- 1Department of General Surgery, Nanjing Drum Tower Hospital, The Affiliated Hospital of Nanjing University Medical School, Nanjing, Jiangsu, China
- 2Laboratory Medicine Center, The Second Affiliated Hospital of Nanjing Medical University, Nanjing, Jiangsu, China
- 3Department of General Surgery, Nanjing Drum Tower Hospital Clinical College of Nanjing Medical University, Nanjing, Jiangsu, China
- 4Department of General Surgery, Nanjing Drum Tower Hospital Clinical College of Xuzhou Medical University, Nanjing, Jiangsu, China
Gastric cancer (GC) is a common cancer worldwide with a high mortality rate. Many microbial factors influence GC, of which the most widely accepted one is Helicobacter pylori (H. pylori) infection. H. pylori causes inflammation, immune reactions and activation of multiple signaling pathways, leading to acid deficiency, epithelial atrophy, dysplasia and ultimately GC. It has been proved that complex microbial populations exist in the human stomach. H. pylori can affect the abundance and diversity of other bacteria. The interactions among gastric microbiota are collectively implicated in the onset of GC. Certain intervention strategies may regulate gastric homeostasis and mitigate gastric disorders. Probiotics, dietary fiber, and microbiota transplantation can potentially restore healthy microbiota. In this review, we elucidate the specific role of the gastric microbiota in GC and hope these data can facilitate the development of effective prevention and therapeutic approaches for GC.
Introduction
Gastric cancer (GC) ranks fifth most common and third most deadly cancer globally (Rawla and Barsouk, 2019). Factors that induce gastric carcinogenesis include gastric microbiota, alcohol, smoking, and unhealthy dietary (Dong and Thrift, 2017; Zhao et al., 2017). Among many risk factors for GC, gastric microbiota act as an emerging one. Human gastric microbiota are subject-specific species and include a variety of bacteria. H. pylori is classified as a Class I risk factor for GC by the World Health Organization (WHO), and H. pylori infection is widely regarded as the strongest threat to GC (Wroblewski et al., 2010). H. pylori has a high infection rate and frequently colonized more than half of the world’s population. The infection of H. pylori usually occurs during childhood and will last for a lifetime (Malaty et al., 2002). H. pylori can disturb the human immune system and promote inflammation responses, leading to acid deficiency, epithelial atrophy, and dysplasia (Doorakkers et al., 2016). Diverse species more common than H. pylori have been found in gastric samples, such as Streptococcus, Prevotella, Veronella, Clostridium, Haemophilus, and Neisseria (Rajilic-Stojanovic et al., 2020). These gastrointestinal microbiota exhibit different biological functions, for instance, preventing the invasion of pathogens, digesting complex carbohydrates, regulating immune response, or regulating the central nervous system (Alarcón et al., 2017).
The process for analyzing the diversity of the gastric microbiota has undergone a change from culture-based methods to molecular assays. Early studies relied on culture-based analysis (Wang et al., 2020a). And the emergence of next-generation sequencing (NGS) enabled researchers to analyze the composition and function of microbiota in a diverse environment with higher throughput and resolution, mainly including targeted amplicon sequencing by 16S ribosomal RNA (rRNA) genes and shotgun metagenomics (Boers et al., 2019), providing fascinating insights into the human gastric microbiota. In this review, we mainly analyzed the basic composition of microbiota in the human stomach, illustrated the changes and interactions of gastric microbiota in GC, and discussed promising strategies to regulate gastric microbiota.
Composition of the gastric microbiota
In earlier times, Monstein et al. used temperature gradient gel electrophoresis of 16S rRNA amplicons to classify the gastric microbiota into three main phyla (Proteobacteria, Firmicutes, and Actinobacteria; Monstein et al., 2000). As high-throughput sequencing developed, more bacteria were found in the human stomach. G2 PhyloChip (16S rRNA chip) data revealed 44 bacterial phyla in the human stomach, of which 4 phyla dominate: Actinobacteria, Firmicutes, Bacteroidetes, and Proteobacteria (Maldonado-Contreras et al., 2011). Based on barcoded 16S pyrosequencing data indicated that the human stomach contains of five phyla: Actinobacteria, Firmicutes, Bacteroidetes, Proteobacteria, and Fusobacteria (Andersson et al., 2008; Figure 1). In addition,researchers found that it contained the most common genera for each phylum, such as Streptococcus (phylum Firmicutes), Neisseria and Haemophilus (Proteobacteria), as well as Prevotella and Porphyromonas (Bacteroidetes; Li et al., 2009).
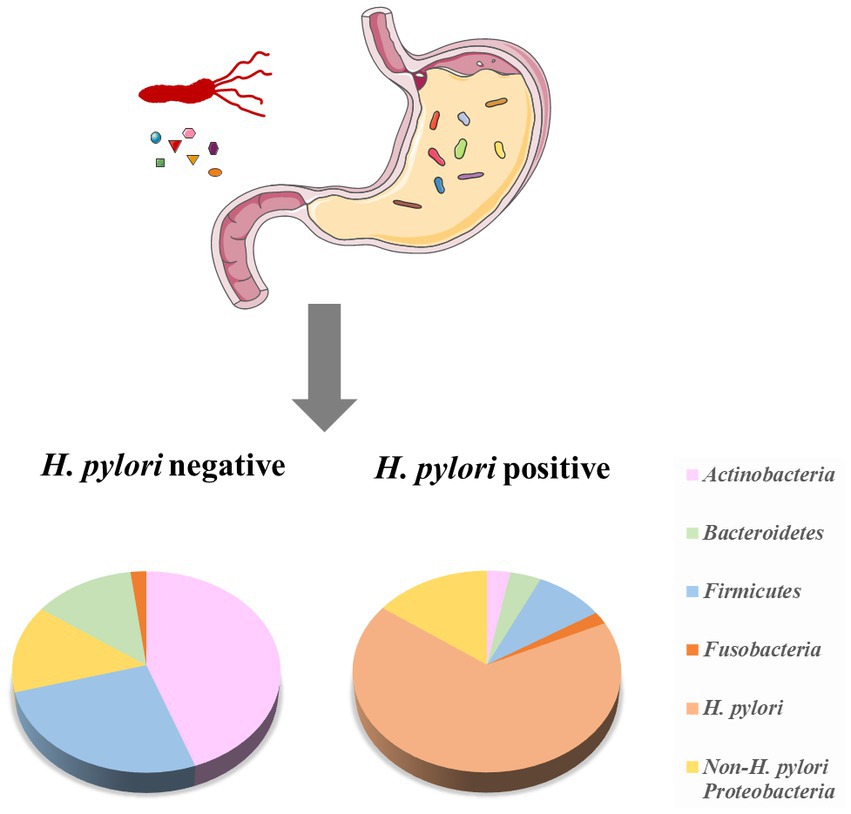
Figure 1. The composition of the human stomach microbiota and the effect of H. pylori on the microbiota. The microbiota of H. pylori (−) cases has higher diversity, with a higher relative abundance of Firmicutes and Actinobacteria. H. pylori (+) cases have a lower prevalence of Actinobacteria, Bacteroidetes, and Firmicutes, while increase Proteobacteria (adopted from Andersson et al., 2008 and Maldonado-Contreras et al., 2011).
H. pylori, a spiral-shaped flagellated bacterium belonging to the Proteobacteria phylum, is considered a constituent of the normal human gastric microbiome (Falush et al., 2003; Linz et al., 2007; Bruno et al., 2018). The remarkable survival capacity of H. pylori in the harsh gastric environment can be attributed to its motility and chemotaxis, which facilitate penetration of the mucus layer and colonization of epithelial cells (Amieva and Peek, 2016). H. pylori can hydrolyze urea and produce urease to increase the pH of its surrounding environment (Schulz et al., 2018).
Apart from H. pylori, many members other than H. pylori have been found in the stomach, including Streptococcus spp., Lactobacillus spp., Neisseria spp., Klebsiella spp., Escherichia coli, Rothia sp., Burkholderia pseudomallei., Bacillus sp., Morganella morganii, Acinetobacter sp., Haemophilus sp., Veillonella sp., Clostridium sp., Corynebacterium sp., Bacteroides sp., and Peptococcus sp. (Zilberstein et al., 2007; Khosravi et al., 2014; Schulz et al., 2018). Interestingly, some uncultured bacteria correlated with the extremophile Deinococcus and members of the enigmatic uncultured bacteria-TM7 group were detected in the stomachs of normal individuals (He et al., 2015; Ye et al., 2016), and another uncultured bacteria-SR1 phylum was also found in the normal stomach (Li et al., 2009).
Factors affecting gastric microbiota
Various factors are affecting the survival and function of gastric microbiota. The harsh environment in the stomach, which contains antibacterial enzymes, defensins, immunoglobulin, and high gastric acid, was a challenge for gastric microbiota (Zhang et al., 2017). These substances could effectively protect the host’s gastric mucosa from the attack of the microbiota. Low pH in the stomach cavity hinders the growth of the gastric microbiota. The hydrochloric acid secreted by parietal cells can convert pepsinogen into pepsin, an effective enzyme that denatures proteins and inhibits the survival of microbiota (Zhang et al., 2017). Immunoglobulin A (IgA) could prevent bacteria from penetrating the epithelial barrier and potentially maintain the diversity of normal gastrointestinal microbiota (Suzuki et al., 2004). Stomach commercial bacteria, especially S24-7, which belonged to Bacteroides, effectively induce the secretion of ILC2-dependent IgA. The secreted IgA is coated with other pathogenic bacteria (such as H. pylori) to prevent it from invading the epithelial mucosa, so as to maintain the gastric bacterial homeostasis (Satoh-Takayama et al., 2020). Children’s ILC2 is immature, unable to activate plasma cells to release enough IgA, resulting in H. pylori susceptibility (Ohno and Satoh-Takayama, 2020; Satoh-Takayama et al., 2020).Other antimicrobial compounds in gastric epithelial cells produced by the host, such as cathelicidins and C-type lectins, also had selective killing effects on microbiota (Walter and Ley, 2011).
Some external factors also influence the human gastric microbiota, including diet (Cires et al., 2016), antibiotics, proton pump inhibitor (PPI; Nardone and Compare, 2015; Tsuda et al., 2015), geography (Yang et al., 2016), and surgical intervention (Tseng et al., 2016). For instance, in the stomach of healthy cases, the most enriching family was Prevotellaceae, followed by Streptococcaceae, Paraprevotellaceae, and Fusobacteriaceae. While among patients who received PPI treatment, Streptococcaceae were the prevalent family, followed by Prevotellaceae, Campylobacteraceae, and Leptotrichiaceae (Parsons et al., 2017). Long-term application of PPI increases the intra-gastric pH, which allows the bacteria to reach the growth phase, resulting in increased bacterial load and increased bacterial translocation (Scarpignato et al., 2016), leading to a new bacterial balance, in which oral bacteria are significantly increased, such as Pepto-streptococcus stomatis, Parvimonas micra, Slackia exigua, Streptococcus anginosus, and Dialist pneumonitis (Bruno et al., 2019). Besides, the diversity of gastric microbiota increased significantly after subtotal gastrectomy. Helicobacter and Ralstonia were the two most abundant genera in stomachs before surgery, while Prevotella and Streptococcus were the two most prevalent bacteria after surgery (Tseng et al., 2016). The parietal cells that secrete gastric acid are mainly located in the stomach body and gastric antrum, so the gastric acid secretion is significantly reduced after distal gastrectomy, as well as highly selective vagotomy and bile reflux after surgery, all of which increase the pH value in the stomach and change the composition of bacteria.
