- 1Department of Pharmacy, University of Salerno, Fisciano, Italy
- 2Department of Chemical, Biological, Pharmaceutical and Environmental Sciences, University of Messina, Messina, Italy
- 3Department for the Earth, Environment and Life Sciences, School of Mathematical, Physical and Natural Sciences, University of Genoa, Genoa, Italy
- 4Essentially Australia, Byron Bay, NSW, Australia
- 5European Herbal and Traditional Medicine Practitioners Association, Norwich, United Kingdom
- 6Institute of Food Sciences, CNR-ISA, Italian National Research Council, Avellino, Italy
The aim of this study was to characterize the chemical composition and to evaluate the antimicrobial and phytotoxic properties of the essential oils (EOs) obtained from leaves of Leptospermum petersonii chemotype “Variety B” and Eucalyptus gunnii, native to Australia. Geranyl acetate, γ-terpinene, geraniol, terpinolene, α-pinene, p-cimene, and linalool were the main components in L. petersonii EO, confirming also the existence of several chemotypes in such taxa; on the other hand, 1,8-cineole, trans-sabinene hydrate acetate, globulol, longicyclene, terpinolene, and camphene were present in major amounts in the E. gunnii EO. Chemical analysis of L. petersonii revealed that it belongs to the variety “B.” E. gunnii EO showed good antibacterial activity, with an MIC of 0.5 and 2 μg/mL against Staphylococcus aureus, and Pectobacterium carotovorum, respectively. The activity of E. gunnii EO was stronger than L. petersonii EO, whose maximum MIC reached 5 μg/mL. E. gunnii and L. petersonii EOs were particularly effective in inhibiting the biofilm formation by S. aureus, already at a concentration of 0.01 μg/mL. The other strains were resistant to both EOs up to a dose of 0.05 μg/mL. The maximum inhibition on biofilm formed by P. carotovorum was recorded for E. gunnii EO, reaching a value of 93.12% at 1.0 μg/mL. This is the first manuscript which studies the biofilm inhibition by EOs and evaluates their effects on biofilm metabolism. Both EOs were more effective against P. carotovorum. In addition, even though L. petersonii EO 0.1 μg/mL was unable to inhibit biofilm formation by Escherichia coli, it decreased the metabolic activity of the biofilm to 78.55% compared to control; furthermore, despite it inducing a relatively low inhibition (66.67%) on biofilm formation, it markedly affected metabolic activity, which decreased to 16.09% with respect to the control. On the contrary, L. petersonii EO 0.5 μg/mL induced a 79.88% inhibition of S. aureus biofilm, maintaining a high metabolic activity (90.89%) compared to the control. Moreover, this EO showed inhibitory activity against radical elongation of Solanum lycopersicum and the germination of radish. On the contrary, E. gunnii EO showed no phytotoxic activity.
Introduction
Leptospermum petersonii F.M. Bailey and Eucalyptus gunnii Hook.f. belong to the Myrtaceae family. This plant family comprises of at least 3000 species widely distributed in several tropical and warm-temperate areas, such as Australia and Central and South America (Mabberley, 1997). Many EOs produced by the Myrtaceae species have been reported for their insecticidal, nematicidal, anti-inflammatory, and antifungal activities and are used as antimicrobial agents in cosmetic products (Lis-Balchin et al., 2000; Lee et al., 2004; Park et al., 2011).
Leptospermum petersonii, commonly known as Australian Rose (lemon-scented tea-tree), is a rare little tree, naturally occurring in lowland or floodplain areas in Northern New South Wales. Previous literature proposed the existence of three chemical varieties of this species, based on the composition of its EO (Brophy et al., 2000). At present, there is no knowledge of indigenous uses of L. petersonii. However, other species of the same genus are known to be used in traditional medicine, such as Leptospermum flavescens Sm., used in Malaysia as a way to relieve stomach disorders and menstrual disorders (Riley, 1994), and the New Zealand species Leptospermum scoparium J. R. Forst. and G. Forst., used by Maori as a food and as a traditional remedy to alleviate coughs (Brooker et al., 1987; Crowe, 1997). The odor of common L. petersonii EO is described as “extremely pleasant and lemony” (Nuadha, 2011). L. petersonii (FM. Bailey) EO showed insecticidal activity against the diamond back moth Plutella xylostella (L.), reducing the feeding and development of larval stages on broccoli leaves and oviposition in adult stage. In light of this, it could be used as an alternative insecticidal strategy, leading to the development of biodegradable and non-toxic products (Purwatiningsih et al., 2012). Several studies reported the antifungal activity of the EO of L. petersonii on Candida albicans and several Aspergillus species (Hood et al., 2010; Kim and Park, 2012) and its antibacterial efficacy (Lis-Balchin et al., 1996; Demuner et al., 2011; Bugarin et al., 2014; Van Vuuren et al., 2014).
Eucalyptus gunnii Hook. F. (cinder gum) is a medium-sized tree, endemic to Tasmania (Brooker and Kleinig, 1996), and widely cultivated in France, the United Kingdom, Ireland, and Italy, mainly for the market of cut foliage (Forrest, 2002). Although Eucalyptus EO has a widely ascertained spectrum of biological activities including anti-microbial, fungicidal, insecticidal/insect repellent, herbicidal, acaricidal, and nematicidal (Batish et al., 2008), few papers have reported the chemical composition or properties of E. gunnii EO (Lucia et al., 2008; Elaissi et al., 2010; Bugarin et al., 2014). It has a weak anti-inflammatory effect (Dhakad et al., 2018) and major antibacterial properties against Pseudomonas aeruginosa when compared to EOs from other species of Eucalyptus genus (Elaissi et al., 2010; Salehi et al., 2019). To date, the phytotoxic activity, as well as the anti-biofilm properties, of these two EOs against several bacterial strains such as Staphylococcus aureus, P. aeruginosa, Listeria monocytogenes, and Pectobacterium carotovorum have not been investigated.
The persistent use of synthetic herbicides may impede sustainable agricultural production, causing ecological and environmental concerns such as increased resistance of weeds, environmental pollution, and health hazards (Rolim de Almeida et al., 2010). EOs are valuable sources of lead molecules in agriculture for weed and pest management. It is thus pertinent to explore and characterize the phytotoxic properties of aromatic plants.
In addition, biofilm formation and bacterial antibiotic resistance constitute problems of particular bearing for human health. For this reason, research aimed at identifying new antimicrobial compounds from natural sources such as medicinal plants are very topical.
Biofilm formation by S. aureus and P. aeruginosa is important when studying infections of the upper airways, because it perpetuates antigen presentation, leading to chronic inflammation and complicating clinical treatments (Camporese, 2013). Similar problems are caused by the uropathogenic Escherichia coli, whose biofilms are difficult to eradicate from the surface of hospital catheters (Bernal-Mercado et al., 2019), and L. monocytogenes, which form biofilms on food products (Djordjevic et al., 2002). Finally, P. carotovorum biofilm causes soft rot in food crops due the release of exoenzymes and increases bacterial resistance during plant disinfection (Gutierrez-Pacheco et al., 2018). P. carotovorum subsp. brasiliense was reported to form biofilm-like aggregates inside xylem vessels of tomato and potato plants (Kubheka et al., 2013). From this point of view, several EOs have already been tested (Hosseini Nezhad et al., 2012). Biofilm cells profoundly differ from planktonic cells of the same species because of phenotypic and metabolic changes that regulate some cell events, such as sporulation, starvation survival, adhesion, rough-smooth phase variations, etc. This also led to differences in the susceptibility of planktonic and biofilm cells to antimicrobial agents because adhesion-dependent phenotypic changes may be important as diffusion barriers or as growth rate-dependent changes in this important phenomenon. The metabolic changes can also make these cells inherently resistant to a particular antibiotic agent, virtually developed against specific targets in planktonic cells.
The present study was carried out to characterize the micromorphological features of L. petersonii and E. gunnii leaves by scanning electron microscopy (SEM) and to investigate the chemical composition of their EOs, as well as their antimicrobial and phytotoxic activities. The antibacterial activity was evaluated against some Gram-positive (S. aureus and L. monocytogenes) and Gram-negative (E. coli and P. aeruginosa) pathogenic strains and against the phytopathogen, P. carotovorum. The potential in vitro phytotoxicity was evaluated against germination and radical elongation of Raphanus sativus L. (radish), Lactuca sativa L.(lettuce), Lepidium sativum L. (garden cress), Solanum lycopersicum L. (tomato), Lolium multiflorum Lam. (Italian ryegrass), and Portulaca oleracea L.(purslane) seeds.
Materials and Methods
Plant Material
Branches with leaves of L. petersonii, belonging to the chemical variety “B CT Australian Rose,” were obtained from plantations situated in the Byron Bay hinterland, Northern New South Wales, Australia. Branches bearing young rounded leaves of E. gunnii were collected from pruning material of trees growing on a private land in Sardinia (Italy). Taxonomic identification of plant samples was carried out by MV for E. gunnii and GT for L. petersonii.
Chemicals and Reagents
Ethanol-FineFix working solution was obtained from Milestone s.r.l., Bergamo, Italy. The bacterial culture medium, PBS, DMSO, tetracycline, ciprofloxacin, and MTT were supplied by Sigma, Milano, Italy. All other reagents were of analytical grade.
Scanning Electron Microscopy
Samples of leaves (1–1.5 cm2) were fixed in a 70% ethanol-FineFix working solution (Milestone s.r.l., Bergamo, Italy) for 24 h at 4°C, and then gradually dehydrated by ethanol series (Chieco et al., 2012). Subsequently, samples were processed in a critical point dryer (CPD processor, K850 2M Strumenti s.r.l., Rome, Italy), mounted on aluminum stubs using glued carbon tabs, and sputter-coated with gold. Specimens were analyzed and photographed using an FE-SEM (Supra VP-40; Zeiss, Oberkochen, Germany) at an accelerating voltage of 20 kV.
Isolation and Analysis of Essential Oils
Isolation of Essential Oils
Fresh plant materials were subjected to steam distillation until no significant increase in the volume of the collected EO was observed (3 h).
The EO yield (w/v, %) was calculated according to the following equation:
where W0 is the plant material weight distillated and VEO is the EO volume obtained.
Essential oils were dried on Na2SO4 and stored in a dark-sealed vial with nitrogen headspace until analysis.
GC-FID Analysis
Analytical GC was performed on a Perkin-Elmer Sigma-115 gas chromatograph equipped with an FID and a data handling processor. The separation was achieved using a HP-5 MS fused-silica capillary column (30 m × 0.25 mm i.d., 0.25 μm film thickness). Column temperature: 40°C, with 5 min initial hold, and then to 270°C at 2°C/min, 270°C (20 min); injection mode splitless (1 μL of a 1:1000 n-hexane solution). Injector and detector temperatures were 250 and 290°C, respectively. Analysis was also run by using a fused silica HP Innowax polyethylene glycol capillary column (50 m × 0.20 mm i.d., 0.25 μm film thickness). In both cases, helium was used as a carrier gas (1.0 mL/min).
