- 1Department of Laboratory Medicine, Nanjing Drum Tower Hospital, The Affiliated Hospital of Nanjing University Medical School, Nanjing, Jiangsu, China
- 2Department of Nosocomial Infection Control, Nanjing Drum Tower Hospital, The Affiliated Hospital of Nanjing University Medical School, Nanjing, Jiangsu, China
- 3Department of Acute Infectious Disease Control and Prevention, Jiangsu Provincial Center for Disease Control and Prevention, Nanjing, China
Objective: To use genomic analysis to identify Acinetobacter spp. and to explore the distribution characteristics of ß-lactamase oxallicinases (blaOXA) among Acinetobacter species globally.
Methods: Genomes of global Acinetobacter spp. were downloaded from GenBank using Aspera batch. After quality check using CheckM and QUAST software, the genomes were annotated using Prokka software to investigate the distribution of blaOXAs across Acinetobacter spp.; a phylogenetic tree was constructed to explore the evolutionary relationship among the blaOXA genes in Acinetobacter spp. Average-nucleotide identification (ANI) was performed to re-type the Acinetobacter spp. BLASTN comparison analysis was implemented to determine the sequence type (ST) of Acinetobacter baumannii strain.
Results: A total of 7,853 genomes were downloaded, of which only 6,639 were left for further analysis after quality check. Among them, 282 blaOXA variants were identified from the genomes of 5,893 Acinetobacter spp.; blaOXA-23 (n = 3,168, 53.8%) and blaOXA-66 (2,630, 44.6%) were the most frequent blaOXAs, accounting for 52.6% (3,489/6639), and the co-carriage of blaOXA-23 and blaOXA-66 was seen in 2223 (37.7%) strains. The 282 blaOXA variants were divided into 27 clusters according to the phylogenetic tree. The biggest clade was blaOXA-51-family carbapenem-hydrolyzing enzymes composed of 108 blaOXA variants. Overall, 4,923 A. baumannii were identified out of the 6,639 Acinetobacter spp. strains and 291 distinct STs were identified among the 4,904 blaOXA-carrying A. baumannii. The most prevalent ST was ST2 (n = 3,023, 61.6%) followed by ST1 (n = 228, 4.6%).
Conclusion: OXA-like carbapenemases were the main blaOXA-type β-lactamase spread widely across Acinetobacter spp. Both blaOXA-23 and blaOXA-66 were the predominant blaOXAs, among all A. baumannii strains, with ST2 (belonging to CC2) being the main clone disseminated globally.
1. Introduction
Acinetobacter spp. is one of the most frequent non-fermentative gram-negative coccobacilli that is widely distributed among humans as well as in the external environment. It predominantly colonizes and infects hospitalized patients, with a variety of nosocomial infections implicated (Bergogne-Bérézin and Towner, 1996). High rates of antibiotic resistance in Acinetobacter spp. have been documented in numerous reports, with strains possessing OXA-type carbapenem-hydrolyzing β-lactamases (CHβLs) being particularly of concern (Lee et al., 2016). Infections caused by such strains are often extremely difficult to eradicate due to their widespread resistance to the major groups of antimicrobial agents.
Currently, Acinetobacter spp. has been assigned into 144 different Acinetobacter species, including 68 with species names and 76 unnamed taxa., with A. baumannii, A. nosocomialis, and A. pittii being the most frequent ones in healthcare setting (Lupo et al., 2018; Qin et al., 2021). With the rapid development of matrix-associated laser desorption ionization–time of flight mass spectrometry (MALDI-TOF MS) and whole genome sequencing (WGS) technology, the current classification of Acinetobacter spp. urgently needs to be updated, since different species have been reported to cause different infections and show different drug resistance characteristics (Antunes et al., 2014; Zajmi et al., 2022; Longo et al., 2023).
Till date, A. baumannii has been found to be of the greatest clinical importance among Acinetobacter species, owing to its association with a variety of nosocomial infections, including pneumonia, bacteremia, urinary tract infection, and secondary meningitis (Lee et al., 2017). Specifically, ventilator-associated pneumonia caused by such strains in intensive care units (ICUs) is of great concern (Harding et al., 2018); even more worrying is the extremely and rapidly developed drug-resistance of the strains, with extensive drug-resistance and pan-drug-resistance of A. baumannii being a public health threat (Magiorakos et al., 2012). Moreover, such strains possess the capacity of long-term survival, resulting in enhanced opportunities of transmission across patients (Antunes et al., 2014).
Multiple investigations have shown that diverse OXA β-lactamases, especially the carbapenem-hydrolyzing oxacillinase (Tooke et al., 2019), play quite important roles in the resistance of A. baumannii (Kengkla et al., 2018). In particular, blaOXA-23, blaOXA-24, blaOXA-51, and blaOXA-58 have been found to be primarily associated with carbapenem resistance in A. baumannii, with blaOXA-23 being the most widespread gene in most countries (Khurshid et al., 2020; Wang et al., 2021), and blaOXA-24 and blaOXA-58 being the dominant genes in specific regions (Salehi et al., 2019). blaOXA-51 is a chromosomally encoded β-lactamase that has been demonstrated to be universally present in all A. baumannii strains, and resistance to carbapenems have been reported when the genetic environment around the gene promoted the expression of blaOXA-51 gene (Takebayashi et al., 2021). Furthermore, global clone groups 1 (ST1) and 2 (ST2) have been the two major clonal groups of carbapenem resistance spreading globally (Douraghi et al., 2020; Palmieri et al., 2020).
In recent years, the number of blaOXA variants have been continuously increasing. Correspondingly, the grouping of blaOXA enzymes has also changed based on amino acid sequence similarity (Walther-Rasmussen and Høiby, 2006). Till date, more than multiple blaOXA variants have been identified (Boyd et al., 2022; https://www.ncbi.nlm.nih.gov/gene/?term=OXA). However, the prevalence and evolution of blaOXAs among Acinetobacter spp. have remained unknown as the major β-lactamase, and whether the dissemination of blaOXA genes is related to specific clones would require further exploration.
In this study, we aimed to analyze the prevalent distribution of blaOXAs among global Acinetobacter spp. from all publicly available genome sequences. A phylogenetic tree of blaOXA variants was constructed to explore the evolutionary relationship among them. Furthermore, all the Acinetobacter spp. analyzed were identified by average nucleotide identity (ANI) comparison, and sequence types (STs) of the blaOXA-carrying A. baumannii were explored to investigate the relationship between prevalent blaOXAs and predominant STs.
2. Materials and methods
2.1. Download of Acinetobacter spp. genomes
A total of 7,853 Acinetobacter spp. genomes were downloaded from NCBI genome database1 using Aspera, on December 21, 2021. The genomic quality of the 7,853 strains was filtered by CheckM and QUAST software (Gurevich et al., 2013; Parks et al., 2015). The conditions for being considered a high-quality genome included completeness ≥ 90% and contamination < 5%. The quantity of contig was required to be ≤500 bp and N50 ≥ 40,000 bp; thus, 1,214 genomes that did not meet the above conditions were filtered out.
