- 1Institute of Infection, Veterinary and Ecological Sciences, University of Liverpool, Neston, United Kingdom
- 2Chakri Naruebodindra Medical Institute, Faculty of Medicine Ramathibodi Hospital, Mahidol University, Salaya, Thailand
- 3NationWide Laboratories/C.A.P.L. Ltd., Knutton, United Kingdom
- 4IDEXX Laboratories Ltd., Wetherby, United Kingdom
- 5Bristol Veterinary School, University of Bristol, Bristol, United Kingdom
Background: Antimicrobial resistance (AMR) is a globally important one health threat. The impact of resistant infections on companion animals, and the potential public health implications of such infections, has not been widely explored, largely due to an absence of structured population-level data.
Objectives: We aimed to efficiently capture and repurpose antimicrobial susceptibility test (AST) results data from several veterinary diagnostic laboratories (VDLs) across the United Kingdom to facilitate national companion animal clinical AMR surveillance. We also sought to harness and genotypically characterize isolates of potential AMR importance from these laboratories.
Methods: We summarized AST results for 29,330 canine and 8,279 feline Enterobacteriaceae isolates originating from companion animal clinical practice, performed between April 2016 and July 2018 from four VDLs, with submissions from 2,237 United Kingdom veterinary practice sites.
Results: Escherichia coli (E. coli) was the most commonly isolated Enterobacteriaceae in dogs (69.4% of AST results, 95% confidence interval, CI, 68.7–70.0) and cats (90.5%, CI 89.8–91.3). Multi-drug resistance was reported in 14.1% (CI 13.5–14.8) of canine and 12.0% (CI 11.1–12.9) of feline E. coli isolates. Referral practices were associated with increased E. coli 3rd generation ≤ cephalosporin resistance odds (dogs: odds ratio 2.0, CI 1.2–3.4). We selected 95 E. coli isolates for whole genome analyses, of which seven belonged to sequence type 131, also carrying the plasmid-associated extended spectrum β-lactamase gene blaCTX–M–15. The plasmid-mediated colistin resistance gene mcr-9 was also identified for the first time in companion animals.
Conclusions: Linking clinical AMR data with genotypic characterization represents an efficient means of identifying important resistance trends in companion animals on a national scale.
Introduction
Antimicrobial resistance (AMR) poses a significant global threat to animal and human health (O’Neill, 2016). The World Health Organization (WHO) has identified Enterobacteriaceae to be of critical importance, due to worldwide dissemination of extended spectrum β-lactamases (ESBLs), ampicillin hydrolyzing (AmpC) β-lactamases, and carbapenemases (Iredell et al., 2016; Bezabih et al., 2021; Salgado-Caxito et al., 2021). Additionally, newly emerging resistance trends, for example mobile colistin resistance (Elbediwi et al., 2019; El-Sayed Ahmed et al., 2020), have led to increased reports of multi-drug resistant (Magiorakos et al., 2012) and pan-resistant strains (Sun et al., 2016).
To address these issues, national AMR surveillance in humans and production animals has been established (VMD, 2019; PHE, 2020). However, companion animal species have been arguably neglected, despite resistance developing in companion animals exposed to antimicrobial therapy (Schmidt et al., 2018), and transmission of pathogens and resistance between humans and companion animals (Lei et al., 2017). This oversight has partly been due to the companion animal veterinary diagnostic laboratory (VDL) sector being largely comprised of independent commercial laboratories, each utilizing often bespoke information management systems (O’Neill et al., 2014), and different protocols for antimicrobial susceptibility testing (AST) (Marques et al., 2016). Such protocols largely focus on relatively time-consuming techniques for demonstrating phenotypic resistance. Recently, genomics-based methodologies have unlocked a wealth of information pertaining to the development and dissemination of resistance genes in and between bacterial species (McCarthy et al., 2015). Though this has only begun to be explored in companion animals (Zhang, 2016), bacterial isolates disposed of by VDLs remain an untapped resource.
Hence, the aim of this study was, using Enterobacteriaceae isolated from clinical samples of dogs and cats as an exemplar, to define national patterns of AST in companion animals, and factors associated with resistance. Further, we sought to develop a method by which Enterobacteriaceae isolates expressing resistance patterns of potential public health importance could be efficiently captured from VDLs for genotypic characterization. We also sought to investigate the AST method and interpretation variation between VDLs, and to outline how such variation might impact on more detailed genotypic investigations as performed here.
Materials and Methods
Antimicrobial Susceptibility Test Data
Clinical AST data were collated by the Small Animal Veterinary Surveillance Network (SAVSNET) from four United Kingdom VDLs (A–D) between 1st April 2016 and 31st July 2018, including a unique sample identifier; submitting veterinary practice site postcode; report date; animal species; breed; sex and neuter status; bacterial species isolated, and AST results. Sample type/site (supplied as free text) was manually categorized into urine, ear(s), oro-nasopharyngeal and respiratory sites, feces, anal region including anal sacs, and other/mixed sites. SAVSNET holds ethical approval to collect such data from the University of Liverpool Ethics Committee (RETH0000964).
The manner with which bacterial species were described varied between VDLs, ranging from genus-level (e.g., Escherichia) alone to species-level (e.g., Escherichia coli). Similarly, bacteria were speciated according to varying methodologies, ranging from commercial biochemical tests (VDLs B–D) to MALDI-TOF (VDL A); Enterobacteriaceae were selected for further analysis. Laboratories utilized disk diffusion (VDL B-D) or minimum inhibitory concentration (MIC) (VDL A) approaches, and used different interpretation guidelines (CLSI, EUCAST, and BSAC). As some VDLs (B–D) only provided clinically interpreted AST results, analysis was restricted to interpreted results alone. Only antimicrobials associated with species-specific acquired resistance were summarized. Tested antimicrobials were grouped to class-level (Supplementary Material 1); ‘intermediate’ results were classified as ‘sensitive,’ with multi-drug resistance (MDR) defined as resistance to three or more classes (Magiorakos et al., 2012).
Veterinary Practice Site Demographic Data
The Royal College of Veterinary Surgeons (RCVS) Veterinary Practice Directory (RCVS, 2018) was interrogated on 12th October 2018, using R packages ‘rvest’ (Wickham, 2016) and ‘purrr’ (Henry, 2018), to extract information for all United Kingdom veterinary practice sites (Table 1); postcodes containing more than one site treating companion animals (n = 148) were designated ‘multi-site postcodes.’ The National Statistics Postcode Look-up was used to provide geographical information, including urban/rural status (NSPL, 2015).
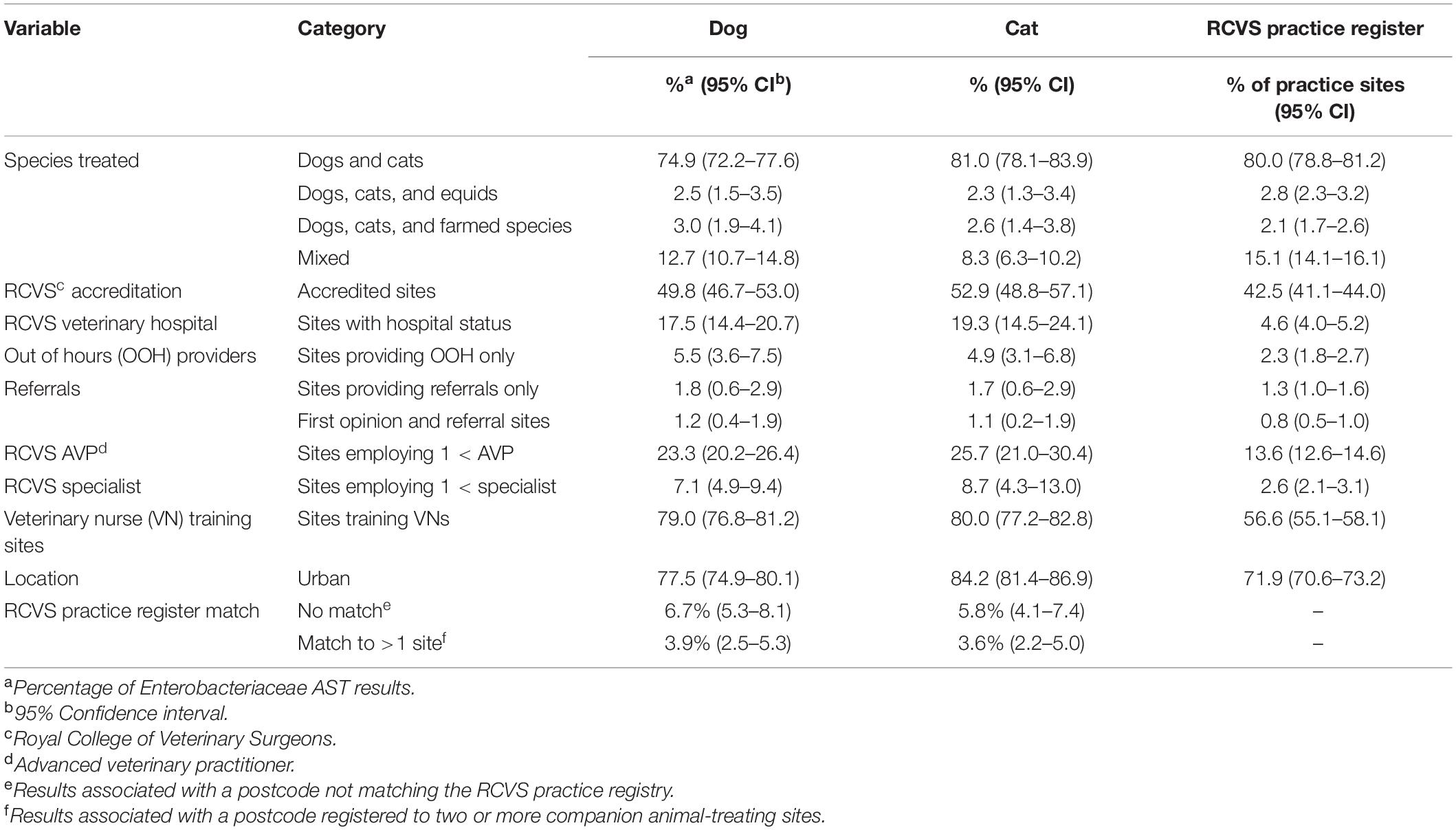
Table 1. Summary of the percentage of Enterobacteriaceae antimicrobial susceptibility test results originating from a range of veterinary practice site categories, as defined by the Royal College of Veterinary Surgeons (RCVS) on 12th October 2018, compared against the percentage of veterinary practice sites in the full RCVS practice register.