GC/MS Analysis and Identification of Single Constituents
Analyses were performed on an Agilent 6850 Ser. II apparatus, fitted with a fused silica DB-5 capillary column (30 m × 0.25 mm i.d., 0.33 μm film thickness), coupled to an Agilent Mass Selective Detector MSD 5973, ionization energy voltage 70 eV, electron multiplier voltage energy 2000 V. Mass spectra were acquired in the range 40–500 amu, scan time 5 scans/s. Gas chromatographic conditions were as reported above, with a transfer line temperature of 295°C. Most constituents were identified by comparison of their Kovats retention indices (Ri) [calculated in relation to retention time of n-alkanes (C10–C35)], with either those of the literature (Jennings and Shibamoto, 1980; Davies, 1990; Adams, 2007; Goodner, 2008), by accurate analysis of mass spectra on both columns and by their comparison with those of authentic compounds available in our laboratories by means of NIST 02 and Wiley 275 libraries (Wiley, 1998). The components’ relative concentrations were obtained by peak area normalization.
Antibacterial Activity
Microorganisms and Culture Conditions
Listeria monocytogenes (ATCC 7644) and P. aeruginosa (ATCC 50071) were purchased from American Type Culture Collection (ATCC), MD, United States. S. aureus, E. coli (DSM 8579), and Pectobacterium carovotorum (DSM 102074) were purchased from Deutsche Sammlung von Mikroorganismen (DSM) collection, Braunschweig, Germany.
Bacteria were grown in Luria–Bertani (LB) broth (Sigma, Milan, Italy) for 18 h at 37°C and 80 r/min (Corning LSE, Pisa, Italy). P. carovotorum was grown at 28°C and 80 r/min.
Determination of the Antibacterial Susceptibility by Agar Diffusion Assay
Different concentrations of Eos, ranging from 0.01 to 2.0 μg/mL, diluted in sterile DMSO (Sigma–Aldrich Italy, Milan, Italy) were spotted onto the plates previously inoculated with 0.5 McFarland (1.5 × 107 cells/mL) bacterial suspension (Densitometer cell density turbidity 0.3–15.0 McFarland, CAMLAB, Cambridge, United Kingdom) (Fratianni et al., 2016). After 10 min in sterile conditions, the plates were incubated for 24 h at 37 or 28°C, depending on the strain. The diameter of the clear zone shown on plates (inhibition zone) was accurately measured by using an Extra steel Caliper mod 0289, mm/inch reading scale, precision 0.05 mm (Mario De Maio, Milan, Italy). Sterile 1% DMSO and tetracycline (7 μg/mL, Sigma–Aldrich Italy, Milan, Italy) were used as negative and positive controls, respectively. The experiments were performed in triplicate and results expressed as mean ± SD.
Minimal Inhibitory Concentration (MIC)
The MIC values were calculated through the application of the resazurin microtiter-plate assay (Sarker et al., 2007). The EOs were dissolved in sterile DMSO. Twofold serial dilutions were prepared to obtain 50 μL of the EOs in serially descending concentrations in each well. Thirty-five μL of 3.3 × strength iso-sensitized broth and 5 μL of resazurin, used as indicator solution, were added to reach a final volume/well of 240 μL with several volumes of sterile Muller–Hinton broth (Sigma–Aldrich, Milan, Italy) previously set. Finally, 10 μL of bacterial suspension was added to each well to reach a concentration of about 5 × 105 cfu/mL. Sterile DMSO and ciprofloxacin (Sigma–Aldrich Italy, Milan, Italy, 1 mg/mL in DMSO) were used as negative and positive controls, respectively. Multiwell plates were prepared in triplicate and incubated at 37°C for 24 h. The lowest concentration at which a color change occurred (from dark purple to colorless) revealed the MIC value.
Biofilm Inhibitory Activity
The effect of the EOs on bacterial adhesion ability was assessed in flat-bottomed 96-well microtiter plates according to the method of O’Toole and Kolter (1998), using EO concentrations ranging from 0.01 to 2.0 μg/mL (corresponding to volumes ranging from 1 to 20 μL, respectively).
In each well, the overnight bacterial cultures were adjusted to 0.5 McFarland (1.5 × 107 cells/mL, Densitometer cell density turbidity 0.3–15.0 McFarland, CAMLAB, Cambridge, United Kingdom) with fresh culture broth. Then, 10 μL of the diluted cultures was distributed in each well, and different volumes of the extracts and Muller–Hinton broth were added, to reach a final volume of 250 μL/well. Microplates were completely covered with parafilm, to avoid the evaporation of samples with relative loss of volume and incubated for 48 h at different temperatures (depending on the strain). Planktonic cells were removed and the attached cells were gently twice washed with sterile physiological saline. After that, 200 μL of methanol was added to each well, retaining it for 15 min to fix the sessile cells. Methanol was then discarded, and each plate was left until complete dryness of samples. The staining of the adhered cells was obtained by adding 200 μL of 2% w/v crystal violet solution to each well that was left for 20 min. Wells were gently washed with sterile physiological solution and left to dry. Two hundred microliters of glacial acetic acid 20% w/v were added to allow the release of the bound dye. The absorbance was measured at OD = 540 nm (Varian Cary Spectrophotometer model 50 MPR, Cernusco sul Naviglio, Italy). The percent value of biofilm inhibition was calculated with respect to control (cells grown without the presence of the EOs). Triplicate tests were done, and the average results were taken for reproducibility.
Metabolic Activity of Biofilm Cells
The effect of different concentrations of Eos, ranging from 0.01 to 2.0 μg/mL on the metabolic activity of biofilm cells, was evaluated through the MTT colorimetric method according to Kairo et al. (1999) and Fratianni et al. (2019), using 96-well microtiter plates.
The overnight bacterial cultures were adjusted to 0.5 McFarland and treated as described in Section “Biofilm Inhibitory Activity.”
After 48 h incubation, bacterial suspension was removed and 150 μL of PBS and 30 μL of 0.3% MTT (Sigma, Milan, Italy) were added, keeping microplates at 37°C. After 2 h, the MTT solution was removed, two washing steps were performed with 200 μL of sterile physiological solution, and 200 μL of DMSO was added to allow the dissolution of the formazan crystals, which were measured after 2 h at OD = 570 nm (Varian Cary Spectrophotometer model 50 MPR, Cernusco sul Naviglio, Italy). Triplicate tests were carried out and the average results were taken for reproducibility.
Phytotoxic Activity
The phytotoxic activity was evaluated on germination and radical elongation of R. sativus L. (radish), L. sativa L. (lettuce), L. sativum L. (garden cress), S. lycopersicum L. (tomato), L. multiflorum Lam. (Italian ryegrass), and P. oleracea L. (purslane). These seeds are usually used in phytotoxicity assays because they easily germinate and are well known from a histological point of view. Radish, lettuce, garden cress, and tomato seeds were purchased from the Blumen Group s.r.l. (Emilia Romagna, Italy), Italian ryegrass seeds were purchased from Fratelli Ingegnoli Spa (Milano, Italy), and purslane seeds from W. Legutko s.r.l. (Jutrosin, Poland). The seeds were surface sterilized in 95% ethanol for 15 s and sown in Petri dishes (Ø = 90 mm), containing three layers of Whatman filter paper, and impregnated with 7 mL of distilled water (control) or 7 mL of the tested solution of EO. The germination conditions were 20 ± 1°C, with a natural photoperiod. The EOs, dissolved into water-acetone mixture (99.5:0.5), were assayed at the doses of 100, 10, 1, and 0.1 μg/mL. Controls performed with water–acetone mixture alone showed no differences in comparison to controls in water alone. Seed germination was observed directly in Petri dishes every 24 h. A seed was considered germinated when the protrusion of the root became evident (Bewley, 1997). After 120 h (on the fifth day), the effects on radicle elongation were measured in cm. Each determination was repeated three times, using Petri dishes containing 10 seeds each. Data were expressed as mean ± SD for both germination and radical elongation.
Statistical Analysis
All experiments were carried out in triplicate. Data from each experiment were statistically analyzed using GraphPad Prism 6.0 software (GraphPad Software Inc., San Diego, CA, United States) followed by comparison of means (two-way ANOVA) using Dunnett’s multiple comparisons test, at the significance level of p < 0.05.
Results and Discussion
Micromorphological Characterization
The leaf anatomical features of L. petersonii and E. gunnii have been scarcely investigated, although leaves are the main section of the plant from which EOs are extracted. Concerning E. gunnii, most recent studies have been focused on the epicuticular waxes of the leaf surface, considering their morphology, composition, function, and biosynthesis, and also regarding the modulatory effects of different stress factors (Shepherd and Griffiths, 2006) and regeneration processes after removal (Huth et al., 2018). A brief anatomical description of L. petersonii leaf anatomy has been reported by Johnson (1980) in his comprehensive revision of the genus Leptospermum, taking into account 40 different species.
Our FE-SEM investigation has highlighted many typical xeromorphic adaptations against water loss, such as sunkenstomata, thick cuticles, and a waxy epidermis in the leaves of L. petersonii (Figures 1A,B), and even more distinctly in those of E. gunnii (Figures 2A,B). The cuticle on the leaf epidermal surfaces of E. gunnii is smooth or slightly striated and shows cuticular papillae and epicuticular waxes shaped as small granules (Figure 2B). These features appear similar to those reported by Migacz et al. (2018) for Eucalyptus dunni Maiden. The mesophyll was dorsiventral in L. petersonii (Figures 1C,D), whereas it appeared isobilateral in E. gunnii (Figures 2C,D). The oil glands of both species have previously been found to develop by schizogony of cell walls (Carr and Carr, 1970; Johnson, 1980). In our observations, these schizogenous cavities appeared scattered in the mesophyll, especially in the sub-epidermal region, on both leaf sides (Figures 1, 2C,D). In transversal sections of E. gunnii leaves, many prismatic crystals and druses of calcium oxalate were also found, mainly around the ribs and near the oil glands (Figures 2C,D, arrows). On the contrary, prismatic crystals were absent on the leaf epidermal surface, being one of the diagnostic features useful to differentiate Eucalyptus species (Migacz et al., 2018).