2.2. Investigation of blaOXAs across Acinetobacter spp.
Prokka (Seemann, 2014) is a fast prokaryotic genome annotation software that was used to annotate the genomes of all 6,639 strains in our study, in order to avoid the differences in genome gene prediction by different annotation methods. Distributions of blaOXA for each genome were determined by Blast analysis using a self-building blaOXA database retrieved from the National Database of Antibiotic Resistant Organisms.2 Genomes harboring blaOXA were selected for further analysis.
2.3. Phylogenetic tree of blaOXA variants within Acinetobacter spp.
MUSCLE version 3.8.31 (Edgar, 2004) was used for nucleotide sequence alignment of 282 blaOXA genes. Then, the generated multiple sequence alignment file was used to build a maximum likelihood (ML) phylogenetic tree by RAxML version 8.2.11 (Alexandros and Stamatakis, 2014), with Bootstrap being set as 500 and M (model setting) being selected as “GTRCAT.” Finally, this tree was visualized with iTOL software (Letunic and Bork, 2019).
2.4. Species identification of Acinetobacter spp.
Average-nucleotide identification was performed for the genomes of all 6,639 strains (Jain et al., 2018; Supplementary Table S1), and 95% was set as the cutoff value for species identification. Briefly, the genome sequences of type strains for Acinetobacter were obtained based on the NCBI type strain list. FastANI version 1.3 (Jain et al., 2018) was used to calculate the ANI values with all 6,639 genomes were as query, and all genomes of type strains belonging to this genus as reference. When the ANI value between a query genome and the type strain genome was greater than or equal to 95%, this query genome were given the same species name with the type strain. When the ANI value was less than 95%, this query genome was treated as Acinetobacter spp.
2.5. Analysis of the sequence types of Acinetobacter baumannii
A self-made Perl program was used to extract the nucleotide coding sequence of the gene from each A. baumannii genome sequence file (GBK format). Concurrently, seven allele sequences sequences and the MLST (Pasteur) profiles of A. baumannii were downloaded.3 The sequence type (ST) for each genome was determined as follows: (1) all genes for each genome were searched against the housekeeping gene sequences via BLASTN, and the blast results were filtered with stringent criteria (E-value = 1e−5, identity = 100%, and coverage = 100%) to obtain the seven conserved gene-type profiles; (2) this conserved gene-type profiles in each genome were compared with the MLST profiles to determine the ST for each genome.
2.6. Strain meta information acquisition
Strain meta information including isolation country, and date, host, and source, etc. was extracted from the downloaded gbk file in batches using perl script. Species identification, OXA distribution as well as STs were integrated in an excel for the further analysis.
2.7. Statistical analysis
SPSS software was used for statistical analysis, and chi-square test was used to compare the difference in distribution of blaOXA between A. baumannii and non-A. baumannii Acinetobacter spp.; p < 0.05 was taken as significant.
3. Results
3.1. Distribution of blaOXA across global Acinetobacter spp.
Out of the 6,639 Acinetobacter spp., 5,893 (88.8%) strains were found to carry 9,581 blaOXAs, which were assigned to 282 blaOXA variants (Figure 1), with blaOXA-23 (n = 3,168, 47.7%) and blaOXA-66 (n = 2,630, 39.6%) being the most predominant blaOXAs. In addition, blaOXA-82 (n = 571, 8.6%), blaOXA-69 (n = 302, 4.5%), blaOXA-58 (n = 202, 3.0%), blaOXA-72 (n = 192, 2.9%), blaOXA-64 (n = 175, 2.6%), and blaOXA-65 (n = 169, 2.5%) were found to be common. Other blaOXAs were scattered, as shown in the Figure 1. Globally, the earliest enzyme identified was blaOXA-78 in 1943 in the United States. In 1980s, blaOXA-64 and blaOXA-69 were the main OXA variants, whereas blaOXA-214, blaOXA-235, blaOXA-500, and blaOXA-506 emerged successively in 1990s. Each Acinetobacter spp. seemed to have contained only one blaOXA before 1996, and subsequently co-existence of two blaOXA variants appeared. Since the identification of the first combination of blaOXA-23 and blaOXA-66 in A. baumannii in Singapore in 1996, it has been increasingly prevalent each year (Figure 2; Supplementary Table S2). The combinations of blaOXA variants detected were diverse, and distributed all over the world; a combination of three distinct blaOXA variants appeared in the cerebrospinal fluid of an inpatient in Italy in 2005.
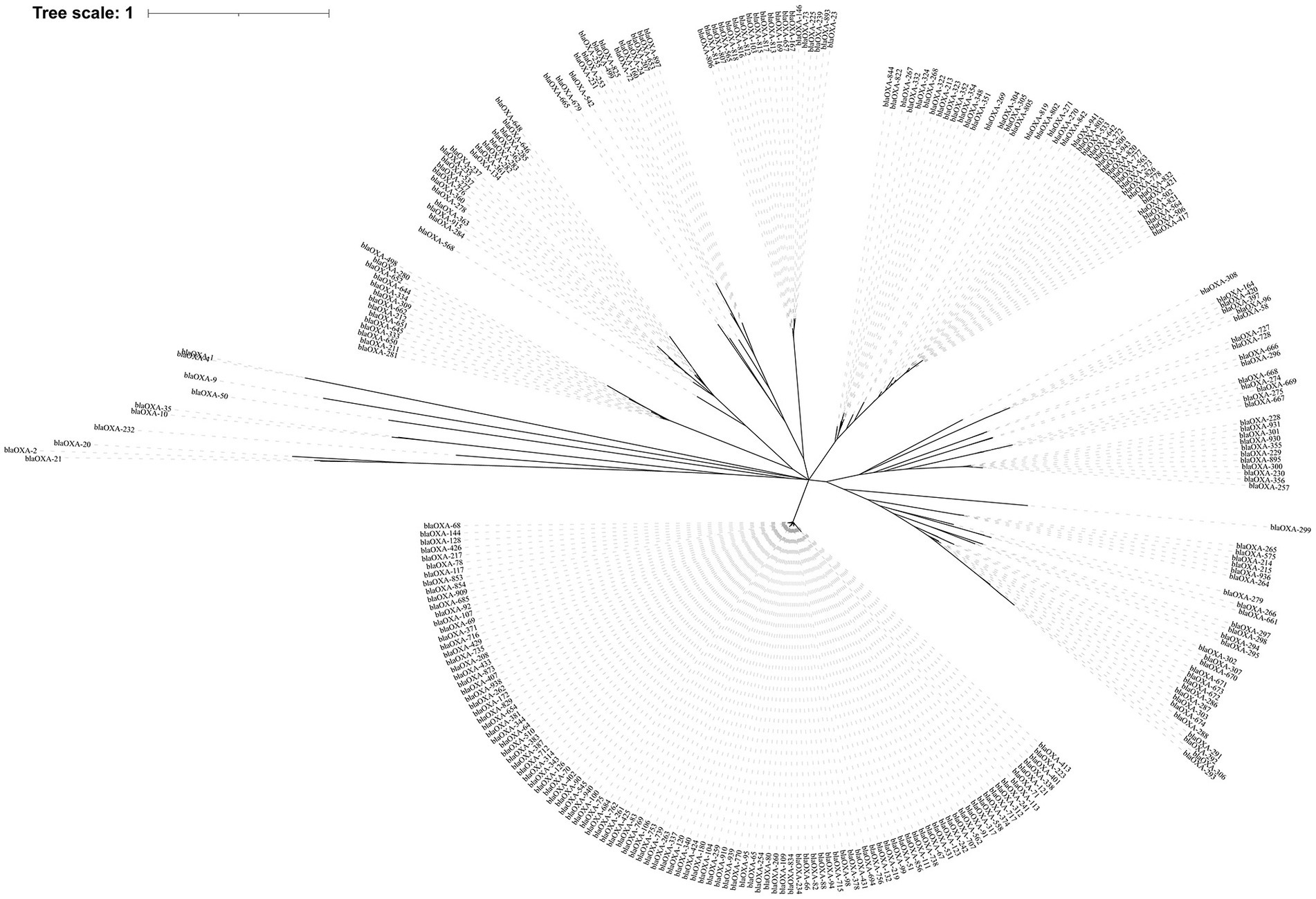
Figure 1. The phylogenetic tree of 282 blaOXA variants detected across global Acinetobacter spp. The phylogenetic tree was constructed using MUSCLE and RAxML software, and finally visualized with iTOL software. The clades were calculated based on the function of OXA variants.