Animal Demographic Data
Dog and cat breeds were summarized to standardized breed terms, which were then grouped into genotypically similar groups. Three further breed groups were defined: crossbreeds, breeds not yet genetically classified (‘unclassified’), and breeds not recorded or recognizable (‘unknown’) (Singleton et al., 2020).
Multidrug Resistant Isolate Collection and Storage
Six VDLs (A–F) were approached regarding collection of Enterobacteriaceae isolates, of which three (A, E, F) participated. Submitted isolates needed to comply with one or more of the following criteria: (1) Phenotypic ESBL-producer, (2) MDR, or (3) non-susceptibility to one or more of the 3rd/4th generation cephalosporin, fluoroquinolone, polymyxin, or carbapenem classes. On receipt at the University of Liverpool, isolates (n = 148) were incubated under aerobic conditions for 18–24 h at 37°C on nutrient agar, and pure colonies stored at −80°C (MicrobankTM cryovial; Pro-Lab Diagnostics, United Kingdom).
Phenotypic Analyses
To mitigate for protocol variation between VDLs, disk diffusion ASTs were undertaken on all received isolates according to CLSI guidelines (CLSI, 2008). Antimicrobial disks (MAST Diagnostics; Liverpool, United Kingdom) represented a range of antimicrobial classes (Supplementary Material 2). Inhibition zones were measured after aerobic incubation at 37°C overnight, and classified as susceptible, intermediate or resistant, or ESBL-producing via a combination disk method (Supplementary Material 2; MAST Diagnostics; Liverpool, United Kingdom) (CLSI, 2008) ‘Intermediate’ results were considered ‘sensitive.’
Genotypic Analyses
Crude nucleic acid extracts were prepared prior to PCR amplification, with isolates classified as E. coli via presence of the uidA (McDaniels et al., 1996) or uspA genes (Bekal et al., 2003) (Supplementary Material 3). All isolates were tested for blaCTX–M (Boyd et al., 2004), with positive isolates characterized into blaCTX–M cluster groups 1, 2, or 9 (Batchelor et al., 2005; Hopkins et al., 2006; Carattoli et al., 2008). Isolates possessing blaCTX–M group 1 genes were characterized into blaCTX–M–1 or blaCTX–M–15 variants via real-time PCR (Isgren et al., 2019). All isolates were tested for presence of blaTEM, blaSHV or blaOXA (Dallenne et al., 2010), and those displaying potential AmpC phenotypes for blaCIT, blaDHA, blaACC, blaEBC and blaFOX (Perez-Perez and Hanson, 2002). All isolates were tested for presence of qnrA, qnrB, or qnrS, genes (Robicsek et al., 2006).
E. coli isolates were selected for whole genome sequencing (WGS) based on evidence of phenotypic ESBL production; MDR status; presence of plasmid-associated AmpC, or plasmid-associated fluoroquinolone resistance genes on PCR. Fragment libraries (NEBNext Ultra II FS Kit; ∼300 base pair inserts) were created from purified genomic DNA (Qiagen QIAmp DNA mini kit) and sequenced using a 2 × 150 base pair paired-end protocol (Illumina HiSeq; CGR, University of Liverpool; GenBank accession numbers: Supplementary Material 4). De novo assembly was performed using SPAdes 3.16.0 (Bankevich et al., 2012), and genome annotation via Prokka 1.14.5 (Seemann, 2014). In silico identification of MLST and AMR genes were analyzed via MLST 2.19.0 (Seemann, 2019), ABRicate 0.8 (Seemann, 2020a), and BLASTP searches. Core genome SNPs were determined via Snippy 4.4.5 (Seemann, 2020b); phylogenies were estimated via maximum likelihood phylogenetic analysis using raxml-ng (Kozlov et al., 2019), with a generalized time-reversible model and 1,000 bootstraps, and annotated using iTOL 4 (Letunic and Bork, 2019). Plasmids were identified via PlasmidFinder (Carattoli et al., 2014), summarizing incompatibility (Inc) group plasmids with 100% coverage and >80% identity.
Statistical Analyses
All statistical analyses were completed using R (v3.4.4). Descriptive proportions and 95% confidence intervals were adjusted for clustering within practice sites (bootstrap method, n = 5,000 samples) (AOD, 2016). Mixed effects logistic regression models exploring odds of E. coli resistance were fitted separately in dogs and cats (LME4, 2016), considering 3rd/4th generation cephalosporin resistance as a binary outcome variable. MDR, fluoroquinolone and potentiated penicillin resistance were also modeled (Supplementary Materials 5–13). Both practice site and VDL were included as random effects if a likelihood ratio test (LRT) suggested improved fit. Initial univariable screening considered a range of categorical explanatory variables, including sample type/site and animal or practice site demographic features (Supplementary Material 8). Explanatory variables were retained for multivariable analysis if a LRT indicated P ≤ 0.20 against a null model.
Multivariable models underwent step-wise backward elimination to minimize Akaike Information Criterion (AIC). Two-way interaction terms were retained if inclusion resulted in an AIC decrease. Multicollinearity was assessed using the Variance Inflation Factor (CAR, 2018). Statistical significance was defined as P < 0.05.
Results
AST Data – Laboratories A–D
Data were obtained for 29,330 canine and 8,279 feline Enterobacteriaceae isolates from 2,237 practice sites, corresponding to 49.1% (95% confidence interval, CI, 47.7–50.6) of registered companion animal-treating sites (Supplementary Material 14), contributing a median of seven canine (range 1–156) and three feline (range 1–166) isolates each. Though practice site details could not be ascertained in 6.7% (CI 5.3–8.1) of canine and 5.8% (CI 4.1–7.4) of feline AST reports, site demographic and animal population features of the remainder are summarized in Table 1 and Supplementary Material 15, respectively.
In total, 65 Enterobacteriaceae species were isolated (Supplementary Material 16). E. coli was most common, representing 69.4% (CI 68.7–70.0) of canine, and 90.5% (CI 89.8–91.3) of feline AST results, with urine being the most recorded sample type/site (58.6% of canine and 83.8% of feline E. coli isolates; Supplementary Material 17). MDR E. coli were reported in 14.1% of canine (CI 13.5–14.8) and 12.0% (CI 11.1–12.9) of feline isolates, MDR Proteus mirabilis in 3.1% (CI 2.5–3.6) of canine, and 7.1% (CI 4.0–10.1) of feline isolates, and MDR Klebsiella pneumoniae in 21.3% (CI 15.3–27.4) of canine and 38.5% (CI 20.1–56.9) of feline isolates (Table 2).
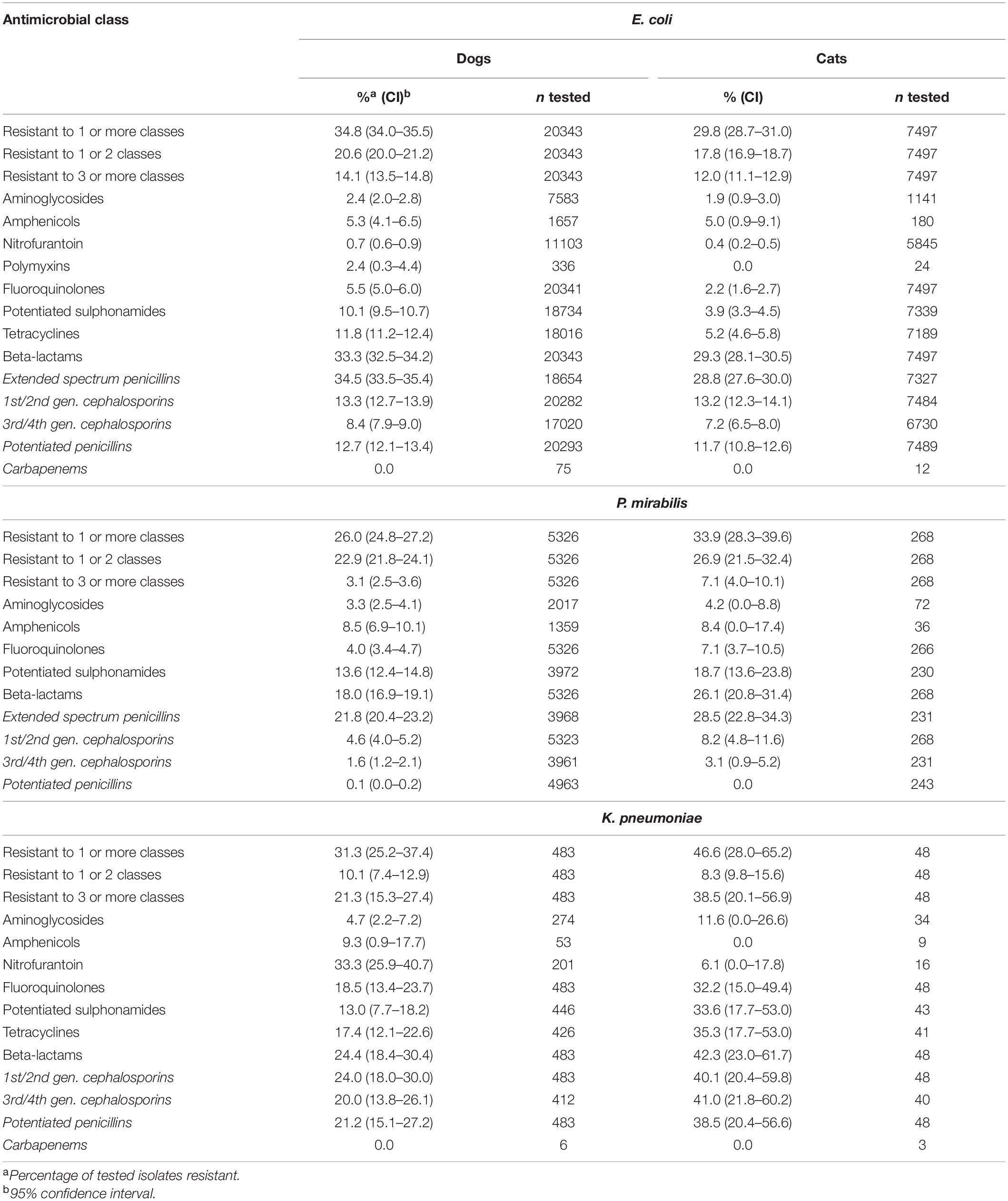
Table 2. Summary of canine and feline E. coli, P. mirabilis, and K. pneumoniae clinical antimicrobial class-level resistance.
