- 1Department of Plant Pathology, Agriculture College, Guizhou University, Guiyang, China
- 2Chongqing Key Laboratory of Economic Plant Biotechnology, College of Landscape Architecture and Life Science/Institute of Special Plants, Chongqing University of Arts and Sciences, Chongqing, China
The effect of exogenous mannitol and sorbitol on the viability of the antagonist yeast, Debaryomyces hansenii, when exposed to oxidative and high-temperature stress was determined. Results indicated that both the 0.1 M mannitol (MT) and 0.1 M sorbitol (ST) treatments improved the tolerance of D. hansenii to subsequent oxidative and high-temperature stress. MT or ST cells had a significantly higher level of cell survival, elevated the gene expression of catalase 1 (CAT1) and copper-zinc superoxide dismutase (SOD1), as well as the corresponding enzyme activity. Treated cells also exhibited a lower accumulation of intracellular reactive oxygen species (ROS), and a higher content of intracellular mannitol and sorbitol relative to non-treated, control yeast cells, when exposed to a subsequent oxidative (30 mM H2O2) or heat (40.5°C) stress for 30 min. Additionally, MT and ST yeast exhibited a higher growth rate in kiwifruit wounds, and a greater ability to inhibit postharvest blue mold (Penicillium expansum) and gray mold (Botrytis cinerea) infections. The present study indicates that increased antioxidant response induced by mannitol and sorbitol in D. hansenii can enhance stress tolerance and biocontrol performance.
Introduction
Postharvest fungal decay of fruits reduces their availability and economic return. While the use of synthetic, chemical fungicides remain the main method of controlling fungal decay organisms, the use of antagonistic yeasts as biological control agents has been comprehensively explored over the last three decades (Liu et al., 2013, 2018; Droby et al., 2016; Usall et al., 2016; Wisniewski et al., 2016; Carmona-Hernandez et al., 2019; Ferraz et al., 2019). Among the yeasts identified as potential biocontrol agents, Debaryomyces hansenii has been reported to be effective during storage against several different postharvest decay fungi in a variety of fruits, including apple (Czarnecka et al., 2019), grapefruit (Droby et al., 1989), lime (Hernández-Montiel et al., 2010), muskmelon (Rivas-Garcia et al., 2019) and papaya (Hernandez-Montiel et al., 2018).
Once antagonistic yeasts have been applied to harvested commodities, a range of factors, such as temperature, oxidative stress, solute stress, and pH can influence their viability. Concomitantly, the level of stress tolerance of antagonistic yeasts is closely related to their ability to survive and proliferate on and in host tissues, as well as to their biocontrol efficacy against pathogens (Castoria et al., 2003; Macarisin et al., 2010; Sui et al., 2015; Spadaro and Droby, 2016). Therefore, enhancing yeast stress tolerance may represent a useful strategy for improving the efficacy of antagonistic yeasts (Abadias et al., 2001; Liu et al., 2012; Wang et al., 2018).
Sugar alcohols from a wide range of sources, including sorbitol and mannitol, are widely used for food, pharmaceutical, and other industrial applications. Mannitol, a six-carbon, non-cyclic sugar alcohol, is the most abundant polyol in nature, occurring in bacteria, fungi, algae, lichens, and in at least 70 species of vascular plants. Various functional roles have been postulated for mannitol in these organisms, including carbon storage, free radical scavenging, osmoregulation, and acting as a compatible solute (Seckin et al., 2009). Mannitol has been reported to play an important role in the osmotic protection of celery (Pharr et al., 1995). Mannitol transport and intracellular metabolism is a major aspect of the response of olive trees to osmotic stress (Conde et al., 2011). Sorbitol also plays an important role in the regulation of osmotic pressure in cells. A growth medium amended with sorbitol induces an adaptive osmotic response in nematodes (Chandler-Brown et al., 2015). Sorbitol in pear trees also plays an important role in osmotic adjustments in pear leaves (Larher et al., 2009). Shen et al. (1999) proposed that the accumulation of mannitol and sorbitol in the model yeast, Saccharomyces cerevisiae, may have dual functions, namely, facilitating osmotic adjustment and also contributing to the maintenance of redox homeostasis. Teixidó et al. (1998) improved the ecological fitness and environmental stress tolerance of the biocontrol yeast, Candida sake, by altering the concentration of intracellular sugar alcohols (glycerol, erythritol, arabitol, and mannitol) and sugars. To the best of our knowledge, however, there is little information about the effect of sugar alcohols on the antioxidant response of antagonistic yeast in the biological control of postharvest diseases.
The objective of the present study was to determine the effect of sugar alcohol (mannitol or sorbitol) on the antioxidant response and subsequent stress tolerance and biocontrol efficacy of the biocontrol yeast, D. hansenii. More specifically, the study determined (1) cell viability of mannitol-treated (MT) or sorbitol-treated (ST) cells to a subsequent exposure to oxidative (H2O2) and high-temperature stress; (2) the effect of mannitol or sorbitol treatment on the expression of antioxidant genes, catalase 1 (CAT1) and copper-zinc superoxide dismutase (SOD1), and their corresponding enzyme activity; (3) the intracellular accumulation of reactive oxygen species (ROS); (4) the growth of the yeast in kiwifruit wounds; and (5) the biocontrol efficacy D. hansenii yeast cells pretreated with sorbitol or mannitol against the infection of kiwifruit by blue mold (Penicillium expansum) and gray mold (Botrytis cinerea).
Materials and Methods
Antagonistic Yeast
The antagonistic yeast, D. hansenii M13, was originally isolated from the surface of mango fruit and identified by its general morphology and DNA sequence of the ITS region of ribosomal DNA (Leaw et al., 2006). It was cultured in a yeast-peptone-dextrose (YPD) broth (10 g of yeast extract, 20 g of peptone, and 20 g of dextrose in 1 L of water). Twenty milliliters of YPD was placed in a 50-mL conical flask and inoculated with D. hansenii at an initial concentration of 105 cells/mL determined using a hemocytometer. Yeast cultures were incubated at 25°C on a rotary shaker at 200 r.p.m. for 16 h.
Fungal Pathogens
The fungal pathogens, B. cinerea and P. expansum, were isolated from infected fruit and maintained on potato dextrose agar (PDA) at 4°C. To reactivate the culture and verify its pathogenicity, the pathogens were inoculated into wounds of kiwifruit fruit and re-isolated onto PDA once the infection was established. Spore suspensions of the two pathogens were obtained from 2-week-old PDA cultures incubated at 25°C. The spore number was calculated with a hemocytometer, and the concentration was adjusted to 1 × 104 spores/mL with sterile distilled water.
Fruit
Kiwifruit (Actinidia deliciosa cv. Hayward) were harvested at commercial maturity. The average quality parameters at the time of harvest were: 9.8 Brix, 67 N firmness, and 96 g fresh weight per fruit. Fruits without wounds or rot were selected based on uniformity of size, disinfected with 2% (v/v) sodium hypochlorite for 2 min, rinsed with tap water, and air-dried. These fruits were used in the biocontrol assay.
Mannitol and Sorbitol Treatment of D. hansenii
Yeast cultures were grown overnight and the culture tubes were then centrifuged at 8,000 × g for 3 min. The yeast cells were subsequently washed three times with sterile distilled water to remove any residual medium, centrifuging the cells between each wash (Liu et al., 2012). Washed cells were resuspended in the same volume (20 mL) of fresh YPD, supplemented with mannitol or sorbitol at a final concentration of 0.1 M and incubated at 25°C for 2 h on a rotary shaker at 200 rpm. The selected concentration of mannitol and sorbitol was based on preliminary experiments. Control cells were subjected to the same process, but in a medium that was not supplemented with mannitol or sorbitol. Cells were harvested by centrifugation at 8,000 × g for 3 min and washed three times with sterile distilled water in order to remove any residual medium. The mannitol-treated (MT), sorbitol-treated (ST) and non-treated (NT) control yeast samples were suspended in water at 1 × 107 cells/mL and used in the subsequent analyses.
Effect of Mannitol and Sorbitol on Stress Tolerance of D. hansenii
The effect of polyol pretreatment on tolerance of D. hansenii to oxidative and high-temperature stress was measured as previously described (Deveau et al., 2010), with slight modification. To measure oxidative stress tolerance, a 10 mL sample of MT, ST, or NT (control) yeast at a concentration of 1 × 107 cells/mL was placed in a 50-mL conical flask and exposed to oxidative stress conditions, 30 mM H2O2, at 25°C for 30 min on a rotary shaker at 200 rpm. To assay high temperature tolerance, 1 mL of MT, ST, or NT (control) yeast cells (1 × 107 cells/mL) was placed into several 1.5 mL Eppendorf tubes. The tubes were then placed in a 40.5°C water bath for 30 min, and manually shaken once every 5 min. At designated time points, 50 μL of serial 10-fold dilutions of the samples were spread on YPD agar plates. The plates were incubated at 25°C for 3 days, and then the number of Colony-Forming Units (CFUs) per plate was determined. Survival rates were expressed as a percentage of the number of colonies produced after the oxidative or high-temperature stress treatments relative to the number of CFUs produced from samples prior to each treatment (Liu et al., 2012). Three replicates were used for each treatment, and each experiment was repeated three times.
