor as described below (Yang et al., 2014). GEdpX9 was identified as a putative c-di-GMP receptor to negatively regulate virulence, EPS production and biofilm formation (Li et al., 2014). These data indicate that most EdpXs and GEdpXs might be PDE active, while Filp and GEdpX9 are experimentally confirmed and putative c-di-GMP effectors, respectively.
HD-GYP domain proteins with the conserved HD and GYP residues hydrolyze both c-di-GMP and 5'-pGpG into GMP (Römling et al., 2013). Sequence alignment analysis of three HGdpXs revealed that the HD-GYP domains were well-conserved in HGdpX1-3 that were predicted as the active PDEs for c-di-GMP hydrolysis (Figure S4).
Besides these regulatory domains mentioned above, several sensory domains in GdpXs, EdpXs, GEdpXs, and HGdpXs were identified through bioinformatic analysis, including the REC, PAS(C), GAF, HAMP, Cache_1, CHASE3, Reg_prop- and Y_Y_Y, MHYT, and DUF domains (Figure 1; Table 2). These data provide information that diverse signal input domains modulate the activity of downstream GGDEF, EAL, or HD-GYP output domains by perceiving various environmental stimuli within the complicated c-di-GMP signaling network in Xoo (Xue et al., 2015).
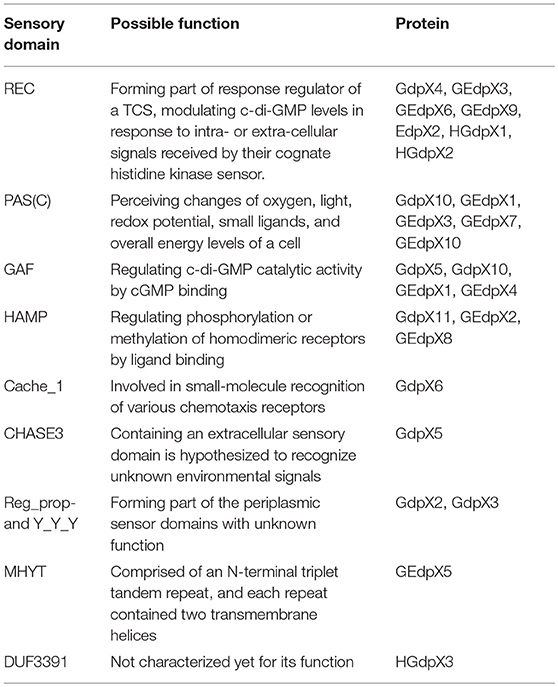
Table 2. Bioinformatic analysis of sensory domains of GdpXs, EdpXs, GEdpXs and HGdpXs in Xanthomonas oryzae pv. Oryzae.
Identification of c-di-GMP Metabolizing-Enzymes
GdpX1 and DgcA, Two DGCs That Similarly Regulate Virulence Factor Traits
GdpX1 (PXO_00649) encodes a well-conserved GGDEF domain standalone protein that was predicted as a putative DGC (Figure 1; Figure S1). Its regulatory function in virulence was identified by genetic and biochemical analysis (Yang et al., 2016). Deletion of gdpX1 resulted in increased virulence, EPS production, and flagellar motility, while overexpression of GdpX1 in the wildtype led to attenuated virulence and decreased EPS synthesis. Moreover, GdpX1 suppressed the transcription of genes involved in type III secretion system (T3SS) activation, EPS synthesis, and flagellar motility. These results imply that GdpX1 is a putative DGC and suppresses bacterial virulence traits in this pathogen (Table 1) (Yang et al., 2016). Further characterization of the DGC activity of GdpX1 and intracellular c-di-GMP levels in the gene deletion mutant and overexpression strains will be helpful for understanding the regulatory mechanisms of virulence by GdpX1 in Xoo.
Similarly, DgcA (XOO3988) from Xoo strain KACC10331 encodes a well conserved GGDEF domain protein with 99.6% sequence identity to GdpX11 (PXO_04275) from PXO99A. The DgcA's DGC activity was first characterized in the study of a c-di-GMP riboswitch (Zhou H. et al., 2016). DgcA negatively affected bacterial pathogenicity on rice, EPS production, motility, and autoaggregation via modulating the intracellular c-di-GMP levels, demonstrating DgcA as an active DGC and major virulence regulator of in Xoo (Su et al., 2016). Furthermore, DgcA affected the expression of an array of genes encoding GGDEF and EAL domains, indicating an additional role of DgcA in the regulation of DGC and PDE expression (Su et al., 2016) (Table 1).
EdpX1, an Active PDE That Promotes Virulence, EPS Production, and Biofilm Formation
EdpX1 (PXO_03877), encoding a well conserved EAL domain standalone protein, was annotated as a PDE (Figures 1, 3). Genetic assays indicated that deletion of edpX1 led to increased intracellular c-di-GMP levels, which can be restored to wildtype levels via complementation of edpX1. In vitro colorimetric assays showed EdpX1 displayed PDE activity for c-di-GMP degradation, and a point mutation of E153A at the EAL motif strongly reduced its activity. ΔedpX1 exhibited a severely impaired virulence phenotype in rice, EPS production, and biofilm formation. In trans expression of wildtype edpX1, but not edpX1E153A, was able to restore these phenotypes to near wild type levels. These observations indicate that EdpX1 functions as an active c-di-GMP-specific PDE and promotes virulence, EPS production, and biofilm formation (Table 1) (Xue et al., 2018).
PdeR, a PDE That Is Promoted by Its Cognate HK PdeK and Interactor TriP
It was previously reported that a HK/RR pair of a TCS of Xcc, RavS (XCC1960)/RavR (XCC1958), interplayed with the DSF-mediated quorum sensing (QS), and regulated EPS and extracellular protease secretion, and their homologs were well-conserved in other close species and pathovars Xoo, Xoc, Xcv, and Xac (He et al., 2009; Wei et al., 2016). Low-oxygen tension is a common stress in the progress of bacterial pathogen infection or in stationary phase growth, and the PAS domain normally senses environmental signals such as oxygen, redox, and light (Girvan and Munro, 2013). RavS/RavR play a key role of in regulation of bacterial virulence in response to low-oxygen tension through the PAS domain of RavS. In addition, RavS/RavR regulates virulence gene expression through the global regulator Clp (He et al., 2009).
In Xoo PXO99A, PXO_01020, and PXO_01019 (PdeR) were the homologs of RavS and RavR, respectively. PdeR, a GGDEF/EAL/REC-domain containing protein was identified as a RR to form a pair of TCS with the cognate histidine kinase (HK) PXO_01018 (PdeK), but not PXO_01020 (Figure 1) (Yang et al., 2012). PdeK showed 95.7% identity with another HK XCC1957 adjacent to RavR in Xcc. PdeR had PDE activity for c-di-GMP hydrolysis in vitro, which was triggered by PdeK. Attenuated virulence in rice and less EPS secretion resulted from the deletion of pdeR or pdeK, but not PXO_01020. These observations suggest that PdeK/PdeR is a novel TCS involved in regulation of c-di-GMP turnover and virulence expression in Xoo (Table 1) (Yang et al., 2012). The differences in TCS constitution between PdeK/PdeR and RavS/RavR might be due to genetic divergence of in Xanthomonas species and pathovars.
