- 1Department of Plant Pathology, Faculty of Agriculture and Environment, The Islamia University of Bahawalpur, Bahawalpur, Punjab, Pakistan
- 2Department of Horticultural Sciences, Faculty of Agriculture and Environment, The Islamia University of Bahawalpur, Bahawalpur, Punjab, Pakistan
- 3The UWA Institute of Agriculture, The University of Western Australia, Perth, WA, Australia
Lemons (Citrus limon L.) are one of the most economically important and consumed fruit worldwide. The species is vulnerable to several postharvest decay pathogens, of which Penicillium italicum associated with blue mold disease is the most damaging. This study investigates the use of integrated management for blue mold of lemon using lipopeptides (LPs) extracted from endophytic Bacillus strains and resistance inducers. Two resistance inducers; salicylic acid (SA) and benzoic acid (BA) were tested at 2, 3, 4, and 5 mM concentrations against the development of blue mold on lemon fruit. The 5 mM SA treatment produced the lowest disease incidence (60%) and lesion diameter (1.4 cm) of blue mold on lemon fruit relative to the control. In an in vitro antagonism assay eighteen Bacillus strains were evaluated for their direct antifungal effect against P. italicum; CHGP13 and CHGP17 had the greatest inhibition zones of 2.30 and 2.14 cm. Lipopeptides (LPs) extracted from CHGP13 and CHGP17 also inhibited the colony growth of P. italicum. LPs extracted from CHGP13 and 5 mM SA were tested as single and combined treatments against disease incidence and lesion diameter of blue mold on lemon fruit. SA + CHGP13 + PI had the lowest disease incidence (30%) and lesion diameter (0.4 cm) of P. italicum on lemon fruit relative to the other treatments. Furthermore, the lemon fruit treated with SA + CHGP13 + PI had the highest PPO, POD, and PAL activities. The postharvest quality analysis of the lemon fruit including fruit firmness, total soluble solids, weight loss, titratable acidity, and ascorbic acid content revealed that the treatment SA + CHGP13 + PI had little effect on fruit quality compared to the healthy control. These findings indicate that Bacillus strains and resistance inducers can be used as components of integrated disease management for the blue mold of lemon.
Introduction
Citrus is an economically and nutritionally important fruit crop worldwide, especially in tropical and subtropical areas (Liu et al., 2012). Lemon (Citrus limon L.) is the third most significant species of the genus Citrus after orange and mandarin. Citrus fruits are susceptible to various biotic and abiotic challenges during the postharvest stage (handling, shipping, storing, and marketing; Tian et al., 2020). Citrus production faces significant economic losses before and after harvest, particularly due to postharvest deterioration. The harvested citrus fruits are stored and handled in packing houses to maintain their postharvest life and quality and to reduce decay due to pathogen infection. Postharvest fungal pathogens account for 30–50% of citrus crop losses (Porat et al., 2000).
Injuries inflicted during postharvest handling are the major cause of infection by Penicillium digitatum Saccardo (associated with the green mold of citrus fruit) and P. italicum Wehmer (associated with blue mold of citrus), allowing these pathogens to enter the fruit (Moosa et al., 2021, 2022). These pathogens are omnipresent and cause significant losses wherever citrus is cultivated (Palou et al., 2001). During postharvest storage, 4°C is the most conducive temperature for developing P. italicum infection (Wyatt et al., 1995), a nesting-type pathogen that produces enzymes to macerate fruit tissues, enabling pathogen colonization. The infection intensity of blue mold of citrus increases with fruit ripeness. A high density of inoculum deposited in wounds and 20–25°C temperature increase the infection intensity of blue mold of citrus (Papoutsis et al., 2019).
Blue mold is controlled primarily by applying synthetic fungicides such as thiabendazole and imazalil (Cunha et al., 2018). While synthetic chemical fungicides can control several diseases effectively, their use is restricted for controlling postharvest deterioration due to their high and acute residual toxicity, long degradation period, environmental pollution, and effects on food quality and human health (Tripathi and Dubey, 2004). Moreover, the widespread use of chemical fungicides proliferates fungicide-resistant strains, compromising the effectiveness of these chemicals (Palou et al., 2008). Thus, the exploration of new and safer alternatives to reduce the use of synthetic fungicides is an emerging field of research.
Biological control of plant diseases is a safer alternative to chemicals with no harmful effects on human health or the environment, offering sustainable, affordable, and eco-friendly management for the control of plant pathogens (He et al., 2021). A biological control agent should be genetically stable, effective at low concentrations, able to survive under adverse environmental conditions, inexpensive to formulate and produce, shelf-stable, resistant to common pesticides, compatible with commercial processing practices, and non-pathogenic to human health and the host (Wisniewski and Wilson, 1992).
Several biocontrol agents have been studied and used successfully to prevent postharvest diseases in fruit, including yeasts and bacteria (Janisiewicz and Korsten, 2002; Tian et al., 2002; Sharma et al., 2009). Bacillus species are widespread endophytic bacteria with impressive biocontrol potential against several plant diseases (Mahaffee and Kloepper, 1997; Algam et al., 2005), mainly by inhibiting the growth of plant pathogens and promoting systemic resistance in plants (Fira et al., 2018). The antagonistic mechanisms of action of endophytic Bacillus species include competition for nutrition and space, production of antimicrobial compounds, direct parasitism, and induced systemic resistance in plants (Farzand et al., 2019a,b), which serve as the foundation for synthesizing commercial bio-formulations to manage plant diseases (Cirvilleri, 2008; Nunes, 2012).
The production of antimicrobial compounds is considered the most significant mechanism for the biological control of plant pathogens (Finking and Marahiel, 2004; Ongena and Jacques, 2008; Zhao et al., 2013). Antifungal lipopeptides (LPs), produced by Bacillus species, are the most effective antimicrobial compounds that directly suppress plant pathogens (Farzand et al., 2019a, 2020). Three important LP families (fengycin, iturin, and surfactin) along with some others (bacilysin, bacillibactin, bacillaene, and bacillomycin) largely contribute to the antifungal activity of Bacillus species (Farzand et al., 2019a,b). Bacillus species have been researched extensively and reported to control citrus fruit diseases. Bacillus subtilis and its metabolites are used to control citrus green and blue molds and are categorized as GRAS (Generally Recognized As Safe) biocontrol agents (Waewthongrak et al., 2015).
The induction of resistance in host plants by natural and synthetic resistance inducers is a widely researched area. Applying resistance inducers can boost the natural defense system of fruit to suppress postharvest pathogen attacks. Non-toxic synthetic resistance inducers such as salicylic acid (SA), benzoic acid (BA), jasmonic acid (JA), and methyl jasmonate (MJ), are also considered a safer alternative to synthetic chemicals (Qin et al., 2003). They are an attractive management strategy employing plants’ natural defense mechanisms to suppress the infection of several threatening plant pathogens (Terry and Joyce, 2004; Walters et al., 2005). SA is an important resistance inducer that increases postharvest disease resistance against multiple diseases of fruit crops, including pear, mango, and citrus (Shaat and Galal, 2004). SA and JA are essential for controlling plant growth and development and improving plant disease resistance (Yao and Tian, 2005). The induction of resistance is accompanied by an oxidative burst and the creation of pathogenesis-related (PR) proteins (Moosa et al., 2018) and other antimicrobial substances, such as phytoalexins (Asghari and Aghdam, 2010). The application of resistance inducers modulates the activity of chitinase, peroxidase, and polyphenol oxidase, which play an important role in plant defense (Sardar et al., 2023). Several studies have reported that exogenous SA application significantly improved the resistance to various postharvest fungal diseases (Asghari and Aghdam, 2010; Iqbal et al., 2012; Zhou et al., 2018; Moosa et al., 2019). However, single applications of resistance inducers have significantly lowered the effectiveness compared to synthetic pesticides and do not offer commercially adequate postharvest decay management (Iqbal et al., 2012; Moosa et al., 2019).
Combining resistance inducers with other therapies can offer comparable disease control to synthetic chemicals. An integrated management strategy is more effective and reliable for the control of several diseases (Sukorini et al., 2013; Moosa et al., 2021, 2022). Combined physical, chemical, and biological controls have been used to manage postharvest diseases of citrus as components of integrated disease control programs (Naqvi, 2004). For example, the combination of yeast and medicinal plant extracts suppressed the infection of P. digitatum on tangerine fruit (Sukorini et al., 2013). Guo et al. (2014) reported that the combined application of Cryptococcus laurentii and MJ inhibited the development of P. digitatum on mandarins. Hao et al. (2011) reported that B. amyloliquefaciens combined with tea saponin (50 μg ml−1) suppressed blue mold, green mold, and sour rot of citrus. While Bacillus species and resistance inducers can significantly suppress blue mold of lemon, they cannot replace the use of pesticides as Singletreatments. Therefore, different management strategies must be combined to enhance the suppression of postharvest diseases during storage.
The biochemical characterization of defense enzymes in diseased plants is critical for understanding the basic mechanisms involved in plant defense (Moosa et al., 2019). The regulation of defense enzymes acts as universal defense against biotic and abiotic stresses (Hasanuzzaman et al., 2020; Zulfiqar and Ashraf, 2021) Necrotrophic pathogens can alter the activity of oxidative enzymes like peroxidase (POD) and polyphenol oxidase (PPO; Bowles, 1990). The pathogenesis-related (PR) class of proteins plays a key role in plant defense against pathogen attack and other physiological stresses like low temperature, salt, drought, and heavy metal toxicity (Ballester et al., 2013). Phenylalanine ammonia-lyase (PAL), POD, and PPO are three important PR proteins that play a role in the biosynthesis and oxidation of phenolic chemicals and disease resistance in plants (Livak and Schmittgen, 2001; Bill et al., 2017). Plant disease resistance is linked to POD activity (Hammerschmidt et al., 1981) which increases due to pathogen infection in host plants (Scott-Craig et al., 1995). POD accelerates the last stages of lignin and hydrogen peroxide synthesis (Ma et al., 2012) and plays a significant role in plant growth and development. Campa (1991) reported that POD is linked to chlorophyll degradation and lipid peroxidation in dormant plant tissues. Chlorophyll degradation is accelerated by the presence of phenol molecules (Kato and Shimizu, 1985).
