- 1Chinese PLA Center for Disease Control and Prevention, Beijing, China
- 2Department of Clinical Laboratory, People’s Liberation Army General Hospital Jingdong Medical District, Beijing, China
- 3Academy of Military Medical Sciences, Academy of Military Sciences, Beijing, China
NDM-1-producing multidrug-resistant Proteus mirabilis brings formidable clinical challenges. We report a nosocomial outbreak of carbapenem-resistant P. mirabilis in China. Six P. mirabilis strains collected in the same ward showed close phylogenetic relatedness, indicating clonal expansion. Illumina and MinION sequencing revealed that three isolates harbored a novel Salmonella genomic island 1 carrying a blaNDM–1 gene (SGI1-1NDM), while three other isolates showed elevated carbapenem resistance and carried a similar SGI1 but with two blaNDM–1 gene copies (SGI1-2NDM). Four new single nucleotide mutations were present in the genomes of the two-blaNDM–1-harboring isolates, indicating later emergence of the SGI1-2NDM structure. Passage experiments indicated that both SGI variants were stably persistent in this clone without blaNDM–1 copy number changes. This study characterizes two novel blaNDM–1-harboring SGI1 variants in P. mirabilis and provides a new insight into resistance gene copy number variation in bacteria.
Introduction
Proteus mirabilis has been recognized as one of the most common pathogens associated with nosocomial infections (Armbruster et al., 2017), particularly of the urinary and respiratory tracts, traumatic wounds, and surgical sites. Salmonella genomic island 1 (SGI1), a site-specific integrative mobilizable element initially found in Salmonella enterica serovar Typhimurium DT104 (Boyd et al., 2000), has emerged recently in P. mirabilis strains from diverse sources in China (Lei et al., 2014, 2015; Qin et al., 2015), France (Schultz et al., 2015; de Curraize et al., 2020), Egypt (Soliman et al., 2017, 2018) and South Korea (Sung et al., 2017). Salmonella genomic island 1 and its variants often carry numerous antimicrobial resistance genes and facilitate the emergence of multidrug resistant (MDR) P. mirabilis, thus posing a significant threat to public health.
New Delhi metallo-β-lactamase 1 (NDM-1), a mediator of carbapenem resistance, has become a serious challenge for clinical management since its first identification in 2009 (Yong et al., 2009). The blaNDM–1 gene is often located on plasmids and is rarely detected in an integrative element. To the best of our knowledge, there has been only one previous description of the integration of blaNDM–1 into an SGI1 relative structure in a P. mirabilis strain, which exhibited an unusual imipenem-resistant but meropenem-susceptible phenotype (Girlich et al., 2015). The clinical impact of P. mirabilis carrying blaNDM–1-positive SGI1 still requires further investigation.
We describe a nosocomial outbreak caused by a carbapenem-resistant P. mirabilis clone in China. Two novel SGI1 variants carrying one and two blaNDM–1 gene copies, respectively, were detected in six P. mirabilis strains through MiSeq and MinION sequencing. Phylogenetic analysis and passage experiments were further performed to gain an insight into the evolutionary relationship of both SGIs.
Materials and Methods
Bacterial Isolation and Antimicrobial Susceptibility Testing
From April to August in 2017, six P. mirabilis isolates were recovered from sputum or urine samples of hospitalized patients in the neurology department of the 263rd Hospital in Beijing, China. All isolates were collected through routine surveillance. Species-level identification and antimicrobial susceptibility were performed by a Vitek 2 compact system (bioMérieux, France). Results were interpreted according to the Clinical and Laboratory Standards Institute guidelines (Clinical and Laboratory Standards Institute, 2017). Escherichia coli ATCC25922 was used for quality control. Minimum inhibitory concentration (MIC) values of imipenem, meropenem and ertapenem were further determined by MIC test strips (Liofilchem, Italy). The entire blaNDM–1 gene was amplified with previously described primers and further confirmed by sequencing (Zou et al., 2015). The study was supervised by Ethics Committee of the Chinese PLA Center for Disease Control and Prevention. Informed consent was not required as no identifiable patient data was presented in this study.
Transcriptional Expression Analysis of blaNDM–1 and Passage Experiments
The blaNDM–1 copy number in six strains were identified by Illumina MiSeq and Nanopore MinION sequencing (see below). Transcriptional expression of blaNDM–1 in PmBJ020-1 and PmBJ023-2 was determined by quantitative RT-PCR (qRT-PCR). Total RNA was isolated from bacterial cultures using RNApure Bacteria Kit (DNase I) (CWBio, China). RT-PCR was performed using UltraSYBR One Step RT-qPCR Kit (CWbio, China) in CFX96 Real-Time System (Bio-Rad, United States) as described previously (Wasfi et al., 2020). Expression levels were normalized relative to the transcriptional level of the constitutive 16S rRNA and interpreted by the ΔΔCt method (Johnson et al., 2013).
A passage experiment was performed on PmBJ020-1 and PmBJ023-2 with imipenem-containing (32 mg/L, Solarbio, China) and antibiotic-free broth (ThermoFisher, United States), respectively, for 12 h at 37°C. Cultures were adjusted to 0.5 McFarland with sterile PBS, 5 μl of which was added to 5ml of fresh broth. The OD600 of the overnight culture was measured using a Spectra Max M5 microplate reader (Molecular Devices, United States). This procedure was repeated for 15 consecutive days. Quantitative PCR (qPCR) was performed to quantify blaNDM–1 at the beginning and end of the passage experiment by QuantStudio3 (ThermoFisher, United States) as described previously (Wasfi et al., 2020). qPCR results were interpreted by the ΔΔCt method (Johnson et al., 2013). Quantitative RT-PCR was also performed to determine the transcriptional expression of blaNDM–1 after 30 passages. Statistical analysis was performed using the Student’s t-test to compare blaNDM–1 gene copy numbers and expression levels.
