- State Key Laboratory of Oral Diseases, National Clinical Research Center for Oral Diseases, West China Hospital of Stomatology, Sichuan University, Chengdu, China
Peri-implant diseases are considered to be a chronic destructive inflammatory destruction/damage occurring in soft and hard peri-implant tissues during the patient’s perennial use after implant restoration and have attracted much attention because of their high incidence. Although most studies seem to suggest that the pathogenesis of peri-implant diseases is similar to that of periodontal diseases and that both begin with microbial infection, the specific mechanism of peri-implant diseases remains unclear. As an oral opportunistic pathogen, Fusobacterium nucleatum (F. nucleatum) has been demonstrated to be vital for the occurrence and development of many oral infectious diseases, especially periodontal diseases. More notably, the latest relevant studies suggest that F. nucleatum may contribute to the occurrence and development of peri-implant diseases. Considering the close connection between peri-implant diseases and periodontal diseases, a summary of the role of Fusobacterium nucleatum in periodontal diseases may provide more research directions and ideas for the peri-implantation mechanism. In this review, we summarize the effects of F. nucleatum on periodontal diseases by biofilm formation, host infection, and host response, and then we establish the relationship between periodontal and peri-implant diseases. Based on the above aspects, we discuss the importance and potential value of F. nucleatum in peri-implant diseases.
Introduction
Peri-implant diseases, including peri-implant mucositis and peri-implantitis, occur in the soft and hard peri-implant tissues around implants during long-term usage after implant restoration (Albrektsson et al., 2016; Papathanasiou et al., 2016; Schwarz et al., 2018). Peri-implant mucositis is mucosal inflammation in peri-implant soft tissue and is clinically characterized by bleeding on gentle probing without supporting bone loss (Berglundh et al., 2018a). In contrast, peri-implantitis is a more serious pathological condition involving the implant supporting tissues, which leads to increased probing depths, bleeding on probing (BOP), and radiographic loss of marginal bone (Heitz-Mayfield and Salvi, 2018; Schwarz et al., 2018; Berglundh et al., 2018a). According to statistics, the prevalence rate of peri-implant mucositis exceeds 50%, while peri-implantitis occurs in approximately 20% of implants (Tarnow, 2016; Buser et al., 2017; Ting et al., 2018). Although several factors, such as the diagnosis method and statistical sources, may affect the results and lead to some biases, the pathogenesis and treatment strategies for peri-implant diseases are momentous issues that have recently received widespread attention but have not yet been resolved (Ritzer et al., 2017; Smith et al., 2017; Berglundh et al., 2018b).
Current studies consider that microbial biofilm accumulation may be a major cause of diseases, and therefore, a complete understanding of the peri-implant microbiota is important for treatment planning. With continuous advances in microbiological techniques, including culture-dependent methods, molecular methods, and sequencing methods, some common pathogens, such as Tannerella forsythia, Porphyromonas gingivalis, Prevotella intermedia, and Fusobacterium nucleatum, have been detected in peri-implant clinical or laboratory samples (Charalampakis and Belibasakis, 2015; Sahrmann et al., 2020). Fusobacterium nucleatum is a common but unique microorganism in the oral environment and has aroused much interest as it participates in not only dental but also extraoral and systemic infectious diseases (Brennan and Garrett, 2019). In the oral environment, on the one hand, F. nucleatum could establish a symbiotic relationship with the host under a healthy state (Dukka et al., 2021). On the other hand, F. nucleatum could destroy the balance as a pathogen, interacting with other pathogens and leading to severe oral diseases (Lamont et al., 2018; Stokowa-Soltys et al., 2021). Existing research has explained how F. nucleatum affects the initiation and development of periodontal diseases to a large extent, but few studies have focused on the relationship between peri-implant diseases and F. nucleatum. Since periodontal diseases and peri-implant diseases exhibit core similarities but also have specific unique characteristics, can the current findings on the association of periodontal disease with F. nucleatum, along with its methodology, be applied to research the pathogenesis of peri-implant diseases? In this review, we mainly focus on summarizing the essential mechanism of F. nucleatum in periodontal diseases and discuss the potential value of F. nucleatum in the pathogenesis of peri-implant diseases.
The Role of Fusobacterium nucleatum in Periodontal Diseases
Biofilm Formation
It is widely accepted that during the transition from a healthy state to an inflammatory state, the composition of dental plaque undergoes a series of complex evolutions. Initial colonizers, such as Actinomyces and Streptococci, are the first microorganisms that adhere to the tooth surface, which contribute to the subsequent coaggregation (Sanchez et al., 2011), and late colonizers, such as P. gingivalis and Treponema denticola, are mostly known as periodontal pathogens that bind to previously bound bacteria (Kolenbrander et al., 2010). In the process of biofilm maturation, however, the intermediate colonizer F. nucleatum acts as a joint and corner stone organism that characteristically binds to representatives of nearly all colonizers, ranging from the initial to the late colonizers (Thurnheer et al., 2019; Zhang et al., 2019).
One of the most well-known adhesins of F. nucleatum is RadD, which contributes to interspecies coaggregation and multispecies biofilm formation. RadD is an outer membrane protein with a high molecular weight (nearly 350 KDa) that is isolated from wild-type F. nucleatum and initially meditates arginine-inhibitable adhesion between F. nucleatum and Streptococcus cristatus as well as Actinomyces naeslundii (Edwards et al., 2007; Kaplan et al., 2009). RadD is encoded by the FN1526 gene, which is now designed as the radD gene, but the expression of RadD may be controlled by a recently revealed Fusobacteria two-component signal transduction system, CarRs (Kaplan et al., 2009; Wu et al., 2021). Using a radD mutant, Kaplan et al. (2009) observed the suppression of F. nucleatum and streptococci coadherence, and this finding demonstrated the unique character of RadD in F. nucleatum binding capacity for the first time. The same approach has been applied in other later RadD-binding related studies, such as the interaction between F. nucleatum RadD and Streptococcus mutans SpaP (Guo et al., 2017) and the RadD-associated coaggregation between F. nucleatum and Candida albicans (Wu et al., 2015), which have all emphasized the significant function of RadD in the coadherence between F. nucleatum and oral microbiota. RadD mediates adhesion not only to oral colonizers but also to intestinal colonizers. It binds to Clostridioides difficile, a well-known anaerobic intestinal pathogen (Sandhu and McBride, 2018), via flagella on C. difficile, promoting biofilm formation, accelerating C. difficile colonization, and ultimately leading to C. difficile infection and severe diarrhea (Engevik et al., 2021).
Apart from RadD, many other outer membrane proteins in F. nucleatum also have prominent roles in bacterial coaggregation and biofilm formation. The 42-kDa F. nucleatum major outer-membrane protein (FomA), which mediates coaggregation with P. gingivalis in periodontal pockets (Kinder and Holt, 1993), has been reported to be a crucial factor for fusobacterial biofilm-bridge function (Zhang et al., 2021). FomA cooperates with RadD and Fap2 to achieve binding between F. nucleatum and P. gingivalis (Brennan and Garrett, 2019). Another F. nucleatum outer membrane protein, coaggregation mediating protein (CmpA), has been targeted as it provides additional adhesion in the binding between F. nucleatum and Streptococcus gordonii, and it is equally important as RadD (Lima et al., 2017). Moreover, in addition to F. nucleatum outer membrane proteins, F. nucleatum outer membrane lectin greatly contributes to dual-species biofilm formation. Both T. denticola major outer sheath protein (MSP) and P. gingivalis lipopolysaccharide (LPS) are able to bind to the F. nucleatum lectin in a galactose-inhibitable manner to enhance interspecies coaggregation (Rosen and Sela, 2006; Rosen et al., 2008). Once a biofilm is formed, it can heighten the survival, invasion, and pathogenic capability of F. nucleatum (Gursoy et al., 2010; Horiuchi et al., 2020), which may influence the proliferation pattern of the oral epithelium and lead to early periodontal disease-related lesions (Pollanen et al., 2012; Figure 1).
