- Department of Pharmaceutical Science and Health, Montepríncipe Campus, CEU San Pablo University, Madrid, Spain
The abuse of chemical fertilizers in intensive agriculture has turned out in the contamination of ground and the soil on which they are applied. Likewise, the generation, storage, and destruction of plant residues from the agri-food industry poses a threat to the environment and human health. The current situation of growing demand for food implies the urgent need to find sustainable alternatives to chemical fertilizers and the management of agricultural waste. Valorization of this plant residue to produce natural biofertilizers using microbiological treatments is presented as a sustainable alternative. The microbial activity allows the transformation into simple molecules that are easily absorbed by plants, as well as the stimulation of plant growth. This double direct and indirect action induced significant increases against the variables of germination, viability, and biomass (dry weight). To guarantee biosafety, it is necessary to use new bio-technological tools, such as metagenomics, which allow the taxonomic analysis of microbial communities, detecting the absence of pathogens. In the present paper, a physicochemical and metagenomic characterization of a fertilizer obtained from agricultural plant waste valorization is carried out. Likewise, fertigation treatments were tested to which the Plant Growth Promoting Bacteria (PGPB) Pseudomonas agronomica and Bacillus pretiosus were added, both independently and in consortium. Metagenomic analysis has identified taxa belonging to the kingdoms Bacteria and Archaea; 10 phyla, 25 families, 32 genera and 34 species, none of them previously described as pathogenic. A 1/512 dilution of the fertilizer increased the germination rate of Medicago sativa (alfalfa) by 16% at 144 h, compared to the treatment without fertilizer. Both the fertilizer and the addition of PGPB in a double direct and indirect action induced significant increases against the variables of germination, viability, and biomass (dry weight). Therefore, the use of an agricultural residue is proposed, which after the addition of two new species is transformed into a biofertilizer that significantly induces plant growth in Mendicago sativa plants.
Introduction
Eradicating hunger, feeding the future population and environmental conservation are the great challenges facing modern societies today. The application of chemical fertilizers in agriculture has resulted in eutrophication processes, an imbalance in soil physicochemical reactions and increased greenhouse gas emissions (Pirttilä et al., 2021). The latter is normal in the transformation of nutrients by plants. The increase in nutrients resulting from the application of chemical fertilizers leads to exponential increases in these emissions (Mikhailova, 2018). In this regard, the Food and Agriculture Organization of the United Nations (FAO), in its 2030 agenda, reports that intensive and short-sighted farming techniques have damaged ecosystems, causing the degradation of one-third of the planet’s soils. It also concludes that food production needs to increase by 50% to meet future food demand and advocates the use of environmentally, socially, and economically sustainable agricultural techniques (Colglazier, 2015).
Knowledge of techniques aimed at the recovery and new uses of plant residues is rising due to their contribution to the physiological and productive balance of soils. Plant remains serve as important points of microbial activity and constitute an elementary part of the preservation of edaphic carbon (Witzgall et al., 2021). Therefore, the use of plant residues as fertilizers can provide a sustainable solution to alleviate the depletion of soil nutrients caused by overexploitation (Chèneby et al., 2010). The recovery of plant waste destined for landfill relies on reclassifying it as having another useful purpose and making it a product with commercial and environmental benefits (Directive (EU) 2008/98/EC opinion of the Economic Committee; V. DIRECTIVA (UE), 2008).
Despite the known value in agriculture of fertilizers, other factors should be considered, such as the type of crop for which they are intended, agronomic practices, seasonality, host variants, location, bacterial strains, soil fertility and interaction with soil micro-biota. It is essential to know the efficacy of fertilizers; for this, biological germination tests are used. There are numerous germination test models, including those using alfalfa (Medicago sativa L.), which has been common in microbiota and agricultural ecology laboratories for more than 20 years (Stewart et al., 2001; Knežević et al., 2021). Alfalfa is, among the 23 fodder legumes, one of the most cultivated. Its annual production amounts to around 450 million tones worldwide. The main exporters are the United States (30%), Europe (25%) and Argentina (23%; Barros et al., 2019).
One of the main problems in the recovery of plant waste is its potential to carry microorganisms that are pathogenic to plants, the majority of which are bacteria. The use of a contaminated fertilizer can threaten crop growth, the environment and human health. To avoid the spread of pathogens and contaminants, it is essential to know their precise microbiological composition. The identification of some of these microorganisms is very complex and expensive, and some may even be undetectable because of the difficulty of their cultivation or because of their low concentrations when using more conventional techniques (Blessing and Oluranti, 2022). Metagenomics enables the rapid and accurate identification of many micro-organisms, including those that are either uncultivable or underrepresented that either pose a risk or that provide value due to their ability to promote plant growth. PGP bacteria can act by increasing the cycle of nutrients, which is why their use is progressively increasing in agriculture as substitutes for chemical fertilizers, herbicides, antibiotics and pesticides (Wang et al., 2021). Numerous studies have been carried out to confirm this promising practice where Bacillus spp. and Pseudomonas spp. They have been proposed as good promoters of plant growth with great resistance to abiotic stress of crops, among other qualities (Katsenios et al., 2022).
For the above reasons, metagenomics is postulated as a suitable technique that allows for more thorough characterization of the composition of the microbial taxa of a certain matrix of a residue/leachate, identification of possible bacterial phytopathogens and the biological interpretation of fertilizer behavior (Blessing and Oluranti, 2022).
Given the impact of the intensive use of chemical fertilizers in cultivation on the environment and human health, it is necessary to increase the recovery of waste as a sustainable measure. To contribute to the knowledge of the techniques and measures that favor the sustainable exploitation of the soil, two objectives are proposed: (i) carry out physicochemical and metagenomic analyzes of ORGAON® for the identification of different taxa, whose results can confirm the safety of a fertilizer through the verification the absence of bacterial plant pathogens; (ii) evaluate the effect on germination, growth promotion and viability of Medicago sativa treated with a biofertilizer composed of the extract of a recovered plant leachate and the addition of the PGPB Pseudomonas agronomica, Bacillus pretiusos and its consortium.
Results
Agricultural leachate residue analysis
To comply with Annex IV statements of Regulation (EC) 2003/2003 and Annex VI of RD 506/2013 (2013), which establishes the physicochemical criteria for plant residues, physicochemical analysis of the ORGAON® fertilizer was done. The results are shown in Table 1.
The results meet the regulatory criteria applicable to fertilizer products with residues and other organic components (Hedo, 2013).
Metagenomic analysis: Sequencing, clustering and taxonomic assignment
Metagenomic analysis was carried out using different processes. As a result of the bioinformatic analysis, metrics and indices were obtained that allowed us to interpret the quality of the processing as well as the composition and taxonomic abundances of the microbial community of the sample. These parameters are shown in Table 2.
The following taxonomic categories were identified: 2 kingdoms, 10 phyla, 25 families, 32 genera and 34 species. An observed relative abundance of bacterial population of 99.62% and archaeobacteria population of 0.38% was found. The main bacterial taxa found in the samples, above 1% relative abundance, were Firmicutes (38.32 ± 0.25%), Proteobacteria (29.09% ± 0.16%) and Bacteroidetes (26.73% ± 0.17%). The taxonomic grouping of the taxa identified at division and order level in ORGAON® fertilizer is shown in Figure 1. The complete list of taxa and their relative abundance are collected in Supplementary Table S1.
No reference has been found in the scientific literature on the phyto-pathogenic potential of any of the identified species.
Alpha diversity indices
Metrics resulting from the alpha diversity indices reflect the structure of a community with respect to its richness (number of taxonomic groups that make up the community) or evenness (distribution of groups with respect to their abundance), or both (Willis, 2019). The analyzed indices and their ranges of interpretation are shown in the Table 3.
Optimum testing conditions for ORGAON® fertilizer
To analyze the efficacy of the fertilizer, its effect on the germination of the Medicago sativa L. plant model was tested. Figure 2 shows the results of the dilution tuning (v/v) of the ORGAON® fertilizer in mineral water. Each condition was tested in triplicate and the percentage of mean values is shown in the graph.