Illumina/MinION Sequencing and Annotation
Total DNA was extracted from cultured bacteria using the High Pure PCR Template Preparation Kit (Roche). Whole genome sequencing (WGS) was conducted using an Illumina MiSeq platform with a 350-bp insert size. Genomic DNA was also sequenced using an Oxford Nanopore MinION sequencer with the RAD004 rapid sequencing kit. Sequencing was performed for 11 h on average for each sample. The de novo hybrid assembly of short Illumina reads and long MinION reads was performed using Unicycler (v0.4.0) with default parameters (Wick et al., 2017). Genome sequences were annotated using RAST (Aziz et al., 2008). Antimicrobial resistance genes were identified through alignment against the Comprehensive Antibiotic Resistance Database (Jia et al., 2017).
Phylogenetic Analysis
Genome sequences of 75 available P. mirabilis isolates were downloaded from the NCBI database for phylogenetic analysis. P. mirabilis strain HI4320 (GenBank accession number AM942759.1) was used as the reference for alignment. Reads were mapped onto the reference genome using BWA (v0.7.12) (Li and Durbin, 2009). SNPs were identified using SAMtools (v1.3) (Li et al., 2009). A Maximum-Likelihood (ML) phylogenetic tree was constructed using RAxML (v8.2.4) with a general time reversible (GTR) model and a gamma distribution based on 1000 bootstraps (Stamatakis, 2014). Average nucleotide identities between genomes were calculated using JSpeciesWS to evaluate genome similarity (Richter et al., 2016).
Nucleotide Sequence Accession Number
The genome sequences of P. mirabilis PmBJ004-1, PmBJ012-2, PmBJ015-2, PmBJ020-1, PmBJ023-2 and PmBJ024-1 have been deposited in GenBank under accession numbers JADOZD000000000, CP065148, CP065147, CP065146, CP065145 and CP065144, respectively.
Results
Clonal Expansion of Proteus mirabilis in the Same Ward
In 2017, six P. mirabilis isolates (designated PmBJ004-1, PmBJ012-2, PmBJ015-2, PmBJ020-1, PmBJ023-2 and PmBJ024-1, respectively) were recovered. The first isolation was from the sputum of a patient in the neurology department diagnosed with cerebral infarction and coronary artery disease. During the following four months, five new cases were identified in the same ward. Four isolates were recovered from the sputum cultures and 1 from the urine culture. Five of the six patients had stayed in three adjacent beds, one of which moved to a separate bed afterward (Figure 1). Patient 24 had lain on a temporarily placed bed.
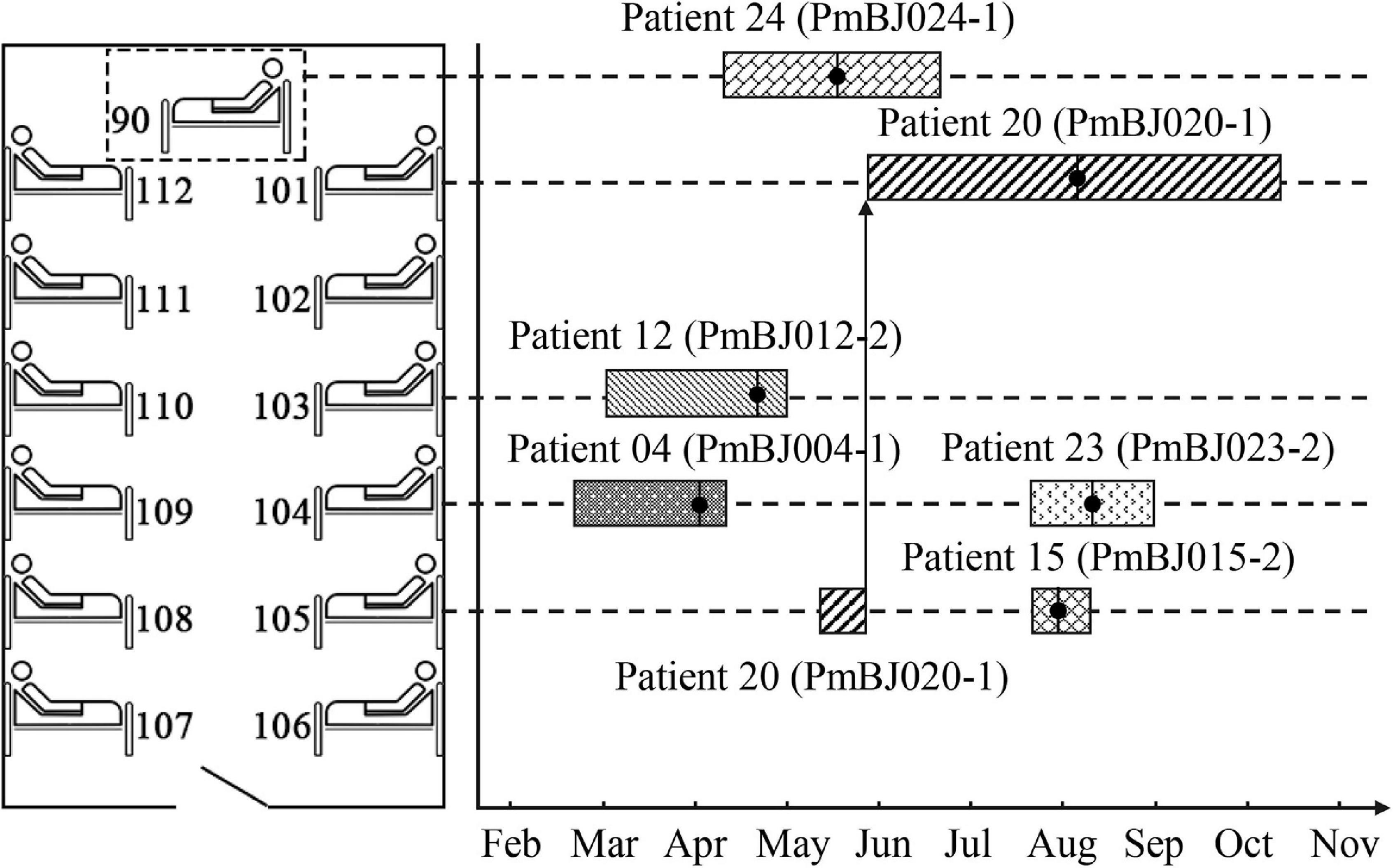
Figure 1. Spatial and temporal distribution of patients during hospitalization. Bed 90 was temporarily placed in the neurology ward; the dotted box does not reflect its exact position. The boxes represent the stay of the patients. The vertical bars with black dots indicate the isolation date of P. mirabilis.