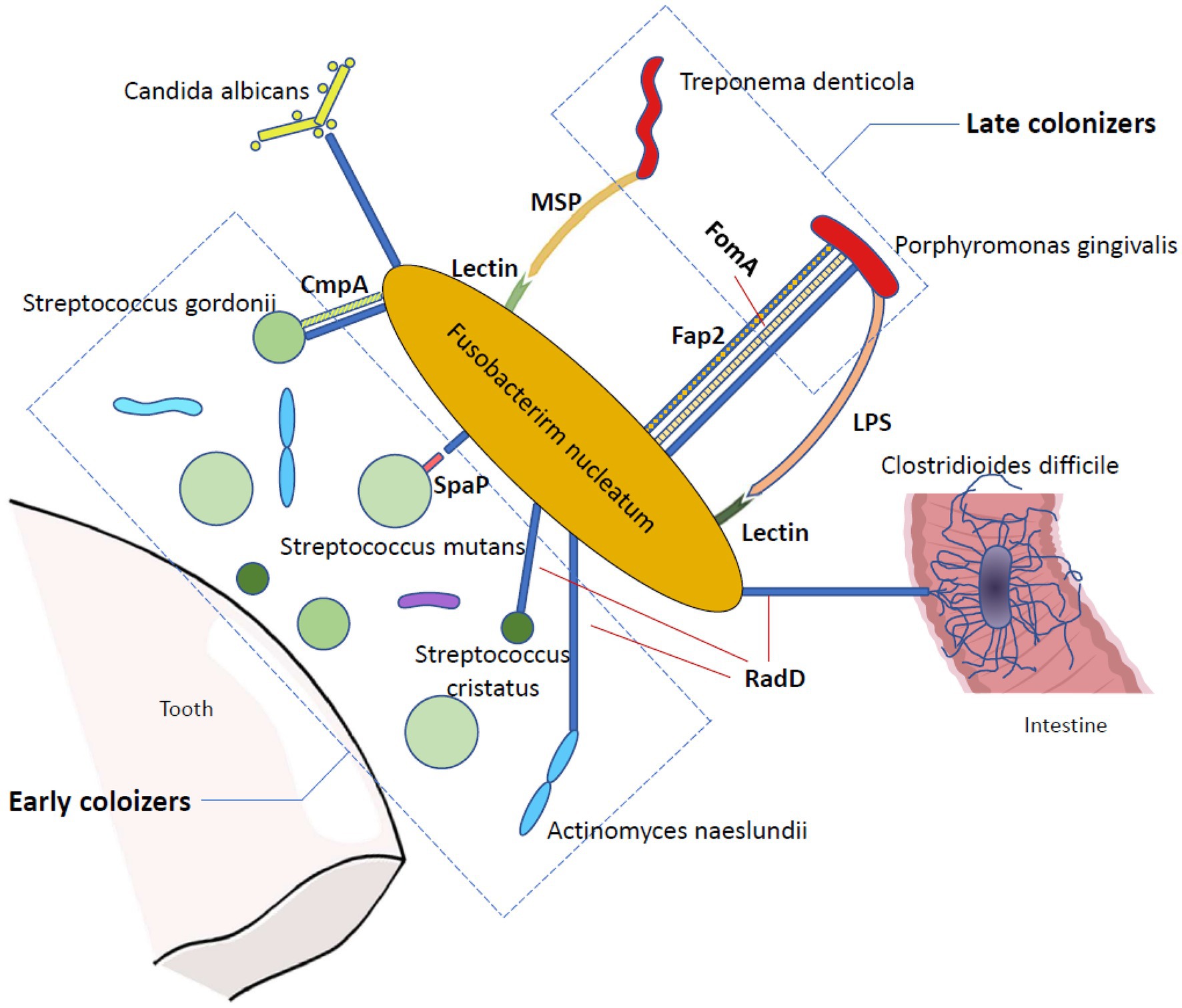
Figure 1. The bridging function of Fusobacterium nucleatum in biofilm formation. Fusobacterium nucleatum is a bridging organism that has a great ability to bind to many other microorganisms. In the oral cavity, Fusobacterium nucleatum adheres to both early and late colonizers, promoting the coaggregation of periodontal disease-related pathogens. In addition, it can bind to Candida albicans and Clostridioides difficile. RadD is one of the most profoundly studied adhesions of Fusobacterium nucleatum and contributes to the binding between Fusobacterium nucleatum and Candida albicans, Streptococcus cristatus, Streptococcus gordonii, Streptococcus mutans, Actinomyces naeslundii, Porphyromonas gingivalis, and Clostridioides difficile via an arginine-inhibitable method. Several other Fusobacterium nucleatum outer membrane proteins are also vital in bacterial coaggregation and biofilm formation, including Fap2, FomA and CmpA. Moreover, the Fusobacterium nucleatum outer membrane lectin also contributes to dual-species biofilm formation in a galactose-inhibitable method.
Host Infection
The important mechanism that elicits infection in the host is the remarkable adherence and/or invasive properties of F. nucleatum. It has been confirmed that F. nucleatum can bind to and/or invade a wide variety of host cells (Han et al., 2000; Dabija-Wolter et al., 2009; Fardini et al., 2011).
A specifically characterized F. nucleatum adhesin, Fusobacterium adhesion A (FadA), which was first found to mediate the binding between F. nucleatum and oral mucosal cells, has been discovered to be a crucial part of these functions (Han et al., 2005). The FadA gene is considerably conserved among two closely related oral fusobacteria, which are referred to as F. nucleatum and F. periodonticum. However, it is absent in almost all the other nonoral fusobacterial species (Han et al., 2005). Using PCR technology to detect this gene in F. nucleatum-positive subgingival biofilm samples, Liu et al. (2014) substantiated that the gingival index directly varies with the detection rate of FadA. The functional form of FadA, the FadA complex (FadAc), is a heterogeneous recombinant gene that is composed of pre-FadA consisting of 129 amino acid residues and mFadA consisting of 111 amino acid residues (Xu et al., 2007). Pre-FadA is a full-length peptide that processes an 18-amino acid signal peptide, which is anchored in the inner membrane, whereas mFadA exists in a mature form that is secreted from the bacterial cell outer membrane (Xu et al., 2007). Neither pre-FadA nor mFadA exhibited little virulence. The crystal structure of mFadA indicated that the mFadA monomers assemble in a head-to-tail pattern by a novel leucine chain motif (Nithianantham et al., 2009). However, this structure is a long, thin, and unstable filament that is defective in curbing the adhesion of F. nucleatum to host cells by itself (Xu et al., 2007; Temoin et al., 2012). When mixed with pre-FadA, the oligomers bind to each other and form short and stable heterogeneous fibrils with the help of signal peptides (Temoin et al., 2012; Meng et al., 2021). Under stress conditions, FadAc assembles into a functional amyloid-like structure, which enhances the pathogenicity of F. nucleatum and ultimately leads to periodontal disease and periodontal bone loss in mice, and pre-FadA is a critical component (Meng et al., 2021). In addition to adhesin, FadA also plays a critical role in invasion as it binds to cadherins, which are known as cell junction molecules (Han, 2015; Rubinstein et al., 2019). FadA binds to endothelial cell vascular endothelial cadherin, relocating its position and loosening intracellular bridges. Ultimately, the structural integrity of the endothelium is destroyed, which may enable noninvasive bacteria to spread systemically and ultimately lead to mixed infection (Fardini et al., 2011).
Fusobacterium nucleatum not only possesses the remarkable ability to adhere to and/or invade host cells itself but also acts as a promoter that enhances the attachment and/or internalization of some early and late colonizers. Streptococcus cristatus, a Streptococcus, is a noninvasive early colonizer that lacks a great ability to bind gingival epithelial cells and internalize by itself (Handley et al., 1991). However, noninvasive bacteria could be transported into host epithelial cells via the coaggregation method when coincubated with F. nucleatum (Edwards et al., 2006). In contrast, P. gingivalis is generally acknowledged as a crucial bacterium in the development of periodontitis (Reyes, 2021) that possesses invasive capacity. However, the invasive feature of P. gingivalis to host cells is dramatically inferior to that of F. nucleatum (Jang et al., 2017), which is in agreement with the finding of De Andrade et al. (2021) that F. nucleatum is more virulent than P. gingivalis to host cells. It has been demonstrated that mixed infection with F. nucleatum strengthens the invasion capacity of P. gingivalis in oral epithelial cells (Zhang et al., 2021). The “promoter” characteristic of F. nucleatum makes it a crucial pathogen in host mixed infection.
Host Responses
As an opportunistic pathogen, F. nucleatum participates in both periodontal health and periodontal disease-related host responses. Under healthy conditions, F. nucleatum constantly stimulates gingival epithelial cells, which leads to the constant expression of human beta-defensin-2, an antimicrobial peptide (AMP; Krisanaprakornkit et al., 2000). AMPs are able to directly defend against invading microbes and intercommunicate with the adaptive immune system, which keeps the oral epithelia from incurring infection and contributes significantly to innate immune responses (Yin and Dale, 2007; Chung and Khanum, 2017; Magana et al., 2020). This mutual effect between F. nucleatum and gingival epithelial cells has been found to be most strongly mediated by F. nucleatum-associated β-defensin inducer (FAD-I), an F. nucleatum cell wall-associated protein encoded by the FN1527 gene (Gupta et al., 2010; Ghosh et al., 2018). It binds to gingival epithelial cell Toll-like receptor 1/2 (TLR-1/2) and TLR-2/6 to promote beta-defension-2 induction (Bhattacharyya et al., 2016). Several other effector molecules that also play a significant role in preserving periodontal health, including cytokines and chemokines, could be promoted by the stimulation of gingival epithelial cells by F. nucleatum (Krisanaprakornkit et al., 2000; Wassing et al., 2021). In the complex oral environment, these molecules are maintained under homeostasis to preserve periodontal health (Han, 2015).
In a state of disease, the balance is broken, and F. nucleatum destroys the epithelial barrier, becoming a pathogen and causing malignant host responses. When the integrity of the epithelium is destroyed, human gingival fibroblasts (GFs), which are most abundant in periodontal connective tissue, become the first line of defense against F. nucleatum invasion (Ahn et al., 2017b; Naruishi and Nagata, 2018). On the one hand, F. nucleatum upregulates the intracellular ROS level, facilitating cell autophagy (Kang et al., 2019); on the other hand, it stimulates the secretion of a number of cytokines, such as IL-6 and IL-8, which are able to recruit immune cells to fight against infection (Ahn et al., 2017a). It seems that with the activation of acquired immunity, the invaded F. nucleatum could be eliminated very quickly. However, the immune system is closely affected by F. nucleatum, so the case is more complicated. One important virulence factor is that F. nucleatum can use its Fap2 and RadD outer membrane proteins to attack human lymphocytes, which enables F. nucleatum to combat the human immune system and kill lymphocytes (Kaplan et al., 2010). Moreover, the influence of F. nucleatum on NK cells could promote the production of TNF-α, which results in more serious alveolar bone loss in mice and probably worsens pathological outcomes in humans (Chaushu et al., 2012; Figure 2; Table 1).