Changes of gastric microbiota in gastric cancer
The dysbiosis of gastric microbiota may be responsible for gastric malignancies. The microbiota changes aggravated gastric environmental disorders and promoted the development of GC (Lofgren et al., 2011; Lertpiriyapong et al., 2014; Guo et al., 2022). The detailed changes in the bacterial community in GC patients were listed as follows (Table 1).
GC patients were more likely to suffer from acid deficiency, which may affect the colonization of microorganisms. In the early stages of GC, the relative abundance of H. pylori, Propionibacterium acnes, and Prevotella copri in the stomach was higher than that of non-cancer-bearing people (Gunathilake et al., 2019). However, Wang LL et al. found that unlike advanced gastric cancer, no significant biodiversity alteration was found in the early stage of gastric cancer (Wang L. et al., 2020). As cancer develops, the prevalence of H. pylori in the stomach may gradually decrease, and the overall microbial population in the stomach may also change. Dicksved et al. found a decreased abundance of H. pylori in the stomach of GC patients and a predominance of different species of the gastric microbial population, which include the genera Lactobacillus, Streptococcus, Prevotella, and Veillonella (Dicksved et al., 2009). Coker et al. found five taxa (Dialister pneumosintes, Parvimonas micra, Peptostreptococcus stomatis, Slackia exigua, and Streptococcus anginosus) as the core of the GC microbiota network (Coker et al., 2018). These studies revealed the difference in gastric microbiota profiles between GC patients and non-cancer-bearing people.
The gastric microbiota also showed different changes in patients with different histological stages from gastritis to GC. Ferreira et al. confirmed that the dysbiosis of gastric microbiota with potential genotoxicity existed in the GC patients, which differed from that of patients with chronic gastritis (CG; Ferreira et al., 2018). From non-atrophic gastritis and intestinal metaplasia (IM) to GC, the abundance of Neisseria, Porphyromonas, Streptococcus sinensis, and TM7 group showed a decreasing trend. In contrast the abundance of Lachnospiraceae and Lactobacillus coleohominis displayed an increasing trend (Aviles-Jimenez et al., 2014). In another study, compared with CG and IM groups, the abundance of Streptococcaceae and Bacilli increased at the class level, and the abundance of Helicobacter decreased at the family level in the stomach of the GC patients (Eun et al., 2014). In H. pylori-negative patients from atrophic gastritis (AG) to dysplasia (Dys) precancerous stage, the abundance of Burkholderiaceae continued to increase, while the abundance of Streptococcaceae and Prevotellaceae continued to decrease (Sun et al., 2022). Interestingly, some oral bacteria, genera Aggregatibacter, Alloprevotella, and Neisseria were abundant in GC patients compared with the superficial gastritis (SG) group. The relative abundance of these bacteria was completely separated between the two groups. This discovery suggested we can distinguish GC from SG patients based on any of the three genera detected in GC (Hu et al., 2018).
Previous studies have primarily focused on the abundance and diversity of the gastric microbiota in diverse patient cohorts. However, uncertain host factors may exert significant influence on research outcomes, thereby confounding interpretation of the results. To minimize the impact of these confounding factors, some investigators have adopted a paired design approach, wherein the profiles of the gastric microbiota in paired tumor tissues and non-malignant tissues from the same GC patient are comparatively analyzed in greater detail. Seo et al. detected 350 bacterial species from paired cancer and non-cancer biopsies among 16 GC patients by 16S rRNA gene sequencing. Compared with non-cancer tissue, the populations of Prevotella spp. and Clostridium spp. were increased, while H. pylori, Propionibacterium spp., Staphylococcus spp., and Corynebacterium spp. were decreased in cancer biopsies (Seo et al., 2014; Dai et al., 2021). In a study of carcinoma and adjacent tissues from 276 GC patients, genera Halomonas, Shewanella, and Helicobacter were enriched in the tumor-adjacent tissues, while Corynebacterium, Fusobacterium, Selenomonas, Propionibacterium, and Streptococcus were enriched in the carcinoma tissues. Similar to the previous reports, the community of H. pylori was also significantly reduced in the tumoral sites (Liu X. et al., 2019). The significant reduction of H. pylori may be due to the changes in the gastric environment of GC patients.
There are significant differences in the composition of gastric microbiota in GC patients based on race and region. A Portuguese cohort study showed an increased abundance of Achromobacter in GC patients compared to gastritis patients, while Achromobacter was completely absent in the validation cohort of Chinese GC subjects (Ferreira et al., 2018). Yu et al. analyzed bacterial abundance and diversity in GC tissues from 80 Chinese and 80 Mexican patients. Similar to the gastric microbiota profiles in non-cancer tissues, microbiota in cancer tissues of Mexican and Chinese patients were also composed mainly of Proteobacteria, followed by Firmicutes in Mexican cancer tissues or Bacteroides in Chinese cancer tissues. Mexican samples showed an increased relative abundance of Clostridium in cancer tissues but no difference in the alpha diversity, while cancer samples from Chinese patients presented substantial differences in alpha diversity and the abundance of several genera has increased, such as Treponema, Helicobacter, Selenomonas, Fusobacterium, Streptococcus, Pseudomonas (Yu et al., 2017a). These studies indicated that geographical and ethnic factors could influence the composition of stomach microbes.
Notably, a robust correlation existed between stomach microbes and the epidemiology of GC. Compared with cases without a family history of upper gastrointestinal cancer, cases with a family history of upper gastrointestinal cancer have lower alpha diversity and a higher abundance of H. pylori (Yu et al., 2017b). Among populations with similar H. pylori prevalence, the gastric microbiota composition significantly differed in populations from two towns with different GC risks in Colombia. Leptotrichia wadei and Veillonella sp. were considerably abundant in populations from Túquerres, a town with high GC risk, while Staphylococcus sp. were strikingly abundant in populations from Tumaco, a town with low GC risk (Yang et al., 2016). These findings demonstrated that the characteristics of the gastric microbiota in GC patients were associated with both familial history of gastrointestinal tumors and diverse environmental conditions.
Helicobacter pylori and gastric cancer
The first animal experiment on the pathogenicity of H. pylori was performed by the Mongolian gerbil model. It revealed that H. pylori induced a continuous development from superficial gastritis to pre-malignant lesions (Hirayama et al., 1996). Compared with age-matched uninfected mice, mice infected with H. pylori had more severe inflammation, acid atrophy, hyperplasia, epithelial defects, and dysplasia (Lee et al., 2008). Likewise, compared with the control gerbils, low differentiated adenocarcinoma and carcinoid were discovered in the gerbils inoculated with H. pylori (Hirayama et al., 1999).
H. pylori infection is one of the main causes of gastric cancer and can increase the risk of gastric cancer by 2.2–21 times (Uemura et al., 2001; Suerbaum and Michetti, 2002). H. pylori infection could induce chronic inflammation in the stomach, which was accompanied by genetic alterations and DNA damage in gastric epithelial cells. H. pylori infection has been found to trigger ubiquitination and proteasomal degradation of p53, a critical regulator of genome stability, thereby impairing the repair of genome damage. H. pylori infection reduces the expression of the transcription factor USF1, which can stabilize the function of P53, and thereby increasing viability of gastric epithelial cells with persistent DNA damage and promoting gastric carcinogenesis (Costa et al., 2020). H. pylori could also downregulate the expression of genes associated with tumor suppression by inducing abnormal DNA methylation (Servetas et al., 2016; Choi et al., 2020). Abnormal DNA methylation in the gene promoter region leads to the inactivation of tumor suppressor and other cancer-related genes in cancer cells, which is the most clear epigenetic marker in gastric cancer (Qu et al., 2013). Chan AO et al. observed that H. pylori infection caused E-cadherin methylation to be more frequent in the gastric mucosa compared to cases without H. pylori infection (Chan et al., 2003). Maekita et al. found that H. pylori infection can effectively induce CpG islands methylation to varying degrees (Maekita et al., 2006). H. pylori infection also delays gastric epithelial cell apoptosis (Sáenz and Mills, 2020; Imai et al., 2021). H. pylori infection induced an increase in cellular spermine oxidase (SMOX), and phosphorylated EGFR (pEGFR), resulting in the generation of a subpopulation of gastric epithelial cells with high levels of DNA damage and resistance to apoptosis (Chaturvedi et al., 2011, 2014).
Among possible explanations of GC caused by H. pylori, the two most widely accepted virulence factors were Cytotoxin-associated gene A (CagA) and Vacuolating cytotoxin A (VacA; Amieva and Peek, 2016), which have been linked to the carcinogenic potential of this bacterium. The CagA gene is the most important pathogenic factor of H. pylori. Compared with the strains without CagA, strains containing CagA increase the risk of gastric cancer by 1.64-fold overall (Censini et al., 1996; Huang et al., 2003). Cag (+) H. pylori induced TP53 gene mutation and aberrant expression of activation-induced cytidine deaminase, which may be responsible for the accumulation of mutation in gastric carcinogenesis (Matsumoto et al., 2007; Yong et al., 2015). CagA (+) H. pylori infection caused the activation of multiple oncogenic pathways, including ERK/MAPK, PI3K/AKT, NF-kB, Wnt/β-catenin, Ras, Hippo, and STAT3 (Udhayakumar et al., 2007; Salama et al., 2013; Yong et al., 2015; Imai et al., 2021). CagA also disturbed the host’sepithelial cells, precursor cells and stem cells (Bessède et al., 2014; Wroblewski et al., 2015). Another virulence factor, VacA, was involved in regulating immune responses and autophagy. VacA regulated host cell metabolism by inhibiting mTORC1 and promoted gastric epithelial cell apoptosis by interfering with the function of mitochondria (Kim et al., 2018). In addition, VacA promotes Treg differentiation by inducing dendritic cell expression and releasing some anti-inflammatory cytokines, such as IL-18 and IL-10, thus suppressing anti-tumor immunity (Kao et al., 2010; Oertli et al., 2012). Prolonged exposure to VacA can interrupt autophagy, which is manifested by the accumulation of P62. Autophagy is an important protective mechanism of the stomach against H. pylori infection. The interruption of this mechanism will cause cell death, inflammation and genetic instability, forming a microenvironment prone to cancer (Raju et al., 2012).