The phylogenetic tree was constructed based on genomic sequences of the six P. mirabilis isolates by short-read and long-read WGS and 75 available P. mirabilis complete genomes in NCBI (Figure 2). Topology revealed that the six P. mirabilis isolates were located within one clade. They exhibited very high average nucleotide identities ranging from 99.93 to 99.99%, indicating a clonal expansion. The six P. mirabilis isolates were distinct from other Chinese strains in the phylogeny but showed closer relationship with overseas strains 360_PMIR and 25_PMIR from the United States, indicating a different evolutionary origin.
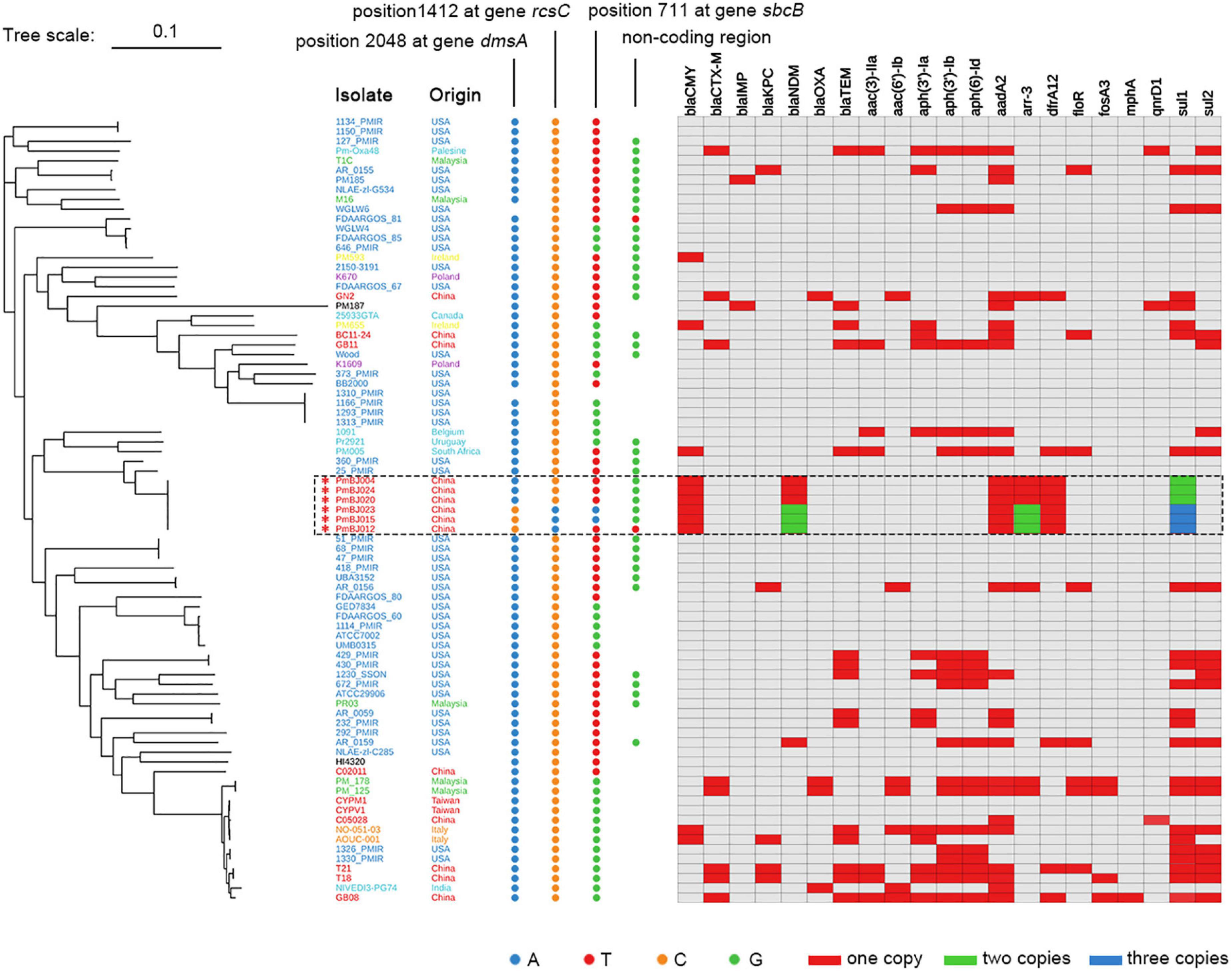
Figure 2. ML Phylogenetic tree of P. mirabilis isolates with key SNPs and resistance gene profiles. The six P. mirabilis strains are marked with an asterisk. Nucleotides at four loci that distinguish the six isolates are indicated by dots in different colors. The colored blocks indicate resistance gene copy numbers.
Further analysis revealed that isolates PmBJ012-2, PmBJ015-2, and PmBJ023-2 were distinguished from PmBJ004-1, PmBJ020-1, and PmBJ024-1 by four single nucleotide mutations (Figure 2). One was located within the dmsA gene associated with dimethyl sulfoxide reduction and resulted in the amino acid substitution of Glu683Gly, and the other was a synonymous mutation at position 1412 (C to T) of the capsular synthesis gene rcsC. PmBJ015-2 and PmBJ023-2 had a synonymous mutation at position 711 (A to T) of exodeoxyribonuclease I gene sbcB, and PmBJ012-2 had a unique nucleotide substitution (G to A) in a non-coding region.
Genetic Features of Two Novel Salmonella Genomic Island 1 Variants Carrying One and Two blaNDM–1 Gene Copies
Sequence analysis revealed that the six P. mirabilis isolates have an SGI1 integrated between the thdF and hipB chromosomal genes. 18-bp direct repeats (DRs) of specific recombination sites attB (DR-R) and attP (DR-L) were identified. Further alignment against existing SGI1s indicated that PmBJ004-1, PmBJ020-1, and PmBJ024-1 harbored a novel SGI1 structure designated SGI1-1NDM; while PmBJ012-2, PmBJ015-2, and PmBJ023-2 carried a different SGI1 variant named SGI1-2NDM (Figure 3A). SGI1-1NDM had a length of 40,293bp, while SGI1-2NDM contained 46,693bp. The two SGI1s shared a backbone of 27.4kb that was highly identical to that of SGI1 from S. Typhimurium DT104 (Boyd et al., 2001). The MDR regions of SGI1-1NDM and SGI1-2NDM were integrated between the backbone genes res (S027) and S044, at the same position as in the Salmonella SGI1.
