- Xinjiang Key Laboratory of Herbivore Drug Research and Creation, College of Veterinary Medicine, Xinjiang Agricultural University, Urumqi, Xinjiang, China
The present study aimed to investigate the therapeutic effects of berberine (BBR) on Salmonella enteritis in broiler chickens and to elucidate its mechanisms of action preliminarily. Blood samples were collected from 21- to 35-day-old Sanhuang male chicks to measure immune and biochemical indicators and to calculate the organ coefficients for the liver, spleen, bursa of Fabricius, and thymus. The caecal microbiota was analysed through 16S ribosomal RNA (rRNA) gene sequencing, and transcriptome sequencing was conducted. Compared with the positive control group (S), the berberine-treated group (BS) presented increased serum immunoglobulin M (IgM) levels, serum IgG levels, and total antioxidant capacity; berberine ameliorated the increase in the thymus index caused by Salmonella administration. The addition of berberine to the diet increased the abundance of beneficial bacterial genera, including Bacteroides and Lactobacillus. It also decreased the abundance of harmful bacterial genera, including Faecalibacterium and Streptococcus. Transcriptome analysis revealed that gene expression in the S and BS groups was associated with T cell selection and B cell receptor signalling pathways, which are enriched primarily in multiple immune-related signalling pathways, including the B cell receptor signalling pathway, NF-κ B signalling pathway, intestinal immune network for IgA production, asthma, and African trypanosomiasis. The significantly expressed genes included ATAD5, ERP29, MGST2, PIK3CA, and HSP90AA1. The present study demonstrated that berberine has a good therapeutic effect on Salmonella infection in chicks, as it inhibits the occurrence and development of Salmonella-induced intestinal inflammation by regulating the balance of the gut microbiota and the expression of related genes, including ATAD5, ERP29, MGST2, PIK3CA, and HSP90AA1.
1 Introduction
Salmonella—a widely distributed Gram-negative enteric bacterium—has long been a focal point of concern in global public health. Since its first description by the American physician, Daniel Elmer Salmon, in 1885, multiple serotypes within the genus Salmonella have been identified. However, more than 2,500 different serotypes have been identified (Zhang Wen-Cheng et al., 2019) as time progressed. As an important zoonotic pathogen, Salmonella can cause various clinical syndromes in both animals and humans, including enteric fever (typhoid fever), gastroenteritis or diarrhoea, bacteraemia, and chronic asymptomatic carriage, which are collectively referred to as salmonellosis (Bao et al., 2022; Johari et al., 2019). Salmonella infection—typically transmitted through ingesting contaminated food or water—poses a severe threat to human health, particularly for children, elderly individuals, and individuals with compromised immune systems. Studies have indicated that Salmonella causes approximately 90 million cases of gastroenteritis globally each year, resulting in about 155,000 deaths. It is estimated that about 10% of these cases are due to direct contact with animals (Galán-Relaño et al., 2023). Globally, Salmonella is one of the leading causes of foodborne illness (Robinson, 1970). According to data from the Centres for Disease Control and Prevention (CDC), there are approximately 1.35 million cases of human Salmonella infections in the United States each year. Additionally, the European Food Safety Authority (EFSA) and the European Centre for Disease Prevention and Control (ECDC) reported that in 2018, Salmonella was responsible for nearly one-third of all foodborne diseases in Europe (Junwei, 2021). Salmonella not only poses a threat to human health but also has a significant effect on the livestock and poultry industry. Salmonella infections can lead to substantial mortality in chicks, a decrease in egg production, a reduction in the hatchability rate of breeder eggs, and an increase in the rate of weak chicks (Liang et al., 2019). Additionally, Salmonella can contaminate eggs through vertical transmission, affecting hatchability rates and the health of chicks, leading to significant economic losses in the poultry industry (Zhai Wendong, 2011).
Currently, antibiotic therapy remains the primary means of controlling Salmonella infections. However, with the extensive use and misuse of antibiotics, the resistance of Salmonella to multiple antibiotics is continuously increasing. Studies have shown that up to 36 mutational genes have emerged in Salmonella, conferring varying degrees of resistance to 14 types of antibiotics, including cephalosporins, penicillin, and tetracycline (Zhao et al., 2023). In a study conducted in the northern region of Jiangsu, Salmonella strains isolated from parent stock and commercial broilers of white feather meat chickens exhibited resistance to multiple antibiotics (Fu et al., 2020). Traditional antibiotic treatments are inadequate in the face of the increasing problem of antibiotic resistance. Therefore, it is crucial to identify new therapeutic strategies and medications.
Berberine (BBR) is a quaternary protoberberine alkaloid isolated from traditional Chinese medicinal plants, such as Coptis chinensis (Huang Lian). Berberine is widely distributed in the plant kingdom and has been identified in approximately four families and 10 genera (Dan, 2022; Fang, 2020). Berberine exhibits potent pharmacological activities, and its medicinal applications have expanded significantly. Initially used clinically for the treatment of diarrhoea, the scope of the medical use of berberine now extends to include antimicrobial (Zhang and Shen, 2023a; Zhang and Shen, 2023b), anti-inflammatory, antitumour (Cheng et al., 2022; Song et al., 2019), blood sugar and lipid modulation (Yuting Chen et al., 2022), diabetes treatment (Shan et al., 2013; Xiao et al., 2021), and adjuvant therapy for liver diseases (Li et al., 2017). Due to its unique chemical structure and diverse biological activities, BBR can act on multiple signalling pathways and target various therapeutic sites (Feng et al., 2012; Izadparast et al., 2022). Additionally, many studies have indicated that berberine has significant efficacy in treating intestinal damage induced by dextran sulphate sodium (DSS) (Dong et al., 2022) and trinitrobenzene sulphonic acid (TNBS) (Li et al., 2015), as well as in genetically modified animal models and models of intestinal damage induced by lipopolysaccharide (LPS) (Guo et al., 2018).
A study mentioned that the combination of berberine with antibiotics can reduce the formation of biofilms in multidrug-resistant bacterial strains and decrease the multidrug resistance of Salmonella by inhibiting messenger RNA (mRNA) expression (Shi et al., 2018). Furthermore, research by Xiao-die Cui and colleagues found that the combination of berberine and ethylenediaminetetraacetic acid (EDTA) significantly restored the sensitivity of Salmonella and Escherichia coli resistant to colistin (Cui et al., 2024). Our previous research revealed that berberine alleviates the inflammation caused by Salmonella lipopolysaccharide stimulation in IPEC-J2 cells (Yang et al., 2022). These studies provide preliminary evidence of berberine’s potential roles and mechanisms in combating Salmonella infections. However, there is currently a lack of research on treating Salmonella infections with berberine, and there have been no reports on whether berberine can treat avian salmonellosis caused by Salmonella and the potential mechanisms involved. Therefore, the present study utilised poultry infected with Salmonella to investigate the mechanisms of action of berberine treatment for Salmonella infection by examining growth performance, histopathology, biochemical indicators, and the gut microbiota through 16S ribosomal RNA (rRNA) analysis and transcriptomics. The present study aimed to identify critical targets for treating Salmonella infection from transcriptomics results, explore the specific mechanisms of BBR in treating this infection, and provide a new traditional Chinese medicine for the Salmonella disease.
2 Materials and methods
2.1 Preparation of BBR and Salmonella
Berberine (catalogue number: 110713) was purchased from the China Food and Drug Administration (CFDA). Salmonella enteritidis (Salmonella enterica subsp. enterica serovar, Typhimurium strain LT2) was provided by Professor Su Zhanqiang from Xinjiang Agricultural University.
2.2 Animals and management
All the experimental procedures adhered to the regulations set by the Animal Care and Use Committee of the College of Veterinary Medicine at Xinjiang Agricultural University.
A total of 120 1-day-old healthy Sanhuang male chickens with similar body weights were purchased from Tiankang Biological Company, Wuhan, Hubei Province, China. The chickens were randomly divided into four groups, with six replicates in each group, and each replicate consisted of five Sanhuang male chickens. All the chicks were housed in an animal facility with adequate lighting, free access to food and water, and a controlled temperature environment. The rearing temperature was maintained at 35–40°C for the first 3 days, at 32–33°C from day 4 to day 7, at 28–32°C from day 8 to day 21, and at room temperature (25°C) after day 21. The relative humidity of the rearing environment for the chicks throughout the experiment was maintained at 55%. The rearing experiment was conducted at the College of Animal Medicine, Xinjiang Agricultural University. During their growth period, all chicks were vaccinated with an inactivated infectious bursal disease vaccine or an inactivated Newcastle disease vaccine. The basal diet for the chickens was purchased from Tiankang Biological Company, and the diet’s nutritional content is shown in Table 1. The standards met the requirements set by the National Research Council (NRC) and satisfied the daily nutritional needs of Sanhuang male chickens.
The experiment utilised a 2 × 2 factorial design, with the following groups: a control group (C), in which the diet did not contain berberine and no Salmonella was administered orally; a positive control group (S), in which the diet did not contain berberine but Salmonella was administered orally; a berberine group (B), in which the diet was supplemented with berberine without oral administration of Salmonella; and a berberine-treated group (BS), in which the diet was supplemented with berberine and Salmonella was administered orally. The feed of groups B and BS was supplemented with 120 mg/kg berberine (Feng et al., 2021), which was freshly provided. Salmonella was dissolved in sterile physiological saline, and at 9 days of age, groups S and BS were orally administered 1.2 × 109 colony-forming units (CFU) of Salmonella for 3 consecutive days (Lamichhane et al., 2024), whereas groups C and B received an equal volume of physiological saline.
2.3 Sample collection and procedures
The chicks were weighed after an overnight fast at 1 day, 14 days, 20 days, and 34 days of age, and the remaining feed amount for each group was recorded. Based on the body weight and feed quantity obtained, the average daily gain (ADG), average daily feed intake (ADFI), and feed conversion ratio (FCR) for the chickens at 15 days, 21 days, and 35 days of age were calculated. At 21 and 35 days of age, five chicks were randomly selected from each group for necropsy. Blood samples (3–5 mL) were collected from the wing vein of the chicks, and the blood was allowed to stand at room temperature for 30 min before being centrifuged at 6,000 rpm for 15 min in a refrigerated centrifuge. The obtained serum was stored at −20°C for future use. After blood collection, the chicks were weighed, euthanized, and subjected to necropsy.
The liver, spleen, and bursa of Fabricius were collected; to retrieve the thymus glands on both sides, the thoracic cavity was opened. After removing the fascia and adipose tissues, the blood was collected and the organs were weighed. The weights were recorded, and the immune organ index—the ratio of the organ weight to the live body weight—was calculated. Additionally, at 21 days of age, the caecum and its contents were collected from the chicks for examination. A portion of the caecum was washed in ice-cold PBS and fixed in 10% formalin solution for 24 h, after which it was sent to the Wuhan Sevilla: Wuhan Sevilla Company, Wuhan, Hubei Province, China for HE staining and tissue sectioning. The remaining caecal tissue was cleaned with RNase-free water, frozen in liquid nitrogen for 30 min, and stored at −80°C. The caecal contents were also frozen in liquid nitrogen for 30 min and stored at −80°C. From each group, three random caecal samples and three caecal content samples were collected and sent to the Shanghai Majorbio Company, Shanghai, China for transcriptome sequencing and 16S rRNA sequencing of the gut microbiota.
2.4 Determination of Salmonella content in faeces
Faeces were collected from 35-day-old broilers. Salmonella DNA was extracted from the faeces via the TaKaRa MiniBEST Universal Genomic DNA Extraction Kit (version 5.0). Absolute quantification was performed via real-time fluorescent quantitative polymerase chain reaction (PCR) (primers invA-F: ACCAGTCGTCTTATCTT GATTGAAG and invA-R: TCATCGCACCGTCAAAGGAA). The copy number of Salmonella in each group was calculated based on the absolute quantification standard curve obtained from a known concentration standard (invA plasmid).