Other pathogenic mechanisms of H. pylori have also been widely reported. Some adhesins, such as sialic acid-binding adhesin (SabA), blood-antigen binding protein A (BabA) and neutrophil-activating protein (NAP), attached to host cell receptors and increased risk of peptic ulcer and GC (Kao et al., 2016). Targosz et al. proved that H. pylori up-regulated the expression of cyclooxygenase-2 (COX-2) mRNA in gastric epithelial cells, which was known to be a carcinogenesis-related rate-limiting enzyme (Targosz et al., 2012; Shao et al., 2014). The accumulation of activated β-catenin in the nucleus of gastric epithelial cells induced by H. pylori was closely connected with tumor invasion (Cheng et al., 2004), indicating the aberrant activation of β-catenin may be a key member in regulating pre-malignant epithelial responses to H. pylori. In addition, H. pylori infection induced the expression of hepatoma-derived growth factor (HDGF), which stimulated the differentiation of human mesenchymal stem cells into myofibroblast-like cells and further promoted the survival and invasion of human GC cells (Liu et al., 2018).
Other gastric microbiota and gastric cancer
Under conditions of absent acidity (such as AG and IM), some non-H. pylori bacteria produce active oxygen or nitrogen to regulate inflammatory reactions, and the gastric micro-ecosystem became more complex (Sheh and Fox, 2013). The abundance of some genera showed a consistent increase in GC patients, including Staphylococcus (Jo et al., 2016; Castaño-Rodríguez et al., 2017; Shen et al., 2022), Lactobacillus (Castaño-Rodríguez et al., 2017; Coker et al., 2018; Ferreira et al., 2018), Clostridium (Castaño-Rodríguez et al., 2017; Ferreira et al., 2018), Fusobacterium (Castaño-Rodríguez et al., 2017; Coker et al., 2018), Streptococcus (Dicksved et al., 2009; Castaño-Rodríguez et al., 2017; Coker et al., 2018), Bifidobacterium, and Lactococcus (Castaño-Rodríguez et al., 2017). These bacteria had different effects on stomach pathogenesis. A prospective study concluded that the characteristics of gastric microbiota in non-tumor patients could accurately classify patients who may develop EC. They identified a constellation of six bacterial taxonomic markers, including the Moryella genus, Vibro genus, Comamonadaceae, Paludibacter, Agrobacterium, and Clostridiales (Png et al., 2022).
Some non-H. pylori bacteria promote the inflammatory response to accelerate the progression of GC, such as Lactobacillus murinus, Clostridium, and Streptococcus salivarius (Lertpiriyapong et al., 2014). It has been proved that the overgrowth of Propionibacterium acnes may contribute to lymphocytic gastritis through in vitro cell experiments. Lymphocytic gastritis caused by Propionibacterium acnes produces pro-inflammatory cytokine IL-15, which is a potential trigger for GC (Montalban-Arques et al., 2016). Prevotella had the classification ability to distinguish GC patients from non-cancer-bearing people with an area under the curve of 0.76 (Wu et al., 2018). The pathogenicity of Prevotella copri has been proven to produce redox proteins in the human body (Hofer, 2014; Wu et al., 2018). Besides, the Insulin-Gastrin (INS-GAS) transgenic mice colonized Lactobacillus murinus, Clostridium and Bacteroides developed gastrointestinal intraepithelial neoplasia, which strongly related to the upregulation of pro-inflammatory and oncogenic genes (Lertpiriyapong et al., 2014). The microflora of non-H. pylori in the stomach also influenced the severity of H. pylori-induced gastric cancer. Shen et al. found that Streptococcus salivarius coinfection with H. pylori induced significantly higher gastric pathology than in H. pylori-monoinfected mice. In contrast, Staphylococcus epidermidis coinfection caused significantly lower H. pylori-induced pro-inflammatory cytokine responses than in H. pylori-monoinfected mice (Shen et al., 2022).
Some non-H. pylori bacteria could disturb the function of immune cells in the tumor microenvironment to promote GC. Previous studies found a positive correlation between Stenotrophomonas in GC tissues and plasmacytoid dendritic cells that have the function of suppressing immune effector cells (Huang et al., 2014; Ling et al., 2019). Similarly, in GC microhabitats, Selenomonas was positively associated with regulatory T cells with immunosuppressive effects (Ahmetlić et al., 2019; Ling et al., 2019). These studies suggest Selenomonas and Stenotrophomonas may promote cancer cells to evade surveillance by the immune system. Besides, it was reported that Fusobacterium nucleatum disturbed the phenotypes and functions of immune cells such as neutrophils, T cells, NK cells, dendritic cells and macrophages, forming an immunosuppressive microenvironment conducive to cancer growth (Wu et al., 2019). The increase of Fusobacterium in H. pylori (−) GC patients may be related to this mechanism (Hsieh et al., 2018). Li Q et al. found that excess Propionibacterium acnes promotes gastric cancer progression by promoting M2 polarization of macrophages through TLR4/PI3K/Akt signaling (Li et al., 2021).
Non-H. pylori bacteria produced metabolites that may promote the occurrence of GC. Higher levels of non-H. pylori nitrosated or nitrate-reducing bacteria (NB) and non-H. pylori urease-producing bacteria (UB) were found in H. pylori (−) GC patients (Jo et al., 2016; Sohn et al., 2017). It is well known that N-nitroso compounds (NOCs) are potent carcinogens (Hernández-Ramírez et al., 2009; Jo et al., 2016). NOCs formed from nitrite and secondary amines and were observed in some nitrate-reducing gastric bacteria, including Clostridium, Veillonella, Haemophilus, Staphylococcus, Streptococcus, and Neisseria (Ayanaba and Alexander, 1973; Hu et al., 2012; Jo et al., 2016). Similarly, urease is the main trigger of innate immune response produced by various non-H. pylori such as Lactococcus, Clostridium, Haemophilus, and Actinomyces (Sohn et al., 2017). These nitrate-reducing and urease-producing bacteria may be involved in the pathological mechanism of stomach disorders. However, the detailed pathogenic mechanism remains to be further confirmed.
Not all microbes in the stomach are harmful, and some studies have found the presence of bacteria in the stomach that can inhibit the progression of gastric cancer. For example, Kim SY et al. found that Lactococcus lactis ssp. lactis can affect the expression of p53 and p21 to induce cell cycle arrest and apoptosis to inhibit the proliferation of gastric cancer cells (Kim et al., 2004, 2009). In addition, Hwang CH et al. studied that Heat-Killed Lactobacillus can induce the expression of pro-apoptotic genes and inhibit the proliferation of gastric cancer cell line AGS in vitro. However it still needs to be verified by in vivo experiments (Hwang et al., 2022).
Interaction between Helicobacter pylori and other gastric microbiota
H. pylori infection altered the composition of the human stomach microbiome (Figure 1). Compared with the gastric microbiota of healthy cases, H. pylori-infection individuals have a lower diversity, with a lower abundance of Actinobacteria, Firmicutes and Bacteroidetes, while increased Proteobacteria (Andersson et al., 2008; Maldonado-Contreras et al., 2011).In H. pylori (+) GC patients, the proportion of the Streptococcus mitis group (such as S. oralis, S. infantis, S. mitis, S. tigurinus, and S. pseudopneumoniae) was significantly lower than that of the H. pylori (−) GC group (Sohn et al., 2017). These reduced bacteria may be related to the unfavorable conditions caused by H. pylori infection. The tendency of co-occurrence/co-competition among gastric microbiota has been further investigated. Das et al. found that in H. pylori (+) patients, H. pylori showed a negative association (inhibit other bacteria) with some gastric members, such as Acidovorax, Aeromonas, Bacillus, Bradyrhizobium, Halomonas, Cloacibacterium, Meiothermus, Methylobacterium, and Ralstonia, while the interactions of these non-H. pylori members were positively correlated (das et al., 2017).
The possible influence of H. pylori on the structure and function of the gastric microbes has been demonstrated by animal experiments. The addition of restricted microbiota (Clostridium ASF356, Bacteroides ASF519, Lactobacillus ASF361) in the stomach of INS-GAS mice was sufficient to promote gastric mucosal lesions such as moderate inflammation, gland atrophy, epithelial defects, dysplasia, but no gastrointestinal intraepithelial neoplasia. However, mice co-infected with these restricted bacteria and H. pylori developed higher-grade glandular abnormalities, and 69% of mice with dysplasia were identified as gastrointestinal intraepithelial neoplasia compared with mice infected only with restricted bacteria (Lertpiriyapong et al., 2014). It indicated that H. pylori synergistically accelerated the onset and progression of gastrointestinal intraepithelial neoplasia in mice infected with restricted bacteria. One year after inoculation with H. pylori, the numbers of Atopobium cluster increased and Bifidobacterium spp., C. coccoides group, and C. leptum subgroup decreased in H. pylori (−) gerbils compared to the uninfected gerbils. Besides, Prevotella spp. and Eubacterium cylindroides group were absent in H. pylori (+) gerbils (Osaki et al., 2012). These results suggested that infection with H. pylori for a long time may disturb the composition of the gastric microbiota in mice (Osaki et al., 2012). Similarly, the localization and levels of Bifidobacterium spp., Bacteroides spp., Enterococcus spp., Staphylococcus aureus and aerobes were modified and caused more severe gastritis in Mongolian gerbils after H. pylori infection. Prolonged colonization of H. pylori made the stomach environment unsuitable for the reproduction of lactobacilli, while Bacteroides, Bifidobacteria, S. aureus and Enterococci could better adapt to the stomach environment (Yin et al., 2011).
H. pylori eradication studies have also demonstrated the relationship between the growth of non-H. pylori and H. pylori. An inverse correlation was observed between the bacterial diversity and relative abundance of H. pylori in GC patients. Compared with non-GC patients with similar levels of H. pylori, GC patients showed lower bacterial diversity. After the eradication of H. pylori, the diversity of the gastric microbes was increased, and the microbiota abundance was restored to be similar to that of cases without H. pylori infection (Li et al., 2017). Likewise, H. pylori in the stomach inhibited the colonization of Enterobacteria, Clostridium leptum and Lactobacillus. After eradicaing of H. pylori, the bacteria in the patient’s stomach increased significantly (Li et al., 2016). In another study, in the H. pylori (+) patients, the total number of non- H. pylori- NB decreased in the eradicated gastric biopsies and increased in the non-eradicated or failed to eradicate samples (Jo et al., 2016). These studies indirectly confirmed the role of H. pylori infection in disturbing gastric microbiota composition.
To sum up, the mechanism of gastric microbiota causing GC is a multi-factor and multi-step process. H. pylori is the main trigger of histopathological changes in GC, and its interactions with non- H. pylori are jointly involved in the development of GC. H. pylori may be more important in the early stages of GC. But the state of achlorhydric induced by H. pylori can disturb gastric microbiota, which may play a key role in the later stages of GC (Dias-Jácome et al., 2016).