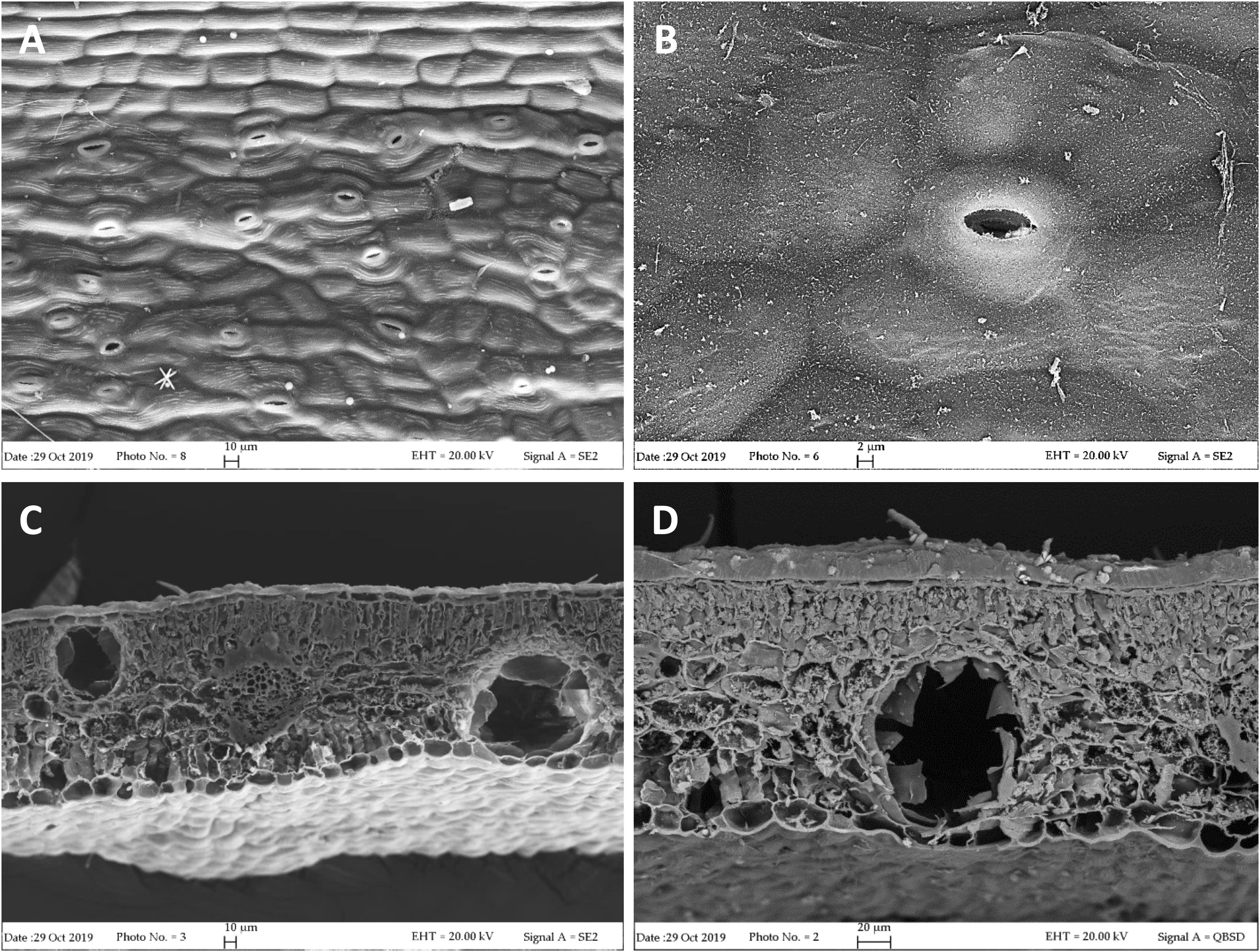
Figure 1. SEM micrographs of L. petersonii leaves. (A,B) Epidermal surface showing subpolygonal striate cells and scattered paracytic stomata. (C) Leaf transversal section showing two secretory cavities, located on each side of the rib, one close to the upper epidermis and the other one to the lower epidermis. (D) Higher magnification of an oil cavity located close to the lower epidermis of the leaf.
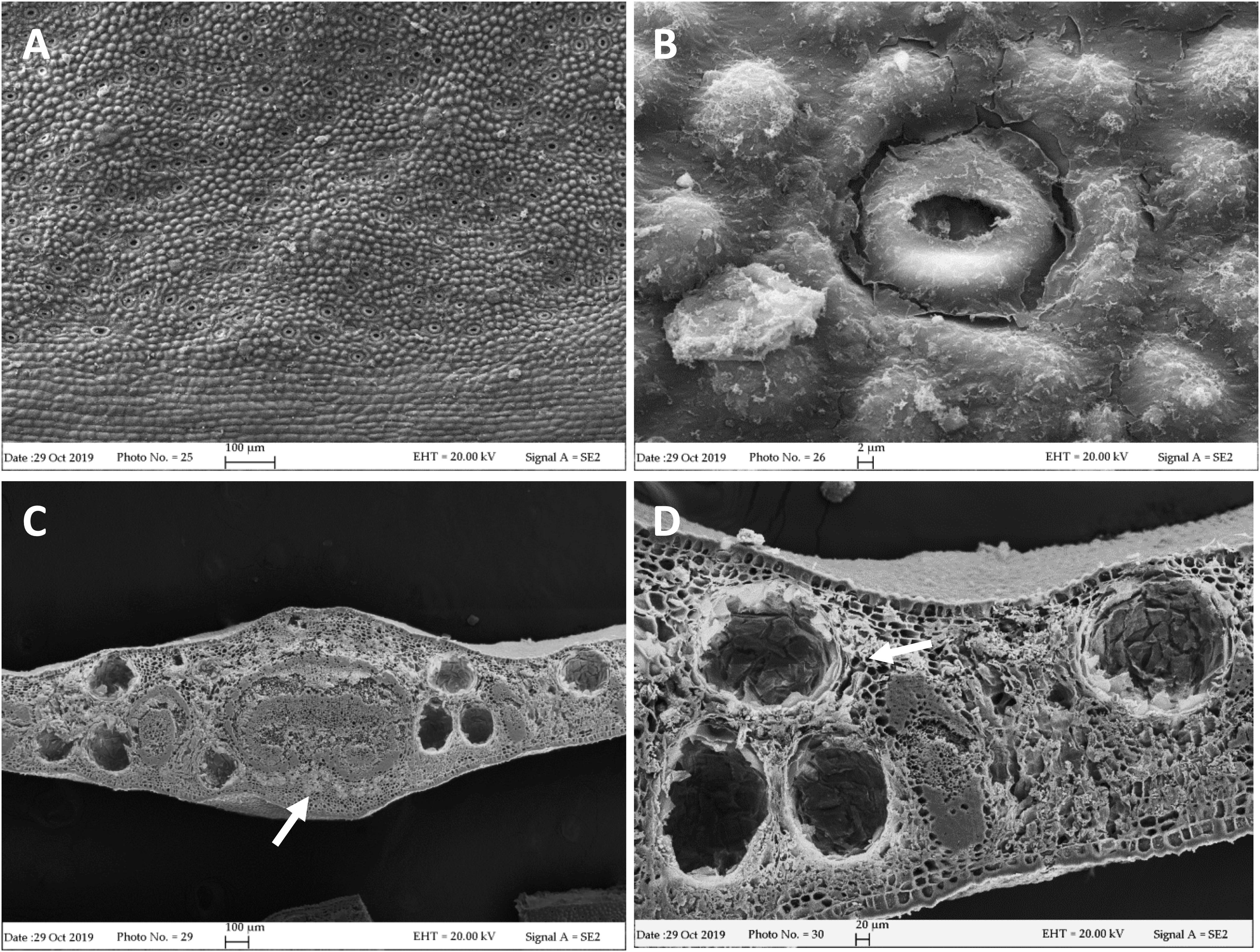
Figure 2. SEM micrographs of E. gunnii leaf. (A,B) Epidermal surface showing papillose cells and many anomocytic stomata. (C) Leaf trasversal section with secretory cavities scattered throughout the mesophyll, around the midrib. (C,D) Prismatic crystals and druses are visible near and around the secretory cavities and the rib (arrows).
The leaf morphological features detected in the examined species can be useful for their identification or in the quality control of herbal material used for EO extraction, to avoid accidental or intentional adulteration.
Essential Oils Yield and Chemical Composition
Essential Oil Yields
Steam distillation of the chemical variety “B CT Australian Rose” of L. petersonii and E. gunnii branchlets and leaves furnished EOs in 0.5 and 2% yields, respectively. The yield of our sample of L. petersonii was higher than other species of genus Leptospermum. In fact, Windsor and Brooks (2012) in their study observed a yield of 0.01–0.06% for Leptospermum laevigatum, 0.06–0.2% Leptospermum speciosum, and 0.2–0.4% for Leptospermum trinervium. Van Vuuren et al. (2014) demonstrated that for L. petersonii annual EO yields ranged from 0.4% (in February) and 1.8% (in March). Moreover, Demuner et al. (2011) showed that flower L. petersonii (0.5%) EO was lower than that found for the leaves (3.7%) in the same period.
Also, for genus Eucalyptus, the yields varied according to the species. In fact, Elaissi et al. (2010) analyzed 13 species from Tunisia and demonstrated yields ranging from 0.5%, for E. gunnii to 3.9% for Eucalyptus cinerea. Moreover, comparing different Eucalyptus species, Lucia et al. (2008) showed a significant relationship between total EO yields and 1.8-cineole concentration.
This shows that there are different factors that can lead to quantitative and qualitative differences in the EOs such as soil, season, temperature, geographic origin, and ecological role of the plant organs that produce EOs (Demuner et al., 2011).
Chemical Composition of Essential Oils
Essential oil compositions with retention indices and area percentages for each compound are reported in Tables 1, 2.
Sixty-four compounds were identified for L. petersonii EO, accounting for 98.9% of the total EO. In particular, the main constituents are geranyl acetate (31.4%), geraniol (9.5%), linalool (5.1%) as oxygenated monoterpenes and γ-terpinene (12.4%), terpinolene (9.3%), α-pinene (5.7%), p-cimene (5.6%), and (E)-β-Ocimene (5.1%) among monoterpenes hydrocarbons.
In the EO from E. gunnii. 24 compounds were identified, accounting for 97.9% of the total EO. The main compounds were: oxygenated monoterpenes with 1,8-cineole (33.0%), trans-sabinene hydrate acetate (15.0%), and globulol (10.3%).
Other minor components were α-fenchene (2.2%), iso-sylvestrene (2.3%), and limonene (2.9%) into L. petersonii EO, and longicyclene (9.1%), terpinolene (5.9%), and camphene (6.3%), aromadendrene (2.7%), viridiflorol (2.6%), and dehydro-linalool (2.3%) into E. gunnii EO.
Seven constituents were present in both EOs but in different amounts. The percentages of α-pinene (5.7%) and γ-terpinene (12.4%) in L. petersonii EO was higher than those in E. gunnii (0.3% for both compounds); instead, camphene (6.3%), 1,8 cineole (33.0%), and rosifoliol (1.4%) were present more in E. gunnii EO than in L. petersoniii (0.1, 0.5, and 0.1%, respectively). δ-2-carene and terpinolene were found in similar amounts in both oils, respectively, 0.7 and 5.9% in E. gunnii and 0.5 and 9.3% in L. petersonii EO.
The composition of the EO of L. petersonii chemical variety “B CT Australian Rose” of this study was partially in agreement with previous data. In fact, structural analogs of geraniol, such as geranial (34.7–29.9%) and neral (19.7–23.5%), have been reported as the main constituents of L. petersonii EO. On the other hand, constituents reported in high amounts in other studies, such as citronellal (33.9–11.4%) or citronellol (17.5%), were absent in our sample (Demuner et al., 2011; Kim and Park, 2012; Van Vuuren et al., 2014).
The different composition of the EOs confirmed the existence of several chemotypes in L. petersonii, as reported by Brophy et al. (2000). The most common chemical variety with a pleasant odor is the “A” type, which consists mainly of aldehydes such as neral, geranial, citronellal, and monoterpene hydrocarbons (γ-terpinene, α-pinene, p-cymene). On the contrary, the chemical variety “B,” with a rose-like odor, is quite rare and contains geraniol and geranyl acetate as main constituents, followed by γ-terpinene and terpinolene (Brophy et al., 2000).