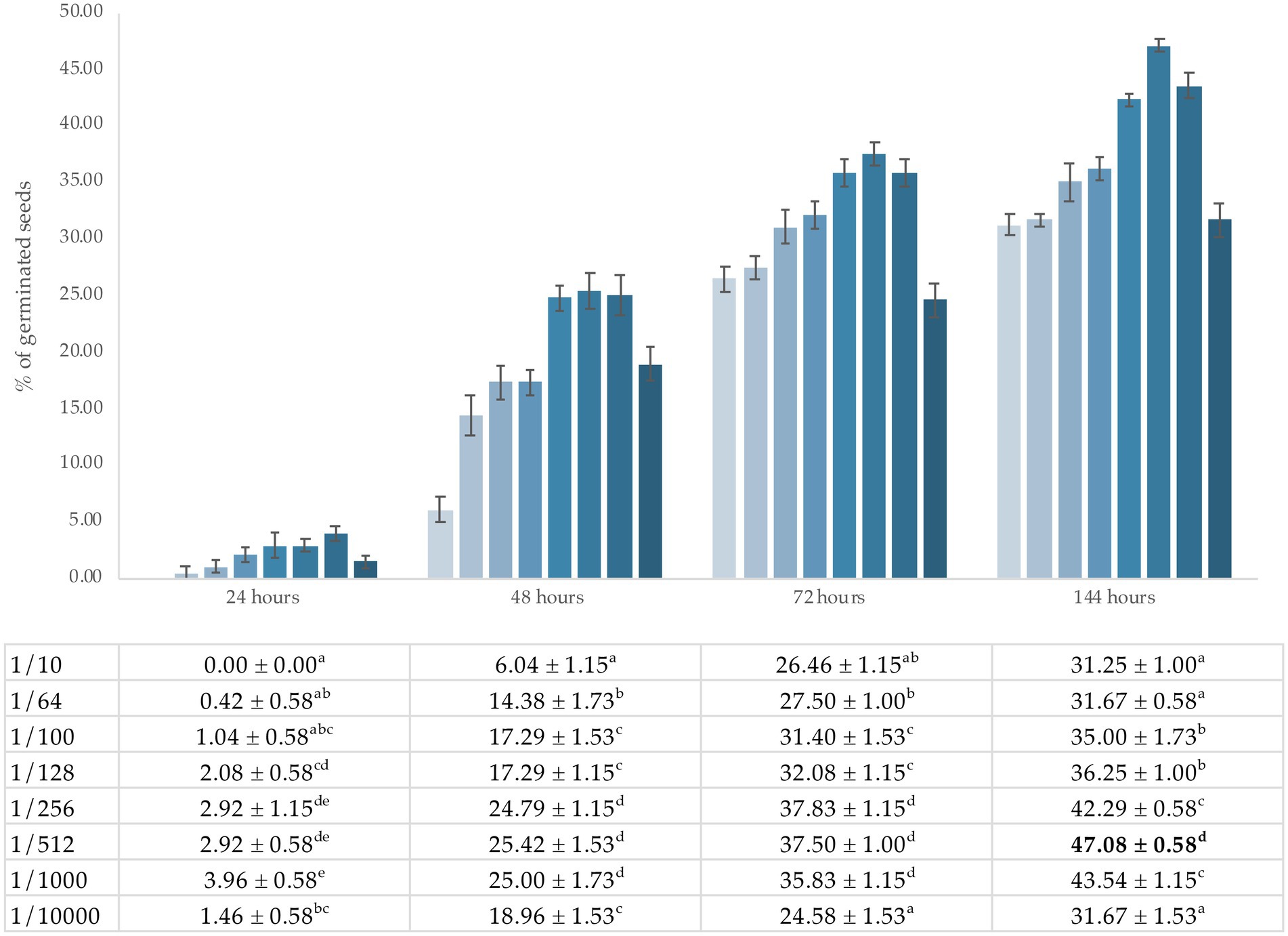
Figure 2. Percentage of germinated seeds after 24, 48, 72, and 144 h for each dilution. All values are the mean of three replicates. Results are followed ± SD (n = 3). The data with identical superscript letters indicate that the mean values are not significantly different.
The maximum germination rate was reached at dilution 1/512 (vFert ORGAON®/vmineral water) 144 h after sowing. Under these conditions, the germination rate was 46.88%.
The comparison between the germination rate in the described conditions versus the traditional treatment with Chem-F irrigation is shown in Figure 3.
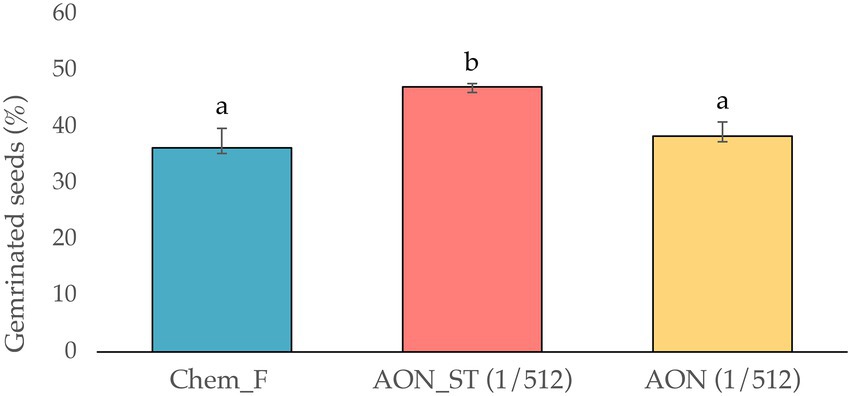
Figure 3. Germinated seeds (%) under different fertigation conditions. Results are followed ± SD (n = 3). Data with identical superscript letters indicate that the mean values are not significantly different.s
Germination trial
To know the effect of the addition of PGPB Pseudomonas agronomica, Bacillus pretiosus and its consortium in the matrix of ORGAON® and ORGAON®_ST fertilizers, a germination assay was carried out with Medicago sativa var. Aragon. The corresponding Chem-F controls were used, to which the same strains and consortium were added. All the measurements collected in Figure 4 correspond to the average of three replicates per treatment.
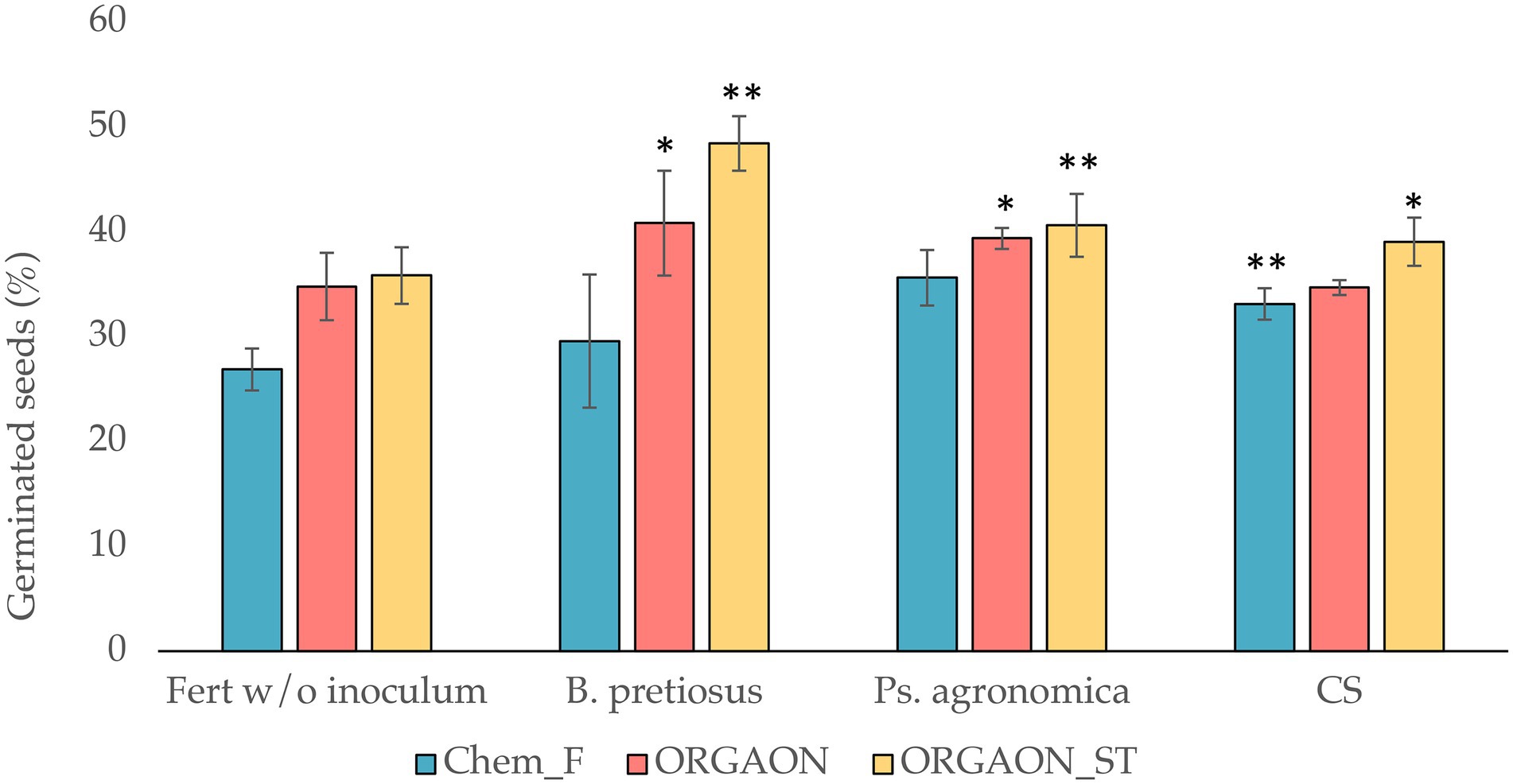
Figure 4. Germinated seeds (%) under the different fertigation conditions. ORGAON® and ORGAON®_ST at the experimental concentration 1/512. Asterisks represent treatments with significant differences in germination compared to their control without inoculum and traditional fertilization (Chem-F; *p < 0.05; **p < 0.01).
