- 1Soil Science Faculty, Lomonosov Moscow State University, Moscow, Russia
- 2Engelhardt Institute of Molecular Biology, Russian Academy of Sciences, Moscow, Russia
- 3Centre for Ecopesticides Research, LLC, Moscow, Russia
Earthworms play a vital role in the terrestrial ecosystem functioning and maintenance of soil fertility. However, many pesticides, for example, imidacloprid, benomyl, and metribuzin that are world-widely used in agriculture, may be potentially dangerous to earthworms. At the same time, standard tests for pesticides acute and chronic toxicity do not reflect all aspects of their negative impact and might not be enough sensitive for effective assessment. In this paper, we studied the effects of non-lethal concentrations of imidacloprid, benomyl, and metribuzin on the gut bacterial community of Lumbricus terrestris using high-throughput sequencing approach. We found that pesticides reduced the total bacterial diversity in the earthworm’s gut even at the recommended application rate. Under the applied pesticides, the structure of the gut prokaryotic community underwent changes in the relative abundance of the phyla Proteobacteria, Actinobacteria, Acidobacteria, Planctomyces, Verrucomicrobia, and Cyanobacteria, as well as the genera Haliangium, Gaiella, Paenisporosarcina, Oryzihumus, Candidatus Udaeobacter, and Aquisphaera. Moreover, the pesticides affected the abundance of Verminephrobacter—the earthworms’ nephridia specific symbionts. In general, the negative impact of pesticides on bacterial biodiversity was significant even under pesticides content, which was much lower than their acute and chronic toxicity values for the earthworms. These results highlighted the fact that the earthworm’s gut microbial community is highly sensitive to soil contamination with pesticides. Therefore, such examination should be considered in the pesticide risk assessment protocols.
Introduction
According to the Food and Agriculture Organization (FAO), more than 4 million tons of pesticides are used in the world annually;1 when released into the environment, most of them might pollute air, soil, ground and surface waters, as well as pose a threat to non-target organisms (Van der Werf, 1996; Carriger et al., 2006; Duke, 2017). Pesticides, even at concentrations not exceeding the Maximum Residue Levels (MRLs), lead to a decrease in biodiversity (Hole et al., 2005; Isenring, 2010; Beketov et al., 2013), in particular, soil microbial biodiversity (Lo, 2010; Puglisi, 2012; Fenner et al., 2013; Astaykina et al., 2020). Moreover, pesticides can change the activity of soil microorganisms. For instance, the fungicide chlorothalonil and insecticide chlorpyrifos have been inhibited dehydrogenase, catalase, urease and acid phosphatase activities (Jastrzȩbska, 2011; Baćmaga et al., 2018).
Earthworms as important representatives of soil invertebrates are often called ‘ecosystem engineers’ because their activity modifies physicochemical and biological properties of the habitat (Jones et al., 1994). Depending on the ecological-trophic group, earthworms can contribute to additional migration of pollutants in the soil (Kuzyakov and Blagodatskaya, 2015). For example, Lumbricus terrestris which belongs to the anecic earthworms (Bouché, 1977; Lavelle et al., 1989) can transport pesticides from the surface to the mineral horizons. L. terrestris, unlike the well-studied compost worm Eisenia fetida, is widespread in the arable horizons of most world soils. Furthermore, L. terrestris appeared to be a more sensitive species than E. fetida in the pesticide toxicity tests (Pelosi et al., 2013). Moreover, due to the structural features, such as the greater length of the intestinal tract, digestion in L. terrestris takes up to 6 h (Nechitaylo et al., 2010), consequently, when pesticides enter the digestive tract of L. terrestris, they are able to have long-term effects on their gut bacteria.
There are plenty of studies devoted to the analysis of the taxonomic composition of the earthworm’s gut microbial community (Horn et al., 2003; Wüst et al., 2011; Sapkota et al., 2020), which usually differ from bacteria isolated from soils or composts (Byzov et al., 2009). The diversity and structure of bacteria in the earthworms’ gut can vary depending on the ecological-trophic group of lumbricid (Egert et al., 2004). However, the study of the pesticide impact on the microbial biodiversity in the intestinal tracts of soil invertebrates remains practically out of sight of researchers, although this locus is generally recognized as a hotspot of microbial activity. For example, it was discovered that the insecticide fipronil may inhibit the growth of Eudrilus eugeniae gut bacteria plated on a sterile nutrient agar media (Salokhe and Deshapande, 2014). Besides, Kavitha et al. (2019) noted that organophosphate insecticide monocrotophos led to a reduction of the bacterial and fungal biodiversity and abundance in the earthworm Lampito mauritii gut. In addition, the herbicide glyphosate affected decrease of the microbial α-diversity in the earthworms Alma millsoni, Eudrilus eugeniae and Libyodrilus violaceus gut, while the relative abundance of Enterobacter spp. DHL-02, Pseudomonas putida, Pantoea agglomerans and Pseudomonas taiwanensis increased (Owagboriaye et al., 2021). Based on the results of molecular genetic analysis using high-throughput sequencing of the 16S RNA gene, it was found that the herbicide fomesafen might reduce bacterial diversity and shift the bacterial community structure of Pheretima guillelmi gut (Chang et al., 2021). Nonetheless, there are no studies which examine the effects of pesticides on the gut microbiota of L. terrestris, one of the most common earthworm species. Undoubtedly, this kind of research is important not only from the ecotoxicology perspective, but also for isolating bacteria which degrade pesticides (Sun et al., 2020).
Thus, based on the previous studies, we hypothesized that (1) pesticides might impact on the earthworm L. terrestris indirectly, through changing the gut microbial community taxonomic structure; (2) pesticides even at the recommended application rate may reduce the earthworm’s gut microbial diversity; (3) the gut microbial community relatively quickly response to the pesticide application; (4) bacteria whose relative abundance increases under the impact of pesticides, can be recommended for further research in order to isolate strains that degrade pesticides. To test these hypotheses, we set up a laboratory incubation experiment to study the relationship between pesticide-contaminated soil and earthworm gut microbiota. The cutting-edge approach of total DNA metabarcoding allowed us to assess the taxonomic diversity of gut-associated bacteria without isolation and cultivation of microorganisms which guarantees the novelty of the obtained results.
Materials and Methods
Soil and Earthworms Collection
For the laboratory experiment soil samples from the surface (0–10 cm) horizon of Umbric Albeluvisols (IUSS Working Group WRB, 2015) were taken in the Odintsovo district of the Moscow region (55°41′ N, 38°05′ E). The experimental site has been fallowed and has not been treated with pesticides and fertilizers over the past 5 years. Five separate soil samples were randomly selected (average distance between sampling points was 2 m) using a shovel. Large plant residues, live rhizomes, and roots were directly removed during collection. In the laboratory, the soil samples were dried at room temperature, sieved (<1 mm) and homogenized. The main properties of the soil are presented in Supplementary Table S1.
Mature specimens of the earthworm L. terrestris (Linnaeus, 1758) were collected on the same soil site. Earthworms were maintained at a constant temperature of +17 ± 1°C in the dark for 2 weeks before the start of the experiment in soil containing fresh litter of Acer platanoides as a food source.
Pesticides
For the experimental treatment we chose three pesticide formulations that were produced by «Avgust» Inc. (Russia): 700 g/kg metribuzin (herbicide), 200 g/L imidacloprid (insecticide), and 500 g/kg benomyl (fungicide). All actives were > 98.0% pure and met international standards Imidacloprid {4,5-dihydro-N-nitro-1-[(6-chloro-3-pyridyl)-methyl]-imidazolidin-2-ylene-amine} is a highly effective worldwide used neonicotinoid insecticide (Tišler et al., 2009), which is very stable in soil (DT50 > 120 days; PPDB: Pesticide Properties DataBase, 2016) and may have long-term effects on non-target species. Metribuzin [4-amino-6-tert-butyl-3-methylthio-1,2,4-triazin-5(4H)-one] is a triazinone herbicide, which has been widely used in agriculture for several decades. Benomyl [N-[1-(butylcarbomoyl)-benzoimidazolyl-2]-0-methylcarbamate] is a systemic benzimidazole fungicide used to control fungal diseases in agriculture, forestry and veterinary. Benomyl, as well as its main metabolite carbendazim are pesticides stable for degradation in soil (DT50 = 61–120 days; PPDB: Pesticide Properties DataBase, 2016). The main properties of these pesticides are provided in Supplementary Table 2S. Imidacloprid, benomyl and metribuzin can be used together for the comprehensive protection of cereals, sugar beets and potatoes. Application of these pesticides includes both seed dressing and soil surface spraying before sowing the seeds so that virtually all the applied pesticide dose enters the soil and is not intercepted by the crop.