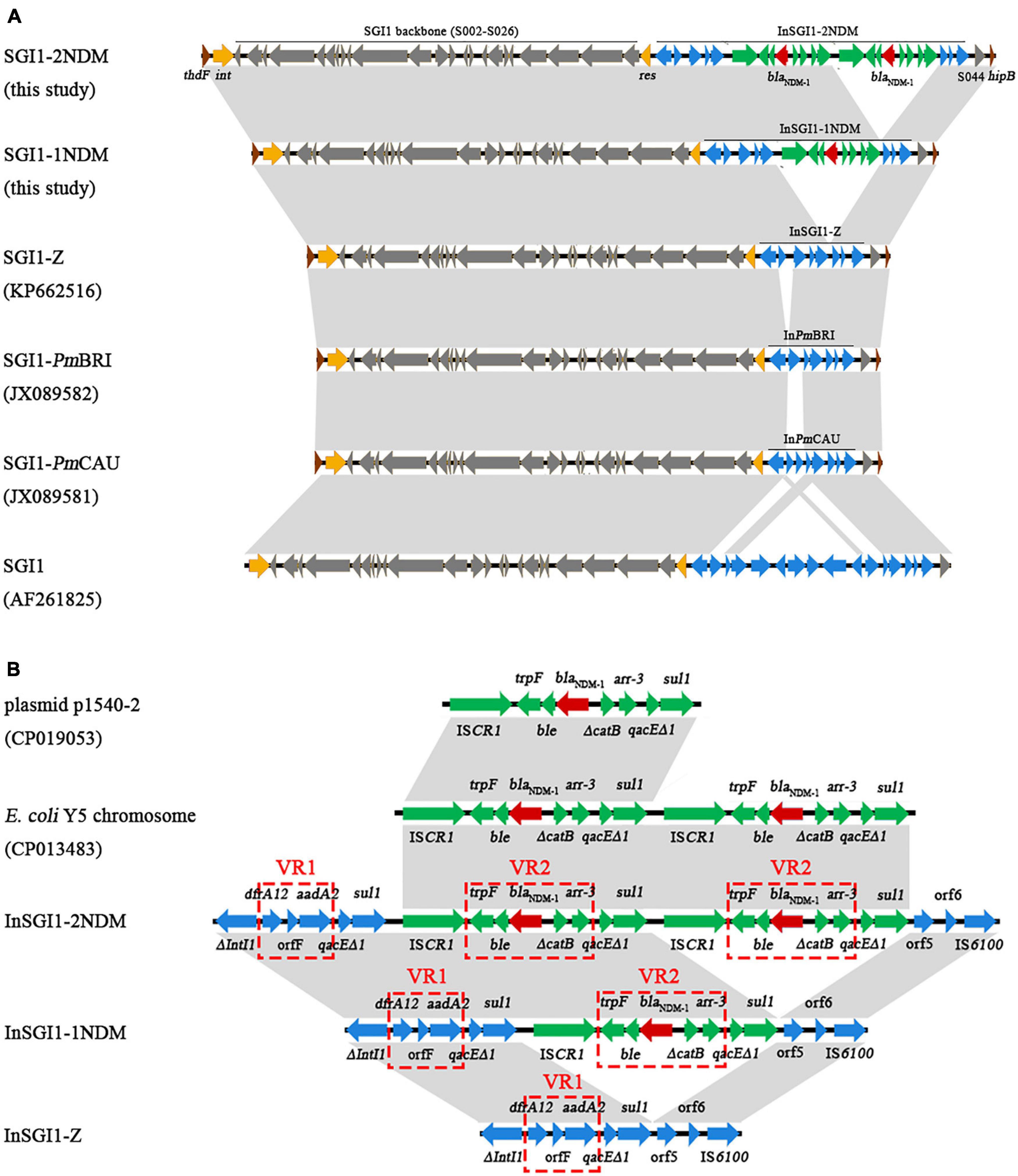
Figure 3. Comparative schematic diagram of (A) SGI1s and (B) the MDR regions of SGI1s. Arrows represent the positions and directions of gene transcription. The red, green and blue arrows indicate genes in the MDR regions. The gray-shaded areas represent regions sharing >99% identity.
The MDR region (InSGI1-1NDM) of SGI1-1NDM harbored a complex class 1 integron of the In4 type (Figure 3B). 5-bp DRs, ACTTG, created upon transposition were detected. This integron was organized as 5′CS (intI1), variable region 1 (VR1), 3′CS-1 (qacEΔ1/sul1), ISCR1, VR2 and 3′CS-2 (qacEΔ1/sul1). The VR1 carried a dfrA12-orfF-aadA2 cassette array conferring resistance to trimethoprim, spectinomycin and streptomycin, which has been found in the class 1 integron of a genomic island SGI1-Z (KP662516.1) in P. mirabilis PM58 (Qin et al., 2015). The VR2 comprised a typical blaNDM–1 unit (trpF-ble-blaNDM–1), a truncated catB, and an arr-3 gene. This VR2 structure flanked by ISCR1 and 3′CS has also been found in several enterobacterial plasmids including E. coli plasmids p1540-2 (CP019053.1), p6061604-KPC (MN823987.1), and pHNSD133T1 (MG196293.1) (Lv et al., 2018), Citrobacter freundii plasmid pCf75 (CP047308.1) (Li et al., 2020), and Klebsiella pneumoniae plasmid pNDM_3214 (CP028851.1) (Liu and Su, 2019).