To further reveal the key elements in the PdeR-mediated c-di-GMP signaling, TriP (PXO_04421), a putative transcriptional regulator interacting with PdeR was identified and characterized. TriP was well-conserved with 100% identifies in other close Xanthomonas species and pathovars. The REC domain of TriP specifically interacted with the EAL domain of PdeR. TriP promoted the PDE activity of PdeR in the presence of PdeK. In-frame deletion in triP abolished polar localization of PdeR in the cells, significantly reduced virulence in rice, and impaired EPS production. The double mutant ΔtriPΔpdeR, like ΔpdeR, caused shorter lesion lengths and produced less EPS than the single mutant ΔtriP. Cross-complementation showed in trans expression of pdeR in ΔtriP restored its EPS production to near wildtype levels, but not vice versa. Accordingly, TriP is a novel regulator epistatic to PdeR in promoting virulence expression (Table 1) (Li H. Y. et al., 2018). This is the first demonstration of the REC-EAL domain interaction of two RRs and TriP, and its function in virulence regulation via PdeR-mediated c-di-GMP signaling in Xanthomonads. Though broader implications of the PdeK/PdeR/TriP/TriK signaling cascade and its conservation in Xanthomonas need to be further explored, a schematic diagram showing the TriP interaction with the EAL domain of PdeR via the REC domain and promotion of the PDE activity of PdeR in the presence of PdeK in Xoo has been presented (Figure 2). PdeR functions as a PDE for c-di-GMP hydrolysis and forms a TCS with PdeK. TriP specifically interacts with PdeR, but not PdeK. The REC and EAL domains are essential for the TriP-PdeR interaction. Such an interaction significantly affects PdeR's PDE ability to degrade c-di-GMP into pGpG and bipolar localization, thereby regulating bacterial virulence and EPS production in a c-di-GMP-dependent manner (Yang et al., 2012; Li H. Y. et al., 2018).
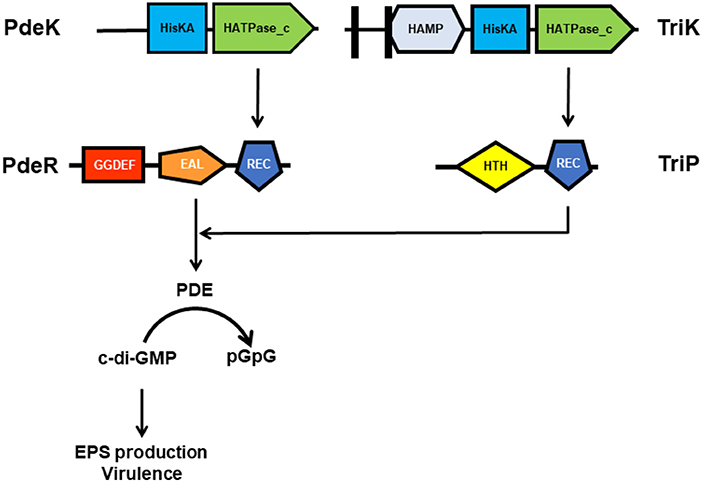
Figure 2. A schematic diagram showing the TriP-PdeR interaction resulting in enhanced PDE activity of PdeR in the presence of PdeK in Xanthomonas oryzae pv. Oryzae. PdeR functions as a PDE for c-di-GMP hydrolysis and forms a TCS with PdeK. TriP specifically interacts with the EAL domain of PdeR via the REC domain. The TriP-PdeR interaction significantly enhances PdeR's PDE ability for degradation of c-di-GMP into pGpG, thereby promoting EPS production and bacterial virulence in a c-di-GMP-dependent manner.
HGdpX1-3, Functional PDEs That Differentially Regulate Virulence Traits
It has been generally known that DSF mediates QS signaling pathways and plays crucial roles in regulation of virulence or virulence factor production, including extracellular enzyme synthesis, EPS production, biofilm formation, and cellular dispersion in Xanthomonas species (Dow, 2008; Barel et al., 2015; Zhou L. et al., 2016). The sensor HK RpfC and RR RpfG constitute a TCS involved in the DSF signal perception and transduction (Slater et al., 2000; He et al., 2010). RpfC senses DSFs to activate its autokinase activity, thereby activating RpfG for PDE activity (Cai et al., 2017). RpfG contains a receiver domain for signal transduction and a HD-GYP domain for c-di-GMP degradation. In addition, RpfC regulates the expression of hrp genes via RpfG and the global transcriptional regulator Clp (Jiang et al., 2018). Furthermore, RpfG's regulation of downstream signaling components might be at the post-transcriptional and post-translational levels. For example, RpfG interacts with some GGDEF domain proteins and the TCS NtrBC (Andrade et al., 2006; Ryan and Dow, 2010). Proteomics analysis revealed that RpfG, RpfC, and RpfF regulated the expression of a number of new virulence factors belonging to several functional categories, including biosynthesis and intermediary metabolism, regulation, oxidative stress or antibiotic resistance, and DNA replication. Among them, the elongation factor P (XC_2359) and a putative outer membrane protein (XC_0650), both widely conserved in bacteria, were characterized as the novel virulence factors regulated by the RpfC/RpfG/RpfF system at post-transcriptional but not transcriptional level (O'Connell et al., 2013). RpfG was also identified as a global regulator controlling EPS production that contribute to biofilm formation and bacterial virulence on rice in Xoc (Zhang Y. et al., 2013).
The RpfC/RpfG-mediated DSF/c-di-GMP signaling pathways and their regulation of virulence factor production was earlier shown to be well-conserved in Xcc and Xoo (He et al., 2010). HGdpX1 (PXO_00070, RpfGxoo), a Xcc RpfG homolog, was identified to promote virulence in rice and EPS production (Figure 1; Figure S4) (Sun et al., 2010). The TCS RpfCxoo/RpfGxoo was reported to negatively regulate the expression of rpfB that played an important role in DSF signal turnover via Clp (Wang X. Y. et al., 2016). In addition, two other HD-GYP domain proteins HGdpX2 (PXO_00476) and HGdpX3 (PXO_03945) have been characterized to degrade c-di-GMP and promote virulence in Xoo (Figure 1; Figure S4) (Qian et al., 2017). These observations imply that HGdpX1-3 might be active PDEs, and differentially regulate bacterial virulence traits (Table 1).
In Xcc, PcrR forms a novel TCS with its cognate HK PcrK that specifically binds the plant cytokinin 2-isopentenyladenine (2iP) via its CHASE domain. Through a four-step phosphorelay, 2iP binding results in decreased autokinase activity of PcrK and phosphorylation level of PcrR, thereby activating PcrR's PDE activity for c-di-GMP degradation. The TCS PcrK/PcrR affects bacterial tolerance to oxidative stress through regulating the gene transcription. These findings reveal an inter-kingdom signaling by which Xcc intercepts a plant derived hormone signal to promote adaptation to oxidative stress (Wang et al., 2017). Furthermore, PcrK and PcrR show 81.4% and 84.91% sequence identities with PXO_00475 and PXO_00476 (HGdpX2) of Xoo, and 82,7% and 85.76% identities with XOC_1985 and XOC_1984 of Xoc, respectively, indicating this TCS is more widely conserved in other Xanthomonads.
Characterization of c-di-GMP Receptors and Effectors
Filp, a Degenerate GGDEF/EAL Domain Protein That Regulates Virulence Expression With Its Interactor PilZX3
FimX was primarily characterized as a PDE regulating twitching motility in Pseudomonas aeruginosa (Huang et al., 2003; Kazmierczak et al., 2006). Subsequent studies revealed that FimX functioned as a receptor for c-di-GMP binding via the degenerate GGDEF/EAL domains (Navarro et al., 2009). The FimX-like proteins in Xac were also characterized to regulate bacterial type IV pilus (T4P) biogenesis through interacting with a degenerate PilZ domain protein to form a FimX-c-di-GMP-PilZ complex; And a novel mechanism underlying the regulation of T4P biogenesis by this complex via binding to an ATPase PilB required for T4P polymerization has been proposed in Xac (Guzzo et al., 2009, 2013). This is the first evidence of direct interactions of FimX and PilZ orthologs with the TP4 machinery.