There have been no large studies on the rare chemical variety “B”: the sample analyzed in this study was evidently derived from L. petersonii chemical variety “B.” In fact, our results agree with Brophy et al. (2000), who reported the chemical composition of three EOs belonging to this variety, with geranyl acetate (ranging from 21 to 38%) and geraniol (ranging from 21 to 29%) as the main components.
The chemical composition of the analyzed E. gunnii EO is quite similar to data reported in literature. 1,8 Cineole was confirmed as the main component, as described in previous studies, with a percentage ranging from 17.9 to 67.8% (Lucia et al., 2008; Bugarin et al., 2014). Conversely, spathulenol (16.5%) was reported as the major component of E. gunnii EO from Tunisia (Elaissi et al., 2011) and it was identified also in E. gunnii EO from Argentina with a percentage of 12.3% (Lucia et al., 2008). This compound was present in a very low amount (0.6%) in our EO. Moreover, viridiflorol and globulol were present in a high percentage in Tunisian EO (11.5 and 12.5%, respectively) (Elaissi et al., 2011). These compounds were also present in our sample, in particular globulolin in a similar quantity (10.3%), while viridiflorol was present in a lower amount (0.6%).
Antibacterial Activity
The antibacterial activity of the EOs was evaluated against Gram-positive and Gram-negative pathogenic strains, through the inhibition zone test and the determination of the MIC. Results are shown in Figure 3 and in Table 3, respectively.
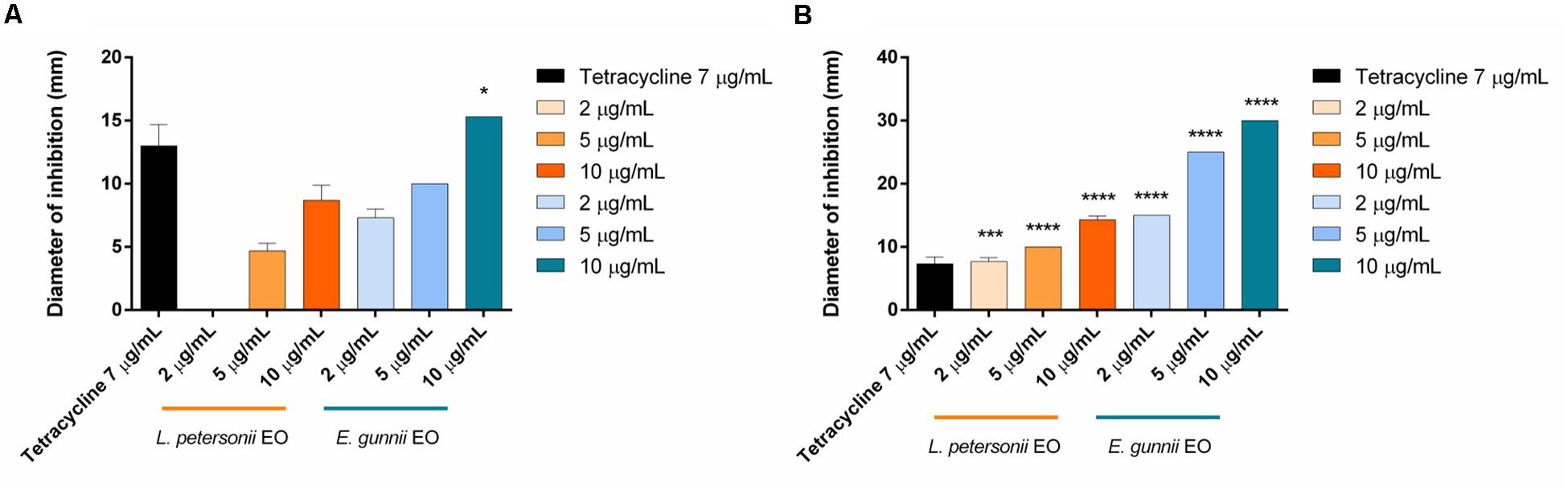
Figure 3. Antibacterial activity of L. petersonii and E. gunnii EOs and of tetracycline against the Gram-positive L. monocytogenes (A) and S. aureus (B). Results are the mean of three experiments. Dunnett’s test vs tetracycline 7 μg/mL (*p < 0.05; ***p < 0.001; ****p < 0.0001).
The behavior of the two EOs against Gram-positive (S. aureus and L. monocytogenes) and Gram-negative (E. coli, P. aeruginosa, and P. carotovorum) strains was completely different. E. gunnii EO showed the best antibacterial activity against Gram-positive strains. Indeed, at highest concentration (10 μg/mL), the inhibition zone vs S. aureus and L. monocytegenes was 2 and 2.5 times greater than those obtained using the same concentration of L. petersonii EO (Figure 3). Moreover, the highest concentration of E. gunnii EO was much more effective than the reference antibiotic against S. aureus and even more so against L. monocytogenes.
The different behavior showed by the two EOs at the highest concentration could be ascribable to their different phytochemical composition. The presence of 1.8-cineole (33%) and trans-sabinene hydrate-acetate (15%) in E. gunnii EO probably contributes to the strong antibacterial activity of this EO, according to Nazzaro et al. (2013). Indeed, 1.8-cineole is a well-known antibacterial agent with proven activity against all the microorganisms used in our experiments (Caputo et al., 2017). Furthermore, γ-terpinene and trans-sabinene hydrate-acetate could enhance the E. gunnii EO ability to block the bacterial growth (Nazzaro et al., 2013), probably due to a synergistic mechanism (Dorman and Deans, 2000). Finally, globulol (10.3%), which was identified in other Eucalyptus EOs, also possesses antibacterial activity (Sebei et al., 2015).
On the contrary, there is no positive correlation between the high amount of geranyl-acetate in L. petersonii EO and antibacterial efficacy vs L. monocytogenes (Lis-Balchin and Deans, 1997).
Considering the Gram-negative microorganisms tested, the results obtained would allow differentiating two subgroups for resistance/sensitivity to the L. petersonii and E. gunnii EOs, a first sub-group represented by E. coli and P. aeruginosa, and a second one by P. carotovorum. E. gunnii EO showed good activity against E. coli, although it was weaker than that exerted on other E. coli strains (Bugarin et al., 2014). It is well known that the resistance/sensitivity to an antimicrobial agent may vary among different strains of the same species (Del Monte et al., 2015). However, we should stress that the inhibition zone induced by E. gunnii EO was almost double than tetracycline (Figure 4A). E. gunnii EO also showed strong activity against P. aeruginosa, with an inhibition diameter twice with respect to tetracycline (Figure 4B). Thus, this EO can represent a product with a wide potential against pathogenic species such as E. coli, P. aeruginosa, L. monocytogenes, and S. aureus, resulting in particular interest due to the increased number of microbial species showing resistance to antibiotic drugs (Heras et al., 2015; Hartmann et al., 2019).
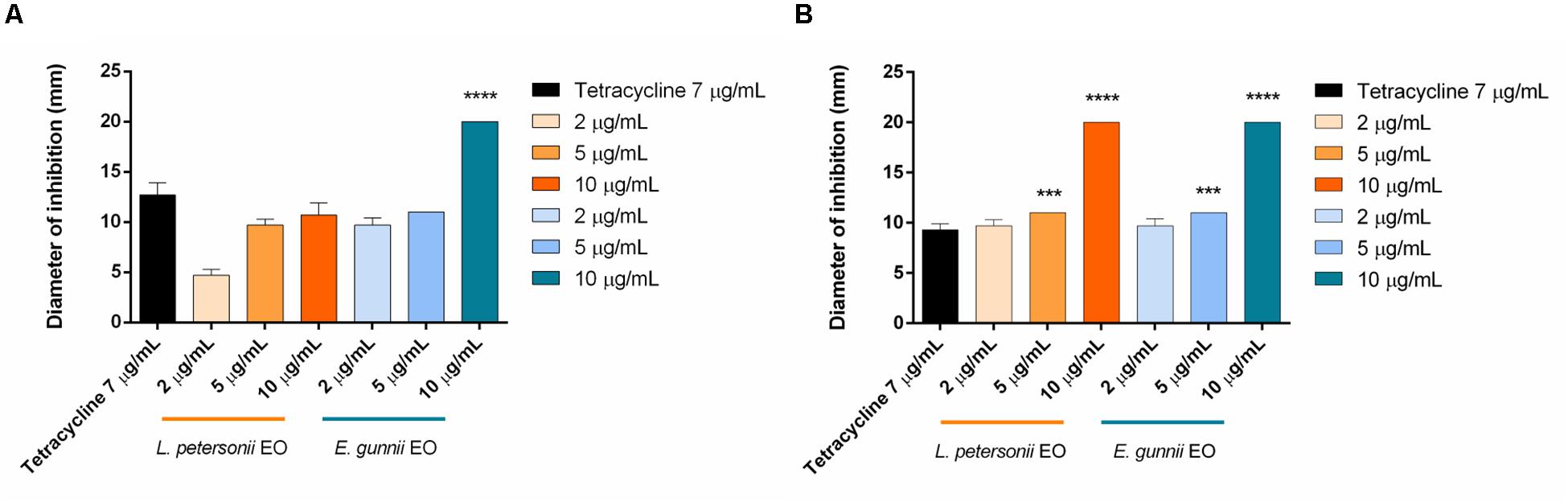
Figure 4. Antibacterial activity of L. petersonii and E. gunnii EOs and of tetracycline against the Gram-negative E. coli (A) and P. aeruginosa (B). Results are the mean of three experiments. Dunnett’s test vs tetracycline 7 μg/mL (***p < 0.001; ****p < 0.0001).
Leptospermum petersonii EO was usually less effective in comparison with E. gunnii EO, as indicated by the MIC values (Table 3). Moreover, despite exercising an inhibitory activity against E coli at all the concentrations used, its efficacy (width of the inhibition zone) was always less than tetracycline (Figure 4A). On the contrary, it was more effective vs Pseudomonas, with inhibition zones of about 20 mm, practically double with respect to tetracycline. Indeed, in this case, the inhibiting force was similar to that exerted by the E. gunnii EO (Figure 4B). The effectiveness shown by L. petersonii EO against P. aeruginosa and S. aureus (Figures 3, 4B) is in accordance with Demuner et al. (2011), which demonstrated a strong efficacy of Leptospermum spp. EOs against these bacteria. The composition of L. petersonii EO from Brazil and South Africa, with considerable amounts of citronellal, geranial, and neral, may confer forceful antimicrobial activity (Van Vuuren et al., 2014).
An MIC test confirmed the more powerful activity of E. gunnii EO (Table 3), with MIC values which ranged between 0.5 and 2.0 μg/mL (against S. aureus and P. carotovorum, respectively). The activity of E. gunnii EO was generally stronger than L. petersonii EO, whose maximum MIC value reached even 5 μg/mL. MIC values of L. petersonii EO were usually higher against L. monocytogenes (Table 3).
Pectobacterium (formerly Erwinia) carotovorum is a Gram-negative bacterium belonging to the Enterobacteriaceae family, which affects several crops such as potato, pineapple, maize, and African violet (Mehrsorosh et al., 2014). It causes soft rot and blackleg of potatoes and vegetables, as well as slime flux on many different tree species. The soft rot erwinias can be present on plant surfaces and in soil where they may penetrate within the plant via wound sites or through natural openings on the plant surface, e.g., lenticels.