The study was undertaken with the hypothesis that the combined application of resistance inducers and Bacillus LPs can provide more effective control of blue mold of lemon than a single application. This study aimed to (a) determine the antifungal efficacy of Bacillus LPs and SA (Single and combined) against blue mold of lemon, and (b) assess the activity of defense enzymes and postharvest quality of treated and untreated lemon fruit.
Materials and methods
Bacterial and fungal cultures
Bacillus strains were obtained from the bacterial culture collection in the Molecular Plant Pathology Laboratory at The Islamia University, Bahawalpur, Pakistan (Table 1; Fatima et al., 2023), which were stored in a 60% glycerol stock solution at −80°C. The bacterial cultures were grown on fresh Luria Bertani (LB) medium, and incubated at 37°C. The fungus P. italicum was isolated on potato dextrose agar medium (PDA) from decaying lemon fruit showing symptoms of blue mold and incubated at 25 ± 2°C. Purified cultures were obtained by using the single spore culturing technique, preserved in 30% glycerol stock solution at −80°C, and later retrieved on the PDA medium for further experimentation.
Fruit
Mature, disease-free, and uniform-sized lemon fruit cv. ‘Meyer Lemon’ were collected from the Citrus orchard at The Islamia University of Bahawalpur, Pakistan. The samples were disinfected with 1% NaOCl for 2 min and, rinsed with sterilized distilled water. Then the samples were air-dried on sterilized paper towels to remove excess moisture from the fruit surface.
Assay of resistance inducers
Resistance inducers SA and BA were used to test their effect on P. italicum infection on lemon fruit. Aqueous solutions of SA and BA were prepared at different concentrations (2, 3, 4, and 5 mM) based on their molar masses. Sterilized fruit were dipped in aqueous solutions of SA and BA for 10 min and then dried on paper towels. A sterilized needle was used to injure the fruit, making a 5 mm artificial wound, before inoculation with 10 μL (1 × 106 spores ml−1) of P. italicum. Then, a 10 μl aqueous solution of SA and BA was deposited into these wounds using a micropipette. The fruit was placed in sterilized square plastic boxes and incubated for 7 days at 25 ± 2°C. The experiment was conducted in a completely randomized design (CRD) with ten replications for each treatment, repeated twice under the same conditions. The lesion diameter of blue mold of lemon was measured, and disease incidence was determined using the following formula (Sukorini et al., 2013):
Antagonism assay
The antifungal activity of 18 Bacillus species was evaluated in a dual culture assay against P. italicum. A 5 mm culture block from 7-day-old pathogen culture was placed at the center of a Petri plate containing PDA and incubated at 25 ± 1°C for 2 days. Sterilized filter paper disks were placed 3 cm from both sides of the plate. Then, 10 μl bacterial culture, grown overnight in LB broth (OD600 = 2.5), was inoculated on both filter paper disks. The plates were incubated for 7 days at 25 ± 1°C. The experiment was performed three times under the same conditions with five replications per treatment.
Extraction of LPs from Bacillus strains
Based on the antagonism assay, the best strains (CHGP13 and CHGP17) were used to extract LPs. Luria Bertani (LB) broth (300 ml) was prepared, inoculated with CHGP13, and placed on a rotary shaker at 180 rpm at 30°C for 3 days. Then the bacterial cultures were centrifuged at 10,000 rpm at 4°C for 15 min to obtain the cell-free supernatant. The supernatant was collected in a sterilized glass beaker and incubated at 4°C for 12 h. After incubation cell-free supernatant was centrifuged at 10,000 rpm for 15 min at 4°C. The supernatant was discarded, and the pellet was collected and dried. The pellet was dissolved in 5 ml HPLC-grade methanol (pH 7) and passed through a 0.22 μm syringe filter to remove impurities.
Antifungal assay with LPs
To determine the effect of LPs on lemon fruit, uniform-sized, disease-free fruit were selected and surface sterilized. A sterilized needle was used to injure the fruit, making a 5 mm artificial wound, before depositing 10 μl LPs solution in each wound. Then each wound was inoculated with 10 μl spore suspension (1 × 106 spores ml−1) of the pathogen. The fruit was placed in sterilized square plastic boxes and incubated at room temperature for 7 days. The experiment was conducted in a CRD, with each treatment replicated ten times, and repeated twice under the same conditions. Disease incidence and severity were determined as per the resistance inducers assay.
Combined assay with resistance inducer and LPs to control blue mold of lemon
To determine the combined effect of the best resistance inducer and LPs, 5 mM SA and the LPs extracted from CHGP13 were used. Mature, disease-free lemon fruit were surface sterilized and dipped in a 5 mm aqueous solution of SA for 10 min. Next, a sterilized needle was used to injure the fruit, making a 5 mm artificial wound, before inoculating with 10 μl of 5 mM SA solution followed by 10 μl of LP solution using a micropipette. Then each wound received 10 μl spore suspension of P. italicum. The fruit was incubated in sterilized square plastic boxes for 7 days at 25 ± 1°C. The experiment was conducted in a CRD with ten replications per treatment, repeated twice under the same conditions. Disease incidence and severity were calculated as per the resistance inducers assay.
Enzyme assays
PAL assay
PAL activity was determined using the method of Martinez-Tellez and Lafuente (1997). The enzyme was extracted from 0.4 g flavedo acetone powder (Sala et al., 2005) in 15 ml of 0.1 M sodium borate buffer (pH 8.8) containing 0.02 M mercaptoethanol at 4°C. The reaction mixture contained 0.1 ml enzyme extract, 1.9 ml of 0.05 M sodium borate buffer (pH 8.8), and 1 ml of 20 mM l-phenylalanine. The absorbance of cinnamic acid was determined at 290 nm after incubation at 40°C for 2 h. PAL activity was calculated on a dry weight basis in nmol cinnamic acid per h per g flavedo acetone powder (nmol h−1 g−1 fresh weight).
POD assay
POD activity was measured using the method of Martinez-Tellez and Lafuente (1997). The enzyme extract was prepared by crushing 0.2 g dry weight of flavedo acetone powder in 6 ml of 100 mM Tris–HCL buffer (pH 8) containing 5 mM mercaptoethanol and centrifuged at 11,000 rpm at 4°C for 30 min. The reaction solution contained 2.15 ml of 10 mM sodium acetate (pH 5.3), comprising guaiacol, 0.25 ml of 0.1% H2O2, and 0.1 ml supernatant. The mixture was incubated at 30°C for 2 min before measuring the absorbance at 470 nm on a spectrophotometer. One unit of POD activity was expressed as a 470 nm absorbance rise per minute. POD activity was calculated in units of POD min−1 g−1 flavedo acetone powder.
PPO assay
PPO activity was assessed using the method of Martinez-Tellez and Lafuente (1997), with some modifications. The enzyme extract comprised 0.2 g flavedo acetone powder (w/w) mixed in 6 mL of 0.05 M potassium phosphate buffer (pH 7.2) containing 1 M KCL and 5% (w/w) polyvinyl–polypyrrolidone. The solution was centrifuged at 15,000 rpm at 4°C for 10 min. The reaction mixture contained 25 μl enzyme extract and 1.25 ml of 0.02 mM caffeic acid. The rate of increase in absorbance at 410 nm and 30°C was measured to determine PPO activity. At 410 nm, an increase of 0.01 absorbance unit per minute was used to define one unit of PPO activity. Thus, PPO activity was calculated in units of PPO activity per minute per gram of fresh weight.
Postharvest quality analysis of lemon fruit
To determine the effect of SA and LPs on lemon fruit quality, fruit firmness (N), total weight loss (%), titratable acidity (%), total soluble solids (%), and ascorbic acid content (mg 100 g−1) were measured. Firmness was measured using a fruit texture analyzer. The reading on the analyzer was set at zero before placing the fruit on the fruit texture analyzer and held tightly. The flat-end probe (8 mm diameter) was pushed into the fruit at 5 mm s−1 to 3 mm, with the penetration (firmness) expressed in Newton. The flat-end probe was cleaned between measurements.
Total soluble solids (TSS) in juice from healthy, disease-infected, and treated fruit was measured using a digital refractometer (Lacey et al., 2009). The fruit was cut in half and squeezed to collect the juice. A few drops of juice were placed on the prism plate of the refractometer, and the reading was recorded. The prism plate was wiped with a clean tissue between measurements.
Each fruit was weighed before treatment and 7 days post-treatment to determine weight loss. Weight loss was calculated as follows:
where A is fruit weight before treatment and B is fruit weight after treatment.
Titratable acidity was measured by titrating fruit juice with 0.1 M NaOH (Hernández et al., 2006). The NaOH solution was slowly added to the fruit juice using a 25 ml clean burette. The titratability of the fruit juice was recorded with a pH meter by placing the electrode in the fruit juice and recording the point when the fruit pH reached neutrality and the amount of NaOH in the burette. The pH meter electrode was washed with distilled water between measurements.