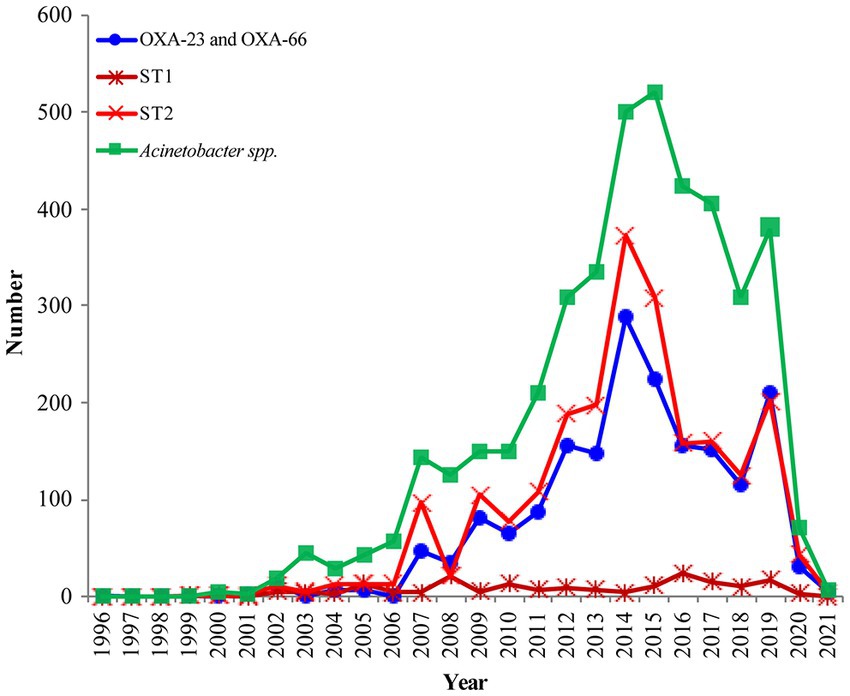
Figure 2. The prevalence of OXA-23 and OXA-66 combination in global Acinetobacter baumannii (The blue line); The distribution of OXA-producing Acinetobacter baumannii ST1 and ST2 during 1999–2021 (The dark red line and light red line); Analysis of the submitted and released genomes of Acinetobacter spp. per year (The green line).
Functionally, the 282 blaOXA variants were assigned to three classes, 239 being carbapenem-hydrolyzing-class-D-β-lactamase (Table 1), and nine belonging to oxacillin-hydrolyzing-class-D-β-lactamase (Table 2); whether the remaining 34 blaOXA variants possessed carbapenem-hydrolyzing activity would require further analysis (Table 3).
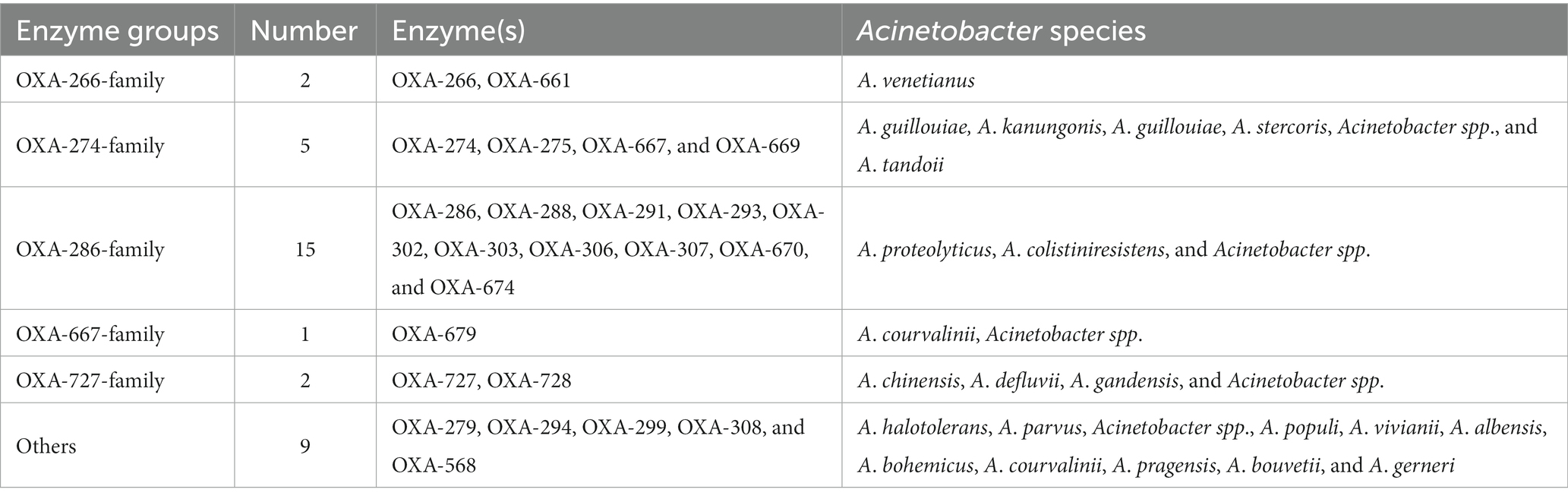
Table 3. Class-D-β-lactamase could not be assigned into detailed classification in Acinetobacter spp.
