- 1Biofuels Institute, School of the Environment and Safety Engineering, Jiangsu University, Zhenjiang, China
- 2Department of Biology, College of Science, Taif University, Taif, Saudi Arabia
- 3Department of Functional and Evolutionary Ecology, University of Vienna, Vienna, Austria
- 4Botany Department, Faculty of Science, Tanta University, Tanta, Egypt
Some essential information on gut bacterial profiles and their unique contributions to food digestion in wood-feeding termites (WFT) and soil-feeding termites (SFT) is still inadequate. The feeding type of termites is hypothesized to influence their gut bacterial composition and its functionality in degrading lignocellulose or other organic chemicals. This could potentially provide alternative approaches for the degradation of some recalcitrant environmental chemicals. Therefore, metagenomic analysis can be employed to examine the composition and functional profiles of gut bacterial symbionts in WFT and SFT. Based on the metagenomic analysis of the 16S rRNA gene sequences of gut bacterial symbionts in the WFT, Microcerotermes sp., and the SFT, Pericapritermes nitobei, the findings revealed a total of 26 major bacterial phyla, with 18 phyla commonly represented in both termites, albeit in varying abundances. Spirochaetes dominated the bacterial symbionts in Microcerotermes sp. at 55%, followed by Fibrobacters, while Firmicutes dominated the gut bacteria symbionts in P. nitobei at 95%, with Actinobacteria coming in second at 2%. Furthermore, the Shannon and phylogenetic tree diversity indices, as well as the observed operational taxonomic units and Chao 1 richness indices, were all found to be higher in the WFT than in the SFT deduced from the alpha diversity analysis. Based on the principal coordinate analysis, exhibited a significant distance dissimilarity between the gut bacterial symbionts. The results showed that the gut bacterial composition differed significantly between the WFT and SFT. Furthermore, Tax4Fun analysis evaluated bacterial functions, revealing the predominance of carbohydrate metabolism, followed by amino acid metabolism and energy metabolism in both Microcerotermes sp. and P. nitobei termites. The results implicated that bacterial symbionts inhabiting the guts of both termites were actively involved in the degradation of lignocellulose and other recalcitrant compounds.
Introduction
Termites are an extraordinarily effective group of lignocellulose-degrading organisms worldwide that contribute to carbon and nitrogen cycling in the ecosystem (Al-Tohamy et al., 2021; Ali et al., 2021; Xie et al., 2024). They transitioned from an omnivore to a wood-feeding lifestyle over 150 million years ago. This was followed by significant modification of their digestive system, including the notable extension of the hindgut and the attainment of cellulolytic flagellates (Brune, 2014). These flagellates are essential for symbiotic digestion in lower termites but disappeared in the most recent lineage, the Termitidae, or “higher” termites, which emerged over 50 million years and possessed an exclusively prokaryotic gut microbiota (Arora et al., 2022). Higher termites are the most varied of all termite families, accounting for nearly 85% of all termite genera. Though higher termites have evolved in various ways, the most significant difference is their diet, which spans beyond “lower termites,” primarily consuming wood (Korb, 2021). Some higher termites ingest sound lignocellulose, such as wood or dried grass, while others consume leaf litter, herbivore dung, humus, and/or soil (Li and Greening, 2022; Xie et al., 2024). The disappearance of symbiotic flagellates in higher termites may be viewed as an evolutionary stride and infer the adoption of a new mechanism for decomposing and obtaining energy from lignocellulose feed (Chouvenc et al., 2021). Although termites secrete endogenous enzymes, their capacity to decompose a lignocellulose diet largely depends on their mutualistic symbiosis with a variety of gut symbionts (Ali et al., 2017; Ali et al., 2019; Ali et al., 2024; Ali et al., 2023). As a result, the study of termite gut symbionts has received much attention in recent years. This aligns with the practical aims of bioprospecting, focusing on industrial lignocellulose conversion and the production of biofuels and other biochemical products (Danso et al., 2022). Despite decades of research, uncovering the gut microbial diversity and its mechanism for lignocellulose degradation has merely been tackled on the surface. There is yet insufficient data to answer the broader ecological questions, including the effect of different feeding regimes on gut microbial community structure and the functional profile of bacterial populations to the hydrolysis of lignocellulose in termites. Noticeably, less molecular data exist for the majority of higher termite species (Marynowska et al., 2020).
More recent research based on culture-independent techniques has provided greater insights into the gut bacterial community structure in wood-feeding termites (WFT) and their potential roles in deconstructing wood (Xie et al., 2024; Nawaz et al., 2023; Santhoshkumar et al., 2024). Nonetheless, the majority of these studies are focused on the Nasutitermes species. To fully understand wood degradation in termites, gut microbial communities and their functional profiles in several other species of WFT must be investigated. In addition, soil-feeding termite (SFT) species are the least studied of all termite lineages, though they are the most abundant (Marynowska et al., 2020). Contrary to wood, which basically consists of lignin, cellulose, and hemicellulose, soil contains humus enriched in lignocellulosic polysaccharides as well as amino acids and peptides (Marynowska et al., 2023; Mao et al., 2019). The comprehension of termites to thrive on such a complex diet is still lacking owing to the less studied species of this feeding guild.
Besides, the question of whether termites have distinct microbial symbiotic populations with unique capabilities tailored to various feeding regimes is still completely unanswered. Hence, the purpose of this study was to describe the bacterial community structure inhabiting the gut of different feeding groups of termites and to ascertain if their bacterial symbionts employ different functional structures or mechanisms toward the hydrolysis of lignocellulose and other recalcitrant compounds. The gut bacteria community structure and functional profile of two understudied and most abundant termite species in the southern part of China, the WFT Microcerotermes sp., and the SFT Pericapritermes nitobei, were investigated in this study employing a culture-independent approach by deploying metagenomics analysis through next generation sequencing of the V3-V4 region of 16S rRNA genes.
Materials and methods
Termite collection, identification, and gut extraction
The termite samples used in this study were collected from Xishuangbanna Tropical Botanical Garden, China. Genomic DNA was extracted using a MiniBEST Universal Genomic DNA Extraction Kit (TaKaRa) as described previously (Danso et al., 2022). The cytochrome c oxidase polypeptide II (COII) gene sequencing PCR was performed using universal primers COIIF (5′CAGATAAGTGCATTGGATTT-3′) and COIIR (5′-GTTTAAGAGACCAGTACTTG-3′). The phylogenetic tree was constructed using molecular evolutionary genetics analysis version 7.0 software.
To extract the whole gut, 100 worker-caste of both termite species were placed in a petri dish and sterilized with 70% ethanol, followed by a quick rinse with sterile double-distilled water. The surface-sterilized termites were dissected using sterile instruments under aseptic conditions in laminar airflow. The guts of sterile termites were gently pulled out using sterilized forceps and deposited in a 2 mL Eppendorf tube for metagenomic microbial DNA extraction. Three replicates were prepared for each termite species.
Amplicon high-throughput sequencing
The extraction of microbial DNA was performed using the HiPure Soil DNA Kits (Magen Guangzhou, China) following the manufacturer’s instructions. The PCR technique was used to amplify the 16S rDNA targeting the V3–V4 region of the ribosomal RNA gene. The amplification process involved subjecting the sample to a temperature of 94°C for 2 min, followed by 30 cycles at 98°C for 10 s, 62°C for 30 s, and 68°C for 30 s. A final extension step was performed at 68°C for 5 min. Specific primers, 341F (5′-CCTACGGGNGGCWGCAG-3′) and 80R (5′-GGACTACHVGGGTATCTAAT-3′), were used for this amplification process according to the standard protocols.
Bioinformatic and statistical analysis
Raw data containing adapters or low-quality reads were filtered using FASTP to obtain high-quality reads (Chen et al., 2018). Paired-end clean reads were merged as raw tags using FLSAH with a minimum overlap of 10 bp and mismatch error rates of 2% (Magoč and Salzberg, 2011). Noisy sequences of raw tags were filtered to obtain high-quality clean tags by QIIME pipeline under specific filtering conditions (Caporaso et al., 2010). Firstly, the low-quality regions (minimum default quality ≤3; default minimum length ≥ 3) in the raw tags have been identified and split the raw tag at the first low-quality base in the region; secondly, the tag length of a continuous sequence of high-quality bases <75% were removed.
