- 1Department of Food Science and Technology, Kaunas University of Technology, Kaunas, Lithuania
- 2Food Institute, Kaunas University of Technology, Kaunas, Lithuania
- 3Institute of Animal Rearing Technologies, Lithuanian University of Health Sciences, Kaunas, Lithuania
- 4Department of Food Safety and Quality, Lithuanian University of Health Sciences, Kaunas, Lithuania
- 5Department of Pharmacy, University of Napoli Federico II, Naples, Italy
Aspergillus spp. are widely occurring fungi in nature; they produce toxic compounds such as aflatoxins (AFs) and mainly target plant products such as corn and nuts. The development of prevention strategies is challenging because AFs are highly toxic and have been regulated to small concentrations. This study proposes a new strategy of AF prevention through the application of rapid methods using acoustic techniques in combination with fermentation for the elimination of contaminated corn from bioethanol production processes. An acoustic device was used for the analysis of model systems consisting of corn and nuts (hazelnuts and peanuts) contaminated with different amounts of AFs. High correlations were obtained between penetrated acoustic signal amplitude (Ap) and corn sample density, and between Ap and AF content. Also, relationships were found between changes in Ap values and AF contamination in the nuts model systems. The results of biotreatment of contaminated corn during bioethanol production confirmed that AFs cannot be completely eliminated in dried distiller’s grains with solubles, a valuable by-product for animal feed. Microbially, contamination of the raw material has a negative impact on bioethanol quality by increasing the content of volatile compounds. Therefore, the application of methods such as acoustic screening is a promising alternative for rapid AF detection in corn and nuts (it can handle multi-layers of grain). With the application of acoustic techniques, the prevention of AFs in contaminated raw plant materials could be achieved.
Introduction
Aspergillus flavus and A. parasiticus are one of the most common fungal strains in the agricultural sector, producing the aflatoxins (AFS) AFB1 and AFB2, and AFG1 and AFG2, which are chemically related to bisfuranocoumarin (Udomkun et al., 2017; He et al., 2018) and found worldwide in soil, air, and plants (Bandyopadhyay et al., 2016; Rushing and Selim, 2019). Aflatoxins are potential carcinogens that frequently contaminate food raw materials such as corn, cottonseed, peanuts, and some tree nuts in high concentrations, representing a high risk to food and feed chains and lowering the nutritional quality (Santini et al., 2009; Mikusova et al., 2010; Ritieni et al., 2010; Weaver et al., 2015; Moretti et al., 2019). Therefore, for the food and feed industry to avoid economical losses, a major task is to control the mycotoxin contamination levels in the end products. The prevention of fungal contamination and the development of methods for the decontamination of foods from mycotoxins are important strategies to protect human and animal health (Ehrlich, 2014; Reinholds et al., 2016; Ismail et al., 2018; Pankaj et al., 2018; Mwakinyali et al., 2019).
The common practice to determine mycotoxins is to use laboratory- and time-intensive fundamental chemical, physical, and enzyme immunoassay analyses (Santos Pereira et al., 2019). Considering the fact that these mycotoxin detection methods are complex and expensive, special attention should be given to innovative mycotoxin determination technology, that will allow quick and cheap detection of mycotoxins in the raw materials. Fungal infection not only results in the accumulation of mycotoxins, but also causes grains to shrivel and become more porous. This phenomenon is known as head blight or scab, one of the indicators of poor wheat grain quality (Juodeikiene et al., 2011; Ropelewska et al., 2019; Zhang and Ji, 2019). Due to changes in grain microstructure a rapid and non-destructive method to evaluate the quality and safety of grains is therefore required to detect and subsequently eliminate these toxins from the food chain. The first portable acoustic device equipped with a wide-range capacity ultrasonic transducer was developed at Kaunas University of Technology (Lithuania) during the implementation of the EUREKA ITEA2 project ACOUSTICS for the prediction of deoxynivalenol (DON) contamination levels in wheat grains. Project results showed that the acoustics method, applied for the first time to grain safety monitoring, is innovative and important in ensuring the safety of grains (ITEA 2 Magazine, 2013; Juodeikiene et al., 2014b). However, until now, no studies of the influence of Aspergillus spp. and their metabolites on corn grain and nut microstructure, their technological properties and the use of an acoustic method for the detection of AFS, have been performed.
To avoid the detrimental effects of feed and food contaminated by AFs, not only prevention of contamination but also decontamination of toxic compounds during processing should be applied (Taheur et al., 2019). Fungal infection not only results in the accumulation of AFs, but due to contamination corn raw materials are no longer available for food or feed consumption and can be used as biomass. One of the possible applications of corn biomass is for the production of bioethanol. It is known that fermentation processes could eliminate grain contamination, with the possibility of using the by-products obtained as feed for cattle (Čolović et al., 2019). For this reason, the degradation or decontamination of mycotoxins using appropriate biological microorganisms have been used in the last decade (Juodeikiene et al., 2012; Oliveira et al., 2013; Peles et al., 2019). Recently, the novel aspects of the biological detoxification of mycotoxins (Vila-Donat et al., 2018) included a strategy that relies on mycotoxin inactivation or transformation to non-toxic products by applying low-cost and economically feasible decontamination technologies, retaining the nutritive quality of feed or food, remaining palatable, and not changing significantly the physical properties of the raw material (Haque et al., 2020). A number of microbial species of bacterial and fungal origin have shown the capability to degrade mycotoxins via sorption/enzymatic degradation (Risa et al., 2017; Wang et al., 2018, 2019).
In the present study, the efficiency of corn biomass bioprocessing was explored by using a multi-step prevention system: in the first step, acoustic screening of grains with the elimination of contaminated corn from the production chain was used, and in the second one, a detoxification approach (e.g., fermentation with selected bio-tools) for bioethanol production was applied.
Materials and Methods
Plant Material
Corn Samples
Uncontaminated corn grains and grains artificially infected with Aspergillus flavus with a high level of total AFs (59.2 μg/kg) were obtained from the USDA (United States). The corn model systems were prepared by mixing the uncontaminated corn kernels with 0, 10, 20, 30, 40, 50, 60, 70, 80, 90, and 100% of contaminated kernels.
Nut Samples
Additionally, this study analyzed different nuts (peanuts and hazelnuts) obtained from a Lithuanian supermarket. The two model systems (peanuts and hazelnuts) were prepared by mixing whole-appearance nuts (peanuts and hazelnuts) with mold-damaged nuts (0, 10, 20, 30, 40, 50, 60, 70, 80, 90, and 100%). The infected nuts were smaller and more shriveled and were selected according to their degree of shriveling.
Methods of Analysis
Determination of Chemical Composition and the Qualitative Characteristics of Grain or Nuts
Humidity was determined by the weight loss on drying of the grain or nuts (130 ± 3°C) to constant weight [AACC method 44-15 (2000)].
The bulk density of the corn or nuts (ρ, kg/m3) was calculated using the equation ρ = G/V, where G is mass and V is volume.
Grain Microstructure Evaluation
Cross-sectional images of infected and healthy grains were taken by scanning electron microscope EVO 50 (LEO Electron Microscopy Ltd., Cambridge, United Kingdom) equipped with a second electron (SE) detector.