ORGAON® and ORGAON®_ST treatments without PGPB additives show higher germination rates than Chem-F. These increases are not significant. However, the addition of the Pseudomonas agronomica and Bacillus pretiosus to organic fertilizers achieve significantly higher germination rates with respect to their controls. The same happens with the treatment of Chem-F added with Pseudomonas agronomica and the bacterial consortium with respect to its control without bacterial inoculum.
In the trials of plants fertigated with ORGAON® and ORGAON®_ST, the greatest differential in the germination rate is observed in those treatments added with the Bacillus pretiosus strain. When this strain is tested in an ORGAON® matrix, the differential increase compared to the uninoculated fertilizer is 17.37% and increases to 35.17% when the bacteria is tested in an ORGAON®_ST matrix. The addition of Pseudmonas agronomica strain to the ORGAON® and ORGAON®_ST matrices also induced an increase in germination, with 13.37 and 17.13%, respectively. Likewise, the consortium of both induced a 9.01% increase in germination in the treatment with ORGAON®_ST with respect to the chemical fertilizer Chem-F added with the same strains.
Plant growth assay
A growth assay was performed, reproducing the irrigation conditions with Chem-F, ORGAON® and ORGAON®_ST in the presence and absence of independent and consortiated PGPB. Figure 5 shows the variation of the dry weight of the plants once the trial is over.
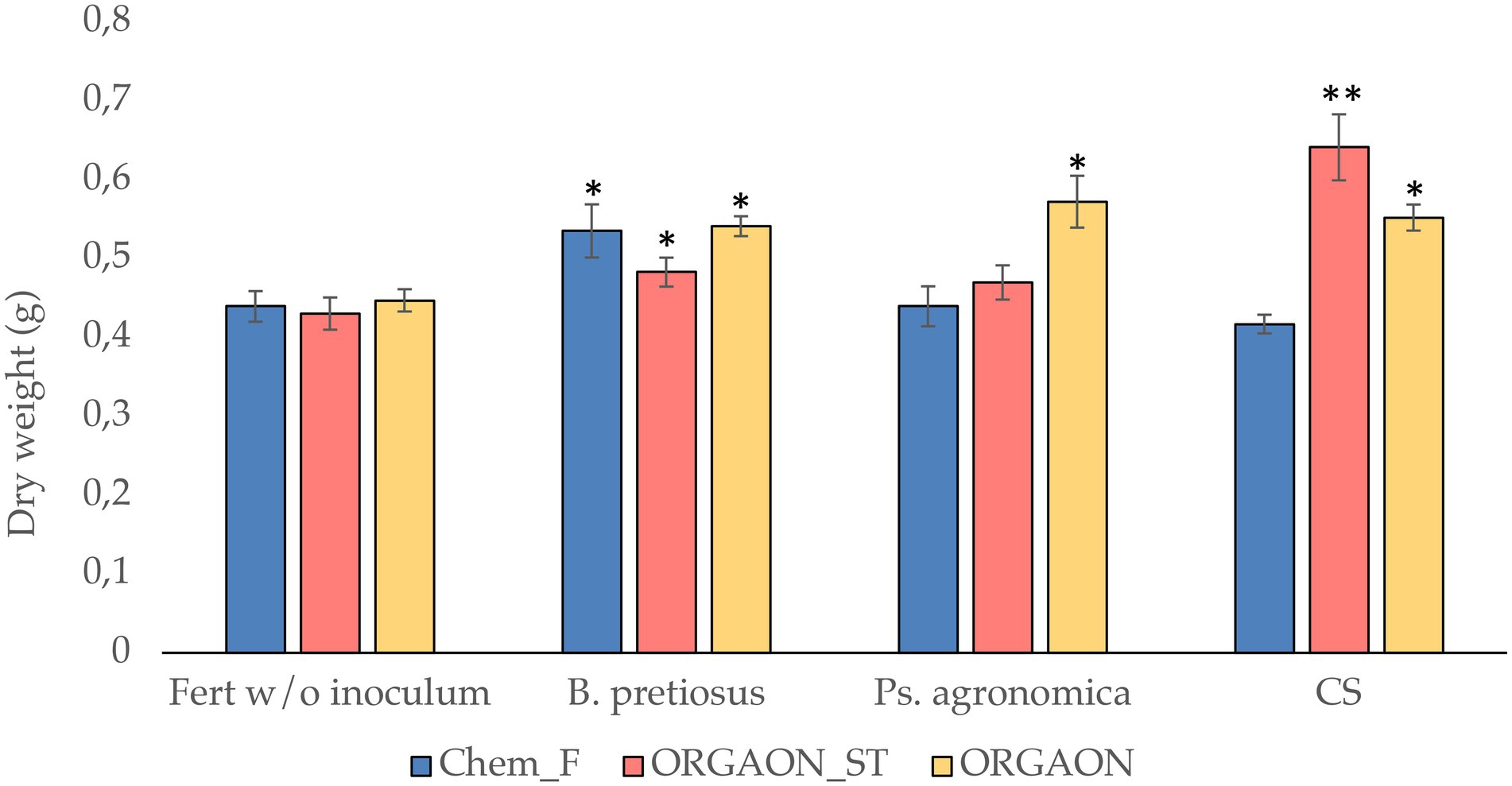
Figure 5. Dry weight (g) of seedlings after 6 weeks of growth. The asterisks represent significant differences with respect to the control without inoculum and traditional fertilization (Chem-F; *p < 0.05; **p < 0.001).
A significant increase in dry weight is observed in those plants treated with ORGAON® added with Pseudomonas agronómica, Bacillus pretiosus and their consortium, compared to any fertilizer control without bacterial addition.
Seedling viability test
Seedling viability was analyzed 6 weeks after germination. Figure 6 shows the results of the count of viable plants.
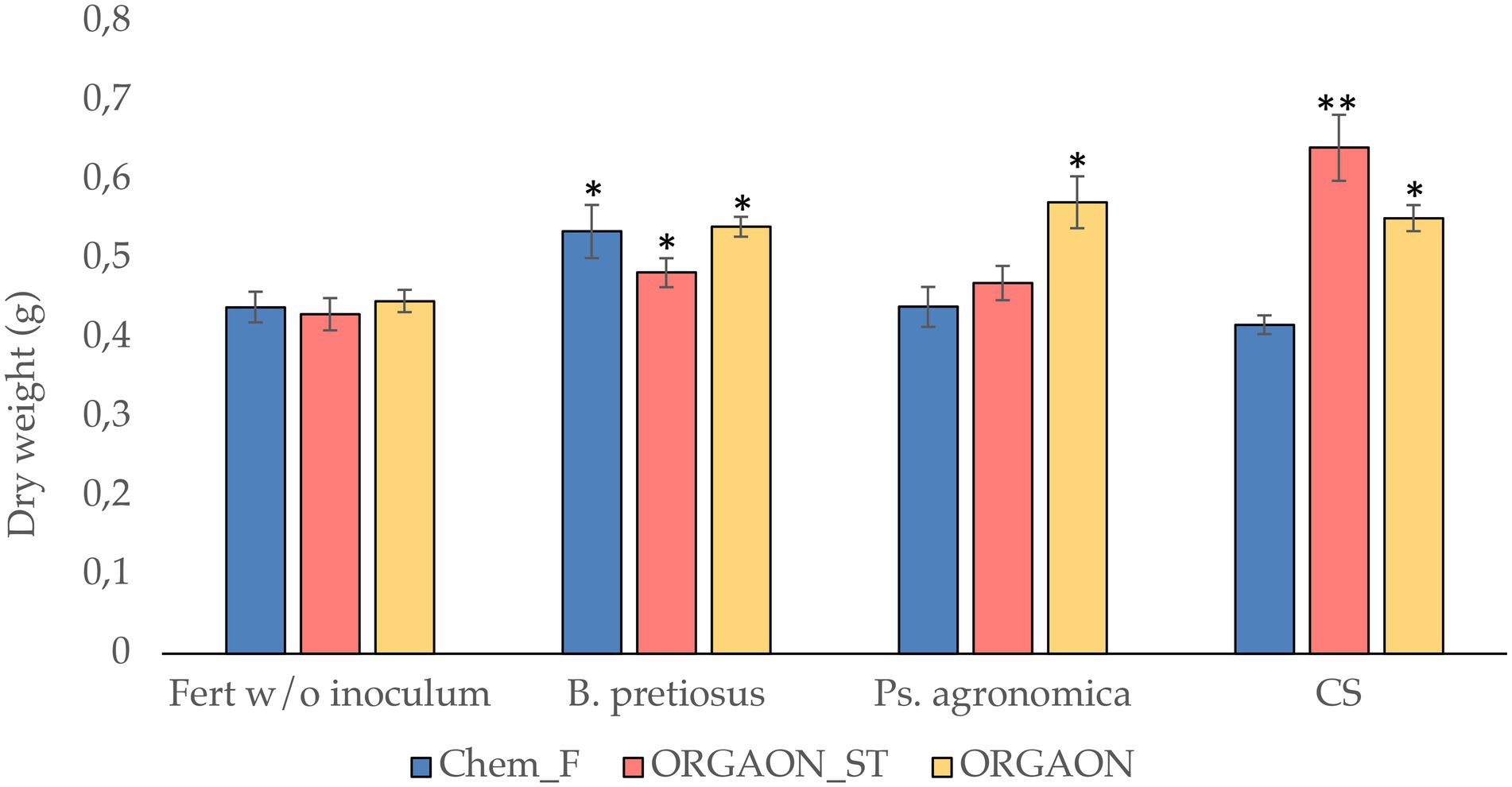
Figure 6. Seed viability (No.) at 6 weeks of growth. Asterisks represent treatments with significant differences in germination compared to their control without inoculum and traditional fertilization (Chem_F; *p < 0.05; **p < 0.01).