The reference database (version r20110519)1 was used to search for clean tags in order to perform reference-based chimaera checking using the UCHIME algorithm (Edgar et al., 2011). All hybrid tags were eliminated, and only efficient tags were retrieved for subsequent study. The efficient labels were grouped into operational taxonomic units (OTUs) with a similarity of at least 97% using the UPARSE pipeline (Edgar, 2013). A representative sequence was chosen from each cluster based on the tag sequence with the highest abundance. OTUs were taxonomically assigned at a confidence threshold of 80% based on the DictDb database (Schloss et al., 2009). Singleton OTUs and sequences identified as chloroplasts or mitochondria were removed from the analysis. OTUs abundance information was normalized with the least sequence number for sample comparison at the same surveying effort. The abundance statistics of each taxonomy were visualized using Krona (Ondov et al., 2011). The stacked bar plot of the community composition was visualized in the R project ggplot2 package (Wickham and Chang, 2008). A heatmap of species abundance in the R project was plotted using the pheatmap package (Kolde and Vilo, 2015). Alpha diversity indices, such as Chao1, Shannon, observed OTUs, and PD-whole tree, were computed using QIIME (Caporaso et al., 2010). The R project ggplot2 package was used to create OTUs rarefaction and rank abundance curves. The alpha index comparison across groups was computed using Welch’s t-test in the R project Vegan package (Oksanen, 2010).
To determine the β diversity, sequence alignment was performed using Muscle version 3.8.31 (Edgar, 2004), and the phylogenetic tree was constructed using FastTree (Price et al., 2010), then a weighted unifrac distance matrix was generated by the GuniFrac package in the R project (Lozupone and Knight, 2005). The Bray-Curtis distance matrix was calculated in the R project Vegan package (Oksanen, 2010). Multivariate statistical techniques, including principal coordinates analysis (PCoA) of weighted unifrac and bray-Curtis distances, were generated in the R project Vegan package and plotted in the R project ggplot2 package (Wickham and Chang, 2008; Oksanen, 2010). The Kyoto encyclopedia of genes and genomes (KEGG) pathway analysis of the OTUs was inferred using Tax4Fun, which has been shown to provide a good correlation of functional profiles with metagenomic profiles derived from direct sequencing (Aßhauer et al., 2015).
Results and discussion
Termite identification and molecular phylogeny
A phylogenetic study based on COII gene sequencing was carried out to taxonomically identify the WFT and SFT used in this study. Searching the attained sequences to similar nucleotides in the Basic Local Alignment Search Tool (BLAST) indicated that the termites were affiliated with the most recent family of higher termites, Termitidae. WFT shared the highest similarity with Microcerotermes sp. MmPP5 (97.7%), while SFT shared a high similarity of 100% with Pericapritermes nitobei (GenBank accession number: MW073099.1). For maximum likelihood phylogeny (Figure 1), the bootstrap values strongly support the branches of the tree, indicating a close relationship between WFT and Microcerotermes sp. (with 98% bootstrap support), as well as SFT and Pericapritermes nitobei (100% bootstrap support).
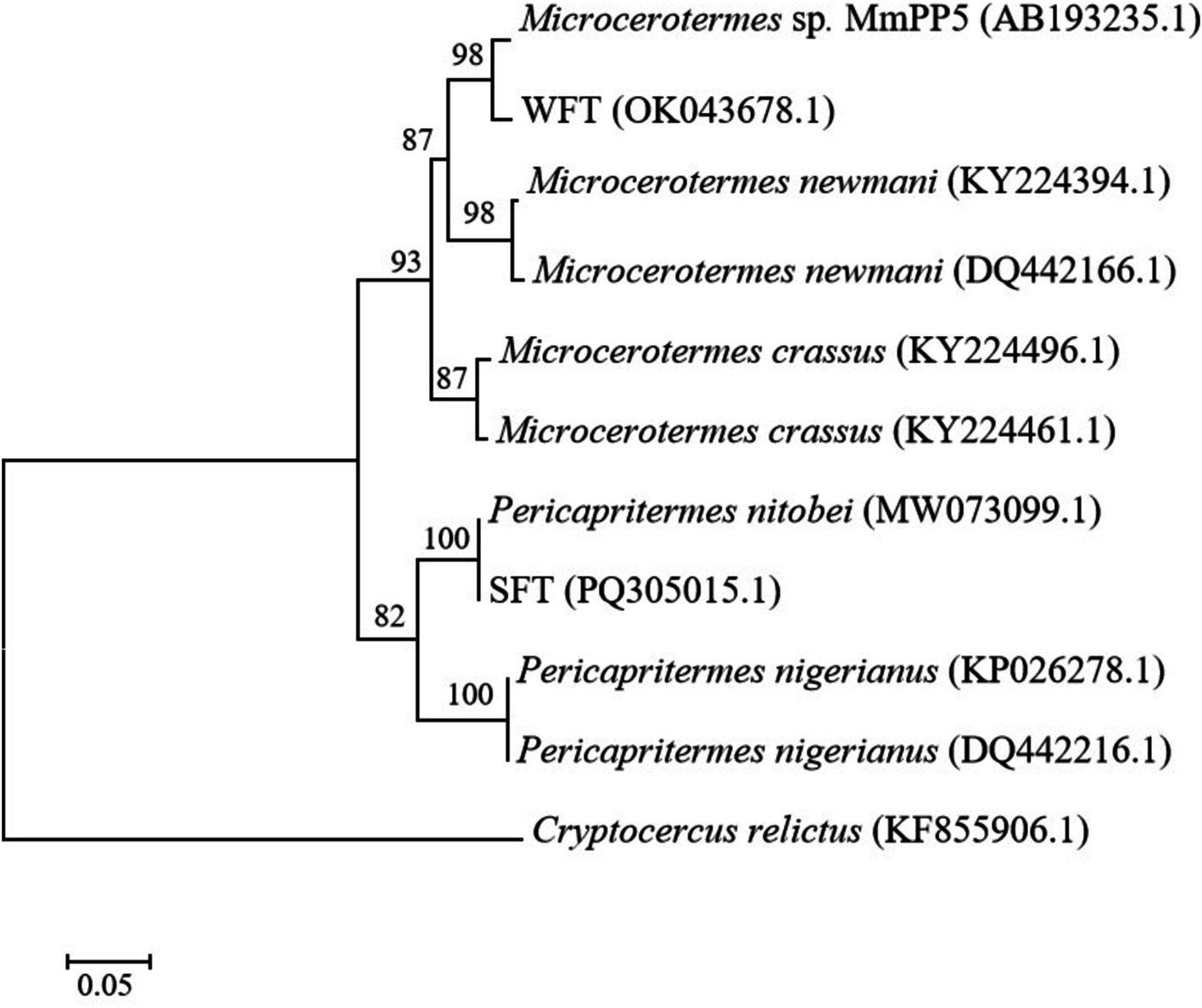
Figure 1. A maximum-likelihood (ML) tree based on the cytochrome oxidase II gene sequences of wood-feeding termites (WFT) and soil-feeding termites (SFT) utilized for this study. Cryptocercus relictus was used as an outgroup. The scale bar indicates 0.05 substitutions per nucleotide position. Bootstrap analysis with 1,000 replicates was performed to determine the statistical significance of the branching order. Values at nodes represent bootstrap support values.
Taxonomic composition of gut bacterial community
A total of 760,285 pair-end reads were obtained from the metagenomic libraries of Microcerotermes sp. and P. nitobei gut samples by sequencing the V3 and V4 regions of the bacterial communities. The high-quality cleaned reads were further binned and filtered into 547,686 effective tags after removing of chimera, which yielded about 77,438–105,870 effective tags per gut sample. Then, the read number in each sample was normalized to the minimum. A total of 2075 OTUs (at 97% sequence similarity) were identified. Reads were assigned to the phyla and genus taxa using the framework of DictDb. The adequacy of the sampling effort for the bacterial diversity in Microcerotermes sp. and P. nitobei was assessed by rarefaction analysis. The outcome of this analysis indicated that the rarefaction curves for each termite gut sample reached a plateau, signifying that the sample size was sufficient to accurately reflect the bacterial diversity community (Figure 2).