Microbiological Contamination of Nuts
Microbiological tests on both types of nuts were carried out using five mixtures: (1) 100% whole nuts, (2) 75% whole nuts + 25% contaminated nuts, (3) 50% whole nuts + 50% contaminated nuts, (4) 25% whole nuts + 75% contaminated nuts, and (5) 100% infected nuts. The total numbers of aerobic microorganisms in the mixtures were determined using the plate-count agar (CM0325, Oxoid, United Kingdom). Nuts (10 g) were mixed with 100 ml of distilled water, and after serial dilution, the obtained homogenate was mixed with the agar medium and incubated at 24°C for 5 days in aerobic conditions. The count of microorganisms was expressed in CFU (colony-forming units) per gram of nuts.
Acoustic Technique
Samples of the corn grain, corn grain model system, and different nuts model systems were screened using a recently developed portable acoustic spectrometer (Figure 1) with penetration (Juodeikiene et al., 2014b).
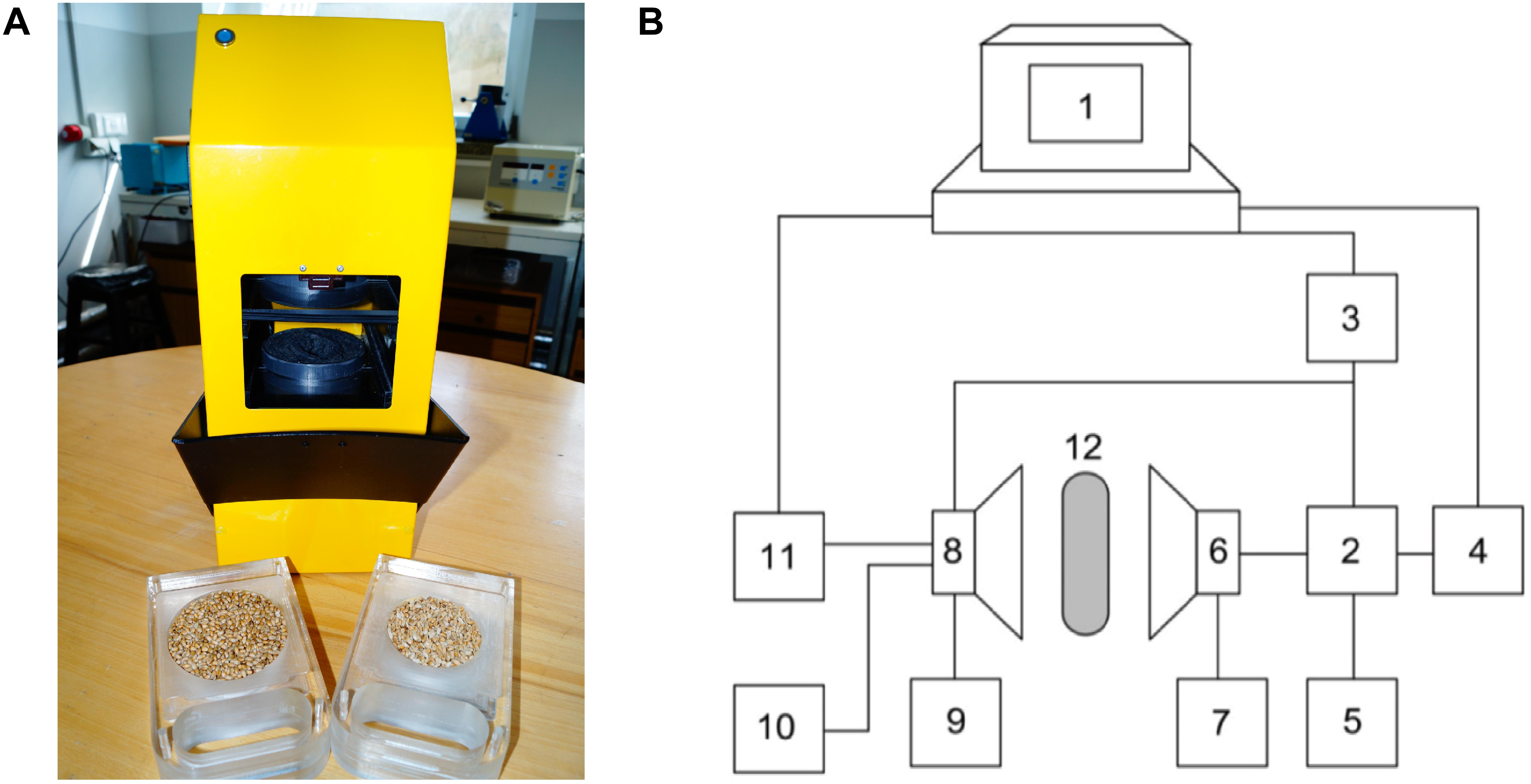
Figure 1. The acoustic spectrometer (A) and its schematic drawing (B) (1 – computer; 2 – sine-wave generator; 3 – pulse generator; 4 – frequency converter; 5 – frequency meter; 6 – transmitting acoustic aerial; 7 – power supply; 8 – receiving acoustic aerial; 9 – power supply; 10 – oscilloscope; 11 – digital voltmeter; 12 – grain seeds sample under test).
The spectrometer measures in relative units the amplitude of the acoustic signal (Ap) that penetrates the sample matrix over the frequency range 10–80 kHz. The 15–40 kHz interval was selected as the optimum frequency range. The duration of each measurement was ∼10 s. The test was carried out by placing the test portion of 200 g of sample into a plastic vessel whose base was covered with sound-transmitting material. The thickness of the sample layer was 50 mm and diameter 80 mm.
Determination of AFs
The quantitative analysis of the total AFs (B1, B2, G1, and G2) in corn samples and corn samples after bioethanol production (the stillage obtained after drying at 50°C for 24 h) was performed by a competitive enzyme linked immunosorbent assay (ELISA) according to the total AF test (AgraQuant®, Romer Labs Ltd., Germany) procedure. The ground test sample amount used in the ELISA assay was 100 g. Mycotoxin extraction and testing was carried out according to the manufacturer’s instructions.
Acidity analysis of fermented broth in bioethanol production was performed according to our previous study (Juodeikiene et al., 2012). The concentration of ethanol was determined using direct distillation and pycnometry.
Volatile compound determination was completed by gas chromatography (GC). Corn samples with different contamination levels were used for bioethanol production (Table 1). The bioethanol production was performed by using the low-temperature process according to Juodeikiene et al. (2014a). A Hewlett-Packard 5890 gas chromatograph equipped with an FID detector was used for the quantitative analysis of volatile compounds as described by Juodeikiene et al. (2014a).
Results and Discussion
The Changes in the Microstructural Composition and Microbiological Contamination of Corn Grains and Nuts Damaged by Aspergillus spp.
Microscopic analysis of the grains contaminated by Aspergillus and the wholesome corn shows visible damage on the surface of the contaminated grain kernels (Figure 2B) and shows what happens to the structure of the grain kernels when attacked by Aspergillus spp. In Figure 2A, the structure of the grain kernel walls is healthy and wholesome; in Figure 2B, the starch granules have been “consumed” by the fungus and a more skeleton-like type of landscape appears. Endosperm cells of healthy kernels (Figure 2A) were filled with regular-shaped starch granules and distributed in the unfolded protein matrix. On the other hand, the endosperm in the Aspergillus-damaged kernels were restructured, fractured, and of irregular form and formed single agglomerates that are influenced by the amylolytic degradation of the starch granules (Figure 2B).