In Xoo, Filp (PXO_00403) was identified as a FimX-like, degenerate GGDEF/EAL domain hybrid protein, which showed 96.8 and 93.8% protein sequence identifies to FimXXac2398 and XccFimX, respectively (Figure 1; Figure S2) (Yang et al., 2014). Filp functions as a c-di-GMP binding protein through its EAL domain in vitro. Filp regulated virulence in rice, in which the REC, PAS, and EAL domains, but not the GGDEF domain, were required for full activity. Notably, Filp specifically interacted with a degenerate PilZ domain protein PilZX3 (PXO_02715). Deletion of pilZX3 led to the same changes in bacterial virulence and T3SS gene expression as the Δfilp mutant. This indicates that Filp is a novel c-di-GMP receptor to co-regulate bacterial virulence with its interactor PilZX3 (Table 1) (Yang et al., 2014). XOC_2102, a Filp homolog in Xoc, showed same ability for c-di-GMP binding (Wei et al., 2016). Taken together, the regulation of bacterial motility and virulence mediated by FimX-like proteins might be well-conserved in P. aeruginosa and Xanthomonas spp.
Deciphering the complexity of c-di-GMP signaling is of importance for a comprehensive understanding of molecular mechanisms underlying bacterial virulence expression and regulation (Römling et al., 2013). To elucidate the regulatory mechanisms of virulence by Filp/PilZX3, isobaric tags for relative and absolute quantitation (iTRAQ) analysis of Δfilp, ΔpilZX3, and ΔfilpΔpilZX3, compared with the wildtype strain was performed. A subset of differentially-expressed proteins including c-di-GMP metabolizing enzymes, HKs, and TonB receptors were identified, in which two PDEs, VieAxoo and HGdpX2, significantly affected bacterial virulence in a Filp/PilZX3-dependent manner (Table 1) (Liang et al., 2011). This provides evidence of the existence of novel feedback regulatory circuits comprising Filp, PilZX3, and VieAxoo or HGdpX2 in c-di-GMP signaling networks of Xoo.
PilZX1-4 Function Differentially in c-di-GMP Binding and Regulation of Virulence
PilZ domain containing proteins have been known as one of the major types of c-di-GMP receptors/effectors in many bacteria (Chou and Galperin, 2016). PliZ domain proteins are widely conserved in the closely-related Xanthomonas spp. Four PilZ domain proteins were previously reported to be involved in the regulation of virulence and related traits in Xcc. Among them, XC0965, XC2249, and XC3221 promoted the virulence in Chinese radish, XC2249 and XC3221 enhanced the motility, and XC2249 and XC0965 regulated extracellular enzyme production of McCarthy et al. (2008).
In Xoo, PilZX1-4, which constitute 4 homologous proteins PXO_00049, PXO_02374, PXO_02715, and PXO_00997/PXO_06169, were identified through bioinformatics analysis. Both PilZX1-2 possessed the conserved PilZ domains, while PilZX3-4 harbored the degenerate ones (Figure S5). PilZX1-2 directly bound to c-di-GMP with high affinity, and negatively affected bacterial virulence and sliding motility, while PilZX3 functioned as a specific interactor of the c-di-GMP receptor Filp, and positively regulated bacterial virulence (Table 1) (Yang et al., 2014, 2015). Furthermore, PilZX1-2 displayed multisite subcellular localizations, whereas PilZX3 showed non-polar distributions in the cells (Yang et al., 2015).
Clpxoo, a c-di-GMP Receptor and Transcription Regulator
Since CRP was first identified to bind with cAMP in Escherichia coli, it has been extensively studied as a global regulator to regulate carbon source metabolism in bacteria and other signaling pathways, through controlling the transcription of downstream genes (Weber and Steitz, 1987; Zheng et al., 2004; Almagro et al., 2018). A CRP-like protein Clp was identified as a c-di-GMP receptor, and c-di-GMP allosterically inhibits the DNA-binding activity of transcription factor Clp in Xac (Leduc and Roberts, 2009). The role of Clp was confirmed as a c-di-GMP effector, whose binding to the promoter of the endoglucanase gene engXCA, was inhibited by c-di-GMP in Xcc (Chin et al., 2010; Tao et al., 2010). Clp regulated the transcription of a number of genes revealed by DNA microarray analysis (He et al., 2007). Structural and functional analysis of Clp showed key c-di-GMP binding sites D70, R154, R166 and D170, and the target promoter binding sites E99, R150, R195, D162, and V165 (Chin et al., 2010; Tao et al., 2010). In Xoo, Clpxoo (PXO_04006) containing the cNMP-/HTH-DNA binding domain was identified as a c-di-GMP receptor and transcription factor (Guan et al., 2009). Structurally, Clpxoo may substantially change upon interacting with c-di-GMP,resulting in inhibition of binding to engAxoo-p (Li et al., 2013). According to the site-directed mutagenesis of Clp in Xcc, ClpxooD70A, ClpxooE99S and ClpxooR166A, site 70 of aspartic acid and 99 of glutamic acid were identified to be two key residues for Clpxoo's binding to c-di-GMP (Li et al., 2016). Furthermore, Clpxoo positively regulated virulence, EPS production, biofilm formation, and resistance to hydrogen peroxide (H2O2) (Guan et al., 2009). These findings manifest that Clpxoo is a c-di-GMP effector to regulate bacterial virulence phenotypes at the transcriptional levels in Xoo.
Summary and Future Issues
Implication of c-di-GMP Signaling Pathways in Regulation of Virulence in Xoo
Resolving the highly complex pathways of c-di-GMP signaling is of utmost importance for a comprehensive understanding of the molecular mechanisms underlying the regulation of virulence expression in plant-pathogenic bacteria, including Xanthomonas spp. Recent studies have uncovered several components involved in c-di-GMP signaling pathways and led to significant gains in our knowledge and understanding of c-di-GMP regulatory circuits of virulence-related traits in Xoo. Here we proposed model outlining the identified c-di-GMP signaling components and their regulation of virulence in Xoo (Figure 3). An array of GGDEF, EAL, and HD-GYP domain proteins encoded in the genome were identified as DGCs and PDEs for c-di-GMP synthesis and degradation. PdeR, EdpX1, RpfGxoo, VieAxoo, and HGdpX3 functioned as PDEs to degrade c-di-GMP, and promotes EPS production, biofilm formation, and virulence. In contrast, GdpX1 and DgcA acted as DGCs and controlled EPS production and virulence in a manner opposite to PdeR, EdpX1, RpfGxoo, VieAxoo and HGdpX3. This regulation scheme is consistent with the general theory that low c-di-GMP levels promote virulence factor production, while high c-di-GMP levels inhibit virulence factor production in pathogenic bacteria.