The addition of Bacillus pretiosus to any fertilizer significantly favors the viability of the treated plants. Said phytoprotection is remarkably superior when the bacterium is in the presence of the ORGAON® fertilizer, both in its natural formulation and in its sterilized form. The PGPB Pseudomonas agronomica and the consortium treatment of both show similar results when tested in the ORGAON® matrix.
Discussion
The use of waste as a raw material for sustainable industrial and agronomic processes is a priority. It is necessary to ensure compliance with the nutrient concentration requirements of current environmental regulations. At the physicochemical level, it is essential that the concentration of toxic substances is minimal (Hedo, 2013). It is also important to characterize the microbiological composition to guarantee the safety and security of the product prior to mass agronomic application. The ORGAON® dilution factor that proved to have a better effect on germination was 1/512 (on mineral water). For this concentration to guarantee the efficacy of the organic fertilizer, the standardization of the physicochemical variables must be guaranteed (Table 1). it must be taken into account to reproduce the same dilution factor and optimize its use in plant growth and development (Phibunwatthanawong and Riddech, 2019).
Metagenomic analysis (mNGS) is based on unbiased sampling. In the present study, this technique achieved taxa identification at the species level for 78.6% of the observed relative abundance in the sample. This method allows the identification of expected, un-expected and unknown microorganisms. Likewise, it allows obtaining quantitative or semiquantitative data on the absolute and relative concentrations of each identified taxon (Gu et al., 2019). For these reasons, it is widely used as a routine method in the analysis of complex environmental samples (Franssen et al., 1784; Borthong et al., 2018; Taş et al., 2021). Authors such as Suttner et al. consider metagenomics to be a useful tool for the identification of microorganisms, risk assessment and the minimization of public health hazards (Suttner et al., 2020).
The bacterial communities present in complex mixtures, such as fertilizers, can be extremely heterogeneous. In the ORGAON® fertilizer studied, the main isolates belong to the phyla Proteobacteria, Actinobacteria, Bacteroidetes and Firmicutes, accounting for 94.38% of the heterogeneity. These results agree with metagenomic studies by other authors, who described these as the predominant taxa (Clúa et al., 2018). Alphaproteobacteria class has been referred to in the use of fertilizers. Its abundance is dependent on the concentration of nutrients, increasing in the presence of phosphate due to their ability to solubilize it and decreasing with increased nitrogen. This class accounts for 25.81% of the sample (Dai et al., 2020). The Clostridiaceae family is identified as one of the groups in which there is biotechnological interest for the development of organic nitrogen fertilizers. This family is present in 3.34% of the sample (Ward et al., 2018). Other authors note the presence of Clostridiales (30.33%) in fertilizers; this taxonomic order is known to have the ability to reduce ferric iron (Fe3+), and most of its species can fix nitrogen [II]. When considering a lower taxonomic resolution, no references in the scientific literature on natural fertilizers have been found regarding the predominance of those taxa identified, in the most abundant sample. However, references to some less preponderant taxa have been identified in studies on the selective gradient of micro-biological diversity along the root–plant continuum, which confirm the presence and abundance of the genus Bacillus. In these samples, species belonging to this genus were present in 3.23%; likewise, the order Pseudomonadales corresponded to 0.66% of the relative microbial abundance of the ORGAON® fertilizer (Clúa et al., 2018).
One of the dangers in the use of natural fertilizers whose origin is a plant extract is the potential transmission of bacterial phytopathogens to the target crops. For this reason, it is necessary to detect these pathogens in samples to ensure environmental biosafety (Franssen et al., 1784; Borthong et al., 2018). Discrimination of the presence or absence of bacterial phytopathogens requires identification at the species, subspecies or pathotype level, as appropriate (Franssen et al., 1784). In taxonomic identification studies of isolated culturable bacteria, the identity index with which the 16S sequence of the bacterium is aligned should not be less than 99%. This ensures accurate identification of the isolated strain. In metagenomics analysis, there is a risk of not detecting a plant pathogen if it is found in very low proportions (Miller et al., 2013; Andrusch et al., 2018) or when the analysis involves unspecific sequences (Borthong et al., 2018). To avoid this, the identity index threshold is reduced. In this way, the detection of incomplete or minority sequences could be used in alignments for determining the presence of the corresponding organisms. In line with what was observed in another study (Hao and Chen, 2012), we reduced the identity index requirement to 90% in the present work. For this reason, any sequence like the identification of a phytopathogen will allow its detection. In the same sense, surveillance based on nontargeted sequencing is being promoted as part of several European initiatives—the European Epidemiological Network (Epi-NET) and the EU Action Plan—intending to include the development of integrated surveillance systems (Duarte et al., 2020). Having applied this criterion, in the present work, the results indicate that the ORGAON® sample is absent of bacterial phytopathogens.
ORGAON® fertilizer combines plant residues from various crops, resulting in a rich liquid fraction that carries PGPB organisms. The alpha diversity metrics of all calculated indices shows a wide diversity. These indices are presented as good indicators for monitoring ecological or phenological samples.
The Shannon index (Dai et al., 2020) is commonly used to characterize the diversity of species in a community and explains both the abundance and evenness of the species present. The analysis of the ORGAON® fertilizer yields a value of 3.64 on the Shannon index, which indicates high microbial diversity. This measure considers the proportion of each species in a studied ecosystem, thus providing a good representation of diversity, beyond a simple count of the number of species (Konopiński, 2020) [50]. However, this index has limitations in the weighting of ZOTUs (Hill et al., 2003). To overcome this, other alpha diversity indices were used. The Pielou (J) index (0–1) applied to the ORGAON® fertilizer sample is 0.80, which corroborates the high diversity estimated by the Shannon index. Likewise, Simpson’s index (D) measures the proportion of observed diversity in relation to the maximum expected diversity. Values close to 1 correspond to situations where all species are equally abundant, and 0 indicates the degree of maximum inequality (Soler et al., 2012). The result obtained for this index reflects a result consistent with the Shannon and Pielou index. Finally, the phylogenetic diversity index (PD) uses phylogenetic patterns of evolutionary diversification to estimate the evolutionary diversity of a community. The result of 10.34 indicates a high phylogenetic diversity, and it can be interpreted that the ORGAON® fertilizer matrix can host taxonomically and functionally very diverse organisms. In the Earth Microbiome Project (EMP), more than 11,000 samples from different ecosystems were analyzed, and the alpha, beta and gamma diversities of bacterial assemblages were calculated. Agricultural soils were found to be one of the richest systems. The EMP has continued its analysis, with more than 27,000 samples from different geographical areas. A recent review of the project pointed out that there is a higher diversity of free-living bacteria than those associated with a host, so that a soil or sediment sample would reach a higher alpha diversity than a gut micro-biome. The results obtained in the ORGAON® metrics agree with current study, in which plant residues and agricultural land provide an optimal environment for microbial richness (Walters and Martiny, 2020). From the above, it can be interpreted that the ORGAON® fertilizer analyzed in the present work respects the diversity of edaphic microbial communities.