Experimental Design
The laboratory incubation experiment was carried out in four replicates at a constant temperature (+17 ± 1°C) in glass flasks covered with a perforated film to prevent water loss. The mass of moist soil (60% WHC) in each vessel was 500 g. The soil moisture was controlled by the weight method every 2 days.
The pesticides were applied according to the manufacturer’s recommended application rates: 0.98 kg/ha metribuzin, 0.02 kg/ha imidacloprid, and 1.5 kg/ha benomyl. We also tested 2-fold and 10-fold application rates that simulated the worst-case scenarios. As was previously mentioned, imidacloprid and benomyl are stable pesticides, therefore, there are likely to accumulate in soil after many years of application on the same site. For a more thorough distribution of pesticides in the soil sample, initially we mixed the pesticide with 20 g of clear sand, and then the already treated sand was thoroughly mixed with the soil aliquot (500 g). In the control, the soil sample was mixed with untreated sand. In total, four variants of the experiment were carried out: a control and a mixture of three pesticides at the manufacturer’s recommended application rates as well as the 2-fold and 10-fold application rates. In each vessel, we placed three specimens of L. terrestris (Figure 1). According to the OECD Guidelines for the Testing of Chemicals (OECD, 1984) the duration of the experiment was 14 days. To analyze the intestinal prokaryotic community, the worms were collected after 7 and 14 days, respectively.
Isolation and Cleaning of Earthworms’ Guts
Before isolating the intestinal tract, the earthworms were washed and kept on wet filter paper for 24 h at a temperature of 6°C–7°C. The earthworms were anesthetized with hot water (100°С) for 3 s and then washed with 70% ethanol. To isolate guts, the worms were frozen on a freezer table (Peltier element) to −16°C and were dissected with a sterile scalpel immediately after defrosting, avoiding repeated freezing–thawing (Byzov et al., 2015). For a molecular genetic analysis, a section of the digestive tract was taken from the clitellum to the anus (Figure 2). The guts cut out in this way were placed in Eppendorf tubes containing 1 ml of sterile Milli-Q quality water and were centrifuged for 10 min at 10,000 g. The supernatant was removed and the obtained isolates of microorganisms were stored at −80°C until the subsequent isolation of total DNA.
Isolation of Total DNA and DNA Metabarcoding
The extraction of total DNA from a 0.5 mg sample of the earthworm’s intestines was performed using the FastDNA SPIN Kit for Soil (MP Biomedicals, United States) according to the manufacturer’s protocol. The isolated DNA extracts were stored at −20°C.
The samples of the isolated DNA were 500-fold diluted. Amplification of the V4 variable region of the 16S rRNA gene was carried out in one round using forward and reverse primers 515F (5′-GTGCCAGCMGCCGCGGTAA-3′) and 806R (5′-GGACTACHVGGGTWTCTAAT-3′) with two-index multiplexing of the samples (Fadrosh et al., 2014). These primers are specific both to bacteria and to archaea. The PCR products were purified using the Cleanup Mini kit (Evrogen, Russia) for DNA isolation from reaction mixtures. The concentration of the obtained 16S rRNA libraries in the solution was measured on a Qubit fluorometer (Invitrogen, United states) using the Quant-iT dsDNA High-Sensitivity Assay Kit. The purified amplicons were mixed equimolarly depending on the concentration. The quality of the library prepared for sequencing was assessed by agarose gel electrophoresis. Further, sample preparation and sequencing of the pooled sample was carried out using the MiSeq Reagent Kit v2 (500 cycles) and an MiSeq sequencer (Illumina, United States).
Bioinformatics and Statistical Analyses
Bioinformatics analysis was performed as described in the work by Fadrosh et al. (2014). We used the DADA2 package for R, including error correction, inferring ribosomal sequencing variants (RSVs) separately for forward and reverse reads, elimination of chimeric RSVs and, finally, merging forward and reverse RSVs. The obtained average RSV length was about 253 bp (with minimal variability) for bacterial 16S V4 fragments. In contrast to OTUs (operation taxonomic units), the analysis based on RSVs which are also referred to as amplicon sequence variants (ASVs) or exact sequence variants (ESVs) does not imply merging of closely related amplicon variants (<3% differences) into a single sequence (i.e., OTU) and therefore can identify single-nucleotide differences between species (Callahan et al., 2017).
For taxonomic annotation of the obtained RSV sequences, we also used the DADA2 package supplied with the Silva database (version 138) for bacterial communities. RSVs annotated as chloroplasts, mitochondria, Cercozoa, etc. were removed. The resulting read count data were normalized between the samples using the read count annotated at the domain (kingdom) level.
The rest of the analysis was also conducted in the R environment (version 3.6.3). To assess α-diversity, we calculated the Shannon, Chao1 and ACE indices using the fossil 0.4.0 and vegan 2.5–6 packages. When calculating these indices, the read count data were rarefied to match the sample with the minimum number of reads. For the beta diversity analysis, we used Bray-Curtis metric. The rest of the analyses and visualization were performed using the phyloseq 1.30.0, plotly 4.9.2.2, phytools 0.7–47, pheatmap 1.0.12, and ggplot2 3.3.3 packages. To visualize differences between the samples, we applied the metrical and non-metrical multidimensional scaling (MDS). The association analysis between time periods and taxon abundance was performed using the Spearman and Pearson correlations. For non-paired comparison of the two groups, we used the multiple t-tests as well as the Mann–Whitney and Pearson tests, and for paired comparisons, we used the Wilcoxon test. The FDR was calculated using the Benjamini–Hochberg adjustment for the obtained value of ps. All analyses were carried out in the R environment. For all types of the statistical analysis, the difference was considered significant at a significance level of value of p < 0.05.
Results
Structure and Composition of Microbial Community of Lumbricus terrestris Gut With or Without Pesticide Impact
The study was based on 1,287,366 high-quality reads of 16S rRNA gene amplicons. In total, 208 bacterial genera from 45 classes that belong to 21 prokaryotic phyla were identified in the gut of L. terrestris.
Proteobacteria (50.3%), Actinobacteria (19.7%), and Firmicutes (17.6%) were found to be dominants of the microbial community. The other phyla were represented to a much lesser extent: Verrucomicrobia, Chloroflexi, Planctomycea up to 4%, Myxococcota, Crenarchaeota, and Acidobacteria up to 1% of all the obtained sequences (Figure 3). At the class level, gram-negative bacteria Gammaproteobacteria and gram-positive Bacilli and Actinobacteria dominated (>10% of all obtained sequences) in the prokaryotic community (Figure 3). At the genus level, Klebsiella, Acinetobacter and Verminephrobacter represented up to 31% of all assigned nucleotide sequences in the earthworm’s gut (Figure 3).