In the antibacterial tests, we observed completely opposed behavior of the two EOs investigated. E. gunnii EO resulted active already at 2 μg/mL (Table 3), causing an inhibition zone of 7 mm (Figure 5). The highest concentration (10 μg/mL) showed an inhibition zone of 17 mm, almost double with respect to the diameter obtained with tetracycline (Figure 5).
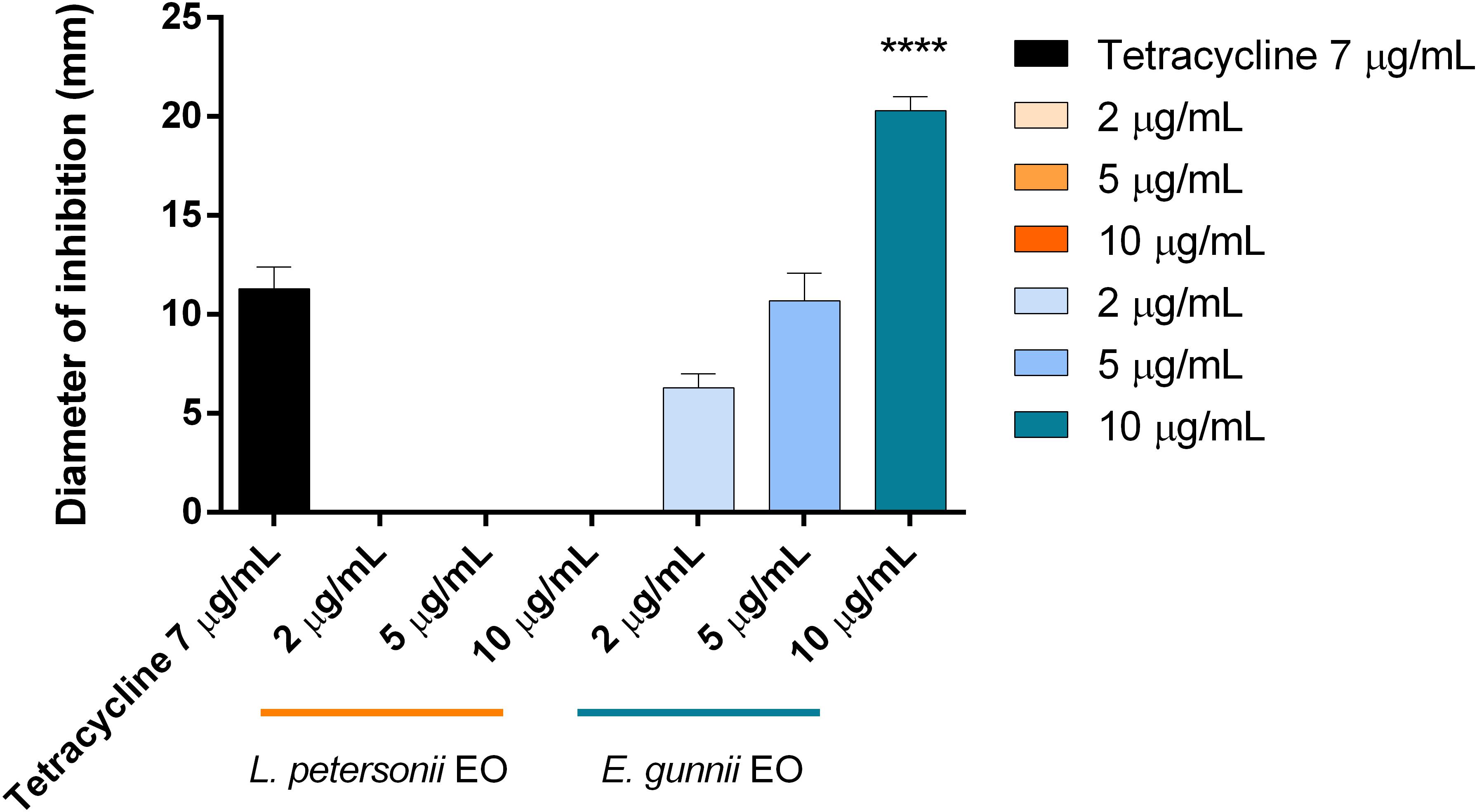
Figure 5. Antibacterial activity of L. petersonii and E. gunnii EOs and of tetracycline against P. carotovorum. Results are the mean of three experiments. Dunnett’s test vs tetracycline 7 μg/mL (****p < 0.0001).
To our knowledge, this is the first time that the antibacterial activity of E. gunnii EO against P. carotovorum has been evaluated. Such activity was stronger than those of EOs obtained from other species belonging to the Eucalyptus genus, such as Eucalyptus caesia Benth., Eucalyptus camaldulensis subsp. obtusa (Blakely Brooker and M. W. McDonald), and Eucalyptus gomphocephala A. Cunn. ex DC., and much more effective with respect to some Lamiaceae EOs (Mehrsorosh et al., 2014; Salem et al., 2015).
This high antibacterial activity against the Gram negative phytopathogen P. carotovorum makes this EO an ideal candidate to treat and prevent bacterial infections in crops; in addition, through the use of new species-specific technologies, such as the encapsulation of EO in mesoporous silica nanoparticles, this EO has high efficacy when applied to this and other phytopathogens (Cadena et al., 2018).
Biofilm and Metabolic Activity of Biofilm Cells
Eucalyptus gunnii and L. petersonii EOs were tested on the above bacterial strains for inhibition of biofilm formation and metabolic activity. In our study, for the first time, biofilm inhibition by these two EOs (Table 4) was integrated with EO effects on biofilm metabolism (Table 5), thus increasing available information on their antibacterial power and potential applications.
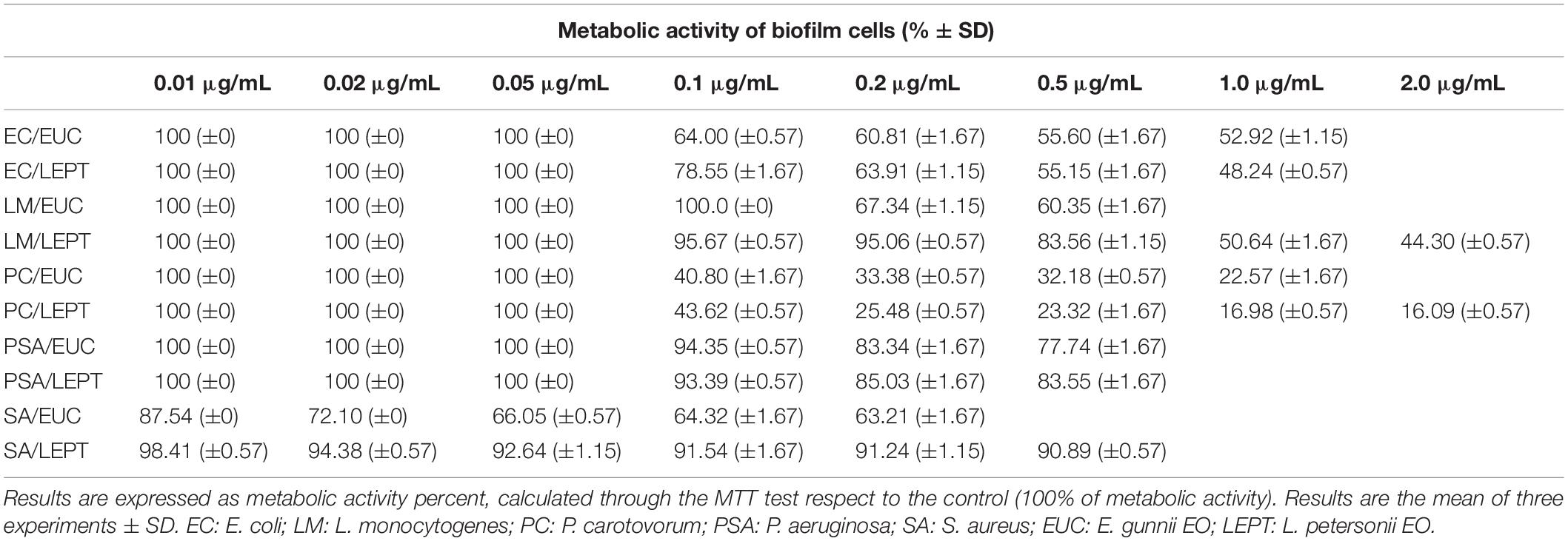
Table 5. Metabolic activity exhibited by the cells present within the bacterial biofilms in the presence of different concentrations of E. gunnii and L. petersonii EOs.
The activity of E. gunnii EO against the uropathogen E. coli was of particular interest. Indeed, at a concentration of only 0.5 μg/mL, this EO causes a biofilm formation inhibition of 80.28%. These results were in accordance with other previous studies (Karpanen et al., 2008; Hendry et al., 2009), which have ascertained the antimicrobial activity of Eucalyptus EO vs E. coli and Staphylococcus epidermidis biofilm formation.
Moreover, E. gunnii EO decreased the metabolic activity of the E. coli biofilm cells to 55.6% with respect to the control, as observed by MTT assay (Table 5). This means that E. gunnii EO was capable not only of strongly inhibiting the E. coli biofilm formation but also to limiting all metabolic changes, which make cells more resistant to the antibacterial agents. L. petersonii EO, although less strong than the previous one, still shows inhibitory properties against E. coli biofilm formation (96.22%) and decreases the metabolic activity (48.24%) at 1.0 μg/mL (Tables 4, 5, respectively). The activity of E. gunnii and L. petersoni EOs against E. coli could be particularly important in clinical settings and patient management.
When EOs were tested to evaluate their ability to block L. monocytogenes biofilm formation, their behavior was different and L. petersonii EO showed, in general, the best antibacterial activity. Indeed, it inhibits the L. monocytogenes biofilm formation at 0.1 μg/mL, with an inhibition rate reaching 78.41% at 2 μg/mL. Moreover, it was able to influence the biofilm cells metabolism by decreasing their activity to 44.30% compared to the control (Table 5). On the contrary, E. gunnii EO was completely inactive until 0.1 μg/mL, showing an inhibition of biofilm formation of 4.8 and 50.21% at 0.2 and 0.5 μg/mL, respectively (Table 4). The latter concentration (0.5 μg/mL) showed a decrease of biofilm cells metabolism to 60.35% with respect to control (Table 5).
Essential oils investigated showed a weak inhibitory activity regarding both biofilm growth (35.43 and 20.15% by E. gunnii and L. petersoni EOs, respectively, Table 4) and metabolic activity of P. aeruginosa (77.74 and 83.55% by E. gunnii and L. petersoni EOs, respectively, Table 5).
This confirmed the weak antibacterial activity of Eucalyptus species against biofilm formation, as previously reported for Eucalyptus globulus Labill. EO (Sambyal et al., 2017), although its action could be enhanced through innovative biotechnological processes. For instance, nanoemulsion containing E. globulus EO showed antimicrobial and anti-biofilm activities against some Gram-negative bacteria, commonly found in immunosuppressed patients, such as P. aeruginosa and C. albicans (Quatrin et al., 2017).