Germination data show that the maximum rate was reached at dilution 1/512 (vFert ORGAON®/vmineral water) 144 h after sowing. Under these conditions, the germination rate was 46.88%. This increase affects both the germination rate and the viability of Medicago sativa seed. These effects depend on the concentration (v/v) of the fertilizer, with the optimum concentration being 1/512 dilution. The average germination time is 2–4 days in a temperature range of 22 ± 2° C, as established by Benabderrahim et al. (2011). The number of germinated seeds was 46%, a percentage that falls within the expected range presented in other studies (Benabderrahim et al., 2011). However, fertilizer concentrations significantly affect the germination percentage and need to be adjusted to optimum levels. The percentage of germinated seeds is in accordance with results described by other authors, who mention that organic fertilizers are nutritional supplements that improve the yield and growth of agricultural crops (Gómez-Álvarez et al., 2008; Li et al., 2020). The reason for this is the increase in the availability of nutrients that are not easily assimilated by the plant under natural conditions and thus improve soil degradation.
The addition of Pseudomonas agronomica and Bacillus pretiosus to ORGAON® and ORGAON®_ST induce significantly higher germination rates with respect to the fertilizer controls without additives with PGPB. The same happens with the treatment of Chem-F added with Pseudomonas agronomica and the bacterial consortium with respect to its control without bacterial inoculum. This fact evidences the promoting effect of both PGPB on the germination of Medicago sativa seeds regardless of the fertilizer matrix that contains them. The addition of PGPB bacteria facilitates the nutrient acquisition in plants, increasing the germination percentage. The fact that it is a liquid fertilizer means that it is distributed in a more homogeneous way, being available in similar concentrations for the entire plant (Scialabba and Hattam, 2002).
Likewise, the plants treated with ORGAON® show significant differences for dry weight in any of their supplemented treatments, both with Pseudomonas agronomica, Bacillus pretiosus as its consortium. Similar results are obtained when the tested fertilizer is ORGAON®_ST. This fact reinforces the explanation of the beneficial effect of the transformation of the organic fertilizer by the metabolic action of PGPB, which gives rise to a better availability of nutrients for the plant (Gómez-Álvarez et al., 2008). Therefore, the bacteria that are added to organic fertilizers improve the conditions of the plant, both directly through the synthesis of biomolecules with PGP activity, and indirectly through the prolonged provision for the plant of easily adsorbed transformed nutrients. This transformation optimizes the use of nutrients, which leads us to think that their use is more efficient and sustainable (Li et al., 2020).
After germination, seedling viability can be compromised by numerous environmental factors. Among the microbial biotic factors that can compromise viability are the colonization of phytopathogens (Kumar and Verma, 2018) or the loss of competitiveness due to the absence of beneficial bacteria for the plant (Cheng et al., 2020). The edaphic and rhizospheric colonization of PGPB hinders the implantation and development of other microorganisms that could behave as bacterial phytopathogens (Parray et al., 2016; Doty, 2018; Gouda et al., 2018). In this sense, the viability of the seedlings is significantly higher when the treatment consists of the contribution of Bacillus pretiosus, Pseudomonas agronomica and their consortium in the presence of the ORGAON® fertilizer in its natural formulation. The HCN synthesis capacity of both Pseudomonas agronomic as of Bacillus pretiosus (Robas Mora et al., 2022) minimizes the possibility of root colonization of phytopathogens. Thus, the presence of PGPB microorganisms in this type of fertilizer could have a phytoprotective effect (Backer et al., 2018; Naeem et al., 2018; Blake et al., 2021). Thus, the joint effect of all these factors can explain the significant difference in viability of the plants treated together with ORGAON® added with the PGPB under study.
Prior to the use of these fertilizers, biosafety must be guaranteed. In this way, such fertilizers can be a sustainable alternative for organic. Liquid biofertilizers are associated with higher crop yields and therefore lower costs (Ye et al., 2020). Previous studies have shown that inoculation with PGPB organisms on crops treated with chemical fertilizers increases nutrient use efficiency. This efficiency is measured by considering plant growth. Likewise, PGPB organisms reduce the need for chemical fertilizers by 50% without causing yield loss. According to the studied parameters, the addition of these fertilizers stimulates germination, promotes plant growth, and can have a positive effect on the edaphic microbial community (Hayat et al., 2010; Good and Beatty, 2011; Da Costa et al., 2013).
For all these reasons, it can be concluded that the fertilizer ORGAON® can be used under biosafety conditions since the metagenomic study of the 16S rRNA amplicons reveals the absence of pathogens and phytopathogens. Experimentally, the maximum germination rate of Medicago sativa seeds (46.88%) was reached at the 1/512 dilution (vFert ORGAON®/vmineral water) 144 h after inoculation. Likewise, irrigation with ORGAON® fertilizer under these conditions results in a significant increase in germination, 16% more than traditional irrigation with inorganic fertilizers (Chem-F). Finally, the incorporation of Pseudomonas agronomica and Bacillus pretiosus strains in ORGAON® (biofertilizer) promotes germination, total biomass, and viability of Medicago sativa var. Aragon.
Materials and methods
Sample collection
The collection of plant waste is carried out over 30,000 hectares of greenhouses, from which two million tons are collected, including stems and leaves, and discarding fruits and complete plants (Biaqui, Almería, Spain). This land extends over different farms in the region of Dalías, province of Almería, Spain (36°49′17″N 2°52′13″W). The average annual temperature is 18°C, and the annual sunshine duration is 3,305 h. These remains must be compulsorily collected and treated for their elimination or revaluation. During composting, a liquid fraction (leachate) is produced, composed mainly of the water from the plants that are being composted. The liquid fraction is subjected to a stabilization process once collected from the composter and then stored (ORGAON®). To evaluate the leachates, a 1 l sample was collected in triplicate. The sample was deposited into a sterile container and transported and stored at 4°C for immediate processing. Each sample was made up of four 250 ml subsamples, taken from different depths of the collection tank (5 m3), where the leachate is collected and stored under aerated conditions.
Fertilizer composition
ORGAON® fertilizer
Table 4 shows the physicochemical analysis carried out on the samples and the respective methods used in accordance with Annex IV to Regulation (EC) 2003/2003 and Annex VI to RD 506/2013 of 28 June, which specify the physicochemical criteria for plant residues.
ORGAON®_ST fertilizer
The synthesis of ORGAON_ST® consisted of exposing a thin film of OR-GAON® to UV light for 15 min, to avoid substantial changes in its physicochemical composition.
Chemical-fertilizer (Chem-F)
PK complex fertilizer is rich in phosphorus and potassium, with magnesium and Sulphur. Low in chloride. Composition: nitrogen 0% + phosphorus 7% + potassium 14% + sulfur 25%. Formulation: (K2HPO4 5%); (K2SO4 14%); (MgSO₄ 2.5%).
Metagenomic analysis
Extraction of DNA and PCR
DNA was extracted from 200 μl of undiluted sample (prepared in Section 2.1) using the All Prep Power Viral RNA/DNA (Qiagen, Hilden, Germany) kit, according to the manufacturer’s instructions. A total of 3 ng of DNA (measured by Picogreen, Thermofisher) was used for the first PCR, with the enzyme Q5Ǐ Hot Start High-Fidelity DNA Polymerase (New England Biolabs), in a volume of 25 μl and with a concentration of primers of 100 nM. The primers used amplified the 16S rRNA V3–V4 regions and added extra sequences, on which the second PCR was performed:
CS1-341F 5′-ACACTGACGACATGGTTCTACACCTACGGGNGGCWGCAG-3′.
CS2-805R ′-TACGGTAGCAGAGACTTGGTCTGACTACHVGGGTATCTAATCC-3′.
The CS1 and CS2 sequences are indicated in bold. The cycling of the first PCR was as follows: 1 × 98°C for 30 s; 20 × (98°C for 10 s, 50°C for 20 s and 72°C for 20 s) and 1 × 72°C for 2 min.
The second PCR was in a volume of 20 μl over 1 μl of the amplification product of the first 1/50 diluted PCR. For this purpose, the enzyme Q5Ǐ Hot Start High-Fidelity DNA Polymerase (New England Biolabs) was used in the presence of 400 nM of the primers 5′-AATGATACGGCGACCACCGAGATCTACACTGACATGGTTCTACA-3′ and 5′-CAAGCAGAAGACGGCATACGAGAT-[10-barcode-nucleotides]-TACGGTAGCAGAGACGGTCT-3′ from the Fluidigm house (see Array Barcode Li-brary for Illumina Sequencers). The cycling conditions for this PCR were as follows: 1 × 98°C for 30 s; 10 × (98°C for 10 s, 60°C for 20 s and 72°C for 20 s) and 1 × 72°C for 2 min. The final product was purified with AMPure Beads (Beckman Coulter, California, United States) and verified and quantified with Bioanalyzer (Agilent, California, United States).