The MDR region (InSGI1-2NDM) of SGI1-2NDM also contained an In4-type complex class 1 integron. Compared with InSGI1-1NDM, the InSGI1-2NDM has acquired a second blaNDM–1-carrying VR2 together with adjacent ISCR1 and 3′CS. Thus, the InSGI1-2NDM could be divided into 5′CS, VR1, 3′CS-1, ISCR1, VR2, 3′CS-2, ISCR1, VR2, and 3′CS-3. The two VR2s presented 100% nucleotide identity, and both ISCR1 elements had oriIS sites detected downstream. This structure with two tandem blaNDM–1 copies was similar to that in the E. coli Y5 chromosome, whereas the latter had a different VR1 carrying aac(6′)lb-cr, arr-3, and aadA16.
Higher Carbapenem Resistance and blaNDM–1 Expression Level of SGI1-2NDM Relative to SGI1-1NDM
All six P. mirabilis isolates were resistant to ampicillin, ciprofloxacin, nitrofurantoin, levofloxacin and most cephalosporins but susceptible to amikacin, aztreonam, gentamicin, and tobramycin (Table 1). MIC test strips showed that all the isolates were resistant to imipenem, but the imipenem MICs of PmBJ012-2, PmBJ015-2 and PmBJ023-2 carrying SGI1-2NDM (96 μg/ml, 96 μg/ml and 64 μg/ml, respectively) were remarkably higher than those of PmBJ004-1, PmBJ020-1 and PmBJ024-1 carrying SGI1-1NDM (16 μg/ml, 16 μg/ml and 12 μg/ml, respectively). MIC test strips also revealed that PmBJ012-2, PmBJ015-2 and PmBJ023-2 were resistant to meropenem and ertapenem, while PmBJ004-1, PmBJ020-1 and PmBJ024-1 were intermediate to meropenem and susceptible to ertapenem. qRT-PCR revealed that the expression level of blaNDM–1 in PmBJ023-2 was 2.63-fold higher than that in PmBJ020-1 (Figure 4A).
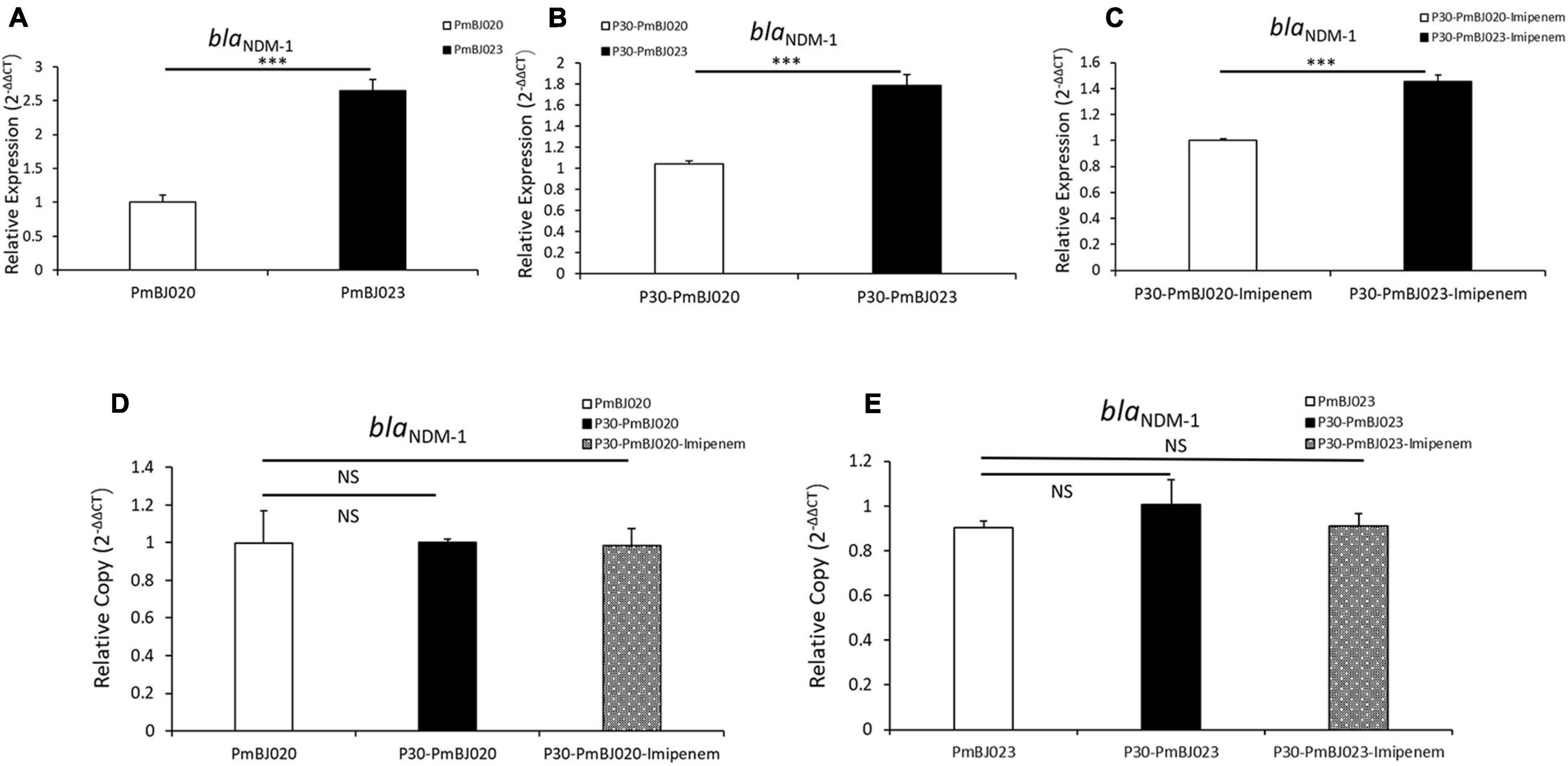
Figure 4. Expression level and copy number of the blaNDM–1 gene in PmBJ020 and PmBJ023 during passage experiment. Relative expression of blaNDM–1 in PmBJ020 and PmBJ023 (A), P30-PmBJ020 and P30-PmBJ023 (B), P30-PmBJ020-Imipenem and P30-PmBJ023-Imipenem (C). Copy number of blaNDM–1 in PmBJ020, P30-PmBJ020 and P30-PmBJ020-Imipenem (D), PmBJ023, P30-PmBJ023 and P30-PmBJ023-Imipenem (E). P30-PmBJ020, PmBJ020 after 30 passages without antibiotic selection; P30-PmBJ023, PmBJ023 after 30 passages without antibiotic selection; P30-PmBJ020-Imipenem, PmBJ020 after 30 passages with imipenem selection; P30-PmBJ023-Imipenem, PmBJ023 after 30 passages with imipenem selection. Significance of difference was determined using the Student’s t-test (*** P < 0.001; NS, not significant).