The ascorbic acid (Vitamin C) content in lemon fruit was determined by titrating the fruit juice with 2,6-dichloro-indophenol. The results were calculated in milligrams of ascorbic acid per 100 g fresh weight (Jones and Hughes, 1983).
Statistical analysis
The experiments were conducted in a completely randomized design. The data was subjected to Tukey’s HSD test after ANOVA at p = 0.05 by using the statistical package Statistix version (Ver. 8.1).
Results
Effect of resistance inducers on lesion diameter and disease incidence of blue mold of lemon
The preventive treatment with resistance inducers decreased the lesion diameter and incidence (Table 2). The 5 mM SA decreased the disease incidence of blue mold to 60% and the lesion diameter to 1.4 cm, compared to the infected control treatment. Treatment with 2 mM SA showed 93.3% disease incidence and a 2.6 cm lesion diameter of blue mold. The treatments were significantly different from each other at p = 0.05. The 5 mM BA treatment decreased disease incidence to 66.7% and lesion diameter to 1.9 cm relative to the infected control treatment. Healthy control fruit remained asymptomatic.
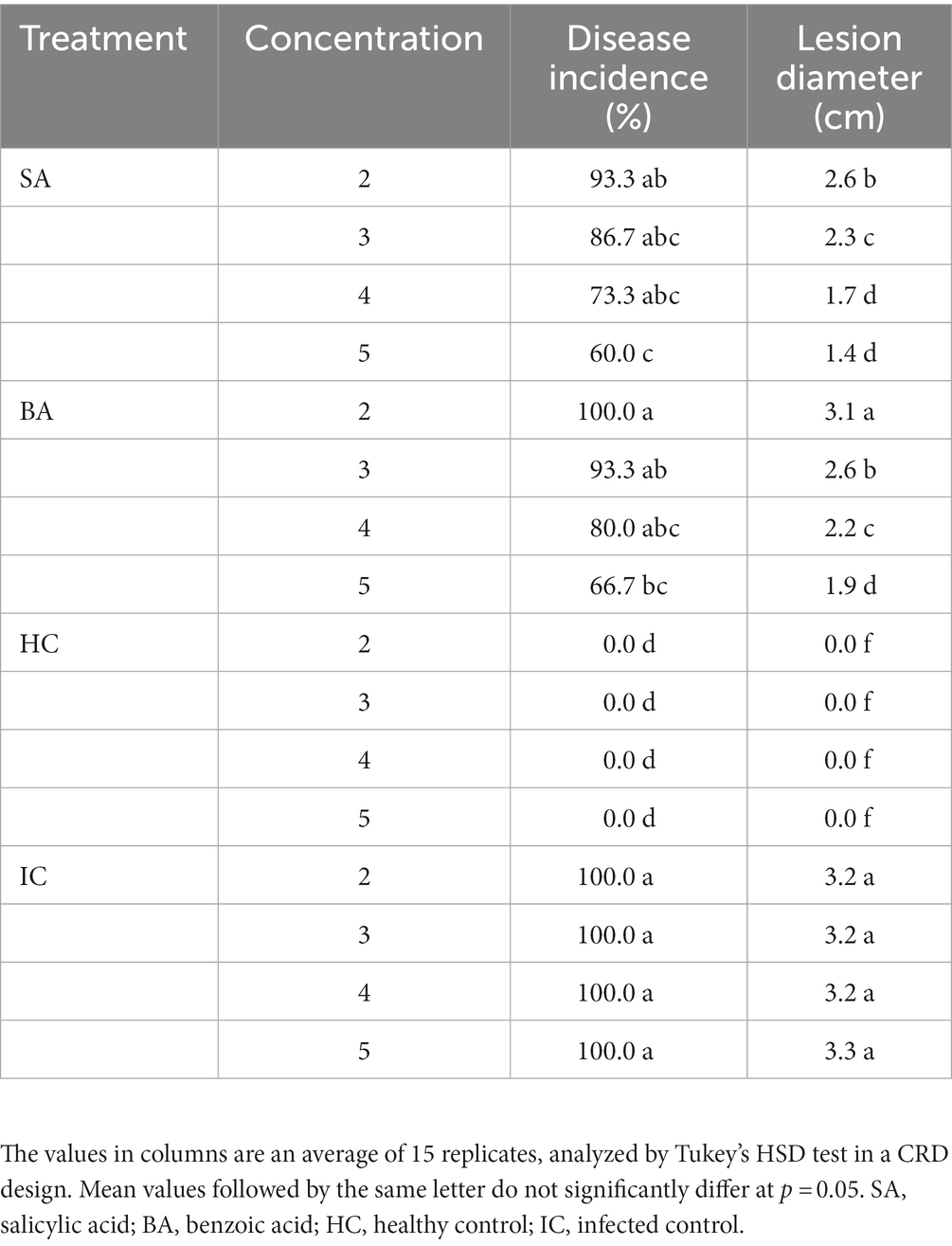
Table 2. Effect of resistance inducers on disease incidence and lesion diameter of blue mold of Lemon.
In vitro antagonism assay
The antifungal effect of 18 Bacillus strains against the colony growth of P. italicum was tested in a filter paper disk assay. All Bacillus strains except CHGP4, CHGP8, CHGP9, and CHGP10 inhibited the colony growth of P. italicum. CHGP13 had the greatest inhibition, followed by CHGP17, with inhibition zones of 2.30 and 2.14 cm, respectively (Figure 1). CHGP2, CHGP5, and CHGP14 had the least inhibition, with inhibition zones 0.46, 0.46, and 0.52 cm, respectively.
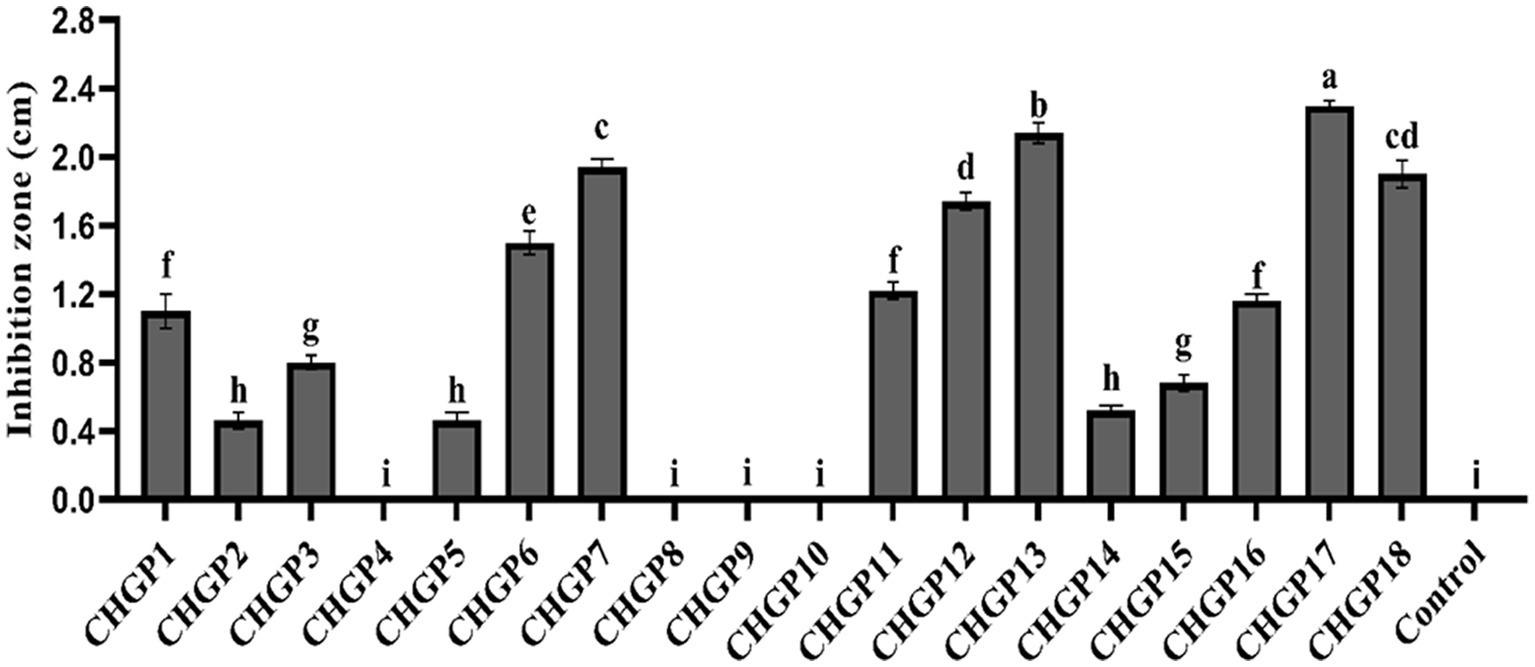
Figure 1. In vitro inhibition of Penicillium italicum by Bacillus strains. Each bar is an average of 15 replicates from three experiments, analyzed by Tukey’s HSD test in a CRD design. Bars with the same letter do not significantly differ at p = 0.05. Error bars are the standard error of the mean.
Antifungal effect of LPs against Penicillium italicum
LPs were extracted from CHGP13 and CHGP17 and tested against the colony growth of P. italicum in an in vitro assay. CHGP13 and CHGP17 significantly inhibited P. italicum growth compared to the control treatment, with inhibition zones of 2.14 and 1.98 cm, respectively, compared with no inhibition zone in the control treatment (Figure 2).