2.5 Biochemical index analysis
From each group, five serum samples were randomly collected for the detection of immunoglobulins (IgA, IgM, and IgG), antioxidant indicators (alkaline phosphatase activity and total antioxidant capacity), high-density lipoprotein (HDL), low-density lipoprotein (LDL), triglyceride, and total cholesterol contents according to the manufacturer’s instructions. The IgA ELISA Kit (YX-E21412C), IgM ELISA Kit (YX-E21856C), and IgG ELISA Kit (YX-E21430C) were purchased from Shanghai Youxuan Biotechnology Co., Ltd., Shanghai, China and the Total Antioxidant Capacity Assay Kit (A015-2-1), Alkaline Phosphatase Assay Kit (A059-2-2), High-Density Lipoprotein Assay Kit (A112-1-1), Low-Density Lipoprotein Assay Kit (A113-1-1), Triglycerides Assay Kit (A110-1-1), and Total Cholesterol Assay Kit (A111-1-1) were obtained from the Nanjing Jiangcheng Bioengineering Research Institute, Nanjing, China.
2.6 Histopathological examination
The caecum of the chicks at 21 days of age was sent to the Wuhan Sevilla Company for tissue sectioning and haematoxylin and eosin (HE) staining. For each sample, three representative intestinal villi and crypts were collected, and the villus height (V) and crypt depth (C) were measured via the ImagePro-Plus 7.0 image analysis software (Media Cybernetics, Rockville, MD, United States) (Unit: μm).
2.7 16S rRNA sequencing and bioinformatics analysis
During the necropsy of 21-day-old chicks, the caecal contents were removed under sterile conditions and quickly frozen in liquid nitrogen. After 30 min, the contents were stored at −80°C. Three samples were randomly selected from each group and sent to Shanghai Majorbio Bio-Pharm Technology Co., Ltd., Shanghai, China. for 16S rRNA sequencing of the gut microbiota. Sequencing was performed via the Illumina MiSeq platform (Illumina, Inc), and initial data quality control was conducted via Fastp (version 0.19.6) (HaploX Biotechnology and Shenzhen Institutes of Advanced Technology, Chinese Academy of Sciences), which removes sequences shorter than 50 base pairs. UPARSE (version 7.0.1090) (Robert C. Edgar) was used for operational taxonomic unit (OTU) clustering analysis. The Ribosomal Database Project (RDP) classifier (version 2.11) (Bayesian algorithm) (Michigan State University) was used to conduct a taxonomic analysis of the representative sequences of OTUs at a 97% similarity level in the database and to calculate the community species composition of each sample.
2.8 Transcriptome sequencing and bioinformatics analysis
During the necropsy of 21-day-old chicks, the caecum was removed under sterile conditions, washed in RNase-free water, and frozen in liquid nitrogen. After 30 min, the caecum was stored at −80°C. From each group, three samples were randomly selected and sent to Shanghai Majorbio Bio-Pharm Technology Co., Ltd. for sequencing on the Illumina MiSeq platform. Fastp (version 0.19.6) was used to remove low-quality reads, adapters, and sequences shorter than 50 base pairs. The quality of the post-QC data was further assessed and statistically analysed, with Q20 (%) and Q30 (%) used as metrics for quality evaluation and alignment with reference sequences. RNA-Seq by Expectation–Maximisation (RSEM version 1.3.3) (Bo Li Colin Dewey and Peng Liu) was used for expression analysis between samples. DESeq2 (version 1.24.0) (Bioconductor) was used for differential expression analysis of genes and transcripts between samples, with a threshold of |log2FC| ≥ 1.000, where FC denotes fold change and a p < 0.05. Goatools software (version 0.6.5) (Xiaoqian Jiang and Yu Lin), China was used for gene ontology (GO) enrichment analysis of the gene set to determine the main GO functions of the genes in the set. Fisher’s exact test was applied, and a corrected p < 0.05 indicated significant enrichment of the GO function. (R scripts) (R Core Team) were used to perform the Kyoto Encyclopedia of Genes and Genomes (KEGG) pathway enrichment analysis on the gene set, with the same computational principles as those used for the GO functional enrichment analysis. A corrected p < 0.05 was considered to indicate significant enrichment of the KEGG pathway function.
2.9 Quantitative real-time PCR
Based on the results of the transcriptome sequencing, the key genes whose expression significantly differed between the positive control group (S) and the berberine-treated group (BS) were screened, and the expression levels of five genes related to the treatment of enteritis caused by Salmonella were detected via qRT-PCR. RT-PCR-specific primers were designed and synthesised (Table 2). The total RNA extracted from the caecal tissue was reverse transcribed into complementary DNA (cDNA) via an RNA reverse transcription kit (catalogue number: AT311-02). The samples were prepared according to the instructions of the Real-Time PCR Easy™-SYBR Green I Kit (version Number: 1.0-1403). Three negative controls were used for each gene, and β-actin was used as the reference gene. The relative quantification of the genes listed in Table 2 was performed via the 2–ΔΔCT method.
2.10 Statistical analysis
All experimental data are presented as the mean values. The standard error of the mean (SEM) was determined, and one-way ANOVA was performed via the general linear model (GLM) procedure in SAS software (version 8.02, SAS Institute, Inc., Cary, NC). Dietary berberine treatment and Salmonella challenge influenced the gene expression levels and serum parameter data obtained from the 2 × 2 factorial arrangement analysis. Differences at Pp < 0.05 and p < 0.01 were considered statistically significant.
3 Results
3.1 Effects of berberine and Salmonella on the growth performance of chicks
To determine the impact of adding BBR to the diet on the growth performance of Salmonella-infected chicks, the average daily gain (ADG), average daily feed intake (ADFI), and feed conversion ratio (FCR) of the chicks in each group at 21 and 35 days of age were calculated based on the obtained data (Table 3). There were no significant differences in the ADG, ADFI, or FCR among the groups from 0 to 35 days of age (p > 0.05). These data indicated that throughout the experimental period, neither berberine nor Salmonella stimulation significantly affected the average daily weight gain, daily feed intake, or feed conversion ratio of the Sanhuang male chicks.
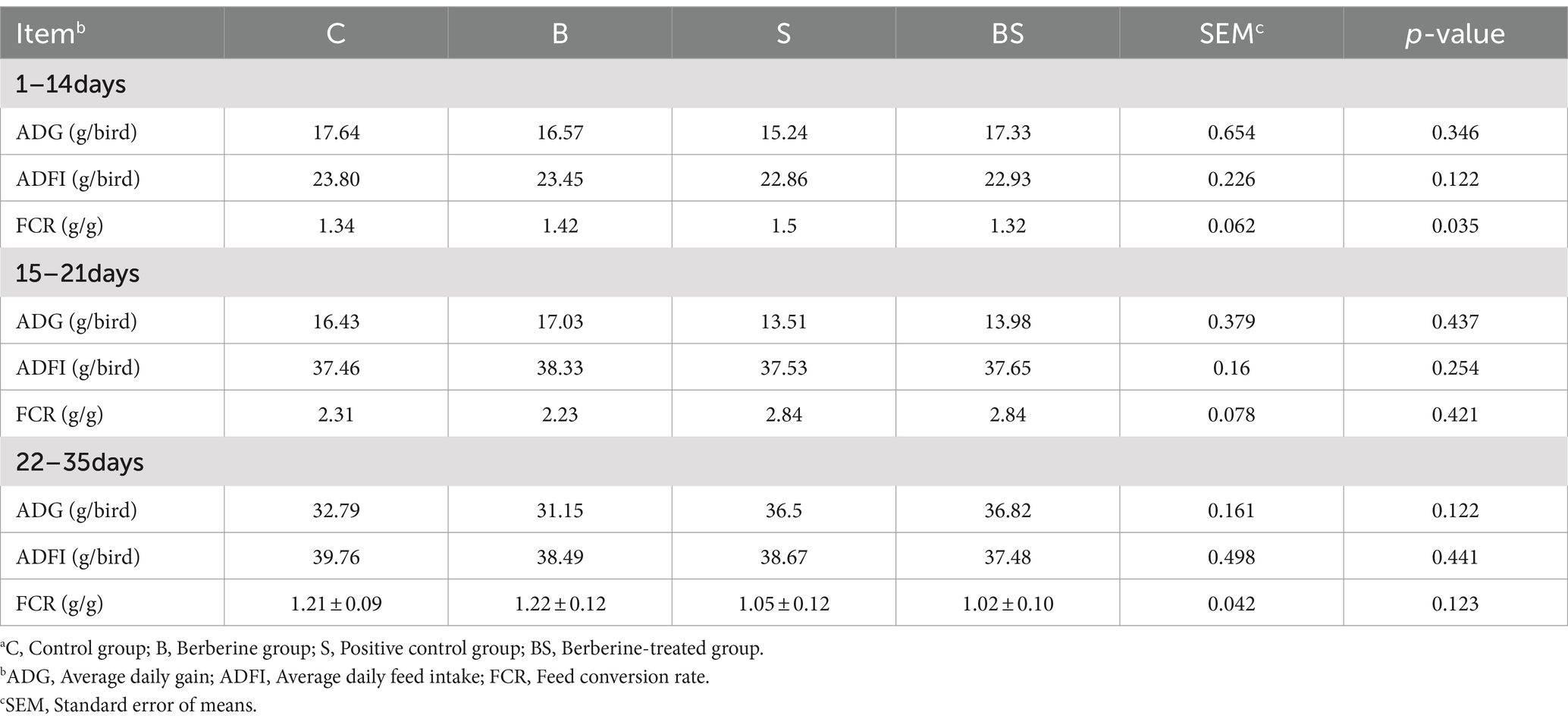
Table 3. Effects of BBR and Salmonella on growth performance of chickensa (N = 120).
3.2 Effects of BBR and Salmonella on the intestinal histomorphology of chicks
To understand the impact of Salmonella infection and berberine treatment on the caecal villi and crypts of chicks, as well as to assess the potential role of berberine in mitigating intestinal tissue damage caused by Salmonella infection, HE staining was performed on caecal tissues from 21-day-old chicks. Figure 1 shows that there were no significant changes in the morphology of the caecum in the berberine group (B) compared with the control group (C). In contrast, Salmonella infection in the positive control group (S) led to a disordered arrangement of caecal epithelial cells and a reduction in villus length, whereas the morphology and villus height in the berberine-treated group (BS) were restored to those of the control group. Table 4 shows variations in the chick caecum’s villus height and crypt depth among the groups. Compared with those in the control group (C), there were changes in the villus height and crypt depth in the BBR group (B). In the Salmonella-infected group (S), the villus height decreased by 25.28%, and the crypt depth increased by 27.88%. Compared with the S group, the villus height in the BS group recovered by 29.34% (p < 0.05), and the crypt depth in the BS group recovered by 28.17% (p < 0.05). These results suggested that berberine alleviates the intestinal tissue damage caused by Salmonella infection to a certain extent.