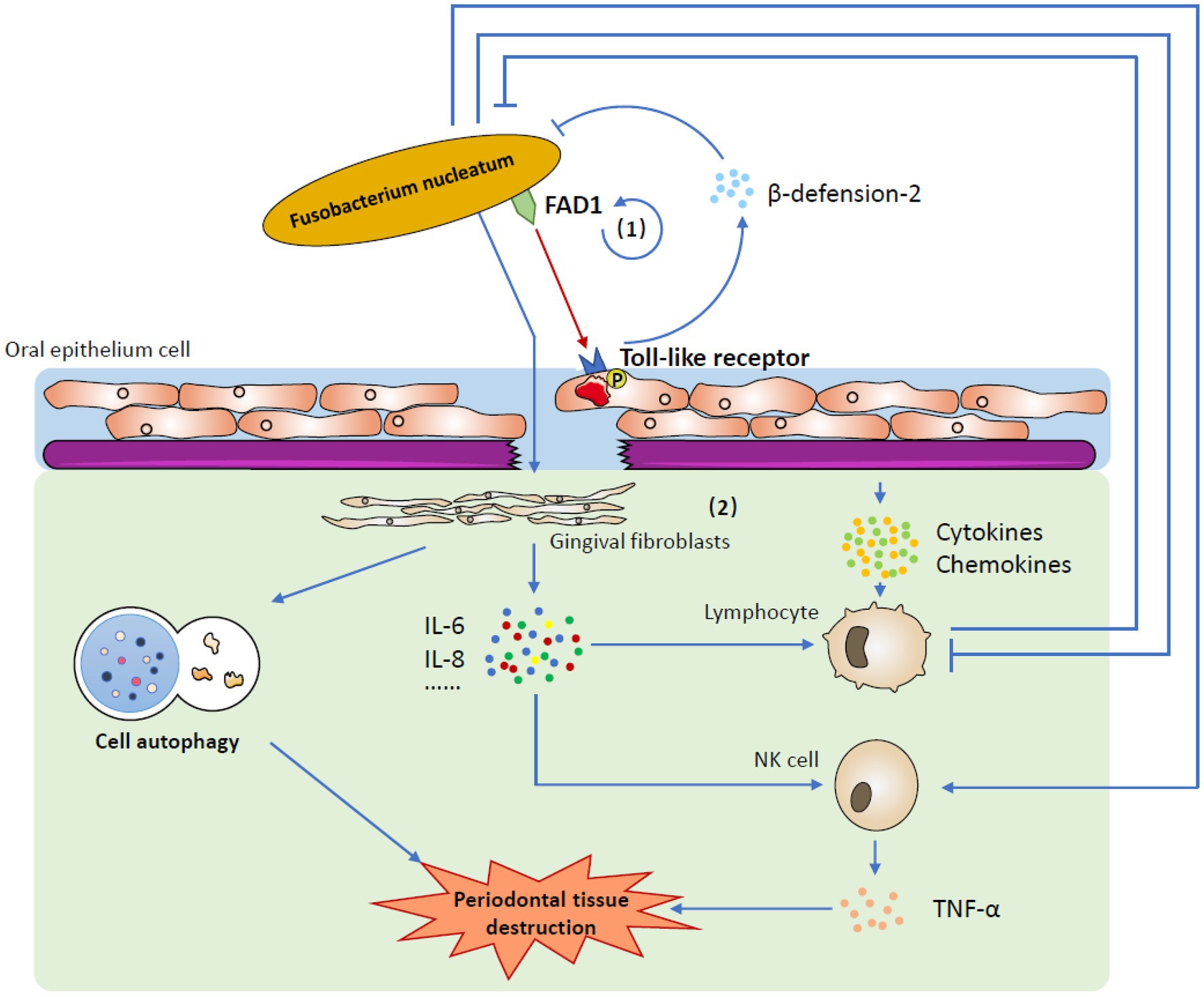
Figure 2. The mechanism by which Fusobacterium nucleatum induces host responses. (1) Under healthy conditions, one of the most important factors is that Fusobacterium nucleatum uses FAD-I to stimulate gingival epithelial cells to express human beta-defensin-2 via gingival epithelial cell Toll-like receptors. Stimulated human beta-defensin-2 helps to defend against invading microbes, including Fusobacterium nucleatum itself, and maintains homeostasis in the complex oral environment. (2) In a state of disease, Fusobacterium nucleatum destroys the epithelial barrier and affects human gingival fibroblasts. It not only induces cell autophagy but also stimulates the secretion of several cytokines and chemokines. When challenged by Fusobacterium nucleatum, the immune system is activated to eliminate invasive bacteria. However, Fusobacterium nucleatum could restrain the human immune system to reduce this removal effect. Moreover, the production of TNF-α from activated NK cells may lead to periodontal tissue destruction.
Special Features of Periodontal Diseases and Peri-Implant Diseases
Considering the similarity between these two diseases, the early peri-implant mucositis and the later peri-implantitis of peri-implant diseases are comparable to gingivitis and periodontitis, respectively (Schincaglia et al., 2017).
As the early stage of the disease, both peri-implant mucositis and gingivitis cause similar symptoms, such as redness, swelling, and bleeding on gentle probing (Heitz-Mayfield and Salvi, 2018). Fusobacterium nucleatum may contribute to this stage by recruiting neutrophils and macrophages to the oral mucosa (Johnson et al., 2018). However, compared with gingivitis, the degree of peri-implant mucositis is more severe, which results in more suppuration and bleeding sites (Meyer et al., 2017; Heitz-Mayfield and Salvi, 2018). Gualini and Berglundh (2003) demonstrated that the proportion of neutrophils and macrophages is higher around lesions from peri-implant mucositis than from gingivitis, which accounts for the more pronounced inflammatory response observed for peri-implant mucositis than gingivitis. Except for the high content of inflammatory cells and inflammatory mediators (Belibasakis, 2014), some specific microbial taxa that have nothing to do with gingivitis have been demonstrated to be positively correlated with peri-implant mucositis (Schincaglia et al., 2017). Even though some crucial differences have been found, the pathogenesis of peri-implant mucositis and gingivitis has not been found to be fundamentally different (Gurlek et al., 2017).
The clinical symptoms of peri-implantitis and periodontitis are also nearly identical (both lead to bone resorption and abscesses; Donos, 2018). However, to date, multiple factors have been found to contribute to the differences between peri-implantitis and periodontitis. From an inflammatory point of view, the extent of peri-implantitis lesions is extraordinarily larger than that of periodontitis lesions, which nearly affects the marginal bone (Dukka et al., 2021). In agreement with peri-implant mucositis and gingivitis, the proportions, numbers and densities of infiltrated neutrophils, macrophages, plasma cells and nitric oxide synthase (iNOS)-positive cells are higher in peri-implantitis than in periodontitis, which reflects the considerably larger quantity of abscesses observed in peri-implantitis clinical symptoms (Carcuac and Berglundh, 2014; Schwarz et al., 2018; Dionigi et al., 2020). From the microorganism point of view, the main distinguishing difference between these two diseases lies in the composition of biofilms, even though both are microbial infectious diseases. First, the plaque construction of peri-implantitis is simpler than that of periodontitis, and its microbial diversity is also low (Kotsakis and Olmedo, 2021). Studies have shown that the abundance of species shared between healthy implants and teeth in most patients is less than 8% (Kotsakis and Olmedo, 2021), while the abundance of species shared between peri-implantitis and periodontitis is less than 50% (Berglundh et al., 2018b). Another finding indicating the divergence between periodontal disease and peri-implantitis biofilms is that peri-implantitis microbes are resistant to beta lactam antibiotics (Leonhardt et al., 2003; Hallstrom et al., 2012), but beta lactam antibiotics show promising therapeutic effects against periodontal disease (Lopez et al., 2006). Moreover, the red complex, which is traditionally enriched in the subgingival plaque of periodontitis, is not detectable in all patients with peri-implantitis (Sanz-Martin et al., 2017). Even when associated flora are detected, the flora tend to be present in much lower numbers in peri-implantitis than in periodontitis (Sanz-Martin et al., 2017). In addition, Eubacterium nodatum, Eubacterium saphenum, and Slackia exigua, for instance, can be detected near peri-implantitis, while these species are rarely detected in periodontal disease (Tamura et al., 2013).
The fundamental reason why peri-implant diseases differ from periodontal diseases in many ways is probably because there have been some discrepancies in their histological structures, especially the surface characteristics, between these two diseases. One of the most important fundamental differences is that the implant surface is directly connected with the crestal bone without involving the periodontal ligament or cementum in periodontal tissue (Ivanovski and Lee, 2018). Thus, in periodontal diseases, the initial substrate for bacterial colonization is mineralized organic dental tissue, whereas bacteria colonize the unmediated titanium base in peri-implant diseases (Kotsakis and Olmedo, 2021). These two substrates differ in chemical composition, roughness and free energy, which seriously affect subsequent microbial adhesion and biofilm formation (Teughels et al., 2006; Busscher et al., 2010; Kotsakis and Olmedo, 2021). Since different microbial taxa are assembled, it is easy to comprehend the variation in the inflammatory response between peri-implant diseases and periodontal diseases. Discrepancies in transmucosal soft tissue could also lead to a distinct inflammatory response. Moon et al. (1999) indicated that the peri-implant transmucosal connective tissue can be divided into two different units. Zone A is closely attached to the implant surface, which possesses almost no blood vessels but a large number of fibroblasts. Zone B is continuous to zone A. It contains more collagen fibers and vascular structures but fewer fibroblasts. Due to the absence of the periodontal ligament, the vascular supply of the peri-implant mucosa is derived only from the supraperiosteal blood vessels lateral to the alveolar bone crest. However, in addition to the supraperiosteal blood vessels, the vascular supply of the periodontium also comes from the periodontal ligament vascular plexus (Ivanovski and Lee, 2018). In general, the peri-implant transmucosal soft tissue contained more collagen fibers with a converted direction (Berglundh et al., 1991) and significantly fewer fibroblasts and vascular structures than periodontal soft tissue. The parallel direction of collagen fibers in peri-implant mucosa may fail to restrict inflammatory cells to the epithelium as the vertical ones do, which leads to the population of inflammatory cells among peri-implant connective tissue (Yuan et al., 2021). A lack of vascular supply has been speculated to weaken the defense capacity of peri-implant tissue and may make it more likely to be invaded by microorganisms (Ivanovski and Lee, 2018). These are all probable reasons why the extent of peri-implantitis lesions is extraordinarily larger than that of periodontitis lesions with more suppuration.