Following the taxonomic annotation of the resulting bacterial OTUs, 26 bacterial phyla were identified. Among these, 18 phyla were commonly shared between both termites, with relatively higher variations in their relative abundance. The gut system of Microcerotermes sp. was dominated by Spirochaetes (Figure 3A), accounting for 55%, followed by Fibrobacteres (10%). Firmicutes and Bacteroidetes were also found in moderate abundance, each representing 8%, followed by Candidate TG3 (4.8%), Proteobacteria (4.1%), and Actinobacteria (3.5%). At the genus distribution, Treponema_1c was the most prevalent and accounted for 28% (Figure 3B). The second most abundant genus was Treponema_1f (11%), followed by Treponema_1a (10.3%), sub-cluster 1a (9.8%), and sub-cluster 1b (4.8%).
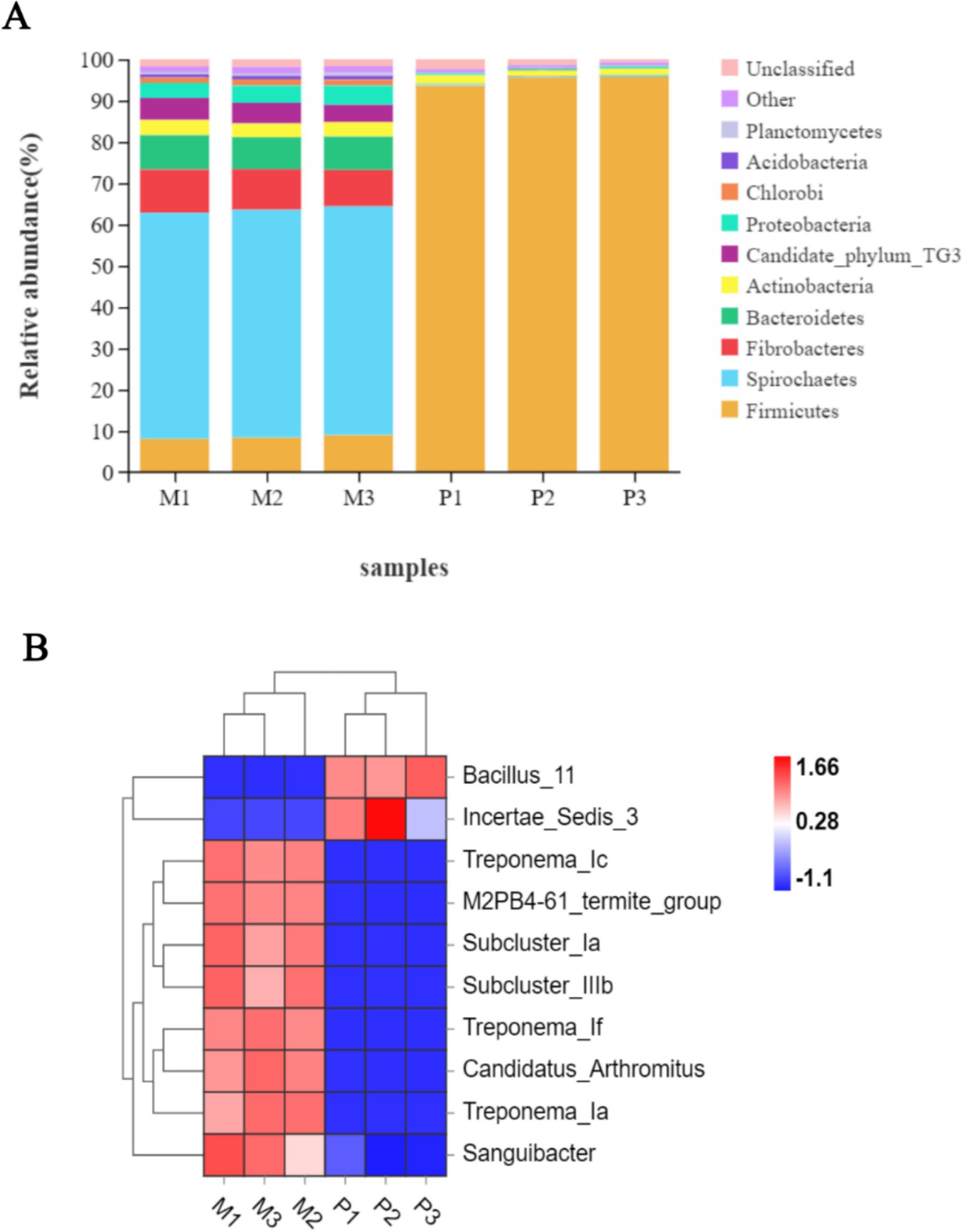
Figure 3. (A) Relative abundance of top 10 bacterial phyla and (B) heat map representation of top 10 genera in the gut of Microcerotermes sp. (M1, M2, M3) and P. nitobei (P1, P2, and P3).
In corroboration to the findings in this study, other researchers have reported similar patterns of bacterial community structure in the gut of several WFT, including Nasutitermes species, Globitermes brachycerastes, Microcerotermes strunckii, Mironasutitermes shangchengensis, and Microcerotermes species (Warnecke et al., 2007; Liu et al., 2019; Makonde et al., 2015). Spirochetes are represented across several termites and have been observed to perform essential metabolic activities essential for termites (Manjula et al., 2016). In addition, Spirochaetes were found in the guts of SFT Nasutitermes corniger and WFT Nasutitermes takasagoensis as part of the wood fiber-associated cellulolytic bacterial community (Mikaelyan et al., 2014). Consequently, the prevalence of Spirochaetes populated by clusters of Treponema in the WFT, Microcerotermes sp., may result from the carbohydrate-rich diet. Besides, recent metagenomic and metatranscriptomics analysis of gut bacterial symbionts has identified Spirochaetes of the Treponema genus, Fibrobacters, and Firmicutes to encode several putative CAZymes (including cellulases and hemicellulases) for lignocellulose degradation (Marynowska et al., 2020; Liu et al., 2019). On the other hand, the phyla Firmicutes (95%) (Figure 3A) populated the gut bacterial community in the SFT, followed by Actinobacteria (2%). Proteobacteria and Candidate TG 3 accounted for 0.43% each, followed by Planctomycetes (0.19%), Chloroflex (0.13%), and Acidobacteria (0.11%). The genus representatives in P. nitobei were dominated by Bacillus_11 (73%) (Figure 3B). Incertae _sedis_3 (15%) accounted for the second most populous genus, followed by Sporosarcina_5 (1.23%) and Gut_cluster_13 (0.58%).
The abundance of Firmicutes in P. nitobei is consistent with preliminary literature on SFT such as Cubitermes niokoloensis, Labiotermes labralis, and Syntermes sp., Cubitermes ugandensis, and Termes riograndensis (Marynowska et al., 2020; Liu et al., 2019; Fall et al., 2007). The presence of the Firmicutes phyla, namely the Bacillus species, in the digestive system of termites has been demonstrated to play a role in breaking down cellulose, obtaining nitrogen, and producing acetate (Manjula et al., 2016). This is due to their mutually beneficial interaction with the termites as endosymbionts. It has also been observed that several Bacillus species identified from insects and other environments could efficiently degrade lignin and other recalcitrant aromatic compounds (Mei et al., 2020); thus, they are considered to contribute to the degradation of lignin and recalcitrant compounds in termites. Additionally, metagenomic sequencing revealed that Firmicutes partake in the secretion of cellulases, hemicellulases, and peptidases in termites (Marynowska et al., 2020). The abundance of Firmicutes phyla in SFT may be influenced by the rich content of organic matter present in the soil diet as well as the high alkalinity of the gut system. Likewise, Mikaelyan et al. (2017) identified Firmicutes as the most prevalent phyla in the most alkaline compartment of several termites, such as Termes hospes, Cornitermes sp., Microcerotermes parvus, and Neocapritermes taracua. Several other phyla, including Proteobacteria, Actinobacteria, Planctomycetes, and Chlorobi, are also implicated in processing lignocellulose components in termites (Diouf et al., 2015). Moreover, Actinobacteria are noted to produce secondary metabolites that offer protection to the termites (Kurtböke et al., 2015).