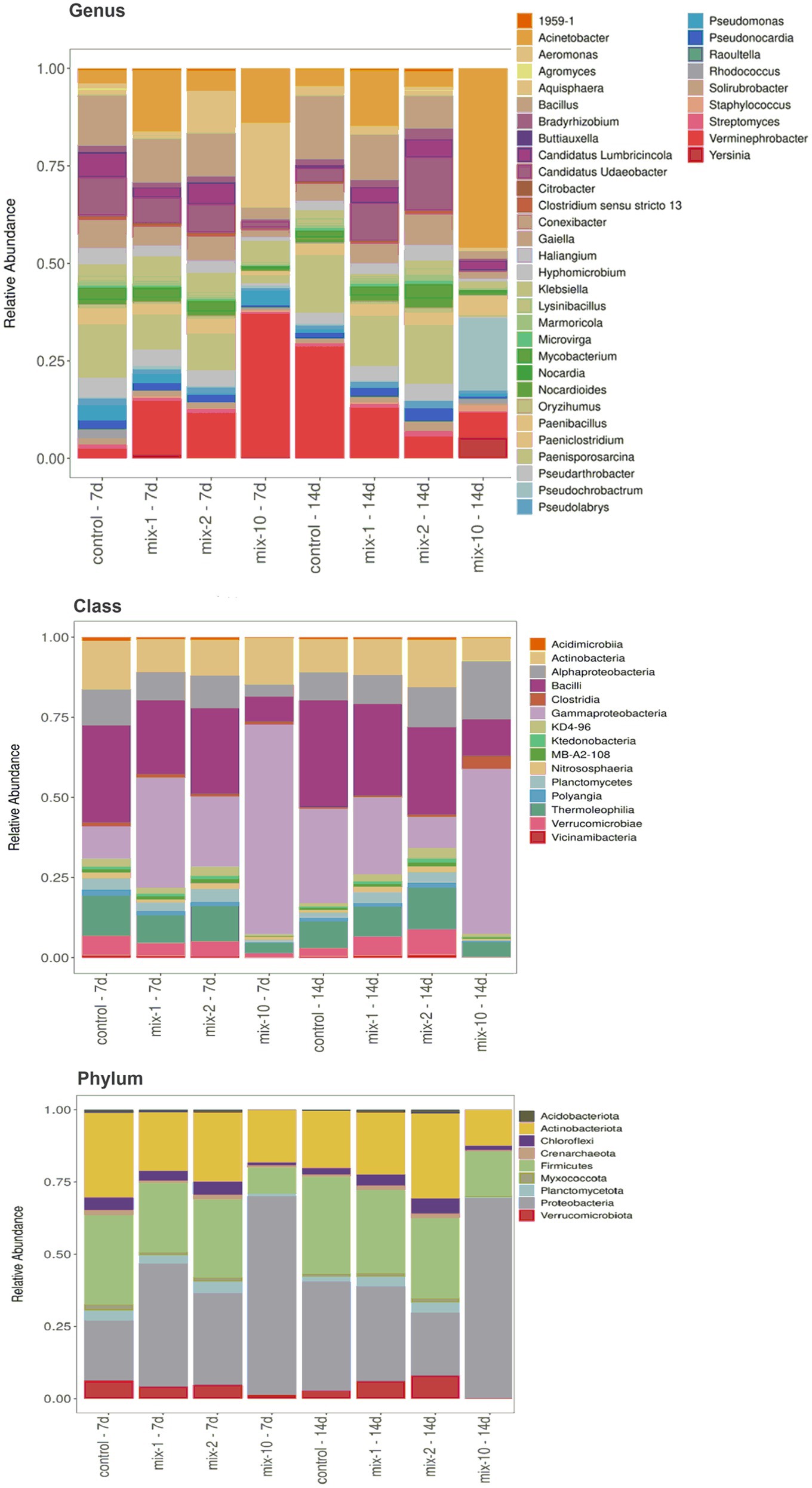
Figure 3. The structure of the prokaryotic community of the intestinal tract of L. terrestris at the levels of phyla, classes and genera (N = 2). Legend: mix indicates pesticides in a mixture; 1/2/10 is the recommended, 2-fold and 10-fold pesticide application rates; 7 day/14 day is the incubation time. The relative abundance is shown for taxa with read counts >0.3%.
Compared to the control, the structure of the prokaryotic community of the gut treated with pesticides changed as follows: (1) on day 7 of incubation, the relative abundance of the phylum Proteobacteria increased and the relative abundance of Actinobacteria decreased; (2) on day 14 of incubation, the relative abundance of the phyla Acidobacteria, Planctomyces, Verrucomicrobia, and Cyanobacteria increased (p < 0.05, Supplementary Table 3S). At the class level, on day 7 of incubation with pesticides, the relative abundance of gram-negative bacteria Gammaproteobacteria increased, while the relative abundance of Chloroflexia, Delta Proteobacteria and Anaerolineae decreased (p < 0.05, Supplementary Table 4S). After a 14-day incubation with pesticides, the relative abundance of the classes Ktedonobacteria, Planctomycetes, Acidobacteriia, Verrucomicrobiae and Cyanobacteriia increased (p < 0.05, Supplementary Table 4S). At the genus level, on day 7 of incubation with pesticides, the relative abundance of Haliangium, Gaiella, Paenisporosarcina, and Oryzihumus in L. terrestris gut decreased, while that of Verminephrobacter increased 3-fold (p < 0.05, Supplementary Table 5S). On day 14 of incubation of earthworms with pesticides, the relative abundance of Candidatus Udaeobacter and Aquisphaera in L. terrestris gut increased, while that of Verminephrobacter decreased 2-fold (p < 0.05, Supplementary Table 5S).
Pesticide-Sensitive Bacteria of Lumbricus terrestris Gut
The earthworm’s gut associated bacteria that considerably changed in relative abundance under pesticide exposure are shown in Figure 4. In contrast to the control variants, as well as to the variants treated with pesticides at the recommended and 2-fold application rate, in the variants treated with pesticides at a 10-fold application rate, the relative abundance of the genera Bacillus, Candidatus Lumbricincola, Nocardioides, Lysinibacillus, Paenisporosarcina, Hyphomicrobium, Pseudolabrys, Aquisphaera, Paenibacillus, Pseudarthrobacter, Bradyrhizobium, Candidatus Udaeobacter, Gaiella, Pseudonocardia, Solirubrobacter, Conexibacter, and Mycobacterium in L. terrestris gut decreased both after 7 and 14 days of the incubation experiment (Figure 4). Conversely, in the variants treated with pesticides at a 10-fold application rate, the relative abundance of Acinetobacter, Yersinia, Paeniclostridium, and Pseudochrobactrum in the earthworm’s guts increased on day 14.
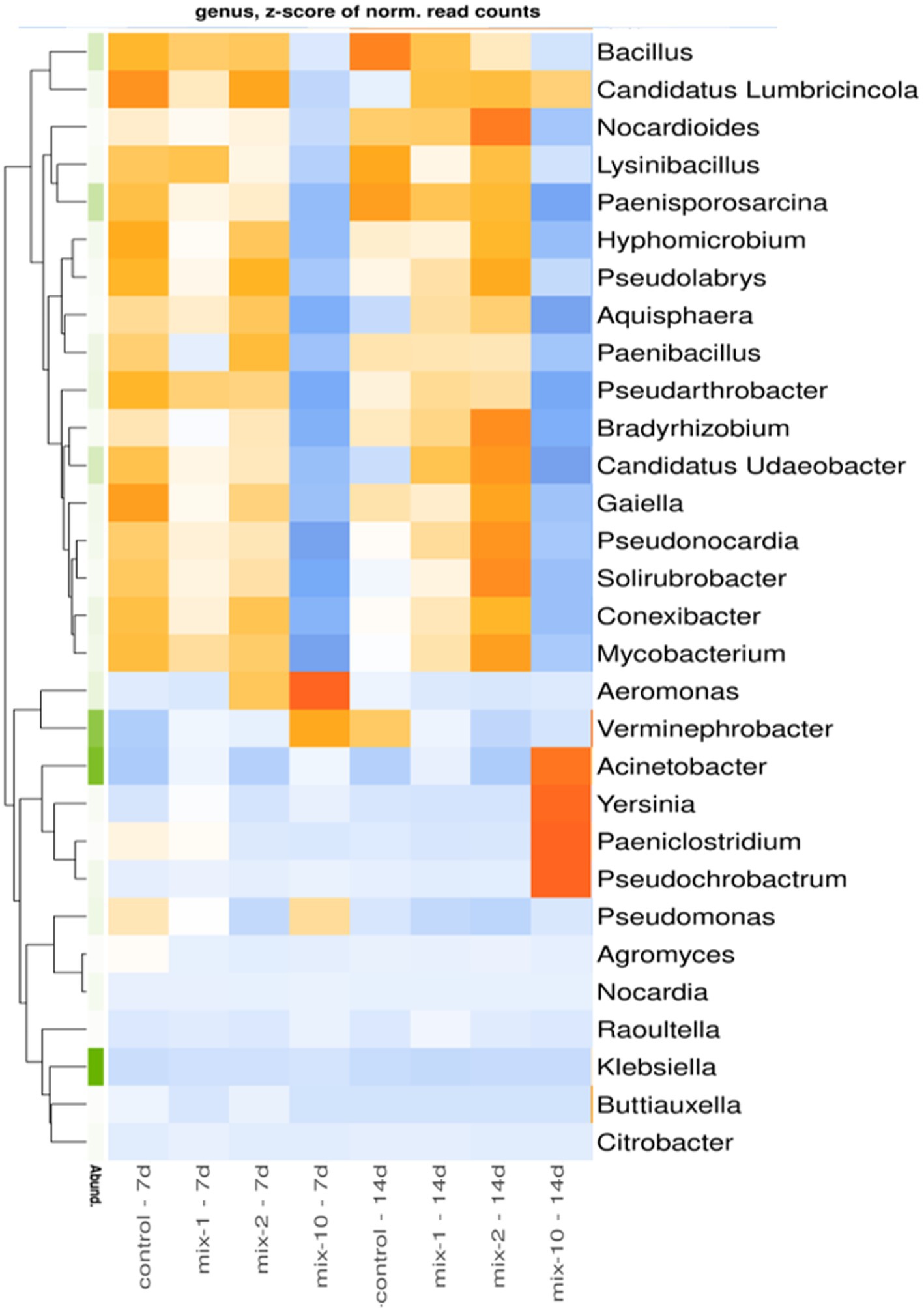
Figure 4. Heatmap for relative abundance of top 30 microbial taxa in prokaryotic communities of the earthworm’s guts at the genus level (N = 2). The data are presented as z-scores. Microbial genera with positive z-scores are marked in orange, genera with negative z-scores in blue. Legend: mix indicates pesticides in a mixture; 1/2/10 is the recommended, 2-fold and 10-fold pesticide application rates; 7 day/14 day is the incubation time.