3rd/4th Generation Cephalosporin Resistant E. coli
While prevalence remained relatively stable over the 2 years summarized (Figure 1), geographical variation was observed (Figure 2). Male dogs were associated with reduced odds of 3rd/4th generation cephalosporin resistance, whereas male cats were associated with increased odds (Table 3). Practice sites examining referral cases only were associated with increased odds, as were RCVS accredited sites. Compared to urine samples, the anal region and oronasopharyngeal/respiratory samples were associated with increased odds in dogs and cats, respectively (univariable findings, Supplementary Materials 18,19).
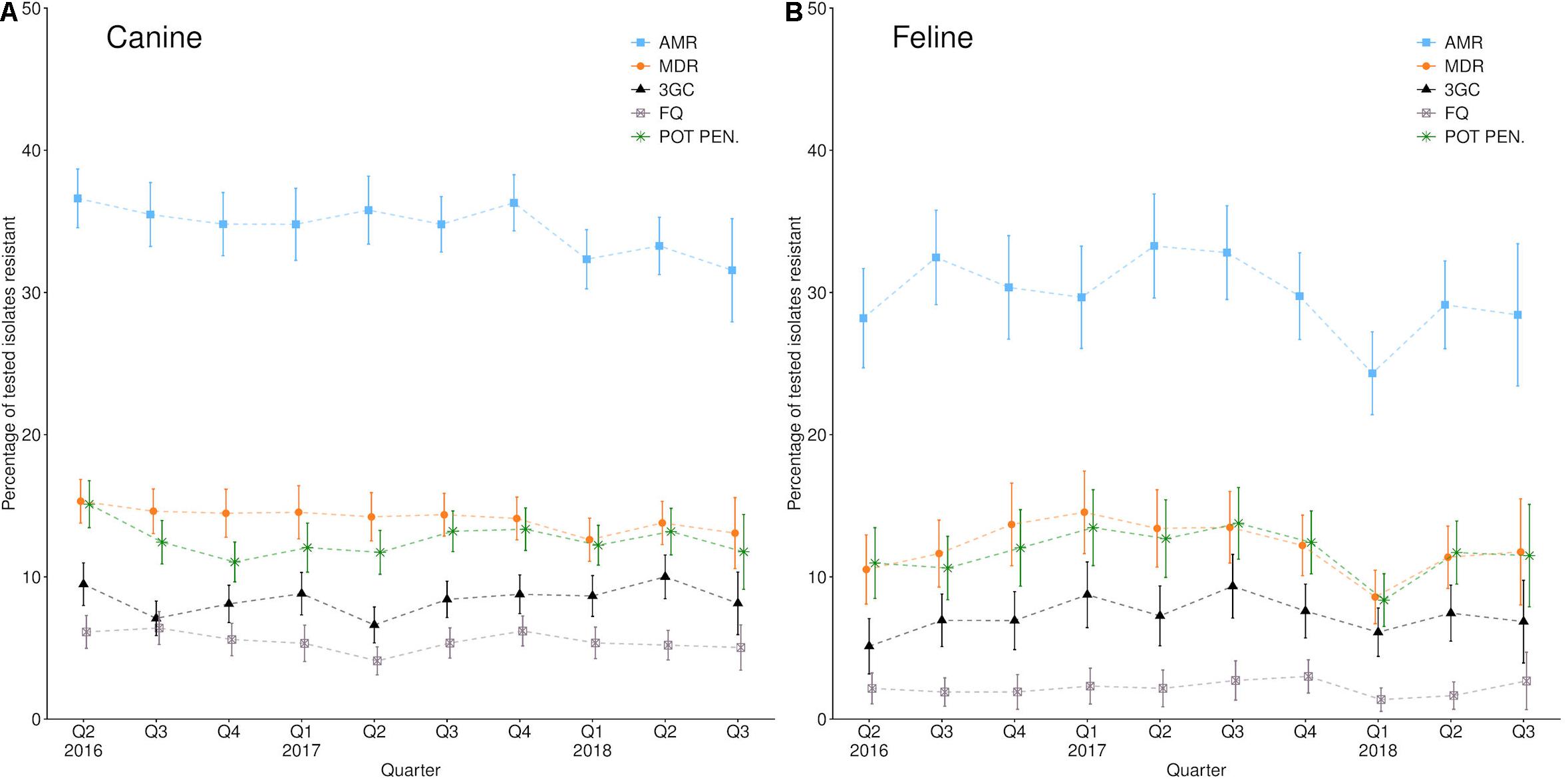
Figure 1. Percentage of (A) canine and (B) feline Enterobacteriaceae AST results reporting single-class resistance (AMR); multi-drug resistance (MDR); 3rd/4th generation cephalosporin resistance (3GC); fluoroquinolone resistance (FQ), or potentiated penicillin resistance (POT PEN.) between the 2nd quarter of 2016 and the third quarter of 2018. Error bars refer to 95% confidence intervals.
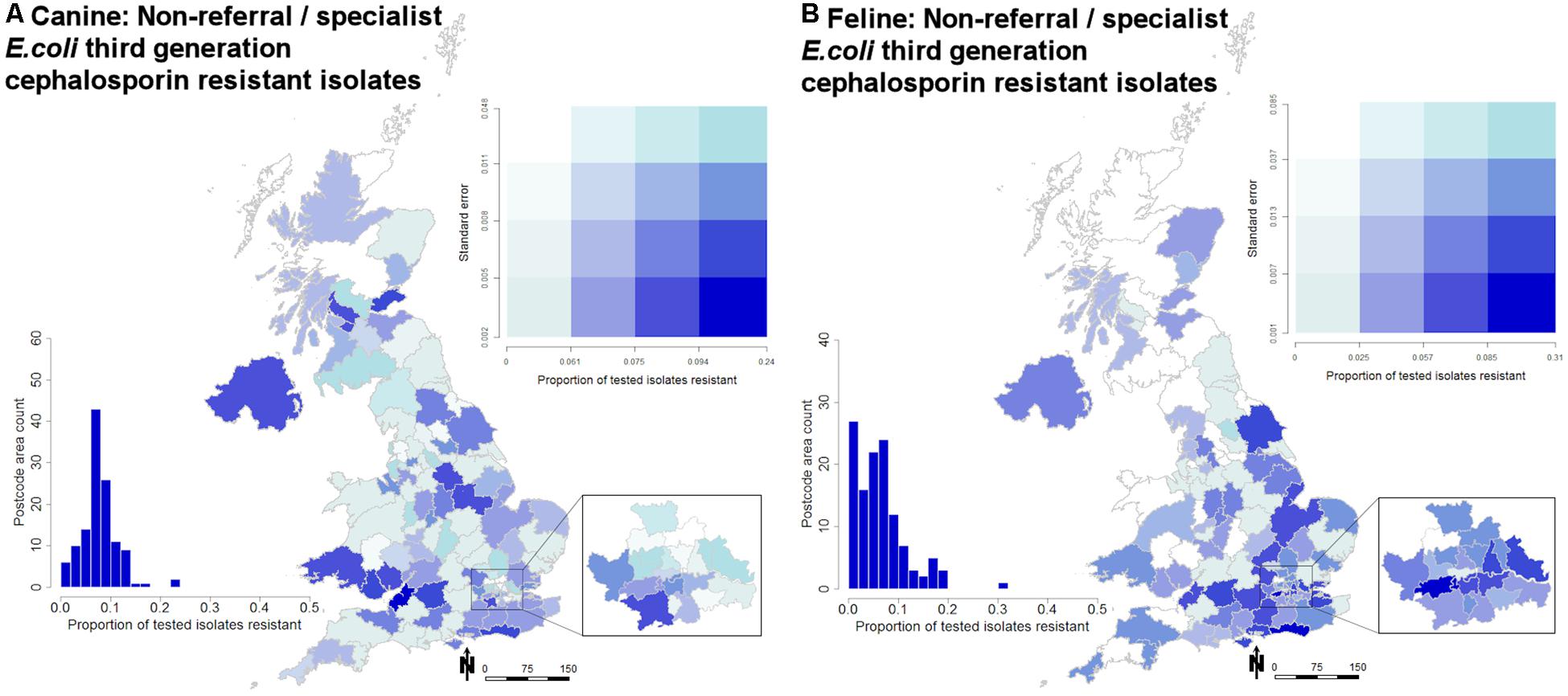
Figure 2. Quartile bivariate postcode map displaying the proportion of tested isolates that were submitted by non-referrals/specialist veterinary practice sites recording phenotypic 3rd/4th generation cephalosporin resistance in (A) dogs and (B) cats. Proportions are displayed against standard error. Scale is in Km. The embedded histogram displays the proportion of tested isolates reporting phenotypic 3rd/4th generation cephalosporin resistance against a count of postcode areas (n = 124).