A potential difference in the composition of the bacterial community related to diet has also been observed in WFT, including Microcerotermes strunckii and Nasutitermes corniger, where Spirochaetes dominated and SFT like Termes riograndensis populated by Firmicutes (Vikram et al., 2021). Similarly, He et al. (2013) identified Spirochaetes and Fibrobacters to dominate in the hindgut of WFT, Nasutitermes corniger, whereas Firmicutes were prevalent in the dung-feeding termite, Amitermes wheeleri. Although these studies depict diet as a primary determinant of gut composition in termites, certain studies have also related the difference in gut bacterial composition to the co-evolution of the termite and the host environment (Abdul Rahman et al., 2015). The variations in bacterial community structure observed in the present study could be associated with the shift in diet during the evolutionary process, such that dietary influences on gut microbiota were prominent by colonizing specific bacterial lineages to adjust to the new diet. Another co-factor is the difference in physiological conditions prevailing in their gut system. Previous studies have established that the gut system of SFT is morphologically elongated and possesses an elevated pH than wood-feeders; thus, it may be dominated by certain bacterial phylotypes like Firmicutes that can survive in the gut environment (Mikaelyan et al., 2017). The findings from this study corroborate with existing literature and implicate that microbial community structures differ in different feeding groups of termites.
Diversity of gut bacterial composition between Microcerotermes sp. and P. nitobei
The bacterial diversity and richness were analyzed employing alpha diversity based on observed OTUs, Shannon, Chao1, and PD tree indices. The observed OTUs (p = 0.001), Shannon 1 (p ≤ 0.001), Chao1 (p = 0.001), and PD tree (p = 0.001) indices of Microcerotermes sp. were all significantly higher than those of P. nitobei (Figure 4), implicating that WFT, Microcerotermes sp., had the highest community diversity and community richness in contrast to SFT, P. nitobei. For P. nitobei, two OTUs (OTU000001 and OTU000002) assigned to Bacillaceae and Planococcaceae were the most abundant members, accounting for more than 85% of all sequence reads in each sample, while the same was represented by 133 OTUs for Microcerotermes sp. The lower bacterial diversity and richness detected in the SFT may result from the preservation of a more specialized microbiota required for the effective digestion of soil organic matter and other aromatic subunits for the survival of the host, as well as the high alkalinity of the gut system. However, results from this study contradict previous studies, which reported higher microbial diversity and richness in the SFT contrary to the WFT and related it to the diverse range of carbon and nitrogen sources available in soil feeders’ diets compared to the limited carbohydrate-rich diet for the wood feeders (Vikram et al., 2021). Nonetheless, the highest diversity observed in the WFT compared to the SFT in this study may be influenced by host phylogeny and host habitat.
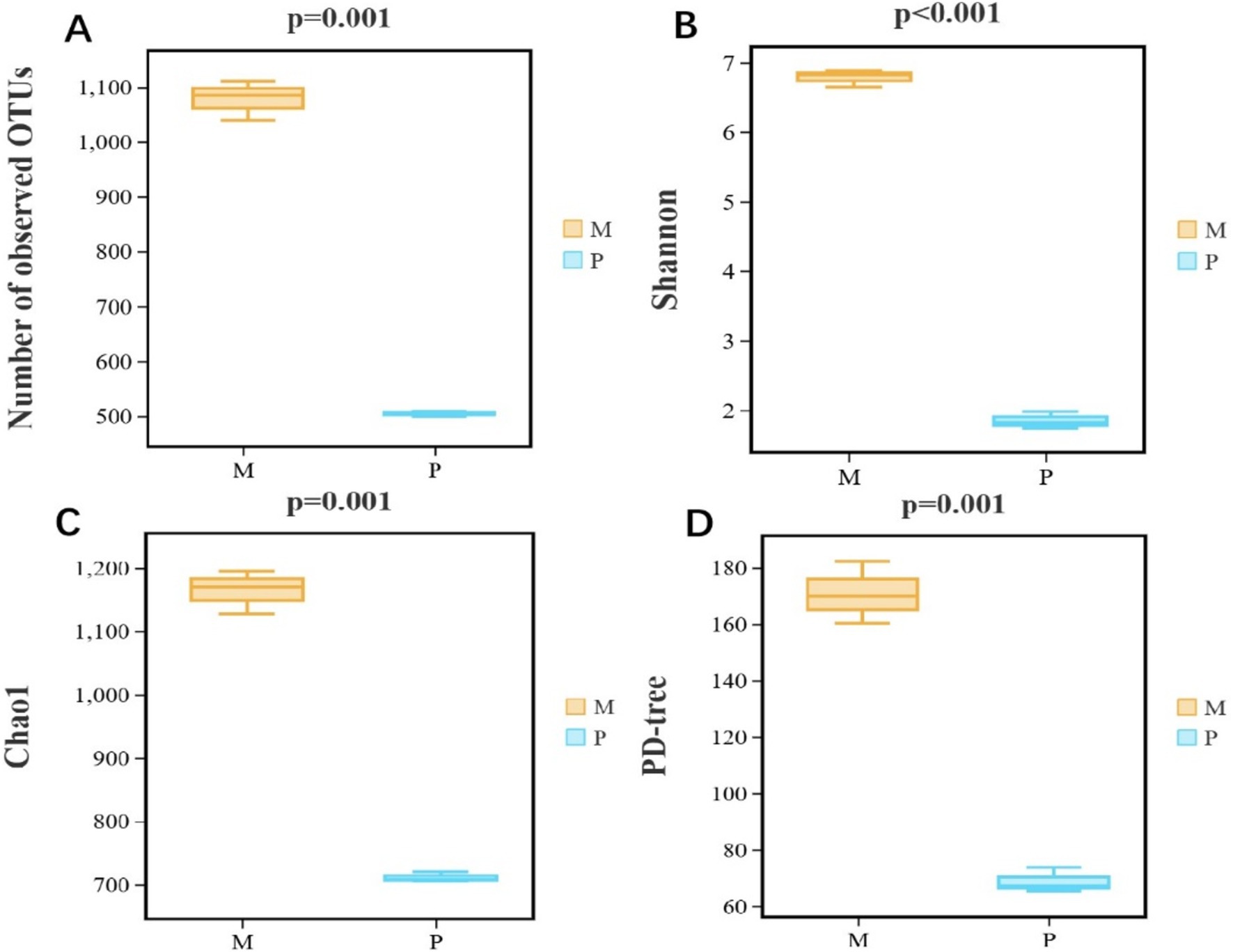
Figure 4. Bacterial α-diversity measures. (A) Number of observed OTUs, (B) Shannon, (C) Chao1, and (D) PD-tree of Microcerotermes sp. (M) and P. nitobei (P) termite species.
The distance between the bacterial community structure of the WFT and SFT species was assessed using the beta diversity analysis based on the weighted UniFrac and Bray-Curtis distances. The UniFrac distances of the triplicate samples of gut bacterial composition in SFT were found to be similar but were significantly distance from the bacterial community structure in the WFT (Figure 5A). Furthermore, the Bray-Curtis distance analysis revealed that the triplicate gut samples from WFT shared high similarity in bacterial composition structure and clustered significantly away from the community in WFT (Figure 5B). A similar trend was also shown by the Bray-Curtis (R2 = 0.96, p < 0.01) and weighted UniFrac distance (R2 = 0.98, p < 0.01) based on the Adonis (PERMANOVA) analysis. Both analyses demonstrate the significant variation of β diversity between the WFT and SFT. Significant differences between the gut bacterial community structure in Microcerotermes sp. and Pericapritermes nitobei have been exhibited. This may be attributed to the evolutionary transition, which caused a change in the composition of gut symbiont to assist the host termite in adapting to their new habitat and feed type (Rossmassler et al., 2024).