The two EOs were particularly effective against S. aureus (Table 4), inhibiting biofilm formation by 10.37 and 14.78%, respectively, at a concentration of 0.01 μg/mL. The activity of E. gunnii EO on S. aureus was stronger than L. petersonii EO at a concentration above 0.01 μg/mL (Table 4). The inhibitory effect of E. gunnii EO against S. aureus confirmed the capability of the EOs recovered by species belonging to the Eucalyptus genus to block the biofilm formation by this methicillin resistant strain (Junka et al., 2019); its activity was more powerful than E. globulus EO (Merghni et al., 2018). The effectiveness shown by both EOs could be taken into consideration, once again, in patient management, mainly to avoid S. aureus infections that concern the central venous, dialysis (Gahlot et al., 2014), and urinary catheters (Murugan et al., 2016), as well as arterio-venous shunts (MacRae et al., 2016) and sutures (Henry-Stanley et al., 2010). Interestingly, L. petersonii EO at 0.5 μg/mL induced a strong inhibition of S. aureus biofilm formation (79.88%), while maintaining a high metabolic activity (90.89%) compared to the control.
Eucalyptus gunnii EO was particularly active in inhibiting the biofilm formation of the Gram-negative phytopathogen P. carotovorum. Indeed, at 1 μg/mL, it was capable of avoiding almost completely the biofilm formation (93.12%, Table 4) and to strongly affect the metabolic activity of the few biofilm cells (22.57% respect to control, Table 5). On the contrary, L. petersoni EO at a concentration of 2.0 μg/mL induced a relatively lower inhibition (66.67%, Table 4) on biofilm formation, while also markedly affecting metabolic activity (16.09% with respect to control) (Table 5). These results are in accordance with what was previously reported about antimicrobial properties of a hydroalcholic extract of L. petersonii leaves (Shirdashtzadeh et al., 2017).
Phytotoxic Activity
A delay in germination or any other adverse effect on plants caused by specific substances is defined as phytotoxicity (Baumgarten and Spiegel, 2004). Moreover, secondary metabolites produced by plants, micro-organisms, viruses, and fungi, can alter the growth of the target species, with both positive and negative effects, through a phenomenon called “allelopathy” (Zeng et al., 2010).
Parameters to analyze the effects of natural or chemical substances on the growing of selected vegetal species are: relative or absolute germination and relative root elongation (Barral and Paradelo, 2011).
In this study, the two EOs were evaluated for their activity against germination and radical elongation of radish, lettuce, garden cress, tomato, rye grass, and purslane. L. petersonii EO showed inhibitory activity against the germination of R. sativus (Figure 6A) and radical elongation of S. lycopersicum (Figure 6B). The treatment of seeds with a concentration of 100 μg/mL inhibited germination of R. sativus; all doses tested seemed to be active against radical elongation of S. lycopersicum. E. gunnii EO showed no phytotoxic activity on the tested seed (data not shown). Its principal component, 1,8 cineole, was inactive against several of the tested seeds, as shown in our previous studies (Caputo et al., 2018).
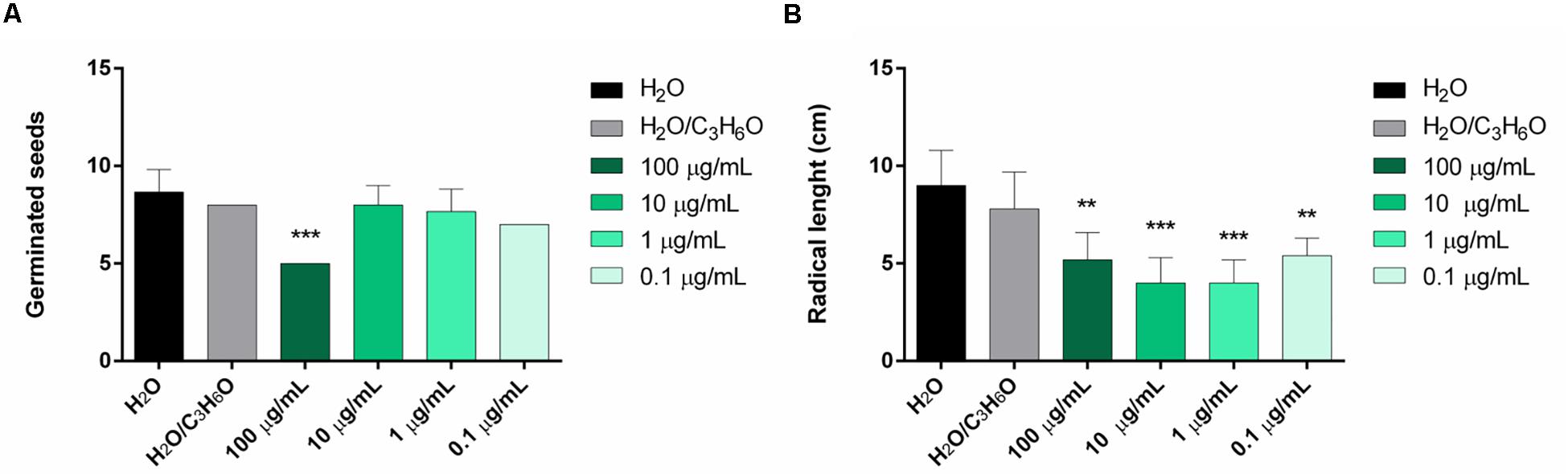
Figure 6. Phytotoxic activity of L. petersonii EO against germination of R. sativus (A) and radical elongation of S. lycopersicum (B), 120 h after sowing. Results are the mean of three experiments ± SD. ** p < 0.01; *** p < 0.001 compared to control (ANOVA followed by Dunnett’s multiple comparison test).
Few previous studies have reported data about the phytotoxicity and/or allelopathy of extracts and or EOs of plants belonging to the Myrtaceae family. Imatomi et al. (2013) studied the phytotoxic potential of leaf extracts of Myrcia tomentosa Glaz. and showed that ethyl acetate extract was the most active on the roots of lettuce, tomato, and onion, as well as on the shoots of lettuce and tomato. Instead, ethyl acetate extract of leaves of Blepharocalyx salicifolius (Kunth) had a high phytotoxic activity against Triticum aestivum L., Sesamum indicum L., Echinochloa crusgalli L. Beauv., and Euphorbia heterophylla L. (Habermann et al., 2015, 2016). Methanol extract of Eugenia flavescens DC leaves showed significant seed germination inhibition of Mimosa pudica and Senna obtusifolia (Cantanhede Filho et al., 2017). Moreover, Callistemon viminalis EO showed dose-dependent allelopathic activity on L. sativa seeds (de Oliveira et al., 2014). Myrtus communis L. EO inhibited seed germinations and seedling growths of Amaranthus retroflexus L., Chenopodium album L., Cirsium arvense (L.) SCOP., Lactuca serriola L., and Rumex crispus L. (Kordali et al., 2016).
In this study, we evaluated for the first time the phytotoxicity of L. petersonii EO; in fact, no previous studies have reported similar data about this or other plants from the genus Leptospermum.
On the contrary, the phytotoxic and allelopathic activities of several Eucalyptus species are well known in both natural and modified ecosystems (Chu et al., 2014) and some EOs from Eucalyptus spp. have been proposed for use in agricultural and forestry management (Ramezani et al., 2008). Despite the fact that the Eucalyptus genus is probably among the most investigated for its allelopathic properties, no studies are currently available on the activity of E. gunnii EO on seeds growing.
Conclusion
This is the first study that provides a detailed SEM analysis of the leaf micromorphology of L. petersonii and E. gunnii. The quali-quantitative analyses showed a superimposable phytochemical profile for E. gunnii EO with respect to other previously published data. In addition, it highlighted the phytochemical composition of the EO of a quite rare L. petersonii chemical variety “B CT Australian Rose,” with a rose-like odor and containing geraniol and geranyl acetate as main constituents.
This is also the first time that the effects of these EOs on the biofilm formation biofilm cells metabolic activity were investigated.
This could open new perspectives for the application of L. petersonii and E. gunnii EOs.
Despite both EOs showing a good antimicrobial activity, the E. gunnii EO was the strongest one. This is probably due to the high content of 1,8-cineole, although a synergism with γ-terpinene and trans-sabinene hydrate-acetate could be postulated in light of previous literature data about the antimicrobial activity of the pure compound 1,8-cineole against the same pathogens.
In addition, both EOs were particularly effective against S. aureus biofilm formation, with E. gunnii EO showing the strongest activity not only with respect to L. petersonii EO, but also compared to other EOs isolated from other Eucalyptus species.
On the contrary, L. petersonii chemical variety “B CT Australian Rose” EO showed inhibitory activity against germination and radical elongation of R. sativus and S. lycopersicum, respectively, while no phytotoxic activity was detected for E. gunnii EO.
Considering these results, further investigations on these EOs could provide useful applications for both the treatment of human microbial infections and for agronomic management.
Data Availability Statement
All datasets generated for this study are included in the article.
Author Contributions
VD, FN, LaC, and DT designed the project. MV and GT distilled the EOs. FN and FF performed the antibacterial assays. LuC studied the phytotoxic activity and performed the data analysis. LaC carried out the micromorphological characterization. AS and LuC performed the chemical characterization. All authors contributed to writing and revising the manuscript.
Conflict of Interest
GT is the owner of Essentially Australia.
The remaining authors declare that the research was conducted in the absence of any commercial or financial relationships that could be construed as a potential conflict of interest.
Abbreviations
DMSO, dimethylsulfoxide; EO(s), essential oil(s); FE-SEM, field-emission—scanning electron microscopy; FID, flame ionization detector; GC, gas chromatography; MIC, minimal inhibitory concentration; MS, mass spectrometry; MTT, 3-(4,5-dimethyl-2-thiazolyl)-2,5-diphenyl-2H-tetrazolium bromide; NIST, National Institute of Standards and Technology; PBS, phosphate buffered saline; SD, standard deviation.
References
Adams, R. P. (2007). Identification of Essential Oil Components by Gas Chromatography/Mass Spectroscopy. Carol Stream, IL: Allured Publishing.
Barral, M. T., and Paradelo, R. (2011). A review on the use of phytotoxicity as a compost quality indicator. Dyn. Soil Dyn. Plant 5, 36–44.
Batish, D. R., Singh, H. P., Kohli, R. K., and Kaur, S. (2008). Eucalyptus essential oil as a natural pesticide. For. Ecol. Manag. 256, 2166–2174. doi: 10.1016/j.foreco.2008.08.008
Baumgarten, A., and Spiegel, H. (2004). Phytotoxicity (Plant Tolerance). Vienna: Agency for Health and Food Safety.
Bernal-Mercado, A. T., Gutierrez Pacheco, M. M., Encinas Basurto, D., MataHaro, V., Lopez Zavala, A. A., Islas Osuna, M. A., et al. (2019). Synergistic mode of action of catechin, vanillic and protocatechuic acids to inhibit the adhesion of uropathogenic Escherichia coli on silicone surfaces. J. Appl. Microbiol. 128, 387–400. doi: 10.1111/jam.14472
Brooker, M. I. H., and Kleinig, D. A. (1996). Eucalyptus. An Illustrated Guide to Identification. Sidney, OH: Reed New Holland.
Brooker, S. G., Cambie, R. C., and Cooper, R. C. (1987). New Zealand Medicinal Plants. London: Heinemann.