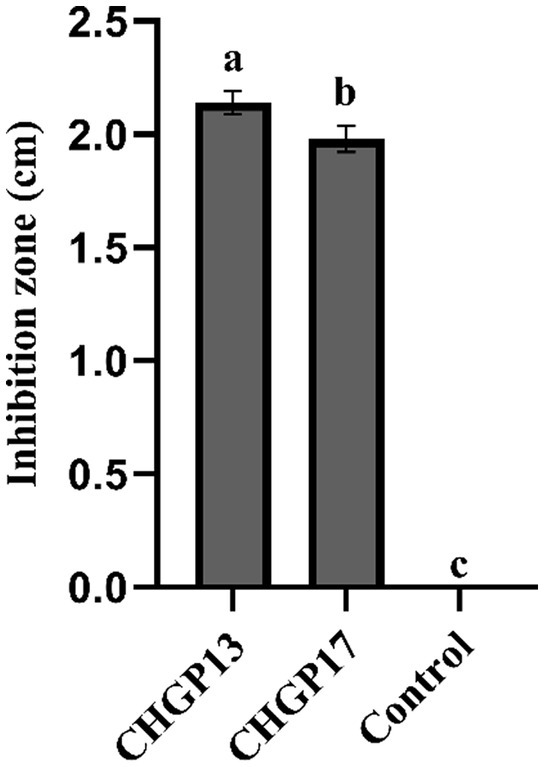
Figure 2. Effect of lipopeptides on in vitro colony growth inhibition of P. italicum. Each bar is an average of 15 replicates from three experiments, analyzed by Tukey’s HSD test in a CRD design. Bars with different letters significantly differ at p = 0.05. Error bars are the standard error of the mean.
Combined assay with resistance inducer and LPs against Penicillium italicum
The effect of 5 mM SA and LPs extracted from CHGP13 as single and combined applications was evaluated on the growth inhibition of P. italicum. The combined treatment (SA + CHGP13) had the lowest disease incidence and lesion diameter of P. italicum compared to the infected control at p = 0.05 (Figure 3). Single CHGP13 and SA also decreased disease incidence and lesion diameter but to a lesser degree. The infected control had the highest disease incidence and lesion diameter.
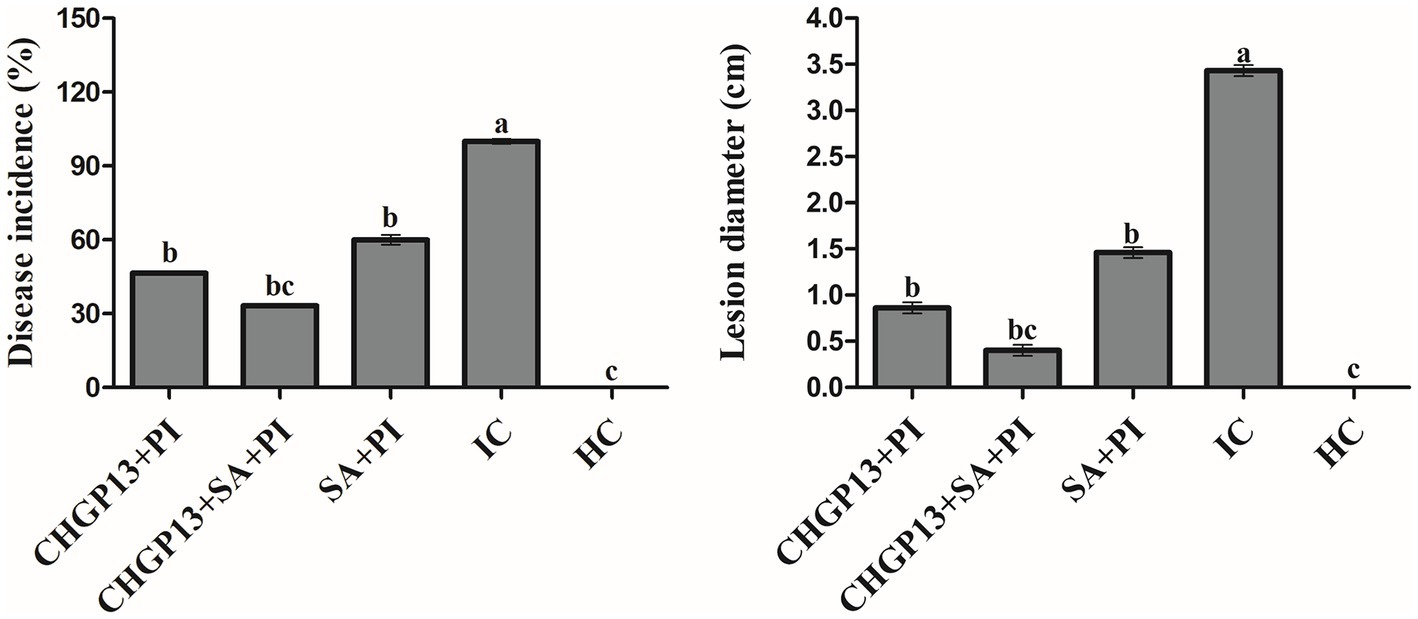
Figure 3. Effect of Bacillus atrophaeus CHGP13 and salicylic acid on disease incidence and lesion diameter of blue mold of lemon in a combined assay. Each bar is an average of 30 replicates from three experiments, analyzed by Tukey’s HSD test in a CRD design. Bars with the same letter do not significantly differ at p = 0.05. Error bars are the standard error of the mean.
Assessment of PAL, PPO, and POD activities
The activities of PAL, PPO, and POD in lemon fruit treated with combined and single applications of SA and LPs extracted from CHGP13 were tested. The activity of all tested enzymes increased in fruit treated with Singleor combined application of SA and LPs extracted from CHGP13 relative to control treatments. The combined application of SA and LPs extracted from CHGP13 had the highest PPO, POD, and PAL activities compared to the infected and healthy control treatments at p = 0.05 (Figure 4). SA and CHGP13 as single treatments also increased PPO, PAL, and POD activities but to a lesser degree. Fruit infected with P. italicum only had the lowest POD, PPO, and PAL activities at p = 0.05 analyzed by Tukey’s HSD test (Figure 4).
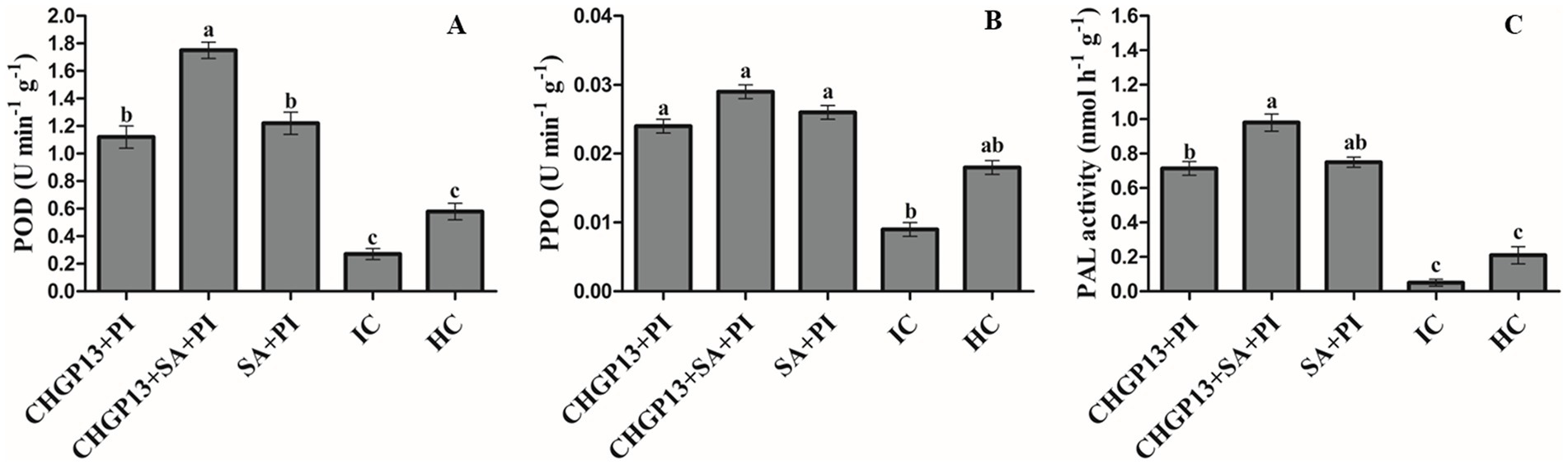
Figure 4. Defense enzyme activities in lemon fruit treated with SA and B. atrophaeus CHGP13 as single and combined treatments to suppress blue mold of lemon. (A) POD (peroxidase), (B) PPO (polyphenol oxidase), and (C) PAL (phenylalanine ammonia lyase). Each bar is an average of 15 replicates from three experiments, analyzed by Tukey’s HSD test in a CRD design. Bars with the same letter do not significantly differ at p = 0.05. Error bars are the standard error of the mean. CHGP13, B. atrophaeus (CHGP13); SA, salicylic acid; PI, P. italicum; IC, infected control; HC, healthy control.
Effect on postharvest quality of lemon fruit
The effect of SA and CHGP13 as single and combined treatments on the postharvest quality of lemon fruit was evaluated. Fruit treated with SA + CHGP13 + PI retained their firmness 7 days post-incubation, while the infected control fruit lost their firmness almost immediately. Fruit treated with SingleSA and CHGP13 also retained their firmness compared to the infected control at p = 0.05 analyzed by Tukey’s HSD test. Furthermore, fruit treated with SA + CHGP13 + PI had comparable TSS values to the healthy control treatment 7 days post incubation, with the highest TSS value in infected control fruit at p = 0.05. Similarly, lemon fruit treated with SA + CHGP13 had the least weight loss compared to the other treatments. Fruit treated with SA + CHGP13 + PI had comparable titratable acidity and ascorbic acid content to the healthy control treatment at p = 0.05 (Figure 5).