3.2. Phylogenetic tree of blaOXA variants across Acinetobacter spp.
Phylogenetically, the blaOXA variants within A. baumannii were divided into 27 distantly related clusters, according to the phylogenetic tree constructed based on SNPs (Figure 1). Among the 27 clades, 11 (including 239 blaOXA variants) belonged to carbapenem-hydrolyzing-class-D-β-lactamase (Figure 1; Table 1). Six clades, including nine blaOXA variants, belonged to oxacillin-hydrolyzing-class-D-β-lactamase (Figure 1; Table 2), and 10, including 34 blaOXA variants, were unknown with regard to antibiotic-hydrolyzing activity (Figure 1; Table 3). It was noteworthy that seven clades consisted of single blaOXA gene, namely blaOXA-9, blaOXA-50, blaOXA-232, blaOXA-568, blaOXA-542, blaOXA-308, and blaOXA-299. Moreover, two clades were unnamed, one group consisting of blaOXA-296 and blaOXA-666, and another group consisting of blaOXA-294, blaOXA-295, blaOXA-297, and blaOXA-298 (Table 2).
3.3. Species assignment of Acinetobacter spp.
Average-nucleotide identification calculations based on BLAST+ (ANIb) analysis (Richter et al., 2016) showed that 6,417 out of 6,639 Acinetobacter spp. belonged to 70 species while the remaining 222 strains could not be identified due to limitation in typing strains (Supplementary Table S1). Furthermore, the 5,893 blaOXA-carrying Acinetobacter spp. were assigned to 52 species, indicating that blaOXA was not identified in at least 18 Acinetobacter species that included A. baretiae (n = 2), A. baylyi (n = 11), A. brisouii (n = 4), A. celticus (n = 1), A. equi (n = 1), A. guerrae (n = 3), A. harbinensis (n = 2), A. lanii (n = 2), A. larvae (n = 1), A. nectaris (n = 1), A. pollinis (n = 4), A. rathckeae (n = 1), A. soli (n = 35), A. terrae (n = 8), A. terrestris (n = 5), A. tjernbergiae (n = 2), A. ursingii (n = 56), and A. wanghuae (n = 2). Further, we observed that the distribution of blaOXAs across Acinetobacter species was different, the most common species being A. baumannii (n = 4,904) followed by A. pittii (n = 299), which was then followed by A. bereziniae (n = 47), A. haemolyticus (n = 39), A. johnsonii (n = 40), A. lwoffii (n = 37), A. oleivorans (n = 34), and A. radioresistens (n = 43; Table 4). Other species were quite rare, as shown in Supplementary Table S1. Notably, 174 blaOXA-carrying Acinetobacter spp. could not be assigned to specific species due to the limited type strains in GenBank.
It would be worth noting that the most prevalent blaOXA variants were different across the Acinetobacter species (Table 4). For example, blaOXA-23 and blaOXA-66 were the predominant blaOXA variants in A. baumannii, whereas blaOXA-500 and blaOXA-506 were the dominant ones in A. pittii. Of note, more than two blaOXA variants were identified across 3,615 strains, and 2,223 were found to simultaneously carry blaOXA-23 and blaOXA-66; co-carriage of blaOXA-23 and blaOXA-66 was only found in A. baumannii. The other difference was that blaOXA-66 was only identified in A. baumannii, whereas blaOXA-23 was not only detected in A. baumannii (n = 3,129), but also in A. cumulans (n = 2), A. gandensis (n = 2), A. indicus (n = 2), A. johnsonii (n = 1), A. nosocomialis (n = 4), A. pittii (n = 6), A. radioresistens (n = 10), A. seifertii (n = 3), and A. wuhouensis (n = 1).
Albeit there was a wide distribution of blaOXAs across A. bereziniae, A. haemolyticus, A. johnsonii, A. lwoffii, A. oleivorans, A. pittii, A. radioresistens, and A. baumannii (Table 4), in general, the prevalence of blaOXAs among A. baumannii (4,904/4,923, 99.6%) was significantly higher than that of blaOXAs among non-A. baumannii Acinetobacter spp. (989/1716, 57.6%, p = 0.000).
The distribution of carbapenem-hydrolyzing-class-D-β-lactamases showed that blaOXA-51 family, the biggest clade, was only found in A. baumannii and A. johnsonii. Other carbapenemases, including blaOXA-134, blaOXA-211, blaOXA-213, blaOXA-214, and blaOXA-229 families, as well as other enzymes not assigned into a specific family were not found in A. baumannii.
3.4. Sequence types of blaOXA-carrying Acinetobacter baumannii
A total of 291 distinct STs were identified for 4,904 blaOXA-carrying A. baumannii. The most identified ST was ST2 (n = 3,023), which was classified as clonal complex 2 (CC2), presenting a global distribution trend. ST1 (n = 228; clonal complex CC1) and ST25 (n = 132), which were the second most common, corresponded to CC2/92 (Pasteur/Oxford scheme; Figure 3A), other distinct STs (ST79, ST78, and ST10) were relatively less common (Figure 3A). The STs of 29 strains were novel (the profile of MLST gene was not assigned into specific ST), and the STs of 125 strains remained unknown (the STs could not be determined since some genes did not match the known MLST loci).