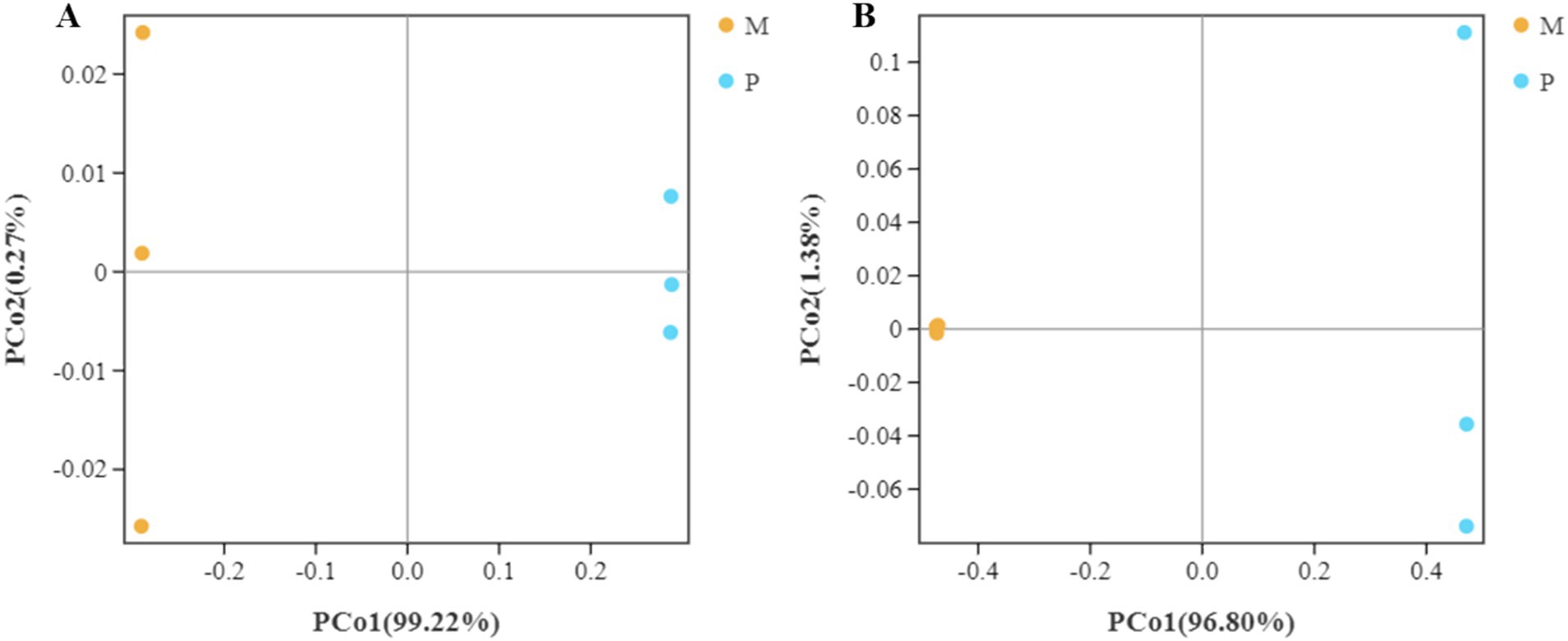
Figure 5. Bacterial β diversity. Principal coordinate analysis (PCoA) of pairwise distances among bacterial communities based on (A) weighted Unifrac and (B) Bray-Curtis distances. The yellow color represents replicate samples from the wood feeding termite, Microcerotermes sp. (M), and the color blue reflects repeated samples from soil feeding termite, P. nitobei (P).
Predicted functional profile of the bacterial communities in the gut of Microcerotermes sp. and P. nitobei
To predict a potential functional composition of the bacterial symbionts in termite guts, a Tax4Fun program was applied to each bacterial sample. The Tax4Fun analysis has been shown to provide a good correlation of predicted functional profiles with metagenomic profiles derived from direct sequencing (Aßhauer et al., 2015). So far, functional profiles using Tax4Fun have been predicted from 16S rRNA gene datasets derived from various environments (Li et al., 2024; Pratama et al., 2019).
As depicted in Figure 6, the dominant metabolic pathways of gut bacterial symbionts in Microcerotermes sp. and P. nitobei were indeed characterized as carbohydrate, amino acid, and energy metabolisms, which suggested that the gut bacterial symbionts might play a vital role in host nutrition and energy supply (Shapira, 2016). A PCoA analysis was performed in terms of the obtained KEGG functional profiles, where the samples from Microcerotermes sp. formed an obvious and independent cluster separated from that of P. nitobei (Supplementary Figure S1). The taxonomic differentiation and the accordingly associated functional differentiation of the symbiotic bacterial community of WFT and SFT presented here suggest a possible type of ecological adaptation mechanism from termites. Statistical analysis employing the Welch’s t-test was carried out to determine the significant (p ≤ 0.05) abundance of metabolic pathways between Microcerotermes sp. gut bacterial symbionts and that of Pericapritermes nitobei. Of all the metabolic functions detected, those associated with carbohydrate metabolism were the most abundant in both Microcerotermes sp. and Pericapritermes nitobei gut bacteria symbionts (Figure 6), reflecting their functional importance for the degradation of their lignocellulose diet. Further statistical analysis indicated that the carbohydrate metabolism pathway was significantly (p ≤ 0.05) enriched in the SFT, P. nitobei gut bacteria symbionts, compared to the WFT, Microcerotermes sp. (Supplementary Figure S2). This outcome is contrary to previous findings, which reported gut bacterial symbionts of several WFT to be enriched in carbohydrate metabolism and encode abundant carbohydrate-active enzymes (CAZymes) than SFT owing to the rich carbohydrate diet of WFT (Arora et al., 2022). The abundance of carbohydrate metabolism pathway from the gut symbionts of P. nitobei, contrary to Microcerotermes sp., is quite interesting and may result from the abundance of non-cellulosic polysaccharides such as chitin in the soil diet. This is further supported by the observation of Marynowska et al. (2020), who reported the high diversity of carbohydrate-active enzymes in the SFT, Labiotermes labralis. In this study, the more abundant carbohydrate metabolic pathway in P. nitobei may be related to the dominant Bacillus (average abundant 73%) in this termite species, which has been demonstrated to play an important role in breaking down carbohydrate (Zhao et al., 2024; Malik and Javed, 2021; Dar et al., 2021; Bisht et al., 2020). However, the significant (p ≤ 0.05) abundance of glycan biosynthesis and metabolism pathways detected in WFT is consistent with previous findings. Generally, wood has a higher content of stored polysaccharides than soil. Therefore, the enrichment of glycan biosynthesis and metabolism pathway from the gut bacteria community in Microcerotermes sp. than P. nitobei is consistent with their diet. In addition, the enrichment of cell motility in Microcerotermes sp. may be related to the dominant of Treponema Ic and If, which can move to wood fibers for cellulose hydrolysis (Mikaelyan et al., 2014).