To conclude, biofilm composition and inflammatory responses are two detectable differences between periodontal diseases and peri-implant diseases that were originally related to histological structures. The surface characteristics determine the group of adhered biological species. The biofilm composition cooperates with the histological structure to influence the inflammatory response (Table 2).
Peri-Implant Diseases and Fusobacterium nucleatum
In essence, both periodontal diseases and peri-implant diseases are mixed inflammations that are microbially derived, with dental plaque being a critical factor for initiating the diseases (Kinane et al., 2017; Salvi et al., 2017). Based on this concept, several assessment methods were applied to determine the microbial profile with the aim of uncovering the pathogenesis of peri-implant diseases. Some early studies utilizing bacterial culture techniques found many microbial colonies containing Gram-negative anaerobes and motile rods around the lesion site of failed implants (Bower et al., 1989; Leonhardt et al., 1999; Mombelli and Decaillet, 2011; Charalampakis and Belibasakis, 2015). Further studies have shown that periodontal disease-associated pathogens can be detected in peri-implant diseases via DNA checkerboard hybridizations (Gatti et al., 2008; Maximo et al., 2009; Lee et al., 2012; Persson and Renvert, 2014). Coupled with the fact that peri-implantitis and periodontitis have very similar clinical symptoms (Donos, 2018), it seems that peri-implant diseases and periodontal diseases are two very similar bacterial infectious diseases. However, with the advancement of research methods, several distinctive lines of evidence have been suggested that demonstrate the differences between these two diseases, as mentioned above. Nonetheless, F. nucleatum, which has been fully studied for 20 years regarding the pathogenesis of periodontal diseases, has been recently found to be the most abundant species and comprises part of the shared core microbiome of periodontal diseases and peri-implant diseases (Belibasakis and Manoil, 2021). Although there have been many studies using F. nucleatum as an experimental species to investigate antibacterial or material antibacterial subjects (Tonon et al., 2021; Wei et al., 2021), the reason why F. nucleatum is selected seems to be ambiguous.
Similarities between periodontal diseases and peri-implant diseases could guide us to pay attention to the relationship between F. nucleatum, periodontal diseases and peri-implant diseases. Thus, the similarities may provide us with new ideas on the pathogenesis of F. nucleatum in peri-implant diseases that originate from periodontal diseases. However, the existing discrepancies oblige us to revise the comprehensively studied and established theories regarding periodontal diseases. Regarding peri-implant diseases, F. nucleatum may also play a role in biofilm formation, host infection and host response. However, the exact mechanism by which F. nucleatum affects the genesis and progression of peri-implant diseases is almost specific and must be precisely deliberated. The development of biofilms has been found to be the fundamental cause of many infectious diseases (Arciola et al., 2018; Del Pozo, 2018), including periodontal and peri-implant diseases. We have learned about the bridge characteristic of F. nucleatum in periodontal biofilm formation, with RadD, Fap2, FomA and CmpA mediating interspecies coaggregation. However, owing to the differences in biofilm composition between implants and teeth, how F. nucleatum interacts with peri-implant microbes and which outer membrane protein plays a key role may vary.
As a whole species, F. nucleatum is considered a core species that has the same proportion in health and disease, but it increases by a 3-log higher load during periodontal disease (Curtis et al., 2020) as the whole microbial community increases in biomass. However, it is worth noting that recent research reports that F. nucleatum is one of the first massively increased species during the progression of mucositis to peri-implantitis (Ghensi et al., 2020). The large increase in F. nucleatum in peri-implant diseases may alter the oral microenvironment differently and ultimately affect biofilm constitution (Renvert et al., 2018). Thus, the bridging characteristics of F. nucleatum in peri-implant diseases seem to be more distinct. The situation is more complex for subspecies. The F. nucleatum subspecies vincentii has been found to be the most abundant subspecies of F. nucleatum in peri-implantitis submucosa, periodontal pocket, healthy peri-implant submucosa, and healthy subgingival sulcus samples, which seems to have nothing to do with either periodontal diseases or peri-implant diseases (Yu et al., 2019; Curtis et al., 2020). However, using the 16S rRNA sequencing method, Schincaglia et al. (2017) found a distinct increase in the F. nucleatum subspecies polymorphum in the development of periodontal inflammation, which is opposite to the F. nucleatum observed in periodontal diseases. Rather, a newly targeted subspecies referred to as F. nucleatum Cluster 5 has been demonstrated to be extremely relevant to peri-implant inflammation (Ghensi et al., 2020). The more complicated discrepancies in F. nucleatum subspecies may also reflect a more intricate pathogenesis of F. nucleatum in peri-implant diseases.
Once biofilms are formed, most colonizers have the capacity to bind to and/or invade host cells. FadA is one of the most important adhesions that helps to adhere biofilms to oral mucosal cells and destroy cell–cell junctions. However, because of the converted histological structure, the method by which F. nucleatum elicits host infection is also probably different. The situation is more complicated for host responses. As mentioned above, both the histological structure and biofilm composition could influence the inflammatory response level. Host responses are not only related to the stimulation of the immune system by F. nucleatum alone but are also caused by the reciprocity between F. nucleatum and the surrounding substrate, and this is referred to as the “host infection” character. Owing to the divergences in biofilm composition, histological structure and F. nucleatum-mediated host response, inflammation is more pronounced in peri-implant diseases than in periodontal diseases. Therefore, although F. nucleatum can be detected in both diseases, it is highly probable that the role it plays in the progression of peri-implant disease is different from that in periodontal disease. Unfortunately, few research studies have covered these aspects (Figure 3).
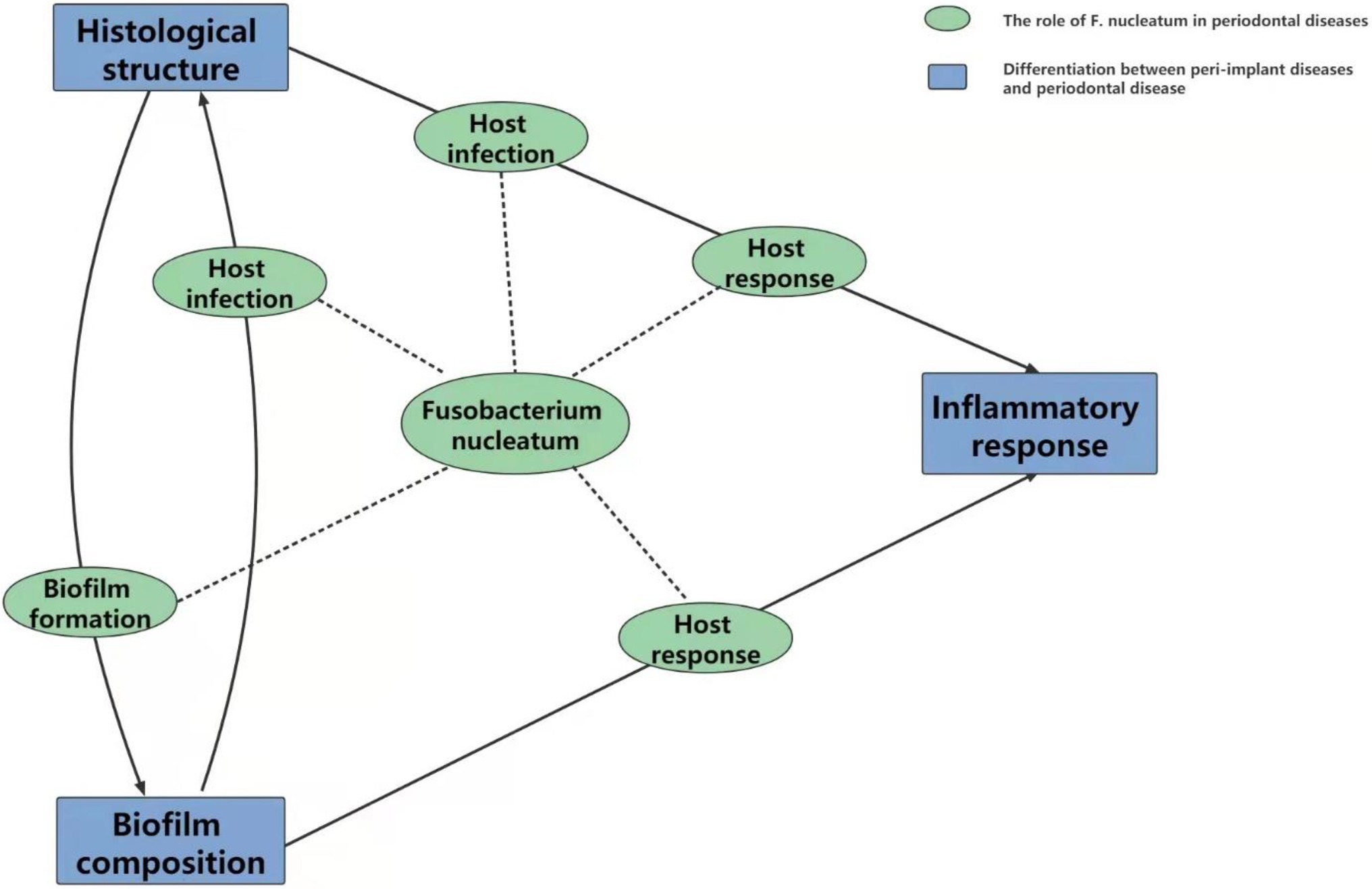
Figure 3. The relationship between Fusobacterium nucleatum periodontal diseases and peri-implant diseases. Biofilms are universally accepted as mutual and critical factors in the initiation of periodontal and peri-implant diseases. The varied substrate for bacterial colonization and probably elevated function of Fusobacterium nucleatum in biofilm formation may be responsible for the reported discrepancies in its composition. With the succession of dental plaque, on the one hand, Fusobacterium nucleatum adheres to and/or invades host cells to induce mixed infection; on the other hand, invaded Fusobacterium nucleatum activates the human immune system to secrete inflammatory factors and accumulate inflammatory cells. These two effects cooperate with each other to elicit the ultimate inflammatory response. Detected differences between periodontal diseases and peri-implant diseases in histological structure and inflammatory response might indicate the specific pathogenesis of Fusobacterium nucleatum in these two diseases individually between the host infection and host response.