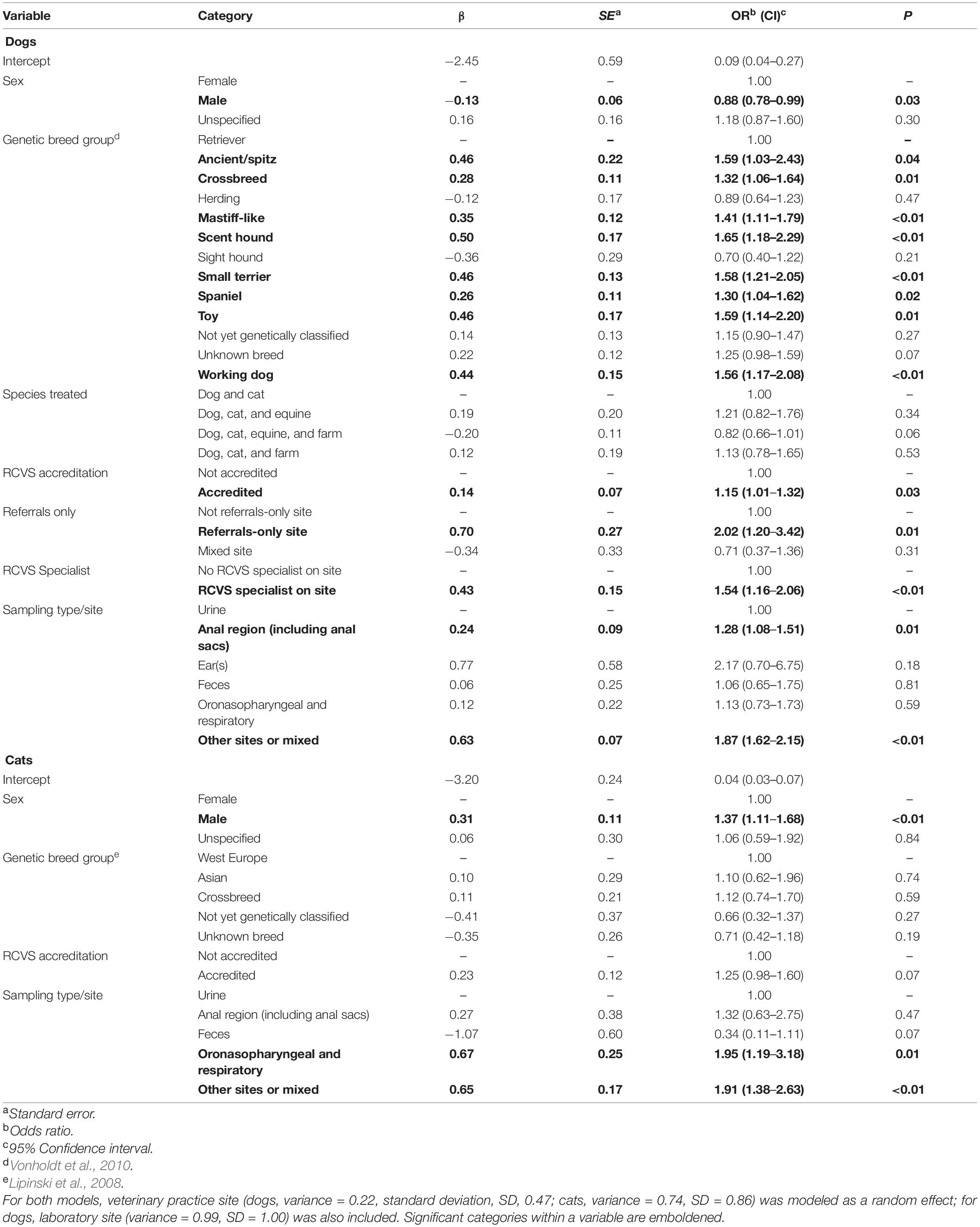
Table 3. Multivariable mixed effects logistic regression models displaying risk factors significantly associated with odds of an E. coli clinical isolate being classed as 3rd/4th generation cephalosporin resistant in dogs and cats.
Laboratory-Based Characterization of Resistant Enterobacteriaceae Isolates
Referring Laboratories
In total, 146 isolates (130 canine, 16 feline) were submitted for further characterization, including E. coli (n = 106), P. mirabilis (n = 12) and K. pneumoniae (n = 10) (Supplementary Material 4). Of these, 30.8% (CI 22.4–39.2) were indicated as having received antimicrobial therapy prior to sample submission, though 68.4%, (CI 60.2–76.6) were of unknown prior therapy status. Most isolates had been characterized via MIC (67.2% of isolates, CI 58.4–75.9), the remainder via disk diffusion (32.9%, CI 23.7–42.1). CLSI was the most utilized interpretation guideline (67.8%, CI 57.8–77.8), followed by EUCAST (19.8%, CI 9.9–29.7); BSAC (9.6%, CI 4.3–15.0), and CLSI-vet (2.7%, CI 0.0–5.3). 82.8% (CI 76.4–89.3) of isolates were reported by referring laboratories as MDR, and 97.8% (CI 95.4–100.0) were reported as phenotypically resistant to >1 3rd/4th generation cephalosporin, fluoroquinolones or polymyxins. An ESBL phenotype was indicated in 13.7% (CI 7.2–20.1) of submitted isolates, though 60.4% (CI 50.3–70.6) did not report an ESBL test.
University of Liverpool
Phenotypic results from our laboratory showed MDR in 83.6% (CI 77.2–90.0) of isolates; 71.3% (CI 63.7–78.9) were resistant to 3rd/4th generation cephalosporins and/or fluoroquinolones. An ESBL-producing phenotype was found in 34.2% (CI 26.3–42.2) of isolates (n = 41 E. coli; n = 5 K. pneumoniae; n = 2 P. mirabilis; n = 1 Proteus spp.; n = 1 Citrobacter koseri), whereas a pattern suggestive of AmpC-production was observed in 8.9% (CI 4.2-13.6) of isolates (Supplementary Material 4; n = 11 E. coli; n = 1 K. pneumoniae; n = 1 Enterobacter asburiae).
Genomic Analysis
In total, 95 E. coli isolates were selected for WGS based on evidence of phenotypic ESBL production (n = 38 isolates), MDR (n = 68) and/or presence of plasmid-associated AmpC (n = 24) or plasmid-associated fluoroquinolone resistance genes (n = 6) on PCR (Supplementary Material 4).
Via WGS, MLST results were obtained from 91 E. coli isolates, identifying 38 sequence types (ST), most notably ST131 (n = 10), ST162 (n = 9), ST88, ST372, and ST1193 (n = 5 each), ST73 (n = 3) and ST69 (n = 3). In total, 17 clonal complexes (CC) were identified, most notably CC131 (n = 10), CC469 (n = 9), CC23 (n = 8) and CC73 (n = 7) (Figure 3). Core genome-based SNP phylogenetic analysis showed ST clustering, though no clear correlation between animal species or sampling site/type could be elucidated.
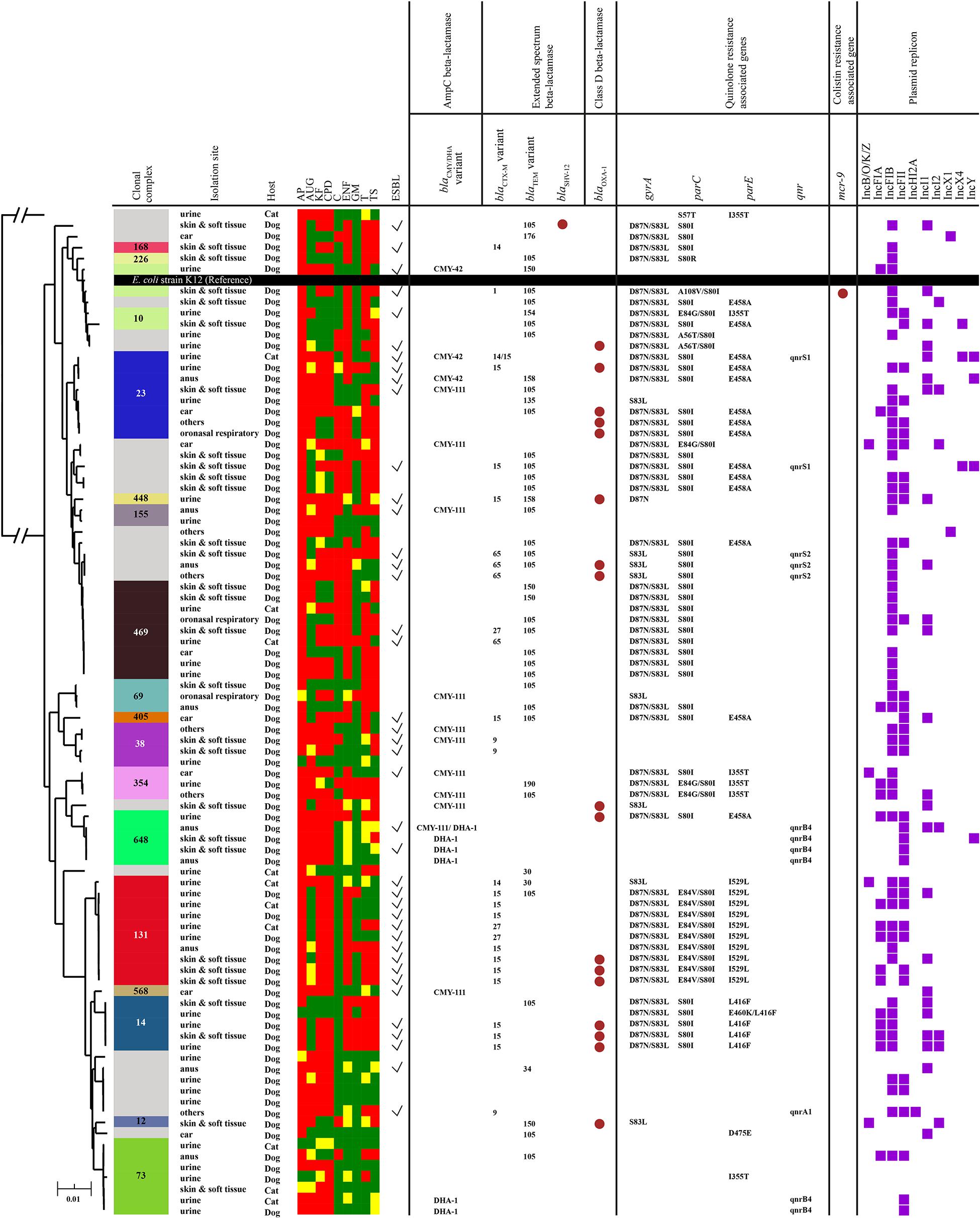
Figure 3. Maximum likelihood phylogenetic tree constructed by core genome SNPs of 91 E. coli isolates using the genome of E. coli K12 as a reference. Colored bands and numbers in the first column indicate clonal complex (CC) of the isolate (gray color indicates unassigned CC). A heatmap represents antimicrobial susceptibility (red = resistance; yellow = intermediate; green = susceptible). Brown circles in columns six and eleven represent the presence of beta-lactamase genes and the mcr-9 gene, respectively. The seventh – ninth columns represent QRDR mutations in gyrA, parC, and parE, respectively. The tenth column shows the presence/absence of the plasmid-mediated quinolone resistance gene, qnr, in each isolate. Purple squares in the last column indicate plasmid replicons identified in each isolate. Abbreviations for antibiotics tested here are as follows: AP, ampicillin; AUG, clavulanic acid potentiated amoxicillin; KF, cephalothin; CPD, cefpodoxime; C, chloramphenicol; ENF, enrofloxacin; GM, gentamicin; T, tetracycline; TS, trimethoprim potentiated sulfamethoxazole.