Measurement of Intracellular ROS
The oxidant-sensitive probe, 2,7-dichlorodihydrofluorescein diacetate (H2DCFDA; Invitrogen, Eugene, OR, United States), was used to assess the intracellular accumulation of ROS in yeast cells (Liu et al., 2011). Yeast cell samples were collected from cultures exposed to 30 mM H2O2 or 40.5°C for 30 min. The cell samples taken prior to exposure to the oxidative or heat stress served as time 0. Yeast cell samples were washed with phosphate buffered saline (PBS) buffer (pH 7.0) and resuspended in the same buffer containing 25 μM H2DCFDA (dissolved in dimethyl sulfoxide). The suspension was incubated in the dark at 30°C for 1 h. After washing twice with PBS buffer, spores were examined under a FV3000 confocal microscope (Olympus, Tokyo, Japan) equipped with a UV-light source using a 488 nm excitation and 510 nm emission filter combination. Ten fields of view from each slide (at least 200 cells) were randomly chosen and the number of cells producing visible levels of ROS was counted. The ROS level was calculated as a percentage (number of fluorescing cells divided by number of cells present in the bright field image × 100). Three biological replicates were used for each sample in each assay, and the experiments were repeated three times.
RNA Isolation and RT-qPCR Analysis of Gene Expression
Total RNA from yeast samples (MT, ST, and NT) was extracted, treated with DNase, and purified using an Ultrapure RNA Kit (CWBIO, Beijing, China) according to the manufacturer’s instructions. RNA quality was evaluated by gel electrophoresis and spectrophotometric analysis (Waltham, MA, United States). First-strand cDNA was synthesized using a TransScript One-Step gDNA Removal and cDNA Synthesis SuperMix kit (TransGen Biotech, Beijing, China). The resulting cDNA was used for RT-qPCR analysis following the manufacturer’s protocol. Briefly, each RT-qPCR reaction was carried out in a 20 μL reaction including 10 μL of GoTaq qPCR Master Mix (Promega, Madison, Wisconsin, United States) and 200 nmol of each gene-specific primer. The RT-qPCR was conducted on a qTOWER 2.2 (Analytik Jena AG, Germany) using the following cycling conditions: 95°C for 30 s; 40 cycles of 95°C for 5 s followed by 58°C for 15 s, 72°C for 10 s. The expression level of two target genes, CAT1 and SOD1, was analyzed using gene-specific primers (Table 1). Transcript levels of 18S rRNA gene served as an internal control. The 2–ΔΔCT method was used to calculate relative expression (Livak and Schmittgen, 2001). To ensure that single products were amplified, each PCR reaction was subjected to a melting curve analysis of the amplification products. PCR products were cloned and sequenced to verify their identity. There were three biological replicates and three technical replicates for each treatment, and the experiment was repeated three times.
Assay of Antioxidant Enzyme Activity
Yeast cell samples were collected from cultures exposed to 40.5°C or 30 mM H2O2 for 30 min. Samples of yeast cells taken prior to exposure to the oxidative or heat stress served as time 0. Yeast cells in the collected samples were disrupted in liquid nitrogen and suspended in chilled potassium phosphate buffer (0.1 M, pH 7.4). The cell homogenate was centrifuged at 10,000 × g for 20 min at 4°C and the supernatant was used for the enzyme assays. The activity of catalase (CAT) and superoxide dismutase (SOD) was measured using a commercial assay kit (Nanjing Jiancheng Bioengineering Institute, Nanjing, China), and expressed as U per mg protein. Protein content was determined using the Bradford assay with bovine serum albumin used to construct a standard curve (Bradford, 1976). One unit of CAT activity was defined as the decomposition of 1 μmol H2O2 per second in the reaction system, while one unit of SOD activity was defined as the amount of enzyme causing 50% inhibition in the nitro blue tetrazolium (NBT) reduction (Reverberi et al., 2005; Liu et al., 2011). Three biological replicates were used for each sample in each assay, and the experiments were repeated three times.
Determination of Intracellular Mannitol and Sorbitol
Intracellular mannitol and sorbitol were extracted as described in a previous study (Hua et al., 2015) with slight modification. Yeast cell samples were collected by centrifugation at 8,000 × g for 3 min and resuspended in HPLC-grade water. The cell samples were then disrupted with the use of a Q125 sonicator (QSonica, Newtown, CT, United States) for 2 min, boiled for 5 min, and then cooled to room temperature. After vortexing, the mixture in each tube was centrifuged at 1,840 × g for 10 min. The supernatant was filtered through a 0.2 μm filter membrane before determination of mannitol and sorbitol levels using an HPLC equipped with a carbohydrate analysis column and a refractive index detector (Agilent Series 1200, Agilent Technologies, Santa Clara, CA, United States). For mannitol, the mobile phase was acetonitrile-water (75:25 in volume) at 1 mL/min (Liu et al., 2009). For sorbitol, the mobile phase was acetonitrile-water (80:20 in volume) at 0.8 mL/min (Hua et al., 2015). Mannitol or sorbitol was quantified using a standard (Sigma-Aldrich, Shanghai, China) with a linear response range of 0.05–10 mg mL–1. Intracellular mannitol and sorbitol concentrations were presented as μmol per gram fresh weight of yeast cells (Abadias et al., 2000). Three biological replicates were used for each sample in each assay and the experiments were repeated three times.
Population Dynamics of D. hansenii in Wounds of Kiwifruit Fruits
Three wounds (4 mm deep × 3 mm wide) were made on the equator of each kiwifruit with a sterile nail, and a 10 μL suspension of MT or ST and NT D. hansenii cells (1 × 107 cells/mL) was administered to each wound to characterize the population dynamics of D. hansenii in wounds of kiwifruit fruit. Fruit samples were collected one hour after their initial application to the wounds and then daily for a period of 4 days. Yeast populations were measured as described by Liu et al. (2012). Briefly, yeasts were recovered by removing ten samples of wounded tissues with a cork borer (1 cm diameter × 1 cm deep). Samples were then ground with a mortar and pestle in 10 mL sterile distilled water. Subsequently, 50 μL of serial 10-fold dilutions were spread on YPD agar plates. Samples taken at 1 h after treatment served as time 0. Fruits stored at 25°C were assessed each day for 4 days. Colonies were counted after incubation of YPD agar plates at 25°C for 2 days and expressed as the Log10 CFU per wound. There were three biological replicates evaluated for each treatment, and the experiment was repeated three times.
Biocontrol Assay
The determination of biocontrol efficacy was determined as described by Wang et al. (2018). Three wounds (4 mm deep × 3 mm wide) were made on the equator of each fruit with a sterile nail. A 10 μL suspension of NT, MT, or ST cells of D. hansenii (1 × 107 cells/mL) was administered to each wound. Sterile distilled water served as a control. After fruits were air-dried for 2 h, 10 μL of either P. expansum or B. cinerea suspension (1 × 104 spores/mL) were inoculated into each wound. Treated fruits were placed in a covered plastic food tray. Each tray was enclosed with a polyethylene bag in order to maintain high humidity (approximately 95% RH) and stored at 25°C. Disease incidence and lesion diameter of kiwifruit fruits were recorded after 4 days. Each treatment contained three biological replicates with ten fruits per replicate and the experiment was repeated three times.
Data Analysis
All statistical analyses were performed using SPSS version 20.0 (SPSS Inc., United States) software. Data with a single variable (treatment) were analyzed by a one-way ANOVA, and mean separations were performed using a Duncan’s multiple range test. Differences at P < 0.05 were considered significant. Data presented in this paper were pooled across three independent repeated experiments.
Results
Effect of Mannitol and Sorbitol on Stress Tolerance of D. hansenii
Mannitol-treated (MT) or sorbitol-treated (ST) yeast cells exhibited significantly higher viability than non-treated (NT) cells. As presented in Figure 1, the viability of NT yeast cells was about 50%, after exposure to 30 mM H2O2 or 40.5°C for 30 min. In contrast, the viability of MT or ST yeast cells was significantly higher, after exposure to 30 mM H2O2 or 40.5°C for 30 min, respectively. These results demonstrate that yeast cells pretreated with mannitol or sorbitol had a statistically significant higher level of viability compared to NT cells.
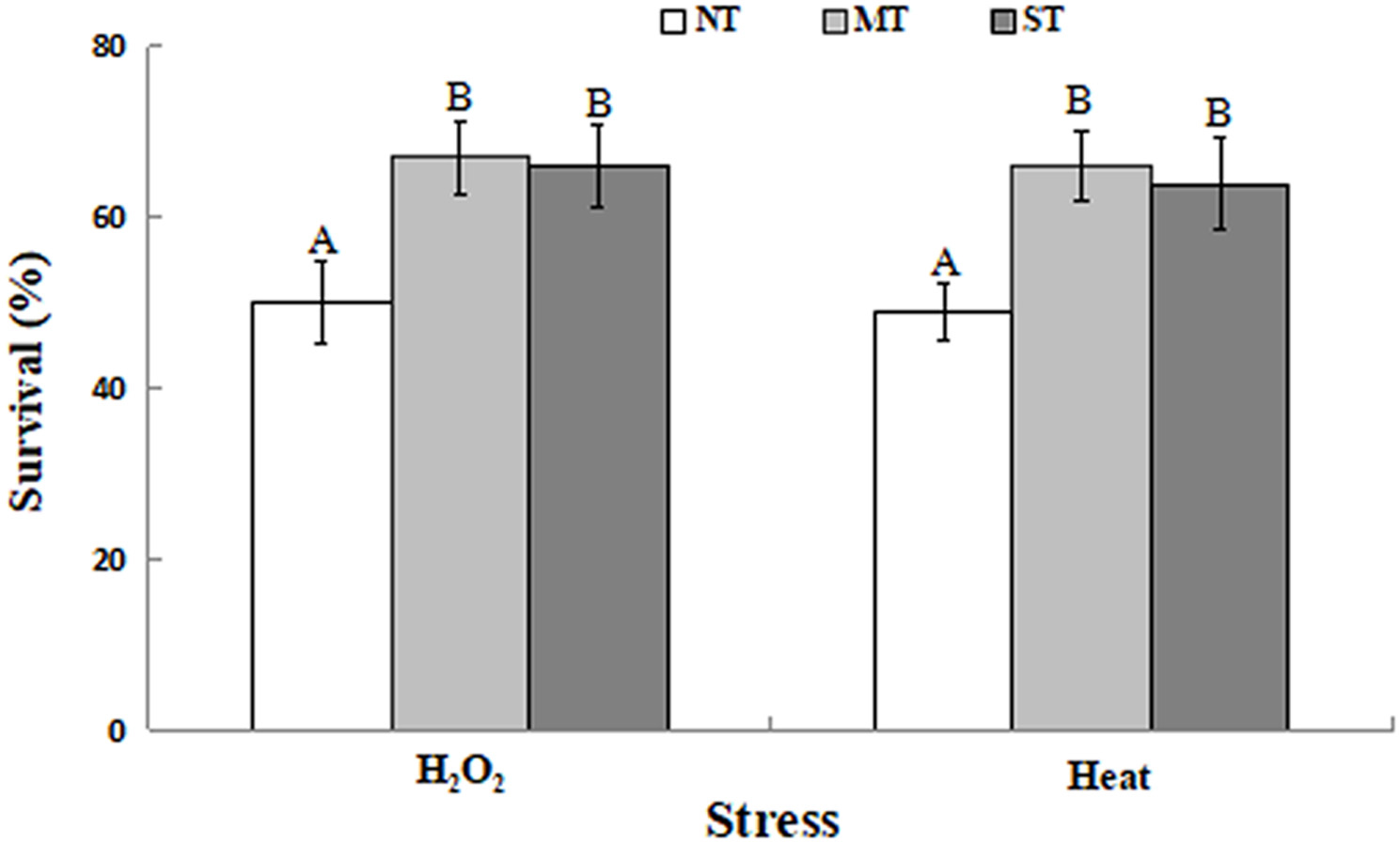
Figure 1. Percent viability of non-treated (NT) control, mannitol-treated (MT), and sorbitol-treated (ST) D. hansenii cells subjected to a subsequent oxidative (30 mM H2O2), or heat (40.5°C) stress for 30 min. Data represent the mean ± standard deviation of three independent experiments, where each experiment consisted of three biological replicates (n = 9). Columns with different letters are significantly different according to a Duncan’s multiple range test at p < 0.05.