Stable Persistence of Novel Salmonella Genomic Island 1 Structures
We assessed the stability of the two SGI1s by passage experiments with and without antibiotic selection. qPCR revealed that the blaNDM–1 copy number remained unchanged in both SGI1s during passage experiments (Figures 4D,E). Quantitative RT-PCR indicated that the expression level of blaNDM–1 in PmBJ023-2 was still 1.46- and 1.72-fold higher than that PmBJ020-1 with and without imipenem selection, respectively, after 30 passages (Figures 4B,C). These results indicated that the two novel SGI1s had strong structural and functional stability and persisted in P. mirabilis.
Discussion
This study documents a nosocomial outbreak caused by six carbapenem-resistant P. mirabilis strains in a Chinese hospital. To the best of our knowledge, this is also the first report of a hospital outbreak of an NDM-1-producing P. mirabilis clone. Since environmental samples were not collected in this ward, the transmission route of the P. mirabilis clone was unidentifiable. Additional cases were identified during the following four months in the same ward, suggesting long term clonal colonization. Infections caused by such MDR pathogens may result in increased morbidity and mortality due to the inefficacy of commonly used antibiotics. To prevent further spread of such pathogens, sterilization of multi-use instruments and caring devices should be vigorously implemented. Contact precautions should be taken with improved hand-hygiene compliance and glove use.
The presence of similar components indicated a close relation of SGI1-1NDM and SGI1-2NDM to SGI1-Z. However, the DR-R diverged in 1bp from the DR-L in SGI1-Z, which was not found in two SGI1 variants in this study. It is likely that SGI1-1NDM or SGI1-2NDM were not derived from SGI1-Z, but from another similar structure. ISCRI1 serves as a common vehicle for the mobilization of antibiotic resistance genes by rolling circle transposition (Janvier et al., 2013; Chen et al., 2014; Li et al., 2014). A previous study demonstrated that the blaNDM–1 gene could be transferred via a circular ISCR1-blaNDM–1 element released from plasmids (Li et al., 2014). Since the oriIS site was detected, we speculate that the ISCR1 element might mediate the acquisition of the blaNDM–1-embedded VR2 to an SGI1-Z-like structure, presumably through recombination at the sul1 locus of the class 1 integron, to form the novel variant SGI1-1NDM.
Although two tandem copies of the blaNDM–1 gene have been found in several bacterial isolates (Jovcić et al., 2013; Shen et al., 2017; Feng et al., 2018), dynamic blaNDM–1 copy number variations during clonal expansion have been detected very rarely under natural conditions. In this study, PmBJ012-2, PmBJ015-2, and PmBJ023-2 with two blaNDM–1 copies were not distinguished from PmBJ004-1, PmBJ020-1, and PmBJ024-1 based on clinical information such as isolation date or source. We also did not find conversion between SGI1-1NDM and SGI1-2NDM during passage experiments. However, four additional nucleotide mutations at the genome-wide level were found in PmBJ012-2, PmBJ015-2, and PmBJ023-2. This observation strongly suggests that the isolates with two blaNDM–1 copies might have evolved later than those with single copy. It is likely that SGI1-1NDM with a single blaNDM–1 copy was the ancestral structure and preceded a second similar transposition event that resulted in the formation of SGI1-2NDM with two copies. The findings of this study also provided evidence that two tandem blaNDM–1 copies were not transferred as a block, but formed due to a multiplication mechanism. Although the strains harboring two blaNDM–1 gene copies showed elevated carbapenem resistance, it was still not clear whether antimicrobial pressure played a crucial role in the emergence of SGI1-2NDM. The strong structural stability of both SGI1s even under antibiotic-free conditions suggest their high potential for long-term persistence, which warrants further studies.
Conclusion
This study describes a nosocomial outbreak caused by carbapenem-resistant P. mirabilis in China. Six P. mirabilis strains collected in the same ward were closely related, indicating clonal expansion. Illumina and MinION sequencing revealed the presence of a novel SGI1-1NDM carrying one blaNDM–1 gene copy and an SGI1-2NDM carrying two blaNDM–1 gene copies. Four new single nucleotide mutations in the genomes of the SGI1-2NDM-harboring isolates indicate the possible later emergence of SGI1-2NDM. SGI1-2NDM confers higher carbapenem resistance and blaNDM–1 expression level relative to SGI1-1NDM. Both SGIs were stably persistent with and without antibiotic selection. Vigorous surveillance and urgent actions should be taken immediately to control the continuous expansion of such pathogens.
Data Availability Statement
The datasets presented in this study can be found in online repositories. The names of the repository/repositories and accession number(s) can be found below: https://www.ncbi.nlm.nih.gov/genbank/, JADOZD000000000; https://www.ncbi.nlm.nih.gov/genbank/, CP065148; https://www.ncbi.nlm.nih.gov/genbank/, CP065147; https://www.ncbi.nlm.nih.gov/genbank/, CP065146; https://www.ncbi.nlm.nih.gov/genbank/, CP065145; and https://www.ncbi.nlm.nih.gov/genbank/, CP065144.
Author Contributions
PnL and LY performed data analysis and prepared the manuscript. HH and QC collected samples and performed susceptibility testing. JL, YL, and KW performed DNA extraction and genome sequencing. PiL, XL, and LJ performed genome assembly and annotation. PnL and HS designed the study and revised the manuscript. All authors contributed to review and revision, and approved the final version.
Funding
The study was supported by grants from National Major Science and Technology Project (no. 2018ZX10201001), National Key Research and Development Project (no. 2018YFC1200100), Beijing Nova Program (no. Z181100006218110), and National Natural Science Foundation of China (no. 31900151).
Conflict of Interest
The authors declare that the research was conducted in the absence of any commercial or financial relationships that could be construed as a potential conflict of interest.