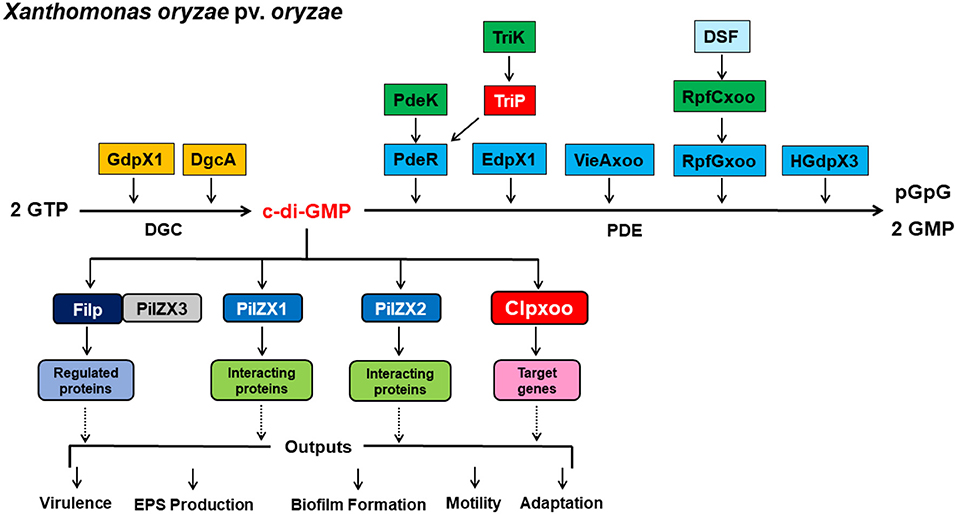
Figure 3. A proposal model outlining c-di-GMP signaling regulation of virulence expression in Xanthomonas oryzae pv. Oryzae. c-di-GMP is a ubiquitous, bacterial second messenger that regulates phenotypes including virulence, EPS production, biofilm formation, motility, and adaptation. c-di-GMP is produced by DGCs GdpX1 and DgcA, and degraded by PDEs PdeR, EdpX1, VieAxoo, RpfGxoo, and HGdpX3. PdeK/PdeR, TriK/TriP, and RpfCxoo/RpfGxoo are 3 pairs of the histidine kinase/response regulator of two component systems (TCSs). c-di-GMP is sensed by receptor proteins Filp, PilZX1, PilZX2, and Clpxoo. Particularly, Filp interacts with PilZX3 for c-di-GMP binding and downstream regulation. Signal receptor and effector proteins then interact with the downstream target proteins or genes to affect a particular cellular function and phenotype, including bacterial virulence, EPS production, biofilm formation, motility, and adaptation at the transcription, post-translation and protein-protein interaction levels.
Functional Diversification of GGDEF/EAL/HD-GYP Domains
GGDEF, EAL, and HD-GYP domain proteins are widely distributed in bacteria, and some of them have been characterized in Xanthomonas spp (Schirmer and Jenal, 2009; Yang et al., 2012; Wei et al., 2016). For example, the roles of 11 GGDEF-EAL domain proteins were revealed in Xoc (Wei et al., 2016). Among them, XOC_2335 and XOC_2393 enhanced bacterial swimming motility, while XOC_2102, XOC_2393, and XOC_4190 inhibited sliding motility. In addition, XOC_2335, XOC_2393, and XOC_4190 were identified to be involved in regulating virulence to rice. Notably, XOC_2335 was a homolog of GEdpX5 unidentified in Xoo, while XOC_2393, XOC_2102, and XOC_4190 were the homologs of PdeR, Filp, and GEdpX9 that were characterized in Xoo, respectively. XOC_2102 showed same ability for c-di-GMP binding as Filp did, and XOC_4190 and GEdpX9 might function as the novel c-di-GMP receptors in these two pathvars. Furthermore, dynamic interactions between RpfG and two GGDEF domain proteins, XC_0249 and XC_0420, were characterized to be dependent on DSF signaling in Xcc (Ryan and Dow, 2010; Ryan et al., 2015). There might exist a complicated protein-protein interaction network between the GGDEF, EAL, and HD-GYP domain proteins in Xoo (Guo et al., 2013). Accordingly, these GGDEF/EAL/HD-GYP domain proteins may function not only as c-di-GMP metabolizing enzymes or receptors, but also act as elements within interacting networks to regulate bacterial processes. Further exploration of the interactome of GGDEF, EAL, and HD-GYP domain proteins will helpful to uncover the c-di-GMP signaling protein network and will hopefully allow a comprehensive understanding of the regulatory mechanisms of bacterial virulence at the post-translational and protein-protein interaction levels.
Further Exploration of Novel c-di-GMP Receptor Domains
Several c-di-GMP receptors have been characterized in Xoo, including one degenerate GGDEF/EAL domain protein, 4 PilZ domain proteins, and one transcriptional regulator (Yang et al., 2017). Since the number of DCGs and PDEs encoded in the genome is far larger than that of known c-di-GMP receptors, it is likely that more receptors remain to be explored in this pathogen. An array of new c-di-GMP receptors and effectors has been recently identified in Xanthomonads and other bacteria. One example is YajQ (XC_3703), a PNPase from Xcc, and XOC_4190 from Xoc, which was well-conserved in Xanthomonas spp (An et al., 2014; Zhao et al., 2016). YajQ showed 96% identity with PXO_03091, and XOC_4190 displayed 97.4% similarity with GEdpX9, suggesting that PXO_03091 and GEdpX9 might be two new c-di-GMP receptors in Xoo. In addition, a Differential Radial Capillary Action of Ligand Assay (DRaCALA) screen identified the c-di-GMP receptor MshE, an ATPase associated with the mannose sensitive hemagglutinin (MSHA) T4P in Vibrio cholerae, meanwhile an ATPase of type II secretion system (T2SS) in Pseudomonas aeruginosa, the homolog of MshE was also identified as a signal receptor, indicating both T4P and T2SS are regulated by c-di-GMP signaling (Roelofs et al., 2015). The N-terminal of MshE (MshEN) was characterized to be the c-di-GMP receptor domain with two 24-residue motifs [RLGxx(L)(V/I)xxG(I/F)(L/V)xxxxLxxxLxxQ] that were linked by a 5-residue spacer to form a complete 53-residue-long domain (Wang Y. C. et al., 2016). This domain was also found present in XpsE or GpsE, a T2SS protein in Xcc (Shiue et al., 2006), while PXO_02675 in Xoo is a homolog of XpsE with 94.26% sequence identity. An affinity capture screen for c-di-GMP binding proteins was performed and flagella export AAA+ ATPase FliI was identified to be c-di-GMP receptor in P. fluorescens. ATPases HrcN of T3SS in P. syringae pv. tomato and ClpB2 of T4SS in P. fluorescens, the homologs of FliI were identified as c-di-GMP receptors (Trampari et al., 2015). Therefore, ATPase is a novel class of c-di-GMP receptor. Among 62 proteins annotated as ATPases in Xoo, PXO_02675 (a T2SS protein), PXO_03396 (a T3SS protein HrcN), and PXO_06188 (a flagellar protein FliI) might be the new c-di-GMP signal receptors. Moreover, diverse proteins and riboswitches were identified as novel c-di-GMP effectors in other pathogenic bacteria, for instance, FleQ, a transcription regulator from P. aeruginosa, and SgmT, a HK from Myxococcus xanthus (Kulshina et al., 2009; Baraquet et al., 2012; Petters et al., 2012; Tang et al., 2016; Weinberg et al., 2017). FleQ's homologs widely existed in Xanthomonads, which were the major transcriptional activators of expression of flagellar genes in Xcc and Xoo (Tian et al., 2015; Bae et al., 2018). Furthermore, Many HKs were extensively characterized for their biological functions in Xcc and Xoo (Qian et al., 2008). Therefore, identification of these novel c-di-GMP receptors in other bacteria would be informative for further exploration of the unknown signal receptors and effectors in Xoo.
Multiple Regulatory Mechanisms Mediated by c-di-GMP Receptors
It has been generally recognized that c-di-GMP receptors exert their regulatory function at the transcriptional and post-transcriptional levels. For example, Clp of Xcc and Clpxoo of Xoo function as transcription factors to affect bacterial functions through direct binding of the target gene promoters to control transcription. Recent studies showed that c-di-GMP receptors including Filp, FimXxac, and YajQ transmitted communication via protein-protein interaction in Xanthomonas spp., and intracellular c-di-GMP concentrations significantly affected the interactions between the signal receptors and their downstream proteins (Guzzo et al., 2009, 2013; An et al., 2014; Zhao et al., 2016). Furthermore, a novel mechanism underlying the Clp-LchP complex formation that results in increased PDE activity of LchP has been recently demonstrated in Lysobacter (Xu et al., 2018). Thus, deciphering the signaling networks formed by c-di-GMP receptors and its interactors, particularly between PDEs and DGCs, would be helpful to comprehensively understand the molecular mechanism underpinning the c-di-GMP receptor-mediated regulation in pathogenic bacteria.