Gut Bacteria α-Diversity
Pesticides concentration affected the gut microbial α-diversity (Figure 5). For instance, the Shannon biodiversity index decreased from 3.6 in the control to 2.2 (p < 0.05) in the variants with a 10-fold application rate (Figure 5). The maximum values of the Chao1 and ACE biodiversity indexes were in the control variants, but decreased (p < 0.05) in the variants with a 10-fold application rate of pesticides (the data are provided in Supplementary Table 6S).
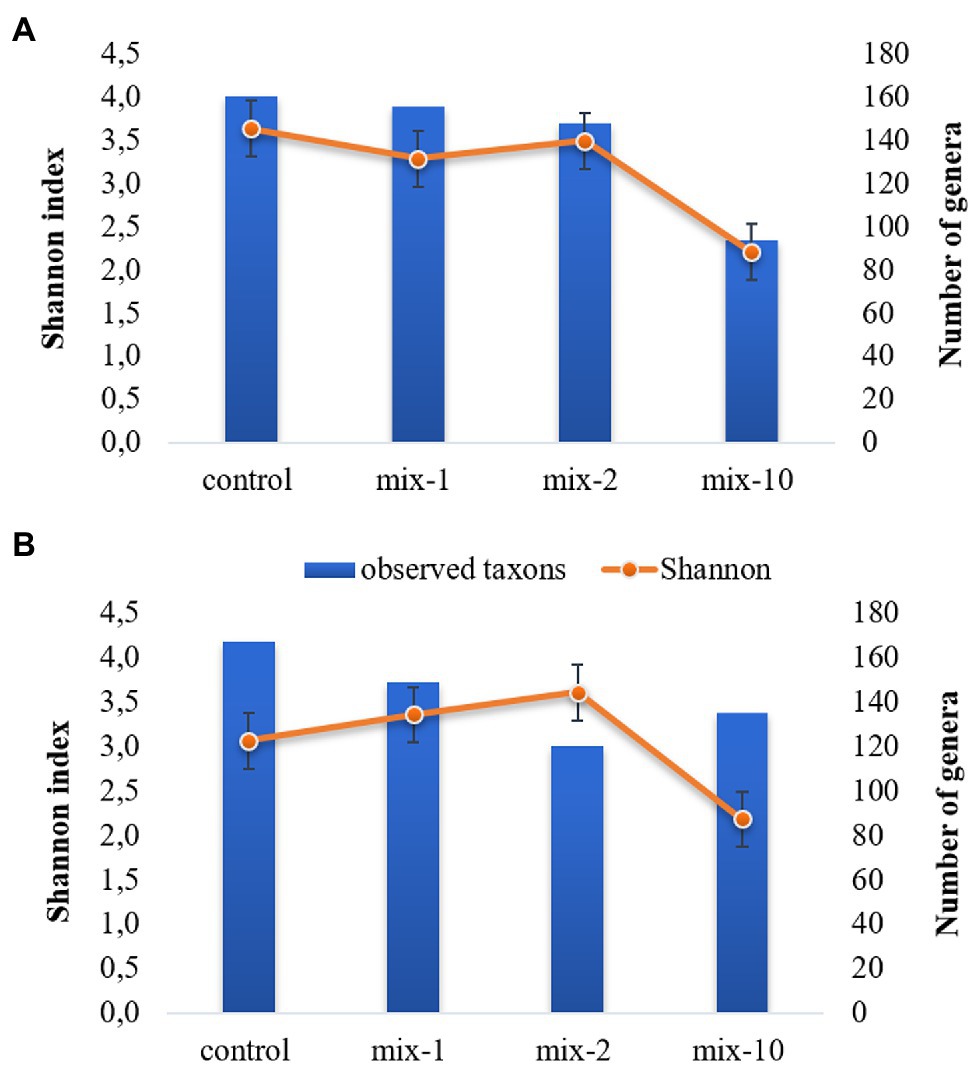
Figure 5. Dynamics of Shannon index and number of genera on day 7 (A) and day 14 (B) of incubation (mean ± SE). 1/2/10 is the recommended, 2-fold and 10-fold pesticide application rates.
The number of identified genera decreased from 161 to 94 on day 7 after the pesticides application. However, on day 14 of the experiment, the number of identified genera increased in the variants with a 10-fold application rate compared to the variants with a 2-fold application rate. At the same time, it was lower than in the control (Figure 5).
Gut Bacteria β-Diversity
Based on the Bray-Curtis metrics and PERMANOVA tests, all samples were divided into two distinct clusters: (1) control variants, samples with the recommended and 2-fold application rates; (2) samples with a 10-fold application rate of pesticides (Figure 6).
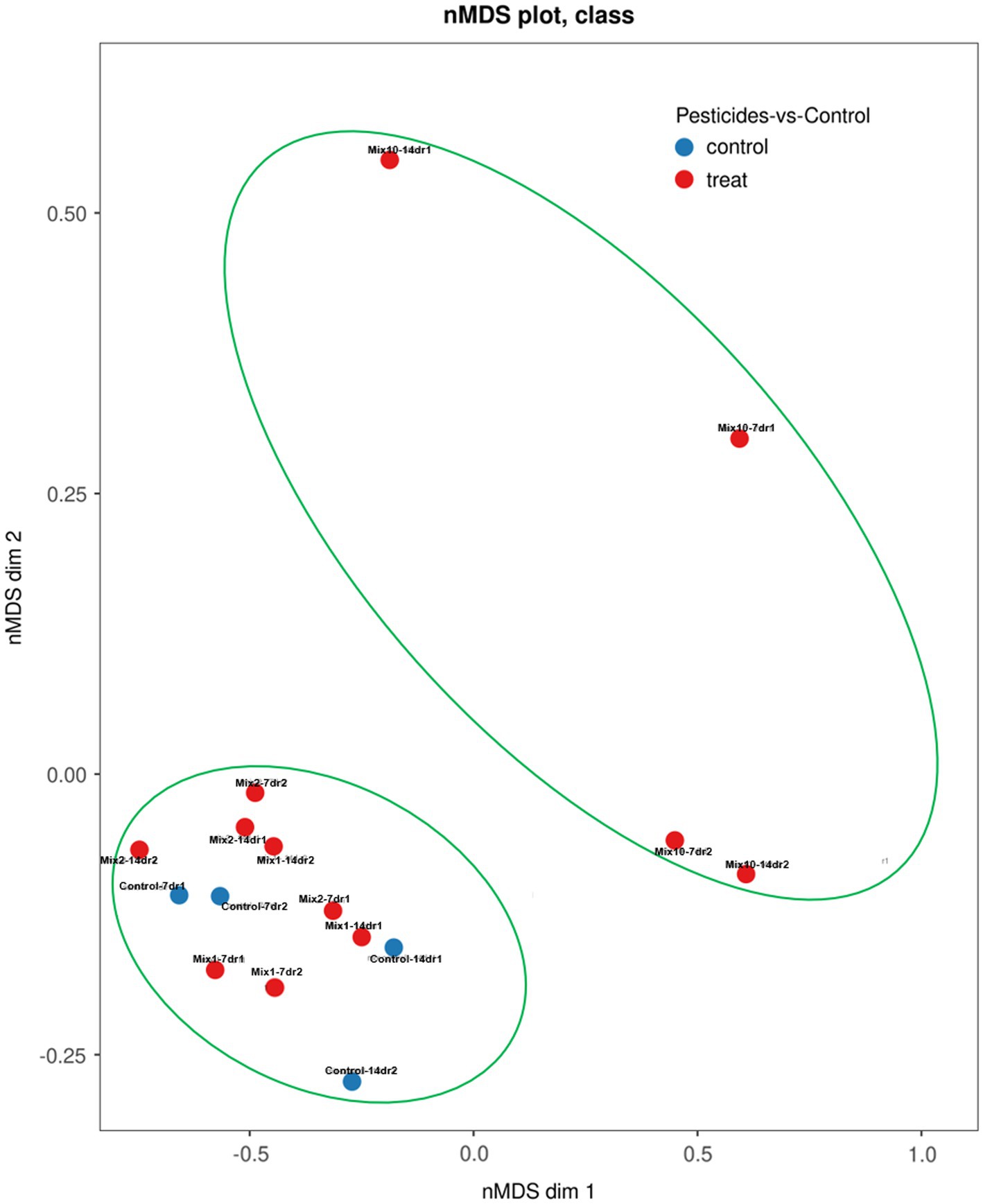
Figure 6. Non-metric multidimensional scaling plot of the assembly patterns of prokaryotic communities of the earthworm’s gut using the Bray–Curtis (BC) distance matrix at the genus level.