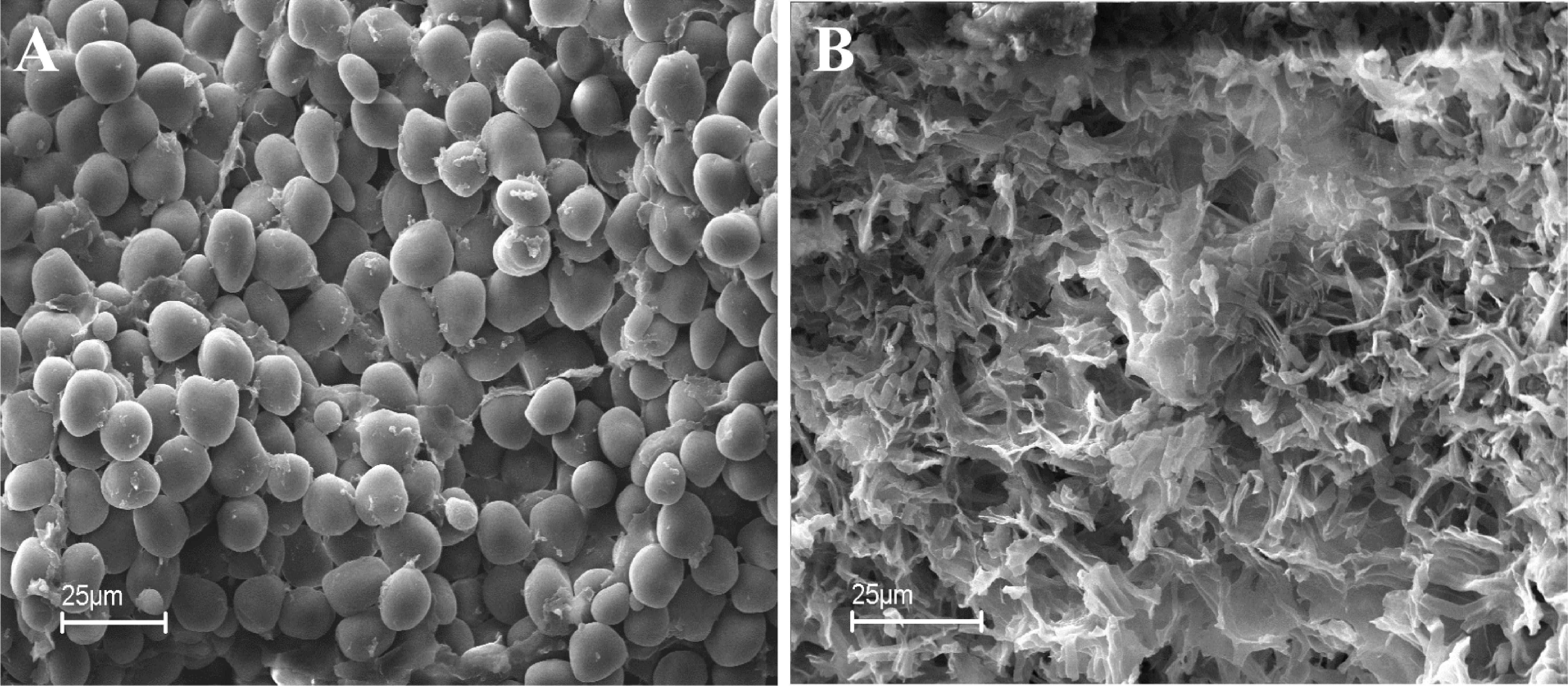
Figure 2. Microscopic view of healthy kernels with intact starch granules (A) and kernels damaged by fungi showing stripped starch granules (B).
Additionally, the microbiological contamination effect was studied on the hazelnut and peanut model systems, which were prepared by mixing visually damaged and contaminated nuts with wholesome ones. Statistical analysis showed a significant positive relationship between the number of scabby hazelnuts (R2 = 0.939, p < 0.05) and peanuts (R2 = 0.874, p < 0.05) in model samples and mold/yeast counts, respectively (Figures 3A,B).
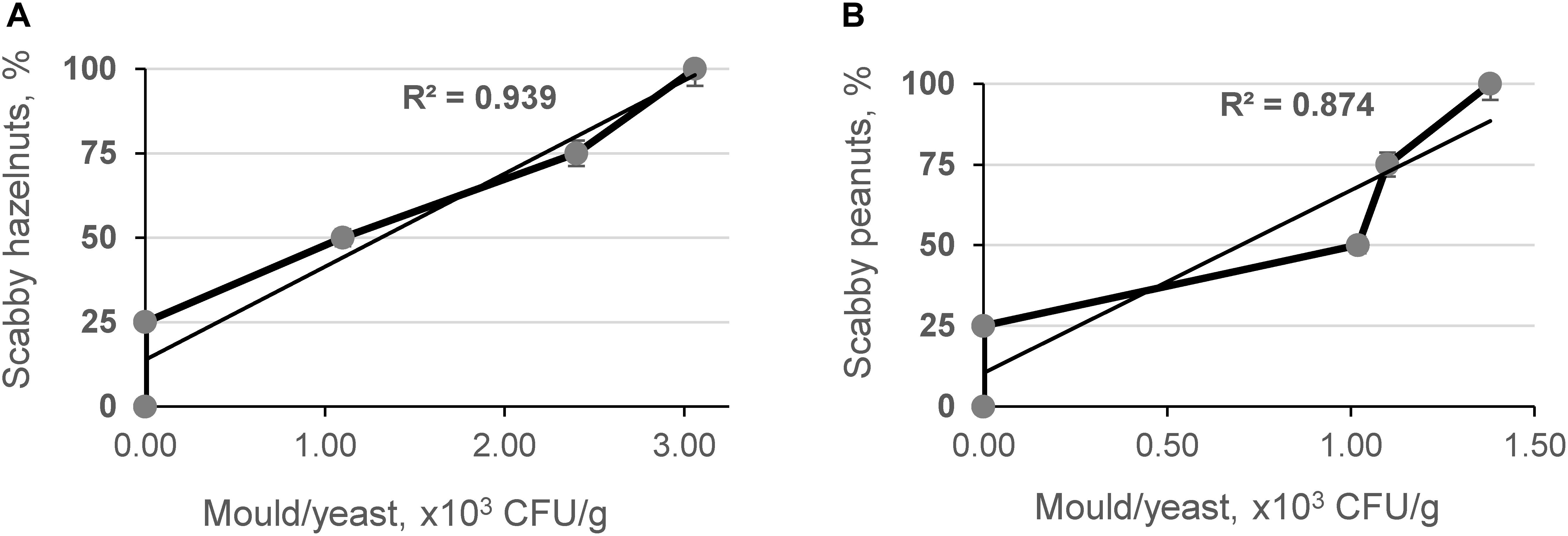
Figure 3. Relationship between content of mold/yeast in scabby hazelnut (A) and peanut (B) model systems.