An ESBL gene was detected in 28/38 phenotypic ESBL-producers. blaCTX–M genes predominated (n = 27); blaCTX–M–15 was most common (n = 15), followed by blaCTX–M–65 (n = 4), blaCTX–M –27 (n = 3), blaCTX–M–14 (n = 3), blaCTX–M–9 (n = 2), and blaCTX–M–1 (n = 1). In addition, blaSHV–12 (n = 1) and the inhibitor resistant blaTEM–158 (n = 2) and blaTEM–154 (n = 1) were also detected. All ten ST131 isolates were associated with ESBL genes, with blaCTX–M–15 (n = 7) most prevalent. Plasmid-associated AmpC genes were detected in 19 E. coli isolates, comprising blaCMY–111 (n = 11), blaDHA–1 (n = 6), and blaCMY–42 (n = 3). Of broad spectrum β-lactamases, blaTEM–105 was most common (n = 29), followed by blaOXA–1 (n = 17), blaTEM–150 (n = 4), blaTEM–135, blaTEM–176 and blaTEM–190 (n = 1 each). Two inhibitor resistant variants, blaTEM–30 (n = 2) and blaTEM–34 (n = 1), were also detected.
Presence of both ESBL and AmpC or inhibitor resistant TEM variant enzymes in the same isolate can produce a phenotypic ‘masking’ effect on double disk ESBL testing, owing to the ability of AmpC to hydrolyze both clavulanic acid-potentiated and non-clavulanic acid potentiated cephalosporins (Jacoby, 2009). This can potentially result in an under-estimate of ESBL prevalence on phenotypic testing, as potentially shown here with non-phenotypic ESBL producers found to be carrying ESBL genes. This was likely due to a masking effect of inhibitor resistant blaTEM–154 in the first isolate; blaTEM–158 and blaCMY–42 in the second, and blaCTX–M–9 and blaCMY–111 in the third isolate. An ESBL gene was not detected in 10 phenotypic ESBL E. coli isolates, with isolates carrying plasmid-associated AmpC (blaCMY–111, n = 3; blaDHA–1, n = 1) or broad spectrum β-lactamase (blaOXA–1, n = 1) genes alone, or co-carried with blaCMY–111 and blaTEM–105 (n = 2), blaCMY–42 and blaTEM–150 (n = 1), blaCMY–111 and blaDHA–1 (n = 1), and blaDHA–1 and blaTEM–34 (n = 1), respectively. An ESBL (blaCTX–M–14 and blaCTX–M–15) and AmpC (blaCMY–42) MDR isolate also carried the narrow-spectrum β-lactamase gene blaLAP–2.
Mutations in the gyrA, parC, or parE quinolone resistance determining region (QRDR) were found in 62 isolates. Twelve isolates carried plasmid-associated quinolone resistance genes (qnrA1, n = 1; qnrB4, n = 6; qnrS1, n = 2; qnrS2, n = 3), though only five had QRDR mutations, and none were associated with significant fluoroquinolone resistance. More broadly, the overall median number of AMR genes per isolate was 6 (range 0–13). Other prominent AMR genes included aadA1 (n = 23), strA (n = 34), strB (n = 35), sul1 (n = 35), sul2 (n = 42), tetA (n = 25), tetB (n = 26), tetR (n = 25), and dfrA17 (n = 30). An ESBL (blaCTX–M–9) ST372 MDR isolate also carried a plasmid-mediated colistin resistance gene, mcr-9 (Supplementary Material 4).
A median of 2 (range 0–4) Inc plasmids per isolate was found, with IncFIB being most numerous (n = 59), followed by IncFII (n = 35), IncI (n = 25) IncFIA (n = 14), IncY (n = 5), IncR (n = 3), IncX4 (n = 3), IncX1 (n = 2), and IncHI2 (n = 1). Though plasmids were widely distributed across STs and resistance genes, nine of ten ST131 isolates possessed F-type plasmids (IncFIB, n = 7; IncFII, n = 7; IncFIA, n = 5). Of blaTEM–105 isolates (n = 29), 26 possessed IncFIB, and of 11 blaCMY–111 isolates, 8 possessed IncFIB. The mcr-9 positive isolate had three plasmids: IncFIB, IncFII, and IncHI2, with IncH12 plasmids previously reported as associated with mcr-9 (Kieffer et al., 2019).
Discussion
Here we have adopted an integrated health informatics and bioinformatics approach to conduct nationally representative surveillance of important clinical AMR trends in companion animals. We have also demonstrated that clinical AMR isolates can be retained by VDLs and further characterized to reveal key AMR insights of one health importance.
Resistance trends in Enterobacteriaceae are of critical importance to human health (Tacconelli et al., 2018). Considering the inherent one health nature of AMR (O’Neill, 2016), it is crucial to gain further understanding of AMR in our companion animal populations. Through collating AST results from half of companion animal-treating practice sites in the United Kingdom, with data being broadly comparative with both the estimated companion animal population (Aegerter et al., 2017) and demographic features of the RCVS practice register (RCVS, 2018), we believe these data are nationally representative. However, mixed species practice sites were under-represented in our dataset. Veterinary practitioners employed in different sectors (companion animal, farm etc.) have reported varied levels of support for ASTs (De Briyne et al., 2013), a disparity perhaps reflected in actual AST results here. Such findings can serve as a stimulus for localized resistance trend monitoring, potentially providing a powerful tool and motivator for antimicrobial stewardship.
Antimicrobial classes rarely (or never) prescribed to companion animals, for example carbapenems (Singleton et al., 2017), were only rarely reported as being tested here. Critically important antimicrobials currently only used in medical practice should not be recommended for veterinary use. However, there would be value in routinely reporting resistance trends to such antimicrobials in veterinary isolates for public health purposes, as demonstrated by our findings relating to colistin resistance here. It should also be noted that differences between AST interpretation guidelines (e.g., EUCAST and CLSI) limited our ability to compare laboratories in this study, re-affirming a need for greater harmonization between laboratories. AST data summarized here also does not include an individual animal identifier, hence it was not possible to designate multiple submissions originating from the same animal. To ameliorate this limitation, we would support efforts at increasing integration between practice and laboratory information management systems.
Considering the above, individual class-level resistance prevalence was generally lower than reported in other studies, though MDR prevalence was broadly comparative (Marques et al., 2016). While others have classified intermediate susceptibility results as resistant, potentially increasing resistance prevalence (VMD, 2019), doing so here would not have elevated findings to levels comparative with previous study (for example, re-classifying intermediate susceptibility findings as resistant would have increased E. coli 3rd generation cephalosporin resistance prevalence in dogs from 8.4 to 10.6%). Previous surveys have largely utilized data supplied by university-based diagnostic laboratories analyzing referral populations (Hernandez et al., 2014; Marques et al., 2016), and here we found increased odds of resistance in isolates originating from referral-only practice sites for dogs. Antimicrobial use prior to culture has been shown to increase odds of isolating resistant bacteria (Hernandez et al., 2014). It is possible that antibiotic therapy prior to referral might in part explain these findings, suggesting a need to stratify surveillance into first opinion and referral populations, broadly in line with human surveillance (PHE, 2020).
Considering animal-level features, genetic breed group, sex and neuter status were associated with 3rd/4th generation cephalosporin resistance odds variation in both species. These findings potentially reflect anatomical (Hernandez et al., 2014) or behavioral differences (Chhetri et al., 2015) between animals, possibly resulting in increased likelihood of bacterial infection or resistance-selecting empirical antimicrobial prescription in some cases. Indeed, we have recently identified such variation in prescribing practices (Singleton et al., 2020). Regarding sample type/site, the anal region in dogs and, oronasopharyngeal and respiratory samples in cats were associated with increased odds. Though resistant E. coli isolated from canine nasal or respiratory sites have been described (Morrissey et al., 2016), it has only rarely been implicated as a causative pathogen in human intensive care settings (de Lastours et al., 2015). In dogs and cats, Enterobacteriaceae are considered minor components of oral, nasopharyngeal and respiratory microbiota, and their role as respiratory pathogens remains debatable (Dorn et al., 2017). Similarly, it is difficult to determine whether anal region samples reflect intestinal carriage or clinical infection, though could be considered to pose a public health risk, nonetheless. Several bacterial species usually considered contaminants were also reported within AST data (e.g., Kluyvera spp.; Pantoea spp. etc.); doing so without thorough evaluation of their association with infection is likely to encourage potentially unnecessary antimicrobial prescription.
There are several Enterobacteriaceae resistance trends of critical one health importance, not least ESBL- or AmpC-production, fluoroquinolone resistance, carbapenemase producing Enterobacteriaceae, and colistin resistance (MacNair et al., 2018). However, due to the primarily clinical purpose behind AST results analyzed here, some phenotypic tests were only rarely reported. Hence, here we aimed to investigate these isolates to gain further insight into dynamics of resistance development and transmission in the companion animal clinical population, utilizing isolates supplied by multiple independent diagnostic laboratories.
Regarding WGS findings, although ST372 E. coli has been previously primarily associated with canine infections and relatively rare resistance (Valat et al., 2020), here we identified an MDR ST372 also possessing mcr-9, a recently identified plasmid-associated colistin resistance gene (Carroll et al., 2019). In medical practice, colistin is considered an antimicrobial of ‘last resort,’ and reported emergence of plasmid-mediated colistin resistance in 2015 instigated widespread concern (Liu et al., 2016). Although there is some debate regarding the clinical relevance of mcr-9 (Tyson et al., 2020) and when it first originated (Elbediwi et al., 2021), our finding represents its first identification in a companion animal, and the first identification of a plasmid-associated colistin resistance gene in companion animals in the United Kingdom. Although companion animals are not prescribed colistin in the United Kingdom, they are relatively frequent recipients of another member of the polymyxin class, polymyxin B (Singleton et al., 2017). Concern has been raised over whether such use might be selecting for colistin resistance (Scott et al., 2019), though this remains to be determined in Enterobacteriaceae. Nevertheless, these findings serve to further reinforce the role of companion animals as carriers of resistance mechanisms of public health importance, and the need for surveillance to highlight the emergence of such trends.