Taken together, more investigation is required for deciphering the molecular mechanisms of c-di-GMP signaling during bacterial pathogenesis and adaptation to environmental stresses. In order to further our understanding, the next 5 steps must be taken: (1) characterize the functions of the remaining GGDEF, EAL, and HD-GYP domain proteins, (2) determine the environmental cues that affect the DGC and PDE activities and intracellular c-di-GMP levels through the sensory input domains, (3) characterize the c-di-GMP receptor-mediated regulatory pathways via the transcription and post-translation mechanisms, (4) assess c-di-GMP regulation of bacterial type III secretion system (T3SS), and (5) uncover the specificity in c-di-GMP signaling through protein-protein interaction mechanisms.
Author Contributions
FY and DX performed experiments. FY, DX, FT, WH, C-HY, and CH analyzed data and wrote the manuscript.
Funding
Work in the He laboratory was supported by grants from the Natural Science Foundation of China (31671990, 31400117, 31370160, 31100947), the Beijing Natural Science Foundation (5142017), the China Postdoctoral Science Foundation (2014T70149, 2012M520476), and the Fundamental Research Funds from Chinese Academy of Agricultural Sciences (1610142017017, Y2015JC20).
Conflict of Interest Statement
The authors declare that the research was conducted in the absence of any commercial or financial relationships that could be construed as a potential conflict of interest.
Supplementary Material
The Supplementary Material for this article can be found online at: https://www.frontiersin.org/articles/10.3389/fmicb.2019.01402/full#supplementary-material
References
Almagro, G., Viale, A. M., Montero, M., Munoz, F. J, Baroja-Fernandez, E., Mori, H., et al. (2018). A cAMP/CRP-controlled mechanism for the incorporation of extracellular ADP-glucose in Escherichia coli involving NupC and NupG nucleoside transporters. Sci. Rep. 8:5509. doi: 10.1038/s41598-018-33647-w
An, S. Q., Caly, D. L., McCarthy, Y., Murdoch, S. L., Ward, J., Febrer, M., et al. (2014). Novel cyclic di-GMP effectors of the YajQ protein family control bacterial virulence. PLoS Pathog. 10:e1004429. doi: 10.1371/journal.ppat.1004429
Andrade, M. O., Alegria, M.C., Guzzo, C. R., Docena, C., Rosa, M. C., Ramos, C. H., et al. (2006). The HD-GYP domain of RpfG mediates a direct linkage between the Rpf quorum-sensing pathway and a subset of diguanylate cyclase proteins in the phytopathogen Xanthomonas axonopodis pv. Citr. Mol. Microbiol. 62, 537–551. doi: 10.1111/j.1365-2958.2006.05386.x
Bae, N., Park, H. J., Park, H., Kim, M., Do, E., and Han, S. W. (2018). Elucidating functions of FleQ in Xanthomonas oryzae pv. oryzae by comparative proteomic and phenotypic analyses. Int. J. Mol. Sci. 19:E3038. doi: 10.3390/ijms19103038
Baena, I., Pérez-Mendoza, D., Sauviac, L., Francesch, K., Martín, M., Rivilla, R., et al. (2019). A partner-switching system controls activation of mixed-linkage β-glucan synthesis by c-di-GMP in Sinorhizobium meliloti. Environ. Microbiol. doi: 10.1111/1462-2920.14624. [Epub ahead of print].
Baraquet, C., Murakami, K., Parsek, M. R., and Harwood, C. S. (2012). The FleQ protein from Pseudomonas aeruginosa functions as both a repressor and an activator to control gene expression from the pel operon promoter in response to c-di-GMP. Nucleic Acids Res. 40, 7207–7218. doi: 10.1093/nar/gks384
Barel, V., Chalupowicz, L., Barash, I., Sharabani, G., Reuven, M., Dror, O., et al. (2015). Virulence and in planta movement of Xanthomonas hortorum pv. pelargonii are affected by the diffusible signal factor (DSF)-dependent quorum sensing system. Mol. Plant Pathol. 16, 710–723. doi: 10.1111/mpp.12230
Cai, Z., Yuan, Z. H., Zhang, H., Pan, Y., Wu, Y., Tian, X. Q., et al. (2017). Fatty acid DSF binds and allosterically activates histidine kinase RpfC of phytopathogenic bacterium Xanthomonas campestris pv. campestris to regulate quorum-sensing and virulence. PLoS Pathog. 13:e1006304. doi: 10.1371/journal.ppat.1006304
Chin, K. H., Lee, Y. C., Tu, Z. L., Chen, C. H., Tseng, Y. H., Yang, J. M., et al. (2010). The cAMP receptor-like protein CLP is a novel c-di-GMP receptor linking cell-cell signaling to virulence gene expression in Xanthomonas campestris. J. Mol. Biol. 396, 646–662. doi: 10.1016/j.jmb.2009.11.076
Chou, S. H., and Galperin, M. Y. (2016). Diversity of cyclic di-GMP-binding proteins and mechanisms. J. Bacteriol. 198, 32–46. doi: 10.1128/JB.00333-15
Das, A., Rangaraj, N., and Sonti, R. V. (2009). Multiple adhesin-like functions of Xanthomonas oryzae pv. oryzae are involved in promoting leaf attachment, entry, and virulence on rice. Mol. Plant Microbe Interact. 22, 73–85. doi: 10.1094/MPMI-22-1-0073
Dow, J. M., Fouhy, Y., Lucey, J. F., and Ryan, R. P. (2006). The HD-GYP domain, cyclic di-GMP signaling, and bacterial virulence to plants. Mol. Plant Microbe Interact. 19, 1378–1384. doi: 10.1094/MPMI-19-1378
Dow, M. (2008). Diversification of the function of cell-to-cell signaling in regulation of virulence within plant pathogenic Xanthomonads. Sci. Signal. 1:23. doi: 10.1126/stke.121pe23
Girvan, H, and, M., and Munro, A. W. (2013). Heme sensor proteins. J. Biol. Chem. 288, 13194–13203. doi: 10.1074/jbc.R112.422642
Guan, W. J., Wu, M. S., and He, C. Y. (2009). Molecular identification and functional analysis of Clpxoo, a homologue to the nucleotide receptor protein in Xanthomonas oryzae pv. oryzae. Acta Microbiol. Sin. 49, 32–37. doi: 10.13343/j.cnki.wsxb.2009.01.013