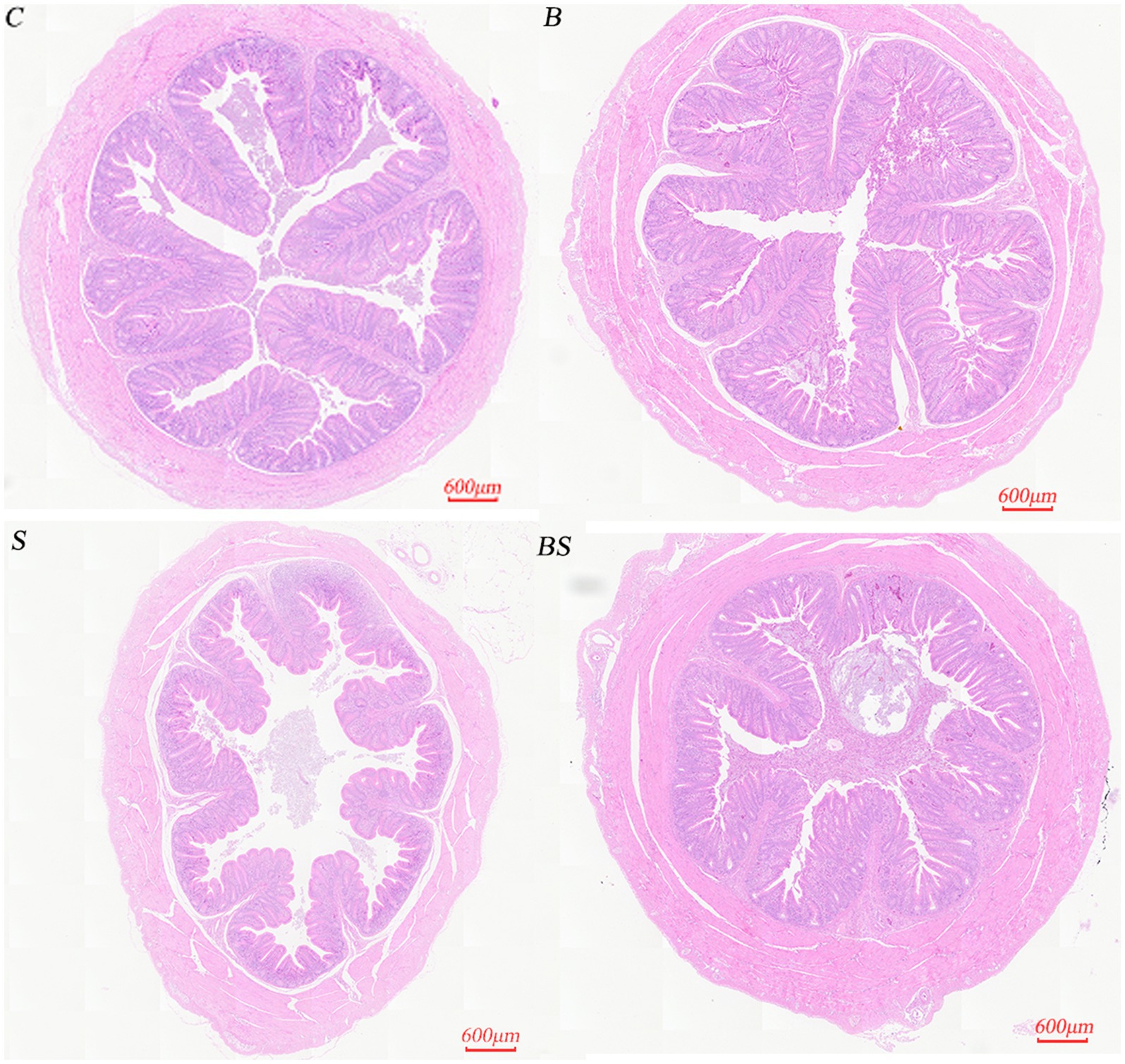
Figure 1. Histological sections of the caecum of a 21-day-old chick (20×). C, Control group; B, Berberine group; S, Positive control group; BS, Berberine-treated group.
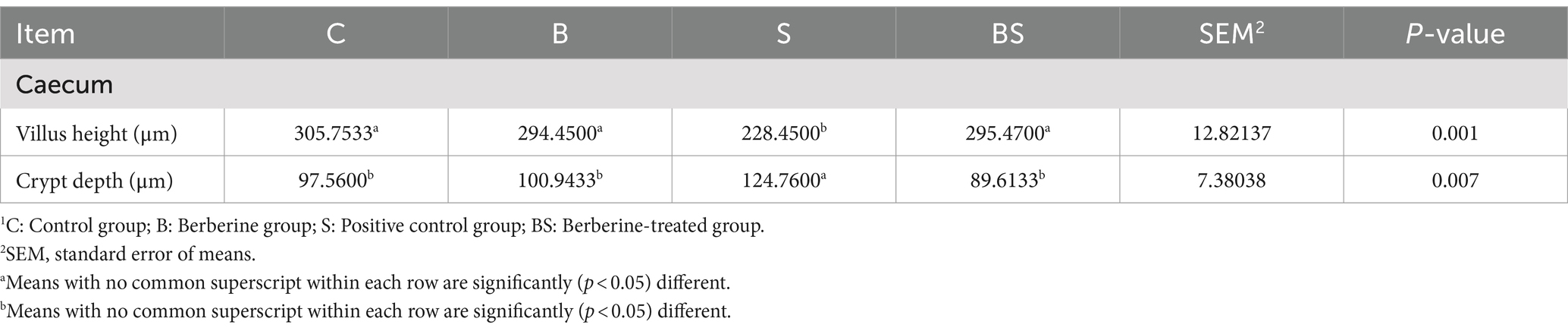
Table 4. Effects of BBR and Salmonella on Intestinal Histomorphology1 (N = 12).
3.3 Effects of berberine and Salmonella on the content of Salmonella in faeces
To determine whether BBR alleviates the presence of Salmonella in chicks after intragastric administration of Salmonella, faeces were collected from chicks in various groups at 35 days of age for Salmonella content determination (Figure 2). Figure 2A shows the Salmonella standard curve obtained from the Salmonella standard product, and Figure 2B shows the copy number corresponding to the groups of Salmonella detected in this test. The content of Salmonella in the faeces of chicks significantly increased after intragastric administration of Salmonella. However, berberine significantly reduced the content of Salmonella.
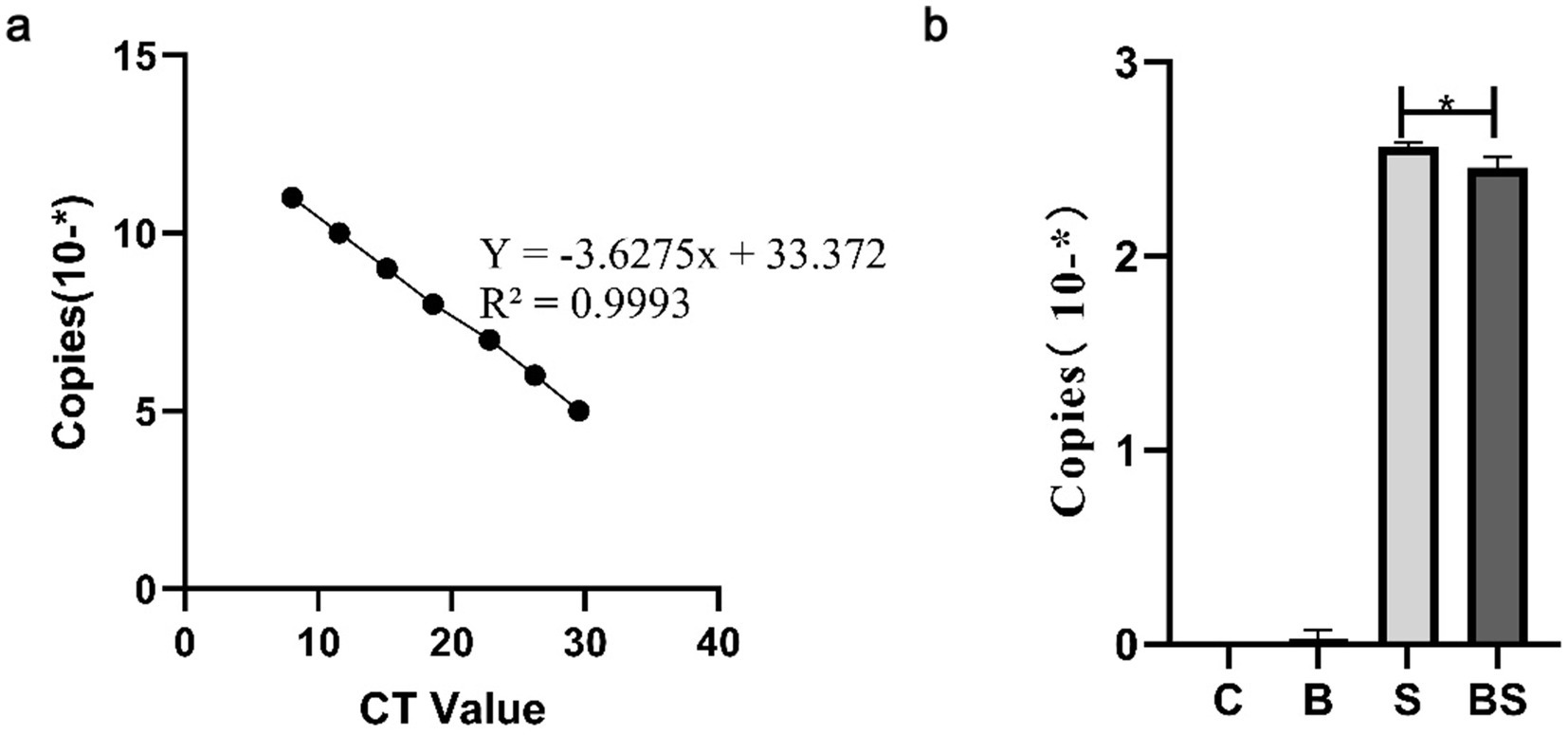
Figure 2. The detection of Salmonella content in the faeces of 35-day-old chicks. (A) Salmonella standard curve; (B) Quantitative PCR detection of Salmonella content in faeces. *Mean p < 0.05.
3.4 Effects of berberine and Salmonella on immune organ indices in chicks
To evaluate whether berberine alleviates or reverses the negative impact of Salmonella infection on indices of immune organs (such as the thymus) in chickens, the liver, spleen, bursa of Fabricius, and thymus from 21-day-old and 35-day-old chicks were weighed. As shown in Table 5, there were slight differences in the liver index, spleen index, and bursa index among the groups, but these differences were not significant (p > 0.05). In contrast, there was a substantial change in the thymus index. At 35 days of age, the thymus index in the S group was significantly lower than that in the C group, while the thymus index in the BS group was restored to the level of the control group (p < 0.05). These results suggested that adding berberine to the diet mitigates the decrease in the thymus index caused by Salmonella infection.
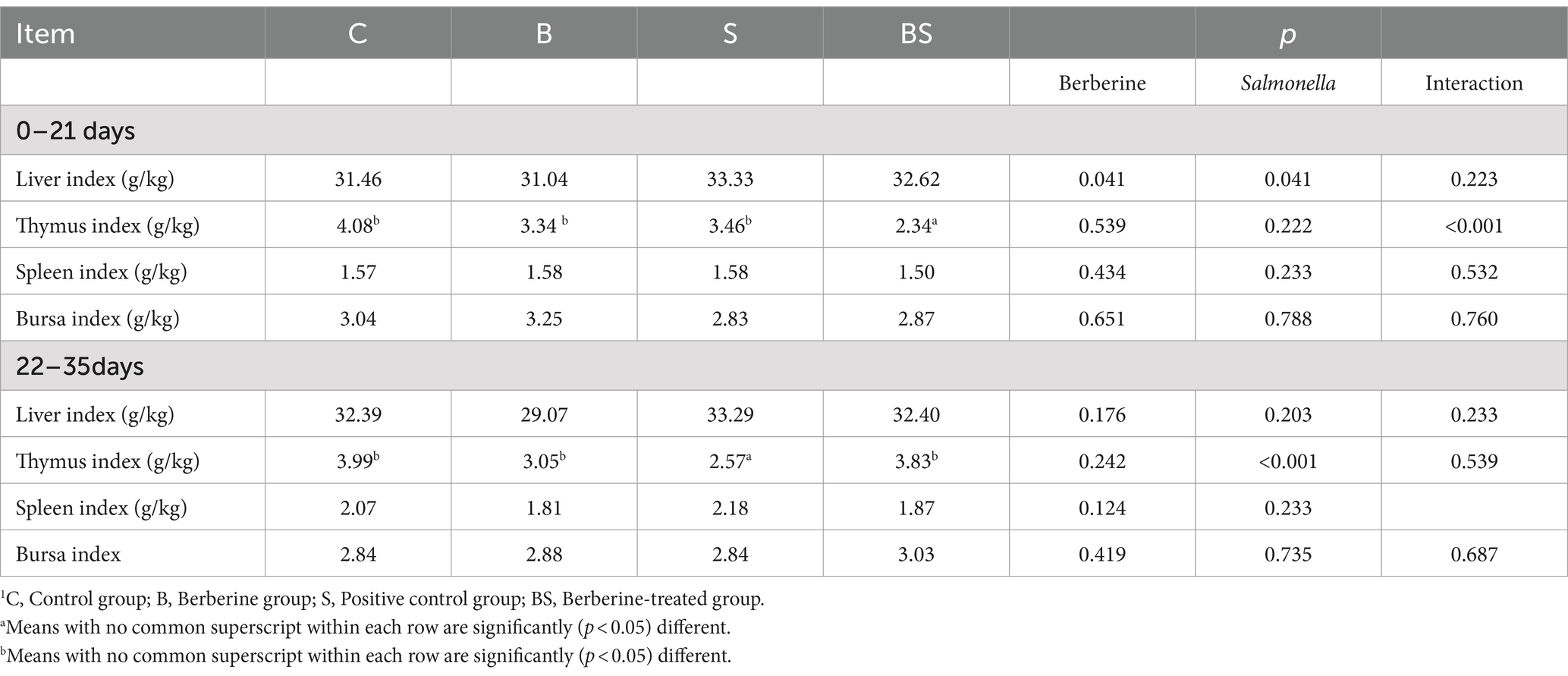
Table 5. Effects of BBR and Salmonella on immune organ index of chicks1 (N = 20).