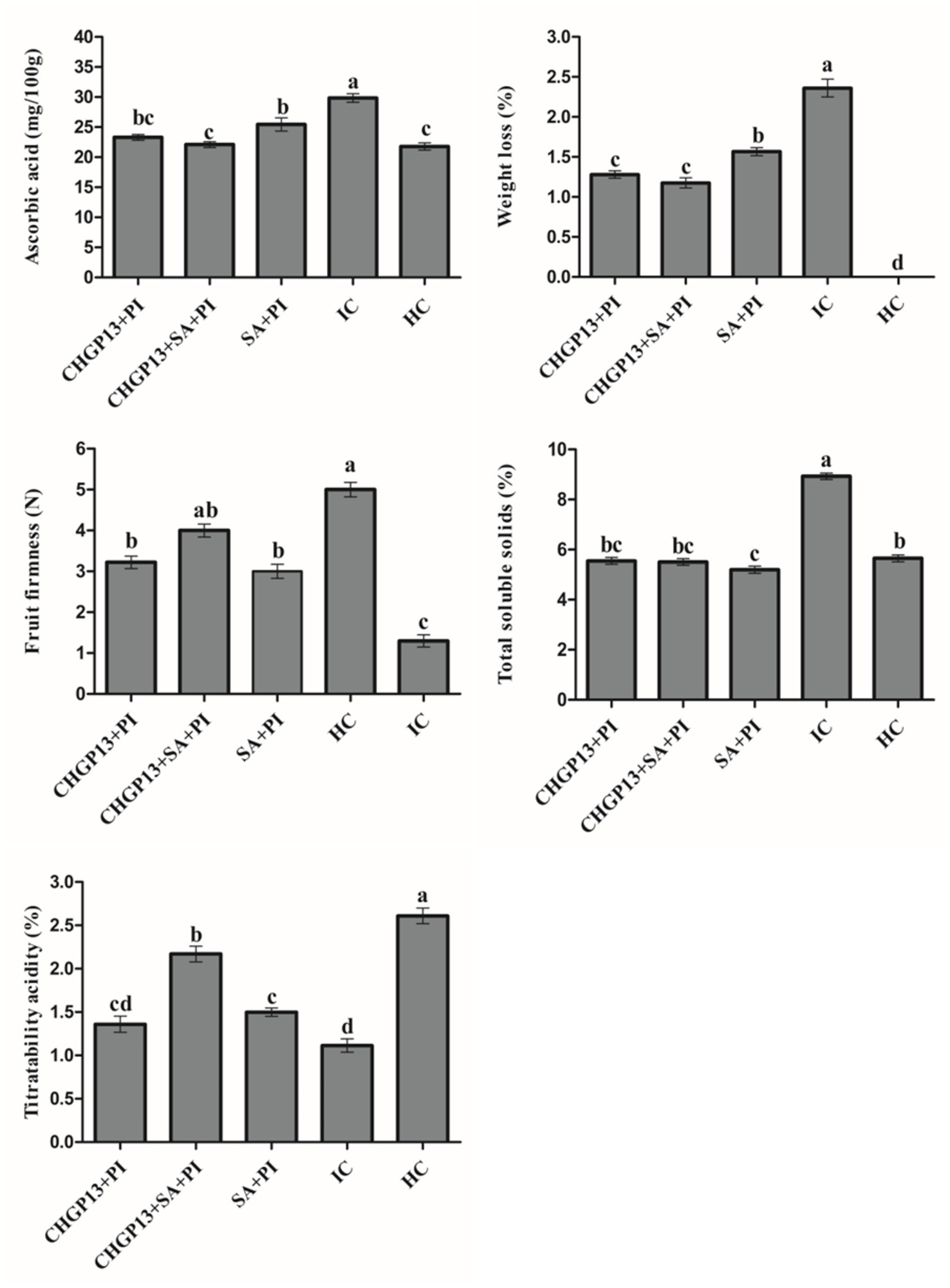
Figure 5. Effect on postharvest quality of lemon fruit after treatment with salicylic acid and lipopeptides extracted from CHGP13 as single and combined treatments. Each bar is an average of 15 replicates from three experiments, analyzed by Tukey’s HSD test in a CRD design. Bars with the same letter do not significantly differ at p = 0.05. Error bars are the standard error of the mean. CHGP13, B. atrophaeus (CHGP13); SA, salicylic acid; PI, P. italicum; IC, infected control; HC, healthy control.
Discussion
Blue mold is a threatening disease causing substantial postharvest losses of citrus fruit (Moosa et al., 2022). Several chemical, physical, and biological control strategies that trigger local and systemic resistance in plants have successfully suppressed blue mold of citrus disease. However, the effectiveness of these treatments is significantly enhanced when combined rather than in single treatments (Moosa et al., 2021, 2022). This study revealed the excellent suppression of blue mold on lemon fruit with the combined application of salicylic acid and lipopeptides extracted from Bacillus.
The induction of defense responses in harvested fruit using non-toxic defense elicitors as an alternative to synthetic chemicals is an outstanding and environmentally friendly management approach for managing postharvest pathogens (Moosa et al., 2021). The use of resistance inducers to improve plant defense has garnered a lot of attention in the last decade (Quaglia et al., 2011; Iqbal et al., 2012; Romanazzi et al., 2016; Moosa et al., 2019, 2021). In this study, the effect of resistance inducers at different concentrations was tested on blue mold of lemon fruit, with SA and BA at the highest concentration producing the best results. In another investigation, the inhibitory effect of SA increased with concentration, successfully stimulating the plant defense response (Verberne et al., 2000). Several studies have reported that the suppressive effect of resistance inducers against green and blue mold of lemon increases in a concentration-dependent manner (Iqbal et al., 2012; Romanazzi et al., 2016; Moosa et al., 2019). SA demonstrated suppressive efficacy against many postharvest pathogens of citrus, pear, and mango, according to earlier research reports (Joyce et al., 2001; Shaat and Galal, 2004; Cao et al., 2006; Moosa et al., 2019).
Bacillus strains are a good alternative to harmful synthetic chemicals for the biological management of postharvest diseases (Janisiewicz and Korsten, 2002). Bacillus-based biopesticide formulations for pre- and post-harvest disease control are predicted to grow in popularity (Fravel, 2005; Droby et al., 2009). The current study intended to investigate the antifungal potential of Bacillus strains against lemon blue mould. Biological control using microbial antagonists, particularly Bacillus species and yeasts is a promising approach for controlling several fruit diseases (Abraham et al., 2010; Osman et al., 2011; Fatima et al., 2023). Bacillus strains tested in this study inhibited the colony growth of P. italicum in a direct antagonism assay. Several studies have reported the biological control potential of Bacillus species against a variety of citrus fruit diseases (Hao et al., 2011; Tian et al., 2020). In support of this work, Mohammadi et al. (2017) reported that B. subtilis, B. pumilus, B. cereus, and B. megaterium suppressed the development of P. digitatum on lemon fruit and inhibited mycelial growth and spore germination. In other investigations, the cell-free supernatant of B. subtilis showed potential antagonistic activity against P. digitatum (Leelasuphakul et al., 2008; Waewthongrak et al., 2015).
Furthermore, the present study was focused on the production of lipopeptides by Bacillus species to control the blue mold of lemon. In a filter paper disc assay, LPs extracted from Bacillus strains CHGP13 and CHGP17 strongly reduced P. italicum growth. Bacillus species have antagonistic potential due to the production of a variety of secondary metabolites (Schneider et al., 1999; Stein, 2005), including antibiotics, lipopeptides, low molecular weight volatile compounds, and antifungal proteins (Huang and Chen, 2008; Zhao et al., 2013; Farzand et al., 2019a). The antimicrobial activity of Bacillus strains has been linked to the production of LPs in several previous reports (Shoda, 2000; Stein, 2005; Ongena and Jacques, 2008; Farzand et al., 2019a,b; Fatima et al., 2023). The LPs produced by Bacillus strains, such as fengycin, iturin, and surfactin, reduced the development of several postharvest diseases, such as gray mold of apple and brown rot of peach (Gueldner et al., 1988; Touré et al., 2004; Ongena et al., 2005). Therefore, the biological control potential of Bacillus strains tested in this study can be attributed to the production of antifungal LPs.
Bacillus strains and resistance inducers alone do not provide comparable disease control to synthetic chemicals (Walters et al., 2005; Moosa et al., 2019). However, the effectiveness of Bacillus strains can be enhanced by combining mixtures of antagonistic agents with other management practices. In the present study, the LPs extracted from Bacillus strain CHGP13 combined with SA further reduced the development of blue mold on lemon fruit and the postharvest quality of fruit including ascorbic acid, titratable acidity, fruit firmness, weight loss, and total soluble solids was not affected. In support of this work, Arrebola et al. (2010) revealed that combining B. amyloliquefaciens PPCB004 and thyme or lemon grass oil with strong antifungal activity inhibited postharvest pathogens of peach, including B. cinerea, P. expansum, and R. stolonifera, compared to single applications. In another instance, El Hamss et al. (2023) reported that the bioefficacy of B. amyloliquefaciens SF14 was enhanced in combination with salicylic acid to suppress green mold of citrus and the treatments were safe to the quality of fruit. Similarly, Zhou et al. (2014) reported that Pichia membranaefaciens and salicylic acid were more effective in combination to suppress green mold decay of citrus fruit and did not impair the postharvest quality of fruit. As a result, based on the current findings and multiple earlier investigations, it may be inferred that combining one or more management methods gives superior disease suppression without affecting the postharvest fruit quality.