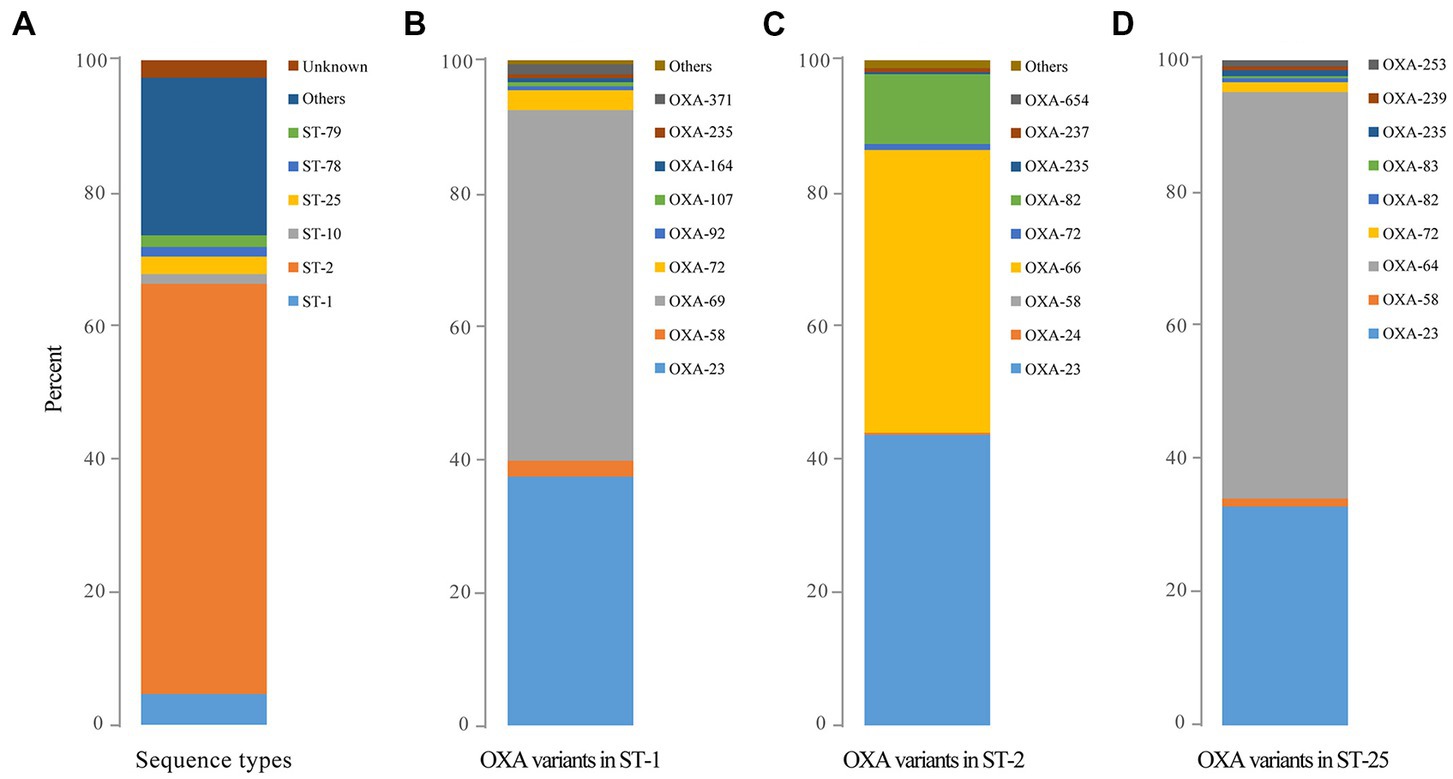
Figure 3. The predominant sequence types of global OXA-producing Acinetobacter baumannii in 1996, 1999, and during 2001–2021 as well as the distribution of OXA variants among the three dominant clones of Acinetobacter baumannii. (A) The predominant sequence types of global OXA-producing Acinetobacter baumannii in 1996, 1999, and during 2001–2021; (B) The distribution of OXA variants among Acinetobacter baumannii ST1; (C) The distribution of OXA variants among Acinetobacter baumannii ST2; and (D) The distribution of OXA variants among Acinetobacter baumannii ST25.
The earliest detected clone in A. baumannii was ST2 collected in Rotterdam, Netherlands, in 1982 from Homo sapiens, which was further found in 1996 in Singapore. The clonal ST2 was continuously and increasingly detected every year all over the world since its first detection in Beijing, China, in 1999 (Figure 2, The light red line). Additionally, ST1 was consecutively collected almost each year, except in 1985, 1995, and 2001 (Figure 2, The dark red line); however, the number was quite less compared to that of ST2 (Figure 2, The light red line).
Ninety-two distinct STs were identified for blaOXA-23-carrying strains, with ST2 (n = 2,429) being the most prevalent clone, followed by ST1 (n = 154). Meanwhile, 25 different STs were found for 2,663 blaOXA-66-carrying A. baumannii with ST2 (n = 2,425) being the most common. Notably, 2081 blaOXA-23 and blaOXA-66 co-carrying A. baumannii belonged to ST2 while the other 22 STs were also identified.
Obvious differences were also observed among the major STs. blaOXA-23 (n = 2,429) and blaOXA-66 (n = 2,374) were the main blaOXAs within ST2, whereas blaOXA-69 (n = 217) and blaOXA-23 (n = 154) were the predominant blaOXAs among ST1, and blaOXA-64 (n = 128) was the dominant blaOXA among ST25 clones (Figures 3B–D).
3.5. Temporal and geographical distribution of global blaOXA-carrying Acinetobacter spp.
The earliest OXA-producing Acinetobacter spp. could be dated back to 1943, and was carried by an A. baumannii from Parthenium argentatum Gray (guayule shrubs) in the United States. The blaOXA-producing Acinetobacter spp. was intermittently isolated over the following years: 1948 (n = 2), 1951 (n = 1), 1953 (n = 1), 1982 (n = 2), 1984 (n = 1), 1985 (n = 1), and 1986 (n = 1), and was found to continuously increase during 1991 to 2021, although the isolation date for 1,585 strains still remains unknown (Figure 2). Overall, the strains were collected from 79 countries (Figure 2; Supplementary Table S3), of which, United States (n = 1,315) and China (n = 1,191) submitted the most number of strains, followed by Germany (n = 205), India (n = 279), South Korea (n = 145), Brazil (n = 134), and France (n = 101; Figure 4; Supplementary Table S3). Unfortunately, the countries from where the other 1,345 strains were collected remain unknown. Furthermore, Homo sapiens were the most common host. The Acinetobacter spp. isolated only from non-Homo sapiens were A. chengduensis, A. chinensis, A. cumulans, A. gandensis, A. kanungonis, A. modestus, A. piscicola, A. populi, A. pragensis, A. pseudolwoffii, A. rongchengensis, A. pullicarnis, A. shaoyimingii, A. sichuanensis, A. terrestris, A. tianfuensis, A. towneri, A. variabilis, and A. wuhouensis.
The distribution of blaOXAs displayed significant regional differences. blaOXA-23 and blaOXA-66, in combination, were the main blaOXAs in Asian regions, such as China, South Korea, Singapore, Pakistan, Malaysia, Lebanon, Kuwait, Thailand, and India. However, blaOXA-23 and blaOXA-82 were the predominant ones in United States.
4. Discussion
In this study, we analyzed the data available on the distribution of blaOXAs across the genomes of the Acinetobacter spp. available in the GenBank, and the evolutionary relationship among the blaOXA variants based on the global genomes of Acinetobacter spp. Furthermore, the relationship between blaOXA variants and STs in A. baumannii was analyzed.
First, consistent with the previous report, we found a wide distribution of diverse OXA-type β-lactamases across the Acinetobacter spp., especially in A. baumannii, indicating that A. baumannii might be the main host of blaOXA genes. It was noteworthy that most of the OXA-type β-lactamase identified in our study belonged to carbapenem-hydrolyzing-β-lactamase, leading to high resistance of Acinetobacter spp. to carbapenem in different degrees (Boral et al., 2019; Tamma et al., 2022). Evidently, the wide distribution of blaOXA-23 and blaOXA-66 across global A. baumannii, in our study, suggested that they may be the main enzyme mediating carbapenem resistance, since the expression of blaOXA-23 within the A. baumannii strain was enough to confer resistance to carbapenems (Evans and Amyes, 2014); however, a much higher turnover rate was observed for imipenem than for meropenem, ertapenem, or doripenem for blaOXA-23 (Smith et al., 2013). In addition, a recent study showed that hyperexpression of blaOXA-23 β-Lactamase in A. baumannii drives significant collateral changes with increased amidase activity, resulting in peptidoglycan integrity and new genetic vulnerabilities (Colquhoun et al., 2021), which may represent novel targets for antimicrobial agents. blaOXA-66 is well known as a chromosomally encoded blaOXA-51-like β-lactamase, and the most prevalent CHβLs in A. baumannii worldwide (Hu et al., 2007). Therefore, the wide co-occurrence of blaOXA-23 and blaOXA-66 in combination with other diverse carbapenem-hydrolyzing enzymes within A. baumannii provides enough explanation for the failure of β-lactam in clinical therapy. Currently, many studies have shown frequent co-carriage of blaOXA-23 and blaOXA-66 among clinical A. baumannii, with high resistance rates (Al-Hassan et al., 2021; Zhang et al., 2021), and the continuously rising co-prevalence of blaOXA-23 and blaOXA-66 over the years indicates the importance of the two variants for high resistance of A. baumannii to carbapenem.