Publisher’s Note
All claims expressed in this article are solely those of the authors and do not necessarily represent those of their affiliated organizations, or those of the publisher, the editors and the reviewers. Any product that may be evaluated in this article, or claim that may be made by its manufacturer, is not guaranteed or endorsed by the publisher.
References
Armbruster, C. E., Smith, S. N., Johnson, A. O., DeOrnellas, V., Eaton, K. A., Yep, A., et al. (2017). The Pathogenic Potential of Proteus mirabilis Is Enhanced by Other Uropathogens during Polymicrobial Urinary Tract Infection. Infect. Immun. 85, e808–e816. doi: 10.1128/IAI.00808-16
Aziz, R. K., Bartels, D., Best, A. A., DeJongh, M., Disz, T., Edwards, R. A., et al. (2008). The RAST Server: rapid annotations using subsystems technology. BMC Genomics 9:75. doi: 10.1186/1471-2164-9-75
Boyd, D., Peters, G. A., Cloeckaert, A., Boumedine, K. S., Chaslus-Dancla, E., Imberechts, H., et al. (2001). Complete nucleotide sequence of a 43-kilobase genomic island associated with the multidrug resistance region of Salmonella enterica serovar Typhimurium DT104 and its identification in phage type DT120 and serovar Agona. J. Bacteriol. 183, 5725–5732. doi: 10.1128/JB.183.19.5725-5732.2001
Boyd, D. A., Peters, G. A., Ng, L., and Mulvey, M. R. (2000). Partial characterization of a genomic island associated with the multidrug resistance region of Salmonella enterica Typhymurium DT104. FEMS Microbiol. Lett. 189, 285–291. doi: 10.1111/j.1574-6968.2000.tb09245.x
Chen, C.-J., Wu, T.-L., Lu, P.-L., Chen, Y.-T., Fung, C.-P., Chuang, Y.-C., et al. (2014). Closely related NDM-1-encoding plasmids from Escherichia coli and Klebsiella pneumoniae in Taiwan. PLoS One 9:e104899. doi: 10.1371/journal.pone.0104899
Clinical and Laboratory Standards Institute (2017). Performance Standards for Antimicrobial Susceptibility Testing: Twenty-seventh Informational Supplement M100-S27. Wayne, PA: CLSI.
de Curraize, C., Siebor, E., Varin, V., Neuwirth, C., and Hall, R. M. (2020). Two New SGI1-LK Variants Found in Proteus mirabilis and Evolution of the SGI1-HKL Group of Salmonella Genomic Islands. mSphere 5:19. doi: 10.1128/mSphere.00875-19
Feng, Y., Liu, L., McNally, A., and Zong, Z. (2018). Coexistence of Two blaNDM–5 Genes on an IncF Plasmid as Revealed by Nanopore Sequencing. Antimicrob. Agents Chemother. 62:18. doi: 10.1128/AAC.00110-18
Girlich, D., Dortet, L., Poirel, L., and Nordmann, P. (2015). Integration of the blaNDM–1 carbapenemase gene into Proteus genomic island 1 (PGI1-PmPEL) in a Proteus mirabilis clinical isolate. J. Antimicrob. Chemother. 70, 98–102. doi: 10.1093/jac/dku371
Janvier, F., Jeannot, K., Tessé, S., Robert-Nicoud, M., Delacour, H., Rapp, C., et al. (2013). Molecular characterization of blaNDM–1 in a sequence type 235 Pseudomonas aeruginosa isolate from France. Antimicrob. Agents Chemother. 57, 3408–3411. doi: 10.1128/AAC.02334-12
Jia, B., Raphenya, A. R., Alcock, B., Waglechner, N., Guo, P., Tsang, K. K., et al. (2017). CARD 2017: expansion and model-centric curation of the comprehensive antibiotic resistance database. Nucleic Acids Res. 45, D566–D573. doi: 10.1093/nar/gkw1004
Johnson, G., Nolan, T., and Bustin, S. A. (2013). Real-time quantitative PCR, pathogen detection and MIQE. Methods Mol. Biol. 943, 1–16. doi: 10.1007/978-1-60327-353-4_1
Jovcić, B., Lepsanović, Z., Begović, J., Rakonjac, B., Perovanović, J., Topisirović, L., et al. (2013). The clinical isolate Pseudomonas aeruginosa MMA83 carries two copies of the blaNDM–1 gene in a novel genetic context. Antimicrob. Agents Chemother. 57, 3405–3407. doi: 10.1128/AAC.02312-12
Lei, C.-W., Zhang, A.-Y., Liu, B.-H., Wang, H.-N., Guan, Z.-B., Xu, C.-W., et al. (2014). Molecular characteristics of Salmonella genomic island 1 in Proteus mirabilis isolates from poultry farms in China. Antimicrob. Agents Chemother. 58, 7570–7572. doi: 10.1128/AAC.03992-14
Lei, C.-W., Zhang, A.-Y., Liu, B.-H., Wang, H.-N., Yang, L.-Q., Guan, Z.-B., et al. (2015). Two novel Salmonella genomic island 1 variants in Proteus mirabilis isolates from swine farms in China. Antimicrob. Agents Chemother. 59, 4336–4338. doi: 10.1128/AAC.00120-15
Li, H., and Durbin, R. (2009). Fast and accurate short read alignment with Burrows-Wheeler transform. Bioinformatics 25, 1754–1760. doi: 10.1093/bioinformatics/btp324
Li, H., Handsaker, B., Wysoker, A., Fennell, T., Ruan, J., Homer, N., et al. (2009). The Sequence Alignment/Map format and SAMtools. Bioinformatics 25, 2078–2079. doi: 10.1093/bioinformatics/btp352
Li, J., Lan, R., Xiong, Y., Ye, C., Yuan, M., Liu, X., et al. (2014). Sequential isolation in a patient of Raoultella planticola and Escherichia coli bearing a novel ISCR1 element carrying blaNDM–1. PLoS One 9:e89893. doi: 10.1371/journal.pone.0089893
Li, Z., Lin, Y., Lu, L., Wang, K., Yang, L., Li, P., et al. (2020). Genetic characterisation of a complex class 1 integron in an NDM-1-producing Citrobacter freundii ST396 clinical strain isolated from a urine sample. J. Glob. Antimicrob. Resist. 23, 64–66. doi: 10.1016/j.jgar.2020.08.002