Effect of Mannitol and Sorbitol on Intracellular Accumulation of ROS in D. hansenii
At time 0 (the time point after the 2-hour pretreatment but prior to exposure to the oxidative or high-temperature stress), the percentage of MT and ST cells, as well as NT cells, exhibiting ROS was less than 10% (Figure 2). This percentage increased when cells were exposed to either a 30-minute treatment of 30 mM H2O2 or 40.5°C. Both MT and ST cells exhibited a significantly lower percentage of cells exhibiting ROS compared to NT cells under each specific stress.
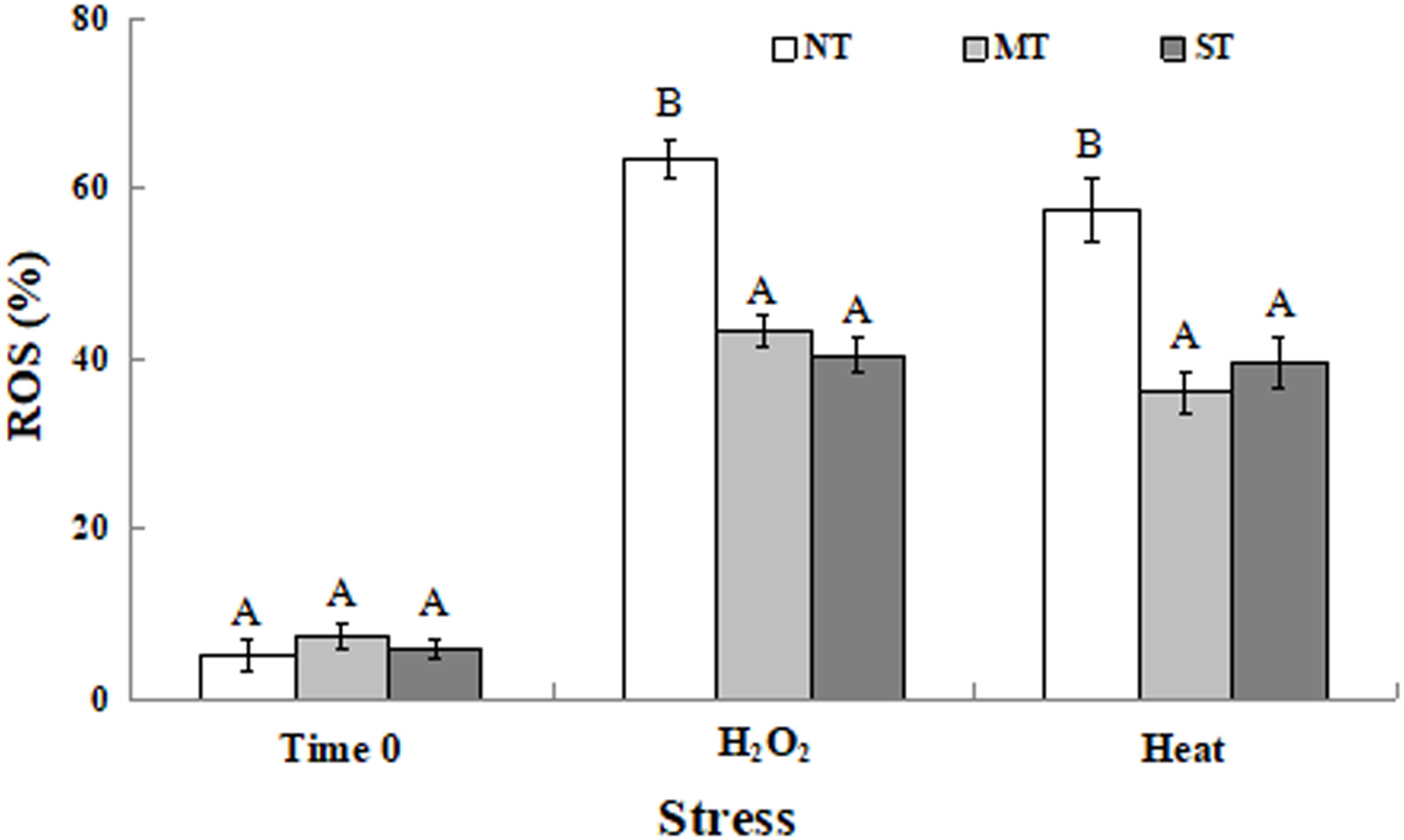
Figure 2. Percentage of non-treated (NT) control, mannitol-treated (MT), and sorbitol-treated (ST) D. hansenii cells exhibiting ROS accumulation subjected to a subsequent oxidative (30 mM H2O2), or heat (40.5°C) stress for 30 min, at time 0 (prior to exposure to the subsequent oxidative or heat stress). Data represent the mean ± standard deviation of three independent experiments, where each experiment consisted of three biological replicates (n = 9). Columns with different letters are significantly different according to a Duncan’s multiple range test at p < 0.05.
Antioxidant Gene Expression
Results of the RT-qPCR analysis on the expression of CAT1, SOD1 transcripts in MT, ST, and NT cells of D. hansenii is presented in Figure 3. The RT-qPCR analysis of gene expression of yeast samples at time 0 (the time point after the 2-hour pretreatment but prior to exposure to the oxidative or high-temperature stress) indicated that pretreatment of yeast cells with sorbitol or mannitol upregulated the expression of CAT1 and SOD1 in D. hansenii cells. The expression of CAT1 was significantly higher in MT and ST cells than in NT cells exposed to 30 mM H2O2 (30 min). Similarly, the expression of CAT1 in cells exposed to 40.5°C (30 min) yeast cells was also elevated (Figure 3A). The expression of SOD1 in pretreated cells was significantly higher than in NT cells exposed to 30 mM H2O2 (30 min). The expression of SOD1 in cells exposed to 40.5°C (30 min) was also significantly higher in MT and ST cells than in NT cells (Figure 3B).