In general, the dissimilarities in the composition of the gut bacterial communities between the samples were high. Both after 7 and 14 days of the incubation experiment, the samples with the highest concentration of pesticides (a 10-fold application rate) tended to position together in the upper right corner of the plot, in contrast to the other variants.
Discussion
Differences in Bacterial Communities of Lumbricus terrestris Gut and Soil
Our previous studies (Astaykina et al., 2020; Streletskii et al., 2022) were devoted to microbial biodiversity of Umbric Albiluvisols under pesticides treatment. We found that the pesticide exposure led to a reduction in the relative abundance of bacterial phyla Myxococcota, Bacteroidetes, Gemmatimonadetes, Proteobacteria. At the genus level pesticides increased the relative abundance of Kitasatospora and Streptomyces which could be explained by the involvement of these bacteria in the degradation of pesticides. Our current data has shown that Proteobacteria and Actinobacteria were dominant in the bacteria communities both in the earthworm’s gut and in the surrounding soil. However, in contrast to the soil, Firmicutes was also the dominant phylum in the gut. This data corresponds with previous research, where Proteobacteria, Actinobacteria, Firmucutes, Bacteroidetes, and Verrucomicrobia have been shown to be the dominant phyla in L. terrestris gut (Knapp et al., 2009; Pass et al., 2015; Ma et al., 2017; Meier et al., 2021). The digestive tract of an earthworm is a microaerophilic and even anaerobic zone (Byzov et al., 2015); hence, phyla Firmicutes and Actinobacteria, which are facultative and/or obligate anaerobes, dominate in the prokaryotic community of the gut (Chang et al., 2021). On the other hand, Chloroflexi, the bacteria phylum which may be among dominants in E. fetida gut (Liu et al., 2018), was not a dominant in L. terrestris gut due to the differences in burrowing and feeding habits of worms.
Pesticides Alter the Structure of the Gut Bacteria Community
Pesticides effect on earthworms might be more complex than described by standard indicators such as LC50, NOEC, body mass changes and behavioral disorders (Pelosi et al., 2014). For instance, some effects on the molecular and cellular levels (e.g., oxidative stress, DNA damage, teratogenesis) have been recently detected (Datta et al., 2016). The fungicide benomyl may cause the disruption of cell’s microtubules (Hess and Nakai, 2000) and affect the development of E. fetida spermatozoa (Sorour and Larink, 2001). The insecticide imidacloprid can lead to a significant reduction in E. fetida fecundity, as well as to a damage of the epidermal and midgut cells of the earthworms (Wang et al., 2015). The fungicide benomyl, insecticide imidacloprid and herbicide metribuzin, separately or combined, have led to changes in the diversity and structure of the soil microbiota (Astaykina et al., 2020). However, there is no data regarding the effect of these pesticides on the earthworm gut microbiota. Our results showed that these pesticides increased the relative abundance of the phyla Proteobacteria, Acidobacteria, Planctomyces, Verrucomicrobia, and Cyanobacteria in the structure of their gut prokaryotic community, while Actinobacteria decreased. A reduction in this phylum indicated the decrease in the ability to produce enzymes necessary for the cellulose, hemicellulose and other natural polymers decomposition (Vieites et al., 2010).
Previously, Chang et al. (2021) also found that the herbicide fomesafen at the recommended application rate caused significant differences in the relative abundance of Actinobacteria, Firmicutes, and Proteobacteria. Furthermore, it was found that the increased abundance of Proteobacteria can be considered as a potential marker of imbalance in the gut microbiota of many earthworm species (Shin et al., 2015; Wang et al., 2022).
At the genus level, pesticides increased the relative abundance of gram-negative Verminephrobacter (the phylum Proteobacteria), while the relative abundance of Haliangium (myxobacteria from the phylum Proteobacteria), Gaiella and Oryzihumus (gram-positive Actinobacteria), Paenisporosarcina (the phylum Firmicutes) decreased after 7 days of pesticide exposure. Conversely, after 14 days of incubation the relative abundance of Verminephrobacter decreased, while the relative abundance of gram-negative Candidatus Udaeobacter (the phylum Verrucomicrobia) and Aquisphaera (the phylum Planctomyces) increased. The genus Verminephrobacter is the extracellular species-specific bacterial symbionts of Lumbricid earthworms inhabiting their nephridia, i.e., kidney-like osmoregulatory organs (Dulla et al., 2012; Lund et al., 2014). Nephridia are located next to the intestinal tract and may enter the sample during resection. Based on the results of molecular genetic analysis, bacteria of the genus Verminephrobacter were found to be more sensitive to the presence of pesticides in the soil than bacteria of the gut. Apparently, the pesticides application may increase the earthworms’ excretory organs activity, consequently, the excretion rate of pesticide decomposition products may increase (Wells and Laverack, 1963). Verminephrobacter are likely to provide enzymes or other essential co-factors for these biochemical reactions (Lund et al., 2014). However, after 14 days of the experiment, the relative abundance of Verminephrobacter in the prokaryotic community of lumbricid significantly decreased. This can be explained by two factors: (1) the earthworms adapted to the presence of pesticides in the soil; (2) their metabolic capacity decreased after pesticide exposure, which can be considered as an indicator of pesticide toxicity.
The verrucomicrobial genus Candidatus Udaeobacter was also sensitive to pesticides in the soil. Our results showed that the pesticides application increased the relative abundance of this genus. According to the previously published research, Candidatus Udaeobacter are the most dominant uncultured soil bacteria that can oxidize the trace gas H2 to generate energy and utilize nutrients due to antibiotic-driven lysis of other soil microbes (Willms et al., 2020). It is possible that pesticide treatment restructured the intestinal complex and amplified the antibiotic activity of Candidatus Udaeobacter. The functional role of these bacteria in the earthworm’s gut remains to be determined.
The dominant gut bacterial genera which are sensitive to pesticide treatment play an important role in organic matter decomposition and nutrient cycling both in earthworms’ guts and soils (Byzov et al., 2015). For instance, Conexibacter is involved in the nitrification as well as Pseudarthrobacter is denitrifiers (Su et al., 2019), and Lysinibacillus is nitrogen fixers. Therefore, a change in these bacteria abundance can affect the nitrogen cycle (Jien et al., 2021). Moreover, Lysinibacillus sphaericus can be used both in soil amendment in the replantation processes (Aguirre-Monroy et al., 2019) and as degraders of pesticides, in particular glyphosate (Pérez Rodríguez et al., 2019). Some Nocardioides species can degrade complex organic pollutants, so reducing their presence may affect the rate of natural remediation (Zhao et al., 2018).