Fungal infection not only results in the accumulation of mycotoxins, but could also considerably influence the structure and the physical criteria of corn grains and nuts. These changes in the structure influence the porosity of the kernels as well as change the packing factor of kernels in the matrix and could be the basis for the development of a screening method for detection of microbial contamination in this type of raw material.
The Application of the Acoustic Screening Technique for the Detection of Microbiological Contamination in Corn Grains and Nuts Damaged by Aspergillus spp.
The influence of contaminated grains on grain bulk density was studied by determining the relationship between the content of damaged grains and the amplitude of the penetrating (Ap) acoustic signal measured by the acoustic spectrometer (Figure 4). As shown in Figures 4A,C, strong inverse linear relationships were obtained between the number of contaminated corn grains in model samples, the AF content in the samples measured by ELISA [AFL(ELISA)], and the amplitude of the acoustic signal in the model samples (R2 = −0.684 and R2 = −0.679, p < 0.05, respectively). A strong positive correlation was observed between the density of model corn samples and the Ap values recorded using the acoustic spectrometer (R2 = 0.729, p < 0.05) as shown in Figure 4B. Further acoustic analyses were performed with contaminated hazelnut and peanut samples (Figures 5A,B).
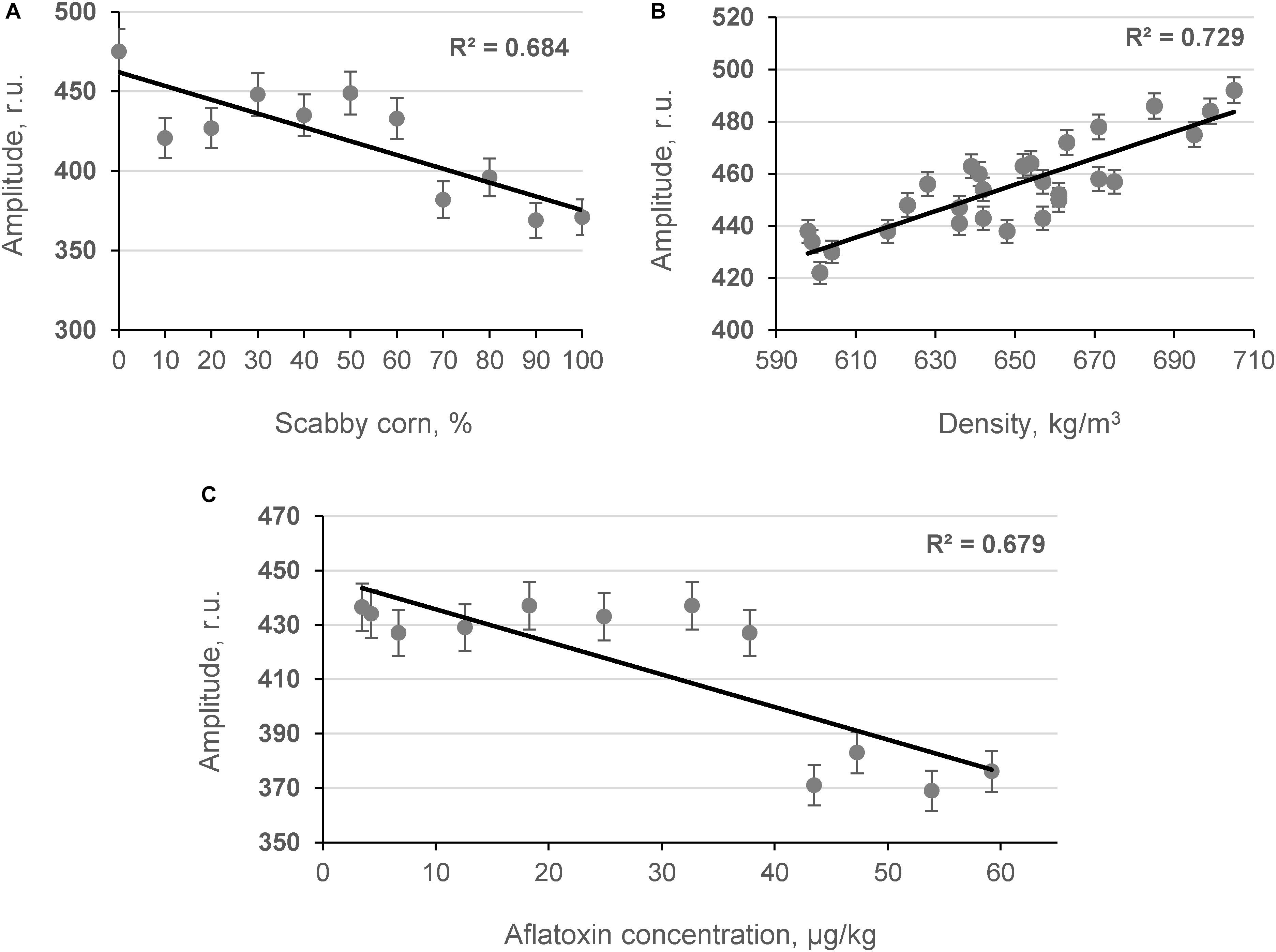
Figure 4. Relationship between content of scabby corn in model systems (A), density of samples (B), AF content in samples (C), and amplitude of the penetrated acoustic signal (Ap, frequency 24.3 kHz; n = 10).
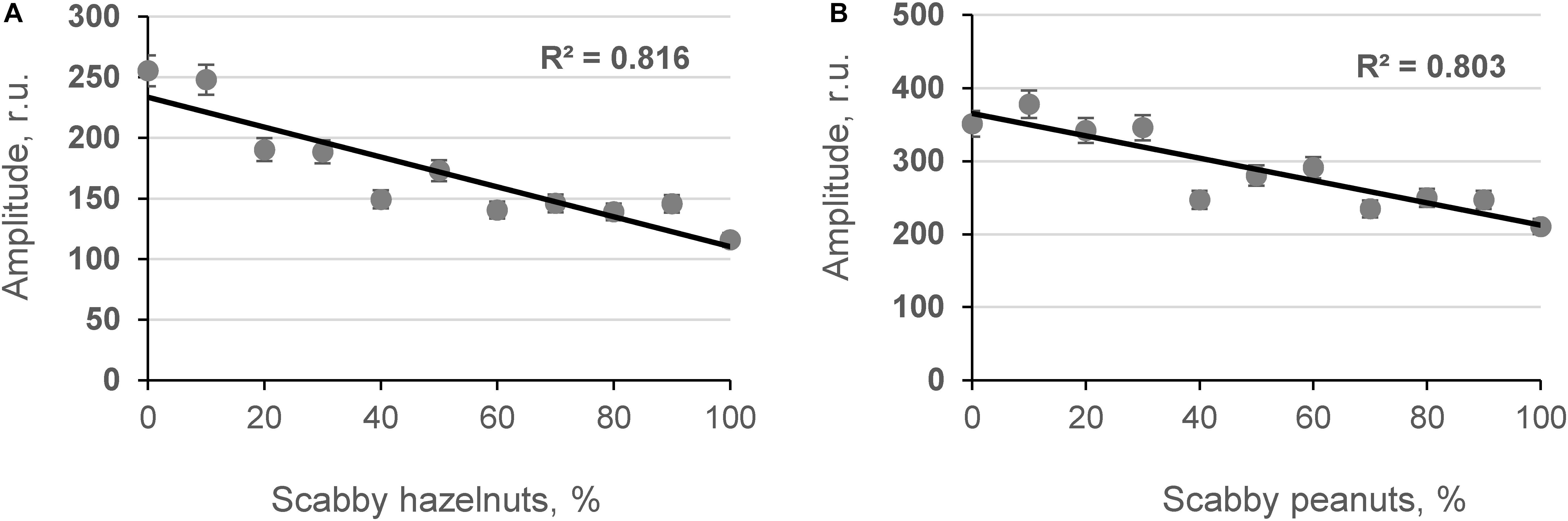
Figure 5. Relationship between content of scabby hazelnuts and peanuts in model systems (A,B, respectively) and amplitude of the penetrated acoustic signal (Ap, frequency 18.1 and 27.0 kHz; n = 10).