Sequencing and obtaining ZOTUs
The total ampoules were sequenced in team MiSeq Illumina (Illumina, California, United States), following instructions from the manufacturer, in a race pair end 2 × 300 using “MiSeq reagent kit v3 600 cycles” (Illumina, California, United States). Preprocessing and quality control were carried out using FastQC (Callahan et al., 2017), visualizing with MultiQC (Edgar, 2010) to eliminate low-quality sequences. Subsequently, the sequences were cleaned, filtering and eliminating those pairs presented too many differences or did not adequately overlap. We proceeded to group all the remaining sequencing reads for establishing ZOTU, determining which were unique and ordering them according to their total abundance. The chimera sequences and possible artifact sequences were then eliminated using the UCHIME algorithm (Andrews, 2010; Ewels et al., 2016). Once the “nonchimeric” sequences were obtained, the clusterization of ZOTU was performed using the USEARCH-UNOISE3 algorithm (Edgar et al., 2011; Edgar, 2018) with 99% identity for the generation of sequence clusters, aiming at the reconstruction of exact biological sequences. Finally, the ZOTU table was created, which collects the count of each ZOTU found per sample. With the ZOTU sequences detected, they were specifically aligned using the USEARCH U-LOCAL (Edgar, 2016a) algorithm in two nested rounds. The first specific round was aligned against a database, developed expressly for this study, of possible recurrent pathogenic bacteria in this type of samples, with a 99% alignment percentage. A second round was conducted with the taxa that remained unallocated in the first round; a specific alignment was made, but with the RDP database (Edgar, 2016b) and 90% identity. Finally, those ZOTU that were left without taxonomic assignment were classified according to the GreenGenes database v13 5 (Thermo Fisher, Madrid, Spain; Edgar, 2017), using the USEARCH predictor algorithm SINTAX (Chelius and Triplett, 2001). A cutoff point was applied based on the identity of this allocation relationship, fixed at a minimum of 97% identity, so that it was filtered to the taxonomic level at which this degree of identity was exceeded.
Indices of diversity
The following indices were used to calculate alpha diversity: Simpson’s index (D; Simpson, 1949), Shannon index (H; Shannon, 2001), Pielou index (J; Pielou, 1969) and phylogenetic diversity index (PD; Faith, 1992).
Biological assays on Medicago sativa (alfalfa) model
Determination of the test conditions of the ORGAON® fertilizer
The optimal concentration of the ORGAON® fertilizer was determined for the germination of alfalfa seeds (Medicago sativa L.) under controlled laboratory conditions. Serial dilutions (v/v) 1/1, 1/10, 1/64, 1/100, 1/128, 1/256, 1/512 and 1/1000 (mL ORGAON®.ml–1 / mineral water) were used. For the germination test, sterile 140 mm Petri dishes were used as containers, in which sterile filter paper (UV light 30 min) was placed on the base. Once sterilized, the filter paper discs were saturated with 4.5 ml of each dilution (experimental volume), avoiding the accumulation of excess liquid. A plate was used for each dilution of ORGAON® fertilizer (Biaqui, Almería, Spain). A total of 160 alfalfa seeds were added for each treatment and evenly distributed using sterile tongs. Plates were then closed with a superimposed lid (under aerobic conditions, avoiding contamination by aerial microorganisms), and left in darkness at controlled temperature (22 ± 2°C). Each condition was assayed in triplicate. Germinated seeds (denoted by visible emerging radicle) were daily checked.
Sterilization of ORGAON® fertilizer
For the control, the ORGAON® (1/512) was sterilized using the same procedure described in section 4.2.2.
Improvement of ORGAON® fertilizer with the incorporation of PGPB strains: Biofertilizer synthesis
The bacterial strains tested in this article were selected from previous studies, in which they were shown to be PGPB (Table 5).
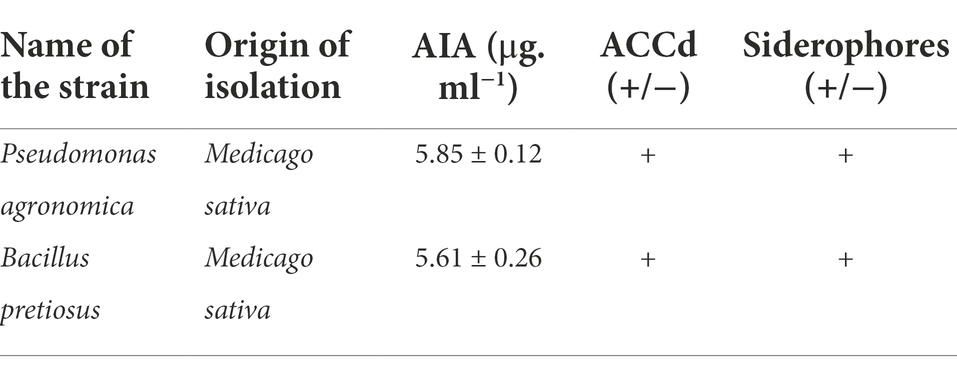
Table 5. Information of the PGPB strains (Robas et al., 2021).
For enrichment of the ORGAON® fertilizer with the proposed PGPB bacterial strains, the inoculum was standardized by making a bacterial suspension in 0.45% sterile saline solution with a final optical density of bacteria equivalent to 0.5 on the Mc Farland scale. 3 ml of these suspensions were added to 3 ml of a prepared 1/256 ORGAON® dilution, achieving a final 1/512 dilution of the fertilizer.
Inoculation of the seeds and irrigation conditions
The preparation of the germination and plant growth tests were identical. A total of 100 seeds per treatment were sown in pots with a bottom of 2 cm3 of sterile river sand and 3 cm3 of agricultural soil. In total, 12 treatments were carried out, with three replicates each. Three types of irrigation were used, Chem-F, non-sterile ORGAON® and sterile ORGAON® (ORGAON®_ST). Each type of irrigation was enriched by adding the proposed bacteria: Pseudomonas agronomica, Bacillus pretiosus, the consortium of both, and an inoculum-free control. Each pot had an initial irrigation of 150 ml of treatment up to field capacity. Subsequently, it was irrigated four times with an experimental volume of 50 ml each.
Germination and plant growth conditions
The first days of germination, the pots were in natural light conditions. Once the seeds began to germinate, the pots were transferred to a programmed phytotron with a photoperiod of 11 h of light, light intensity of 505 μmoles.m−2.s−1 and a temperature range of 15–30°C.
Dry weight determination
Six weeks after the start of the trial, the seedlings were harvested. The collected biomass was dried at room temperature for 2 days. It was then weighed.
Statistical analysis
Once the null hypothesis of equality of means (ANOVA) had been rejected, to verify the existence of statistically significant differences between the different concentrations of ORGAON®, the post-hoc multiple comparison test of Duncan was performed. Differences were considered statistically significant when p < 0.05. To study the existence of significant differences between seeds irrigated with the different treatments, ORGAON® fertilizer, sterilized ORGAON® fertilizer, and the traditional Chem-F irrigation, the nonparametric Kruskal–Wallis test (Mann and Whitney, 1947; N < 30) was carried out. Differences were considered statistically significant when p < 0.05. To verify the effect of each treatment and the incorporation of the PGPB strains, an ANOVA was carried out. Rejecting the null hypothesis of mean equality, a pairwise comparison was carried out using Duncan’s post-hoc statistic. Differences were considered statistically significant when p < 0.05. The statistical package SPSS (Version 27.0, IBM Corp.) was used for all analyses.
Conclusion
In view of the results obtained, the following conclusions can be drawn:
1. The fertilizer can be used in biosafety conditions. The metagenomic study of 16S rRNA amplicons reveals the absence of pathogens and phytopathogens.
2. Irrigation with ORGAON® fertilizer (v/v; 1/512) results in a significant germination increase, 16% over regular Chem-F irrigation.
3. The maximum germination rate was reached at dilution 1/512 (vFert ORGAON®/vmineral water) 144 h after sowing. Under these conditions, the germination rate was 46.88%.
4. The addition of the bacterial strain Pseudomonas agronomica or Bacillus pretiosus in the fertilizer promotes an increase in germination, biomass, and viability of Medicago sativa var. Aragón.
Data availability statement
The data presented in the study are deposited in the BioProyect repository, accession number PRJNA906533.
Author contributions
VF, MR, AP, and PJ: conceptualization, methodology, formal analysis, and writing—review and editing. VF, MR, and PJ: software and investigation. AP: validation and data curation. AP and PJ: resources, supervision, project administration, and funding acquisition. VF and MR: writing—original draft preparation and visualization. All authors contributed to the article and approved the submitted version.
Funding
This research has been funded by FUNDACIÓN UNIVERSITARIA SAN PABLO CEU (Microbiology SAI) and BANCOs SANTANDER, grant number FUSP-BS-PPC01/2014. Likewise, this project has been financed with the European Funds oriented to the ecological transition and digital transition, of the national plan for scientific, technical and innovation research 2021-2023, within the framework of the recovery, transformation and resilience plan. File number TED2021-132285A-I00.