3.5 Effects of berberine and Salmonella on biochemical indices of chicks
To explore whether berberine modulates the body’s response to Salmonella infection, the levels of immunoglobulins (IgA, IgM, and IgG), antioxidant indicators (alkaline phosphatase activity and total antioxidant capacity), high-density lipoprotein (HDL), low-density lipoprotein (LDL), triglycerides, and total cholesterol were measured in the serum of chicks from each group at 21 and 35 days of age. Compared with those in the control group (C), there was an increasing trend in the concentrations of IgA, IgM, and IgG in the B group (Figure 3A). Still, IgA, IgM, and IgG concentrations were lower in the Salmonella-infected group (S). Compared with those in the S group, IgA, IgM, and IgG concentrations in the BS group were more remarkable, with significant increases in the IgM and IgG concentrations (p < 0.05). The effects of berberine and Salmonella on serum antioxidant indicators are shown in Figure 3B. At 21 days of age, compared with that in the C group, the total antioxidant capacity in the S group significantly decreased (p < 0.05), and the concentration of alkaline phosphatase also reduced, which was mitigated in the BS group. At 35 days of age, compared with that in the C group, the total antioxidant capacity in the B group significantly increased (p < 0.05). Compared with that in the B group, the total antioxidant capacity in the S group decreased, which was mitigated in the BS group. Compared with those in the C group, the concentrations of high-density lipoprotein (HDL) and low-density lipoprotein (LDL), as well as the total cholesterol content, were elevated in the B group; however, the opposite was observed after Salmonella gavage, but no significant differences were observed (p > 0.05) (Figure 3C). These results indicated that BBR modulates various biochemical indicators in the body after Salmonella infection.
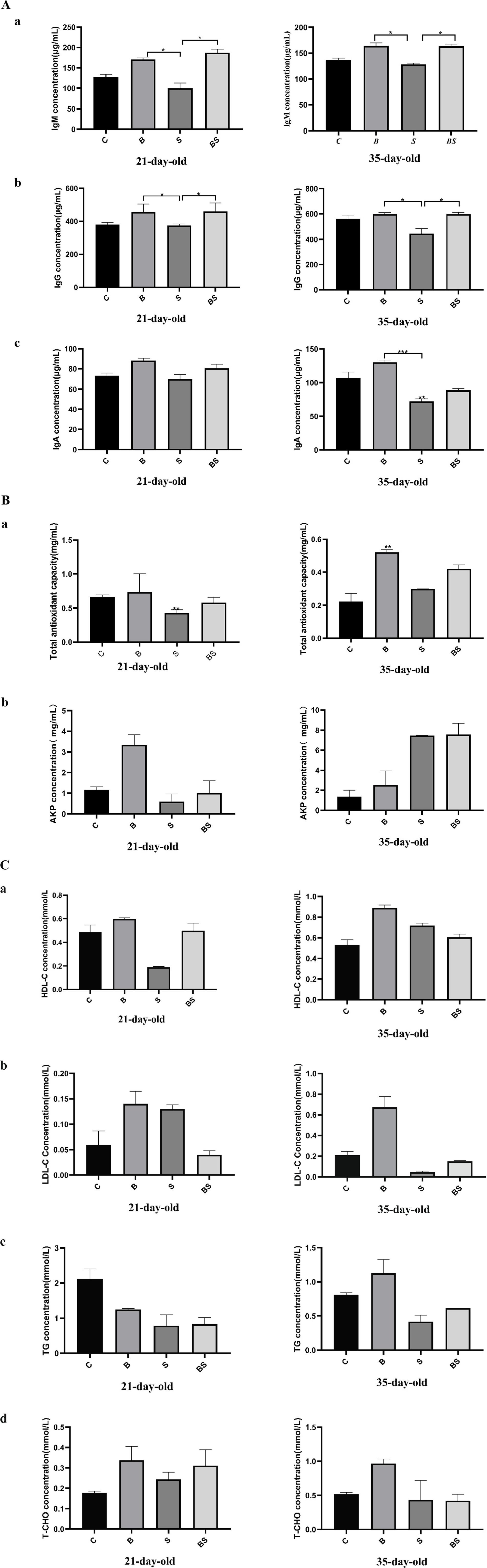
Figure 3. Effects of BBR and Salmonella on serum biochemical indexes of chickens. C, Control group; B, Berberine group; S, Positive control group; BS, Berberine-treated group. (a) Effects of BBR and Salmonella on IgM; (b) Effects of BBR and Salmonella on IgG; (c) Effects of BBR and Salmonella on IgA. *Mean p < 0.05; **Mean p < 0.01; ***Mean p < 0.001. (a) Effects of BBR and Salmonella on total antioxidant capacity; (b) Effects of BBR and Salmonella on alkaline phosphatase. *Mean p < 0.05; **Mean p < 0.01; ***Mean p < 0.001. (a) Effects of BBR and Salmonella on high-density lipoprotein; (b) Effects of BBR and Salmonella on low-density lipoprotein; (c) Effects of BBR and Salmonella on triglycerides; (d) Effects of BBR and Salmonella on total cholesterol. *Mean p < 0.05; **Mean p < 0.01; ***Mean p < 0.001.
3.6 Effects of berberine and Salmonella on the intestinal microbial flora of chicks
To explore the protective effects of adding berberine to the diet on Salmonella-induced enteritis and gut microbiota dysbiosis in chicks, caecal contents from 21-day-old chicks were sent to Shanghai Majorbio Bio-Pharm Technology Co., Ltd. for 16S rRNA sequencing of the gut microbiota. After rarefying the gut microbiota sequences to 29,026 sequence counts for each group, α-diversity analysis was conducted, including species diversity indices (Shannon index and Simpson index) and species richness indices (Sobs, Ace, and Chao indices) (Table 6). The Sobs and Chao indices in both the S group and BS group were significantly lower than those in the C group (p < 0.05), indicating that Salmonella gavage reduced the variety and quantity of the gut microbial populations in the chicks.
Analysis of the composition of the caecal microbiota across the groups revealed 16 predominant bacterial genera (Figure 4A), including Faecalibacterium, norank_f__norank_o__Clostridia_UCG-014, Bacteroides, unclassified_f__Lachnospiraceae, Streptococcus, norank_f__norank_o__Clostridia_vadinBB60_group, Lactobacillus, Blautia, Alistipes, Ruminococcus_torques_group, Eisenbergiella, Subdoligranulum, Parabacteroides, Sellimonas, Lachnoclostridium, and Butyricicoccus.
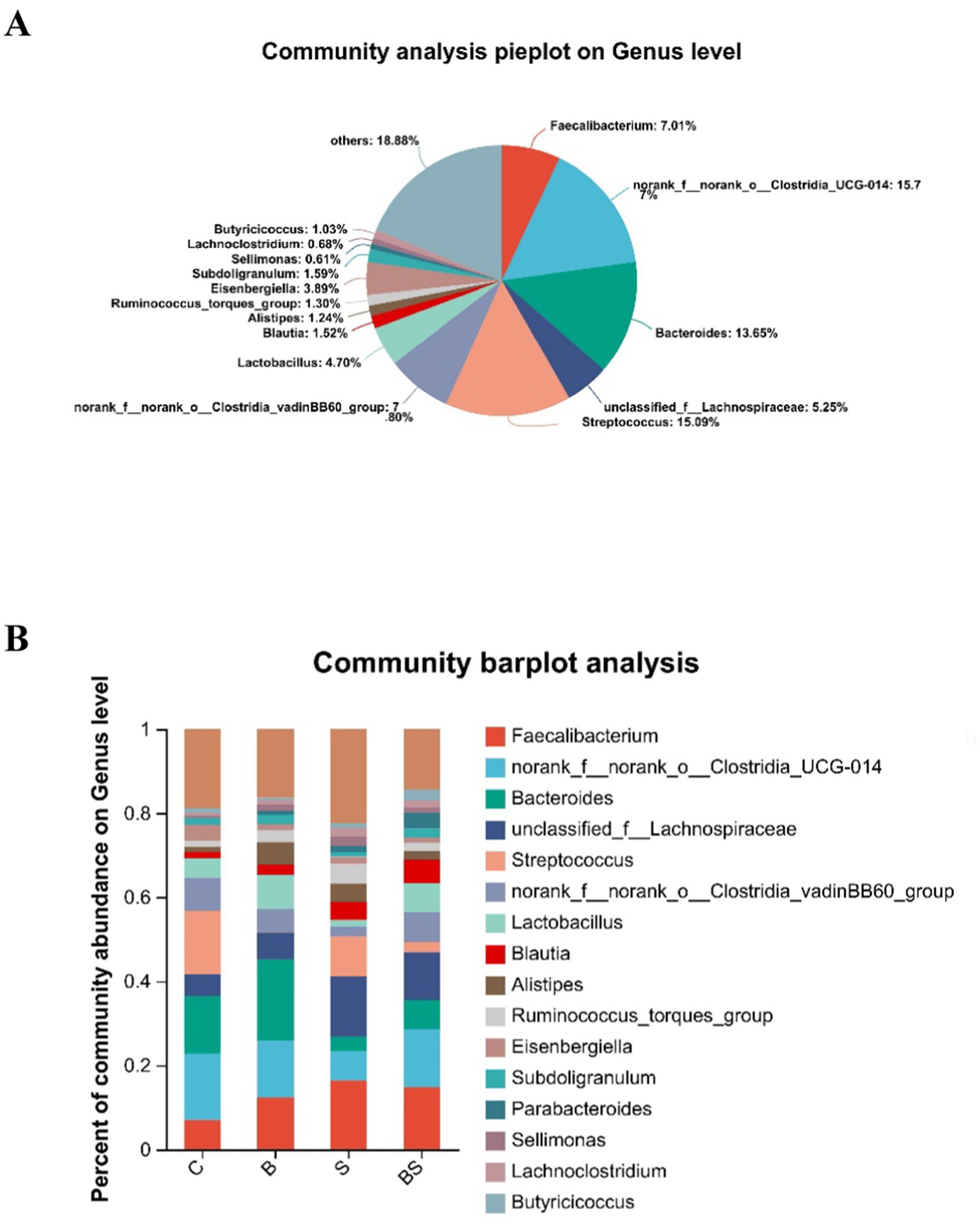
Figure 4. Gut microbiota composition. (A) Community composition at the genus level. Different colours represent distinct bacterial genera, and the area of the pie chart segments corresponds to the percentage that each genus constitutes within the community. (B) Variation in dominant genera composition across different groups. The bars depicted in various colours represent different bacterial genera, with the length of the bars indicating the proportional representation of each genus.