Discussion
As an oral opportunistic pathogen, F. nucleatum is one of the most important microorganisms in the pathogenesis of oral diseases. We have concluded how F. nucleatum affects the occurrence and development of periodontal diseases. In periodontitis, F. nucleatum first acts as a joint organism to bind to most colonizers throughout the whole process. Then, the bacteria adhere to and/or invade host cells and act as promoters, enhancing the attachment and/or internalization of some early and late colonizers. Once the balance between F. nucleatum and gingival epithelial cells is damaged, F. nucleatum destroys the epithelial barrier, becoming a pathogen and causing malignant host responses. However, in peri-implant diseases, no definite mechanism is currently available. The results of next-generation sequencing have shown that F. nucleatum contributes significantly to the formation and maturation of biofilms in peri-implant diseases. This indicates that F. nucleatum also plays a significant role in peri-implant diseases, similar to periodontal diseases. Although the bridging role of F. nucleatum in dental plaque is common, there are many differences between these two diseases in bacterial colonization, adhesion of microorganisms, and the formation of biofilms, leading to the high recurrence rate of peri-implantitis. Therefore, the specific mechanism by which F. nucleatum influences the disease seems different.
We are trying to put forward the following three aspects for exploring the pathogenesis of peri-implant diseases from the F. nucleatum point of view. First, more analyses and comparisons of clinical samples (healthy gingiva, healthy peri-implant, gingivitis, peri-implant mucositis, periodontitis, and peri-implantitis) are needed (de Waal et al., 2017; Yeh et al., 2019) since there have been slightly different results from different studies. Some previous studies have raised the bias that the prevalence and levels of F. nucleatum are not associated with peri-implantitis (Zhuang et al., 2016). Fusobacterium nucleatum should be confirmed as a pathogen of peri-implant diseases based on further new studies. Moreover, F. nucleatum subspecies should also be evaluated due to their more complicated situation. Although microbial sequencing of peri-implant diseases is available, there are currently few reports on single-cell RNA-seq and spatial transcriptome analyses around implants. If relevant data are available, it may promote the rapid progress of related research. Second, the in vivo dynamic changes between F. nucleatum and other microorganisms during the maturation of peri-implant biofilms should be considered to illustrate the biofilm formation characteristics of F. nucleatum. Jiang et al. (2021) demonstrated the important role of F. nucleatum in biofilm formation by investigating the in vivo microbial shift using a canine model. However, the construction of animal models of peri-implant diseases does not seem to be unified at present because in the actual process of use, the implant has an upper repair structure, which cannot be achieved with animal models, thus lacking considerations such as mechanical factors. Not only F. nucleatum itself but also some outer membrane proteins in F. nucleatum, such as RadD and FomA, should be considered. Finally, how F. nucleatum interacts with the host during the evolution of peri-implant diseases, including host infection and host responses, is the most operative issue that needs to be addressed. The host immune microenvironment of peri-implant issues determines the microbial composition (Wang et al., 2021). Patients with different risk levels exhibit different immune microenvironments, and F. nucleatum is distinctly detected in high-risk individuals (Wang et al., 2021). Host-derived marker analyses have pointed out that IL-1β is positively correlated with the probing pocket depth in experimental peri-implant models (Monje et al., 2021). High expression of IL-1β is associated with significant enrichment of F. nucleatum in periodontal diseases secondary to neuroinflammation (Martinez et al., 2021). The Fusobacterium genus tends to have a relatively higher proportion in deeper peri-implant pockets (Polymeri et al., 2021). However, whether F. nucleatum stimulates the expression of IL-1β, causing the typical clinical symptoms of peri-implant diseases, remains unknown. Since the relationship between F. nucleatum and peri-implant diseases has garnered increasing attention recently, some new technologies targeting F. nucleatum have been developed to treat peri-implant diseases. A new brushing solution has been tested to inhibit the development of peri-implant biofilms and kill F. nucleatum, which may have a wide application foreground in the treatment of peri-implant diseases (Virto et al., 2022).
In conclusion, our review summarizes the specific mechanism of F. nucleatum in periodontitis and analyzes the resemblances and discrepancies between periodontal diseases and peri-implant diseases. We propose a conjecture for the important role of F. nucleatum in periodontal diseases and provide an idea and direction for exploring the pathogenesis of peri-implant diseases. More research is still needed on how F. nucleatum affects the pathogenesis of peri-implant diseases.
Author Contributions
DY and YC conceived the idea and edited the manuscript. YC and TS collected information and drafted the manuscript. YL and LH assisted in assessing the study quality and reviewing the manuscript. YC, DY, TS, YL, and LH revised the manuscript. All authors contributed to the article and approved the submitted version.
Funding
This work was supported by Research Funding for Talent Development, West China Hospital of Stomatology, Sichuan University (RCDWJS2021-6) and Research and Development Funding, West China Hospital of Stomatology, Sichuan University (RD-02-202009).
Conflict of Interest
The authors declare that the research was conducted in the absence of any commercial or financial relationships that could be construed as a potential conflict of interest.
Publisher’s Note
All claims expressed in this article are solely those of the authors and do not necessarily represent those of their affiliated organizations, or those of the publisher, the editors and the reviewers. Any product that may be evaluated in this article, or claim that may be made by its manufacturer, is not guaranteed or endorsed by the publisher.
References
Ahn, S. H., Cho, S. H., Song, J. E., Kim, S., Oh, S. S., Jung, S., et al. (2017a). Caveolin-1 serves as a negative effector in senescent human gingival fibroblasts during Fusobacterium nucleatum infection. Mol Oral Microbiol 32, 236–249. doi: 10.1111/omi.12167
Ahn, S. H., Chun, S., Park, C., Lee, J. H., Lee, S. W., and Lee, T. H. (2017b). Transcriptome profiling analysis of senescent gingival fibroblasts in response to Fusobacterium nucleatum infection. PLoS One 12:e0188755. doi: 10.1371/journal.pone.0188755
Albrektsson, T., Canullo, L., Cochran, D., and De Bruyn, H. (2016). “Peri-Implantitis”: a complication of a foreign body or a man-made “disease”. Facts and fiction. Clin. Implant. Dent. Relat. Res. 18, 840–849. doi: 10.1111/cid.12427
Arciola, C. R., Campoccia, D., and Montanaro, L. (2018). Implant infections: adhesion, biofilm formation and immune evasion. Nat. Rev. Microbiol. 16, 397–409. doi: 10.1038/s41579-018-0019-y
Belibasakis, G. N. (2014). Microbiological and immuno-pathological aspects of peri-implant diseases. Arch. Oral Biol. 59, 66–72. doi: 10.1016/j.archoralbio.2013.09.013
Belibasakis, G. N., and Manoil, D. (2021). Microbial community-driven etiopathogenesis of peri-implantitis. J. Dent. Res. 100, 21–28. doi: 10.1177/0022034520949851
Berglundh, T., Armitage, G., Araujo, M. G., Avila-Ortiz, G., Blanco, J., Camargo, P. M., et al. (2018a). Peri-implant diseases and conditions: consensus report of workgroup 4 of the 2017 world workshop on the classification of periodontal and peri-implant diseases and conditions. J. Clin. Periodontol. 45, S286–S291. doi: 10.1111/jcpe.12957
Berglundh, T., Armitage, G., Araujo, M. G., Avila-Ortiz, G., Blanco, J., Camargo, P. M., et al. (2018b). Peri-implant diseases and conditions: consensus report of workgroup 4 of the 2017 world workshop on the classification of periodontal and peri-implant diseases and conditions. J. Periodontol. 89(Suppl 1), S313–S318. doi: 10.1002/JPER.17-0739
Berglundh, T., Lindhe, J., Ericsson, I., Marinello, C., Liljenberg, B., and Thomsen, P. (1991). The soft tissue barrier at implants and teeth. Clin. Oral Implants Res. 2, 81–90. doi: 10.1034/j.1600-0501.1991.020206.x