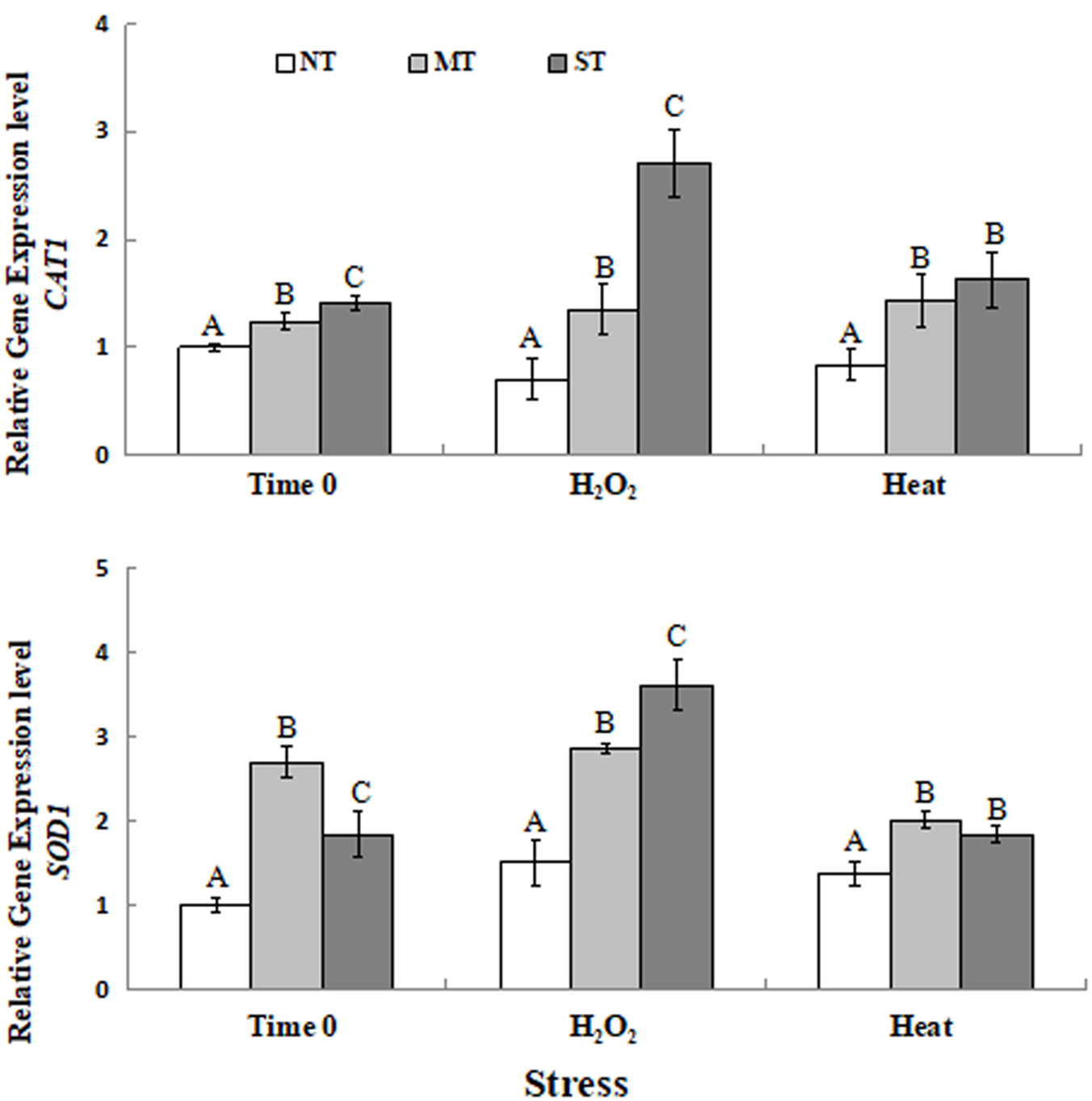
Figure 3. Expression of two antioxidant genes [CAT1 (A) and SOD1 (B)] in non-treated (NT) control, mannitol-treated (MT), and sorbitol-treated (ST) D. hansenii cells subjected to a subsequent oxidative (30 mM H2O2), or heat (40.5°C) stress for 30 min, at time 0 (prior to exposure to the subsequent oxidative or heat stress). Data represent the mean ± standard deviation of three independent experiments, where each experiment consisted of three biological replicates (n = 9). Columns with different letters are significantly different according to a Duncan’s multiple range test at p < 0.05.
Effect of Mannitol and Sorbitol Treatments on Antioxidant Enzyme Activity of D. hansenii
The activity of catalase and SOD activity were measured in the pretreated and control samples in response to the oxidative and high-temperature stress treatments. Results indicated that CAT and SOD activity was significantly higher in MT and ST yeast cells than in NT yeast cells (Figure 4). The activity of these antioxidant enzymes, as with the level of expression of their corresponding genes, was significantly higher in yeast cells of D. hansenii pretreated with mannitol or sorbitol than in untreated cells prior to and after exposure (time 0 and after 30 min) to the subsequent high-temperature and oxidative stress. CAT activity in MT and ST cells in response to exposure to the high-temperature and oxidative stress was significantly higher than in NT cells (Figure 4A). SOD activity was also significant higher in MT and ST cells exposed to the high-temperature and oxidative stress than in NT cells at all of the evaluated time points (Figure 4B).
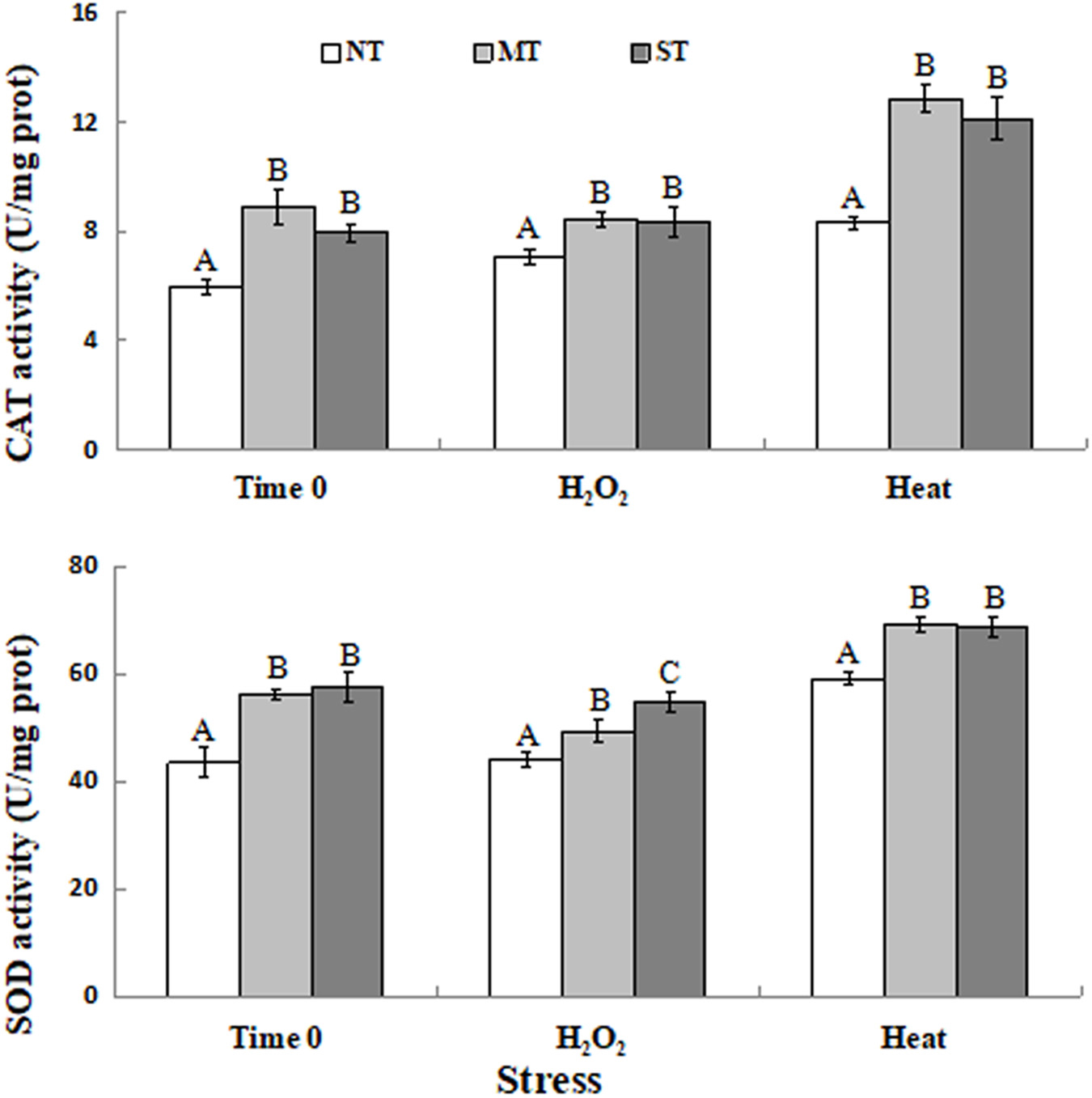
Figure 4. CAT (A) and SOD (B) activity in non-treated (NT) control, mannitol-treated (MT), and sorbitol-treated (ST) of D. hansenii cells subjected to a subsequent oxidative (30 mM H2O2), or heat (40.5°C) stress for 30 min, at time 0 (prior to exposure to the subsequent oxidative or heat stress). Data represent the mean ± standard deviation of three independent experiments, where each experiment consisted of three biological replicates (n = 9). Columns with different letters are significantly different according to a Duncan’s multiple range test at p < 0.05.
Intracellular Content of Mannitol and Sorbitol in D. hansenii
As indicated in Figure 5, the intracellular level of mannitol in MT cells was significantly higher than it was in ST and NT cells at time 0 (the time point after the 2-hour pretreatment but prior to exposure to the oxidative or high-temperature stress). Exposure to either a 30-minute treatment of 30 mM H2O2 or 40.5°C elevated intracellular mannitol content, with MT cells still exhibiting the highest mannitol content (Figure 5A). Similarly, ST cells had the highest intracellular sorbitol content at time 0 and under the stress conditions (Figure 5B).