Compared with those in the control group (C), adding BBR increased the abundance of several genera, including Faecalibacterium, Bacteroides, Lactobacillus, and Alistipes. Still, it reduced the abundance of Streptococcus and Eisenbergiella (Figure 4B). Compared with those in the control group (C), the abundances of Faecalibacterium, unclassified_f__Lachnospiraceae, Blautia, and Alistipes were more significant in the Salmonella-infected group (S). In contrast, the abundances of norank_f__norank_o__Clostridia_UCG-014, Bacteroides, Streptococcus, norank_f__norank_o__Clostridia_vadinBB60_group, and Lactobacillus were lower in the S group. Compared with those in the S group, the abundances of norank_f__norank_o__Clostridia_UCG-014, Bacteroides, norank_f__norank_o__Clostridia_vadinBB60_group, and Lactobacillus were more significant in the BS group. On the contrary, the abundances of Faecalibacterium, Streptococcus, and Alistipes were lower in the BS group. These results indicated that Salmonella gavage increases the levels of harmful bacterial genera in the gut. In contrast, adding berberine to the feed reduces the levels of harmful bacterial genera and increases the levels of beneficial bacterial genera.
3.7 Transcriptome sequencing and bioinformatics analysis
To further explore the molecular mechanisms of BBR treatment for Salmonella-induced enteritis in chicks, the caecum from 21-day-old chicks was collected and sent to Shanghai Majorbio Bio-Pharm Technology Co., Ltd. for transcriptome sequencing. A total of 96.93 Gb of clean data were obtained from the sequencing, with each sample yielding more than 6.1 Gb of clean data. The percentage of Q30 bases was more significant than 93.82%, and a total of 20,577 genes were detected, including 19,472 known genes. Figure 5A shows that the correlation coefficients among all samples are high, ranging from 0.912 to 0.973, indicating a strong correlation between these samples. The differential gene expression analysis among the groups is shown in Figure 5B. Compared with the control group (C), the BBR group (B) had 515 upregulated genes and 255 downregulated genes. Compared with the control group, the Salmonella-infected group (S) had 564 upregulated genes and 223 downregulated genes. Compared with the Salmonella-challenged group (S), the BBR-treated group (BS) had 272 upregulated genes and 498 downregulated genes.
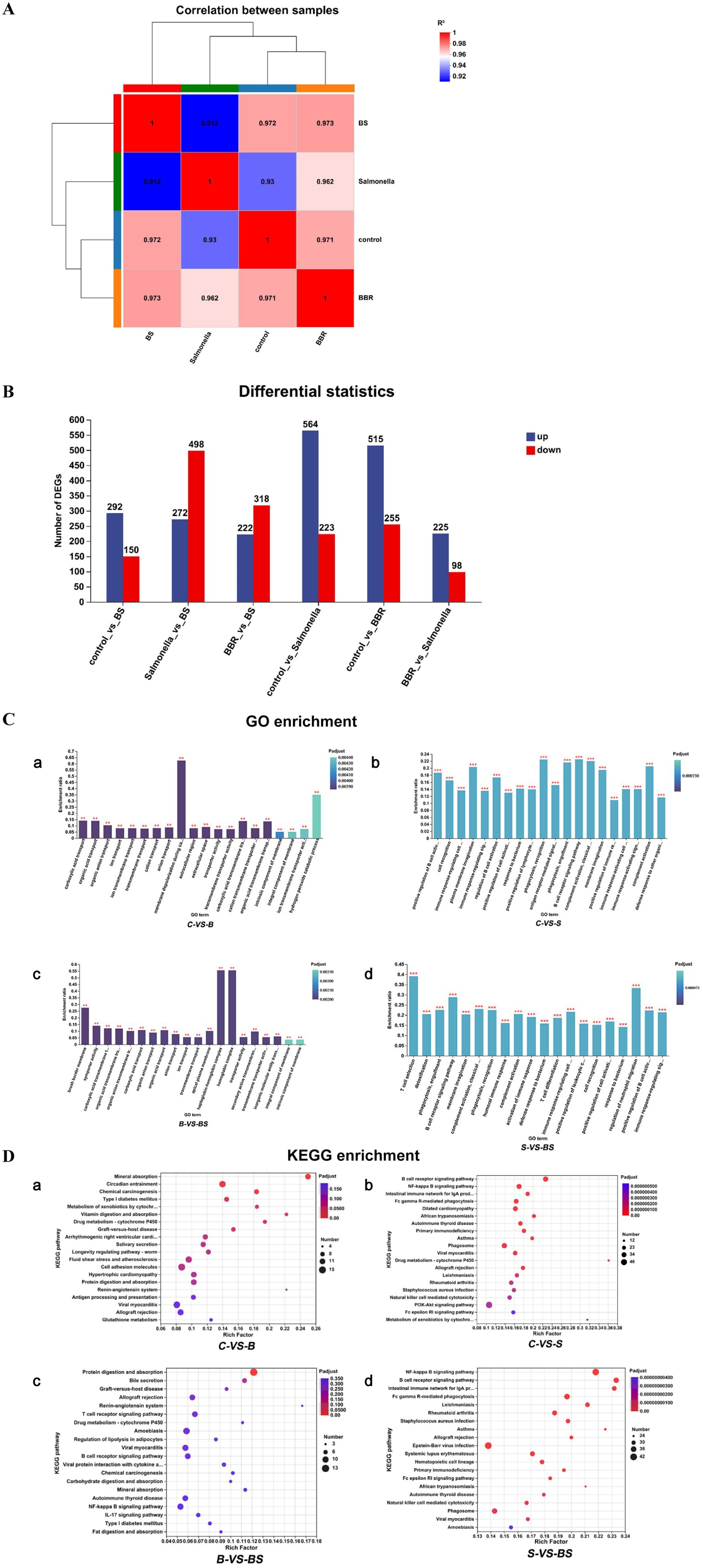
Figure 5. Transcriptome sequencing result analysis. (A) Sample correlation analysis. The right and bottom sides of the chart list the sample names, while the left and upper sides depict the clustering of the samples. The coloured squares represent the degree of correlation between two samples, with different colours indicating a higher or lower correlation. (B) Statistical chart of expression difference between groups. (C) GO Enrichment Analysis. The horizontal axis represents the GO term, and the vertical axis represents the enrichment factor. The gradient of the bar colour indicates the significance of the enrichment, *Mean p < 0.05; **Mean p < 0.01; ***Mean p < 0.001. (D) KEGG enrichment analysis. The vertical axis represents the pathway name, and the horizontal axis represents the enrichment factor. The size of the dots indicates the number of genes in a particular pathway, while the colour of the dots corresponds to different padjust ranges.
According to the GO enrichment results (Figure 5C), compared with those in the control group (C), the differentially expressed genes (DEGs) in the Salmonella group (S) were enriched predominantly in the following biological processes: positive regulation of B cell activation, plasma membrane invagination, phagocytosis recognition, phagocytosis engulfment, the B cell receptor signalling pathway, complement activation, and the classical pathway (Figure 5C-b). Compared with those in the Salmonella group (S), the DEGs in the berberine-treated group (BS) were enriched mainly in T cell selection, the B cell receptor signalling pathway, regulation of neutrophil migration, and positive regulation of B cell activation (Figure 5C-d).
The KEGG pathway enrichment analysis results (Figure 5D) revealed that, compared with those in the control group (C), the DEGs in the berberine group (B) were enriched primarily in the following pathways: mineral absorption, chemical carcinogenesis, vitamin digestion and absorption, drug metabolism (cytochrome P450), and graft vs. host disease (Figure 5D-a). Compared with those in the control group (C), the DEGs in the Salmonella group (S) were enriched mainly in pathways related to the B cell receptor signalling pathway, the NF-κ B signalling pathway, the intestinal immune network for IgA production, African trypanosomiasis, drug metabolism (cytochrome P450), and asthma (Figure 5D-b). Compared with those in the berberine group (B), the differentially expressed genes in the berberine-treated group (BS) were predominantly enriched in the following pathways: protein digestion and absorption; renin–angiotensin system; and mineral absorption (Figure 5D-c). Compared with those in the Salmonella group (S), the DEGs in the berberine-treated group (BS) were enriched mainly in the following pathways: the NF-κ B signalling pathway, the B cell receptor signalling pathway, the intestinal immune network for IgA production, asthma, and the African trypanosomiasis pathway (Figure 5D-d).
3.8 Quantitative real-time PCR analysis
The transcriptome sequencing results were verified via quantitative real-time PCR analysis and gene expression detection. On the basis of the results of the transcriptome sequencing, five genes whose expression significantly differed were selected, namely, ATAD5, ERP29, MGST2, HSP90AA1, and PIK3CA, and verified using quantitative real-time PCR (Figure 6). Compared with those in the control group, the expression levels of ATAD5, ERP29, MGST2, and PIK3CA were downregulated in the Salmonella-challenged group (S) and upregulated in the berberine-treated group (BS), whereas the opposite trend was observed for the expression of HSP90AA1.
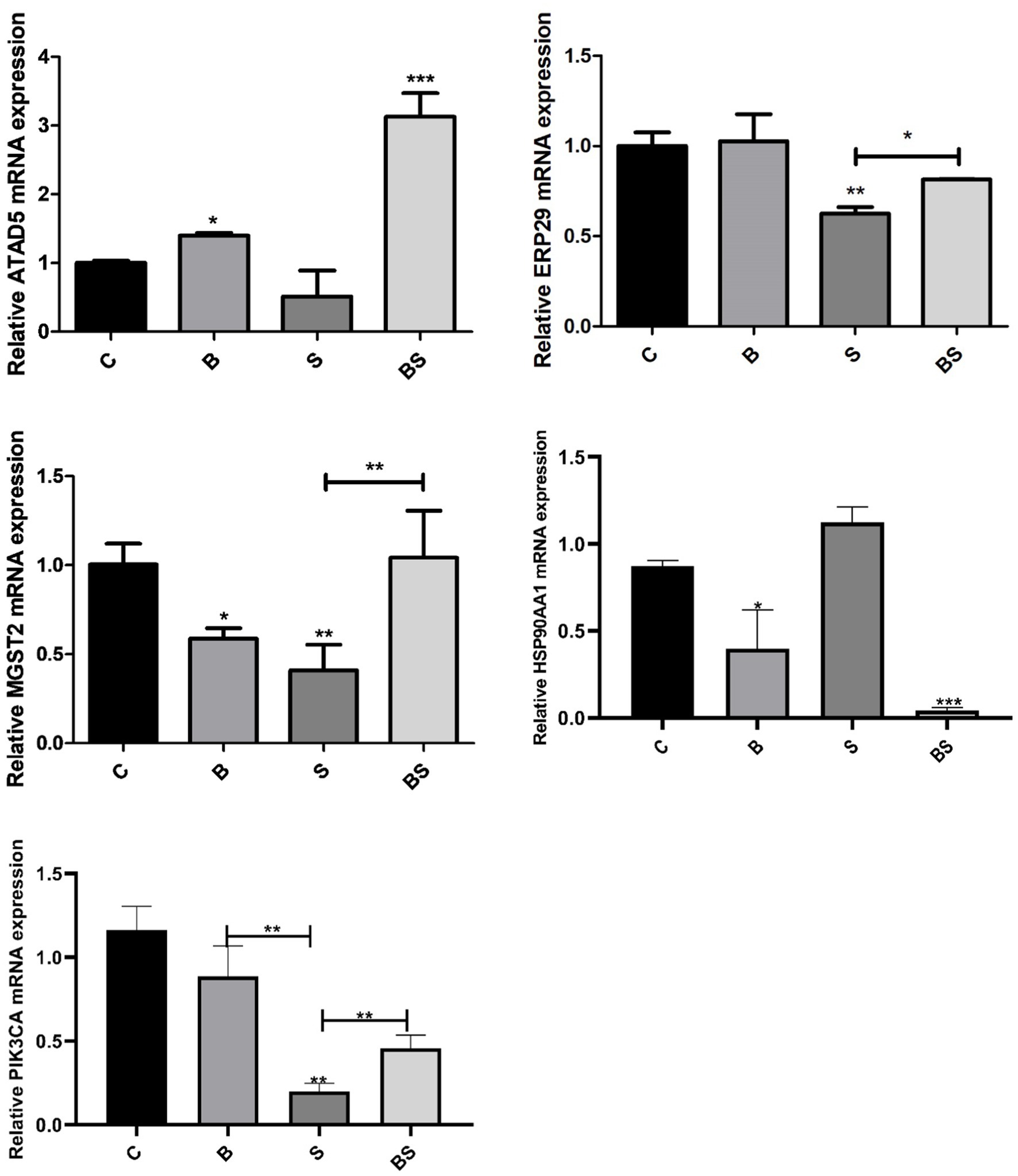
Figure 6. Effects of Berberine on the expression of ATAD5, ERP29, MGST2, HSP90AA1, and PIK3CA genes in the caecum of broilers. *Mean p < 0.05; **Mean p < 0.01; ***Mean p < 0.001.