Brophy, J. J., Goldsack, R. J., Punruckvong, A., Bean, A. R., Forster, P. I., Lepschi, B. J., et al. (2000). Leaf essential oils of the genus Leptospermum (Myrtaceae) in eastern Australia, Part 7. Leptospermum petersonii, L. liversidgei and allies. Flavour Fragr. J. 15, 342–351. doi: 10.1002/1099-1026(200009/10)15:5<342::aid-ffj924>3.0.co;2-v
Bugarin, D., Grbovixć, S., Orèiè, D., Mitić-Ćulafić, D., Knežević-Vukèević, J., and Mimica-Dukić, N. (2014). Essential oil of Eucalyptus Gunnii hook. As a novel source of antioxidant, antimutagenic and antibacterial agents. Molecules 19:19007. doi: 10.3390/molecules191119007
Cadena, M. B., Preston, G. M., Van der Hoorn, R. A. L., Townley, H. E., and Thompson, I. P. (2018). Species-specific antimicrobial activity of essential oils and enhancement by encapsulation in mesoporous silica nanoparticles. Ind. Crops Prod. 122, 582–590. doi: 10.1016/j.indcrop.2018.05.081
Camporese, A. (2013). In vitro activity of Eucalyptus smithii and Juniperus communis essential oils against bacterial biofilms and efficacy perspectives of complementary inhalation therapy in chronic and recurrent upper respiratory tract infections. Infez. Med. 21, 117–124.
Cantanhede Filho, A. J., Santos, L. S., Guilhon, G., Zoghbi, M. D. G. B., Ports, P. S., and Rodrigues, I. (2017). Triterpenoids, phenolics and phytotoxic effects from Eugenia flavescens DC (Myrtaceae) leaves.Quim. Nova 40, 252–259.
Caputo, L., Nazzaro, F., Souza, L., Aliberti, L., De Martino, L., Fratianni, F., et al. (2017). Laurus nobilis: composition of essential oil and its biological activities. Molecules 22:930. doi: 10.3390/molecules22060930
Caputo, L., Trotta, M., Romaniello, A., and De Feo, V. (2018). Chemical composition and phytotoxic activity of Rosmarinus officinalis essential oil. Nat. Prod. Commun. 13, 1367–1370.
Carr, D., and Carr, S. G. (1970). Oil glands and ducts in Eucalyptus L’Herit. II. Development and structure of oil glands in the embryo. Aust. J. Bot. 18, 191–212.
Chieco, C., Rotondi, A., Morrone, L., Rapparini, F., and Baraldi, R. (2012). An ethanol-based fixation method for anatomical and micro-morphological characterization of leaves of various tree species. Biotech. Histochem. 88, 109–119. doi: 10.3109/10520295.2012.746472
Chu, C., Mortimer, P. E., Wang, H., Wang, Y., Liu, X., and Yu, S. (2014). Allelopathic effects of Eucalyptus on native and introduced tree species. For. Ecol. Manage. 323, 79–84. doi: 10.1016/j.foreco.2014.03.004
Crowe, A. (1997). A Field Guide to the Native Edible Plants of New Zealand. Auckland: Penguin Books.
Davies, N. W. (1990). Gas chromatographic retention indices of monoterpenes and sesquiterpenes on methyl silicone and Carbowax 20M phases. J. Chromatogr. 503, 1–24. doi: 10.1016/s0021-9673(01)81487-4
de Oliveira, C. M., das Graças Cardoso, M., da Silva, Figueiredo, A. C., de Carvalho, M. L. M., de Miranda, C. A. S. F., et al. (2014). Chemical composition and allelopathic activity of the essential oil from Callistemon viminalis (myrtaceae) blossoms on lettuce (Lactuca sativa L.) seedlings. Am. J. Plant Sci. 5, 3551–3557. doi: 10.4236/ajps.2014.524371
Del Monte, D., De Martino, L., Marandino, A., Fratianni, F., Nazzaro, F., and De Feo, V. (2015). Phenolic content, antimicrobial and antioxidant activities of Hypericum perfoliatum L. Ind. Crop. Prod. 74, 342–347. doi: 10.1080/13880209.2016.1270973
Demuner, A. J., Almeida Barbosa, L. C., Gonçalves Magalhaes, C., Da Silva, C. J., Alvares Maltha, C. R., and Lelis Pinheiro, A. (2011). Seasonal variation in the chemical composition and antimicrobial activity of volatile oils of three species of Leptospermum (Myrtaceae) grown in Brazil. Molecules 16, 1181–1191. doi: 10.3390/molecules16021181
Dhakad, A. K., Pandey, V. V., Beg, S., Rawat, J. M., and Singh, A. (2018). Biological, medicinal and toxicological significance of Eucalyptus leaf essential oil:a review.J. Sci. Food Agric. 98, 833–848. doi: 10.1002/jsfa.8600
Djordjevic, D., Wiedmann, M., and McLandsborough, L. A. (2002). Microtiter plate assay for assessment of Listeria monocytogenes biofilm formation. Appl. Environ. Microbiol. 68, 2950–2958. doi: 10.1128/aem.68.6.2950-2958.2002
Dorman, H. J. D., and Deans, S. G. (2000). Antimicrobial agents from plants: antibacterial activity of volatile plant oils. J. Appl. Microbiol. 88, 308–316. doi: 10.1046/j.1365-2672.2000.00969.x
Elaissi, A., Marzouki, H., Medini, H., Larbi Khouja, M., Farhat, F., Lynene, F., et al. (2010). Variation in volatile leaf oils of 13 Eucalyptus species harvested from Souinet Arboreta (Tunisia). Chem. Biodivers. 7, 909–921. doi: 10.1002/cbdv.200900229
Elaissi, A., Salah, K. H., Mabrouk, S., Larbi, K. M., Chemli, R., and Harzallah-Skhiri, F. (2011). Antibacterial activity and chemical composition of 20 Eucalyptus species’ essential oils. Food Chem. 129, 1427–1434. doi: 10.1016/j.foodchem.2011.05.100
Forrest, M. (2002). The performance of a Eucalyptus gunnii cut foliage plantation over 7 years. Irish J. Agr. Food Res. 41, 235–245.
Fratianni, F., Cozzolino, A., De Feo, V., Coppola, R. Ombra, M. N., and Nazzaro, F. (2019). Polyphenols, antioxidant, antibacterial, and biofilm inhibitory activities of peel and pulp of Citrus medica L., Citrus bergamia, and Citrus medica cv. Salò Cultivated in Southern Italy. Molecules 24:4577. doi: 10.3390/molecules24244577
Fratianni, F., Ombra, M. N., Cozzolino, A., Riccardi, R., Spigno, P., Tremonte, P., et al. (2016). Phenolic constituents, antioxidant, antimicrobial and anti-proliferative activities of different endemic Italian varieties of garlic (Allium sativum L.). J. Funct. Foods 21, 240–248. doi: 10.1016/j.jff.2015.12.019
Gahlot, R., Nigam, C., Kumar, V., Yadav, G., Anupurba, S., Gahlot, R., et al. (2014). Catheter-related bloodstream infections. Int. J. Crit. Illn. Inj. Sci. 4, 162–167.
Goodner, K. L. (2008). Practical retention index models of OV-101, DB-1, DB-5, and DB-Wax for flavor and fragrance compounds. LWT Food Sci. Technol. 41, 951–958. doi: 10.1016/j.lwt.2007.07.007
Gutierrez-Pacheco, M. M., Gonzalez-Aguilar, G. A., Martinez-Tellez, M. A., Lizardi-Mendoza, J., Madera-Santana, T. J., Bernal-Mercado, A. T., et al. (2018). Carvacrol inhibits biofilm formation and production of extracellular polymeric substances of Pectobacterium carotovorum subsp. carotovorum. Food Control 89, 210–218. doi: 10.1016/j.foodcont.2018.02.007
Habermann, E., Imatomi, M., De Cassia Pereira, V., Cevithereza Pontes, F., Gualtieri, J., and Cristina, S. (2015). Phytotoxic activity of stem bark and leaves of Blepharocalyx salicifolius (Myrtaceae) on Weeds. Acta Biolo. Colom. 20, 153–162. doi: 10.15446/abc.v20n1.42756
Habermann, E., Pontes, F. C., Pereira, V. C., Imatomi, M., and Gualtieri, S. C. J. (2016). Phytotoxic potential of young leaves from Blepharocalyx salicifolius (Kunth) O. Berg (Myrtaceae). Braz. J. Biol. 76, 531–538. doi: 10.1590/1519-6984.24114
Hartmann, R., Singh, K. P., Pearce, P., Mok, R., Boya Song, B., Francisco Díaz-Pascual, F., et al. (2019). Emergence of three-dimensional order and structure in growing biofilms. Nat. Phys. 15, 251–256. doi: 10.1038/s41567-018-0356-9
Hendry, E. R., Worthington, T., Conway, B. R., and Lambert, P. A. (2009). Antimicrobial efficacy of eucalyptus oil and 1,8-cineole alone and in combination with chlorhexidine digluconate against microorganisms grown in planktonic and biofilm cultures. J. Antim. Chemoth. 64, 1219–1225. doi: 10.1093/jac/dkp362
Henry-Stanley, M. J., Hess, D. J., Barnes, A. M. T., Dunny, G. M., Carol, L., and Wells, C. L. (2010). Bacterial contamination of surgical suture resembles a biofilm. Surg. Infect. (Larchmt) 11, 433–439. doi: 10.1089/sur.2010.006
Heras, B., Scanlon, M. J., and Martin, J. L. (2015). Targeting virulence not viability in the search for future antibacterials. Br. J. Pharmacol. 79, 208–215. doi: 10.1111/bcp.12356
Hood, J. R., Burton, D. M., Wilkinson, J. M., and Cavanagh, H. M. A. (2010). The effect of Leptospermum petersonii essential oil on Candida albicans and Aspergillus fumigatus. Med. Mycol. 48, 922–931. doi: 10.3109/13693781003774697
Hosseini Nezhad, M., Alamshahi, L., and Panjehkeh, N. (2012). Biocontrol efficiency of medicinal plants against Pectobacterium carotovorum, Ralstonia solanacearum and Escherichia coli. Open Conf. Proc. J. 3, 46–51. doi: 10.2174/1876326x01203020046
Huth, M. A., Huth, A., and Koch, K. (2018). Morphological diversity of β-diketone wax tubules on Eucalyptus gunnii leaves and real time observation of self-healing of defects in the wax layer. Aust. J. Bot. 66, 313–324.
Imatomi, M., Novaes, P., Matos, A. P., Gualtieri, S. C., Molinillo, J. M., Lacret, R., et al. (2013). Phytotoxic effect of bioactive compounds isolated from Myrcia tomentosa (Myrtaceae) leaves. Biochem. Syst. Ecol. 46, 29–35. doi: 10.1016/j.bse.2012.09.005
Jennings, W., and Shibamoto, T. (1980). Qualitative Analysis of Flavour and Fragrance Volatiles by Glass Capillary Gas Chromatography. New York, NY: Academic Press.
Johnson, C. T. (1980). The leaf anatomy of Leptospermum Forst. (Myrtaceae). Aust. J. Bot. 28, 77–104.