Liu, B.-T., and Su, W.-Q. (2019). Whole genome sequencing of NDM-1-producing serotype K1 ST23 hypervirulent Klebsiella pneumoniae in China. J. Med. Microbiol. 68, 866–873. doi: 10.1099/jmm.0.000996
Lv, L., Zeng, Z., Song, Q., Cao, Y., Wang, J., Li, W., et al. (2018). Emergence of XDR Escherichia coli carrying both blaNDM and mcr-1 genes in chickens at slaughter and the characterization of two novel blaNDM-bearing plasmids. J. Antimicrob. Chemother. 73, 2261–2263. doi: 10.1093/jac/dky176
Qin, S., Qi, H., Zhang, Q., Zhao, D., Liu, Z.-Z., Tian, H., et al. (2015). Emergence of Extensively Drug-Resistant Proteus mirabilis Harboring a Conjugative NDM-1 Plasmid and a Novel Salmonella Genomic Island 1 Variant, SGI1-Z. Antimicrob. Agents Chemother. 59, 6601–6604. doi: 10.1128/AAC.00292-15
Richter, M., Rosselló-Móra, R., Oliver Glöckner, F., and Peplies, J. (2016). JSpeciesWS: a web server for prokaryotic species circumscription based on pairwise genome comparison. Bioinformatics 32, 929–931. doi: 10.1093/bioinformatics/btv681
Schultz, E., Haenni, M., Mereghetti, L., Siebor, E., Neuwirth, C., Madec, J.-Y., et al. (2015). Survey of multidrug resistance integrative mobilizable elements SGI1 and PGI1 in Proteus mirabilis in humans and dogs in France, 2010-13. J. Antimicrob. Chemother. 70, 2543–2546. doi: 10.1093/jac/dkv154
Shen, P., Yi, M., Fu, Y., Ruan, Z., Du, X., Yu, Y., et al. (2017). Detection of an Escherichia coli Sequence Type 167 Strain with Two Tandem Copies of blaNDM–1 in the Chromosome. J. Clin. Microbiol. 55, 199–205. doi: 10.1128/JCM.01581-16
Soliman, A. M., Ahmed, A. M., Shimamoto, T., El-Domany, R. A., Nariya, H., and Shimamoto, T. (2017). First report in Africa of two clinical isolates of Proteus mirabilis carrying Salmonella genomic island (SGI1) variants, SGI1-PmABB and SGI1-W. Infect. Genet. Evol. 51, 132–137. doi: 10.1016/j.meegid.2017.03.029
Soliman, A. M., Saad, A. M., Ahmed, A. M., Al-Baqir, A., Hussein, A., Shimamoto, T., et al. (2018). Occurrence of Salmonella genomic island 1 (SGI1) in two African Proteus mirabilis strains isolated from diseased chicken flocks. Infect. Genet. Evol. 62, 8–10. doi: 10.1016/j.meegid.2018.04.008
Stamatakis, A. (2014). RAxML version 8: a tool for phylogenetic analysis and post-analysis of large phylogenies. Bioinformatics 30, 1312–1313. doi: 10.1093/bioinformatics/btu033
Sung, J. Y., Kim, S., Kwon, G., and Koo, S. H. (2017). Molecular Characterization of Salmonella Genomic Island 1 in Proteus mirabilis Isolates from Chungcheong Province, Korea. J. Microbiol. Biotechnol. 27, 2052–2059. doi: 10.4014/jmb.1708.08040
Wasfi, R., Abdellatif, G. R., Elshishtawy, H. M., and Ashour, H. M. (2020). First-time characterization of viable but non-culturable Proteus mirabilis: Induction and resuscitation. J. Cell. Mol. Med. 24, 2791–2801. doi: 10.1111/jcmm.15031
Wick, R. R., Judd, L. M., Gorrie, C. L., and Holt, K. E. (2017). Unicycler: Resolving bacterial genome assemblies from short and long sequencing reads. PLoS Comput. Biol. 13:e1005595. doi: 10.1371/journal.pcbi.1005595
Yong, D., Toleman, M. A., Giske, C. G., Cho, H. S., Sundman, K., Lee, K., et al. (2009). Characterization of a new metallo-beta-lactamase gene, bla(NDM-1), and a novel erythromycin esterase gene carried on a unique genetic structure in Klebsiella pneumoniae sequence type 14 from India. Antimicrob. Agents Chemother. 53, 5046–5054. doi: 10.1128/AAC.00774-09
Keywords: Proteus mirabilis, Salmonella genomic island 1, clonal expansion, NDM-1, copy number variation
Citation: Yang L, He H, Chen Q, Wang K, Lin Y, Li P, Li J, Liu X, Jia L, Song H and Li P (2022) Nosocomial Outbreak of Carbapenemase-Producing Proteus mirabilis With Two Novel Salmonella Genomic Island 1 Variants Carrying Different blaNDM–1 Gene Copies in China. Front. Microbiol. 12:800938. doi: 10.3389/fmicb.2021.800938
Received: 24 October 2021; Accepted: 10 December 2021;
Published: 13 January 2022.
Edited by:
Octavio Luiz Franco, Catholic University of Brasilia (UCB), BrazilReviewed by:
Hamid Solgi, Isfahan University of Medical Sciences, IranJean-Philippe Lavigne, Centre Hospitalier Universitaire de Nîmes, France
Copyright © 2022 Yang, He, Chen, Wang, Lin, Li, Li, Liu, Jia, Song and Li. This is an open-access article distributed under the terms of the Creative Commons Attribution License (CC BY). The use, distribution or reproduction in other forums is permitted, provided the original author(s) and the copyright owner(s) are credited and that the original publication in this journal is cited, in accordance with accepted academic practice. No use, distribution or reproduction is permitted which does not comply with these terms.
*Correspondence: Hongbin Song, aG9uZ2JpbnNvbmdAMjYzLm5ldA==; Peng Li, amlla2VubGVlQDEyNi5jb20=
†These authors have contributed equally to this work