In our study, blaOXA-78 (OXA-51 family) was the earliest blaOXA in A. baumannii isolated in 1943 in United States. However, searching blaOXA-78 as a keyword in PubMed, it was first reported in a clinical multi-drug resistant A. baumannii from a hospitalized patient in a major hospital in Kuwait in 2015 (Vali et al., 2015). As is well known, carbapenem was approved for clinical use in 1980s, indicating that class-D-CHßLs had already existed in A. baumannii before carbapenem usage. Analysis from our study showed that blaOXA-51 family, including blaOXA-66, blaOXA-69, and blaOXA-98, appeared in bacterial genomes submitted in the 1980s, and OXA-213 family, including blaOXA-500, blaOXA-506, and blaOXA-417, emerged in strains collected in the 1990s, although the blaOXAs were first reported in 2007 (Zhou et al., 2007), and blaOXA-69 in 2005 (Héritier et al., 2005), blaOXA-417 in 2014 (D'Souza et al., 2019), and blaOXA-500 in 2019 (Sun et al., 2014), indicating the presence of these blaOXA before the use of carbapenems. Whether there was an association between the evolution of class D CHβLs and the carbapenem use would require further investigation. Importantly, all the blaOXA-48-like CHβLs, were quite rare, except blaOXA-232, which was quite popular in Enterobacterales (Pitout et al., 2019). This could be due to the good fitness between blaOXA-48 and Enterobacterales, especially Klebsiella pneumoniae.
In our study, the clades of blaOXA variants were in accordance with the subgroups categorized by enzyme function, from an overall perspective, and blaOXA-51 family included the most members. However, not all the A. baumannii isolates contained blaOXA-51-family genes, providing evidence that this gene was not omnipresent in the species, but rather distributed in subpopulations of A. baumannii (Walther-Rasmussen and Høiby, 2006). Nevertheless, the members of this family were reported to diverge by amino acid modifications in A. baumannii (Brown and Amyes, 2005; Héritier et al., 2005), contributing to intrinsic resistance to imipenem (Hu et al., 2007). In addition, blaOXA family appears to be evolving quite quickly in recent years as supported by the presence of 282 blaOXA variants across all Acinetobacter spp. globally, along with more yet-unknown variants, since most of the recently submitted genomes had not been released when the related genomes were downloaded for analysis.
We found quite a wide distribution of ST2, most of them co-carrying blaOXA-23 and blaOXA-66, indicating an international clonal dissemination of the strains, mainly among Homo sapiens in health care centers in 38 countries and six continents across the world, posing a serious threat to global public health. As the clone secondary to ST2, although ST1 clone only accounted for little part of the strains, we not only found an increasing trend of ST1 clone every year, but also a wide distribution across 30 countries, mainly Brazil, United States, and Australia. Interestingly, ST1 was not found in isolates from China. The other multiple STs identified in our study indicated the diversity of blaOXA-carrying A. baumannii strains.
According to the current WGS identification and typing methods for Acinetobacter spp., at least 70 members of this genus were identified. It would be worth mentioning that the naming of at least 27 existing genomes was wrong. For example, “A. colistiniresistens” was mistaken as “A. baumannii,” indicating that most Acinetobacter species were misidentified previously, and the underlying reason could be that NCBI started ANI verification-taxonomy nomenclature around 2016, and there was no ANI verification for previously submitted genomes. Moreover, the genomes of typing strains submitted have been increasing, which will also affect their taxonomy verification. If there is no obvious error, the submitted genome with spp. may pass automatically.
There were some limitations in our current study. First, some strains may have been excluded from the analysis, since only the A. baumannii strains, whose genomes were submitted to GenBank and released, were analyzed, even though the analysis was based on global data. Second, the resistant profiles of these strains were missing; we could not check the accordance between the phenotype and genotypes of these strains. Third, all the results were acquired based on WGS, and the strains were not available for further confirmation by molecular methods.
In summary, class D β-lactamase blaOXA variants in Acinetobacter spp. have been rapidly evolving, with CHβLs being the most predominate class D β-lactamase, widely distributed within Acinetobacter spp. blaOXA-23- and blaOXA-66-co-carrying A. baumannii ST2 is a predominant international high-risk clone spreading globally that poses potential threat to global public health.
Data availability statement
The datasets presented in this study can be found in online repositories. The names of the repository/repositories and accession number(s) can be found in the article/Supplementary material.
Author contributions
JL, YL, and XC performed the bioinformatics analysis and writing. JZ and YZ sorted the data and helped with the writing. HX interpreted the data regarding resistance determinants and plasmid replicons. CLi performed statistical analysis. CLiu and HS designed the work and were major contributors in revising the manuscript. All authors contributed to the article and approved the submitted version.
Funding
This work was supported by the National Natural Science Foundation of China (grant numbers 81902124 and 82002205).
Acknowledgments
We are very grateful to Xiangyang Li for providing technical help with data sorting and bioinformatics analysis.
Conflict of interest
The authors declare that the research was conducted in the absence of any commercial or financial relationships that could be construed as a potential conflict of interest.
Publisher’s note
All claims expressed in this article are solely those of the authors and do not necessarily represent those of their affiliated organizations, or those of the publisher, the editors and the reviewers. Any product that may be evaluated in this article, or claim that may be made by its manufacturer, is not guaranteed or endorsed by the publisher.
Author disclaimer
The views expressed in this article are those of the authors and do not necessarily reflect the official policy or position of the Department of Laboratory Medicine, Nanjing Drum Tower Hospital, the Affiliated Hospital of Nanjing University Medical School, and the Clinical Research Center, the Second Hospital of Nanjing, Nanjing University of Chinese Medicine, Nanjing, China.
Supplementary material
The Supplementary material for this article can be found online at: https://www.frontiersin.org/articles/10.3389/fmicb.2023.1174200/full#supplementary-material
Abbreviations
CHβL, Carbapenem-hydrolyzing β-lactamase; MALDI-TOF MS, Matrix-associated laser desorption ionization–time of flight mass spectrometry; WGS, Whole genome sequencing; ICU, Intensive care unit; ANI, Average nucleotide identification; ST, Sequence type; SNP, Single nucleotide polymorphism; MLST, Multi-locus sequence typing.