Bhattacharyya, S., Ghosh, S. K., Shokeen, B., Eapan, B., Lux, R., Kiselar, J., et al. (2016). FAD-I, a Fusobacterium nucleatum cell wall-associated diacylated lipoprotein that mediates human beta defensin 2 induction through toll-like receptor-1/2 (TLR-1/2) and TLR-2/6. Infect. Immun. 84, 1446–1456. doi: 10.1128/IAI.01311-15
Bower, R. C., Radny, N. R., Wall, C. D., and Henry, P. J. (1989). Clinical and microscopic findings in edentulous patients 3 years after incorporation of osseointegrated implant-supported bridgework. J. Clin. Periodontol. 16, 580–587. doi: 10.1111/j.1600-051x.1989.tb02141.x
Brennan, C. A., and Garrett, W. S. (2019). Fusobacterium nucleatum—symbiont, opportunist and oncobacterium. Nat. Rev. Microbiol. 17, 156–166. doi: 10.1038/s41579-018-0129-6
Buser, D., Sennerby, L., and De Bruyn, H. (2017). Modern implant dentistry based on osseointegration: 50 years of progress, current trends and open questions. Periodontol. 73(1), 7–21. doi: 10.1111/prd.12185
Busscher, H. J., Rinastiti, M., Siswomihardjo, W., and van der Mei, H. C. (2010). Biofilm formation on dental restorative and implant materials. J. Dent. Res. 89, 657–665. doi: 10.1177/0022034510368644
Carcuac, O., and Berglundh, T. (2014). Composition of human peri-implantitis and periodontitis lesions. J. Dent. Res. 93, 1083–1088. doi: 10.1177/0022034514551754
Charalampakis, G., and Belibasakis, G. N. (2015). Microbiome of peri-implant infections: lessons from conventional, molecular and metagenomic analyses. Virulence 6, 183–187. doi: 10.4161/21505594.2014.980661
Chaushu, S., Wilensky, A., Gur, C., Shapira, L., Elboim, M., Halftek, G., et al. (2012). Direct recognition of Fusobacterium nucleatum by the NK cell natural cytotoxicity receptor NKp46 aggravates periodontal disease. PLoS Pathog. 8:e1002601. doi: 10.1371/journal.ppat.1002601
Chung, P. Y., and Khanum, R. (2017). Antimicrobial peptides as potential anti-biofilm agents against multidrug-resistant bacteria. J. Microbiol. Immunol. Infect. 50, 405–410. doi: 10.1016/j.jmii.2016.12.005
Curtis, M. A., Diaz, P. I., and Van Dyke, T. E. (2020). The role of the microbiota in periodontal disease. Periodontol. 83, 14–25. doi: 10.1111/prd.12296
Dabija-Wolter, G., Cimpan, M. R., Costea, D. E., Johannessen, A. C., Sornes, S., Neppelberg, E., et al. (2009). Fusobacterium nucleatum enters normal human oral fibroblasts in vitro. J. Periodontol. 80, 1174–1183. doi: 10.1902/jop.2009.090051
De Andrade, K. Q., Almeida-da-Silva, C. L. C., Ojcius, D. M., and Coutinho-Silva, R. (2021). Differential involvement of the canonical and noncanonical inflammasomes in the immune response against infection by the periodontal bacteria Porphyromonas gingivalis and Fusobacterium nucleatum. Curr. Res. Microb. Sci. 2:100023. doi: 10.1016/j.crmicr.2021.100023
de Waal, Y. C., Eijsbouts, H. V., Winkel, E. G., and van Winkelhoff, A. J. (2017). Microbial characteristics of peri-implantitis: a case-control study. J. Periodontol. 88, 209–217. doi: 10.1902/jop.2016.160231
Del Pozo, J. L. (2018). Biofilm-related disease. Expert Rev. Anti-Infect. Ther. 16, 51–65. doi: 10.1080/14787210.2018.1417036
Dionigi, C., Larsson, L., Carcuac, O., and Berglundh, T. (2020). Cellular expression of DNA damage/repair and reactive oxygen/nitrogen species in human periodontitis and peri-implantitis lesions. J. Clin. Periodontol. 47, 1466–1475. doi: 10.1111/jcpe.13370
Dukka, H., Saleh, M. H. A., Ravida, A., Greenwell, H., and Wang, H. L. (2021). Is bleeding on probing a reliable clinical indicator of peri-implant diseases? J. Periodontol. 92, 1669–1674. doi: 10.1002/JPER.20-0890
Edwards, A. M., Grossman, T. J., and Rudney, J. D. (2006). Fusobacterium nucleatum transports noninvasive Streptococcus cristatus into human epithelial cells. Infect. Immun. 74, 654–662. doi: 10.1128/IAI.74.1.654-662.2006
Edwards, A. M., Grossman, T. J., and Rudney, J. D. (2007). Association of a high-molecular weight arginine-binding protein of Fusobacterium nucleatum ATCC 10953 with adhesion to secretory immunoglobulin A and coaggregation with Streptococcus cristatus. Oral Microbiol. Immunol. 22, 217–224. doi: 10.1111/j.1399-302X.2006.00343.x
Engevik, M. A., Danhof, H. A., Auchtung, J., Endres, B. T., Ruan, W., Basseres, E., et al. (2021). Fusobacterium nucleatum adheres to Clostridioides difficile via the RadD adhesin to enhance biofilm formation in intestinal mucus. Gastroenterology 160, 1301–1314.e8. doi: 10.1053/j.gastro.2020.11.034
Fardini, Y., Wang, X., Temoin, S., Nithianantham, S., Lee, D., Shoham, M., et al. (2011). Fusobacterium nucleatum adhesin FadA binds vascular endothelial cadherin and alters endothelial integrity. Mol. Microbiol. 82, 1468–1480. doi: 10.1111/j.1365-2958.2011.07905.x
Gatti, C., Gatti, F., Chiapasco, M., and Esposito, M. (2008). Outcome of dental implants in partially edentulous patients with and without a history of periodontitis: a 5-year interim analysis of a cohort study. Eur. J. Oral Implantol. 1, 45–51. doi: 10.1259/dmfr/75888462
Ghensi, P., Manghi, P., Zolfo, M., Armanini, F., Pasolli, E., Bolzan, M., et al. (2020). Strong oral plaque microbiome signatures for dental implant diseases identified by strain-resolution metagenomics. NPJ Biofilms Microbiomes 6:47. doi: 10.1038/s41522-020-00155-7
Ghosh, S. K., Feng, Z., Fujioka, H., Lux, R., McCormick, T. S., and Weinberg, A. (2018). Conceptual perspectives: bacterial antimicrobial peptide induction as a novel strategy for symbiosis with the human host. Front. Microbiol. 9:302. doi: 10.3389/fmicb.2018.00302
Gualini, F., and Berglundh, T. (2003). Immunohistochemical characteristics of inflammatory lesions at implants. J. Clin. Periodontol. 30, 14–18. doi: 10.1034/j.1600-051x.2003.300103.x
Guo, L., Shokeen, B., He, X., Shi, W., and Lux, R. (2017). Streptococcus mutans SpaP binds to RadD of Fusobacterium nucleatum ssp. polymorphum. Mol Oral Microbiol 32, 355–364. doi: 10.1111/omi.12177
Gupta, S., Ghosh, S. K., Scott, M. E., Bainbridge, B., Jiang, B., Lamont, R. J., et al. (2010). Fusobacterium nucleatum-associated beta-defensin inducer (FAD-I): identification, isolation, and functional evaluation. J. Biol. Chem. 285, 36523–36531. doi: 10.1074/jbc.M110.133140
Gurlek, O., Gumus, P., Nile, C. J., Lappin, D. F., and Buduneli, N. (2017). Biomarkers and bacteria around implants and natural teeth in the same individuals. J. Periodontol. 88, 752–761. doi: 10.1902/jop.2017.160751
Gursoy, U. K., Pollanen, M., Kononen, E., and Uitto, V. J. (2010). Biofilm formation enhances the oxygen tolerance and invasiveness of Fusobacterium nucleatum in an oral mucosa culture model. J. Periodontol. 81, 1084–1091. doi: 10.1902/jop.2010.090664
Hallstrom, H., Persson, G. R., Lindgren, S., Olofsson, M., and Renvert, S. (2012). Systemic antibiotics and debridement of peri-implant mucositis. A randomized clinical trial. J. Clin. Periodontol. 39, 574–581. doi: 10.1111/j.1600-051X.2012.01884.x
Han, Y. W. (2015). Fusobacterium nucleatum: a commensal-turned pathogen. Curr. Opin. Microbiol. 23, 141–147. doi: 10.1016/j.mib.2014.11.013
Han, Y. W., Ikegami, A., Rajanna, C., Kawsar, H. I., Zhou, Y., Li, M., et al. (2005). Identification and characterization of a novel adhesin unique to oral fusobacteria. J. Bacteriol. 187, 5330–5340. doi: 10.1128/JB.187.15.5330-5340.2005
Han, Y. W., Shi, W., Huang, G. T., Haake, S. K., Park, N. H., Kuramitsu, H., et al. (2000). Interactions between periodontal bacteria and human oral epithelial cells: Fusobacterium nucleatum adheres to and invades epithelial cells. Infect. Immun. 68, 3140–3146. doi: 10.1128/IAI.68.6.3140-3146.2000
Handley, P., Coykendall, A., Beighton, D., Hardie, J. M., and Whiley, R. A. (1991). Streptococcus crista sp. nov., a viridans streptococcus with tufted fibrils, isolated from the human oral cavity and throat. Int. J. Syst. Bacteriol. 41, 543–547. doi: 10.1099/00207713-41-4-543
Heitz-Mayfield, L. J. A., and Salvi, G. E. (2018). Peri-implant mucositis. J. Clin. Periodontol. 45, S237–S245. doi: 10.1111/jcpe.12953
Horiuchi, A., Kokubu, E., Warita, T., and Ishihara, K. (2020). Synergistic biofilm formation by Parvimonas micra and Fusobacterium nucleatum. Anaerobe 62:102100. doi: 10.1016/j.anaerobe.2019.102100
Ivanovski, S., and Lee, R. (2018). Comparison of peri-implant and periodontal marginal soft tissues in health and disease. Periodontol. 76, 116–130. doi: 10.1111/prd.12150