A diverse collection of ESBL genes were found in E. coli isolates undergoing WGS, with the blaCTX–M (1, 9, 14, 15, 27, and 65) family dominating. In people, blaCTX–M–14 and blaCTX–M–15 are of greatest clinical importance (Canton et al., 2012), whereas agricultural species are more commonly associated with blaCTX–M–1 carriage (Abraham et al., 2018). In companion animals, blaCTX–M–1 (Wedley et al., 2017), blaCTX–M–14 (Sun et al., 2016), and blaCTX–M–15 (Timofte et al., 2016) have each been identified. Here blaCTX–M–15 was most prevalent; concerningly, the human pandemic clone ST131 predominated, further demonstrating links between people and companion animals for important resistance and pathogenic clones (Zhang, 2016). This clone has been detected in companion animal clinical isolates previously, and is considered a driver of blaCTX–M–15 dissemination in human and animal populations (Timofte et al., 2016). F-type Inc plasmids were also frequently identified, this group of plasmids (particularly IncFII) being commonly implicated with global dissemination of blaCTX–M (Canton et al., 2012). Inhibitor resistant ESBL genes were also detected at low frequency, the blaTEM–158 gene having been previously reported in a companion animal referral hospital in the United Kingdom (Tuerena et al., 2016).
Although less frequently identified than ESBL genes, AmpC genes were also identified in these isolates. Despite blaCMY–2 being the most well distributed blaCMY allele worldwide (Jacoby, 2009), including in companion animals (Wedley et al., 2011), it was not detected here. Instead, the comparatively recently identified blaCMY–111 (Kao et al., 2018), representing the first identification in companion animals, and blaDHA–1, this gene only recently reported in European companion animals (Belas et al., 2014), were found to predominate. Although the blaCMY gene has many variants (Jacoby, 2009), and as such a measured view of this novel finding should be taken, our results do suggest increased AmpC diversity in companion animals. We also detected ST410 in this population, this ST being previously implicated in human and companion animal infection (Timofte et al., 2016), including harboring a carbapenemase resistance gene in a sample of canine origin (Reynolds et al., 2019).
Regarding treatment of ESBL and/or AmpC-mediated infections in companion animals, the appropriateness of using alternative agents, such as the carbapenems and piperacillin-tazobactam, is a topic of ongoing debate in the medical field due to concerns surrounding developing resistance to these ‘last report’ agents (Tamma and Mathers, 2021). Indeed the prescription of such agents to companion animals is not recommended (BSAVA, 2018), severely limiting treatment options for such cases. Accordingly, whilst emergence of these troubling clinical resistance trends in companion animals is often viewed primarily through the lens of associated public health risk, it should also be remembered that enhanced treatment failure risk may well also carry an increased risk to animal welfare.
Quinolone resistance mechanisms are relatively complex, and consist of interactions between chromosomal mutations and plasmid-mediated genes (Hamed et al., 2018). In this study, chromosomal mutations were commonplace, contrasting with the relative scarcity of plasmid-mediated genes. This finding suggests a greater role of chromosomal mutations in conferring clinical resistance in companion animals, in agreement with previous study (de Jong et al., 2018). Considering AMR genes identified more widely, although many of the AMR genes identified here are commonly associated with plasmid types frequently identified in this study, specific, in-depth investigation was out of the scope of this study. Hence, though unlikely, it is possible that some of the assumed transmissible AMR genes discussed here might be chromosomal in origin.
Although this study was successful in identifying clinical isolates of one health importance, we revealed further evidence of AST method and interpretation variation between VDLs. When confirmatory additional testing was performed at the University of Liverpool, although a similar percentage of isolates were reported as MDR by referring laboratories (82.8%) to that found at Liverpool (83.6%), ESBL testing was only reported for a minority of referring VDLs, potentially systematically under-estimating the impact of ESBLs on companion animal practice, and the potential risk posed to public health. This is likely due notification of ESBL presence being of an arguably greater importance for public health/surveillance than for clinical decision making. Hence, we suggest an urgent need to harmonize standard AST testing procedures, methods, and interpretation more closely, including tests of primary public health/surveillance relevance, if companion animal clinical bacterial isolates are to be used for wide scale surveillance in the future.
Conclusion
This study demonstrated a method by which clinical AST results could be repurposed for near real-time passive and active AMR clinical surveillance at a nationally representative scale in companion animals, providing insights of importance and use to both veterinary practitioners and public health. Using Enterobacteriaceae as an exemplar, we found MDR to be common, with prevalence remaining relatively static between 2016 and 2018. We also conducted a pilot study to determine whether MDR E. coli isolates could be efficiently retrieved from VDLs and further characterized at the University of Liverpool, revealing presence of resistance genes and sequence types of importance to human and animal health.
Data Availability Statement
Data utilized during this study is available upon written request to DS (d.a.singleton@liverpool.ac.uk). Whole genome sequence data is freely available via GenBank (accession numbers available, Supplementary Material 4).
Author Contributions
NW, AR, GP, P-JN, SD, and FS-V conceived the study. DT, StS, MJ, and LR contributed data and isolates to this project. DS and ShS completed laboratory work. PP, RB, and KB completed or supervised bioinformatic components of this work. DS completed primary analyses and wrote the manuscript under the supervision of NW. All authors contributed to the draft review.
Funding
This work was funded by The Veterinary Medicines Directorate (VM0520), the University of Liverpool and SAVSNET. We are extremely grateful for the support and major funding from BBSRC (BB/N019547/1) and BSAVA. KB was funded through a Wellcome Trust Clinical Research Career Development Award (106690/A/14/Z), and supported RB through a United Kingdom Research and Innovation Medical Research Council New Investigator Research Grant (MR/R020787/1).
Conflict of Interest
MJ and LR were employed by the veterinary diagnostic company IDEXX Laboratories Ltd. StS was employed by the veterinary diagnostic company NationWide Laboratories/CAPL Ltd. Although employed by the University of Liverpool, DT held primary responsibility for managing a commercial veterinary diagnostic laboratory within the university.
The remaining authors declare that the research was conducted in the absence of any commercial or financial relationships that could be construed as a potential conflict of interest.
Publisher’s Note
All claims expressed in this article are solely those of the authors and do not necessarily represent those of their affiliated organizations, or those of the publisher, the editors and the reviewers. Any product that may be evaluated in this article, or claim that may be made by its manufacturer, is not guaranteed or endorsed by the publisher.
Acknowledgments
We wish to thank data providers both in veterinary practice (VetSolutions, Teleos, CVS, and other practitioners) and especially in veterinary diagnostic laboratories, without whose support and participation this research would not be possible. Finally, we are especially grateful for the help and support provided by SAVSNET team members Susan Bolan, Bethaney Brant, and Steven Smyth.
Supplementary Material
The Supplementary Material for this article can be found online at: https://www.frontiersin.org/articles/10.3389/fmicb.2021.700698/full#supplementary-material
References
Abraham, S., Kirkwood, R. N., Laird, T., Saputra, S., Mitchell, T., Singh, M., et al. (2018). Dissemination and persistence of extended-spectrum cephalosporin-resistance encoding IncI1-blaCTXM-1 plasmid among Escherichia coli in pigs. ISME J. 12, 2352–2362. doi: 10.1038/s41396-018-0200-3
Aegerter, J., Fouracre, D., and Smith, G. C. (2017). A first estimate of the structure and density of the populations of pet cats and dogs across Great Britain. PLoS One 12:e0174709. doi: 10.1371/journal.pone.0174709
AOD. (2016). AOD R Packages. Available online at: http://cran.r-project.org/package=aod (accessed January 26, 2019).
Bankevich, A., Nurk, S., Antipov, D., Gurevich, A. A., Dvorkin, M., Kulikov, A. S., et al. (2012). SPAdes: a new genome assembly algorithm and its applications to single-cell sequencing. J. Comput. Biol. 19, 455–477. doi: 10.1089/cmb.2012.0021
Batchelor, M., Hopkins, K., Threlfall, E. J., Clifton-Hadley, F. A., Stallwood, A. D., Davies, R. H., et al. (2005). bla(CTX-M) genes in clinical Salmonella isolates recovered from humans in England and Wales from 1992 to 2003. Antimicrob. Agents Chemother. 49, 1319–1322. doi: 10.1128/AAC.49.4.1319-1322.2005
Bekal, S., Brousseau, R., Masson, L., Prefontaine, G., Fairbrother, J., and Harel, J. (2003). Rapid identification of Escherichia coli pathotypes by virulence gene detection with DNA microarrays. J. Clin. Microbiol. 41, 2113–2125.
Belas, A., Salazar, A. S., Gama, L. T., Couto, N., and Pomba, C. (2014). Risk factors for faecal colonisation with Escherichia coli producing extended-spectrum and plasmid-mediated AmpC beta-lactamases in dogs. Vet. Rec. 175:202. doi: 10.1136/vr.101978
Bezabih, Y. M., Sabiiti, W., Alamneh, E., Bezabih, A., Peterson, G. M., Bezabhe, W. M., et al. (2021). The global prevalence and trend of human intestinal carriage of ESBL-producing Escherichia coli in the community. J. Antimicrob. Chemother. 76, 22–29. doi: 10.1093/jac/dkaa399
Boyd, D. A., Tyler, S., Christianson, S., McGeer, A., Muller, M. P., Willey, B. M., et al. (2004). Complete nucleotide sequence of a 92-kilobase plasmid harboring the CTX-M-15 extended-spectrum beta-lactamase involved in an outbreak in long-term-care facilities in Toronto. Can. Antimicrob. Agents Chemother. 48, 3758–3764. doi: 10.1128/AAC.48.10.3758-3764.2004
BSAVA (2018). BSAVA/SAMSoc Guide to Responsible Use of Antibacterials: PROTECT ME. Available online at: https://www.bsavalibrary.com/content/book/10.22233/9781910443644 (accessed November 11, 2018).