Footnotes
1. ^https://www.ncbi.nlm.nih.gov/genome/browse/
References
Alexandros,, and Stamatakis, (2014). RAxML version 8: a tool for phylogenetic analysis and post-analysis of large phylogenies. Bioinformatics 30, 1312–1313. doi: 10.1093/bioinformatics/btu033
Al-Hassan, L., Elbadawi, H., Osman, E., Ali, S., Elhag, K., Cantillon, D., et al. (2021). Molecular epidemiology of Carbapenem-resistant Acinetobacter baumannii from Khartoum state, Sudan. Front. Microbiol. 12:628736. doi: 10.3389/fmicb.2021.628736
Antunes, L. C., Visca, P., and Towner, K. J. (2014). Acinetobacter baumannii: evolution of a global pathogen. Pathog Dis 71, 292–301. doi: 10.1111/2049-632x.12125
Bergogne-Bérézin, E., and Towner, K. J. (1996). Acinetobacter spp. as nosocomial pathogens: microbiological, clinical, and epidemiological features. Clin. Microbiol. Rev. 9, 148–165. doi: 10.1128/cmr.9.2.148
Boral, B., Unaldi, O., Ergin, A., Durmaz, R., and Eser, O. K., Acinetobacter Study, G (2019). A prospective multicenter study on the evaluation of antimicrobial resistance and molecular epidemiology of multidrug-resistant Acinetobacter baumannii infections in intensive care units with clinical and environmental features. Ann. Clin. Microbiol. Antimicrob. 18:19. doi: 10.1186/s12941-019-0319-8
Boyd, S. E., Holmes, A., Peck, R., Livermore, D. M., and Hope, W. (2022). OXA-48-like β-lactamases: global epidemiology, treatment options, and development pipeline. Antimicrob. Agents Chemother. 66:e0021622. doi: 10.1128/aac.00216-22
Brown, S., and Amyes, S. G. (2005). The sequences of seven class D beta-lactamases isolated from carbapenem-resistant Acinetobacter baumannii from four continents. Clin. Microbiol. Infect. 11, 326–329. doi: 10.1111/j.1469-0691.2005.01096.x
Colquhoun, J. M., Farokhyfar, M., Hutcheson, A. R., Anderson, A., Bethel, C. R., Bonomo, R. A., et al. (2021). OXA-23 β-lactamase overexpression in Acinetobacter baumannii drives physiological changes resulting in new genetic vulnerabilities. MBio 12:e0313721. doi: 10.1128/mBio.03137-21
Douraghi, M., Kenyon, J. J., Aris, P., Asadian, M., Ghourchian, S., and Hamidian, M. (2020). Accumulation of antibiotic resistance genes in Carbapenem-resistant Acinetobacter baumannii isolates belonging to lineage 2, global clone 1, from outbreaks in 2012-2013 at a Tehran burns hospital. mSphere. 5:e00164–20. doi: 10.1128/mSphere.00164-20
D'Souza, R., Pinto, N. A., Phuong, N. L., Higgins, P. G., Vu, T. N., Byun, J. H., et al. (2019). Phenotypic and genotypic characterization of Acinetobacter spp. panel strains: a cornerstone to facilitate antimicrobial development. Front. Microbiol. 10:559. doi: 10.3389/fmicb.2019.00559
Edgar, R. C. (2004). MUSCLE: multiple sequence alignment with high accuracy and high throughput. Nucleic Acids Res. 32, 1792–1797. doi: 10.1093/nar/gkh340
Evans, B. A., and Amyes, S. G. (2014). OXA β-lactamases. Clin. Microbiol. Rev. 27, 241–263. doi: 10.1128/cmr.00117-13
Gurevich, A., Saveliev, V., Vyahhi, N., and Tesler, G. (2013). QUAST: quality assessment tool for genome assemblies. Bioinformatics 29, 1072–1075. doi: 10.1093/bioinformatics/btt086
Harding, C. M., Hennon, S. W., and Feldman, M. F. (2018). Uncovering the mechanisms of Acinetobacter baumannii virulence. Nat. Rev. Microbiol. 16, 91–102. doi: 10.1038/nrmicro.2017.148
Héritier, C., Poirel, L., Fournier, P. E., Claverie, J. M., Raoult, D., and Nordmann, P. (2005). Characterization of the naturally occurring oxacillinase of Acinetobacter baumannii. Antimicrob. Agents Chemother. 49, 4174–4179. doi: 10.1128/aac.49.10.4174-4179.2005
Hu, W. S., Yao, S. M., Fung, C. P., Hsieh, Y. P., Liu, C. P., and Lin, J. F. (2007). An OXA-66/OXA-51-like carbapenemase and possibly an efflux pump are associated with resistance to imipenem in Acinetobacter baumannii. Antimicrob. Agents Chemother. 51, 3844–3852. doi: 10.1128/aac.01512-06
Jain, C., Rodriguez, R. L., Phillippy, A. M., Konstantinidis, K. T., and Aluru, S. (2018). High throughput ANI analysis of 90K prokaryotic genomes reveals clear species boundaries. Nat. Commun. 9:5114. doi: 10.1038/s41467-018-07641-9
Kengkla, K., Kongpakwattana, K., Saokaew, S., Apisarnthanarak, A., and Chaiyakunapruk, N. (2018). Comparative efficacy and safety of treatment options for MDR and XDR Acinetobacter baumannii infections: a systematic review and network meta-analysis. J. Antimicrob. Chemother. 73, 22–32. doi: 10.1093/jac/dkx368
Khurshid, M., Rasool, M. H., Ashfaq, U. A., Aslam, B., Waseem, M., Xu, Q., et al. (2020). Dissemination of Bla(OXA-23)-harbouring carbapenem-resistant Acinetobacter baumannii clones in Pakistan. J. Glob. Antimicrob. Resist. 21, 357–362. doi: 10.1016/j.jgar.2020.01.001
Lee, C. R., Lee, J. H., Park, K. S., Kim, Y. B., Jeong, B. C., and Lee, S. H. (2016). Global dissemination of Carbapenemase-producing Klebsiella pneumoniae: epidemiology, genetic context, treatment options, and detection methods. Front. Microbiol. 7:895. doi: 10.3389/fmicb.2016.00895
Lee, C. R., Lee, J. H., Park, M., Park, K. S., Bae, I. K., Kim, Y. B., et al. (2017). Biology of Acinetobacter baumannii: pathogenesis, antibiotic resistance mechanisms, and prospective treatment options. Front. Cell. Infect. Microbiol. 7:55. doi: 10.3389/fcimb.2017.00055
Letunic, I., and Bork, P. (2019). Interactive tree of life (iTOL) v4: recent updates and new developments. Nucleic Acids Res. 47, 256–259. doi: 10.1093/nar/gkz239
Longo, B., Fazio, C., Meini, S., Neri, A., Andreoli, E., Vacca, P., et al. (2023). Primary septic arthritis of the knee caused by Neisseria meningitidis serogroup B in an elderly patient. Case report and review of the literature. Infection 51, 499–506. doi: 10.1007/s15010-022-01928-2
Lupo, A., Haenni, M., and Madec, J.-Y. (2018). Antimicrobial resistance in Acinetobacter spp. and Pseudomonas spp. Microbiol. Spectr. 6:01. doi: 10.1128/microbiolspec.ARBA-0007-2017