Effects of Pesticides Application on the Earthworm Gut Bacterial Diversity
It was found that pesticides both at the recommended application rate and at the rate increased by 10 times decreased the values of α-biodiversity indices. It is similar with the effect of other xenobiotics, such as heavy metals, microplastics, antibiotics (triclosan), pesticides (fomesafen), which significantly reduced the bacterial biodiversity in the digestive tracts of soil invertebrates (Ma et al., 2017; Chen et al., 2020; Sun et al., 2020; Chang et al., 2021). Biodiversity reduction and changing of dominants in the bacterial community of the digestive tract led to a decrease in the biochemical activity of the intestinal contents and, therefore, affected the ability of the earthworm to assimilate the substrate. The pesticides may also alter the feeding behavior, leading to changes in the composition of intestinal microbiota (Zhu et al., 2018).
The β-diversity assessment showed that bacterial complexes of L. terrestris gut cluster according to the application rate of pesticides. The variants with a 10-fold pesticide application rate formed a separate cluster both after 7 and 14 days of incubation. Comparison of pesticide concentrations in the variants with a 10-fold application rate with the values of acute and chronic toxicity of these pesticides for earthworms showed that even when the recommended application rate is 10-fold increased, the final concentration of the pesticide is more than 100 times lower than the values of acute and chronic toxicity of the same pesticide (0.008 mg/kg metribuzin versus LC50 = 427 mg/kg and NOEC >52.3 mg/kg; 0.0002 mg/kg imidacloprid versus LC50 = 10.7 mg/kg and NOEC = 0.178 mg/kg; 0.01 mg/kg benomyl versus LC50 = 5.4 mg/kg and NOEC = 1.0 mg/kg; Supplementary Table S2). Thus, the microbial community of the intestinal tract is highly sensitive to soil pollutants (Chang et al., 2021), hence, this type of research should be included in the practice of assessing the risks of pesticide application for non-target organisms.
Pesticide-Degrading Bacteria
Numerous studies have demonstrated the crucial role of bacteria inhabiting the earthworm’s guts in the transformation of organic pollutants in the environment (Sun et al., 2020). It is known that there are different ways to transform organic pollutants in the earthworm’s gut such as the activity of transit bacteria, their free enzymes and intestinal symbionts (Byzov et al., 2015). It has been established that Rhodococcus and Bacillus bacteria from the earthworm’s intestine can degrade pesticides (Kuipa et al., 2016). In this study, we showed that pesticide treatment increases the relative abundance of Proteobacteria phylum and at the genus level, increases the relative abundance of Acinetobacter, Pseudochrobactrum and bacterial symbionts Verminephrobacter.
Acinetobacter and Pseudochrobactrum are active microbiodegraders which can degrade many xenobiotics, including pesticides (Pawar and Mali, 2014; Kafilzadeh et al., 2015; Doolotkeldieva et al., 2018; Zhan et al., 2018). The discovered fact allows us to assume that bacteria of Proteobacteria phylum which possess high hydrolytic activity can be considered as pesticide-degrading bacteria. Therefore, further studies are required to assess the physiological and biochemical potential of Proteobacteria in the digestive tract of earthworms. For instance, it would be of great interest to design primers corresponding to carboxylesterases of bioscavengers involved in pesticide detoxification (Sanchez-Hernandez et al., 2009). It is also important to determine the role of Verminephrobacter, as a nephridium symbiont, in the mechanisms of pesticide detoxification.
Conclusion
In summary, based on the metagenomic analysis, pesticide-sensitive taxa, such as Verminephrobacter, Acinetobacter, Candidatus Udaeobacter, Pseudochrobactrum were identified in the Lumbricus terrestris gut. Depending on the duration of incubation, the reaction of the gut bacteria community on the presence of pesticides in the soil was different. Our results indicated that after 7 days of pesticide exposure the relative abundance of gram-negative Verminephrobacter increased, while the relative abundance of Haliangium, Gaiella and Oryzihumus, Paenisporosarcina decreased. On the contrary, after 14 days of incubation the relative abundance of Verminephrobacter decreased, while the relative abundance of gram-negative Candidatus Udaeobacter and Aquisphaera increased. It is possible that the gut microbiota adapted, and taxa that were initially subjected to toxic influence soon restored their abundance. We have discovered that pesticides can have a significant effect on the composition of the earthworms gut bacterial community at concentrations that were many times less than their toxicity to earthworms. Therefore, standard methods for assessing risks of pesticides application do not have enough sensitivity and the NGS methods might be recommended for a better understanding of possible changes in the soil environment under pesticides application.
Data Availability Statement
The datasets presented in this study can be found in online repositories. The names of the repository/repositories and accession number(s) can be found at: NCBI BioProject—PRJNA797445.
Author Contributions
AA, RS, and MM: conceptualization. RS and VG: methodology, resources, and funding acquisition. AA: software, formal analysis, data curation, writing—review and editing, visualization, and project administration. AA and GK: validation. AA and RS: investigation. MM and GK: writing—original draft preparation. MM and VG: supervision. All authors contributed to the article and approved the submitted version.
Funding
The DNA sequencing was funded by a grant from the President of the Russian Federation (project МК-92.2021.1.5). The bioinformatic analysis was supported by the Russian Foundation for Basic Research (project 18-29-25027). The conceptualization was supported by the MSU Eurasian Center for Food Security.
Conflict of Interest
VG was employed by the company Centre for Ecopesticides Research, LLC.
The remaining authors declare that the research was conducted in the absence of any commercial or financial relationships that could be construed as a potential conflict of interest.
Publisher’s Note
All claims expressed in this article are solely those of the authors and do not necessarily represent those of their affiliated organizations, or those of the publisher, the editors and the reviewers. Any product that may be evaluated in this article, or claim that may be made by its manufacturer, is not guaranteed or endorsed by the publisher.
Supplementary Material
The Supplementary Material for this article can be found online at: https://www.frontiersin.org/articles/10.3389/fmicb. 2022.853535/full#supplementary-material
Footnotes
References
Aguirre-Monroy, A. M., Santana-Martínez, J. C., and Dussán, J. (2019). Lysinibacillus sphaericus as a nutrient enhancer during fire-impacted soil replantation. Appl. Environ. Soil Sci. 2019, 1–8. doi: 10.1155/2019/3075153
Astaykina, A. A., Streletskii, R. A., Maslov, M. N., Belov, A. A., Gorbatov, V. S., and Stepanov, A. L. (2020). The impact of pesticides on the microbial community of agrosoddy-podzolic Soil. Eurasian Soil Sci. 53, 696–706. doi: 10.1134/S1064229320050038
Baćmaga, M., Wyszkowska, J., and Kucharski, J. (2018). The influence of chlorothalonil on the activity of soil microorganisms and enzymes. Ecotoxicology 27, 1188–1202. doi: 10.1007/s10646-018-1968-7
Beketov, M. A., Kefford, B. J., Schäfer, R. B., and Liess, M. (2013). Pesticides reduce regional biodiversity of stream invertebrates. Proc. Natl. Acad. Sci. U. S. A. 110, 11039–11043. doi: 10.1073/pnas.1305618110
Bouché, M. B. (1977). “Stratégies lombriciennes,” Soil organisms as components of ecosystems. eds. U. Lohm and T. Persson (Stockholm, Ecology Bulletin), 122–132.