Results indicated that as microbiological contamination of nuts increased, the amplitude of the acoustic signal penetrating the nut sample decreased. The obtained results are in agreement with previous studies in which a strong dependence between DON and the content of scabby kernels in wheat matrix was found by Juodeikiene et al. (2008, 2014b) and Tutelyan (2004). The results of the fungal invasion are that the attacked grains shrivel (become scabby in the case of wheat) and become more porous. The same is true for corn kernels affected by A. flavus, although the shriveling is less manifested compared to wheat grains because the pericarp of the corn kernel is sturdier. At the point of harvest, a mixture of wholesome and shriveled grains (or more porous kernels) is seen. The differences between the wholesome (less scabby nuts) and contaminated nuts (more scabby nuts) can also be detected by screening acoustic techniques (Figure 5). Experiments have shown that the acoustic behavior of porous granular materials can be characterized essentially in terms of porosity and airflow resistance (Guo et al., 2019; Pereira et al., 2019). Furthermore, it was found (Guo et al., 2005) that in beads of cereal grains, the absorption of the acoustic signal depends on the size and shape of the particles. Therefore, it is advisable to use developed equipment at point of harvest where one strain of cereal usually dominates (with one particle size and shape).
The Effect of Microbial Contamination by Aspergillus spp. on the Fermentation Processes During Bioethanol Production
The influence of corn biomass being contaminated with AFs at different levels on alcoholic fermentation was evaluated by chromatographic analysis of the yield ethanol and fusel oils.
The process of fermentation of corn contaminated with AFs resulted in a higher organic acid formation (on average 7.03 times) in wort compared to the control sample (Figure 6).
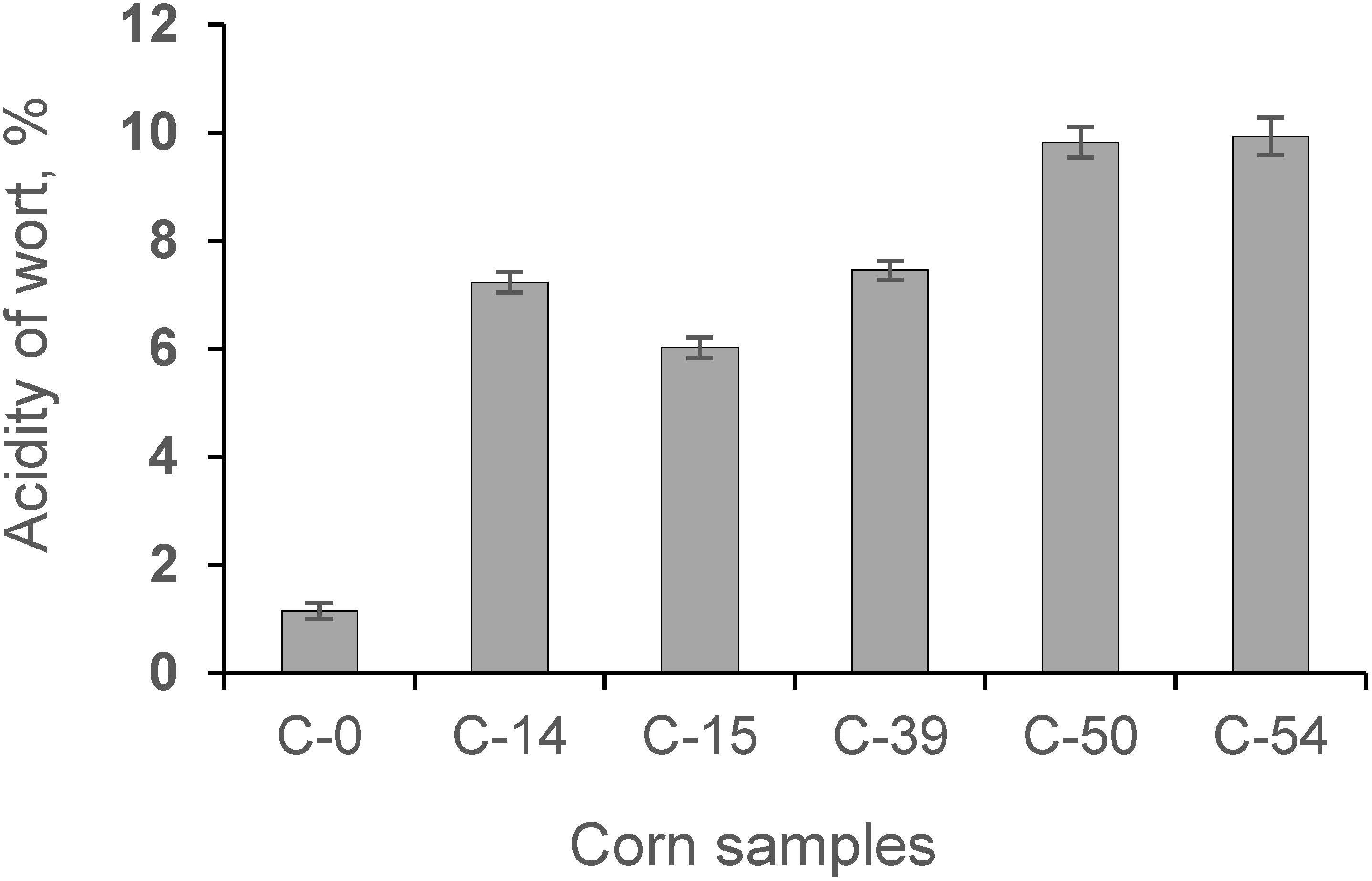
Figure 6. The acidity of wort obtained during fermentation of whole corn grains and contaminated grain samples (C-0, C-14, C-15, C-39, C-50, C-54).
Qualitative and quantitative analysis of bioethanol showed (Figure 7) that fermentation of AF-contaminated corn produced, on average, higher levels of higher alcohols: isoamyl alcohol (35.57%), propyl alcohol (8.63%), and methanol (88.62%). During the experiment, the levels of AF in the infected corn before and after fermentation were examined (Figure 8).