Magiorakos, A. P., Srinivasan, A., Carey, R. B., Carmeli, Y., Falagas, M. E., Giske, C. G., et al. (2012). Multidrug-resistant, extensively drug-resistant and pandrug-resistant bacteria: an international expert proposal for interim standard definitions for acquired resistance. Clin. Microbiol. Infect. 18, 268–281. doi: 10.1111/j.1469-0691.2011.03570.x
Palmieri, M., D'Andrea, M. M., Pelegrin, A. C., Perrot, N., Mirande, C., Blanc, B., et al. (2020). Abundance of Colistin-resistant, OXA-23- and ArmA-producing Acinetobacter baumannii belonging to international clone 2 in Greece. Front. Microbiol. 11:668. doi: 10.3389/fmicb.2020.00668
Parks, D. H., Imelfort, M., Skennerton, C. T., Hugenholtz, P., and Tyson, G. W. (2015). CheckM: assessing the quality of microbial genomes recovered from isolates, single cells, and metagenomes. Genome Res. 25, 1043–1055. doi: 10.1101/gr.186072.114
Pitout, J. D. D., Peirano, G., Kock, M. M., Strydom, K. A., and Matsumura, Y. (2019). The global ascendency of OXA-48-type Carbapenemases. Clin. Microbiol. Rev. 33:e00102–19. doi: 10.1128/cmr.00102-19
Qin, J., Feng, Y., Lü, X., and Zong, Z. (2021). Precise species identification for Acinetobacter: a genome-based study with description of two novel Acinetobacter species. mSystems 6, e00237–e00221. doi: 10.1128/mSystems.00237-21
Richter, M., Rosselló-Móra, R., Oliver Glöckner, F., and Peplies, J. (2016). JSpeciesWS: a web server for prokaryotic species circumscription based on pairwise genome comparison. Bioinformatics 32, 929–931. doi: 10.1093/bioinformatics/btv681
Salehi, B., Ghalavand, Z., Mohammadzadeh, M., Maleki, D. T., Kodori, M., and Kadkhoda, H. (2019). Clonal relatedness and resistance characteristics of OXA-24 and -58 producing carbapenem-resistant Acinetobacter baumannii isolates in Tehran, Iran. J. Appl. Microbiol. 127, 1421–1429. doi: 10.1111/jam.14409
Seemann, T. (2014). Prokka: rapid prokaryotic genome annotation. Bioinformatics 30, 2068–2069. doi: 10.1093/bioinformatics/btu153
Smith, C. A., Antunes, N. T., Stewart, N. K., Toth, M., Kumarasiri, M., Chang, M., et al. (2013). Structural basis for carbapenemase activity of the OXA-23 β-lactamase from Acinetobacter baumannii. Chem. Biol. 20, 1107–1115. doi: 10.1016/j.chembiol.2013.07.015
Sun, Y., Liu, Q., Chen, S., Song, Y., Liu, J., Guo, X., et al. (2014). Characterization and plasmid elimination of NDM-1-producing Acinetobacter calcoaceticus from China. PLoS One 9:e106555. doi: 10.1371/journal.pone.0106555
Takebayashi, Y., Findlay, J., Heesom, K. J., Warburton, P. J., Avison, M. B., and Evans, B. A. (2021). Variability in carbapenemase activity of intrinsic OxaAb (OXA-51-like) β-lactamase enzymes in Acinetobacter baumannii. J. Antimicrob. Chemother. 76, 587–595. doi: 10.1093/jac/dkaa502
Tamma, P. D., Aitken, S. L., Bonomo, R. A., Mathers, A. J., van Duin, D., and Clancy, C. J. (2022). Infectious Diseases Society of America guidance on the treatment of AmpC beta-lactamase-producing Enterobacterales, Carbapenem-resistant Acinetobacter baumannii, and Stenotrophomonas maltophilia infections. Clin. Infect. Dis. 74, 2089–2114. doi: 10.1093/cid/ciab1013
Tooke, C. L., Hinchliffe, P., Bragginton, E. C., Colenso, C. K., Hirvonen, V. H. A., Takebayashi, Y., et al. (2019). β-Lactamases and β-lactamase inhibitors in the 21st century. J. Mol. Biol. 431, 3472–3500. doi: 10.1016/j.jmb.2019.04.002
Vali, L., Dashti, K., Opazo-Capurro, A. F., Dashti, A. A., Al Obaid, K., and Evans, B. A. (2015). Diversity of multi-drug resistant Acinetobacter baumannii population in a major hospital in Kuwait. Front. Microbiol. 6:743. doi: 10.3389/fmicb.2015.00743
Walther-Rasmussen, J., and Høiby, N. (2006). OXA-type carbapenemases. J. Antimicrob. Chemother. 57, 373–383. doi: 10.1093/jac/dki482
Wang, X., Du, Z., Huang, W., Zhang, X., and Zhou, Y. (2021). Outbreak of multidrug-resistant Acinetobacter baumannii ST208 producing OXA-23-like Carbapenemase in a Children's Hospital in Shanghai. Microb. Drug Resist. 27, 816–822. doi: 10.1089/mdr.2019.0232
Zajmi, A., Teo, J., and Yeo, C. C. (2022). Epidemiology and characteristics of Elizabethkingia spp. infections in Southeast Asia. Microorganisms 10:882. doi: 10.3390/microorganisms10050882
Zhang, X., Li, F., Awan, F., Jiang, H., Zeng, Z., and Lv, W. (2021). Molecular epidemiology and clone transmission of Carbapenem-resistant Acinetobacter baumannii in ICU rooms. Front. Cell. Infect. Microbiol. 11:633817. doi: 10.3389/fcimb.2021.633817
Keywords: Acinetobacter spp., Acinetobacter baumannii , OXA-23, OXA-66, ST2
Citation: Li J, Li Y, Cao X, Zheng J, Zhang Y, Xie H, Li C, Liu C and Shen H (2023) Genome-wide identification and oxacillinase OXA distribution characteristics of Acinetobacter spp. based on a global database. Front. Microbiol. 14:1174200. doi: 10.3389/fmicb.2023.1174200
Edited by:
Qixia Luo, Zhejiang University, ChinaReviewed by:
Ulises Garza-Ramos, National Institute of Public Health (Mexico), MexicoPawan Kumar Kanaujia, Mahayogi Gorakhnath University, Gorakhpur (Uttar Pradesh), India
Copyright © 2023 Li, Li, Cao, Zheng, Zhang, Xie, Li, Liu and Shen. This is an open-access article distributed under the terms of the Creative Commons Attribution License (CC BY). The use, distribution or reproduction in other forums is permitted, provided the original author(s) and the copyright owner(s) are credited and that the original publication in this journal is cited, in accordance with accepted academic practice. No use, distribution or reproduction is permitted which does not comply with these terms.
*Correspondence: Chang Liu, bGl1Y2hhbmdiaW9AMTYzLmNvbQ==; Han Shen, c2hlbmhhbjEwMzY2QHNpbmEuY29t
†These authors have contributed equally to this work