Regulation of gastric microbiota
Medication
Among the drug interventions to reduce the risk of GC, one of the most studied approaches is H. pylori eradication therapy. All patients who test positive for H. pylori should be offered eradication therapy. The internationally recommended treatment is the combination of PPI, 2–3 antibiotics, and bismuth, and it should be taken in strictly accordance with the course of treatment. Antibiotic abuse and irregular medication during the treatment of H. pylori have made H. pylori resistant to clarithromycin, levofloxacin, metronidazole and other drugs. The combination of drugs and the course of treatment vary according to different populations. The main recommended first-line treatment options were Bismuth quadruple therapy, Concomitant therapy, Sequential therapy, Levofloxacin triple therapy and so on (Chey et al., 2017). After 8 weeks of eradication treatment for CG or IM patients, H. pylori was significantly reduced, and the diversity of the microbiota increased (increased 31 operational taxonomic units; Li et al., 2017). It indicated that eradication treatment restored the diversity of gastric microbiota. Some studies have shown that eradicating H. pylori effectively alleviated stomach pathology (Bae et al., 2018; Sakitani et al., 2018). Eradication treatment also reduced the incidence of GC in healthy cases and patients with gastric neoplasia, reducing GC-related mortality (Choi et al., 2018; Doorakkers et al., 2020; Ford et al., 2020). However, there is evidence that H. pylori-eradicated patients were associated with an increased risk of GC (Cheung et al., 2019). Therefore, the effect of H. pylori eradication on the incidence of GC still needs to be clarified.
Probiotics and dietary
Probiotics and dietary fiber regulate the gastrointestinal microbiota and immune response; supplementing them was considered a preventive intervention (Zhang et al., 2013; Hill et al., 2014). It was reported that probiotics increased the eradication rate of H. pylori and reduced the incidence of side effects of antibacterial treatment (especially diarrhea; de Bortoli et al., 2007; Ojetti et al., 2012; Wang et al., 2013; Keikha and Karbalaei, 2021). It is worth noting that probiotics are not effective per se and can only be used as adjunctive therapy for clinical improvement (Wang et al., 2013). Probiotics also improve anticancer properties by producing lactic acid and other organic acids to inhibit the growth of microorganisms that produce mutagens and carcinogens (Dugas et al., 1999). Lactobacillus spp. is one of the best-known probiotics and their anti-H. pylori properties have been proven (Bahmanyar and Ye, 2006).Apart from probiotics, prebiotics and dietary fiber also synergistically affected H. pylori eradication therapy and were strongly associated with a lower risk of GC (Zhang et al., 2013; Shafaghi et al., 2016). Wheat bran acted as a nitrite scavenger, potentially offsetting the carcinogenic effects of NOCs produced by nitrate-reducing bacteria (Møller et al., 1988). The inhibitory effect of garlic on the growth of H. pylori was observed (Jonkers et al., 1999). The antibacterial properties of garlic may be attributed to allicin. Allicin has been confirmed to have a direct antibacterial effect on the growth of H. pylori in vitro (Cañizares et al., 2004).
Studies have reported synergistic relationships between multiple dietary components, such as vegetables, fruits, pickles, and soy products, in the development of GC (Bahmanyar and Ye, 2006).Fresh vegetables contain a various of antioxidants that acted as protectants, potentially ameliorating the effects of microbial dysbiosis (Epplein et al., 2008). Broccoli sprouts were rich in sulforaphane, the sulforaphane which had a strong bactericidal effect on H. pylori (Yanaka et al., 2009). Dietary patterns of high vegetables and seafood were associated with lower gastric dysbiosis index and lower the risk of GC in males. Sheu et al. pointed out that yogurt containing lactobacilli and bifidobacteria can improve the cure rate of H. pylori infection (Sheu et al., 2002).And the high-dairy dietary pattern was associated with a lower gastric dysbiosis index to reduce GC risk in females (Gunathilake et al., 2021). The intake of red meat and processed meat is related to the increased risk of gastric cancer, especially in H. pylori (+) subjects (González et al., 2006; Huang et al., 2021). Salt induces gastritis by directly damaging the gastric mucosa and increasing the rate of mitosis, and excess salt intake enhances H. pylori colonization. Therefore, long-term excessive salt intake will increase the risk of gastric cancer (Yamaguchi and Kakizoe, 2001; Peleteiro et al., 2011; D’Elia et al., 2012; Smyth et al., 2020). Therefore, the change of dietary structure, including reducing the intake of salt and red meat, and increasing the intake of vegetables and fruits, is a possible strategy to prevent gastric cancer.
Transplantation of rumen microbes
In recent years, fecal bacteria transplantation has been a focus to restore the healthy microbiota of the recipient. This effective strategy has been demonstrated in the treatment of various diseases, such as recurrent Clostridium difficile infection (Garza-González et al., 2019), inflammatory bowel disease (Colman and Rubin, 2014) and cancers (McQuade et al., 2020; Baruch et al., 2021). Liu et al. investigated the beneficial effects of rumen fluid transplantation on rumen morphology and function in a sheep model of rumen acidosis. Rumen fluid transplantation accelerated the rapid reconstruction of bacterial homeostasis in the rumen from an obvious acidosis state to a healthy level (similar to that of the donor). Furthermore, it reduced the damage of rumen epithelial cells caused by acute rumen acidosis (Liu J. et al., 2019). The results indicated that rumen microbiota transplantation is a promising strategy for reconstructing bacterial homeostasis. However, rumen transplantation is only an early attempt at the animal level, and it needs to be further verified in cancer models and clinical trials.
Conclusion
A healthy stomach environment is a basis for disease prevention, while the imbalance of gastric microbiota is a potential trigger of stomach carcinogenesis. Although H. pylori is considered the main cause of GC, studies have shown that other gastric microbiota are also involved in the development of cancer. Therefore, we analyzed the specific interactions between H. pylori and non-H. pylori in the progression of GC and discussed effective measures to reestablish a balance stomach environment. Probiotics and dietary fiber are considered to be a preventive intervention and adjuvant treatment, while gastric microbiota transplantation may fundamentally rebuild a normal microbiota in the stomach.
In summary, the gastric microbiota is an extremely complex group. The specific mechanism that causes the occurrence and development of GC is still unclear. Gastric tumorigenesis studies should take into account the virulence diversity of H. pylori strains, host genetic features, entire microbiota community and diverse environmental conditions.
Author contributions
SZ and CL conceived and designed the study, collected, and drafted the manuscript. LL, QY, and JM revised it critically for important intellectual content. WG, CD, and HW revised the manuscript. All authors contributed to the article and approved the submitted version.
Funding
This research was funded the Fundamental Research Funds for the Central Universities (0214-14380502).
Conflict of interest
The authors declare that the research was conducted in the absence of any commercial or financial relationships that could be construed as a potential conflict of interest.
Publisher’s note
All claims expressed in this article are solely those of the authors and do not necessarily represent those of their affiliated organizations, or those of the publisher, the editors and the reviewers. Any product that may be evaluated in this article, or claim that may be made by its manufacturer, is not guaranteed or endorsed by the publisher.
References
Ahmetlić, F., Riedel, T., Hömberg, N., Bauer, V., Trautwein, N., Geishauser, A., et al. (2019). Regulatory T cells in an endogenous mouse lymphoma recognize specific antigen peptides and contribute to immune escape. Cancer Immunol. Res. 7, 600–608. doi: 10.1158/2326-6066.CIR-18-0419
Alarcón, T., Llorca, L., and Perez-Perez, G. (2017). Impact of the microbiota and gastric disease development by Helicobacter pylori. Curr. Top. Microbiol. Immunol. 400, 253–275. doi: 10.1007/978-3-319-50520-6_11
Amieva, M., and Peek, R. M. Jr. (2016). Pathobiology of Helicobacter pylori-induced gastric cancer. Gastroenterology 150, 64–78. doi: 10.1053/j.gastro.2015.09.004
Andersson, A. F., Lindberg, M., Jakobsson, H., Bäckhed, F., Nyrén, P., and Engstrand, L. (2008). Comparative analysis of human gut microbiota by barcoded pyrosequencing. PLoS One 3:e2836. doi: 10.1371/journal.pone.0002836
Aviles-Jimenez, F., Vazquez-Jimenez, F., Medrano-Guzman, R., Mantilla, A., and Torres, J. (2014). Stomach microbiota composition varies between patients with non-atrophic gastritis and patients with intestinal type of gastric cancer. Sci. Rep. 4:4202. doi: 10.1038/srep04202
Ayanaba, A., and Alexander, M. (1973). Microbial formation of nitrosamines in vitro. Appl. Microbiol. 25, 862–868. doi: 10.1128/am.25.6.862-868.1973