Guo, J., Li, H., Chang, J. W., Lei, Y., Li, S., and Chen, L. L. (2013). Prediction and characterization of protein-protein interaction network in Xanthomonas oryzae pv. oryzae PXO99A. Res. Microbiol. 164, 1035–1044. doi: 10.1016/j.resmic.2013.09.001
Guzzo, C. R., Dunger, G., Salinas, R. K., and Farah, C. S. (2013). Structure of the PilZFimXEALc-di-GMP complex responsible for the regulation of bacterial type IV pilus biogenesis. J. Mol. Biol. 425, 2174–2197. doi: 10.1016/j.jmb.2013.03.021
Guzzo, C. R., Salinas, R. K., Andrade, M. O., and Farah, C. S. (2009). PILZ protein structure and interactions with PILB and the FIMX EAL domain: implications for control of type IV pilus biogenesis. J. Mol. Biol. 393, 848–866. doi: 10.1016/j.jmb.2009.07.065
He, Y. W., Boon, C., Zhou, L., and Zhang, L. H. (2009). Co-regulation of Xanthomonas campestris virulence by quorum sensing and a novel two-component regulatory system RavS/RavR. Mol. Microbiol. 71, 1464–1476. doi: 10.1111/j.1365-2958.2009.06617.x
He, Y. W., Ng, A. Y., Xu, M., Lin, K., Wang, L. H., Dong, Y. H., et al. (2007). Xanthomonas campestris cell-cell communication involves a putative nucleotide receptor protein Clp and a hierarchical signaling network. Mol. Microbiol. 64, 281–292. doi: 10.1111/j.1365-2958.2007.05670.x
He, Y. W., Wu, J, Cha, J. S., and Zhang, L. H. (2010). Rice bacterial blight pathogen Xanthomonas oryzae pv. oryzae produces multiple DSF-family signals in regulation of virulence factor production. BMC Microbiol. 10:187. doi: 10.1186/1471-2180-10-187
Hengge, R. (2009). Principles of c-di-GMP signalling in bacteria. Nat. Rev. Microbiol. 7, 263–273. doi: 10.1038/nrmicro2109
Hengge, R. (2013). Novel tricks played by the second messenger c-di-GMP in bacterial biofilm formation. EMBO J. 32, 322–323. doi: 10.1038/emboj.2012.351
Huang, B., Whitchurch, C. B., and Mattick, J. S. (2003). FimX, a multidomain protein connecting environmental signals to twitching motility in Pseudomonas aeruginosa. J. Bacteriol. 185, 7068–7076. doi: 10.1128/JB.185.24.7068-7076.2003
Hunter, J. L., Severin, G. B., Koestler, B. J., and Waters, C. M. (2014). The Vibrio cholerae diguanylate cyclase VCA0965 has an AGDEF active site and synthesizes cyclic di-GMP. BMC Microbiol. 14:22. doi: 10.1186/1471-2180-14-22
Jenal, U., Reinders, A., and Lori, C. (2017). Cyclic di-GMP: second messenger extraordinaire. Nat. Rev. Microbiol. 15, 271–284. doi: 10.1038/nrmicro.2016.190
Jha, G., Rajeshwari, R., and Sonti, R. V. (2007). Functional interplay between two Xanthomonas oryzae pv,. oryzae secretion systems in modulating virulence on rice. Mol. Plant Microbe Interact. 20, 31–40. doi: 10.1094/MPMI-20-0031
Jiang, B. L., Jiang, G. F., Liu, W., Yang, L. C., Yang, L. Y., Wang, L., et al. (2018). RpfC regulates the expression of the key regulator hrpX of the hrp/T3SS system in Xanthomonas campestris pv. campestris. BMC Microbiol. 18:103. doi: 10.1186/s12866-018-1233-5
Kazmierczak, B. I., Lebron, M. B., and Murray, T. S. (2006). Analysis of FimX, a phosphodiesterase that governs twitching motility in Pseudomonas aeruginosa. Mol. Microbiol. 60, 1026–1043. doi: 10.1111/j.1365-2958.2006.05156.x
Kim, S., Cho, Y. J., Song, E. S., Lee, S. H., Kim, J. G., and Kang, L. W. (2016). Time-resolved pathogenic gene expression analysis of the plant pathogen Xanthomonas oryzae pv. oryzae. BMC Genom. 17:345. doi: 10.1186/s12864-016-2657-7
Kulshina, N., Baird, N. J., and Ferre-D'Amare, A. R. (2009). Recognition of the bacterial second messenger cyclic diguanylate by its cognate riboswitch. Nat. Struct. Mol. Biol. 16, 1212–1217. doi: 10.1038/nsmb.1701
Leduc, J. L., and Roberts, G. P. (2009). Cyclic di-GMP allosterically inhibits the CRP-like protein (Clp) of Xanthomonas axonopodis pv. citri. J. Bacteriol. 191, 7121–7122. doi: 10.1128/JB.00845-09
Li, B., Tian, F., Chen, H. M., Wu, M. S., and He, C. Y. (2013). Binding of transcription activator Clpxoo to promoter of endoglucanase gene engAxoo was inhibited by c-di-GMP in Xanthomonas oryzae pv. oryzae. Acta Microbiol. Sinica 53, 1116–1171. doi: 10.13343/j.cnki.wsxb.2013.11.002
Li, H. Y., Xue, D. R., Tian, F., Yuan, X. C., Yang, F. H., Chen, H. M., et al. (2018). Xanthomonas oryzae pv. oryzae response regulator TriP regulates virulence and exopolysaccharide production via interacting with c-di-GMP phosphodiesterase PdeR. Mol. Plant Microbe Interact. 32, 729–739. doi: 10.1094/MPMI-09-18-0260-R
Li, J. Y., Li, B., Chen, H. M., Yang, F. H., He, C. Y., and Tian, F. (2016). Identification of critical residues in c-di-GMP receptor Clpxoo from Xanthomonas oryzae pv. oryzae. Biotechnol. Bull. 32, 124–129. doi: 10.13560/j.cnki.biotech.bull.1985.2016.12.020
Li, X. T., Yang, F. H., Liang, S. M., Tian, F., Chen, H. M., and He, C. Y. (2014). Molecular characterization of PXO_02944, a negative regulator in virulence, extracellular polysaccharide production and biofilm formation in Xanthomonas oryzae pv. oryzae. Sci. Agricult. Sin. 47, 2563–2570. doi: 10.3864/j.issn.0578-1752.2014.13.008
Liang, S. M., Yang, F. H., Guan, W. J., Wu, M. S., Chen, H. M., Tian, F., et al. (2011). Gene deletion and functional analysis of the EAL domain protein VieAxoo in Xanthomonas oryzae pv. oryzae. Acta Microbiol. Sin. 51, 29–34. doi: 10.13343/j.cnki.wsxb.2011.01.009
McCarthy, Y., Ryan, R. P., O'Donovan, K., He, Y. Q., Jiang, B. L., Feng, J. X., et al. (2008). The role of PilZ domain proteins in the virulence of Xanthomonas campestris pv. campestris. Mol. Plant Pathol. 9, 819–824. doi: 10.1111/j.1364-3703.2008.00495
Mew, T. W., Alvarez, A. M., Leach, J. E., and Swings, J. (1993). Focus on bacterial blight of rice. Plant Dis 77, 5–12.