4 Discussion
In recent years, berberine has been included in the Chinese Pharmacopoeia because of its anti-inflammatory, antioxidant, antibacterial, and antiapoptotic properties, and it has garnered widespread attention in the prevention and treatment of gastrointestinal diseases (Song et al., 2020). The present study revealed that the addition of 120 mg/kg berberine to the diet had no significant effect on the average daily gain (ADG), average daily feed intake (ADFI), or feed conversion ratio (FCR) of the broiler chicks aged 0–35 days (p > 0.05), which may be due to the different growth stages of the broilers or that the feeding period was too short. In addition, research has confirmed that the appropriate addition of berberine to the diet improves the growth performance of poultry. Zhu et al. reported that adding 250 mg/kg berberine to the diet effectively enhances the growth performance of broilers at 63 days of age. Zhang et al. reported that adding 100 mg/kg berberine to the diet effectively improves the growth performance of broilers at 42 days of age (Zhang et al., 2013; Zhu et al., 2021).
Villus height and crypt depth are essential indicators for assessing the intestinal tissue health because they directly reflect intestinal epithelial cells’ renewal and regenerative capacity. Villi are the main structures involved in nutrient absorption in the intestine, whereas crypts are the locations of intestinal stem cells and are responsible for generating new epithelial cells. When the intestine is infected or damaged, these structures may change, such as a reduction in villus height and an increase in crypt depth. These changes are usually associated with a decline in intestinal function and a disease state (Jing et al., 2021). In the present study, the villus height in the S group (Salmonella-infected) was significantly reduced, and the S group’s crypt depth was significantly increased. The villus height and crypt depth in the BS group (Berberine-treated) were both restored compared with those in the S group. This may be due to BBR directly inhibiting or killing invading Salmonella in the intestine or to BBR repairing epithelial cells, thereby alleviating the intestinal structural damage caused by Salmonella. Berberine has antibacterial properties and can directly inhibit or kill bacteria, reducing pathogen attacks on the intestine (Sun et al., 2023). In addition, BBR promotes the repair and regeneration of intestinal epithelial cells, helping to restore the typical structure and function of the intestine (Huo and Wang, 2024).
Immune organs govern the proliferation and development of immune cells. The weights of the thymus, spleen, and bursa of Fabricius, representing the most critical immune organs in poultry, increased, which reflected the growth and reproduction of immune cells, further indicating an increase in the immune capabilities of the organism. The present study revealed that after broilers were inoculated with 1.2 × 109 CFU/mL Salmonella, there was a significant decrease in the thymus index of 35-day-old chicks, suggesting that the invasion of Salmonella decreased the immune system performance in broilers. Wang Shasha et al. also confirmed that the thymus index of chicks significantly decreases after inoculation with 1 × 109 CFU/mL Salmonella for 21 days (Xian-Yao, 2015). Immunoglobulins are an essential part of the immune system in animals, and IgM, IgA, and IgG are essential indicators for measuring humoural immune responses. Higher levels of these immunoglobulins indicate stronger immune functions in the organism (Jili, 2011). The present study revealed that at 21 days of age, the concentrations of IgM and IgG in the serum of chicks in the treatment group were significantly greater than those in the serum of chicks in the challenge group, indicating that the addition of BBR to feed reversed the decrease in immune function caused by Salmonella infection in chicks. Oxidative stress is a negative impact caused by the production of free radicals, such as hydroxyl radicals and superoxide anions, within the animal body. Excessive reactive oxygen species damage proteins and nucleic acids, as well as produce large amounts of MDA to injure tissues, and they cause inflammation and disease in organisms (He Yun and Xiaoying, 2020). At 35 days of age, the addition of berberine significantly increased the total antioxidant capacity in the serum of chicks, which was consistent with previously published results (Zhu Cui et al., 2022).
16S ribosomal RNA (16S rRNA) is a crucial component of the ribosome’s small subunit (30S subunit) in prokaryotes, including bacteria and archaea. 16 rRNA plays a significant role in bacterial taxonomy, phylogenetic analysis, and microbial community research. The sequence of the 16S rRNA gene contains both highly conserved regions and highly variable regions, making it an ideal molecular marker for studying microbial diversity and evolutionary relationships (Nelson et al., 2014). In the present study, caecal contents from 21-day-old chicks were sent to a Shanghai Majorbio Bio-Pharm Technology Co., Ltd. for 16S rRNA sequencing of the gut microbiota. α-Diversity assessment reflects the microbial community’s richness and diversity, including a series of statistical analysis indices to estimate species abundance and diversity within environmental communities. The Sobs, Ace, and Chao indices of the Salmonella-challenged and the berberine-treated groups were significantly lower than those of the control group, indicating that Salmonella gavage effectively reduced the microbial abundance of the caecal microbiota. Similarly, Wang et al. revealed that Salmonella reduces the microbial abundance of the gut microbiota (Chen and Stappenbeck, 2019).
The genus-level gut microbiota diversity results revealed that the addition of berberine to the diet increased the abundance of genera, such as Faecalibacterium, Bacteroides, and Lactobacillus, but reduced the abundance of other genera, such as Streptococcus and Eisenbergiella. In contrast, gavage with Salmonella led to an increase in the abundance of some genera, such as Faecalibacterium, Blautia, and Alistipes, but a decrease in the abundance of Bacteroides, Streptococcus, and Lactobacillus. The genus Lactobacillus plays an important role in maintaining the health of the animal body. Lactobacillus enhances the absorption of nutrients by the body, whereas it inhibits the proliferation of pathogenic bacteria in the intestine. The gut is protected from harmful bacteria by the competitive exclusion of pathogens and the production of antimicrobial substances, which are probiotics of the gut (Rajab et al., 2020). The genus Faecalibacterium promotes the production of short-chain fatty acids (such as butyrate), which are crucial for maintaining the health of intestinal epithelial cells and strengthening intestinal barrier function (Jiang et al., 2024). The genus Streptococcus is widely distributed in nature, and the majority of the species of Streptococcus are nonpathogenic; however, Group A Streptococcus has strong pathogenicity, and Gallid Streptococcus causes causing streptococcosis in chickens, which can severely damage the economic benefits of breeders (Barnham et al., 1980; Wollein Waldetoft and Råberg, 2014). Compared with those in the Salmonella-challenged group (S), the abundances of Bacteroides and Lactobacillus in the berberine-treated group (BS) increased, whereas the abundances of Faecalibacterium, Streptococcus, and Alistipes decreased in the BS group. These results indicated that BBR alleviates the negative effects of Salmonella infection by regulating the balance of the gut microbiota, which agreed with previously published findings (Zhang et al., 2013).
Transcriptome sequencing is a rapid, efficient, and accurate method for identifying differences in gene expression levels among various samples. The present study sent caecal tissues from 21-day-old chicks to Shanghai Majorbio Bio-Pharm Technology Co., for transcriptome sequencing. This study aimed to screen for key genes that may be involved in the alleviation of Salmonella infection in chicks by berberine and to explore the molecular mechanisms by which berberine alleviates Salmonella infection in chicks at the mRNA level. Compared with those in the blank control group (C), there were 787 DEGs in the Salmonella-challenged group (S). These genes were significantly enriched in the following GO terms: positive regulation of B cell activation, plasma membrane invagination, phagocytosis recognition, phagocytosis engulfment, the B cell receptor signalling pathway, and complement activation of the classical pathway. The enrichment of ‘positive regulation of B-cell activation’ and the ‘B cell receptor signalling pathway’ was related to immune system processes, indicating that Salmonella infection may activate B cell-mediated immune responses, in which B cells play a crucial role in antibody production and the formation of immune memory (Okkenhaug, 2016; Wang and Zheng, 2013). The enrichment of ‘phagocytosis recognition’ and ‘phagocytosis engulfment’ is related to phagocytosis, suggesting that Salmonella infection may increase the phagocytic activity of phagocytes (such as macrophages and neutrophils), which is an essential mechanism by which the host clears pathogens (Jiang et al., 2021; Wang and Ding, 2020). The enrichment of the ‘complement activation’ and ‘classical pathway’ indicated that the complement system is activated after Salmonella infection, which is part of the host’s innate immunity and plays a vital role in pathogen clearance and inflammatory responses (Bajic et al., 2015; Noris and Remuzzi, 2013). Compared with those in the Salmonella-challenged group (S), there were 770 DEGs in the berberine-treated group (BS), which were enriched mainly in GO terms related to T cell selection, the B cell receptor signalling pathway, the regulation of neutrophil migration, and the positive regulation of B cell activation. The enrichment of ‘T cell selection’ suggested that berberine may affect the T cells’ selection and maturation process, which is crucial for the development of adaptive immune responses (Turner et al., 2009). The enrichment of ‘regulation of neutrophil migration’ suggested that berberine may have a regulatory effect on the migration of neutrophils, which is essential for the localization and control of inflammatory responses (Brazil and Parkos, 2016). Moreover, the enrichment of the ‘B cell receptor signalling pathway’ and ‘positive regulation of B cell activation’ indicated that berberine might alleviate the negative effects of Salmonella infection in chicks by modulating the function of B cells and antibody production.
KEGG enrichment analysis was performed on the significantly differentially expressed genes. Compared with those in the control group (C), the DEGs in the Salmonella-infected group (S) were enriched predominantly in the following pathways: the B cell receptor signalling pathway, NF-κ B signalling pathway, intestinal immune network for IgA production, African trypanosomiasis, drug metabolism (cytochrome P450), and asthma pathways. The enrichment of the ‘B-cell receptor signalling pathway’ and ‘intestinal immune network for IgA production’ pathways highlighted the impact of Salmonella infection on the intestinal immune network, which may be related to the host’s defence mechanisms against pathogens. IgA is the most abundant type of antibody in the mucosal immune response of the gut. The enrichment of this pathway may reflect the impact of Salmonella infection on IgA production in the intestinal mucosa, which is crucial for local immune defence and pathogen clearance (Gutzeit et al., 2014). The enrichment of the ‘NF–κ B signalling pathway’ suggested that infection may activate the NF-κB pathway, which is a key transcription factor that regulates the expression of inflammatory genes (Hayden and Ghosh, 2012). Salmonella infection may induce the expression of inflammatory genes and trigger the host inflammatory response by activating the NF-κB pathway. Compared with those in the S group, the DEGs in the berberine-treated group (BS) were enriched mainly in the following pathways: the NF–κ B signalling pathway, B cell receptor signalling pathway, the intestinal immune network for IgA production, asthma pathway, and African trypanosomiasis pathway. The enrichment of the ‘NF–κ B signalling pathway’, ‘B cell receptor signalling pathway’, and ‘intestinal immune network for IgA production’ suggested that the addition of berberine to the diet may regulate the upregulation or downregulation of key genes in these pathways, thereby balancing the inflammatory and immune responses induced by Salmonella infection. The results of the GO and KEGG enrichment analyses suggested that berberine may alleviate the negative effects of Salmonella infection in chicks by regulating immune responses, enhancing phagocytosis, affecting complement activation, modulating drug metabolism, and modulating digestive absorption mechanisms.