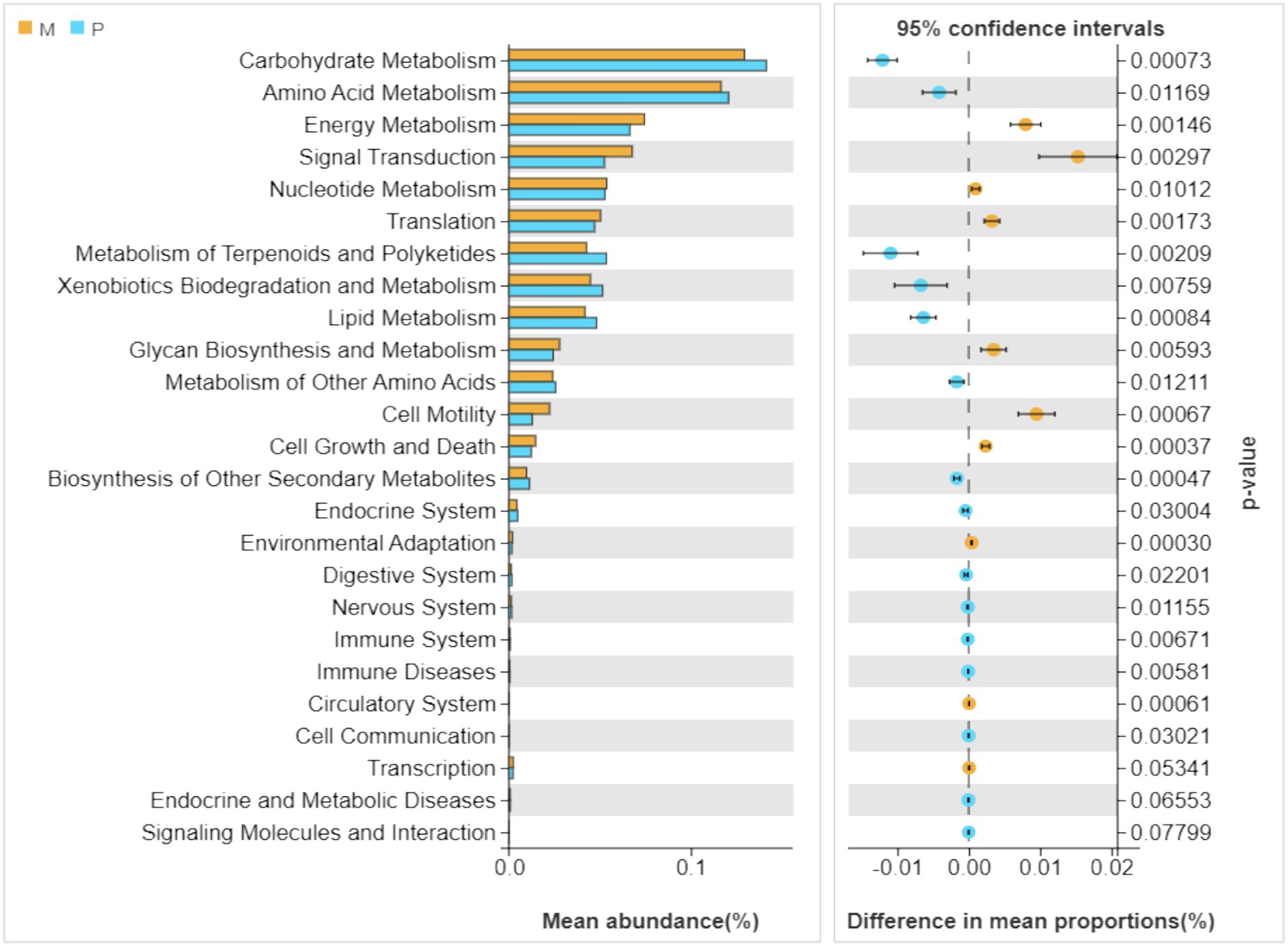
Figure 6. Relative abundances (top 20) of the putative metabolic pathways in the gut bacteria of Microcerotermes sp. (M) and Pericapritermes nitobei (P). The Tax4Fun program was used to predict the functional capabilities of the microbial communities based on 16S rRNA gene amplicon sequencing data. The significantly varied metabolic pathways between wood feeding and soil-feeding termites are indicated by p ≤ 0.05. Values are presented as the mean (n = 3).
In contrast to wood, soil contains a variety of recalcitrant and nitrogenous compounds originating from the humic components (peptides, aromatic subunits, amino acids, microbial excretions, and plant debris). Thus, several KEGG pathways, including amino acid metabolism, metabolism of terpenoids and polyketides, xenobiotic degradation and metabolism, lipid metabolism, and metabolism of other secondary metabolites, were significantly (p ≤ 0.05) enriched in the P. nitobei gut bacterial community. Similarly, the SFT Cubitermes orthognathus has been observed to metabolize peptidoglycan and other microbial excretion components at a higher rate than cellulose (Ji and Brune, 2001). In addition, recent findings by Rossmassler et al. identified gut symbionts of the P3 compartment of Cubitermes ugandensis, SFT to encode more peptidase genes than those in Nasutitermes corniger and Microcerotermes parvus WFT (Rossmassler et al., 2024). Though bacteria composition and community structure significantly differed between the WFT and SFT in this study, the symbiotic gut bacteria communities demonstrated a similar functional structure toward the degradation of lignocellulose and recalcitrant compounds.
Conclusion
This study employed metagenomic analysis to investigate the bacterial communities and functional profiles of understudied termite species, Microcerotermes sp. and P. nitobei. In the gut of Microcerotermes sp., the bacteria phylum Spirochetes (mainly the genus Treponema clusters) were the most common. In the gut of P. nitobei, on the other hand, Firmicutes (mainly the Bacillus genus) were the most common. Further analysis revealed that the diversity of bacteria was higher in the WFT than in the SFT. Furthermore, the β-diversity analysis revealed significant differences between the gut bacterial community structures of Microcerotermes sp. and P. nitobei. Furthermore, the functional metabolic pathways of gut bacteria from both termites were assessed. The bacterial communities were enriched in carbohydrate metabolism independent of feeding behavior. Ultimately, the outcome of this study depicts that the individual termite gut system contains unique bacterial flora yet exhibits a functional congruence toward lignocellulose degradation and other recalcitrant compounds. Although the metagenomic study provided an overview of the bacterial community structure and potential metabolic pathways associated with lignocellulosic biomass degradation, such an approach does not allow for estimating microbial physiology or ecology. Furthermore, cultivable microbes are suitable for studying symbiotic relationships within the host and exploring their potential applications. Thus, enrichment techniques are under investigation to ascertain the composition of culturable lignocellulolytic gut bacteria symbionts in Microcerotermes sp. and P. nitobei.
Data availability statement
The datasets presented in this study can be found in online repositories. The names of the repository/repositories and accession number(s) can be found in the article/Supplementary material.
Author contributions
RX: Writing – review & editing, Conceptualization, Methodology, Writing – original draft. BD: Methodology, Writing – original draft. JS: Conceptualization, Validation, Visualization, Writing – review & editing. RA-T: Writing – review & editing, Formal analysis. MK: Visualization, Writing – review & editing, Investigation. MS: Investigation, Writing – review & editing. SA: Conceptualization, Data curation, Formal analysis, Software, Writing – review & editing.
Funding
The author(s) declare that financial support was received for the research, authorship, and/or publication of this article. This work was supported by the National Key Research and Development Program of China (2023YFC3403600), the Grant-in-aid from the National Natural Science Foundation of China (31900367, 32250410285). The authors extended their appreciation to Taif University, Saudi Arabia, for supporting this work through project number (TU-DSPP-2024–267). This work was also supported in part by the Grant-in-Aid Startup Foundation (10JDG127) of Jiangsu University, China.
Conflict of interest
The authors declare that the research was conducted in the absence of any commercial or financial relationships that could be construed as a potential conflict of interest.
The author(s) declared that they were an editorial board member of Frontiers, at the time of submission. This had no impact on the peer review process and the final decision.
Publisher’s note
All claims expressed in this article are solely those of the authors and do not necessarily represent those of their affiliated organizations, or those of the publisher, the editors and the reviewers. Any product that may be evaluated in this article, or claim that may be made by its manufacturer, is not guaranteed or endorsed by the publisher.