Conflict of interest
The authors declare that the research was conducted in the absence of any commercial or financial relationships that could be construed as a potential conflict of interest.
Publisher’s note
All claims expressed in this article are solely those of the authors and do not necessarily represent those of their affiliated organizations, or those of the publisher, the editors and the reviewers. Any product that may be evaluated in this article, or claim that may be made by its manufacturer, is not guaranteed or endorsed by the publisher.
Supplementary material
The Supplementary material for this article can be found online at: https://www.frontiersin.org/articles/10.3389/fmicb.2022.1048154/full#supplementary-material
References
Andrusch, A., Dabrowski, P. W., Klenner, J., Tausch, S. H., Kohl, C., Osman, A. A., et al. (2018). PAIPline: pathogen identification in metagenomic and clinical next generation sequencing samples. Bioinformatics 34, i715–i721. doi: 10.1093/bioinformatics/bty595
Backer, R., Rokem, J. S., Ilangumaran, G., Lamont, J., Praslickova, D., Ricci, E., et al. (2018). Plant growth-promoting rhizobacteria: context, mechanisms of action, and roadmap to commercialization of biostimulants for sustainable agriculture. Front. Plant Sci. 9:1473. doi: 10.3389/fpls.2018.01473
Barros, J., Temple, S., and Dixon, R. A. (2019). Development and commercialization of reduced lignin alfalfa. Curr. Opin. Biotechnol. 56, 48–54. doi: 10.1016/j.copbio.2018.09.003
Benabderrahim, M., Haddad, M., Hamza, H., and Ferchichi, A. (2011). Germination and emergence variability of alfalfa (Medicago sativa L.) landraces collected in southern Tunisia oases. Span. J. Agric. Res. 9, 135–143. doi: 10.5424/sjar/20110901-075-10
Blake, C., Christensen, M. N., and Kovács, Á. T. (2021). Molecular aspects of plant growth promotion and protection by Bacillus subtilis. Mol. Plant-Microbe Interact. 34, 15–25. doi: 10.1094/MPMI-08-20-0225-CR
Blessing, N., and Oluranti, O. (2022). Metagenomics: a tool for exploring key microbiome with the potentials for improving sustainable agriculture. Front. Sustain. Food Syst. 6:886987. doi: 10.3389/fsufs.2022.886987
Borthong, J., Omori, R., Sugimoto, C., Suthienkul, O., Nakao, R., and Ito, K. (2018). Comparison of database search methods for the detection of legionella pneumophila in water samples using metagenomic analysis. Front. Microbiol. 9:1272. doi: 10.3389/fmicb.2018.01272
Callahan, B. J., McMurdie, P. J., and Holmes, S. P. (2017). Exact sequence variants should replace operational taxonomic units in marker-gene data analysis. ISME J. 11, 2639–2643. doi: 10.1038/ismej.2017.119
Chelius, M., and Triplett, E. (2001). The diversity of Archaea and bacteria in association with the roots of Zea mays L. Microb. Ecol. 41, 252–263. doi: 10.1007/s002480000087
Chèneby, D., Bru, D., Pascault, N., Maron, P.-A., Ranjard, L., and Philippot, L. (2010). Role of plant residues in determining temporal patterns of the activity, size, and structure of nitrate reducer communities in soil. Appl. Environ. Microbiol. 76, 7136–7143. doi: 10.1128/AEM.01497-10
Cheng, H., Zhang, D., Huang, B., Song, Z., Ren, L., Hao, B., et al. (2020). Organic fertilizer improves soil fertility and restores the bacterial community after 1, 3-dichloropropene fumigation. Sci. Total Environ. 738:140345. doi: 10.1016/j.scitotenv.2020.140345
Clúa, J., Roda, C., Zanetti, M. E., and Blanco, F. A. (2018). Compatibility between legumes and rhizobia for the establishment of a successful nitrogen-fixing symbiosis. Genes 9:125. doi: 10.3390/genes9030125
Colglazier, W. (2015). Sustainable development agenda: 2030. Science 349, 1048–1050. doi: 10.1126/science.aad2333
Da Costa, P. B., Beneduzi, A., de Souza, R., Schoenfeld, R., Vargas, L. K., and Passaglia, L. M. (2013). The effects of different fertilization conditions on bacterial plant growth promoting traits: guidelines for directed bacterial prospection and testing. Plant Soil 368, 267–280. doi: 10.1007/s11104-012-1513-z
Dai, Z., Liu, G., Chen, H., Chen, C., Wang, J., Ai, S., et al. (2020). Long-term nutrient inputs shift soil microbial functional profiles of phosphorus cycling in diverse agroecosystems. ISME J. 14, 757–770. doi: 10.1038/s41396-019-0567-9
Doty, S. L. (2018). Symbiotic plant-bacterial Endospheric interactions. Microorganisms 6:28. doi: 10.3390/microorganisms6020028
Duarte, A. S. R., Stärk, K. D., Munk, P., Leekitcharoenphon, P., Bossers, A., Luiken, R., et al. (2020). Addressing learning needs on the use of metagenomics in antimicrobial resistance surveillance. Front. Public Health 8:38. doi: 10.3389/fpubh.2020.00038
Edgar, R. C. (2010). Search and clustering orders of magnitude faster than BLAST. Bioinformatics 26, 2460–2461. doi: 10.1093/bioinformatics/btq461
Edgar, R. C. (2016a). UCHIME2: improved chimera prediction for amplicon sequencing. Bio Rxiv [Epub ahead of preprint], doi: 10.1101/074252
Edgar, R. C. (2016b). UNOISE2: improved error-correction for Illumina 16S and ITS amplicon sequencing. Bio Rxiv [Epub ahead of preprint], doi: 10.1101/081257
Edgar, R. C. (2017). Accuracy of microbial community diversity estimated by closed-and open-reference OTUs. Peer J. 5:e3889. doi: 10.7717/peerj.3889
Edgar, R. C. (2018). Updating the 97% identity threshold for 16S ribosomal RNA OTUs. Bioinformatics 34, 2371–2375. doi: 10.1093/bioinformatics/bty113
Edgar, R. C., Haas, B. J., Clemente, J. C., Quince, C., and Knight, R. (2011). UCHIME improves sensitivity and speed of chimera detection. Bioinformatics 27, 2194–2200. doi: 10.1093/bioinformatics/btr381
Ewels, P., Magnusson, M., Lundin, S., and Käller, M. (2016). Multi QC: summarize analysis results for multiple tools and samples in a single report. Bioinformatics 32, 3047–3048. doi: 10.1093/bioinformatics/btw354
Faith, D. P. (1992). Conservation evaluation and phylogenetic diversity. Biol. Conserv. 61, 1–10. doi: 10.1016/0006-3207(92)91201-3
Franssen, F. F., Janse, I., Janssen, D., Caccio, S. M., Vatta, P., van der Giessen, J. W., et al. (1784). Mining public metagenomes for environmental surveillance of parasites: a proof of principle. Front. Microbiol. 12:622356. doi: 10.3389/fmicb.2021.622356
Gómez-Álvarez, R., Lázaro-Jerónimo, G., and León-Nájera, J. (2008). Producción de frijol (Phaseolus vulgaris L.) y rábano (Rhabanus sativus L.) en huertos biointensivos en el trópico húmedo de Tabasco. Universidad y ciencia 24, 11–20. doi: 10.19136/era.a24n1.275
Good, A. G., and Beatty, P. H. (2011). Fertilizing nature: a tragedy of excess in the commons. PLoS Biol. 9:e1001124. doi: 10.1371/journal.pbio.1001124
Gouda, S., Kerry, R. G., Das, G., Paramithiotis, S., Shin, H.-S., and Patra, J. K. (2018). Revitalization of plant growth promoting rhizobacteria for sustainable development in agriculture. Microbiol. Res. 206, 131–140. doi: 10.1016/j.micres.2017.08.016
Gu, W., Miller, S., and Chiu, C. Y. (2019). Clinical metagenomic next-generation sequencing for pathogen detection. Annu. Rev. Pathol. 14, 319–338. doi: 10.1146/annurev-pathmechdis-012418-012751
Hao, X., and Chen, T. (2012). OTU analysis using metagenomic shotgun sequencing data. PLoS One 7:e49785. doi: 10.1371/journal.pone.0049785
Hayat, R., Ali, S., Amara, U., Khalid, R., and Ahmed, I. (2010). Soil beneficial bacteria and their role in plant growth promotion: a review. Ann. Microbiol. 60, 579–598. doi: 10.1007/s13213-010-0117-1
Hedo, E. B. (2013). Real Decreto 506/2013, de 28 de junio, sobre productos fertilizantes.BOE núm. 164, de 10 de julio de 2013. Actualidad Jurídica Ambiental, 55–56.