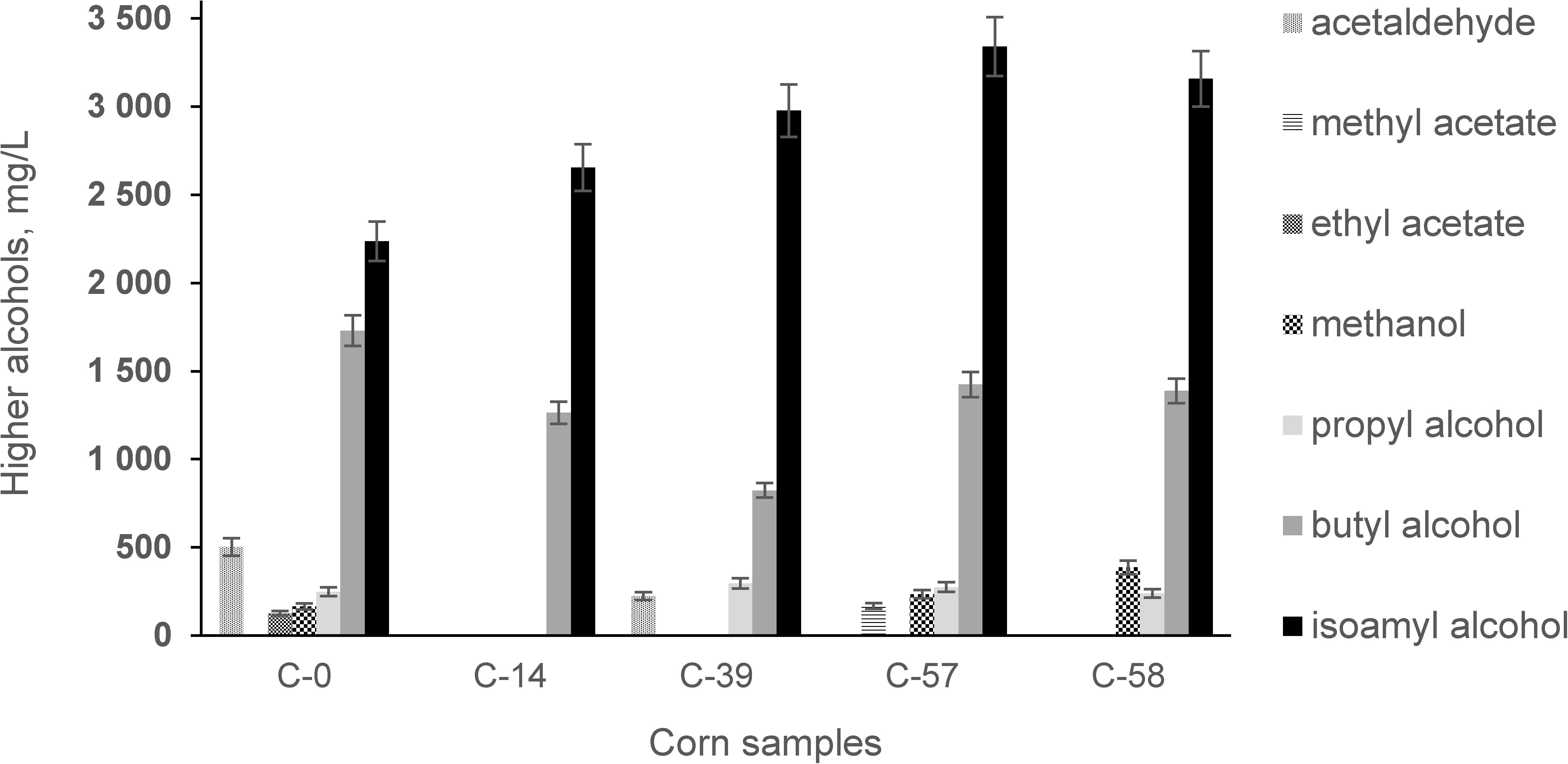
Figure 7. Concentrations of metabolic products in bioethanol during fermentation of corn contaminated with AFs.
Our study showed that contamination of corn with AFs has a negative influence on the fermentation process by increasing the acidity profile (Figure 6) and increasing secondary metabolites in the final product (Figure 7).
However, the positive detoxification effect of fermentation was achieved by reducing the AF content by ∼29,71% in the by-product of ethanol production (DDGS) (Figure 8). DDGS is characterized by its high contents of protein, fiber, and various minerals and vitamins (Chatzifragkou and Charalampopoulos, 2018; Chen et al., 2019), which are valuable as animal nutrition in feed. Increasing supply and demand for DDGS (Wu and Munkvold, 2008) is expected to be driven by increased bioethanol production (Mohanty and Swain, 2019), which will allow DDGS to be used as a renewable source (Kumar and Singh, 2019). There is still no information offering biological tools for the complete elimination of mycotoxins from fermentation media, including raw materials.
The obtained results of the possible biological decontamination are in agreement with other reviewed papers (Mahmood Fashandi et al., 2018; Chiocchetti et al., 2019). Fermentation is influenced by microorganisms occurring naturally in the raw materials or by the addition of starter cultures of microorganisms. Yeasts, such as S. cerevisiae and various lactic acid bacteria (LAB), occur naturally and spontaneously as a natural part of fermentation in the food industry. Detoxification of mycotoxins usually occurs in two stages: sorption and enzymatic degradation of mycotoxins (Perczak et al., 2018; Pereyra et al., 2018).
Conclusion
A portable acoustic penetration spectrometer was applied for high-throughput monitoring of contaminated corn and nuts (hazelnuts and peanuts). Strong correlation coefficients were achieved between acoustic results and corn grain density and AF concentrations in model systems (R2 = 0.729 and −0.684, p < 0.05, respectively). The relationships between the amplitude of the acoustic signal penetrating the samples and AF contamination of hazelnuts and peanuts presented strong relationships (R2 = 0.816 and 0.803, p < 0.05, respectively). Our results show that bioprocesses such as bioethanol production cannot completely eliminate AF contamination of dried distillers’ grains with solubles (AF removal was on average 29.71%). In addition, there is a problem with bioethanol quality (lower ethanol content and more volatile metabolites). Therefore, the development and use of rapid methods, such as the use of broadband capacitive acoustic transducers, are still very attractive solutions for AF prevention. Because of its speed, non-invasive character, and quantification ability, this method is comparable in precision to wet-chemistry methods such as ELISA and is far faster and cheaper per analysis to set up than wet-chemistry methods. It lends itself to the monitoring and high-throughput detection of AFs in corn and nuts and eliminates their contamination of the food chain.
Data Availability Statement
All datasets generated for this study are included in the article.
Author Contributions
GJ, EB, and AS conceived and designed the experiments. GB and KT-R performed the experiments. DC and DZ analyzed the data. GJ and EB contributed the reagents, materials, and analysis tools. GJ, DC, and AS wrote the manuscript. All authors read and approved the final version of manuscript.
Conflict of Interest
The authors declare that the research was conducted in the absence of any commercial or financial relationships that could be construed as a potential conflict of interest.
Acknowledgments
The authors gratefully acknowledge the COST Action CA18101 “SOURDOugh biotechnology network toward novel, healthier and sustainable food and bIoproCesseS” and the EUREKA Network Project E!13309 “SUSFEETECH” (Nr. 01.2.2-MITA-K-702-05-0001).