Bae, S. E., Choi, K. D., Choe, J., Kim, S. O., Na, H. K., Choi, J. Y., et al. (2018). The effect of eradication of Helicobacter pylori on gastric cancer prevention in healthy asymptomatic populations. Helicobacter 23:e12464. doi: 10.1111/hel.12464
Bahmanyar, S., and Ye, W. (2006). Dietary patterns and risk of squamous-cell carcinoma and adenocarcinoma of the esophagus and adenocarcinoma of the gastric cardia: a population-based case-control study in Sweden. Nutr. Cancer 54, 171–178. doi: 10.1207/s15327914nc5402_3
Baruch, E. N., Youngster, I., Ben-Betzalel, G., Ortenberg, R., Lahat, A., Katz, L., et al. (2021). Fecal microbiota transplant promotes response in immunotherapy-refractory melanoma patients. Science 371, 602–609. doi: 10.1126/science.abb5920
Bessède, E., Staedel, C., Acuña Amador, L. A., Nguyen, P. H., Chambonnier, L., Hatakeyama, M., et al. (2014). Helicobacter pylori generates cells with cancer stem cell properties via epithelial-mesenchymal transition-like changes. Oncogene 33, 4123–4131. doi: 10.1038/onc.2013.380
Boers, S. A., Jansen, R., and Hays, J. P. (2019). Understanding and overcoming the pitfalls and biases of next-generation sequencing (NGS) methods for use in the routine clinical microbiological diagnostic laboratory. Eur. J. Clin. Microbiol. Infect. Dis. 38, 1059–1070. doi: 10.1007/s10096-019-03520-3
Bruno, G., Rocco, G., Zaccari, P., Porowska, B., Mascellino, M. T., and Severi, C. (2018). Helicobacter pylori infection and gastric Dysbiosis: can probiotics administration be useful to treat this condition? Can. J. Infect. Dis. Med. Microbiol. 2018:6237239. doi: 10.1155/2018/6237239
Bruno, G., Zaccari, P., Rocco, G., Scalese, G., Panetta, C., Porowska, B., et al. (2019). Proton pump inhibitors and dysbiosis: current knowledge and aspects to be clarified. World J. Gastroenterol. 25, 2706–2719. doi: 10.3748/wjg.v25.i22.2706
Cañizares, P., Gracia, I., Gómez, L. A., García, A., Martín de Argila, C., Boixeda, D., et al. (2004). Thermal degradation of allicin in garlic extracts and its implication on the inhibition of the in-vitro growth of Helicobacter pylori. Biotechnol. Prog. 20, 32–37. doi: 10.1021/bp034135v
Castaño-Rodríguez, N., Goh, K. L., Fock, K. M., Mitchell, H. M., and Kaakoush, N. O. (2017). Dysbiosis of the microbiome in gastric carcinogenesis. Sci. Rep. 7:15957. doi: 10.1038/s41598-017-16289-2
Censini, S., Lange, C., Xiang, Z., Crabtree, J. E., Ghiara, P., Borodovsky, M., et al. (1996). Cag, a pathogenicity island of Helicobacter pylori, encodes type I-specific and disease-associated virulence factors. Proc. Natl. Acad. Sci. U. S. A. 93, 14648–14653. doi: 10.1073/pnas.93.25.14648
Chan, A. O., Lam, S. K., Wong, B. C., Wong, W. M., Yuen, M. F., Yeung, Y. H., et al. (2003). Promoter methylation of E-cadherin gene in gastric mucosa associated with Helicobacter pylori infection and in gastric cancer. Gut 52, 502–506. doi: 10.1136/gut.52.4.502
Chaturvedi, R., Asim, M., Piazuelo, M. B., Yan, F., Barry, D. P., Sierra, J. C., et al. (2014). Activation of EGFR and ERBB2 by Helicobacter pylori results in survival of gastric epithelial cells with DNA damage. Gastroenterology 146, 1739–51.e14. doi: 10.1053/j.gastro.2014.02.005
Chaturvedi, R., Asim, M., Romero–Gallo, J., Barry, D. P., Hoge, S., de Sablet, T., et al. (2011). Spermine oxidase mediates the gastric cancer risk associated with Helicobacter pylori CagA. Gastroenterology 141, 1696–1708.e2. doi: 10.1053/j.gastro.2011.07.045
Chen, X. H., Wang, A., Chu, A. N., Gong, Y. H., and Yuan, Y. (2019). Mucosa-associated microbiota in gastric cancer tissues compared with non-cancer tissues. Front. Microbiol. 10:1261. doi: 10.3389/fmicb.2019.01261
Cheng, X. X., Sun, Y., Chen, X. Y., Zhang, K. L., Kong, Q. Y., Liu, J., et al. (2004). Frequent translocalization of beta-catenin in gastric cancers and its relevance to tumor progression. Oncol. Rep. 11, 1201–1207. doi: 10.3892/or.11.6.1201
Cheung, K. S., Chan, E. W., Chen, L., Seto, W. K., Wong, I. C. K., and Leung, W. K. (2019). Diabetes increases risk of gastric cancer after Helicobacter pylori eradication: a territory-wide study with propensity score analysis. Diabetes Care 42, 1769–1775. doi: 10.2337/dc19-0437
Chey, W. D., Leontiadis, G. I., Howden, C. W., and Moss, S. F. (2017). ACG clinical guideline: treatment of Helicobacter pylori infection. Am. J. Gastroenterol. 112, 212–239. doi: 10.1038/ajg.2016.563
Choi, J. M., Kim, S. G., Yang, H. J., Lim, J. H., Cho, N. Y., Kim, W. H., et al. (2020). Helicobacter pylori eradication can reverse the methylation-associated regulation of miR-200a/b in gastric carcinogenesis. Gut Liver 14, 571–580. doi: 10.5009/gnl19299
Choi, I. J., Kook, M. C., Kim, Y. I., Cho, S. J., Lee, J. Y., Kim, C. G., et al. (2018). Helicobacter pylori therapy for the prevention of Metachronous gastric cancer. N. Engl. J. Med. 378, 1085–1095. doi: 10.1056/NEJMoa1708423
Cires, M. J., Wong, X., Carrasco-Pozo, C., and Gotteland, M. (2016). The gastrointestinal tract as a Key target organ for the health-promoting effects of dietary Proanthocyanidins. Front. Nutr. 3:57. doi: 10.3389/fnut.2016.00057
Coker, O. O., Dai, Z., Nie, Y., Zhao, G., Cao, L., Nakatsu, G., et al. (2018). Mucosal microbiome dysbiosis in gastric carcinogenesis. Gut 67, 1024–1032. doi: 10.1136/gutjnl-2017-314281
Colman, R. J., and Rubin, D. T. (2014). Fecal microbiota transplantation as therapy for inflammatory bowel disease: a systematic review and meta-analysis. J. Crohns Colitis 8, 1569–1581. doi: 10.1016/j.crohns.2014.08.006
Costa, L., Corre, S., Michel, V., le Luel, K., Fernandes, J., Ziveri, J., et al. (2020). USF1 defect drives p53 degradation during Helicobacter pylori infection and accelerates gastric carcinogenesis. Gut 69, 1582–1591. doi: 10.1136/gutjnl-2019-318640
D’Elia, L., Rossi, G., Ippolito, R., Cappuccio, F. P., and Strazzullo, P. (2012). Habitual salt intake and risk of gastric cancer: a meta-analysis of prospective studies. Clin. Nutr. 31, 489–498. doi: 10.1016/j.clnu.2012.01.003
Dai, D., Yang, Y., Yu, J., Dang, T., Qin, W., Teng, L., et al. (2021). Interactions between gastric microbiota and metabolites in gastric cancer. Cell Death Dis. 12:1104. doi: 10.1038/s41419-021-04396-y
das, A., Pereira, V., Saxena, S., Ghosh, T. S., Anbumani, D., Bag, S., et al. (2017). Gastric microbiome of Indian patients with Helicobacter pylori infection, and their interaction networks. Sci. Rep. 7:15438. doi: 10.1038/s41598-017-15510-6
de Bortoli, N., Leonardi, G., Ciancia, E., Merlo, A., Bellini, M., Costa, F., et al. (2007). Helicobacter pylori eradication: a randomized prospective study of triple therapy versus triple therapy plus lactoferrin and probiotics. Am. J. Gastroenterol. 102, 951–956. doi: 10.1111/j.1572-0241.2007.01085.x
Dias-Jácome, E., Libânio, D., Borges-Canha, M., Galaghar, A., and Pimentel-Nunes, P. (2016). Gastric microbiota and carcinogenesis: the role of non-Helicobacter pylori bacteria - a systematic review. Rev. Esp. Enferm. Dig. 108, 530–540. doi: 10.17235/reed.2016.4261/2016
Dicksved, J., Lindberg, M., Rosenquist, M., Enroth, H., Jansson, J. K., and Engstrand, L. (2009). Molecular characterization of the stomach microbiota in patients with gastric cancer and in controls. J. Med. Microbiol. 58, 509–516. doi: 10.1099/jmm.0.007302-0
Dong, J., and Thrift, A. P. (2017). Alcohol, smoking and risk of oesophago-gastric cancer. Best Pract. Res. Clin. Gastroenterol. 31, 509–517. doi: 10.1016/j.bpg.2017.09.002
Doorakkers, E., Lagergren, J., Engstrand, L., and Brusselaers, N. (2016). Eradication of Helicobacter pylori and gastric cancer: a systematic review and meta-analysis of cohort studies. J. Natl. Cancer Inst. 108:djw132. doi: 10.1093/jnci/djw132
Doorakkers, E., Lagergren, J., Engstrand, L., and Brusselaers, N. (2020). Reply to: Helicobacter pylori eradication treatment and the risk of gastric adenocarcinoma in a western population. Gut 69, 1149–1150. doi: 10.1136/gutjnl-2019-319000
Dugas, B., Mercenier, A., Lenoir-Wijnkoop, I., Arnaud, C., Dugas, N., and Postaire, E. (1999). Immunity and probiotics. Immunol. Today 20, 387–390. doi: 10.1016/S0167-5699(99)01448-6
Epplein, M., Nomura, A. M. Y., Hankin, J. H., Blaser, M. J., Perez-Perez, G., Stemmermann, G. N., et al. (2008). Association of Helicobacter pylori infection and diet on the risk of gastric cancer: a case-control study in Hawaii. Cancer Causes Control 19, 869–877. doi: 10.1007/s10552-008-9149-2
Eun, C. S., Kim, B. K., Han, D. S., Kim, S. Y., Kim, K. M., Choi, B. Y., et al. (2014). Differences in gastric mucosal microbiota profiling in patients with chronic gastritis, intestinal metaplasia, and gastric cancer using pyrosequencing methods. Helicobacter 19, 407–416. doi: 10.1111/hel.12145
Falush, D., Wirth, T., Linz, B., Pritchard, J. K., Stephens, M., Kidd, M., et al. (2003). Traces of human migrations in Helicobacter pylori populations. Science 299, 1582–1585. doi: 10.1126/science.1080857
Ferreira, R. M., Pereira-Marques, J., Pinto-Ribeiro, I., Costa, J. L., Carneiro, F., Machado, J. C., et al. (2018). Gastric microbial community profiling reveals a dysbiotic cancer-associated microbiota. Gut 67, 226–236. doi: 10.1136/gutjnl-2017-314205
Ford, A. C., Yuan, Y., and Moayyedi, P. (2020). Helicobacter pylori eradication therapy to prevent gastric cancer: systematic review and meta-analysis. Gut 69, 2113–2121. doi: 10.1136/gutjnl-2020-320839