Navarro, M. V., De, N., Bae, N., Wang, Q., and Sondermann, H. (2009). Structural analysis of the GGDEFEAL domain-containing c-di-GMP receptor FimX. Structure 17, 1104–1116. doi: 10.1016/j.str.2009.06.010
Niño-Liu, D. O., Ronald, P. C., and Bogdanove, A. J. (2006). Xanthomonas oryzae pathovars: model pathogens of a model crop. Mol. Plant Pathol. 7, 303–324. doi: 10.1111/j.1364-3703.2006.00344.x
O'Connell, A., An, S. Q., McCarthy, Y., Schulte, F., Niehaus, K., He, Y. Q., et al. (2013). Proteomics analysis of the regulatory role of Rpf/DSF cell-to-cell signaling system in the virulence of Xanthomonas campestris. Mol. Plant Microbe Interact. 26, 1131–1137. doi: 10.1094/MPMI-05-13-0155-R
Pérez-Mendoza, D., Coulthurst, S. J., Humphris, S., Campbell, E., Welch, M., Toth, I. K., et al. (2011). A multi-repeat adhesin of the phytopathogen, Pectobacterium atrosepticum, is secreted by a Type I pathway and is subject to complex regulation involving a non-canonical diguanylate cyclase. Mol. Microbiol. 82, 719–733. doi: 10.1111/j.1365-2958.2011.07849.x
Petters, T., Zhang, X., Nesper, J., Treuner-Lange, A., Gomez-Santos, N., Hoppert, M., et al. (2012). The orphan histidine protein kinase SgmT is a c-di-GMP receptor and regulates composition of the extracellular matrix together with the orphan DNA binding response regulator DigR in Myxococcus xanthus. Mol. Microbiol. 84, 147–165. doi: 10.1111/j.1365-2958.2012.08015.x
Qian, S. S., Yang, F. H., Tian, F., Chen, H. M., and He, C. Y. (2017). Functional identification of the HD-GYP domain protein PXO_03945 in Xanthomonas oryzae pv. oryzae. Acta Phytopathol. Sin. 47, 605–611. doi: 10.13926/j.cnki.apps.000114
Qian, W., Han, Z. J., Tao, J., and He, C. (2008). Genome-scale mutagenesis and phenotypic characterization of two-component signal transduction systems in Xanthomonas campestris pv. campestris ATCC 33913. Mol. Plant Microbe Interact. 21, 1128–1138. doi: 10.1094/MPMI-21-8-1128
Ray, S. K., Rajeshwari, R., and Sonti, R. V. (2000). Mutants of Xanthomonas oryzae pv. oryzae deficient in general secretory pathway are virulence deficient and unable to secrete xylanase. Mol. Plant Microbe. Interact. 13, 394–401. doi: 10.1094/MPMI.2000.13.4.394
Roelofs, K. G., Jones, C. J., Helman, S. R., Shang, X., Orr, M. W., Goodson, J. R., et al. (2015). Systematic identification of cyclic-di-GMP binding proteins in Vibrio cholerae reveals a novel class of cyclic-di-GMP-binding ATPases associated with type II secretion systems. PLoS Pathog 11:e1005232. doi: 10.1371/journal.ppat.1005232
Römling, U., Galperi, M. Y., and Gomelsky, M. (2013). Cyclic di-GMP: the first 25 years of a universal bacterial second messenger. Microbiol. Mol. Biol. Rev. 77, 1–52. doi: 10.1128/MMBR.00043-12
Römling, U., and Galperin, M. Y. (2017). Discovery of the second messenger cyclic di-GMP. Methods Mol. Biol. 1657, 1–8. doi: 10.1007/978-1-4939-7240-1_1
Römling, U., Liang, Z. X., and Dow, J. M. (2017). Progress in understanding the Molecular basis underlying functional diversification of cyclic dinucleotide turnover proteins. J. Bacteriol. 199, e00790–e00716. doi: 10.1128/JB.00790-16
Ryan, R. P., An, S. Q, Allan, J. H., McCarthy, Y., and Dow, J. M. (2015). The DSF family of cell–cell signals: an expanding class of bacterial virulence regulators. PLoS Pathog. 11:e1004986. doi: 10.1371/journal.ppat.1004986
Ryan, R. P., and Dow, J. M. (2010). Intermolecular interactions between HD-GYP and GGDEF domain proteins mediate virulence-related signal transduction in Xanthomonas campestris. Virulence 1, 404–408. doi: 10.4161/viru.1.5.12704
Salzberg, S. L., Sommer, D. D., Schatz, M. C., Phillippy, A. M., Rabinowicz, P. D., Tsuge, S., et al. (2008). Genome sequence and rapid evolution of the rice pathogen Xanthomonas oryzae pv. oryzae PXO99A. BMC Genomics 9:204. doi: 10.1186/1471-2164-9-204
Schirmer, T. (2016). C-di-GMP synthesis: structural aspects of evolution, catalysis and regulation. J. Mol. Biol. 428, 3683–3701. doi: 10.1016/j.jmb.2016.07.023
Schirmer, T., and Jenal, U. (2009). Structural and mechanistic determinants of c-di-GMP signalling. Nat. Rev. Microbiol. 7, 724–735. doi: 10.1038/nrmicro2203
Shiue, S. J., Kao, K. M., Leu, W. M., Chen, L. Y., Chan, N. L., and Hu, N. T. (2006). XpsE oligomerization triggered by ATP binding, not hydrolysis, leads to its association with XpsL. EMBO J. 25, 1426–1435. doi: 10.1038/sj.emboj.7601036
Slater, H., Alvarez-Morales, A., Barber, C. E., Daniels, M. J., and Dow, J. M. (2000). A two-component system involving an HD-GYP domain protein links cell-cell signaling to pathogenicity gene expression in Xanthomonas campestris. Mol. Microbiol. 38, 986–1003. doi: 10.1046/j.1365-2958.2000.02196.x
Su, J., Zou, X., Huang, L., Bai, T., Liu, S., Yuan, M., et al. (2016). DgcA, a diguanylate cyclase from Xanthomonas oryzae pv. oryzae regulates bacterial pathogenicity on rice. Sci. Rep. 6:25978. doi: 10.1038/srep25978
Sun, L., Wu, M. S., Chen, H. M., and He, C. Y. (2010). Diffusible signal factor production and virulence expression in ΔrpfFxoo, ΔrpfGxoo and ΔrpfCxoo, the gene deletion mutants of DSF/Rpf signaling proteins of Xanthomonas oryzae pv. oryzae. Acta Microbiol. Sin. 50, 717–723. doi: 10.13343/j.cnki.wsxb.2010.06.015
Tang, Q., Yin, K., Qian, H., Zhao, Y., Wang, W., Chou, S. H., et al. (2016). Cyclic di-GMP contributes to adaption and virulence of Bacillus thuringiensis through a riboswitch-regulated collagen adhesion protein. Sci. Rep. 6:28807. doi: 10.1038/srep28807
Tao, F., He, Y. W., Wu, D. H., Swarup, S., and Zhang, L. H. (2010). The cyclic nucleotide monophosphate domain of Xanthomonas campestris global regulator Clp defines a new class of cyclic di-GMP effectors. J. Bacteriol. 192, 1020–1029. doi: 10.1128/JB.01253-09
Tian, F., Yu, C., Li, H., Wu, X., Li, B., Chen, H., et al. (2015). Alternative sigma factor RpoN2 is required for flagellar motility and full virulence of Xanthomonas oryzae pv. oryzae. Microbiol. Res. 170, 177–183. doi: 10.1016/j.micres.2014.07.002
Trampari, E., Stevenson, C. E., Little, R. H., Wilhelm, T., Lawson, D. M., and Malone, J. G. (2015). Bacterial rotary export ATPases are allosterically regulated by the nucleotide second messenger cyclic-di-GMP. J. Biol. Chem. 290, 24470–24483. doi: 10.1074/jbc.M115.661439
Tsuge, S., Furutani, A., and Ikawa, Y. (2014). Regulatory network of hrp gene expression in Xanthomonas oryzae pv. oryzae. J. Gen. Plant Pathol. 80, 303–313. doi: 10.1007/s10327-014-0525-3
Tsuge, S., Nakayama, T., Terashima, S., Ochiai, H., Furutani, A., Oku, T., et al. (2006). Gene involved in transcriptional activation of the hrp regulatory gene hrpG in Xanthomonas oryzae pv. oryzae. J. Bacteriol. 188, 4158–4162. doi: 10.1128/JB.00006-06