References
Bandyopadhyay, R., Ortega-Beltran, A., Akande, A., Mutegi, C., Atehnkeng, J., and Kaptoge, L. (2016). Biological control of aflatoxins in Africa: current status and potential challenges in the face of climate changes. Word Mycotoxin. J. 9, 771–789. doi: 10.3920/wmj2016.2130
Chatzifragkou, A., and Charalampopoulos, D. (2018). “Distiller’s dried grains with solubles (DDGS) and intermediate products as starting materials in biorefinery strategies,” in Sustainable Recovery and Reutilization of Cereal Processing By-Products, ed. C. M. Galanakis (Sawston: Woodhead Publishing), 63–86. doi: 10.1016/B978-0-08-102162-0.00003-4
Chen, Y. F., Huang, C. F., Liu, L., Lai, C. H., and Wang, F. L. (2019). Concentration of vitamins in the 13 feed ingredients commonly used in pig diets. Anim. Feed Sci. Technol. 247, 1–8. doi: 10.1016/j.anifeedsci.2018.10.011
Chiocchetti, G. M., Jadán-Piedra, C., Monedero, V., Zúñiga, M., Vélez, D., and Devesa, V. (2019). Use of lactic acid bacteria and yeasts to reduce exposure to chemical food contaminants and toxicity. Crit. Rev. Food Sci. Nutr. 59, 1534–1545. doi: 10.1080/10408398.2017.1421521
Čolović, R., Puvaèa, N., Cheli, F., Avantaggiato, G., Greco, D., Ðuragić, O., et al. (2019). Decontamination of Mycotoxin-contaminated feedstuffs and compound feed. Toxins 11:617. doi: 10.3390/toxins11110617
Ehrlich, K. C. (2014). Non-aflatoxigenic Aspergillus flavus to prevent aflatoxin contamination in crops: advantages and limitations. Front. Microbiol. 5:1–9. doi: 10.3389/fmicb.2014.00050
Guo, M., Ma, Y., Yang, X., and Mankin, R. W. (2019). Detection of damaged wheat kernels using an impact acoustic signal processing technique based on Gaussian modelling and an improved extreme learning machine algorithm. Biosyst. Eng. 184, 37–44. doi: 10.1016/j.biosystemseng.2019.04.022
Guo, M., Shang, Z., and Shi, H. (2005). Sound absorption measurements of various types of grain. Acta Acust. United Acust. 91, 915–919.
Haque, M. A., Wang, Y., Shen, Z., Li, X., Saleemi, M. K., and He, C. (2020). Mycotoxin contamination and control strategy in human, domestic animal and poultry: a review. Microb. Pathog. 142:104095. doi: 10.1016/j.micpath.2020.104095
He, Y., Wang, B., Chen, W., Cox, R. J., He, J., and Chen, F. (2018). Recent advances in reconstructing microbial secondary metabolites biosynthesis in Aspergillus spp. Biotechnol. Adv. 36, 739–783. doi: 10.1016/j.biotechadv.2018.02.001
Ismail, A., Gonçalves, B. L., de Neeff, D. V., Ponzilacqua, B., Coppa, C. F. S. C., Hintzsche, H., et al. (2018). Aflatoxin in foodstuffs: occurrence and recent advances in decontamination. Food Res. Int. 113, 74–85. doi: 10.1016/j.foodres.2018.06.067
ITEA 2 Magazine (2013). First Acoustic Equipment for Screening Mycotoxins in Cereals September. Available at: itea3.org/publication/download/919-itea-magazine-16-web-pdf.pdf (accessed September, 2013).
Juodeikiene, G., Basinskiene, L., Bartkiene, E., and Matusevicius, P. (2012). Mycotoxin decontamination aspects in food, feed and renewables using fermentation processes. INTECH 8, 171–204. doi: 10.5772/46184
Juodeikiene, G., Basinskienë, L., Vidmantiene, D., Bartkiene, E., Bakutis, B., and Baliukoniene, V. (2011). Acoustic sensing of deoxynivalenol in co-occurrence with zearalenone and T-2/HT-2 toxin in winter wheat cultivar Sirvinta from Lithuania. World Mycotoxin J. 4, 395–404. doi: 10.3920/WMJ2011.1297
Juodeikiene, G., Basinskiene, L., Vidmantiene, D., Bartkiene, E., Kunigelis, V., and de Koe, W. J. (2008). Rapid acoustic screening of deoxynivalenol (DON) in grain. World Mycotoxin J. 1, 267–274. doi: 10.3920/WMJ2008.1018
Juodeikiene, G., Cernauskas, D., Vidmantiene, D., Basinskiene, L., Bartkiene, E., Bakutis, B., et al. (2014a). Combined fermentation for increasing efficiency of bioethanol production from Fusarium sp. contaminated barley biomass. Catalysis Today 223, 108–114. doi: 10.1016/j.cattod.2013.09.028
Juodeikiene, G., Vidmantiene, D., Basinskiene, L., Cernauskas, D., Klupsaite, D., Bartkiene, E., et al. (2014b). Recent advances in the rapid acoustic screening of deoxynivalenol in wheat grains. World Mycotoxin J. 7, 517–525. doi: 10.3920/WMJ2013.1677
Kumar, D., and Singh, V. (2019). “Bioethanol production from corn,” in Corn, ed. G. Gibbons (Arnold, MA: AACC International Press), 615–631. doi: 10.1016/B978-0-12-811971-6.00022-X
Mahmood Fashandi, H., Abbasi, R., and Mousavi Khaneghah, A. (2018). The detoxification of aflatoxin M1 by Lactobacillus acidophilus and Bifidobacterium spp.: a review. J. Food Process. Preserv. 42:13704. doi: 10.1111/jfpp.13704
Mikušová, P., Ritieni, A., Santini, A., Juhasová, G., and Šrobárová, A. (2010). Contamination by moulds of grape berries in Slovakia. Food Addit. Contamin. Pt. A 27, 738–747. doi: 10.1080/19440040903571754
Mohanty, S. K., and Swain, M. R. (2019). “Bioethanol production from corn and wheat: food, fuel, and future,” in Bioethanol Production from Food Crops, eds C. R. Ramesh and S. Ramachandran (Cambridge, MA: Academic Press), 45–59. doi: 10.1016/B978-0-12-813766-6.00003-5
Moretti, A., Pascale, M., and Logrieco, A. F. (2019). Mycotoxin risks under a climate change scenario in Europe. Trends Food Sci. Technol. 84, 38–40. doi: 10.1016/j.tifs.2018.03.008
Mwakinyali, S. E., Ding, X., Ming, Z., Tong, W., Zhang, Q., and Li, P. (2019). Recent development of aflatoxin contamination biocontrol in agricultural products. Biol. Control 128, 31–39. doi: 10.1016/j.biocontrol.2018.09.012
Oliveira, C. A. F., Bovo, F., Corassin, C. H., Jager, A. V., and Reddy, K. R. (2013). “Recent trends in microbiological decontamination of aflatoxins in foodstuffs,” in INTECH No 4, Aflatoxins - Recent Advances and Future Prospects, ed. M. Razzaghi-Abyaneh (London: IntechOpen), 59–92. doi: 10.5772/51120