Garza-González, E., Mendoza-Olazarán, S., Morfin-Otero, R., Ramírez-Fontes, A., Rodríguez-Zulueta, P., Flores-Treviño, S., et al. (2019). Intestinal microbiome changes in fecal microbiota transplant (FMT) vs. FMT enriched with lactobacillus in the treatment of recurrent Clostridioides difficile infection. Can. J. Gastroenterol. Hepatol. 2019:4549298. doi: 10.1155/2019/4549298
González, C. A., Jakszyn, P., Pera, G., Agudo, A., Bingham, S., Palli, D., et al. (2006). Meat intake and risk of stomach and esophageal adenocarcinoma within the European prospective investigation into cancer and nutrition (EPIC). J. Natl. Cancer Inst. 98, 345–354. doi: 10.1093/jnci/djj071
Gunathilake, M. N., Lee, J., Choi, I. J., Kim, Y. I., Ahn, Y., Park, C., et al. (2019). Association between the relative abundance of gastric microbiota and the risk of gastric cancer: a case-control study. Sci. Rep. 9:13589. doi: 10.1038/s41598-019-50054-x
Gunathilake, M., Lee, J. H., Choi, I. J., Kim, Y. I., and Kim, J. S. (2021). Effect of the Interaction between Dietary Patterns and the Gastric Microbiome on the Risk of Gastric Cancer. Nutrients 13:2692. doi: 10.3390/nu13082692
Guo, Y., Cao, X. S., Zhou, M. G., and Yu, B. (2022). Gastric microbiota in gastric cancer: different roles of Helicobacter pylori and other microbes. Front. Cell. Infect. Microbiol. 12:1105811. doi: 10.3389/fcimb.2022.1105811
He, X., McLean, J. S., Edlund, A., Yooseph, S., Hall, A. P., Liu, S. Y., et al. (2015). Cultivation of a human-associated TM7 phylotype reveals a reduced genome and epibiotic parasitic lifestyle. Proc. Natl. Acad. Sci. U. S. A. 112, 244–249. doi: 10.1073/pnas.1419038112
Hernández-Ramírez, R. U., Galván-Portillo, M. V., Ward, M. H., Agudo, A., González, C. A., Oñate-Ocaña, L. F., et al. (2009). Dietary intake of polyphenols, nitrate and nitrite and gastric cancer risk in Mexico City. Int. J. Cancer 125, 1424–1430. doi: 10.1002/ijc.24454
Hill, C., Guarner, F., Reid, G., Gibson, G. R., Merenstein, D. J., Pot, B., et al. (2014). Expert consensus document. The international scientific Association for Probiotics and Prebiotics consensus statement on the scope and appropriate use of the term probiotic. Nat. Rev. Gastroenterol. Hepatol. 11, 506–514. doi: 10.1038/nrgastro.2014.66
Hirayama, F., Takagi, S., Iwao, E., Yokoyama, Y., Haga, K., and Hanada, S. (1999). Development of poorly differentiated adenocarcinoma and carcinoid due to long-term Helicobacter pylori colonization in Mongolian gerbils. J. Gastroenterol. 34, 450–454. doi: 10.1007/s005350050295
Hirayama, F., Takagi, S., Kusuhara, H., Iwao, E., Yokoyama, Y., and Ikeda, Y. (1996). Induction of gastric ulcer and intestinal metaplasia in mongolian gerbils infected with Helicobacter pylori. J. Gastroenterol. 31, 755–757. doi: 10.1007/BF02347631
Hofer, U. (2014). Microbiome: pro-inflammatory Prevotella? Nat. Rev. Microbiol. 12:5. doi: 10.1038/nrmicro3180
Hsieh, Y. Y., Tung, S. Y., Pan, H. Y., Yen, C. W., Xu, H. W., Lin, Y. J., et al. (2018). Increased abundance of clostridium and Fusobacterium in gastric microbiota of patients with gastric cancer in Taiwan. Sci. Rep. 8:158. doi: 10.1038/s41598-017-18596-0
Hu, Y., He, L. H., Xiao, D., Liu, G. D., Gu, Y. X., Tao, X. X., et al. (2012). Bacterial flora concurrent with Helicobacter pylori in the stomach of patients with upper gastrointestinal diseases. World J. Gastroenterol. 18, 1257–1261. doi: 10.3748/wjg.v18.i11.1257
Hu, Y. L., Pang, W., Huang, Y., Zhang, Y., and Zhang, C. J. (2018). The gastric microbiome is perturbed in advanced gastric adenocarcinoma identified through shotgun Metagenomics. Front. Cell. Infect. Microbiol. 8:433. doi: 10.3389/fcimb.2018.00433
Huang, Y., Cao, D., Chen, Z., Chen, B., Li, J., Guo, J., et al. (2021). Red and processed meat consumption and cancer outcomes: umbrella review. Food Chem. 356:129697. doi: 10.1016/j.foodchem.2021.129697
Huang, X. M., Liu, X. S., Lin, X. K., Yu, H., Sun, J. Y., Liu, X. K., et al. (2014). Role of plasmacytoid dendritic cells and inducible costimulator-positive regulatory T cells in the immunosuppression microenvironment of gastric cancer. Cancer Sci. 105, 150–158. doi: 10.1111/cas.12327
Huang, J. Q., Zheng, G. F., Sumanac, K., Irvine, E. J., and Hunt, R. H. (2003). Meta-analysis of the relationship between cagA seropositivity and gastric cancer. Gastroenterology 125, 1636–1644. doi: 10.1053/j.gastro.2003.08.033
Hwang, C. H., Lee, N. K., and Paik, H. D. (2022). The anti-cancer potential of heat-killed lactobacillus brevis KU15176 upon AGS cell lines through intrinsic apoptosis pathway. Int. J. Mol. Sci. 23:4073. doi: 10.3390/ijms23084073
Imai, S., Ooki, T., Murata-Kamiya, N., Komura, D., Tahmina, K., Wu, W., et al. (2021). Helicobacter pylori CagA elicits BRCAness to induce genome instability that may underlie bacterial gastric carcinogenesis. Cell Host Microbe 29, 941–58.e10. doi: 10.1016/j.chom.2021.04.006
Jo, H. J., Kim, J., Kim, N., Park, J. H., Nam, R. H., Seok, Y. J., et al. (2016). Analysis of gastric microbiota by pyrosequencing: minor role of bacteria other than Helicobacter pylori in the gastric carcinogenesis. Helicobacter 21, 364–374. doi: 10.1111/hel.12293
Jonkers, D., van den Broek, E., van Dooren, I., Thijs, C., Dorant, E., Hageman, G., et al. (1999). Antibacterial effect of garlic and omeprazole on Helicobacter pylori. J. Antimicrob. Chemother. 43, 837–839. doi: 10.1093/jac/43.6.837
Kao, C. Y., Sheu, B. S., and Wu, J. J. (2016). Helicobacter pylori infection: an overview of bacterial virulence factors and pathogenesis. Biom. J. 39, 14–23. doi: 10.1016/j.bj.2015.06.002
Kao, J. Y., Zhang, M., Miller, M. J., Mills, J. C., Wang, B., Liu, M., et al. (2010). Helicobacter pylori immune escape is mediated by dendritic cell-induced Treg skewing and Th17 suppression in mice. Gastroenterology 138, 1046–1054. doi: 10.1053/j.gastro.2009.11.043
Keikha, M., and Karbalaei, M. (2021). Probiotics as the live microscopic fighters against Helicobacter pylori gastric infections. BMC Gastroenterol. 21:388. doi: 10.1186/s12876-021-01977-1
Khosravi, Y., Dieye, Y., Poh, B. H., Ng, C. G., Loke, M. F., Goh, K. L., et al. (2014). Culturable bacterial microbiota of the stomach of Helicobacter pylori positive and negative gastric disease patients. ScientificWorldJournal 2014:610421. doi: 10.1155/2014/610421
Kim, S. Y., Kim, J. E., Lee, K. W., and Lee, H. J. (2009). Lactococcus lactis ssp. lactis inhibits the proliferation of SNU-1 human stomach cancer cells through induction of G0/G1 cell cycle arrest and apoptosis via p53 and p21 expression. Ann. N. Y. Acad. Sci. 1171, 270–275. doi: 10.1111/j.1749-6632.2009.04721.x
Kim, S. Y., Lee, K. W., Kim, J. Y., and Lee, H. J. (2004). Cytoplasmic fraction of Lactococcus lactis ssp. lactis induces apoptosis in SNU-1 stomach adenocarcinoma cells. Biofactors 22, 119–122. doi: 10.1002/biof.5520220123
Kim, I. J., Lee, J., Oh, S. J., Yoon, M. S., Jang, S. S., Holland, R. L., et al. (2018). Helicobacter pylori infection modulates host cell metabolism through VacA-dependent inhibition of mTORC1. Cell Host Microbe 23, 583–93.e8. doi: 10.1016/j.chom.2018.04.006
Lee, C. W., Rickman, B., Rogers, A. B., Ge, Z., Wang, T. C., and Fox, J. G. (2008). Helicobacter pylori eradication prevents progression of gastric cancer in hypergastrinemic INS-GAS mice. Cancer Res. 68, 3540–3548. doi: 10.1158/0008-5472.CAN-07-6786
Lertpiriyapong, K., Whary, M. T., Muthupalani, S., Lofgren, J. L., Gamazon, E. R., Feng, Y., et al. (2014). Gastric colonisation with a restricted commensal microbiota replicates the promotion of neoplastic lesions by diverse intestinal microbiota in the Helicobacter pylori INS-GAS mouse model of gastric carcinogenesis. Gut 63, 54–63. doi: 10.1136/gutjnl-2013-305178
Li, T. H., Qin, Y., Sham, P. C., Lau, K. S., Chu, K. M., and Leung, W. K. (2017). Alterations in gastric microbiota after H. pylori eradication and in different histological stages of gastric carcinogenesis. Sci. Rep. 7:44935. doi: 10.1038/srep44935
Li, X. X., Wong, G. L. H., To, K. F., Wong, V. W. S., Lai, L. H., Chow, D. K. L., et al. (2009). Bacterial microbiota profiling in gastritis without Helicobacter pylori infection or non-steroidal anti-inflammatory drug use. PLoS One 4:e7985. doi: 10.1371/journal.pone.0007985
Li, Q., Wu, W., Gong, D., Shang, R., Wang, J., and Yu, H. (2021). Propionibacterium acnes overabundance in gastric cancer promote M2 polarization of macrophages via a TLR4/PI3K/Akt signaling. Gastric Cancer 24, 1242–1253. doi: 10.1007/s10120-021-01202-8
Li, L., Zhou, X., Xiao, S., Ye, F., and Zhang, G. (2016). The effect of Helicobacter pylori eradication on the gastrointestinal microbiota in patients with duodenal ulcer. J. Gastrointestin. Liver Dis. 25, 139–146. doi: 10.15403/jgld.2014.1121.252.hpe
Ling, Z., Shao, L., Liu, X., Cheng, Y., Yan, C., Mei, Y., et al. (2019). Regulatory T cells and Plasmacytoid dendritic cells within the tumor microenvironment in gastric cancer are correlated with gastric microbiota Dysbiosis: a preliminary study. Front. Immunol. 10:533. doi: 10.3389/fimmu.2019.00533
Linz, B., Balloux, F., Moodley, Y., Manica, A., Liu, H., Roumagnac, P., et al. (2007). An African origin for the intimate association between humans and Helicobacter pylori. Nature 445, 915–918. doi: 10.1038/nature05562