Canton, R., Gonzalez-Alba, J. M., and Galan, J. C. (2012). CTX-M enzymes: origin and diffusion. Front. Microbiol. 3:110. doi: 10.3389/fmicb.2012.00110
CAR (2018). CAR R Package. Available online at: https://cran.r-project.org/package=car (accessed June 27, 2021).
Carattoli, A., Garcia-Fernandez, A., Varesi, P., Fortini, D., Gerardi, S., Penni, A., et al. (2008). Molecular epidemiology of Escherichia coli producing extended-spectrum beta-lactamases isolated in Rome, Italy. J. Clin. Microbiol. 46, 103–108. doi: 10.1128/JCM.01542-07
Carattoli, A., Zankari, E., García-Fernández, A., Voldby Larsen, M., Lund, O., Villa, L., et al. (2014). In silico detection and typing of plasmids using PlasmidFinder and plasmid multilocus sequence typing. Antimicrob. Agents Chemother. 58, 3895–3903. doi: 10.1128/AAC.02412-14
Carroll, L. M., Gaballa, A., Guldimann, C., Sullivan, G., Henderson, L. O., and Wiedmann, M. (2019). Identification of novel mobilized colistin resistance gene mcr-9 in a multidrug-resistant, colistin-susceptible Salmonella enterica. MBio 10:e00853-19. doi: 10.1128/mBio.00853-19
Chhetri, B. K., Berke, O., Pearl, D. L., and Bienzle, D. (2015). Comparison of risk factors for seropositivity to feline immunodeficiency virus and feline leukemia virus among cats: a case-case study. BMC Vet. Res. 11:30. doi: 10.1186/s12917-015-0339-3
CLSI (2008). Performance Standards for Antimicrobial Disk and Dilution Susceptibility Tests for Bacteria Isolated From Animals; Approved Standard, 3rd Edn. Wayne, PA: Clinical and Laboratory Standards Institute.
Dallenne, C., Da Costa, A., Decre, D., Favier, C., and Arlet, G. (2010). Development of a set of multiplex PCR assays for the detection of genes encoding important beta-lactamases in Enterobacteriaceae. J. Antimicrob. Chemother. 65, 490–495. doi: 10.1093/jac/dkp498
De Briyne, N., Atkinson, J., Pokludova, L., Borriello, S. P., and Price, S. (2013). Factors influencing antibiotic prescribing habits and use of sensitivity testing amongst veterinarians in Europe. Vet. Rec. 173:475. doi: 10.1136/vr.101454
de Jong, A., Muggeo, A., El Garch, F., Moyaert, H., de Champs, C., and Guillard, T. (2018). Characterization of quinolone resistance mechanisms in Enterobacteriaceae isolated from companion animals in Europe (ComPath II study). Vet. Microbiol. 216, 159–167. doi: 10.1016/j.vetmic.2018.02.002
de Lastours, V., Malosh, R. E., Aiello, A. E., and Foxman, B. (2015). Prevalence of Escherichia coli carriage in the oropharynx of ambulatory children and adults with and without upper respiratory symptoms. Ann. Am. Thorac. Soc. 12, 461–463. doi: 10.1513/AnnalsATS.201412-586LE
Dorn, E. S., Tress, B., Suchodolski, J. S., Nisar, T., Ravindran, P., Weber, K., et al. (2017). Bacterial microbiome in the nose of healthy cats and in cats with nasal disease. PLoS One 12:e0180299. doi: 10.1371/journal.pone.0180299
Elbediwi, M., Li, Y., Paudyal, N., Pan, H., Li, X., Xie, S., et al. (2019). Global Burden of colistin-resistant bacteria: mobilized colistin resistance genes study (1980–2018). Microorganisms 7:461. doi: 10.3390/microorganisms7100461
Elbediwi, M., Pan, H., Zhou, X., Rankin, S. C., Schifferli, D. M., and Yue, M. (2021). Detection of mcr-9-harbouring ESBL-producing Salmonella Newport isolated from an outbreak in a large-animal teaching hospital in the USA. J. Antimicrob. Chemother. 76, 1107–1109. doi: 10.1093/jac/dkaa544
El-Sayed Ahmed, M. A. E.-G., Zhong, L.-L., Shen, C., Yang, Y., Doi, Y., and Tian, G.-B. (2020). Colistin and its role in the Era of antibiotic resistance: an extended review (2000–2019). Emerg. Microbes Infect. 9, 868–885. doi: 10.1080/22221751.2020.1754133
Hamed, S. M., Elkhatib, W. F., El-Mahallawy, H. A., Helmy, M. M., Ashour, M. S., and Aboshanab, K. M. A. (2018). Multiple mechanisms contributing to ciprofloxacin resistance among Gram negative bacteria causing infections to cancer patients. Sci. Rep. 8:12268. doi: 10.1038/s41598-018-30756-4
Henry. (2018). purrr R package. Available online at: https://cran.r-project.org/web/packages/purrr/purrr.pdf (accessed April 16, 2020).
Hernandez, J., Bota, D., Farbos, M., Bernardin, F., Ragetly, G., and Medaille, C. (2014). Risk factors for urinary tract infection with multiple drug-resistant Escherichia coli in cats. J. Feline Med. Surg. 16, 75–81. doi: 10.1177/1098612X13504407
Hopkins, K. L., Batchelor, M. J., Liebana, E., Deheer-Graham, A. P., and Threlfall, E. J. (2006). Characterisation of CTX-M and AmpC genes in human isolates of Escherichia coli identified between 1995 and 2003 in England and Wales. Int. J. Antimicrob. Agents 28, 180–192. doi: 10.1016/j.ijantimicag.2006.03.027
Iredell, J., Brown, J., and Tagg, K. (2016). Antibiotic resistance in Enterobacteriaceae: mechanisms and clinical implications. BMJ 352:h6420. doi: 10.1136/bmj.h6420
Isgren, C. M., Edwards, T., Pinchbeck, G. L., Winward, E., Adams, E. R., Norton, P., et al. (2019). Emergence of carriage of CTX-M-15 in faecal Escherichia coli in horses at an equine hospital in the UK; increasing prevalence over a decade (2008–2017). BMC Vet. Res. 15:268. doi: 10.1186/s12917-019-2011-9
Jacoby, G. A. (2009). AmpC beta-lactamases. Clin. Microbiol. Rev. 22, 161–182. doi: 10.1128/CMR.00036-08
Kao, C.-Y., Chen, J.-W., Liu, T.-L., Yan, J.-J., and Wu, J.-J. (2018). Comparative genomics of Escherichia coli sequence type 219 clones from the same patient: evolution of the IncI1 bla(CMY)-carrying plasmid in vivo. Front. Microbiol. 9:1518. doi: 10.3389/fmicb.2018.01518
Kieffer, N., Royer, G., Decousser, J.-W., Bourrel, A.-S., Palmieri, M., Ortiz De La Rosa, J.-M., et al. (2019). mcr-9, an inducible gene encoding an acquired phosphoethanolamine transferase in Escherichia coli, and its origin. Antimicrob. Agents Chemother. 63:e00965-19. doi: 10.1128/AAC.00965-19
Kozlov, A. M., Darriba, D., Flouri, T., Morel, B., and Stamatakis, A. (2019). RAxML-NG: a fast, scalable and user-friendly tool for maximum likelihood phylogenetic inference. Bioinformatics 35, 4453–4455. doi: 10.1093/bioinformatics/btz305
Lei, L., Wang, Y., Schwarz, S., Walsh, T. R., Ou, Y., Wu, Y., et al. (2017). mcr-1 in Enterobacteriaceae from companion animals. Beijing, China, 2012-2016. Emerg. Infect. Dis. 23, 710–711. doi: 10.3201/eid2304.161732
Letunic, I., and Bork, P. (2019). Interactive tree of life (iTOL) v4: recent updates and new developments. Nucleic Acids Res. 47, W256–W259. doi: 10.1093/nar/gkz239
Lipinski, M. J., Froenicke, L., Baysac, K. C., Billings, N. C., Leutenegger, C. M., Levy, A. M., et al. (2008). The ascent of cat breeds: genetic evaluations of breeds and worldwide random-bred populations. Genomics 91, 12–21. doi: 10.1016/j.ygeno.2007.10.009
Liu, Y. Y., Wang, Y., Walsh, T. R., Yi, L. X., Zhang, R., Spencer, J., et al. (2016). Emergence of plasmid-mediated colistin resistance mechanism MCR-1 in animals and human beings in China: a microbiological and molecular biological study. Lancet Infect. Dis. 16, 161–168. doi: 10.1016/S1473-3099(15)00424-7
LME4 (2016). LME4 R Package. Available online at: https://cran.r-project.org/package=lme4 (accessed June 22, 2021).
MacNair, C. R., Stokes, J. M., Carfrae, L. A., Fiebig-Comyn, A. A., Coombes, B. K., Mulvey, M. R., et al. (2018). Overcoming mcr-1 mediated colistin resistance with colistin in combination with other antibiotics. Nat. Commun. 9:458. doi: 10.1038/s41467-018-02875-z
Magiorakos, A. P., Srinivasan, A., Carey, R. B., Carmeli, Y., Falagas, M. E., Giske, C. G., et al. (2012). Multidrug-resistant, extensively drug-resistant and pandrug-resistant bacteria: an international expert proposal for interim standard definitions for acquired resistance. Clin. Microbiol. Infect. 18, 268–281. doi: 10.1111/j.1469-0691.2011.03570.x
Marques, C., Gama, L. T., Belas, A., Bergstrom, K., Beurlet, S., Briend-Marchal, A., et al. (2016). European multicenter study on antimicrobial resistance in bacteria isolated from companion animal urinary tract infections. BMC Vet. Res. 12:213. doi: 10.1186/s12917-016-0840-3
McCarthy, A. J., Harrison, E. M., Stanczak-Mrozek, K., Leggett, B., Waller, A., Holmes, M. A., et al. (2015). Genomic insights into the rapid emergence and evolution of MDR in Staphylococcus pseudintermedius. J. Antimicrob. Chemother. 70, 997–1007. doi: 10.1093/jac/dku496
McDaniels, A. E., Rice, E. W., Reyes, A. L., Johnson, C. H., Haugland, R. A., and Stelma, G. N. Jr. (1996). Confirmational identification of Escherichia coli, a comparison of genotypic and phenotypic assays for glutamate decarboxylase and beta-D-glucuronidase. Appl. Environ. Microbiol. 62, 3350–3354.