Pankaj, S. K., Shi, H., and Keener, K. M. (2018). A review of novel physical and chemical decontamination technologies for aflatoxin in food. Trends Food Sci. Technol. 71, 73–83. doi: 10.1016/j.tifs.2017.11.007
Peles, F., Sipos, P., Gyõri, Z., Pfliegler, W. P., Giacometti, F., Serraino, A., et al. (2019). Adverse effects, transformation and channeling of aflatoxins into food raw materials in livestock. Front. Microbiol. 10:2861. doi: 10.3389/fmicb.2019.02861
Perczak, A., Goliñski, P., Bryła, M., and Waśkiewicz, A. (2018). The efficiency of lactic acid bacteria against pathogenic fungi and mycotoxins. Arch. Indus. Hyg. Toxicol. 69, 32–45. doi: 10.2478/aiht-2018-69-3051
Pereira, M., Carbajo San Martín, J., Godinho, L., Amado-Mendes, P., Mateus, D., and Ramis-Soriano, J. (2019). Acoustic behavior of porous concrete. Characterization by experimental and inversion methods. Mater. Construc. 69:e202. doi: 10.3989/mc.2019.03619
Pereyra, C. M., Gil, S., Cristofolini, A., Bonci, M., Makita, M., Monge, M. P., et al. (2018). The production of yeast cell wall using an agroindustrial waste influences the wall thickness and is implicated on the aflatoxin B1 adsorption process. Food Res. Int. 111, 306–313. doi: 10.1016/j.foodres.2018.05.026
Reinholds, I., Juodeikiene, G., Bartkiene, E., Zadeike, D., Bartkevics, V., Krungleviciute, V., et al. (2016). Evaluation of ozonation as a method for mycotoxins degradation in malting wheat grains. World Mycotoxin J. 9, 409–417. doi: 10.3920/WMJ2015.2011
Risa, A., Divinyi, D. M., Baka, E., and Krifaton, C. (2017). Aflatoxin B1 detoxification by cell-free extracts of rhodococcus strains. Acta Microbiol. Immunol. Hung. 64, 423–438. doi: 10.1556/030.64.2017.023
Ritieni, A., Santini, A., Mussap, M., Ferracane, R., Bosco, P., Gazzolo, D., et al. (2010). Simultaneous determination of mycotoxins in biological fluids by LC-MS/MS. Front. Biosci. 2:151–158. doi: 10.2741/e77
Ropelewska, E., Jurczak, S., Bilska, K., Kulik, T., and Zapotoczny, P. (2019). Correlations between the textural features of wheat kernels and the quantity of DNA of Fusarium fungi. Eur. Food Res. Technol. 245, 1161–1167. doi: 10.1007/s00217-019-03240-0
Rushing, B. R., and Selim, M. I. (2019). Aflatoxin B1: a review on metabolism, toxicity, occurrence in food, occupational exposure, and detoxification methods. Food Chem. Toxicol. 124, 81–100. doi: 10.1016/j.fct.2018.11.047
Santini, A., Ferracane, R., Meca, G., and Ritieni, A. (2009). Overview of analytical methods for beauvericin and fusaproliferin in food matrices. Anal. Bioanal. Chem. 395:1253. doi: 10.1007/s00216-009-3117-x
Santos Pereira, C., Cunha, S., and Fernandes, J. O. (2019). Prevalent mycotoxins in animal feed: occurrence and analytical methods. Toxins 11:290. doi: 10.3390/toxins11050290
Taheur, F. B., Kouidhi, B., Al Qurashi, Y. M. A., Salah-Abbès, J. B., and Chaieb, K. (2019). Biotechnology of mycotoxins detoxification using microorganisms and enzymes. Toxicon 160, 12–22. doi: 10.1016/j.toxicon.2019.02.001
Tutelyan, V. A. (2004). Deoxynivalenol in cereals in Russia. Toxicol. Lett. 153, 173–179. doi: 10.1016/j.toxlet.2004.04.042
Udomkun, P., Wiredu, A. N., Nagle, M., Müller, J., Vanlauwe, B., and Bandyopadhyay, R. (2017). Innovative technologies to manage aflatoxins in foods and feeds and the profitability of application - A review. Food Control 76, 127–138. doi: 10.1016/j.foodcont.2017.01.008
Vila-Donat, P., Marín, S., Sanchis, V., and Ramos, A. J. (2018). A review of the mycotoxin adsorbing agents, with an emphasis on their multi-binding capacity, for animal feed decontamination. Food Chem. Toxicol. 114, 246–259. doi: 10.1016/j.fct.2018.02.044
Wang, L., Wu, J., Liu, Z., Shi, Y., Liu, J., Xu, X., et al. (2019). Aflatoxin B1 degradation and detoxification by Escherichia coli CG1061 isolated from chicken cecum. Front. Pharmacol. 9:1548. doi: 10.3389/fphar.2018.01548
Wang, Y., Zhang, H., Yan, H., Yin, C., Liu, Y., Xu, Q., et al. (2018). Effective biodegradation of aflatoxin B1 using the Bacillus licheniformis (BL010) strain. J. Toxins 10, 2–16. doi: 10.3390/toxins10120497
Weaver, M. A., Abbas, H. K., Falconer, L. L., Allen, T. W., Pringle, H. C., and Sciumbato, G. (2015). Biological control of aflatoxin is effective and economial in Mississippi field trials. Crop Protect. 69, 52–55. doi: 10.1016/j.cropro.2014.12.009
Wu, F., and Munkvold, G. P. (2008). Mycotoxins in ethanol co-products: modelling economic impacts on the livestock industry and management strategies. J. Agric. Food. Chem. 56, 3900–3911. doi: 10.1021/jf072697e
Keywords: Aspergillus spp., corn, nuts, rapid method, aflatoxins screening, acoustic sensors, bioethanol, detoxification
Citation: Juodeikiene G, Cernauskas D, Trakselyte-Rupsiene K, Bartkiene E, Zadeike D, Banyte G and Santini A (2020) Acoustic-Based Screening Method for the Detection of Total Aflatoxin in Corn and Biological Detoxification in Bioethanol Production. Front. Microbiol. 11:543. doi: 10.3389/fmicb.2020.00543
Received: 23 November 2019; Accepted: 12 March 2020;
Published: 15 April 2020.
Edited by:
Mehdi Razzaghi-Abyaneh, Pasteur Institute of Iran (PII), IranReviewed by:
Ebrahim Hadavi, Islamic Azad University Karaj Branch, IranFuguo Xing, Institute of Food Science & Technology (CAAS), China
Copyright © 2020 Juodeikiene, Cernauskas, Trakselyte-Rupsiene, Bartkiene, Zadeike, Banyte and Santini. This is an open-access article distributed under the terms of the Creative Commons Attribution License (CC BY). The use, distribution or reproduction in other forums is permitted, provided the original author(s) and the copyright owner(s) are credited and that the original publication in this journal is cited, in accordance with accepted academic practice. No use, distribution or reproduction is permitted which does not comply with these terms.
*Correspondence: Darius Cernauskas, darius.cernauskas@ktu.lt