Liu, J., Li, H., Zhu, W., and Mao, S. (2019). Dynamic changes in rumen fermentation and bacterial community following rumen fluid transplantation in a sheep model of rumen acidosis: implications for rumen health in ruminants. FASEB J. 33, 8453–8467. doi: 10.1096/fj.201802456R
Liu, X., Shao, L., Liu, X., Ji, F., Mei, Y., Cheng, Y., et al. (2019). Alterations of gastric mucosal microbiota across different stomach microhabitats in a cohort of 276 patients with gastric cancer. EBioMedicine 40, 336–348. doi: 10.1016/j.ebiom.2018.12.034
Liu, C. J., Wang, Y. K., Kuo, F. C., Hsu, W. H., Yu, F. J., Hsieh, S., et al. (2018). Helicobacter pylori infection-induced Hepatoma-derived growth factor regulates the differentiation of human Mesenchymal stem cells to Myofibroblast-like cells. Cancers 10:479. doi: 10.3390/cancers10120479
Lofgren, J. L., Whary, M. T., Ge, Z., Muthupalani, S., Taylor, N. S., Mobley, M., et al. (2011). Lack of commensal flora in Helicobacter pylori-infected INS-GAS mice reduces gastritis and delays intraepithelial neoplasia. Gastroenterology 140, 210–220.e4. doi: 10.1053/j.gastro.2010.09.048
Maekita, T., Nakazawa, K., Mihara, M., Nakajima, T., Yanaoka, K., Iguchi, M., et al. (2006). High levels of aberrant DNA methylation in Helicobacter pylori - infected gastric mucosae and its possible association with gastric cancer risk. Clin. Cancer Res. 12, 989–995. doi: 10.1158/1078-0432.CCR-05-2096
Malaty, H. M., el-Kasabany, A., Graham, D. Y., Miller, C. C., Reddy, S. G., Srinivasan, S. R., et al. (2002). Age at acquisition of Helicobacter pylori infection: a follow-up study from infancy to adulthood. Lancet 359, 931–935. doi: 10.1016/S0140-6736(02)08025-X
Maldonado-Contreras, A., Goldfarb, K. C., Godoy-Vitorino, F., Karaoz, U., Contreras, M., Blaser, M. J., et al. (2011). Structure of the human gastric bacterial community in relation to Helicobacter pylori status. ISME J. 5, 574–579. doi: 10.1038/ismej.2010.149
Matsumoto, Y., Marusawa, H., Kinoshita, K., Endo, Y., Kou, T., Morisawa, T., et al. (2007). Helicobacter pylori infection triggers aberrant expression of activation-induced cytidine deaminase in gastric epithelium. Nat. Med. 13, 470–476. doi: 10.1038/nm1566
McQuade, J. L., Ologun, G. O., Arora, R., and Wargo, J. A. (2020). Gut microbiome modulation via fecal microbiota transplant to augment immunotherapy in patients with melanoma or other cancers. Curr. Oncol. Rep. 22:74. doi: 10.1007/s11912-020-00913-y
Møller, M. E., Dahl, R., and Bøckman, O. C. (1988). A possible role of the dietary fibre product, wheat bran, as a nitrite scavenger. Food Chem. Toxicol. 26, 841–845. doi: 10.1016/0278-6915(88)90024-5
Monstein, H. J., Tiveljung, A., Kraft, C. H., Borch, K., and Jonasson, J. (2000). Profiling of bacterial flora in gastric biopsies from patients with Helicobacter pylori-associated gastritis and histologically normal control individuals by temperature gradient gel electrophoresis and 16S rDNA sequence analysis. J. Med. Microbiol. 49, 817–822. doi: 10.1099/0022-1317-49-9-817
Montalban-Arques, A., Wurm, P., Trajanoski, S., Schauer, S., Kienesberger, S., Halwachs, B., et al. (2016). Propionibacterium acnes overabundance and natural killer group 2 member D system activation in corpus-dominant lymphocytic gastritis. J. Pathol. 240, 425–436. doi: 10.1002/path.4782
Nardone, G., and Compare, D. (2015). The human gastric microbiota: is it time to rethink the pathogenesis of stomach diseases? United European Gastroenterol J 3, 255–260. doi: 10.1177/2050640614566846
Oertli, M., Sundquist, M., Hitzler, I., Engler, D. B., Arnold, I. C., Reuter, S., et al. (2012). DC-derived IL-18 drives Treg differentiation, murine Helicobacter pylori-specific immune tolerance, and asthma protection. J. Clin. Invest. 122, 1082–1096. doi: 10.1172/JCI61029
Ohno, H., and Satoh-Takayama, N. (2020). Stomach microbiota, Helicobacter pylori, and group 2 innate lymphoid cells. Exp. Mol. Med. 52, 1377–1382. doi: 10.1038/s12276-020-00485-8
Ojetti, V., Bruno, G., Ainora, M. E., Gigante, G., Rizzo, G., Roccarina, D., et al. (2012). Impact of lactobacillus reuteri supplementation on anti-Helicobacter pylori levofloxacin-based second-line therapy. Gastroenterol. Res. Pract. 2012:740381. doi: 10.1155/2012/740381
Osaki, T., Matsuki, T., Asahara, T., Zaman, C., Hanawa, T., Yonezawa, H., et al. (2012). Comparative analysis of gastric bacterial microbiota in Mongolian gerbils after long-term infection with Helicobacter pylori. Microb. Pathog. 53, 12–18. doi: 10.1016/j.micpath.2012.03.008
Park, C. H., Lee, A. R., Lee, Y. R., Eun, C. S., Lee, S. K., and Han, D. S. (2019). Evaluation of gastric microbiome and metagenomic function in patients with intestinal metaplasia using 16S rRNA gene sequencing. Helicobacter 24:e12547. doi: 10.1111/hel.12547
Parsons, B. N., Ijaz, U. Z., D’Amore, R., Burkitt, M. D., Eccles, R., Lenzi, L., et al. (2017). Comparison of the human gastric microbiota in hypochlorhydric states arising as a result of Helicobacter pylori-induced atrophic gastritis, autoimmune atrophic gastritis and proton pump inhibitor use. PLoS Pathog. 13:e1006653. doi: 10.1371/journal.ppat.1006653
Peleteiro, B., Lopes, C., Figueiredo, C., and Lunet, N. (2011). Salt intake and gastric cancer risk according to Helicobacter pylori infection, smoking, tumour site and histological type. Br. J. Cancer 104, 198–207. doi: 10.1038/sj.bjc.6605993
Png, C. W., Lee, W. J. J., Chua, S. J., Zhu, F., Gastric Consortium5, Yeoh, K. G., et al. (2022). Mucosal microbiome associates with progression to gastric cancer. Theranostics 12, 48–58. doi: 10.7150/thno.65302
Qu, Y., Dang, S., and Hou, P. (2013). Gene methylation in gastric cancer. Clin. Chim. Acta 424, 53–65. doi: 10.1016/j.cca.2013.05.002
Rajilic-Stojanovic, M., Figueiredo, C., Smet, A., Hansen, R., Kupcinskas, J., Rokkas, T., et al. (2020). Systematic review: gastric microbiota in health and disease. Aliment. Pharmacol. Ther. 51, 582–602. doi: 10.1111/apt.15650
Raju, D., Hussey, S., Ang, M., Terebiznik, M. R., Sibony, M., Galindo–Mata, E., et al. (2012). Vacuolating cytotoxin and variants in Atg16L1 that disrupt autophagy promote Helicobacter pylori infection in humans. Gastroenterology 142, 1160–1171. doi: 10.1053/j.gastro.2012.01.043
Rawla, P., and Barsouk, A. (2019). Epidemiology of gastric cancer: global trends, risk factors and prevention. Prz. Gastroenterol. 14, 26–38. doi: 10.5114/pg.2018.80001
Sáenz, J. B., and Mills, J. C. (2020). Helicobacter pylori: preying on SIVA for survival in the stomach. J. Clin. Invest. 130, 2183–2185. doi: 10.1172/JCI135508
Sakitani, K., Nishizawa, T., Arita, M., Yoshida, S., Kataoka, Y., Ohki, D., et al. (2018). Early detection of gastric cancer after Helicobacter pylori eradication due to endoscopic surveillance. Helicobacter 23:e12503. doi: 10.1111/hel.12503
Salama, N. R., Hartung, M. L., and Müller, A. (2013). Life in the human stomach: persistence strategies of the bacterial pathogen Helicobacter pylori. Nat. Rev. Microbiol. 11, 385–399. doi: 10.1038/nrmicro3016
Satoh-Takayama, N., Kato, T., Motomura, Y., Kageyama, T., Taguchi-Atarashi, N., Kinoshita-Daitoku, R., et al. (2020). Bacteria-induced group 2 innate lymphoid cells in the stomach provide immune protection through induction of IgA. Immunity 52, 635–49.e4. doi: 10.1016/j.immuni.2020.03.002
Scarpignato, C., Gatta, L., Zullo, A., and Blandizzi, C., for the SIF-AIGO-FIMMG Group, on behalf of the Italian Society of Pharmacology, the Italian Association of Hospital Gastroenterologists, and the Italian Federation of General Practitioners (2016). Effective and safe proton pump inhibitor therapy in acid-related diseases - a position paper addressing benefits and potential harms of acid suppression. BMC Med. 14:179. doi: 10.1186/s12916-016-0718-z
Schulz, C., Schütte, K., Koch, N., Vilchez-Vargas, R., Wos-Oxley, M. L., Oxley, A. P. A., et al. (2018). The active bacterial assemblages of the upper GI tract in individuals with and without helicobacter infection. Gut 67, 216–225. doi: 10.1136/gutjnl-2016-312904
Seo, I., Jha, B. K., Suh, S. I., Suh, M. H., and Baek, W. K. (2014). Microbial profile of the stomach: comparison between Normal mucosa and cancer tissue in the same patient. J. Bus. Ventur. 44, 162–169. doi: 10.4167/jbv.2014.44.2.162
Servetas, S. L., Bridge, D. R., and Merrell, D. S. (2016). Molecular mechanisms of gastric cancer initiation and progression by Helicobacter pylori. Curr. Opin. Infect. Dis. 29, 304–310. doi: 10.1097/QCO.0000000000000248
Shafaghi, A., Pourkazemi, A., Khosravani, M., Fakhrie Asl, S., Amir Maafi, A., Atrkar Roshan, Z., et al. (2016). The effect of probiotic plus prebiotic supplementation on the tolerance and efficacy of Helicobacter pylori eradication quadruple therapy: a randomized prospective double blind controlled trial. Middle East J. Dig. Dis. 8, 179–188. doi: 10.15171/mejdd.2016.30
Shao, Y., Sun, K., Xu, W., Li, X. L., Shen, H., and Sun, W. H. (2014). Helicobacter pylori infection, gastrin and cyclooxygenase-2 in gastric carcinogenesis. World J. Gastroenterol. 20, 12860–12873. doi: 10.3748/wjg.v20.i36.12860
Shao, D., Vogtmann, E., Liu, A., Qin, J., Chen, W., Abnet, C. C., et al. (2019). Microbial characterization of esophageal squamous cell carcinoma and gastric cardia adenocarcinoma from a high-risk region of China. Cancer 125, 3993–4002. doi: 10.1002/cncr.32403
Sheh, A., and Fox, J. G. (2013). The role of the gastrointestinal microbiome in Helicobacter pylori pathogenesis. Gut Microbes 4, 505–531. doi: 10.4161/gmic.26205