Morrissey, I., Moyaert, H., de Jong, A., El Garch, F., Klein, U., Ludwig, C., et al. (2016). Antimicrobial susceptibility monitoring of bacterial pathogens isolated from respiratory tract infections in dogs and cats across Europe: compath results. Vet. Microbiol. 191, 44–51. doi: 10.1016/j.vetmic.2016.05.020
NSPL (2015). National Statistics Postcode Directory. Available online at: https://data.gov.uk/dataset/7ec10db7-c8f4-4a40-8d82-8921935b4865/national-statistics-postcode-lookup-uk. (accessed January 26, 2016).
O’Neill, D. G., Church, D. B., McGreevy, P. D., Thomson, P. C., and Brodbelt, D. C. (2014). Approaches to canine health surveillance. Canine Genet. Epidemiol. 1:2. doi: 10.1186/2052-6687-1-2
O’Neill, J. (2016). Tackling Drug-Resistant Infections Globally: Final Report and Recommendations. Available online at: http://amr-review.org/ (accessed June 1, 2018).
Perez-Perez, F. J., and Hanson, N. D. (2002). Detection of plasmid-mediated AmpC beta-lactamase genes in clinical isolates by using multiplex PCR. J. Clin. Microbiol. 40, 2153–2162.
PHE (2020). English Surveillance Programme for Antimicrobial Utilisation and Resistance (ESPAUR) Report 2017. Available online at: https://www.gov.uk/government/publications/english-surveillance-programme-antimicrobial-utilisation-and-resistance-espaur-report (accessed July 19, 2021).
RCVS (2018). Royal College of Veterinary Surgeons Veterinary Practice Directory. Available online at: https://findavet.rcvs.org.uk/find-a-vet-practice/ (accessed November 7, 2018).
Reynolds, M. E., Phan, H. T. T., George, S., Hubbard, A. T. M., Stoesser, N., Maciuca, I. E., et al. (2019). Occurrence and characterization of Escherichia coli ST410 co-harbouring blaNDM-5, blaCMY-42 and blaTEM-190 in a dog from the UK. J. Antimicrob. Chemother. 74, 1207–1211. doi: 10.1093/jac/dkz017
Robicsek, A., Strahilevitz, J., Jacoby, G. A., Macielag, M., Abbanat, D., Park, C. H., et al. (2006). Fluoroquinolone-modifying enzyme: a new adaptation of a common aminoglycoside acetyltransferase. Nat. Med. 12, 83–88. doi: 10.1038/nm1347
Salgado-Caxito, M., Benavides, J. A., Adell, A. D., Paes, A. C., and Moreno-Switt, A. I. (2021). Global prevalence and molecular characterization of extended-spectrum β-lactamase producing-Escherichia coli in dogs and cats – a scoping review and meta-analysis. One Health 12:100236. doi: 10.1016/j.onehlt.2021.100236
Schmidt, V. M., Pinchbeck, G., McIntyre, K. M., Nuttall, T., McEwan, N., Dawson, S., et al. (2018). Routine antibiotic therapy in dogs increases the detection of antimicrobial-resistant faecal Escherichia coli. J. Antimicrob. Chemother. 73, 3305–3316. doi: 10.1093/jac/dky352
Scott, A., Pottenger, S., Timofte, D., Moore, M., Wright, L., Kukavica-Ibrulj, I., et al. (2019). Reservoirs of resistance: polymyxin resistance in veterinary-associated companion animal isolates of Pseudomonas aeruginosa. Vet. Rec. 185:206. doi: 10.1136/vr.105075
Seemann, T. (2014). Prokka: rapid prokaryotic genome annotation. Bioinformatics 30, 2068–2069. doi: 10.1093/bioinformatics/btu153
Seemann, T. (2019). MLST 2.19.0. Available online at: https://github.com/tseemann/mlst (accessed July 16, 2020).
Seemann, T. (2020a). Abricate 0.8. Available online at: https://github.com/tseemann/abricate (accessed July 16, 2020).
Seemann, T. (2020b). Snippy: Fast Bacterial Variant Calling From NGS Reads. Available online at: https://github.com/tseemann/snippy (accessed September 27, 2020).
Singleton, D. A., Pinchbeck, G. L., Radford, A. D., Arsevska, E., Dawson, S., Jones, P. H., et al. (2020). A large multi-centre study utilising electronic health records to identify antimicrobial prescription risk factors for dogs and cats. Emerg. Infect. Dis. 26, 1778–1791.
Singleton, D. A., Sánchez-Vizcaíno, F., Dawson, S., Jones, P. H., Noble, P. J. M. M., Pinchbeck, G. L., et al. (2017). Patterns of antimicrobial agent prescription in a sentinel population of canine and feline veterinary practices in the United Kingdom. Vet. J. 224, 18–24. doi: 10.1016/j.tvjl.2017.03.010
Sun, J., Yang, R. S., Zhang, Q., Feng, Y., Fang, L. X., Xia, J., et al. (2016). Co-transfer of blaNDM-5 and mcr-1 by an IncX3-X4 hybrid plasmid in Escherichia coli. Nat. Microbiol. 1:16176. doi: 10.1038/nmicrobiol.2016.176
Tacconelli, E., Carrara, E., Savoldi, A., Harbarth, S., Mendelson, M., Monnet, D. L., et al. (2018). Discovery, research, and development of new antibiotics: the WHO priority list of antibiotic-resistant bacteria and tuberculosis. Lancet Infect. Dis. 18, 318–327. doi: 10.1016/S1473-3099(17)30753-3
Tamma, P. D., and Mathers, A. J. (2021). Navigating treatment approaches for presumed ESBL-producing infections. JAC Antimicrob. Resist. 3:dlaa111. doi: 10.1093/jacamr/dlaa111
Timofte, D., Maciuca, I. E., Williams, N. J., Wattret, A., and Schmidt, V. (2016). Veterinary hospital dissemination of CTX-M-15 extended-spectrum beta-lactamase-producing Escherichia coli ST410 in the United Kingdom. Microb. Drug Resist. 22, 609–615. doi: 10.1089/mdr.2016.0036
Tuerena, I., Williams, N. J., Nuttall, T., and Pinchbeck, G. (2016). Antimicrobial-resistant Escherichia coli in hospitalised companion animals and their hospital environment. J. Small Anim. Pract. 57, 339–347. doi: 10.1111/jsap.12525
Tyson, G. H., Li, C., Hsu, C.-H., Ayers, S., Borenstein, S., Mukherjee, S., et al. (2020). The mcr-9 Gene of Salmonella and E. coli is not associated with colistin resistance in the United States. Antimicrob. Agents Chemother. 64:e00573-20. doi: 10.1128/AAC.00573-20
Valat, C., Drapeau, A., Beurlet, S., Bachy, V., Boulouis, H.-J., Pin, R., et al. (2020). Pathogenic Escherichia coli in dogs reveals the predominance of ST372 and the human-associated ST73 extra-intestinal lineages. Front. Microbiol. 11:580. doi: 10.3389/fmicb.2020.00580
VMD (2019). Veterinary Antimicrobial Resistance and Sales Surveillance 2018. Available online at: https://www.gov.uk/government/publications/veterinary-antimicrobial-resistance-and-sales-surveillance-2018 (accessed July 6, 2020).
Vonholdt, B. M., Pollinger, J. P., Lohmueller, K. E., Han, E., Parker, H. G., Quignon, P., et al. (2010). Genome-wide SNP and haplotype analyses reveal a rich history underlying dog domestication. Nature 464, 898–902. doi: 10.1038/nature08837
Wedley, A. L., Dawson, S., Maddox, T. W., Coyne, K. P., Pinchbeck, G. L., Clegg, P., et al. (2017). Carriage of antimicrobial resistant Escherichia coli in dogs: prevalence, associated risk factors and molecular characteristics. Vet. Microbiol. 199, 23–30. doi: 10.1016/j.vetmic.2016.11.017
Wedley, A. L., Maddox, T. W., Westgarth, C., Coyne, K. P., Pinchbeck, G. L., Williams, N. J., et al. (2011). Prevalence of antimicrobial-resistant Escherichia coli in dogs in a cross-sectional, community-based study. Vet. Rec. 168:354. doi: 10.1136/vr.d1540
Wickham (2016). rvest R package. Available online at: https://cran.r-project.org/web/packages/rvest/rvest.pdf (accessed April 16, 2020).
Keywords: antimicrobial resistance, companion animal, surveillance, digital health, Escherichia coli, one health
Citation: Singleton DA, Pongchaikul P, Smith S, Bengtsson RJ, Baker K, Timofte D, Steen S, Jones M, Roberts L, Sánchez-Vizcaíno F, Dawson S, Noble P-JM, Radford AD, Pinchbeck GL and Williams NJ (2021) Temporal, Spatial, and Genomic Analyses of Enterobacteriaceae Clinical Antimicrobial Resistance in Companion Animals Reveals Phenotypes and Genotypes of One Health Concern. Front. Microbiol. 12:700698. doi: 10.3389/fmicb.2021.700698
Received: 26 April 2021; Accepted: 08 July 2021;
Published: 30 July 2021.
Edited by:
Benjamin Andrew Evans, University of East Anglia, United KingdomReviewed by:
Min Yue, Zhejiang University, ChinaCaray Anne Walker, Anglia Ruskin University, United Kingdom
Copyright © 2021 Singleton, Pongchaikul, Smith, Bengtsson, Baker, Timofte, Steen, Jones, Roberts, Sánchez-Vizcaíno, Dawson, Noble, Radford, Pinchbeck and Williams. This is an open-access article distributed under the terms of the Creative Commons Attribution License (CC BY). The use, distribution or reproduction in other forums is permitted, provided the original author(s) and the copyright owner(s) are credited and that the original publication in this journal is cited, in accordance with accepted academic practice. No use, distribution or reproduction is permitted which does not comply with these terms.
*Correspondence: David A. Singleton, D.A.Singleton@liverpool.ac.uk