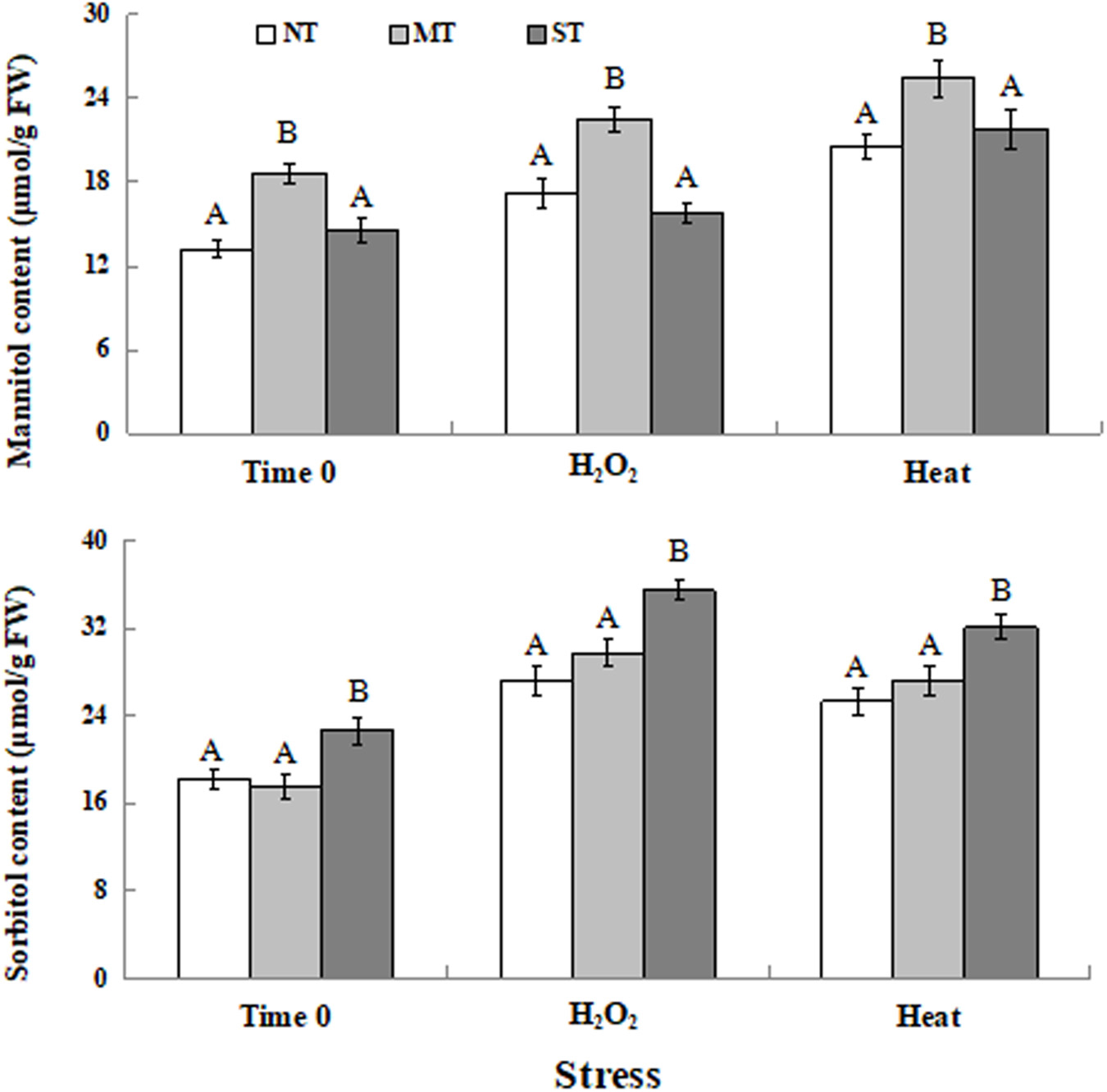
Figure 5. Intracellular contents of mannitol (A) and sorbitol (B) in D. hansenii cells subjected to a subsequent oxidative (30 mM H2O2), or heat (40.5°C) stress for 30 min, at time 0 (prior to exposure to the subsequent oxidative or heat stress). Mannitol and sorbitol concentrations represented as μmol per gram of yeast cell fresh weight (μmol/g FW). Data represent the mean ± standard deviation of three independent experiments, where each experiment consisted of three biological replicates (n = 9). Columns with different letters are significantly different according to a Duncan’s multiple range test at p < 0.05.
Growth of D. hansenii in Kiwifruit Wounds
All of the D. hansenii cultures (NT, MT, and ST) grew rapidly after they were administered to fruit wounds. The population of MT and ST cells, however, was significantly higher than the population of NT cells on each of 4 days following their inoculation into wounds (Figure 6). Importantly, the ability of yeast biocontrol agents to rapidly establish themselves and grow in fruit wounds once they are administered is considered a biocontrol trait that is essential for effective control.
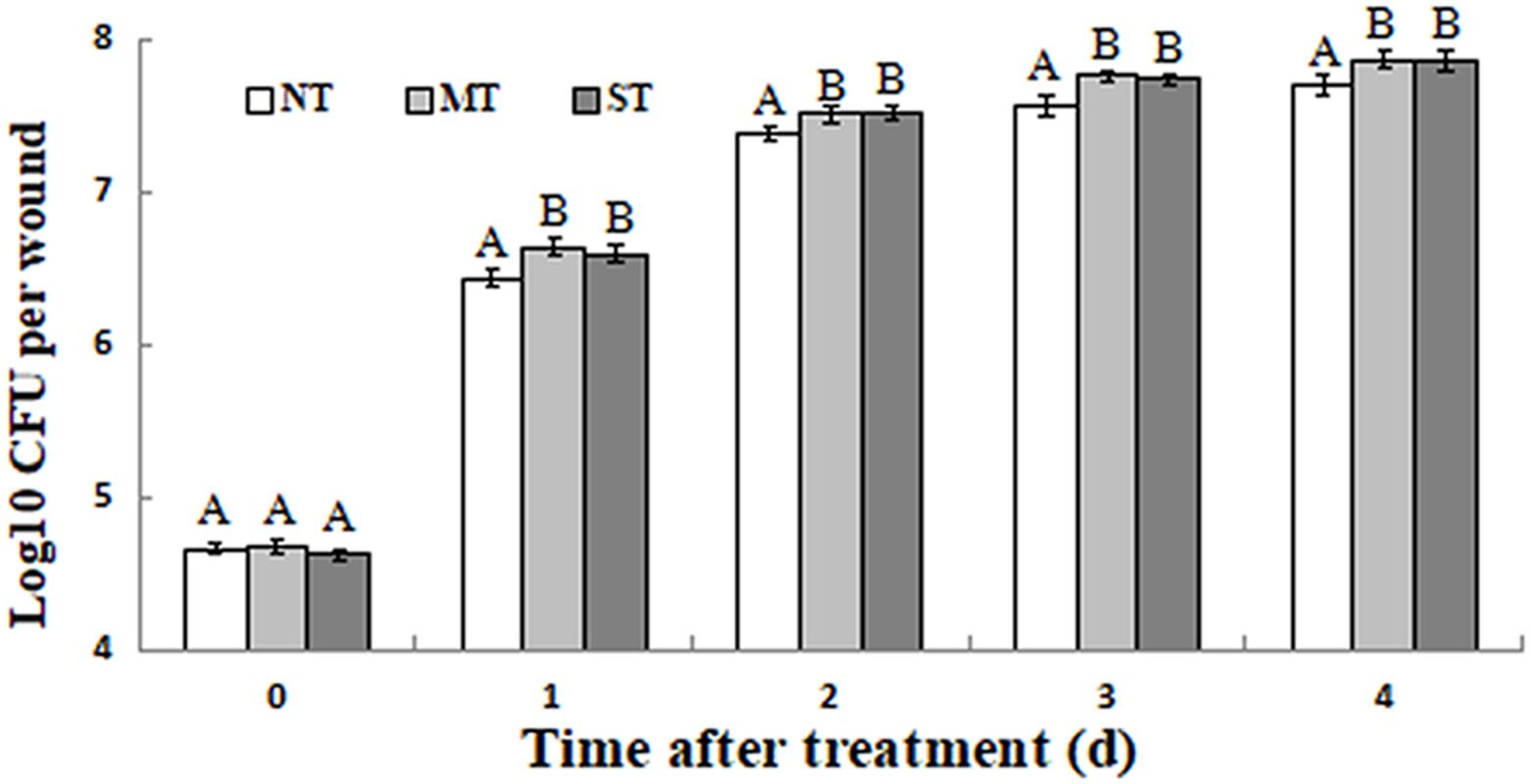
Figure 6. Growth dynamics of non-treated (NT) control, mannitol-treated (MT), and sorbitol-treated (ST) D. hansenii in kiwifruit wounds at 25°C. Fruits were wounded and inoculated with 10 μL suspension of MT or ST and NT yeast cells at 1 × 107 cells/mL. Data represent the mean ± standard deviation of three independent experiments, where each experiment consisted of three biological replicates (n = 9). Columns with different letters are significantly different according to a Duncan’s multiple range test at p < 0.05.
Biocontrol Assay of D. hansenii Against Postharvest Diseases of Kiwifruit Fruits
As shown in Figure 7, the antagonistic yeast, D. hansenii, significantly reduced both disease incidence and lesion diameter of both blue mold and gray mold infections of kiwifruit caused by P. expansum and B. cinerea, respectively. Notably, the incidence of both blue and gray mold decay in fruit treated with NT cultures was about 20% lower than the control group where no yeast cells were administered, which had an incidence level of 100% for both pathogens. In contrast, disease incidence in kiwifruit treated with MT or ST cell groups was reduced significantly, relative to the NT control (Figure 7A). Correspondingly, lesion diameters caused by P. expansum and B. cinerea were significantly smaller on kiwifruit fruits inoculated with MT or ST cells, compared to lesion size in kiwifruit treated with NT cells (Figure 7B). These data indicate that D. hansenii significantly reduced both disease incidence and lesion size for both gray mold and blue mold pathogens, however, the use of yeast cells pretreated with mannitol or sorbitol further increased the level of control beyond the level observed for non-treated (NT) cells.