Furthermore, in order to decipher the defense mechanisms underlying the suppression of blue mold of lemon, the activity of PPO, POD, and PAL enzymes were measured using a spectrophotometer. The activity of PPO, POD, and PAL was highest in lemon fruit treated with combined application of CHGP13 and SA. Resistance induction in harvested fruit is an effective strategy for improving the suppression of fungal pathogens associated with postharvest rots (Cao et al., 2006). According to our findings, SA and CHGP13 boosted the activity of PPO, POD, and PAL in suppressing blue mold infection on lemon. PPO is a critical enzyme in plant defense responses that converts phenols to poisonous quinones, contributing to its high toxicity against a variety of invading pathogens (Mohammadi and Kazemi, 2002; Moosa et al., 2022). Another key defense enzyme is POD, which catalyses the final stage in the synthesis of lignin and transforms phenolic chemicals to highly toxic quinones. Previously, an increase in PPO and POD activity was seen in citrus fruit treated with a combination of P. membranaefaciens and salicylic acid (Zhou et al., 2014). The phenolic pathway is regulated by PAL, which catalyses the conversion of phenylalanine to trans-cinnamate. Furthermore, PAL is a precursor to scoparone and scopoletin, both of which have been reported to be antifungal against a variety of fungal diseases (Droby et al., 2002). Our findings revealed a link between increased PPO, POD, and PAL activity and the suppression of blue mold of lemon, implying that these defense enzymes play an important role in plant defence. Several prior studies have found that increase in the activity of PPO, PAL, and POD confers increased resistance to fungal pathogens (Shao et al., 2013; Zhou et al., 2014; Moosa et al., 2021, 2022). Therefore, the current study suggests that PAL, PPO, and POD defence enzymes may be associated with better defense of lemons against blue mold disease.
Conclusion
In conclusion, the study presents the promising antifungal potential of LPs extracted from B. atrophaeus CHGP13 and SA against the development of blue mold of lemon. The combined application of LPs and SA shower higher antifungal activity than the single application by enhancing the activity of defense enzymes PAL, PPO, and POD. Furthermore, the treatments had a very little impact on the postharvest quality of lemon fruit. The underlying mechanisms behind the antifungal potential of SA and LPs must be investigated further before these therapies can be included in the integrated disease management programme for blue mold of lemon.
Data availability statement
The raw data supporting the conclusions of this article will be made available by the authors, without undue reservation.
Author contributions
AM conceived the idea of the research. SM and TM performed the experiments. AM, MNA, and FZ supervised the work. SM, AM, and FZ prepared the manuscript draft. AM, FZ, and KHMS reviewed and revised the draft. All authors contributed to the article and approved the submitted version.
Conflict of interest
The authors declare that the research was conducted in the absence of any commercial or financial relationships that could be construed as a potential conflict of interest.
Publisher’s note
All claims expressed in this article are solely those of the authors and do not necessarily represent those of their affiliated organizations, or those of the publisher, the editors and the reviewers. Any product that may be evaluated in this article, or claim that may be made by its manufacturer, is not guaranteed or endorsed by the publisher.
References
Abraham, A. O., Laing, M. D., and Bower, J. P. (2010). Isolation and in vivo screening of yeast and Bacillus antagonists for the control of Penicillium digitatum of citrus fruit. Biol. Control 53, 32–38. doi: 10.1016/j.biocontrol.2009.12.009
Algam, S. A., Guan-lin, X., and Coosemans, J. (2005). Delivery methods for introducing endophytic Bacillus into tomato and their effect on growth promotion and suppression of tomato wilt. Plant Pathol. J. 4, 69–74. doi: 10.3923/ppj.2005.69.74
Arrebola, E., Jacobs, R., and Korsten, L. (2010). Iturin a is the principal inhibitor in the biocontrol activity of Bacillus amyloliquefaciens PPCB004 against postharvest fungal pathogens. J. Applied Microbiol. 108, 386–395. doi: 10.1111/j.1365-2672.2009.04438.x
Asghari, M., and Aghdam, M. S. (2010). Impact of salicylic acid on postharvest physiology of horticultural crops. Trends Food Sci. Technol. 21, 502–509. doi: 10.1016/j.tifs.2010.07.009
Ballester, A.-R., Teresa Lafuente, M., and González-Candelas, L. (2013). Citrus phenylpropanoids and defense against pathogens. Part II: gene expression and metabolite accumulation in the response of fruit to Penicillium digitatum infection. Food Chem. 136, 285–291. doi: 10.1016/j.foodchem.2012.08.006
Bill, M., Korsten, L., Remize, F., Glowacz, M., and Sivakumar, D. (2017). Effect of thyme oil vapours exposure on phenylalanine ammonia-lyase (PAL) and lipoxygenase (LOX) genes expression, and control of anthracnose in ‘Hass’ and ‘Ryan’ avocado fruit. Sci. Hort. 224, 232–237. doi: 10.1016/j.scienta.2017.06.026
Bowles, D. J. (1990). Defense-related proteins in higher plants. Annu. Rev. Biochem. 59, 873–907. doi: 10.1146/annurev.bi.59.070190.004301
Campa, A. (1991). “Biological role of plant peroxidases: known and potential functions,” in Peroxidases in chemistry and biology. eds. J. Everse, K. E. Everse, and M. B. Grisham (FL: CRC Press. Inc.), 25–50.
Cao, J., Zeng, K., and Jiang, W. (2006). Enhancement of postharvest disease resistance in Ya Li pear (Pyrus bretschneideri) fruit by salicylic acid sprays on the trees during fruit growth. European J. Plant Pathol. 114, 363–370. doi: 10.1007/s10658-005-5401-8
Cirvilleri, G. (2008). “Bacteria for biological control of posthar- vest diseases of fruit,” in Plant-microbe interactions. eds. E. Ait Barka and C. Clément (India, Research Sign- post: Kerala), 1–2.
Cunha, T., Ferraz, L. P., Wehr, P. P., and Kupper, K. C. (2018). Antifungal activity and action mechanisms of yeasts isolates from citrus against Penicillium italicum. Int. J. Food Microbiol. 276, 20–27. doi: 10.1016/j.ijfoodmicro.2018.03.019
Droby, S., Vinokur, V., Weiss, B., Cohen, L., Daus, A., Goldschmidt, E. E., et al. (2002). Induction of resistance to Penicillium digitatum in grapefruit by the yeast biocontrol agent candida oleophila. Phytopathology 92, 393–399. doi: 10.1094/PHYTO.2002.92.4.393
Droby, S., Wisniewski, M., Macarisin, D., and Wilson, C. (2009). Twenty years of postharvest biocontrol research: is it time for a new paradigm? Postharvest Biol. Technol. 52, 137–145. doi: 10.1016/j.postharvbio.2008.11.009
El Hamss, H., Kajad, N., Belabess, Z., and Lahlali, R. (2023). Enhancing bioefficacy of Bacillus amyloliquefaciens SF14 with salicylic acid for the control of the postharvest citrus green mould. Plant Stress. 7:100144. doi: 10.1016/j.stress.2023.100144
Farzand, A., Moosa, A., Zubair, M., Khan, A. R., Ayaz, M., Massawe, V. C., et al. (2020). Transcriptional profiling of diffusible lipopeptides and fungal virulence genes during Bacillus amyloliquefaciens EZ1509-mediated suppression of Sclerotinia sclerotiorum. Phytopathology 110, 317–326. doi: 10.1094/PHYTO-05-19-0156-R
Farzand, A., Moosa, A., Zubair, M., Khan, A. R., Hanif, A., Tahir, H. A. S., et al. (2019a). Marker assisted detection and LC-MS analysis of antimicrobial compounds in different Bacillus strains and their antifungal effect on Sclerotinia sclerotiorum. Biol. Control 133, 91–102. doi: 10.1016/j.biocontrol.2019.03.014
Farzand, A., Moosa, A., Zubair, M., Khan, A. R., Massawe, V. C., Tahir, H. A. S., et al. (2019b). Suppression of Sclerotinia sclerotiorum by the induction of systemic resistance and regulation of antioxidant pathways in tomato using fengycin produced by Bacillus amyloliquefaciens FZB42. Biomol. Ther. 9:613. doi: 10.3390/biom9100613
Fatima, R., Mahmood, T., Moosa, A., Aslam, M. N., Shakeel, M. T., Maqsood, A., et al. (2023). Bacillus thuringiensis CHGP12 uses a multifaceted approach for the suppression of fusarium oxysporum f. sp. ciceris and to enhance the biomass of chickpea plants. Pest Management Sci. 79, 336–348. doi: 10.1002/ps.7203
Finking, R., and Marahiel, M. A. (2004). Biosynthesis of nonribosomal peptides. Annu. Rev. Microbiol. 58, 453–488. doi: 10.1146/annurev.micro.58.030603.123615
Fira, D., Dimkić, I., Berić, T., Lozo, J., and Stanković, S. (2018). Biological control of plant pathogens by Bacillus species. J. Biotechnol. 285, 44–55. doi: 10.1016/j.jbiotec.2018.07.044
Fravel, D. R. (2005). Commercialization and implementation of biocontrol. Annu. Rev. Phytopathol. 43, 337–359. doi: 10.1146/annurev.phyto.43.032904.092924
Gueldner, R. C., Reilly, C. C., Pusey, P. L., Costello, C. E., Arrendale, R. F., Cox, R. H., et al. (1988). Isolation and identification of iturins as antifungal peptides in biological control of peach brown rot with Bacillus subtilis. J. Agric. Food Chem. 36, 366–370. doi: 10.1021/jf00080a031
Guo, J., Fang, W., Lu, H., Zhu, R., Lu, L., Zheng, X., et al. (2014). Inhibition of green mold disease in mandarins by preventive applications of methyl jasmonate and antagonistic yeast Cryptococcus laurentii. Postharvest Biol. Technol. 88, 72–78. doi: 10.1016/j.postharvbio.2013.09.008
Hammerschmidt, R., Nuckles, E. M., and Kuc, J. (1981). Association of enhanced peroxidase activity with induced systemic resistance of cucumber to Colletotrichum lagenarium. Physiol. Plant Pathol. 20, 73–82. doi: 10.1016/0048-4059(82)90025-X
Hao, W., Li, H., Hu, M., Yang, L., and Rizwan-ul-Haq, M. (2011). Integrated control of citrus green and blue mold and sour rot by Bacillus amyloliquefaciens in combination with tea saponin. Postharvest Biol. Technol. 59, 316–323. doi: 10.1016/j.postharvbio.2010.10.002
Hasanuzzaman, M., Bhuyan, M. B., Zulfiqar, F., Raza, A., Mohsin, S. M., Mahmud, J. A., et al. (2020). Reactive oxygen species and antioxidant defense in plants under abiotic stress: revisiting the crucial role of a universal defense regulator. Antioxidants. 9:681. doi: 10.3390/antiox9080681
He, D. C., He, M. H., Amalin, D. M., Liu, W., Alvindia, D. G., and Zhan, J. (2021). Biological control of plant diseases: an evolutionary and eco-economic consideration. Pathogens. 10:1311. doi: 10.3390/pathogens10101311
Hernández, Y., Lobo, M. G., and González, M. (2006). Determination of vitamin C in tropical fruits: A comparative evaluation of methods. Food Chem. 96, 654–664. doi: 10.1016/j.foodchem.2005.04.012
Huang, C. J., and Chen, C. Y. (2008). Synergistic interactions between chitinase ChicCW and fungicides against plant fungal pathogens. J. Microbiol. Biotechnol. 18, 784–787.