Based on the transcriptome data, five genes with significantly differential expression, namely, ATAD5, ERP29, MGST2, PIK3CA, and HSP90AA1, were selected and qRT–PCR was used to measure their mRNA expression levels in the caecal tissues of broilers after Salmonella challenge. ATAD5 is a member of the nucleotide adenosine triphosphatase (ATPase) family involved in DNA replication and cell cycle control. ATAD5 interacts with proliferating cell nuclear antigen (PCNA) to facilitate normal DNA replication and cell division. Studies have shown that downregulation of ATAD5 may be associated with DNA replication stress and genomic instability (Kim et al., 2020). According to the present results, the downregulation of ATAD5 after Salmonella infection may indicate an impaired cell cycle and DNA replication in the intestine, and berberine may alleviate this obstruction. Endoplasmic reticulum protein 29 (ERp29) is a molecular chaperone that plays a crucial role in protein folding, transport, and secretion (Ye et al., 2017). In the present study, the ERP29 gene expression level was downregulated after Salmonella infection in chicks, and this downregulation was partially reversed by berberine treatment. Thus, berberine may block the association between Salmonella invasion and ERP29, protecting the tight junctions of intestinal tissue, which is also supported by the present histopathological results and previous laboratory findings (McLaughlin et al., 2018). Phosphatidylinositol-4,5-bisphosphate 3-kinase, catalytic subunit α (PIK3CA) is a crucial component of the phosphatidylinositol-3-kinase (PI3K) signalling pathway. Studies have shown that this pathway plays a vital role in various biological processes within the cell, including cell survival, proliferation, apoptosis, metabolism, and cell migration (Iorga et al., 2017). The overexpression or activation of PIK3CA often leads to abnormal activation of the PI3K–Akt pathway, which may be related to cell survival, apoptosis, and oxidative stress status (Engelman et al., 2006). In the present study, berberine regulated the gene expression of PIK3CA, suggesting that berberine may have an inhibitory effect on the PIK3CA/PI3K-Akt signalling pathway by reducing its abnormal activation, which may help regulate cell proliferation and survival, as well as reduce the risk of apoptosis (Manning, 2017; Vanhaesebroeck et al., 2010). HSP90AA1 is closely related to inflammation and regulates cell proliferation and apoptosis. Previous studies have shown that inhibiting HSP90AA1 induces autophagy, thereby improving ulcerative colitis (Song et al., 2020; Yang et al., 2020). Su et al. revealed that berberine has functions similar to those of HSP90 inhibitors and inhibits the expression of the HSP90 protein in colon cancer SW840 cells, thereby inhibiting the proliferation of SW840 cells (Su et al., 2015).
Based on the above findings, the prevention and treatment of Salmonella infection by berberine may be related to the coordinated action of multiple targets. Berberine is likely involved in treating Salmonella infection by regulating cellular processes, such as the cell cycle, apoptosis, oxidative stress, and inflammatory responses.
5 Conclusion
The present study revealed that berberine has a promising therapeutic effect on Salmonella infection in chicks. Moreover, berberine regulates the expression of multiple genes involved in several pathways. These results suggest that BBR treatment of Salmonella has potential clinical value, and its specific molecular mechanisms warrant further in-depth investigation.
Data availability statement
The datasets presented in this study can be found in online repositories. The names of the repository/repositories and accession number(s) can be found in the article/supplementary material.
Ethics statement
The animal study was approved by Xinjiang Agricultural University College of Veterinary Medicine Animal Care and Use Committee. The study was conducted in accordance with the local legislation and institutional requirements.
Author contributions
LY: Conceptualization, Formal analysis, Funding acquisition, Investigation, Methodology, Supervision, Writing – original draft, Writing – review & editing. JS: Data curation, Methodology, Software, Validation, Writing – original draft. TY: Data curation, Methodology, Validation, Writing – original draft. XZ: Validation, Writing – original draft. CX: Validation, Writing – original draft. YW: Validation, Writing – original draft. YL: Validation, Writing – original draft. YZ: Validation, Writing – original draft. SZ: Validation, Writing – original draft. QW: Validation, Writing – original draft. HS: Conceptualization, Formal analysis, Investigation, Supervision, Writing – review & editing. QF: Conceptualization, Formal analysis, Investigation, Project administration, Writing – review & editing. LX: Conceptualization, Formal analysis, Investigation, Methodology, Project administration, Supervision, Writing – review & editing.
Funding
The author(s) declare that financial support was received for the research, authorship, and/or publication of this article. The present study received funding from the Open project of Xinjiang Key Laboratory of New Drug Research and Development for Herbivores (XJCDVM-HDRC-T202305), the Tianshan Elite Youth Top-notch Talent Project of the Autonomous Region (2023TSYCQNTJ0022), the National Natural Science Foundation of China (2522GZRJJ), the Autonomous Region Innovation Team Project (2023D14018), the National Key Research and Development Program Projects (2021YFD1600702-3), and the Youth Science Foundation Project of the Natural Science Foundation of Xinjiang Uygur Autonomous Region (2022D01B21).
Acknowledgments
We are grateful for the resources and equipment provided by the College of Veterinary Medicine at Xinjiang Agricultural University and Xinjiang Key Laboratory of Herbivore Drug Research and Creation.
Conflict of interest
The authors declare that the research was conducted in the absence of any commercial or financial relationships that could be construed as potential conflicts of interest.
Publisher’s note
All claims expressed in this article are solely those of the authors and do not necessarily represent those of their affiliated organizations, or those of the publisher, the editors and the reviewers. Any product that may be evaluated in this article, or claim that may be made by its manufacturer, is not guaranteed or endorsed by the publisher.
References
Bajic, G., Degn, S. E., Thiel, S., and Andersen, G. R. (2015). Complement activation, regulation, and molecular basis for complement-related diseases. EMBO J. 34, 2735–2757. doi: 10.15252/embj.201591881
Bao, H. D., Hu, S. J., and Wang, R. (2022). Research progress on biological prevention and control of Salmonella phage in animals and foods. J. Food Saf. Qual. 13, 605–611. doi: 10.19812/j.cnki.jfsq11-5956/ts.2022.02.023
Barnham, M., Kerby, J., and Skillin, J. (1980). An outbreak of streptococcal infection in a chicken factory. J. Hygiene 84, 71–75. doi: 10.1017/S002217240002653X
Brazil, J. C., and Parkos, C. A. (2016). Pathobiology of neutrophil-epithelial interactions. Immunol. Rev. 273, 94–111. doi: 10.1111/imr.12446
Chen, F., and Stappenbeck, T. S. (2019). Microbiome control of innate reactivity. Curr. Opin. Immunol. 56, 107–113. doi: 10.1016/j.coi.2018.12.003
Cheng, H., Liu, J., Tan, Y., Feng, W., and Peng, C. (2022). Interactions between gut microbiota and berberine, a necessary procedure to understand the mechanisms of berberine. J. Pharm. Anal. 12, 541–555. doi: 10.1016/j.jpha.2021.10.003
Cui, X.-D., Liu, X. K., Ma, X. Y., Li, S. H., Zhang, J. K., Han, R. J., et al. (2024). Restoring colistin sensitivity in colistin-resistant Salmonella and Escherichia coli: combinatorial use of berberine and EDTA with colistin. mSphere 9:e0018224. doi: 10.1128/msphere.00182-24
Dan, C. Y.-J. Z. X. Z. (2022). Progress in the formulation of Berberine and its salts. Chin. J. Drug Eval. 39, 140–147. doi: 10.3389/fphar.2022.940282
Dong, Y., Fan, H., Zhang, Z., Jiang, F., Li, M., Zhou, H., et al. (2022). Berberine ameliorates DSS-induced intestinal mucosal barrier dysfunction through microbiota-dependence and Wnt/β-catenin pathway. Int. J. Biol. Sci. 18, 1381–1397. doi: 10.7150/ijbs.65476
Engelman, J. A., Luo, J., and Cantley, L. C. (2006). The evolution of phosphatidylinositol 3-kinases as regulators of growth and metabolism. Nat. Rev. Genet. 7, 606–619. doi: 10.1038/nrg1879
Fang, W. L.-L. F. (2020). Advances in structural modification and structure-activity relationship of berberine. Chin. J. New Drugs 29, 1257–1264. doi: 10.1007/s00210-024-03199-0
Feng, A. W., Gao, W., Zhou, G. R., Yu, R., Li, N., Huang, X. L., et al. (2012). Berberine ameliorates COX-2 expression in rat small intestinal mucosa partially through PPARγ pathway during acute endotoxemia. Int. Immunopharmacol. 12, 182–188. doi: 10.1016/j.intimp.2011.11.009
Feng, X., Wang, K., Cao, S., Ding, L., and Qiu, F. (2021). Pharmacokinetics and excretion of Berberine and its nine metabolites in rats. Front. Pharmacol. 11:594852. doi: 10.3389/fphar.2020.594852
Fu, H. Q., Li, L., Yao, Z. L., and Wang, Y. J. (2020). Isolation, identification and characterization of Salmonella from a parental breeder farm of white broiler and its commercial chickens in northern Jiangsu. Microbiol. Chin. 47, 4105–4112. doi: 10.13344/j.microbiol.china.200631
Galán-Relaño, Á., Valero Díaz, A., Huerta Lorenzo, B., Gómez-Gascón, L., Mena Rodríguez, M. Á., Carrasco Jiménez, E., et al. (2023). Salmonella and salmonellosis: an update on public health implications and control strategies. Animals 13:3666. doi: 10.3390/ani13233666
Guo, Y., Wang, S., Liu, G., and Zhou, C. (2018). Regulation mechanisms of Berberine on inflammatory bowel disease. Chin. J. Anim. Nutr. 30, 4363–4368. doi: 10.1016/j.phrs.2018.09.010
Gutzeit, C., Magri, G., and Cerutti, A. (2014). Intestinal IgA production and its role in host-microbe interaction. Immunol. Rev. 260, 76–85. doi: 10.1111/imr.12189
Hayden, M. S., and Ghosh, S. (2012). NF-κB, the first quarter-century: remarkable progress and outstanding questions. Genes Dev. 26, 203–234. doi: 10.1101/gad.183434.111
He Yun, Y. L., and Xiaoying, G. (2020). Progress in the study of Berberine's mechanism of action in the treatment of type 2 diabetes. Chin. J. Exp. Tradition. 33, 69–73. doi: 10.3969/j.issn.1001-6910.2020.12.23
Huo, A., and Wang, F. (2024). Berberine alleviates ischemia reperfusion injury induced AKI by regulation of intestinal microbiota and reducing intestinal inflammation. BMC Complement. Med. Ther. 24:66. doi: 10.1186/s12906-023-04323-y
Iorga, A., Dara, L., and Kaplowitz, N. (2017). Drug-induced liver injury: Cascade of events leading to cell death, apoptosis or necrosis. Int. J. Mol. Sci. 18:1018. doi: 10.3390/ijms18051018
Izadparast, F., Riahi-Zajani, B., Yarmohammadi, F., Hayes, A. W., and Karimi, G. (2022). Protective effect of berberine against LPS-induced injury in the intestine: a review. Cell Cycle 21, 2365–2378. doi: 10.1080/15384101.2022.2100682
Jiang, J., Li, C., Li, Z., Liu, J., Tian, Q., and Tingting, Z. (2024). Research progress on the regulation of intestinal flora and effect on intestinal absorption and transport by TCM components. J, Pharm. Pract. Serv. 42, 89–120. doi: 10.12206/j.issn.2097-2024.202303012
Jiang, L., Wang, P., Song, X., Zhang, H., Ma, S., Wang, J., et al. (2021). Salmonella Typhimurium reprograms macrophage metabolism via T3SS effector SopE2 to promote intracellular replication and virulence. Nat. Commun. 12:879. doi: 10.1038/s41467-021-21186-4
Jili, X. (2011). Effects of different Lactobacillus strains and dosages on growth performance, Cecal microflora and immunity of broilers. Northeast Agricultural University.