Jang, J. Y., Baek, K. J., Choi, Y., and Ji, S. (2017). Relatively low invasive capacity of Porphyromonas gingivalis strains into human gingival fibroblasts in vitro. Arch. Oral Biol. 83, 265–271. doi: 10.1016/j.archoralbio.2017.08.007
Jiang, Q., Yu, Y., Xu, R., Zhang, Z., Liang, C., Sun, H., et al. (2021). The temporal shift of peri-implant microbiota during the biofilm formation and maturation in a canine model. Microb. Pathog. 158:105100. doi: 10.1016/j.micpath.2021.105100
Johnson, L., Almeida-da-Silva, C. L. C., Takiya, C. M., Figliuolo, V., Rocha, G. M., Weissmuller, G., et al. (2018). Oral infection of mice with Fusobacterium nucleatum results in macrophage recruitment to the dental pulp and bone resorption. Biom. J. 41, 184–193. doi: 10.1016/j.bj.2018.05.001
Kang, W., Jia, Z., Tang, D., Zhang, Z., Gao, H., He, K., et al. (2019). Fusobacterium nucleatum facilitates apoptosis, ROS generation, and inflammatory cytokine production by activating AKT/MAPK and NF-kappaB signaling pathways in human gingival fibroblasts. Oxidative Med. Cell. Longev. 2019:1681972. doi: 10.1155/2019/1681972
Kaplan, C. W., Lux, R., Haake, S. K., and Shi, W. (2009). The Fusobacterium nucleatum outer membrane protein RadD is an arginine-inhibitable adhesin required for inter-species adherence and the structured architecture of multispecies biofilm. Mol. Microbiol. 71, 35–47. doi: 10.1111/j.1365-2958.2008.06503.x
Kaplan, C. W., Ma, X., Paranjpe, A., Jewett, A., Lux, R., Kinder-Haake, S., et al. (2010). Fusobacterium nucleatum outer membrane proteins Fap2 and RadD induce cell death in human lymphocytes. Infect. Immun. 78, 4773–4778. doi: 10.1128/IAI.00567-10
Kinane, D. F., Stathopoulou, P. G., and Papapanou, P. N. (2017). Periodontal diseases. Nat. Rev. Dis. Primers 3:17038. doi: 10.1038/nrdp.2017.38
Kinder, S. A., and Holt, S. C. (1993). Localization of the Fusobacterium nucleatum T18 adhesin activity mediating coaggregation with Porphyromonas gingivalis T22. J. Bacteriol. 175, 840–850. doi: 10.1128/jb.175.3.840-850.1993
Kolenbrander, P. E., Palmer, R. J., Periasamy, S., and Jakubovics, N. S. (2010). Oral multispecies biofilm development and the key role of cell-cell distance. Nat. Rev. Microbiol. 8, 471–480. doi: 10.1038/nrmicro2381
Kotsakis, G., and Olmedo, D. J. P. (2021). Peri-implantitis is not periodontitis: Scientific discoveries shed light on microbiome-biomaterial interactions that may determine disease phenotype. Periodontol. 86, 231–240. doi: 10.1111/prd.12372
Krisanaprakornkit, S., Kimball, J. R., Weinberg, A., Darveau, R. P., Bainbridge, B. W., and Dale, B. A. (2000). Inducible expression of human beta-defensin 2 by Fusobacterium nucleatum in oral epithelial cells: multiple signaling pathways and role of commensal bacteria in innate immunity and the epithelial barrier. Infect. Immun. 68, 2907–2915. doi: 10.1128/IAI.68.5.2907-2915.2000
Lamont, R. J., Koo, H., and Hajishengallis, G. (2018). The oral microbiota: dynamic communities and host interactions. Nat. Rev. Microbiol. 16, 745–759. doi: 10.1038/s41579-018-0089-x
Lee, J. C. Y., Mattheos, N., Nixon, K. C., and Ivanovski, S. (2012). Residual periodontal pockets are a risk indicator for peri-implantitis in patients treated for periodontitis. Clin. Oral Implants Res. 23, 325–333. doi: 10.1111/j.1600-0501.2011.02264.x
Leonhardt, A., Dahlen, G., and Renvert, S. (2003). Five-year clinical, microbiological, and radiological outcome following treatment of peri-implantitis in man. J. Periodontol. 74, 1415–1422. doi: 10.1902/jop.2003.74.10.1415
Leonhardt, A., Renvert, S., and Dahlen, G. (1999). Microbial findings at failing implants. Clin. Oral Implants Res. 10, 339–345. doi: 10.1034/j.1600-0501.1999.100501.x
Lima, B. P., Shi, W., and Lux, R. (2017). Identification and characterization of a novel Fusobacterium nucleatum adhesin involved in physical interaction and biofilm formation with Streptococcus gordonii. Microbiology 6:e00444. doi: 10.1002/mbo3.444
Liu, P., Liu, Y., Wang, J., Guo, Y., Zhang, Y., and Xiao, S. (2014). Detection of Fusobacterium nucleatum and fadA adhesin gene in patients with orthodontic gingivitis and non-orthodontic periodontal inflammation. PLoS One 9:e85280. doi: 10.1371/journal.pone.0085280
Lopez, N. J., Socransky, S. S., Da Silva, I., Japlit, M. R., and Haffajee, A. D. (2006). Effects of metronidazole plus amoxicillin as the only therapy on the microbiological and clinical parameters of untreated chronic periodontitis. J. Clin. Periodontol. 33, 648–660. doi: 10.1111/j.1600-051X.2006.00957.x
Magana, M., Pushpanathan, M., Santos, A. L., Leanse, L., Fernandez, M., Ioannidis, A., et al. (2020). The value of antimicrobial peptides in the age of resistance. Lancet Infect. Dis. 20, e216–e230. doi: 10.1016/S1473-3099(20)30327-3
Martinez, M., Martin-Hernandez, D., Virto, L., MacDowell, K. S., Montero, E., Gonzalez-Bris, A., et al. (2021). Periodontal diseases and depression: a pre-clinical in vivo study. J. Clin. Periodontol. 48, 503–527. doi: 10.1111/jcpe.13420
Maximo, M. B., de Mendonca, A. C., Renata Santos, V., Figueiredo, L. C., Feres, M., and Duarte, P. M. (2009). Short-term clinical and microbiological evaluations of peri-implant diseases before and after mechanical anti-infective therapies. Clin. Oral Implants Res. 20, 99–108. doi: 10.1111/j.1600-0501.2008.01618.x
Meng, Q., Gao, Q., Mehrazarin, S., Tangwanichgapong, K., Wang, Y., Huang, Y., et al. (2021). Fusobacterium nucleatum secretes amyloid-like FadA to enhance pathogenicity. EMBO Rep. 22:e52891. doi: 10.15252/embr.202152891
Meyer, S., Giannopoulou, C., Courvoisier, D., Schimmel, M., Muller, F., and Mombelli, A. (2017). Experimental mucositis and experimental gingivitis in persons aged 70 or over. Clinical and biological responses. Clin. Oral Implants Res. 28, 1005–1012. doi: 10.1111/clr.12912
Mombelli, A., and Decaillet, F. (2011). The characteristics of biofilms in peri-implant disease. J. Clin. Periodontol. 38, 203–213. doi: 10.1111/j.1600-051X.2010.01666.x
Monje, A., Eick, S., Buser, D., and Salvi, G. E. (2021). Microbial and host-derived biomarker changes during ligature-induced and spontaneous peri-implantitis in the beagle dog. J. Periodontal Res. 56, 93–100. doi: 10.1111/jre.12797
Moon, I., Berglundh, T., Abrahamsson, I., Linder, E., Lindhe, J. J. J., and o.c.p., (1999). The barrier between the keratinized mucosa and the dental implant. An experimental study in the dog. J. Clin. Periodontol. 26, 658–663. doi: 10.1034/j.1600-051x.1999.261005.x
Naruishi, K., and Nagata, T. (2018). Biological effects of interleukin-6 on gingival fibroblasts: cytokine regulation in periodontitis. J. Cell. Physiol. 233, 6393–6400. doi: 10.1002/jcp.26521
Nithianantham, S., Xu, M., Yamada, M., Ikegami, A., Shoham, M., and Han, Y. W. (2009). Crystal structure of FadA adhesin from Fusobacterium nucleatum reveals a novel oligomerization motif, the leucine chain. J. Biol. Chem. 284, 3865–3872. doi: 10.1074/jbc.M805503200
Papathanasiou, E., Finkelman, M., Hanley, J., and Parashis, A. O. (2016). Prevalence, etiology and treatment of peri-implant mucositis and peri-implantitis: a survey of periodontists in the United States. J. Periodontol. 87, 493–501. doi: 10.1902/jop.2015.150476
Persson, G. R., and Renvert, S. (2014). Cluster of bacteria associated with peri-implantitis. Clin. Implant. Dent. Relat. Res. 16, 783–793. doi: 10.1111/cid.12052
Pollanen, M. T., Gursoy, U. K., Kononen, E., and Uitto, V. J. (2012). Fusobacterium nucleatum biofilm induces epithelial migration in an organotypic model of dento-gingival junction. J. Periodontol. 83, 1329–1335. doi: 10.1902/jop.2012.110535
Polymeri, A., van der Horst, J., Buijs, M. J., Zaura, E., Wismeijer, D., Crielaard, W., et al. (2021). Submucosal microbiome of peri-implant sites: a cross-sectional study. J. Clin. Periodontol. 48, 1228–1239. doi: 10.1111/jcpe.13502
Renvert, S., Persson, G. R., Pirih, F. Q., and Camargo, P. M. (2018). Peri-implant health, peri-implant mucositis, and peri-implantitis: case definitions and diagnostic considerations. J. Periodontol. 89, S304–S312. doi: 10.1002/JPER.17-0588
Reyes, L. (2021). Porphyromonas gingivalis. Trends Microbiol. 29, 376–377. doi: 10.1016/j.tim.2021.01.010
Ritzer, J., Luhmann, T., Rode, C., Pein-Hackelbusch, M., Immohr, I., Schedler, U., et al. (2017). Diagnosing peri-implant disease using the tongue as a 24/7 detector. Nat. Commun. 8:264. doi: 10.1038/s41467-017-00340-x
Rosen, G., Genzler, T., and Sela, M. N. (2008). Coaggregation of Treponema denticola with Porphyromonas gingivalis and Fusobacterium nucleatum is mediated by the major outer sheath protein of Treponema denticola. FEMS Microbiol. Lett. 289, 59–66. doi: 10.1111/j.1574-6968.2008.01373.x