Wang, F. F., Cheng, S. T., Wu, Y., Ren, B. Z., and Qian, W. (2017). A bacterial receptor PcrK senses the plant hormone cytokinin to promote adaptation to oxidative stress. Cell Rep. 21, 2940–2951. doi: 10.1016/j.celrep.2017.11.017
Wang, X. Y., Zhou, L., Yang, J., Ji, G. H., and He, Y. W. (2016). The RpfB-dependent quorum sensing signal turnover system Is required for adaptation and virulence in rice bacterial blight pathogen Xanthomonas oryzae pv. oryzae. Mol. Plant Microbe Interact. 29, 220–230. doi: 10.1094/MPMI-09-15-0206-R
Wang, Y. C., Chin, K. H., Chuah, M. L., Liang, Z. X., and Chou, S. H. (2012). Crystallization and preliminary X-ray diffraction studies of Xanthomonas campestris PNPase in the presence of c-di-GMP. Acta Crystallogr. Sect. F Struct Biol Cryst. Commun. 68, 1247–1250. doi: 10.1107/S1744309112036202
Wang, Y. C., Chin, K. H., Tu, Z. L., He, J., Jones, C. J., Sanchez, D. Z., et al. (2016). Nucleotide binding by the widespread high-affinity cyclic di-GMP receptor MshEN domain. Nat. Commun. 7:12481. doi: 10.1038/ncomms12481
Weber, I. T., and Steitz, T. A. (1987). Structure of a complex of catabolite gene activator protein and cyclic AMP refined at 2.5 Å resolution. J. Mol. Biol. 198, 311–326. doi: 10.1016/0022-2836(87)90315-9
Wei, C., Jiang, W., Zhao, M., Ling, J., Zeng, X., Deng, J., et al. (2016). A systematic analysis of the role of GGDEFEAL domain proteins in virulence and motility in Xanthomonas oryzae pv. oryzicola. Sci. Rep. 6:23769. doi: 10.1038/srep23769
Weinberg, Z., Nelson, J. W., Lunse, C. E., Sherlock, M. E., and Breaker, R. R. (2017). Bioinformatic analysis of riboswitch structures uncovers variant classes with altered ligand specificity. Proc. Natl. Acad. Sci. U.S.A. 114, E2077–E2085. doi: 10.1073/pnas.1619581114
White, F. F., and Yang, B. (2009). Host and pathogen factors controlling the rice-Xanthomonas oryzae interaction. Plant Physiol. 150, 1677–1686. doi: 10.1104/pp.109.139360
Xu, G., Han, S., Huo, C., Chin, K. H., Chou, S. H., Gomelsky, M., et al. (2018). Signaling specificity in the c-di-GMP-dependent network regulating antibiotic synthesis in Lysobacter. Nucleic Acids Res. 46, 9276–9288. doi: 10.1093/nar/gky803
Xue, D. R., Tian, F., Li, H. Y., Yang, F. H., Chen, H. M., and He, C. Y. (2015). Prediction and analysis of genes encoding c-di-GMP-metabolizing enzymes in Xanthomonas oryzae pv. oryzae. Biotechnol. Bull. 31, 131–138. doi: 10.13560/j.cnki.biotech.bull.1985.2015.11.016
Xue, D. R., Tian, F., Yang, F. H., Chen, H. M., Yuan, X. C., Yang, C. H., et al. (2018). Phosphodiesterase EdpX1 promotes virulence, exopolysaccharide production and biofilm formation in Xanthomonas oryzae pv. oryzae. Appl. Environ. Microbiol. 84, e01717–e01718. doi: 10.1128/AEM.01717-18
Yang, F. H., Qian, S. S., Tian, F., Chen, H. M., Hutchins, W., Yang, C.-H., et al. (2016). The GGDEFdomain protein GdpX1 attenuates motility, exopolysaccharide production, and virulence in Xanthomonas oryzae pv. oryzae. J. Appl. Microbiol. 120,1646–1657. doi: 10.1111/jam.13115
Yang, F. H., Tian, F., Chen, H. M., and He, C. Y. (2017). Cyclic di-GMP signal receptor-mediated regulation of bacterial behaviors. Plant Protect. 1, 9–14. doi: 10.3969/j.issn.0529-1542.2017.01.002
Yang, F. H., Tian, F., Chen, H. M., Hutchins, W., Yang, C.-H., and He, C. Y. (2015). The Xanthomonas oryzae pv. oryzae PilZ domain proteins function differentially in cyclic di-GMP binding, and regulation of virulence and motility. Appl. Env. Microbiol. 81, 1–8. doi: 10.1128/AEM.04044-14
Yang, F. H., Tian, F., Li, X. T., Fan, S. S., Chen, H. M., Wu, M. S., et al. (2014). The degenerate EAL-GGDEF domain protein Filp functions as a cyclic di-GMP receptor and specifically interacts with the PilZ domain protein PXO_02715 to regulate virulence in Xanthomonas oryzae pv. oryzae. Mol. Plant-Microbe Interact. 27, 578–589. doi: 10.1094/MPMI-12-13-0371-R
Yang, F. H., Tian, F., Sun, L., Chen, H. M., Wu, M., Yang, C.-H., et al. (2012). A novel two-component system PdeK/PdeR regulates c-di-GMP turnover and virulence of Xanthomonas oryzae pv. oryzae. Mol. Plant Microbe Interact. 25, 1361–1369. doi: 10.1094/MPMI-01-12-0014-R
Zhang, F., Du, Z., Huang, L., Vera Cruz, C., Zhou, Y., and Li, Z. (2013). Comparative transcriptome profiling reveals different expression patterns in Xanthomonas oryzae pv. oryzae strains with putative virulence-relevant genes. PLoS ONE 8:e64267. doi: 10.1371/journal.pone.0064267
Zhang, Y., Wei, C., Jiang, W., Wang, L., Li, C., Wang, Y., et al. (2013). The HD-GYP domain protein RpfG of Xanthomonas oryzae pv. oryzicola regulates synthesis of extracellular polysaccharides that contribute to biofilm formation and virulence on rice. PLoS ONE 8:e59428. doi: 10.1371/journal.pone.0059428
Zhao, Z., Wu, Z., and Zhang, J. (2016). Crystal structure of the YajQ-family protein XC_3703 from Xanthomonas campestris pv. campestris. Acta Crystallogr. F Struct. Biol. Commun. 72, 720–725. doi: 10.1107/S2053230X16013017
Zheng, D., Constantinidou, C., Hobman, J. L., and Minchin, S. D. (2004). Identification of the CRP regulon using in vitro and in vivo transcriptional profiling. Nucleic Acids Res. 32, 5874–5893. doi: 10.1093/nar/gkh908
Zhou, H., Zheng, C., Su, J., Chen, B., Fu, Y., Xie, Y., et al. (2016). Characterization of a natural triple-tandem c-di-GMP riboswitch and application of the riboswitch-based dual-fluorescence reporter. Sci. Rep. 6:20871.doi: 10.1038/srep20871
Keywords: c-di-GMP signaling, regulation, virulence, pathogenesis, Xanthomonads
Citation: Yang F, Xue D, Tian F, Hutchins W, Yang C-H and He C (2019) Identification of c-di-GMP Signaling Components in Xanthomonas oryzae and Their Orthologs in Xanthomonads Involved in Regulation of Bacterial Virulence Expression. Front. Microbiol. 10:1402. doi: 10.3389/fmicb.2019.01402
Received: 27 December 2018; Accepted: 04 June 2019;
Published: 11 July 2019.
Edited by:
Daniel Perez-Mendoza, Experimental Station of Zaidín (EEZ), SpainReviewed by:
Max Dow, University College Cork, IrelandGeorge W. Sundin, Michigan State University, United States
Copyright © 2019 Yang, Xue, Tian, Hutchins, Yang and He. This is an open-access article distributed under the terms of the Creative Commons Attribution License (CC BY). The use, distribution or reproduction in other forums is permitted, provided the original author(s) and the copyright owner(s) are credited and that the original publication in this journal is cited, in accordance with accepted academic practice. No use, distribution or reproduction is permitted which does not comply with these terms.
*Correspondence: Chenyang He, aGVjaGVueWFuZ0BjYWFzLmNu
†These authors have contributed equally to this work