Iqbal, Z., Singh, Z., Khangura, R., and Ahmad, S. (2012). Management of citrus blue and green moulds through application of organic elicitors. Australas. Plant Pathol. 41, 69–77. doi: 10.1007/s13313-011-0091-5
Janisiewicz, W., and Korsten, L. (2002). Biological control of postharvest diseases of fruit. Annu. Rev. Phytopathol. 40, 411–441. doi: 10.1146/annurev.phyto.40.120401.130158
Jones, E., and Hughes, R. E. (1983). Foliar ascorbic acid in some angiosperms. Phytochemistry 22, 2493–2499. doi: 10.1016/0031-9422(83)80147-2
Joyce, D. C., Wearing, H., Coates, L., and Terry, L. (2001). Effects of phosphonate and salicylic acid treatments on anthracnose disease development and ripening of' Kensington Pride' mango fruit. Australian J. Exp. Agric. 41, 805–813. doi: 10.1071/EA99104
Kato, M., and Shimizu, S. (1985). Chlorophyll metabolism in higher plants VI. Involvement of peroxidase in chlorophyll degradation. Plant Cell Physiol. 26, 1291–1301.
Lacey, K., Hancock, N., and Ramsey, H. (2009). Measuring internal maturity of citrus. Farm Note. 354, 1–4.
Leelasuphakul, W., Hemmanee, P., and Chuenchitt, S. (2008). Growth inhibitory properties of Bacillus subtilis strains and their metabolites against the green mold pathogen (Penicillium digitatum Sacc.) of citrus fruit. Postharvest Biol. Technol. 48, 113–121. doi: 10.1016/j.postharvbio.2007.09.024
Liu, Y. Q., Heying, E., and Tanumihardjo, S. A. (2012). History, global distribution, and nutritional importance of citrus fruit. Compr. Rev. Food Sci. Food Saf. 11, 530–545. doi: 10.1111/j.1541-4337.2012.00201.x
Livak, K. J., and Schmittgen, T. D. (2001). Analysis of relative gene expression data using real-time quantitative PCR and the 2−∆∆CT method. Methods 25, 402–408. doi: 10.1006/meth.2001.1262
Ma, B., Gao, L., Zhang, H., Cui, J., and Shen, Z. (2012). Aluminum-induced oxidative stress and changes in antioxidant defenses in the roots of rice varieties differing in Al tolerance. Plant Protect. Sci. 31, 687–696. doi: 10.1007/s00299-011-1187-7
Mahaffee, W. F., and Kloepper, J. W. (1997). Temporal changes in the bacterial communities of soil, rhizosphere, and Endorhiza associated with field-grown cucumber (Cucumis sativus L.). Microb. Ecol. 34, 210–223. doi: 10.1007/s002489900050
Martinez-Tellez, M. A., and Lafuente, M. T. (1997). Effect of high temperature conditioning on ethylene, phenylalanine ammonia-lyase, peroxidase and polyphenol oxidase activities in flavedo of chilled‹ fortune› mandarin fruit. J. Plant Physiol. 150, 674–678. doi: 10.1016/S0176-1617(97)80282-9
Mohammadi, M., and Kazemi, H. (2002). Changes in peroxidase and polyphenol oxidase activities in susceptible and resistant wheat heads inoculated with fusarium graminearum and induced resistance. Plant Sci. 162, 491–498. doi: 10.1016/S0168-9452(01)00538-6
Mohammadi, P., Tozlu, E., Kotan, R., and Kotan, M. Ş. (2017). Potential of some bacteria for biological control of postharvest citrus green mould caused by Penicillium digitatum. Plant Protect. Sci. 53, 134–143. doi: 10.17221/55/2016-PPS
Moosa, A., Farzand, A., Sahi, S. T., and Khan, S. A. (2018). Transgenic expression of antifungal pathogenesis-related proteins against phytopathogenic fungi–15 years of success. Israel J. Plant Sci. 65, 1–17. doi: 10.1080/07929978.2017.1288407
Moosa, A., Farzand, A., Sahi, S. T., Khan, S. A., Aslam, M. N., and Zubair, M. (2021). Salicylic acid and Cinnamomum verum confer resistance against Penicillium rot by modulating the expression of defense linked genes in Citrus reticulata Blanco. Postharvest Biol. Technol. 181:111649. doi: 10.1016/j.postharvbio.2021.111649
Moosa, A., Sahi, S. T., Khan, S. A., and Malik, A. U. (2019). Salicylic acid and jasmonic acid can suppress green and blue moulds of citrus fruit and induce the activity of polyphenol oxidase and peroxidase. Folia Hortic. 31, 195–204. doi: 10.2478/fhort-2019-0014
Moosa, A., Zulfiqar, F., and Siddique, K. H. (2022). Transcriptional and biochemical profiling of defense enzymes in Citrus sinensis during salicylic acid and cinnamon mediated suppression of green and blue mold. Front. Plant Sci. 13:1048433. doi: 10.3389/fpls.2022.1048433
Naqvi, S. A. M. H. (2004). “Diagnosis and Management of pre and Postharvest Diseases of Citrus fruit” in Diseases of Fruit and Vegetables Volume I: Diagnosis and Management. ed. S. A. M. H. Naqvi (Netherlands, Dordrecht: Springer), 339–359.