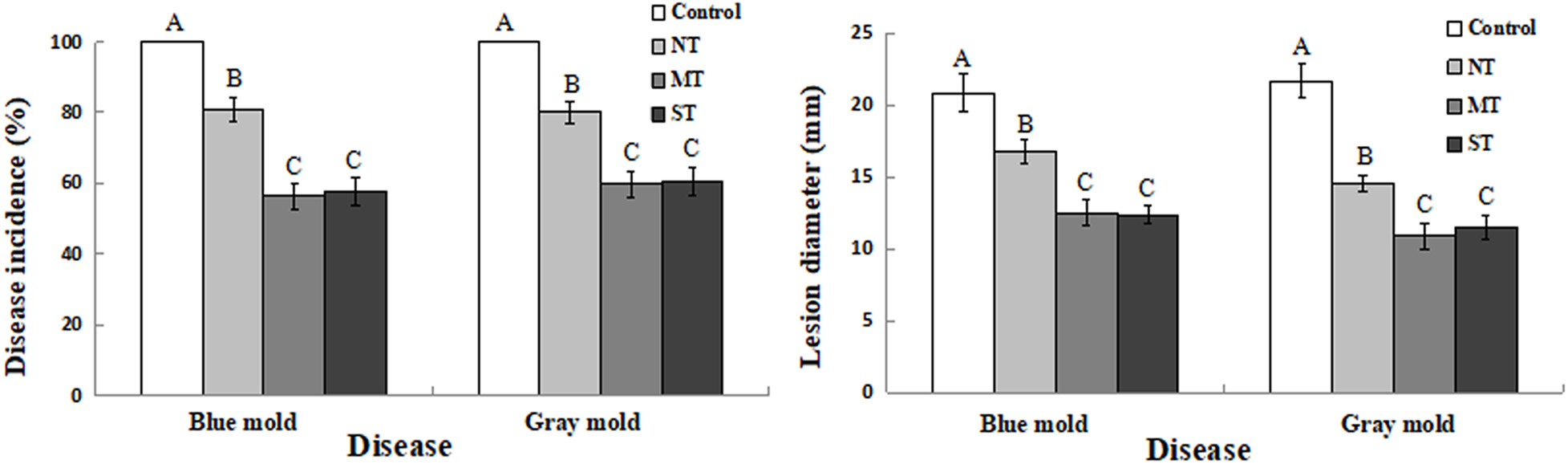
Figure 7. Biocontrol efficacy of D. hansenii against P. expansum and B. cinerea on kiwifruit stored at 25°C. Fruits were wounded inoculated with 10 μl of sterile water (control), and non-treated (NT), mannitol-treated (MT), and sorbitol-treated (ST) cell suspension of D. hansenii at 1 × 107 cells/ml. Fruits were air-dried and then wounds were inoculated with either 10 μl of P. expansum or B. cinerea at 1 × 104 spores/ml. Disease incidence (A) and lesion diameter (B) in kiwifruit were recorded after 4 days. Data represent the mean ± sd of the pooled data from three experiments (n = 9). Columns with different letters at each time point indicate significant differences according to a Duncan’s multiple range test at P < 0.05.
Discussion
Biocontrol agents used to manage postharvest diseases encounter a variety of abiotic stresses under packinghouse conditions, including oxidative stress, high temperature, lack of nutrients, and adverse pH. Enhancing the viability and efficacy of yeasts exposed to these stresses represents a useful strategy for increasing the performance of postharvest biocontrol agents in both packinghouses and in preharvest field applications (Teixidó et al., 2006; Wang et al., 2010; An et al., 2012). In the present study, yeast viability under oxidative and high temperature conditions was determined for yeast cultures that were pretreated with a 2 h exposure to 0.1 M mannitol (MT) or 0.1 M sorbitol (ST). Results indicated that the survival rate of MT and ST yeast determined by CFU counting was significantly higher compared to untreated (NT) yeast cells that had not been exposed to either mannitol or sorbitol (Figure 1). Several methods for enhancing stress tolerance and improving the efficacy of biological control have been previously reported. These include, stress adaptation (Liu et al., 2012; Wang et al., 2018), physiological manipulation (Abadias et al., 2001; Mokiou and Magan, 2008), and the use of exogenous anti-stress substances (Liu et al., 2011; An et al., 2012; Sui et al., 2012). Notably, viability in the present study was estimated as CFUs that only took into account viable cells with growth capacity. Some yeast cells with metabolic activity and still able to inhibit molds, but not able to multiply viable but non-culturable cells (VBNC) may have been present. These cells may exhibit a low but detectable level of metabolic activity and gene expression, as well as maintain membrane integrity, but not able to grow on the culture medium and therefore do not form a CFU. In this case, actual levels of survival could be higher than the level determined by CFUs.
Mannitol is a common metabolite in many organisms where it is synthesized in response to abiotic stress and functions as an osmoprotectant, a free radical scavenger, and a stabilizer of protein and membrane structure (including in chloroplasts, thus helping to maintain photosynthesis) (Seckin et al., 2009). Sorbitol stabilizes the native conformation of proteins, counteracting the detrimental effects of desiccation and high temperature stresses (Wolfe et al., 1998). Sugar alcohols have been reported to enhance stress tolerance and have a significant antioxidant effect in many plants (Pharr et al., 1995). Debaryomyces hansenii is a halotolerant yeast that produces and assimilates a wide variety of polyols (Pereira et al., 2014). In our study, both mannitol and sorbitol enhanced the viability of D. hansenii under oxidative stress and high temperature conditions (Figure 1). When yeasts are exposed to severe stresses, such as oxidative and heat stress, large amounts of intracellular ROS are generated that impair cell viability (Liu et al., 2012; Sui and Liu, 2014). An et al. (2012) reported that exogenous calcium improves the viability of the biocontrol yeasts, D. hansenii and Pichia membranaefaciens, under heat stress by reducing ROS accumulation and oxidative damage to cellular proteins. Liu et al. (2017) found that three ion-modified (Mg2+, Fe2+, and Zn2+) minimal mineral media enhanced viable biomass production and biocontrol efficacy of the biocontrol yeast Candida diversa by protecting cells from oxidative damage and elevating of the activity of SOD and glutathione peroxidase enzymes. In the present study, a lower percentage of cells exhibiting ROS was observed in MT and ST yeast cells (Figure 2), and lower ROS production was associated with a higher level of viability in MT and ST cells exposed to oxidative/heat stress (Figure 1). These data indicate that exogenous mannitol and sorbitol can play an important role in increasing the level of stress tolerance in yeast used as postharvest biocontrol agents.
The expression of antioxidant genes has been reported to be upregulated in yeast cells by a variety of treatments that enhance stress tolerance (Martínez-Pastor et al., 2010; Wang et al., 2018). In the current study, pretreatment of yeast cells with sorbitol or mannitol induced a higher level of expression of the genes, CAT1 and SOD1, relative to untreated cells, when all three types of yeast cells (NT, MT and ST) were exposed to a subsequent oxidative stress or high temperature (Figure 3). The increase in expression was especially evident when the yeast cells were exposed to oxidative stress (30 mM H2O2). The lack of an effective antioxidant defense system leads to an accumulation of hydrogen peroxide and/or other reactive oxygen species (ROS). The enzymatic detoxification of ROS is partially dependent on the activation of antioxidant genes such as CAT1 and SOD1 (Deveau et al., 2010; Huang et al., 2014; Jung and Kim, 2014). In the present study, the expression of two antioxidant genes (Figure 3) was elevated in MT and ST cells, which may have contributed to an increased ability to scavenge intracellular ROS (Figure 2) in yeast cells, and thus a higher level of viability (Figure 1).
When yeast cells are exposed to an abiotic stress, a significant increase in the level of ROS is observed. Excessive ROS levels can result in oxidative damage to cellular constituents, including nucleic acids and proteins, resulting in a decreased enzyme activity in cells (Reverter-Branchat et al., 2004; Branduardi et al., 2007). In our study, CAT enzyme activity in MT and ST cells exposed to subsequent oxidative (30 mM H2O2 for 30 min) or high-temperature (40.5°C for 30 min) stress was significantly higher than in NT cells. Similarly, MT and ST yeast cells also exhibited significantly higher SOD enzyme activity compared to NT yeast cells, when the different groups of yeast cells were exposed to a subsequent oxidative or high-temperature stress (Figure 4). This pattern of enzyme activity did not exactly match levels of gene expression (Figure 3). This may be attributed to the fact that the measurement of enzyme activity was for total CAT and SOD activity, while the level of gene expression was for specific genes, CAT1 and SOD1. Alternatively, post-transcriptional, post-translational, or different rates of protein turnover vs. transcript turnover may have also account for the discrepancy between levels of gene expression and enzyme activity. However, the inducive effect of MT and ST on both transcription and enzyme levels was clearly evident. Previous researches have reported that increased antioxidant enzyme activity contributes to improved abiotic stress tolerance (Collinson et al., 2002; Chi et al., 2015; Sui et al., 2015; Akshya and Sukesh, 2017; Wang et al., 2018).
Mannitol and sorbitol are reported to play important roles in the response of yeast cells to a variety of abiotic stresses (Teixidó et al., 1998; Abadias et al., 2000; Kumar and Gummadi, 2009; Hua et al., 2015). Managbanag and Torzilli (2002) reported that mannitol accumulated in cells of a yeast-like fungus, Aureobasidium pullulans, during exposure to heat and salt stress. Aguilera and Prieto (2001) observed that intracellular sorbitol content in Saccharomyces cerevisiae cells increased when cells were exposed to oxidative, heat and salt stresses. Similar increases in intracellular mannitol and sorbitol levels were observed in D. hansenii under oxidative and heat stress in the present study (Figure 5). Moreover, exposure to exogenous MT increased the concentration of intracellular mannitol (Figure 5A), while exposure to exogenous ST increased the intracellular level of sorbitol (Figure 5B). This indicated that D. hansenii cells were able to absorb a certain amount of exogenous mannitol or sorbitol. The increased levels of mannitol and sorbitol may play a crucial antioxidant function in yeast cells exposed to oxidative or heat stress, thus enabling cells to maintain a higher level of viability (Figure 1).
Rapid growth of yeast biocontrol agents in wounds and fruit surfaces is an advantage for microbial antagonists competing for nutrients and space (Liu et al., 2013). MT and ST yeast cells grew more quickly than NT cells in wounds of kiwifruit fruits over the sampled 4-day time period (Figure 6). Previous studies have also reported that pretreatment of yeast cells with different exogenous compounds can significantly enhance their biocontrol efficacy (Sui et al., 2012). In the present study, MT and ST cells reduced the incidence of blue mold and gray mold to a significantly greater degree than NT yeast (Figure 7A). The lesion diameters caused by pathogenic fungi on kiwifruit were also significantly smaller in fruits treated with MT and ST yeast cells, compared to kiwifruit treated with NT cells (Figure 7B). These results are in agreement with previous studies indicating that specific types of pretreatment of antagonistic yeast can activate their antioxidant defense system and increase their biocontrol efficacy (Chi et al., 2015; Sui et al., 2015; Wang et al., 2018).