Junka, A., Żywicka, A., Chodaczek, G., Dziadas, M., Czajkowska, J., Duda-Madej, A., et al. (2019). Potential of biocellulose carrier impregnated with essential oils to fight against bioflms formed on hydroxyapatite. Sci. Rep 9:1256. doi: 10.1038/s41598-018-37628-x
Kairo, S. K., Bedwell, J., Tyler, P. C., Carter, A., and Corbel, M. J. (1999). Development of a tetrazolium salt assay for rapid determination of viability of BCG vaccines. Vaccine 17, 2423–2428. doi: 10.1016/s0264-410x(99)00023-7
Karpanen, T. J., Worthington, T., Hendry, E. R., Conway, B. R., and Lambert, P. A. (2008). Antimicrobial efficacy of chlorhexidine digluconate alone and in combination with eucalyptus oil, tea tree oil and thymol against planktonic and biofilm cultures of Staphylococcus epidermidis. J Antimicrob. Chemother. 62, 1031–1036. doi: 10.1093/jac/dkn325
Kim, E., and Park, I. K. (2012). Fumigant antifungal activity of Myrtaceae essential oils and constituents from Leptospermum petersonii against three Aspergillus species. Molecules. 17, 10459–10469. doi: 10.3390/molecules170910459
Kordali, S., Usanmaz, A., Cakir, A., Komaki, A., and Ercisli, S. (2016). Antifungal and herbicidal effects of fruit essential oils of four Myrtus communis genotypes. Chem. Biodivers. 13, 77–84. doi: 10.1002/cbdv.201500018
Kubheka, G. C., Coutinho, T. A., Moleleki, N., and Moleleki, L. N. (2013). Colonization patterns of an mCherry-tagged Pectobacterium carotovorum subsp. brasiliense strain in potato plants. Phytopathology 103, 1268–1279. doi: 10.1094/PHYTO-02-13-0049-R
Lee, B. H., Annis, P. C., Tumaalii, F., and Choi, W. S. (2004). Fumigant toxicity of essential oils from the Myrtaceae family and 1,8-cineole against 3 major stored-grain insects. J. Stored Prod. Res. 40, 553–564. doi: 10.1016/j.jspr.2003.09.001
Lis-Balchin, M., Deans, S., and Hart, S. (1996). Bioactivity of New Zealand medicinal plant essential oils. Acta Hortic. 426, 13–30. doi: 10.17660/actahortic.1996.426.1
Lis-Balchin, M., and Deans, S. G. (1997). Bioactivity of selected plant essential oils against Listeria monocytogenes. J. Appl. Bacteriol. 82, 759–762. doi: 10.1046/j.1365-2672.1997.00153.x
Lis-Balchin, M., Hart, S. L., and Deans, S. G. (2000). Pharmacological and antimicrobial studies on different tea-tree oils (Melaleuca alternifolia, Leptospermum scoparium or manuka and Kunzeaericoidesor kanuka), originating in Australia and New Zealand. Phytother. Res. 14, 623–629. doi: 10.1002/1099-1573(200012)14:8<623::aid-ptr763>3.0.co;2-z
Lucia, A., Licastro, S., Zerba, E., and Masuh, H. (2008). Yield, chemical composition, and bioactivity of essential oils from 12 species of Eucalyptus on Aedes aegypti larvae. Entomol. Exp. Appl. 129, 107–114. doi: 10.1111/j.1570-7458.2008.00757.x
Mabberley, D. J. (1997). The Plant-Book: A Portable Dictionary of the Vascular Plants. Cambridge: Cambridge University Press.
MacRae, J. M., Dipchand, C., Matthew Oliver, M., Moist, L., Yilmaz, S., Lok, C., et al. (2016). Arteriovenous access infection, neuropathy, and other complications. Can. J. Kidney Health Dis. 3, 1–13. doi: 10.1177/2054358116669127
Mehrsorosh, H., Gavanji, S., Larki, B., Mohammadi, M. D., Karbasiun, A., Bakhtari, A., et al. (2014). Essential oil composition and antimicrobial screening of some Iranian herbal plants on Pectobacterium carotovorum. Glob. NEST J. 16, 240–251. doi: 10.30955/gnj.001205
Merghni, A., Noumi, E., Hadded, O., Dridi, N., Panwar, H., Ceylan, O., et al. (2018). Assessment of the antibiofilm and antiquorum sensing activities of Eucalyptus globulus essential oil and its main component 1,8-cineole against methicillin-resistant Staphylococcus aureus strains. Microb. Pathog. 118, 74–80. doi: 10.1016/j.micpath.2018.03.006
Migacz, I. P., Raeski, P. A., Paes de Almeida, V., Raman, V., Nisgoski, S., Bolzón de Muniz, G. I., et al. (2018). Comparative leaf morpho-anatomy of six species of Eucalyptus cultivated in Brazil. Rev. Bras. Farmacogn. 28, 273–281. doi: 10.1016/j.bjp.2018.04.006
Murugan, K., Selvanayaki, K., and Al-Sohaibani, S. (2016). Urinary catheter indwelling clinical pathogen biofilm formation, exopolysaccharide characterization and their growth influencing parameters Saudi. J. Biol. Sci. 23, 150–159. doi: 10.1016/j.sjbs.2015.04.016
Nazzaro, F., Fratianni, F., De Martino, L., Coppola, R., and De Feo, V. (2013). Effect of essential oils on pathogenic bacteria. Pharmaceuticals 6, 1451–1474. doi: 10.3390/ph6121451
O’Toole, G. A., and Kolter, R. (1998). Flagellar and twitching motility are necessary for Pseudomonas aeruginosa biofilm development. Mol. Microbiol. 30, 295–304. doi: 10.1046/j.1365-2958.1998.01062.x
Park, H. M., Kim, J., Chang, K. S., Kim, B. S., Yang, Y. J., Kim, G. H., et al. (2011). Larvicidal activity of Myrtaceae essential oils and their components against Aedes aetypti, acutetoxicity on Daphnia magna, and aqueous residue. J. Med. Entomol. 48, 405–410. doi: 10.1603/me10108
Purwatiningsih, Heather, N. E., and Hassan, E. (2012). Efficacy of Leptospermum petersonii oil, on Plutella xylostella, and its parasitoid, Trichogramma pretiosum. J. Econ. Entom. 105, 1379–1384. doi: 10.1603/ec11382
Quatrin, P. M., Verdi, C. M., de Souza, M. E., de Godoi, S. N., Klein, B., Gundel, A., et al. (2017). Antimicrobial and antibiofilm activities of nanoemulsions containing Eucalyptus globulus oil against Pseudomonas aeruginosa and Candida spp. Microb. Pathog. 112, 230–242. doi: 10.1016/j.micpath.2017.09.062
Ramezani, S., Saharkhiz, M. J., Ramezani, F., and Fotokian, M. H. (2008). Use of essential oils as bioherbicides. J. Essent. Oil Bear.Plants 1, 319–327. doi: 10.1080/0972060X.2008.10643636
Riley, M. (1994). Mâori Healing and Herbal: New Zealand Ethnobotanical Sourcebook. Paraparaumu: Viking Seven seas.
Rolim de Almeida, L. F., Fernando Frei, F., Mancini, E., De Martino, L., and De Feo, V. (2010). Phytotoxic activities of mediterranean essential oils. Molecules 15, 4309–4323. doi: 10.3390/molecules15064309
Salehi, B., Sharifi-Rad, J., Quispe, C., Llaique, H., Villalobos, M., Smeriglio, A. et al. (2019). Insights into Eucalyptus genus chemical constituents, biological activities and health-promoting effects. Trends Food Sci. Technol. 91, 609–624. doi: 10.1016/j.tifs.2019.08.003
Salem, M. Z. M., Ashmawy, A. N., Elansary, H. O., and El-Settawy, A. A. (2015). Chemotyping of diverse Eucalyptus species grown in Egypt and antioxidant and antibacterial activities of its respective essential oils. Nat. Prod. Res. 29, 681–685. doi: 10.1080/14786419.2014.981539
Sambyal, S. S., Sharma, P., and Shrivastava, D. (2017). Anti-biofilm activity of selected plant essential oils against Pseudomonas aeruginosa and Staphylococcus aureus. Int. J. Curr. Microbiol. Appl. Sci. 6, 444–450. doi: 10.20546/ijcmas.2017.603.051
Sarker, S. D., Nahar, L., and Kumarasamy, Y. (2007). Microtitre plate-based antibacterial assay incorporating resazurin as an indicator of cell growth, and its application in the in vitro antibacterial screening of phytochemicals. Methods 42, 321–324. doi: 10.1016/j.ymeth.2007.01.006
Sebei, K., Sakouhi, F., Herchi, W., Larbi Khouja, M., and Boukhchina, S. (2015). Chemical composition and antibacterial activities of seven Eucalyptus species essential oils leaves. Biol. Res. 48:7. doi: 10.1186/0717-6287-48-7
Shepherd, T., and Griffiths, D. W. (2006). The effects of stress on plant cuticular waxes. New Phytol. 171, 469–499. doi: 10.1111/j.1469-8137.2006.01826.x
Shirdashtzadeh, M., Chandrasena, G. I., Henry, R., and McCarthy, D. T. (2017). Plants that can kill; improving E. coli removal in stormwater treatment systems using Australian plants with antibacterial activity. Ecol. Eng. 107, 120–125. doi: 10.1016/j.ecoleng.2017.07.009
Van Vuuren, S. F., Docrat, Y., Kamatou, G. P. P., and Viljoen, A. M. (2014). Essential oil composition and antimicrobial interactions of understudied tea tree species. S. Afr. J. Bot. 92, 7–14. doi: 10.1016/j.sajb.2014.01.005
Wiley (1998). The Wiley Registry of Mass Spectral Data, with NIST Spectral Data CD Rom. New York, NY: John Wiley & Sons.
Windsor, S. A. M., and Brooks, P. (2012). Essential oils from Leptospermums of the sunshine coast and northern rivers regions. Chem. Cent. J. 6:38. doi: 10.1186/1752-153X-6-38
Keywords: Myrtaceae, essential oil, phytochemical profile, antibacterial activity, biofilm, phytotoxic activity
Citation: Caputo L, Smeriglio A, Trombetta D, Cornara L, Trevena G, Valussi M, Fratianni F, De Feo V and Nazzaro F (2020) Chemical Composition and Biological Activities of the Essential Oils of Leptospermum petersonii and Eucalyptus gunnii. Front. Microbiol. 11:409. doi: 10.3389/fmicb.2020.00409
Received: 07 December 2019; Accepted: 27 February 2020;
Published: 15 April 2020.
Edited by:
Fatih Ozogul, Çukurova University, TurkeyReviewed by:
Abderrahmane Houicher, University of Laghouat, AlgeriaAbdelkader Bensid, Ziane Achour University of Djelfa, Algeria
Nariman El Abed, National Institute of Applied Science and Technology, Tunisia
Copyright © 2020 Caputo, Smeriglio, Trombetta, Cornara, Trevena, Valussi, Fratianni, De Feo and Nazzaro. This is an open-access article distributed under the terms of the Creative Commons Attribution License (CC BY). The use, distribution or reproduction in other forums is permitted, provided the original author(s) and the copyright owner(s) are credited and that the original publication in this journal is cited, in accordance with accepted academic practice. No use, distribution or reproduction is permitted which does not comply with these terms.
*Correspondence: Vincenzo De Feo, defeo@unisa.it