Byzov, B. A., Nechitaylo, T. Y., Bumazhkin, B. K., Kurakov, A. V., Golyshin, P. N., and Zvyagintsev, D. G. (2009). Culturable microorganisms from the earthworm digestive tract. Microbiology 78, 360–368. doi: 10.1134/S0026261709030151
Byzov, B. A., Tikhonov, V., Nechitailo, T. Y., Demin, V. V., and Zvyagintsev, D. G. (2015). Taxonomic composition and physiological and biochemical properties of bacteria in the digestive tracts of earthworms. Eurasian Soil Sci. 48, 268–275. doi: 10.1134/S1064229315030035
Callahan, B. J., McMurdie, P. J., and Holmes, S. P. (2017). Exact sequence variants should replace operational taxonomic units in marker-gene data analysis. ISME J. 11, 2639–2643. doi: 10.1038/ismej.2017.119
Carriger, J. F., Rand, G. M., Gardinali, P. R., Perry, W. B., Tompkins, M. S., and Fernandez, A. M. (2006). Pesticides of potential ecological concern in sediment from south Florida canals: An ecological risk prioritization for aquatic arthropods. Soil and Sediment Contamination 15. doi: 10.1080/15320380500363095
Chang, X., Sun, Y., Zhao, L., Li, X., Yang, S., Weng, L., et al. (2021). Exposure to fomesafen alters the gut microbiota and the physiology of the earthworm Pheretima guillelmi. Chemosphere 284:131290. doi: 10.1016/j.chemosphere.2021.131290
Chen, X., Ma, X., Pan, Y., Ji, R., Gu, X., Luo, S., et al. (2020). Dissipation, transformation and accumulation of triclosan in soil-earthworm system and effects of biosolids application. Sci. Total Environ. 712:136563. doi: 10.1016/j.scitotenv.2020.136563
Datta, S., Singh, J., Singh, S., and Singh, J. (2016). Earthworms, pesticides and sustainable agriculture: a review. Environ. Sci. Pollut. Res. 23, 8227–8243. doi: 10.1007/s11356-016-6375-0
Doolotkeldieva, T., Konurbaeva, M., and Bobusheva, S. (2018). Microbial communities in pesticide-contaminated soils in Kyrgyzstan and bioremediation possibilities. Environ. Sci. Pollut. Res. 25, 31848–31862. doi: 10.1007/s11356-017-0048-5
Duke, S. O. (2017). Pesticide dose - A parameter with many implications. ACS Symposium Series 1–13. doi: 10.1021/bk-2017-1249.ch001
Dulla, G. F. J., Go, R. A., Stahl, D. A., and Davidson, S. K. (2012). Verminephrobacter eiseniae type IV pili and flagella are required to colonize earthworm Nephridia. ISME J. 6, 1166–1175. doi: 10.1038/ismej.2011.183
Egert, M., Marhan, S., Wagner, B., Scheu, S., and Friedrich, M. W. (2004). Molecular profiling of 16S rRNA genes reveals diet-related differences of microbial communities in soil, gut, and casts of Lumbricus terrestris L. (Oligochaeta: Lumbricidae). FEMS Microbiol. Ecol. 48, 187–197. doi: 10.1016/j.femsec.2004.01.007
Fadrosh, D. W., Ma, B., Gajer, P., Sengamalay, N., Ott, S., Brotman, R. M., et al. (2014). An improved dual-indexing approach for multiplexed 16S rRNA gene sequencing on the Illumina MiSeq platform. Microbiome 2:6. doi: 10.1186/2049-2618-2-6
Fenner, K., Canonica, S., Wackett, L. P., and Elsner, M. (2013). Evaluating pesticide degradation in the environment: blind spots and emerging opportunities. Science 341, 752–758. doi: 10.1126/science.1236281
Hess, R. A., and Nakai, M. (2000). Histopathology of the male reproductive system induced by the fungicide benomyl. Histol. Histopathol. 15, 207–224. doi: 10.14670/HH-15.207
Hole, D. G., Perkins, A. J., Wilson, J. D., Alexander, I. H., Grice, P. V., and Evans, A. D. (2005). Does organic farming benefit biodiversity? Biol. Conserv. 122, 113–130. doi: 10.1016/j.biocon.2004.07.018
Horn, M. A., Schramm, A., and Drake, H. L. (2003). The earthworm gut: an ideal habitat for ingested N2O-producing microorganisms. Appl. Environ. Microbiol. 69, 1662–1669. doi: 10.1128/AEM.69.3.1662-1669.2003
IUSS Working Group WRB (2015). World Reference Base for Soil Resources 2014. International Soil Classification System for Naming Soils and Creating Legends for Soil Maps. Food and Agriculture Organization of the United Nations, Rome.
Jastrzȩbska, E. (2011). The effect of chlorpyrifos and teflubenzuron on the enzymatic activity of soil. Pol. J. Environ. Stud. 20, 903–910.
Jien, S. H., Kuo, Y. L., Liao, C. S., Wu, Y. T., Igalavithana, A. D., Tsang, D. C. W., et al. (2021). Effects of field scale in situ biochar incorporation on soil environment in a tropical highly weathered soil. Environ. Pollut. 272:116009. doi: 10.1016/J.ENVPOL.2020.116009
Jones, C. G., Lawton, J. H., and Shachak, M. (1994). Organisms as ecosystem engineers. Oikos 69:373. doi: 10.2307/3545850
Kafilzadeh, F., Ebrahimnezhad, M., and Tahery, Y. (2015). Isolation and identification of Endosulfan-degrading bacteria and evaluation of their bioremediation in Kor River, Iran. Osong Public Health Res. Perspect. 6, 39–46. doi: 10.1016/j.phrp.2014.12.003
Kavitha, V., Anandhan, R., Alharbi, N. S., Kadaikunnan, S., Khaled, J. M., Almanaa, T. N., et al. (2019). Impact of pesticide monocrotophos on microbial populations and histology of intestine in the Indian earthworm Lampito mauritii (Kinberg). Microb. Pathogensis. doi: 10.1016/j.micpath.2019.103893
Knapp, B. A., Podmirseg, S. M., Seeber, J., Meyer, E., and Insam, H. (2009). Diet-related composition of the gut microbiota of Lumbricus rubellus as revealed by a molecular fingerprinting technique and cloning. Soil Biol. Biochem. 41, 2299–2307. doi: 10.1016/j.soilbio.2009.08.011
Kuipa, P., Mudziwapasi, R., Mlambo, S., Chigu, N., Kuipa, P., and Sanyika, T. (2016). Isolation and molecular characterization of bacteria from the gut of Eisenia fetida for biodegradation of 4,4 DDT. J. Appl. Biol. Biotechnol. 4, 41–47. doi: 10.7324/JABB.2016.40507
Kuzyakov, Y., and Blagodatskaya, E. (2015). Microbial hotspots and hot moments in soil: concept and review. Soil Biol. Biochem. 83, 184–199. doi: 10.1016/j.soilbio.2015.01.025
Lavelle, P., Barois, I., Martin, A., Zaidi, Z., and Schaefer, R. (1989). “Management of earthworm populations in agro-ecosystems: a possible way to maintain soil quality?” in Ecology of Arable Land—Perspectives and Challenges. Springer, Dordrecht, 109–122.
Liu, D., Lian, B., Wu, C., and Guo, P. (2018). A comparative study of gut microbiota profiles of earthworms fed in three different substrates. Symbiosis 74, 21–29. doi: 10.1007/s13199-017-0491-6
Linnaeus, C. (1758). Systema naturae per regna tria naturae: secundum classes, ordines, genera, species, cum characteribus, differentiis, synonymis, locis. Stockholm, Sweden: Laurentii Salvii (in Latin).
Lo, C. C. (2010). Effect of pesticides on soil microbial community. J. Environ. Sci. Health – Part B Pesticides Food Contam. Agric. Wastes 45, 348–359. doi: 10.1080/03601231003799804
Lund, M. B., Kjeldsen, K. U., and Schramm, A. (2014). The earthworm-verminephrobacter symbiosis: an emerging experimental system to study extracellular symbiosis. Front. Microbiol. 5:128. doi: 10.3389/fmicb.2014.00128
Ma, L., Xie, Y., Han, Z., Giesy, J. P., and Zhang, X. (2017). Responses of earthworms and microbial communities in their guts to Triclosan. Chemosphere 168, 1194–1202. doi: 10.1016/j.chemosphere.2016.10.079
Meier, A. B., Hunger, S., and Drake, H. L. (2021). Differential engagement of fermentative taxa in gut contents of the earthworm Lumbricus terrestris. Appl. Environ. Microbiol. 84, e01851–e01817. doi: 10.1128/AEM.01851-17
Nechitaylo, T. Y., Yakimov, M. M., Godinho, M., Timmis, K. N., Belogolova, E., Byzov, B. A., et al. (2010). Effect of the earthworms Lumbricus terrestris and Aporrectodea caliginosa on bacterial diversity in soil. Microb. Ecol. 59, 574–587. doi: 10.1007/s00248-009-9604-y
OECD (1984). Test No 207: Earthworm, Acute Toxicity Tests. OECD Guidelines for the Testing of Chemicals.