Shen, Z., Dzink-Fox, J. A., Feng, Y., Muthupalani, S., Mannion, A. J., Sheh, A., et al. (2022). Gastric non-Helicobacter pylori urease-positive Staphylococcus epidermidis and streptococcus salivarius isolated from humans have contrasting effects on H. pylori-associated gastric pathology and host immune responses in a murine model of gastric cancer. mSphere 7:e0077221. doi: 10.1128/msphere.00772-21
Sheu, B. S., Wu, J. J., Lo, C. Y., Wu, H. W., Chen, J. H., Lin, Y. S., et al. (2002). Impact of supplement with lactobacillus- and Bifidobacterium-containing yogurt on triple therapy for Helicobacter pylori eradication. Aliment. Pharmacol. Ther. 16, 1669–1675. doi: 10.1046/j.1365-2036.2002.01335.x
Smyth, E. C., Nilsson, M., Grabsch, H. I., van Grieken, N. C. T., and Lordick, F. (2020). Gastric cancer. Lancet 396, 635–648. doi: 10.1016/S0140-6736(20)31288-5
Sohn, S. H., Kim, N., Jo, H. J., Kim, J., Park, J. H., Nam, R. H., et al. (2017). Analysis of gastric body microbiota by pyrosequencing: possible role of bacteria other than Helicobacter pylori in the gastric carcinogenesis. J. Cancer Prev. 22, 115–125. doi: 10.15430/JCP.2017.22.2.115
Suerbaum, S., and Michetti, P. (2002). Medical progress: Helicobacter pylori infection. N. Engl. J. Med. 347, 1175–1186. doi: 10.1056/NEJMra020542
Sun, Q. H., Zhang, J., Shi, Y. Y., Zhang, J., Fu, W. W., and Ding, S. G. (2022). Microbiome changes in the gastric mucosa and gastric juice in different histological stages of Helicobacter pylori-negative gastric cancers. World J. Gastroenterol. 28, 365–380. doi: 10.3748/wjg.v28.i3.365
Suzuki, K., Meek, B., Doi, Y., Muramatsu, M., Chiba, T., Honjo, T., et al. (2004). Aberrant expansion of segmented filamentous bacteria in IgA-deficient gut. Proc. Natl. Acad. Sci. U. S. A. 101, 1981–1986. doi: 10.1073/pnas.0307317101
Targosz, A., Brzozowski, T., Pierzchalski, P., Szczyrk, U., Ptak-Belowska, A., Konturek, S. J., et al. (2012). Helicobacter pylori promotes apoptosis, activates cyclooxygenase (COX)-2 and inhibits heat shock protein HSP70 in gastric cancer epithelial cells. Inflamm. Res. 61, 955–966. doi: 10.1007/s00011-012-0487-x
Tseng, C. H., Lin, J. T., Ho, H. J., Lai, Z. L., Wang, C. B., Tang, S. L., et al. (2016). Gastric microbiota and predicted gene functions are altered after subtotal gastrectomy in patients with gastric cancer. Sci. Rep. 6:20701. doi: 10.1038/srep20701
Tsuda, A., Suda, W., Morita, H., Takanashi, K., Takagi, A., Koga, Y., et al. (2015). Influence of proton-pump inhibitors on the luminal microbiota in the gastrointestinal tract. Clin. Transl. Gastroenterol. 6:e89. doi: 10.1038/ctg.2015.20
Udhayakumar, G., Jayanthi, V., Devaraj, N., and Devaraj, H. (2007). Interaction of MUC1 with beta-catenin modulates the Wnt target gene cyclinD1 in H. pylori-induced gastric cancer. Mol. Carcinog. 46, 807–817. doi: 10.1002/mc.20311
Uemura, N., Okamoto, S., Yamamoto, S., Matsumura, N., Yamaguchi, S., Yamakido, M., et al. (2001). Helicobacter pylori infection and the development of gastric cancer. N. Engl. J. Med. 345, 784–789. doi: 10.1056/NEJMoa001999
Walter, J., and Ley, R. (2011). The human gut microbiome: ecology and recent evolutionary changes. Annu. Rev. Microbiol. 65, 411–429. doi: 10.1146/annurev-micro-090110-102830
Wang, Z. H., Gao, Q. Y., and Fang, J. Y. (2013). Meta-analysis of the efficacy and safety of lactobacillus-containing and Bifidobacterium-containing probiotic compound preparation in Helicobacter pylori eradication therapy. J. Clin. Gastroenterol. 47, 25–32. doi: 10.1097/MCG.0b013e318266f6cf
Wang, Z., Ren, R., and Yang, Y. (2020a). Mucosa microbiome of gastric lesions: fungi and bacteria interactions. Prog. Mol. Biol. Transl. Sci. 171, 195–213. doi: 10.1016/bs.pmbts.2020.03.004
Wang, L., Xin, Y., Zhou, J., Tian, Z., Liu, C., Yu, X., et al. (2020). Gastric mucosa-associated microbial signatures of early gastric cancer. Front. Microbiol. 11:1548. doi: 10.3389/fmicb.2020.01548
Wang, L., Zhou, J., Xin, Y., Geng, C., Tian, Z., Yu, X., et al. (2016). Bacterial overgrowth and diversification of microbiota in gastric cancer. Eur. J. Gastroenterol. Hepatol. 28, 261–266. doi: 10.1097/MEG.0000000000000542
Wang, Z., Gao, X., Zeng, R., Wu, Q., Sun, H., Wu, W., et al. (2020b). Changes of the gastric mucosal microbiome associated with histological stages of gastric carcinogenesis. Front. Microbiol. 11:997. doi: 10.3389/fmicb.2020.00997
Wroblewski, L. E., Peek, R. M. Jr., and Wilson, K. T. (2010). Helicobacter pylori and gastric cancer: factors that modulate disease risk. Clin. Microbiol. Rev. 23, 713–739. doi: 10.1128/CMR.00011-10
Wroblewski, L. E., Piazuelo, M. B., Chaturvedi, R., Schumacher, M., Aihara, E., Feng, R., et al. (2015). Helicobacter pylori targets cancer-associated apical-junctional constituents in gastroids and gastric epithelial cells. Gut 64, 720–730. doi: 10.1136/gutjnl-2014-307650
Wu, J., Li, Q., and Fu, X. (2019). Fusobacterium nucleatum contributes to the carcinogenesis of colorectal cancer by inducing inflammation and suppressing host immunity. Transl. Oncol. 12, 846–851. doi: 10.1016/j.tranon.2019.03.003
Wu, J., Xu, S., Xiang, C., Cao, Q., Li, Q., Huang, J., et al. (2018). Tongue coating microbiota community and risk effect on gastric cancer. J. Cancer 9, 4039–4048. doi: 10.7150/jca.25280
Wu, Z. F., Zou, K., Wu, G.-N., Jin, Z.-J., Xiang, C.-J., Xu, S., et al. (2020). A comparison of tumor-associated and non-tumor-associated gastric microbiota in gastric cancer patients. Dig. Dis. Sci. 66, 1673–1682. doi: 10.1007/s10620-020-06415-y
Yamaguchi, N., and Kakizoe, T. (2001). Synergistic interaction between Helicobacter pylori gastritis and diet in gastric cancer. Lancet Oncol. 2, 88–94. doi: 10.1016/S1470-2045(00)00225-4
Yanaka, A., Fahey, J. W., Fukumoto, A., Nakayama, M., Inoue, S., Zhang, S., et al. (2009). Dietary sulforaphane-rich broccoli sprouts reduce colonization and attenuate gastritis in Helicobacter pylori-infected mice and humans. Cancer Prev. Res. (Phila.) 2, 353–360. doi: 10.1158/1940-6207.CAPR-08-0192
Yang, I., Woltemate, S., Piazuelo, M. B., Bravo, L. E., Yepez, M. C., Romero-Gallo, J., et al. (2016). Different gastric microbiota compositions in two human populations with high and low gastric cancer risk in Colombia. Sci. Rep. 6:18594. doi: 10.1038/srep18594
Ye, F., Shen, H., Li, Z., Meng, F., Li, L., Yang, J., et al. (2016). Influence of the biliary system on biliary bacteria revealed by bacterial communities of the human biliary and upper digestive tracts. PLoS One 11:e0150519. doi: 10.1371/journal.pone.0150519
Yin, Y. N., Wang, C. L., Liu, X. W., Cui, Y., Xie, N., Yu, Q. F., et al. (2011). Gastric and duodenum microflora analysis after long-term Helicobacter pylori infection in Mongolian gerbils. Helicobacter 16, 389–397. doi: 10.1111/j.1523-5378.2011.00862.x
Yong, X., Tang, B., Li, B. S., Xie, R., Hu, C. J., Luo, G., et al. (2015). Helicobacter pylori virulence factor CagA promotes tumorigenesis of gastric cancer via multiple signaling pathways. Cell Commun. Signal 13:30. doi: 10.1186/s12964-015-0111-0
Yu, G., Hu, N., Wang, L., Wang, C., Han, X. Y., Humphry, M., et al. (2017b). Gastric microbiota features associated with cancer risk factors and clinical outcomes: a pilot study in gastric cardia cancer patients from Shanxi, China. Int. J. Cancer 141, 45–51. doi: 10.1002/ijc.30700
Yu, G., Torres, J., Hu, N., Medrano-Guzman, R., Herrera-Goepfert, R., Humphrys, M. S., et al. (2017a). Molecular characterization of the human stomach microbiota in gastric cancer patients. Front. Cell. Infect. Microbiol. 7:302. doi: 10.3389/fcimb.2017.00302
Zhang, C., Powell, S. E., Betel, D., and Shah, M. A. (2017). The gastric microbiome and its influence on gastric carcinogenesis: current knowledge and ongoing research. Hematol. Oncol. Clin. North Am. 31, 389–408. doi: 10.1016/j.hoc.2017.01.002
Zhang, Z., Xu, G., Ma, M., Yang, J., and Liu, X. (2013). Dietary fiber intake reduces risk for gastric cancer: a meta-analysis. Gastroenterology 145, 113–20.e3. doi: 10.1053/j.gastro.2013.04.001
Zhao, Z., Yin, Z., and Zhao, Q. (2017). Red and processed meat consumption and gastric cancer risk: a systematic review and meta-analysis. Oncotarget 8, 30563–30575. doi: 10.18632/oncotarget.15699
Keywords: gastric cancer, H. pylori , gastric microbiota, non-H. pylori, microbiome diversity
Citation: Zhou S, Li C, Liu L, Yuan Q, Miao J, Wang H, Ding C and Guan W (2023) Gastric microbiota: an emerging player in gastric cancer. Front. Microbiol. 14:1130001. doi: 10.3389/fmicb.2023.1130001
Edited by:
Paula Roszczenko-Jasinska, University of Warsaw, PolandReviewed by:
Dong Yang, Tianjin Institute of Environmental and Operational Medicine, ChinaKathryn Haley, Grand Valley State University, United States
Copyright © 2023 Zhou, Li, Liu, Yuan, Miao, Wang, Ding and Guan. This is an open-access article distributed under the terms of the Creative Commons Attribution License (CC BY). The use, distribution or reproduction in other forums is permitted, provided the original author(s) and the copyright owner(s) are credited and that the original publication in this journal is cited, in accordance with accepted academic practice. No use, distribution or reproduction is permitted which does not comply with these terms.
*Correspondence: Wenxian Guan, bWVkZ3Vhbnd4QDE2My5jb20=; Chao Ding, ZGluZ2NoYW8xOTkxMDUyMUAxMjYuY29t; Hao Wang, d2FuZ2hhbzAzNDlAc2luYS5jb20=
†These authors have contributed equally to this work