Supplementary material
The Supplementary material for this article can be found online at: https://www.frontiersin.org/articles/10.3389/fmicb.2024.1424982/full#supplementary-material
Footnotes
References
Abdul Rahman, N., Parks, D. H., Willner, D. L., Engelbrektson, A. L., Goffredi, S. K., Warnecke, F., et al. (2015). A molecular survey of Australian and north American termite genera indicates that vertical inheritance is the primary force shaping termite gut microbiomes. Microbiome 3, 1–16. doi: 10.1186/s40168-015-0067-8
Ali, S. S., Al-Tohamy, R., Sun, J., Wu, J., and Huizi, L. (2019). Screening and construction of a novel microbial consortium SSA-6 enriched from the gut symbionts of wood-feeding termite, Coptotermes formosanus and its biomass-based biorefineries. Fuel 236, 1128–1145. doi: 10.1016/j.fuel.2018.08.117
Ali, S. S., Elsamahy, T., El-Sapagh, S., Khalil, M. A., Al-Tohamy, R., Zhu, D., et al. (2024). Exploring the potential of insect gut symbionts for polyethylene biodegradation. Process Saf. Environ. Prot. 190, 22–33. doi: 10.1016/j.psep.2024.08.008
Ali, S. S., Elsamahy, T., Zhu, D., and Sun, J. (2023). Biodegradability of polyethylene by efficient bacteria from the guts of plastic-eating waxworms and investigation of its degradation mechanism. J. Hazard. Mater. 443:130287. doi: 10.1016/j.jhazmat.2022.130287
Ali, S. S., Mustafa, A. M., and Sun, J. (2021). Wood-feeding termites as an obscure yet promising source of bacteria for biodegradation and detoxification of creosote-treated wood along with methane production enhancement. Bioresour. Technol. 338:125521. doi: 10.1016/j.biortech.2021.125521
Ali, S. S., Wu, J., Xie, R., Zhou, F., Sun, J., and Huang, M. (2017). Screening and characterizing of xylanolytic and xylose-fermenting yeasts isolated from the wood-feeding termite, Reticulitermes chinensis. PLoS One 12:e0181141. doi: 10.1371/journal.pone.0181141
Al-Tohamy, R., Sun, J., Khalil, M. A., Kornaros, M., and Ali, S. S. (2021). Wood-feeding termite gut symbionts as an obscure yet promising source of novel manganese peroxidase-producing oleaginous yeasts intended for azo dye decolorization and biodiesel production. Biotechnol. Biofuels 14, 1–27. doi: 10.1186/s13068-021-02080-z
Arora, J., Kinjo, Y., Šobotník, J., Buček, A., Clitheroe, C., Stiblik, P., et al. (2022). The functional evolution of termite gut microbiota. Microbiome 10:78. doi: 10.1186/s40168-022-01258-3
Aßhauer, K. P., Wemheuer, B., Daniel, R., and Meinicke, P. (2015). Tax4Fun: predicting functional profiles from metagenomic 16S rRNA data. Bioinformatics 31, 2882–2884. doi: 10.1093/bioinformatics/btv287
Bisht, N., Mishra, S. K., and Chauhan, P. S. (2020). Bacillus amyloliquefaciens inoculation alters physiology of rice (Oryza sativa L. var. IR-36) through modulating carbohydrate metabolism to mitigate stress induced by nutrient starvation. Int. J. Biol. Macromol. 143, 937–951. doi: 10.1016/j.ijbiomac.2019.09.154
Brune, A. (2014). Symbiotic digestion of lignocellulose in termite guts. Nat. Rev. Microbiol. 12, 168–180. doi: 10.1038/nrmicro3182
Caporaso, J. G., Kuczynski, J., Stombaugh, J., Bittinger, K., Bushman, F. D., Costello, E. K., et al. (2010). QIIME allows analysis of high-throughput community sequencing data. Nat. Methods 7, 335–336. doi: 10.1038/nmeth.f.303
Chen, S., Zhou, Y., Chen, Y., and Gu, J. (2018). Fastp: an ultra-fast all-in-one FASTQ preprocessor. Bioinformatics 34, i884–i890. doi: 10.1093/bioinformatics/bty560
Chouvenc, T., Šobotník, J., Engel, M. S., and Bourguignon, T. (2021). Termite evolution: mutualistic associations, key innovations, and the rise of Termitidae. Cell. Mol. Life Sci. 78, 2749–2769. doi: 10.1007/s00018-020-03728-z
Danso, B., Ali, S. S., Xie, R., and Sun, J. (2022). Valorisation of wheat straw and bioethanol production by a novel xylanase-and cellulase-producing Streptomyces strain isolated from the wood-feeding termite. Microcerotermes species. Fuel 310:122333. doi: 10.1016/j.fuel.2021.122333
Dar, M. A., Dhole, N. P., Xie, R., Pawar, K. D., Ullah, K., Rahi, P., et al. (2021). Valorization potential of a novel bacterial strain, Bacillus altitudinis RSP75, towards lignocellulose bioconversion: an assessment of symbiotic bacteria from the stored grain pest, Tribolium castaneum. Microorganisms 9:1952. doi: 10.3390/microorganisms9091952
Diouf, M., Roy, V., Mora, P., Frechault, S., Lefebvre, T., Herve, V., et al. (2015). Profiling the succession of bacterial communities throughout the life stages of a higher termite Nasutitermes arborum (Termitidae, Nasutitermitinae) using 16S rRNA gene pyrosequencing. PLoS One 10:e0140014. doi: 10.1371/journal.pone.0140014
Edgar, R. C. (2004). MUSCLE: multiple sequence alignment with high accuracy and high throughput. Nucleic Acids Res. 32, 1792–1797. doi: 10.1093/nar/gkh340
Edgar, R. C. (2013). UPARSE: highly accurate OTU sequences from microbial amplicon reads. Nat. Methods 10, 996–998. doi: 10.1038/nmeth.2604
Edgar, R. C., Haas, B. J., Clemente, J. C., Quince, C., and Knight, R. (2011). UCHIME improves sensitivity and speed of chimera detection. Bioinformatics 27, 2194–2200. doi: 10.1093/bioinformatics/btr381
Fall, S., Hamelin, J., Ndiaye, F., Assigbetse, K., Aragno, M., Chotte, J. L., et al. (2007). Differences between bacterial communities in the gut of a soil-feeding termite (Cubitermes niokoloensis) and its mounds. Appl. Environ. Microbiol. 73, 5199–5208. doi: 10.1128/AEM.02616-06
He, S., Ivanova, N., Kirton, E., Allgaier, M., Bergin, C., Scheffrahn, R. H., et al. (2013). Comparative metagenomic and metatranscriptomic analysis of hindgut paunch microbiota in wood-and dung-feeding higher termites. PLoS One 8:e61126. doi: 10.1371/journal.pone.0061126
Ji, R., and Brune, A. (2001). Transformation and mineralization of 14C-labeled cellulose, peptidoglycan, and protein by the soil-feeding termite Cubitermes orthognathus. Biol. Fertil. Soils 33, 166–174. doi: 10.1007/s003740000310
Kolde, R., and Vilo, J. (2015). GOsummaries: an R package for visual functional annotation of experimental data. F1000Res 4:574. doi: 10.12688/f1000research.6925.1
Kurtböke, D. İ., French, J. R., Hayes, R. A., and Quinn, R. J. (2015). Eco-taxonomic insights into actinomycete symbionts of termites for discovery of novel bioactive compounds. Biotechnol. Appl. Biodiv. 147, 111–135. doi: 10.1007/10_2014_270
Li, H., and Greening, C. (2022). Termite-engineered microbial communities of termite nest structures: a new dimension to the extended phenotype. FEMS Microbiol. Rev. 46:fuac034. doi: 10.1093/femsre/fuac034
Li, L., Li, W., Xue, Y., Wang, Z., Wang, Y., Li, R., et al. (2024). Perfluorooctanoic acid effect and microbial mechanism to methane production in anaerobic digestion. J. Environ. Manag. 369:122412. doi: 10.1016/j.jenvman.2024.122412
Liu, N., Li, H., Chevrette, M. G., Zhang, L., Cao, L., Zhou, H., et al. (2019). Functional metagenomics reveals abundant polysaccharide-degrading gene clusters and cellobiose utilization pathways within gut microbiota of a wood-feeding higher termite. ISME J. 13, 104–117. doi: 10.1038/s41396-018-0255-1
Lozupone, C., and Knight, R. (2005). UniFrac: a new phylogenetic method for comparing microbial communities. Appl. Environ. Microbiol. 71, 8228–8235. doi: 10.1128/AEM.71.12.8228-8235.2005