Owagboriaye, F., Mesnage, R., Dedeke, G., Adegboyega, T., Aladesida, A., Adeleke, M., et al. (2021). Impacts of a glyphosate-based herbicide on the gut microbiome of three earthworm species (Alma millsoni, Eudrilus eugeniae and Libyodrilus violaceus): a pilot study. Toxicol. Rep. 8, 753–758. doi: 10.1016/J.TOXREP.2021.03.021
Pass, D. A., Morgan, A. J., Read, D. S., Field, D., Weightman, A. J., and Kille, P. (2015). The effect of anthropogenic arsenic contamination on the earthworm microbiome. Environ. Microbiol. 17, 1884–1896. doi: 10.1111/1462-2920.12712
Pawar, K. R., and Mali, G. V. (2014). Biodegradation of the organophosphorus insecticide Dichlorvas by Bacillus species isolated from grape wine yard soils from Sangli District, M.S., India. Int. Res. J. Environ. Sci. 3, 8–12.
Pelosi, C., Barot, S., Capowiez, Y., Hedde, M., and Vandenbulcke, F. (2014). Pesticides and earthworms. A review. Agron. Sustain. Dev. 34, 199–228. doi: 10.1007/s13593-013-0151-z
Pelosi, C., Joimel, S., and Makowski, D. (2013). Searching for a more sensitive earthworm species to be used in pesticide homologation tests—a meta-analysis. Chemosphere 90, 895–900. doi: 10.1016/j.chemosphere.2012.09.034
Pérez Rodríguez, M., Melo, C., Jiménez, E., and Dussán, J. (2019). Glyphosate bioremediation through the sarcosine oxidase pathway mediated by Lysinibacillus sphaericus in soils cultivated with potatoes. Agriculture 9:217. doi: 10.3390/agriculture9100217
PPDB: Pesticide Properties DataBase (2016). PPDB: Pesticide Properties DataBase. Available at: http://sitem.herts.ac.uk/aeru/ppdb/ (Accessed December 20, 2021).
Puglisi, E. (2012). Response of Microbial Organisms (Aquatic and Terrestrial) to Pesticides, Vol. 9. EFSA Supporting Publications, 359E.
Salokhe, S., and Deshapande, H. (2014). Laboratory evaluation of Fipronil on biological parameters, gut microflora and physiology of Eudrilus eugeniae. Int. J. Sci. Res. Publ. 4, 1–6.
Sanchez-Hernandez, J. C., Mazzia, C., Capowiez, Y., and Rault, M. (2009). Carboxylesterase activity in earthworm gut contents: potential (eco)toxicological implications. Comp. Biochem. Physiol. Part C: Toxicol. Pharmacol. 150, 503–511. doi: 10.1016/J.CBPC.2009.07.009
Sapkota, R., Santos, S., Farias, P., Krogh, P. H., and Winding, A. (2020). Insights into the earthworm gut multi-kingdom microbial communities. Sci. Total Environ. 727:138301. doi: 10.1016/J.SCITOTENV.2020.138301
Shin, N.-R., Whon, T. W., and Bae, J.-W. (2015). Proteobacteria: microbial signature of dysbiosis in gut microbiota. Trends Biotechnol. 33, 496–503. doi: 10.1016/j.tibtech.2015.06.011
Sorour, J., and Larink, O. (2001). Toxic effects of benomyl on the ultrastructure during spermatogenesis of the earthworm Eisenia fetida. Ecotoxicol. Environ. Saf. 50, 180–188. doi: 10.1006/eesa.2001.2067
Streletskii, R., Astaykina, A., Krasnov, G., and Gorbatov, V. (2022). Changes in bacterial and fungal community of soil under treatment of pesticides. Agronomy 12, 32–41. doi: 10.3390/agronomy12010124
Su, X., Wang, Y., He, Q., Hu, X., and Chen, Y. (2019). Biochar remediates denitrification process and N2O emission in pesticide chlorothalonil-polluted soil: role of electron transport chain. Chem. Eng. J. 370, 587–594. doi: 10.1016/J.CEJ.2019.03.195
Sun, M., Chao, H., Zheng, X., Deng, S., Ye, M., and Hu, F. (2020). Ecological role of earthworm intestinal bacteria in terrestrial environments: a review. Sci. Total Environ. 740:140008. doi: 10.1016/j.scitotenv.2020.140008
Tišler, T., Jemec, A., Mozetič, B., and Trebše, P. (2009). Hazard identification of imidacloprid to aquatic environment. Chemosphere 76, 907–914. doi: 10.1016/J.CHEMOSPHERE.2009.05.002
van der Werf, H. M. G. (1996). Assessing the impact of pesticides on the environment. Agric. Ecosyst. Environ. 60, 81–96. doi: 10.1016/S0167-8809(96)01096-1
Vieites, J. M., Ghazi, A., Beloqui, A., Polaina, J., Andreu, J. M., Golyshina, O. V., et al. (2010). Inter-conversion of catalytic abilities in a bifunctional carboxyl/feruloyl-esterase from earthworm gut metagenome. Microb. Biotechnol. 3, 48–58. doi: 10.1111/j.1751-7915.2009.00135.x
Wang, H.-T., Ma, L., Zhu, D., Ding, J., Li, G., Jin, B.-J., et al. (2022). Responses of earthworm Metaphire vulgaris gut microbiota to arsenic and nanoplastics contamination. Sci. Total Environ. 806:150279. doi: 10.1016/j.scitotenv.2021.150279
Wang, K., Pang, S., Mu, X., Qi, S., Li, D., Cui, F., et al. (2015). Biological response of earthworm, Eisenia fetida, to five neonicotinoid insecticides. Chemosphere 132, 120–126. doi: 10.1016/j.chemosphere.2015.03.002
Wells, G. P., and Laverack, M. S. (1963). The physiology of earthworms. J. Anim. Ecol. 32:577. doi: 10.2307/2613
Willms, I. M., Rudolph, A. Y., Göschel, I., Bolz, S. H., Schneider, D., Penone, C., et al. (2020). Globally abundant “Candidatus udaeobacter,” benefits from release of antibiotics in soil and potentially performs trace gas scavenging. mSphere 5, e00186–e001820. doi: 10.1128/msphere.00186-20
Wüst, P. K., Horn, M. A., and Drake, H. L. (2011). Clostridiaceae and Enterobacteriaceae as active fermenters in earthworm gut content. ISME J. 5, 92–106. doi: 10.1038/ismej.2010.99
Zhan, H., Wang, H., Liao, L., Feng, Y., Fan, X., Zhang, L., et al. (2018). Kinetics and novel degradation pathway of permethrin in Acinetobacter baumannii ZH-14. Front. Microbiol. 9:98. doi: 10.3389/fmicb.2018.00098
Zhao, X., Fan, F., Zhou, H., Zhang, P., and Zhao, G. (2018). Microbial diversity and activity of an aged soil contaminated by polycyclic aromatic hydrocarbons. Bioprocess Biosyst. Eng. 41, 871–883. doi: 10.1007/s00449-018-1921-4
Keywords: pesticides, next-generation sequencing, earthworm, gut microbiota, bacterial biodiversity
Citation: Astaykina A, Streletskii R, Maslov M, Krasnov G and Gorbatov V (2022) Effects of Three Pesticides on the Earthworm Lumbricus terrestris Gut Microbiota. Front. Microbiol. 13:853535. doi: 10.3389/fmicb.2022.853535
Edited by:
Shaohua Chen, South China Agricultural University, ChinaReviewed by:
Inna Solyanikova, Belgorod National Research University, RussiaSikandar I. Mulla, REVA University, India
Willian Garcia Birolli, Barretos Cancer Hospital, Brazil
Copyright © 2022 Astaykina, Streletskii, Maslov, Krasnov and Gorbatov. This is an open-access article distributed under the terms of the Creative Commons Attribution License (CC BY). The use, distribution or reproduction in other forums is permitted, provided the original author(s) and the copyright owner(s) are credited and that the original publication in this journal is cited, in accordance with accepted academic practice. No use, distribution or reproduction is permitted which does not comply with these terms.
*Correspondence: Angelika Astaykina, YXN0YWlraW5hLWFuemhlbEBtYWlsLnJ1