Rosen, G., and Sela, M. N. (2006). Coaggregation of Porphyromonas gingivalis and Fusobacterium nucleatum PK 1594 is mediated by capsular polysaccharide and lipopolysaccharide. FEMS Microbiol. Lett. 256, 304–310. doi: 10.1111/j.1574-6968.2006.00131.x
Rubinstein, M. R., Baik, J. E., Lagana, S. M., Han, R. P., Raab, W. J., Sahoo, D., et al. (2019). Fusobacterium nucleatum promotes colorectal cancer by inducing Wnt/beta-catenin modulator Annexin A1. EMBO Rep. 20:e47638. doi: 10.15252/embr.201847638
Sahrmann, P., Gilli, F., Wiedemeier, D. B., Attin, T., Schmidlin, P. R., and Karygianni, L. (2020). The microbiome of peri-implantitis: a systematic review and meta-analysis. Microorganisms 8, 661. doi: 10.3390/microorganisms8050661
Salvi, G. E., Cosgarea, R., and Sculean, A. (2017). Prevalence and mechanisms of peri-implant diseases. J. Dent. Res. 96, 31–37. doi: 10.1177/0022034516667484
Sanchez, M. C., Llama-Palacios, A., Blanc, V., Leon, R., Herrera, D., and Sanz, M. (2011). Structure, viability and bacterial kinetics of an in vitro biofilm model using six bacteria from the subgingival microbiota. J. Periodontal Res. 46, 252–260. doi: 10.1111/j.1600-0765.2010.01341.x
Sandhu, B. K., and McBride, S. M. (2018). Clostridioides difficile. Trends Microbiol. 26, 1049–1050. doi: 10.1016/j.tim.2018.09.004
Sanz-Martin, I., Doolittle-Hall, J., Teles, R. P., Patel, M., Belibasakis, G. N., Hammerle, C. H. F., et al. (2017). Exploring the microbiome of healthy and diseased peri-implant sites using Illumina sequencing. J. Clin. Periodontol. 44, 1274–1284. doi: 10.1111/jcpe.12788
Schincaglia, G. P., Hong, B. Y., Rosania, A., Barasz, J., Thompson, A., Sobue, T., et al. (2017). Clinical, immune, and microbiome traits of gingivitis and peri-implant mucositis. J. Dent. Res. 96, 47–55. doi: 10.1177/0022034516668847
Schwarz, F., Derks, J., Monje, A., and Wang, H. L. (2018). Peri-implantitis. J. Periodontol. 89, S267–S290. doi: 10.1002/JPER.16-0350
Smith, M. M., Knight, E. T., Al-Harthi, L., and Leichter, J. W. (2017). Chronic periodontitis and implant dentistry. Periodontol 2000, 63–73. doi: 10.1111/prd.12190
Stokowa-Soltys, K., Wojtkowiak, K., and Jagiello, K. (2021). Fusobacterium nucleatum—Friend or foe? J. Inorg. Biochem. 224:111586. doi: 10.1016/j.jinorgbio.2021.111586
Tamura, N., Ochi, M., Miyakawa, H., and Nakazawa, F. (2013). Analysis of bacterial flora associated with peri-implantitis using obligate anaerobic culture technique and 16S rDNA gene sequence. Int. J. Oral Maxillofac. Implants 28, 1521–1529. doi: 10.11607/jomi.2570
Tarnow, D. P. (2016). Increasing prevalence of peri-implantitis: how will we manage? J. Dent. Res. 95, 7–8. doi: 10.1177/0022034515616557
Temoin, S., Wu, K. L., Wu, V., Shoham, M., and Han, Y. W. (2012). Signal peptide of FadA adhesin from Fusobacterium nucleatum plays a novel structural role by modulating the filament’s length and width. FEBS Lett. 586, 1–6. doi: 10.1016/j.febslet.2011.10.047
Teughels, W., Van Assche, N., Sliepen, I., and Quirynen, M. (2006). Effect of material characteristics and/or surface topography on biofilm development. Clin. Oral Implants Res. 17, 68–81. doi: 10.1111/j.1600-0501.2006.01353.x
Thurnheer, T., Karygianni, L., Flury, M., and Belibasakis, G. N. (2019). Fusobacterium species and subspecies differentially affect the composition and architecture of supra- and subgingival biofilms models. Front. Microbiol. 10:1716. doi: 10.3389/fmicb.2019.01716
Ting, M., Craig, J., Balkin, B. E., and Suzuki, J. B. (2018). Peri-implantitis: a comprehensive overview of systematic reviews. J. Oral Implantol. 44, 225–247. doi: 10.1563/aaid-joi-D-16-00122
Tonon, C. C., Panariello, B. H. D., Spolidorio, D. M. P., Gossweiler, A. G., and Duarte, S. (2021). Anti-biofilm effect of ozonized physiological saline solution on peri-implant-related biofilm. J. Periodontol. 92, 1151–1162. doi: 10.1002/Jper.20-0333
Virto, L., Simoes-Martins, D., Sanchez, M. C., Encinas, A., Sanz, M., and Herrera, D. (2022). Antimicrobial effects of a new brushing solution concept on a multispecies in vitro biofilm model growing on titanium surfaces. Clin. Oral Implants Res. 33, 209–220. doi: 10.1111/clr.13884
Wang, C. W., Hao, Y., Di Gianfilippo, R., Sugai, J., Li, J., Gong, W., et al. (2021). Machine learning-assisted immune profiling stratifies peri-implantitis patients with unique microbial colonization and clinical outcomes. Theranostics 11, 6703–6716. doi: 10.7150/thno.57775
Wassing, G. M., Ilehag, N., Frey, J., and Jonsson, A. B. (2021). Modulation of human beta-defensin 2 expression by pathogenic Neisseria meningitidis and commensal lactobacilli. Antimicrob. Agents Chemother. 65, e02002–e02020. doi: 10.1128/AAC.02002-20
Wei, J., Qiao, S., Zhang, X., Li, Y., Zhang, Y., Wei, S., et al. (2021). Graphene-reinforced titanium enhances soft tissue seal. Front. Bioeng. Biotechnol. 9:665305. doi: 10.3389/fbioe.2021.665305
Wu, T., Cen, L., Kaplan, C., Zhou, X., Lux, R., Shi, W., et al. (2015). Cellular components mediating coadherence of Candida albicans and Fusobacterium nucleatum. J. Dent. Res. 94, 1432–1438. doi: 10.1177/0022034515593706
Wu, C., Chen, Y. W., Scheible, M., Chang, C., Wittchen, M., Lee, J. H., et al. (2021). Genetic and molecular determinants of polymicrobial interactions in Fusobacterium nucleatum. Proc. Natl. Acad. Sci. U. S. A. 118:e2006482118. doi: 10.1073/pnas.2006482118
Xu, M., Yamada, M., Li, M., Liu, H., Chen, S. G., and Han, Y. W. (2007). FadA from Fusobacterium nucleatum utilizes both secreted and nonsecreted forms for functional oligomerization for attachment and invasion of host cells. J. Biol. Chem. 282, 25000–25009. doi: 10.1074/jbc.M611567200
Yeh, H. C., Lu, J. J., Chang, S. C., and Ge, M. C. (2019). Identification of microbiota in peri-implantitis pockets by matrix-assisted laser desorption/ionization time-of-flight mass spectrometry. Sci. Rep. 9:774. doi: 10.1038/s41598-018-37450-5
Yin, L., and Dale, B. A. (2007). Activation of protective responses in oral epithelial cells by Fusobacterium nucleatum and human beta-defensin-2. J. Med. Microbiol. 56, 976–987. doi: 10.1099/jmm.0.47198-0
Yu, X. L., Chan, Y., Zhuang, L., Lai, H. C., Lang, N. P., Leung, W. K., et al. (2019). Intra-oral single-site comparisons of periodontal and peri-implant microbiota in health and disease. Clin. Oral Implants Res. 30, 760–776. doi: 10.1111/clr.13459
Yuan, X., Pei, X., Chen, J., Zhao, Y., Brunski, J. B., and Helms, J. A. (2021). Comparative analyses of the soft tissue interfaces around teeth and implants: insights from a pre-clinical implant model. J. Clin. Periodontol. 48, 745–753. doi: 10.1111/jcpe.13446
Zhang, Z., Liu, S., Zhang, S., Li, Y., Shi, X., Liu, D., et al. (2021). Porphyromonas gingivalis outer membrane vesicles inhibit the invasion of Fusobacterium nucleatum into oral epithelial cells by downregulating FadA and FomA. J. Periodontol. doi: 10.1002/JPER.21-0144 [Epub ahead of print]
Zhang, Y., Shi, W., Song, Y., and Wang, J. (2019). Metatranscriptomic analysis of an in vitro biofilm model reveals strain-specific interactions among multiple bacterial species. J. Oral Microbiol. 11:1599670. doi: 10.1080/20002297.2019.1599670
Keywords: Fusobacterium nucleatum, periodontal diseases, peri-implant diseases, oral microbiology, pathogeny
Citation: Chen Y, Shi T, Li Y, Huang L and Yin D (2022) Fusobacterium nucleatum: The Opportunistic Pathogen of Periodontal and Peri-Implant Diseases. Front. Microbiol. 13:860149. doi: 10.3389/fmicb.2022.860149
Edited by:
Axel Cloeckaert, Institut National de recherche pour l’agriculture, l’alimentation et l’environnement (INRAE), FranceReviewed by:
Marwan Mansoor Ali Mohammed, University of Sharjah, United Arab EmiratesSasanka Chukkapalli, Texas A&M University College Station, United States
Eija Könönen, University of Turku, Finland
Xuesong He, The Forsyth Institute, United States
Cassio Almeida-da-Silva, University of the Pacific, United States
Copyright © 2022 Chen, Shi, Li, Huang and Yin. This is an open-access article distributed under the terms of the Creative Commons Attribution License (CC BY). The use, distribution or reproduction in other forums is permitted, provided the original author(s) and the copyright owner(s) are credited and that the original publication in this journal is cited, in accordance with accepted academic practice. No use, distribution or reproduction is permitted which does not comply with these terms.
*Correspondence: Derong Yin, ZGVyb25neWluQHNjdS5lZHUuY24=