Hill, T. C., Walsh, K. A., Harris, J. A., and Moffett, B. F. (2003). Using ecological diversity measures with bacterial communities. FEMS Microbiol. Ecol. 43, 1–11. doi: 10.1111/j.1574-6941.2003.tb01040.x
Katsenios, N., Andreou, V., Sparangis, P., Djordjevic, N., Giannoglou, M., Chanioti, S., et al. (2022). Assessment of plant growth promoting bacteria strains on growth, yield and quality of sweet corn. Sci. Rep. 12:11598. doi: 10.1038/s41598-022-16044-2
Knežević, M., Berić, T., Buntić, A., Delić, D., Nikolić, I., Stanković, S., et al. (2021). Potential of root nodule nonrhizobial endophytic bacteria for growth promotion of Lotus corniculatus L. and Dactylis glomerata L. J. Appl. Microbiol. 131, 2929–2940. doi: 10.1111/jam.15152
Konopiński, M. K. (2020). Shannon diversity index: a call to replace the original Shannon’s formula with unbiased estimator in the population genetics studies. PeerJ 8:e9391. doi: 10.7717/peerj.9391
Kumar, A., and Verma, J. P. (2018). Does plant—microbe interaction confer stress tolerance in plants: a review? Microbiol. Res. 207, 41–52. doi: 10.1016/j.micres.2017.11.004
Li, R., Pang, Z., Zhou, Y., Fallah, N., Hu, C., Lin, W., et al. (2020). Metagenomic analysis exploring taxonomic and functional diversity of soil microbial communities in sugarcane fields applied with organic fertilizer. Bio. Med. Res. Int. 2020:9381506. doi: 10.1155/2020/9381506
Mann, H. B., and Whitney, D. R. (1947). On a test of whether one of two random variables is stochastically larger than the other. Ann. Math. Stat. 18, 50–60. doi: 10.1214/aoms/1177730491
Mikhailova, N. (2018). Fertilizer and an atomic balancing act to increase productivity and protect the environment. Organismo Internacional de Energía Atómica Boletin 59, 11–13.
Miller, R. R., Montoya, V., Gardy, J. L., Patrick, D. M., and Tang, P. (2013). Metagenomics for pathogen detection in public health. Genome Med. 5, 81–14. doi: 10.1186/gm485
Naeem, M., Aslam, Z., Khaliq, A., Ahmed, J. N., Nawaz, A., and Hussain, M. (2018). Plant growth promoting rhizobacteria reduce aphid population and enhance the productivity of bread wheat. Braz. J. Microbiol. 49, 9–14. doi: 10.1016/j.bjm.2017.10.005
Parray, J. A., Jan, S., Kamili, A. N., Qadri, R. A., Egamberdieva, D., and Ahmad, P. (2016). Current perspectives on plant growth-promoting rhizobacteria. J. Plant Growth Regul. 35, 877–902. doi: 10.1007/s00344-016-9583-4
Phibunwatthanawong, T., and Riddech, N. (2019). Liquid organic fertilizer production for growing vegetables under hydroponic condition. Int. J. Recycl. Org. Waste Agric. 8, 369–380. doi: 10.1007/s40093-019-0257-7
Pirttilä, A. M., Mohammad Parast Tabas, H., Baruah, N., and Koskimäki, J. J. (2021). Biofertilizers and biocontrol agents for agriculture: how to identify and develop new potent microbial strains and traits. Microorganisms 9:817. doi: 10.3390/microorganisms9040817
Robas, M., Jiménez, P. A., González, D., and Probanza, A. (2021). Bio-mercury remediation suitability index: a novel proposal that compiles the PGPR features of bacterial strains and its potential use in phytoremediation. Int. J. Environ. Res. Public Health 18:4213. doi: 10.3390/ijerph18084213
Robas Mora, M., Fernández Pastrana, V. M., Gutiérrez Oliva, L. L., Probanza Lobo, A., and Jiménez Gómez, P. A. (2022). Plant growth promotion of the forage plant Lupinus albus Var. Orden dorado using Pseudomonas agronomica sp. nov. and Bacillus pretiosus sp. nov. added over a valorized agricultural biowaste. Front. Microbiol.
Scialabba, N., and Hattam, C. (2002). Organic Agriculture, Environment and Food Security. Rome: Food & Agriculture Org.
Shannon, C. E. (2001). A mathematical theory of communication. ACM SIGMOBILE Mobile Comput. Commun. Rev. 5, 3–55. doi: 10.1145/584091.584093
Soler, P., Berroterán, J., Gil, J., and Acosta, R. (2012). Índice valor de importancia, diversidad y similaridad florística de especies leñosas en tres ecosistemas de los llanos centrales de Venezuela. Agronomía Trop. 62, 025–038.
Stewart, D., Reineke, K., Ulaszek, J., Fu, T., and Tortorello, M. (2001). Growth of Escherichia coli O157: H7 during sprouting of alfalfa seeds. Lett. Appl. Microbiol. 33, 95–99. doi: 10.1046/j.1472-765x.2001.00957.x
Suttner, B., Johnston, E. R., Orellana, L. H., Rodriguez-R, L. M., Hatt, J. K., Carychao, D., et al. (2020). Metagenomics as a public health risk assessment tool in a study of natural creek sediments influenced by agricultural and livestock runoff: potential and limitations. Appl. Environ. Microbiol. 86, e02525–e02519. doi: 10.1128/AEM.02525-19
Taş, N., de Jong, A. E., Li, Y., Trubl, G., Xue, Y., and Dove, N. C. (2021). Metagenomic tools in microbial ecology research. Curr. Opin. Biotechnol. 67, 184–191. doi: 10.1016/j.copbio.2021.01.019
V. DIRECTIVA (UE) (2008). 2015/720 DEL PARLAMENTO EUROPEO Y DEL CONSEJO de 29 de abril de 2015 por la que se modifica la Directiva 94/62/CE en lo que se refiere a la reducción del consumo de bolsas de plástico ligeras. El Dictamen del Comité Económico.
Walters, K. E., and Martiny, J. B. (2020). Alpha-, beta-, and gamma-diversity of bacteria varies across habitats. PLoS One 15:e0233872. doi: 10.1371/journal.pone.0233872
Wang, S., Wang, J., Zhou, Y., Huang, Y., and Tang, X. (2021). Prospecting the plant growth–promoting activities of endophytic bacteria Franconibacter sp. YSD YN2 isolated from Cyperus esculentus L. var. sativus leaves. Ann. Microbiol. 72:1. doi: 10.1186/s13213-021-01656-2
Ward, B. K., Dufault, R. J., Hassell, R., and Cutulle, M. A. (2018). Affinity of hyperammonia-producing bacteria to produce bioammonium/ammonia utilizing five organic nitrogen substrates for potential use as an organic liquid fertilizer. ACS Omega 3, 11817–11822. doi: 10.1021/acsomega.7b02083
Willis, A. D. (2019). Rarefaction, alpha diversity, and statistics. Front. Microbiol. 10:2407. doi: 10.3389/fmicb.2019.02407
Witzgall, K., Vidal, A., Schubert, D. I., Höschen, C., Schweizer, S. A., Buegger, F., et al. (2021). Particulate organic matter as a functional soil component for persistent soil organic carbon. Nat. Commun. 12, 1–10. doi: 10.1038/s41467-021-24192-8
Keywords: alpha diversity, bacterial phytopathogens, biofertilizer, metagenomics, PGPB, waste recovery, germination
Citation: Robas Mora M, Fernández Pastrana VM, Probanza Lobo A and Jiménez Gómez PA (2022) Valorization as a biofertilizer of an agricultural residue leachate: Metagenomic characterization and growth promotion test by PGPB in the forage plant Medicago sativa (alfalfa). Front. Microbiol. 13:1048154. doi: 10.3389/fmicb.2022.1048154
Edited by:
Shashi Kant Bhatia, Konkuk University, South KoreaReviewed by:
Bansh Narayan Singh, Banaras Hindu University, IndiaRohit Joshi, Institute of Himalayan Bioresource Technology (CSIR), India
Shuchi Singh, University of Illinois at Urbana-Champaign, United States
Copyright © 2022 Robas Mora, Fernández Pastrana, Probanza Lobo and Jiménez Gómez. This is an open-access article distributed under the terms of the Creative Commons Attribution License (CC BY). The use, distribution or reproduction in other forums is permitted, provided the original author(s) and the copyright owner(s) are credited and that the original publication in this journal is cited, in accordance with accepted academic practice. No use, distribution or reproduction is permitted which does not comply with these terms.
*Correspondence: Marina Robas Mora, bWFyaW5hLnJvYmFzbW9yYUBjZXUuZXM=; Vanesa M. Fernández Pastrana, dmFuZXNhLmZlcm5hbmRlenBhc3RyYW5hQGNldS5lcw==