Magoč, T., and Salzberg, S. L. (2011). FLASH: fast length adjustment of short reads to improve genome assemblies. Bioinformatics 27, 2957–2963. doi: 10.1093/bioinformatics/btr507
Makonde, H. M., Mwirichia, R., Osiemo, Z., Boga, H. I., and Klenk, H. P. (2015). 454 pyrosequencing-based assessment of bacterial diversity and community structure in termite guts, mounds and surrounding soils. Springerplus 4, 1–11. doi: 10.1186/s40064-015-1262-6
Malik, W. A., and Javed, S. (2021). Biochemical characterization of cellulase from Bacillus subtilis strain and its effect on digestibility and structural modifications of lignocellulose rich biomass. Front. Bioeng. Biotechnol. 9:800265. doi: 10.3389/fbioe.2021.800265
Manjula, A., Pushpanathan, M., Sathyavathi, S., Gunasekaran, P., and Rajendhran, J. (2016). Comparative analysis of microbial diversity in termite gut and termite nest using ion sequencing. Curr. Microbiol. 72, 267–275. doi: 10.1007/s00284-015-0947-y
Mao, G. H., Zhang, Z. H., Fei, F., Ding, Y. Y., Zhang, W. J., Chen, H., et al. (2019). Effect of Grifola frondosa polysaccharide on anti-tumor activity in combination with 5-Fu in Heps-bearing mice. Int. J. Biol. Macromol. 121, 930–935. doi: 10.1016/j.ijbiomac.2018.10.073
Marynowska, M., Goux, X., Sillam-Dussès, D., Rouland-Lefèvre, C., Halder, R., Wilmes, P., et al. (2020). Compositional and functional characterisation of biomass-degrading microbial communities in guts of plant fibre-and soil-feeding higher termites. Microbiome 8, 1–18. doi: 10.1186/s40168-020-00872-3
Marynowska, M., Sillam-Dussès, D., Untereiner, B., Klimek, D., Goux, X., Gawron, P., et al. (2023). A holobiont approach towards polysaccharide degradation by the highly compartmentalised gut system of the soil-feeding higher termite Labiotermes labralis. BMC Genomics 24:115. doi: 10.1186/s12864-023-09224-5
Mei, J., Shen, X., Gang, L., Xu, H., Wu, F., and Sheng, L. (2020). A novel lignin degradation bacteria-Bacillus amyloliquefaciens SL-7 used to degrade straw lignin efficiently. Bioresour. Technol. 310:123445. doi: 10.1016/j.biortech.2020.123445
Mikaelyan, A., Meuser, K., and Brune, A. (2017). Microenvironmental heterogeneity of gut compartments drives bacterial community structure in wood-and humus-feeding higher termites. FEMS Microbiol. Ecol. 93:fiw210. doi: 10.1093/femsec/fiw210
Mikaelyan, A., Strassert, J. F., Tokuda, G., and Brune, A. (2014). The fibre-associated cellulolytic bacterial community in the hindgut of wood-feeding higher termites (Nasutitermes spp.). Environ. Microbiol. 16, 2711–2722. doi: 10.1111/1462-2920.12425
Nawaz, M. Z., Shang, H., Sun, J., Geng, A., Ali, S. S., and Zhu, D. (2023). Genomic insights into the metabolic potential of a novel lignin-degrading and polyhydroxyalkanoates producing bacterium Pseudomonas sp. Hu109A. Chemosphere 310:136754. doi: 10.1016/j.chemosphere.2022.136754
Oksanen, J. (2010). vegan: community ecology package. Available at: http://vegan.r-forge.r-project.org/ (Accessed July 2, 2023).
Ondov, B. D., Bergman, N. H., and Phillippy, A. M. (2011). Interactive metagenomic visualization in a web browser. BMC Bioinform. 12, 1–10. doi: 10.1186/1471-2105-12-385
Pratama, R., Schneider, D., Böer, T., and Daniel, R. (2019). First insights into bacterial gastrointestinal tract communities of the Eurasian beaver (Castor fiber). Front. Microbiol. 10:1646. doi: 10.3389/fmicb.2019.01646
Price, M. N., Dehal, P. S., and Arkin, A. P. (2010). FastTree 2–approximately maximum-likelihood trees for large alignments. PLoS One 5:e9490. doi: 10.1371/journal.pone.0009490
Rossmassler, K., Dietrich, C., Thompson, C. L., Mikaelyan, A., and Brune, A. (2024). Comparative metagenomic analyses reveal bacterial functional profiles in the hindgut compartments of higher termites. Ecol. Evol. Driv. Microb. Commun. Struct. Termi. Gut 139, 139–161.
Santhoshkumar, S., Gomathi, V., Meenakshisundaram, P., and Mary, J. K. (2024). Comparative insights of soil properties of termite hill in relation to the microbial community using culture-independent approach. Total Environ. Adv. 9:200094. doi: 10.1016/j.teadva.2023.200094
Schloss, P. D., Westcott, S. L., Ryabin, T., Hall, J. R., Hartmann, M., Hollister, E. B., et al. (2009). Introducing mothur: open-source, platform-independent, community-supported software for describing and comparing microbial communities. Appl. Environ. Microbiol. 75, 7537–7541. doi: 10.1128/AEM.01541-09
Shapira, M. (2016). Gut microbiotas and host evolution: scaling up symbiosis. Trends Ecol. Evol. 31, 539–549. doi: 10.1016/j.tree.2016.03.006
Vikram, S., Arneodo, J. D., Calcagno, J., Ortiz, M., Mon, M. L., Etcheverry, C., et al. (2021). Diversity structure of the microbial communities in the guts of four neotropical termite species. PeerJ 9:e10959. doi: 10.7717/peerj.10959
Warnecke, F., Luginbühl, P., Ivanova, N., Ghassemian, M., Richardson, T. H., Stege, J. T., et al. (2007). Metagenomic and functional analysis of hindgut microbiota of a wood-feeding higher termite. Nature 450, 560–565. doi: 10.1038/nature06269
Wickham, H., and Chang, W. (2008). ggplot2: an implementation of the grammar of graphics. R package version 0.7. Available at: http://CRAN.R-project.org/package=ggplot2 (Accessed December 6, 2021).
Xie, R., Danso, B., Sun, J., Khalil, M. A., Al-Tohamy, R., and Ali, S. S. (2024). Exploring the composition and function profiles of bacteria from wood-and soil-feeding termites for effective degradation of lignin-based aromatics. Process Saf. Environ. Prot. 191, 1672–1683. doi: 10.1016/j.psep.2024.09.081
Xie, R., Danso, B., Sun, J., Schagerl, M., Al-Tohamy, R., and Ali, S. S. (2024). Harnessing the potential of a novel lignin-degrading Streptomyces sp. MS-S2 from wood-feeding termite for malachite green decolorization and detoxification. Process Saf. Environ. Prot. 186, 189–199. doi: 10.1016/j.psep.2024.04.016
Keywords: metagenomics, recalcitrant pollutants, termite gut symbionts, bacterial community, operational taxonomic unit, metabolic pathways
Citation: Xie R, Danso B, Sun J, Al-Tohamy R, Khalil MA, Schagerl M and Ali SS (2024) Metagenomics analysis of bacterial community structure from wood- and soil-feeding termites: metabolic pathways and functional structures toward the degradation of lignocellulose and recalcitrant compounds. Front. Microbiol. 15:1424982. doi: 10.3389/fmicb.2024.1424982
Edited by:
Liwen He, China Agricultural University, ChinaReviewed by:
Varinia López, Instituto Tecnológico Superior de Irapuato, MexicoBassem Jaouadi, Centre of Biotechnology of Sfax, Tunisia
Cai Wang, South China Agricultural University, China
Copyright © 2024 Xie, Danso, Sun, Al-Tohamy, Khalil, Schagerl and Ali. This is an open-access article distributed under the terms of the Creative Commons Attribution License (CC BY). The use, distribution or reproduction in other forums is permitted, provided the original author(s) and the copyright owner(s) are credited and that the original publication in this journal is cited, in accordance with accepted academic practice. No use, distribution or reproduction is permitted which does not comply with these terms.
*Correspondence: Jianzhong Sun, SnpzdW4xMDAyQHVqcy5lZHUuY24=; Michael Schagerl, bWljaGFlbC5zY2hhZ2VybEB1bml2aWUuYWMuYXQ=; Sameh S. Ali, c2FtaEB1anMuZWR1LmNu;c2FtaF9zYW1pckBzY2llbmNlLnRhbnRhLmVkdS5lZw==