Conclusion
Pretreatment of D. hansenii yeast cells with mannitol or sorbitol increased their survival rate, antioxidant enzyme activity and gene expression, and decreased intracellular ROS level in the pretreated yeast when subsequently exposed to oxidative or high-temperature stress. The pretreatment of yeast with mannitol or sorbitol was also associated with the upregulation of intracellular mannitol or sorbitol level. The enhancement in stress tolerance increased the biocontrol efficacy of D. hansenii in controlling blue mold and gray mold decay on kiwifruit. While many studies on the function and use of mannitol and sorbitol have been conducted on animals and higher plants, few studies have examined their effect on biocontrol yeast. The results of our study may have practical implications for the use of mannitol and sorbitol to improve ability of yeast to control postharvest diseases of fruits. However, the effects of mannitol and sorbitol treatment on the tolerance of biocontrol yeasts to additional stresses, like osmotic and cell wall stresses, need to be further investigated.
Data Availability Statement
The datasets generated for this study are available on request to the corresponding author.
Author Contributions
YW and YS conceived and designed the experiments. All authors performed the experiments and analyzed the data, read and approved the final manuscript. YS drafted the manuscript.
Funding
This work was supported by National Natural Science Foundation of China (31972133), Science and Technology Research Program of Chongqing Education Commission (KJQN201801331), Scientific Research Program for Graduate Students of Chongqing University of Arts and Sciences, and Program of Introducing Talents of Discipline to Universities of China (111 Program, D20023).
Conflict of Interest
The authors declare that the research was conducted in the absence of any commercial or financial relationships that could be construed as a potential conflict of interest.
References
Abadias, M., Teixidó, N., Usall, J., Viñas, I., and Magan, N. (2000). Solute stresses affect growth patterns, endogenous water potentials and accumulation of sugars and sugar alcohols in cells of the biocontrol yeast Candida sake. J. Appl. Microbiol. 89, 1009–1017. doi: 10.1046/j.1365-2672.2000.01207.x
Abadias, M., Teixidó, N., Usall, J., Viñas, I., and Magan, N. (2001). Improving water stress tolerance of the biocontrol yeast Candida sake grown in molasses-based media by physiological manipulation. Can. J. Microbiol. 47, 123–129. doi: 10.1139/cjm-47-2-123
Aguilera, J., and Prieto, J. A. (2001). The Saccharomyces cerevisiae aldose reductase is implied in the metabolism of methylglyoxal in response to stress conditions. Curr. Genet. 39, 273–283. doi: 10.1007/s002940100213
Akshya, S., and Sukesh, C. S. (2017). Physiological basis for the tolerance of yeast Zygosaccharomyces bisporus to salt stress. Hayati J. Biosci. 24, 176–181. doi: 10.1016/j.hjb.2017.11.001
An, B., Li, B., Qin, G., and Tian, S. (2012). Exogenous calcium improves viability of biocontrol yeasts under heat stress by reducing ROS accumulation and oxidative damage of cellular protein. Curr. Microbiol. 65, 122–127. doi: 10.1007/s00284-012-0133-4
Bradford, M. M. (1976). A rapid and sensitive method for the quantitation of microgram quantities of protein utilizing the principle of protein-dye binding. Anal. Biochem. 72, 248–254. doi: 10.1016/0003-2697(76)90527-3
Branduardi, P., Fossati, T., Sauer, M., Pagani, R., Mattanovich, D., and Porro, D. (2007). Biosynthesis of vitamin C by yeast leads to increased stress resistance. PLoS One 2:e1092. doi: 10.1371/journal.pone.0001092
Carmona-Hernandez, S., Reyes-Pérez, J. J., Chiquito-Contreras, R. G., Rincon-Enriquez, G., Cerdan-Cabrera, C. R., and Hernandez-Montiel, L. G. (2019). Biocontrol of postharvest fruit fungal diseases by bacterial antagonists: a review. Agronomy 9:121. doi: 10.3390/agronomy9030121
Castoria, R., Caputo, L., Curtis, F. D., and Cicco, V. D. (2003). Resistance of postharvest biocontrol yeasts to oxidative stress: a possible new mechanism of action. Phytopathology 93, 564–572. doi: 10.1094/phyto.2003.93.5.564
Chandler-Brown, D., Choi, H., Park, S., Ocampo, B. R., Chen, S., Le, A., et al. (2015). Sorbitol treatment extends lifespan and induces the osmotic stress response in Caenorhabditis elegans. Front. Genet. 6:316. doi: 10.3389/fgene.2015.00316
Chi, M., Li, G., Liu, Y., Liu, G., Li, M., Zhang, X., et al. (2015). Increase in antioxidant enzyme activity, stress tolerance and biocontrol efficacy of Pichia kudriavzevii with the transition from a yeast-like to biofilm morphology. Biol. Control 90, 113–119. doi: 10.1016/j.biocontrol.2015.06.006
Collinson, E. J., Wheeler, G. L., Garrido, E. O., Avery, A. M., Avery, S. V., and Grant, C. M. (2002). The yeast glutaredoxins are active as glutathione peroxidases. J. Biol. Chem. 277, 16712–16717. doi: 10.1074/jbc.m111686200
Conde, A., Silva, P., Agasse, A., Conde, C., and Gerós, H. (2011). Mannitol transport and mannitol dehydrogenase activities are coordinated in Olea europaea under salt and osmotic stresses. Plant Cell Physiol. 52, 1766–1775. doi: 10.1093/pcp/pcr121
Czarnecka, M., Żarowska, B., Połomska, X., Restuccia, C., and Cirvilleri, G. (2019). Role of biocontrol yeasts Debaryomyces hansenii and Wickerhamomyces anomalus in plants’ defence mechanisms against Monilinia fructicola in apple fruits. Food Microbiol. 83, 1–8. doi: 10.1016/j.fm.2019.04.004
Deveau, A., Piispanen, A. E., Jackson, A. A., and Hogan, D. A. (2010). Farnesol induces hydrogen peroxide resistance in Candida albicans yeast by inhibiting the ras-cyclic AMP signaling pathway. Eukaryot. Cell 9, 569–577. doi: 10.1128/ec.00321-09
Droby, S., Chalutz, E., Wilson, C. L., and Wisniewski, M. (1989). Characterization of the biocontrol activity of Debaryomyces hansenii in the control of Penicillium digitatum on grapefruit. Can. J. Microbiol. 35, 794–800. doi: 10.1139/m89-132
Droby, S., Wisniewski, M., Teixidó, N., Spadaro, D., and Jijakli, M. H. (2016). The science, development, and commercialization of postharvest biocontrol products. Postharvest Biol. Technol. 122, 22–29. doi: 10.1016/j.postharvbio.2016.04.006
Ferraz, P., Cássio, F., and Lucas, C. (2019). Potential of yeasts as biocontrol agents of the phytopathogen causing cacao Witches’ broom disease: is microbial warfare a solution? Front. Microbiol. 10:1766. doi: 10.3389/fmicb.2019.01766
Hernandez-Montiel, L. G., Gutierrez-Perez, E. D., Murillo-Amador, B., Vero, S., Chiquito-Contreras, R. G., and Rincon-Enriquez, G. (2018). Mechanisms employed by Debaryomyces hansenii in biological control of anthracnose disease on papaya fruit. Postharvest Biol. Technol. 139, 31–37. doi: 10.1016/j.postharvbio.2018.01.015
Hernández-Montiel, L. G., Ochoa, J. L., Troyo-Diéguez, E., and Larralde-Corona, C. P. (2010). Biocontrol of postharvest blue mold (Penicillium italicum Wehmer) on Mexican lime by marine and citrus Debaryomyces hansenii isolates. Postharvest Biol. Technol. 56, 181–187. doi: 10.1016/j.postharvbio.2009.12.010
Hua, S. S., Hernlem, B. J., Yokoyama, W., and Sarreal, S. B. (2015). Intracellular trehalose and sorbitol synergistically promoting cell viability of a biocontrol yeast, Pichia anomala, for aflatoxin reduction. World J. Microbiol. Biotechnol. 31, 729–734. doi: 10.1007/s11274-015-1824-3
Huang, M., Xu, Q., Mitsui, K., and Xu, Z. (2014). PSK1 regulates expression of SOD1 involved in oxidative stress tolerance in yeast. FEMS Microbiol. Lett. 350, 154–160. doi: 10.1111/1574-6968.12329
Jung, J. H., and Kim, J. (2014). Roles of Edc3 in the oxidative stress response and CaMCA1-encoded metacaspase expression in Candida albicans. FEBS J. 281, 4841–4851. doi: 10.1111/febs.13022
Kumar, S., and Gummadi, S. N. (2009). Osmotic adaptation in halotolerant yeast, Debaryomyces nepalensis NCYC 3413: role of osmolytes and cation transport. Extremophiles 13, 793–805. doi: 10.1007/s00792-009-0267-x
Larher, F. R., Lugana, R., Gagneul, D., Guyot, S., Monnier, C., Lespinasse, Y., et al. (2009). A reassessment of the prevalent organic solutes constitutively accumulated and potentially involved in osmotic adjustment in pear leaves. Environ. Exp. Bot. 66, 230–241. doi: 10.1016/j.envexpbot.2009.02.005
Leaw, S. N., Chang, H. C., Sun, H. F., Barton, R., Bouchara, J. P., and Chang, T. C. (2006). Identification of medically important yeast species by sequence analysis of the internal transcribed spacer regions. J. Clin. Microbiol. 44, 693–699. doi: 10.1128/jcm.44.3.693-699.2006