Nunes, C. A. (2012). Biological control of postharvest diseases of fruit. Eur. J. Plant Pathol. 133, 181–196. doi: 10.1007/s10658-011-9919-7
Ongena, M., and Jacques, P. (2008). Bacillus lipopeptides: versatile weapons for plant disease biocontrol. Trends Microbiol. 16, 115–125. doi: 10.1146/annurev.phyto.40.120401.130158
Ongena, M., Jacques, P., Touré, Y., Destain, J., Jabrane, A., and Thonart, P. (2005). Involvement of fengycin-type lipopeptides in the multifaceted biocontrol potential of Bacillus subtilis. Appl. Microbiol. Biotechnol. 69, 29–38. doi: 10.1007/s00253-005-1940-3
Osman, M. S., Sivakumar, D., and Korsten, L. (2011). Effect of biocontrol agent Bacillus amyloliquefaciens and 1-methyl cyclopropene on the control of postharvest diseases and maintenance of fruit quality. Crop Prot. 30, 173–178. doi: 10.1016/j.cropro.2010.09.014
Palou, L., Smilanick, J. L., and Droby, S. (2008). Alternatives to conventional fungicides for the control of citrus postharvest green and blue moulds. Stewart Postharvest Rev. 4, 1–16. doi: 10.2212/spr.2008.2.2
Palou, L., Smilanick, J. L., Usall, J., and Vigrıas, I. (2001). Control of postharvest blue and green molds of oranges by hot water, sodium carbonate and sodium bicarbonate. Plant Dis. 85, 371–376. doi: 10.1094/PDIS.2001.85.4.371
Papoutsis, K., Mathioudakis, M. M., Hasperué, J. H., and Ziogas, V. (2019). Nonchemical treatments for preventing the postharvest fungal rotting of citrus caused by Penicillium digitatum (green Mold) and Penicillium italicum (blue Mold). Trends Food Sci. Technol. 86, 479–491. doi: 10.1016/j.tifs.2019.02.053
Porat, R., Daus, A., Weiss, B., Cohen, L., Fallik, E., and Droby, S. (2000). Reduction of postharvest decay in organic citrus fruit by a short hot water brushing treatment. Postharvest Biol. Technol. 18, 151–157. doi: 10.1016/S0925-5214(99)00065-4
Qin, G. Z., Tian, S. P., Xu, Y., and Wan, Y. K. (2003). Enhancement of biocontrol efficacy of antagonistic yeasts by salicylic acid in sweet cherry fruit. Physiol. Mol. Plant Pathol. 62, 147–154. doi: 10.1016/S0885-5765(03)00046-8
Quaglia, M., Ederli, L., Pasqualini, S., and Zazzerini, A. (2011). Biological control agents and chemical inducers of resistance for postharvest control of Penicillium expansum link. On apple fruit. Posthar. Biol. Technol. 59, 307–315. doi: 10.1016/j.postharvbio.2010.09.007
Romanazzi, G., Sanzani, S. M., Bi, Y., Tian, S., Martınez, P. G., and Alkan, N. (2016). Induced resistance to control postharvest decay of fruit and vegetables. Postharvest Biol. Technol. 122, 82–94. doi: 10.1016/j.postharvbio.2016.08.003
Sala, J. M., Sanchez-Ballesta, M. T., Alférez, F., Mulas, M., Zacarias, L., and Lafuente, M. T. (2005). A comparative study of the postharvest performance of an ABA deficient mutant of oranges II. Antioxidant enzymatic system and phenylalanine ammonia-lyase in non-chilling and chilling peel disorders of citrus fruit. Postharvest Biol. Technol. 37, 232–240. doi: 10.1016/j.postharvbio.2005.05.006
Sardar, H., Khalid, Z., Ahsan, M., Naz, S., Nawaz, A., Ahmad, R., et al. (2023). Enhancement of salinity stress tolerance in lettuce (Lactuca sativa L.) via foliar application of nitric oxide. Plan. Theory 12:1115. doi: 10.3390/plants12051115
Schneider, J., Taraz, K., Budzikiewicz, H., Deleu, M., Thonart, P., and Jacques, P. (1999). The structure of two fengycins from Bacillus subtilis S499. Zeitschrift für Naturforschung C. 54, 859–866. doi: 10.1515/znc-1999-1102
Scott-Craig, J. S., Kerby, K. B., Stein, B. D., and Somerville, S. C. (1995). Expression of an extracellular peroxidase that is induced in barley (Hordeum vulgare) by the powdery mildew pathogen (Erysiphe graminis f. sp. hordei). Physiol. Mol. Plant Pathol. 47, 407–418. doi: 10.1006/pmpp.1995.1068
Shaat, M., and Galal, A. (2004). Response of citrus fruit to pre-harvest antioxidant spraying and infection with Alternaria fruit rot and green mould. Ann. Agr. Sci. (Cairo) 49, 747–758.
Shao, X., Wang, H., Xu, F., and Cheng, S. (2013). Effects and possible mechanisms of tea tree oil vapor treatment on the main disease in postharvest strawberry fruit. Postharvest Biol. Technol. 77, 94–101. doi: 10.1016/j.postharvbio.2012.11.010
Sharma, R. R., Singh, D., and Singh, R. (2009). Biological control of postharvest diseases of fruit and vegetables by microbial antagonists: a review. Biol. Control 50, 205–221. doi: 10.1016/j.biocontrol.2009.05.001
Shoda, M. (2000). Bacterial control of plant diseases. J. Biosci. Bioeng. 89, 515–521. doi: 10.1016/S1389-1723(00)80049-3
Stein, T. (2005). Bacillus subtilis antibiotics: structures, syntheses and specific functions. Mol. Microbiol. 56, 845–857. doi: 10.1111/j.1365-2958.2005.04587.x
Sukorini, H., Sangchote, S., and Khewkhom, N. (2013). Plant crude extracts and yeast as alternative to synthetic fungicide for controlling postharvest green mould on citrus Kinnow. Acta Univ. Agric. Silvic. Mendelianae Brun. 61, 795–801. doi: 10.11118/actaun201361030795
Terry, L. A., and Joyce, D. C. (2004). Elicitors of induced disease resistance in postharvest horticultural crops: a brief review. Postharvest Biol. Technol. 32, 1–13. doi: 10.1016/j.postharvbio.2003.09.016
Tian, Z., Chen, C., Chen, K., Liu, P., Fan, Q., Zhao, J., et al. (2020). Biocontrol and the mechanisms of Bacillus sp. w176 against postharvest green mold in citrus. Postharvest Biol. Technol. 159:111022. doi: 10.1016/j.postharvbio.2019.111022
Tian, S. P., Fan, Q., Xu, Y., and Liu, H. B. (2002). Biocontrol efficacy of antagonist yeasts to grey mold and blue mold on apples and pears in controlled atmospheres. Plant Dis. 86, 848–853. doi: 10.1094/PDIS.2002.86.8.848
Touré, Y., Ongena, M., Jacques, P., Guiro, A., and Thonart, P. (2004). Role of lipopeptides produced by Bacillus subtilis GA1 in the reduction of grey mould disease caused by Botrytis cinerea on apple. J. App. Microbiol. 96, 1151–1160. doi: 10.1111/j.1365-2672.2004.02252.x
Tripathi, P., and Dubey, N. K. (2004). Exploitation of natural products as an alternative strategy to control postharvest fungal rotting of fruit and vegetables. Postharvest Biol. Technol. 32, 235–245. doi: 10.1016/j.postharvbio.2003.11.005
Verberne, M. C., Verpoorte, R., Bol, J. F., Mercado-Blanco, J., and Linthorst, H. J. (2000). Overproduction of salicylic acid in plants by bacterial transgenes enhances pathogen resistance. Nat. Biotechnol. 18, 779–783. doi: 10.1038/77347
Waewthongrak, W., Pisuchpen, S., and Leelasuphakul, W. (2015). Effect of Bacillus subtilis and chitosan applications on green mold (Penicilium digitatum Sacc.) decay in citrus fruit. Postharvest Biol. Technol. 99, 44–49. doi: 10.1016/j.postharvbio.2014.07.016
Walters, D., Walsh, D., Newton, A., and Lyon, G. (2005). Induced resistance for plant disease control: maximizing the efficacy of resistance elicitors. Phytopathology 95, 1368–1373. doi: 10.1094/PHYTO-95-1368
Wisniewski, M. E., and Wilson, C. L. (1992). Biological control of postharvest diseases of fruit and vegetables: recent advances. HortSci. 27, 94–98. doi: 10.21273/HORTSCI.27.2.94
Wyatt, M. K., Parish, M. E., Widmer, W. W., and Kimbrough, J. (1995). Characterization of mould growth in orange juice. Food Microbiol. 12, 347–355. doi: 10.1016/S0740-0020(95)80115-4
Yao, H., and Tian, S. (2005). Effects of pre-and postharvest application of salicylic acid or methyl jasmonate on inducing disease resistance of sweet cherry fruit in storage. Postharvest Biol. Technol. 35, 253–262. doi: 10.1016/j.postharvbio.2004.09.001
Zhao, X., Zhao, X., Wei, Y., Shang, Q., and Liu, Z. (2013). Isolation and identification of a novel antifungal protein from a rhizobacterium Bacillus subtilis strain F3. J. Phytopathol. 161, 43–48. doi: 10.1111/jph.12015
Zhou, Y., Ma, J., Xie, J., Deng, L., Yao, S., and Zeng, K. (2018). Transcriptomic and biochemical analysis of highlighted induction of phenylpropanoid pathway metabolism of citrus fruit in response to salicylic acid, Pichia membranaefaciens and oligochitosan. Postharvest Biol. Technol. 142, 81–92. doi: 10.1016/j.postharvbio.2018.01.021
Zhou, Y., Ming, J., Deng, L., and Zeng, K. (2014). Effect of Pichia membranaefaciens in combination with salicylic acid on postharvest blue and green mold decay in citrus fruits. Biol. Control 74, 21–29. doi: 10.1016/j.biocontrol.2014.03.007
Keywords: Penicillium italicum, resistance inducer, benzoic acid, Citrus limon, fruit, postharvest, enzyme activity
Citation: Maalik S, Moosa A, Zulfiqar F, Aslam MN, Mahmood T and Siddique KHM (2023) Endophytic Bacillus atrophaeus CHGP13 and salicylic acid inhibit blue mold of lemon by regulating defense enzymes. Front. Microbiol. 14:1184297. doi: 10.3389/fmicb.2023.1184297
Edited by:
Yan Li, Chinese Academy of Agricultural Sciences, ChinaReviewed by:
Sami Abou Fayssal, University of Forestry, BulgariaKaituo Wang, Chongqing Three Gorges University, China
Sunjeet Kumar, Hainan University, China
Copyright © 2023 Maalik, Moosa, Zulfiqar, Aslam, Mahmood and Siddique. This is an open-access article distributed under the terms of the Creative Commons Attribution License (CC BY). The use, distribution or reproduction in other forums is permitted, provided the original author(s) and the copyright owner(s) are credited and that the original publication in this journal is cited, in accordance with accepted academic practice. No use, distribution or reproduction is permitted which does not comply with these terms.
*Correspondence: Anam Moosa, YW5hbW1vb3NhMUBvdXRsb29rLmNvbQ==; Faisal Zulfiqar, Y2guZmFpc2FsLnp1bGZpcWFyQGdtYWlsLmNvbQ==; Kadambot H. M. Siddique, a2FkYW1ib3Quc2lkZGlxdWVAdXdhLmVkdS5hdQ==
†These authors have contributed equally to this work and share first authorship