Jing, W., Dong, S., Luo, X., Liu, J., Wei, B., du, W., et al. (2021). Berberine improves colitis by triggering AhR activation by microbial tryptophan catabolites. Pharmacol. Res. 164:105358. doi: 10.1016/j.phrs.2020.105358
Johari, M. I., Besari, A. M., Wan Ghazali, W. S., and Yusof, Z. (2019). Disseminated Salmonella infection. BMJ Case Rep. 12:e226337. doi: 10.1136/bcr-2018-226337
Junwei, L. Y. Z. G. Z. J. L. J. W. (2021). Discussion on the monitoring status of Salmonella during livestock and poultry slaughtering in EU, the united state and China. Chin. Anim. Health Inspect. 38, 69–75. doi: 10.3969/j.issn.1005-944X.2021.06.016
Kim, S., Kang, N., Park, S. H., Wells, J., Hwang, T., Ryu, E., et al. (2020). ATAD5 restricts R-loop formation through PCNA unloading and RNA helicase maintenance at the replication fork. Nucleic Acids Res. 48, 7218–7238. doi: 10.1093/nar/gkaa501
Lamichhane, B., Mawad, A. M. M., Saleh, M., Kelley, W. G., Harrington, P. J., Lovestad, C. W., et al. (2024). Salmonellosis: an overview of epidemiology, pathogenesis, and innovative approaches to mitigate the antimicrobial resistant infections. Antibiotics 13:76. doi: 10.3390/antibiotics13010076
Li, C., Xi, Y., Li, S., Zhao, Q., Cheng, W., Wang, Z., et al. (2015). Berberine ameliorates TNBS induced colitis by inhibiting inflammatory responses and Th1/Th17 differentiation. Mol. Immunol. 67, 444–454. doi: 10.1016/j.molimm.2015.07.013
Li, M., Zhang, M., Zhang, Z. L., Liu, N., Han, X. Y., Liu, Q. C., et al. (2017). Induction of apoptosis by Berberine in hepatocellular carcinoma HepG2 cells via downregulation of NF-κB. Oncol. Res. 25, 233–239. doi: 10.3727/096504016X14742891049073
Liang, H., Wu, X. J., Xu, X., Tian, M. X., Chan, D., Huan-Rong, Z., et al. (2019). Multiplex PCR assay for detection of avian pathogenic E. coli, Salmonella enteritidis, Salmonella typhimurium, Salmonella gallinarum and Salmonella pullorum. Microbiol. Chin. 46, 960–966. doi: 10.13344/j.microbiol.china.180269
Manning, B. D. (2017). AKT/PKB signaling: navigating the network. Cell 169, 381–405. doi: 10.1016/j.cell.2017.04.001
McLaughlin, T., Falkowski, M., Wang, J. J., and Zhang, S. X. (2018). Molecular chaperone ERp29: A potential target for cellular protection in retinal and neurodegenerative diseases. Adv. Exp. Med. Biol. 1074, 421–427. doi: 10.1007/978-3-319-75402-4_52
Nelson, M. C., Morrison, H. G., Benjamino, J., Grim, S. L., and Graf, J. (2014). Analysis, optimization and verification of Illumina-generated 16S rRNA gene amplicon surveys. PLoS One 9:e94249. doi: 10.1371/journal.pone.0094249
Noris, M., and Remuzzi, G. (2013). Overview of complement activation and regulation. Semin. Nephrol. 33, 479–492. doi: 10.1016/j.semnephrol.2013.08.001
Okkenhaug, K. B. J. (2016). PI3K signaling in Normal B cells and chronic lymphocytic leukemia (CLL). Curr. Top. Microbiol. Immunol. 393, 123–142. doi: 10.1007/82_2015_484
Rajab, S., Tabandeh, F., Shahraky, M. K., and Alahyaribeik, S. (2020). The effect of lactobacillus cell size on its probiotic characteristics. Anaerobe 62:102103. doi: 10.1016/j.anaerobe.2019.102103
Robinson, R. A. (1970). Salmonella infection diagnosis and control. N. Z. Vet. J. 18, 259–277. doi: 10.1080/00480169.1970.33918
Shan, C. Y., Yang, J. H., Kong, Y., Wang, X. Y., Zheng, M. Y., Xu, Y. G., et al. (2013). Alteration of the intestinal barrier and GLP2 secretion in Berberine-treated type 2 diabetic rats. J. Endocrinol. 218, 255–262. doi: 10.1530/JOE-13-0184
Shi, C., Li, M., Muhammad, I., Ma, X., Chang, Y., Li, R., et al. (2018). Combination of berberine and ciprofloxacin reduces multi-resistant Salmonella strain biofilm formation by depressing mRNA expressions of luxS, rpoE, and ompR. J. Vet. Sci. 19:808. doi: 10.4142/jvs.2018.19.6.808
Song, D., Hao, J., and Fan, D. (2020). Biological properties and clinical applications of berberine. Front. Med. 14, 564–582. doi: 10.1007/s11684-019-0724-6
Song, L., Luo, Y., Wang, X., Almutairi, M. M., Pan, H., Li, W., et al. (2019). Exploring the active mechanism of berberine against HCC by systematic pharmacology and experimental validation. Mol. Med. Rep. 20, 4654–4664. doi: 10.3892/mmr.2019.10698
Song, K. H., Oh, S. J., Kim, S., Cho, H., Lee, H. J., Song, J. S., et al. (2020). HSP90A inhibition promotes anti-tumor immunity by reversing multi-modal resistance and stem-like property of immune-refractory tumors. Nat. Commun. 11:562. doi: 10.1038/s41467-019-14259-y
Su, Y. H., Tang, W. C., Cheng, Y. W., Sia, P., Huang, C. C., Lee, Y. C., et al. (2015). Targeting of multiple oncogenic signaling pathways by Hsp90 inhibitor alone or in combination with berberine for treatment of colorectal cancer. Biochim. Biophys. Acta 1853, 2261–2272. doi: 10.1016/j.bbamcr.2015.05.012
Sun, X., Zhang, Y., Cheng, G., Zhu, T., Zhang, Z., Xiong, L., et al. (2023). Berberine improves DSS-induced colitis in mice by modulating the fecal-bacteria-related bile acid metabolism. Biomed. Pharmacother. 167:115430. doi: 10.1016/j.biopha.2023.115430
Turner, S. J., la Gruta, N. L., Kedzierska, K., Thomas, P. G., and Doherty, P. C. (2009). Functional implications of T cell receptor diversity. Curr. Opin. Immunol. 21, 286–290. doi: 10.1016/j.coi.2009.05.004
Vanhaesebroeck, B., Guillermet-Guibert, J., Graupera, M., and Bilanges, B. (2010). The emerging mechanisms of isoform-specific PI3K signalling. Nat. Rev. Mol. Cell Biol. 11, 329–341. doi: 10.1038/nrm2882
Wang, Z., and Ding, H. (2020). Fighting between bacteria and autophagy——death or rebirth. Chin. J. Biotechnol. 36, 1471–1483. doi: 10.13345/j.cjb.190545
Wang, P., and Zheng, S. G. (2013). Regulatory T cells and B cells: implication on autoimmune diseases. Int. J. Clin. Exp. Pathol. 6, 2668–2674. doi: 10.3389/fimmu.2013.02668
Wollein Waldetoft, K., and Råberg, L. (2014). To harm or not to harm? On the evolution and expression of virulence in group A streptococci. Trends Microbiol. 22, 7–13. doi: 10.1016/j.tim.2013.10.006
Xian-Yao, W. S.-S. L. L.-Y. W. G.-X. T. H. L. X.-Y. Z. H. L. S.-H. L. (2015). The effect of Salmonella Enteritidis inoculation on immune organ index of Jining Bairi chicken. Chin. Poultry Sci. 12, 12–17. doi: 10.1186/s12917-020-02474-5
Xiao, Y., Liu, Y., Lai, Z., Huang, J., Li, C., Zhang, Y., et al. (2021). An integrated network pharmacology and transcriptomic method to explore the mechanism of the total Rhizoma Coptidis alkaloids in improving diabetic nephropathy. J. Ethnopharmacol. 270:113806. doi: 10.1016/j.jep.2021.113806
Yang, T., Zhang, X., Xu, C., Wen, X., Zhao, S., Shi, H., et al. (2022). Improving effect of berberine on Salmonella LPS-induced barrier damage of intestinal porcine epithelial cells. Anim. Husb. Vet. Med. 54, 64–71. doi: 10.1177/1753425919873102
Yang, M., Zhang, F., Yang, C., Wang, L., Sung, J., Garg, P., et al. (2020). Oral targeted delivery by nanoparticles enhances efficacy of an Hsp90 inhibitor by reducing systemic exposure in murine models of colitis and colitis-associated Cancer. J. Crohns Colitis 14, 130–141. doi: 10.1093/ecco-jcc/jjz113
Ye, J., Huang, J., Xu, J., Huang, Q., Wang, J., Zhong, W., et al. (2017). ERp29 controls invasion and metastasis of gastric carcinoma by inhibition of epithelial-mesenchymal transition via PI3K/Aktsignaling pathway. BMC Cancer 17:626. doi: 10.1186/s12885-017-3613-x
Yuting Chen, K. L., Zhao, H., Hao, Z., Yang, Y., Gao, M., and Zhao, D. (2022). Integrated lipidomics and network pharmacology analysis to reveal the mechanisms of berberine in the treatment of hyperlipidemia. J. Transl. Med. 20:412. doi: 10.1186/s12967-022-03623-0
Zhai Wendong, S. M. S. (2011). Salmonella—A crucial component of the Salmonella enteritis prevention program. Anim. Sci. Abroad 31, 17–20. doi: 10.1586/erv.12.138
Zhang, M. F., and Shen, Y. Q. (2023a). Research Progress in antifungal pharmacological effects and mechanism of Berberine. Anti-Infect. Pharm. 20, 453–474. doi: 10.13493/j.issn.1672-7878.2023.05-002
Zhang, M. F., and Shen, Y. Q. (2023b). Research Progress in pharmacological effects and mechanism berberine against Salmonella, Shigella, Vibrio, Mycobacterium and Klebsiella. Anti-Infect. Pharm. 20, 1117–1122. doi: 10.13493/j.issn.1672-7878.2023.11-001
Zhang, H. Y., Piao, X. S., Zhang, Q., Li, P., Yi, J. Q., Liu, J. D., et al. (2013). The effects of Forsythia suspensa extract and berberine on growth performance, immunity, antioxidant activities, and intestinal microbiota in broilers under high stocking density. Poult. Sci. 92, 1981–1988. doi: 10.3382/ps.2013-03081
Zhang Wen-Cheng, Z. L.-Z., Fu-qiang, L. I., Li-ping, Z. H. U., and Shi-gan, Y. A. N. (2019). Progress in serotype of Salmonella. J. Qilu Univ. Technol. 33, 10–14. doi: 10.1016/S1473-3099(18)30685-6
Zhao, S., Xu, Q., Cui, Y., Yao, S., Jin, S., Zhang, Q., et al. (2023). Salmonella effector SopB reorganizes cytoskeletal vimentin to maintain replication vacuoles for efficient infection. Nat. Commun. 14:478. doi: 10.1038/s41467-023-36123-w
Zhu Cui, H. K., Lina, L., Aiji, W., Peng, W., Fangmiao, F., Yuanhua, L., et al. (2022). Effects of Berberine on organ index, antioxidant capacity and intestinal immunity in yellow-feathered broilers. Chin. Anim. Husb. Vet. Med. 49, 932–941. doi: 10.1111/asj.13255
Keywords: berberine, salmonellosis, intestinal flora, transcriptome, broiler
Citation: Yang L, Sun J, Yang T, Zhang X, Xu C, Wei Y, Li Y, Zhao Y, Zhang S, Wu Q, Shi H, Fu Q and Xia L (2024) Therapeutic effects and mechanisms of berberine on enteritis caused by Salmonella in poultry. Front. Microbiol. 15:1458579. doi: 10.3389/fmicb.2024.1458579
Edited by:
Ming Jiang, Guangdong Academy of Agricultural Sciences, ChinaReviewed by:
Dexi Li, Henan Agricultural University, ChinaAbhishek Trivedi, Arizona State University, United States
Copyright © 2024 Yang, Sun, Yang, Zhang, Xu, Wei, Li, Zhao, Zhang, Wu, Shi, Fu and Xia. This is an open-access article distributed under the terms of the Creative Commons Attribution License (CC BY). The use, distribution or reproduction in other forums is permitted, provided the original author(s) and the copyright owner(s) are credited and that the original publication in this journal is cited, in accordance with accepted academic practice. No use, distribution or reproduction is permitted which does not comply with these terms.
*Correspondence: Lining Xia, eGxuNzUwNTMwQDE2My5jb20=
†These authors share first authorship