Liu, J., Li, G., and Sui, Y. (2017). Optimization of culture medium enhances viable biomass production and biocontrol efficacy of the antagonistic yeast, Candida diversa. Front. Microbiol. 8:2021. doi: 10.3389/fmicb.2017.02021
Liu, J., Sui, Y., Wisniewski, M., Droby, S., and Liu, Y. (2013). Review: utilization of antagonistic yeasts to manage postharvest fungal diseases of fruit. Int. J. Food Microbiol. 167, 153–160. doi: 10.1016/j.ijfoodmicro.2013.09.004
Liu, J., Sui, Y., Wisniewski, M., Xie, Z., Liu, Y., You, Y., et al. (2018). The impact of the postharvest environment on the viability and virulence of decay fungi. Crit. Rev. Food Sci. Nutr. 58, 1681–1687. doi: 10.1080/10408398.2017.1279122
Liu, J., Wisniewski, M., Droby, S., Norelli, J., Hershkovitz, V., Tian, S., et al. (2012). Increase in antioxidant gene transcripts, stress tolerance and biocontrol efficacy of Candida oleophila following sublethal oxidative stress exposure. FEMS Microbiol. Ecol. 80, 578–590. doi: 10.1111/j.1574-6941.2012.01324.x
Liu, J., Wisniewski, M., Droby, S., Vero, S., Tian, S., and Hershkovitz, V. (2011). Glycine betaine improves oxidative stress tolerance and biocontrol efficacy of the antagonistic yeast Cystofilobasidium infirmominiatum. Int. J. Food Microbiol. 146, 76–83. doi: 10.1016/j.ijfoodmicro.2011.02.007
Liu, Q., Ying, S. H., Feng, M. G., and Jiang, X. H. (2009). Physiological implication of intracellular trehalose and mannitol changes in response of entomopathogenic fungus Beauveria bassiana to thermal stress. Antonie Van Leeuwenhoek 95, 65–75. doi: 10.1007/s10482-008-9288-1
Livak, K. J., and Schmittgen, T. D. (2001). Analysis of relative gene expression datausing realtime quantitative PCR and the 2–ΔΔCT method. Methods 25, 402–408. doi: 10.1006/meth.2001.1262
Macarisin, D., Droby, S., Bauchan, G., and Wisniewski, M. (2010). Superoxide anion and hydrogen peroxide in the yeast antagonist fruit interaction: a new role for reactive oxygen species in postharvest biocontrol? Postharvest Biol. Technol. 58, 194–202. doi: 10.1016/j.postharvbio.2010.07.008
Managbanag, J. R., and Torzilli, A. P. (2002). An analysis of trehalose, glycerol, and mannitol accumulation during heat and salt stress in a salt marsh isolate of Aureobasidium pullulan. Mycologia 94, 384–391. doi: 10.1080/15572536.2003.11833203
Martínez-Pastor, M., Proft, M., and Pascual-Ahuir, A. (2010). Adaptive changes of the yeast mitochondrial proteome in response to salt stress. OMICS 14, 541–552. doi: 10.1089/omi.2010.0020
Mokiou, S., and Magan, N. (2008). Physiological manipulation and formulation of the biocontrol yeast Pichia anomala for control of Penicillium verrucosum and ochratoxin A contamination of moist grain. Biocontrol Sci. Tech. 18, 1063–1073. doi: 10.1080/09583150802585769
Pereira, I., Madeira, A., Prista, C., Loureiro-Dias, M. C., and Leandro, M. J. (2014). Characterization of new Polyol/H+ symporters in Debaryomyces hansenii. PLoS One 9:e88180. doi: 10.1371/journal.pone.0088180
Pharr, D. M., Stoop, M. H., Williamson, J. D., Studer Feusi, M. E., Masse, M. O., and Conkling, M. A. (1995). The dual role of mannitol as osmoprotectant and photoassimilate in celery. Colloquium 30, 1182–1188. doi: 10.21273/hortsci.30.6.1182
Reverberi, M., Fabbri, A. A., Zjalic, S., Ricelli, A., Punelli, F., and Fanelli, C. (2005). Antioxidant enzymes stimulation in Aspergillus parasiticus by Lentinula edodes inhibits aflatoxin production. Appl. Microbiol. Biotech. 69, 207–215. doi: 10.1007/s00253-005-1979-1
Reverter-Branchat, G., Cabiscol, E., Tamarit, J., and Ros, J. (2004). Oxidative damage to specific proteins in replicative and chronological-aged Saccharomyces cerevisiae: common targets and prevention by calorie restriction. J. Biol. Chem. 279, 31983–31989. doi: 10.1074/jbc.m404849200
Rivas-Garcia, T., Murillo-Amador, B., Nieto-Garibay, A., Rincon-Enriquez, G., Chiquito-Contreras, R. G., and Hernandez-Montiela, L. G. (2019). Enhanced biocontrol of fruit rot on muskmelon by combination treatment with marine Debaryomyces hansenii and Stenotrophomonas rhizophila and their potential modes of action. Postharvest Biol. Technol. 151, 61–67. doi: 10.1016/j.postharvbio.2019.01.013
Seckin, B., Sekmen, A. H., and Türkan, I. (2009). An enhancing effect of exogenous mannitol on the antioxidant enzyme activities in roots of wheat under salt stress. J. Plant Growth Regul. 28, 12–20. doi: 10.1007/s00344-008-9068-1
Shen, B., Hohmann, S., Jensen, R. G., and Bohnert, H. J. (1999). Roles of sugar alcohols in osmotic stress adaptation. Replacement of glycerol by mannitol and sorbitol in yeast. Plant Physiol. 121, 45–52. doi: 10.1104/pp.121.1.45
Spadaro, D., and Droby, S. (2016). Development of biocontrol products for postharvest diseases of fruit: the importance of elucidating the mechanisms of action of yeast antagonists. Trends Food Sci. Tech. 47, 39–49. doi: 10.1016/j.tifs.2015.11.003
Sui, Y., and Liu, J. (2014). Effect of glucose on thermotolerance and biocontrol efficacy of the antagonistic yeast Pichia guilliermondii. Biol. Control 74, 59–64. doi: 10.1016/j.biocontrol.2014.04.003
Sui, Y., Liu, J., Wisniewski, M., Droby, S., Norelli, J., and Hershkovitz, V. (2012). Pretreatment of the yeast antagonist, Candida oleophila, with glycine betaine increases oxidative stress tolerance in the microenvironment of apple wounds. Int. J. Food Microbiol. 157, 45–51. doi: 10.1016/j.ijfoodmicro.2012.04.010
Sui, Y., Wisniewski, M., Droby, S., and Liu, J. (2015). Responses of yeast biocontrol agents to environmental stress. Appl. Environ. Microbiol. 81, 2968–2975. doi: 10.1128/aem.04203-14
Teixidó, N., Cañamás, T. P., Abadias, M., Usall, J., Solsona, C., Casals, C., et al. (2006). Improving low water activity and desiccation tolerance of the biocontrol agent Pantoea agglomerans CPA-2 by osmotic treatments. J. Appl. Microbiol. 101, 927–937. doi: 10.1111/j.1365-2672.2006.02948.x
Teixidó, N., Viñas, I., Usall, J., and Magan, N. (1998). Improving ecological fitness and environmental stress tolerance of the biocontrol yeast Candida sake by manipulation of intracellular sugar alcohol and sugar content. Mycol. Res. 102, 1409–1417. doi: 10.1017/s0953756298006716
Usall, J., Torres, R., and Teixidó, N. (2016). Biological control of postharvest diseases on fruit: a suitable alternative? Curr. Opin. Food Sci. 11, 51–55. doi: 10.1016/j.cofs.2016.09.002
Wang, Y., Luo, Y., Sui, Y., Xie, Z., Liu, Y., Jiang, M., et al. (2018). Exposure of Candida oleophila to sublethal salt stress induces an antioxidant response and improves biocontrol efficacy. Biol. Control 127, 109–115. doi: 10.1016/j.biocontrol.2018.09.002
Wang, Y., Wang, P., Xia, J., Yu, T., Lou, B., Wang, J., et al. (2010). Effect of water activity on stress tolerance and biocontrol activity in antagonistic yeast Rhodosporidium paludigenum. Int. J. Food Microbiol. 143, 103–108. doi: 10.1016/j.ijfoodmicro.2010.07.035
Wisniewski, M., Droby, S., Norelli, J., Liu, J., and Schena, L. (2016). Alternative management technologies for postharvest disease control: the journey from simplicity to complexity. Postharvest Biol. Technol. 122, 3–10. doi: 10.1016/j.postharvbio.2016.05.012
Keywords: antagonistic yeast, biocontrol efficacy, mannitol, sorbitol, stress tolerance
Citation: Ming X, Wang Y and Sui Y (2020) Pretreatment of the Antagonistic Yeast, Debaryomyces hansenii, With Mannitol and Sorbitol Improves Stress Tolerance and Biocontrol Efficacy. Front. Microbiol. 11:601. doi: 10.3389/fmicb.2020.00601
Received: 12 December 2019; Accepted: 18 March 2020;
Published: 15 April 2020.
Edited by:
Gianluigi Cardinali, University of Perugia, ItalyReviewed by:
Catarina Prista, University of Lisbon, PortugalIstván Pócsi, University of Debrecen, Hungary
Copyright © 2020 Ming, Wang and Sui. This is an open-access article distributed under the terms of the Creative Commons Attribution License (CC BY). The use, distribution or reproduction in other forums is permitted, provided the original author(s) and the copyright owner(s) are credited and that the original publication in this journal is cited, in accordance with accepted academic practice. No use, distribution or reproduction is permitted which does not comply with these terms.
*Correspondence: Yong Wang, eW9uZ3dhbmdiaXNAYWxpeXVuLmNvbQ==; Yuan Sui, c3VpeXVhbi1taW5lQDE2My5jb20=